- 1Department of Biology, Valdosta State University, Valdosta, GA, United States
- 2Department of Biological Sciences, University of Idaho, Moscow, ID, United States
Endocrine disruptors have devastating impacts on the reproductive physiology of aquatic organisms. The Gulf pipefish, Syngnathus scovelli, is a sexually dimorphic species, which demonstrates predictable morphological, physiological, behavioral, and genetic responses to synthetic estrogen exposure. It has a broad geographic range, spanning freshwater and marine environments, making it a potential sentinel species across a wide range of habitats. In this study, we investigated the effects of ecologically relevant levels of 17α-ethinylestradiol (EE2) exposure on gene expression patterns in the male pipefish’s brood pouch. We also characterized the extent to which EE2-exposed males developed coloration patterns that are normally restricted to females. We identified differentially expressed genes in the brood pouches of pregnant and non-pregnant males when males were exposed to 5ng/L EE2 from the second to eighth day of pregnancy (which normally lasts about 2 weeks). Our result revealed several potential candidate genes that have a role in the brood pouch’s response to environmental estrogens. We also identified genes that were differentially expressed between mid-gestation pregnant males and non-pregnant males. We found an overall greater effect of EE2 exposure in the transcriptomes of non-pregnant males, which may explain why estrogen-exposed males exhibited difficulty receiving eggs in previous studies. The offspring developed similarly in the control and estrogen treatments, highlighting a potential link between the timing of EE2 exposure and its effects on male pregnancy. These results provide insight into how breeding pipefish populations may still exist even though they are found in freshwater and coastal locations where they are periodically exposed to potentially high concentrations of endocrine-disrupting compounds. We also present examples of female-typical coloration development on males due to EE2 exposure and identify candidate brood pouch genes that can be utilized as biomarkers, contributing to the development of the Gulf pipefish as a sentinel model for ecotoxicology.
Introduction
In aquatic environments, endocrine-disrupting compounds, such as 17α-ethinylestradiol (EE2), have been shown to affect physiology, gene expression patterns, and reproductive behaviors (Porte et al., 2006; Orlando and Guillette, 2007; Saaristo et al., 2010). For example, EE2 contamination can cause severe physiological changes in exposed aquatic male organisms, resulting in male sterilization, partial feminization, and even complete sex reversal in some cases (Allen et al., 1999; Jobling et al., 2003). Historically, the model systems for ecotoxicology research have been freshwater species, such as zebrafish and fathead minnows (Porte et al., 2006). More recently, marine species have revealed valuable insights into intraspecific variation in responses to endocrine disruptor contamination in coastal ecosystems (Gouveia et al., 2019; DeCourten et al., 2020). Long-term exposure to low levels of synthetic estrogens can cause entire breeding populations of fish to collapse as a consequence of reproductive failure in both fresh and saltwater environments (Nash et al., 2004; Kidd et al., 2007; Sárria et al., 2013).
Syngnathid fishes (i.e., seahorses, pipefishes, and seadragons) have served as flagship species for coastal environments due to their site fidelity and reliance on these threatened habitats during their entire lifespans (Shokri et al., 2009). Recently, they have emerged as sentinel species for ecotoxicology, with an increase in research utilizing several pipefish species, such as Syngnathus abaster and S. scovelli (Ueda et al., 2005; Partridge et al., 2010; Sárria et al., 2011; Rose et al., 2013; Sárria et al., 2013; Rose et al., 2015), and seahorse species, including Hippocampus erectus and H. guttulatus (Qin et al., 2020; D’Alvise et al., 2020). The Gulf pipefish (S. scovelli) has many advantageous characteristics for a toxicology model system. For instance, it is an estuarine fish and carries out its entire lifecycle in shallow habitats throughout the Gulf of Mexico and along the Florida Atlantic coast near large, populated cities, where effluent from wastewater treatment facilities is released (Dawson, 1982). These characteristics suggest that the Gulf pipefish might be useful as a bioindicator of coastal contamination throughout its life stages. Additional benefits include that Gulf pipefish can be collected throughout the year in many locations (Flanagan et al., 2016; Flanagan et al., 2021), that all ages can be maintained in laboratory conditions (Anderson and Jones, 2019), and that the developmental stages of embryos can be assessed through the male’s transparent brood pouch (Paczolt and Jones, 2010). This species exhibits sexual dimorphism, has a polyandrous mating system, and is sex-role-reversed (Jones and Avise, 1997; Jones et al., 2000). Female pipefish are deeper bodied, have larger dorsal fins, and possess iridescent bands on their abdomens at sexual maturity. These traits are likely targets of sexual selection (Flanagan et al., 2014). These female secondary sexual traits can also develop in male Gulf pipefish that are exposed to EE2, so changes in male morphology can signal estrogen contamination in surrounding waters (Partridge et al., 2010).
In a review of integrative behavioral ecotoxicology, Peterson et al. (2017) observed that only a few studies have assessed the role of behavioral changes caused by toxin exposure on an organism’s overall fitness. The well-described mating system of the Gulf pipefish has provided the opportunity to establish a link between endocrine disruptors and reproductive success in a sex-role-reversed species (Jones and Avise, 1997; Jones et al., 2001; Rose et al., 2013). Gulf pipefish research over the past two decades has investigated the effects of endocrine disruptors on morphological changes, particularly secondary sexual traits (Ueda et al., 2005; Partridge et al., 2010), courtship behaviors, the strength of sexual selection, and fitness (Partridge et al., 2010; Rose et al., 2013). In addition, the effects of endocrine disruptors on the transcriptomes of the liver, skin, and now brood pouch have been documented (Rose et al., 2015; Anderson et al., 2020). A well-annotated, chromosome-scale genome has provided the necessary backdrop for the development of biomarkers emerging from RNA-seq studies (Small et al., 2016; Ramesh et al., 2023). Studies of the population genomics of Gulf pipefish across saltwater and freshwater habitats (Flanagan et al., 2016; Flanagan et al., 2021) provide insights into population structure that lay a groundwork for future studies of population-level effects of endocrine disruptors in nature (Martyniuk et al., 2020).
High concentrations of synthetic estrogen contamination alter the function of the brood pouch when males are exposed prior to mating, and females prefer to mate with control males over those exposed to estrogen (Partridge et al., 2010; Rose et al., 2013). However, little is known about the effects of estrogen exposure on brood pouches of Gulf pipefish during a male’s gestation period. Given the diversity of processes that take place in the brood pouch during pregnancy, such as waste removal and osmoregulation (Ripley, 2009), vascularization of the growing epithelial tissues (Ripley et al., 2010), adaptive immune responses (Parker et al., 2021), and many other vital processes to ensure embryo development (Carcupino et al., 1997), there is a need to understand the effects of EE2 exposure during pregnancy (Whittington et al., 2015). The brood pouch in seahorses displays morphological changes when exposed to 5 ng/L of EE2, with modifications in the expression patterns of genes related to the collagen family and many other cell proliferation and differentiation processes (Qin et al., 2020), but no pipefish has yet been studied in this regard.
The first goal of this study was to determine the effects of low, ecologically relevant levels of 17α-ethinylestradiol (EE2) exposure during and prior to pregnancy in the Gulf pipefish by identifying changes in gene expression patterns in the male pipefish’s brood pouch. Since the study aimed to identify the impacts of EE2 exposure in the brood pouch during the male’s pregnancy and not alter the process of egg transfer or fertilization, we chose to expose the males to contamination 24 hours after copulation. The second goal was to identify the effects of EE2 exposure on the success of the pregnancy and the changes in morphological patterning on the male’s abdomens. This required an identification of stages in the banding ornamentation on the abdomen of male Gulf pipefish exposed to varying concentrations of EE2, which were then used to assess the levels of feminization for the males. The stages of ornamentation identified in this study provides a standard that can be used for future studies of both laboratory-exposed and wild-caught Gulf pipefish. To determine if the pregnancy was successful at the midpoint, we allowed the males to gestate for approximately half their pregnancy and compared the offspring from each treatment after eight days of pregnancy (Scobell and MacKenzie, 2011). The chosen concentration of 5 ng/L EE2 exposure was selected because this level of contamination is ecologically relevant (Zuo et al., 2006; Martyniuk et al., 2020; Klaic and Jirsa, 2022), has been shown to affect the reproductive abilities of laboratory exposed male Gulf pipefish (Rose et al., 2013), and has been detected in wastewater effluent in Florida seagrass communities where wild Gulf pipefish reside (Cook, 2015).
