- 1Key Laboratory of Mariculture (Ocean University of China), Ministry of Education, Qingdao, China
- 2The Marine Ecological Civilization Comprehensive Experimental Area of Changdao, Yantai, China
Phyllospadix spp. play a crucial role in the supply of ecosystem services in rocky shores, but they have been rapidly reduced because of natural and anthropogenic causes. Since Phyllospadix spp. are attached to rocks in the surf zone with a strong hydrodynamic condition, their transplanted shoots easily disappear due to the high current velocity, resulting in the failure of transplantation. We described a leaf trimming method for transplanting shoots of surfgrass Phyllospadix iwatensis and evaluated the method’s establishment success for potential use in large-scale restoration projects. A 30-day laboratory experiment was conducted to determine the effect of leaf trimming on shoot survival, growth, and physiology. Successful establishment of new patches from shoots transplanted with the leaf trimming method was assessed through a shoot-transplanting field experiment over 15 months. The survival of P. iwatensis shoots was not significantly affected by the leaf trimming proportion of 10%–60%. The leaf elongation rate and total leaf area of the shoots exposed to 10%–50% leaf trimming reached or exceeded those of the control at the end of the laboratory experiment; however, the growth of the shoots under 60% leaf trimming was significantly lower than those under the control (p < 0.05). The soluble sugar and starch contents of the shoots also displayed a similar trend to the growth, with the lowest values attained at 60% of leaf trimming. The survival rate of shoots exposed to 50% leaf trimming treatment was 70% after 15 months following transplantation, which was 1.23 times higher than that of shoots in the control (p < 0.05). The shoot height in the 50% leaf trimming treatment was 1.02 times higher than that of shoots in the control at the end of the field experiment. There were no significant differences in photosynthetic pigment contents of P. iwatensis shoots between the leaf trimming treatment and the control (p > 0.05). Our study suggested that the leaf trimming method is an efficient restoration technique for the transplantation of P. iwatensis shoots, and is potentially useful in facilitating large-scale restoration projects.
1 Introduction
As one of the most important coastal ecosystems, seagrass meadows serve pivotal roles in providing important ecosystem services such as forming habitat for marine species (Duarte and Chiscano, 1999; Lefcheck et al., 2019; Unsworth et al., 2019), improving seawater clarity (Moore, 2004; Potouroglou et al., 2017), carbon sequestration (Fourqurean et al., 2012; Duarte et al., 2013), and pathogenic bacteria removal (Lamb et al., 2017). However, seagrass meadows have been reduced rapidly worldwide by ~30% during the past century due to environmental changes and human intervention (Orth et al., 2006; Waycott et al., 2009; Dunic et al., 2021; Xu et al., 2021). In view of the vital ecological service value of seagrass meadows, including plant transplantation and seed sowing, diverse strategies have been implemented to mitigate these declines (Orth et al., 1999; van Katwijk et al., 2016; Tan et al., 2020; Deng et al., 2022).
Surfgrass (Phyllospadix spp.) includes five seagrass species, all of which are found in temperate coastal areas (McKenzie et al., 2020). Three of five species, P. torreyi, P. scouleri, and P. serrulatus, are usually distributed on the Pacific coast of North America (Phillips, 1979), while P. japonicus and P. iwatensis mainly grow in the coastal areas of Northeast Asia (Park and Lee, 2014; Zheng et al., 2013). The Phyllospadix spp. live and attach to rocks in the surf zone and have distinct morphological adaptations compared with seagrasses that grow in soft substrates. Their rhizomes are cespitose and contain a large number of fibrous roots with dense short root hairs, which significantly increase the area of fixation between the fibrous roots and the rock, allowing them to anchor themselves on the rocks (Cox et al., 1992; Reed et al., 1998).
Phyllospadix spp. have higher leaf productivity compared with other seagrass species. For example, Kim et al. (2018) indicated that the leaf productivity of P. iwatensis was 5–48 times higher than that of Thalassia testudinum and 4.4–8.5 times higher than that of Zostera japonica (Fourqurean et al., 2001; Shafer et al., 2008). The complex physical habitats of Phyllospadix meadow play an important role in the improvement of environment and community structure in the nearshore rocky coastal ecosystem. Shelton (2010) found that once Phyllospadix spp. are removed from tidepools, the temperature of the pools will be 10°C higher than that of the control, leading to alterations in invertebrate community composition and a significant loss in biological abundance, indicating that Phyllospadix spp. play a crucial role in stabilizing and mitigating intertidal habitats. However, surfgrass meadows are sensitive to human disturbance due to their unique ecological characteristics, resulting in serious habitat losses. The area of surfgrass meadows along the eastern coast of Korea has dropped by more than 80% compared with the area in pre-1970s (Park et al., 2018). Phyllospadix spp. were once widespread in Shandong province, China, but most of them have now disappeared (Zheng et al., 2013). The International Union for the Conservation of Nature (IUCN) Red List has classified P. japonicus and P. iwatensis as endangered species and vulnerable species, respectively (Short et al., 2011).
In the last decades, a variety of seagrass restoration techniques have been implemented, including seed planting and shoot transplantation. However, these restoration efforts mostly focused on seagrasses that grow on soft substrates with gentle flows, such as Zostera marina, Enhalus acoroides, and Posidonia oceanica (Zhang et al., 2015; Curiel et al., 2021; Li et al., 2021; Zhang et al., 2022b). Surfgrass communities have typically extreme female-biased sex ratios (Cox et al., 1992; Williams, 1995); thus, it is difficult to restore the degraded Phyllopadix spp. through seed planting. Hence, shoot transplantation is the most important method to mitigate the decline of Phyllopadix meadows. Because surfgrass grows on rock matrix in a high-energy, fast-flowing environment (Park and Lee, 2009), the fixation method of their transplanted plants is crucial for the success of transplantation. Bull et al. (2004) revealed that the survival rate of P. torreyi seedlings placed on nylon ropes was lower than 5% 6 months after transplantation, but the survival rate increased to 47%–71% when the transplanted sprigs were glued to the reef with epoxy. Also, several studies indicated that the survival rate of P. japonicus was as high as 96%–98% by using a wire net or artificial underwater structure to anchor the seedlings to the substrate (Park and Lee, 2014; Park et al., 2018). However, these methods were labor- and material-consuming, which may limit their potential use in large-scale restoration projects.
Hydrodynamic forces caused by currents and waves can lead to strong damage to aquatic plants and even uproot those plants (Bornette and Puijalon, 2011). The physical drag forces that apply to the aquatic plants are proportional to the area of the plants and the flow velocity (Carmen et al., 2016). Therefore, plants with smaller leaf areas and more linear leaves would be subject to less drag forces and thus have a lower risk of being removed under conditions of high flow velocity. Bull et al. (2004) evaluated the effect of leaf trimming on the dislodgement of transplanted P. japonicus sprigs and indicated that trimming the leaves of the sprigs to 20 cm in length before attachment could reduce the drag force and thus increase the fixation of the transplants. This research revealed that trimming leaves to reduce drag was a necessary condition for effective attachment of surfgrass transplants. However, the leaves of the sprigs in the research were only trimmed to 20 cm in length; an optimum leaf trimming method is still unclear.
P. iwatensis is the dominant species of surfgrass in temperate zones and mainly distributed in the littoral zone of Northeast Asia (Short et al., 2007; McKenzie et al., 2020). In the present study, we describe a leaf trimming method of transplanting P. iwatensis shoots for potential use in large-scale restoration projects. Our aims are to (i) determine the optimum proportion of leaf trimming through a cultivation experiment over a 30-day period focusing on shoot survival, growth, and physiology, and (ii) assess the effectiveness of this method to increase the successful establishment of new patches by the transplants through a shoot-transplanting field experiment over 15 months.
2 Materials and methods
2.1 Experimental site
The study was conducted in Mashanli Bay (37°19.3′ N, 122°35.3′ E) on the eastern coast of the Shandong Peninsula, China. The habitat in this area is complex and diverse, including seagrass beds, rock reefs, bare sands, and artificial concrete reefs. The area of seagrass beds is 58.26 hm2, and P. iwatensis is estimated to occupy approximately 93.5% of the area (Deng et al., 2022). This study was conducted in bare areas adjacent to patches of P. iwatensis on rocky substrates.
2.2 Plant collection
Forty 5 cm × 5 cm patches of plants with attached roots and rhizomes were gently collected by hand with a collecting density of one patch per approximately 50 m2 from a 50 × 50 m donor bed of P. iwatensis in Mashanli coast using SCUBA in July 2019. The total area of these patches was 0.1 m2, which was only 0.004% of the area of the donor bed, and thus the collection of the patches did not have a detrimental effect on donor populations. During plant collection, the water temperature of the seagrass bed was 25 ± 0.8°C and the salinity was 31 ± 1.0 PSU. The patches were immediately placed in boxes and transported to the aquaculture farm within 2 h. Patches were held in the farm for 5 days for shoot acclimatization in a 12 m × 1.5 m × 1.2 m (length × width × height) concrete tank with flowing seawater at a temperature of 25 ± 1.0°C, under natural light conditions (13,655 ± 3 lux), and at a salinity of 31 ± 1 PSU until prepared for the experiment. The P. iwatensis shoots for the field experiment were collected in June 2020 with the same site and method described above.
