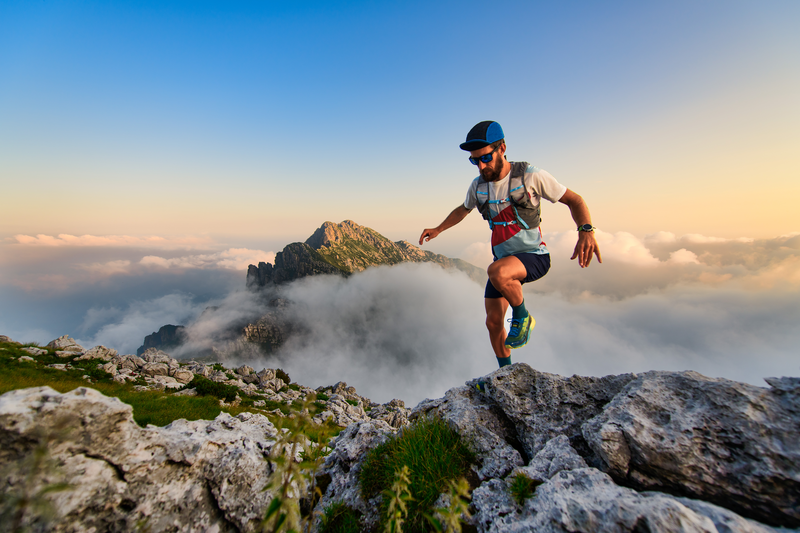
95% of researchers rate our articles as excellent or good
Learn more about the work of our research integrity team to safeguard the quality of each article we publish.
Find out more
ORIGINAL RESEARCH article
Front. Mar. Sci. , 28 June 2023
Sec. Marine Ecosystem Ecology
Volume 10 - 2023 | https://doi.org/10.3389/fmars.2023.1176780
This article is part of the Research Topic Restoration of Coastal Marine Ecosystems View all 22 articles
Grazing is one of the most important biological factors controlling the abundance of early-life stages of fucoids and one of the major issues when restoring marine forests. Benthic macroinvertebrates (e.g., sea urchins) and fish shape and regulate benthic macroalgal communities from polar to tropical regions and can be responsible for regime shifts leading to the predominance of turfs and/or barren grounds. However, other herbivores (i.e., mesograzers) could also significantly participate in the grazing, especially on early-life stages, hampering the persistence and capacity of Cystoseira sensu lato populations to recover after major disturbances and being a cause of failure of restoration actions. We performed experiments in the field and in mesocosm in order to investigate the herbivory pressure and the effects of different grazers on recruits of Cystoseira compressa. The results highlight that non-strict herbivorous invertebrates, such as Clibanarius erythropus, Cerithium vulgatum, and Idotea balthica, graze on recruits of Cystoseira s.l. spp., with I. balthica showing the highest consumption rate. We concluded that biotic factors such as herbivory, which affect key life stages, can be crucial for the conservation of Cystoseira s.l. forests and need to be better understood and considered on a case-by-case basis when planning restoration actions.
Marine forests of large brown macroalgae represented by the species from the orders Laminariales, Tylopteridales, Desmarestiales, and Fucales form structurally complex habitats in polar and temperate regions around the world (Wernberg and Filbee-Dexter, 2019). These forests, commonly located on intertidal and subtidal rocky bottoms, provide important ecosystem functions, such as habitat, food, and shelter to multiple species. They are also one of the world’s most productive ecosystems, participating in capturing carbon dioxide (Boudouresque et al., 2016; Wernberg and Filbee-Dexter, 2019; Pessarrodona et al., 2022). Despite the important ecological functions marine forests provide, multiple anthropogenic stressors are pushing them to the edge (Filbee-Dexter and Wernberg, 2018; Carnell and Keough, 2019; Wernberg et al., 2019). A worldwide decline of marine forests is occurring, mainly driven by the destruction of habitats, reduced water quality, global change, and proliferation of herbivores (Wernberg et al., 2019; Orfanidis et al., 2021; Pessarrodona et al., 2021). In particular, overgrazing by sea urchins and herbivorous fish species is, in some locations, responsible for such declines (Foster and Schiel, 2010; Gianni et al., 2017; Sharma et al., 2021; Barrientos et al., 2022).
Herbivores shape and regulate benthic macroalgal communities in a variety of natural rocky reef systems (Scheibling et al., 1999; Vanderklift et al., 2009; Vergés et al., 2009), and in turn, plant–herbivore interactions are controlled by biotic and abiotic factors (Korpinen et al., 2007). Ocean warming, increased pCO2, and oligotrophic and eutrophic conditions can modify top-down and bottom-up macroalgal communities interactions, increasing grazing and threatening the persistence of habitat-forming macroalgae by altering the density and the species composition of the macroalgal community (Lotze et al., 2001; Hereu et al., 2008; Illa-López et al., 2023). Although local stressors and regional variations can control marine forest dynamics (Krumhansl et al., 2016; Smale, 2020; Verdura et al., 2021; Smith et al., 2022), many studies have shown how sea urchins, native and invasive herbivorous fish, and even omnivorous fish are potential threats to macroalgal communities and responsible for the formation of turfs or barren ground extensions (Tegner et al., 1995; Vergés et al., 2014; Vergés et al., 2016; Papadakis et al., 2021). Mesograzers associated with marine forests such as decapods, gastropods, amphipods, and isopods can significantly participate in the grazing of different life stages of forest-forming species, but little is known about the magnitude of their possible effects (Arrontes et al., 2004; Jonne et al., 2006; Molis et al., 2010; Gunnarsson and Berglund, 2012; Hong et al., 2021; Navarro-Barranco et al., 2022).
In the Mediterranean Sea, the density and abundance of macroalgae are also controlled by herbivores (Vergés et al., 2009; Giakoumi et al., 2012). The two species of sea urchin, Paracentrotus lividus and Arbacia lixula, are the most common benthic macroherbivores of sublittoral rocky bottoms (Bulleri et al., 1999; Agnetta et al., 2015). Both species have been responsible, alongside anthropogenic stressors such as habitat destruction, for the degradation and regime shifts from forest-forming and shrub-forming macroalgal assemblages to barren grounds, favoring the maintenance of stable post-regime shift states (e.g., turfs or barren grounds) in many areas (Sala et al., 1998a; Bulleri et al., 1999; Guidetti and Dulčić, 2007; Guidetti, 2011; Giakoumi et al., 2012). However, sea urchins are not the only macroherbivores that play a role structuring Mediterranean macroalgal assemblages. Sarpa salpa (Salema fish), the only true native herbivorous fish in the Mediterranean Sea (Verlaque, 1990), plays also an important role in structuring macroalgal communities and can be responsible for the depletion of macroalgal forests (Gianni et al., 2017). More recently, the range expansion and invasion of tropical herbivorous fishes such as Siganus spp. (rabbitfish) have contributed to reducing the biomass and diversity of macroalgal species in the Mediterranean Sea, driving regime shifts from macroalgal-dominated communities to barrens (Azzurro et al., 2007; Giakoumi, 2014; Vergés et al., 2014; Vergés et al., 2016; Zarco-Perello et al., 2020).
Mediterranean marine forests are dominated by Cystoseira sensu lato species, including the genera Cystoseira, Ericaria, and Gongolaria (Molinari - Novoa and Guiry, 2020). These canopy-forming macroalgae can form prominent and dense canopies that are analogous to kelp forests of other temperate rocky coasts (Giaccone, 1973; Ballesteros et al., 1998) and constitute one of the most productive and complex habitats of the Mediterranean Sea (Ballesteros, 1989a; Ballesteros, 1990; Clayton, 1990). The zonation of Cystoseira s.l. spp. depends on multiple physical factors such as light, hydrodynamics, temperature, and availability of nutrients (Ballesteros, 1989b) but also on biotic factors such as herbivory (Ruitton et al., 2000; Vergés et al., 2009; Sala et al., 2011). Considering the widespread deforestation of Mediterranean macroalgal forests (Cormaci and Furnari, 1999; Thibaut et al., 2015; Mariani et al., 2019) and the important ecosystem services they provide, important efforts have recently been made to promote their protection and restoration (Eger et al., 2021). Currently, restoration techniques based on recruitment enhancement (by obtaining new recruits from both ex-situ and in-situ techniques) are increasingly being used to restore marine forests (Falace et al., 2018; Verdura et al., 2018; De La Fuente et al., 2019; Cebrian et al., 2021; Savonitto et al., 2021). However, herbivory pressure still constitutes one of the major problems when planning marine forests restoration actions, since it is one of the main causes of failure (Gianni et al., 2013; Gianni et al., 2018; Tamburello et al., 2019b; Savonitto et al., 2021).