Materials and methods
Pipefish collection, experimental design, and EE2 exposure
Male Gulf pipefish (S. scovelli) were collected from Redfish Bay, Texas (N 27 53 39.07, W 97 7 51.69) in July of 2013 under the Texas Parks and Wildlife permit number SPR-0808-307. Only sexually mature females and pregnant males, those that had visible and functional brood pouches with developing offspring, were collected and brought into the lab under IACUC approval (Animal Use Protocol # 2013-0020, Reference #001898). All fish were dipped in freshwater for 10 minutes to remove any external parasites and then housed in 26-ppt salinity tanks at Texas A&M University (College Station, Texas). All field-caught males gave birth in lab prior to being used in the experiment and were assigned to either the non-pregnant category or mated in the lab to serve as a pregnant male for the experiment.
Methods used for the EE2 exposure treatments were stated in Rose et al. (2015). Powdered 17α-ethinylestradiol, of 98% purity, was acquired from Sigma and dissolved in ethanol. Tanks, holding 7-liters of saltwater, were separated and treated as either experimental or control tanks. Experimental tanks were dosed with 50 μl of 7ng/10μl stock EE2 ethanol solution to obtain a final concentration of 5ng of EE2 per liter of saltwater (5ng/L of EE2). Control tanks were dosed with 50 μl of pure ethanol with no EE2. Ten percent water changes were completed daily to ensure the concentrations remained at constant 5ng/L as established by Partridge et al. (2010).
To generate the pregnant males and establish successful pregnancies prior to assigning the fish to either the control or EE2-exposure treatments, the non-pregnant males and females were paired together in clean, EE2-free saltwater tanks until the male became pregnant. Pregnant males were then assigned a non-pregnant male for each replication. The males used in the study ranged from 84-94 mm in body length, and the size distribution did not differ between the treatments (mean = 89 mm). On the second day of the male’s pregnancy, the fish were photographed, measured, and randomly assigned to treatments in either experimental tanks containing 5ng/L EE2 or the EE2-free control tanks. After seven days of exposure, that is, on the eighth day of the male’s pregnancy, fish were humanely euthanized in MS-222 (IACUC-2013-0020) and their brood pouches dissected, imaged, and coated in RNA-later solution for the removal of offspring. Photographs were taken of the offspring, both in the pouch to detect any brood reduction (Paczolt and Jones, 2010) and after being dissected out of the pouch to assess the development of the embryos.
RNA extraction and sequencing
RNA from brood pouch flap tissues was isolated from 8 control males and 8 EE2 exposed males using a TRIzol® Reagent (Life Technologies, Carlsbad, CA) extraction method modified from (Leung and Dowling, 2005) as described in (Rose et al., 2015). All RNA samples were confirmed to be of high quality using a Caliper GX instrument before library construction. Each sample had its library individually prepared using the TruSeq mRNA Library Prep Kit v2 at the RTSF Genomics Core at Michigan State University. Caliper GX and qPCR methods were used for quality control on prepared libraries. Libraries were sequenced on an Illumina HiSeq 2500. Illumina Real Time Analysis (RTA, v1.17.21.3) was used for base calling. The RTA output was demultiplexed and converted to FASTQ using Illumina Bcl2fastq (v1.8.4) resulting in 150bp paired-end reads. This resulted in the following RNA-sequencing (RNA-seq) read files for each of the sixteen samples: (1) EE2-exposed and not pregnant (E51NPP, E52NPP, E54NPP, E57NPP), (2) EE2-exposed and pregnant (E51PP, E52PP, E54PP, E57PP), (3) control (i.e., non-exposed) and pregnant (C4PP, C7PP, C8PP, C9PP), and (4) control and not pregnant (C4NPP, C7NPP, C88NPP , C9NPP). The number of reads per sample ranged from 7,038,156 to 24,648,065 read pairs.
Transcriptome assembly and quantification
Reads from each sample were trimmed to remove Illumina adapters and low quality bases using Trimmomatic (v0.39) (ILLUMINACLIP : TruSeq3-PE.fa:2:30:10:2:keepBothReads LEADING:3 TRAILING:3 MINLEN:75) (Bolger et al., 2014). Paired-end reads were aligned and mapped to the Gulf pipefish (S. scovelli) genome (obtained from the William Cresko Lab at the University of Oregon) using HISAT2 (v2.2.1) on default parameters (Kim et al., 2015; Kim et al., 2019). The overall alignment rate averaged across all samples was 88.61%, with the lowest sample alignment rate being 83.08%. Reads were then assembled and quantified using StringTie (v.2.1.3b) without a reference annotation file in transcript merge mode (Pertea et al., 2015; Pertea et al., 2016). We chose not to use a reference annotation file to prevent the relatively poor annotation quality of the Gulf pipefish genome from biasing resulting read counts. Transcripts were then merged from all samples into a non-redundant set of transcripts (n = 21,721). A gene count matrix was generated from the abundances of the merged transcripts using the prepDE.py script from StringTie. Read counts and TPM values for each gene are provided in Supplemental Table 3.
Differential expression analysis
From this gene count matrix, we used the R (R Core team, 2015) and Bioconductor package edgeR (v.3.1.3) to identify transcripts that were differentially expressed between the conditions of EE2-exposure and pregnancy status (Robinson et al., 2010). Our goal was to understand whether EE2-exposure had an overall effect on the male brood pouch regardless of pregnancy, and whether EE2-exposure influenced the brood pouches of males that were pregnant. This resulted in four pairwise comparisons based on the following conditions: (1) EE2-exposure regardless of pregnancy status, (2) pregnancy status regardless of EE2-exposure, (3) EE2-exposure for only pregnant fish, and (4) EE2-exposure for only non-pregnant fish. Only genes with a false discovery rate ≤ 0.05 for differential expression were used for downstream analyses. For each of the four comparisons, we were left with a list of differentially expressed genes (Figure 1). The nucleotide sequences for the corresponding transcripts were obtained from the Gulf pipefish genome using the program gffread (v12.7) and FastaRecordExtractor (v2) (Pertea and Pertea, 2020). Each resulting sequence was converted to its longest open reading frame via FastaToORF (v1). Both FastaRecordExtractor and FastaToORF are available via the Jones Lab Github (https://github.com/JonesLabIdaho/). Sequences from differentially expressed genes were compared against all Syngnathus species present in the NCBI database using BLASTX, and the best matching protein ID was retained (Supplemental File 1: DE_syngnathus.xlsx). Sequences from differentially expressed genes were also used in the downstream gene ontology analysis.
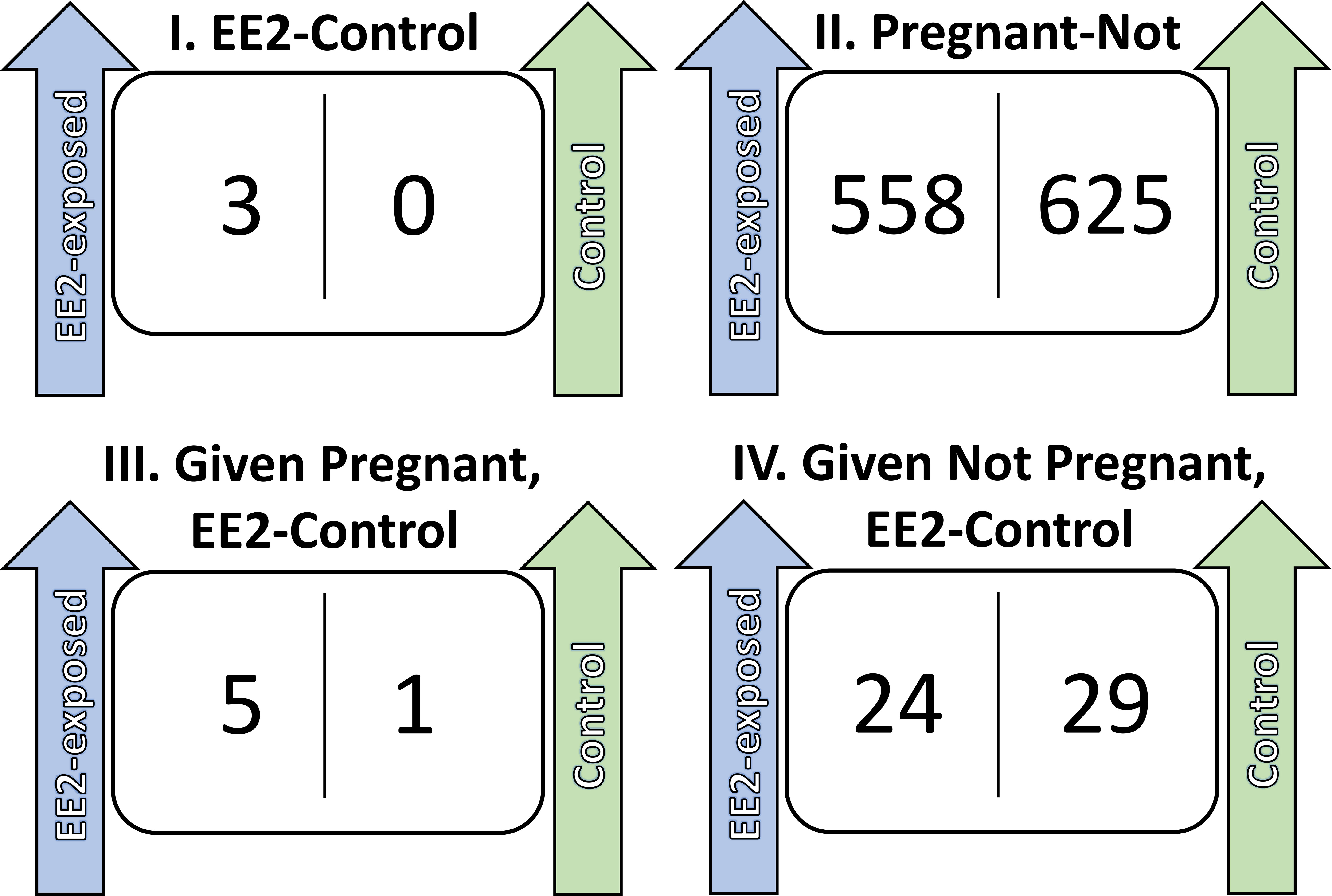
Figure 1 Differentially expressed genes from pairwise analyses. These are the number of significantly differentially expressed genes (FDR ≤ 0.05), that are up-regulated for their respective condition. There are four pairwise tests: (1) EE2-exposed versus control, including all males (regardless of pregnancy status), (2) pregnant versus not, including all males (regardless of EE2-exposure), (3) EE2-exposed versus control, for only pregnant males, and (4) EE2-exposed versus not, for only non-pregnant males.
