- 1Research Institute for Basic Science, Chonnam National University, Gwangju, Republic of Korea
- 2Division of Microbiology, Honam National Institute of Biological Resources, Mokpo-si, Jeollanam-do, Republic of Korea
- 3Department of Oceanography, Chonnam National University, Gwangju, Republic of Korea
Rising seawater temperatures are causing geographic shifts in the composition and abundance of the marine plankton that make up the microbial food web. Over the past few years, the water temperature around the Korea Strait has been increasing rapidly. Twelve cruise surveys were conducted in the Jeju Strait between 2017 and 2019 to monitor the presence of the tropical dinoflagellate genera Ornithocercus and Triposolenia, which are brought in by the Jeju Warm Current. Additionally, passive tracer experiments were performed using the Regional Ocean Modeling System to understand how the warm currents impact the Jeju Strait. The results of these simulations were then compared against the distribution ranges and abundance of Ornithocercus and Triposolenia. The study found that there were significant seasonal variations in abundance and spatial distribution of the tropical dinoflagellates during the sampling period, particularly during September and November when they were highly abundant and widely distributed. These patterns were closely related to the intensity and path of the Jeju Warm Current, particularly from southeastern offshore (EKB02; Eastern Kuroshio Branch02) rather than from southwestern offshore (EKB01; Eastern Kuroshio Branch01) of Jeju Island. The study also found no tropical dinoflagellates at any of the stations near the south coast of Korea during the sampling period. These findings suggest that the Ornithocercus and Triposolenia tropical dinoflagellate genera may serve as useful biological indicators to monitor the advection of warm currents into the Jeju Strait, Korea.
Introduction
Dispersal is a fundamental component of the ecological and evolutionary process whereby an individual or multiple individuals move away from their birthplace to another location (Croteau, 2010). The dispersal of individuals can be either active or passive. Active dispersal, which is more common in animals, is where the animal redistributes itself through its own actions, while passive dispersal, which is more common in plants, is where organisms require assistance to move to new habitats (Putman and Mansfield, 2015). In marine environments, many microorganisms are expected to have a relatively broad distribution range because they are small in size and are affected by numerous atmospheric and oceanographic parameters (Finlay, 2002; Palumbi, 2003; Smayda, 2007). Therefore, understanding the dynamics of a geographic distribution range is essential for understanding biogeography and ecological niche (Crickenberger, 2014).
Since the mid-1970s, there has been increasing research on the effect of global climate change, particularly regarding the effect of increasing seawater temperatures on marine species (Cushing and Dickson, 1976; Southward et al., 1995; Intergovernmental Panel On Climate Change, 2007; Jung et al., 2017). An increase in seawater temperature can significantly affect the distribution of marine species by either widening or narrowing their range (Poloczanska et al., 2008). In addition, it can lead to changes in species composition, which is directly related to changes in the structure and function of the various marine ecosystems (Poff et al., 2002; Doney et al., 2012). The marginal seas surrounding the Korean peninsula are also affected by global climate change and marine ecosystems are gradually switching from temperate to tropical and/or subtropical conditions. The mean seawater temperature surrounding Korea has already increased by as much as 1.7°C in the East Sea, 1.4°C in the Korean Strait, and 0.3°C in the Yellow Sea over the past half century (KMA, 2019). Along with the increase in seawater temperature caused by changes in atmospheric circulation (Yeh and Kim, 2010), and the northward expansion of the Kuroshio Current with high salinity and temperature (Pang et al., 1996), the occurrence and habitats of tropical and/or subtropical marine organisms (e.g., fish, invertebrates, and macroalgae) surrounding the Korean peninsula are expanding northwards (Kim, 1992; Ju and Kim, 2012; Kim et al., 2020).
Lie and Cho (2016) reviewed the system of currents in the Yellow and East China Seas (YECS) and shows that the Kuroshio Current consists of the Western Kuroshio Branch (WKB) off the northeast coast of Taiwan, and the Eastern Kuroshio Branch (EKB) off the southwest coast of Kyushu, Japan (Figure 1). The EKB, which flows northward toward the Korea Strait (KS) divides into three further branches off western Kyushu: one turns clockwise to flow southwestern coast of Kyushu, a second flows northward to the Korea Strait and is known as the Tsushima Warm Current (TWC), and the third flows northwestward to the south and west of Jeju Island. A portion of the TWC flows northwestward and turns clockwise around Jeju Island, which is called as the Jeju Warm Current (JWC). The TWC and JWC bring warmer, saltier water from the East China Sea (ECS) to the East Sea through the Korea Strait and the Jeju Strait (Lie and Cho, 1997; Chang et al., 2004). The Jeju Strait is located between the southwestern coast of Korean peninsula and Jeju Island. This region is known to be affected by the variability of the JWC and is directly affected by increase in seawater temperature. The currents through the Jeju Strait have been investigated using a variety of methods (e.g. drift bottle, satellite-tracked drifter, short-term current meter observations, drift plate tracking, and acoustic Doppler current profilers) (Lee, 1983; Kim and Rho, 1997; Lie et al., 2000; Suk et al., 2000; Pang et al., 2003; Shin et al., 2022), and it is estimated that the temperature and salinity distribution range in the Jeju Strait varies greatly depending on the season. Because of the inflow of the JWC, it would be easy to observe tropical and/or subtropical marine microorganisms that are introduced into the Jeju Strait. While several studies have investigated phytoplankton community temporally and spatially in coastal sites very close to the Jeju Island (e.g., Lee et al., 1990; Lee et al., 1993; Choa and Lee, 2000; Kim et al., 2019), unfortunately, none has been done in the Jeju Strait until now, thereby making it difficult to understand seasonal succession pattern on phytoplankton community in the Jeju Strait.
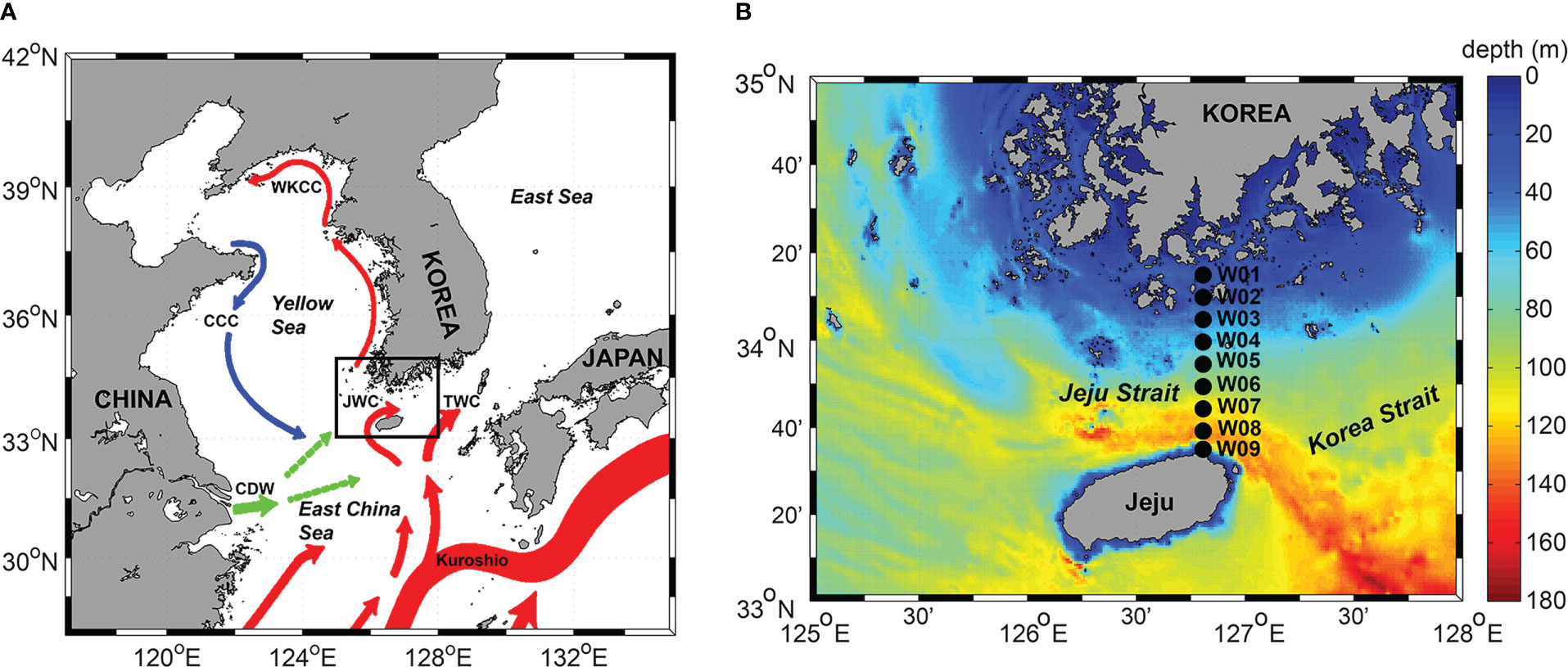
Figure 1 (A) Map of the study area (black solid rectangle) along with surface ocean currents system (colored vector; Park et al., 2017). Blue lines indicate cold currents and red lines indicate warm currents. Green lines indicate Changjiang Diluted Water (CDW). CCC, Chinese Coastal Current; WKCC, West Korea Coastal Current; JWC, Jeju Warm Current; TWC, Tsushima Warm Current. (B) Map of the study area showing the sampling sites. Black circles represent the sampling sites in the Jeju Strait during this study (W01 – W09).
The heterotrophic dinoflagellates of the order Dinophysiales, (which include Amphisolenia, Histoneis, Ornithocercus, Parahistoneis, and Triposolenia) are widespread in tropical and subtropical marine environments (Hallegraeff and Jeffrey, 1984; Hernández-Becerril et al., 2008; Saifullah et al., 2008; Tarangkoon et al., 2010; de Aquino et al., 2014; Lavanya et al., 2020) and are characterized by having symbiotic cyanobacteria and eukaryotes (Nakayama et al., 2019; Kim et al., 2021). If these dinoflagellates are therefore beginning to be found in new oceanic environments such as temperate regions, it would mean that those regions are becoming suitable habitats for them as a result of environmental changes such as increased seawater temperature. Indeed, tropical dinoflagellates can now be observed off Jeju Island all year round (Kim et al., 2008; Jung and Kim, 2013; Kim et al., 2013; Lee et al., 2015; Kim et al., 2021), implying that it can no longer be considered a temperate region. In this context, a growing concern is the extent to which these (sub) tropical dinoflagellates are expanding northwards in coastal waters around Korea. Their northward expansion range could be caused by an increase in seawater temperature because of global warming, thereby the subsequent adaptation of the introduced dinoflagellates to new oceanic environments, or changes in intensity and range of warm currents influencing Korean coastal waters and accompanying the introduction of the dinoflagellates, or both, although the exact reason for the expansion remains unknown.
