- 1Department of Ocean Systems, Royal Netherlands Institute for Sea Research (NIOZ), Den Burg, Netherlands
- 2Department of Renewable Marine Resources, Institut de Ciències del Mar (ICM- Spanish National Research Council), Passeig Maritim de la Barceloneta, Barcelona, Spain
- 3Department of Research Infrastructures for Marine Biological Resources, Anton Dohrn Zoological Sation, Naples, Italy
- 4Department of Fisheries and Oceans, Ocean Ecology Section, Bedford Institute of Oceanography, Dartmouth, NS, Canada
- 5Consiglio per la ricerca in agricoltura e l’analisi dell’economia agraria (CREA) – Centro di ricerca Ingegneria e Trasformazioni agroalimentari, Monterotondo, Italy
- 6Bentho-Pelagische Prozesse (BPP), Alfred Wegener Institute Helmholtz Centre for Polar and Marine Research (AWI), Bremerhaven, Germany
The genus Sebastes is a morphologically and ecologically diverse genus of rockfish characterized by high longevity, late-maturity and low natural mortality. On the northwest Atlantic continental shelf, the Acadian redfish (Sebastes fasciatus) is the most common rockfish species above 300 m depth. This species has been widely exploited resulting in the depletion or collapse of most of its stocks. Management of long-lived species with intricate life-history characteristics is challenging and requires highly integrated biological and oceanographic monitoring, which allow the identification of environmental drivers and demographic and behavioral trends. The present study uses high-temporal resolution imaging and environmental data, acquired with an autonomous lander deployed for 10-months at the Sambro Bank Sponge Conservation Area (Scotian Shelf) to elucidate S. fasciatus temporal dynamics and behavioral trends in response to near-bed environmental conditions. S. fasciatus, mostly displayed passive locomotion and static behaviors, in common with other shelf-dwelling Sebastes species. Structural complexity provided by sponges positively influenced S. fasciatus presence. Fish used sponges to avoid being dragged by bottom currents. Hydrodynamics appear to act as a synchronizing factor conditioning its swimming behavior. S. fasciatus total counts exhibited a seasonal shift in rhythm’s phase likely reflecting changes in lifestyle requirements. This study provides new insights on S. fasciatus dynamics and behavior. Nonintrusive monitoring approaches, such as the one used in this study, will be key to monitor this threatened species populations. Especially, since it is expected that S. fasciatus will experience distribution shifts to higher latitudes due to future climate stressors.
1 Introduction
The genus Sebastes is a morphologically and ecologically diverse genus of rockfish with a wide species diversity (i.e., 111 named species) (Magnuson-Ford et al., 2009). Most Sebastes species are found in the North Pacific, but few occur in the North Atlantic and Southern Hemisphere (Hyde and Vetter, 2007). Sebastes species are ovoviviparous, late maturing, with low natural mortality (Planque et al., 2013) and long-lived (several decades), certain species can easily reach 40 years and exceed 75 years of age, at which point they can measure 42 cm (Senay et al., 2021). They have adapted to a wide diversity of ecological niches but are frequently associated with rocky benthic structures (boulders, outcrops, or reefs), and hence also found in association with emergent sessile epibenthic fauna (Love et al., 2002; Auster, 2005).
Along the northwest Atlantic margin, three different species occur; deep-water redfish (Sebastes mentella) and Acadian redfish (Sebastes fasciatus), are the most abundant, while the golden redfish (Sebastes marinus) is found sporadically and can be easily differentiated from the other two species (Sévigny et al., 2008). Both S. mentella and S. fasciatus have very similar external morphological features, which makes their identification difficult. However, they are somewhat bathymetrically segregated; S. mentella occurring primarily between 350 m and 700 m depth and S. fasciatus is found primarily in shallower waters between 150 and 300 m depth (Brassard et al., 2017; Voronin et al., 2021). Their geographic distribution also differs. S. mentella mainly occurs from the Labrador Sea to the Gulf of St. Lawrence, while S. fasciatus is mostly distributed from the southern Grand Banks to the Gulf of Main (Valentin et al., 2006). Both species mostly overlay their distributions in the Grand Banks, Cape Flemish, and the Laurentian Channel, whereas on the Scotian shelf and Gulf of Main, S. fasciatus is almost the only species present (Valentin et al., 2006; Campana et al., 2016).
Both species are economically relevant in the northwest Atlantic Ocean where they are marketed as ‘ocean perch’, and their stocks have been commercially exploited since the second half of the 20th century (Cadigan and Campana, 2017). Due to their spasmodic recruitment behavior, which is characterized by irregular and infrequent pulse events, both species are highly vulnerable to fishing pressures and population fluctuations (Valentin et al., 2015). For example, in the Gulf of St. Lawrence, recruitment of redfish was consistently poor for 30 years with most stocks being collapsed or depleted, and fisheries-independent surveys initiated in 1984 showing decline in the biomass index through to 1995 (Morin et al., 1995). As a result, the redfish fishery in that region was put under a moratorium in 1995 to protect the dwindling stocks. Based on these demographics, S. fasciatus was classified as an endangered species in 1996 by the International Union for Conservation Nature – a status that has not been revisited and which requires updating (IUCN, 2021). In 2010, the Canadian Committee on the Status of Endangered Wildlife designated the population of S. fasciatus in the area as ‘Threatened’, and S. mentella as ‘Endangered’ (Benestan et al., 2021). However, demonstrative of the extreme nature of population fluctuations in these species, unprecedently strong recruitment of S. mentella, from very low population abundance, occurred in the Gulf of St. Lawrence from 2011-2013 (Burns et al., 2021). Survivorship was high, resulting in massive population increases and record-breaking biomass today.
Calanus finmarchicus nauplii, have been identified as an important dietary component of recently extruded Sebastes spp. larvae in the Gulf of St. Lawrance (Burns et al., 2021). The phenology of C. finmarchicus is primarily correlated with the timing of spring warming, and to a lesser extent with the timing of the spring bloom (Lehoux et al., 2022). Scientists have not yet been able to identify the enabling conditions for the strong Sebastes spp. recruitment pulse, however warming waters may have promoted shifts in the larval prey fields mediated through phenological changes in availability of naupliar prey resulting in faster larval growth and ultimately contributing to higher recruitment success (Burns et al, 2021). Small (< 20 cm) and medium sized (20–30 cm) redfish also saw a dietary shift from amphipods in the early 1990s to copepods in the 2010s (Brown-Vuillemin et al., 2022).
Currently, fishery closures have been implemented in Canada’s Atlantic waters, to promote the recovery of both species (Senay et al., 2021), and fisheries management plans are being re-evaluated given the unprecedented high biomass of S. mentella in the Gulf of St. Lawrence. Clearly, management of long-lived species with such complex life history characteristics poses a special challenge which will only be more difficult in the face of changing environmental conditions.
Conservation strategies based on data obtained by highly integrated multiparametric biological and oceanographic monitoring approaches can help to improve estimates of population abundance and biomass (Danovaro et al., 2017) and to anticipate the effects of climate change. Specifically, consideration of behavioral data has been shown to augment knowledge on species’ traits such as swimming rhythms, that influence dispersal and ultimately connectivity in some deep-sea species (Aguzzi et al., 2010; Aguzzi et al., 2011). Behavior associated with other events such as feeding or predation influence demographic processes, and many behaviors are influenced by environmental factors (Merrick and Koprowski, 2017). Fishers have long considered fish behavior as a means to optimize their catches (Wardle, 1986), although data on fish behavior is not commonly incorporated into management plans, largely because it is poorly understood, particularly in deep-sea species.
Behavioral data are typically obtained through direct observation by snorkeling or diving, through surface observation (e.g., through viewing windows or acoustic methods), or through use of remotely-operated underwater vehicles (Thurow et al., 2012). Each of these methods generates data over relatively short time scales and introduces sampling gears into the environment that may themselves evoke behavioral change. Video-imaging from fixed autonomous platforms such as landers or land-connected cabled observatories, allow in situ monitoring of deep-sea organisms over long periods of time (i.e., from months to years) at a high-frequency (i.e., from minutes to hours), allowing for sufficient time for the species to adjust to the presence of the instrumentation. Such devices extract new knowledge on the effects of behavioral rhythms on species presence, populations abundance, and estimated biodiversity (e.g., Matabos et al., 2014; Francescangeli et al., 2022) which may ultimately be useful in guiding fisheries management plans.
Few studies have addressed behavioral aspects of deep-sea species such as S. fasciatus and there is still a wide knowledge gap on how this species responds to changing oceanographic conditions, due to its deep distribution (Auster, 2005). Burns et al. (2021) identified changes in larval feeding behavior of Sebastes sp. through examination of gut content and otolith microstructure. However, the most well-known behavior of this species is the diel vertical migration that occurs during feeding (e.g., Gauthier and Rose, 2005). Acoustic techniques applied from surface vessels showed that Sebastes spp. were on or near the seabed by day and migrated vertically to feed in the water column around sunset in all seasons – returning to the seabed at dawn. They asserted that acoustic measurements of Sebastes spp. abundance made at night provide the most reliable and least variable density estimates and suggested that acoustic surveys could be supported by research trawling to determine species composition and size distribution. Acoustic techniques are associated with a dead zone near the bottom where fish cannot be accurately detected (Mello and Rose, 2009) and so little is known about their behavior during the day when they are associated with the seafloor. The present study uses high temporal resolution imaging and environmental data, acquired with an Autonomous Lander for Biological Experiments (ALBEX) designed by the Royal Netherlands Institute for Sea Research and deployed for a 10-month period in the Sambro Bank Sponge Conservation Area on the Scotian Shelf (Hanz et al., 2021). The study area hosts globally unique, mono-specific glass sponge aggregations of Vazella pourtalesii that provides important habitat for benthic and pelagic fauna, including redfish (Beazley et al., 2013; Hawkes et al., 2019). In this study lander data were used to characterize the behavioral rhythms of S. fasciatus in relation to: a) the near-bed hydrodynamic conditions; b) the temporal dynamic of its local population; c) its behavior trends and its response to changes in environmental conditions; and finally, d) its gregarious behavior in the Sambro Bank sponge ground (Scotian Shelf, northwestern Atlantic). Implications for the management of this species are discussed.
2 Material and methods
2.1 Study area
The Sambro Bank Sponge Conservation Area located on the Scotian Shelf (Figure 1) covers an area 62 km2 between 150 and 175 m depth. In 2013, Fisheries and Oceans Canada (DFO) closed this area to bottom fishing activities as it hosts one of the densest aggregations of the hexactinellid sponge Vazella pourtalesii, which provides habitat to a wide variety of species, including S. fasciatus (Hawkes et al., 2019).