Knowing which species graze on different life stages of forest-forming species could be crucial for successful protection and restoration implementation. Grazing is believed to be one of the most important biological factors controlling the abundance of early-life stages of fucoids (Chapman, 1995), whereas the persistence and the resilience of populations to future impacts can be largely dependent on efficient recruitment and growth of the recruits (Ang, 1991; Capdevila et al., 2015; Clausing et al., 2023). Therefore, it is of special interest to study the herbivory pressure on early-life stages as they are usually more vulnerable than adults and high mortality rates are naturally observed during this life stage (Aberg, 1992; Coelho et al., 2000; Schiel and Foster, 2006). The main objective of this study was to investigate the herbivory pressure of different grazers (sea urchins, fishes, decapods, gastropods, amphipods, and isopods) on recruits of Cystoseira compressa. To assess species-specific herbivory pressure on the survival and growth of recruits of C. compressa, we performed experiments in the field (herbivory exclusion) and in mesocosm (grazing rate of decapods, gastropods, amphipods, and isopods) on recruits of this species. C. compressa was selected as a model because it is considered one of the most resistant Cystoseira s.l. spp. to manipulation and the only not protected under the Barcelona Convention Annex II and the United Nations Environment Programme/Mediterranean Action Plan-UNEP/MAP (Mangialajo et al., 2012; Verlaque et al., 2019). This species, which could also have a role in facilitating the settlement of other Cystoseira s.l. spp. (Mangialajo et al., 2012), is still present in the French Riviera, where patches can be found along exposed rocky shores. However, dense populations are only found in rockpools in Lérins Islands (authors’ personal information; Thibaut et al., 2015).
Three sites were selected to study the herbivory pressure on recruits of Cystoseira compressa: (i) a site where a Cystoseira s.l. forest is present (Sainte Marguerite Island) and (ii) two sites where C. compressa was reported in the past but is lost nowadays (Beaulieu-sur-Mer and Passable; Thibaut et al., 2015). The Sainte Marguerite Island site (hereafter Sainte Marguerite; WN-Mediterranean Sea, Lérins Islands, Cannes, France) is a rockpool system located in a protected Natura 2000 site. Situated 1.3 km from the coast of Cannes is where there are some of the last well-conserved shallow marine forests in the Côte d’Azur (Figure 1). The rockpool system (between 0.2 and 1.0 m depth) is composed mainly of C. compressa, Gongolaria barbata, Ericaria crinita, and Ericaria brachycarpa. The two sites deprived of Cystoseira s.l. forests are located on an urbanized continental open coast in (i) Fourmis’ Bay in Beaulieu-sur-Mer (hereafter Beaulieu), a semi-exposed site between 0.5 and 1 m depth, and (ii) Passable in Saint-Jean-Cap-Ferrat (hereafter Passable), a sheltered site around 0.5 m depth (Figure 1).
Figure 1 Location of the three experimental sites: (i) the rockpool system in Sainte Marguerite Island in Lérins Islands (43°30′57.6″N, 7°3′14.4″E) and (ii) the two open coast sites Beaulieu (43°42′3.6″N, 7°19′48″ E) and Passable (43°41′42″N, 7°16′33.6″E).
The presence and density of known and potential herbivorous species, including sea urchins, decapods, and gastropods, were measured at three experimental sites from summer to autumn 2020 and 2021 on five different days in Sainte Marguerite (14/07/2020, 10/09/2020, 20/08/2021, 30/09/2021, 27/10/2021) and in 2021 on three different days in Beaulieu and Passable (30/09/2021, 27/10/2021, 02/11/2021). Herbivorous fish, mainly Sarpa salpa larger or equal to 5 cm in length, were visually quantified in Sainte Marguerite by stationary recording the number of individuals during 5 min, whereas in Beaulieu and Passable, herbivorous fish densities were quantified by recording stationary videos at random points and counting the number of S. salpa individuals in a radius of 5 m during 5 min (n = 10; Sala and Ballesteros, 1997; Vergés et al., 2009). The density of sea urchins was assessed using 50 × 50 cm quadrats (n = 20 per site; Guidetti et al., 2003). The density of decapods and gastropods was determined using 20 × 20 cm quadrats (Barnes, 2003; Jethva et al., 2022) in the rockpool system in Sainte Marguerite (n = 40) and in the open coast in Beaulieu (n = 20) and Passable (n = 20).
An herbivory exclusion experiment was performed in the rockpool system in Sainte Marguerite to study the effectiveness of the in-situ recruitment enhancement and assess the herbivory pressure on recruits in the field (Figure 1). There were 18 natural clean rock substrates randomly fixed using Epoxy putty to the bottom of the rockpool system, to provide free substrates. To test the effect of recruitment enhancement (factor Recruitment enhancement, two levels), half of these substrates were seeded using a non-destructive in-situ recruitment enhancement technique (Verdura et al., 2018). In July 2020, the presence of mature conceptacles of C. compressa in the rockpool system in Sainte Marguerite was confirmed under the microscope and apical fertile branches (ca. 3 cm in length) were manually collected and transported in cold and dark conditions in plastic bags to the laboratory. The apical fertile branches were conserved at 4°C in the dark overnight before being placed in-situ on top of the selected substrates the day after. Above each substrate, a net bag containing 5 g fresh weight (FW) of apical fertile branches was placed floating in the water column for 3 days. In order to assess the herbivory impact on the recruits, some of the substrates were protected (factor Herbivory protection, three levels) to avoid herbivory (mainly from fishes and sea urchins, factor level Protected). Artefact controls and substrates with no protection (levels Artefact control and Open, respectively) were also included (Figure 2; Supplementary material S1; Recruitment enhancement × Herbivory Protection, n = 3). The density (number of recruits per 0.04 m2) and size (length in cm of the longest axis) of C. compressa individuals growing on the substrates were monitored at 2, 9, and 12 months and used as response variables. The size of the recruits was determined by measuring 10 individuals from each substrate. All the individuals were measured when less than 10 individuals were present on the substrate.
Figure 2 Representation of the different experiments performed. Two herbivory experiments were performed in the field, to assess the herbivory pressure on recruits of Cystoseira compressa. A mesocosm experiment to assess the grazing on recruits of C. compressa by the decapod Clibanarius erythropus, the gastropod Cerithium vulgatum, the amphipod Gammarus sp., and the isopod Idotea balthica.
The natural density of the C. compressa individuals naturally present in the rockpool system of Sainte Marguerite was monthly monitored from May to October 2020 using 20 × 20 cm quadrats (n = 25), and the global mean value was used as a reference to assess the evolution of the individuals on our experimental substrates (Verdura et al., 2018).
An herbivory exclusion experiment was performed in the two deforested open coast sites (Beaulieu and Passable; Figure 1) to study the herbivory pressure in the field and the success of the ex-situ recruitment enhancement technique in the absence of a Cystoseira s.l. forest. In August 2021, after having observed mature conceptacles under the microscope, around 180 g FW apical branches of C. compressa containing fertile receptacles were collected from the C. compressa donor population located in the rockpool system in Sainte Marguerite. The apical branches were transported in cold and dark conditions, and once in the laboratory, they were gently cleaned with tweezers. To stimulate the release of gametes, the apical branches were maintained at 4°C in the dark overnight before placing them on the culture tanks. The day after, the apical fertile branches were placed on the surface of three 30-l closed system tanks (60 g FW of apical fertile branches per tank) to obtain recruits on the clean natural rock substrates previously placed on the bottom of the tanks (Verdura et al., 2018; De La Fuente et al., 2019). The apical fertile branches were kept for 4 days on the surface of the tanks to release the zygotes. The recruits of C. compressa were kept for 2.5 months in the aquarium facilities, with air pumps and under natural light conditions, before transplanting them to the two open coast sites. Filtered seawater (200 µm mesh) from Anse des Fosses (Saint-Jean-Cap-Ferrat, France) was used for the tanks and was changed every 2 days; no culture medium was provided to the cultures. After 2.5 months in tanks (in October 2021), 24 substrates with similar densities of recruits of C. compressa were transplanted and fixed using Epoxy putty in the two deforested open coast sites. Some substrates with recruits were maintained in the aquarium facilities and used as controls (n = 6). As for experiment 1 (see Section 2.3), half of the transplanted substrates were protected (factor Herbivory protection, two levels, n = 6) to avoid herbivory (mainly fishes and sea urchins) whereas the others were not (Figure 2; Supplementary material S1). The density (number of recruits per 0.04 m2) of C. compressa individuals growing on the substrates was used as a response variable and was monitored at the beginning of the experiment and after 1 and 12 days.
The potential herbivory pressure on recruits of C. compressa of a decapod (Clibanarius erythropus), a gastropod (Cerithium vulgatum), an amphipod (Gammarus sp.), and an isopod (Idotea balthica) was assessed in laboratory. The species and their densities were selected in function of field observations and information reported in the literature for fucoid species (Engkvist et al., 2000; Chemello and Milazzo, 2002; Gozler et al., 2010; Gunnarsson and Berglund, 2012; Suzuki et al., 2020; Hong et al., 2021; Navarro-Barranco et al., 2022).
