- Laboratory of Marine Ecology, Department of Ecology and Marine Resources, Institute of Biosciences, Federal University of the State of Rio de Janeiro, Rio de Janeiro, Brazil
Sandy beaches are ecologically important, physically dynamic, and heterogeneous habitats intrinsically related to human culture. However, these interactions present challenges for sandy beaches in the Anthropocene as stressors from urbanization increase. This study investigated sandy beach community responses to local urbanization in two periods. Beaches were classified into three urbanization categories: conserved (C), modified (M), and urbanized (U), and compared temporally (1997/1998 – first period; 2012 – second period). We hypothesized that community structure descriptors (total abundance, biomass, and richness) and bioindicator abundance (Emerita brasiliensis and Atlantorchestoidea brasiliensis) decreased temporally and be lower in urbanized beaches. The results partially corroborate the main hypothesis since there were different temporal responses from biological variables between each urbanization category (C, M, and U). The data supported that community structure descriptors decreased as local urbanization increased between categories (C, M, and U). Conserved beaches (C) presented higher values for community descriptors, and populations of E. brasiliensis and A. brasiliensis were more abundant compared to other groups (M and U). Modified beaches (M) presented resilience to local urbanization pressures since they are recently urbanized habitats, and some beaches are inside protected areas. The urbanized beaches (U) were impacted by the long-term pressures of urbanization and recreation, and community descriptors and bioindicators presented the lowest values in both periods. Species undergoing direct development, such as A. brasiliensis, should preferably be used as indicators of temporal changes due to local urbanization on sandy beaches, rather than indirect developers like E. brasiliensis. Identifying suitable indicators for long-term anthropogenic impacts from increasing urbanization is necessary for protecting sandy beach ecology.
1 Introduction
Sandy beaches are of great ecological importance, constituting one-third of the world’s coastline (Luijendijk et al., 2018; McLachlan and Defeo, 2018). Sandy beaches are physically dynamic and heterogeneous habitats defined by the interactions of tides and waves, have varying sand grain size, and shelter biota that varies according to morphodynamic conditions (Defeo and McLachlan, 2005). They provide ecosystem services by protecting the coast, maintaining coastal functions such as erosion control, and supporting human activities such as tourism and recreation (Barbier et al., 2011). Sandy beaches are intrinsically related to human culture and are the most explored ecosystem for recreational activities, offering high socioeconomic value (Schlacher et al., 2007). However, the complex interactions between ecological attributes and human use present challenges for sandy beach management in the Anthropocene (Orlando et al., 2020).
Urbanization refers to the physical transformation of natural environments for human activities (Cabral and Cândido, 2019). Currently, about 40% of the world’s population lives in coastal areas (Martínez et al., 2007), and as urban development accelerates, it causes severe ecological and ecosystem management issues (Baird, 2009). Urbanization, climate change, and resource overuse are the three major threats to coasts worldwide, leading to the transformation of the natural landscape and causing several ecological impacts (Defeo and Elliott, 2021). In the case of sandy beach ecosystems, urbanization can alter these habitats at a local scale (1-10 km) through coastal armoring, breakwaters, artificial night lighting, or at larger scales (>10 km), such as the construction of ports (Defeo et al., 2009; Becchi et al., 2014; Laurino et al., 2022). At a local scale, direct anthropogenic pressures caused by human activities on beaches tend to increase with efficient public transportation and facilities to support recreation and tourism, such as toilets, kiosks, and parking spots (McLachlan et al., 2013; González and Holtmann-Ahumada, 2017).
Recognizing whether a beach is suitable for recreation or conservation is essential for ensuring its ecosystem services and prioritizing uses (McLachlan et al., 2013). Beach management requires a holistic approach that considers the Littoral Active Zone (LAZ) as an ecological and geomorphological unit that summarizes dunes, the beach, and the surf zone to preserve ecosystem resilience (Defeo et al., 2021a; Jorge-Romero et al., 2022). Despite increasing anthropogenic pressures, coastal management often neglects the ecological aspects of sandy beaches and focuses on securing dune vegetation stability and restoring physical attributes such as beach nourishment and breakwaters (Schlacher et al., 2008; Jones et al., 2017). Human occupancy often transforms the natural landscape of beaches and reduces biodiversity by removing dunes and beach vegetation, usually for coastal armoring or construction of beach facilities (Peña-Alonso et al., 2019; Salgado et al., 2022). Exposure to off-road motorized vehicles and the deposition of debris affects birds’ and turtles’ behavior (Schlacher et al., 2013; Fujisaki and Lamont, 2016). Urbanization and recreation also negatively influence macrofaunal community densities and richness (Orlando et al., 2020; Wu et al., 2020), and as human activities increase, sandy beach biota is subjected to increased trampling, improper solid waste disposal and grooming (Afghan et al., 2020). Beach cleaning has intensified to meet recreational demands, which contribute to aesthetics but have negative ecological consequences for communities (Zielinski et al., 2019), and mechanized cleaning causes direct mortality and reduces species richness, abundance, and biomass (Schooler et al., 2019).
Macrofaunal organisms play a crucial role in understanding anthropogenic impacts. Crustaceans are commonly used as ecological indicators on exposed sandy beaches because they are abundant, sensitive to human activities, and can be monitored easily (Suciu et al., 2018; Barboza et al., 2021). Talitrid amphipods with direct development are considered reliable indicators of local urbanization pressures such as trampling and mechanized cleaning (Costa et al., 2020). Beaches with coastal armoring results in lower abundances of talitrids than natural beaches (Sobocinski et al., 2010), and artificial night lights affect their feeding behavior (Luarte et al., 2016). In addition, hippid decapods are widely used in environmental pollution studies due to their filter-feeding habit (Sauco et al., 2010; Donohoe et al., 2021). Studies report their capacity to bioaccumulate metals (Pérez, 1999; Cabrini et al., 2017) and microplastics (Horn et al., 2019), and they are also sensitive to harmful algal blooms (Bretz et al., 2002). The mole crab Emerita brasiliensis (Schmitt, 1935) and sandhopper Atlantorchestoidea brasiliensis (Dana, 1853) are considered suitable indicators of sandy beach health, and the combined use of these organisms can be effective because of their different life-history traits (Cardoso et al., 2016; Frota et al., 2019). E. brasiliensis is a eucarid crustacean with an oceanic planktonic larval stage, whereas A. brasiliensis is a peracarid crustacean that incubates its eggs in their marsupium and hatches as pre-juveniles. They have distinct feeding strategies and inhabit different beach zones. E. brasiliensis filters phytoplankton in the swash zone and lives in the infralittoral zone, whereas A. brasiliensis is detritivorous, feeds on wrack or other biological deposits, and ranges from mesolittoral to supralittoral zone. These organisms respond negatively to intensive recreational activities leading to decreased abundance and even disappearance on heavily urbanized beaches (Veloso et al., 2008; Cardoso et al., 2016).
Sandy beaches are ecosystems at risk and require more research to fill information gaps which can ultimately be integrated into beach management and conservation policies (Nel et al., 2014; Lercari, 2023). To undertake comprehensive impact assessments, it is essential to comprehend the resistance and resilience of beaches to long-term perturbations and to identify effective ecological indicators (Fanini et al., 2020). There have been recent efforts to analyze the temporal dynamics of sandy beaches induced by anthropogenic factors such as fisheries (Lercari et al., 2018), artificial freshwater discharges (Lercari and Defeo, 1999; Jorge-Romero et al., 2019), urbanization (Bessa et al., 2014), and coastal squeeze (Luisa Martínez et al., 2014), but there is still a gap in temporal studies. Cumulative and long-lasting stressors can alter these ecosystems, and identifying ecological indicators that respond to those pressures can help improve sandy beach conservation. A series of indices have been proposed as an alternative to estimate anthropogenic pressures and assist in decision-making and public policy (González and Holtmann-Ahumada, 2017). When integrated with ecological indicators such as macrofaunal organisms, these metrics help assess the impact and provide new insights for conservation (Costa et al., 2017; Laitano et al., 2019).
In this study, we investigated the structure of sandy beach communities and their responses to anthropogenic pressures during two different periods. Beaches were classified into three urbanization categories (conserved, modified, and urbanized) and compared temporally (1997 – first period; 2012 – second period). We hypothesized that community structure descriptors (total abundance, biomass, and richness) and bioindicator abundance (Emerita brasiliensis and Atlantorchestoidea brasiliensis) decreased temporally and be lower in urbanized beaches. The results partially corroborate the main hypothesis since there were different temporal responses from biological variables between each urbanization category (C, M, and U).
2 Material and methods
2.1 Study area
Eighteen exposed sandy beaches were evaluated along the Rio de Janeiro State in Southeast Brazil. All beaches were selected in the 1990s, contemplating beaches with varied coastal development and socio-economic activities, comprising 295 kilometers and covering a large portion of the state’s coastal region. Our study replicated the same design on the same previously selected beaches. In addition to this limitation, the current comparison found the expected pattern of more urbanized beaches in more urban areas of the State. Rio de Janeiro has the highest population density (IBGE, 2010), the third-longest coastline (IBGE, 2017), and is the leading tourist destination in Brazil (Ministério do Turismo, 2018). In recent decades, urban development in the state has accelerated to support population growth, recreational activities, and tourism. From 1991 to 2010, the state of Rio de Janeiro’s population grew by more than three million people, from 12,807,706 to 15,989,929 inhabitants (IBGE, 2017). In 2010, only 54.8% of the population had access to basic sanitation, contributing to untreated sewage disposal in the ocean (SNIS, 2022). Brazil is the 4th largest waste producer globally, and Rio de Janeiro generates approximately 3.5 million metric tons of solid waste annually (Climate and Clean Air Coalition, 2014; WWF, 2019). Coastal environments are widely considered polluted, and the major coastal bays of the state are eutrophic and abundant in multiple pollutants, such as Guanabara Bay and Sepetiba Bay (Olivatto et al., 2019; de Souza et al., 2021; da Silva et al., 2022). Rio de Janeiro has undergone massive coastal modifications due to urbanization, especially in the last 20 years, as previously less-developed zones started to attract real estate investments because of their scenic beauty and large beach arcs (Barickman, 2014). An important factor that intensified pressure in the region was the expansion of urban mobility, as highway construction started connecting more populated to less populated zones and municipalities (Brandão, 2006; Pessanha, 2015). Coastal erosion intensified in recently developed areas, mainly from the urbanization of the waterfront with the construction of bike paths and recreational structures, reaching the post-beach area (Sousa, 2011). The urbanization process around the beaches was represented in thematic maps (Figures S1 and S2) based on Geographic Information Systems (GIS), using information about the number of residents, irregular sanitary sewage, and road construction between 2000 and 2010 (Supplementary materials for more details on building the maps).
The beaches under study were grouped into three categories based on their level of urbanization, recreation, and conservation indices (further details in Section 2.4.1). The three categories were subsequently identified as follows: Conserved beaches included Marambaia, Grumari, Formosa, Massambaba, Carapebus, and Fora beaches. Modified beaches included Barra da Tijuca, Foguete, Itaipu, Itaipuaçu, Jaconé, Pecado, Peró, Tucuns, and Unamar. Urbanized beaches included Copacabana, Ipanema, and São Conrado (Figure 1).