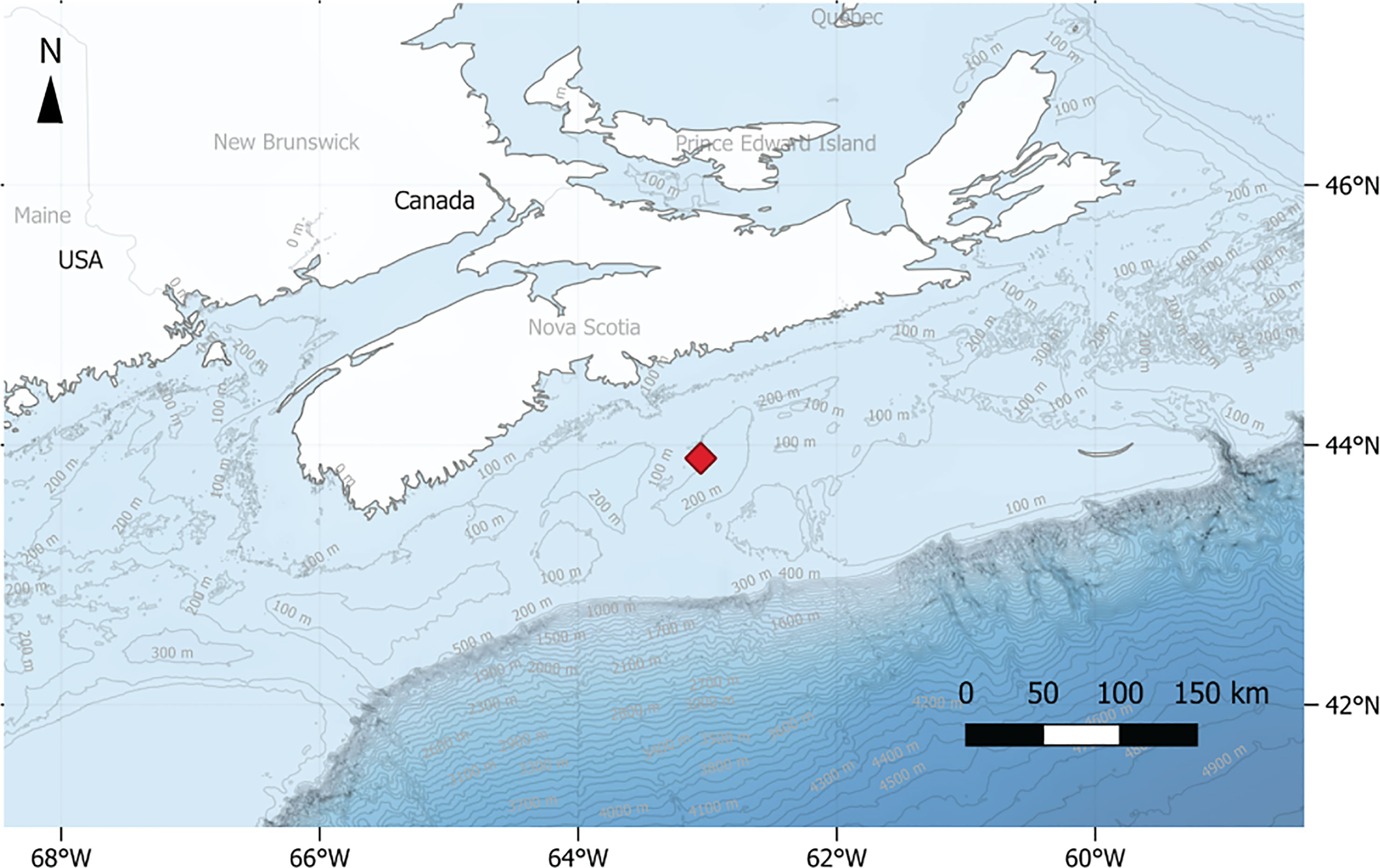
Figure 1 Location of the Sambro bank sponge conservation area on the Scotian Shelf. Bathymetric data was obtained from the European Commission, Directorate-General for Maritime Affairs and Fisheries, “EMODnet Digital Bathymetry (DTM)”, 2018, http://data.europa.eu/88u/dataset/EMODnet_bathymetry.
Two major ocean currents influence the Sambro Bank: the Gulf Stream, and the equatorward flowing Labrador Current (Hannah et al., 2001). The latter plays a major role for the benthic communities on the Scotian Shelf. Seasonally, this current can reach the bottom resulting in strong hydrodynamic events which can displace benthic organisms and sediments (Hannah et al., 2001). Currents on the Scotian Shelf are dominated by a semi-diurnal tidal cycle (Chen et al., 2011; Hanz et al., 2021). Additionally, it was recently observed that storm events can result in benthic storms, producing extreme sediment resuspension events and sponge displacements (Hanz et al., 2021).
2.2 Lander deployment
From the 6th of September 2017 until the 23rd of June 2018, the ALBEX multiparametric video-lander (Duineveld et al., 2004) was deployed at 154 m depth at the center of the Sambro Bank closure zone (43.898 N, 63.052 E) (Figure 1). The lander was equipped with a NIOZ custom-made HD video camera equipped with two white LED lights. Every 4 h the camera recorded a video that had a duration of 20 s. The 4 h video-recording frequency resulted in S. fasciatus time series of counts structured in 6 sampling events (00:00, 04:00, 08:00, 12:00, 16:00, 20:00).
The lander was also equipped with a CTD sensor (Sea-Bird SBE37SM-RS232, sampling interval = 15 min, accuracy for temperature of 0.002 °C, and conductivity of 0.003 mS cm-1) and with an ARO-USB oxygen sensor (JFE Advantech TM, sampling interval = 15 min, accuracy of nonlinearity 2% of full scale, at 1 atm and 25 C°). A two-point calibration was carried out before deployment to compensate for the time drift and ensure reliable and accurate dissolved oxygen data. An Aquadopp2 MHz (Nortek TM, sampling interval = 15 min) Acoustic Doppler Current Profiler (ADCP) was attached to the lander 2 m above the bottom (mab). The ADCP measured acoustic backscatter and velocity in 50 cm bins with an accuracy of 1% of the measured value (0.05 cm s-1).
On the 5th of January 2018, 120 days after the lander was deployed, strong hydrodynamic processes turned the lander, shifting the video camera’s Field Of View (FOV; Figure 2) (Hanz et al., 2021). The ADCP heading changed during the storm event by 6° from 265 to 259°. The lander remained in this position 168 days until the end of the study. Both FOVs recorded mixed sandy and muddy seabed areas, scattered with cobbles colonized by anemones and V. pourtalesii sponges that occurred as isolated individuals or formed small aggregations (two to three individuals). V. pourtalesii abundances were higher in the first FOV (FOV1), where 13 individuals occurred. In the second FOV (FOV2) sponge abundance fluctuated between three and four individuals, as detached sponges were dragged with the current (see Figure 2).
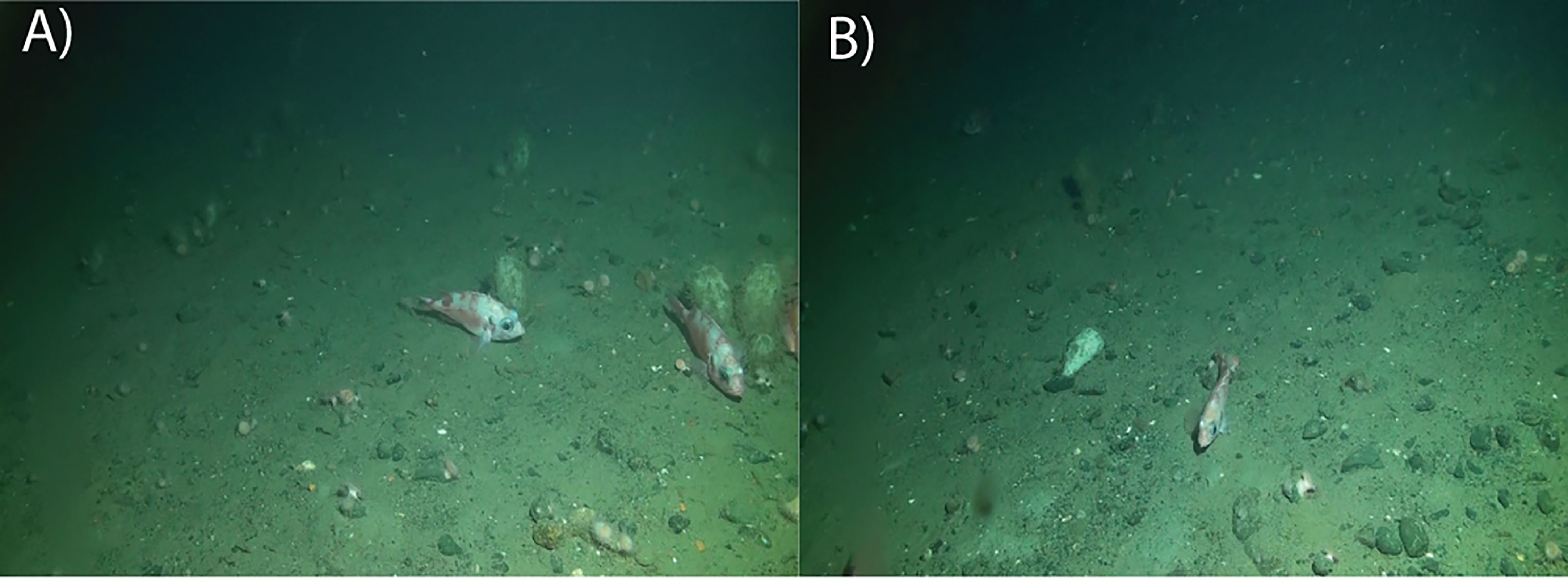
Figure 2 (A) FOV 1 with two static Sebastes fasciatus in contact with Vazella pourtalesii individuals. (B) FOV 2 with one Sebastes fasciatus sitting on the seafloor.