Analysis of abundant transcripts
Apart from the differential expression analysis, we were also interested in the nature of abundant transcripts within the brood pouches of the different experimental groups. We assessed the overall expression profile and clustering of samples with a heatmap and the variance between samples with a PCA. For these analyses, we used the TPM (transcripts per million) values calculated by StringTie. We only included transcripts that had a minimum expression level in at least one of the four experimental groups. Our minimum expression level was set by averaging the TPM values across transcripts between samples of the same condition (EE2-exposure and pregnancy status) and keeping transcripts that had an average TPM value ≥ 25 in at least one of these four groups (n = 4,892). We excluded transcripts that have very low expression across all samples because they do not provide enough information on the variance between the samples.
To identify the variance between and clustering of samples, we performed a principal component analysis using the R function prcomp() within the package stats (v.3.6.2) (Figure 2). For all samples, we used only the TPM-filtered transcripts, with the expression value for these transcripts calculated as the log(TPM +1). Samples are labelled and plotted by pregnancy and EE2-exposure status on the first two principal components (Figure 2A). The first two principal components were also plotted in comparison with the third (Figures 2B, C).
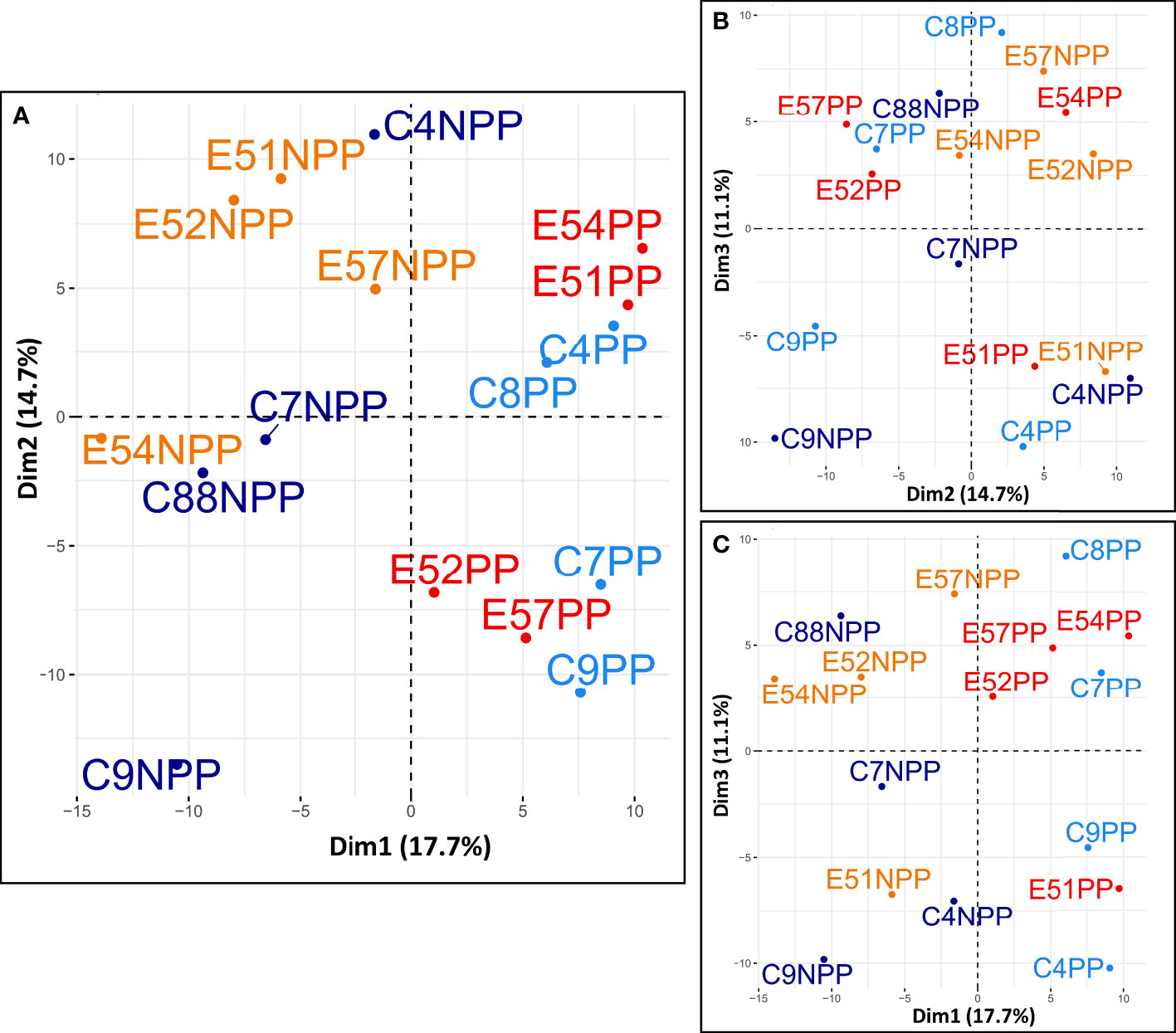
Figure 2 PCA between all individual male samples. Samples are labelled by their EE2-exposure status (E-EE2 exposed or C-control) and their pregnancy status (NPP-not pregnant, PP-pregnant). The first principal component separates males by pregnancy status (A, C). The first two principal components explain 32.4% of the total variance between samples (A–C). The third principal component explains an additional 11.1% of the total variance (B, C).
We then took fifty transcripts with the highest average TPM value in each of the four experimental groups and searched for their corresponding protein ID and name in NCBI’s S. scovelli database using BLASTX. The protein sequence ID with the best E-value score per transcript was retained (Supplemental File 2: top50_genes.xlsx).
Gene ontology analysis of differentially expressed genes
To explore the genes present within our differential expression comparison, we performed a gene ontology analysis through the PANTHER database using a common reference proteome. To begin, the zebrafish (Danio rerio) proteome (UP000000437_7955), was obtained from UniProt and used to construct a local NCBI BLAST database. The differentially expressed transcripts were searched against the zebrafish proteome using BLASTX to identify potential orthologs. When multiple differentially expressed transcripts within the pairwise comparison matched to the same zebrafish protein sequence ID, duplicates were removed to prevent potential inflation bias. If there was no match for a differentially expressed transcript, its sequence was not included for the PANTHER analysis. For these BLAST searches, matching protein sequence IDs with an E-value score of 1x10-20 or less were retained, and the protein sequence ID with the best E-value score per transcript was used.
For the gene ontology analysis, we used the PANTHER database (v16.0) (Thomas et al., 2003; Mi et al., 2013; Mi et al., 2019). The PANTHER database is a protein classification system that provides information on gene ontology and gene families. It also provides details on the molecular function, biological process, and pathways in which a gene is implicated. Use of PANTHER requires a reference organism for which PANTHER has an established database. For this reason, we used zebrafish, as it is one of the most heavily studied fish with respect to gene function. For the PANTHER analysis, we uploaded the list of protein sequence IDs for each of the differential expression comparisons. We performed a gene ontology analysis for biological processes, cellular components, and molecular function using the curated PANTHER GO-Slim database, and an analysis using the PANTHER Protein Class database. Results were recorded and assessed as percentages of the total hits in each database (Figure 3).
