- 1School of Natural Sciences, Macquarie University, Macquarie Park, NSW, Australia
- 2Department of Primary Industries Fisheries, Port Stephens Fisheries Institute, Taylors Beach, NSW, Australia
- 3Department of Primary Industries Fisheries, Byron Bay, NSW, Australia
- 4Department of Primary Industries Fisheries, Southern Regional Fisheries Office, Batemans Bay, NSW, Australia
- 5The Nature Conservancy, Ultimo, NSW, Australia
- 6Sydney Institute of Marine Science, Mosman, NSW, Australia
Globally, oyster reef restoration is on the rise. In many instances, restoration is occurring alongside established oyster aquaculture industries that grew to prominence following oyster reef demise. This paper examines the potential positive and negative interactions between the two industries and identifies key factors that may promote positive interactions. Interactions between the two industries result from shared resource requirements (e.g., space, clean water, brood-stock, breeding programs), shared knowledge requirements (e.g. around threats and their mitigation, factors optimizing growth/survival) and biological interactions (e.g. over-catch, disease spill-over, competition for resources). Many of these interactions are reciprocated, and can shift from positive to negative depending on environmental, biological and socio-economic conditions. From our examination, three key factors emerge as shaping the strength and direction (positive or negative) of interactions: (1) whether the focal species is common or different between the two industries; (2) the physicochemical and socio-economic environment in which the two industries are occurring; and (3) whether there is open dialogue and consultation between the two industries and relevant stakeholders. Positive interactions can be maximized where the two industries are able to co-invest in and share infrastructure (e.g. hatcheries, breeding programs), resources (e.g. spat, broodstock, shell) and knowledge (e.g. optimal conditions of growth) – an easier task where the target oyster species is in common. Positive interactions may also be maximized by utilizing marine spatial planning tools, such as suitability modelling, to inform optimal siting of the two industries. As the two industries continue to grow, open and inclusive dialogue between these and key stakeholders will be essential for mitigating risk and maximising positive synergies.
Introduction
Oyster reefs are important estuarine habitats that support abundant and diverse communities of invertebrates and fish (Peterson et al., 2003; Grabowski et al., 2005; McLeod et al., 2020; Cole et al., 2022). Historic overharvest using destructive fishing practices has, in combination with more recent declines in water quality and disease, resulted in the loss of 85% of the world’s oyster reefs (Beck et al., 2011; Gillies et al., 2020). In many jurisdictions, the decline in wild oyster reefs, and the fisheries they once sustained, was accompanied by the rise of shellfish aquaculture (Nell, 2001; Ruesink et al., 2005; Pouvreau et al., 2023). Today, molluscs are the second largest category of farmed seafood, in quantity and value, with oysters the leading molluscan species cultivated (Botta et al., 2020). Besides food and pearl production, oyster aquaculture industries can support a range of ecosystem services, including habitat provision, water filtration and shoreline protection (Alleway et al., 2019). Consequently, oyster aquaculture is often considered among the most sustainable forms of aquaculture (Williamson et al., 2015).
For over a century lost oyster reefs were a forgotten ecosystem across much of the globe (e.g. Alleway and Connell, 2015). However, over recent decades analyses of historical records have reilluminated the important role oyster reefs once played (e.g Beck et al., 2011; Zu Ermgassen et al., 2012; Alleway and Connell, 2015) and reinvigorated efforts to restore oyster reefs (Blomberg et al., 2018; Pogoda et al., 2019; McAfee et al., 2020). Due to shared environmental and resource requirements for farming and restoring oysters (Nell, 2001; Botta et al., 2020), many attempts to restore oysters have occurred proximally to oyster aquaculture industries. Oyster reef restoration may be aimed at enhancing molluscan and associated fin fisheries production (Peterson et al., 2003), stabilizing and protecting shorelines, and improving water quality (Nelson et al., 2004; McClenachan et al., 2020; Morris et al., 2021). Both the process of oyster restoration, and the growth of associated industries (e.g. tourism, fishing), can stimulate jobs and the economy (Kroeger, 2012; Grabowski et al., 2012; McAfee et al., 2022). However, poorly planned projects that are progressed without adequate stakeholder consultation can also cause significant economic, environmental, and/or social losses, particularly where conducted using non-native species (Paolisso et al., 2006) or in areas that did not historically support oyster reef (Polk and Eulie, 2018).
Oyster reef restoration typically involves the provision of a hard substrate (e.g. clean recycled shell base, quarried rock, pre-fabricated concrete units) and/or live oysters (introduced as juveniles on shell or adults) at environmentally suitable sites for oyster growth and survival (Fitzsimons et al., 2020; Howie and Bishop, 2021). The resources and knowledge required for successful oyster reef restoration are similar as for oyster aquaculture, such that the two industries can benefit from co-location (Ben-Horin et al., 2018; Wasson et al., 2020; Figure 1). However, co-location of the industries can also lead to negative (often reciprocated) interactions from competition for food and space and exchange of disease, parasites and pests (Forrest et al., 2009). Additionally, restored oyster reefs may increase unwanted recruitment on farmed oysters (O'Connor and Dove, 2009). The strength of these positive and negative interactions may depend on whether the two industries focus on the same or different oyster species, the environmental and social contexts in which the industries are operating, and the extent of consultation and dialogue between the two. Negative interactions will, presumably, be greatest in instances where they are not properly considered in the early planning stage of a restoration project and, hence, appropriately controlled.
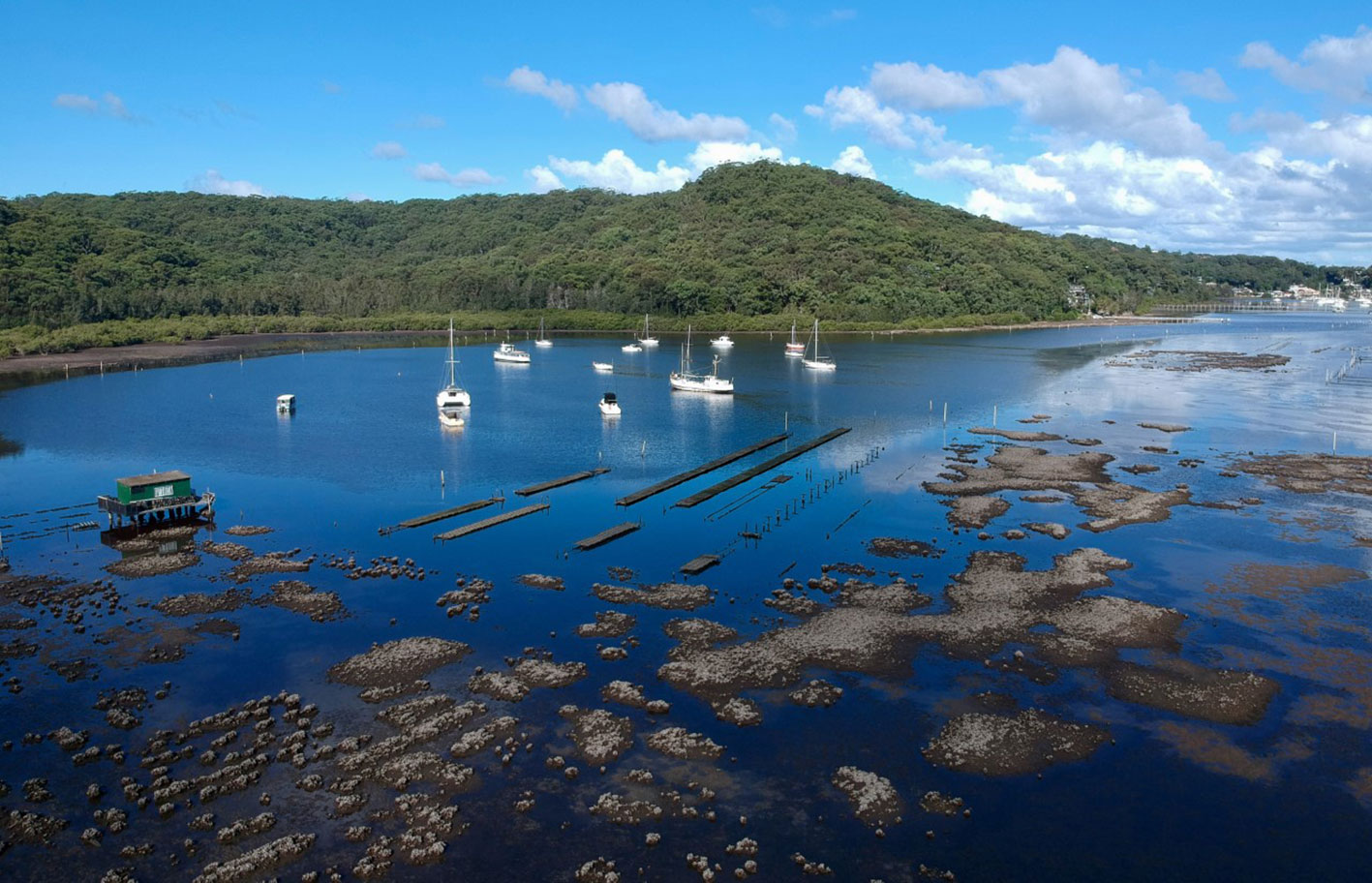
Figure 1 Co-location of oyster aquaculture and wild oyster reefs at Daley’s Point, New South Wales, Australia.
This paper examines positive and negative interactions between restoration and oyster aquaculture industries and identifies factors that promote positive interactions. Based on this examination we provide recommendations for maximising positive interactions between oyster aquaculture and restoration.