2.3 Image analysis
Physical samples of the redfish observed in the images were not available and species identification was inferred from the known distribution that attributes redfish on the Scotian Shelf to S. fasciatus (Ni, 1981; Valentin et al., 2006). Visual counts of putative S. fasciatus were made for each 20 s video under the assumption that fluctuating counts are a reliable proxy for averaged swimming activity into populations (hence varying the overall chance of video-spotting; Francescangeli et al., 2022). Time series of visual counts from fixed video camera stations indicate a general level of activity into monitored populations since counted specimens are proportional to the overall population. When animals are present in higher abundances or are more behaviorally active their counts increment as the probability to enter the field of view is higher. Additionally, each individual’s behavior was registered during the first 5 s of its appearance in the FOV to avoid any potential disturbance generated by the lander (e.g., illumination, noise; e.g., Doya et al., 2014). Innovatively the behavioral activity of S. fasciatus was subdivided into four categories:
i) descending: sinking almost static towards the seafloor (Supplementary video 1);
ii) drifting: drifting with current few centimeters from the seafloor (Supplementary video 2);
iii) static: remaining still on the seafloor (Supplementary video 3); and finally,
iv) swimming: active natation sufficient to overcome currents (Supplementary video 4).
Static individuals interacting with V. pourtalesii, by leaning against it or sheltering from currents a few centimeters from it, were also reported. Current direction was deduced from the movement of suspended particles.
2.4 Data treatment
To obtain a global overview of S. fasciatus behavioral rhythms and explore potential differences between the two FOVs, visual count time series for the 10-month sampling period were plotted. The occurrence of significant differences in S. fasciatus counts between both FOVs was tested with a Wilcoxon test, as data did not display a normal distribution. The Wilcoxon test was performed using the R-language function “wilcox.test” (Oksanen et al., 2016).
To explore the relationship between S. fasciatus count rhythms and bottom current velocity, monthly average waveforms were calculated and plotted together for both factors (Doya et al., 2014). Firstly, to obtain information on S. fasciatus activity rhythms, waveforms analysis was conducted on 4-hour counts time series. Segments’ values were averaged per each month at corresponding timings (00:00, 04:00, 08:00, 12:00, 16:00, 20:00). Resulting averages were then plotted along with the standard error (SE) and a horizontal threshold line, the Midline Estimated Statistic of Rhythm (MESOR, Francescangeli et al., 2022). The MESOR were calculated by averaging monthly interval average values. Values above the MESOR are considered as composing the rhythm phase (i.e., peaks). Similarly, tidal patterns were studied by waveform analysis of time series in bottom current velocity data. ADCP readings 3 meters above the bottom (mab) were compiled into one hour interval via values averaging at corresponding one hour time intervals. Then, the monthly waveform curves and MESOR were calculated by averaging those hourly data, which were plotted with their SE.
The relationship S. fasciatus behavior and environmental factors (temperature, salinity, oxygen concentration and current velocities and directions registered between 3 and 4.5 mab) was explored by means of a Canonical Correspondence Analysis (CANOCO) (Legendre and Legendre., 1998). This analysis determined the relative contributions of behavioral rhythms (as indicated by count fluctuations) and environmental status (by multiparametric oceanographic monitoring) in the same multidimensional space (Aguzzi et al., 2018). The ordination axes are linear combinations of the cultivars. Both matrices (species counts and environmental data) were standardized using correlation matrices. The CANOCO was performed using the free software PAST 2.17c (Hammer et al., 2001).
To study the gregarious behavior of S. fasciatus, defined as individuals shoaling (loosely organized group of fish) (Larsson, 2012) or remaining static in small groups on the seafloor, videos were ranked based on the number of individuals occurring simultaneously in the same field of view. Posteriorly the frequency of groups of individuals was represented with a histogram plot. Differences in gregariousness frequency between sampling events (00:00, 04:00, 08:00, 12:00, 16:00, 20:00) and seasons, (defined by the winter solstice and spring and autumn equinox) were determined by means of an Adonis multivariate analysis and subsequent pairwise test using the R-language function “adonis” (Oksanen et al., 2016). Summer was excluded from this analysis as it was underrepresented (16 days) compared to the other seasons. Demersal fish can aggregate over wide areas (Williams et al., 2021) to discard potential spatial constraints intrinsic to the lander field view, gregarious data was also assessed in six photo-transects recorded with the ROV Ropos, equipped with a Pacific-Zeux HD Camera with a 96-pixel resolution, that covered several hundreds of meters.
3 Results
3.1 Environmental setting
The water temperature at the seafloor ranged between 8.8 and 12°C and salinity was between 34.1 and 35.3 psu from summer 2017 to 2018 (Figure 3). Currents at 3 mab were on average 0.11 m s-1 with peaks of 0.38 m s-1 during late autumn (November) and early spring (March) storm events (see Figure 3).Current direction oscillated between a NW and SE direction with a semi-diurnal cycle. In January and especially March average current direction changed in a more westward direction (Hanz et al., 2021).
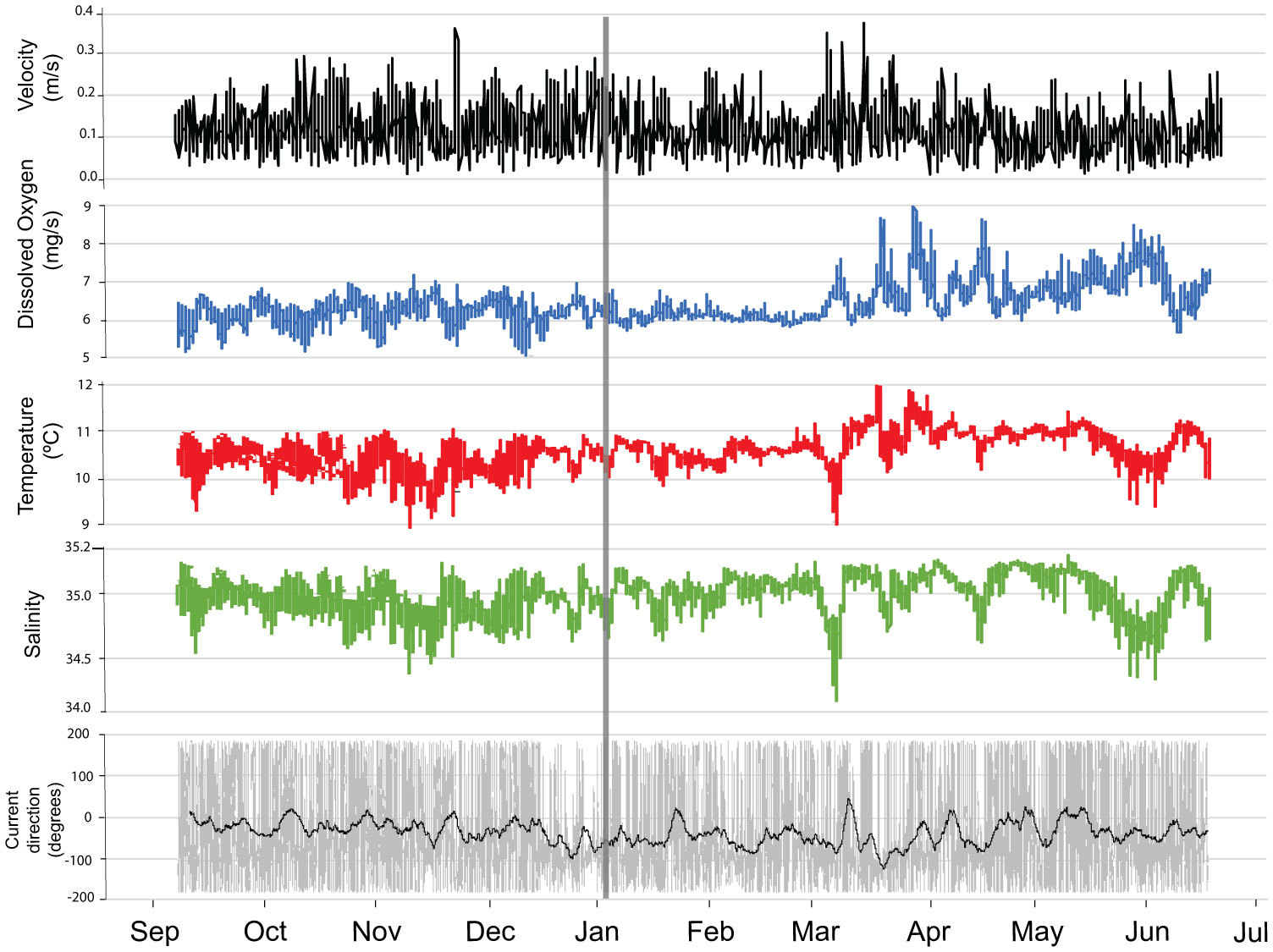
Figure 3 Near-seabed environmental conditions, recorded from September 2017 to June 2018. Current velocity and direction time series is only for the ADCP bin of 3 mab. In the current direction panel, grey lines represent current direction values obtained every 15 minutes and the black line represents daily current direction average values. The gray vertical line represents the shift from FOV1 to FOV2.
3.2 S. fasciatus counts
A total of 1740 videos were obtained (six daily sampling events for 290 days), although due to intense turbid conditions, 10 videos could not be analyzed. S. fasciatus were present in 852 videos (49.2% of all analyzed). A total of 2504 individuals were counted, 47.48% of which in FOV1 and 52.52% in FOV2. In both FOVs, individuals mainly displayed descending and static behaviors, jointly accounting for 81.4% and 73.4% in FOV1 and FOV2, respectively (Figure 4). Conversely, swimming was the scarcest behavior pattern displayed by 7.2% and 5.2% of all observed individuals, respectively in FOV1 and FOV2 (Figure 4).
S. fasciatus was unevenly distributed in both FOVs, with most count peaks registered in FOV1 (Figure 5). In this regard, average count values were higher in FOV1 than in FOV2 (Table 1), and when comparing behaviors, significant differences were only found for descending fish counts.
