- Department of Biology and Environmental Science, Ecology and Evolution in Microbial Model Systems (EEMiS), Linnaeus University, Kalmar, Sweden
Climate change causes earlier and warmer springs in seasonal environments and a higher incidence of extreme weather events. In aquatic environments, this changes the thermal conditions during spawning, and the thermal performance of eggs and embryos may determine the consequences of climate change on recruitment. In iteroparous species with indeterminate growth, the eggs produced by a given female in successive years will increase in size as the female grows larger and likely be exposed to different temperatures during incubation due to annual variation in spring phenology. Still, we know little about whether differences in maternal size impact the temperature-dependent performance and viability of the offspring. Here we utilised a thermal gradient laboratory experiment on Baltic Sea perch (Perca fluviatilis) to investigate how maternal size influence the temperature dependent hatching success of the offspring. The results uncovered a positive relationship between maternal size and average hatching success, but the shape of the relationship (reaction norm) linking hatching success to incubation temperature was independent of maternal size. However, we did find an association between maternal size and the variance (S.D. and CV) in hatching success across temperatures, with larger females producing offspring with maintained performance (less sensitive) across temperature treatments, indicative of flatter reaction norms and broader thermal niches. This suggests that maintaining the size distribution of fish populations, for instance through regulations of size-selective fisheries, may be important to aid the long-term productivity and viability of fish populations and ultimately conserve the function and services of ecosystems.
1 Introduction
Global warming is impacting the thermal conditions that organisms experience during key life-history events, such as reproduction and embryo development, which may ultimately influence the dynamics and viability of populations and species (Dahlke et al., 2020). In high latitude biomes, global warming is already causing altered seasonality, increased annual mean temperatures and a higher incidence of extreme weather events that generate more variable and unpredictable thermal conditions among and within years. These effects are also predicted to increase in severity over the next decades (Tamarin-Brodsky et al., 2020). The capacity to tolerate high, variable, and unpredictable thermal conditions during life-history events, such as reproduction, is thus assumed critical for organisms to cope with climate change (Dahlke et al., 2020; Morgan et al., 2020). Previous research has highlighted that this may be mediated both through adaptive evolution of, and phenotypic plasticity in, thermal performance of eggs and embryos (Salinas and Munch, 2012; Seebacher et al., 2015; Chen et al., 2018). This is supported by a firm body of evidence based on comparisons of thermal performance and tolerance among species and populations inhabiting, and exposed to, different thermal regimes (Comte and Olden, 2017; Sunde et al., 2019; Carbonell et al., 2021). Compared with the existing work and understanding of the mechanistic underpinnings of variation among populations and species, much less is known regarding the sources of variation in thermal performance (tolerance) among individuals within populations. For example, a better knowledge of the sources of variation in the thermal performance and tolerance of offspring within and between different families, including the relative contribution of genes, developmental plasticity and maternal effects, is key for understanding evolutionary modifications of thermal tolerance and to generate predictive models on the consequences of global warming for biodiversity and the function and services of ecosystems (Salinas and Munch, 2012; Wennersten and Forsman, 2012; Forsman, 2015; Hall et al., 2021).
Maternal size affects the allocation of resources for reproduction and the distribution of those resources among offspring in most vertebrates (Roff, 1992; Marshall et al., 2018). For example, in fish, larger females tend to produce more and larger eggs with higher nutrient content (Barneche et al., 2018), which can lead to increased offspring size and survival (Heath et al., 1999; Kamler, 2005; Hixon et al., 2014). It is also known that oxygen consumption and metabolic rates often increases with increasing egg size. These are physiological factors that can influence susceptibility to thermal stress, especially in aquatic environments where oxygen solubility decreases with increasing temperature (Martin et al., 2017). From a mechanistic perspective, it has been proposed that because smaller eggs have a higher surface to volume ratio the oxygen demand of the developing embryos can more easily be met (via diffusion) in small than in large eggs, such that smaller eggs should be favorable in low oxygen (i.e. warm) environments (van den Berghe and Gross, 1989; Robertson and Collin, 2015). Still, there are also examples to the opposite effect where larger eggs perform better in low oxygen environments (Einum et al., 2002; Hendry and Day, 2003). Together, this means that there is potential for maternal size to also impact offspring performance in different temperatures, due to size-dependent changes in egg size, although evidence for this effect is limited (Martin et al., 2020; Olin et al., 2022).
Important knowledge on how and why species, populations, and individuals may respond differently to changes in temperature can be achieved by studying their performance under various thermal conditions (i.e., reaction norms or performance curves)(Via et al., 1995; Hall et al., 2021). Flat (shallow) reaction norms would suggest tolerance to environmental fluctuations (i.e., maintained performance), while narrow curvilinear responses are instead indicative of a narrow environmental range for optimal performance, and directional reaction norms suggest that optimal performance peaks at one end of the environmental spectrum. Directional and/or narrow curvilinear recreation norms may increase vulnerability to temperature variation because they reduce the ability to adjust to changing temperatures without incurring fitness costs (Hall et al., 2021). However, not only the shapes of reaction norms are informative concerning the response of genotypes to different environments but also the sensitivity in performance since it may influence population dynamics (e.g. to what degree the number of recruits may be impacted in different environments).