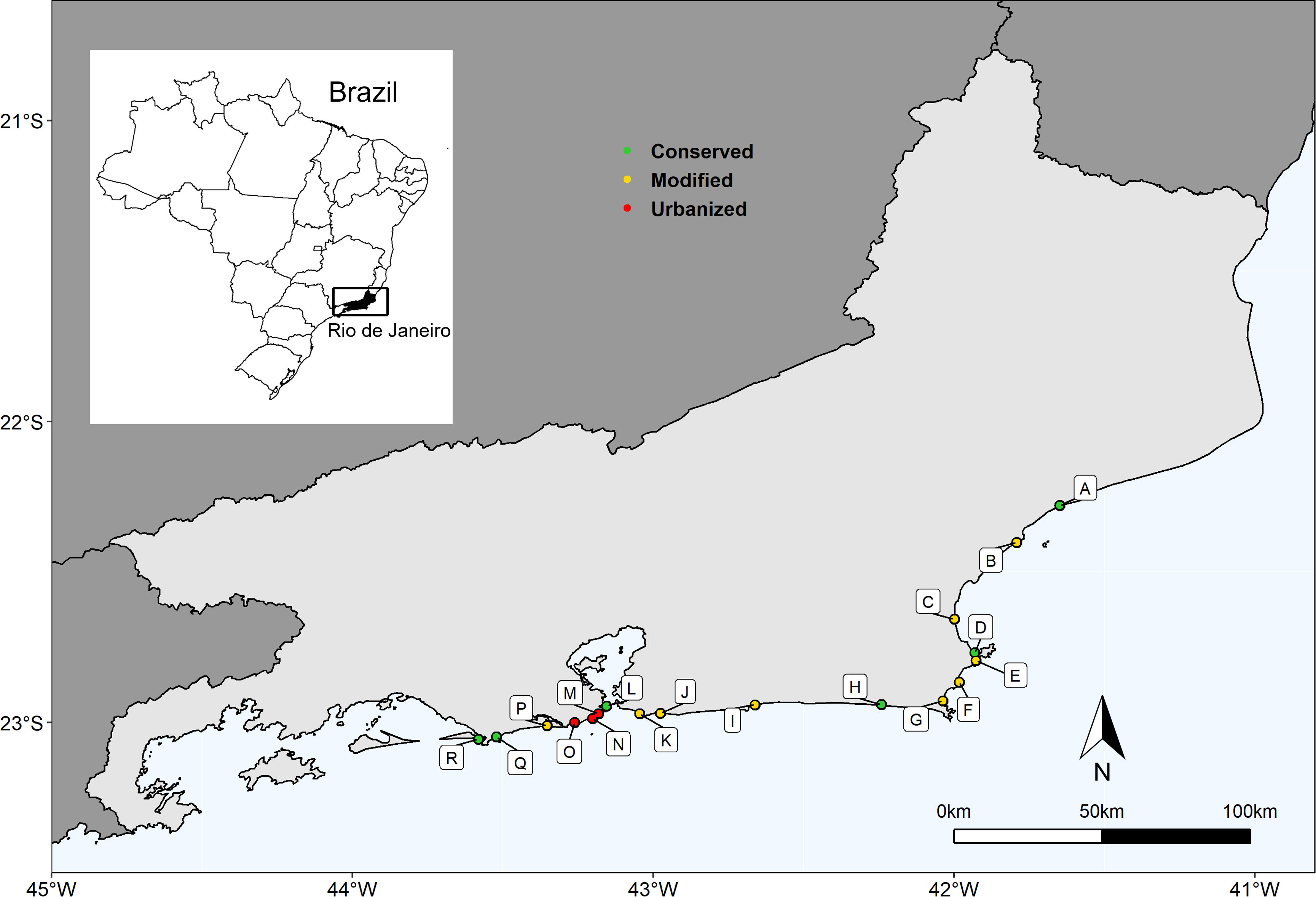
Figure 1 Map of the study area showing 18 sandy beaches along the Rio de Janeiro state coast. A-Carapebus, B- Pecado, C- Unamar, D- Formosa, E- Tucuns, F- Peró, G- Foguete, H- Massambaba, I- Jaconé, J- Itaipuaçu, K- Itaipu, L- Fora, M- Copacabana, N- Ipanema, O- São Conrado, P- Barra da Tijuca, Q- Grumari and R- Marambaia.
Conserved beaches (C) are characterized by low levels of urbanization and recreation, and their accessibility is limited. The Marambaia and Fora beaches are located within military areas with restricted access, where entry is only permitted with formal authorization and exclusively for educational and research activities. Grumari, Formosa, Massambaba, and Carapebus are located within no-take protected areas (Category II of IUCN). These beaches have low visitation rates due to physical limitations such as trails, distance from urban centers, lack of public transportation, and a low number of parking spaces. Except for Fora, all these beaches have high levels of conserved vegetation. Beach grooming is done manually when it occurs.
Modified beaches (M) are characterized by recently urbanized habitats and show intermediate disturbance. They are in the areas of Rio de Janeiro State that have been recently occupied, such as the West Zone of the Capital and the Coastal Lowlands Region. These areas have experienced a population doubling and intensification of beach-and-sun tourism between the studied years. The intensity of use and frequentation is high on holidays and weekends and less intensive on weekdays. The infrastructure to support beachgoers is limited, with a low number of parking spaces and kiosks. Some of these beaches still have vegetation, but less than conserved beaches. Jaconé, Pecado, Peró, Tucuns, and Unamar beaches are inside no-take protected areas (Category II of IUCN).
Urbanized beaches (U) are in the most densely populated area of the metropolitan region of Rio de Janeiro. The impacts in this group have been accumulating since the 1920s, making it one of the first urbanized regions of the state. Additionally, it has been a traditionally important region for culture and tourism, receiving large events and tourists year-round. These beaches are located near urban centers, with efficient public transportation in the surrounding areas. The number of users is high and frequent regardless of the day of the week. Beach vegetation is sparse or absent, with buildings present on the post-shore and high infrastructure for beachgoers, such as kiosks, lifeguard stations, and parking spaces.
2.2 Sampling
Eighteen sandy beaches were sampled during the summer of 1997/1998 (first period) and again during the summer of 2012 (second period). Each beach was sampled once per period, following the same methodology for all procedures to compare possible changes in the community descriptors of macrofauna and physical variables over 15 years. Samplings were carried out during spring low tides, all during summer to reduce interannual biotic and abiotic variability linked to the seasonal cycle (Defeo and Rueda, 2002).
Biological samples were collected from six transects perpendicular to the shoreline, spaced equally at each beach, and each was divided into ten sampling units (N10–N1), totaling 60 samples per beach per period and 2,160 samples overall. N10 represents the highest level of a supralittoral location, and N1 represents the lowest level of an infralittoral location at the waterline. Macrofauna communities were sampled using a 0.04 m² quadrat sampler to a depth of 25 cm and washed through a 0.50 mm mesh. The collected materials were transported to the laboratory for further analysis. Sediment samples were collected with a 3.5 cm diameter corer to a depth of 15 cm at N2 (infralittoral), N6 (mediolittoral), and N10 (supralittoral) at all transects of each sandy beach, totaling 18 samples per beach per period and 648 samples overall. The beach face slope was determined using the method described by Emery (1961) by measuring the height difference between the supralittoral and waterline along all six transects.
2.3 Laboratory procedures
The collected specimens were sorted, counted, and fixed in 70% ethanol. The species were identified by experts from each taxonomic group. To estimate biomass, the specimens were dried at 70°C for 24 h and weighed on a balance with a precision of 0.001 g to obtain their dry weight values. The sediment samples were also oven-dried at 70°C, and the sediment texture was assessed using a series of sieves ranging in size from 2.5–4.0 Φ (Suguio, 1973), graded from boulders (> 256 mm) to ultra-clay (< 0.0020 mm). Sedimentary parameters, including asymmetry (ranging from very negative to very positive) and kurtosis (ranging from very platykurtic to extremely leptokurtic), were estimated using the method described by Folk and Ward (1957). Sediment analysis was performed on Microsoft Excel using the GRADISTAT package (Blott and Pye, 2001).
2.4 Data analysis
2.4.1 Beach groups classification
We applied urbanization, recreation, and conservation indices to estimate local urbanization at all sampled beaches during both periods. For the urbanization index created by González et al. (2014), we made some adaptations. We removed qualitative indicators of human intervention for both periods, such as the quality of the night sky and solid residues in the sand. The other evaluated indicators were calculated based on information provided by urban cleaning companies (beach cleaning), municipal laws (vehicle traffic in the sand, including mechanized cleaning), Google Earth, and available literature (Veloso and Cardoso, 2001; Cardoso et al., 2003; Veloso et al., 2006) to qualitative indicators of human intervention: proximity to urban centers, buildings in the sand, and frequency of visitors. In addition, we used personal communication from Cardoso R.S., the author of this work and of the base article for comparing this study (Veloso et al., 2003), carried out in the 90s.
The urbanization index (UI) was adapted from González et al. (2014), and levels of urbanization were estimated using five indicators: (1) proximity to urban centers, (2) presence of buildings on the beach, (3) beach cleaning method, (4) frequency of visitors, and (5) vehicle traffic on the beach. The conservation index (CI) and recreation index (RI) were calculated according to the methods of McLachlan et al. (2013). The CI indicators were scored as follows: (1) the extent, nature, and condition of the dunes and their vegetation and their connection to the beach; (2) the presence of rare, endangered, or iconic species that are particularly susceptible to disturbance; and (3) the abundance and diversity of intertidal benthic macrofauna. The indicators used for RI were: (1) infrastructure, (2) safety and health, and (3) physical carrying capacity. To calculate these indices, we extracted information from available literature, Google Earth images, and data from the Brazilian Institute of Geography and Statistics (IBGE).
The beaches were categorized according to the degree of urbanization as determined by the conservation (CI), urbanization (UI), and recreation (RI) indices ascertained for the first and second periods. These three variables were subjected to a Principal Component Analysis (PCA) in R using the “vegan” package (Legendre and Legendre, 2012). The PCA divided the beaches along axis 1, with 76.7% explanation, into urbanized beaches on the positive side and conserved ones on the negative side. Beaches associated with the CI (negative side) in both periods (1997 and 2012) were classified as “conserved” (C). Beaches located on the negative side (CI) in 1997 and on the positive side (UI and RI) in 2012 were classified as “modified” (M). A third category was created for beaches located on the positive side (UI and RI) in both periods, categorized as “urbanized” (U) (see Figure S3).