This study aimed to investigate the spatiotemporal distribution of species belonging to two (sub) tropical dinoflagellates genera, Ornithocercus and Triposolenia, in the Jeju Strait off the south coast of Korea and then to determine whether their distribution is related to the intensity and path of the JWC, a branch of TWC originating from the Kuroshio Current. To this end, we determined two (sub) tropical dinoflagellates abundance in March, June, September, and November for a three-year period from 2017 to 2019, and simulated the spatiotemporal distribution of warm current using dye release experiments to determine whether their occurrence is related to the flow of warm currents through the Jeju Strait.
Materials and methods
Study area and sampling strategy
The study was carried out at nine stations (W01 – W09) between Wando (located off the south coast of Korea) and Jeju Island in March, June, September, and November 2017, 2018, and 2019 (Figure 1; Supplementary Table S1). Vertical profiles of seawater temperature and salinity were measured using a Conductivity-Temperature-Depth sensor (SBE 19plus V2, Sea-Bird Electronics, USA) mounted on a rosette sampler. Plankton samples were hauled vertically through the water column from a depth of 30 m (which corresponded to a thermocline depth, if present) to the surface using a 0.6 m diameter bongo net with a 20 µm mesh. At the center of the net mouth, a digital flowmeter (Hydro-Bios, Germany) was mounted to determine the volume of the seawater passing through the net. Highly concentrated samples were immediately fixed with neutral Lugol’s solution (final concentration 2%) in a 500 ml polyethylene bottle and wrapped with aluminum foil for microscopic observation.
Identification and abundance of tropical dinoflagellates
In the laboratory, the 10 – 50 mL subsamples were withdrawn from each fixed sample and transferred to either 60 mm petri dishes (SPL Life Sciences, Korea) or plant culture dishes (SPL Life Sciences, Korea). These dishes were used for species identification and enumeration of all tropical Ornithocercus and Triposolenia dinoflagellates using an inverted microscope (Axio Vert.A1, ZEISS, Germany) equipped with a full HD mini-box camera (MediCAM-Z, Comart System, Korea) at 200 or 400x magnifications. Ornithocercus and Triposolenia species were identified in line with the studies by Abe (1967) and Taylor (1971), and the species names were followed by Gómez (2005). Some species were not able to be classified because of a lack of micrographs or uncertain cell shapes, so they were assigned as either “Ornithocercus sp.” or “Triposolenia sp.”, respectively. Cell abundance (cells·m-3) was calculated based on the cell count data and the flowmeter revolutions.
Model configuration and dye release experiment
In this study, the Regional Ocean Modeling Systems (ROMS), which is a free-surface ocean model, was used to study the possibility of transport of tropical dinoflagellates to the northern Jeju Strait by the northward expansion of the JWC (Song and Haidvogel, 1994; Shchepetkin and Mcwilliams, 2005). The Boussinesq and hydrostatic approximations were used and the orthogonal curvilinear and stretched terrain-following coordinates were adopted as the horizontal and vertical coordinates, respectively. In the numerical model, the split-explicit time stepping method was used, which is efficient for the time integration of the equations because the barotropic and baroclinic modes are calculated separately. This model has a horizontal grid resolution of approximately 1/100° and its vertical grids consist of 41 layers. For the bottom topography data, the Korbathy30s (Seo, 2008) is used in the tilted rectangular model domain. The depth of the water greater than 120m in the Jeju Strait and become shallower toward the south coast of Korea. Open boundary data including temperature, salinity, currents, and sea level are supplied by the Mercator Ocean global 1/12° system PSY4V3R1, which was provided by Copernicus Marine Environment Monitoring Service (Lellouche et al., 2018; CMEMS, http://marine.copernicus.eu/). The ERA-interim reanalysis data were used as daily mean atmospheric forcing data including wind, relative humidity, air temperature, pressure, and solar radiation, and these were provided by the European Centre for Medium-Range Weather Forecasts (ECMWF, https://www.ecmwf.inf). Heat flux calculations at the ocean surface boundary were performed using the bulk formulation (Fairall et al., 2003). Also, the ten tidal constituents (M2, S2, N2, K2, K1, O1, P1, Q1, Mf, and Mm) were provided by the Topex/Poseidon version 6 barotropic tidal model (TPXO6; Egbert and Erofeeva, 2002), which is applied for the propagation of tides from the open boundary to the model interior. The initial condition of this model was obtained from a spin-up simulation of ocean circulation for two years using atmospheric forcing and open boundary data from 2016.
Dye release experiments have previously been useful in tracing the transport path of the zooplankton and water masses (Keister et al., 2011; White, 2015). For the dye release experiment in this study, a multidimensional positive definite advection transport algorithm was applied to the tracer advection method, while the third-order upstream scheme and the fourth-order centered scheme were applied to the horizontal and vertical advection schemes for momentum, respectively. The K-profile parameterization (KPP) scheme was used to calculate the vertical mixing in the numerical model (Large et al., 1994). The equation for the advection and diffusion of a dye is as follows:
where, C represents the passive tracer concentration, and Ah and Av represents the horizontal and vertical diffusivities, respectively. The second term on the left-hand side represents the advection term, while on the right-hand side, the first and the second terms represent horizontal and vertical diffusion terms, respectively. τ represents the decay timescale of the tracer and Q indicates the source of the tracer supplied into the model domain.
To identify the inflow regions of warm water flowing into the Korea Strait from the northwestern Pacific, the vertical distribution of the temporal mean v-velocity along the southern open boundary from 2017 to 2019 was examined (Figure 2). The two strong v-velocity cores with current speed higher than 0.1 m·s-1 were exhibited over the entire depth from 126°E to 128°E, respectively (Figure 2B). The regions where the two v-velocity cores appeared were similar to the regions of the two EKB branches defined by Lie and Cho (2016), which are named EKB01 and EKB02, in this study. EKB01 and EKB02 are main pathways of the JWC and the current of the TWC, respectively. For the dye release experiment, dyes at concentrations of 10 kg·m-3 were injected over the entire depth profile of the EKB01 and EKB02 along the southern open boundary into the model interior at every time step from 2017 to 2019 (Figure 2A).
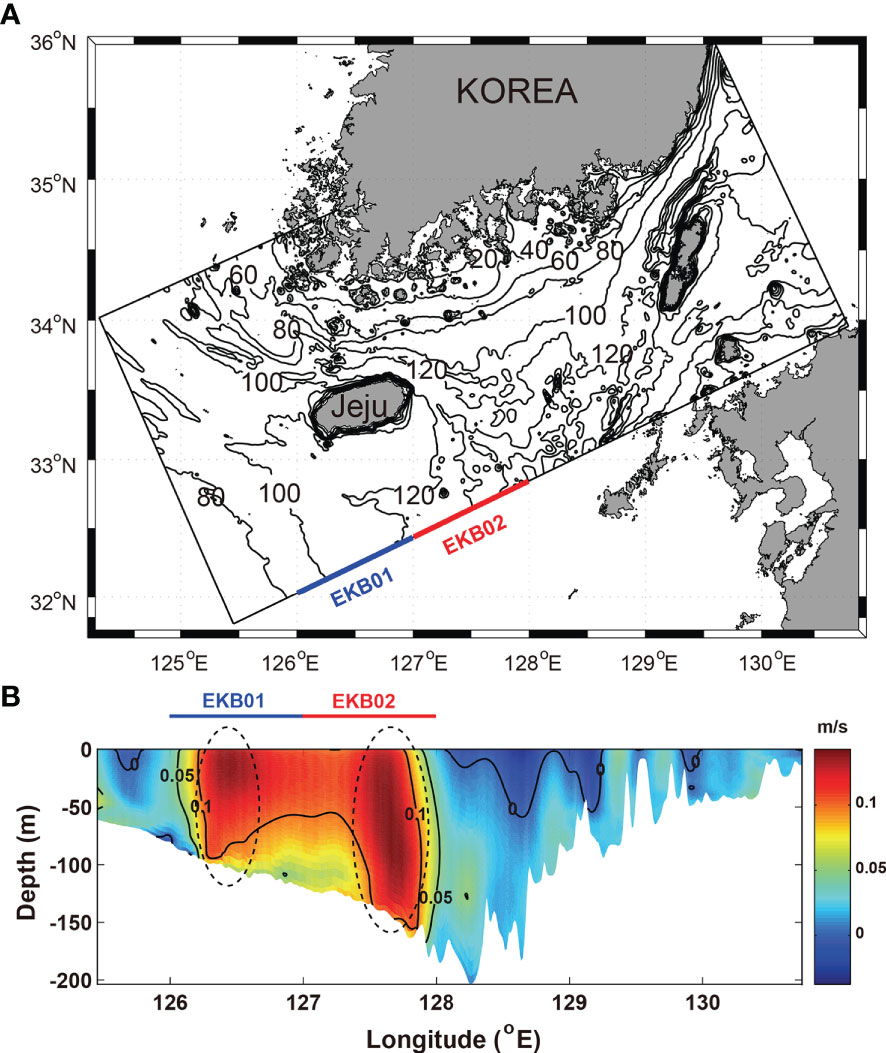
Figure 2 (A) Model domain and bathymetry and (B) vertical distribution of temporal mean v-velocity in the southern open boundary from 2017 to 2019.
Data analysis
To analyze the relationship between total cell abundance of Ornithocercus and Triposolenia and concentration of dyes originating from EKB01 and EKB02, the data were analyzed or processed using the SigmaPlot (Version 13, Systat Software Inc., San Jose, CA, USA).
Results
Microscopic observations of the Ornithocercus and Triposolenia
A total of nine species from two genera were identified during this sampling period. The majority (seven species) belonged to the genus Ornithocercus within the family Dinophysiaceae and had cyanobacterial ectosymbionts. The species were O. heteroporoides, O. magnificus, O. quadratus, O. cf. skogsbergii, O. splendidus, O. steinii, and O. thumii. The remaining two species had endosymbionts and belonged to the genus Triposolenia within the family Amphisoleniaceae. The species were T. bicornis and T. cf. depressa (Figure 3).