Potential benefits of oyster aquaculture for oyster reef restoration
The growing fields of conservation aquaculture (Froehlich et al., 2017; Wasson et al., 2020; Ridlon et al., 2021) and restorative aquaculture (Theuerkauf et al., 2019b; Carranza and Zu Ermgassen, 2020; Overton et al., 2023) recognise the important role aquaculture can play in supporting conservation efforts. Oyster aquaculture can benefit oyster reef restoration by: (1) providing contemporary knowledge and historical context of those conditions and sites that maximise oyster recruitment, growth and survival; (2) providing resources, such as water quality and environmental data, stock and genetic resources for projects that require seeding, and shell substrate; and (3) providing environmental and biological conditions, and stewardship, that support healthy reefs (Ridlon et al., 2021; Ridlon et al., 2023). Additionally, in jurisdictions where the harvest of wild shellfish is permitted, aquaculture may take harvest pressure off wild reefs (Anderson, 1985).
Especially where the species of oyster to be restored is common to the aquaculture industry, oyster farmers can be important sources of knowledge on conditions and practices that maximise oyster recruitment, growth and survival. Both oyster aquaculture and oyster reef restoration must overcome similar stressors, to maximise oyster growth and survival. These include environmental stressors such as sedimentation, heat stress and poor water quality, as well as biotic stressors, such as predation, disease and parasitism (Wasson, 2010; Coen and Bishop, 2015; Scanes et al., 2020; Esquivel-Muelbert et al., 2022). The successful operation of an oyster aquaculture industry in an estuary can therefore be a key indicator of the suitability of environmental conditions for establishment of oyster reefs alongside. Additionally, in some instances, the management actions used by the aquaculture industry to mitigate these stressors may also be applied to restored oyster reefs (Thomson, 1954; Buestel et al., 2009). For example, this may include working alongside land managers to manage sediment and nutrient inputs from sources such as unsealed roads and unfenced livestock.
Traditionally, the livelihood of oyster farmers depended on the collection of wild spat (juvenile oysters), and subsequently maximising their growth and survival. Many oyster farmers consequently have developed a strong knowledge base of the locations and conditions under which there is high larval supply (the ‘catching areas’), there is reliable growth (‘grow-out leases’), and where oyster meat yield is maximal (the ‘paddock’ or ‘fattening grounds’), as well as the timing of recruitment events. Additionally, with many oyster farms held within families across generations, the aquaculture industry can be an important source of knowledge of changes in conditions through time, including past and present distributions of oyster reefs. A recent survey of oyster farmers in New South Wales, Australia, revealed that 91% had knowledge of extant and 23% knowledge of extinct oyster reef locations (New South Wales Department of Primary Industries, 2019). Particularly where historical records of past oyster reef distributions are not available, this knowledge can be invaluable in planning oyster reef restoration projects.
In some instances, this local-knowledge, held within generations of farming communities, has been accompanied by industry-focused research programs, that quantify how key parameters such as growth, reproduction and survival respond to environmental factors (e.g. Nell and Holliday, 1988; Dove and O'Connor, 2007). Knowledge of the ecological ‘niche’ (environmental tolerances) of oyster species has formed the basis of GIS-based Habitat Suitability Models, which match environmental data to niche data to predict the suitability of locations for future oyster reefs (e.g. Cake, 1983; Barnes et al., 2007; Soniat et al., 2013). Such Habitat Suitability Models, augmented with additional factors including the potential for social interactions, built marine infrastructure, land use zoning, and logistical and legislative considerations, form a Restoration Suitability Model (RSM; Howie and Bishop, 2021). RSMs are often an important first step in shortlisting sites that might be suitable for oyster reef restoration, particularly in areas where historic distributions are unknown or in which physico-chemical conditions have changed through time (Fitzsimons et al., 2020; Howie and Bishop, 2021). More recently they have been adapted to also integrate ecosystem service considerations, such as the optimisation of water quality enhancement by oyster filtration (Thauerkauf et al., 2019a) or nature-based shoreline protection (La Peyre et al., 2015).
Shellfish aquaculture industries are also often supported by spatially and temporally replicated water quality monitoring programs, which can serve as important data inputs to Restoration Suitability Models. Oysters readily acquire contaminants and pathogens from the water through their filter feeding (Portnoy et al., 1975; Silva et al., 2001), and hence the industry is highly regulated for sanitation (de Souza et al., 2018). Whereas in some jurisdictions, regulation is based around sampling of shellfish flesh (European Union; de Souza et al., 2018), in others, water quality is monitored to classify production areas in relation to standards (e.g. The National Shellfish Sanitation Program, USA; de Souza et al., 2018). Alongside indicators of public health risk such as biotoxins and faecal coliforms, these programs may include collection of environmental data such as temperature and salinity (e.g. NSW Food Authority – NSW Shellfish Program). These environmental data, as well as data collected by local, state and national government agencies, research projects, and other industries, can be fed into Restoration Suitability Models.
Besides knowledge of environmental conditions, the oyster aquaculture industry is an important direct and indirect source of material resources for restoration, which may include equipment, oyster shell substrate, larvae, spat or mature transplants for seeding, and/or genetic resources (Wasson et al., 2020). Oysters typically require multiple years of growth in order to reach a size suitable for commercial harvest (Bosch et al., 2010; Palmer et al., 2020). Consequently, broadcast spawning genera of oysters, which can be reproductively capable from their first year (e.g. Saccostrea glomerata, Dinamani, 1974), may spawn one or more times on oyster farms, if cultivated as reproductively capable diploids. In New Zealand, spill-over of mussel larvae from aquaculture industries at some locations is subsidising restoration efforts (Norrie et al., 2020). Oyster aquaculture likely plays a similar role in supplying larvae in areas where oyster reefs have been depleted.
Oyster aquaculture industries can supply the seed for restoration projects at localities where oysters have been extirpated, or where the effective breeding population is small, maladapted or genetically impoverished. This seed may be donated by oyster farmers from their collections of wild-caught spat (Pouvreau et al., 2023), or produced in hatcheries (Froehlich et al., 2017; Wasson et al., 2020). In Quiberon Bay France, since 2015 oyster farmers have provided 1% of their annual collected spat for seeding a former oyster bed in the bay (Pouvreau et al., 2023). In other parts of the world, such as eastern Australia and the USA, hatcheries and their selective breeding programs are being harnessed to supply seed for restoration (termed, “conservation aquaculture”, Froehlich et al., 2017; Wasson et al., 2020). These hatchery-produced spat are often selectively bred for desirable traits such as rapid growth and disease resistance and in some instances also resilience to warming waters (Naciri-Graven et al., 1998; O'Connor and Dove, 2009; Tan et al., 2020; Nascimento-Schulze et al., 2021; Overton et al., 2023). Larvae may be settled onto sanitised shell (cultch) in the hatchery or pediveliger larvae can be transported to the reef restoration location and settled onto cultch using remote settlement techniques (Fitzsimons et al., 2020). Alternatively, cultchless spat or adults may be introduced directly to restoration sites (Fitzsimons et al., 2020). The latter may be ‘gardened’, whereby oysters are reared non-commercially by community members in baskets under jetties or docks, before being introduced to restoration sites at a size that is more robust to predators and stressors (Brumbaugh et al., 2002; Anderson et al., 2019). Where disease has led to genetic bottlenecks in wild populations (Thompson et al., 2017), appropriately designed selective breeding programs may be a way of reintroducing genetic diversity to wild populations (Ridlon et al., 2021). Increased genetic diversity will also enhance the capacity of reef building oysters to adapt to changing conditions (Ridlon et al., 2023).
Shell waste produced by routine oyster aquaculture operations can provide a source of substrate on which reefs can grow and accrete (Morris et al., 2019). These activities include culling of over-catch, removal of dead oysters, and shucking oysters for half-shell and canning industries (Morris et al., 2019; New South Wales Department of Primary Industries, 2019). As the natural substrate on which oyster reefs grow, shell is often favoured over artificial materials and rock as the substrate for oyster reef establishment (Goelz et al., 2020). Oysters display greater recruitment to oyster shell than other commonly used substrates, including concrete, limestone, granite and other types of shell (e.g. surf clams, scallops; Hemraj et al., 2022). Oyster shell can be deployed loose, in biodegradable mesh bags or gabion baskets, or embedded in substrate (e.g. Ysebaert et al., 2019; Uddin et al., 2021; Comba et al., 2022). Furthermore, living oyster clumps removed from oyster farm infrastructure during routine farming operations may provide an alternative combined supply of oyster stock and substrate for restoration projects within the estuary from which the clumps were sourced. The use of this more developed and seasoned oyster stock may potentially accelerate oyster reef development, though this is yet to be trialled. However, before being transported to be deployed at distant restoration sites, shell should be treated (typically by heating, drying, acid or bleaching) to remove any pathogens or hitchhikers that may pose a biosecurity risk (Diggles, 2021).
Finally, oyster farms may enhance the probability of establishing healthy reef ecosystems by providing a source of fish and invertebrate colonists, by improving environmental conditions, and by lowering parasite loads. Oyster farms often support many of the same species of fish and macro-invertebrates as oyster reefs, as a result of their habitat structure, and filter-feeding activities (Dumbauld et al., 2009; Martínez-Baena et al., 2022). Consequently, in locations where remnant oyster reefs are scarce or lacking, oyster farms can play an important role in supplying those populations of fish and macro-invertebrates that characterise reef ecosystems (Martínez-Baena et al., 2022). Additionally, filter feeding by cultivated oysters improves water quality (Dumbauld et al., 2009; Humphries et al., 2016) which might facilitate the recruitment of wild oysters. The water-based infrastructure required for oyster farming may ameliorate environmental stressors like wave and wind energy that may otherwise be inhibitory of the establishment of oyster reefs (Dumbauld et al., 2009). Moreover, despite the misconception that aquaculture will necessarily lead to increased disease incidence among sympatric wild populations, in some instances aquaculture may dilute the effects of disease agents by serving as hosts that can be harvested before disease peaks (Ben-Horin et al., 2018).