Clibanarius erythropus and Cerithium vulgatum individuals were obtained from the rockpool system in Sainte Marguerite, within the Cystoseira s.l. forest, whereas I. balthica and Gammarus sp. were obtained from Anse des Fosses (Saint-Jean-Cap-Ferrat, France) within leaves of Posidonia oceanica (Tracheophyta) detritus between 0 and 0.5 m depth. Recruits of Cystoseira compressa were obtained in the laboratory following the same ex-situ cultivation technique as in Section 2.4 and kept in 30-l tanks until the beginning of the mesocosm experiment (2.5 months). The substrates with C. compressa recruits were cleaned from epiphytes and/or growing biofilm with tweezers and a brush before the start of the experiment. Individual substrates with similar biomass of recruits of C. compressa were placed in 2.5-l experimental tanks (100 cm2). Different numbers of herbivores of each species were considered for the experiment: one, two, and three individuals of Clibanarius erythropus were placed in a total of 15 tanks (n = 5); one, three, and five individuals Cerithium vulgatum were placed in a total of 15 tanks (n = 5); and one individual per tank was considered for Gammarus sp. and I. balthica (n = 7; 7 experimental tanks per species). Controls with 0 herbivorous were included. As the experiments with Clibanarius erythropus and Cerithium vulgatum were performed simultaneously, the same controls (n = 5; 5 experimental tanks) were used for both species. The experiments with Gammarus sp. and I. balthica were performed later and shared the same control experimental tanks with 0 individuals (n = 6; 6 experimental tanks; Supplementary material S1).
All the herbivores were kept without food for 48 h before the start of the experiment. Every 2 days, the water temperature was measured and the filtered seawater (200 µm mesh) in the experimental tanks was changed, and faeces were cleaned every day. Clibanarius erythropus and Cerithium vulgatum were maintained in the experimental tanks for 23 days and Gammarus sp. and I. balthica for 4 days, until they consumed all, or almost all, of the available biomass of Cystoseira compressa. Clibanarius erythropus and Cerithium vulgatum were weighted once at the end of the experiment (mg FW) after extracting them from their shells. Gammarus sp. and I. balthica individuals where weighted (mg FW) every 24 h. The mean biomass (FW) of Gammarus sp. individuals was 0.08 ± 0.01 g FW, and the mean biomass of I. balthica individuals was 0.07 ± 0.01 g FW (mean ± se hereafter) at the beginning of the experiment. The consumption of the different species was assessed as the consumed biomass (mg FW) of recruits and extrapolated from the number of Cystoseira compressa recruits. At the start of the experiment, the mean biomass of C. compressa in each experimental tank was 4.96 ± 0.13 mg FW for Clibanarius erythropus, 5.08 ± 0.14 mg FW for Cerithium vulgatum, 4.37 ± 0.37 mg FW for Gammarus sp., and 5.47 ± 0.28 mg FW for I. balthica (Supplementary material S2). The biomass consumption of recruits (mg FW) was evaluated every 24 h the first week and then twice a week, which, with the consumption rate (mg FW Cystoseira compressa mg FW herbivores-1 day-1), were used as response variables.
The biomass (mg FW) of the C. compressa recruits was extrapolated from a linear regression [volume (mm3) ~ biomass (mg FW)]. To do so, the volume of each recruit was calculated approximating its shape to a cone and semi sphere (Supplementary material S2). The maximum length and maximum width of each recruit were measured under a stereomicroscope. Then, this volume was transformed to g FW, using the previously adjusted volume (mm3) ~ biomass (mg FW) linear regression determined on other recruits (R2 = 0.994). To obtain the linear regression, the volume of 38 recruits was determined by a water displacement technique (mL). The volume of each recruit was measured six times, and the mean of the measurements was used. Then, each recruit was dried with absorbent paper before being weighed three times (± 1 mg) for obtaining the FW (Supplementary material S2).
Field experiment 1: The density (ind. 0.04 m-2) and the size (cm) of recruits of C. compressa were used as response variables. A generalized linear model (GLM), with a “Quasipoisson link log distribution function, was used to test the effect of the herbivory protection and recruitment enhancement on the density of recruits after 2 months, with herbivory protection (three levels) and recruitment enhancement (two levels) as fixed factors. The factor recruitment enhancement was no longer considered for further analysis because it was not significant for the first sampling date (2 months). Then, a generalized linear mixed-effect model (GLMM) with a Poisson link log distribution function was used to test the effect of the factor herbivory protection over time with herbivory protection and time (both three levels) as fixed factors and substrate as a random factor, in order to cope with repeated measures over time (Pinheiro and Bates, 2000). A GLMM with an inverse Gaussian link log distribution was used to test the effects of the herbivory protection on the size of the recruits, with herbivory protection and time (both three levels) as fixed factors and the substrate as a random factor.
Field experiment 2: The density of recruits of C. compressa (ind. 0.04 m-2) was used as a response variable. A GLMM with a Poisson link log distribution function was used to test the effect of the herbivory protection on the density of recruits, with herbivory protection (two levels) and time (three levels) as fixed factors and site (two levels) and substrate as random factors to cope with repeated measures over time (Pinheiro and Bates, 2000).
Mesocosm experiment: The cumulative biomass consumption (mg FW) and the consumption rate (mg FW C. compressa mg FW herbivores -1 day -1) of recruits of C. compressa were used as response variables. A linear mixed-effect model (LMM) was used to test the cumulative biomass consumption of recruits by Clibanarius erythropus, Cerithium vulgatum, and I. balthica, with the density of herbivores (four levels for Clibanarius erythropus and Cerithium vulgatum and two levels for I. balthica) as a fixed factor and the time and the tank as random factors as the LMM models can cope with repeated measures over time. Finally, a linear model (LM) was used to test the consumption rate of the different species, with the species (three levels) as a fixed factor. Gammarus sp. was excluded of this analysis as no consumption was detected.
All the GLM, GLMM, LMM, and LM models were fitted to analyze the effect of the variables, and the AIC likelihood minimum was used to select the best model among the possible combinations. All the different models were fitted using the functions “glm,” “glmer,” “lmer,” and “lm” from the package lme4 (Bates et al., 2015) in the statistical environment R (R Core Team, 2019). For all the models, the assumptions of normality and equality of variance were evaluated through graphical analyses of residuals using QQ plot functions. P-values were obtained by means of a Wald χ2 test using the ‘ANOVA’ function from the car package (Fox and Weisberg, 2019). Finally, the function ‘emmeans’ from the package emmeans (Lenth et al., 2022) was used to perform the post-hoc analysis.
In the rockpool system in Sainte Marguerite, neither Sarpa salpa (>5 cm) or sea urchins (Paracentrotus lividus or Arbacia lixula) were observed during the experiment. In this site, the mean density of Paguroidea decapods was 45.90 ± 13.08 ind. m-2 and the mean density of Cerithium vulgatum was 36.46 ± 9.44 ind. m-2. In the open coast sites, mean densities of S. salpa of 1.1 ± 0.50 ind. 39.27 m-2 and of 1.2 ± 0.33 ind. 39.27 m-2 were respectively found in Beaulieu and Passable. Sea urchins (including P. lividus and A. lixula) were present at densities of 6.00 ± 1.83 and 3.20 ± 1.04 ind. m-2; Paguroidea decapods were present at densities of 2.91 ± 1.35 ind. m-2 and 49.45 ± 14.77 ind. m-2; and C. vulgatum was present at densities of 5.09 ± 3.85 and 96.73 ± 24.79 ind. m-2, respectively, for Beaulieu and Passable (Figure 3). Paguroidea decapods in the three sites consisted mainly of Clibanarius erythropus, but few individuals of Diogenes pugilator and of Calcinus tubularis were also observed. In general, higher densities of herbivores were observed in Passable (Figure 3).
Figure 3 In-situ density of herbivores at the three studied sites: the two open coast sites (Beaulieu and Passable) and the rockpool system (Sainte Marguerite). The errors bars show the standard error.
The density of recruits (ind. 0.04 m-2) on the experimental substrates in Sainte Marguerite was affected at 2 months only by the factor herbivory protection, and not by the recruitment enhancement (GLM, P-value < 0.001; Figure 4 and Table 1). At this time, the post-hoc analysis found higher densities of recruits on protected substrates than on open or artefact control substrates. Due to the non-significance of the recruitment enhancement on the density of recruits at 2 months, this factor was no longer considered and the data were pooled together in the next analysis. When considering the three sampling dates, the herbivory protection and the time exerted an interactive effect on the density of recruits (GLMM, P-value < 0.001 for the interaction Herbivory protection × Time; Figure 5 and Table 1). The post-hoc analysis detected differences in densities of Cystoseira compressa between the levels of herbivory protection for all sampling days. At months 2 and 12, densities of C. compressa were higher on protected substrates, whereas at month 7, there was no difference between protected and open substrates. For all the herbivory protection levels, the densities of C. compressa decreased over time, but at month 12, the densities on the experimental substrates were still higher than the ones observed in the natural population (3.05 ± 0.26 ind. C. compressa 0.04 m-2 in natural populations; Figure 4).
Figure 4 Boxplot of the density of recruits of Cystoseira compressa per 0.04 m2 of natural substrates in the rockpool system in Sainte Marguerite 2 months after the starting of the experiment. The bold horizontal lines indicate the median value (Q2), the box marks the interquartile distances (Q1 and Q3), and the whiskers mark the values that are less than Q3 + 1.5 * IQR but greater than Q1–1.5 * IQR.
Figure 5 Density of recruits of Cystoseira compressa per 0.04 m2 of natural substrates in the rockpool system in Sainte Marguerite in function of the herbivory protection level (protected, artefact control, and open). The natural population densities of C. compressa in this site are represented in red considering the mean and the standard error (3.05 ± 0.26 individuals 0.04 m-2, mean ± se). The errors bars show the standard error.
