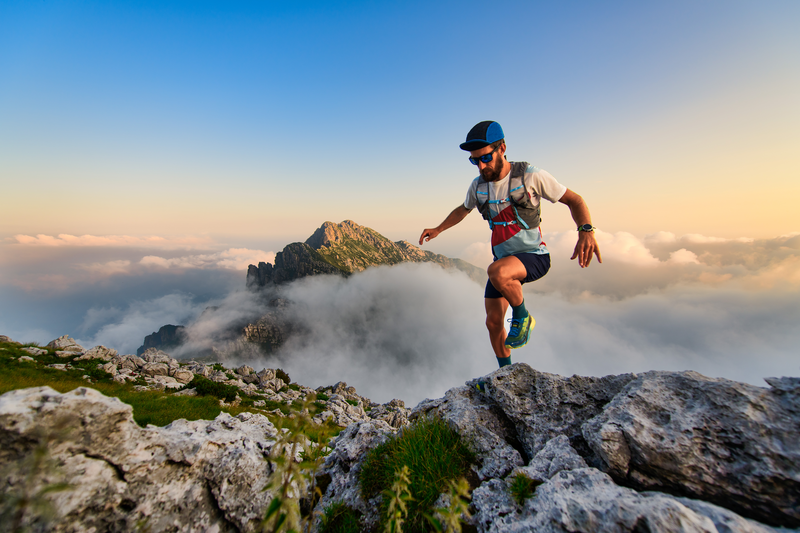
95% of researchers rate our articles as excellent or good
Learn more about the work of our research integrity team to safeguard the quality of each article we publish.
Find out more
ORIGINAL RESEARCH article
Front. Mar. Sci. , 07 March 2023
Sec. Marine Conservation and Sustainability
Volume 10 - 2023 | https://doi.org/10.3389/fmars.2023.1135521
This article is part of the Research Topic The Dolphins of Sarasota Bay: Lessons from 50 years of Research and Conservation View all 14 articles
Multiple techniques have been used for estimating age in bottlenose dolphins (Tursiops truncatus). The longest established technique is via counts of growth later groups (GLGs) in the longitudinal section of an extracted mandibular tooth. Previous studies to validate GLGs have primarily used younger dolphins (less than 16yrs old) due to the limited number of known age older animals. This study assessed the accuracy of age estimates for bottlenose dolphins using both GLG analysis and a newer technique, pectoral flipper radiography (PFR) for dolphins ranging from 0 to 58yrs, with a majority of samples (70%) over 16yrs. GLGs were assessed by two expert independent readers on tooth sections from 52 different dolphins (85% over 16yrs, maximum age 58yrs), and assessments of PFRs were performed by two experienced veterinarians on 37 dolphins (54% over 16yrs, maximum age 54yrs). Results demonstrated both techniques became less accurate in older dolphins, particularly those > 30yrs, with errors as large as 37yrs for GLG estimates. The root mean squared error (RMSE) for age estimation using GLGs for dolphins ≤15 years of age was 1.46yrs compared to 1.58yrs using PFRs demonstrating the accuracy of both techniques in this age bracket. For dolphins >30yrs, RMSE increased to 17yrs in GLG aging and 8.25yrs in PFR. Challenges in obtaining accurate age estimates in GLGs were primarily due to sectioning and staining difficulties in visualizing the newest GLG layers, in the very old animals. Complications in reading the layers included obtaining a good section, the presence of accessory layers, GLG compression, and tooth curvature or wear removing the neonatal line influencing aging biases. In pectoral flipper aging, the primary challenge was obtaining a true dorsoventral radiograph in a live dolphin during a health assessment, as well as the subjective assessment of scoring and reliance on degenerative changes accurately estimate age in geriatric dolphins. While access to the radiography equipment can be a limiting factor, the improved accuracy for age estimation in adult dolphins, the less invasive nature, and reduced processing time to results make pectoral radiography a preferred alternative technique for estimating age in live dolphins.
Accurate knowledge of age is important for understanding population demographics and interpreting biological data. In marine mammals, age estimation can be challenging, particularly in species with a long life span that show minimal external signs of aging and for which growth slows or ceases as they approach adulthood (Read et al., 2018). In live-stranded individuals, knowledge of age can be paramount in the decision to release, attempt to rehabilitate, or euthanize (Moore et al., 2007). In addition, during mass stranding or unusual mortality events, determining the demographic groups affected can help to understand the underlying factors for mortality and the potential consequences for the population (Calzada et al., 1994). Age estimates at sexual maturation are important for predicting population dynamics and recovery (Schwacke et al., 2022). In bottlenose dolphins (Tursiops truncatus), growth models have established sexual dimorphism with adult males being larger than females (Read et al., 1993; Stolen et al., 2002), and multiple phases of growth, including a rapid increase around the age of sexual maturity (Mcfee et al., 2010). Consequently, total straight length can be roughly used as an indicator of categorical age class (calf<200cm vs subadult 200-240cm vs adult >240cm); however it is less useful for estimating chronological age, particularly following sexual maturity when growth rate approaches zero (Mcfee and Hopkins-Murphy, 2002).
Determining the age of dolphins via tooth examination has been explored since the first dolphins were successfully maintained under human care in the 1950’s. Dentine analysis was first used to age striped dolphins (Stenella coeruleoalba) in 1953 and then applied to bottlenose dolphins five years later (Nishiwaki and Yagi, 1953; Sergeant, 1959; Scheffer and Myrick, 1980). Scientific and veterinary research developed methods to extract a tooth from a live dolphin under local anesthesia, section the tooth and count the growth layer groups (GLGs) to provide an indication of age (Sergeant et al., 1973; Ridgway et al., 1975). Numerous studies have been performed to understand the timing of dentine deposits in odontocete teeth, determining the annual deposition by extracting multiple teeth from the same individual at defined time periods (Perrin and Myrick, 1980; Myrick Jr et al., 1984; Hohn et al., 1989). It is important to denote that GLGs do not automatically represent annual layers with specific exceptions (such as the first layer or accessory layers) described, however the repetitive pattern of a growth layer group is understood to be representative of a single year of growth, within context of other dental anatomy (Figure 1) (Hohn et al., 1989). In some species such as the Franciscana dolphin (Pontoporia blainvillei) there is value in including cementum in the tooth reads, even though comparison of dentine and cementum found dentine to be more accurate (Kasuya and Brownell Jr, 1979). In other species such as (Steno bredanensis) regular growth layers are present in both dentine and cementum (Miyazaki, 1980). For Tursiops truncatus cementum has been found to be inconsistent and while there is value in some specific cases generally it is too thin to be included in most Tursiops truncatus age estimates (Myrick et al., 1990; Klevezal, 1996).