Potential benefits of oyster reef restoration for oyster aquaculture
Oyster reef restoration projects in estuaries with oyster farms are also likely to have positive, reciprocal, feedbacks for oyster aquaculture.
First, restoring oyster reefs may in and of itself be beneficial to oyster aquaculture. In some instances, the greater total biomass of oysters and filter feeding service resulting from oyster reef restoration may benefit oyster cultivation by improving water quality (Humphries et al., 2016). This may be particularly beneficial in estuaries with large agricultural or urbanised catchments. Generations of additional larvae produced by restored oyster reefs could also benefit spat collecting activities of farmers (Breitburg et al., 2000), though at grow-out sites over-catch (fouling) by the additional larval supply may be problematic (see negative interactions below). Complimentary coastal and catchment management activities put in place to assist oyster reef restoration (for example strategies that effectively reduce suspended sediment, nutrient and/or contaminant loads) may benefit oyster farms through the improvement of water quality.
Second, just as oyster reef restoration may benefit from the knowledge base generated by oyster farming the reverse may also apply. For example, habitat suitability models used to inform the selection of oyster reef restoration sites may also be applied to select new sites for oyster farms and/or spat collection (Brown and Hartwick, 1988; Cho et al., 2012). Additionally, research and development around oyster reef restoration may lead to more efficient and/or effective aquaculture practices. For example, pilot studies for restoration projects addressing growth and survival of oysters across environmental gradients (e.g. McAfee and Connell, 2020; Esquivel-Muelbert et al., 2022) may lead to new knowledge that can be harnessed by aquaculture on optimal conditions for grow-out, and studies of wild oysters may lead to better understanding of patterns of disease incidence along environmental gradients (Bushek et al., 2012).
Third, oyster reef restoration may provide opportunities for oyster farmers to diversify their income, through the provision of animals and/or shell substrate, specialist equipment, and experienced labour for restoration projects (Ridlon et al., 2021). Where the species targeted by restoration is distinct from that cultivated for consumption, this can build resilience in the industry as many of the oyster diseases that can cause catastrophic loss are specific to certain genera. Provision by the aquaculture industry of substrate for reef growth can also decrease costs associated with waste disposal (McAfee and Connell, 2020), though as oyster-reef restoration projects are often one-off events, the longevity of this financial benefit may be limited.
Fourth, the restoration of rare or depleted oyster species may increase the availability of seed and broodstock for aquaculture. For example, in Europe, demand for O. edulis seed for restoration is leading to investment in hatcheries and may drive re-establishment of breed polls (shallow-water oyster lagoons) and ponds, that also enhance seed supply for aquaculture (Colsoul et al., 2021). In southern Australia, the fledgling O. angasi aquaculture industry requires the collection of wild, sexually mature adults for spawning (Crawford, 2016). Oyster reef restoration projects targeting this presently rare species may increase the number of viable adults in the wild, reducing the time spent by farmers searching for viable adults as well as potentially increasing the likelihood of collecting high-quality genetically diverse broodstock.
Finally, oyster reef restoration, particularly where community led or accompanied by citizen scientist monitoring programs, is likely to improve public awareness of the important ecosystem services that oysters provide (Toomey et al., 2020). This may help increase the environmental (‘green’) image of the oyster aquaculture industry, fuelling support for growth and expansion of the industry.
Potential negative effects of oyster aquaculture on oyster reef restoration
Despite the many benefits oyster aquaculture may confer to oyster reef restoration, negative impacts of oyster aquaculture may also arise where it creates genetic pollution, facilitates proliferation of non-native species, serves as a source of disease, creates estuarine use conflict and/or leads to environmental degradation.
Because oyster aquaculture industries are increasingly dependent on selectively bred stock, the potential for genetic pollution exists (Šegvić-Bubić et al., 2020). Genetic pollution occurs when genotypes that are otherwise rare or absent in the wild are enhanced through interbreeding with aquaculture stocks. Studies to date have, however, found little evidence for genetic introgression of aquaculture lines into wild oyster populations (Hare et al., 2006; Carlsson et al., 2008; Thompson et al., 2017). This may be because aquaculture lines, which are typically bred for fast growth but not necessarily resilience to environmental stressors, are maladapted to survival in the wild. Alternatively this lack of genetic introgression could be because in many estuaries the biomass of cultivated oysters is small relative to that of remnant wild oysters. As an example, in New South Wales, Australia, the three estuaries, Port Stephens, Botany Bay and Wagonga, in which significant oyster reef restoration projects have been conducted or planned, cultivated oysters account for 2, 0, and 27% of total oysters respectively (NSW DPI, unpublished data).
In estuaries where non-native species are utilised in aquaculture (e.g. Crassostrea/Magallana gigas outside of Japan), there is the potential for these to proliferate in the wild, competing with native oyster species (Ruesink et al., 2005). Globally, there are over 182 records of 18 oyster species being moved to 73 countries, largely for aquaculture purposes (Ruesink et al., 2005). The Pacific oyster, the most translocated oyster, is now considered naturalised in at least 17 countries, significantly altering diversity, community structure and ecosystem processes (Herbert et al., 2016). Though in some jurisdictions (e.g. the Netherlands), naturalised populations of oysters are being harnessed in nature based solutions (Ysebaert et al., 2019), the proliferation of non-native oysters in the wild is generally seen at odds with efforts to restore native oysters (e.g. Ostrea edulis; Zu Ermgassen et al., 2020). The risk of non-native species proliferation in the wild may be reduced through the cultivation of sterile triploids (Piferrer et al., 2009).
Oyster farms, and oyster translocations, can also be the source of disease-causing parasites, predators and competitors that may spill over to negatively affect shellfish reefs (Forrest et al., 2009; Coen and Bishop, 2015; Goedknegt et al., 2017). For example, large-scale movements of oysters for aquaculture have contributed to the introduction and spread of pathogens including Marteilia refringens, Bonamia ostreae and B. exitiosa in European waters (Culloty and Mulcahy, 2007; Wolff and Reise, 2002). These pathogens represent a significant threat to the remaining wild O. edulis populations, and are a key hurdle to overcome in re-establishing functional reefs (Zu Ermgassen et al., 2020). Many of these pathogens are notifiable to the World Organization for Animal Health and regulations are in place to prevent movement of oysters from infected to non-infected waters. The widespread occurrence of Bonamia spp. among O. edulis, however, places considerable constraints on restoration, in some instances necessitating that it is conducted in disease affected waters (Sas et al., 2020; Zu Ermgassen et al., 2020). Emerging diseases, often first identified from aquaculture, represent an ongoing threat to both aquaculture and restoration industries alike (Bishop et al., 2006). The high densities of oysters on farms, and the movement of stock as well as aquaculture infrastructure (e.g., barges, boats, equipment, personnel) facilitate the rapid spread of parasites and pathogens (Bishop et al., 2006; Ogburn et al., 2007; Forrest et al., 2009). Biosecurity measures such as quarantining and disease screening translocated oysters prior to introduction to new location can assist in reducing risk (Sas et al., 2020).
Estuary use conflicts with aquaculture can also be an impediment to restoration in some parts of the world. For example, in New South Wales, Australia many areas suitable for oyster reef restoration are zoned for oyster aquaculture – Priority Oyster Aquaculture Areas (POAA)– and consequently cannot be used as restoration sites unless an application is made to the relevant State Government Authority to extinguish the POAA for the purposes of restoration. Even where zoning allows for co-location of restoration and farming activities, the areas most suitable for oyster reef restoration may already be occupied by oyster farms, leading to the selection of sub-optimal sites for restoration. Additionally, the scope for restoration may also be limited in estuaries with a significant oyster aquaculture industry (Smaal and Van Duren, 2019) because cultivated oysters and biofouling communities on farming infrastructure reduce the availability of food for other filter-feeders through top-down control (Souchu et al., 2001; Huang et al., 2008).
Aquaculture operations that replace or damage other biogenic habitats may disrupt seascape connectivity of developing oyster reefs. Oyster farms are often situated in shallow and sheltered waters, where seagrass meadows naturally occur. Mobile species, such as fish, may move between oyster reefs and other coastal habitats at time scales of tides to years, to shelter and feed (Martínez-Baena et al., 2022). When poorly managed, oyster farms can have negative impacts on seagrass beds below or adjacent to structures (Everett et al., 1995; Forrest et al., 2009). Such impacts may compromise the biodiversity of oyster reefs.
Potential negative effects of oyster reef restoration on oyster aquaculture
Increasing the number of oysters within an estuary through restoration activities may present biological and social challenges to aquaculture. These potential challenges are recognized by aquaculture industries with 30% of respondents to a 2019 survey of New South Wales, Australia, oyster farmers expressing concerns about possible risks to oyster aquaculture from oyster reef restoration (New South Wales Department of Primary Industries, 2019). Restored oyster reefs could act as a reservoir for diseases or pests or introduce new ones, compete with aquaculture stocks for food, create problems of over-catch for farms and lead to estuarine use conflict. Nevertheless, in the many parts of the world where oyster reef restoration is a new endeavour, the spatial footprint of restoration sites is small compared to that of oyster aquaculture.
There is growing evidence that oysters on farms are more susceptible to diseases than their wild counterparts (Wilkie et al., 2013). This may be a consequence of their higher stocking density, greater genetic homogeneity and/or greater physiological stress. Consequently, parasites that may exist in small sub-lethal reservoirs in oyster reefs may produce severe disease impacts on oyster farms (Bishop et al., 2006). Increasing the abundance of oyster reefs will increase this reservoir potential. Additionally, oyster reefs may promote the population growth of oyster predators such as flatworms, drills and fishes (Grabowski and Peterson, 2007) that “spill over” and may reduce yields from aquaculture. Predation is one of the major hurdles to the successful field aquaculture of oysters in many parts of the world (Jory et al., 1984). Though cultivation in bags and baskets off-bottom, manual removal of predators, regularly cleaning, controlled air exposure and chemical treatment can reduce predation (Jory et al., 1984; Pit and Southgate, 2003), none are entirely effective (Jory et al., 1984).