2.3 Effect of leaf trimming on shoot survival, growth, and physiology
To establish whether leaf trimming affected the survival, growth, and physiology of P. iwatensis shoots, a 30-day cultivation experiment was conducted under controlled mesocosm conditions. Seven different proportions of leaf trimming (0% [reference], 10%, 20%, 30%, 40%, 50%, and 60% of the longest leaf with a corresponding average leaf length of 51.8 cm, 46.6 cm, 41.4 cm, 36.3 cm, 31.1 cm, 25.9 cm, and 20.7 cm, respectively) were set in this experiment. A total of 35 patches were randomly selected from those that had been acclimated to mesocosm conditions over 5 days, and then made into grass plugs of 5 cm × 5 cm in the rhizome coverage area with 9–15 shoots each. The shoot height of each shoot was measured and the average value was 60.6 cm. These plugs were randomly divided into seven groups of five plugs each. Five replicates were prepared for each treatment. All shoots were marked for leaf growth estimations using the leaf punching method (Short and Duarte, 2001). The leaves of the shoots in each treatment were trimmed using scissors with the designed proportions. A plug was fixed on a 15 cm × 10 cm concrete block by passing the aboveground tissues through a polyethylene net with a hole in the center and then tying the net on the block with nylon ropes at both ends of the block (Figure 1). The number of shoots in each plug was counted and the block was marked with digits using red paint.
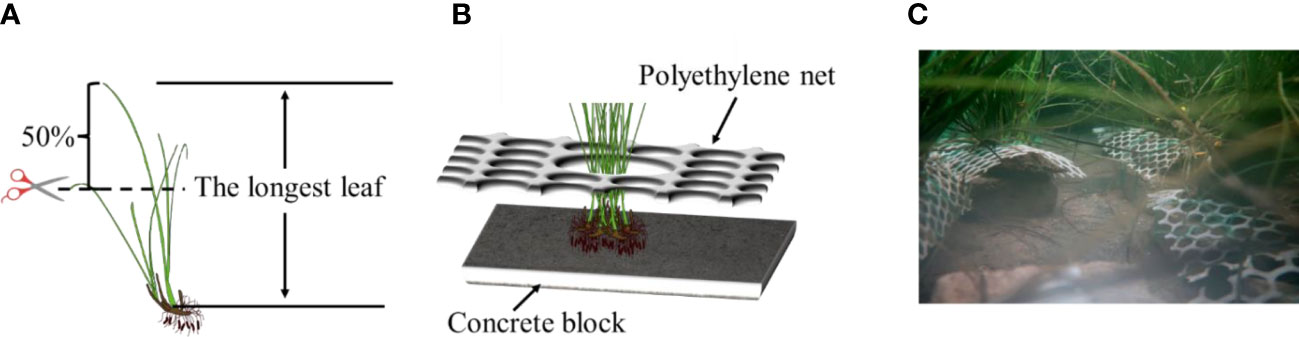
Figure 1 The process of leaf trimming. (A) Leaf trimming; (B) fixation of grass plug; (C) cultivation of grass plugs.
The experiment was conducted in a 12 m × 1.5 m × 1.2 m (length × width × height) concrete tank with a circulating-water system, a transport tank with a 400-W aquarium pump (with a flow capacity of 2,000 L h−1) for recycling water, and a programmed temperature controller for the holding of the water temperature. The position of the plugs in the tank was changed randomly every day to reduce the error in light intensity. During the course of the experiment, the water depth, salinity, and temperature of the water in the tank were maintained at 1.0 m, 31 ± 1 PSU, and 25 ± 1°C, respectively. Salinity and temperature were checked daily using a portable refractometer (Atago) and a mercury thermometer. The water in the tank was renewed daily at a rate of 20%.
At the end of the experiment, the number of shoots in each plug was counted, and the survival rate of P. iwatensis shoots in each treatment was calculated as the number of shoots that were still alive at the end of the experiment over the number of shoots transplanted at the beginning of the experiment. Four shoots were randomly collected from each plug for morphological and growth analysis. Newly formed leaf segments (those without the mark) were separated from the shoots and washed with tap water. The morphometrics of new leaf segments were analyzed by measuring leaf length (cm) and leaf width (cm). The total leaf area (TLA, cm2 shoot−1) and leaf elongation rate (LER, cm shoot−1 day−1) were also calculated to estimate the shoot dynamic properties according to Zhang et al. (2022a). Six shoots were randomly collected from each treatment to analyze the pigment contents of the leaves. Three shoots were randomly collected from each treatment to analyze the non-structural carbohydrate contents (soluble sugar and starch) of the leaves. The remainder were used for analysis of proline content of the leaves. The analysis of the physiological parameters was referenced to the methods as described by Xiao and Wang (2005) and Zhang et al. (2022a).
2.4 Assessment of the successful establishment of new patches
Based on the results of the laboratory experiment, two leaf trimming treatments (0% of the longest leaf with an average leaf length of 47.8 cm, control group; 50% of the longest leaf with an average leaf length of 28.1 cm, leaf trimming group) were set in the field experiment. Twelve replicates were prepared for each leaf trimming treatment.
For the field transplantation, 12 transplant reefs with 96 kg in average weight each were designed and made using concrete and rebar into 60 cm × 40 cm × 20 cm (length × width × height) frames. Then, the reef was made into a groove structure (40 cm × 30 cm × 8 cm in length × width × height) and attached with four steel rings for transportation, lifting, and deployment (Figure 2A). These reefs were kept submerged in seawater for 30 days until the shoots were planted.
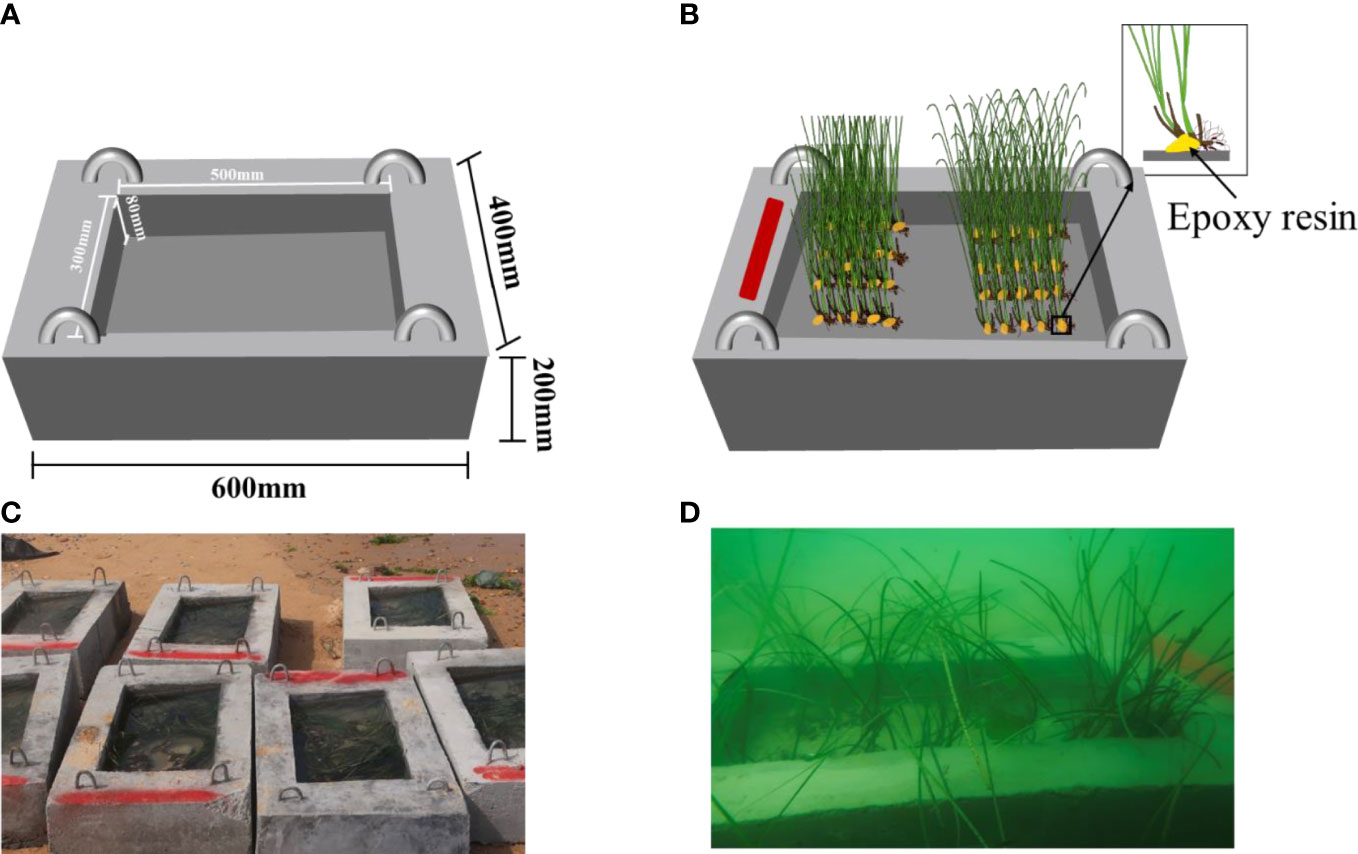
Figure 2 Artificial transplant reefs used in the field experiment. (A) Structure of transplant reef; (B) transplanting unit; (C) image of transplant reefs; (D) underwater image of transplant reef.