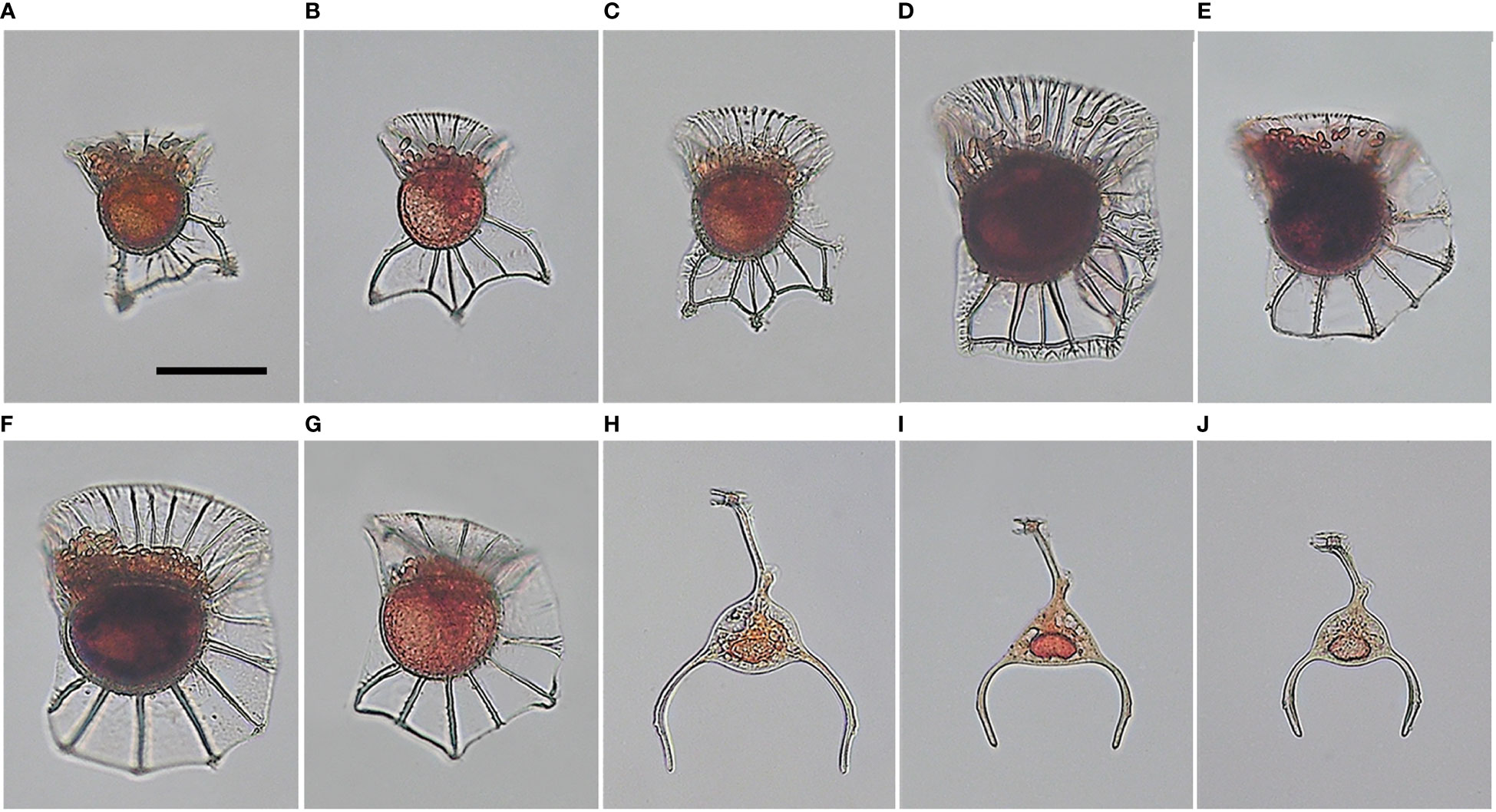
Figure 3 Light micrographs of tropical dinoflagellates Ornithocercus and Triposolenia cells from field samples. (A), Ornithocercus heteroporoides; (B, C), O. magnificus; (D) O. quadratus; (E), O. cf. skogsbergii; (F), O. steinii; (G), O. thumii; (H), Triposolenia bicornis; (I, J), T. cf. depressa. Scale bars = 50 µm.
All Ornithocercus spp. cells observed in this study had the typical morphological features of Ornithocercus, and rod-shaped cyanobacterial symbionts were observed inside the girdle list (Figures 3A–G). Unfortunately, O. splendidus was lost during observation under the microscope, so a micrograph could not be obtained. The Triposolenia species were observed to have endosymbionts typical of the genus (Figure 3H–J). Although their morphology was similar between the species, there were differences in size. Overall, T. bicornis was larger than T. cf. depressa (140 and 100 µm, respectively).
Seasonal fluctuation in the abundance of Ornithocercus and Triposolenia
The abundance of the tropical dinoflagellates Ornithocercus and Triposolenia cells showed large seasonal variations. During the study period, Ornithocercus spp. were observed more frequently than Triposolenia. The abundance of tropical dinoflagellates Ornithocercus and Triposolenia averaged 742 and 506 cells·m-3, respectively. Ornithocercus species were observed throughout the entire duration of the sampling period, regardless of the season, and in water temperatures of 12.4 – 24.5°C and salinities of 31 – 34.6 (Figure 4A). By comparison, Triposolenia spp. occurred only in March and November and in water temperatures of 15.2 – 20.2°C and salinities of 33.4 – 34.2 (Figure 4B).
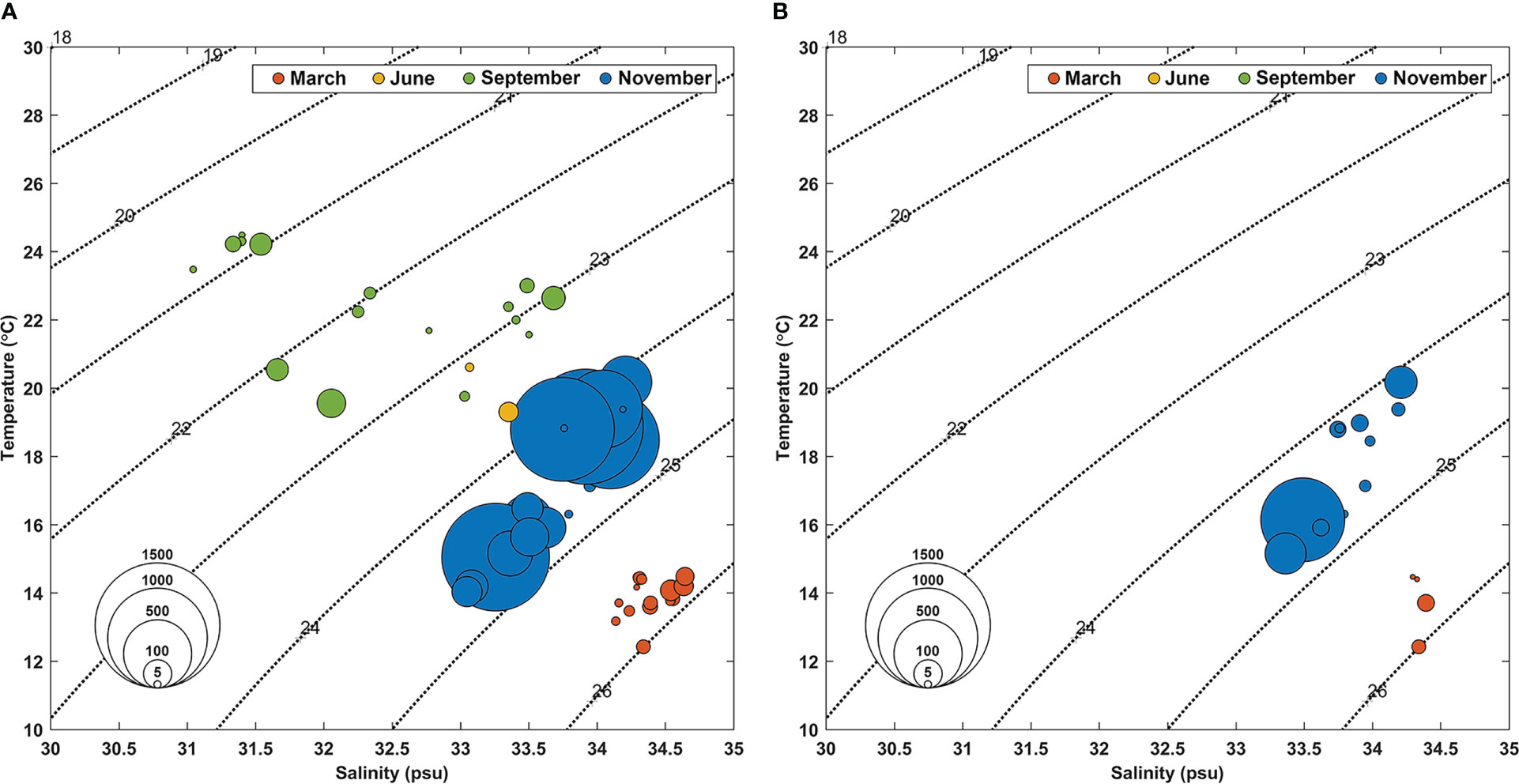
Figure 4 Temperature-salinity diagram showing the abundance of Ornithocercus (A) and Triposolenia species during this study (B). Circle sizes are proportional to the abundance (cells·m-3). The colors of the circles indicate each sampling month. Red: March, Yellow: June, Green: September, Blue: November.