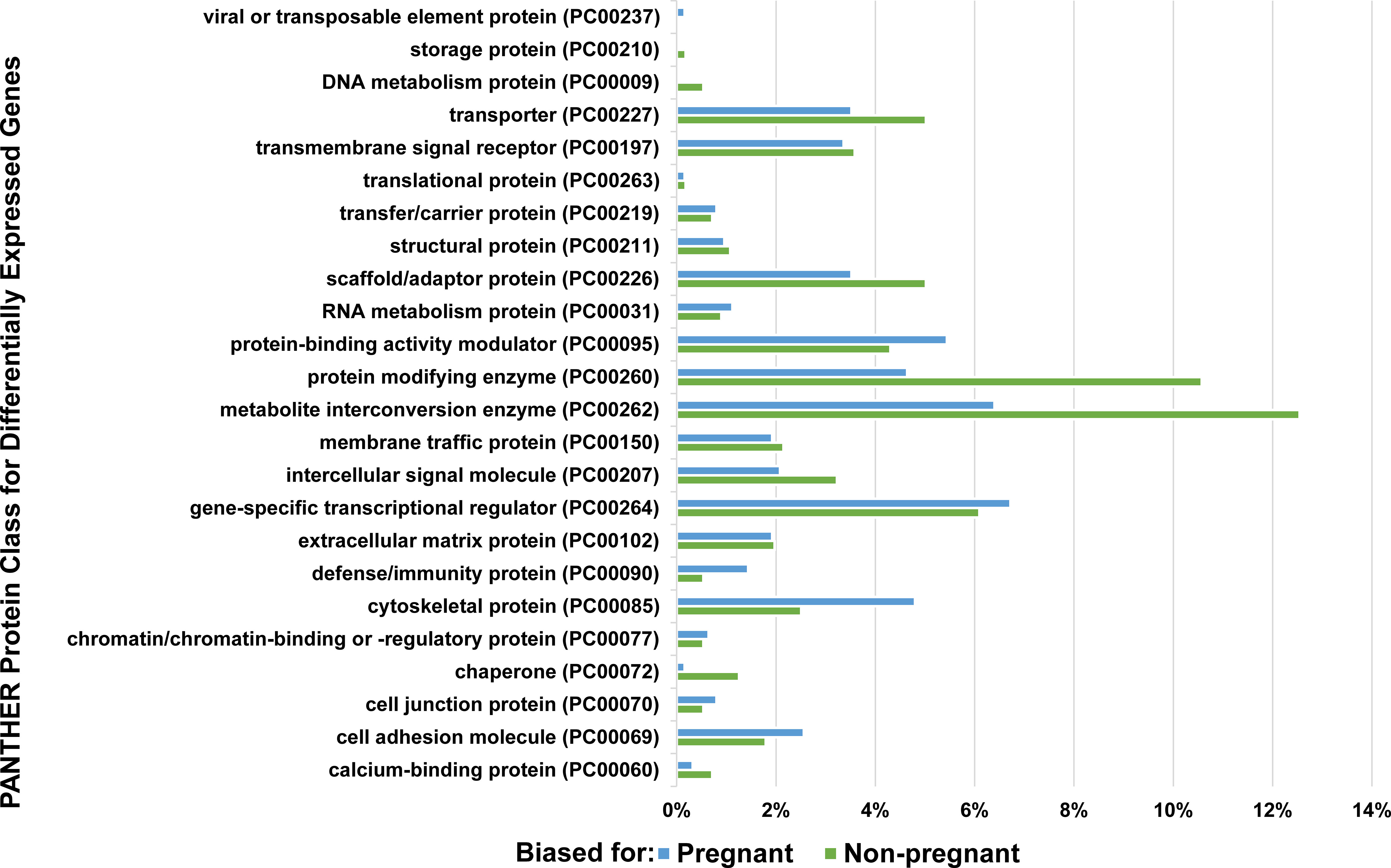
Figure 3 Protein classes from PANTHER for pregnancy-related differentially expressed genes. Differentially expressed genes from the second pairwise comparison (pregnant versus not, including all males, regardless of EE2-exposure) were BLAST searched for in the zebrafish (Danio rerio) proteome to find a corresponding protein ortholog. Resulting zebrafish protein IDs were used for a PANTHER protein class analysis. The percentage of proteins that fall into a specific protein class are report as the total number of proteins in a protein class divided by the total number of differentially expressed genes (pregnant males = 625 and non-pregnant males = 558). Differentially expressed genes that did not match to a zebrafish protein, and zebrafish proteins that did not have a PANTHER protein class are not displayed (pregnant males = 47%, and non-pregnant males = 34%).
Assessment of male banding ornamentation stages
The morphological changes in the male abdomen were categorized using a scale of ornamental band intensity, similar to that used to quantify ornamentation in female S. scovelli by Jones and colleagues (2001). To generate the ornamentation scale, we produced images representing a wide range of variation among exposed males. The requisite variation in male morphology was generated by exposing them to 4 combinations of EE2 concentration and exposure time. We used a 2ng/L exposure for seven days (n = 8 male fish) to replicate the concentrations from Rose et al. (2013). A 5ng/L exposure for seven days (n= 8 fish not used in the present study) represented the EE2 exposure of the present study. We also exposed males to 5ng/L for 18 days (n= 5) and 10ng/L for 18 days (n =5) to establish males that had full iridescence, approaching that observed in typical female specimens. Images were taken under a dissecting microscope to create the scale ranging from no banding pattern in control fish to female-like iridescent bands in the high exposure treatments (Figure 4). We used this newly established scale of ornamentation intensity to score the fish in the present study (which, as noted above, were not involved in the establishment of the scale). In a double-blind process, three reviewers were assigned the images of the male abdomens for the 16 fish included in the RNA-seq dataset for staging. The average score for each fish was taken across the three reviewers for statistical analysis. A Wilcoxon rank sum test was conducted due to the violation of normality for the data comparing the control males (n=8) with the EE2-exposed males (n=8) for band development.
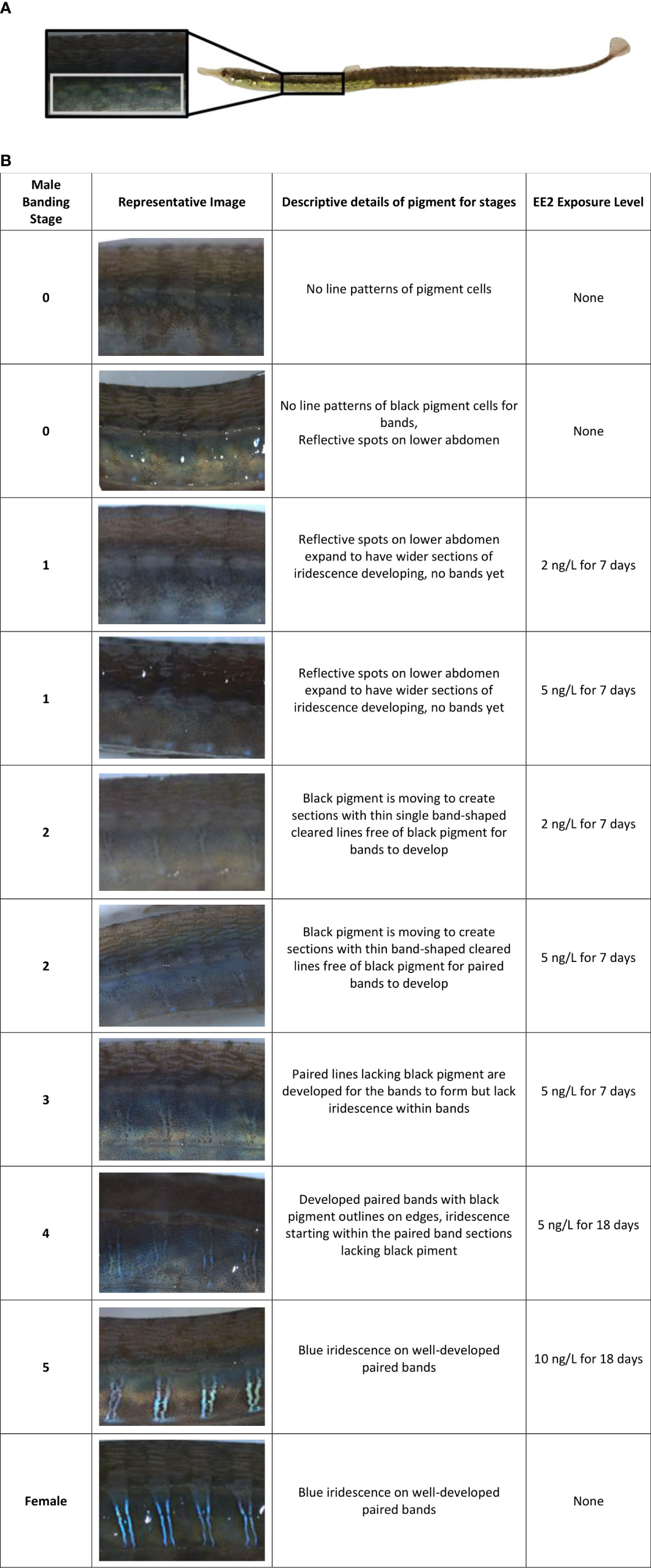
Figure 4 Developmental stages of the male banding pattern resulting from exposure to estrogen. The torso section outlined in gray indicates the location of the banding pattern on the abdomen of the Gulf pipefish on this non-exposed male in (A). The table for (B) contains images for the stages ranging from 0 with no band development through stage 5 with fully developed paired bands, similar to the last column displaying female bands. Descriptions with details of the pigmentation patterns and the EE2-exposure level of the fish is provided for each stage.