Furthermore, the use of cultch in restoration projects especially shell collected from unknown sources such as via restaurant shell recycling schemes, comes with inherent risk attached in terms of biosecurity. Dead shell can be infected or contaminated with disease, even when it has been out of the water for significant periods of time (Diggles, 2021). The movement of people, equipment, shell and oysters between estuaries as part of a restoration project also brings the risk of diseases and pests being translocated (Zu Ermgassen et al., 2020). Though emerging oyster restoration networks (e.g. Native Oyster Restoration Alliance) have recognised this risk and are attempting to control it (Pogoda et al., 2019), there are countless examples where recreational boating and aquaculture translocations have facilitated spread of emerging diseases and pests despite protective measures being in place. A recent example is the spread of Ostereid herpesvirus-1 µVar in Europe and New Zealand as a consequence of oyster translocations (Pernet et al., 2016).
Increasing oyster biomass through restoration may in small, low productivity and/or heavily cultivated estuaries result in the carrying capacity of the environment for oysters being approached or even exceeded (Smaal and Van Duren, 2019). Where competition for food resources results, reductions in growth rates of farmed oyster populations will be seen. The potentially significant added oyster biomass from restoration may also lead to enhanced larval supply and unwanted over-catch of oyster recruits biofouling cultivated oysters and oyster farming infrastructure. Over-catch control is one of the most significant costs in oyster farming (Cox et al., 2012) as it reduces growth rates of oysters, and can make them less aesthetically appealing for sale on the half shell, or even unmarketable. Heavy encrustations can cause the collapse of infrastructure. Consequently, oyster farmers invest considerable time, and hence money, in removing over-catch.
Coastal restoration projects that proceed without adequate stakeholder consultation may lead to estuarine use conflict and social impacts that undermine the success of the restoration project (La Peyre et al., 2012). Oyster reefs may serve as navigational hazards to oyster farmers, impose future space limitations on the growth of oyster aquaculture, and where the oyster reef restoration leads to development of a wild capture shellfishery, introduce market competition with aquaculture (New South Wales Department of Primary Industries, 2019). Further, where oyster reef restoration produces heightened awareness of the demise of oyster reefs, legislation may be introduced at national, state or local level to aid in their protection and restoration. This may have negative consequences for the industry if this restricts the sites and/or activities that are permitted in support of farming. It is important that in shortlisting sites for oyster reef restoration, not only environmental suitability and logistics, but also social conflict are considered (Puckett et al., 2018). Early engagement of stakeholders in the decision making and planning process may not only assist in avoiding conflict, but also improve environmental outcomes by identifying key indicators of suitability that may otherwise be missed (Puckett et al., 2018).
The way forward: maximising positive and minimising negative interactions
This paper has identified both positive and negative interactions that may occur between oyster aquaculture and oyster reef restoration (Table 1). These interactions result from shared resource (e.g. space, animals, breeding programs) and environmental (e.g. physico-chemical) requirements, as well as biological interactions (e.g. competition, disease, predation, facilitation) between the two. Many of these interactions are reciprocated, and can shift from positive to negative depending on environmental, biological and socio-economic conditions. From our examination, key factors that emerge that drive the nature of interactions are: (1) whether the focal species is common or different between the two industries; (2) the physico-chemical and socio-economic environment in which the two industries are occurring, and (3) whether there is open dialogue and consultation between the two industries and relevant stakeholders. In order to grow prosperous oyster aquaculture industries and functional oyster reefs, there is need to recognise these drivers of interactions and adopt management strategies and consultation practices that favour positive interactions.
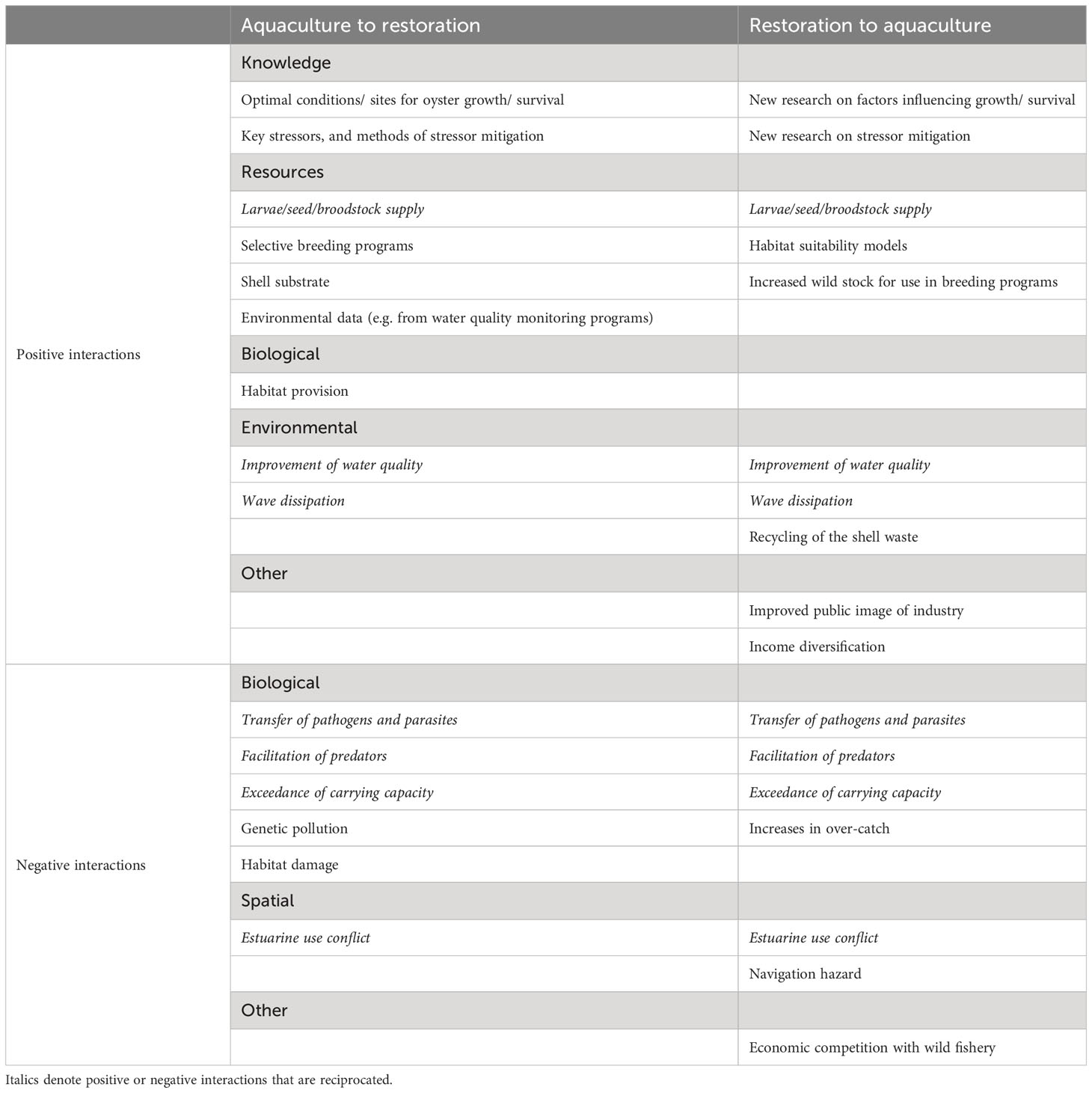
Table 1 Summary of the positive and negative interactions between oyster aquaculture and restoration.
With many aquaculture industries continuing to focus on non-native species, but most restoration targeting native species, the commonality (or not) of species between the industries can be a key determinant of interactions. Where the oyster species is common, there is greater potential for knowledge exchange and sharing of genetic resources, brood stock and spat between the two. However, the risk of disease transfer can be greater. Where the target species differs, there is in some instances greater potential for unwanted over-catch and competitive interactions. However, as many oyster diseases are species- or genus-specific, the risk of disease transfer is reduced. Restoration of native oyster reefs may help to facilitate a shift from non-native to native species cultivation in areas where it has not previously been feasible, strengthening opportunities for knowledge sharing and sharing of genetic and brood-stock resources.
Focusing the two industries on common species will provide greater opportunity for co-design and co-investment in infrastructure and research and development programs that benefit both industries. For example, breeding programs are in place throughout the world, to produce fast growing and disease resilient oysters for aquaculture. These breeding programs may be adapted to produce oysters with enhanced resilience to growing environmental threats, such as heat waves, acidification and/or low or variable food supply (Dove and O'Connor, 2009; Nascimento-Schulze et al., 2021). Additionally, these breeding programs may be harnessed to enhance genetic diversity of wild oyster populations (Thompson et al., 2017). Maintenance, and even enhancement, of genetic diversity and the identification of locally adapted populations will benefit both the aquaculture and oyster reef restoration industries (Hughes et al., 2017; Li et al., 2018), particularly given rapidly changing environmental conditions due to increasing coastal development and climate change.
Furthermore, whilst not acting as a substitute for oyster reef restoration, investment in commercial native oyster aquaculture industries may aid ecosystem recovery in instances where public funds to support coastal restoration efforts are limited or unavailable (Theueurkauf et al., 2019). Both sustainable aquaculture and oyster reef restoration can provide food and habitat to invertebrates and fish, provide filtration services and add oyster spawning stock biomass to coastal ecosystems.
The physico-chemical and socio-economic environment in which aquaculture and restoration activities occurs also appeared to be a key determinant of interactions between the two. Over-catch, competition for food and space, and disease exchange can all be problematic in small semi-enclosed embayments where water retention is high and exchange (and hence carrying capacity) is low (Smaal and Van Duren, 2019). Zoning arrangements, designed to regulate aquaculture, could in these instances also inhibit restoration activities. Modelling the carrying capacity of estuaries, though challenging (Filgueira et al., 2014), could provide mutual benefits to aquaculture and restoration industries by avoiding over populating estuaries whilst maximising the benefits associated with healthy oyster populations, such as improved water quality (Figure 2).