A total of 500 P. iwatensis shoots were collected in June 2020. Twenty shoots were randomly collected from these shoots and were analyzed for morphological characteristics and pigment contents of the leaves. Then, the remanent shoots, with an average shoot height of 56.2 cm, were randomly divided into 24 groups of 20 shoots each. Twelve groups were randomly collected and the leaves of the shoots in each group were trimmed with a proportion of 50% of the longest leaf using scissors.
To avoid the effect of the deployment location of reefs on the growth of transplants, the shoots of the two treatments in each replicate were transplanted in the same reef. The area of the groove structure of each transplant reef was equally divided into two parts, and one of the two parts was randomly selected and used for the leaf trimming group, the other for the reference group. The side of the reef transplanted with the leaf trimming group was marked with red paint to distinguish it from the reference group (Figure 2B). The rhizomes of the shoots were attached using epoxy resin on the surface of the groove structure of the reef. The end of the rhizome was exposed at least 1 cm outside the epoxy resin (Figure 2B). Then, the reefs were kept submerged in seawater for 2 h to allow the epoxy resin to solidify and harden. Subsequently, these reefs were transported and deployed into the experimental site in Mashanli Bay in June 2020 (Figures 2C, D). These reefs were arranged into a 2 × 6 grid by placing 12 reefs one by one within a 2 m × 4 m flat, unvegetated sediment transect with a water depth of 2.5 m on neaps. A permanent buoy was fixed to the seabed near the transect using a steel stake as a marker.
On months 1, 2, 3, and 15 after planting, the number of shoots in each reef was counted using SCUBA, and the percentage of shoots that survived in each leaf trimming treatment was calculated. On each visit, three reefs were randomly selected from the deployed reefs, and the shoots were carefully separated from the reef using a small shovel. These shoots in each treatment were rinsed thoroughly in seawater to remove the sediments and other debris. The samples were then placed in sealed polythene bags, marked using a marking pen, transported to the laboratory, and stored at 4°C before being sorted for analysis. In the laboratory, shoots in the reef were counted to correct the potential error in the survival estimate obtained by SCUBA observation. The samples were sorted, gently cleaned with gauze to remove epiphytes, and washed with tap water. Morphometrics were analyzed by measuring shoot height, leaf width, and leaf length (cm). The TLA was determined as the sum of all the leaf areas of each shoot, which was calculated by multiplying the length and width of each leaf. Subsequently, four shoots were randomly collected from each treatment to analyze the pigment contents of the leaves using the method described above.
2.5 Statistical analysis
All data and errors in tables and figures were reported as means ± SD. Data were tested with one-way analysis of variance (ANOVA) to analyze the effect of different leaf trimming ratios on the growth and physiology of the shoots. All data were tested for normality and homogeneity of variance to meet the assumptions of parametric statistical analysis. Duncan’s multiple range test was performed to identify the significance of differences among treatments when the ANOVAs were significant at α = 0.05. Independent sample t-test was used to analyze the differences in survival and growth of shoots between the two treatments in the field experiment. Statistical analyses of all data were performed using the SPSS Windows Program (Release 25.0, SPSS Inc.).
3 Results
3.1 Effect of leaf trimming on shoot survival, growth, and physiology
3.1.1 Survival and growth
All plants under different treatments survived at the end of the experiment. The results of the one-way ANOVA showed that leaf trimming had a significant influence on the LER and TLA of P. iwatensis shoots (p < 0.05; Figure 3). The LER and TLA of P. iwatensis shoots showed a similar variation trend, and the values of plants under 10%–30% leaf trimming treatments were 1.12–1.14 times and 1.17–1.21 times significantly higher than those of plants under the control, respectively. There were no significant differences in these values between the 40%–50% leaf trimming treatments and the control (p > 0.05). However, the LER and TLA of the plants at the 60% leaf trimming treatment were 53.7% and 48.7% significantly lower than those of the plants at the control, respectively.
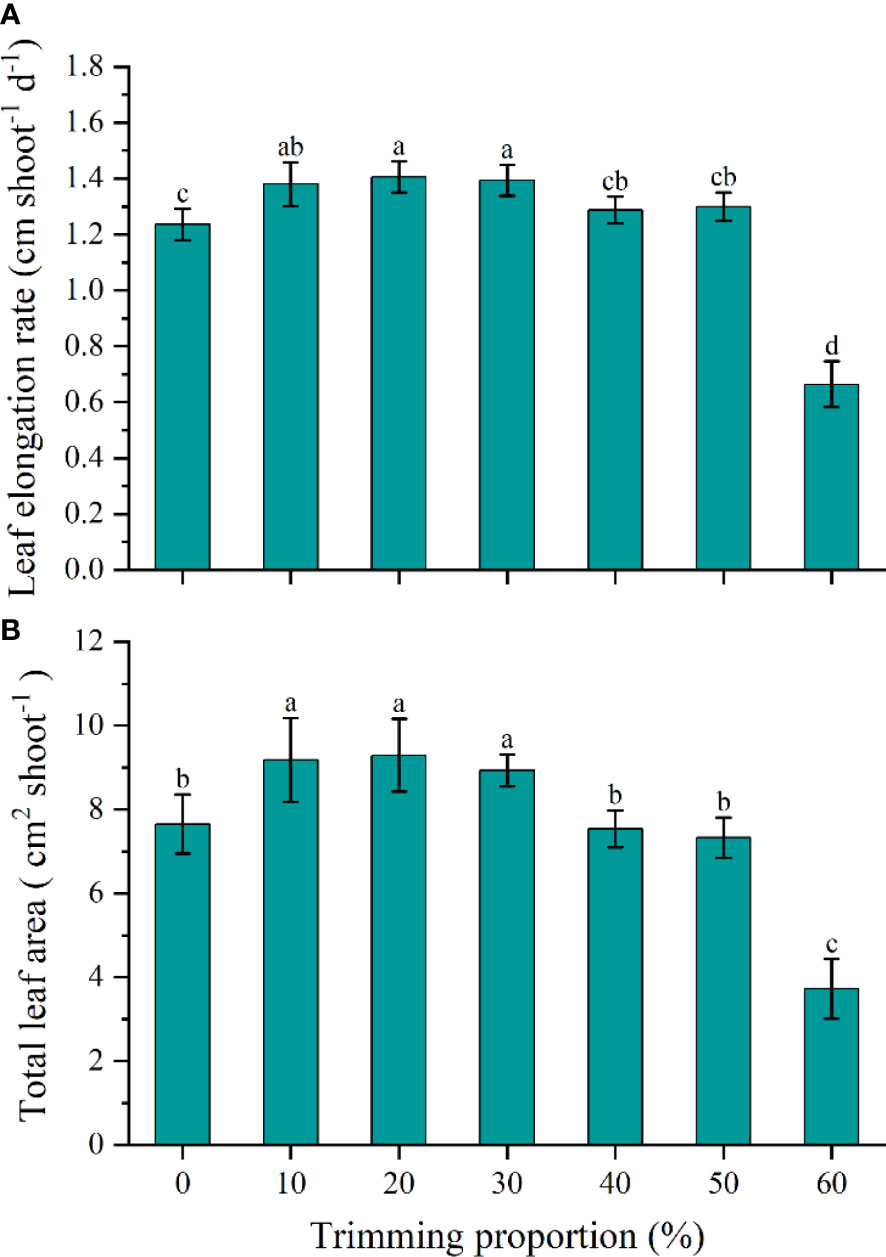
Figure 3 The change in leaf elongation rate (A) and total leaf area (B) of P. iwatensis shoots exposed to different leaf trimming proportions under controlled mesocosm conditions. Data are expressed as means ± SD. Different letters above error bars indicate significant differences among different leaf trimming treatments (p < 0.05).
3.1.2 Physiological parameters
The results of the one-way ANOVA indicated that there were no significant differences in the pigment and proline contents of the P. iwatensis shoots among the different leaf trimming treatments (p > 0.05; Table 1).
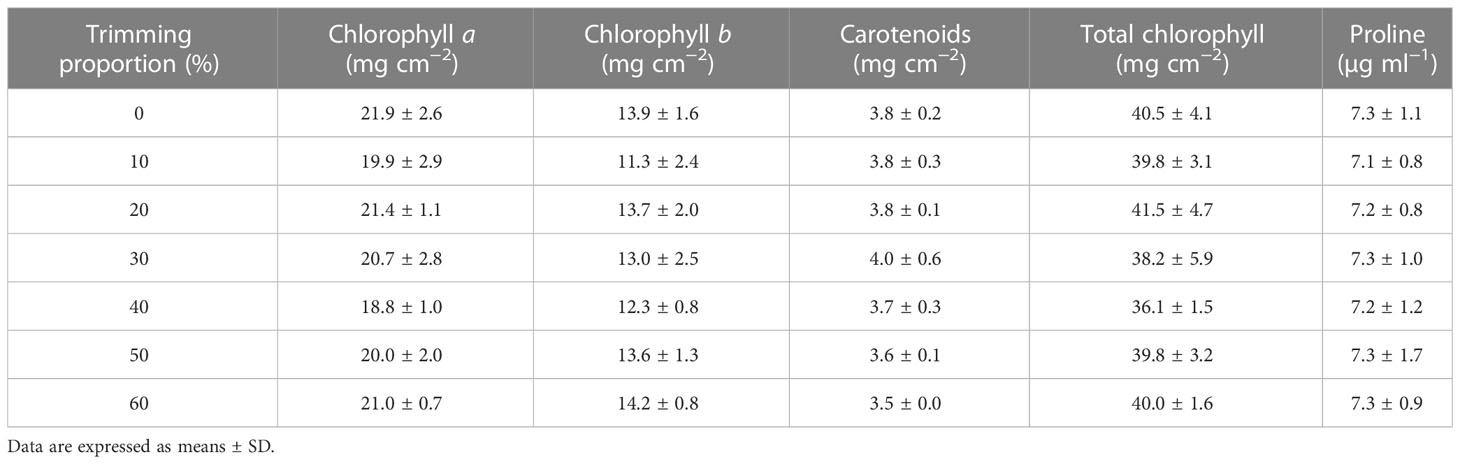
Table 1 The change in photosynthetic pigment and proline contents of Phyllospadix iwatensis shoots exposed to different leaf trimming proportions under controlled mesocosm conditions.