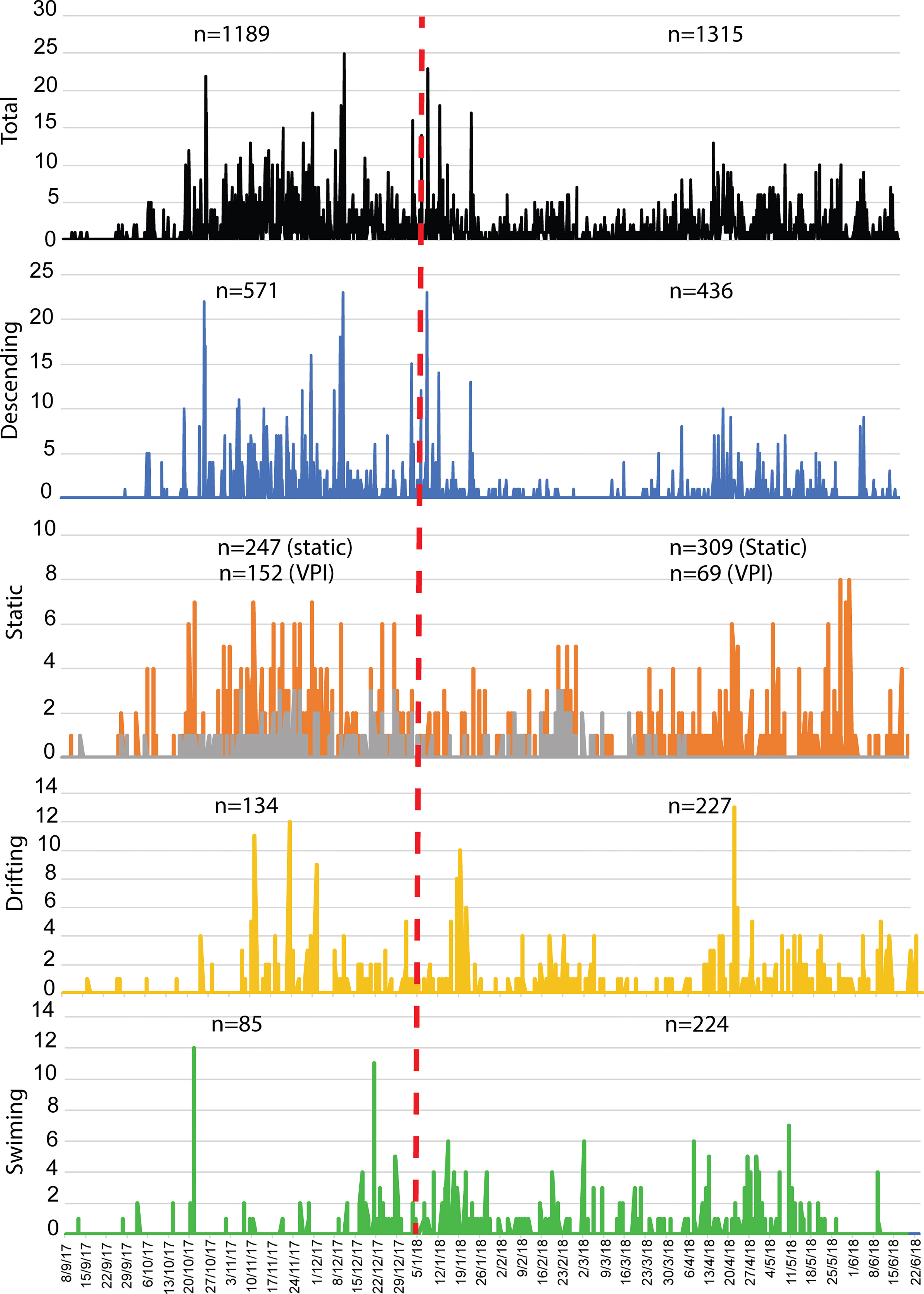
Figure 5 Ten-month time series of Sebastes fasciatus visual counts. In the Static plot, the gray time series represents individuals interacting (leaning or remaining static few cm from sponges) with Vazella pourtalesii (VPI) while orange time series those which do not. Dashed vertical red line separates FOV1 from FOV2. (n= number of individuals).
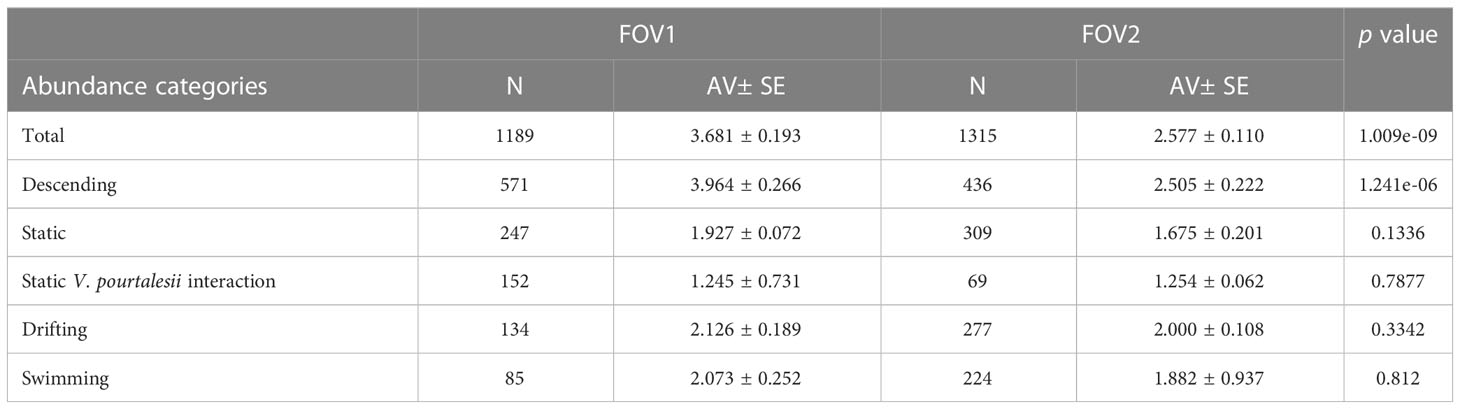
Table 1 Sebastes fasciatus averaged counts (AV± SE) abundances and Pairwise test (Wilcoxon test) analysis for differences between FOV (p value).
3.3 Wave form analysis
Waveform analyses showed that S. fasciatus averaged counts exhibited a temporal shift in peak timing (i.e., values above the MESOR defining the phase of the count rhythm) (Figure 6). In autumn (September – October) peaks occurred from 12:00 to 20:00, shifting towards 00:00 to 04:00 in early winter (November – January), and from 08:00 to 16:00 in late winter and spring (February – June). Waveform analysis for current velocity (i.e., the bin at 3 mab) showed a bimodal peak profile in most months as a proxy for a semidiurnal tidal regime (see Figure 6). For several months (September, October, December, and June) S. fasciatus total counts peaked simultaneously with peaks of highest bottom current velocity (see Figure 6).
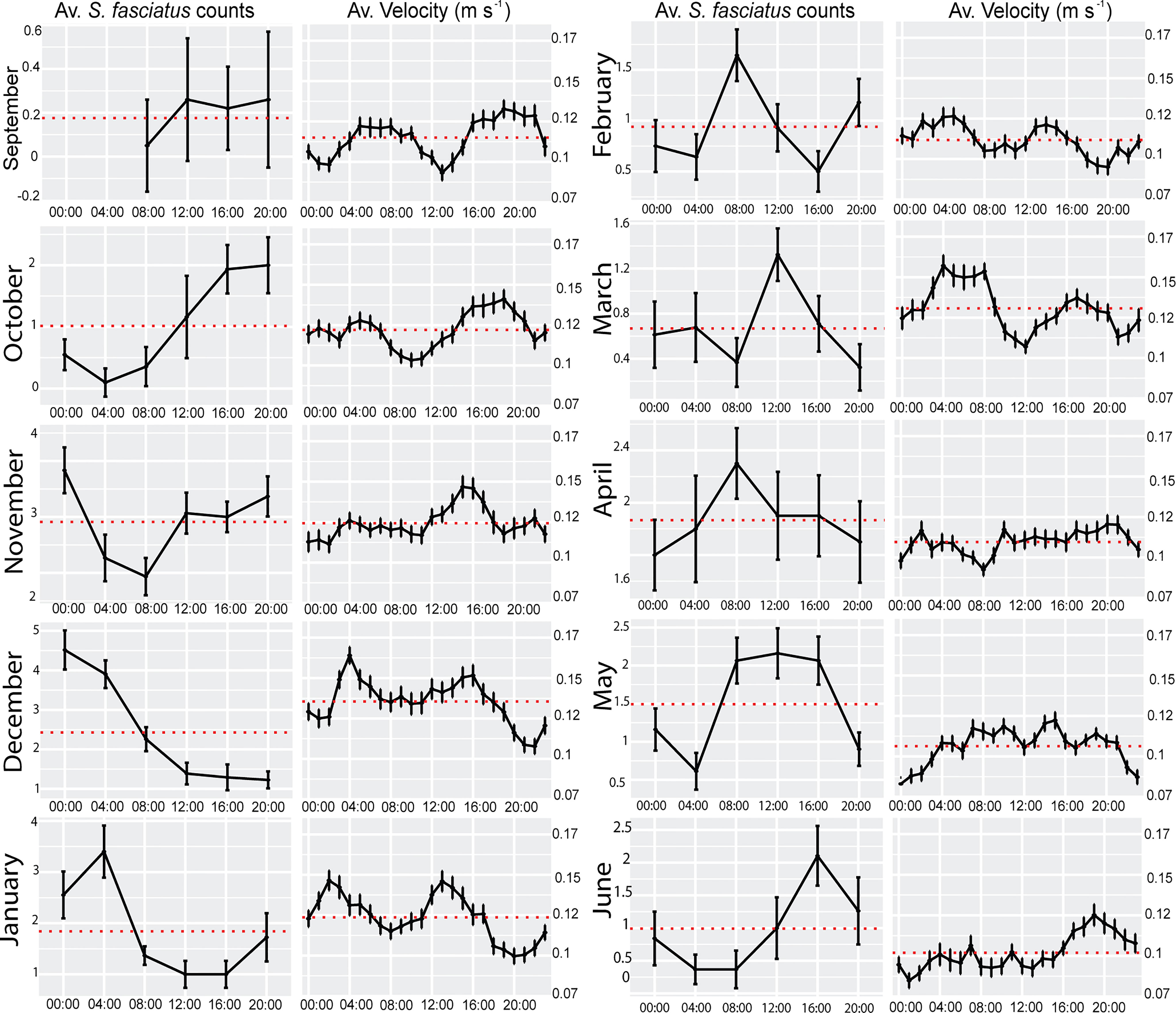
Figure 6 Waveform analysis of hourly means of Sebastes fasciatus counts and bottom current velocity for each month. Red horizontal dashed red lines represent the MESOR. Error bars indicate the SE.
Additionally, the waveform analysis for counted fishes within the 4 different behavioral categories (Supplementary figure 1) generally displayed a similar pattern to the one of total counts. All behaviors were similarly affected by the tidal regime.
3.4 Influence of environmental variables on S. fasciatus behavior
In the CANOCO the first two axis accounted for 83.9% and 9.2% of the behavioral categories-environment relation variance (Figure 7). Descending and static individuals interacting with sponges were negatively associated with temperature, oxygen concentration, and current direction (Figure 7). Contrariwise, drifting individuals were positively associated with current velocities between 3.5 and 4.5 mab and higher oxygen concentrations (see Figure 7). Static individuals which did not interact with sponges were positively associated with current velocities 3 mab and current directions (3 to 4.5 mab). Finally, swimming individuals were associated with salinity, temperature, and current direction (see Figure 7).