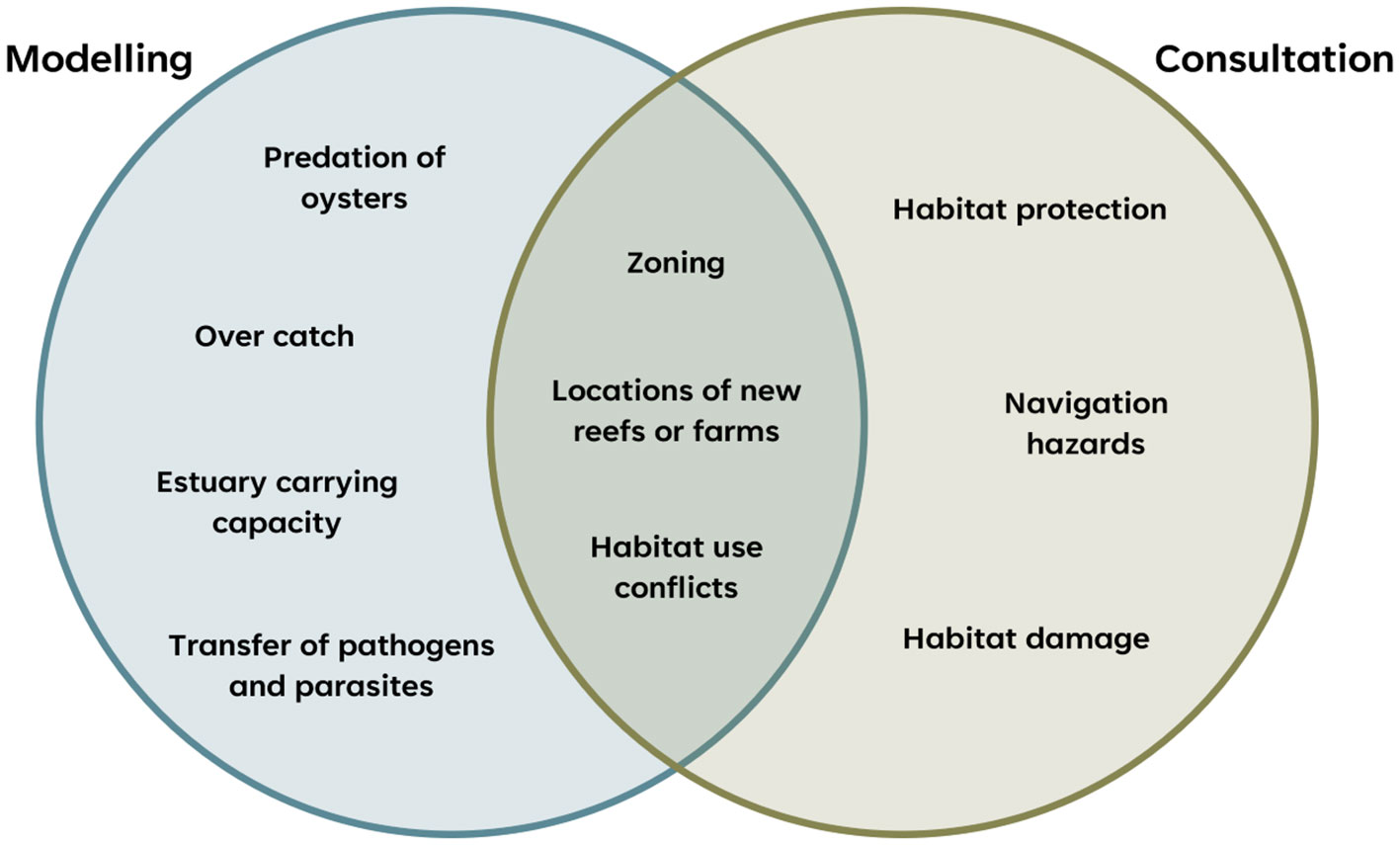
Figure 2 Venn diagram highlighting when negative feedbacks can be mitigated by informed habitat suitability modelling, or well-constructed stakeholder consultation.
In areas where there is already a significant aquaculture industry, habitat and restoration suitability modelling can be used to shortlist sites that are environmentally suitable for oyster reef restoration but minimize potential negative interactions (e.g. over-catch, introduction of navigation hazards) and maximise positive interactions (e.g. habitat provision for desired biodiversity; Figure 2). Similar models may also, potentially, be applied to the siting of new aquaculture leases, to optimise oyster production and minimise negative environmental interactions. For example, the assessment criteria outlined in the NSW Oyster Industry Sustainable Aquaculture Strategy (New South Wales Department of Primary Industries, 2021) for new POAA in NSW estuaries assesses proximity to navigation hazards, conservation areas (including estuarine habitats), and Aboriginal and non-Indigenous heritage items, amongst other variables.
Marine spatial planning, that is responsive to the needs of both industries, and considers the carrying capacity of the environment, will be critical in enabling the industries to coexist. Swam et al. (2022) provide an example of how spatial planning can support management of oyster metapopulations, while identifying zones supportive of off-bottom aquaculture. Using GIS mapping, they classified coastal Louisana into zones supportive of (1) broodstock sanctuary reefs (i.e., reproductive populations), (2) productive reefs during dry (salty) years, (3) productive reefs during wet (fresh) years, and (4) off-bottom aquaculture development. Whereas the Broodstock Sanctuary Zone was defined around environmental parameters that optimize reef sustainability and fecundity across years, the Aquaculture Zone was defined by conditions that maximise oyster growth and survival, but not reproduction and excluded the influence of predation (as oysters are grown in predator-excluding baskets from hatchery provided seed). The Dry and Wet year zones supported reproduction in some but not all years, enabling the persistence of reefs in areas that may not be suitable for aquaculture.
Consultation between the two industries is also key to minimising negative interactions. For example, given the deep-rooted knowledge oyster farmers have of oysters and estuarine ecosystems they should be key stakeholders that inform the location, design and implementation of oyster reef restoration projects. Early engagement is critical to avoiding habitat use conflicts and potential zoning issues (Figure 2). In some instances, positive interactions between aquaculture and restoration industries may be enhanced through the creation of multiple use zones where aquaculture and restoration can occur alongside one another. This may facilitate shared surveillance/monitoring programs for diseases and pests and water quality issues that affect both aquaculture and reef restoration.
Overall, this paper highlights the considerable synergies between oyster aquaculture and restoration industries, and the potential for the two to work together for the mutual benefit of both. In order to ensure that opportunities for co-benefits are maximised, and mal-adaptive outcomes are avoided it is essential that an open dialogue is established between aquaculture and restoration early in the expansion of one or other industry, and that this and consultation with other relevant stakeholders is maintained.
Author contributions
MB, BL and JE-M conceived the manuscript, and MB and BL wrote the first draft. All authors contributed to the article and approved the submitted version.
Funding
JE-M was supported by the RTP-PhD scholarship and KF by the RTP-MRes scholarship from Macquarie University during the preparation of this manuscript. This project contributes to ARC Linkage Grant LP180100732 and to NSW Environmental Trust Grant 2018-RD-0023.
Conflict of interest
The author(s) declared that they were an editorial board member of Frontiers, at the time of submission. This had no impact on the peer review process and the final decision.
The handling editor HA declared a past co-authorship with the author MB.
Publisher’s note
All claims expressed in this article are solely those of the authors and do not necessarily represent those of their affiliated organizations, or those of the publisher, the editors and the reviewers. Any product that may be evaluated in this article, or claim that may be made by its manufacturer, is not guaranteed or endorsed by the publisher.
References
Alleway H. K., Connell S. D. (2015). Loss of an ecological baseline through the eradication of oyster reefs from coastal ecosystems and human memory. Conserv. Biol. 29, 795–804. doi: 10.1111/cobi.12452
Alleway H. K., Gillies C. L., Bishop M. J., Gentry R. R., Theuerkauf S. J., Jones R. (2019). The ecosystem services of marine aquaculture: Valuing benefits to people and nature. BioScience 69, 59–68. doi: 10.1093/biosci/biy137
Anderson J. L. (1985). Market interactions between aquaculture and the common-property commercial fishery. Mar. Resource Economics 2, 1–24. doi: 10.1086/mre.2.1.42628874
Anderson L., Sacks P., Donnelly M., Barker V., Anderson S., Walters L. (2019). Oyster reef enhancement utilizing gardened oysters in a subtropical estuary. Restor. Ecol. 27, 966–973. doi: 10.1111/rec.12975
Barnes T. K., Volety A. K., Chartier K., Mazzotti F. J., Pearlstine L. (2007). A habitat suitability index model for the eastern oyster (Crassostrea virginica), a tool for restoration of the Caloosahatchee Estuary, Florida. J. Shellfish Res. 26, 949–959. doi: 10.2983/0730-8000(2007)26[949:AHSIMF]2.0.CO;2
Beck M. W., Brumbaugh R. D., Airoldi L., Carranza A., Coen L. D., Crawford C., et al. (2011). Oyster reefs at risk and recommendations for conservation, restoration, and management. BioScience 61, 107–116. doi: 10.1525/bio.2011.61.2.5
Ben-Horin T., Burge C. A., Bushek D., Groner M. L., Proestou D. A., Huey L. I., et al. (2018). Intensive oyster aquaculture can reduce disease impacts on sympatric wild oysters. Aquaculture Environ. Interact. 10, 557–567. doi: 10.3354/aei00290
Bishop M. J., Carnegie R. B., Stokes N. A., Peterson C. H., Burreson E. M. (2006). Complications of a non-native oyster introduction: facilitation of a local parasite. Mar. Ecol. Prog. Ser. 325, 145–152. doi: 10.3354/meps325145
Blomberg B. N., Pollack J. B., Montagna P. A., Yoskowitz D. W. (2018). Evaluating the US Estuary Restoration Act to inform restoration policy implementation: A case study focusing on oyster reef projects. Mar. Policy 91, 161–166. doi: 10.1016/j.marpol.2018.02.014
Bosch D., Kuminoff N., Stephenson K., Miller A., Pope J., Harris A. (2010). Evaluation of policy options for expanding oyster aquaculture in Virginia. Aquaculture Economics Manage. 14, 145–163. doi: 10.1080/13657301003776698
Botta R., Asche F., Borsum J. S., Camp E. V. (2020). A review of global oyster aquaculture production and consumption. Mar. Policy 117, 103952. doi: 10.1016/j.marpol.2020.103952
Breitburg D. L., Coen L. D., Luckenbach M., Mann R. L., Posey M., Wesson J. A. (2000). Oyster reef restoration: convergence of harvest and conservation strategies. J. Shellfish Res. 19, 371.