2.4.2 Ecological and urbanization variables relationships
The variations in species richness, total abundance, biomass, and abundance of mole crabs and talitrids were investigated between beaches at different categories of urbanization and between two periods (1997/1998 and 2012) using Generalized Linear Mixed Models (GLMM) implemented in R with the “glmmTMB” package (Zuur et al., 2009). We validated the models using graphical quantile analysis of the randomized residuals using the “DHARMa” package (Dunn and Smyth, 1996; Gelman and Hill, 2006). A Wald chi-square test (Type II) was applied to each term and interaction to obtain the Analysis of Deviance (ANODEV) table with the “car” package. The significance of the interactions was tested using the analysis of contrasts with Tukey’s test in the “lsmeans” package. The predicted values (± CI) for each predictor variable were graphically displayed using the “ggeffects” package.
The Generalized Additive Mixed Models (GAMM; R “mgcv” package) were used to test the relationship between each response variable (species richness, total abundance, biomass, abundance of E. brasiliensis, and A. brasiliensis) with indices (CI, UI, RI) and environmental variables (beach granulometry and slope) (Zuur et al., 2009). A Negative Binomial distribution was used, except for biomass, where a two-part zero-truncated model was applied: Gamma distribution (link=log) to model values greater than zero and binomial distribution (link=logit) to model zeros vs. non-zeros. The predictor variables were smoothed using the cubic regression spline* method, and the amount of smoothing was fixed for the variables CI, UI, and RI (k = 3) and kept free for the variables granulometry and beach slope, where optimal smoothing was estimated by cross-validation (Zuur et al., 2009). We conducted all analyses and plots in the R environment (R Core Team, 2021) using the following packages: ‘HH’ (Heiberger and Holland, 2004), ‘vegan’ (Oksanen et al., 2016), ‘glmmTMB’ (Brooks et al., 2017), ‘mgcv’ (Wood, 2004), ‘DHARMa’ (Hartig, 2016), ‘car’ (Fox and Weisberg, 2019), ‘lsmeans’ (Lenth, 2016), ‘ggplot2’ (Wickham, 2016), and ‘ggeffects’ (Lüdecke, 2018).
3 Results
The community structure descriptors, including species richness, total abundance, biomass, and the abundance of Emerita brasiliensis, decreased temporally, as shown in Table 1. However, the abundance of Atlantorchestoidea brasiliensis did not differ among beaches with different categories of urbanization and periods, as shown in Table S5. Each urbanization category showed different temporal responses for biological variables (C, M, and U). The total community abundance, species richness, biomass, and abundance of A. brasiliensis were higher in conserved beaches and lower in urbanized beaches in both the first and second periods (Figures 2A-C, E). The abundance of E. brasiliensis was higher in conserved beaches and lower in modified beaches, and this pattern was also repeated in the two periods (Figure 2D). The dominant species of the conserved and modified beaches changed compared to the first and second periods while remaining the same in urbanized beaches.

Table 1 Significance values obtained from contrast analysis (Tukey test) of the interaction period vs. degree of urbanization.
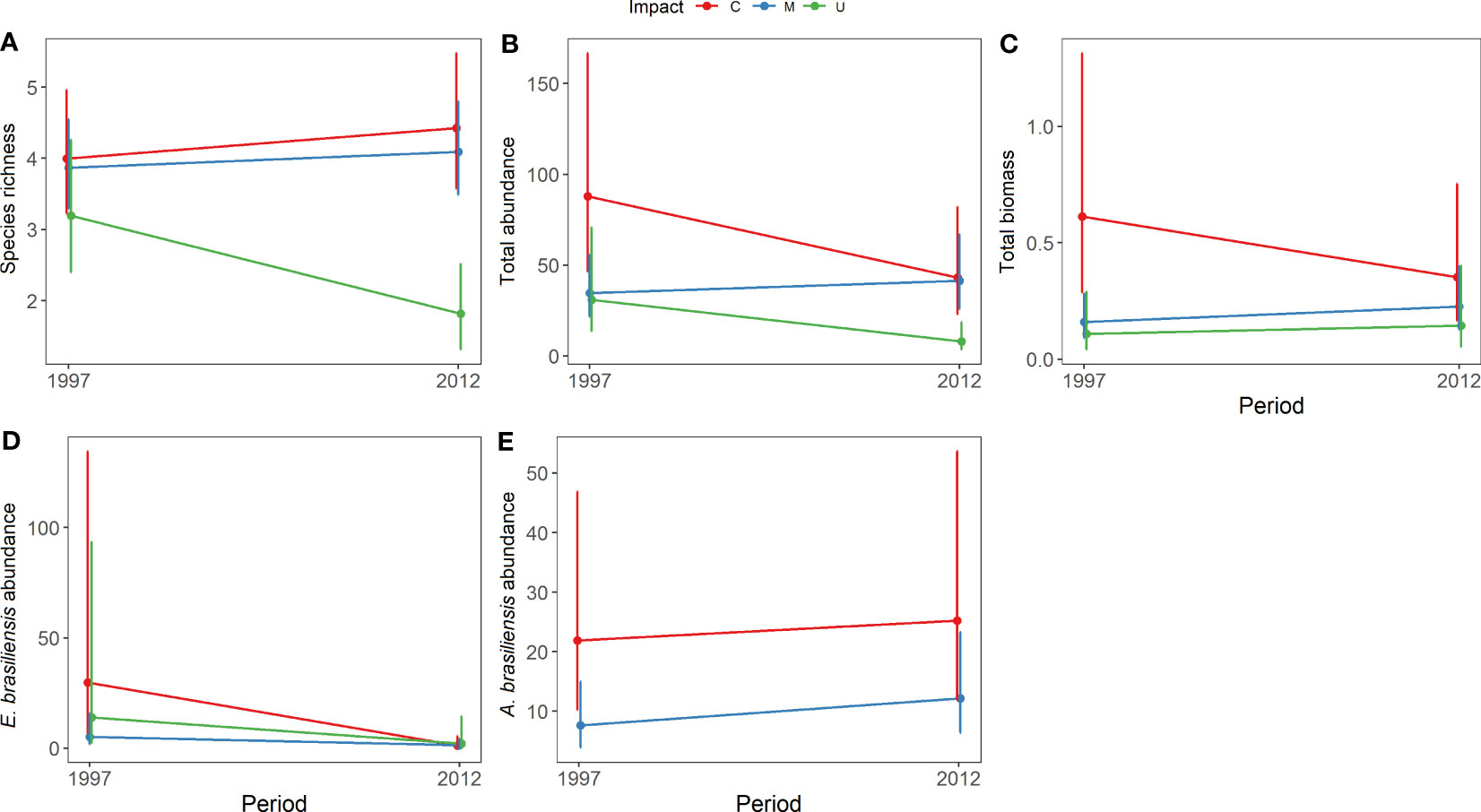
Figure 2 Model prediction for the mean (± CI) between urbanization degree and periods for: (A) species richness, (B) total abundance, (C) total biomass, (D) abundance of Emerita brasiliensis, and (E) abundance of Atlantorchestoidea brasiliensis.
Conserved beaches (C) showed increased richness and decreased total abundance, biomass, and abundance of E. brasiliensis between the two periods (Figure 2). This group had the highest values for all variables in both the first period (1997/1998) and the second period (2012) compared to the other groups (M and U). On conserved beaches, the dominant species were E. brasiliensis in 1997 and A. brasiliensis in 2012. The density of the isopod Excirolana braziliensis (Richardson, 1912) increased between the two periods and surpassed that of E. brasiliensis in 2012 (Table S6).
Modified beaches (M) exhibited an increase in richness, total abundance, and biomass and a reduction in the abundance of E. brasiliensis. This group presented intermediate values for the variables, except for E. brasiliensis abundance, which was lower than the other two groups (C and U). The species with the highest density in 1997/1998 in this group was Excirolana braziliensis, whereas, in 2012, A. brasiliensis presented the highest density.
In urbanized beaches (U), all variables except for biomass decreased. During both periods, urbanized beaches showed lower richness, total abundance, and biomass than conserved (C) and modified beaches (M). The dominant species were E. brasiliensis and Phaleria testacea (Say, 1824), and the densities of both species were lower in 2012.
The variables in this study showed similar relationships with each predictor variable, except for the relationship between total biomass and UI (Figure 3). The increase in the CI was related to increased species richness and abundance of E. brasiliensis. Four of the response variables also showed an increase with UI, except for total biomass, which decreased with increases in urbanization to a value where it appeared to reach stabilization (UI ≈ 10). The total abundance, biomass, and abundance of A. brasiliensis showed a decrease in RI values greater than six. Except for total biomass, all variables showed a bimodal relationship with beach granulometry and slope, with two maximum peaks for granulometry values equal to 0.4 and 1.0 and slope values equal to 0.08 and 0.14. Biomass values equal to zero were recorded on five beaches that showed no relationship with the covariates evaluated.
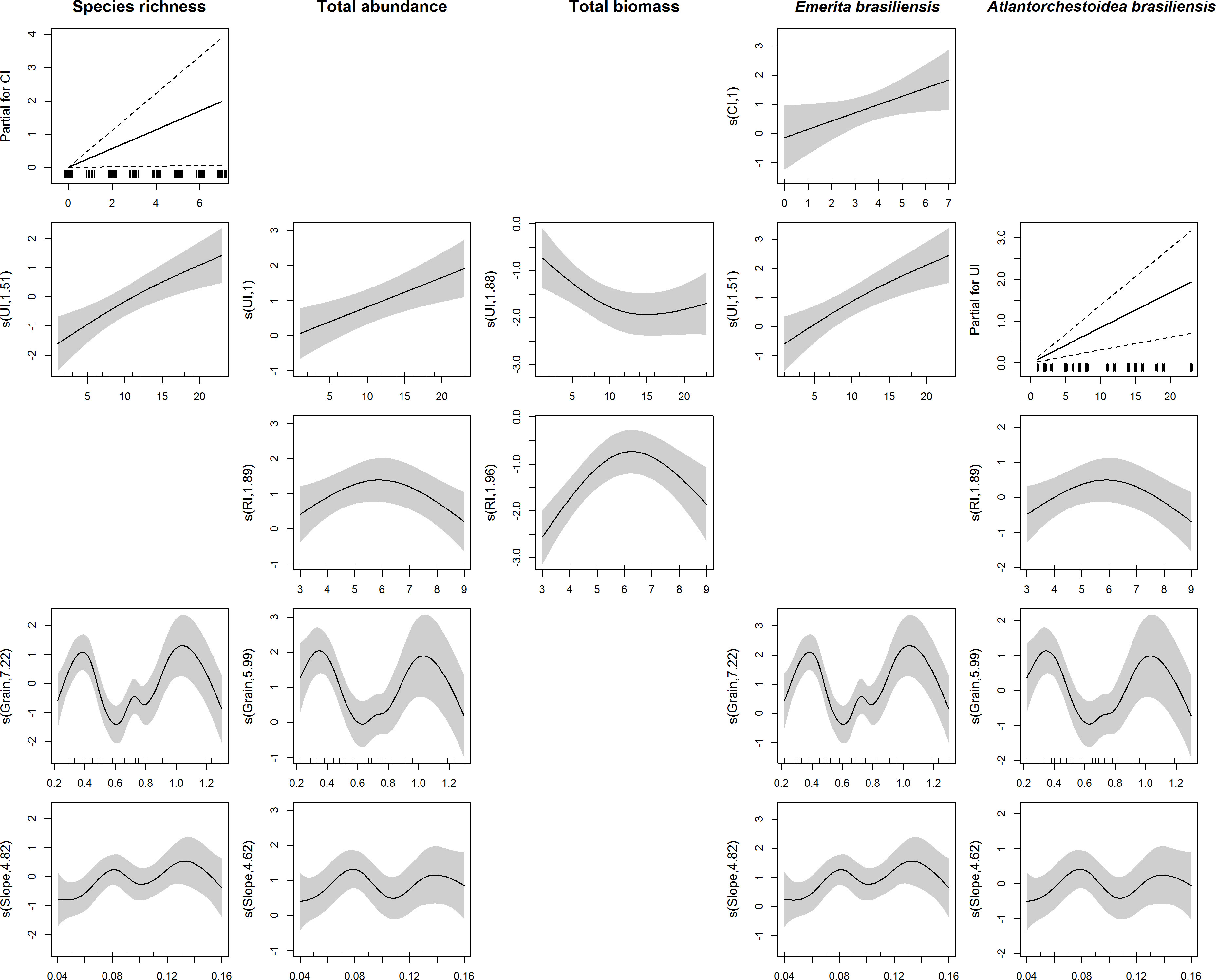
Figure 3 Smoothed curves and linear relations (partial for CI or UI) of the variables species richness, total abundance, total biomass, Emerita brasiliensis abundance, and Atlantorchestoidea brasiliensis abundance, with the predictors conservation index (CI), urbanization index (UI), recreation index (RI), granulometry and beach slope.