Only time and not the herbivory protection had a significant effect on the size of the recruits (GLMM, P-value < 0.001 for the factor Time; Figure 6 and Table 1). The size increased with time with a maximum at month 12 (July 2021), with a mean size of 9.00 ± 0.52 cm, and maximum and minimum individual sizes of 18 and 3 cm, respectively (Figure 6 and Table 1). The natural density of C. compressa in Sainte Marguerite was 3.05 ± 0.26 ind. 0.04 m-2 (76.36 ± 6.40 individuals m-2), with maximum densities of 5 and a minimum of 1 ind. 0.04 m-2.
Figure 6 Size class distribution of the Cystoseira compressa recruits on Sainte Marguerite’s substrates over time in function of the herbivory protection level (protected, artefact control, and open). The X-axis represents the size classes (length of the of the longest axis of each individual) in 1-cm intervals, and the Y-axis is the proportion of each size class per treatment.
The herbivory protection and time had an interactive effect on the density of recruits of C. compressa on the deforested open coast (GLMM, P-value < 0.001 for the interaction Herbivory protection × Time; Figure 7 and Table 1). The post-hoc analysis found higher densities of recruits of C. compressa on protected substrates after 1 and 12 days. However, most of the recruits (>50%), even on protected substrates, disappeared after 24 h (Figure 7), whereas densities of the recruits that remained in the tanks in the laboratory (substrates that were not transplanted to the field) were stable after 12 days.
Figure 7 Density of recruits of Cystoseira compressa per 0.04 m2 of natural substrate on the deforested open coast sites (Beaulieu and Passable) in function of the herbivory protection level (protected, artefact control and open). The errors bars show the standard error.
All the studied mesograzers, except Gammarus sp., consumed recruits of C. compressa. For Clibanarius erythropus, Cerithium vulgatum, and Idotea balthica, the post-hoc analysis found significant differences in the consumption of Cystoseira compressa biomass when compared with the controls (factor number of individuals; LMM, P-value < 0.001; Figure 8 and Table 1). No consumption and neither significant mortality of C. compressa was found in the tanks with Gammarus sp. (Figure 8). For Clibanarius erythropus, a significant effect was observed between the treatments and the control, independently of the density of individuals. A density effect was detected for Cerithium vulgatum, with the consumption being higher in the treatment with five individuals (Figure 8 and Table 1). I. balthica individuals consumed 100% of the biomass of recruits 24 h after the start of the experiment in four out of the seven experimental tanks, and more than 85% in two of them (Figure 8; Supplementary Material S3). The consumption rate of I. balthica on Cystoseira compressa recruits was by far the highest of the three grazers; no significant differences were observed between Clibanarius erythropus and Cerithium vulgatum grazing rate (LM, P-value < 0.001; Figure 9 and Table 1).
Figure 8 Cumulative biomass consumption of Cystoseira compressa (mg FW) by Clibanarius erythropus, Cerithium vulgatum, Gammarus sp., and Idotea balthica, and control substrates. Not that the biomass loss in the controls (0 individuals) it is not due to herbivory. The grey dashed lines represent the total mean available biomass of C. compressa in the experimental tanks. The errors bars show the standard error.
Figure 9 Consumption rate (mg FW Cystoseira compressa mg FW herbivore -1 day -1) for the three mesoherbivores for the whole duration of each experiment. Gammarus sp. is not represented as no consumption of C. compressa by this species was observed. The error bars show the standard error.
Our study aimed at elucidating the herbivory pressure of different mesograzers on early-life stages of shallow Cystoseira s.l. spp. Our results are also very relevant in the framework of ecological restoration, as grazing can affect marine forest restoration actions and the species chosen (Tamburello et al., 2019a; Savonitto et al., 2021). During the field experiments, Cerithium vulgatum and decapods (mainly Clibanarius erythropus) were the most abundant herbivores observed at all sampling sites (excepted in Beaulieu), and interestingly, high densities were observed on patches of bare rocks just next to the macroalgal communities (author’s personal observation). In the two open coast sites, the densities of Sarpa salpa and sea urchins were similar with the ones reported on other Mediterranean studies at the same depth (Sala et al., 1998b; Guidetti et al., 2003; Vergés et al., 2009). Similarly, although no data were found for the Mediterranean Sea, the observed densities of Paguroidea decapods and Cerithium vulgatum in the three sites were between the ranges reported for species of the same genera in other locations (Barnes, 2003; Halpern, 2004; Jethva et al., 2022).
In the experiment performed in the rockpool system situated in Sainte Marguerite, the density of Cystoseira compressa recruits changed in function of the herbivory protection, showing that herbivory pressure can affect the recruitment success and, therefore, how important it is to control herbivory on early-life stages for increasing restoration success. The first assessment of the density and size of recruits at this site was performed 2 months after the setup, a reasonable time to allow a correct visual identification and quantification of the recruits in the field. The density of recruits drastically decreased in month 7, likely due to density-dependant effects: the density decreases as the size increases (Chapman, 1995). The significantly higher number of individuals found on the protected substrates than on the artefact control and open substrates at month 2 was still observed at month 12. No significant patterns were observed at month 7, likely due to some difficulties in the individual quantification at this particular stage of the growth, when they start ramifying (when the ramifications are close to the holdfast and the densities of individuals high, it can be difficult to quantify single individuals). At month 12, when significant differences linked to the protection were observed, the individuals were bigger and their quantification easier. Interestingly, the density of individuals of C. compressa on the experimental substrates after 12 months was higher than the density observed in the surrounding natural populations. On the other hand, there were no differences in the size of the recruits independently of the herbivory protection. No effect of the recruitment enhancement was observed in the experiment. This could be due to the presence of fertile C. compressa adults in the rockpool system, which could have released new zygotes recruiting on all our experimental substrates, but also to a larger effect of the recruitment enhancement scale: every bag with receptacles could have spread zygotes at a wider scale than the size of the experimental substrates.
In the two deforested open coast sites, Beaulieu and Passable, we observed a dramatic decrease in density of the transplanted recruits after only 24 h. The density of recruits was significantly higher in the protected treatments after 24 h, but almost no individuals were present after 12 days in both treatments. A high grazing rate in these sites could be at the origin of this result (showing a delay in time in the loss of recruits in the protected substrates), but other reasons could explain the observed patterns. While in Sainte Marguerite, the good conditions for the growth of Cystoseira s.l. spp. were ensured by the presence of a healthy forest, in Beaulieu and Passable, this was not the case, and we cannot exclude that the environmental conditions for the survival of the recruits might have not been adequate (e.g., water quality, sedimentation, irradiation, absence of a canopy; Irving et al., 2009), despite the presence of C. compressa in the past (Thibaut et al., 2015). It is also worth noting that the recruits transplanted to the open coast sites were grown in tanks (ex-situ technique) for 2.5 months, eventually becoming more sensitive to the natural environmental parameters (Clausing et al., 2023). Replicating natural conditions in experimental tanks is difficult, and recruits in the laboratory grew less than the ones in Sainte Marguerite (obtained from the in-situ technique). For restoration actions it is counselled to transplant the recruits after a few weeks (Falace et al., 2018; De La Fuente et al., 2019; Savonitto et al., 2021).
The recruits of the two field experiments were obtained using different techniques that were chosen in function of the characteristic of each site (e.g., forested/no forested, past presence of a forest, potential herbivory pressure; Cebrian et al., 2021). It is therefore not possible to compare the results obtained at Sainte Marguerite with the ones obtained at Beaulieu and Passable. In the rockpool system in Sainte Marguerite, natural and artificial seedling (in-situ technique) with substrate provision was used, as it is the one preferred for sheltered locations with low herbivory pressure (Cebrian et al., 2021). While in the deforested open coast, in Beaulieu and Passable, the ex-situ technique was prioritized as it is the one recommended for deforested and more hydrodynamic locations (Cebrian et al., 2021). However, the herbivory pressure can change in function of the characteristics of the experimental locations (e.g., the physical environmental conditions and the structure of the benthic communities; Lubchenco, 1986; Medrano et al., 2020). Ferrario et al. (2016) observed how individuals from the same species (fishes and decapods) exerted a stronger herbivory pressure on artificial habitats than on natural ones, and the same could have happened during our experiments in the urbanized coast of Beaulieu and Passable. Another study (Gianni et al., 2018) performed on the open infralittoral fringe of the French Riviera (N-W Mediterranean Sea), where sea urchins were not present, already concluded that S. salpa was the most efficient grazer on adult Ericaria amentacea (formerly Cystoseira amentacea), potentially limiting the success of restoration actions. Therefore, recruits obtained in the rockpool system within an existing Cystoseira s.l. forest (Sainte Marguerite) could have been less affected by herbivores because of the lager abundance and variety of other palatable species of macroalgae present in the site.