The non-structural carbohydrates of the plants, including soluble sugar and starch, displayed a similar trend to the change in plant growth. One-way ANOVA indicated that leaf trimming had a significant effect on the non-structural carbohydrates of P. iwatensis shoots (p < 0.05; Figure 4). There were no significant differences in these values between the 10%–50% leaf trimming treatments and the control (p > 0.05). However, the soluble sugar and starch contents of the plants at the 60% leaf trimming treatment were 89.0% and 68.2% significantly lower than those of the plants at the control, respectively.
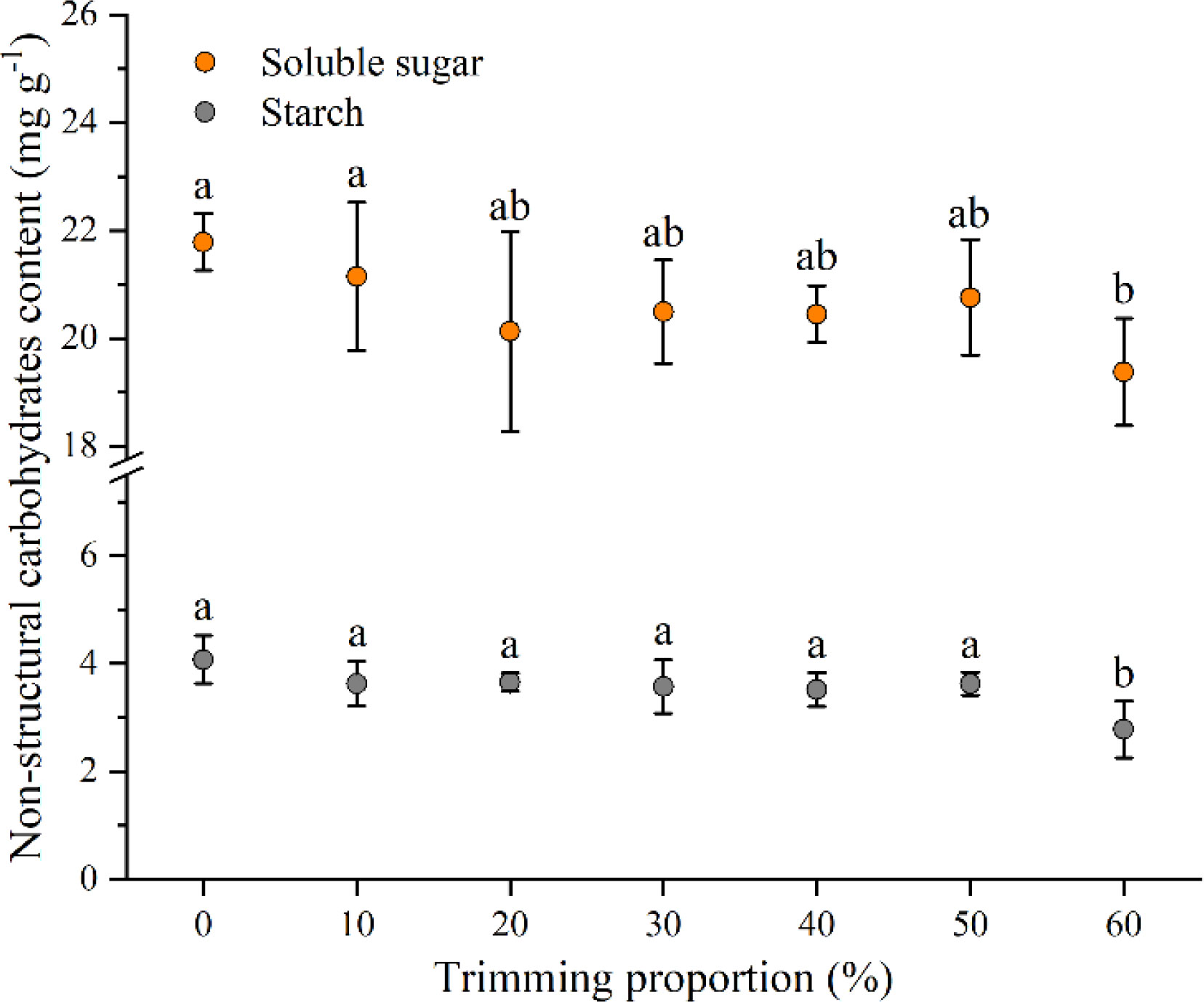
Figure 4 The effect of different leaf trimming proportions on the soluble sugar and starch contents of P. iwatensis shoots. Data are expressed as means ± SD. Different letters above the error bars in the same physiological parameter indicate significant differences among different leaf trimming treatments (p < 0.05).
3.2 Effect of leaf trimming on shoot survival, growth, and physiology
3.2.1 Survival
The survival of P. iwatensis shoots displayed a decrease during the experimental period under the two treatments (Figure 5). The result of independent sample t-tests indicated that the leaf trimming had a significant effect on the survival of P. iwatensis shoots after 2 months following planting (p < 0.05). The survival rate of P. iwatensis in the leaf trimming treatment was 70.0% 15 months following planting, which was 1.5 times significantly higher than the survival rate of plants in the non-trimming treatment.
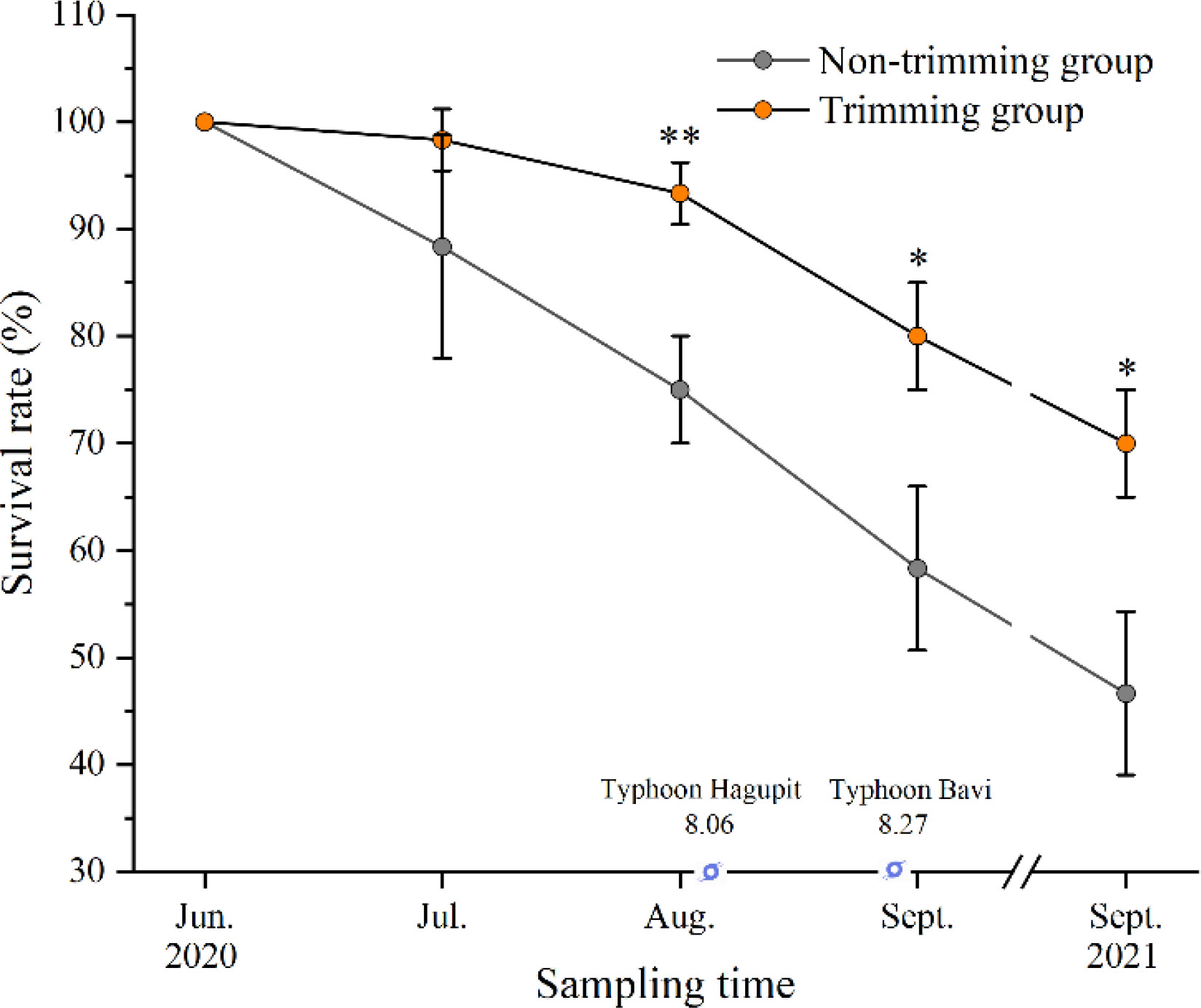
Figure 5 The change in survival rate of P. iwatensis shoots under the leaf trimming treatment and non-trimming treatment at Mashanli Bay. Data are expressed as means ± SD. Asterisk (*) indicates significant difference in survival rate between leaf trimming and non-trimming treatments on the same sampling month (p < 0.05), ** indicates very significant difference (p < 0.01).