This study aimed to determine whether and how maternal size affects offspring quality and the offspring’s ability to perform in different thermal conditions by examining the size-dependent hatching success of anadromous Baltic Sea perch (Perca fluviatilis) using a split-brood laboratory temperature gradient experiment. Perch is an iteroparous total spawner with females depositing their eggs in a single egg strand at a specific location and at a single time point (Craig, 2000). The incubation of eggs until hatching is subjected to variable thermal conditions depending on the timing of egg deposition and the prevailing spring phenology (Tibblin et al., 2012; Hall et al., 2021) such that maintained offspring performance in different temperatures may be key for individual reproductive success. For this study, we collected eggs from 69 newly deposited egg strands (the total weight of which served as a proxy for maternal size similar to Lang, 1987) in the field and conducted an experiment in which eggs from the focal females were incubated in the laboratory at four different temperatures (10, 12, 15, and 18 ˚C). The experiment was designed to answer the following questions: (1) Does maternal size influence the overall hatching success?; (2) Does the shape of reaction norms and/or the sensitivity of egg hatching success to temperature vary among families depending on maternal size?; and (3) Could size-dependent maternal effects on thermal sensitivity of offspring performance be accounted for by differences in egg size?
2 Methods
2.1 Field collection of egg strands
In 2019, we conducted a field survey to identify and sample perch egg strands in the lower stretches of Hossmoån river (56°C 37.530’N, 16°C 14.331’E southeast Sweden) which have previously been identified as an important spawning habitat for Baltic Sea anadromous perch (Tibblin et al., 2012). Perch typically spawns in shallow water and their eggs can be detected from their characteristic egg strands which are highly visible within reasonable sight depth conditions, as is the case for perch in Hossmoån (Tibblin et al., 2012; Hall et al., 2021). The study took place from April 3 to June 24 during which we identified 511 egg strands (unique females spawning) along transects set in the lower stretch of the river. We monitored the transects three days a week using a combination of polarizing glasses and a bathyscope to detect the egg strands. Each detected egg strand was marked with a red cellulose tape in the nearest reed strand, and its location was marked on a map to avoid double sampling of egg strands. During each sampling occasion (day), we sampled 3-6 of the newly detected egg strands (pending availably of egg strands). The egg strands sampled were chosen haphazardly but with the aim to comprise size variation among strands during each sample occasion. In sampling the egg strands, we first gently loosened them from the spawning substrate (typically reed), and then transferred the egg strand as a whole to a water-filled bucket using a fine-meshed net (see Hall et al., 2021 for details) after which it was weighed (gram wet weight). From each of the weighed egg strands, we took a ~10 cm subsample which was placed in a small water-filled (stream water) container (0.8 L) and brought to the to the laboratory facility at the Linnaeus University. At the laboratory, we divided each egg strand sample into four subsamples after which they were randomly distributed to the different temperature-controlled rooms, set at 10, 12, 15 and 18 C°, to be used for the split-brood temperature hatching experiment.
2.2 Hatching experiment in the laboratory
Once the subsamples had been brought into each of the temperature-controlled rooms and allowed to slowly adjust to room temperatures (~1h), we cut out two replicates (replicate weight 1.23 g ± 0.18 S.D.) from each subsample per temperature treatment. We then transferred each replicate to 0.8 L containers, filled with aerated room temperature adjusted tap-water. The container was made of two stacked 0.8 L containers, with the bottom part cut out of the top container and replaced with a 1.5*1.5 mm mesh-net to allow for throughflow during water exchanges (as water flows out from the bottom container). The containers were placed on racks within the temperature-controlled rooms, with an average light intensity of ~60 πE/m2/S. The position of each sample and replicate was assigned randomly prior to the start of the experiment, to minimize potential bias stemming from temperature and light differences within the racks. We conducted complete water exchanges regularly (daily in the 15 and 18 C° temperature-controlled room, and about every second day in the 10 and 12 C° rooms), by overflowing the containers with treatment specific temperature adjusted water. This was done to maintain high oxygen concentrations throughout the incubation period and to remove biological byproducts.
To capture the initial number of eggs and number of viable eggs in each replicate, we took a picture of each replicate at the start of the laboratory experiment (Nikon D5600, F/7.1, 1/60 sec, ISO-400, Focal length 50 mm). In a similar fashion, we also captured images of hatched larvae at the end of the experiment (Nikon D5600, F/11, 1/80 sec, ISO-400, Focal length 50 mm). These images provided us with a starting estimate of the number of viable eggs in each replicate, and the final number of hatched larvae (i.e. hatching success).
2.2.1 Counting of eggs and larvae and estimating larvae length
We counted the initial number of eggs and the number of viable eggs in each replicate using ImageJs multipoint tool (Schneider et al., 2012), we excluded dead eggs (opaque) from the estimate of viable eggs. We also counted the number of hatched larvae at the end of the experiment, using the same approach. Hatching success was then estimated as the number of hatched larvae/viable eggs.
2.3 Statistics
All statistical analyses were performed in R v. 3.5.2 and Rstudio v. 1.1.46 (Team, R. S, 2016; Team, R. C, 2018).
2.3.1 Maternal size effects on hatching success
To examine whether and how maternal size influenced hatching success, we used a linear mixed model with hatching success set as the response variable, egg strand size as a continuous fixed effect and family was included as a random effect. Here, we included all egg strand samples (n = 67 in the analysis, while later analyses focused on families with an overall hatching success above 5% to examine the influence of maternal size on thermal performance.