There is a vast body of literature documenting the progression of the GLG aging technique, applying the results of GLG ages to biological data (Hui, 1978; Hohn and Fernandez, 1999; Read et al., 2018), but also acknowledging its limitations. It has been widely accepted that GLG layers are deposited annually despite early publications that acknowledged the unpredictability of the dentine deposition when more than 15 GLGs are present (Hui, 1980). Historically, studies concluded the maximum dolphin lifespan to be 25 years of age based on GLG layers (Sergeant et al., 1973). However, more recent GLG studies with improved section preparation, the longevity of dolphins in human care, and longitudinal photo-identification studies of a wild dolphin population demonstrate dolphins can live far past 25 years with lifespans extending beyond 60 years (Hohn and Fernandez, 1999; Venn-Watson et al., 2011; Wells, 2014). The factors driving the deposition are currently unknown, however seasonal changes, nutrition, growth rate and health status are likely to influence the rate of deposition. It is unknown whether the deposition of growth layer groups slows down once a dolphin’s growth has slowed and maximum length is approached, however theoretically, GLGs should continue to be deposited as long as the tooth pulp cavity remains open (Figure 2).
Figure 2 A 58 year old female bottlenose dolphin tooth section. Only 22 GLG are clearly visible highlighting the challenges of aging old dolphins via this technique. Image courtesy of W. McFee.
Validation of the GLG technique has not previously been possible in dolphins over 16 years of age due to a lack of samples available from known aged dolphins (Hohn et al., 1989). Despite the well-documented limitations of tooth aging, GLG tooth aging remains the primary method to estimate the age of wild bottlenose dolphins due to the insufficiency of alternative aging techniques. Alternative methods to estimate age have included morphometrics such as total straight length, however the asymptotic nature of dolphin growth curves results in length being an inaccurate assessment of age in older animals (Mcfee et al., 2012). More recently, dental radiography was demonstrated as a viable alternative to tooth extraction, however accuracy in age estimation decreased significantly in dolphins > 11 years of age, therefore this technique could not be used across the dolphin’s full lifespan (Herrman et al., 2020). Bone density was extensively researched but unfortunately did not provide an accurate correlation with age (Powell et al., 2019). Pectoral flipper radiography is highly accurate in young animals up to adulthood, but relies upon degenerative osteoarthritic changes to accurately estimate age in older individuals when all bone growth plates are fully consolidated. Therefore flipper radiography becomes more subjective in dolphins >25 years of age (Barratclough et al., 2019a) (Figure 3). Epigenetics via DNA methylation is a promising new technology to accurately estimate age, and may be applicable across the dolphin’s lifespan (Barratclough et al., 2021; Peters et al., 2022).
Figure 3 A pectoral flipper radiograph demonstrating the open physeal plates present in a young sexually immature dolphin. The scores for each of the 16 locations are provided on the radiograph. L, Left pectoral flipper.
The primary objective of this study was to examine the accuracy of the two aging techniques (dentinal GLG and pectoral flipper radiography) in dolphins confirmed to be older than 15 years of age. The second objective was the application of the previously established PFR technique for real time age estimates during dolphin health assessments. Understanding the uncertainty in age estimates from the two techniques across the dolphin lifespan will aid in interpretation and application of estimates for future population demographic analyses, and for decisions related to management of live-stranded cetaceans.
Fifty-two teeth were obtained via standard extraction procedure during necropsy at the Navy Marine Mammal Program (n=27) and the Mote Marine Laboratory Stranding Investigations Program (SIP) (n=25). Of the 52 teeth examined in this study, 23 were from females and 29 were from males. The Navy Marine Mammal Program (MMP) was established in 1959; dolphins acquired for the program had their ages estimated via tooth GLGs and morphometrics (Ridgway et al., 1975). Dolphins born at the MMP are of exact known age, currently up to 38 years. Samples from these animals provide the opportunity to validate the tooth aging technique in dolphins > 16 years old (Venn-Watson et al., 2015). From the 27 MMP teeth, seven dolphins were born at the program and were of exact known age. The remaining 20 animals were of known minimum age, i.e. they were not born at the program but had been part of the program for a specific number of years and are referred to as inferred age. Minimum age (time at the MMP) for these 20 individuals ranged from 21 – 47 years with estimated ages at the time of entry ranging from 4-9 years.
In Sarasota Bay, Florida, wild dolphins have been monitored by the Sarasota Dolphin Research Program through tagging, photo-identification surveys and veterinary health assessments since 1970 allowing documentation of wild dolphins estimated to be > 50 years of age (Wells et al., 1987; Scott et al., 1990; Wells and Scott, 1999; Wells, 2014; Barratclough et al., 2019b). Of the 25 stranded Sarasota dolphins, collected by SIP for necropsy 10 animals had been sighted as young calves and had known year of birth. The remaining 15 animals had inferred age estimates provided from first the known sighting as an adult (as early as 1975) providing a known minimum age or from previous tooth extraction and GLG age.
Inferred ages for the 52 teeth ranged from six to 58 years. Where possible, tooth 13-18 was extracted; however, due to tooth wear or missing teeth, this was not always possible. Teeth were submitted for sectioning and staining to either W. McFee (n=22) at NOAA’s Hollings Marine Laboratory, South Carolina or A. Hohn at NOAA’s Southeast Fisheries Science Center, Beaufort, North Carolina (n=30). Samples were processed according to standardized techniques as described below with the method adapted from the protocol established at the NOAA Southwest Fisheries Science Center and based on previous publications (Sergeant, 1959; Sergeant et al., 1973; Hohn, 1980; Wainwright and Walker, 1988; Hohn et al., 1989; Myrick et al., 1990; Hohn and Fernandez, 1999; Mcfee et al., 2012). Dentinal GLGs were assessed throughout this study, cementum GLGs were not examined, hereafter GLGs is referring to dentinal GLGs unless otherwise stated.
Thick sectioning was performed using a Buehler ® Isomet™ Low Speed Saw (Lake Bluff, Illinois, USA) and diamond wafer blade. RDO decalcifying agent was applied to the wedged tooth section until the wedge was pliable for the full section then rinsed in water for a minimum of 12 hours. Thin sectioning was performed using a Leica SM 2000R (Nussloch, Germany) sledge microtome with a Physitemp thermoelectric freezing stage (Clifton, New Jersey, USA). The thin sections were individually stained using Harris’ or Mayer’s hematoxylin prior to mounting on a slide for microscopic review. W. McFee provided an initial blind review. The slides were then submitted to M. Stolen (BWRI) for a second blind review. The two readers provided age estimates read to the nearest 0.25 GLGs for animals with fewer than three GLGs and the nearest 0.5 GLGs for greater than three GLGs. Ideally readings that vary by more than 3 GLGs should require a new tooth section and a repeat of the process, however a second tooth was not available for the archived cases. For every tooth, an average score was produced based on the three readings 1 week apart by each reader. The final score and age assigned to the GLG was calculated by combing each of the readers final estimates and dividing this by two. Readers evaluated each tooth and made notes on curvature, excessive wear, and the presence of pulp stones. Digital photographs of the slides made by W. McFee were also provided to A. Hohn (NOAA) for an opinion on reading quality of the tooth sections.pt?