Crustaceans were the most abundant group in both periods, with the hippid decapod E. brasiliensis being the most representative in 1997 and the talitrid amphipod A. brasiliensis in 2012. E. brasiliensis densities varied from 8547.80 to 361.63 ind/m² between the periods, while A. brasiliensis varied from 460.30 to 957.98 ind/m². The cirolanid isopod Excirolana braziliensis was the third (1997/1998) and second (2012) most abundant species, increasing from 398.50 to 600.91 ind/m². The bivalve Donax hanleyanus (MolluscaBase, 2023) was the most common and abundant mollusk in both periods, with densities ranging from 74.00 to 78.36 ind/m². Among polychaetes, the spionid genus Dispio (Hartman, 1951) showed higher density (132.80 ind/m²) in 1997/1998, despite occurring in only two sites. Hemipodia californiensis (Hartman, 1951) is a widely distributed polychaete, and its densities varied from 52.20 to 23.90 ind/m² between the periods. In 2012, Orbinia riseri (Pettibone, 1957) was the most abundant, with 46.42 ind/m². The only sampled insect species was the tenebrionid beetle P. testacea, which ranged from 124.20 to 53.08 ind/m² between the periods and occurred in almost all sampled beaches. A complete list of species densities is shown in Table S6.
4 Discussion
The results partially support the main hypothesis since there were different responses from biological variables between each urbanization category (C, M, and U). The data supported that community structure descriptors (species richness, total abundance, and biomass) decreased as urbanization increased from conserved to urbanized beaches. Between the periods, there was a reduction of community structure descriptors and abundance of E. brasiliensis. Despite the study covering an extensive spatial area, there was a lack of temporal replication to conclude that recorded temporal variations were solely due to increases in local urbanization. While there was a decline in E. brasiliensis abundance in all categories comparing the first and second periods, the abundance of A. brasiliensis responded to urbanization spatially, increasing from more to less urbanized categories. The observed patterns, where urbanized beaches presented lower values for all community structure descriptors and non-occurrence of A. brasiliensis, while conserved beaches presented the highest values in the two different periods, show evidence of urbanization effects on sandy beach ecosystems. However, caution should be exercised when interpreting the effects of local urbanization on temporal variations.
It was expected that conserved beaches (C) would act as a control group, but instead, most biological variables decreased except for species richness. Although local urbanization pressures and recreational activities are considerably lower in this group, they are still susceptible to impacts, such as those derived from marine pollution. But they still maintained higher values for community descriptors, and populations of E. brasiliensis and A. brasiliensis were more abundant compared to other groups (M and U). Many coastal habitats around the study area are eutrophic and are daily impacted by domestic and industrial sewage disposal (Fistarol et al., 2015; Castelo et al., 2021). Chemical pollutants are also present, and there is a report of metal bioaccumulation by macrofauna of sandy beaches in the study area (Cabrini et al., 2017). Solid waste, including microplastics, is a frequent issue on beaches (Carvalho and Baptista-Neto, 2016; Iannilli et al., 2018; da Silva et al., 2022). Accessibility is a major driver of the volume and type of solid waste accumulation on beaches, but even those with restricted access can present high densities (Abude et al., 2021). Trampling is a major impact on benthic fauna, especially in recreational areas with high numbers of users (Schlacher et al., 2012; Machado et al., 2017), and is lower in conserved beaches due to access restrictions in military areas and physical limitations of protected areas. Manual cleaning is performed on most conserved beaches and is less harmful to benthic fauna than mechanized cleaning (Zielinski et al., 2019).
The modified beaches (M) seem to present resilience to the estimated pressures as they have intermediate levels of disturbance and are recently urbanized habitats. Some beaches in this group were included inside new no-take protected areas (Category II of IUCN) between the periods, which contributed to reducing the pressures of local urbanization, especially by protecting dunes and vegetation (Rio de Janeiro, 2011). All community descriptors increased in this group between the periods, and only the abundance of E. brasiliensis declined. The peracarid crustaceans A. brasiliensis and Excirolana braziliensis increased between years, contributing to increased community descriptors (total abundance and biomass). High abundances of A. brasiliensis could be attributed to the conserved vegetation in some of these beaches since beaches that present shoreline armoring has a lower abundance of talitrids (Sobocinski et al., 2010; Dugan et al., 2008). Increases in A. brasiliensis demographic parameters, such as density, size, and biomass, are all correlated with beach vegetal availability (Weber et al., 2019). Also, visitation in these habitats is not as constant as in urbanized beaches and tends to increase only on weekends and holidays. Talitrids are widely used as indicators of human trampling, but their populations can recover after periods of reduced activities (Ugolini et al., 2008; Veloso et al., 2008, Veloso et al., 2009). Compared to other crustaceans, Excirolana braziliensis is more tolerant to environmental stress and remains abundant in moderately urbanized habitats (Veloso et al., 2011; Suciu et al., 2018).
The urbanized beaches (U) are the most impacted and least conserved group, and community descriptors and bioindicators present the lowest values in both periods. They are in the most densely populated region of the capital and have a long history of urbanization and recreation impacting these sandy beach habitats (Cardoso et al., 2016). Shoreline modifications on these beaches started around the 1920s, and beach-going by locals has been a cultural habit ever since (Barickman, 2014). Beach nourishment, a widely adopted engineering intervention to extend beach width, was already performed in Copacabana and Ipanema beaches around the 70s and 80s and causes ecological damage to beach fauna (Schlacher et al., 2012; de Schipper et al., 2021). Anthropogenic stressors are highly associated with human activity intensity, as shown by Covid-19 anthropause (Soto et al., 2021; Ben-Haddad et al., 2022; Costa et al., 2022; Neves et al., 2022), and those beaches are daily used by locals and tourists for recreational purposes.
The absence of A. brasiliensis in urbanized beaches (U) in both periods is noteworthy, as talitrids are considered reliable indicators of urbanization, particularly trampling and mechanized cleaning (Cardoso et al., 2016; Costa et al., 2020). Other local urbanization factors, such as artificial lighting and coastal armoring, reportedly have a more detrimental effect on supralittoral organisms like sandhoppers (Devon-Lynn et al., 2021; Jaramillo et al., 2021). Deposited biological material from the sea and land is a determinant factor in maintaining populations of supralittoral detritivores and is removed by beach cleaning (Hyndes et al., 2022), which is mechanized and frequent in urbanized beaches. The non-occurrence of talitrids has been previously reported in highly impacted environments along an urbanization gradient (Fanini et al., 2005; Veloso et al., 2006; Veloso et al., 2008; Cardoso et al., 2016). It is important to consider the sampling design when using sandhoppers for impact assessments (Costa and Zalmon, 2019a) and a more consistent species-oriented sampling design is needed to confirm local extirpation. However, the long-term high levels of urbanization probably affected A. brasiliensis’ capacity to sustain resident populations on urbanized beaches.
The abundance and occurrence of Emerita brasiliensis declined in all beach categories (C, M, and U). It is hypothesized that mole crab populations are defined by source-sink dynamics, in which some beaches act as a “source” of larval supply and others as “sinks”, receiving more individuals that it generates, and that coastal development could disrupt metapopulation dynamics by impacting populations of “source” beaches (Celentano et al., 2009; Costa et al., 2022). An overall decline in E. brasiliensis abundance, even in conserved beaches, could be related to this conjecture. Despite the widely studied benthic phase of the species, the larval dynamics are poorly understood. E. brasiliensis exhibits great phenotypic plasticity and can inhabit beaches with contrasting morphodynamics in high densities (Defeo et al., 2001; Defeo and Cardoso, 2002). They may present fluctuations due to recruitment periods, but reproduction in subtropical environments is continuous, and peaks are registered in warmer seasons (Veloso and Cardoso, 1999; Cardoso et al., 2003; Petracco et al., 2003). Mole crabs are filter feeders living in the swash zone and could be more susceptible to impacts beyond direct human pressure than supralittoral species. This genus plays an important role as a bioindicator of chemical and organic pollution on sandy beaches. Mole crabs are negatively affected by urban sewage effluents (Boere et al., 2011), accumulate high concentrations of algal toxins (Ferdin et al., 2002; Powell et al., 2002), and heavy metals (Cabrini et al., 2017). The Emerita genus (Stimpson 1857) can also accumulate microplastics (Horn et al., 2019). The response of E. brasiliensis could be derived not only from local pressures from recreation and urbanization but also from large-scale factors (e.g., marine pollution) that were not captured by the indices.
The indices (CI, UI, and RI) are efficient tools for rapidly estimating local human modification parameters on beaches and can assist policymakers in prioritizing the use of space and decision-making (McLachlan et al., 2013; González and Holtmann-Ahumada, 2017). The indices provided a scenario of increasing urban development around sandy beaches in the study area, where most sites changed from conserved to urbanized status, classified here as “modified.” Various studies have found relationships between the indices and ecological data when integrated into sandy beach ecology. For example, Cardoso et al. (2016) showed that E. brasiliensis and A. brasiliensis abundances can be predicted by the conservation and urbanization indices and are positively associated with conservation and negatively associated with urbanization. Morphological traits like fluctuating asymmetry of both species seem to be related to conservation levels estimated by the CI, as beaches with the highest conservation levels showed low asymmetry in Frota et al. (2019). Regarding other sandy beach species, the high levels of UI are related to a lower abundance of beetles like tenebrionid Phaleria maculata (Kulzer, 1959) on the Chilean coast (González et al., 2014) and cicindelid Cylindera nivea (Kirby, 1818) (Costa and Zalmon, 2019b). Laitano et al. (2019) applied the UI and found that beach urbanization negatively affected the abundance of recruits and juveniles of clam Amarilladesma mactroides (Reeve, 1854). Despite their efficiency, these indices only assess local factors, and other metrics need to be integrated into sandy beach impact evaluations.