Cell abundance of Ornithocercus spp. was highly variable and ranged from undetectable levels to 4818 cells·m-3 in November 2018 (Figure 5A). Abundances were either negligible or undetectable in March and June, before starting to gradually increase from September and reaching a peak in November. Similar trends were seen for each of the three years. Ornithocercus species diversity was also highest in November (five or six species) and lowest in March and June (two or three species). Among them, O. magnificus was the most abundant, accounting for about half of the total cell abundance in almost all sampling times, and accounted for 100% in March 2019, although the total cell abundance was low (Figure 5A). Surprisingly, however, O. magnificus cells were absent in June 2018 and represented less than half of the total Ornithocercus abundance in September 2017 and 2018. The second most common species was O. thumii, which had the highest abundance at 85.7% in June 2018, although its mean abundance was 17.7% over the study period. Some Ornithocercus species only occurred at certain times. For example, O. heteroporoides and O. splendidus occurred only in November 2018, while O. cf. skogsbergii was observed in September and November but not in March.
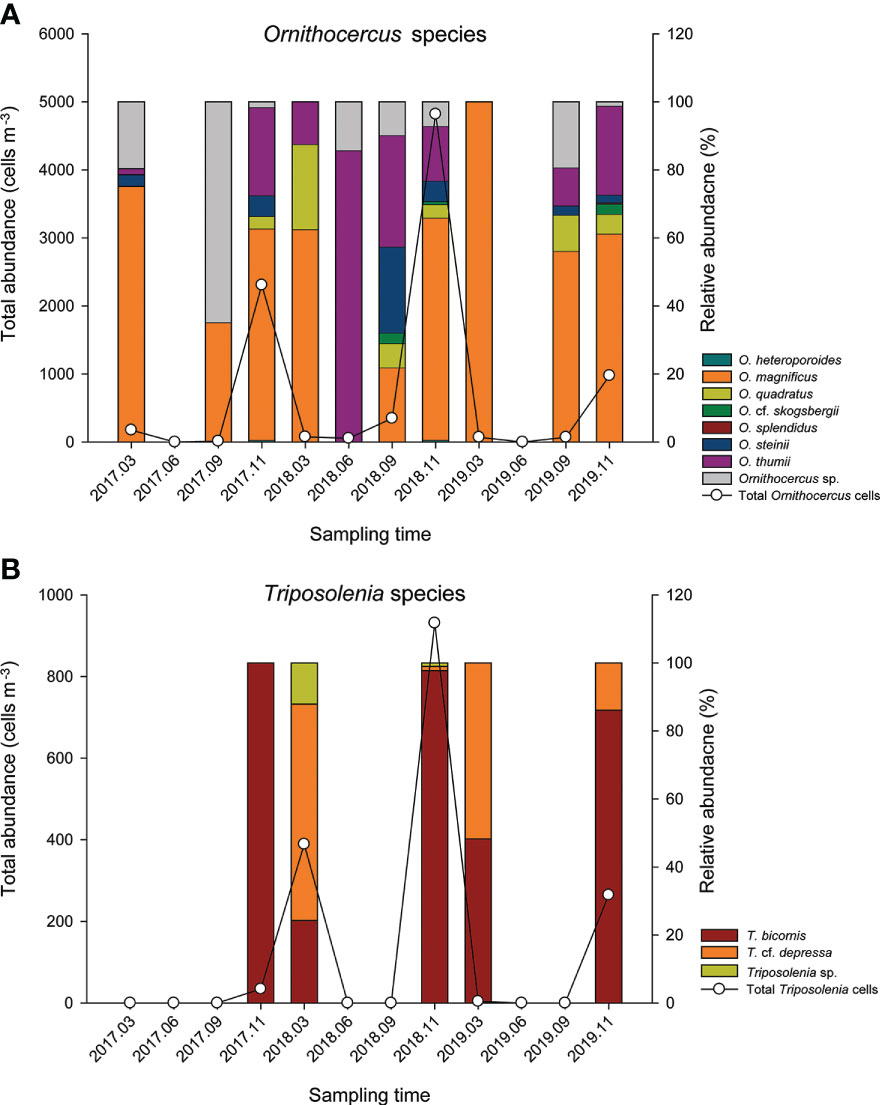
Figure 5 Total abundance of the Ornithocercus (A) and Triposolenia species (B) as functions of sampling dates. Absolute total number (lines, left y-axis scale) and proportion (bar, right y-axis scale) of two genera. Bar colors indicate each species within a genus.
Triposolenia cells were only observed in March and November, ranging from undetectable levels to 931 cells·m-3 in November 2018 (Figure 5B). Although the abundance of Triposolenia species was attributed to either T. bicornis or T. cf. depressa, T. bicornis was only relatively predominant in their abundance in November (>86% of total Triposolenia cells), while T. cf. depressa cells were highest in March (64 and 52% in 2018 and 2019, respectively), excluding 2017 (Figure 5B).
Spatial distribution of Ornithocercus and Triposolenia
The sea surface temperature (SST) supplied by Operational Sea Surface Temperature and Ice Analysis (OSTIA; Donlon et al., 2012) showed a remarkable seasonal change between 10.8 and 27.3°C in the study area. SST ranged from 11.1 to 14.5°C in March, 14.7 – 20.8°C in June, 19.6 – 25.5°C in September, and 14.0 – 20.2°C in November. There was a large difference in SST depending on the sampling stations. The salinity ranged from 29.6 to 34.7 during the sampling period, with the mean salinity in March, June, September, and November being 34.4, 33.3, 32.2, and 33.7, respectively.
The spatial distribution of Ornithocercus showed a pronounced seasonal variation (Figure 6), displaying the widest spatial distribution along the transect in November (Figures 6J–L). In November, they were found from station W09 adjacent to Jeju Island to station W02 close to the south coast of Korea. Their maximum abundance (1298 cells·m-3) was observed in 2018 at station W06 located in the middle of the transect (Figure 6K). In March, they were found from stations W09 to W03, with peak abundance (53 cells·m-3) observed at station W05 in 2017 (Figure 6A). No cells were found in June 2017 and 2019 (Figures 6D, F), but small populations (8 and 47 cells·m-3) were observed at stations W08 and W09 adjacent to Jeju Island in June 2018 (Figure 6E). Their spatial distribution in September was usually similar to that in November, although they were less abundant in September. Interestingly, however, the pattern of spatial distribution observed in September 2017 differed from that in September 2018 and 2019. In September 2017, they were only observed at stations W03 and W06 located in the middle of the transect (Figure 6G). Triposolenia cells had a narrow spatial distribution compared with Ornithocercus. For example, in March, Ornithocercus cells were found between stations W03 and W09, whereas Triposolenia cells were observed in a relatively narrow range, from stations W03 to W07 in 2018 and only W06 and W08 in 2019 (Figures 6B, C). Even in November, Triposolenia cells were distributed over a narrow range from stations W03 to W05, which were located in the middle of the transect (Figures 6J–L).
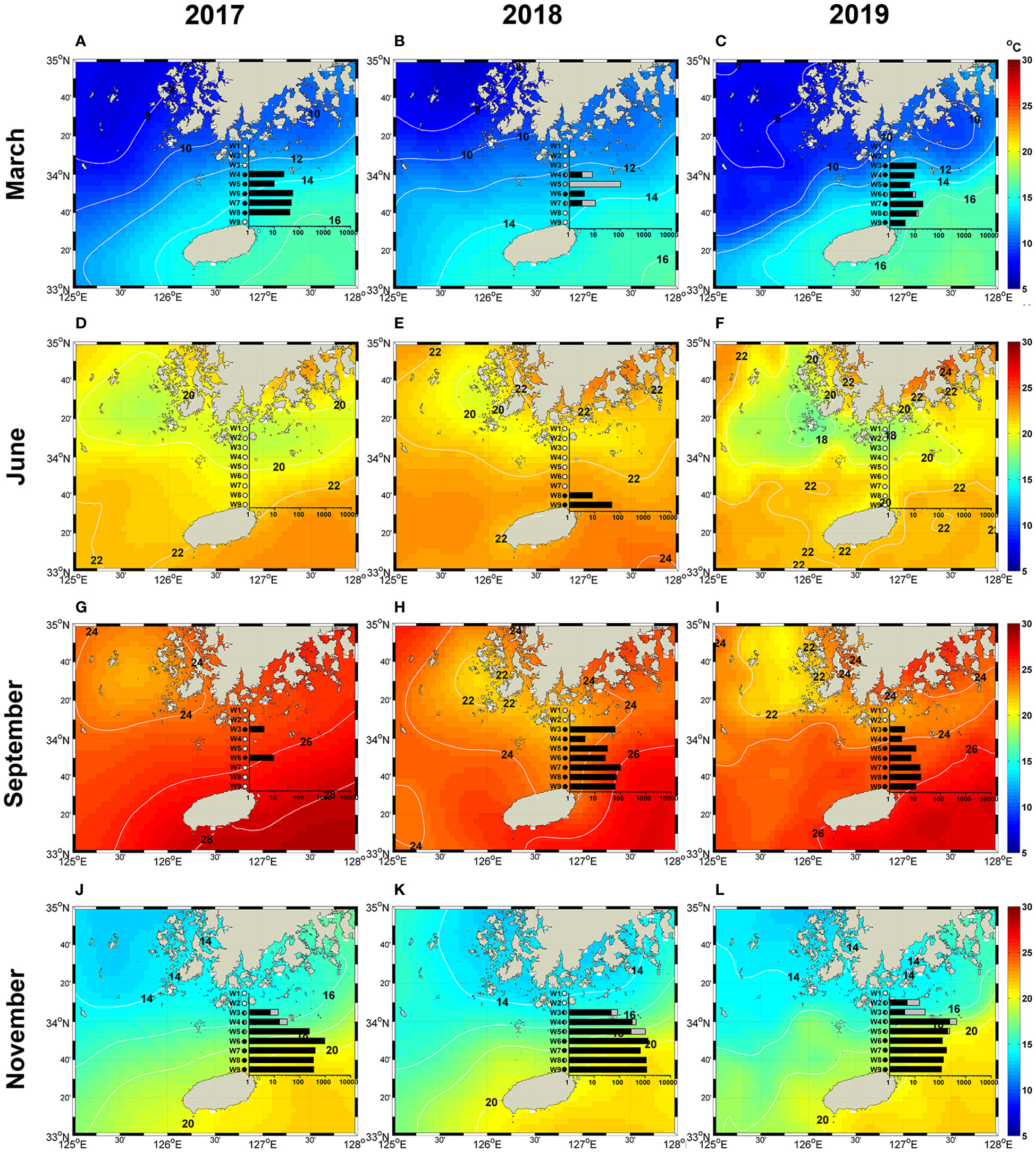
Figure 6 Spatial distribution of the total cell abundance of Ornithocercus (Black bars) and Triposolenia (Gray bars) at each sampling station during the sampling period, superimposed on the sea surface temperature supplied from Operational Sea Surface Temperature and Ice Analysis (OSTIA; Donlon et al., 2012). (A–C), March; (D–F), June; (G–I), September; (J–L), November.