Brown J. R., Hartwick E. B. (1988). A habitat suitability index model for suspended tray culture of the Pacific oyster, Crassostrea gigas Thunberg. Aquaculture Res. 19, 109–126. doi: 10.1111/j.1365-2109.1988.tb00414.x
Brumbaugh R. D., Sorabella L. A., Garcia C. O., Goldsborough W. J., Wesson J. A. (2002). Making a case for community-based oyster restoration: an example from Hampton Roads, Virginia, USA. J. Shellfish Res. 19, 467–472.
Buestel D., Ropert M., Prou J., Goulletquer P. (2009). History, status, and future of oyster culture in France. J. Shellfish Res. 28, 813–820. doi: 10.2983/035.028.0410
Bushek D., Ford S. E., Burt I. (2012). Long-term patterns of an estuarine pathogen along a salinity gradient. J. Mar. Res. 70, 225–251. doi: 10.1357/002224012802851968
Cake E. W. (1983). Habitat suitability index models: Gulf of Mexico American oyster (Washington DC USA: National Coastal Ecosystems Team, Division of Biological Services, Research and Development, Fish and Wildlife Service, US Department of the Interior).
Carlsson J., Carnegie R. B., Cordes J. F., Hare M. P., Leggett A. T., Reece K. S. (2008). Evaluating recruitment contribution of a selectively bred aquaculture line of the oyster, Crassostrea virginica used in restoration efforts. J. Shellfish Res. 27, 1117–1124. doi: 10.2983/0730-8000-27.5.1117
Carranza A., Zu Ermgassen P. S. (2020). A global overview of restorative shellfish mariculture. Front. Mar. Sci. 7, 722. doi: 10.3389/fmars.2020.00722
Cho Y., Lee W. C., Hong S., Kim H. C., Kim J. B. (2012). GIS-based suitable site selection using habitat suitability index for oyster farms in Geoje-Hansan Bay, Korea. Ocean Coast. Manage. 56, 10–16. doi: 10.1016/j.ocecoaman.2011.10.009
Coen L. D., Bishop M. J. (2015). The ecology, evolution, impacts and management of host–parasite interactions of marine molluscs. J. Invertebrate Pathol. 131, 177–211. doi: 10.1016/j.jip.2015.08.005
Cole V. J., Harasti D., Lines R., Stat M. (2022). Estuarine fishes associated with intertidal oyster reefs characterized using environmental DNA and baited remote underwater video. Environ. DNA 4, 50–62. doi: 10.1002/edn3.190
Colsoul B., Boudry P., Pérez-Parallé M. L., Bratoš Cetinić A., Hugh-Jones T., Arzul I., et al. (2021). Sustainable large-scale production of European flat oyster (Ostrea edulis) seed for ecological restoration and aquaculture: a review. Rev. Aquaculture 13, 1423–1468. doi: 10.1111/raq.12529
Comba D., Palmer T. A., Breaux N. J., Pollack J. B. (2022). Evaluating biodegradable alternatives to plastic mesh for small-scale oyster reef restoration. Restor. Ecol. 32, e13762. doi: 10.1111/rec.13762
Cox B., Kosmeyer P., O’Connor W., Dove M., Johnstone K. (2012). Oyster over-catch: Cold shock treatment. Bedford Park SA: Australian Seafood Cooperative Research Centre. https://www.frdc.com.au/sites/default/files/products/2010-734-DLD.pdf.
Crawford C. M. (2016). National review of Ostrea angasi aquaculture: historical culture, current methods and future priorities (Hobart: Institute for Marine and Antarctic Studies). Available at: http://www.imas.utas.edu.au/:data/assets/pdf_file/0005/936536/160181-UTAS-Scientific-Report_-Angasi-aquaculture.pdf.
Culloty S., Mulcahy M. (2007). Bonamia ostreae in the native oyster Ostrea edulis: a review. Marine Environment and Health Series No. 29. Rinville: Marine Institute.
de Souza R. V., Campos C., Garbossa L. H. P., Vianna L. F. D. N., Vanz A., Rupp G. S., et al. (2018). A critical analysis of the international legal framework regulating the microbiological classification of bivalve shellfish production areas. Rev. Aquaculture 10, 1025–1033. doi: 10.1111/raq.12222
Diggles B. K. (2021). Biosecurity risks related to recycling of mollusc shell waste for shellfish reef restoration in Australia. Ecol. Manage. Restor. 22 (2), 145–159. doi: 10.1111/emr.12472
Dinamani P. (1974). Reproductive cycle and gonadial changes in the New Zealand rock oyster Crassostrea glomerata. New Z. J. Mar. Freshw. Res. 8, 39–65. doi: 10.1080/00288330.1974.9515490
Dove M. C., O'Connor W. A. (2007). Salinity and temperature tolerance of Sydney rock oysters Saccostrea glomerata during early ontogeny. J. Shellfish Res. 26, 939–947. doi: 10.2983/0730-8000(2007)26[939:SATTOS]2.0.CO;2
Dove M. C., O'Connor W. A. (2009). Commercial assessment of growth and mortality of fifth-generation Sydney rock oysters Saccostrea glomerata (Gould 1850) selectively bred for faster growth. Aquaculture Res. 40, 1439–1450. doi: 10.1111/j.1365-2109.2009.02243.x
Dumbauld B. R., Ruesink J. L., Rumrill S. S. (2009). The ecological role of bivalve shellfish aquaculture in the estuarine environment: a review with application to oyster and clam culture in West Coast (USA) estuaries. Aquaculture 290, 196–223. doi: 10.1016/j.aquaculture.2009.02.033
Esquivel-Muelbert J. R., Lanham B. S., Martínez-Baena F., Dafforn K. A., Gribben P. E., Bishop M. J. (2022). Spatial variation in the biotic and abiotic filters of oyster recruitment: Implications for restoration. J. Appl. Ecol. 59, 953–964. doi: 10.1111/1365-2664.14107
Everett R. A., Ruiz G. M., Carlton J. T. (1995). Effect of oyster mariculture on submerged aquatic vegetation: an experimental test in a Pacific Northwest estuary. Mar. Ecol. Prog. Ser. 125, 205–217. doi: 10.3354/meps125205
Filgueira R., Guyondet T., Comeau L. A., Grant J. (2014). A fully-spatial ecosystem-DEB model of oyster (Crassostrea virginica) carrying capacity in the Richibucto Estuary, Eastern Canada. J. Mar. Syst. 136, 42–54. doi: 10.1016/j.jmarsys.2014.03.015
Fitzsimons J. A., Branigan S., Gillies C. L., Brumbaugh R. D., Cheng J., DeAngelis B. M., et al. (2020). Restoring shellfish reefs: Global guidelines for practitioners and scientists. Conserv. Sci. Pract. 2, e198. doi: 10.1111/csp2.198
Forrest B. M., Keeley N. B., Hopkins G. A., Webb S. C., Clement D. M. (2009). Bivalve aquaculture in estuaries: review and synthesis of oyster cultivation effects. Aquaculture 298, 1–15. doi: 10.1016/j.aquaculture.2009.09.032
Froehlich H. E., Gentry R. R., Halpern B. S. (2017). Conservation aquaculture: Shifting the narrative and paradigm of aquaculture’s role in resource management. Biol. Conserv. 215, 162–168. doi: 10.1016/j.biocon.2017.09.012
Gillies C. L., Castine S. A., Alleway H. K., Crawford C., Fitzsimons J. A., Hancock B., et al. (2020). Conservation status of the oyster reef ecosystem of southern and eastern Australia. Global Ecol. Conserv. 22, e00988. doi: 10.1016/j.gecco.2020.e00988
Goedknegt M. A., Schuster A. K., Buschbaum C., Gergs R., Jung A. S., Luttikhuizen P. C., et al. (2017). Spillover but no spillback of two invasive parasitic copepods from invasive Pacific oysters (Crassostrea gigas) to native bivalve hosts. Biol. Invasions 19, 365–379. doi: 10.1007/s10530-016-1285-0
Goelz T., Vogt B., Hartley T. (2020). Alternative substrates used for oyster reef restoration: a review. J. Shellfish Res. 39, 1–12. doi: 10.2983/035.039.0101
Grabowski J. H., Brumbaugh R. D., Conrad R. F., Keeler A. G., Opaluch J. J., Peterson C. H., et al. (2012). Economic valuation of ecosystem services provided by oyster reefs. Bioscience 62, 900–909. doi: 10.1525/bio.2012.62.10.10
Grabowski J. H., Hughes A. R., Kimbro D. L., Dolan M. A. (2005). How habitat setting influences restored oyster reef communities. Ecology 86, 1926–1935. doi: 10.1890/04-0690
Grabowski J. H., Peterson C. H. (2007). Restoring oyster reefs to recover ecosystem services. Ecosystem Engineers: Plants to Protists 4, 281–298. doi: 10.1016/S1875-306X(07)80017-7
Hare M. P., Allen S. K., Bloomer P., Camara M. D., Carnegie R. B., Murfree J., et al. (2006). A genetic test for recruitment enhancement in Chesapeake Bay oysters, Crassostrea virginica, after population supplementation with a disease tolerant strain. Conserv. Genet. 7, 717–734. doi: 10.1007/s10592-005-9108-3
Hemraj D. A., Bishop M. J., Hancock B., Minuti J. J., Thurstan R. H., Zu Ermgassen P. S., et al. (2022). Oyster reef restoration fails to recoup global historic ecosystem losses despite substantial biodiversity gain. Sci. Adv. 8, eabp8747. doi: 10.1126/sciadv.abp8747
Herbert R. J., Humphreys J., Davies C. J., Roberts C., Fletcher S., Crowe T. P. (2016). Ecological impacts of non-native Pacific oysters (Crassostrea gigas) and management measures for protected areas in Europe. Biodiversity Conserv. 25, 2835–2865. doi: 10.1007/s10531-016-1209-4
Howie A. H., Bishop M. J. (2021). Contemporary oyster reef restoration: responding to a changing world. Front. Ecol. Evol. 9, 689915. doi: 10.3389/fevo.2021.689915
Huang C. H., Lin H. J., Huang T. C., Su H. M., Hung J. J. (2008). Responses of phytoplankton and periphyton to system-scale removal of oyster-culture racks from a eutrophic tropical lagoon. Mar. Ecol. Prog. Ser. 358, 1–12. doi: 10.3354/meps07465
Hughes A. R., Hanley T. C., Byers J. E., Grabowski J. H., Malek J. C., Piehler M. F., et al. (2017). Genetic by environmental variation but no local adaptation in oysters (Crassostrea virginica). Ecol. Evol. 7, 697–709. doi: 10.1002/ece3.2614
Humphries A. T., Ayvazian S. G., Carey J. C., Hancock B. T., Grabbert S., Cobb D., et al. (2016). Directly measured denitrification reveals oyster aquaculture and restored oyster reefs remove nitrogen at comparable high rates. Front. Mar. Sci. 3, 74. doi: 10.3389/fmars.2016.00074
Jory D. E., Carriker M. R., Iversen E. S. (1984). Preventing predation in molluscan mariculture: an overview. J. World Mariculture Soc. 15, 421–432. doi: 10.1111/j.1749-7345.1984.tb00176.x
Kroeger T. (2012). Dollars and sense: economic benefits and impacts from two oyster reef restoration projects in the Northern Gulf of Mexico. Nat. Conservancy, 101. https://www.conservationgateway.org/Documents/2_Oyster%20restoration%20study_Kroeger%20May%209%202012.pdf.