Sandy beaches are not closed systems; several impacts that may originate in the ocean or by large-scale urban modification influence this ecosystem, and therefore, other unmeasured impacts may have influenced the results. Given the age of the ecological data from the 90s, complementary information such as the physical and chemical properties of water was not found in the available databases. Therefore, it was not possible to make inferences about the effects of climate change on the study area, such as increases in sea surface temperature, increases in the frequency of extreme climatic events, or changes in marine current patterns. Populations distributions can be affected by warmer waters, as the tropicalization phenomenon registered in Uruguayan beach communities, although its effects are still briefly studied (Defeo, 2003; Schoeman et al., 2014). The Brazil Current is intensifying and shifting southwards during the past decades in response to changes in near-surface wind patterns, leading to intense ocean warming along its path (Franco et al., 2020).
Assessing anthropogenic impacts via suitable indicators is a major topic within sandy beach research (Fanini et al., 2020) and may serve as useful resources for managers. However, research on conservation and management practices is less prevalent (Nel et al., 2014). Lercari (2023) highlights that sandy beaches still do not receive the same scientific attention as other coastal environments; however, scientific studies are growing moderately, and human impacts derived from urbanization are a primary research field. Nevertheless, few studies address the temporal dimension (medium or long-term) in sandy beach habitats, and even fewer include cumulative impacts from urbanization on communities. Although the present study is not a long or medium-term assessment to comprehend a temporal trend, and the aim is to compare two distinct points in time, even evaluations like this are scarce. Bessa et al. (2014) suggested that the talitrid amphipod Talitrus saltator (Montagu, 1808) and isopod Tylos europaeus (Arcangeli, 1938) were potential indicators of medium-term human pressures on the Portugal coast. In southern California (USA), a historical data survey showed that intertidal isopods Tylos punctatus (Holmes & Gay, 1909) and Alloniscus perconvexus (Dana, 1853) were extirpated from numerous locations, and populations persist primarily on non-urbanized sites (Hubbard et al., 2014). Also, on the Californian coast, local-scale urbanization processes were the primary drivers of change in beach ecosystems, and wrack-associated species richness declined over time at impacted beaches (e.g., beach nourishment and grooming) (Schooler et al., 2017). This shows that sandy beach populations can be disrupted by urbanization over time and corroborates the absence of A. brasiliensis in urbanized beaches in both periods of the current study. A. brasiliensis can be a potential indicator of long-term local urban pressures on sandy beach habitats.
Different community and population responses must be considered when assessing the impacts and challenges of conservation policymaking. Marine connectivity is often disregarded when creating and managing protected areas (Balbar and Metaxas, 2019). Currently, the scenario in Rio de Janeiro consists of a few pristine beaches inside protected areas with good levels of conservation (Categories I and II of the IUCN) and large areas with high urbanization and recreation. Many studied beaches have been classified as protected areas since 1997; however, many are of sustainable use and lack management plans, although Brazilian legislation determines that they must be elaborated within five years from the date of the creation of the protected area (BRASIL, 2000). Often, beaches inside protected areas are attractive for their scenic beauty and are unmanaged for purposes beyond recreation and tourism. Even beaches in the urbanized category (U) are protected areas for sustainable use (RIO DE JANEIRO, 1988), despite not conserving their biodiversity. The conservation target of 10% for coastal and marine systems proposed by the Convention on Biological Diversity can be considered low for conserving beaches and their biota, and coastal reserve networks do not necessarily confer conservation benefits to sandy beaches because the LAZ can often be neglected (Harris et al., 2014a; Harris et al., 2014b). This scenario repeats itself on many coasts subject to increasing urbanization, use of resources, and climate change effects (Defeo and Elliott, 2021). The status of sandy beaches in Rio de Janeiro reflects others worldwide: poor management, increasing urbanization, and impacted biodiversity.
5 Conclusions
Our results support that local urbanization impacts sandy beach ecosystems over time, and long-term monitoring is necessary to distinguish community and population responses better. The decline of E. brasiliensis was not entirely explained by local urbanization pressures, as there are other factors, natural or human-induced, that could be influencing the abundance of this species. Thus, species with direct development, such as A. brasiliensis, stand out as potential indicators of changes caused by local urbanization on sandy beaches. Simple metrics such as indices are low-cost tools for quick assessments of impacts and are easily integrated into ecological information. However, they do not preclude the need for more robust surveys that provide a holistic picture of the changes caused by urbanization. Urbanization is a complex phenomenon that alters coastal systems, with many consequences that are still poorly understood. There are possibilities for conservation in Rio de Janeiro since there are still preserved beaches with low levels of human intervention. Managing these ecosystems requires an effective protected area network and not only a few conserved beaches in remote areas of the state. It remains to be seen whether biodiversity will endure until conservation measures are efficiently implemented.
Data availability statement
The original contributions presented in the study are included in the article/Supplementary Material. Further inquiries can be directed to the corresponding author.
Author contributions
MA: Data curation, Investigation, Formal analysis, Visualization, Writing – original draft. RA: Data curation, Investigation, Visualization, Writing – review & editing. RC: Conceptualization, Resources, Project administration, Validation. TC: Investigation, Supervision, Project administration, Formal analysis, Writing – review & editing, Validation. All authors contributed to the article and approved the submitted version.
Funding
MA was supported by Federal University of the State of Rio de Janeiro (UNIRIO) graduate research scholarships. RA was supported by Coordination for the Improvement of Higher Education Personnel (CAPES) post-graduate research scholarships. RC was supported by Foundation Carlos Chagas Filho Research Support of the State of Rio de Janeiro – FAPERJ (E-26/110.013/2011) and Brazilian National Council for Scientific and Technological Development (CNPq). TC was supported by FAPERJ (E-26/010.001576/2019).
Acknowledgments
The authors express their deepest gratitude to all fieldwork participants. We would like to thank Dra. Kátia Regina Góes Souza for developing the thematic maps (Figures S1 and S2). We also would like to thank Editage [http://www.editage.com] for editing and reviewing this manuscript for English language.
Conflict of interest
The authors declare that the research was conducted in the absence of any commercial or financial relationships that could be construed as a potential conflict of interest.
Publisher’s note
All claims expressed in this article are solely those of the authors and do not necessarily represent those of their affiliated organizations, or those of the publisher, the editors and the reviewers. Any product that may be evaluated in this article, or claim that may be made by its manufacturer, is not guaranteed or endorsed by the publisher.
Supplementary material
The Supplementary Material for this article can be found online at: https://www.frontiersin.org/articles/10.3389/fmars.2023.1158413/full#supplementary-material
References
Abude R. R. S., Augusto M., Cardoso R. S., Cabrini T. M. B. (2021). Spatiotemporal variability of solid waste on sandy beaches with different access restrictions. Mar. pollut. Bull. 171, 112743. doi: 10.1016/j.marpolbul.2021.112743
Afghan A., Cerrano C., Luzi G., Calcinai B., Puce S., Mantas T. P., et al. (2020). Main anthropogenic impacts on benthic macrofauna of sandy beaches: a review. J. Mar. Sci. Eng. 8 (6), 405. doi: 10.3390/jmse8060405
Baird R. C. (2009). Coastal urbanization: the challenge of management lag. Manage. Environ. Qual. Int. J. 20, 371–382. doi: 10.1108/14777830910963726
Balbar A. C., Metaxas A. (2019). The current application of ecological connectivity in the design of marine protected areas. Glob. Ecol. Conserv. 17, e00569. doi: 10.1016/j.gecco.2019.e00569
Barbier E. B., Hacker S. D., Kennedy C., Koch E. W., Stier A. C., Silliman B. R. (2011). The value of estuarine and coastal ecosystem services. Ecol. Monogr. 81 (2), 169–193. doi: 10.1890/10-1510.1
Barboza C. A., de M., Mattos G., Soares-Gomes A., Zalmon I. R., Costa L. L. (2021). Low densities of the ghost crab Ocypode quadrata is related to large scale human modification of sandy shores. Front. Mar. Sci. 8. doi: 10.3389/fmars.2021.589542
Barickman B. J. (2014). Not many flew down to Rio: tourism and the history of beach-going in twentieth-century Rio de Janeiro. J. Tour. Hist. 6, 223–241. doi: 10.1080/1755182X.2014.969788
Becchi C., Ortolani I., Muir A. I., Cannicci S. (2014). The effect of breakwaters on the structure of marine soft-bottom assemblages: a case study from a north-Western Mediterranean basin. Mar. pollut. Bull. 87, 131–139. doi: 10.1016/j.marpolbul.2014.08.002
Ben-Haddad M., Hajji S., Abelouah M. R., Costa L. L. L., Rangel-Buitrago N., Alla A. A. (2022). Has the “Covid-19” lockdown an impact on beach faunal communities? the central Atlantic coast of morocco as a case study. Mar. pollut. Bull. 185, 114259. doi: 10.1016/j.marpolbul.2022.114259
Bessa F., Gonçalves S. C., Franco J. N., André J. N., Cunha P. P., Marques J. C. (2014). Temporal changes in macrofauna as response indicator to potential human pressures on sandy beaches. Ecol. Indic. 41, 49–57. doi: 10.1016/j.ecolind.2014.01.023
Blott S. J., Pye K. (2001). GRADISTAT: a grain size distribution and statistics package for the analysis of unconsolidated sediments. Earth Surface Processes Landforms 26, 1237–1248. doi: 10.1002/esp.261
Boere V., Cansi E. R., Alvarenga A. B. B., Silva I. O. (2011). The burying behavior of the mole crabs before and after an accident with urban sewage efluents in bombinhas beach, Santa catarina, Brazil. AmbiAgua Taubaté. 6 (3), 70–76. doi: 10.4136/ambi-agua.627
Boyko C. B., Bruce N. L., Hadfield K. A., Merrin K. L., Ota Y., Poore G. C. B., Taiti ,. S. (Eds.) (2008 onwards). “World marine, freshwater and terrestrial isopod crustaceans database,” in Tylos europaeus Arcangeli 1938. Available at: https://www.marinespecies.org/aphia.php?p=taxdetails&id=156476.
Brandão Z. (2006). Urban planning in Rio de Janeiro: a critical review of the urban design practice in the twentieth century. City Time 2, 37–50. Available at: http://www.ct.ceci-br.org.
Brasil (2000). Law n°9985, July 18, 2000. SNUC - Sistema Nacional de Unidades de Conservação. Accessed on 06/28/2023. Available at: https://www.planalto.gov.br/ccivil_03/leis/l9985.htm.