A total of 37 pectoral flipper radiographs (PFR) were obtained from 36 dolphins; 12 from MMP dolphins, 10 from Sarasota health assessments and 15 from stranded dolphins in Sarasota Bay, following previously published techniques (Barratclough et al., 2019a). Radiographs can be taken of either flipper and a dorsal ventral view of the flipper including the distal radius to the distal phalanges (Figure 2). Of the 37 radiographs 13 were from females and 24 were from males. Of the 12 MMP dolphins, nine were known age from observed birth. Two had age estimated between 2-4 years of age based on morphometrics at the time of acquisition and one animal was estimated as 3yrs by GLG. Of the 25 Sarasota cases, 20 were known age from observed birth and five were known minimum age. In addition, 10 pectoral flipper radiographs were taken during 2019 and 2022 Sarasota Bay live dolphin health assessments, with one individual radiographed in both 2019 and 2022. Nine of these cases had known age and one individual was known minimum age. Of the 15 stranded cases, six were of known age and four had previous sighting data providing a minimum age estimate. Figure 4 depicts four radiographs with suggested scores for the growth plates and the given ages of the different stages allowing depiction of the predicted sequential changes in growth plate closure over time.
Radiographs were obtained as part of routine animal care under the authorization of U.S. Code, Title 10, USC 7524. Secretary of Navy Instruction 3900.41H directs that Navy marine mammals be provided the highest quality of care. The U.S. Navy Marine Mammal Program (MMP), Naval Information Warfare Center (NIWC) Pacific, houses and cares for a population of bottlenose dolphins and California sea lions in San Diego Bay (CA, USA). The MMP is accredited by AAALAC International and adheres to the national standards of the U.S. Public Health Service Policy on the Humane Care and Use of Laboratory Animals and the Animal Welfare Act. Pectoral flipper bone maturation scores were assigned independently by two blind reviewers, both marine mammal veterinarians with experience of the previously published technique (A.Barratclough and D. Garcia-Parraga). The established methodology requires a score of -1 to 8 to be applied to 16 different anatomic locations within the pectoral flipper with each score denoting the degree of development of the growth plate (Barratclough et al., 2019a). The metaphyseal region of the radius and ulna are the most informative along with the proximal and distal epiphysis of metacarpals II and III. The sex-specific equations reported by Barratclough et al. (2019a) were applied to predict the chronological age:
Sex-specific equations are required due to the different growth rates between male and female bottlenose dolphins and the different ages at which sexual and skeletal maturity occur. For example closure of the female radius growth plate is expected at 6-8 years whereas in males this does not occur until 10-12 years. By including 16 different scoring locations, the accuracy of age prediction is improved. Scores from the two reviewers were combined to provide an average age estimation for each individual. The known ages of the animals included for pectoral radiographs ranged from 0 – 58 years of age.
As archived tooth samples were used in this study, it was generally not possible to obtain both a radiograph and a tooth sample from the same individuals. There were eight dolphins which had both age estimation methods applied but the tooth extraction and PFR did not occur at the same age (one method performed several years before the other). Subsequently, analyses to assess the accuracy of GLG and PFR estimates were conducted separately using the two different sample sets.
The samples were stratified into three age categories: ≤15 years; 16-30 years; and > 30 years to facilitate comparison between the two sample sets. Error was calculated as the absolute difference between the actual and estimated ages, and proportional error as this value divided by the actual age. Root-mean-square error (RMSE) was calculated as the square root of the average of the squared differences. An analysis of variance (ANOVA) was performed to examine the potential influence of sex (male/female) and population (Sarasota/MMP) on errors. All statistical analyses were performed using R Studio version 4.2.1. The Metrics package was used to calculate the RMSE.
The median absolute error between the average age estimate from GLGs and the actual age increased with age category from 0.5 years in dolphins ≤15 years of age and up to 11.5 years in dolphins >30 years. The proportional error ranged from 0.0 to 0.60 (Table 1). The difference between the average estimate from the two readers ranged from 0 to 23 years. The scoring results are provided in Supplementary Materials. Of the 52 teeth reviewed digitally by A. Hohn, five were determined to be too poor quality to assess age accurately. It was recommended that these cases had new teeth sectioned and processed as the area above the pulp cavity was difficult to view. Unfortunately a duplicate sample was not available therefore these five cases were kept within the dataset as their margin of error was low in comparison to other specimens and their sections likely representative of the challenges of tooth sectioning. The RMSE for dolphins ≤15 years of age was 1.46 years increasing to 17 years of age for dolphins over the age of 30 (Table 1). ANOVA results did not find evidence that sex (p = 0.74) or population (p = 0.41) influenced errors, but differences among age categories were highly significant (p = 0.000007). Notes on the curvature and wear of each tooth found 2/27 MMP teeth to be very curved with no excessive wear reported. In Sarasota wear was reported in 3/25 teeth, but no curvatures reported. Three Sarasota teeth also had pulp stones observed. Four teeth were from dolphins first sighted as adults therefore, only a known minimum age was available, so these were not included in the boxplot analysis.
Table 1 Comparison of median error by age group estimated by growth layer group (GLG) analysis and pectoral flipper radiography (PFR). Values in parentheses are minimum and maximum.
The median absolute error between the average of the two radiograph scores and the actual age also increased with age category from 0.04 years in dolphins ≤15 years and up to 4.0 in dolphins >30 years of age. The difference in age estimation between the two reviewers ranged from 0 years to 10.2 years. PFR scoring results are presented in Supplementary Materials. RMSE error ranges from 3 months in those< 5 years and increases to +/- 5 years when >20 years of age. Of the 37 radiographs, age estimates were available for 30 cases (with known minimum age on 7 cases), PFR age estimates were within the expected RMSE in 70% of cases. The nine cases with RMSE > 4 years from the estimated age were all over 28 years of age with some as old as 57 years. For the radiographs included in this study we had one outlier which is a known age dolphin F123 from Sarasota who was known to be 22 years of age however the radiograph appeared much older at approximately 45 years of age. The RMSE for dolphins ≤15 years of age was 1.58 years and for dolphins >30 years of age was 8.25 years with all age categories presented in Table 1. ANOVA results showed sex (p=0.39) and location (p=0.07) not to influence results with age class (p=0.001) having significant influence on the accuracy of age prediction.
Scatter plots comparing the actual age with the predicted age for each method demonstrate the increased variance with age and the lack of difference between the two populations (Navy in blue and Sarasota in orange, Figure 5). Box plots of errors for the three different age categories (<15 years, 16-30 years and >30 years) show accurate predictions in the<15 year category with increases in variance observed with increasing age for both GLG and PFR (Figure 6). GLG errors in the >30 year age class not only had a wide range but were negatively biased (Figure 6).
Figure 5 Scatter plots of Inferred age versus Estimated age for (A) Tooth growth layer group analysis, (B) Pectoral Flipper Radiography. Dolphins from the Navy Marine Mammal program are represented in blue and dolphins from Sarasota Bay are in orange. Dashed 45° line represents exact agreement.
Figure 6 Boxplots of prediction error grouped by age range for (A) tooth growth layer group (GLG) age estimates, and (B) pectoral flipper radiography (PFR) age estimates. Boxes represent interquartile range, line within boxes represents median, whiskers represent non-outlier range, and points represent outliers.