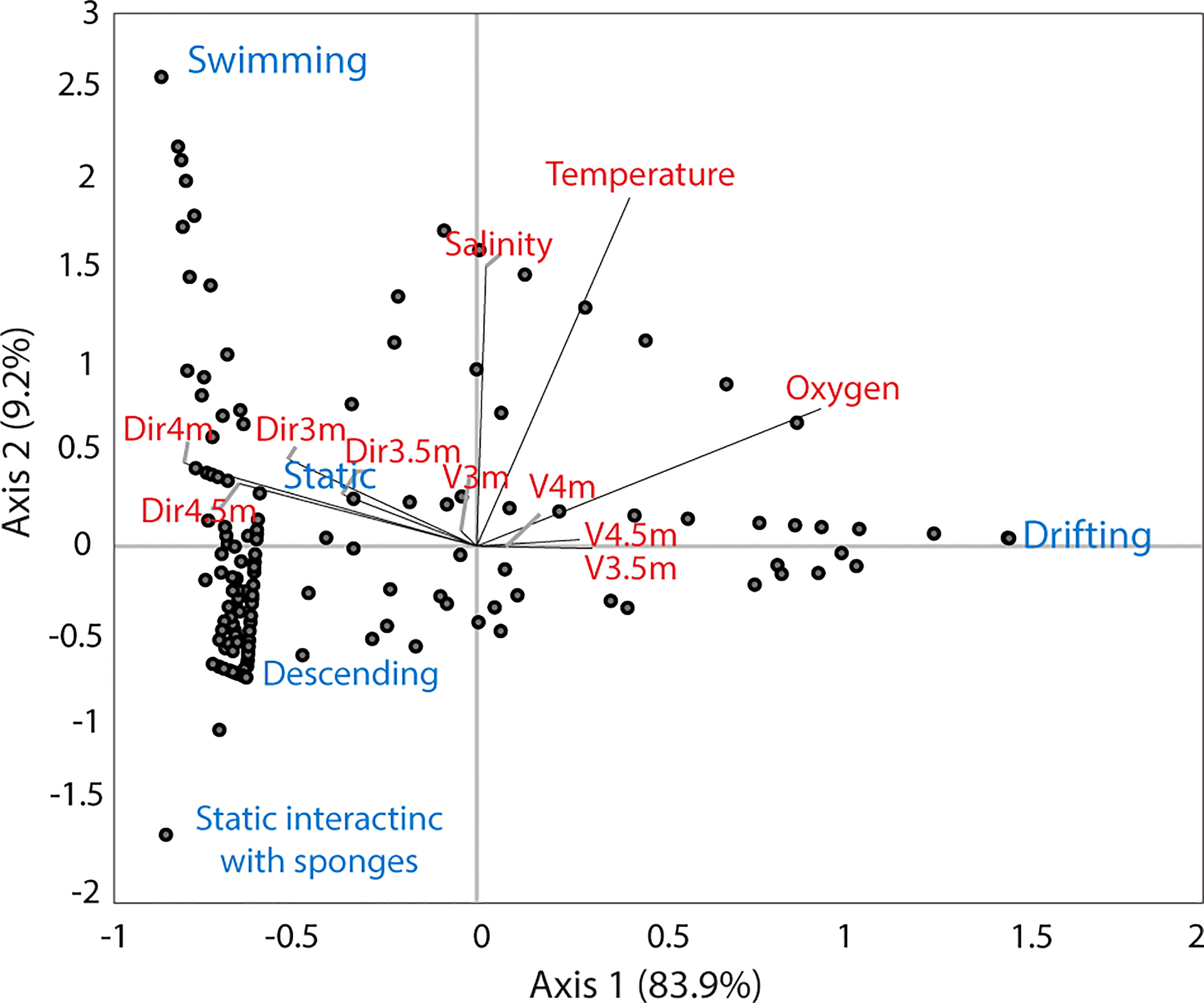
Figure 7 CANOCO bi-plot showing the ordination of behavioral categories, observation made during the videos (points) and the roles of the significant environmental variables (red vectors), whose length is proportional to their significance in explaining the distribution of biological data as response variables. Dir3m: current direction 3 mab, Dir3.5m: current direction 3.5 mab, Dir4m: current direction 4 mab, Dir4.5m: current direction 4.5 mab. V3m: current velocity 3 mab, V3.5m: current velocity 3.5 mab, V4m: current velocity 4 mab, V4.5m: current velocity 4.5 mab.
3.5 S. fasciatus gregarious behavior
Mostly, S. fasciatus was observed as isolated individuals or in pairs generally occurring close to one another (Figure 2), representing 42% and 20% of all observations, respectively (Figure 8). Gregarious behavior was mainly displayed while fish were descending or drifting (36.6% and 40%, respectively). Aggregations of three to six individuals jointly accounted for 29% of observations while aggregations between seven to 23 individuals represented 8% of all observations (Figure 8). Adonis permutation multivariate analysis of variance revealed that there were no significant differences (p value: 0.1639) in gregarious occurrence among daily sampling events (00:00, 04:00, 08:00, 12:00, 16:00, 20:00). Yet, in terms of seasonality, autumn (23 of September to 22 of December) presented significantly higher (p value< 0.001) aggregation occurrence than the other seasons.
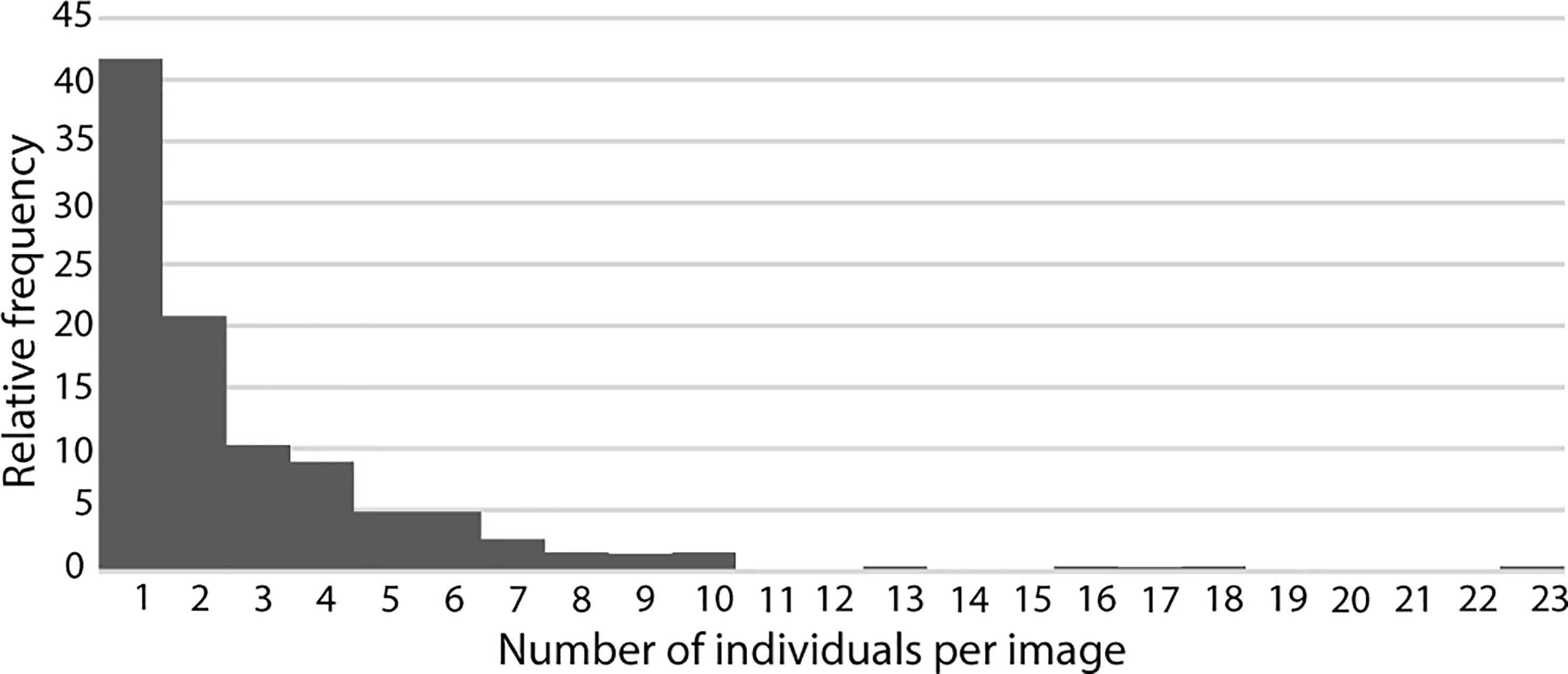
Figure 8 Relative percentages of videos with different counts of Sebastes fasciatus as an indication of their level of aggregation.
4 Discussion
4.1 S. fasciatus behavioral assessment
In this study, we describe the temporal count dynamics of the Acadian redfish, S. fasciatus on a V. pourtalesii aggregation on the Scotian continental shelf and identify the main environmental processes influencing their occurrence and behavior while on or near the seabed in the acoustic dead zone. Most individuals displayed sitting or passively displacing (descending or drifting) behaviors (94.8% and 92.8% in FOV1 and FOV2, respectively; see Figure 3). Northwest Atlantic Sebastes species exhibit a semi-pelagic shoaling behavior vertically migrating to shallow depth on a diurnal bases to feed (Cadigan et al., 2022). The stomach content of S. fasciatus are mainly pelagic organisms (i.e., up to a 90% of total stomach volume) only occasionally feeding on benthic organisms (Moran et al., 1996). Of the 2504 individuals here detected, only one was observed feeding on a swimming crustacean. Thus, the low abundance of prey in benthic environments may explain the predominance of passive and static behaviors as fish do not require to engage in active behaviors such as the pursue of pelagic prey.
In this sense, Sebastes species occurring in benthic environments have frequently been described to display a sluggish behavior hovering over the seafloor, drifting with currents, or resting near benthic structures or sessile organisms based on short period observations (Brodeur, 2001; Auster et al., 2003; Johnson et al., 2003; Stierhoff et al., 2013). Boulders or epibenthic organisms (e.g., sponges, gorgonians, cerianhtids) have been described to positively influence Sebastes species occurrence at a local scale (Tissot et al., 2006; Parker et al., 2008) and of S. fasciatus specifically (Hawkes et al., 2019). The highly resolved temporal data collected from the lander shows that these observations are typical. Higher S. fasciatus counts registered in FOV1 may result from a higher number of V. pourtalesii individuals that provide greater structural complexity (Figures 2, 4; Table 1), as difference in the density of V. pourtalesii was the main difference between the two sampling FOVs. This is consistent with other image-based studies, where S. fasciatus and other shelf dwelling Sebsates species, were more abundant in areas hosting sponge and anthozoan aggregations than in surrounding areas (Auster, 2005; Stone, 2006). Anthozoans and sponges, contribute to vertical relief enhancing microhabitat availability from which ichthyofauna may benefit (Tissot et al., 2006). Indeed, other deep-living Sebastes species have been reported to use sessile organisms, as nursery (Wilborn et al., 2022), shelter, and feeding grounds (Krieger and Wing, 2002; Wilborn et al., 2022).