Results
Phenotypic response to EE2 exposure
During the seven days of 5ng/L of EE2 exposure, all males maintained their pregnancies with normal pouches and their offspring did not show any signs of brood reduction. The offspring developmental stages were assessed during the brood pouch dissection. The embryos for the control and EE2-exposed pregnant males were determined to be at the correct developmental stage for embryos at mid-gestation according to Sommer et al., 2012 and Schneider et al., 2023. Adult male fish in the EE2 treatment had begun demonstrating morphological signs of feminization during their seven days of exposure to estrogen, as seen in Partridge et al., 2010, whereas the control males did not show any phenotypic changes. Through an assessment of the images taken after seven days of EE2 contamination, all of the estrogen exposed males, including the pregnant and non-pregnant fish, had begun developing the female secondary sexual traits with banding patterns on their torsos. The staging of the band development was significantly higher in the EE2-exposed males with an average stage of 1.88 ± 0.37 compared to 0.17 ± 0.09 for the non-exposed males (Wilcoxon rank sum: W=1.5, p=0.0012).
Transcriptional response to EE2 exposure
The sequencing of the brood pouch RNA resulted in a total of 1,245 differentially expressed genes across pairwise comparisons between the four treatments (n=16, Figure 1). The brood pouch expression profiles were more greatly influenced by their pregnancy status than their exposure to EE2 (Figure 1). The largest number of differentially expressed genes were within the males in regard to their pregnancy status. There was 558 genes significantly upregulated in pregnant males and 625 genes upregulated in non-pregnant males (Figure 1, Supplemental Table 1A). There were only three genes that were statistically significantly different (p<0.05), as a result of EE2 exposure for all males, regardless of their pregnancy status, with all three genes being upregulated in the EE2-exposed males. These three estrogen-responsive genes included coagulation factor XIII A chain-like, peptidyl-prolyl cis-trans isomerase FKBP11, and transcription factor Sp7 isoform X1 (Supplemental Table 1B). Gene expression patterns were less altered by EE2 exposure in pregnant males compared with their non-pregnant counterparts. There were only 6 genes differentially expressed when comparing the control pregnant males with the pregnant males in the EE2 treatment (Supplemental Table 1C) However, when comparing the non-pregnant males across treatments, there were 53 genes reported as differentially expressed due to EE2 exposure (Supplemental Table 1D).
The most highly expressed genes in all four categories of male brood pouches included overrepresentation of genes related to cell proliferation, including lectin, keratin, actin, and myosin proteins (Supplemental Table 2). Additional genes detected in the top 25 of all four male groups included glyceraldehyde-3-phosphate dehydrogenase, creatine kinase muscle b, cytochrome c oxidase, cAMP-specific 3’ 5’-cyclic phosphodiesterase 4C-like isoform X, aldolase a fructose-bisphosphate b, and parvalbumin beta-like. The top expressed genes in this study for the brood pouch parallel the top expressed genes found in the Gulf pipefish skin and muscle transcriptome (Anderson et al., 2020). The top four genes for muscle and skin tissues included lectin proteins, glyceraldehyde-3-phosphate dehydrogenase, actin proteins, and creatine kinase muscle b, which were all found in the top 10 highest expressed genes in the brood pouches of pregnant males. The EE2-exposed males had similar highly expressed genes when compared with their control counterparts.
We identified genes regulating embryo development that were only highly expressed in the brood pouches for the control and EE2 exposed pregnant males. The reproductive gene hatching enzyme 1.2-like isoform X1 was the third most highly expressed transcript for both pregnant groups. This gene is likely categorized as a patristacin-like gene (Small et al., 2016) and was significantly downregulated in expression for non-pregnant males compared to the pregnant males. Another reproductive gene that was differentially expressed in pregnant males compared with non-pregnant males was low choriolytic enzyme-like isoform, which is likely another patristacin or patristacin-like gene. Given that the pregnant males were dissected during their mid-gestation stage, the offspring were likely nearing the developmental stage where embryos begin hatching from their chorion (Sommer et al., 2012; Whittington et al., 2015; Schneider et al., 2023).
The PANTHER analyses identified protein classes for all the differentially expressed genes that could be assigned to the zebrafish proteome. The protein classes with the largest number of proteins assigned, particularly for the non-pregnant males, included metabolite interconversion enzyme, protein modifying enzyme, and gene-specific transcriptional regulator. The pregnant male genes were assigned to the cytoskeletal protein and protein binding activity modulator categories in higher percentages compared to their non-pregnant counterparts. Many of the protein classes were related to restructuring occurring in the pouches either during or in preparation for pregnancy, such as extracellular matrix proteins, cytoskeletal proteins, cell adhesion proteins and cell junction proteins (Qin et al., 2020). Although the results indicated that the defense/immunity protein group had greater representation for the pregnant males, lectin proteins were found to be one of the most highly expressed genes across all four male categories rather than being differentially expressed, thus not being reported in the PANTHER data set. Interestingly, C-type lectin transcripts, which have been shown to be highly expressed in seahorse brood pouches and suggested as an immune response gene in the brood pouch, were upregulated in EE2-exposed non-pregnant males compared to their control counterparts (Small et al., 2013; Whittington et al., 2015; Kawaguchi et al., 2017).
Discussion
This study’s findings provide valuable insights into the effects of EE2 contamination on the brood pouch and stages of morphological changes in ornamentation of males, contributing to the development of the Gulf pipefish as an ecotoxicology model system. We also provide additional estrogen-responsive biomarkers for male brood pouches and a photographic scale for quantifying EE2-induced banding patterns in males that can be used in wild populations for predicting EE2-exposure. Additionally, we did not detect a substantial alteration in embryo morphology or developmental stage when comparing the offspring from the control and treatment males. We observed no direct evidence of brood reduction (Paczolt and Jones, 2010), and the staging of the embryos was similar to that of, Syngnathus typhle during mid prerelease developmental stages, as outlined by Schneider and colleagues (2023), when the eye is well developed and pigment has spread across the head and trunk. These results provide insight into why pipefish populations can remain reproductively active in locations of known endocrine-disruptor contamination. Gene expression data for the brood pouch also revealed that non-pregnant males show a greater transcriptional response to EE2 exposure when compared to pregnant males, providing support for previous studies where males were unable to receive eggs after exposure as non-pregnant or late-stage pregnant males when exposed to similar estrogen concentrations (Rose et al., 2013). Many of the top expressed transcripts were the same genes as the top expressed genes in the muscle and skin tissues studied by Anderson and colleagues (2020). The overall fold changes in gene expression patterns of the brood pouch paralleled those of the Gulf pipefish skin and muscle tissues (Anderson et al., 2020). The brood pouch transcriptome showed substantially fewer differentially expressed genes between control and estrogen treated fish (see Supplemental Table 1) compared to the dramatic effects of EE2 on the liver transcriptome (Rose et al., 2015), indicating variation in EE2 responsiveness across different organs.
The results from the current study indicate that timing of exposure to EE2 can affect the male pipefish’s ability to successfully carry developing offspring in their brood pouches. Previous studies have shown that male pipefish and seahorses, when exposed prior to mating, experience reduced or receding pouch tissue, difficulties receiving eggs from females, or termination of the pregnancy with non-surviving offspring (Partridge et al., 2010; Rose et al., 2013; Sárria et al., 2013; Qin et al., 2020). However, in this study the males in the EE2 treatment were not exposed until 24 hours after mating, and all showed normal development of their offspring at the midpoint of the pregnancy. Previous studies conducted at 2ng/L allowed for Gulf pipefish males to become impregnated and an increase in female reproductive yields, yet there was complete reproductive failure documented for males at 5 ng/L (Rose et al., 2013). Partridge and colleagues (2010) reported that males exposed to 5 ng/L of EE2 had difficulty receiving eggs and continued to have reduced mating activity several days after being removed from exposure. Interestingly, the male pipefish in this study did display feminizing morphological changes as a result of the 5 ng/L EE2 contamination, similar to the males in other studies (Partridge et al., 2010; Rose et al., 2013) with the major difference in the studies being the timing of the exposure relative to the pregnancy.