2.3.2 Maternal size and its influence on offspring reaction norms and thermal performance
To examine whether and how maternal size influenced the shape of the reaction norm linking hatching success to incubation temperature we first excluded egg strands (families) with a hatching success lower than 5% in all incubation temperatures. These egg strand samples were excluded to avoid estimating reaction norms and variance estimates from samples with 0 - few hatching individuals, which could severely influence the shape of reaction norms and variance estimates without reflecting true differences in thermal performance (e.g., due to differences in infections/virulence among the replicates). Following that, we used a generalized linear mixed model (GLMM) with a binomial error structure to estimate whether and how the thermal reaction norm changed with maternal size. This was analyzed using the glmer function in the lme4-package (Bates et al., 2014). Here, we set hatching success as the response variable, egg strand size (total weight in gram) and incubation temperature as continuous fixed effects with the effect of incubation temperature estimated using a curvilinear function, and with the interaction between these two factors included to examine whether size influenced temperatures effect on hatching success (i.e., the shape of the reaction norm). Family and its interaction with temperature (family-by-temperature interaction) was included as a random effect to account for among family variation in the temperature dependent reaction norms (slopes), again using a curvilinear function for the effect of temperature. Significance of fixed effects were evaluated with a type II Wald’s chi-square test.
To further investigate how maternal size influenced the thermal sensitivity of performance of the offspring, we used variance estimates to investigate the variance in hatching success among the temperature treatments. Here, we first calculated the mean hatching success within each temperature treatment for each family (from the two replicates). These mean values of hatching success in the temperature treatments (10, 12, 15 and 18 °C) were then used to estimate the standard deviation (S.D.) and the coefficient of variance (CV) in hatching success. Thereafter, we examined the relationship between egg strand size and variance estimates (S.D. and CV, respectively) with a linear regression, using the lm function in R.
2.3.3 Egg size and its influence on temperature-dependent hatching success
As egg size influences metabolism and oxygen diffusion, both of which depend on temperature, and commonly increases with maternal size in fish, we wanted to examine whether egg size per se was associated with changes in the thermal performance of the offspring. We first examined if egg size also increased with increasing maternal size (egg strand size) within this population. Egg size was estimated by dividing the weight of each replicate with its initial number of eggs, and then averaged across replicates and treatments to gather a robust estimate of the average egg size for each egg strand. We then assessed the relationship between egg size and the size of the egg strand with a linear regression, again using the lm function in R. Finally, we examined whether and how egg size influenced the thermal sensitivity of offspring performance across the temperature treatments. Here, the variance estimates (S.D. and CV, respectively) were set as response variables and egg size were set as the explanatory variable, and its relationship was analysed with a linear regression, again using the lm function in R.
2.4 Ethical approval
The laboratory was approved as research facility (Dnr 5.2.18–17988/18), and the study was granted ethical approval (approval Dnr 168677-2018) by the Ethical Committee on Animal Experiments in Linköping, Swedish Board of Agriculture, Sweden.
3 Results
3.1 Maternal size and offspring success