La Peyre M. K., Nix A., Laborde L., Piazza B. P. (2012). Gauging state-level and user group views of oyster reef restoration activities in the northern Gulf of Mexico. Ocean Coast. Manage. 67, 1–8. doi: 10.1016/j.ocecoaman.2012.06.001
La Peyre M. K., Serra K., Joyner T. A., Humphries A. (2015). Assessing shoreline exposure and oyster habitat suitability maximizes potential success for sustainable shoreline protection using restored oyster reefs. PeerJ 3, e1317. doi: 10.7717/peerj.1317
Li L., Li A., Song K., Meng J., Guo X., Li S., et al. (2018). Divergence and plasticity shape adaptive potential of the Pacific oyster. Nat. Ecol. Evol. 2, 1751–1760. doi: 10.1038/s41559-018-0668-2
Martínez-Baena F., Lanham B. S., McLeod I., Taylor M. D., McOrrie S., Bishop M. J. (2022). De novo reefs: Fish habitat provision by oyster aquaculture varies with farming method. Aquaculture Environ. Interact. 14, 71–84. doi: 10.3354/aei00431
McAfee D., Connell S. D. (2020). Cuing oyster recruitment with shell and rock: implications for timing reef restoration. Restor. Ecol. 28, 506–511. doi: 10.1111/rec.13134
McAfee D., McLeod I. M., Alleway H. K., Bishop M. J., Branigan S., Connell S. D., et al. (2022). Turning a lost reef ecosystem into a national restoration program. Conserv. Biol. 36, e13958. doi: 10.1111/cobi.13958
McAfee D., McLeod I. M., Boström-Einarsson L., Gillies C. L. (2020). The value and opportunity of restoring Australia's lost rock oyster reefs. Restor. Ecol. 28, 304–314. doi: 10.1111/rec.13125
McClenachan G. M., Donnelly M. J., Shaffer M. N., Sacks P. E., Walters L. J. (2020). Does size matter? Quantifying the cumulative impact of small-scale living shoreline and oyster reef restoration projects on shoreline erosion. Restor. Ecol. 28, 1365–1371. doi: 10.1111/rec.13235
McLeod I. M., Boström-Einarsson L., Creighton C., D’Anastasi B., Diggles B., Dwyer P. G., et al. (2020). Habitat value of Sydney rock oyster (Saccostrea glomerata) reefs on soft sediments. Mar. Freshw. Res. 71, 771–781. doi: 10.1071/MF18197
Morris J. P., Backeljau T., Chapelle G. (2019). Shells from aquaculture: a valuable biomaterial, not a nuisance waste product. Rev. Aquaculture 11, 42–57. doi: 10.1111/raq.12225
Morris R. L., La Peyre M. K., Webb B. M., Marshall D. A., Bilkovic D. M., Cebrian J., et al. (2021). Large-scale variation in wave attenuation of oyster reef living shorelines and the influence of inundation duration. Ecol. Appl. 31, e2382. doi: 10.1002/eap.2382
Naciri-Graven Y., Martin A. G., Baud J. P., Renault T., Gérard A. (1998). Selecting the flat oyster Ostrea edulis (L.) for survival when infected with the parasite Bonamia ostreae. J. Exp. Mar. Biol. Ecology. 224, 91–107. doi: 10.1016/S0022-0981(97)00171-8
Nascimento-Schulze J. C., Bean T. P., Houston R. D., Santos E. M., Sanders M. B., Lewis C., et al. (2021). Optimizing hatchery practices for genetic improvement of marine bivalves. Rev. Aquaculture 13, 2289–2304. doi: 10.1111/raq.12568
Nell J. A., Holliday J. E. (1988). Effects of salinity on the growth and survival of Sydney rock oyster (Saccostrea commercialis) and Pacific oyster (Crassostrea gigas) larvae and spat. Aquaculture 68, 39–44. doi: 10.1016/0044-8486(88)90289-X
Nelson K. A., Leonard L. A., Posey M. H., Alphin T. D., Mallin M. A. (2004). Using transplanted oyster (Crassostrea virginica) beds to improve water quality in small tidal creeks: a pilot study. J. Exp. Mar. Biol. Ecol. 298, 347–368. doi: 10.1016/S0022-0981(03)00367-8
New South Wales Department of Primary Industries (2019). Oyster reef restoration: NSW oyster industry survey. (NSW Department of Planning, Industry and Environment).
New South Wales Department of Primary Industries (2021). NSW Oyster Industry Sustainable Aquaculture Strategy Fourth Edition 2021. (Department of Regional NSW).