It was also observed that S. fasciatus used V. pourtalesii to shelter from currents. Most static individuals interacting with sponges (67%) were observed during relatively high current events ranging between 0.12 and 0.32 m s-1. Under these circumstances, interacting individuals would lean or shelter behind sponges while, individuals that did not interact with sponges would face current to reduce water resistance (Figure 9). These behaviors are consistent with previous observations made on several demersal fish species, occurring on continental shelves and canyons that have been observed to use sessile fauna to shelter from current (Button et al., 2021; Chatzievangelou et al., 2022).
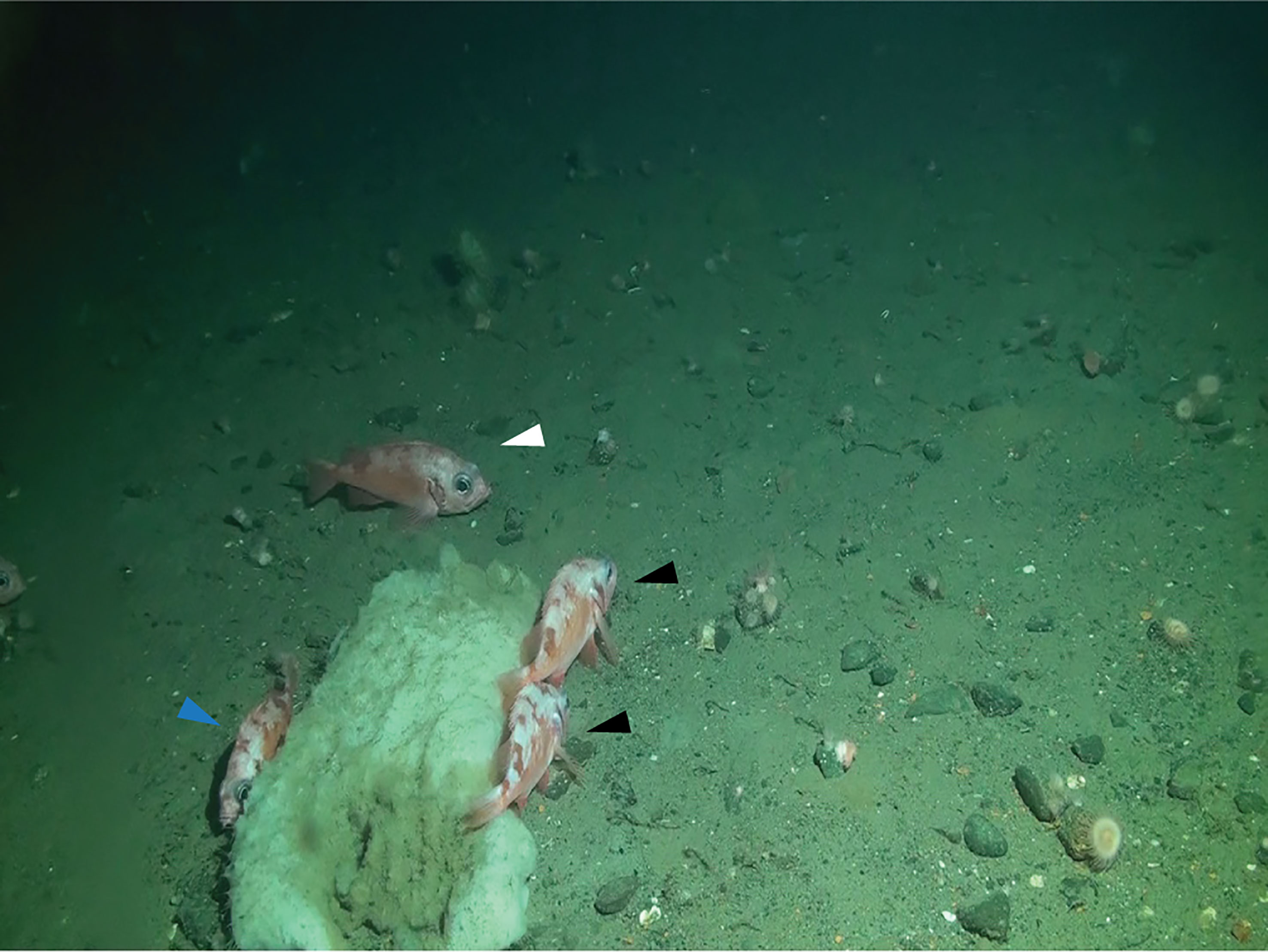
Figure 9 Static Sebastes fasciatus facing current (white arrowhead), leaning (black arrowhead) and standing behind (blue arrowhead) Vazella pourtalesii. This image’s associated current velocity reached 0.17 m s-1, current direction in relation to S. fasciatus position was determined by observing suspended particle movement.
Current velocity and direction appeared to be the main forcing mechanisms behind S. fasciatus passive movement behaviors (descending and drifting) (Figure 7). In this study, most descending and drifting individuals (57% and 72%, respectively) were associated with currents below 0.12 m s-1. S. fasciatus may use these low currents to disperse across the shelf investing little energy, which is a common strategy used by deep-sea demersal fish species (Uiblein et al., 2003). A variable relationship between species counts fluctuations and tidal cycles has been found, depending on the species movement life habit (i.e., stronger in swimmers as fishes, intermediate in crustacean decapods as walkers, and weaker in gastropod as crawling movers; Aguzzi et al., 2010). Here, actively swimming individuals were mostly influenced by temperature, salinity, and current direction (Figure 7). In the study area fluctuations in water temperature, and salinity have been associated with variations in the main water masses entering the Scotian Shelf and with storm events (Urrego-Blanco and Sheng, 2014; Hanz et al., 2021). This might indicate that swimming behavior is associated with variations in hydrodynamic conditions triggered by changes in water mass and benthic storm events.
4.2 S. fasciatus population temporal dynamics
In the present study, bottom currents displayed a bimodal velocity peak. During several months, S. fasciatus abundance peaks simultaneously occurred with the highest current velocity peak (see Figure 6). Bottom currents can also affect demersal fish species abundances (Doya et al., 2016) influencing species biological clocks and synchronizing swimming activity (Doya et al., 2014; Chatzievangelou et al., 2016). In this scenario, the data indicate that under high current velocity S. fasciatus remains on the seafloor likely to avoid being displaced by tidal flows. This behavior has been proposed as a mechanism that deep-sea demersal organisms display to keep seabed positioning, simultaneously decreasing energy consumption (Aguzzi et al., 2010). As the species is associated with the seabed following an extended (dusk to dawn) feeding period (Gauthier and Rose, 2005), it is possible that this phase is critical to digestion and energy conversion. Furthermore, S. fasciatus total counts exhibited a seasonal shift (abundance peaks shifted from 12:00 to 20:00 in autumn, to 00:00 to 04:00 in early winter and 08:00 to 16:00 in late winter and spring) in timing of rhythm’s phase as indicated by monthly waveform analysis (see Figure 6). This seasonal shift in abundance may reflect changes in the lifestyle requirement associated to this species. For example, S. fasciatus has been reported to change their distribution between summer and winter locations (Campana et al., 2007). Also, in the northwest Atlantic, Sebastes spp. are suspected to mate on mid-waters from late summer to early winter and larvae are speculated to be released in deeper waters below the intermediate cold layer during spring months (Cadigan et al., 2022).
4.3 S. fasciatus gregarious behavior
Finally, S. fasciatus mainly occurred as isolated or pairs of individuals (see Figure 8). Gregariousness was most common while individuals were in the water column generally forming shoals. This grouping behavior agrees with sonar observations in which Sebastes spp. appear to form densely packed aggregations in the water column close to the seafloor and appear more scattered while on the seafloor (Gauthier and Rose, 2005). Additionally, the fact that there were no significant differences between sampling events contrasts with previous observations on shallow water visual predatory fish which mainly grouped during daytime (Francescangeli et al., 2022). Finally, aggregation occurrences were significantly higher during autumn. This seasonal trend could be related to the fact northwest Atlantic Sebastes species mate from September to December (Gascon, 2003). Nevertheless, it should also be considered that during autumn, the lander was oriented towards FOV1, which as it has been previously explained presented a higher structural complexity that likely favored higher S. fasciatus occurrence. Therefore, it is unclear if this seasonal trend responds to S. fasciatus biology or its ecological requirements. To address this matter future image-based nonintrusive monitoring studies should be enforced with the following specifications: over complete and consecutive 24-h cycles; estimates should be derived at count peak timing as indicated by waveform analysis and completed over at least two consecutive years to provide valuable data on the effects that behavior, reproduction, and growth may exert on perceived demographic estimates of this threatened species.
4.4 Considerations on future scenarios and management
Species distribution models indicate that under future climate scenarios, suitable thermal habitat of S. fasciatus will shift to higher latitudes (Kleisner et al., 2017). However, it is unclear how this species will respond to variations in other factors such as oxygen concentration, acidification, primary and secondary production or change in benthic habitat. Recently, trait-based approaches indicate that under future climate stressors (ocean warming, deoxygenation, acidification, decrease in food supply) long-lived demersal fish species, with slow growth and maturation rates, such as S. fasciatus, will experience high hazard levels that will challenge their long-term survival (Cheung et al., 2022). However, this contrasts with the increased biomass of the Deepwater redfish in the Gulf of St. Lawrence which has been attributed to phenological changes in prey cycles favoring Sebastes larval growth and survivorship (Burns et al., 2021). If the close association with the seabed during the daylight hours is necessary for digestion, then environmental changes occurring there also have the potential to disrupt the energy budgets and ultimately growth rates. Under this scenario, monitoring activities in relation to fishery independent assessment procedures will be highly relevant specially when dealing with threatened species (Aguzzi et al., 2022).
Implications of these results for fisheries management lay in the observed passive behavior of the fish on the seabed during daylight hours. This provides further evidence for the higher catchability during the day hours as reported by Atkinson (1989) and should be factored into data analyses for both fishery-dependent and fishery-independent trawl catches. Although dense aggregations were not observed, neither are they found during pelagic feeding at night when redfish are dispersed through the upper water layers (Gauthier and Rose, 2005).