Egg strand sizes varied between 31 and 1502 g, showcasing a substantial variation in the size of the reproducing individuals. These maternal size differences were associated with differences in hatching success, with larger egg strands (females) having an overall higher hatching success (χ2 = 12.08, d.f. = 1, p < 0.001, Figure 1).
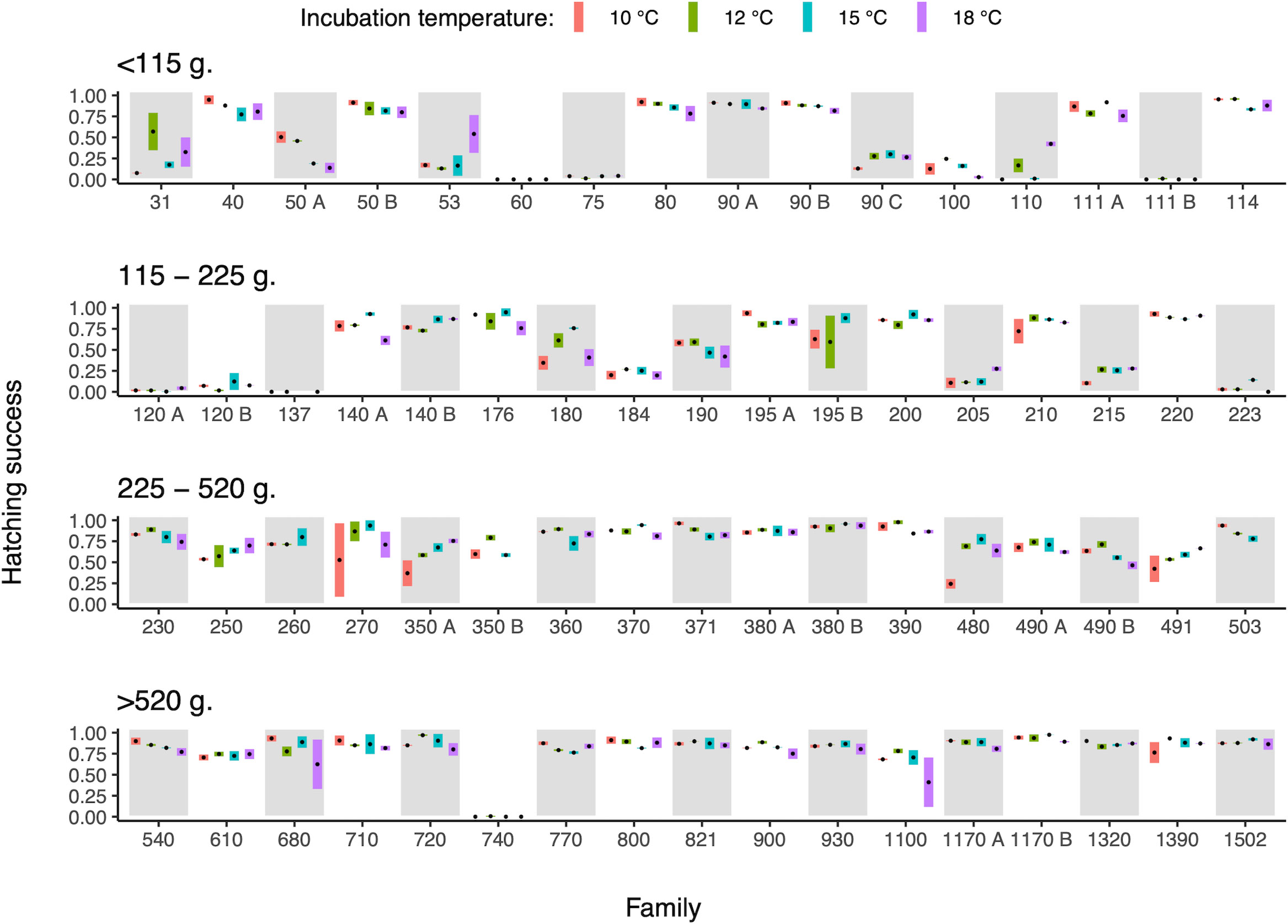
Figure 1 Relationship between maternal size (estimated through egg strand weight) and hatching success of the eggs when exposed to different incubation temperatures. The bar within each temperature treatment shows the range between the two replicates, and the black mark is the mean temperature specific hatching success of that family. Families have been ordered based on egg strand weight and split into four panels with different size classes. Families are labelled with their egg strand weight (g) with the addition of letters (A, B) to separate families that shared the same size. Data from temperature treatments are ordered from lowest to highest temperature within each family.
3.2 Maternal size and its influence on offspring reaction norms and thermal performance
The shape of the reaction norm linking hatching success to incubation temperature did not change with maternal size (egg strand size × incubation temperature interaction; χ2 = 0.73, d.f. = 2, p = 0.69, Figure 1), while temperature and maternal size, respectively, influenced hatching success (egg strand size, χ2 = 10.39, d.f. = 1, p = 0.001; incubation temperature - curvilinear relationship; χ2 = 7.82, d.f. = 2, p = 0.02). However, variance estimates (S.D. and CV) of the temperature dependent hatching success decreased with increasing maternal size (linear regression; S.D., R2 = 0.079, F1,59 = 5.06, p = 0.028; CV, R2 = 0.118, F1,59 = 7.87, p = 0.007; Figure 1), indicating that the performance of offspring produced by larger females was less sensitive to differences in incubation temperature.
3.3 Egg size and its influence on temperature performance
As egg size increased with increasing female size (linear regression; R2 = 0.19, F1,65 = 15.28, p < 0.001; Figure 2), we wanted to examine whether egg size was a driving mechanism behind the variation in thermal performance of offspring according to maternal size. We did this by examining whether egg size was associated with tolerance in temperature performance (S.D. and CV). Here, we found that larger eggs indeed were associated with maintained offspring performance across temperatures (linear regression; S.D., R2 = 0.09 F1, 59 = 5.56, p = 0.022; CV, R2 = 0.10, F1,59 = 6.47, p = 0.0136).
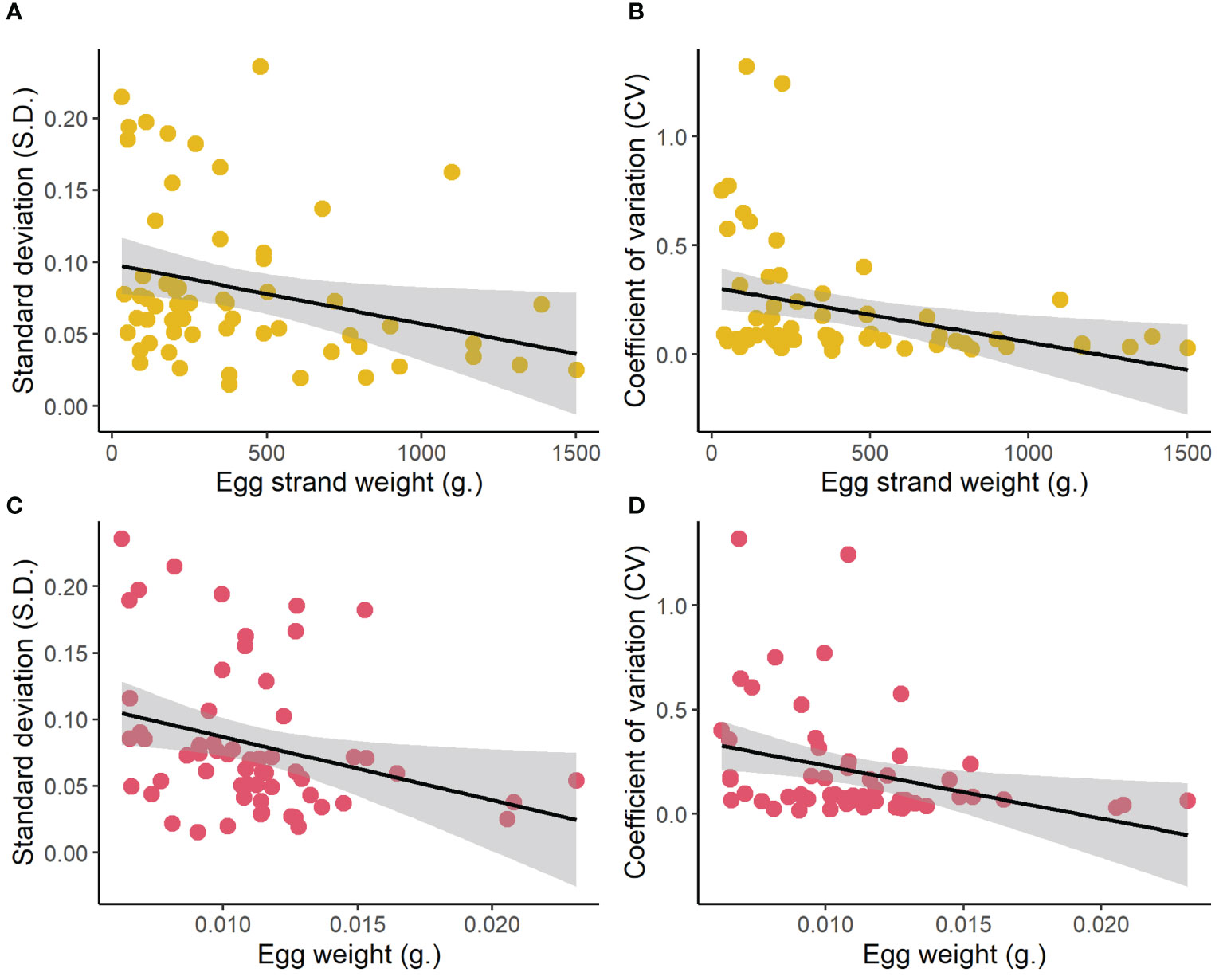
Figure 2 The relationship between: maternal size (egg strand weight) (A, B) and egg size (C, D) with the temperature sensitivity of the offspring estimated as the variance (standard deviation S.D, and coefficient of variance CV) in hatching success across the temperature treatments.
4 Discussion
Thermal regimes are shifting due to climate change which result in higher and more unpredictable temperatures during spawning for fish populations in temperate regions (Lema et al., 2019; IPCC, 2022). Simultaneously, the size distribution of many fish populations is impacted by intense fisheries harvest which typically removes large individuals (Uusi-Heikkilä et al., 2016). This emphasizes the need to understand whether and how the size structure within populations and species impacts their thermal dependence of performance and resilience to climate change. In this study we examined how maternal size of perch was related to offspring size (egg size), offspring quality (hatching success), and whether it influenced the thermal performance of offspring across a laboratory temperature gradient. We found that egg size and the overall hatching success increased with increasing maternal size. We also found that the shape (i.e. slope or curvature) of the reaction