The mesocosm experiment performed in this study is a first approach for elucidating which other species, apart from the most studied ones (mainly sea urchins and herbivorous fish), can have a role in the grazing of recruits of Cystoseira s.l. spp. Ferrario et al. (2016) already highlighted that most species consuming or interacting with Cystoseira s.l. spp. are usually classified as omnivorous rather than herbivorous. During our mesocosm experiment, the mesograzer that had the highest and fastest consumption rate of recruits of C. compressa was Idotea balthica. It is worth noting that different results could have been obtained if proposing other extra food sources to the mesograzers in the tanks. I. balthica is the most widespread Idoteidae in European Seas (Guarino et al., 1993) and is frequently used as model species to study the interactions between macroalgae and herbivores (Gutow et al., 2014; Lavaut et al., 2022). This species can be found living in macroalgal communities including marine forests (Guarino et al., 1993; Gozler et al., 2010; Lavaut et al., 2022), and it is an important consumer of forest-forming brown macroalgae, such as the shallow Fucus vesiculosus in the Atlantic (Kotta et al., 2000; Jonne et al., 2006; Vesakoski et al., 2008; Molis et al., 2010; Schaal et al., 2016). I. balthica has an important grazing effect on structuring F. vesiculosus populations in the Baltic Sea, preferring younger tissue over older (Engkvist et al., 2000; Boström and Mattila, 2005) and, according to our results, could also play an important role in grazing recruits of shallow Cystoseira s.l. spp. in the Mediterranean Sea.
A significant biomass loss of recruits of C. compressa recruits was also observed in the presence of Cerithium vulgatum and Clibanarius erythropus (both omnivores). Few previous studies have shown that molluscs and decapods in particular can have consumptive (i.e., grazing) and non-consumptive (i.e., clipping and cutting the thalli) behaviours with recruits and adults of macroalgae, including Cystoseira compressa (Lubchenco, 1983; Perkol-Finkel et al., 2012; Ferrario et al., 2016; Gianni et al., 2017). Even if, in our experiment, the amphipod (Gammarus sp.) did not graze on recruits of C. compressa, it is reported that amphipods can heavily feed on brown macroalgae (Duffy and Hay, 2000) including Fucoids (Jonne et al., 2006). Even if macroalgal species, including Cystoseira s.l. spp., contain metabolites such as phenolic compounds, especially on young tissue, that deter herbivores’ feeding, this chemical defence is not enough to prevent grazing. Interestingly, Cerithium vulgatum, known to be resistant to the chemical deterrence of Posidonia oceanica extracts (Vergés et al., 2007), was one of the more abundant species in our experimental sites and was observed grazing on Cystoseira compressa recruits. Nevertheless, the feeding behaviour and preferences of different native and invasive species (e.g., sea urchins and herbivorous fishes) could be modified under global change (Vergés et al., 2014; Asnaghi et al., 2020). Chemical defences, such as phlorotannins, present in Fucoids could be as well affected by climate change, as is the case of F. vesiculosus, making macroalgae more susceptible to grazing (Raddatz et al., 2017; Kinnby et al., 2021). The palatability of macroalgal species can change under ocean warming, elevated pCO2, increased salinity, and nutrient conditions, enhancing grazing and constituting an additional threat under future climate change scenarios (Asnaghi et al., 2013; Gutow et al., 2014; Kinnby et al., 2021; Mitterwallner et al., 2021; Illa-López et al., 2023).
In conclusion, several mesograzers can potentially feed on Cystoseira s.l. recruits and they have to be taken into account when planning marine forests protection and restoration actions. A case-by-case analyses of the particularities of each location is necessary, including both abiotic (e.g., water quality) and biotic (i.e., grazing pressure) factors, both natural and human-induced. Where the grazing pressure is high, solutions should be found, such as anti-herbivory devices adapted to the grazers present in the site (Gianni et al., 2020; Cebrian et al., 2021; Savonitto et al., 2021) and/or the regulation of grazer populations (Medrano et al., 2020; Sharma et al., 2021; Miller et al., 2022). The use of ex-situ cultivation techniques, allowing the installation in the field of larger individuals, can increase the survival of recruits against mesograzers (Verdura et al., 2018; Cebrian et al., 2021). These measures, however, will not address the underlying causes of the proliferation of herbivores and are unlikely to provide a long-term solution for the protection and restoration of marine forests and their ecosystem function and services (Miller et al., 2022). More studies are needed to predict how the feeding behaviour and preferences of different native and invasive species could evolve under climate change to evaluate the threat they can pose for future the conservation of marine forests.
The raw data supporting the conclusions of this article will be made available by the authors, without undue reservation.
LM got the funding. LM, MM, JV, and SC designed the experiments, LM, MM, JV, SC, J-MC, FP, and GR performed the experiments in the field and laboratory activities. MM and JV analysed the data and wrote the first draft of the manuscript, which was then finalized by all co-authors. All authors contributed to the article and approved the submitted version.
This work is supported by a PhD grant funded by the Région Provence-Alpes-Côte d’Azur (contract Emplois Jeunes Doctorants 2019–2022), the “Algal Forest Restoration In the MEDiterranean sea” - AFRIMED project funded by the Executive Agency for Small and Medium Enterprise (EASME) and European Maritime and Fisheries Fund (EMFF) under grant agreement N° 789059 and the “Innovative approaches FOr RESCUE and management of algal forests in the Mediterranean Sea” – FORESCUE project, funded in the framework of the Biodiversa+ 2021 Call for Proposals “Supporting the protection of biodiversity and ecosystems across land and sea”, Project number Biodiversa2021-134.
The authors declare that the research was conducted in the absence of any commercial or financial relationships that could be construed as a potential conflict of interest.
All claims expressed in this article are solely those of the authors and do not necessarily represent those of their affiliated organizations, or those of the publisher, the editors and the reviewers. Any product that may be evaluated in this article, or claim that may be made by its manufacturer, is not guaranteed or endorsed by the publisher.
The Supplementary Material for this article can be found online at: https://www.frontiersin.org/articles/10.3389/fmars.2023.1176780/full#supplementary-material.
Supplementary Material S3 | available on: https://youtu.be/BFJDBZFh9mo.
Aberg (1992). A demographic study of two populations of the seaweed Ascophyllum nodosum. Ecology 73, 1473–1487. doi: 10.2307/1940691
Agnetta D., Badalamenti F., Ceccherelli G., Di Trapani F., Bonaviri C., Gianguzza P. (2015). Role of two co-occurring Mediterranean sea urchins in the formation of barren from Cystoseira canopy. Estuar. Coast. Shelf Sci. 152, 73–77. doi: 10.1016/j.ecss.2014.11.023
Ang P. O. (1991). Natural dynamics of a Fucus distichus (Phaeophyceae, fucales) population: reproduction and recruitment. Mar. Ecol. Prog. Ser. 78, 71–85.
Arrontes J., Arenas F., Fernández C., Rico J. M., Oliveros J., Martínez B., et al. (2004). Effect of grazing by limpets on mid-shore species assemblages in northern Spain. Mar. Ecol. Prog. Ser. 277, 117–133. doi: 10.3354/meps277117
Asnaghi V., Chiantore M., Mangialajo L., Gazeau F., Francour P., Alliouane S., et al. (2013). Cascading effects of ocean acidification in a rocky subtidal community. PloS One 8, e61978. doi: 10.1371/journal.pone.0061978
Asnaghi V., Chindris A., Leggieri F., Scolamacchia M., Brundu G., Guala I., et al. (2020). Decreased pH impairs sea urchin resistance to predatory fish: a combined laboratory-field study to understand the fate of top-down processes in future oceans. Mar. Environ. Res. 162, 105194. doi: 10.1016/j.marenvres.2020.105194
Azzurro E., Fanelli E., Mostarda E., Catra M., Andaloro F. (2007). Resource partitioning among early colonizing Siganus luridus and native herbivorous fish in the Mediterranean: an integrated study based on gut-content analysis and stable isotope signatures. J. Mar. Biol. Assoc. U. K. 87, 991–998. doi: 10.1017/S0025315407056342
Ballesteros E. (1989a). Production of seaweeds in northwestern Mediterranean marine communities: its relation with environmental factors. Sci. Mar. Barc. 53, 357–364.
Ballesteros E. (1989b). Surface-dependent strategies and energy flux in benthic marine communities or, why corals do not exist in the Mediterranean. Scientia Marina 53, 3–17.
Ballesteros E. (1990). Structure and dynamics of the community of Cystoseira zosteroides (Turner) C. Agardh (Fucales, phaeophyceae) in the northwestern Mediterranean. Sci. Mar. 54, 217–229.