Accuracy of age estimates in dolphins has been extensively researched, but prior studies have primarily used samples from younger dolphins for which age was definitively known from an observed birth (Kimura, 1980; Hohn et al., 1989; Barratclough et al., 2019a). Our study was the first to focus on assessing the accuracy of predictions for older dolphins (> 16 years), and our results demonstrates increased error in age estimates for older age classes. The largest errors were observed for dolphins in the 30+ age group, with maximum errors of 37 years and 23 years for GLG and PFR, respectively.
Over the last 30 years, numerous studies have been published on wild dolphins that use age estimates from GLGs to interpret biological data, determine age demographics, and to model age-specific survival (Stolen and Barlow, 2003; Mattson et al., 2006; Mcfee and Lipscomb, 2009; Wells, 2014; Schwacke et al., 2017; Mccormack et al., 2021). The results of this study provide evidence of negative age bias through both methods when estimating age in older dolphins (Figure 6). The cause of the bias is not completely understood. The likely primary source of error in obtaining an accurate estimate of age from GLGs is sections that do not fully include the compressed GLGs around the pulp cavity (for example, see Figure 7). In older animals, the correct orientation of the section relative to the pulp cavity is essential; obtaining such sections is affected by whether the tooth is curved or twisted or the actual location of small pulp cavity in a tooth. Excessive tooth wear in older dolphins can also impact GLG accuracy. It is not unusual that more than one tooth from each individual be sectioned, which was not possible in the current study using single archived samples. The open pulp cavity observed in the older MMP dolphins (Figure 2) demonstrates the capacity to continue depositing GLGs into old age, therefore with precise sectioning it is theoretically possible to use this technique in older dolphins. The challenges of achieving this precise section make the GLG technique highly specialized and not always obtainable.
Figure 7 Tooth cross section highlighting the area where the GLG counts are produced and the level of detail and perfect cross-section required to view the newest layers. Image courtesy of A. Hohn.
Deviations in tooth formation, potentially influenced by disease or environment, could also influence the accuracy of GLG aging. Varying dental pathologies, which could interfere with GLG counts, have been recorded in wild dolphins with conditions ranging from amelogenesis imperfecta, tooth impaction, accessory roots and dental caries (Brooks and Anderson, 1998). Dental disease in bottlenose dolphins in managed care has also been reported even with great lengths taken to maintain oral health (Meegan, 2016). It is possible that layer deposition could be influenced by nutrition, activity level, health status or environment, leading to variation in tooth formation or wear that compounds over time. Previous studies have documented mineral density dentinal markers associated with nutritional stress in short-finned pilot whales (Globicephala macrorhynchus) and Peruvian dusky dolphins (Lagenorhynchus obscurus) (Manzanilla, 1989; Lockyer, 1993). Given this, we might expect to see disparity in GLG estimation errors between managed and wild populations due to the differences in diet consistency, types of prey, nutritional quality or seasonal variability of prey. Fresh live fish being actively caught vs hand feeding of (thawed) frozen dead fish could also impact tooth wear (Ardente et al., 2017). Indeed, we noted that wild dolphin teeth appeared more worn for a given age than MMP teeth. However, despite these differences in feeding and tooth wear, we did not find evidence for a difference in the accuracy of GLG estimates between the two cohorts (p=0.41).
This study also explored potential sex-related differences in GLG error. Females live longer than males and also reach a lower asymptotic length at a faster rate, therefore it is possible the rate of GLG deposition could vary between sexes (Mcfee et al., 2010). In addition, data suggest variation in growth rates, skeletal maturity, possible additional GLG layers post parturition and lactation, and life expectancy variations between sexes (Klevezal and Myrick Jr, 1984; Read et al., 1993). However, our results did not indicate an influence of sex on GLG age (p=0.74).
Several additional factors which could influence the accuracy of the results include tooth quality and selection. In 1975, Ridgway et al. highlighted the best technique for extracting a dolphin’s tooth in a live animal (Ridgway et al., 1975). Lower jaw teeth 13-18 are recommended as the primary teeth for aging due to their minimal wear and straight appearance, with rostral teeth more likely to be worn and caudal teeth more likely to be curved. Elliptical teeth are more challenging to age as they cannot be cross sectioned and read as a single piece (Figure 8). Previous studies have confirmed the lack of variation between left and right, mandibular and maxillary tooth ages when compared from the same animal (Hui, 1980). However, differences were observed between teeth from the same individual between anterior and posterior teeth, with anterior teeth ceasing the deposition of dentine after approximately 10-12 GLGs were deposited however the posterior teeth could deposit up to 15. Hui (1978) concluded that posterior teeth are more reliable indicators of age and may allow a minimum age greater than 15 to be estimated. Not all teeth included in this study followed Ridgway’s recommendation of mandibular tooth 13-18 primarily due to accessibility, availability or in the older wild animals worn teeth. The variation in which teeth were extracted and the subsequent elliptical nature of some teeth could have influenced the accuracy of the age estimates produced. For future reference standardizing tooth selection is recommended, and where possible obtaining multiple teeth to account for any sectioning challenges is advised. Recommended storage is either dry at room temperature or in 70% ethanol. Freezing is not recommended due to the risk of tooth cracking. This study cannot address discrepancies between teeth that were extracted from live vs deceased dolphins as all teeth included in the study were extracted post-mortem.
Figure 8 A tooth from an adult male dolphin demonstrating the potential elliptical curvature which can make aging via GLG more challenging. Image courtesy of W. McFee.
There has been ample discussion regarding the influence of preparation methodology on age estimation in marine mammals (Perrin and Myrick, 1980), particularly on the inaccuracy of using unstained sections compared to stained sections with differences in age estimates between the techniques reaching 31 years (Hohn and Fernandez, 1999). Subsequently, standardized sectioning and staining has been recommended to ensure GLG reading accuracy. In addition, experienced readers are essential when interpreting GLGs, as errors such as misidentification of early deposited GLG layers or accessory layers can lead to inaccurate results even in young animals (Kimura, 1980). Species-specific differences warrant inclusion or exclusion of cementum GLG counts. For example, harbor porpoise, Phocoena phocoena, did not have a regular layering pattern in cementum (Grue-Nielsen, 1972) whereas in Stenella attenuata, Stenella coeruleoalba and South Asian river dolphins (Platanista gangetica minor and P. g. gangetica) cementum was found to be of value (Kasuya, 1976; Lockyer and Braulik, 2014), and in Franciscana dolphins only a couple of dentinal GLGs are deposited, after which cemental layers must be used (Pinedo and Hohn, 2000). Cemental GLGs have not routinely been used for age estimation in bottlenose dolphins which is why they were not included in this study however they are of value in other odontocetes (Kasuya et al., 1988; Amano et al., 2014). Other factors such as enlarged pulp cavities in younger animals or pulp stones can also cause compression of GLGs leading to reading errors (Hohn et al., 1989). It has been noted that during later years dentinal deposition shifts from primary to secondary with irregular deposition in some cases and a 20 year discrepancy has been observed between age estimates (Hohn and Fernandez, 1999). An additional challenge in the application of this technique to older animals is the small amount of dentine deposited in older animals resulting in very thin annular layers (Hohn et al., 1989). The possibility of the time frame of GLG shifting to biannually was explored in the beluga whale (Delphinapterus leucas) with the conclusion that inter-reader variability was more likely than two GLGs per year (Waugh et al., 2018). However, too few older animals with known age has resulted in GLGs in belugas only being validated up to 23 years of age (Lockyer et al., 2007).