norm linking hatching success to incubation temperature did not seem to differ significantly depending on maternal size (as indirectly estimated by the weight of the egg strand). However, the variance estimates of the thermal performance (CV/S.D.) decreased with increasing maternal size, indicating higher tolerance in performance across temperatures in offspring produced by large females. Further analysis indicated that this association between variance in offspring thermal performance and maternal size was mediated by egg size rather than maternal size itself, such that larger eggs had higher tolerance in performance. Although our study does not implicitly investigate whether and how this was related to oxygen conditions, the results support a growing body of evidence that challenge the conventional “bigger is worse during incubation” hypothesis stating that smaller eggs should be favoured in high temperatures due to improved diffusion of oxygen relating to the higher surface to volume ratio (van den Berghe and Gross, 1989; Hendry et al., 2001; Einum et al., 2002; Hendry and Day, 2003; Martin et al., 2017). Together, our findings suggest that maintaining the size structure of fish populations, for instance through fisheries regulations, may be fundamental for the ability to cope with the future climate change (Hidalgo et al., 2011).
Our finding of an increase in egg size with increasing maternal size aligns with numerous previous studies in fish (Berggren et al., 2016; Rollinson and Rowe, 2016), and in perch specifically (Olin et al., 2012), where egg size commonly increases with maternal size. This pattern is also consistent across many other taxa including birds, amphibians and reptiles (see Lim et al., 2014 for a meta-analysis). This points towards a general size dependent shift in the optimal trade-off between fecundity and individual offspring investment, increasing the likelihood that offspring quality also increases with maternal size (Rollinson and Rowe, 2016). Indeed, our results also support this notion as offspring quality, estimated through hatching success, increased with maternal size. This in turn indicates that the size structure within populations could affect the overall recruitment success and ultimately impact population dynamics (Shelton et al., 2006).
Our split-brood hatching experiment across a temperature gradient did not reveal any statistically significant differences in the shape of the reaction norms depending on maternal size. This could perhaps partly be attributed to that smaller females had rather variable reactions norms (positive, negative, and curvilinear) whereas the reaction norms of larger females were predominantly flat (Figure 1) resulting in similar mean reaction norms across size classes. In agreement with this interpretation, the intraindividual variation (CV and S.D.) in hatching success across the temperature gradient decreased with increasing maternal size, indicating that the thermal tolerance of performance of offspring produced by larger individuals was broader. This suggests that the recruitment potential of larger females was less sensitive to thermal variation than that of smaller females (Sinclair et al., 2016). In the light of that climate change is expected to skew the size distributions of many temperate fish species and populations towards smaller individuals (Walters and Hassall, 2006; Estlander et al., 2015; Campana et al., 2020), our result of a positive size-dependent resilience to temperature variation suggests that there may be additive negative effects of climate change on the viability and productivity of populations. This finding also constitutes an important argument to why it is essential for fisheries management to maintain the size distribution of exploited fish stocks in the context of maintaining their capacity to cope with climate change (Hidalgo et al., 2011; Dahlke et al., 2020).