Bretz C. K., Manouki T. J., Kvitek R. G. (2002). Emerita analoga (Stimpson) as an indicator species for paralytic shellfish poisoning toxicity along the California coast. Toxicon 40, 1189–1196. doi: 10.1016/S0041-0101(02)00127-7
Brooks M. E., Kristensen K., Benthem K. J.v., Magnusson A., Berg C. W., Nielsen A., et al. (2017). glmmTMB Balances Speed and Flexibility Among Packages for Zero-inflated Generalized Linear Mixed Modeling. The R Journal 9 (2), 378–400. doi: 10.32614/RJ-2017-066
Cabral L. N., Cândido G. A. (2019). Urbanization, vulnerability, resilience: conceptual relations and understanding of cause and effect. Rev. Bras. Gest. Urbana 11, e20180063. doi: 10.1590/2175-3369.011.002.AO08
Cabrini T. M. B., Barboza C. A. M., Skinner V. B., Hauser-Davis R. A., Rocha R. C., Saint’Pierre T. D., et al. (2017). Heavy metal contamination in sandy beach macrofauna communities from the Rio de Janeiro coast, southeastern Brazil. Environ. pollut. 221, 116–129. doi: 10.1016/j.envpol.2016.11.053
Cardoso R. S., Barboza C. A. M., Skinner V. B., Cabrini T. M. B. (2016). Crustaceans as ecological indicators of metropolitan sandy beaches health. Ecol. Indic 62, 154–162. doi: 10.1016/j.ecolind.2015.11.039
Cardoso R., Veloso V. G., Caetano C. H. S. (2003). Life history of Emerita brasiliensis (Decapoda: hippidae) on two beaches with different morphodynamic characteristics. J. Coast. Res. 35, 392–401.
Carvalho D. G., Baptista Neto J. A. (2016). Microplastic pollution of the beaches of guanabara bay, southeast Brazil. Ocean Coast. Manage. 128, 10–17. doi: 10.1016/j.ocecoaman.2016.04.009
Castelo W. F. L., Martins M. V. A., de Lima Ferreira P. A., Figueira R., da Costa C. F., da Fonseca L. B., et al. (2021). Long-term eutrophication and contamination of the central area of sepetiba bay (SW Brazil). Environ. Monit. Assess. 193, 100. doi: 10.1007/s10661-021-08861-1
Celentano E., Gutiérrez N. L., Defeo O. (2009). Effects of morphodynamic and estuarine gradients on the demography and distribution of a sandy beach mole crab: implications for source-sink habitat dynamics. Mar. Ecol. Prog. Ser. 398, 193–205. doi: 10.3354/meps08342
Climate and Clean Air Coalition (2014) Climate and clean air coalition annual report: September 2013 - august 2014. 1-44. Available at: https://www.ccacoalition.org/en/resources/climate-and-clean-air-coalition-ccac-annual-report-2013-2014.
COMLURB (2015) Companhia municipal de limpeza Urbana. Available at: http://www.rio.rj.gov.br/web/comlurb.
Costa L. L., Fanini L., Zalmon I. R., Defeo O., McLachlan A. (2022). Cumulative stressors impact macrofauna differentially according to sandy beach type: a meta-analysis. J. Environ. Manage 307, 114594. doi: 10.1016/j.jenvman.2022.114594
Costa L. L., Rosental I., Fanini L., Defeo O. (2020). Macroinvertebrates as indicators of human disturbances on sandy beaches : a global review. Ecol. Indic. 118, 106764. doi: 10.1016/j.ecolind.2020.106764
Costa L. L., Tavares D. C., Suciu M. C., Rangel D. F., Zalmon I. R. (2017). Human-induced changes in the trophic functioning of sandy beaches. Ecol. Indic. 82, 304–315. doi: 10.1016/j.ecolind.2017.07.016
Costa L. L., Zalmon I. R. (2019a). Issues to consider when sampling the sandhopper atlantorchestoidea brasiliensis (Dana 1853) for impact assessments on sandy beaches. Mar. Biol. Res. 15, 451–457. doi: 10.1080/17451000.2019.1680848
Costa L. L., Zalmon I. R. (2019b). Sensitivity of macroinvertebrates to human impacts on sandy beaches: a case study with tiger beetles (Insecta, cicindelidae). Estuar. Coast. Shelf Sci. 220, 142–151. doi: 10.1016/j.ecss.2019.02.017
Dana J. D. (1853). “Crustacea. Part II,” in United States Exploring Expedition. During the Years 1838, 1839, 1840, 1841, 1842. Under the Command of Charles Wilkes, U.S.N. 14:691-161. Available at: https://www.biodiversitylibrary.org/page/40412910.
da Silva E. F., do Carmo D., de F., Muniz M. C., dos Santos C. A., Cardozo B. B. I., et al. (2022). Evaluation of microplastic and marine debris on the beaches of niterói oceanic region, Rio de Janeiro, Brazil. Mar. pollut. Bull. 175, 113161. doi: 10.1016/j.marpolbul.2021.113161
Defeo O. (2003). Marine invertebrate fisheries in sandy beaches: an overview. J. Coast. Res. 35, 56–65.
Defeo O., Cardoso R. S. (2002). Macroecology of population dynamics and life history traits of the mole crab emerita brasiliensis in Atlantic sandy beaches of south America. Mar. Ecol. Prog. Ser. 239, 169–179. doi: 10.3354/meps239169
Defeo O., Elliott M. (2021). The ‘triple whammy’ of coasts under threat – why we should be worried! Mar. pollut. Bull. 163, 111832. doi: 10.1016/j.marpolbul.2020.111832
Defeo O., Gomez J., Lercari D. (2001). Testing the swash exclusion hypothesis in sandy beach populations: the mole crab emerita brasi- liensis in uruguay. Mar. Ecol. Prog. Ser. 212, 159–170. doi: 10.3354/MEPS212159
Defeo O., McLachlan A. (2005). Patterns, processes, and regulatory mechanisms in sandy beach macrofauna: a multi–scale analysis. Mar. Ecol. Prog. Ser. 295, 1–20. doi: 10.3354/meps295001
Defeo O., McLachlan A., Armitage D., Elliott M., Pittman J. (2021). Sandy beach social–ecological systems at risk: regime shifts, collapses, and governance challenges. Front. Ecol. Environ. 19, 564–573. doi: 10.1002/fee.2406
Defeo O., Mclachlan A., Schoeman D. S., Schlacher T. A., Dugan J., Jones A., et al. (2009). Threats to sandy beach ecosystems : a review. Estuar. Coast. Shelf Sci. 81, 1–12. doi: 10.1016/j.ecss.2008.09.022
Defeo O., Rueda M. (2002). Spatial structure, sampling design and abundance estimates in sandy beach macroinfauna: some warnings and new perspectives. Mar. Biol. 140, 1215–1225. doi: 10.1007/s00227-002-0783-z
de Schipper M. A., Ludka B. C., Raubenheimer B., Luijendijk A. P., Schlacher T. A. (2021). Beach nourishment has complex implications for the future of sandy shores. Nat. Rev. Earth Environ. 2, 70–84. doi: 10.1038/s43017-020-00109-9
de Souza A. M., Rocha D. S., Guerra J. V., Cunha B. A., Martins M. V. A., Geraldes M. C. (2021). Metal concentrations in marine sediments of the Rio de Janeiro coast (Brazil): a proposal to establish new acceptable levels of contamination. Mar. pollut. Bull. 165, 112113. doi: 10.1016/j.marpolbul.2021.112113
Devon-Lynn K., Quintanilla-Ahumada D., Anguita C., Widdicombe S., Pulgar J., Manríquez P. H., et al. (2021). Artificial light at night alters the activity and feeding behaviour of sandy beach amphipods and pose a threat to their ecological role in Atlantic Canada. Sci. Total Environ. 780, 146568. doi: 10.1016/j.scitotenv.2021.146568
Donohoe R. M., Duke B. M., Clark S. L., Joab B. M., Dugan J. E., Hubbard D. M., et al. (2021). Toxicity of Refugio beach oil to sand crabs (Emerita analoga), blue mussels (Mytilus sp.), and inland silversides (Menidia beryllina). Environ. Toxicol. Chem. 40, 2578–2586. doi: 10.1002/etc.5148
Dugan J. E., Hubbard D. M., Rodil I. F., Revell D. L., Schroeter S. (2008). Ecological effects of coastal armoring on sandy beaches. Mar. Ecol. 29, 160e170. doi: 10.1111/j.1439-0485.2008.00231.x
Dunn K. P., Smyth G. K. (1996). Randomized quantile residuals. J. Comput. Graphical Stat 5, 1–10. doi: 10.1080/10618600.1996.10474708
Emery K. O. (1961). A simple method of measuring beach profiles. Limnol. Oceanogr. 6, 90–93. doi: 10.4319/lo.1961.6.1.0090
Fanini L., Cantarino C. M., Scapini F. (2005). Relationships between the dynamics of two talitrus saltator populations and the impacts of activities linked to tourism. Oceanologia 47, 93–112.
Fanini L., Defeo O., Elliott M. (2020). Advances in sandy beach research – local and global perspectives. Estuar. Coast. Shelf Sci. 234, 106646. doi: 10.1016/j.ecss.2020.106646
Ferdin M. E., Kvitek R. G., Bretz C. K., Powell C. L., Doucette G. J., Lefebvre K. A., et al. (2002). Emerita analoga (Stimpson) - possible new indicator species for the phycotoxin domoic acid in California coastal waters. Toxicon 40, 1259–1265. doi: 10.1016/S0041-0101(02)00129-0
Fistarol G. O., Coutinho F. H., Moreira A. P. B., Venas T., Cánovas A., de Paula S. E. M. Jr., et al. (2015). Environmental and sanitary conditions of guanabara bay, rio de janeiro. Front. Microbiol. 6, 1232. doi: 10.3389/fmicb.2015.0120.3389/fmicb.2015.012
Folk R. L., Ward W. C. (1957). Brazos river bar, a study in significance of grain size parameters. J. Sedimentary Petrol. 27, 3–26. doi: 10.1306/74D70646-2B21-11D7-8648000102C1865D
Fox J., Weisberg S. (2019). An r companion to applied regression, third edition (Thousand Oaks CA: Sage). Available at: https://socialsciences.mcmaster.ca/jfox/Books/Companion/.
Franco B. C., Defeo O., Piola A. R., Barreiro M., Yang H., Ortega L., et al. (2020). Climate change impacts on the atmospheric circulation, ocean, and fisheries in the southwest south Atlantic ocean: a review. Clim. Change 162, 2359–2377. doi: 10.1007/s10584-020-02783-6
Frota G. P., Cabrini T. M. B., Cardoso R. S. (2019). Fluctuating asymmetry of two crustacean species on fourteen sandy beaches of Rio de Janeiro state. Estuar. Coast. Shelf Sci. 223, 138–146. doi: 10.1016/j.ecss.2019.03.013
Fujisaki I., Lamont M. M. (2016). The effects of large beach debris on nesting sea turtles. J. Exp. Mar. Bio. Ecol. 482, 33–37. doi: 10.1016/j.jembe.2016.04.005
Gelman A., Hill J. (2006). Data analysis using regression and multilevel/hierarchical models (Cambridge University Press).