The methodology of storage, sectioning and staining performed in this research has been well established by multiple scientists over several decades (Sergeant, 1959; Sergeant et al., 1973; Hohn, 1980; Wainwright and Walker, 1988; Hohn et al., 1989; Myrick et al., 1990; Hohn and Fernandez, 1999; Mcfee et al., 2012). Nevertheless, there were still tooth sections that did not stain well and could have been improved upon to ensure accurate reads. This highlights the challenging nature of this technique and the need to extract multiple teeth where possible to allow for multiple sections. We also highly recommend having the actual slides in hand to estimate the age via GLGs rather than digital images, as having the actual slides allows the reader to magnify sections that are otherwise difficult to read. Some of the teeth sectioned contained “pulp stones” as demonstrated in Figure 9 however none of these three teeth were those with larger errors. The teeth with the greatest errors were actually described as clear on section but did have some curvatures which demonstrates the need to try and select straight teeth from number 13-18 where possible as this could influence the accuracy of the age estimate. A scoring system similar to that used for the radiographs that indicated a reader’s expectation of the reliability of an age estimate from a particular tooth section may also be useful as some tooth sections were noted to be sub-par.
Figure 9 Cross section of a bottlenose dolphin tooth showing a “pulp stone” within the GLG layers which could interfere with GLG counts. Image courtesy of W. McFee.
Although our study confirms GLG estimates for dolphins 16 years or older were inaccurate, the actual age for samples with 16 or more GLG layers was always above 16 years. With this in mind, we can confidently state that a reading of 16 or more GLGs provides a lower bound for the dolphin’s actual age (i.e. at least 16 years). This is important because numerous prior studies have relied at least partially on GLG age estimates to investigate various aspects of life-history and population dynamics in bottlenose dolphins (Wells et al., 1987; Wells, 2014). While exact age may not be known, the longitudinal life history data and the known time since GLG was performed can still inform a known minimum age with the potential for the individual to be older than expected. Growth patterns have been investigated using functional forms such as logistic or Gompertz functions fitted to GLGs versus length to model a growth rate that decreases as length increases and approaches zero at some asymptotic length. However, the growth analyses have consistently shown that asymptotic length is reached between 12-15 years of age in both males and females (Read et al., 1993; Fernandez and Hohn, 1998; Stolen and Barlow, 2003; Mattson et al., 2006; Mcfee et al., 2010), ages that can be estimated quite accurately from GLGs. In addition, since dolphins with GLG estimates above 16 are almost certainly at least 16 years old, the inaccuracies in GLG estimates would only affect samples for dolphins that have reached asymptotic length; therefore there would be negligible impact on estimated growth functions.
Age estimated from GLG analyses have also been applied for modeling age-specific survival in bottlenose dolphins by fitting a Siler competing risk function to age-at-death data (Siler, 1979; Stolen and Barlow, 2003; Schwacke et al., 2017; Schwacke et al., 2022). The Siler model uses a continuous function to estimate mortality risk as three competing components: an exponentially decreasing risk up to about five years of age due to juvenile factors, a constant risk experience by all age classes, and an exponentially increasing risk beginning at around 20 years of age due to senescence (Schwacke et al., 2017). GLG estimation errors as we observed could affect estimation of the senescent risk, but juvenile risk would be unaffected and the median age at death estimates (nine and 16 years for males and females, respectively) would not be affected. For survival estimates above 16 years, the impact would likely be small for any single year class, but overall the negatively biased estimates could have a significant influence on estimates of longevity. Schwacke et al. (2017) estimated cumulative survival probability to be very close to zero by the age of 60 years for female dolphins, yet a dolphin living to the estimated age of 67 years has been observed in the long-studied Sarasota Bay resident dolphin community (age was estimated in 1984 via GLGs at 34 years, and then the dolphin was observed for an additional 33 years). Prior research has suggested fitting the Siler competing risk model using existing data on well-studied mammalian species and scaling the model using estimates of longevity for species for which data are lacking (Barlow and Boveng, 1991). Given our results, we recommend considering alternative parameters to use for such interspecies scaling; for example, instead of longevity, Marques et al. (submitted) used gestation length, a parameter more straightforward to estimate, for scaling reproduction and survival parameters among different cetacean species.
Pectoral flipper radiography also demonstrated a wider discrepancy in age estimates for older individuals. This was expected, as accurate age estimation in geriatric individuals is dependent on the presence of degenerative changes which is a varied process, not systematic like the growth plate closure assessed in the younger ages. For example, two 58 year old radiographs demonstrate vast differences in degenerative changes for the same age (Figure 10). In other species, numerous factors can influence the presence and progression of degenerative changes, to include genetic predisposition, previous injury or infection, and underlying metabolic disorders. Therefore, further investigation is warranted to determine the drivers for degenerative change in dolphins, in addition to than age. In the Sarasota dolphins, some of the larger PFR errors could also have been influenced by poor quality radiographs. It is essential to obtain a dorsoventral view of the carpus, as an oblique view will distort the growth plate appearance leading to inaccurate scoring. Assessment of the carpal bones is used to assess the degree of rotation, and caution should be taken in scoring if an oblique view is present. It can be challenging to obtain a dorsoventral view in a wild dolphin in a field situation if the dolphin is not conducive to extending the pectoral flipper or perhaps has a pathology that prevents full extension such as poor joint mobility.
Figure 10 Geriatric radiographs in two 58year old dolphins. (A) Is the radiograph of a 57 year old female dolphin. (B) is a 58 year old male dolphin. (A) was underestimated in age as there are minimal degenerative changes. These radiographs demonstrate both the variability and subjectivity of this technique at the older age range. Both of these dolphins were in managed care.
A limitation of this study was the retrospective nature of the sample collection which prevented performing both techniques on the same individual. As the tooth aging aspect was retrospective with no tooth extracted for this study, teeth were only available from archived post-mortem samples, therefore the pectoral flipper was no longer present to facilitate a paired radiograph. While having paired samples would likely not have changed our conclusions of increased error in older age classes and a negative bias for GLG ages in the oldest dolphins, having a tooth and radiographs from the same individuals would have allowed us to examine the correlation in error between the two techniques. In addition, having the opportunity to section additional teeth from the same animal can help with obtaining a section which better displays all of the GLGs.