Based on a rather large body of evidence, we predicted that differences in egg size would offer a mechanistic explanation to putative patterns of size-dependent maternal effects on offspring thermal performance (Parker and Begon, 1986; Hendry et al., 2001; Martin et al., 2017) which was indeed supported by our data. However, our results did not cohere to the notion of smaller eggs performing better in high temperatures (and assumedly lower oxygen concentrations) due to improved oxygen diffusion through a higher surface to volume ratio (van den Berghe and Gross, 1989; Fleming and Gross, 1990), instead larger eggs had both higher and less variable performance across temperatures. A similar pattern of a positive association between egg size and thermal performance have been shown in salmonids (Einum et al., 2002) which was attributed to that the rate of oxygen consumption does not, as previously assumed, increase more rapidly with increasing egg size than the rate at which the egg surface area available for oxygen diffusion increases. Unfortunately, our study design does not allow to make any casual inference about the effects of egg size on thermal performance and it is possible that it may be related to other factors correlating with increasing size of the females and the eggs. For instance, the number of spawning events (which is strongly correlated with age and body size) may influence the temperature performance in eggs through epigenetic mechanisms relating to the variation in temperature conditions during spawning experienced by the female (Salinas and Munch, 2012; Tibblin et al., 2016). Another explanation may be that the higher temperature tolerance of offspring produced by larger mothers indicated by our results is accompanied by a higher buffering capacity and temperature tolerance also of other aspects of individual performance, and that broader thermal niches improve survival (Franzen et al., 2022).
Taken together, our results show that larger females, in general, produce larger eggs and eggs with an overall higher quality (increased hatching success). Furthermore, we also found indications of an increased tolerance to different temperature conditions in eggs from larger females, and that these differences may be due to maternally driven differences in egg sizes. At times when many fish populations are challenged by intense size-selective harvest this knowledge emphasize the great value in conserving the natural size distribution to avoid further negative impacts of climate change on the productivity and viability of fish populations.
Data availability statement
The raw data supporting the conclusions of this article will be made available by the authors, without undue reservation.
Ethics statement
The animal study was reviewed and approved by the Ethical Committee on Animal Experiments in Linköping, Swedish Board of Agriculture, Sweden. Dnr 168677-2018.
Author contributions
MH and PT conceived the study. MH, ON, AF and PT designed the study. MH, ON and PT conducted the field and laboratory work. MH analyzed the data with support from PT and AF. All authors contributed to interpretation of results. MH and PT wrote the manuscript, with input from ON and AF. All authors contributed to the revision process and approved the submitted version.
Funding
This work was supported by funds kindly provided by the Swedish Research Council FORMAS (grant 2018-00605 to PT; grant 2017-00346 to AF and PT; and via EcoChange grant to PT).
Acknowledgments
We are grateful to Ole Tiesch, Emma Svahn and Christofer Osbeck for excellent field assistance, and Per Koch-Schmidt, Per Larsson and Johanna Sunde for important input on the research questions and advise on the set-up of the laboratory experiment. We thank Mikko Olin and Xian Sun for constructive feedback during the review process. We are grateful to Hossmo FVOF, Ola Sennefjord and Kjell Linyr for granting us access to the study system.
Conflict of interest
The authors declare that the research was conducted in the absence of any commercial or financial relationships that could be construed as a potential conflict of interest.
Publisher’s note
All claims expressed in this article are solely those of the authors and do not necessarily represent those of their affiliated organizations, or those of the publisher, the editors and the reviewers. Any product that may be evaluated in this article, or claim that may be made by its manufacturer, is not guaranteed or endorsed by the publisher.
References
Barneche D. R., Robertson D. R., White C. R., Marshall D. J. (2018). Fish reproductive-energy output increases disproportionately with body size. Science 360, 642–645. doi: 10.1126/science.aao6868
Bates D., Mächler M., Bolker B., Walker S. (2014). Fitting linear mixed-effects models using lme4. arXiv 1–51. doi: 10.18637/jss,v067.i01
Berggren H., Nordahl O., Tibblin P., Larsson P., Forsman A. (2016). Testing for local adaptation to spawning habitat in sympatric subpopulations of pike by reciprocal translocation of embryos. PloS One 11, e0154488. doi: 10.1371/journal.pone.0154488
Campana S. E., Stefánsdóttir R. B., Jakobsdóttir K., Sólmundsson J. (2020). Shifting fish distributions in warming sub-Arctic oceans. Sci. Rep. 10, 16448. doi: 10.1038/s41598-020-73444-y
Carbonell J. A., Wang Y.-J., Stoks R. (2021). Evolution of cold tolerance and thermal plasticity in life history, behaviour and physiology during a poleward range expansion. J. Anim. Ecol. 90, 1666–1677. doi: 10.1111/1365-2656.13482
Chen Z., Farrell A. P., Matala A., Narum S. R. (2018). Mechanisms of thermal adaptation and evolutionary potential of conspecific populations to changing environments. Mol. Ecol. 27, 659–674. doi: 10.1111/mec.14475
Comte L., Olden J. D. (2017). Evolutionary and environmental determinants of freshwater fish thermal tolerance and plasticity. Global Change Biol. 23, 728–736. doi: 10.1111/gcb.13427
Craig J. F. (2000). Percid fishes: systematics, ecology and exploitation (Oxford: Blackwell Science).