González S. A., Holtmann-Ahumada G. (2017). Quality of tourist beaches of northern Chile: a first approach for ecosystem-based management. Ocean Coast. Manage. 137, 154–164. doi: 10.1016/j.ocecoaman.2016.12.022
González S. A., Yáñez-Navea K., Muñoz M. (2014). Effect of coastal urbanization on sandy beach coleoptera phaleria maculata (Kulzer 1959) in northern Chile. Mar. pollut. Bull. 83, 265–274. doi: 10.1016/j.marpolbul.2014.03.042
Harris L., Campbell E. E., Nel R., Schoeman D. (2014a). Rich diversity, strong endemism, but poor protection: addressing the neglect of sandy beach ecosystems in coastal conservation planning. Divers. Distrib. 20, 1120–1135. doi: 10.1111/ddi.12226
Harris L., Nel R., Holness S., Sink K., Schoeman D. (2014b). Setting conservation targets for sandy beach ecosystems. Estuar. Coast. Shelf Sci. 150, 45–57. doi: 10.1016/j.ecss.2013.05.016
Hartig F. (2016). DHARMa: residual diagnostics for hierarchical (multi-level/mixed) regression models (R package version 0.1. 0. CRAN / GitHub). Accessed on 30/06/2023. Available at: https://cran.r-project.org/web/packages/DHARMa/vignettes/DHARMa.html.
Hartman O. (1951). The littoral marine annelids of the gulf of Mexico (Port Aransas, Texas: Publications of the Institute of Marine Science), 7–124.
Heiberger R. M., Holland B. (2004). Statistical analysis and data display: an intermediate course with examples in s-plus, r, and SAS, first edition (Springer Texts in Statistics. Springer). doi: 10.1007/978-1-4757-4284-8
Holmes S., Gay M. E. (1909). Four new species of isopods from the coast of california. Proc. United States Natl. Museum 36 (1670), 375–379.
Horn D., Miller M., Anderson S., Steele C. (2019). Microplastics are ubiquitous on California beaches and enter the coastal food web through consumption by pacific mole crabs. Mar. pollut. Bull. 139, 231–237. doi: 10.1016/j.marpolbul.2018.12.039
Hubbard D. M., Dugan J. E., Schooler N. K., Viola S. M. (2014). Local extirpations and regional declines of endemic upper beach invertebrates in southern California. Estuar. Coast. Shelf Sci. 150, 67–75. doi: 10.1016/j.ecss.2013.06.017
Hyndes G. A., Berdan E. L., Duarte C., Dugan J. E., Emery K. A., Hambäck P. A., et al. (2022). The role of inputs of marine wrack and carrion in sandy-beach ecosystems: a global review. Biol. Rev. 000–000. doi: 10.1111/brv.12886
Iannilli V., Di Gennaro A., Lecce F., Sighicelli M., Falconieri M., Pietrelli L., et al. (2018). Microplastics in talitrus saltator (Crustacea, amphipoda): new evidence of ingestion from natural contexts. Environ. Sci. pollut. Res. Int. 25, 28725–28729. doi: 10.1007/s11356-018-2932-z
IBGE (2010) Cidades. Available at: https://cidades.ibge.gov.br/ (Accessed 15/07/2020).
IBGE (2017) O Recorte das regiões geográficas imediatas e intermediárias de 2017. Available at: https://ia600603.us.archive.org/2/items/RegiesGeogrrficasBrasil2017/Regi%C3%B5es%20geogr%C3%A1ficas_Brasil%202017.pdf (Accessed 10/01/2021).
Instituto Pereira Passos (2013) Data.Rio. Available at: https://www.data.rio/search?groupIds=0f4009068ec74e17b25eb3e70891b95f&sort=–modified (Accessed 15/07/2020).
Jaramillo E., Dugan J., Hubbard D., Manzano M., Duarte C. (2021). Ranking the ecological effects of coastal armoring on mobile macroinvertebrates across intertidal zones on sandy beaches. Sci. Total Environ. 755, 142573. doi: 10.1016/j.scitotenv.2020.142573
Jones A. R., Schlacher T. A., Schoeman D. S., Weston M. A., Withycombe G. M. (2017). Ecological research questions to inform policy and the management of sandy beaches. Ocean Coast. Manage. 148, 158–163. doi: 10.1016/j.ocecoaman.2017.07.020
Jorge-Romero G., Elliott M., Defeo O. (2022). Managing beyond ecosystem limits at the land-sea interface: the case of sandy beaches. Mar. pollut. Bull. 181, 113838. doi: 10.1016/j.marpolbul.2022.113838
Jorge-Romero G., Lercari D., Ortega L., Defeo O. (2019). Long-term ecological footprints of a man-made freshwater discharge onto a sandy beach ecosystem. Ecol. Indic. 96, 412–420. doi: 10.1016/j.ecolind.2018.09.024
Laitano M. V., Chiaradia N. M., Nuñez J. D. (2019). Clam population dynamics as an indicator of beach urbanization impacts. Ecol. Indic. 101, 926–932. doi: 10.1016/j.ecolind.2019.02.006
Laurino I. R. A., Checon H. H., Corte G. N., Turra A. (2022). Does coastal armoring affect biodiversity and its functional composition on sandy beaches? Mar. Envir. Res. 181, 105760. doi: 10.1016/j.marenvres.2022.105760
Legendre P., Legendre L. (2012). Numerical ecology, 3rd english edition (Amsterdam, The Netherlands: Elsevier Science BV).
Lenth R. V. (2016). Least-squares means: the r package lsmeans. J. Stat. Software 69 (1), 1–33. doi: 10.18637/jss.v069.i01
Lercari D. (2023). Sandy beaches: publication features, thematic areas and collaborative networks between 2009 and 2019. Estuar. Coast. Shelf Sci. 281, 108211. doi: 10.1016/j.ecss.2023.108211
Lercari D., Defeo O. (1999). Effects of freshwater discharge in sandy beach populations: the mole crab emerita brasiliensis in Uruguay. Estuar. Coast. Shelf Sci. 49, 457–468. doi: 10.1006/ecss.1999.0521
Lercari D., Defeo O., Ortega L., Orlando L., Gianelli I., Celentano E. (2018).Long-term structural and functional changes driven by climate variability and fishery regimes in a sandy beach ecosystem. Ecol. Model. 368, 41–51. doi: 10.1016/j.ecolmodel.2017.11.007
Luarte T., Bonta C. C., Silva-Rodriguez E. A., Quijón P. A., Miranda C., Farias A. A., et al. (2016). Light pollution reduces activity, food consumption and growth rates in a sandy beach invertebrate. Environ. pollut. 218, 1147–1153. doi: 10.1016/j.envpol.2016.08.068
Lüdecke (2018). Ggeffects: tidy data frames of marginal effects from regression models. J. Open Source Software 3 (26), 772. doi: 10.21105/joss.0077
Luijendijk A., Hagenaars G., Ranasinghe R., Baart F., Donchyts G., Aarninkhof S. (2018). The state of the world’s beaches. Sci. Rep. 8, 6641. doi: 10.1038/s41598-018-24630-6
Luisa Martínez M., Mendoza-González G., Silva-Casarín R., Mendoza-Baldwin E. (2014). Land use changes and sea level rise may induce a “coastal squeeze” on the coasts of veracruz, Mexico. Glob. Environ. Change 29, 180–188. doi: 10.1016/j.gloenvcha.2014.09.009
Machado P. M., Suciu M. C., Costa L. L., Tavares D. C., Zalmon I. R. (2017). Tourism impacts on benthic communities of sandy beaches. Mar. Ecol. 38, 1–11. doi: 10.1111/maec.12440
Martínez M. L., Intralawan A., Vázquez G., Pérez–Maqueo O., Sutton P., Landgrave R. (2007). The coasts of our world: economic and social importance. Ecol. Econ 63, 254–272. doi: 10.1016/j.ecolecon.2006.10.022
McLachlan A., Defeo O., Jaramillo E., Short A. D. (2013). Sandy beach conservation and recreation: guidelines for optimising management strategies for multi-purpose use. Ocean Coast. Manage. 71, 256–268. doi: 10.1016/j.ocecoaman.2012.10.005
Ministério do Turismo (2018) Estudo da demanda turística internacional brasil – 2018. Available at: http://www.dadosefatos.turismo.gov.br/2016–02–04–11–54–03/demanda–tur%C3%ADstica–internacional/ (Accessed 15/07/2020).
MolluscaBase eds. (2023). MolluscaBase. In: Donax hanleyanus R. A. Philippi 1847. Available at: https://www.marinespecies.org/aphia.php?p=taxdetails&id=494710.
Montagu G. (1808). Description of several marine animals found on the south coast of devonshire. Trans. Linn. Soc. London 9, 81–114. Available at: https://www.biodiversitylibrary.org/page/757838.
Nel R., Campbell E. E., Harris L., Hauser L., Schoeman D. S., McLachlan A., et al. (2014). The status of sandy beach science: past trends, progress, and possible futures. Estuar. Coast. Shelf Sci. 150, 1–10. doi: 10.1016/j.ecss.2014.07.016
Neves R. A. F., Seixas J. T. C., Rodrigues N., Santos L. N. (2022). Impacts of the COVID-19 pandemic restrictions on solid waste pollution in the worldwide iconic copacabana beach (Rio de janeiro, brazil). Mar. pollut. Bull. 181, 113865. doi: 10.1016/j.marpolbul.2022.113865
Oksanen J. F., Blanchet G., Kindt R., Legendre P., Minchin P. R., O'Hara R. B., et al. (2016). Vegan: Community Ecology Package. R Package Version 2.3-5. Accessed on 06/30/2023. Available at: http://CRAN.R-project.org/package=vegan.
Olivatto G. P., Martins M. C. T., Montagner C. C., Henry T. B., Carreira R. S. (2019). Microplastic contamination in surface waters in guanabara bay, Rio de Janeiro, Brazil. Mar. pollut. Bull. 139, 157–162. doi: 10.1016/j.marpolbul.2018.12.042
Orlando L., Ortega L., Defeo O. (2020). Urbanization effects on sandy beach macrofauna along an estuarine gradient. Ecol. Indic. 111, 106036. doi: 10.1016/j.ecolind.2019.106036
Peña-Alonso C., García-Romero L., Hernández-Cordero A. I., Hernández-Calvento L. (2019). Beach vegetation as an indicator of human impacts in arid environments: environmental conditions and landscape perception in the canary islands. J. Environ. Manage. 240, 311–320. doi: 10.1016/j.jenvman.2019.03.096
Pérez D. (1999). Mercury levels in mole crabs hippa cubensis, emerita brasiliensis, e. portoricensis, and lepidopa richmondi (Crustacea: decapoda: hippidae) from a sandy beach at Venezuela. Bull. Environ. Contam Toxicol. 63, 320–326. doi: 10.1007/s001289900983
Pessanha R. M. (2015). A ampliação da fronteira de exploração petrolífera no brasil é parte da geopolítica da energia: oportunidades e riscos de inserção global em meio às novas territorialidades regionais e ao desafio da abundância na economia dos royalties no estado do Rio de Janeiro (Espaço e Economia: Revista Brasileira de Geografia Econômica), 6.