Norrie C., Dunphy B., Roughan M., Weppe S., Lundquist C. (2020). Spill-over from aquaculture may provide a larval subsidy for the restoration of mussel reefs. Aquaculture Environ. Interact. 12, 231–249. doi: 10.3354/aei00363
O'Connor W. A., Dove M. C. (2009). The changing face of oyster culture in New South Wales, Australia. J. Shellfish Res. 28, 803–811. doi: 10.2983/035.028.0409
Ogburn D. M., White I., McPhee D. P. (2007). The disappearance of oyster reefs from eastern Australian estuaries — impact of colonial settlement or mudworm invasion? Coast. Manage. 35, 271–287. doi: 10.1080/08920750601169618
Overton K., Dempster T., Swearer S. E., Morris R. L., Barrett L. T. (2023). Achieving conservation and restoration outcomes through ecologically beneficial aquaculture. Conserv. Biol., e14065. doi: 10.1111/cobi.14065
Palmer S. C., Gernez P. M., Thomas Y., Simis S., Miller P. I., Glize P., et al. (2020). Remote sensing-driven Pacific oyster (Crassostrea gigas) growth modeling to inform offshore aquaculture site selection. Front. Mar. Sci. 6, 802. doi: 10.3389/fmars.2019.00802
Paolisso M., Dery N., Herman S. (2006). Restoration of the Chesapeake Bay using a non-native oyster: ecological and fishery considerations. Hum. Organ. 65, 253–267. doi: 10.17730/humo.65.3.bqqr1mh15fkdxwv8
Pernet F., Lupo C., Bacher C., Whittington R. J. (2016). Infectious diseases in oyster aquaculture require a new integrated approach. Philos. Trans. R. Soc. B: Biol. Sci. 371, 20150213. doi: 10.1098/rstb.2015.0213
Peterson C. H., Grabowski J. H., Powers S. P. (2003). Estimated enhancement of fish production resulting from restoring oyster reef habitat: quantitative valuation. Mar. Ecol. Prog. Ser. 264, 249–264. doi: 10.3354/meps264249
Piferrer F., Beaumont A., Falguière J. C., Flajšhans M., Haffray P., Colombo L. (2009). Polyploid fish and shellfish: production, biology and applications to aquaculture for performance improvement and genetic containment. Aquaculture 293, 125–156. doi: 10.1016/j.aquaculture.2009.04.036
Pit J. H., Southgate P. C. (2003). Fouling and predation; how do they affect growth and survival of the blacklip pearl oyster, Pinctada margaritifera, during nursery culture? Aquaculture Int. 11, 545–555. doi: 10.1023/B:AQUI.0000013310.17400.97
Pogoda B., Brown J., Hancock B., Preston J., Pouvreau S., Kamermans P., et al. (2019). The Native Oyster Restoration Alliance (NORA) and the Berlin Oyster Recommendation: bringing back a key ecosystem engineer by developing and supporting best practice in Europe. Aquat. Living Resour. 32, 13. doi: 10.1051/alr/2019012
Polk M. A., Eulie D. O. (2018). Effectiveness of living shorelines as an erosion control method in North Carolina. Estuaries Coasts 41, 2212–2222. doi: 10.1007/s12237-018-0439-y
Portnoy B. L., Mackowiak P. A., Caraway C. T., Walker J. A., McKinley T. W., Klein C. A. (1975). Oyster-associated hepatitis: failure of shellfish certification programs to prevent outbreaks. Jama 233, 1065–1068. doi: 10.1001/jama.1975.03260100035016
Pouvreau S., Lapègue S., Arzul I., Boudry P. (2023). Fifty years of research to counter the decline of the European flat oyster (Ostrea edulis): a review of French achievements and prospects for the restoration of remaining beds and revival of aquaculture production. Aquat. Living Resour. 36, 13. doi: 10.1051/alr/2023006
Puckett B. J., Theuerkauf S. J., Eggleston D. B., Guajardo R., Hardy C., Gao J., et al. (2018). Integrating larval dispersal, permitting, and logistical factors within a validated habitat suitability index for oyster restoration. Front. Mar. Sci. 5, 76. doi: 10.3389/fmars.2018.00076
Ridlon A. D., Grosholz E. D., Hancock B., Miller M. W., Bickel A., Froehlich H. E., et al. (2023). Culturing for conservation: the need for timely investments in reef aquaculture. Front. Mar. Sci. 10, 1069494. doi: 10.3389/fmars.2023.1069494
Ridlon A. D., Wasson K., Waters T., Adams J., Donatuto J., Fleener G., et al. (2021). Conservation aquaculture as a tool for imperiled marine species: Evaluation of opportunities and risks for Olympia oysters, Ostrea lurida. PloS One 16, e0252810. doi: 10.1371/journal.pone.0252810
Ruesink J. L., Lenihan H. S., Trimble A. C., Heiman K. W., Micheli F., Byers J. E., et al. (2005). Introduction of non-native oysters: ecosystem effects and restoration implications. Annu. Rev. Ecology Evol. Systematics 36, 643–689. doi: 10.1146/annurev.ecolsys.36.102003.152638
Sas H., Kamermans P., zu Ermgassen P. S., Pogoda B., Preston J., Helmer L., et al. (2020). Bonamia infection in native oysters (Ostrea edulis) in relation to European restoration projects. Aquat. Conservation-Marine Freshw. Ecosystem 30, 2150–2162. doi: 10.1002/aqc.3430
Scanes E., Parker L. M., O'Connor W. A., Dove M. C., Ross P. M. (2020). Heatwaves alter survival of the Sydney rock oyster, Saccostrea glomerata. Mar. pollut. Bull. 158, 111389. doi: 10.1016/j.marpolbul.2020.111389
Šegvić-Bubić T., Žužul I., Talijančić I., Ugrin N., Lepen Pleić I., Žuvić L., et al. (2020). Translocation and aquaculture impact on genetic diversity and composition of wild self-sustainable Ostrea edulis populations in the Adriatic sea. Front. Mar. Sci. 7, 84. doi: 10.3389/fmars.2020.00084
Silva C. A. R., Rainbow P. S., Smith B. D., Santos Z. L. (2001). Biomonitoring of trace metal contamination in the Potengi estuary, Natal (Brazil), using the oyster Crassostrea rhizophorae, a local food source. Water Res. 35 (17), 4072–4078. doi: 10.1016/S0043-1354(01)00144-0
Smaal A. C., Van Duren L. A. (2019). “Bivalve aquaculture carrying capacity: concepts and assessment tools,” in Goods and services of marine bivalves (Cham: Springer), 451–483.
Soniat T. M., Conzelmann C. P., Byrd J. D., Roszell D. P., Bridevaux J. L., Suir K. J., et al. (2013). Predicting the effects of proposed Mississippi River diversions on oyster habitat quality; application of an oyster habitat suitability index model. J. Shellfish Res. 32, 629–638. doi: 10.2983/035.032.0302
Souchu P., Vaquer A., Collos Y., Landrein S., Deslous-Paoli J. M., Bibent B. (2001). Influence of shellfish farming activities on the biogeochemical composition of the water column in Thau lagoon. Mar. Ecol. Prog. Ser. 218, 141–152. doi: 10.3354/meps218141
Swam L. M., Couvillion B., Callam B., La Peyre J. F., La Peyre M. K. (2022). Defining oyster resource zones across coastal Louisiana for restoration and aquaculture. Ocean Coast. Manage. 225, 106178. doi: 10.1016/j.ocecoaman.2022.106178
Tan K., Zhang H., Zheng H. (2020). Selective breeding of edible bivalves and its implication of global climate change. Rev. Aquaculture 12, 2559–2572. doi: 10.1111/raq.12458
Theuerkauf S. J., Eggleston D. B., Puckett B. J. (2019a). Integrating ecosystem services considerations within a GIS-based habitat suitability index for oyster restoration. PloS One 14, e0210936. doi: 10.1371/journal.pone.0210936
Theuerkauf S. J., Morris J. J.A., Waters T. J., Wickliffe L. C., Alleway H. K., Jones R. C. (2019b). A global spatial analysis reveals where marine aquaculture can benefit nature and people. PloS One 14, e0222282. doi: 10.1371/journal.pone.0222282
Thompson J., Stow A., Raftos D. (2017). Lack of genetic introgression between wild and selectively bred Sydney rock oysters Saccostrea glomerata. Mar. Ecol. Prog. Ser. 570, 127–139. doi: 10.3354/meps12109
Toomey A. H., Strehlau-Howay L., Manzolillo B., Thomas C. (2020). The place-making potential of citizen science: Creating social-ecological connections in an urbanized world. Landscape Urban Plann. 200, 103824. doi: 10.1016/j.landurbplan.2020.103824
Uddin M. J., Smith K. J., Hargis C. W. (2021). Development of pervious oyster shell habitat (POSH) concrete for reef restoration and living shorelines. Construction Building Materials 295, 123685. doi: 10.1016/j.conbuildmat.2021.123685
Wasson K. (2010). Informing Olympia oyster restoration: evaluation of factors that limit populations in a California estuary. Wetlands 30, 449–459. doi: 10.1007/s13157-010-0056-4
Wasson K., Gossard D. J., Gardner L., Hain P. R., Zabin C. J., Fork S., et al. (2020). A scientific framework for conservation aquaculture: A case study of oyster restoration in central California. Biol. Conserv. 250, 108745. doi: 10.1016/j.biocon.2020.108745
Wilkie E. M., Bishop M. J., O’Connor W. A., McPherson R. G. (2013). Status of the Sydney rock oyster in a disease-afflicted estuary: persistence of wild populations despite severe impacts on cultured counterparts. Marine and Freshwater Res 64 (3), 267–276.
Williamson T. R., Tilley D. R., Campbell E. (2015). Emergy analysis to evaluate the sustainability of two oyster aquaculture systems in the Chesapeake Bay. Ecol. Eng. 85, 103–120. doi: 10.1016/j.ecoleng.2015.09.052
Wolff W. J., Reise K. (2002). Oyster imports as a vector for the introduction of alien species into northern and western European coastal waters. In Invasive aquatic species of Europe. Distribution, impacts and management. (Dordrecht: Springer Netherlands), 193–205.
Ysebaert T., Walles B., Haner J., Hancock B. (2019). “Habitat modification and coastal protection by ecosystem-engineering reef-building bivalves,” in Goods and services of marine bivalves. Eds. Smaal A., Ferreira J., Grant J., Petersen J., Strand Ø. (Cham: Springer), 253–273. Goods and Services of Marine Bivalves.
Zu Ermgassen P. S., Bonačić K., Boudry P., Bromley C. A., Cameron T. C., Colsoul B., et al. (2020). Forty questions of importance to the policy and practice of native oyster reef restoration in Europe. Aquat. conservation: Mar. Freshw. Ecosyst. 30, 2038–2049. doi: 10.1002/aqc.3462
Keywords: aquaculture, restoration, oyster, shellfish, spatial planning, stakeholder, conflict, ecosystem service
Citation: Bishop MJ, Lanham BS, Esquivel-Muelbert JR, Cole VJ, Faelnar KM, Jenkins C, Keating J, Martínez-Baena F and O’Connor WA (2023) Oyster reef restoration - aquaculture interactions: maximizing positive synergies. Front. Mar. Sci. 10:1162487. doi: 10.3389/fmars.2023.1162487
Received: 09 February 2023; Accepted: 25 August 2023;
Published: 19 September 2023.
Edited by:
Heidi K. Alleway, The Nature Conservancy, United StatesReviewed by:
Rebecca Gentry, The Nature Conservancy, United StatesAlice R. Jones, University of Adelaide, Australia
Elizabeth Mansfield, Florida State University, United States
Copyright © 2023 Bishop, Lanham, Esquivel-Muelbert, Cole, Faelnar, Jenkins, Keating, Martínez-Baena and O’Connor. This is an open-access article distributed under the terms of the Creative Commons Attribution License (CC BY). The use, distribution or reproduction in other forums is permitted, provided the original author(s) and the copyright owner(s) are credited and that the original publication in this journal is cited, in accordance with accepted academic practice. No use, distribution or reproduction is permitted which does not comply with these terms.
*Correspondence: Melanie J. Bishop, melanie.bishop@mq.edu.au
†Present address: Brendan S. Lanham, National Centre for Coasts and Climate, University of Melbourne, Parkville, VIC, Australia
‡These authors have contributed equally to this work and share first authorship