3.2.2 Morphology and growth
The transplants displayed a fine growth (Figure 6). The shoot height of P. iwatensis in both leaf trimming and non-trimming treatments showed a similar variation trend (Figure 7A). The shoot height of P. iwatensis shoots in the leaf trimming treatment had grown to 57.2 cm after 1 month following planting, which had no significant difference with that of the plants in the non-trimming treatment (p > 0.05). In addition, there was also no significant difference in shoot height between the two treatments in subsequent sampling months, with the average value of 37.8–59.4 cm in the non-trimming treatment and 39.2–57.2 cm in the leaf trimming treatment.
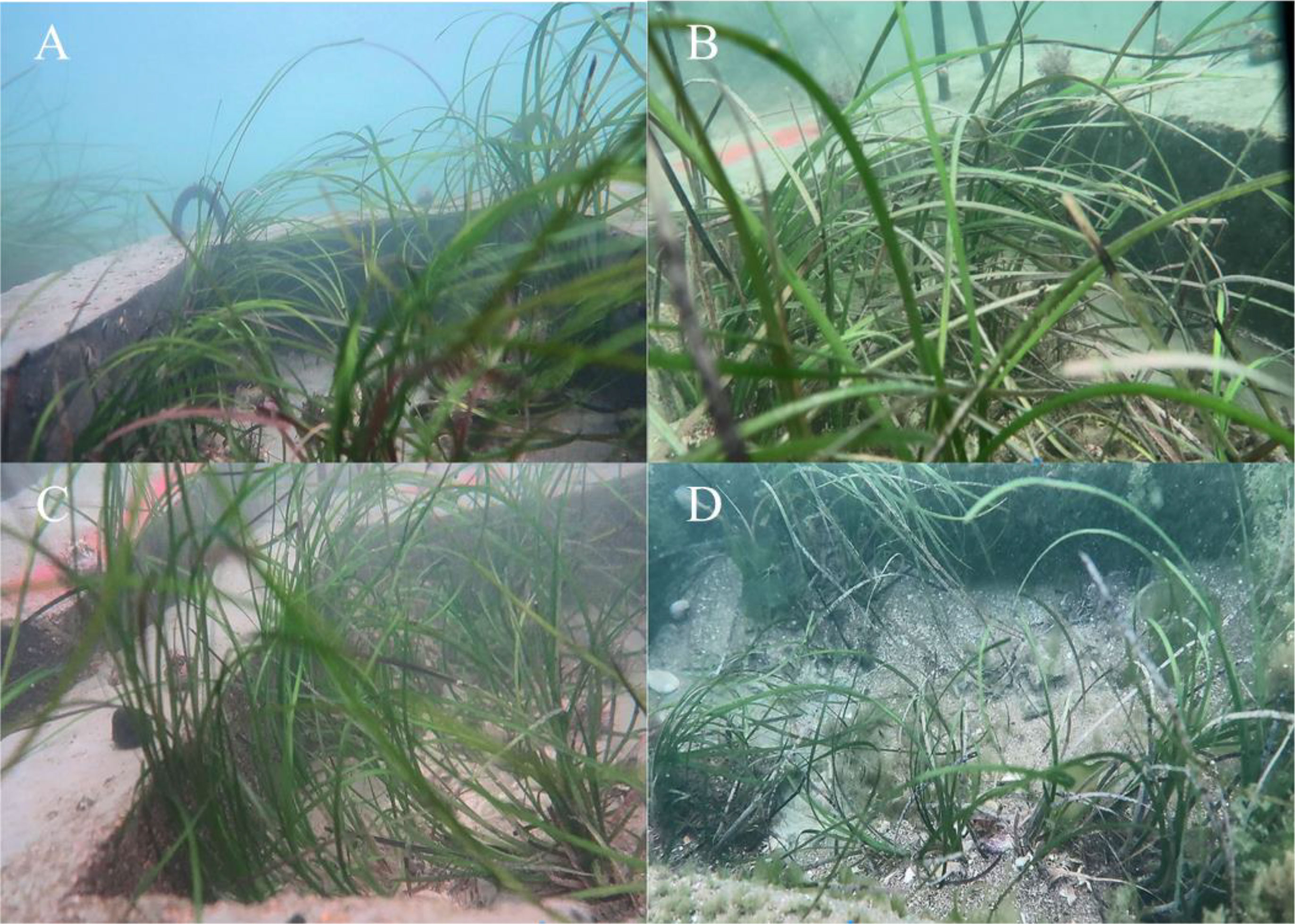
Figure 6 Shoots growth of P. iwatensis transplants in the field experiment in July 2020 (A), August 2020 (B), September 2020 (C), and September 2021 (D).
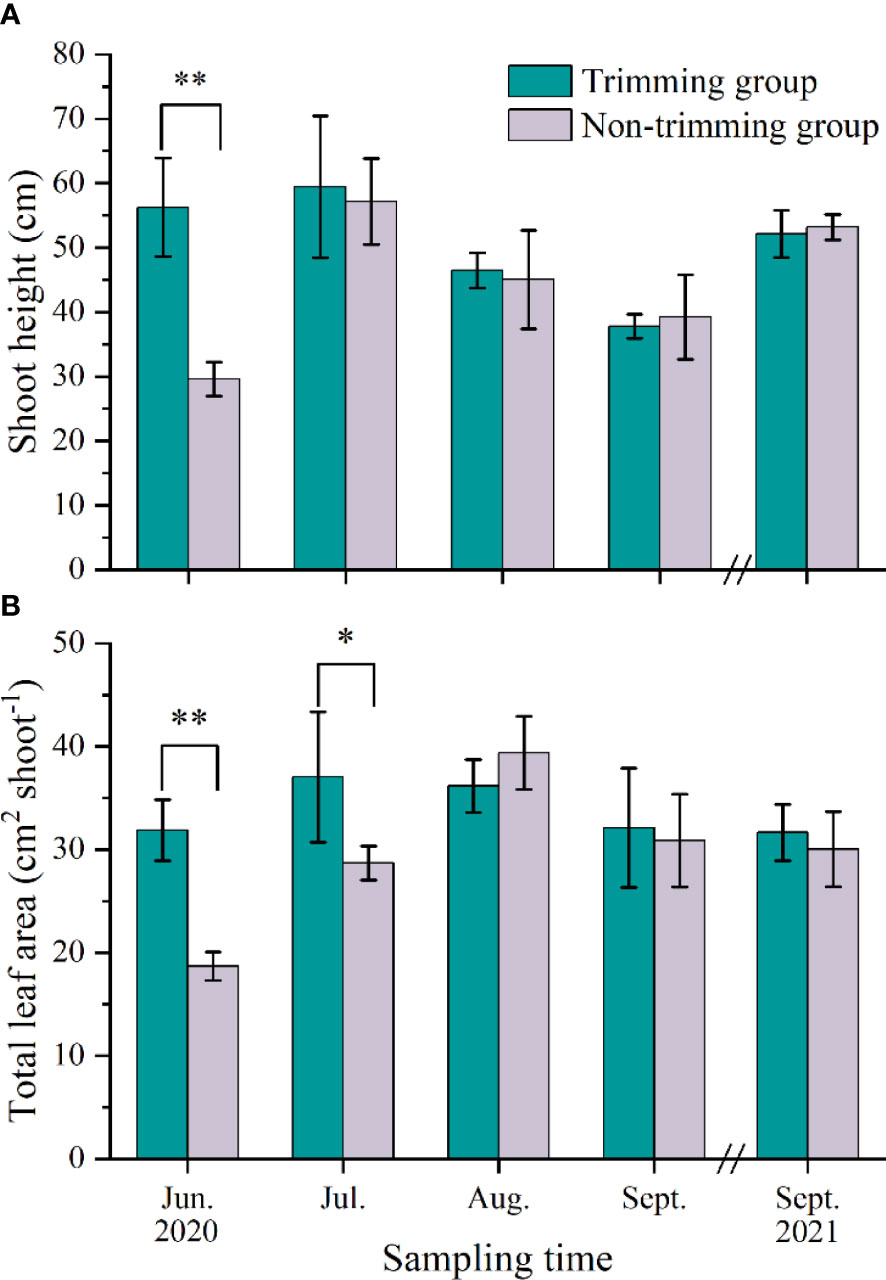
Figure 7 The change in shoot height (A) and total leaf area (B) of P. iwatensis shoots exposed to different leaf trimming treatments under the field experiment. Data are expressed as means ± SD. Asterisk (*) indicates significant differences in shoot height and total leaf area between leaf trimming and non-trimming treatments on the same sampling month (p < 0.05), ** indicates very significant difference (p < 0.01).