Ballesteros E., Sala E., Garrabou J., Zabala M. (1998). Community structure and frond size distribution of a deep water stand of Cystoseira spinosa (Phaeophyta) in the northwestern Mediterranean. Eur. J. Phycol. 33, 121–128. doi: 10.1080/09670269810001736613
Barnes R. S. K. (2003). Interactions between benthic molluscs in a sulawesi mangal, Indonesia: the cerithiid mud-creeper Cerithium coralium and potamidid mud-whelks, Terebralia spp. J. Mar. Biol. Assoc. U. K. 83, 483–487. doi: 10.1017/S0025315403007380h
Barrientos S., Piñeiro-Corbeira C., Barreiro R. (2022). Temperate kelp forest collapse by fish herbivory: a detailed demographic study. Front. Mar. Sci. 9. doi: 10.3389/fmars.2022.817021
Bates D., Mächler M., Bolker B., Walker S. (2015). Fitting linear mixed-effects models using lme4. J. Stat. Software 64, 1–48. doi: 10.18637/jss.v067.i01
Boström C., Mattila J. (2005). Effects of isopod grazing: an experimental comparison in temperate (Idotea balthica, Baltic Sea, Finland) and subtropical (Erichsonella attenuata, gulf of Mexico, U.S.A.) ecosystems. Crustaceana 78, 185–200. doi: 10.1163/1568540054020541
Boudouresque C., Blanfune A., Harmelin M., Personnic S., Ruitton S., Thibaut T., et al. (2016). Where seaweed forests meet animal forests: the examples of macroalgae in coral reefs and the Mediterranean coralligenous ecosystem. Marine Animal Forests. The Ecology of Benthic Biodiversity Hotspots. Springer Verlag, 1–28. doi: 10.1007/978-3-319-17001-5_48-1
Bulleri F., Benedetti-Cecchi L., Cinelli F. (1999). Grazing by the sea urchins Arbacia lixula L. and Paracentrotus lividus Lam. in the Northwest Mediterranean. J. Exp. Mar. Biol. Ecol. 241, 81–95. doi: 10.1016/S0022-0981(99)00073-8
Capdevila P., Linares C., Aspillaga E., Navarro L., Kersting D. K., Hereu B. (2015). Recruitment patterns in the Mediterranean deep-water alga Cystoseira zosteroides. Mar. Biol. 162, 1165–1174. doi: 10.1007/s00227-015-2658-0
Carnell P. E., Keough M. J. (2019). Reconstructing historical marine populations reveals major decline of a kelp forest ecosystem in Australia. Estuaries Coasts 42, 765–778. doi: 10.1007/s12237-019-00525-1
Cebrian E., Tamburello L., Verdura Brugarola J., Guarnieri G., Medrano A., Linares C., et al. (2021). A roadmap for the restoration of Mediterranean macroalgal forests. Front. Mar. Sci. 8. doi: 10.3389/fmars.2021.709219
Chapman A. R. O. (1995). Functional ecology of fucoid algae: twenty-three years of progress. Phycologia 34, 1–32. doi: 10.2216/i0031-8884-34-1-1.1
Chemello R., Milazzo M. (2002). Effect of algal architecture on associated fauna: some evidence from phytal molluscs. Mar. Biol. 140, 981–990. doi: 10.1007/s00227-002-0777-x
Clausing R. J., de la Fuente G., Falace A., Chiantore M. (2023). Accounting for environmental stress in restoration of intertidal foundation species. J. Appl. Ecol. 60, 305–318. doi: 10.1111/1365-2664.14334
Clayton M. N. (1990). The adaptive significance of life history characters in selectedorders of marine brown maeroalgae. Aust. J. Ecol. 15, 439–452. doi: 10.1111/j.1442-9993.1990.tb01469.x
Coelho S. M., Rijstenbil J. W., Brown M. T. (2000). Impacts of anthropogenic stresses on the early development stages of seaweeds. J. Aquat. Ecosyst. Stress Recovery 7, 317–333. doi: 10.1023/A:1009916129009
Cormaci M., Furnari G. (1999). “Changes of the benthic algal flora of the tremiti islands (southern Adriatic) Italy,” in Sixteenth international seaweed symposium developments in hydrobiology. Eds. Kain J. M., Brown M. T., Lahaye M. (Dordrecht: Springer Netherlands), 75–79. doi: 10.1007/978-94-011-4449-0_9
De La Fuente G., Chiantore M., Asnaghi V., Kaleb S., Falace A. (2019). First ex situ outplanting of the habitat-forming seaweed Cystoseira amentacea var. stricta from a restoration perspective. PeerJ 7, e7290. doi: 10.7717/peerj.7290
Duffy J. E., Hay M. E. (2000). Strong impacts of grazing amphipods on the organization of a benthic community. Ecol. Monogr. 70, 237–263. doi: 10.1890/0012-9615(2000)070[0237:SIOGAO]2.0.CO;2
Eger A., Marzinelli E., Christie H., Fagerli C. W., Fujita D., Hong S., et al. (2022). Global kelp forest restoration: past lessons, status, and future goals. EcoEvoRxiv. doi: 10.32942/osf.io/emaz2
Engkvist R., Malm T., Tobiasson S. (2000). Density dependent grazing effects of the isopod Idotea baltica Pallas on Fucus vesiculosus L in the Baltic Sea. Aquat. Ecol. 34, 253–260. doi: 10.1023/A:1009919526259
Falace A., Kaleb S., Fuente G. D. L., Asnaghi V., Chiantore M. (2018). Ex situ cultivation protocol for Cystoseira amentacea var. stricta (Fucales, phaeophyceae) from a restoration perspective. PloS One 13, e0193011. doi: 10.1371/journal.pone.0193011
Ferrario F., Iveša L., Jaklin A., Perkol-Finkel S., Airoldi L. (2016). The overlooked role of biotic factors in controlling the ecological performance of artificial marine habitats. J. Appl. Ecol. 53, 16–24. doi: 10.1111/1365-2664.12533
Filbee-Dexter K., Wernberg T. (2018). Rise of turfs: a new battlefront for globally declining kelp forests. BioScience 68, 64–76. doi: 10.1093/biosci/bix147
Foster M. S., Schiel D. R. (2010). Loss of predators and the collapse of southern California kelp forests (?): alternatives, explanations and generalizations. J. Exp. Mar. Biol. Ecol. 393, 59–70. doi: 10.1016/j.jembe.2010.07.002
Fox J., Weisberg S. (2018). An r companion to applied regression. Third edit. (Thousand Oaks, California, USA: SAGE Publications). https://cran.r-project.org/web/packages/car/index.html.
Giaccone G. (1973). Écologie et chorologie des Cystoseira de Méditerranée. Rapp. Com. Int. Mer Médi. 22 (4), 49–50.
Giakoumi S. (2014). Distribution patterns of the invasive herbivore Siganus luridus (Rüppell 1829) and its relation to native benthic communities in the central Aegean Sea, northeastern Mediterranean. Mar. Ecol. 35, 96–105. doi: 10.1111/maec.12059
Giakoumi S., Cebrian E., Kokkoris G. D., Ballesteros E., Sala E. (2012). Relationships between fish, sea urchins and macroalgae: the structure of shallow rocky sublittoral communities in the Cyclades, Eastern Mediterranean. Estuar. Coast. Shelf Sci. 109, 1–10. doi: 10.1016/j.ecss.2011.06.004
Gianni F., Bartolini F., Airoldi L., Ballesteros E., Francour P., Guidetti P., et al. (2013). Conservation and restoration of marine forests in the Mediterranean Sea and the potential role of marine protected areas. Adv. Oceanogr. Limnol. 4, 83–101. doi: 10.1080/19475721.2013.845604
Gianni F., Bartolini F., Airoldi L., Mangialajo L. (2018). Reduction of herbivorous fish pressure can facilitate focal algal species forestation on artificial structures. Mar. Environ. Res. 138, 102–109. doi: 10.1016/j.marenvres.2018.04.007
Gianni F., Bartolini F., Pey A., Laurent M., Martins G. M., Airoldi L., et al. (2017). Threats to large brown algal forests in temperate seas: the overlooked role of native herbivorous fish. Sci. Rep. 7, 6012. doi: 10.1038/s41598-017-06394-7
Gianni F., Mačić V., Bartolini F., Pey A., Laurent M., Mangialajo L. (2020). Optimizing canopy-forming algae conservation and restoration with a new herbivorous fish deterrent device. Restor. Ecol. 28, 750–756. doi: 10.1111/rec.13143
Gozler A. M., Kopuz U., Agirbas E. (2010). Seasonal changes of invertebrate fauna associated with Cystoseira barbata facies of southeastern Black Sea coast. Afr. J. Biotechnol. 9, 8852–8859. doi: 10.4314/ajb.v9i51
Guarino S. M., Gambardella C., De Nicola (1993). Biology and population dynamics of Idotea baltica (Crustacea, isopoda) in the gulf of Naples, the tyrrhenian Sea. Vie Milieu Life Environ. 43, 125–136.