While no single method is ideal there are advantages and disadvantages to each of the aforementioned aging techniques. The advantage of GLG aging is the breadth of data available with this technique being applied for many decades. The disadvantages are the specialized skill and equipment required to section the tooth and read the GLG layers as well as the tooth extraction being an invasive procedure for live dolphins, with a small risk of post extraction infection. Following sectioning the tooth is effectively destroyed so it’s not viable in valuable samples or museum specimens for example. The ability to share data and submit samples to experts could require additional permits for import and export potentially limiting access from an international conservation perspective. In pectoral flipper radiographs, the advantages are the ability to obtain estimates in real-time at the time of health assessment or necropsy, the electronically shareable data sources allowing international collaboration and no tissue permit or shipping required and the quick, accessible non-invasive approach which can also be used on valuable archived samples. Another advantage is the ability to estimate sexual maturity across all species with documentation of physeal plate closure demonstrating the presence or absences of sexual maturity with sexual hormones being a primary driver for growth plate closure. The disadvantages of pectoral flipper radiography are the radiograph machine requirement and the need for species-specific equations to estimate age as well as some experience to perform the radiograph assessment. Both techniques can be performed on Code 1-5 individuals or in properly preserved museum specimens (Code 1 = live stranding, Code 2 = fresh dead, Code 3 = moderate decomposition, Code 4 = advanced decomposition, Code 5 = skeletal remains). From a conservation perspective, a technique needs to be accurate, practical, and cost-effective. Considering all of the above factors, PFR is recommended over GLG analysis when assessing the age of live animals, given the less invasive nature of the procedure.
This study demonstrated the challenges in accurately determining the age of older bottlenose dolphins. We hypothesize that the reason for the reduction in accuracy in older dolphins could be multifactorial with challenges of sectioning and visualizing the oldest GLG layers, compression of GLG layers, lack of laying down of GLG layers at a certain age or worn teeth inhibiting accuracy due to loss of the perinatal line. In PFR estimating age in older dolphins is reliant upon degenerative changes which can be highly variable reducing the accuracy of this technique in this age group. Caution should be taken when estimating age in older dolphins and the effect of the potential negative bias should be considered in incorporating estimates for life-history analyses.
The original contributions presented in the study are included in the article/Supplementary Material. Further inquiries can be directed to the corresponding authors.
Ethical review and approval was not required for the animal study because Samples were obtained post mortem or as part of routine care.
AB, LS, FMG, CS contributed to conception and design of the study. CP, GL, RW provided sample submission. AH, WM, MS provided age estimates from tooth samples. AB and DG-P provided age estimates from pectoral flipper radiography. RD and SR provided historical data for samples. AB and LS wrote the first draft of the manuscript and performed statistical analysis. All authors contributed to the article and approved the submitted version.
This research was made possible by a generous grant from NOAA Prescott funding NA20NMF4390132. These data and related items of information have not been formally disseminated by NOAA, and do not represent any agency determination, view, or policy. Open access was made possible by the Jeanette Fuller Ridgway Scientific Writing Fund.
The authors wish to thank the involvement of Dr. Eric Jensen and the U.S. Navy Marine Mammal Program dolphins, biotechnicians, and managers, and the National Marine Mammal Foundation trainers, veterinary, and medical records staff who facilitated this study. In addition, we thank Todd Speakman and Jason Allen for providing additional information on these cases through photo-identification surveys or clinical records. The authors would like to specifically thank Veronica Cendejas and Brittany Novick for their instrumental help with sample management and thank you to Erin Brodie, Dr. Carolina Le Bert and Dr. Betsy Lutmerding for sample procurement. For the wild dolphin radiographs thank you to Dr. Shelly Marquardt, Dr. Craig Pelton and Dr. Mike Walsh for performing field radiographs. Thank you to Lynne Byrd at Mote Marine Laboratory for the radiographs of the stranded dolphins. This is scientific contribution 350 of the National Marine Mammal Foundation. Finally a huge thank you to Jacqulynne Stuart at Sound Imaging for access to x-ray equipment to obtain field radiographs.
The authors declare that the research was conducted in the absence of any commercial or financial relationships that could be construed as a potential conflict of interest.
All claims expressed in this article are solely those of the authors and do not necessarily represent those of their affiliated organizations, or those of the publisher, the editors and the reviewers. Any product that may be evaluated in this article, or claim that may be made by its manufacturer, is not guaranteed or endorsed by the publisher.
The Supplementary Material for this article can be found online at: https://www.frontiersin.org/articles/10.3389/fmars.2023.1135521/full#supplementary-material
Amano M., Yamada T. K., Kuramochi T., Hayano A., Kazumi A., Sakai T. (2014). Life history and group composition of melon-headed whales based on mass strandings in Japan. Mar. Mammal Sci. 30 (2), 480–493. doi: 10.1111/mms.12050
Ardente A., Wells R., Smith C., Walsh M., Jensen E., Schmitt T., et al. (2017). Dietary cation–anion difference may explain why ammonium urate nephrolithiasis occurs more frequently in common bottlenose dolphins (Tursiops truncatus) under human care than in free-ranging common bottlenose dolphins. J. Anim. Sci. 95, 1396–1406. doi: 10.2527/jas.2016.1113
Barlow J., Boveng P. (1991). Modeling age-specific mortality for marine mammal populations. Mar. Mammal Sci. 7, 50–65. doi: 10.1111/j.1748-7692.1991.tb00550.x
Barratclough A., Sanz-Requena R., Marti-Bonmati L., Schmitt T. L., Jensen E., García-Párraga D. (2019a). Radiographic assessment of pectoral flipper bone maturation in bottlenose dolphins (Tursiops truncatus), as a novel technique to accurately estimate chronological age. PloS One 14, e0222722. doi: 10.1371/journal.pone.0222722
Barratclough A., Smith C. R., Gomez F. M., Photopoulou T., Takeshita R., Pirotta E., et al. (2021). Accurate epigenetic aging in bottlenose dolphins (Tursiops truncatus), an essential step in the conservation of at-risk dolphins. J. Zoological Botanical Gardens 2, 416–420. doi: 10.3390/jzbg2030030
Barratclough A., Wells R. S., Schwacke L. H., Rowles T. K., Gomez F. M., Fauquier D. A., et al. (2019b). Health assessments of common bottlenose dolphins (Tursiops truncatus): Past, present, and potential conservation applications. Front. Vet. Sci. 6. doi: 10.3389/fvets
Brooks L., Anderson H. F. (1998). Dental anomalies in bottlenose dolphins, Tursiops truncatus, from the west coast of Florida. Mar. mammal Sci. 14, 849–853. doi: 10.1111/j.1748-7692.1998.tb00769.x
Calzada N., Lockyer C. H., Aguilar A. (1994). Age and sex composition of the striped dolphin die-off in the western Mediterranean. Mar. Mammal Sci. 10, 299–310. doi: 10.1111/j.1748-7692.1994.tb00484.x
Fernandez S., Hohn A. A. (1998). Age, growth, and calving season of bottlenose dolphins, Tursiops truncatus, off coastal Texas. Fishery Bull. 96, 357–365.
Grue-Nielsen H. (1972). Age determination of the harbour porpoise phocoena phocoena (L.) (Cetacea).Vid. Medd. Dan. Naturhist. Foren. 135, 61–84.