Relation between the tropical dinoflagellates and the inflow of tropical water mass
The advection and diffusion of dyes from the surface to 30 m depth injected from regions of EKB01 and EKB02 to the model interior from 2017 to 2019 were compared in Figures 7, 8. In this experiment, dyes originating from EKB01 and EKB02 were distributed in the regions of both warm currents which affected significantly to advection of dyes. Additionally, the paths of warm current from EKB01 and EKB02 had interannual and seasonal variations, which led to temporal and spatial differences in dye distribution (Figures 7, 8). Overall, the dye originating from EKB01 highly affected the southwestern and southern shallow coastal regions of Korea as well as the Jeju Strait (Figure 7), while dye originating from EKB02 flowed predominantly to the southeast of Jeju Island through the Korea Strait (Figure 8). Although the dye originating from EKB01 dispersed from the East China Sea to the southern shallow coastal regions through the Jeju Strait with seasonal variations, the concentration of dye originating from EKB01 was relatively low around Jeju Island in September 2018 and in November for every year while the dye originating from EKB02 predominated in this region (Figures 7, 8). In September 2018, dyes originating from EKB01 did not flow close to the northern coast of Jeju Island because of the northwestward intrusion of the dye originating from EKB02, which pushed up the dyes originating from EKB01 to the southwestern shallows in the Jeju Strait (Figures 7H, 8H). At that time, in the southeastern offshore of Jeju Island, the northwestward flowing current from EKB02 was stronger than it was in September 2017 and 2019. It flowed along the southern and southwestern coast of Jeju Island, causing advection of the dye originating from EKB02 into the Jeju Strait (Figures 7H, 8H). Moreover, the total cell abundance of Ornithocercus and Triposolenia was also larger in September 2018 compared with September 2017 and 2019 (Figure 6H). In November each year, most of the dye originating from EKB01 flowed southwestern offshore to northwest off Jeju Island before being advected to the Yellow Sea (Figures 7J–L). Therefore, instead of the dyes originating from EKB01, the dyes originating from EKB02 moved around Jeju Island (Figures 8J–L).
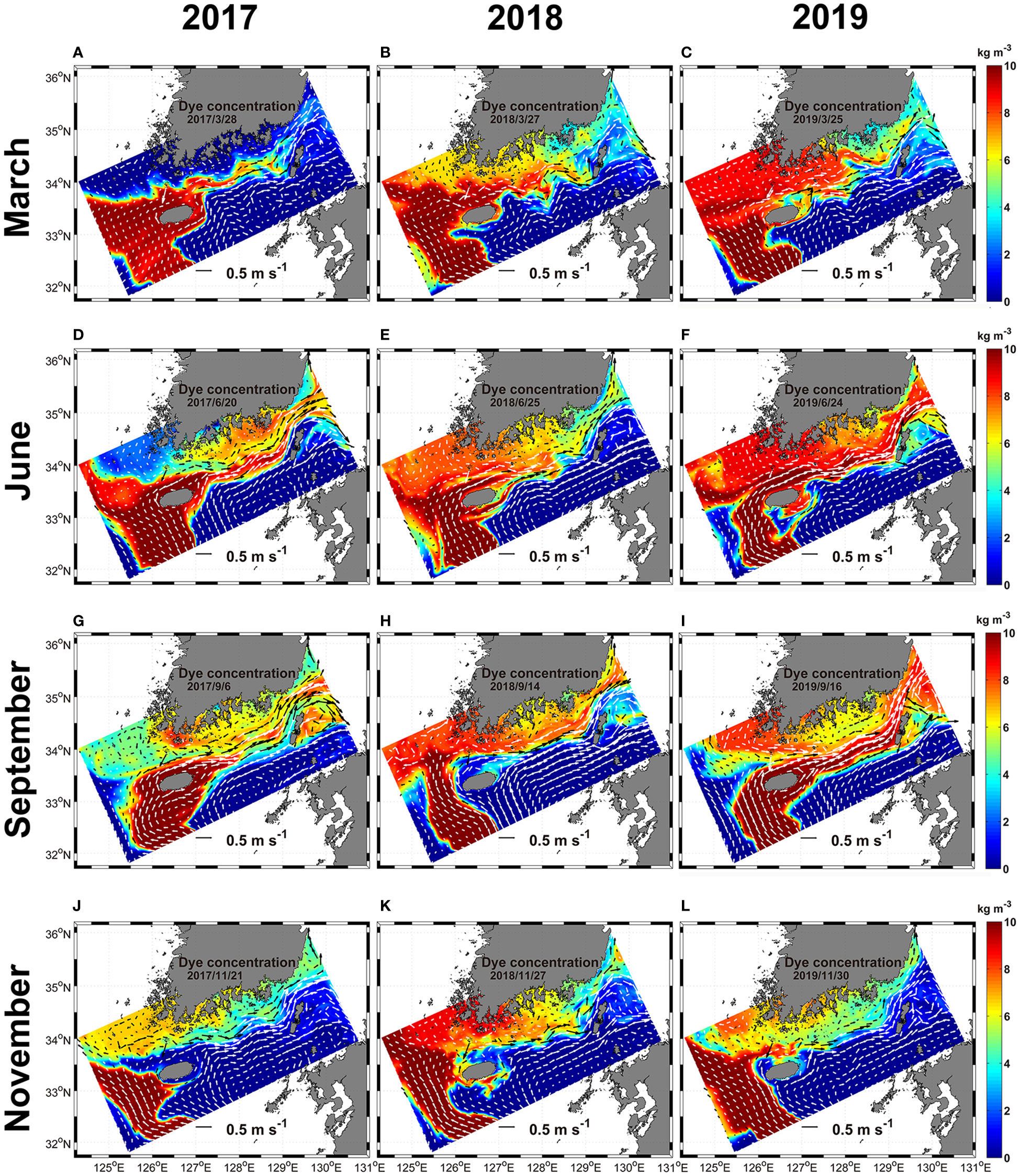
Figure 7 The horizontal distribution of the dye originated from EKB01 from the surface to 30 m depth from 2017 to 2019. White and black arrows, which are represented in two colors to make visible clearly, indicate ocean current vectors. (A–C), March; (D–F), June; (G–I), September; (J–L), November.
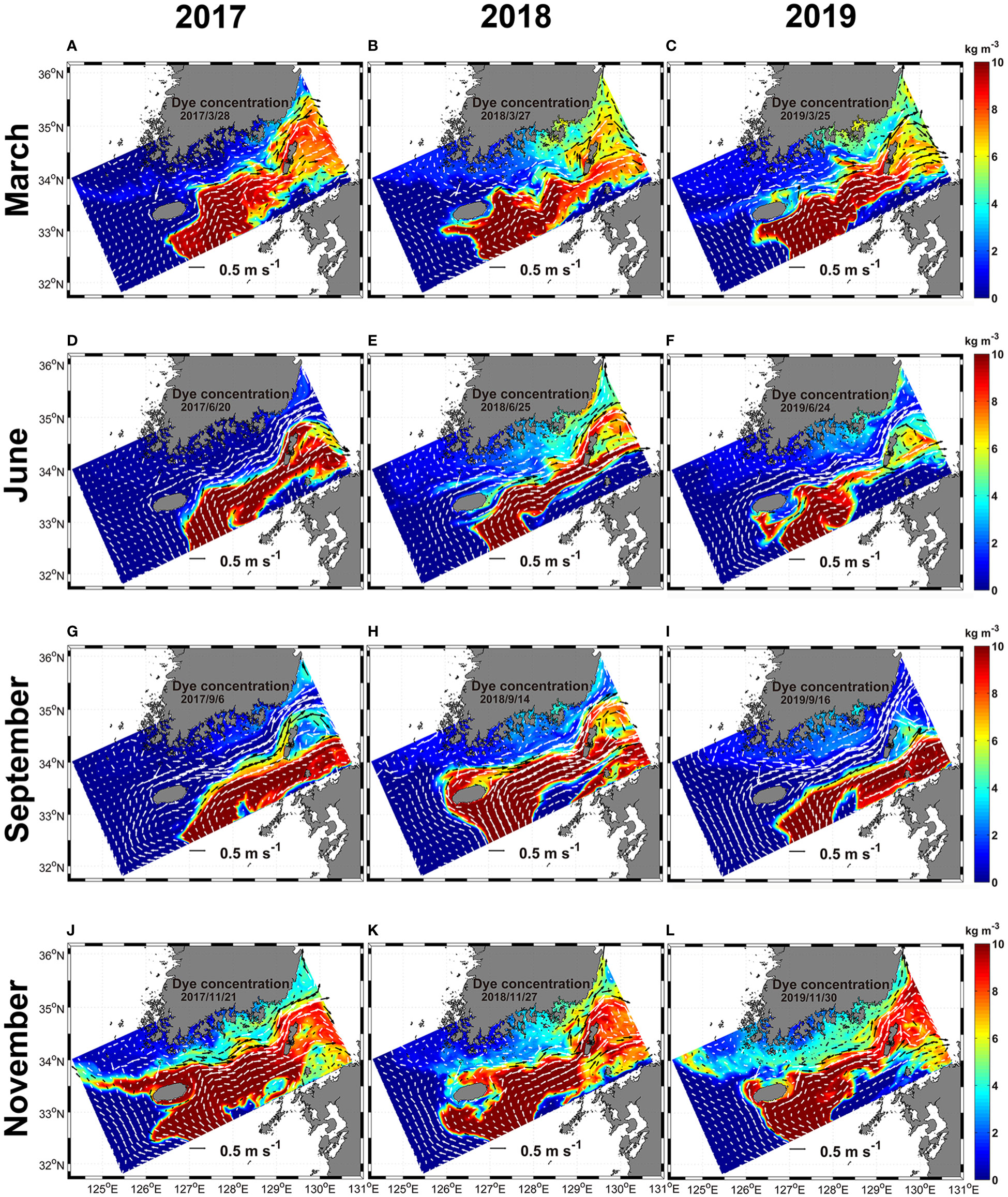
Figure 8 The horizontal distribution of the dye originated from EKB02 from the surface to 30 m depth from 2017 to 2019. White and black arrows, which are represented in two colors to make visible clearly, indicate ocean current vectors. The horizontal current is equal to it in Figure 7. (A–C), March; (D–F), June; (G–I), September; (J–L), November.