Guidetti P. (2011). The destructive date-mussel fishery and the persistence of barrens in Mediterranean rocky reefs. Mar. pollut. Bull. 62, 691–695. doi: 10.1016/j.marpolbul.2011.01.029
Guidetti P., Dulčić J. (2007). Relationships among predatory fish, sea urchins and barrens in Mediterranean rocky reefs across a latitudinal gradient. Mar. Environ. Res. 63, 168–184. doi: 10.1016/j.marenvres.2006.08.002
Guidetti P., Fraschetti S., Terlizzi A., Boero F. (2003). Distribution patterns of sea urchins and barrens in shallow Mediterranean rocky reefs impacted by the illegal fishery of the rock-boring mollusc Lithophaga lithophaga. Mar. Biol. 143, 1135–1142. doi: 10.1007/s00227-003-1163-z
Gunnarsson K., Berglund A. (2012). The brown alga Fucus radicans suffers heavy grazing by the isopod Idotea baltica. Mar. Biol. Res. 8, 87–89. doi: 10.1080/17451000.2011.594890
Gutow L., Rahman M. M., Bartl K., Saborowski R., Bartsch I., Wiencke C. (2014). Ocean acidification affects growth but not nutritional quality of the seaweed Fucus vesiculosus (Phaeophyceae, fucales). J. Exp. Mar. Biol. Ecol. 453, 84–90. doi: 10.1016/j.jembe.2014.01.005
Halpern B. S. (2004). Habitat bottlenecks in stage-structured species: hermit crabs as a model system. Mar. Ecol. Prog. Ser. 276, 197–207. doi: 10.3354/meps276197
Hereu B., Zabala M., Sala E. (2008). Multiple controls of community structure and dynamics in a sublittoral marine environment. Ecology 89, 3423–3435. doi: 10.1890/07-0613.1
Hong S., Kim J., Ko Y. W., Yang K. M., Macias D., Kim J.H. (2021). Effects of sea urchin and herbivorous gastropod removal, coupled with transplantation, on seaweed forest restoration. Bot. Mar. 64, 438. doi: 10.1515/bot-2021-0043
Illa-López L., Aubach-Masip À., Alcoverro T., Ceccherelli G., Piazzi L., Kleitou P., et al. (2023). Nutrient conditions determine the strength of herbivore-mediated stabilizing feedbacks in barrens. Ecol. Evol. 13, e9929. doi: 10.1002/ece3.9929
Irving A. D., Balata D., Colosio F., Ferrando G. A., Airoldi L. (2009). Light, sediment, temperature, and the early life-history of the habitat-forming alga Cystoseira barbata. Mar. Biol. 156, 1223–1231. doi: 10.1007/s00227-009-1164-7
Jethva S., Liversage K., Kundu R. (2022). Does topography of rocky intertidal habitat affect aggregation of cerithiid gastropods and co-occurring macroinvertebrates? Oceanologia 64, 387–395. doi: 10.1016/j.oceano.2022.01.006
Jonne K., Helen O.-K., Tiina P., Ilmar K., Henn K. (2006). Seasonal changes in situ grazing of the mesoherbivores Idotea baltica and Gammarus oceanicus on the brown algae Fucus vesiculosus and Pylaiella littoralis in the central gulf of Finland, Baltic Sea. Hydrobiologia 554, 117–125. doi: 10.1007/s10750-005-1011-x
Kinnby A., Toth G. B., Pavia H. (2021). Climate change increases susceptibility to grazers in a foundation seaweed. Front. Mar. Sci. 8. doi: 10.3389/fmars.2021.688406
Korpinen S., Honkanen T., Vesakoski O., Hemmi A., Koivikko R., Loponen J., et al. (2007). Macroalgal communities face the challenge of changing biotic interactions: review with focus on the Baltic Sea. AMBIO J. Hum. Environ. 36, 203–211. doi: 10.1579/0044-7447(2007)36[203:MCFTCO]2.0.CO;2
Kotta J., Paalme T., Martin G., Mäkinen A. (2000). Major changes in macroalgae community composition affectthe food and habitat preference of Idotea baltica. Int. Rev. Hydrobiol. 85, 697–705. doi: 10.1002/1522-2632(200011)85:5/6<697::AID-IROH697>3.0.CO;2-0
Krumhansl K. A., Okamoto D. K., Rassweiler A., Novak M., Bolton J. J., Cavanaugh K. C., et al. (2016). Global patterns of kelp forest change over the past half-century. Proc. Natl. Acad. Sci. 113, 13785–13790. doi: 10.1073/pnas.1606102113
Lavaut E., Guillemin M.-L., Colin S., Faure A., Coudret J., Destombe C., et al. (2022). Pollinators of the sea: a discovery of animal-mediated fertilization in seaweed. Science 377, 528–530. doi: 10.1126/science.abo6661
Lenth R. V., Buerkner P., Herve M., Love J., Miguez F., Riebl H., et al. (2022). Emmeans: estimated marginal means, aka least-squares means. J. Stat. Software 69, 1–33. doi: 10.1080/00031305.1980.10483031
Lotze H. K., Worm B., Sommer U. (2001). Strong bottom-up and top-down control of early life stages of macroalgae. Limnol. Oceanogr. 46, 749–757. doi: 10.4319/lo.2001.46.4.0749
Lubchenco J. (1983). Littornia and Fucus: effects of herbivores, substratum heterogeneity, and plant escapes during succession. Ecology 64, 1116–1123. doi: 10.2307/1937822
Lubchenco J. (1986). Relative importance of competition and predation: early colonization by seaweeds in new England. Comm. Ecol. 537–555.
Mangialajo L., Chiantore M., Susini M.-L., Meinesz A., Cattaneo-Vietti R., Thibaut T. (2012). Zonation patterns and interspecific relationships of fucoids in microtidal environments. J. Exp. Mar. Biol. Ecol. 412, 72–80. doi: 10.1016/j.jembe.2011.10.031
Mariani S., Cefalì M. E., Chappuis E., Terradas M., Pinedo S., Torras X., et al. (2019). Past and present of fucales from shallow and sheltered shores in Catalonia. Reg. Stud. Mar. Sci. 32, 100824. doi: 10.1016/j.rsma.2019.100824
Medrano A., Hereu B., Cleminson M., Pagès-Escolà M., Rovira G., Solà J., et al. (2020). From marine deserts to algal beds: Treptacantha elegans revegetation to reverse stable degraded ecosystems inside and outside a no-take marine reserve. Restor. Ecol. 28, 632–644. doi: 10.1111/rec.13123
Miller K., Blain C., Shears N. (2022). Sea Urchin removal as a tool for macroalgal restoration: a review on removing “the spiny enemies”. Front. Mar. Sci. 9. doi: 10.3389/fmars.2022.831001
Mitterwallner V., Suci A. N. N., Zamani N., Lenz M. (2021). Exposure to moderately elevated temperatures changes food preferences in the tropical marine herbivore Haliotis squamata. Mar. Biol. 168, 110. doi: 10.1007/s00227-021-03922-y
Molinari - Novoa E. A., Guiry E. (2020). Reinstatement of the genera Gongolaria Boehmer and Ericaria Stackhouse (Sargassaceae, phaeophyceae). Notulae Algarum 172, 1–10.
Molis M., Enge A., Karsten U. (2010). Grazing impact of, and indirect interactions between mesograzers associated with kelp (Laminaria digitata)1. J. Phycol. 46, 76–84. doi: 10.1111/j.1529-8817.2009.00787.x
Navarro-Barranco C., Lanza-Arroyo P., Serrano J. G., Rocha J. M. D. (2022). Amphipod assemblages associated with native habitat-forming seaweeds of the Alboran Sea: influence of environmental protection and biogeographical patterns. Mar. Freshw. Res. 74(1) 50–64. doi: 10.1071/MF22080
Orfanidis S., Rindi F., Cebrian E., Fraschetti S., Nasto I., Taskin E., et al. (2021). Effects of natural and anthropogenic stressors on fucalean brown seaweeds across different spatial scales in the Mediterranean Sea. Front. Mar. Sci. 8. doi: 10.3389/fmars.2021.658417
Papadakis O., Tsirintanis K., Lioupa V., Katsanevakis S. (2021). The neglected role of omnivore fish in the overgrazing of Mediterranean rocky reefs. Mar. Ecol. Prog. Ser. 673, 107–116. doi: 10.3354/meps13810
Perkol-Finkel S., Ferrario F., Nicotera V., Airoldi L. (2012). Conservation challenges in urban seascapes: promoting the growth of threatened species on coastal infrastructures. J. Appl. Ecol. 49, 1457–1466. doi: 10.1111/j.1365-2664.2012.02204.x
Pessarrodona A., Assis J., Filbee-Dexter K., Burrows M. T., Gattuso J.-P., Duarte C. M., et al. (2022). Global seaweed productivity. Sci. Adv. 8, eabn2465. doi: 10.1126/sciadv.abn2465
Pessarrodona A., Filbee-Dexter K., Alcoverro T., Boada J., Feehan C. J., Fredriksen S., et al. (2021). Homogenization and miniaturization of habitat structure in temperate marine forests. Glob. Change Biol. 27, 5262–5275. doi: 10.1111/gcb.15759
Pinheiro J. C., Bates D. M. (Eds.) (2000). “Linear mixed-effects models: basic concepts and examples,” in Mixed-effects models in sand s-PLUS statistics and computing (New York, NY: Springer), 3–56. doi: 10.1007/978-1-4419-0318-1_1
Raddatz S., Guy-Haim T., Rilov G., Wahl M. (2017). Future warming and acidification effects on anti-fouling and anti-herbivory traits of the brown alga Fucus vesiculosus (Phaeophyceae). J. Phycol. 53, 44–58. doi: 10.1111/jpy.12473
R Core Team (2019). R: a language and environment for statistical computing. R Foundation for Statistical Computing, Vienna, Austria.