Herrman J. M., Morey J. S., Takeshita R., De Guise S., Wells R. S., Mcfee W., et al. (2020). Age determination of common bottlenose dolphins (Tursiops truncatus) using dental radiography pulp: tooth area ratio measurements. PloS One 15, e0242273. doi: 10.1371/journal.pone.0242273
Hohn A. A. (1980). Age determination and age related factors in the teeth of Western north Atlantic bottlenose dolphins. Sci. Rep. Whales Res. Institute 32, 39–66.
Hohn A. A., Fernandez S. (1999). Biases in dolphin age structure due to age estimation technique. Mar. Mammal Sci. 15, 1124–1132. doi: 10.1111/j.1748-7692.1999.tb00881.x
Hohn A. A., Scott M. D., Wells R. S., Sweeney J. C., Irvine A. B. (1989). Growth layers in teeth from known-age, free-ranging bottlenose dolphins. Mar. Mammal Sci. 5, 315–342. doi: 10.1111/j.1748-7692.1989.tb00346.x
Hui C. A. (1978). Reliability of using dentin layers for age determination in Tursiops truncatus. Naval Ocean Syst. Center Biosci. Department.
Hui C. A. (1980). Variability of dentin deposits in Tursiops truncatus. Can. J. Fisheries Aquat. Sci. 37, 712–716. doi: 10.1139/f80-090
Kasuya T. (1976). Reconsideration of life history parameters of the spotted and striped dolphins based on cemental layers. Sci. Rep. Whales Res. Inst. 28, 73–106.
Kasuya T., Brownell R. L. Jr (1979). Age determination, reproduction, and growth of the franciscana dolphin, pontoporia blainvillei. Sci. Rep. Whales Res. Institute 31, 45–67.
Kasuya T. O., Sergeant D. E., Tanaka K. E. (1988). Re-examination of life history parameters of long-finned pilot whales in the Newfoundland waters. Sci. Rep. Whales Res. Inst 39, 103–119.
Kimura M. (1980). Variability in techniques of counting dentinal growth layer groups in a tooth of a known-age dolphin, Tursiops truncatus. Age Determination toothed whales sirenians 1980 (3), 161.
Klevezal G. (1996). Recording Structures of Mammals Determination of Age and Reconstructions of LIfe Hsitory. Chapter 6.7. Brookfield, VT, USA: Taylor and Francis. pp. 165.
Klevezal G. A., Myrick A. C. Jr (1984). Marks in tooth dentine of female dolphins (Genus stenella) as indicators of parturition. J. Mammalogy 65, 103–110. doi: 10.2307/1381206
Lockyer C. (1993). A report on patterns of deposition of dentine and cement in teeth of pilot whales, genus globicephala. Rep. Int. Whal. Comm 14, 137–161.
Lockyer C. H., Braulik G. T. (2014). An evaluation of age estimation using teeth from south Asian river dolphins (Platanistidae). (NAMMCO Scientific Publications), 10. doi: 10.7557/3.3268
Lockyer C., Hohn A. A., Doidge D. W., Heide-Jørgensen M. P., Suydam R. (2007). Age determination in belugas (Delphinapterus leucas in belugas): a quest for validation of dentinal layering. Aquat. Mamm 33, 293–304. doi: 10.1578/AM.33.3.2007.293
Manzanilla S. R. (1989). The 1982–1983 El niño event recorded in dentinal growth layers in teeth of Peruvian dusky dolphins (Lagenorhynchus obscurus). Can. J. Zoology 67, 2120–2125. doi: 10.1139/z89-301
Mattson M. C., Mullin K. D., Ingram G. W. Jr., Hoggard W. (2006). Age structure and growth of the bottlenose dolphin (Tursiops truncatus) from strandings in the Mississippi sound region of the north-central gulf of Mexico from 1986 to 2003. Mar. Mammal Sci. 22, 654–666. doi: 10.1111/j.1748-7692.2006.00057.x
Mccormack M. A., Mcfee W. E., Whitehead H. R., Piwetz S., Dutton J. (2022). Exploring the use of SEM–EDS analysis to measure the distribution of major, minor, and trace elements in bottlenose dolphin (Tursiops truncatus) teeth. Biol. Trace Element Res. 200, 2147–2159. doi: 10.1007/s12011-021-02809-9
Mcfee W. E., Adams J. D., Fair P. A., Bossart G. D. (2012). Age distribution and growth of two bottlenose dolphin (Tursiops truncatus) populations from capture-release studies in the southeastern united states. Aquat. Mammals 38, 258–265. doi: 10.1578/AM.38.1.2012.17
Mcfee W. E., Hopkins-Murphy S. R. (2002). Bottlenose dolphin (Tursiops truncatus) strandings in south Carolina 1992–1996. (South Carolina State Documents Depository: Fishery Bulletin), 100 (2), 258–265. Available at: http://fishbull.noaa.gov/1002/09mcfeef.pdf.
Mcfee W. E., Lipscomb T. P. (2009). Major pathologic findings and probable causes of mortality in bottlenose dolphins stranded in south Carolina from 1993 to 2006. J. Wildlife Dis. 45, 575–593. doi: 10.7589/0090-3558-45.3.575
Mcfee W. E., Schwacke J. H., Stolen M. K., Mullin K. D., Schwacke L. H. (2010). Investigation of growth phases for bottlenose dolphins using a Bayesian modeling approach. Mar. Mammal Sci. 26, 67–85. doi: 10.1111/j.1748-7692.2009.00306.x
Meegan J. M. (2016). Surgical management of osteomyelitis and dental disease in a bottlenose dolphin (Tursiops truncatus) using general anesthesia IAAAM 2016. In IAAAM Conference Proceedings.
Miyazaki N. (1980). “Preliminary note on age determination and growth of the rough-toothed dolphin, steno bredanensis, off the pacific coast of Japan,” in Age determination of toothed whales and sirenians, vol. 3 . Eds. Perrin W. F., Myrick A. C. (Cambridge: Rep. Intern. Whaling Commis. Spec. Iss), 171–179.
Moore M., Early G., Touhey K., Barco S., Gulland F., Wells R. (2007). Rehabilitation and release of marine mammals in the united states: risks and benefits. Mar. Mammal Sci. 23, 731–750. doi: 10.1111/j.1748-7692.2007.00146.x
Myrick A. C. J., Cornell L. H., Leatherwood S., Reeves R. R. (1990). Calibrating dental layers bottlenose dolphins from tetracycline labels and tooth extractions (The Bottlenose dolphin. San Diego: Academic Press, Inc), 587–608.
Myrick A. C. Jr., Shallenberger E. W., Kang I., Mackay D. B. (1984). Calibration of dental layers in seven captive Hawaiian spinner dolphins, stenella longirostris, based on tetracycline labeling. Fishery Bull. 82, 207–225.
Nishiwaki M., Yagi T. (1953). On the age and the growth of teeth in a dolphin (Prodelphinus caeruleo-albus). Sci. Rep. Whales Res. Inst 8, 133–146.
Perrin W. F., Myrick A. C. (1980). Age determination of toothed whales and sirenians. Int. Whaling Commission.