The TLA of P. iwatensis shoots displayed a similar trend to the change in shoot height (Figure 7B). The TLA of P. iwatensis shoots in the leaf trimming treatment was 28.7 cm2 shoot−1 after 1 month following planting, which was significantly higher than that of the plants in the non-trimming treatment (p < 0.05). There was no significant difference in TLA between the two treatments in each subsequent sampling month, with the average value of 31.7–37.0 cm2 shoot−1 in non-trimming treatment and 28.7–39.4 cm2 shoot−1 in leaf trimming treatment.
3.2.3 Pigment
The results of the independent sample t-tests indicated that there were no significant differences in pigment contents of P. iwatensis shoots between the two leaf trimming treatments during the field transplantation experiment (p > 0.05; Table 2).
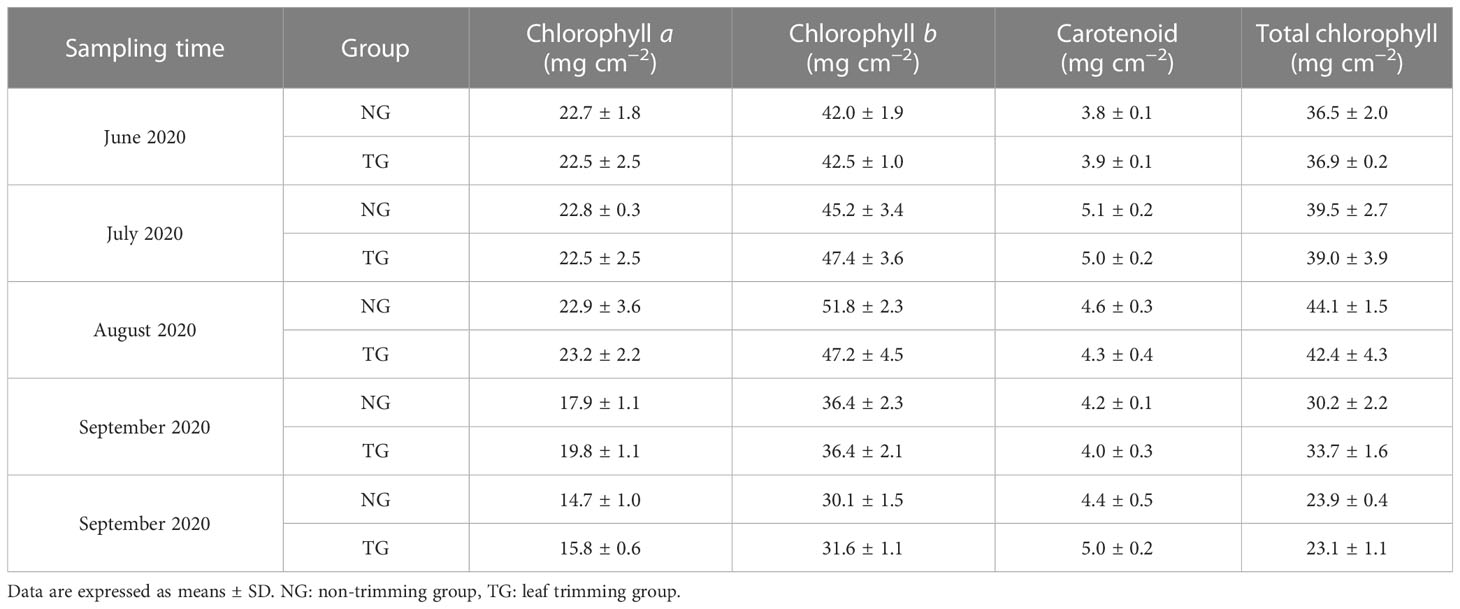
Table 2 The change in photosynthetic pigment contents of Phyllospadix iwatensis shoots exposed to different leaf trimming treatments under the field experiment.
4 Discussion
4.1 The effect of leaf trimming on the survival and growth of seagrasses
Our results showed that leaf trimming had a significant effect on the growth of P. iwatensis shoots under controlled mesocosm conditions. Growth analysis combined with physiology assessment indicated that the optimal ratio of leaf trimming for the transplantation of P. iwatensis shoots is 50%. The survival rate of P. iwatensis shoots exposed to the optimal ratio of leaf trimming was 1.5 times higher than that of shoots under the control in the field experiment. Results in this study support the hypothesis that appropriate leaf trimming may promote the survival and growth of transplanted P. iwatensis shoots. It was worth noting that typhoons “Hagupit” and “Bavi” impacted our transplantation area in August 2020 (2 months after transplantation). This implies that the combination of leaf trimming and adhesive material (epoxy resin) is an effective transplantation method for surfgrass plants in high-energy conditions.
The intense drag caused by waves and currents is one of the obstacles that seagrasses must overcome in order to adapt to life beneath (Paul and Santos, 2019). Some similar studies have demonstrated that reducing the contact area between seagrass leaves and water has a positive impact on the growth and survival of shoots. For example, the plant Zostera noltii exhibited phenotypic plasticity (i.e., a short leaf strategy) to ensure the survival and growth of the plant in highly hydrodynamic water environments (Peralta et al., 2006; Paul and Santos, 2019). Removing the uppermost portion of the leaves could lessen the resistance caused by the leaves and hence decrease the possibility of their uprooting, resulting in a higher survival of the transplantation (de los Santos et al., 2016). On the other hand, many studies have indicated that shade stress could reduce the growth, cause the loss of leaves, decrease the shoot density, and even result in the death of many seagrasses (Yaakub et al., 2014; Benham et al., 2016). For example, Collier et al. (2012) found that the length of Halodule uninervis, Thalassia hemprichii, and Zostera muelleri leaves was reduced by 48%–77% and the density of shoots was reduced by 62%–100% under shade stress. Leaf trimming could reduce self-shading and increase the photosynthesis rate, which could enhance the survival of transplanted seagrass (Tang et al., 2019).
In addition, we also found that the growth of P. iwatensis plants exposed to 10%–30% leaf trimming was significantly higher than that of plants at the control. Some studies have also indicated that plants treated with adequate leaf trimming exhibit a compensatory growth to maintain or improve their growth (Meyer, 1998; Zhao et al., 2008), particularly the increase in leaf area in response to leaf loss (Gao et al., 2008). For example, Prado et al. (2011) found that P. oceanica could resist injury from herbivores and continued to grow even after losing 57% of leaf tissue. Dos Santos et al. (2013) indicated that low-intensity leaf trimming (~40% biomass removed) did not negatively affect seagrass growth. This may be because the treatment of leaf trimming can modify the resource allocation of plants (Aragones et al., 2006). However, the similar studies on Zostera capricorni and Cymodocea serrulate exhibited that leaf trimming adversely affected leaf growth (Kuiper-Linley et al., 2007), indicating that the response of seagrasses to leaf trimming depended on the species and the proportion of the leaf trimming.
However, over-trimming has a negative effect on seagrass. For instance, studies have shown that overgrazing on Z. muelleri damaged the above-ground structure of the plants and depleted nutrients, which limited the acquisition of resources for seagrasses and ultimately impacted their growth and reproduction (Prado et al., 2011; Dos Santos et al., 2013). The results in the present study also showed that the LER and TLA of plants exposed to 60% leaf trimming were significantly 53.7% and 48.7% lower than those of plants in the control, respectively. Dos Santos et al. (2013) conducted a simulative grazing experiment and demonstrated that the high grazing intensity (~100% biomass removed) of Z. muelleri resulted in a failing restoration (only 25%–42% of control in biomass) after 1 year. These studies have proved that only appropriate leaf trimming ratios can promote the survival and growth of seagrass plant.
4.2 The effect of leaf trimming on the physiological response of seagrasses
Our study showed that leaf trimming had a significant effect on the non-structural carbohydrate (including the soluble sugar and starch) contents of the aboveground tissues of the surfgrass plants. Both soluble sugars and starch are non-structural carbohydrates and principal metabolites, and they serve as the plant’s primary source of energy throughout growth and metabolism (Schönbeck et al., 2018). Tang et al. (2019) conducted a long-term trimming experiment and demonstrated that the increase in soluble sugar content may help plants increase their tolerance to stress. In addition, leaf-trimming plants often accumulate fewer reactive oxygen species (ROS), leaf senescence is delayed, and more carbohydrates are accumulated in plants; as a result, compensatory growth occurs in trimming plants (You and Chan, 2015; Tang et al., 2019). Pigments are responsible for absorbing, transmitting, and converting light energy. However, there was no significant difference in the content of photosynthetic pigments between the trimming and non-trimming treatments. This may be because P. iwatensis is able to compensate for the loss of photosynthetic area caused by trimming by raising the photosynthetic rate of the remaining tissues (Detling et al., 1979; Cebrián et al., 1998; Zhao et al., 2008).
Although plants could compensate for tissue removal through some morphological and physiological responses, intense trimming would result in a significant decrease in biomass and growth rate (Zhao et al., 2008; Van Staalduinen et al., 2010). This is due to the fact that the plant exposed to over-trimming requires large amounts of energy investment to recover, and the limited non-structural carbohydrates, starches, etc. stored in the residual tissues are not enough to support this consumption (Reichman and Smith, 1991). Similarly, Kuiper-Linley et al. (2007) found that there was a significant decrease in carbohydrate content in rhizomes of Cymodocea serrulata with increasing intensity of grazing. Therefore, under-compensatory growth is frequently found when regrowth tissues are less than the proportion of biomass lost due to excessive leaf trimming.