Understanding how populations of pipefish can remain reproductively active despite the threats of contamination is an important aspect of learning about ecotoxicology model systems in wild populations (Matthiessen et al., 2018). One potential explanation is that estrogen or other contaminants can vary in their concentrations over time depending on the frequency and quantity of contaminated effluent being introduced into coastal environments (Zuo et al., 2006). For example, the concentrations of EE2 detected in water bodies can vary seasonally in the dry season compared to the wet season and the distance of the fishes from where the wastewater effluent is introduced (Klaic and Jirsa, 2022). The range in detected concentrations in natural bodies of water could be due in part to varying rates of photodegradation of EE2 contamination, as a result of variation in sun exposure and water depths within pipefish habitats, given that EE2 has a half-life of less than 1.5 days during days with maximum sun (Zuo et al., 2006). Although the pipefish in this study were exposed to 5 ng/L of EE2, which is higher than the concentrations for surface waters reported in marine locations further from the wastewater effluent sources reported in Klaic and Jirsa (2022), wildlife are often exposed to several endocrine disrupting compounds that have similar estrogenic effects on the physiology and behaviors of exposed organisms (Matthiessen et al., 2018). The combination of varying concentration at the site of introduction and potential movement patterns of the fish can lead to individuals being exposed at different stages of their reproductive cycle. Future directions for this model system include monitoring wild populations for contamination levels and long-term surveys of their reproduction in locations with evidence of estrogen contamination. Studies in Tampa Bay have identified locations where 5ng/L EE2 has been detected entering the coastal waters where pipefish reside, providing the opportunity for testing multibiomarkers (Cook, 2015). Implementing the tools developed in the Gulf pipefish through comparative RNA-sequencing conducted over the past several years and identification of biomarker candidates will enable the next stage in biomonitoring in situ (Gouveia et al., 2019; Martyniuk et al., 2020).
One of the primary goals of the present study was to identify gene expression patterns in the brood pouch prior to mating and during the male’s pregnancy when success of developing offspring could be confirmed. The males in the present study were either non-pregnant after recently having given birth or halfway through the gestation of their offspring, representing a diverse array of metabolic and epithelial changes occurring in the male’s reproductive structure during the two different stages of reproduction. For example, non-pregnant males are typically preparing their pouch for mating (Carcupino et al., 1997). Thus, this stage could require the differentiation of the epithelial cells and other changes to the structure of the pouch flaps. On the other hand, pregnant males would likely experience epithelial proliferation, growth of new blood vessels, and waste removal within the pouch as the offspring develop (Scobell and MacKenzie, 2011). Since males included in this study were dissected during the middle of their pregnancy, the data set does not include genes related to fertilization, early gestation or parturition. Nevertheless, a time-series of the brood pouch transcriptome of the big-bellied seahorse (H. abdominalis) by Whittington and colleagues (2015) indicated that the mid-pregnancy stage differed the most from non-pregnant males, with 966 genes up or down regulated. In contrast, early, late, and post-parturition stages showed 527, 535, and 325 genes, respectively, that were differentially expressed compared to non-pregnant males. Comparatively, the largest difference in gene expression patterns in the seahorse brood pouch transcriptome occurred between mid-stage pregnant males and post-parturition males, indicating that the pouch undergoes a remodeling after the offspring are born to prepare for the next pregnancy (Whittington et al., 2015). This pattern is also seen in the pipefish brooding structures for Nerophis ophidion and Syngnathus rostellatus, where transcriptomes from early-stage pregnant males showed greater numbers of differentially expressed genes when compared to the parturition males, whereas the late stage transcriptomes were not as different from the parturition males (Parker et al., 2021). The serine/threonine-protein kinase transcripts were significantly biased in non-pregnant males in the present study and also in the Gulf pipefish brood pouch study conducted by Small et al. (2016). Seahorse males during post parturition experience an increase in estrogen receptor 2a expression in the brood pouch (Whittington et al., 2015), consistent with our findings in the Gulf pipefish. Curiously, neither seahorses nor pipefish in the present study showed a significant change in estrogen receptor expression when exposed to EE2 contamination (Qin et al., 2020).
Our differential expression analyses identified three EE2 responsive genes to use as biomarkers for contamination in all male brood pouches. One of these genes is coagulation factor XIII A chain-like, which is also upregulated by EE2 contamination in Gulf pipefish male’s skin tissues where the female-specific iridescent bands develop (Anderson et al., 2020). The exposure to EE2 resulted in a greater change in gene expression patterns for non-pregnant male brood pouches compared to pregnant males, which could lead to a decline in pouch function for males or their ability to receive eggs from females during future courtship events. EE2 exposure and pregnancy stage did not lead to differences in the representation of genes with the greatest expression levels, as seen by the similar breakdowns in the makeup of the top 25 most expressed genes across groups reported in Supplemental Table 2. The gene cathepsin was upregulated in the brood pouch of EE2 exposed pregnant males compare to their control counterparts and has also been identified as an estrogen biomarker in the skin and the liver of male Gulf pipefish (Rose et al., 2015; Anderson et al., 2020). The gene with the greatest upregulation in EE2 non-pregnant males, C-type lectin domain family 4 member M-like, which potentially regulates cell growth and immune responses, has also been shown to be over expressed in brood pouches of non-pregnant male seahorses compared to pregnant males (Whittington et al., 2015).
In conclusion, the gene expression changes identified in the brood pouch transcriptome and the documentation of male morphological changes in male banding patterns due to EE2 exposure contribute to the growing development of the Gulf pipefish as a model system for ecotoxicology. The EE2-responsive genes identified in the brood pouch for this study, along with the extreme gene expression patterns in the livers of EE2-exposed pipefish (Rose et al., 2015) and the genes previously identified that underlie the development of female secondary sexual traits in the skin of EE2-exposed males (Anderson et al., 2020), provide several excellent candidate genes for developing biomarkers to detect endocrine disruptor contamination in wild populations of pipefish. While previous studies indicated EE2-exposed pipefish males experienced a reduction in the function of their brood pouches prior to mating, males in this study successfully maintained their pregnancies when exposed 24 hours after copulation. Although the EE2 exposed males in this study displayed morphological feminization and began developing the female-specific sexually selected banding on their abdomen, the offspring displayed normal developmental patterns through the 7 days of exposure, indicating that the timing of exposure to EE2 is a critical factor affecting male pouches. Given that EE2 can alter the strength of sexual selection in the Gulf pipefish (Rose et al., 2013), affect the preferences of choosy females (Partridge et al., 2010), and has the potential to alter the operational sex ratios and effective population sizes due to non-receptive or sterile males (Lane et al., 2011), there is still a need to test the effects of contaminants on wild populations. The combination of the molecular tools and the well characterized morphological changes in EE2 exposed males provide an excellent arsenal of techniques for detecting estrogen contamination in wild populations across various ecosystems where the Gulf pipefish resides, particularly when quantification of contamination in water samples is difficult. The collection of previous laboratory research has now set the stage for the Gulf pipefish to become a well-established model system for investigating how endocrine disruptors, such as EE2, can alter the mating system of exposed pipefish populations in the natural environment.
Data availability statement
The RNA-seq raw reads generated in this study were deposited in NCBI's SRA database under BioProject PRJNA980712. The morphological data generated for this study is available https://zenodo.org/record/7996477.
Author contributions
Conceived and designed the experiments: ER and AJ. Performed the experiments: ER. Analyzed the data: ER, BJ, VA, AJ. Wrote the paper: ER, BJ, AJ. All authors contributed to the article and approved the submitted version.
Funding
Funding was provided by the National Science Foundation (DEB-1119261 and DEB-1953170 to AJ).
Acknowledgments
The authors would like to thank Caitlin Leslie for her assistance with animal husbandry and RNA extractions, Clay Small for providing the Gulf pipefish genome, and Megan Sims and Marie Hill for their assistance with the staging analyses.
Conflict of interest
The authors declare that the research was conducted in the absence of any commercial or financial relationships that could be construed as a potential conflict of interest.
Publisher’s note
All claims expressed in this article are solely those of the authors and do not necessarily represent those of their affiliated organizations, or those of the publisher, the editors and the reviewers. Any product that may be evaluated in this article, or claim that may be made by its manufacturer, is not guaranteed or endorsed by the publisher.