Petracco M., Veloso V. G., Cardoso R. S. (2003). Population dynamics and secondary production of emerita brasiliensis (Crustacea: hippidae) at prainha beach, Brazil. Mar. Ecol. 24, 231–245. doi: 10.1046/j.0173-9565.2003.00837.x
Pettibone M. H. (1957). North American genera of the family orbiniidae (Annelida: polychaeta), with descriptions of new species. J. Wash Acad. Sci. 47, 159–167.
Powell C. L., Ferdin M. E., Busman M., Kvitek R. G., Doucette G. J. (2002). Development of a protocol for determination of domoic acid in the sand crab (Emerita analoga): a possible new indicator species. Toxicon 40, 485–492. doi: 10.1016/S0041-0101(01)00236-7
R Core Team (2021). R: a language and environment for statistical computing (Vienna: R Foundation for Statistical Computing). Available at: https://www.R-project.org.
Reeve L. A. (1854). “Monograph of the genus mesodesma,” in Conchologia iconica, or, illustrations of the shells of molluscous animals, vol. 8. (London: L. Reeve & Co.), 1–4. Available at: https://www.biodiversitylibrary.org/page/11120513.
Richardson H. (1912). Descriptions of a new genus of isopod crustaceans, and of two new species from south America. Proc. U S Natl. Mus 43, 201–204. doi: 10.5479/si.00963801.43-1929.201
Rio de Janeiro (1988). LEI n° 1.272 (DE 06 DE JULHO DE 1988). Available at: http://mail.camara.rj.gov.br/APL/Legislativos/contlei.nsf/d38566aa34930b4d03257960005fdc91/dd35f2a978ea32d2032576ac0073895b?OpenDocument.
Rio de Janeiro (2011). Decreto Estadual n° 42.929 de 18 de abril de 2011. In: State decree no 42.929 of april 18, 2011 (Costa do Sol State Park). Available at: http://www.pesquisaatosdoexecutivo.rj.gov.br/Home/Detalhe/84905.
Salgado K., Martínez M. L., Álvarez-Molina L. L., Hesp P., Equihua M., Mariño-Tapia I. (2022). Impact of urbanization and landscape changes on the vegetation of coastal dunes along the gulf of Mexico. Écoscience 29, 103–116. doi: 10.1080/11956860.2021.1934299
Sauco S., Eguren G., Heinzen H., Defeo O. (2010). Effects of herbicides and freshwater discharge on water chemistry, toxicity and benthos in a Uruguayan sandy beach. Mar. Environ. Res. 70, 300–307. doi: 10.1016/j.marenvres.2010.06.002
Say P. T. 1824 in GBIF secretaria). GBIF backbone taxonomy. doi: 10.15468/39omei accessed via GBIF.org on 2023-06-30
Schlacher T. A., Dugan J., Schoeman D. S., Lastra M., Jones A., Scapini F., et al. (2007). Sandy beaches at the brink. Distrib. 13, 556–560. doi: 10.1111/j.1472-4642.2007.00363.x
Schlacher T. A., Nielsen T., Weston M. A. (2013). Human recreation alters behaviour profiles of non-breeding birds on open-coast sandy shores. Estuar. Coast. Shelf Sci. 118, 31–42. doi: 10.1016/j.ecss.2012.12.016
Schlacher T. A., Noriega R., Jones A., Dye T. (2012). The effects of beach nourishment on benthic invertebrates in eastern Australia: impacts and variable recovery. Sci. Total Environ. 435–436, 411–417. doi: 10.1016/j.scitotenv.2012.06.071
Schlacher T. A., Schoeman D. S., Dugan J., Lastra M., Jones A., Scapini F., et al. (2008). Sandy beach ecosystems: key features, sampling issues, management challenges and climate change impacts. Mar. Ecol. 29, 70–90. doi: 10.1111/j.1439-0485.2007.00204.x
Schmitt W. L. (1935). “Crustacea Macrura and anomura of Porto Rico and the virgin islands,” in Scientific survey of Porto Rico and the virgin islands, vol. 15. (New York: Academy of Sciences), 125–227.
Schoeman D. S., Schlacher T. A., Defeo O. (2014). Climate-change impacts on sandy-beach biota: crossing a line in the sand. Glob. Change. Biol. 20, 2383–2392. doi: 10.1111/gcb.12505
Schooler N. K., Dugan J. E., Hubbard D. M. (2019). No lines in the sand: impacts of intense mechanized maintenance regimes on sandy beach ecosystems span the intertidal zone on urban coasts. Ecol. Indic. 106, 105457. doi: 10.1016/j.ecolind.2019.105457
Schooler N. K., Dugan J. E., Hubbard D. M., Straughan D. (2017). Local scale processes drive long-term change in biodiversity of sandy beach ecosystems. Ecol. Evol. 7, 4822–4834. doi: 10.1002/ece3.3064
SNIS (2022) Sistema nacional de informações sobre saneamento. Available at: http://app4.mdr.gov.br/serieHistorica/.
Sobocinski K. L., Cordell J. R., Simenstad C. A. (2010). Effects of shoreline modifications on supratidal macroinvertebrate fauna on puget sound, Washington beaches. Estuaries Coasts 33, 699–711. doi: 10.1007/s12237-009-9262-9
Soto E. H., Botero C. M., Milanés C. B., Rodríguez-Santiago A., Palacios-Moreno M., Díaz-Ferguson E., et al. (2021). How does the beach ecosystem change without tourists during COVID-19 lockdown? Biol. Conserv. 255, 108972. doi: 10.1016/j.biocon.2021.108972
Sousa R. S. (2011). Erosão costeira nas praias da barra da tijuca, reserva e recreio dos bandeirantes, Rio de Janeiro (Rio de Janeiro: Universidade Federal do Rio de Janeiro).
Stimpson W. (1857). Notices of new species of crustacea of western north america; being an abstract from a paper to be published in the journal of the society. Proc. Boston Soc. Natural History. 6, 84–89.
Suciu M. C., Tavares D. C., Zalmon I. R. (2018). Comparative evaluation of crustaceans as bioindicators of human impact on Brazilian sandy beaches. J. Crustac Biol. 38, 420–428. doi: 10.1093/jcbiol/ruy027
Ugolini A., Ungherese G., Somigli S., Galanti G., Baroni D., Borghini F., et al. (2008). The amphipod talitrus saltator as a bioindicator of human trampling on sandy beaches. Mar. Environ. Res. 65, 349–357. doi: 10.1016/j.marenvres.2007.12.002
Veloso V. G., Caetano C. H. S., Cardoso R. S. (2003). Composition, structure and zonation of intertidal macrofauna in relation to physical factors in microtidal sandy beaches at río de Janeiro state, Brazil. Sci. Mar. 67, 393–402. doi: 10.3989/scimar.2003.67n4393
Veloso V. G., Cardoso R. S. (1999). Population biology of the mole crab emerita brasiliensis (Decapoda: hippidae) at fora beach, Brazil. J. Crustacean Biol. 19 (1), 147–153. doi: 10.2307/1549556
Veloso V. G., Cardoso R. (2001). The effect of morphodynamics on the spatial and temporal variation of the macrofauna of three sandy beaches on the Rio de Janeiro state, Brazil. J. Mar. Biol. Assoc. United Kingdom 81, 369–375. doi: 10.1017/S0025315401003976
Veloso V. G., Neves G., De Almeida Capper L. (2011). Sensitivity of a cirolanid isopod to human pressure. Ecol. Indic. 11, 782–788. doi: 10.1016/j.ecolind.2010.10.004
Veloso V. G., Neves G., Lozano M., Perez-Hurtado A., Gago C. G., Hortas F., et al. (2008). Responses of talitrid amphipods to a gradient of recreational pressure caused by beach urbanization. Mar. Ecol. 29, 126–133. doi: 10.1111/j.1439-0485.2008.00222.x
Veloso V. G., Silva E. S., Caetano C. H. S., Cardoso R. S. (2006). Comparison between the macroinfauna of urbanized and protected beaches in Rio de Janeiro state, Brazil. Biol. Conserv. 127, 510–515. doi: 10.1016/j.biocon.2005.09.027
Veloso V., Sallorenzo I., Ferreira B., de Souza G. (2009). Atlantorchestoidea brasiliensis (Crustacea: Amphipoda) as an indicator of disturbance caused by urbanization of a beach ecosystem. Braz. J. Oceanogr. 58, 13–21. doi: 10.1590/S1679-87592010000100002
Weber L. I., Barbosa T. P., Beraldini N. G., Silva V. C. (2019). Two–year study of food availability, demographic structure, and genetic diversity of the most common sandhopper in southeastern Brazil. Regional Stud. Mar. Sci. 32, 100876. doi: 10.1016/j.rsma.2019.100876
Wickham H. (2016). ggplot2 – elegant graphics for data analysis (2nd edition) (Springer International Publishing).
Wood S. N. (2004). Stable and efficient multiple smoothing parameter estimation for generalized additive models. J. Am. Stat. Assoc. 99 (467), 673–686. doi: 10.1198/016214504000000980
Wu X., Zou X., Zhong C., Yu W., Li Y., Wang T. (2020). Assessing the response of sandy-beach macrobenthos to recreation and the ecological status of the beach ecosystem at liandao, China. Mar. Ecol. 41, 1–16. doi: 10.1111/maec.12580
WWF (2019) Annual report 2019. Available at: https://wwfbr.awsassets.panda.org/downloads/wwfbra_report_2019_eng.pdf.
Zielinski S., Botero C. M., Yanes A. (2019). To clean or not to clean? a critical review of beach cleaning methods and impacts. Mar. pollut. Bull. 139, 390–401. doi: 10.1016/j.marpo
Keywords: sandy beach ecology, ecological indicators, macrofaunal community, urbanization, conservation, recreation
Citation: Augusto M, Abude RRS, Cardoso RS and Cabrini TMB (2023) Local urbanization impacts sandy beach macrofauna communities over time. Front. Mar. Sci. 10:1158413. doi: 10.3389/fmars.2023.1158413
Received: 03 February 2023; Accepted: 14 June 2023;
Published: 17 July 2023.
Edited by:
Leonardo Costa, Universidade Estadual do Norte Fluminense Darcy Ribeiro, BrazilReviewed by:
Mohamed Ben-Haddad, Ibn Zohr University, MoroccoNicholas Schooler, Ventura College, United States
Phillipe Mota Machado, Federal University of Espirito Santo, Brazil
Copyright © 2023 Augusto, Abude, Cardoso and Cabrini. This is an open-access article distributed under the terms of the Creative Commons Attribution License (CC BY). The use, distribution or reproduction in other forums is permitted, provided the original author(s) and the copyright owner(s) are credited and that the original publication in this journal is cited, in accordance with accepted academic practice. No use, distribution or reproduction is permitted which does not comply with these terms.
*Correspondence: Matheus Augusto, bS5hdWd1c3RvQGVkdS51bmlyaW8uYnI=