Hovmöller diagrams showing the concentration of the dyes originating from EKB01 and EKB02 at the sampling sites in the Jeju Strait also showed similar variations (Figures 9, S2). The concentrations of the dyes originating from EKB01 and EKB02 offset each other. In particular, the dye originating from EKB01 mainly appeared close to Wando, whereas dye originating from EKB02 was abundant at W05 to W09 where the tropical dinoflagellates were mainly observed. During the study period, these two pathways were clearly separate in autumn and late winter. However, during the summer of 2018, dye originating from EKB02 showed short-term fluctuations that were greater than they were during the summers of 2017 and 2019 (Figure 9E). At that time, the total abundance and spatial distribution of the tropical dinoflagellates in June and September 2018 were greater than they were in 2017 and 2019 (Figure 6H).
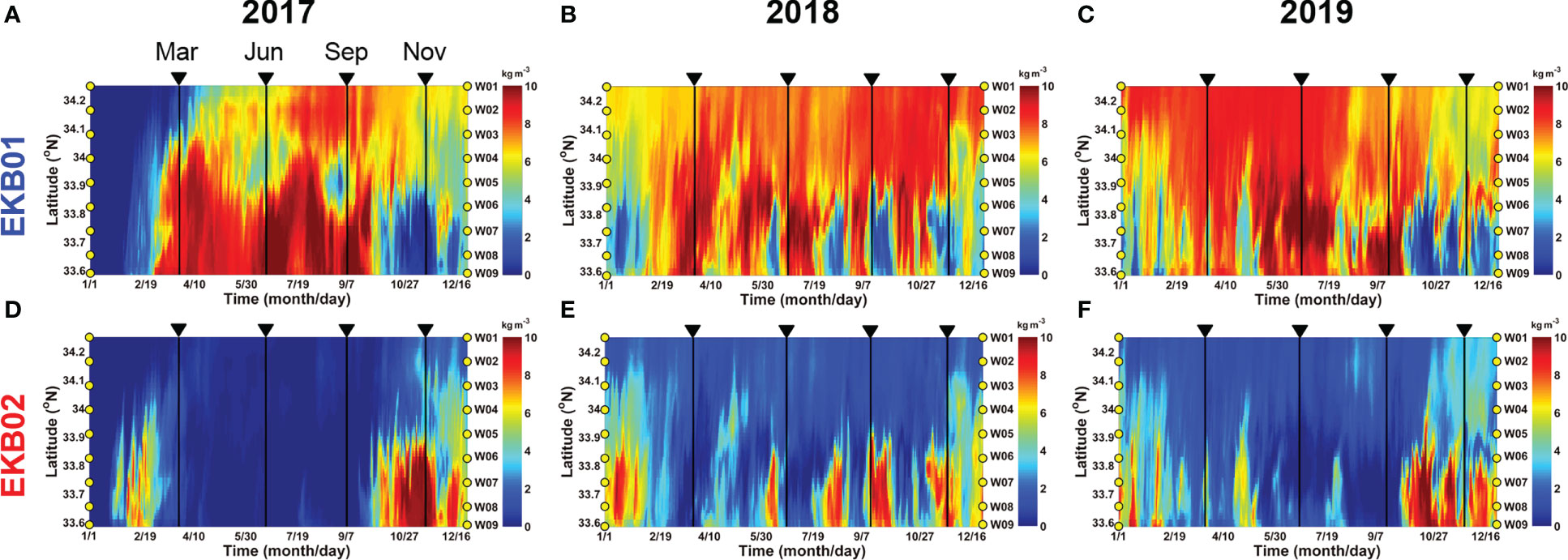
Figure 9 Hovmöller diagram showing the concentration of dyes originating from EKB01 (A–C) and EKB02 (D–F) averaged from the surface to 30 m depth from 2017 to 2019. Along the axis of ordinates, the yellow circles indicate the sampling sites in Figure 1B.
Correlation analysis between the total cell abundance of Ornithocercus and Triposolenia and the concentration of dyes originating from EKB01 and EKB02 showed that their total cell abundances have a strong positive correlation with the concentration of dyes originating from EKB02 (r = 0.637, p<0.0001), but a moderately negative relationship with the concentration of dyes originating from EKB01 (r = -0.512, p<0.0001) (Supplementary Figure 1).
Discussion
The major findings of the present study were as follows. (1) Abundances and species diversity of the tropical dinoflagellates genera Ornithocercus and Triposolenia in the Jeju Strait show strong seasonality, with the highest abundance observed in November and being almost undetectable in June. (2) Spatial distribution and a northward expansion range of the tropical dinoflagellates in the Jeju Strait are strongly associated with the path and intensity of the warm current (i.e., Jeju Warm Current) originating from EKB02.
During a cruise across the Indian Ocean from Cape Town, South Africa to Broome, Australia, where surface water temperatures ranged from 12 to 30°C, Tarangkoon et al. (2010) found that cell densities of ectosymbiont-bearing dinoflagellates, including Ornithocercus, were positively correlated with water temperature, with those in the euphotic zone not observed at water temperatures< 16°C. Unlike their result, however, the result from the present study showed a different pattern in cell abundance with respect to water temperature. In this study, the highest cell abundance of Ornithocercus was observed in November, when water temperatures ranged from 14.0 to 20.2°C. Interestingly, all Ornithocercus cells observed in March were found in relatively low-temperature waters, in the range of 12.4 to 14.5°C, although cell abundance in March was lower than in November. The highest species diversity of Ornithocercus was found in November (five or six species) than in March and June (two or three species). By comparison, all Triposolenia cells observed in this study were found only in water temperatures of 12.4 to 18.8°C. This pattern of occurrence of Triposolenia cell abundance also differs from Tarangkoon et al.’s (Tarangkoon et al., 2010) result, in which endosymbiont-bearing dinoflagellates such as Triposolenia were not observed in water temperatures below 23°C. Our data, therefore, imply that cell abundance and species diversity (and particularly Ornithocercus in this study) of these symbiont-bearing dinoflagellates were not dependent on the high water temperature in the Jeju Strait undergoing large seasonal variations in water temperature, down to as little as 16°C, which is typical for temperate waters. In fact, their highest cell abundances and species diversity were found in relatively cold water temperatures. Therefore, the results from the present study strongly indicate that seasonal variations observed in cell abundance and species diversity in the Jeju Strait located in temperate regions may be attributed to the introduction of the dinoflagellates by currents originating from warm waters that subsequently mix with the cooler coastal waters. Alternatively, it could not be excluded the possibility that their high abundance in November each year might result from in situ growth of their populations in the Jeju Strait and/or subsequent later stages of the seasonal succession. However, this seems not to be the case. When calculated in situ growth rate by using the cell abundances in November and September, their growth rates were too low (about 0.0478 day-1; data not shown) to achieve such a high cell density observed in November each year, again supporting the idea that the peak abundance shown in November is highly related to the inflow of a large number of Ornithocercus and Triposolenia through the inflow of warm current.
Ocean warming has dramatically shifted the geographic distributions of tropical species into more temperate waters. Indeed, the distribution ranges of a variety of marine organisms, including seaweed, plankton, invertebrates, and fish have been steadily expanding northwards (Lubchenco et al., 1993; Beaugrand et al., 2002; Zacherl et al., 2003; Perry et al., 2005). Plankton species, in particular, are seen as good potential climate change indicators in the marine environment because they can easily respond to changes in water temperature and exhibit rapid distribution changes in which their habitat range expands or contracts. For example, free-floating dinoflagellates belonging to the genus Tripos (previously known as Ceratium) are considered biological indicators for monitoring water masses, oceanic currents, and climate change (Dodge and Marshall, 1994; Johns et al., 2003; Tunin-Ley et al., 2009; Tunin-Ley and Lemée, 2013). Similarly, the result from the present study also showed that the warm water dinoflagellates Ornithocercus and Triposolenia are distributed throughout the warm current system. This suggests, therefore, that Ornithocercus and Triposolenia could also be used as biological indicators for warm water mass and ocean currents originating from subtropical and tropical seas.
In the Jeju Strait, the shoreward limit and spatial distribution of the tropical dinoflagellates were strongly linked with the intensity of warm current water originating from the EKB02 in the numerical model simulation. The result of correlation analysis between the total cell abundance and the concentration of dyes again supports that spatial distribution and a northward expansion range of the tropical dinoflagellates in the Jeju Strait are strongly associated with the path and intensity of the warm current (i.e., Jeju Warm Current) originating from EKB02. Indeed, in November, when the warm current carrying water originating from EKB02 expanded northwest, the tropical dinoflagellates were observed at station W03, a northern station, along the transect. By comparison, in June, water carried by the warm current originating from the EKB02 was not observed along the meridional transect or was only found at stations W08 and W09, the southernmost stations, off the northern coast of Jeju Island. In addition to the intensity of the warm current, the present study suggests that the path of the warm current is also an important factor for determining the spatial distribution of the tropical dinoflagellates in the Jeju Strait. Specifically, in September 2018 and 2019 when the warm current flowed over a broad range across the observation transect, the tropical dinoflagellates were found from stations W03 to W09, whereas in September 2017, they were only found at stations W03 and W06 located in the middle of the transect where the warm current passed through. Unlike the results in June of 2017 and 2019 when the tropical dinoflagellates were not observed, their occurrence in 2018 only at stations W08 and W09, adjacent to the northern coast of Jeju Island, again supports that the path of the warm current is an important determinant for the spatial distribution of tropical dinoflagellates. During the study period, no tropical dinoflagellates were found at stations W01 and W02, which were located close to the southern coast of Korea. In other words, it can be inferred that the JWC provides warm water originating from the subtropical regions to the southwestern coast of Korea up to W02 station and that this region marks the northern limit of tropical dinoflagellates distribution in the Jeju Strait.