Dahlke F. T., Wohlrab S., Butzin M., Pörtner H.-O. (2020). Thermal bottlenecks in the life cycle define climate vulnerability of fish. Science 369, 65. doi: 10.1126/science.aaz3658
Einum S., Hendry A., Fleming I. (2002). Egg-size evolution in aquatic environments: does oxygen availability constrain size? Proc. R. Soc. B: Biol. Sci. 269, 2325–2330. doi: 10.1098/rspb.2002.2150
Estlander S., Nurminen L., Mrkvička T., Olin M., Rask M., Lehtonen H. (2015). Sex-dependent responses of perch to changes in water clarity and temperature. Ecol. Freshw. Fish 24, 544–552. doi: 10.1111/eff.12167
Fleming I. A., Gross M. R. (1990). Latitudinal clines: a trade-off between egg number and size in pacific salmon. Ecology 71, 2–11. doi: 10.2307/1940241
Forsman A. (2015). Rethinking phenotypic plasticity and its consequences for individuals, populations and species. Heredity 115, 276–284. doi: 10.1038/hdy.2014.92
Franzen M., Francioli Y., Askling J., Kindvall O., Johansson V., Forsman A. (2022). Differences in phenology, daily timing of activity, and associations of temperature utilization with survival in three threatened butterflies. Sci. Rep. 12, 7534. doi: 10.1038/s41598-022-10676-0
Hall M., Nordahl O., Larsson P., Forsman A., Tibblin P. (2021). Intra-population variation in reproductive timing co-varies with thermal plasticity of offspring performance in perch (Perca fluviatilis). J. Anim. Ecol. 90, 2236–2247. doi: 10.1111/1365-2656.13542
Heath D. D., Fox C. W., Heath J. W. (1999). Maternal effects on offspring size: variation through early development of chinook salmon. Evolution 53, 1605–1611. doi: 10.2307/2640906
Hendry A., Day T. (2003). Revisiting the positive correlation between female size and egg size. Evolutionary Ecol. Res. 5, 421–429.
Hendry A. P., Day T., Cooper A. B. (2001). Optimal size and number of propagules: allowance for discrete stages and effects of maternal size on reproductive output and offspring fitness. Am. Nat. 157, 387–407. doi: 10.1086/319316
Hidalgo M., Rouyer T., Molinero J. C., Massutí E., Moranta J., Guijarro B., et al. (2011). Synergistic effects of fishing-induced demographic changes and climate variation on fish population dynamics. Mar. Ecol. Prog. Ser. 426, 1–12. doi: 10.3354/meps09077
Hixon M. A., Johnson D. W., Sogard S. M. (2014). BOFFFFs: on the importance of conserving old-growth age structure in fishery populations. ICES J. Mar. Sci. 71, 2171–2185. doi: 10.1093/icesjms/fst200
IPCC (2022). Climate change 2022: impacts, adaptation, and vulnerability (Cambridge, UK and New York, NY, USA: Cambridge University Press).
Kamler E. (2005). Parent-egg-progeny relationships in teleost fishes: an energetics perspective. Rev. Fish Biol. Fisheries 15, 399. doi: 10.1007/s11160-006-0002-y
Lang C. (1987). Mortality of perch, Perca fluviatilis l., estimated from the size and abundance of egg strands. J. Fish Biol. 31, 715–720. doi: 10.1111/j.1095-8649.1987.tb05274.x
Lema S. C., Bock S. L., Malley M. M., Elkins E. A. (2019). Warming waters beget smaller fish: evidence for reduced size and altered morphology in a desert fish following anthropogenic temperature change. Biol. Lett. 15, 20190518. doi: 10.1098/rsbl.2019.0518
Lim J. N., Senior A. M., Nakagawa S. (2014). Heterogeneity in individual quality and reproductive trade-offs within species. Evolution 68, 2306–2318. doi: 10.1111/evo.12446
Marshall D. J., Pettersen A. K., Cameron H. (2018). A global synthesis of offspring size variation, its eco-evolutionary causes and consequences. Funct. Ecol. 32, 1436–1446. doi: 10.1111/1365-2435.13099
Martin B. T., Dudley P. N., Kashef N. S., Stafford D. M., Reeder W. J., Tonina D., et al. (2020). The biophysical basis of thermal tolerance in fish eggs. Proc. R. Soc. B: Biol. Sci. 287, 20201550. doi: 10.1098/rspb.2020.1550
Martin B. T., Pike A., John S. N., Hamda N., Roberts J., Lindley S. T., et al. (2017). Phenomenological vs. biophysical models of thermal stress in aquatic eggs. Ecol. Lett. 20, 50–59. doi: 10.1111/ele.12705
Morgan R., Finnøen M. H., Jensen H., Pélabon C., Jutfelt F. (2020). Low potential for evolutionary rescue from climate change in a tropical fish. Proc. Natl. Acad. Sci. 117, 33365–33372. doi: 10.1073/pnas.2011419117
Olin M., Jutila J., Lehtonen H., Vinni M., Ruuhijärvi J., Estlander S., et al. (2012). Importance of maternal size on the reproductive success of perch, Perca fluviatilis, in small forest lakes: implications for fisheries management. Fisheries Manage. Ecol. 19, 363–374. doi: 10.1111/j.1365-2400.2012.00845.x
Olin M., Kotakorpi M., Nurminen L., Ruuhijärvi J. (2022). The maternal effects on pikeperch (Sander lucioperca) larvae depend on temperature. Ecol. Freshw. Fish 31, 280–290. doi: 10.1111/eff.12629