5 Conclusions
Like most shelf dwelling Sebastes species, S. fasciatus, mostly displayed passive locomotion and static behaviors when near the seabed. The structural complexity provided by V. pourtalesii appeared to positively influence S. fasciatus presence. It was observed that S. fasciatus used this sponge to shelter from current drag. Hydrodynamics likely act as a synchronizing cue for its swimming behavior. S. fasciatus total counts exhibited a seasonal shift in peak phase contrasting with the fact that this species performs dial migrations, remain close to the bottom during the day and redistribute into the water column at night. Shoaling behavior was mostly observed while fishes where above the seafloor mainly while descending or drifting. Conversely, static individuals remained solitary.
Data availability statement
The Raw data supporting this article are in the article/Supplementary Material. Further inquiries can be directed to the corresponding author.
Ethics statement
Ethical review and approval was not required for the animal study because this study is based on images recorded in the deep-sea benthic environments. No organisms were captured nor manipulated in any way.
Author contributions
JG, JA, FM, and EK were involved in the conceptualization; CC provided the CANOCO analysis and wrote a section of the data analysis section. UH provided study area figure and wrote a section of the environmental results. JG, EK, and FM, acquired the funding. JG analysed images, treated the data and wrote the original draft. JG, JA, EK, CC, UH, and FM reviewed and edited the manuscript. All authors contributed to the article and approved the submitted version.
Funding
This study is part of the SponGES project, which received funding from the European Union’s Horizon 2020 Research and Innovation Program under grant agreement no. 679849. Cruises were funded by Fisheries and Ocean’s Canada’s (DFO) International Governance Strategy awarded to EK, Bedford Institute of Oceanography, and supported by Hans Tore Rapp (University of Bergen, Norway). JG has received funding form the European Union’s Horizon 2020 research and Innovation programme. Action MSCA-IF-EF-ST fellowship, CWCC-Dynamics Grant agreement no. 101028621.
Acknowledgments
The authors would like to thank Trevor Kenchington and Camile Lirette, from Bedford Institute of Oceanography, for their help while elaborating the manuscript.
Conflict of interest
The authors declare that the research was conducted in the absence of any commercial or financial relationships that could be construed as a potential conflict of interest.
Publisher’s note
All claims expressed in this article are solely those of the authors and do not necessarily represent those of their affiliated organizations, or those of the publisher, the editors and the reviewers. Any product that may be evaluated in this article, or claim that may be made by its manufacturer, is not guaranteed or endorsed by the publisher.
Supplementary material
The Supplementary Material for this article can be found online at: https://www.frontiersin.org/articles/10.3389/fmars.2023.1158283/full#supplementary-material
References
Aguzzi J., Chatzievangelou D., Robinso N. J., Bahamon N., Berry A., Carreras M., et al. (2022). Advancing fishery-independent stock assessments for the Norway lobster (Nephrops norvergicus) with new monitoring technologies. Front. Mar. Sci. 9. doi: 10.3389/fmars.2022.969071
Aguzzi J., Company J. B., Costa C., Menesatti P., Garcia J. A., Bahamon N., et al. (2011). Activity rhythms in the deep-sea: a chronobiological approach. Front. Biosci. 16, 131–150. doi: 10.2741/3680
Aguzzi J., Costa C., Furushima Y., Chiesa J. J., Company J. B., Menesatti P., et al. (2010). Behavioral rhythms of hydrocarbon seep fauna in relation to internal tides. Mar. Ecol. Prog. Ser. 418, 47–56. doi: 10.3354/meps08835
Aguzzi J., Fanelli E., Ciuffardi T., Schirone A., De Leo F., Doya C., et al. (2018). Faunal activity rhythms influencing early community succession of an implanted whale carcass offshore sagami bay Japan. Sci. Rep. 8, 1–15. doi: 10.1038/s41598-018-29431-5
Atkinson D. B. (1989). Diel movements of beaked redfish and the implications of these for stratified random bottom trawl estimates of biomass and abundance. N. Am. J. Fish. Manage. 9, 163–170.
Auster P. J. (2005). ““Are deep-water corals important habitats for fishes?”,” in Cold-water corals and ecosystems. Eds. Freiwald A., Robert M. J. (Berlin, Heidelberg: Springer), 747–760.
Auster P. J., Lindholm J., Valentine P. C. (2003). Variation in habitat use by juvenile acadian redfish, Sebastes fasciatus. Environ. Biol. Fish. 68, 381–389. doi: 10.1023/B:EBFI.0000005751.30906.d5
Beazley L. I., Kenchington E. L., Murillo F. J., Sacau M. D. (2013). Deep-sea sponge grounds enhance diversity and abundance of epibenthic megafauna in the Northwest Atlantic. ICES J. Mar. Sci. 70, 1471–1490. doi: 10.1093/icesjms/fst124
Benestan L. M., Rougemont Q., Senay C., Normandeau E., Parent E., Rideout R., et al. (2021). Population genomics and history of speciation reveal fishery management gaps in two related redfish species (Sebastes mentella and Sebastes fasciatus). Evol. Appl. 14, 588–606. doi: 10.1111/eva.13143
Brassard C., Bourdages H., Duplisea D., Gauthier J., Valentin A. (2017). The status of the redfish stocks (Sebastes fasciatus and S. mentella) in unit 1 (Gulf of st. Lawrence) in 2015. Can. Sci. Advisory Secretariat 2017/023. ix + 53 p.
Brodeur R. D. (2001). Habitat-specific distribution of pacific ocean perch (Sebastes alutus) in pribilof canyon, Bering Sea. Cont. Shelf. Res. 21, 207–224. doi: 10.1016/S0278-4343(00)00083-2
Brown-Vuillemin S., Chabot D., Nozères C., Tremblay R., Sirois P., Robert D. (2022). Diet composition of redfish (Sebastes sp.) during periods of population collapse and massive resurgence in the gulf of st. Lawrence. Front. Mar. Sci. 9. doi: 10.3389/fmars.2022.963039
Burns C. M., Pepin P., Plourde S., Veillet G., Sirois P., Robert D. (2021). Revealing the relationship between feeding and growth of larval redfish (Sebastes sp.) in the gulf of st. Lawrence. ICES J. Mar. Sci. 78, 3757–3766. doi: 10.1093/icesjms/fsab221
Button R. E., Parker D., Coetzee V., Samaai T., Palmer R. M., Sink K., et al. (2021). ROV assessment of mesophotic fish and associated habitats across the continental shelf of the amathole region. Sci. Rep. 11, 1–11. doi: 10.1038/s41598-021-97369-2
Cadigan N. G., Campana S. E. (2017). Hierarchical model-based estimation of population growth curves for redfish (Sebastes mentella and Sebastes fasciatus) off the Eastern coast of Canada. ICES J. Mar. Sci. 74, 687–697. doi: 10.1093/icesjms/fsw195
Cadigan N. G., Duplisea D. E., Senay C., Parent G. J., Winger P. D., Linton B., et al. (2022). Northwest Atlantic Redfish science priorities for managing an enigmatic species complex. Can. J. Fish. Aquat. Sci. 79, 1572–1589. doi: 10.1139/cjfas-2021-0266
Campana S. E., Valentin A. E., MacLellan S. E., Groot J. B. (2016). Image-enhanced burnt otoliths, bomb radiocarbon and the growth dynamics of redfish (Sebastes mentella and S. fasciatus) off the eastern coast of Canada. Mar. Freshw. Res. 67, 925–936. doi: 10.1071/MF15002
Campana S. E., Valentin A., Sévigny J. M., Power D. (2007). Tracking seasonal migrations of redfish (Sebastes spp.) in and around the gulf of st. Lawrence using otolith elemental fingerprints. Can. J. Fish. Aquat. Sci. 64, 6–18. doi: 10.1139/f06-162
Chatzievangelou D., Doya C., Thomsen L., Purser A., Aguzzi J. (2016). High-frequency patterns in the abundance of benthic species near a cold-seep – an Internet operated vehicle application. PloS One 11, e0163808. doi: 10.1371/journal.pone.0163808
Chatzievangelou D., Thomsen L., Doya C., Purser A., Aguzzi J. (2022). Transects in the deep: opportunities with tele-operated resident seafloor robot. Front. Mar. Sci. 9. doi: 10.3389/fmars.2022.833617
Chen C., Huang H., Beardsley R. C., Xu Q., Limeburner R., Cowles G. W., et al. (2011). Tidal dynamics in the gulf of Maine and new England shelf: an application of FVCOM. J. Geophys. Res. Oceans. 116, 12010. doi: 10.1029/2011JC007054oi.org/10.1029/2011JC007054
Cheung W. W., Wei C. L., Levin L. A. (2022). Vulnerability of exploited deep-sea demersal species to ocean warming, deoxygenation, and acidification. Environ. Biol. Fishes 105, 1301–1315. doi: 10.1007/s10641-022-01321-w
Danovaro R., Aguzzi J., Fanelli E., Billet D., Gjerde K., Jamieson A., et al. (2017). A new international ecosystem-based strategy for the global deep ocean. Science. 355, 452–454. doi: 10.1126/science.aah7178
Doya C., Aguzzi J., Chatzievangelou D., Costa C., Company J. B., Tunnicliffe V. (2016). The seasonal use of small-scale space by benthic species in a transiently hypoxic area. J. Mar. Syst. 154, 280–290. doi: 10.1016/j.jmarsys.2015.09.005
Doya C., Aguzzi J., Pardo M., Matabos M., Company J. B., Costa C., et al. (2014). Diel behavioral rhythms in the sablefish (Anoplopoma fimbria) and other benthic species, as recorded by deep-sea cabled observatories in barkley canyon (NEPTUNE-Canada). J. Mar. Syst. 130, 69–78. doi: 10.1016/j.jmarsays.2013.04.003
Duineveld G., Lavaleye M., Berghuis E. (2004). Particle flux and food supply to a seamount cold-water coral community (Galicia bank, NW Spain). Mar. Ecol. Prog. Ser. 277, 13–23. doi: 10.3354/meps277013
Francescangeli M., Sbragaglia V., del Río Fernandez J., Trullols E., Antonijuan J., Massana I., et al. (2022). Long-term monitoring of diel and seasonal rhythm of Dentex dentex at an artificial reef. Front. Mar. Sci. 9. doi: 10.3389/fmars.2022.837216
Gascon D. (2003). Redfish multidisciplinary research zonal program, (1995-1998). Can. Tech. Rep. Fisheries Aquat. Sci., 2462.