Supplementary material
The Supplementary Material for this article can be found online at: https://www.frontiersin.org/articles/10.3389/fmars.2023.1138597/full#supplementary-material
Supplementary Table 1 | Gene expression patterns for all comparative groups. Differentially expressed genes for all control and EE2-exposed males (A), all pregnant and non-pregnant males (B), pregnant control and EE2-exposed males (C), and non-pregnant control and EE2-exposed males (D).
Supplementary Table 2 | Top 50 expressed transcripts for all four male groups. Average TPM is provided along with the NCBI protein match for each of the highest expressed transcripts for the four male groups.
Supplementary Table 3 | Counts for all genes. TPM counts provided for all the transcripts generated from the brood pouches for all control and EE2-exposed males.
References
Allen Y., Scott A., Matthiessen P., Haworth S., Thain J., Feist S. (1999). Survey of estrogenic activity in united kingdom estuarine and coastal waters and its effects on gonadal development of the flounder Platichthys flesus. Environ. Toxicol. Chem. 18, 1791–1800. doi: 10.1002/etc.5620180827
Anderson A. P., Jones A. G. (2019). Choosy gulf pipefish males ignore age but prefer active females with deeply keeled bodies. Anim. Behav. 155, 37–44. doi: 10.1016/j.anbehav.2019.05.018
Anderson A. P., Rose E., Flanagan S. P., Jones A. G. (2020). The estrogen-responsive transcriptome of female secondary sexual traits in the gulf pipefish. J. Heredity 111 (3), 294–306. doi: 10.1093/jhered/esaa008
Bolger A. M., Lohse M., Usadel B. (2014). Trimmomatic: a flexible trimmer for illumina sequence data. Bioinformatics 30 (15), 2114–2120. doi: 10.1093/bioinformatics/btu170
Carcupino M., Baldacci A., Mazzini M., Franzoi P. (1997). Morphological organization of the male brood pouch epithelium of Syngnathus abaster risso (Teleostea, syngnathidae) before, during, and after egg incubation. Tissue Cell 29 (1), pp.21–pp.30. doi: 10.1016/S0040-8166(97)80068-7
Cook M. M. (2015). Endocrine-disrupting compounds: measurement in Tampa bay, removal from sewage and development of an estrogen receptor model (University of South Florida ProQuest Dissertations Publishing, 3688382).
D’Alvise N. P., Richard S., Aublanc P., Bunet R., Bonnefont J. L. (2020). When male seahorses take the female contraceptive pill. Environ. Sci. pollut. Res. 27 (14), 16528–16538. doi: 10.1007/s11356-020-08152-1
Dawson C. E. (1982). Fishes of the Western north Atlantic, part 8, order gasterosteiformes, suborder syngnathoidei (New Haven, CT: Sears Foundation for Marine Research, Yale University).
DeCourten B. M., Forbes J. P., Roark H. K., Burns N. P., Major K. M., White J. W., et al. (2020). Multigenerational and transgenerational effects of environmentally relevant concentrations of endocrine disruptors in an estuarine fish model. Environ. Sci. Technol. 54 (21), 13849–13860. doi: 10.1021/acs.est.0c02892
Flanagan S. P., Johnson J. B., Rose E., Jones A. G. (2014). Sexual selection on female ornaments in the sex-role-reversed gulf pipefish (Syngnathus scovelli). J. Evolution. Biol. 27 (11), 2457–2467. doi: 10.1111/jeb.12487
Flanagan S. P., Rose E., Jones A. G. (2016). Population genomics reveals multiple drivers of population differentiation in a sex-role-reversed pipefish. Mol. Ecol. 25 (20), 5043–5072. doi: 10.1111/mec.13794
Flanagan S. P., Rose E., Jones A. G. (2021). The population genomics of repeated freshwater colonizations by gulf pipefish. Mol. Ecol. 30 (7), 1672–1687. doi: 10.1111/mec.15841
Gouveia D., Almunia C., Cogne Y., Pible O., Degli-Esposti D., Salvador A., et al. (2019). Ecotoxicoproteomics: a decade of progress in our understanding of anthropogenic impact on the environment. J. Proteomics 198, 66–77. doi: 10.1016/j.jprot.2018.12.001
Jobling S., Casey D., Rodgers-Gray T., Oehlmann J., Schulte-Oehlmann U., Pawlowski S., et al. (2003). Comparative responses of molluscs and fish to environmental estrogens and an estrogenic effluent. Aquat. Toxicol. 65 (2), 205–220. doi: 10.1016/S0166-445X(03)00134-6
Jones A. G., Avise J. C. (1997). Microsatellite analysis of maternity and the mating system in the gulf pipefish Syngnathus scovelli, a species with male pregnancy and sex-role reversal. Mol. Ecol. 6 (3), 203–213. doi: 10.1046/j.1365-294X.1997.00173.x
Jones A. G., Rosenqvist G., Berglund A., Arnold S. J., Avise J. C. (2000). The Bateman gradient and the cause of sexual selection in a sex–role–reversed pipefish. Proc. R. Soc. London. Ser. B: Biol. Sci. 267 (1444), 677–680. doi: 10.1098/rspb.2000.1055
Jones A. G., Walker D., Avise J. C. (2001). Genetic evidence for extreme polyandry and extraordinary sex-role reversal in a pipefish. Proc. R. Soc. London. Ser. B: Biol. Sci. 268 (1485), 2531–2535. doi: 10.1098/rspb.2001.1841
Kawaguchi M., Okubo R., Harada A., Miyasaka K., Takada K., Hiroi J., et al. (2017). Morphology of brood pouch formation in the pot-bellied seahorse hippocampus abdominalis. Zool. Lett. 3, 1–16. doi: 10.1186/s40851-017-0080-9
Kidd K., Blanchfield P., Mills K., Palace V., Evan R., Lazorchak J., et al. (2007). Collapse of a fish population after exposure to synthetic estrogen. PNAS 104, 8897–8901. doi: 10.1073/pnas.0609568104
Kim D., Langmead B., Salzberg S. L. (2015). HISAT: a fast spliced aligner with low memory requirements. Nat. Methods 12 (4), 357–360. doi: 10.1038/nmeth.3317
Kim D., Paggi J. M., Park C., Bennett C., Salzberg S. L. (2019). Graph-based genome alignment and genotyping with HISAT2 and HISAT-genotype. Nat. Biotechnol. 37 (8), 907–915. doi: 10.1038/s41587-019-0201-4
Klaic M., Jirsa F. (2022). 17α-ethinylestradiol (EE2): concentrations in the environment and methods for wastewater treatment–an update. RSC Adv. 12 (20), 12794–12805. doi: 10.1039/D2RA00915C
Lane J. E., Forrest M. N., Willis C. K. (2011). Anthropogenic influences on natural animal mating systems. Anim. Behav. 81 (5), 909–917. doi: 10.1016/j.anbehav.2011.02.003
Leung Y. F., Dowling J. E. (2005). Gene expression profiling of zebrafish embryonic retina. Zebrafish 2 (4), 269–283. doi: 10.1089/zeb.2005.2.269
Martyniuk C. J., Feswick A., Munkittrick K. R., Dreier D. A., Denslow N. D. (2020). Twenty years of transcriptomics, 17alpha-ethinylestradiol, and fish. Gen. Comp. Endocrinol. 286, 113325. doi: 10.1016/j.ygcen.2019.113325
Matthiessen P., Wheeler J. R., Weltje L. (2018). A review of the evidence for endocrine disrupting effects of current-use chemicals on wildlife populations. Crit. Rev. Toxicol. 48 (3), 195–216. doi: 10.1080/10408444.2017.1397099
Mi H., Muruganujan A., Casagrande J. T., Thomas P. D. (2013). Large-Scale gene function analysis with the PANTHER classification system. Nat. Protoc. 8 (8), 1551–1566. doi: 10.1038/nprot.2013.092
Mi H., Muruganujan A., Ebert D., Huang X., Thomas P. D. (2019). PANTHER version 14: more genomes, a new PANTHER GO-slim and improvements in enrichment analysis tools. Nucleic Acids Res. 47 (D1), D419–D426. doi: 10.1093/nar/gky1038
Nash J. P., Kime D. E., van der Ven L. T., Wester P. W., Brion F., Maack G., et al. (2004). Long-term exposure to environmental concentrations of the pharmaceutical ethynylestradiol causes reproductive failure in fish. Environ. Health Perspect. 112 (17), 1725–1733. doi: 10.1289/ehp.7209
Orlando E. F., Guillette L. J. Jr. (2007). Sexual dimorphic responses in wildlife exposed to endocrine disrupting chemicals. Environ. Res. 104 (1), 163–173. doi: 10.1016/j.envres.2006.06.002