5 Conclusion
Transplantation methods have the potential to facilitate large-scale surfgrass restoration projects, but because they grow in the rocky intertidal zone, dragging forces of flow result in a low survival rate that remains a bottleneck. In the present study, we demonstrated that 50% leaf trimming could develop a positive effect on the survival of transplanted P. iwatensis plants. It could be used as the maximum trimming ratio for transplanting to minimize the damage caused by the dragging force due to currents and waves. Compared with other methods of surfgrass restoration proposed by other researchers (Park and Lee, 2014; Park et al., 2018), the leaf trimming method not only has a higher survival rate and fine growth of transplants but also has a simpler operation to lower the transplantation cost, facilitating large-scale surfgrass restoration projects. However, this study only conducted trimming in different proportions for P. iwatensis shoots, and further studies are needed to determine the appropriate trimming proportions for other seagrasses.
Data availability statement
The raw data supporting the conclusions of this article will be made available by the authors, without undue reservation.
Author contributions
JS analyzed the data and wrote the manuscript. FS-L, G-XY and H-YZ designed the study, performed the experiment, and helped perform the experiment and contributed substantially to revisions. P-DZ and W-TL designed the study, performed the experiment, and contributed substantially to revisions. All authors contributed to the article and approved the submitted version.
Funding
This study was supported by funds from the National Natural Science Foundation of China (42076100) and Joint Funds of the National Natural Science Foundation of China (U2006214).
Acknowledgments
We thank Shi-Qi Li and Chao Li for their assistance during experimental apparatus construction, and we thank Yan-Hao Zhang and Zheng Li for their assistance during sample collection and calculations.
Conflict of interest
The authors declare that the research was conducted in the absence of any commercial or financial relationships that could be construed as a potential conflict of interest.
Publisher’s note
All claims expressed in this article are solely those of the authors and do not necessarily represent those of their affiliated organizations, or those of the publisher, the editors and the reviewers. Any product that may be evaluated in this article, or claim that may be made by its manufacturer, is not guaranteed or endorsed by the publisher.
References
Aragones L. V., Lawler I. R., Foley W. J., Marsh H. (2006). Dugong grazing and turtle cropping: grazing optimization in tropical seagrass systems? Oecologia. 149, 635–647. doi: 10.1007/s00442-006-0477-1
Benham C. F., Beavis S. G., Hendry R. A., Jackson E. L. (2016). Growth effects of shading and sedimentation in two tropical seagrass species: implications for port management and impact assessment. Mar. pollut. Bull. 109, 461–470. doi: 10.1016/j.marpolbul.2016.05.027
Bornette G., Puijalon S. (2011). Response of aquatic plants to abiotic factors: a review. Aquat. Sci. 73, 1–14. doi: 10.1007/s00027-010-0162-7
Bull J. S., Reed D. C., Holbrook S. J. (2004). An experimental evaluation of different methods of restoring Phyllospadix torreyi (surfgrass). Restor. Ecol. 12, 70–79. doi: 10.1111/j.1061-2971.2004.00258.x
Carmen B., Onoda Y., Vergara J. J., Pérez-Lloréns J. L., Bouma T. J., La Nafie Y. A., et al. (2016). A comprehensive analysis of mechanical and morphological traits in temperate and tropical seagrass species. Mar. Ecol. Prog. Ser. 551, 81–94. doi: 10.3354/meps11717
Cebrián J., Duarte C. M., Agawin N. S. R., Merino M.N. (1998). Leaf growth response to simulated herbivory: a comparison among seagrass species. J. Exp. Mar. Biol. Ecol. 220, 67–81. doi: 10.1016/s0022-0981(97)00084-1
Collier C. J., Waycott M., Ospina A. G. (2012). Responses of four indo-West pacific seagrass species to shading. Mar. pollut. Bull. 65, 342–354. doi: 10.1016/j.marpolbul.2011.06.017
Cox P., Tomlinson P., Nieznanski K. (1992). Hydrophilous pollination and reproductive morphology in the seagrass Phyllospadix scouleri (Zosteraceae). Plant Syst. Evol. 180, 65–75. doi: 10.1007/bf00940398
Curiel D., Pavelić S. K., Kovacev A., Miotti C., Rismondo A. (2021). Marine seagrasses transplantation in confined and coastal adriatic environments: methods and results. Water. 13, 2289. doi: 10.3390/w13162289
de los Santos C. B., Vicencio R. B., Lepoint G., Remy F., Bouma T. J., Gobert S. (2016). Ontogenic variation and effect of collection procedure on leaf biomechanical properties of Mediterranean seagrass Posidonia oceanica (L.). Delile. Mar. Ecol. 37, 750–759. doi: 10.1111/maec.12340
Deng X., Zhang H., Wu Z., Li W., Zhang P. (2022). Distribution and ecological characteristics of seagrass bed of mashanli sea area in rongcheng. Acta Oceanol. Sin. 44, 97–109. doi: 10.12284/hyxb2022160
Detling J., Dyer M., Winn D. (1979). Net photosynthesis, root respiration, and regrowth of Bouteloua gracilis following simulated grazing. Oecologia 41, 127–134. doi: 10.1007/bf00344997
Dos Santos V. M., Matheson F. E., Pilditch C. A., Elger A. (2013). Seagrass resilience to waterfowl grazing in a temperate estuary: a multi-site experimental study. J. Exp. Mar. Biol. Ecol. 446, 194–201. doi: 10.1016/j.jembe.2013.05.030
Duarte C. M., Chiscano C. L. (1999). Seagrass biomass and production: a reassessment. Aquat. Bot. 65, 159–174. doi: 10.1016/S0304-3770(99)00038-8
Duarte C. M., Kennedy H., Marbà N.R., Hendriks I. (2013). Assessing the capacity of seagrass meadows for carbon burial: current limitations and future strategies. Ocean Coast. Manage. 83, 32–38. doi: 10.1016/j.ocecoaman.2011.09.001
Dunic J. C., Brown C. J., Connolly R. M., Turschwell M. P., Côté I. M. (2021). Long term declines and recovery of meadow area across the worlds seagrass bioregions. Global Change Biol. 27, 4096–4109. doi: 10.1038/s41559-021-01433-z
Fourqurean J. W., Duarte C. M., Kennedy H., Marbà N., Holmer M., Mateo M. A., et al. (2012). Seagrass ecosystems as a globally significant carbon stock. Nat. Geosci. 5, 505–509. doi: 10.1038/ngeo1477
Fourqurean J. W., Willsie A., Rose C. D., Rutten L. M. (2001). Spatial and temporal pattern in seagrass community composition and productivity in south Florida. Mar. Biol. 138, 341–354. doi: 10.1007/s002270000448
Gao Y., Wang D., Ba L., Bai Y., Liu B. (2008). Interactions between herbivory and resource availability on grazing tolerance of Leymus chinensis. Environ. Exp. Bot. 63, 113–122. doi: 10.1016/j.envexpbot.2007.10.030
Kim J. H., Kim J. H., Kim G. Y., Park J. I. (2018). Growth dynamics of the surfgrass, Phyllospadix iwatensis on the eastern coast of Korea. J. Korean Soc Oceanogr. 23, 192–203. doi: 10.7850/jkso.2018.23.4.192
Kuiper-Linley M., Johnson C. R., Lanyon J. M. (2007). Effects of simulated green turtle regrazing on seagrass abundance, growth and nutritional status in moreton bay, south-east Queensland, Australia. Mar. Freshw. Res. 58, 492–503. doi: 10.1071/mf06241
Lamb J. B., Van De Water J. A., Bourne D. G., Altier C., Hein M. Y., Fiorenza E. A., et al. (2017). Seagrass ecosystems reduce exposure to bacterial pathogens of humans, fishes, and invertebrates. Science 355, 731–733. doi: 10.1126/science.aal1956
Lefcheck J. S., Hughes B. B., Johnson A. J., Pfirrmann B. W., Rasher D. B., Smyth A. R., et al. (2019). Are coastal habitats important nurseries? a meta-analysis. Conserv. Lett. 12, e12645. doi: 10.1111/conl.12645
Li H., Liu J. G., Che X. K. (2021). Establishing healthy seedlings of Enhalus acoroides for the tropical seagrass restoration. J. Environ. Manage. 286, 112200. doi: 10.1016/j.jenvman.2021.112200
McKenzie L. J., Nordlund L. M., Jones B.L., Cullen-Unsworth L. C., Roelfsema C., Unsworth P. K. (2020). The global distribution of seagrass meadows. Environ. Res. Lett. 15, 074041. doi: 10.1088/1748-9326/ab7d06
Meyer G. (1998). Mechanisms promoting recovery from defoliation in goldenrod (Solidago altissima). Can. J. Bot. 76, 450–459. doi: 10.1139/b98-004
Moore K. A. (2004). Influence of seagrasses on water quality in shallow regions of the lower Chesapeake bay. J. Coast. Res. 2009, 162–178. doi: 10.2112/si45-162.1