Parker G. A., Begon M. (1986). Optimal egg size and clutch size: effects of environment and maternal phenotype. Am. Nat. 128, 573–592. doi: 10.1086/284589
Robertson D. R., Collin R. (2015). Inter- and intra-specific variation in egg size among reef fishes across the isthmus of Panama. Front. Ecol. Evol. 2. doi: 10.3389/fevo.2014.00084
Rollinson N., Rowe L. (2016). The positive correlation between maternal size and offspring size: fitting pieces of a life-history puzzle. Biol. Rev. 91, 1134–1148. doi: 10.1111/brv.12214
Salinas S., Munch S. B. (2012). Thermal legacies: transgenerational effects of temperature on growth in a vertebrate. Ecol. Lett. 15, 159–163. doi: 10.1111/j.1461-0248.2011.01721.x
Schneider C. A., Rasband W. S., Eliceiri K. W. (2012). NIH Image to ImageJ: 25 years of image analysis. Nat. Methods 9, 671. doi: 10.1038/nmeth.2089
Seebacher F., White C. R., Franklin C. E. (2015). Physiological plasticity increases resilience of ectothermic animals to climate change. Nat. Climate Change 5, 61–66. doi: 10.1038/nclimate2457
Shelton P. A., Sinclair A. F., Chouinard G. A., Mohn R., Duplisea D. E. (2006). Fishing under low productivity conditions is further delaying recovery of Northwest Atlantic cod (Gadus morhua). Can. J. Fisheries Aquat. Sci. 63, 235–238. doi: 10.1139/f05-253
Sinclair B. J., Marshall K. E., Sewell M. A., Levesque D. L., Willett C. S., Slotsbo S., et al. (2016). Can we predict ectotherm responses to climate change using thermal performance curves and body temperatures? Ecol. Lett. 19, 1372–1385. doi: 10.1111/ele.12686
Sunde J., Larsson P., Forsman A. (2019). Adaptations of early development to local spawning temperature in anadromous populations of pike (Esox lucius). BMC Evolutionary Biol. 19, 148. doi: 10.1186/s12862-019-1475-3
Tamarin-Brodsky T., Hodges K., Hoskins B. J., Shepherd T. G. (2020). Changes in northern hemisphere temperature variability shaped by regional warming patterns. Nat. Geosci. 13, 414–421. doi: 10.1038/s41561-020-0576-3
Team, R.C (2018). A language and environment for statistical computing (Vienna, Austria: R Foundation for Statistical Computing).
Tibblin P., Forsman A., Borger T., Larsson P. (2016). Causes and consequences of repeatability, flexibility and individual fine-tuning of migratory timing in pike. J. Anim. Ecol. 85, 136–145. doi: 10.1111/1365-2656.12439
Tibblin P., Koch-Schmidt P., Larsson P., Stenroth P. (2012). Effects of salinity on growth and mortality of migratory and resident forms of Eurasian perch in the Baltic Sea. Ecol. Freshw. Fish 21, 200–206. doi: 10.1111/j.1600-0633.2011.00537.x
Uusi-Heikkilä S., Lindström K., Parre N., Arlinghaus R., Alós J., Kuparinen A. (2016). Altered trait variability in response to size-selective mortality. Biol. Lett. 12, 20160584. doi: 10.1098/rsbl.2016.0584
van den Berghe E. P., Gross M. R. (1989). Natural selection resulting from female breeding competition in a pacific salmon (coho: Oncorhynchus kisutch). Evolution 43, 125–140. doi: 10.2307/2409169
Via S., Gomulkiewicz R., Dejong G., Scheiner S. M., Schlichting C. D., Vantienderen P. H. (1995). Adaptive phenotypic plasticity - consensus and controversy. Trends Ecol. Evol. 10, 212–217. doi: 10.1016/S0169-5347(00)89061-8
Walters R. j., Hassall M. (2006). The temperature-size rule in ectotherms: may a general explanation exist after all? Am. Nat. 167, 510–523. doi: 10.1086/501029
Keywords: Baltic Sea, climate change, hatching, fish, global warming, reproduction, spawning, thermal tolerance
Citation: Hall M, Nordahl O, Forsman A and Tibblin P (2023) Maternal size in perch (Perca fluviatilis) influences the capacity of offspring to cope with different temperatures. Front. Mar. Sci. 10:1175176. doi: 10.3389/fmars.2023.1175176
Received: 27 February 2023; Accepted: 13 June 2023;
Published: 03 July 2023.
Edited by:
Anke Kremp, Leibniz Institute for Baltic Sea Research (LG), GermanyReviewed by:
Mikko Olin, Natural Resources Institute Finland (Luke), FinlandXian Sun, Sun Yat-sen University, China
Copyright © 2023 Hall, Nordahl, Forsman and Tibblin. This is an open-access article distributed under the terms of the Creative Commons Attribution License (CC BY). The use, distribution or reproduction in other forums is permitted, provided the original author(s) and the copyright owner(s) are credited and that the original publication in this journal is cited, in accordance with accepted academic practice. No use, distribution or reproduction is permitted which does not comply with these terms.
*Correspondence: Petter Tibblin, cGV0dGVyLnRpYmJsaW5AbG51LnNl