Ruitton S., Francour P., Boudouresque C. F. (2000). Relationships between algae, benthic herbivorous invertebrates and fishes in rocky sublittoral communities of a temperate Sea (Mediterranean). Estuar. Coast. Shelf Sci. 50, 217–230. doi: 10.1006/ecss.1999.0546
Sala E., Ballesteros E. (1997). Partitioning of space and food resources by three fish of the genus Diplodus (Sparidae) in a Mediterranean rocky infralittoral ecosystem. Mar. Ecol. Prog. Ser. 152, 273–283. doi: 10.3354/meps152273
Sala E., Boudouresque C. F., Harmelin-Vivien M. (1998a). Fishing, trophic cascades, and the structure of algal assemblages: evaluation of an old but untested paradigm. Oikos 82, 425–439. doi: 10.2307/3546364
Sala E., Kizilkaya Z., Yildirim D., Ballesteros E. (2011). Alien marine fishes deplete algal biomass in the Eastern Mediterranean. PloS One 6, e17356. doi: 10.1371/journal.pone.0017356
Sala E., Ribes M., Hereu B., Zabala M., Alvà V., Coma R., et al. (1998b). Temporal variability in abundance of the sea urchins Paracentrotus lividus and Arbacia lixula in the northwestern Mediterranean: comparison between a marine reserve and an unprotected area. Mar. Ecol. Prog. Ser. 168, 135–145. doi: 10.3354/meps168135
Savonitto G., de la Fuente G., Tordoni E., Ciriaco S., Srijemsi M., Bacaro G., et al. (2021). Addressing reproductive stochasticity and grazing impacts in the restoration of a canopy-forming brown alga by implementing mitigation solutions. Aquat. Conserv. Mar. Freshw. Ecosyst. 31, 1611–1623. doi: 10.1002/aqc.3555
Schaal G., Leclerc J.-C., Droual G., Leroux C., Riera P. (2016). Biodiversity and trophic structure of invertebrate assemblages associated with understorey red algae in a Laminaria digitata bed. Mar. Biol. Res. 12, 513–523. doi: 10.1080/17451000.2016.1164318
Scheibling R. E., Hennigar A. W., Balch T. (1999). Destructive grazing, epiphytism, and disease: the dynamics of sea urchin - kelp interactions in Nova Scotia. Can. J. Fish. Aquat. Sci. 56, 2300–2314. doi: 10.1139/f99-163
Schiel D. R., Foster M. S. (2006). The population biology of Large brown seaweeds: ecological consequences of multiphase life histories in dynamic coastal environments. Annu. Rev. Ecol. Evol. Syst. 37, 343–372. doi: 10.1146/annurev.ecolsys.37.091305.110251
Sharma R., Swearer S. E., Morris R. L., Strain E. M. A. (2021). Testing the efficacy of sea urchin exclusion methods for restoring kelp. Mar. Environ. Res. 107, 105439. doi: 10.1016/j.marenvres.2021.105439
Smale D. A. (2020). Impacts of ocean warming on kelp forest ecosystems. New Phytol. 225, 1447–1454. doi: 10.1111/nph.16107
Smith K. E., Moore P. J., King N. G., Smale D. A. (2022). Examining the influence of regional-scale variability in temperature and light availability on the depth distribution of subtidal kelp forests. Limnol. Oceanogr. 67, 314–328. doi: 10.1002/lno.11994
Suzuki H., Kubo Y., Inomata E., Agatsuma Y., Aoki M. N. (2020). Effects of herbivorous gastropod grazing on the sedimentation and succession of subtidal macroalgal assemblages. Mar. Ecol. Prog. Ser. 656, 123–138. doi: 10.3354/meps13582
Tamburello L., Papa L., Guarnieri G., Basconi L., Zampardi S., Scipione M. B., et al. (2019a). Are we ready for scaling up restoration actions? an insight from Mediterranean macroalgal canopies. PloS One 14, e0224477. doi: 10.1371/journal.pone.0224477
Tamburello L., Ravaglioli C., Mori G., Nuccio C., Bulleri F. (2019b). Enhanced nutrient loading and herbivory do not depress the resilience of subtidal canopy forests in Mediterranean oligotrophic waters. Mar. Environ. Res. 149, 7–17. doi: 10.1016/j.marenvres.2019.05.015
Tegner M. J., Dayton P. K., Edwards P. B., Riser K. L. (1995). Sea Urchin cavitation of giant kelp (Macrocystis pyrifera C. Agardh) holdfasts and its effects on kelp mortality across a large California forest. J. Exp. Mar. Biol. Ecol. 191, 83–99. doi: 10.1016/0022-0981(95)00053-T
Thibaut T., Blanfune A., Boudouresque C.-F., Verlaque M. (2015). Decline and local extinction of fucales in French Riviera: the harbinger of future extinctions? Mediterr. Mar. Sci. 16, 206–224. doi: 10.12681/mms.1032
Vanderklift M. A., Lavery P. S., Waddington K. I. (2009). Intensity of herbivory on kelp by fish and sea urchins differs between inshore and offshore reefs. Mar. Ecol. Prog. Ser. 376, 203–211. doi: 10.3354/meps07811
Verdura J., Sales M., Ballesteros E., Cefalì M. E., Cebrian E. (2018). Restoration of a canopy-forming alga based on recruitment enhancement: methods and long-term success assessment. Front. Plant Sci. 9. doi: 10.3389/fpls.2018.01832
Verdura J., Santamaría J., Ballesteros E., Smale D., Cefalì M. E., Golo R., et al. (2021). Local-scale climatic refugia offer sanctuary for a habitat-forming species during a marine heatwaves. J. Ecol. 109, 1758–1773. doi: 10.1111/1365-2745.13599
Vergés A., Alcoverro T., Ballesteros E. (2009). Role of fish herbivory in structuring the vertical distribution of canopy algae Cystoseira spp. in the Mediterranean Sea. Mar. Ecol. Prog. Ser. 375, 1–11. doi: 10.3354/meps07778
Vergés A., Becerro M. A., Alcoverro T., Romero J. (2007). Experimental evidence of chemical deterrence against multiple herbivores in the seagrass Posidonia oceanica. Mar. Ecol. Prog. Ser. 343, 107–114. doi: 10.3354/meps06885
Vergés A., Doropoulos C., Malcolm H. A., Skye M., Garcia-Pizá M., Marzinelli E. M., et al. (2016). Long-term empirical evidence of ocean warming leading to tropicalization of fish communities, increased herbivory, and loss of kelp. Proc. Natl. Acad. Sci. 113, 13791–13796. doi: 10.1073/pnas.1610725113
Vergés A., Tomas F., Cebrian E., Ballesteros E., Kizilkaya Z., Dendrinos P., et al. (2014). Tropical rabbitfish and the deforestation of a warming temperate sea. J. Ecol. 102, 1518–1527. doi: 10.1111/1365-2745.12324
Verlaque M. (1990). Relations entre Sarpa salpa (Linnaeus 1758) (Téléostéen, sparidae), les autres poissons broteurs et le phytobenthos algal méditerranéen. Oceanol Acta 13 (3), 373–388.
Verlaque M., Boudouresque C.-F., Perret-Boudouresque M. (2019). Mediterranean Seaweeds listed as threatened under the Barcelona convention: a critical analysis. Sci. Rep. Port-Cros Natl. Park 33, 179–214.
Vesakoski O., Boström C., Ramsay T., Jormalainen V. (2008). Sexual and local divergence in host exploitation in the marine herbivore Idotea baltica (Isopoda). J. Exp. Mar. Biol. Ecol. 367, 118–126. doi: 10.1016/j.jembe.2008.09.006
Wernberg T., Filbee-Dexter K. (2019). Missing the marine forest for the trees. Mar. Ecol. Prog. Ser. 612, 209–215. doi: 10.3354/meps12867
Wernberg T., Krumhansl K., Filbee-Dexter K., Pedersen M. F. (2019). Status and trends for the world’s kelp forests, in World seas: an environmental evaluation (Amsterdam: Elsevier), 57–78. doi: 10.1016/B978-0-12-805052-1.00003-6
Keywords: marine forests, Cystoseira s.l., Cystoseira compressa, herbivory, plant-herbivore interactions, grazing, restoration, mesograzers
Citation: Monserrat M, Verdura J, Comeau S, Cottalorda J-M, Priouzeau F, Romero G and Mangialajo L (2023) The role of grazers in early-life stages of Cystoseira sensu lato can be crucial in the restoration of marine forests. Front. Mar. Sci. 10:1176780. doi: 10.3389/fmars.2023.1176780
Received: 28 February 2023; Accepted: 30 May 2023;
Published: 28 June 2023.
Edited by:
Ronald Osinga, Wageningen University and Research, NetherlandsReviewed by:
Sotiris Orfanidis, Institute of Fisheries Research, GreeceCopyright © 2023 Monserrat, Verdura, Comeau, Cottalorda, Priouzeau, Romero and Mangialajo. This is an open-access article distributed under the terms of the Creative Commons Attribution License (CC BY). The use, distribution or reproduction in other forums is permitted, provided the original author(s) and the copyright owner(s) are credited and that the original publication in this journal is cited, in accordance with accepted academic practice. No use, distribution or reproduction is permitted which does not comply with these terms.
*Correspondence: Margalida Monserrat, bW1vbnNlcnJhdGJAb3V0bG9vay5jb20=; Jana Verdura, amFuYS52ZXJkdXJhQHVuaXYtY290ZWRhenVyLmZy
†Present address: Margalida Monserrat, CoNISMa, Roma, Italy
‡These authors have contributed equally to this work and share first authorship
Disclaimer: All claims expressed in this article are solely those of the authors and do not necessarily represent those of their affiliated organizations, or those of the publisher, the editors and the reviewers. Any product that may be evaluated in this article or claim that may be made by its manufacturer is not guaranteed or endorsed by the publisher.
Research integrity at Frontiers
Learn more about the work of our research integrity team to safeguard the quality of each article we publish.