Orth R. J., Carruthers T. J., Dennison W. C., Duarte C. M., Fourqurean J. W., Heck K. L., et al. (2006). A global crisis for seagrass ecosystems. BioSci. 56, 987–996. doi: 10.1641/0006-3568(2006)56[987:agcfse]2.0.co;2
Orth R. J., Harwell M. C., Fishman J. R. (1999). A rapid and simple method for transplanting eelgrass using single, unanchored shoots. Aquat. Bot. 64, 77–85. doi: 10.1016/S0304-3770(99)00007-8
Park J. I., Kim J. H., Kim J. H., Shim J. H. (2018). A new and effective transplanting technique using wire netting for restoration of the surfgrass, Phyllospadix japonicus, in exposed rocky shores. J. Coast. Res. 85, 326–330. doi: 10.2112/SI85-066.1
Park J. I., Lee K. S. (2009). Peculiar growth dynamics of the surfgrass Phyllospadix japonicus on the southeastern coast of Korea. Mar. Biol. 156, 2221–2233. doi: 10.1007/s00227-009-1250-x
Park J. I., Son M. H., Kim J. B., Lee K. S. (2014). An effective seeding method for restoring the surfgrass Phyllospadix japonicus using an artificial reef. Ocean Sci. J. 49, 403–410. doi: 10.1007/s12601-014-0037-2
Paul M., Santos C. B. D. L. (2019). Variation in flexural, morphological, and biochemical leaf properties of eelgrass (Zostera marina) along the European Atlantic climate regions. Mar. Biol. 166, 1–12. doi: 10.1007/s00227-019-3577-2
Peralta G., Brun F. G., Pérez-Lloréns J. L., Bouma T. J. (2006). Direct effects of current velocity on the growth, morphometry and architecture of seagrasses: a case study on Zostera noltii. Mar. Ecol. Prog. Ser. 327, 135–142. doi: 10.3354/meps327135
Phillips R. C. (1979). Ecological notes on Phyllospadix (Potamogetonaceae) in the northeast pacific. Aquat. Bot. 6, 159–170. doi: 10.1016/0304-3770(79)90059-7
Potouroglou M., Bull J. C., Krauss K. W., Kennedy H. A., Fusi M., Daffonchio D., et al. (2017). Measuring the role of seagrasses in regulating sediment surface elevation. Sci. Rep. 7, 1–11. doi: 10.1038/s41598-017-12354-y
Prado P., Collier C. J., Romero J., Alcoverro T. (2011). Distinctive types of leaf tissue damage influence nutrient supply to growing tissues within seagrass shoots. Mar. Biol. 158, 1473–1482. doi: 10.1007/s00227-011-1664-0
Reed D. C., Holbrook S. J., Solomon E., Anghera M. (1998). Studies on germination and root development in the surfgrass Phyllospadix torreyi: implications for habitat restoration. Aquat. Bot. 62, 71–80. doi: 10.1016/S0304-3770(98)00088-6
Reichman O., Smith S. C. (1991). Responses to simulated leaf and root herbivory by a biennial, tragopogon dubius. Ecology 72, 116–124. doi: 10.2307/1938907
Schönbeck L., Gessler A., Hoch G., McDowell N. G., Rigling A., Schaub M., et al. (2018). Homeostatic levels of nonstructural carbohydrates after 13 yr of drought and irrigation in pinus sylvestris. New Phytol. 219, 1314–1324. doi: 10.1111/nph.15224
Shafer D. J., Wyllie-Echeverria S., Sherman T. D. (2008). The potential role of climate in the distribution and zonation of the introduced seagrass Zostera japonica in north America. Aquat. Bot. 89, 297–302. doi: 10.1016/j.aquabot.2008.03.005
Shelton A. O. (2010). Temperature and community consequences of the loss of foundation species: surfgrass (Phyllospadix spp., hooker) in tidepools. J. Exp. Mar. Biol. Ecol. 391, 35–42. doi: 10.1016/j.jembe.2010.06.003
Short F., Carruthers T., Dennison W., Waycott M. (2007). Global seagrass distribution and diversity: a bioregional model. J. Exp. Mar. Biol. Ecol. 350, 3–20. doi: 10.1016/j.jembe.2007.06.012
Short F. T., Duarte C. M. (2001). “Methods for the measurement of seagrass growth and production,” in Global seagrass research methods. Eds. Short F. T., Coles R. G. (Amsterdam: Elsevier), 155–182. doi: 10.1016/S0025-3227(02)00475-9
Short F. T., Polidoro B., Livingstone S. R., Carpenter K. E., Bandeira S., Bujang J. S., et al. (2011). Extinction risk assessment of the world's seagrass species. Biol. Conserv. 144, 1961–1971. doi: 10.1016/j.biocon.2011.04.010
Tan Y. M., Dalby O., Kendrick G. A., Statton J., Sinclair E. A., Fraser M. W., et al. (2020). Seagrass restoration is possible: insights and lessons from Australia and New Zealand. Front. Mar. Sci. 7. doi: 10.3389/fmars.2020.00617
Tang G. L., Guo Z. C., Zhang B., Li X. Y., Zeng F. J. (2019). Long-term clipping causes carbohydrate accumulation and induced transition of Alhagi sparsifolia from herbs to shrubs. Funct. Plant Biol. 46, 967–985. doi: 10.1071/fp18072
Unsworth R. K., Nordlund L. M., Cullen-Unsworth L. C. (2019). Seagrass meadows support global fisheries production. Conserv. Lett. 12, e12566. doi: 10.1111/conl.12566
van Katwijk M. M., Thorhaug A., Marba N., Orth R. J., Duarte C. M., Kendrick G. A., et al. (2016). Global analysis of seagrass restoration: the importance of large-scale planting. J. Appl. Ecol. 53, 567–578. doi: 10.3354/meps11717
Van Staalduinen M. A., Dobarro I., Peco B. (2010). Interactive effects of clipping and nutrient availability on the compensatory growth of a grass species. Plant Ecol. 208, 55–64. doi: 10.1007/s11258-009-9686-0
Waycott M., Duarte C. M., Carruthers T. J., Orth R. J., Dennison W. C., Olyarnik S., et al. (2009). Accelerating loss of seagrasses across the globe threatens coastal ecosystems. Proc. Natl. Acad. Sci. 106, 12377–12381. doi: 10.1073/pnas.0905620106
Williams S. L. (1995). Surfgrass (Phyllospadix torreyi) reproduction: reproductive phenology, resource allocation, and male rarity. Ecology 76, 1953–1970. doi: 10.2307/1940726
Xiao L. T., Wang S. G. (2005). Experimental techniques of plant physiology (Beijing: China: Agricultural Press), 262–279.
Xu S., Xu S., Zhou Y., Yue S., Zhang X., Gu R., et al. (2021). Long-term changes in the unique and largest seagrass meadows in the bohai Sea (China) using satellite (1974-2019) and sonar data: implication for conservation and restoration. Remote Sens. 13, 856. doi: 10.3390/rs13050856
Yaakub S. M., Chen E., Bouma T. J., Erftemeijer P. L., Todd P. A. (2014). Chronic light reduction reduces overall resilience to additional shading stress in the seagrass Halophila ovalis. Mar. pollut. Bull. 83, 467–474. doi: 10.1016/j.marpolbul.2013.11.030
You J., Chan Z. (2015). ROS regulation during abiotic stress responses in crop plants. Front. Plant Sci. 6. doi: 10.3389/fpls.2015.01092
Zhang P. D., Fang C., Liu J., Xu Q., Li W. T., Liu Y. S. (2015). An effective seed protection method for planting Zostera marina (eelgrass) seeds: implications for their large-scale restoration. Mar. pollut. Bull. 95, 89–99. doi: 10.1016/j.marpolbul.2015.04.036
Zhang Y. H., Li J. D., Wu Z. X., Yuan S. J., Li W. T., Zhang P. D. (2022a). Effects of different prolonged light durations on survival, growth and physiology of the eelgrass Zostera marina. Front. Environ. Sci. 10. doi: 10.3389/fenvs.2022.893377
Zhang Y. H., Wang H. H., Li F., Sun J., Li W. T., Zhang P. D. (2022b). The combined effect of planting density and sediment fertilization on survival, growth and physiology of eelgrass zostera marina. Mar. pollut. Bull. 184, 114136. doi: 10.1016/j.marpolbul.2022.114136
Zhao W., Chen S. P., Lin G. H. (2008). Compensatory growth responses to clipping defoliation in Leymus chinensis (Poaceae) under nutrient addition and water deficiency conditions. Plant Ecol. 196, 85–99. doi: 10.1007/s11258-007-9336-3
Keywords: phyllospadix iwatensis, rocky shores, leaf trimming, survival, restoration technique
Citation: Sun J, Luo F-S, Yu G-X, Zhang H-Y, Li W-T and Zhang P-D (2023) Assessment of the establishment success of surfgrass Phyllospadix iwatensis from shoots in a leaf trimming method: implications for large-scale restoration. Front. Mar. Sci. 10:1165354. doi: 10.3389/fmars.2023.1165354
Received: 14 February 2023; Accepted: 20 April 2023;
Published: 05 May 2023.
Edited by:
Stanislao Bevilacqua, University of Trieste, ItalyCopyright © 2023 Sun, Luo, Yu, Zhang, Li and Zhang. This is an open-access article distributed under the terms of the Creative Commons Attribution License (CC BY). The use, distribution or reproduction in other forums is permitted, provided the original author(s) and the copyright owner(s) are credited and that the original publication in this journal is cited, in accordance with accepted academic practice. No use, distribution or reproduction is permitted which does not comply with these terms.
*Correspondence: Pei-Dong Zhang, zhangpdsg@ouc.edu.cn