Gauthier S., Rose G. A. (2005). Diel vertical migration and shoaling heterogeneity in Atlantic redfish: effects on acoustic and bottom-trawl surveys. ICES J. Mar. Sci. 62, 75–85. doi: 10.1016/j.icesjms.2004.10.001
Hammer Ø., Harper D. A., Ryan P. D. (2001). PAST: paleontological statistics software package for education and data analysis. Palaeontol. Electron. 4, 9.
Hannah C. G., Shore J. A., Loder J. W., Naimie C. E. (2001). Seasonal circulation on the western and central scotian shelf. J. Phys. Oceanogr. 31, 591–615. doi: 10.1175/1520-0485(2001)031<0591:SCOTWA>2.0.CO;2
Hanz U., Beazley L., Kenchington E., Duineveld G., Rapp H. T., Mienis F. (2021). Seasonal variability in near-bed environmental conditions in the Vazella pourtalesii glass sponge grounds of the scotian shelf. Front. Mar. Sci. 7. doi: 10.3389/fmars.2020.597682
Hawkes N. J., Korabik M., Beazley L. I., Rapp H. T., Xavier J. R., Kenchington E. (2019). Glass sponge grounds on the scotian shelf and their associated biodiversity. Mar. Ecol. Prog. Ser. 614, 91–109. doi: 10.3354/meps12903
Hyde J. R., Vetter R. D. (2007). The origin, evolution, and diversification of rockfishes of the genus sebastes (Cuvier). Mol. Phylogenet. Evol. 44, 790–811. doi: 10.1016/j.ympev.2006.12.026
IUCN (2021) The IUCN red list of threatened species. version 2021-3. Available at: https://www.iucnredlist.org/species/20084/9144739 (Accessed February 1, 2023).
Johnson S. W., Murphy M. L., Csepp D. J. (2003). Distribution, habitat, and behavior of rockfishes, sebastes spp., in nearshore waters of southeastern Alaska: observations from a remotely operated vehicle. Environ. Biol. Fishes. 66, 259–270. doi: 10.1023/A:1023981908146
Kleisner K. M., Fogarty M. J., McGee S., Hare J. A., Moret S., Perretti C. T., et al. (2017). Marine species distribution shifts on the U.S. northeast continental shelf under continued ocean warming. Prog. Oceanogr. 153, 24–36. doi: 10.1016/j.pocean.2017.04.001
Krieger K. J., Wing B. L. (2002). Megafauna associations with deep water corals (Primnoa spp.) in the gulf of Alaska. Hydrobiologia 471, 83–90. doi: 10.1023/A:1016597119297
Lehoux C., Plourde S., Chamberland J. M., Benoit H. (2022). Linking interannual variations of capelin abundance indices in the gulf of st. Lawrence to environmental proxies of bottom-up regulation of cohort strength. Can. Sci. Advisory Secretariat (CSAS) 2021/068. iv + 51 p.
Love M. S., Yoklavich M., Thorsteinson L. K. (2002). The rockfishes of the northeast pacific (Barkley and Los Angeles: Universty of California Press).
Magnuson-Ford K., Ingram T., Redding D. W., Mooers A.Ø. (2009). Rockfish (Sebastes) that are evolutionarily isolated are also large, morphologically distinctive and vulnerable to overfishing. Biol. Conserv. 142, 1787–1796. doi: 10.1016/j.boicon.2009.03.020
Matabos M., Bui A. O., Mihály S., Aguzzi J., Juniper S. K., Ajayamohan R. S. (2014). High-frequency study of epibenthic megafaunal community dynamics in barkley canyon: a multi-disciplinary approach using the NEPTUNE Canada network. J. Mar. Syst. 130, 56–68. doi: 10.1016/j.jmarsys.2013.05.002
Mello L. G. S., Rose G. A. (2009). The acoustic dead zone: theoretical vs. empirical estimates, and its effect on density measurements of semi-demersal fish. ICES J. Mar. Sci. 66, 1364–1369. doi: 10.1093/icesjms/fsp099
Merrick M. J., Koprowski J. L. (2017). Should we consider individual behavior differences in applied wildlife conservation studies? Biol. Conserv. 209, 34–44. doi: 10.1016/j.biocon.2017.01.021
Moran J. D. W., Arthur J. R., Burt M. D. (1996). Parasites of sharp-beaked redfishes (Sebastes fasciatus and Sebates mentella) collected from the gulf of st. Lawrence, Canada. Can. J. Fish. Aquat. Sci. 53, 1821–1826. doi: 10.1139/f96115
Morin B., Bernier B., Fréchet A. (1995). “The status of redfish in unit 1 (Gulf of st. Lawrence),” in DFO atlantic. fish. res. doc. 95/109.
Ni I.-H. (1981). “The use of vertebrae frequencies to indicate the distribution of sharp-beaked redfish, Sebastes mentella and S. fasciatus,” in NAFO SCR doc., no. 70, serial no. N354.
Oksanen J., Blanchet F. G., Friendly M., Kindt R., Legendre P., McGlinn D., et al. (2016) Vegan: community ecology package. r package version 2.4–1. Available at: https://CRAN.R-project.org/package=vegan.
Parker S. J., Olson J. M., Rankin P. S., Malvitch J. S. (2008). Patterns in vertical movements of black rockfish Sebastes melanops. Aquat. Biol. 2, 57–65. doi: 10.3354/ab00036
Planque B., Kristinsson K., Astakhov A., Bernreuther M., Bethke E., Drevetnyak K., et al. (2013). Monitoring beaked redfish (Sebastes mentella) in the north Atlantic, current challenges and future prospects. Aquat. Living Resour. 26, 293–306. doi: 10.1051/alr/2013062
Senay C., Ouellette-Plante J., Bourdages H., Bermingham T., Parent G., Chabot D., et al. (2021). Unit 1 redfish (Sebastes mentella and S. fasciatus) stock status in 2019 and updated information on population structure, biology, ecology, and current fishery closures (pp. xi+-119). Can. Sci. Advisory Secretariat 2021/015. xi + 119 p.
Sévigny J. M., Méthot R., Bourdages H., Power D., Comeau P. (2008). “Review of the structure, the abundance and distribution of Sebastes mentella and S. fasciatus in Atlantic Canada” in a species-at-risk context. Can Sci Advis Sec, Res (Fisheries and Oceans Canada), Doc 2007/085.
Stierhoff K. L., Mau S. A., Murfin D. W. (2013). “A fishery-independent survey of cowcod (Sebastes levis) in the southern CA bight using a remotely operated vehicle (ROV),” in US Dep. Commer. NOA technical memorandum NMSF, vol. 520.
Stone R. P. (2006). Coral habitat in the Aleutian islands of Alaska: depth distribution, fine-scale species associations, and fisheries interactions. Coral reefs 25, 229–238. doi: 10.1007/s00338-006-0091-z
Thurow R. F., Dolloff C. A., Marsden J. E. (2012). “Visual observation of fish and aquatic habitat”, in Fisheries and Oceans Canada Eds. Zale A. V., Parrish D. L., Sutton T. M. (Bethesda, MD: American Fisheries Society), 781–817.
Tissot B. N., Yoklavich M. M., Love M. S., York K., Amend M. (2006). Benthic invertebrates that form habitat on deep banks off southern California, with special reference to deep sea coral. Fish. Bull. 104, 167–181.
Uiblein F., Lorance P., Latrouite D. (2003). Behaviour and habitat utilisation of seven demersal fish species on the bay of Biscay continental slope, NE Atlantic. Mar. Ecol. Prog. Ser. 257, 223–232. doi: 10.3354/meps257223
Urrego-Blanco J., Sheng J. (2014). Study on subtidal circulation and variability in the gulf of st. Lawrence, scotian shelf, and gulf of Maine using a nested-grid shelf circulation model. Ocean Dyn. 64, 385–412. doi: 10.1007/s10236-013-0688-z
Valentin A. E., Power D., Sévigny J. M. (2015). Understanding recruitment patterns of historically strong juvenile year classes in redfish (Sebastes spp.): the importance of species identity, population structure, and juvenile migration. Can. J. Fish. Aquat. Sci. 72, 774–784. doi: 10.1139/cjfas-2014-0149
Valentin A., Sévigny J. M., Morin B. (2006). Extensive sampling and concomitant use of meristic characteristics and variation at the MDH-a* locus reveal new information on redfish species distribution and spatial pattern of introgressive hybridization in the Northwest Atlantic. J. Northw. Atl. Fish. 36, 65–80.
Voronin V. P., Nemova N. N., Ruokolainen T. R., Artemenkov D. V., Rolskii A. Y., Orlov A. M., et al. (2021). Into the deep: new data on the lipid and fatty acid profile of redfish sebastes mentella inhabiting different depths in the irminger Sea. Biomolecules 11, 704. doi: 10.3390/biom11050704
Wardle C. S. (1986). ““Fish behaviour and fishing gear”,” in The behaviour of teleost fishes. Ed. Pitcher T. J. (Boston, MA: Springer), 463–495.
Wilborn R. E., Spies I., Goddard P., Rooper C. N., Orr J. W. (2022). First observation of the use of coral habitat by larval northern rockfish (Sebastes polyspinis) in the western gulf of Alaska. Fish. Bull. 36, 75–78. doi: 10.7755/FB.120.1.7
Keywords: Sebastes, rockfish, behavior, rhythms, habitat use, environmental drivers, lander, monitoring
Citation: Grinyó J, Aguzzi J, Kenchington E, Costa C, Hanz U and Mienis F (2023) Occurrence and behavioral rhythms of the endangered Acadian redfish (Sebastes fasciatus) in the Sambro Bank (Scotian Shelf). Front. Mar. Sci. 10:1158283. doi: 10.3389/fmars.2023.1158283
Received: 03 February 2023; Accepted: 03 April 2023;
Published: 21 April 2023.
Edited by:
Stefanie Kaiser, Senckenberg Research Institute and Natural History Museum Frankfurt, GermanyReviewed by:
Sarah Brown-Vuillemin, Université du Québec à Rimouski, CanadaBrian Linton, Northeast Fisheries Science Center (NOAA), United States
Copyright © 2023 Grinyó, Aguzzi, Kenchington, Costa, Hanz and Mienis. This is an open-access article distributed under the terms of the Creative Commons Attribution License (CC BY). The use, distribution or reproduction in other forums is permitted, provided the original author(s) and the copyright owner(s) are credited and that the original publication in this journal is cited, in accordance with accepted academic practice. No use, distribution or reproduction is permitted which does not comply with these terms.
*Correspondence: Jordi Grinyó, jordi.grinyo@nioz.nl; jordigrinyo85@gmail.com