Paczolt K. A., Jones A. G. (2010). Post-copulatory sexual selection and sexual conflict in the evolution of male pregnancy. Nature 464 (7287), 401–404. doi: 10.1038/nature08861
Parker J., Dubin A., Schneider R., Wagner K. S., Jentoft S., Böhne A., et al. (2021). Immunological tolerance in the evolution of male pregnancy. Mol. Ecol. 32 (4), 819–840. doi: 10.1111/mec.16333
Partridge C., Boettcher A., Jones A. G. (2010). Short-term exposure to a synthetic estrogen disrupts mating dynamics in a pipefish. Horm. Behav. 58 (5), 800–807. doi: 10.1016/j.yhbeh.2010.08.002
Pertea M., Kim D., Pertea G. M., Leek J. T., Salzberg S. L. (2016). Transcript-level expression analysis of RNA-seq experiments with HISAT, StringTie and ballgown. Nat. Protoc. 11 (9), 1650–1667. doi: 10.1038/nprot.2016.095
Pertea G., Pertea M. (2020). GFF utilities: GffRead and GffCompare. F1000Research 9, 304. doi: 10.12688/f1000research.23297.1
Pertea M., Pertea G. M., Antonescu C. M., Chang T. C., Mendell J. T., Salzberg S. L. (2015). StringTie enables improved reconstruction of a transcriptome from RNA-seq reads. Nat. Biotechnol. 33 (3), 290–295. doi: 10.1038/nbt.3122
Peterson E. K., Buchwalter D. B., Kerby J. L., LeFauve M. K., Varian-Ramos C. W., Swaddle J. P. (2017). Integrative behavioral ecotoxicology: bringing together fields to establish new insight to behavioral ecology, toxicology, and conservation. Curr. Zool. 63 (2), 185–194. doi: 10.1093/cz/zox010
Porte C., Janer G., Lorusso L. C., Ortiz-Zarragoitia M., Cajaraville M. P., Fossi M. C., et al. (2006). Endocrine disruptors in marine organisms: approaches and perspectives. Comp. Biochem. Physiol. Part C: Toxicol. Pharmacol. 143 (3), 303–315. doi: 10.1016/j.cbpc.2006.03.004
Qin G., Zhang Y., Zhang B., Zhang Y., Liu Y., Lin Q. (2020). Environmental estrogens and progestins disturb testis and brood pouch development with modifying transcriptomes in male-pregnancy lined seahorse. Hippocampus erectus. Sci. Total Environ. 715, 136840. doi: 10.1016/j.scitotenv.2020.136840
Ramesh B., Small C. M., Healey H., Johnson B., Barker E., Currey M., et al. (2023). Improvements to the gulf pipefish syngnathus scovelli genome. Gigabyte. doi: 10.46471/gigabyte.76
R Core Team (2015). R: a language and environment for statistical computing (Vienna, Austria: R Foundation for Statistical Computing). Available at: http://www.R-project.org/.
Ripley J. L. (2009). Osmoregulatory role of the paternal brood pouch for two syngnathus species. Comp. Biochem. Physiol. Part A: Mol. Integr. Physiol. 154 (1), 98–104. doi: 10.1016/j.cbpa.2009.05.003
Ripley J. L., Williams P. S., Foran C. M. (2010). Morphological and quantitative changes in paternal brood-pouch vasculature during embryonic development in two syngnathus pipefishes. J. Fish Biol. 77 (1), 67–79. doi: 10.1111/j.1095-8649.2010.02659.x
Robinson M. D., McCarthy D. J., Smyth G. K. (2010). edgeR: a bioconductor package for differential expression analysis of digital gene expression data. bioinformatics 26 (1), 139–140. doi: 10.1093/bioinformatics/btp616
Rose E., Flanagan S. P., Jones A. G. (2015). The effects of synthetic estrogen exposure on the sexually dimorphic liver transcriptome of the sex-role-reversed gulf pipefish. PloS One 10 (10), e0139401. doi: 10.1371/journal.pone.0139401
Rose E., Paczolt K. A., Jones A. G. (2013). The effects of synthetic estrogen exposure on premating and postmating episodes of selection in sex-role-reversed gulf pipefish. Evolution. Appl. 6 (8), 1160–1170. doi: 10.1111/eva.12093
Saaristo M., Craft J. A., Lehtonen K. K., Lindström K. (2010). An endocrine disrupting chemical changes courtship and parental care in the sand goby. Aquat. Toxicol. 97, 285–292. doi: 10.1016/j.aquatox.2009.12.015
Sárria M. P., Santos M. M., Castro L. F. C., Vieira N. M., Monteiro N. M. (2013). Estrogenic chemical effects are independent from the degree of sex role reversal in pipefish. J. Hazard Mater. 88 (1), 75–80. doi: 10.1016/j.jhazmat.2013.10.043
Sárria M. P., Santos M. M., Reis-Henriques M. A., Vieira N. M., Monteiro N. M. (2011). The unpredictable effects of mixtures of androgenic and estrogenic chemicals on fish early life. Environ. Int. 37 (2), 418–424. doi: 10.1016/j.envint.2010.11.004
Schneider R. F., Woltering J. M., Adriaens D., Roth O. (2023). A comparative analysis of the ontogeny of syngnathids (pipefishes and seahorses) reveals how heterochrony contributed to their diversification. Dev. Dyn. 252 (5), 553–588. doi: 10.1002/dvdy.551
Scobell S. K., MacKenzie D. S. (2011). Reproductive endocrinology of syngnathidae. J. Fish Biol. 78 (6), 1662–1680. doi: 10.1111/j.1095-8649.2011.02994.x
Shokri M. R., Gladstone W., Jelbart J. (2009). The effectiveness of seahorses and pipefish (Pisces: syngnathidae) as a flagship group to evaluate the conservation value of estuarine seagrass beds. Aquat. Conserv: Mar. Freshw. Ecosyst. 19, 588–595. doi: 10.1002/aqc.1009
Small C. M., Bassham S., Catchen J., Amores A., Fuiten A. M., Brown R. S., et al. (2016). The genome of the gulf pipefish enables understanding of evolutionary innovations. Genome Biol. 17 (1), 1–23. doi: 10.1186/s13059-016-1126-6
Small C. M., Harlin-Cognato A. D., Jones A. G. (2013). Functional similarity and molecular divergence of a novel reproductive transcriptome in two male-pregnant Syngnathus pipefish species. Ecol. Evol. 3 (12), 4092–4108. doi: 10.1002/ece3.763
Sommer S., Whittington C. M., Wilson A. B. (2012). Standardised classification of pre-release development in male-brooding pipefish, seahorses, and seadragons (Family syngnathidae). BMC Dev. Biol. 12 (1), 1–6. doi: 10.1186/1471-213X-12-39
Thomas P. D., Campbell M. J., Kejariwal A., Mi H., Karlak B., Daverman R., et al. (2003). PANTHER: a library of protein families and subfamilies indexed by function. Genome Res. 13 (9), 2129–2141. doi: 10.1101/gr.772403
Ueda N., Partridge C., Bolland J., Hemming J., Sherman T., Boettcher A. (2005). Effects of an environmental estrogen on male gulf pipefish, Syngnathus scovelli (Evermann and Kendall), a male brooding teleost. Bull. Environ. contam. Toxicol. 74 (6), 1207–1212. doi: 10.1007/s00128-005-0709-9
Whittington C. M., Griffith O. W., Qi W., Thompson M. B., Wilson A. B. (2015). Seahorse brood pouch transcriptome reveals common genes associated with vertebrate pregnancy. Mol. Biol. Evol. 32 (12), 3114–3131. doi: 10.1093/molbev/msv177
Keywords: syngnathidae, synthetic estrogen, brood pouch, transcriptomics, male pregnancy
Citation: Rose E, Johnson BD, Armitage V and Jones AG (2023) The response of the brood pouch transcriptome to synthetic estrogen exposure in the Gulf pipefish (Syngnathus scovelli). Front. Mar. Sci. 10:1138597. doi: 10.3389/fmars.2023.1138597
Received: 05 January 2023; Accepted: 29 May 2023;
Published: 29 June 2023.
Edited by:
Siu Gin Cheung, City University of Hong Kong SAR, ChinaReviewed by:
Ralf Friedrich Schneider, Helmholtz Association of German Research Centres (HZ), GermanyJorge Palma, University of Algarve, Portugal
Copyright © 2023 Rose, Johnson, Armitage and Jones. This is an open-access article distributed under the terms of the Creative Commons Attribution License (CC BY). The use, distribution or reproduction in other forums is permitted, provided the original author(s) and the copyright owner(s) are credited and that the original publication in this journal is cited, in accordance with accepted academic practice. No use, distribution or reproduction is permitted which does not comply with these terms.
*Correspondence: Emily Rose, ZXJvc2VAdmFsZG9zdGEuZWR1