Between autumn and spring, a thermohaline front forms between W01 and W03 stations marking the boundary between the coastal waters and the JWC water. In summer, the warm saline water flowing northwestward from EKB01 in the subsurface layer forms a subsurface thermohaline front with a cooler and less-saline water mass originating from the Yellow Sea in the East China Sea and the Jeju Strait (Pang et al., 1992; Chen et al., 1994; Hur et al., 1999; Lie et al., 2000; Qi et al., 2014). Although the waters of EKB01 and EKB02 are similar waters originating from the KWC, the hydrographic properties of the water from EKB01 might be modified in the East China Sea by the mixing that occurs along the subsurface thermohaline front. The water originating from EKB02 is less affected by other coastal water masses than the water from EKB01 and it can maintain the warm and saline water characteristics in the Korea Strait, meaning that tropical dinoflagellates are likely to be able to distribute throughout the warm, saline waters originating from EKB02. Mixing of the water masses originating from EKB01 and EKB02 with other coastal water masses in the East China Sea means that the formation of the thermohaline fronts in the Jeju Strait, and the main path of the JWC might affect the distribution of tropical dinoflagellates throughout the Jeju Strait.
In conclusion, our results demonstrate the presence of various and numerous tropical dinoflagellate species from the Ornithocercus and Triposolenia genera in the Jeju Strait. The populations of these genera fluctuate seasonally, with the highest abundance in November, and are strongly associated with the path and intensity of JWC, which flows into the strait. As suggested in our results, these tropical dinoflagellates could potentially be used as biological indicators to monitor the JWC inflowing in the Jeju Strait, and they are presumed to have not yet settled in the coastal region off the south coast of Korea, despite their continuing northward movement toward the coastal regions in autumn and winter.
Data availability statement
The original contributions presented in the study are included in the article/Supplementary Material. Further inquiries can be directed to the corresponding author.
Author contributions
All authors listed have made a substantial, direct and intellectual contribution to the work and approved the manuscript for publication.
Funding
This study was supported by the National Research Foundation of Korea (research grants NRF-2016R1A6A1A03012647 and NRF-2022M3I6A1085991).
Conflict of interest
The authors declare that the research was conducted in the absence of any commercial or financial relationships that could be construed as a potential conflict of interest.
Publisher’s note
All claims expressed in this article are solely those of the authors and do not necessarily represent those of their affiliated organizations, or those of the publisher, the editors and the reviewers. Any product that may be evaluated in this article, or claim that may be made by its manufacturer, is not guaranteed or endorsed by the publisher.
Supplementary material
The Supplementary Material for this article can be found online at: https://www.frontiersin.org/articles/10.3389/fmars.2023.1156121/full#supplementary-material
Supplementary Figure 1 | Correlation between total cell abundances of Ornithocercus and Triposolenia and the concentration of dyes originating from EKB01 (A) and EKB02 (B) at each sampling station and dates.
Supplementary Figure 2 | The concentraion of dyes originating from EKB01 (dotted line) and EKB02 (solid line) averaged from W01 to W09 stations during the study period.
References
Abe T. H. (1967). The armoured dinoflagellata: II. prorocentridae and dinophysidae (C)-Ornithocercus, Histioneis, Amphisolenia and others. Pub. Seto Mar. Biol. Lab. 15, 79–116. doi: 10.5134/175463
Beaugrand G., Reid P. C., Ibanez F., Lindley J. A., Edwards M. (2002). Reorganization of north Atlantic marine copepod biodiversity and climate. Science 296, 1692–1694. doi: 10.1126/science.1071329
Chang K.-I., Teague W., Lyu S., Perkins H., Lee D.-K., Watts D., et al. (2004). Circulation and currents in the southwestern East/Japan Sea: Overview and review. Prog. Oceanogr. 61, 105–156. doi: 10.1016/j.pocean.2004.06.005
Chen C., Beardsley R. C., Limeburner R., Kim K. (1994). Comparison of winter and summer hydrographic observations in the yellow and East China seas and adjacent kuroshio during 1986. Continent. Shelf Res. 14, 909–929. doi: 10.1016/0278-4343(94)90079-5
Choa J. H., Lee J.-B. (2000). Bioecological characteristics of coral habitats around moonsom, cheju island, Korea i. environment properties and community structures of phytoplankton. Sea: J. korean Soc. oceanogr. 5 (1), 59–69.
Crickenberger S. E. (2014). Range limits, range shifts, and lower thermal tolerance in the tropical barnacle megabalanus coccopoma. Ph.D. Dissertation (Clemson, SC, USA: Clemson University).
Croteau E. (2010). Causes and consequences of dispersal in plants and animals. Nat. Educ. Knowl. 3, 12.
Cushing D. H., Dickson R. R. (1976). The biological response in the sea to climatic changes. Adv. Mar. Biol. 14, 1–122. doi: 10.1016/S0065-2881(08)60446-0
de Aquino E. P., Honorato-Da-Silva M., Do Nascimento Feitosa F. A., Koening M. L., De Oliveira Passavante J. Z. (2014). Field observation of Ornithocercus spp. (Dinophysiales, dinophyta): Reproductive stages and phased cell division (South Atlantic ocean, Brazil). Trop. Oceanogr. Recife 42, 170–176. doi: 10.5914/tropocean.v42i2.5812
Dodge J. D., Marshall H. G. (1994). Biogeographic analysis of the armored planktonic dinoflagellate Ceratium in the north atrlantic and adjacent seas 1. J. Phycol. 30, 905–922. doi: 10.1111/j.0022-3646.1994.00905.x
Doney S. C., Ruckelshaus M., Emmett Duffy J., Barry J. P., Chan F., English C. A., et al. (2012). Climate change impacts on marine ecosystems. Annu. Rev. Mar. Sci. 4, 11–37. doi: 10.1146/annurev-marine-041911-111611
Donlon C. J., Martin M., Stark J., Roberts-Jones J., Fiedler E., Wimmer W. (2012). The operational sea surface temperature and sea ice analysis (OSTIA) system. Remote Sens. Environ. 116, 140–158. doi: 10.1016/j.rse.2010.10.017
Egbert G. D., Erofeeva S. Y. (2002). Efficient inverse modeling of barotropic ocean tides. J. Atmos. Ocean. Technol. 19, 183–204. doi: 10.1175/1520-0426(2002)019<0183:EIMOBO>2.0.CO;2
Fairall C. W., Bradley E. F., Hare J., Grachev A. A., Edson J. B. (2003). Bulk parameterization of air–sea fluxes: Updates and verification for the COARE algorithm. J. Climate 16, 571–591. doi: 10.1175/1520-0442(2003)016<0571:BPOASF>2.0.CO;2
Finlay B. J. (2002). Global dispersal of free-living microbial eukaryote species. science 296, 1061–1063. doi: 10.1126/science.1070710
Gómez F. (2005). A list of free-living dinoflagellate species in the world’s oceans. Acta Botanica Croatica 64, 129–212.
Hallegraeff G., Jeffrey S. (1984). Tropical phytoplankton species and pigments of continental shelf waters of north and north-west Australia. Mar. Ecol. Prog. Ser. 20, 59–74. doi: 10.3354/meps020059
Hernández-Becerril D. U., Ceballos-Corona J. G. A., Esqueda-Lara K., Tovar-Salazar M. A., León- lvarez D. (2008). Marine planktonic dinoflagellates of the order dinophysiales (Dinophyta) from coasts of the tropical Mexican pacific, including two new species of the genus Amphisolenia. J. Mar. Biol. Assoc. U.K. 88, 1–15. doi: 10.1017/S0025315408000143
Hur H., Jacobs G., Teague W. (1999). Monthly variations of water masses in the yellow and East China seas, November 6, 1998. J. Oceanogr. 55, 171–184. doi: 10.1023/A:1007885828278
Intergovernmental Panel On Climate Change. (2007) Climate change 2007: The physical science basis. Agenda, 6, 333.
Johns D., Edwards M., Richardson A., Spicer J. (2003). Increased blooms of a dinoflagellate in the NW Atlantic. Mar. Ecol. Prog. Ser. 265, 283–287. doi: 10.3354/meps265283
Ju S.-J., Kim S.-J. (2012). Assessment of the impact of climate change on marine ecosystem in the south sea of Korea. Ocean Polar Res. 34, 197–199. doi: 10.4217/OPR.2012.34.2.197
Jung M.-M., Kim H.-S. (2013). Morphology of four dinoflagellate species (Amphisolenia and Triposolenia) newly recorded from Korea. J. Fish. Mar. Sci. Educ. 25, 1239–1244.
Jung H. K., Rahman S. M., Kang C.-K., Park S.-Y., Lee S. H., Park H. J., et al. (2017). The influence of climate regime shifts on the marine environment and ecosystems in the East Asian marginal seas and their mechanisms. Deep Sea Res. Part II: Topical Stud. Oceanogr. 143, 110–120. doi: 10.1016/j.dsr2.2017.06.010
Keister J. E., Di Lorenzo E., Morgan C., Combes V., Peterson W. (2011). Zooplankton species composition is linked to ocean transport in the northern California current. Global Change Biol. 17, 2498–2511. doi: 10.1111/j.1365-2486.2010.02383.x
Kim H. K., Chan B. K., Lee S.-K., Kim W. (2020). Biogeography of intertidal and subtidal native and invasive barnacles in Korea in relation to oceanographic current ecoregions and global climatic changes. J. Mar. Biol. Assoc. U.K. 100, 1079–1091. doi: 10.1017/S0025315420001009
Kim M., Choi D. H., Park M. G. (2021). Cyanobiont genetic diversity and host specificity of cyanobiont-bearing dinoflagellate Ornithocercus in temperate coastal waters. Sci. Rep. 11 (1), 1–13. doi: 10.1038/s41598-021-89072-z
Kim H.-S., Jung M.-M., Lee J.-B. (2008). The Korean peninsula warming based on appearance trend of tropical dinoflagellate species, genus Ornithocercus. Sea: J. Korean Soc. Oceanogr. 13, 303–307.
Kim G. B., Kang S. M., Lee J.-B. (2019). Spatial and temporal variation of phytoplankton community in the coastal waters of jeju island. Sea: J. Korean Soc. Oceanogr. 24 (1), 92–105. doi: 10.7850/jkso.2019.24.1.092
Kim H.-S., Kim S.-H., Jung M.-M., Lee J.-B. (2013). New record of dinoflagellates around jeju island. J. Ecol. Environ. 36, 273–291. doi: 10.5141/ecoenv.2013.273
Kim S. H., Rho H. K. (1997). A study on the residual current in the cheju strait. Korean J. Fish. Aquat. Sci. 30, 759–770.