Peters K. J., Gerber L., Scheu L., Cicciarella R., Zoller J. A., Fei Z., et al. (2023). An epigenetic DNA methylation clock for age estimates in Indo‐Pacific bottlenose dolphins (Tursiops aduncus). Evol. Appl. 16 (1), 126–133. doi: 10.1111/eva.13516
Pinedo M. C., Hohn A. A.. (2000). Growth layer patterns in teeth from the franciscana, Pontoporia blainvillei: Developing a model for precision in age estimation. Mar. Mam. Sci. 16 (1), 1–27. doi: 10.1111/j.1748-7692.2000.tb00901.x
Powell J. W. B., Duffield D. A., Kaufman J. J., Mcfee W. (2019). Bone density cannot accurately predict age in the common bottlenose dolphin, Tursiops truncatus. Mar. Mam. Sci. 35, 1597–1602. doi: 10.1111/mms.12591
Read F. L., Hohn A. A., Lockyer C. H. (2018). A review of age estimation methods in marine mammals with special reference to monodontids. (NAMMCO Scientific Publications), 10. doi: 10.7557/3.4474
Read A., Wells R., Hohn A., Scott M. (1993). Patterns of growth in wild bottlenose dolphins, Tursiops truncatus. J. Zoology 231, 107–123. doi: 10.1111/j.1469-7998.1993.tb05356.x
Ridgway S. H., Green R. F., Sweeney J. C. (1975). Mandibular anesthesia and tooth extraction in the bottlenosed dolphin. J. wildlife Dis. 11, 415–418. doi: 10.7589/0090-3558-11.3.415
Scheffer V. B., Myrick A. C. (1980). A review of studies to 1970 of growth layers in the teeth of marine mammals. Rep. Int. Whal. Commn 3, 51–63.
Schwacke L. H., Marques T. A., Thomas L., Booth C. G., Balmer B. C., Barratclough A., et al. (2022). Modeling population effects of the deepwater horizon oil spill on a long-lived species. Conserv. Biol. 36 (4), e13878. doi: 10.1111/cobi.13878
Schwacke L. H., Thomas L., Wells R. S., Mcfee W. E., Hohn A. A., Mullin K. D., et al. (2017). Quantifying injury to common bottlenose dolphins from the deepwater horizon oil spill using an age-, sex-and class-structured population model. Endang Species Res. 33, 265–279. doi: 10.3354/esr00777
Scott M. D., Wells R. S., Irvine A. B. (1990). A long-term study of bottlenose dolphins on the West coast of Florida 11. bottlenose dolphin 235. doi: 10.1016/B978-0-12-440280-5.50015-9
Sergeant D. E. (1959). Age determination in odontocete whales from dentinal growth layers. Norwegian Whaling Gazette.
Sergeant D. E., Caldwell D. K., Caldwell M. C. (1973). Age, growth, and maturity of bottlenosed dolphin (Tursiops truncatus) from northeast Florida. J. Fisheries Board Canada 30, 1009–1011. doi: 10.1139/f73-165
Siler W. (1979). A competing-risk model for animal mortality. Ecology 60, 750–757. doi: 10.2307/1936612
Stolen M. K., Barlow J. (2003). A model life table for bottlenose dolphins (Tursiops truncatus) from the Indian river lagoon system, Florida, USA. Mar. mammal Sci. 19, 630–649. doi: 10.1111/j.1748-7692.2003.tb01121.x
Stolen M. K., Odell D. K., Barros N. B. (2002). Growth of bottlenose dolphins (Tursiops truncatus) from the Indian river lagoon system, Florida, USA. Mar. Mammal Sci. 18, 348–357. doi: 10.1111/j.1748-7692.2002.tb01042.x
Venn-Watson S. K., Jensen E. D., Smith C. R., Xitco M., Ridgway S. H. (2015). Evaluation of annual survival and mortality rates and longevity of bottlenose dolphins (Tursiops truncatus) at the united states navy marine mammal program from 2004 through 2013. J. Am. Veterinary Med. Assoc. 246, 893–898. doi: 10.2460/javma.246.8.893
Venn-Watson S., Smith C. R., Gomez F., Jensen E. D. (2011). Physiology of aging among healthy, older bottlenose dolphins (Tursiops truncatus): comparisons with aging humans. J. Comp. Physiol. B 181, 667–680. doi: 10.1007/s00360-011-0549-3
Wainwright K. L., Walker R. S. (1988). A method for preparing beluga (white whale), delphinapterus leucas, teeth for ageing. Fisheries Oceans. Canada.
Waugh D. A., Suydam R. S., Ortiz J. D., Thewissen J. G. M. (2018). Validation of growth layer group (GLG) depositional rate using daily incremental growth lines in the dentin of beluga (Delphinapterus leucas (Pallas 1776)) teeth. PloS One 13, e0190498. doi: 10.1371/journal.pone.0190498
Wells R. S. (2014). “Social structure and life history of bottlenose dolphins near Sarasota bay, Florida: insights from four decades and five generations,” in Primates and cetaceans. (Tokyo, Japan: Springer), 149–172.
Wells R. S., Scott M. D. (1999). Bottlenose dolphin Tursiops truncatus (montagu 1821). Handb. Mar. mammals 6, 137–182.
Keywords: aging, cetacean, geriatric, growth layer group, novel technique, x-ray
Citation: Barratclough A, McFee WE, Stolen M, Hohn AA, Lovewell GN, Gomez FM, Smith CR, Garcia-Parraga D, Wells RS, Parry C, Daniels R, Ridgway SH and Schwacke L (2023) How to estimate age of old bottlenose dolphins (Tursiops truncatus); by tooth or pectoral flipper? Front. Mar. Sci. 10:1135521. doi: 10.3389/fmars.2023.1135521
Received: 01 January 2023; Accepted: 16 February 2023;
Published: 07 March 2023.
Edited by:
Salvatore Siciliano, Fundação Oswaldo Cruz (Fiocruz), BrazilReviewed by:
Pavel Gol’din, National Academy of Sciences of Ukraine (NAN Ukraine), UkraineCopyright © 2023 Barratclough, McFee, Stolen, Hohn, Lovewell, Gomez, Smith, Garcia-Parraga, Wells, Parry, Daniels, Ridgway and Schwacke. This is an open-access article distributed under the terms of the Creative Commons Attribution License (CC BY). The use, distribution or reproduction in other forums is permitted, provided the original author(s) and the copyright owner(s) are credited and that the original publication in this journal is cited, in accordance with accepted academic practice. No use, distribution or reproduction is permitted which does not comply with these terms.
*Correspondence: Ashley Barratclough, YXNobGV5LmJhcnJhdGNsb3VnaEBubW1mLm9yZw==
Disclaimer: All claims expressed in this article are solely those of the authors and do not necessarily represent those of their affiliated organizations, or those of the publisher, the editors and the reviewers. Any product that may be evaluated in this article or claim that may be made by its manufacturer is not guaranteed or endorsed by the publisher.
Research integrity at Frontiers
Learn more about the work of our research integrity team to safeguard the quality of each article we publish.