KMA (2019). Report of global atmosphere watch 2018 Vol. 268 (Seogwipo, Korea: National Institute of Meteorological Sciences).
Large W. G., Mcwilliams J. C., Doney S. C. (1994). Oceanic vertical mixing: A review and a model with a nonlocal boundary layer parameterization. Rev. geophys. 32, 363–403. doi: 10.1029/94RG01872
Lavanya S., Joseph R. V., Parvathy R., Padua S., Prema D., Kripa V., et al. (2020). First report of a rare bloom of Ornithocercus magnificus, stein 1883 along the coastal waters of kochi; a possible indicator of increasing sea surface temperature. J. Mar. Biol. Assoc. India 62, 92–98. doi: 10.6024/jmbai.2020.62.2.2193-11
Lee J. C. (1983). Characteristics of front near the cheju strait in early winter. Korean J. Fish. Aquat. Sci. 16, 51–58.
Lee J.-B., Go Y. B., Choa J. H. (1990). The structure and dynamics of phytoplankton communities around the coastline of cheju island, Korea. Korean J. Phycol. 5, 159–171.
Lee J.-B., Jwa J. H., Go Y. B., Choe Y. C. (1993). Bioecological studies of the Eastern coastal area in cheju island (II) phytoplankton dynamics and primary productivity around U-do. J. Korean Earth Sci. Soc. 14 (4), 458–458.
Lee J.-B., Kim H.-S., Chung H.-S. (2015). New records of three dinophycean genera Dinophysis, Histioneis, and Parahistioneis (Dinophysiales, dinophyceae) from coastal waters of jeju island, Korea. J. Ecol. Environ. 38, 599–609. doi: 10.5141/ecoenv.2015.060
Lellouche J.-M., Greiner E., Le Galloudec O., Garric G., Regnier C., Drevillon M., et al. (2018). Recent updates to the Copernicus marine service global ocean monitoring and forecasting real-time 1/12 high-resolution system. Ocean Sci. 14, 1093–1126. doi: 10.5194/os-14-1093-2018
Lie H.-J., Cho C.-H. (1997). Surface current fields in the eastern East China Sea. J. Korean Soc. Oceanogr. 32, 1–7.
Lie H.-J., Cho C.-H. (2016). Seasonal circulation patterns of the yellow and East China seas derived from satellite-tracked drifter trajectories and hydrographic observations. Prog. Oceanogr. 146, 121–141. doi: 10.1016/j.pocean.2016.06.004
Lie H.-J., Cho C.-H., Lee J.-H., Lee S., Tang Y. (2000). Seasonal variation of the cheju warm current in the northern East China Sea. J. Oceanogr. 56, 197–211. doi: 10.1023/A:1011139313988
Lubchenco J., Navarrete S.A., Tissot B.N., Castilla J.C. (1993). Possible ecological responses to global climate change: near-shore benthic biota of Northeastern Pacific coastal ecosystems. In: Earth System Responses to Global Climate Change: Contrasts between North and South America (eds Mooney H.A., Fuentes E.R., Kronberg B.I.). Academic Press, San Diego, CA, 147–166.
Nakayama T., Nomura M., Takano Y., Tanifuji G., Shiba K., Inaba K., et al. (2019). Single-cell genomics unveiled a cryptic cyanobacterial lineage with a worldwide distribution hidden by a dinoflagellate host. Proc. Natl. Acad. Sci. 116, 15973–15978. doi: 10.1073/pnas.1902538116
Palumbi S. R. (2003). Population genetics, demographic connectivity, and the design of marine reserves. Ecol. Appl. 13, 146–158. doi: 10.1890/1051-0761(2003)013[0146:PGDCAT]2.0.CO;2
Pang I.-C., Hong C.-S., Chang K.-I., Lee J.-C., Klm J.-T. (2003). Monthly variation of water mass distribution and current in the cheju strait. J. Korean Soc. Oceanogr. 38, 87–100.
Pang I.-C., Rho H.-K., Kim T.-H. (1992). Seasonal variations of water mass distributions and their causes in the yellow Sea, the East China Sea and the adjacent seas of cheju island. Korean J. Fish. Aquat. Sci. 25, 151–163.
Pang I.-C., Rho H.-K., Lee J.-H., Lie H.-J. (1996). Water mass distribution and seasonal circulation northwest of cheju island in 1994. Korean J. Fish. Aquat. Sci. 29, 862–875.
Park K.-A., Park J.-E., Choi B.-J., Lee S.-H., Shin H.-R., Lee S.-R., et al. (2017). Schematic maps of ocean currents in the yellow Sea and the East China Sea for science textbooks based on scientific knowledge from oceanic measurements. Sea: J. Korean Soc. Oceanogr. 22, 151–171. doi: 10.7850/jkso.2017.22.4.151
Perry A. L., Low P. J., Ellis J. R., Reynolds J. D. (2005). Climate change and distribution shifts in marine fishes. Science 308, 1912–1915. doi: 10.1126/science.1111322
Poff N., Brinson M. M., Day J. (2002). Aquatic ecosystems and global climate change. Pew Center Global Climate Change Arlington VA 44, 1–36.
Poloczanska E. S., Hawkins S. J., Southward A. J., Burrows M. T. (2008). Modeling the response of populations of competing species to climate change. Ecology 89, 3138–3149. doi: 10.1890/07-1169.1
Putman N. F., Mansfield K. L. (2015). Direct evidence of swimming demonstrates active dispersal in the sea turtle “lost years”. Curr. Biol. 25, 1221–1227. doi: 10.1016/j.cub.2015.03.014
Qi J., Yin B., Zhang Q., Yang D., Xu Z. (2014). Analysis of seasonal variation of water masses in East China Sea. Chin. J. Oceanol. Limnol. 32, 958–971. doi: 10.1007/s00343-014-3269-1
Saifullah S., Gul S., Khan M. (2008). The dinoflagellate genus Ornithocercus stein from north Arabian Sea shelf of Pakistan. Pakistan J. Bot. 40, 849–857.
Seo S.-N. (2008). Digital 30sec gridded bathymetric data of Korea marginal seas-KorBathy30s. J. Korean Soc. Coast. Ocean Eng. 20, 110–120.
Shchepetkin A. F., Mcwilliams J. C. (2005). The regional oceanic modeling system (ROMS): A split-explicit, free-surface, topography-following-coordinate oceanic model. Ocean Model. 9, 347–404. doi: 10.1016/j.ocemod.2004.08.002
Shin C.-W., Min H. S., Lee S., Kang H.-W., Ku B., Kim D. G., et al. (2022). Current structure and volume transport in the jeju strait observed for a year with multiple ADCP moorings. Ocean Sci. J. 57 (3), 365–380. doi: 10.1007/s12601-022-00079-7
Smayda T. J. (2007). Reflections on the ballast water dispersal–harmful algal bloom paradigm. Harmful Algae 6, 601–622. doi: 10.1016/j.hal.2007.02.003
Song Y., Haidvogel D. (1994). A semi-implicit ocean circulation model using a generalized topography-following coordinate system. J. Comput. Phys. 115, 228–244. doi: 10.1006/jcph.1994.1189
Southward A., Hawkins S., Burrows M. (1995). Seventy years' observations of changes in distribution and abundance of zooplankton and intertidal organisms in the western English channel in relation to rising sea temperature. J. Thermal Biol. 20, 127–155. doi: 10.1016/0306-4565(94)00043-I
Suk M.-S., Pang I.-C., Teague W. J., Chang K.-I. (2000). Observations of the cheju current. J. Korean Soc. Oceanogr. 35, 129–152.
Tarangkoon W., Hansen G., Hansen P. J. (2010). Spatial distribution of symbiont-bearing dinoflagellates in the Indian ocean in relation to oceanographic regimes. Aquat. Microbial Ecol. 58, 197–213. doi: 10.3354/ame01356
Taylor F. (1971). Scanning electron microscopy of thecae of the dinoflagellate genus ornithocercus 1. J. Phycol. 7, 249–258. doi: 10.1111/j.1529-8817.1971.tb01510.x
Tunin-Ley A., Ibañez F., Labat J.-P., Zingone A., Lemée R. (2009). Phytoplankton biodiversity and NW Mediterranean Sea warming: Changes in the dinoflagellate genus Ceratium in the 20th century. Mar. Ecol. Prog. Ser. 375, 85–99. doi: 10.3354/meps07730
Tunin-Ley A., Lemée R. (2013). The genus Neoceratium (planktonic dinoflagellates) as a potential indicator of ocean warming. Microorganisms 1, 58–70. doi: 10.3390/microorganisms1010058
White R. (2015). Using multiple passive tracers to identify the importance of the north Brazil undercurrent for Atlantic cold tongue variability. Q. J. R. Meteorol. Soc. 141, 2505–2517. doi: 10.1002/qj.2536
Yeh S.-W., Kim C.-H. (2010). Recent warming in the Yellow/East China Sea during winter and the associated atmospheric circulation. Continent. Shelf Res. 30, 1428–1434. doi: 10.1016/j.csr.2010.05.002
Keywords: Kuroshio Current, passive tracer, tropical dinoflagellate, biological indicator, Jeju Strait
Citation: Lee B, Kim JK, Kim M, Choi B-J, Kim KY and Park MG (2023) Northward movement of the tropical dinoflagellate Ornithocercus and Triposolenia genera in Korean coastal waters is strongly associated with the inflow of the Jeju Warm Current. Front. Mar. Sci. 10:1156121. doi: 10.3389/fmars.2023.1156121
Received: 01 February 2023; Accepted: 13 March 2023;
Published: 22 March 2023.
Edited by:
Haifeng Gu, State Oceanic Administration, ChinaReviewed by:
Jihai Dong, Nanjing University of Information Science and Technology, ChinaEnrique Nogueira, Spanish Institute of Oceanography (IEO), Spain
Copyright © 2023 Lee, Kim, Kim, Choi, Kim and Park. This is an open-access article distributed under the terms of the Creative Commons Attribution License (CC BY). The use, distribution or reproduction in other forums is permitted, provided the original author(s) and the copyright owner(s) are credited and that the original publication in this journal is cited, in accordance with accepted academic practice. No use, distribution or reproduction is permitted which does not comply with these terms.
*Correspondence: Myung Gil Park, bXBhcmtAY2hvbm5hbS5hYy5rcg==