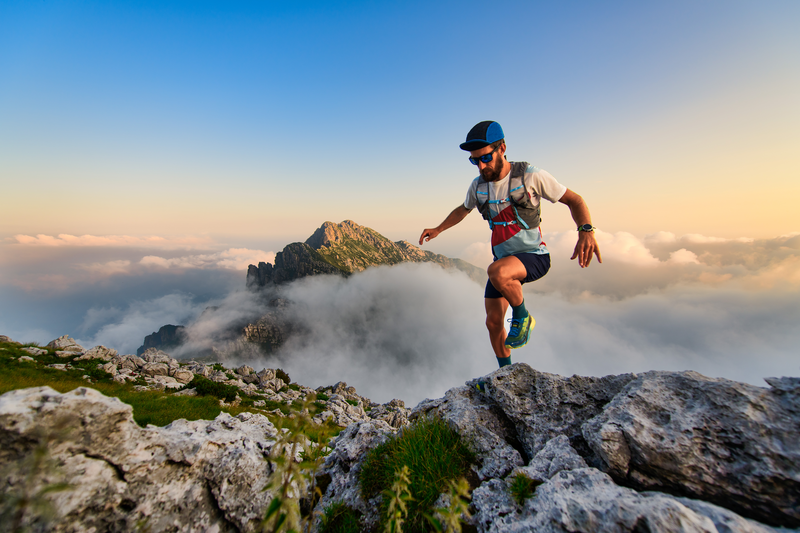
94% of researchers rate our articles as excellent or good
Learn more about the work of our research integrity team to safeguard the quality of each article we publish.
Find out more
ORIGINAL RESEARCH article
Front. Mar. Sci. , 12 May 2023
Sec. Marine Biology
Volume 10 - 2023 | https://doi.org/10.3389/fmars.2023.1135250
This article is part of the Research Topic Syngnathid Fishes: Biology, Ecology, Physiology, Conservation and Innovative Rearing Techniques View all 13 articles
Syngnathids (seahorses, pipefishes and seadragons) are an attractive resource for Traditional Chinese Medicine (TCM). Despite few scientific studies supporting seahorse nutritional benefits, they are believed to possess medicinal properties that enhance human health. The European short-snout seahorse Hippocampus hippocampus is classified as Data Deficient by the IUCN Red List of Threatened Species. Nevertheless, there are increasing records of this species being illegally captured and traded to supply TCM. This study investigated the fatty acid (FA) profiles of the trunk muscles of cultured female and male H. hippocampus, to assess sex and intraspecific variation, as well as their potential nutritional value. The contents of crude lipid (4.05 ± 2.15% dry weight, DW in females and 2.82 ± 1.48% DW in males) and phospholipid (8.23 ± 3.34 μg mg−1 DW in females and 7.91 ± 2.36 μg mg−1 DW in males) were not significantly different between the two sexes. The absolute FA compositions of H. hippocampus trunk muscles revealed higher mean values for FA 16:0, 18:0, 18:1 n-9 and 22:6 n-3 (DHA), in both female (2.82 ± 1.11, 1.81 ± 0.89, 0.90 ± 0.41 and 0.93 ± 0.35 μg mg−1 DW, respectively) and male specimens (1.99 ± 0.95, 1.52 ± 0.78, 0.74 ± 0.44 and 0.80 ± 0.41 μg mg−1 DW, respectively). In terms of FA classes, saturated fatty acids (SFA) showed the highest absolute value of the total pool of FA, for both sexes (4.73 ± 1.94 μg mg−1 DW in females and 3.58 ± 1.76 μg mg−1 DW in males). Males tended to exhibit a more suitable profile for human nutrition, displaying a lower atherogenic index (AI) and thrombogenic index (TI). The relative composition of H. hippocampus trunk muscles followed the patterns of seahorse species valued in TCM, with DHA ranking amongst the PUFA with higher mean relative abundances (12.0% of total FA). While seahorse FA profiles may be of interest in terms of their nutritional value for humans, only specimens originating from sustainable production practices should be traded and the conservation of their populations in the wild should continue to be a global priority.
Seahorse species within genus Hippocampus are vulnerable to illegal, unregulated and unreported (IUU) trade (Foster et al., 2019) mostly due to their demand by Traditional Chinese Medicine (TCM) and, to a lesser extent, to the marine ornamental and curio trades (Cohen et al., 2017). Global seahorse extraction is substantial, rounding tens of millions of specimens per year (Vincent et al., 2007; Vincent et al., 2011; Foster et al., 2019; Vaidyanathan et al., 2021). One way to address the pressure on wild populations is the implementation of sustainable aquaculture practices with the upside of supporting small communities that are dependent on seahorses as a source of income (Cohen et al., 2017). In 2002, the increase recorded in the live trade of farmed seahorses somehow signaled the growing international effort towards their aquaculture (Koldewey and Martin-Smith, 2010; Olivotto et al., 2011; Planas et al., 2017). There are, however, several seahorse species that by being caught in large numbers from the wild, highlight the pressing need to diversify the scope of seahorse aquaculture (Koldewey and Martin-Smith, 2010).
Most seahorse specimens supplying TCM are obtained from the wild to be consumed dried (Foster et al., 2019), as they are believed to have nutritional properties that are beneficial for human health (Kim et al., 2019). Dried seahorses are placed whole in beverages or consumed in the form of powder to treat several illnesses such as respiratory diseases and arteriosclerosis (Vincent, 1996; Vincent et al., 2011). Biomolecules with nutritional relevance already reported from Hippocampus spp. include lipids and amino acids, as well as minerals, namely some trace elements (Lin et al., 2009; Kim et al., 2019). Among the health promoting benefits that have been described to date from pharmacological studies addressing natural derivatives from seahorse species valued in TCM one can highlight the anti-oxidant activity reported from H. abdominalis (Kim et al., 2019) and its, as well as H. comes, anti-cancer potential (Xu et al., 2020; Ghasemi et al., 2021). Additionally, the phenolic compound paeonol isolated from H. kuda has been reported to display activity against neuro-inflammation (Himaya et al., 2012).
Marine fish are commonly recognized as a rich source of polyunsaturated fatty acids (PUFA), especially omega-3 fatty acids (FA), namely docosahexaenoic acid (DHA, 22:6 n-3) and eicosapentaenoic acid (EPA, 20:5 n-3) (Woods, 2003; Huang et al., 2010). Docosahexaenoic acid and EPA are abundant FA in fish cell membranes (Johnston et al., 2020), being the first a relevant component of cell membranes involved in the synthesis of muscle fibers (Planas et al., 2020). These omega-3 FA, as well as some omega-6 FA (arachidonic acid, ARA, 20:4 n-6), are known to be of major importance in human metabolism, for contributing to the prevention of multiple non-communicable diseases (e.g., cardiovascular disorders) and modulate immune responses (Crawford et al., 1999; Nestel, 2000; Sonnweber et al., 2018). Nevertheless, the biosynthesis pathway that allow de novo synthesis of DHA and EPA from its precursor, the essential α-linoleic acid (18:3 n-3, ALA) that needs to be obtained from dietary sources and cannot be biosynthesized, is very limited in humans (Burdge and Calder, 2005; Scaioli et al., 2017). For this reason, consumers and nutritionists value marine fish and seafood as important sources of omega-3 FA (Tocher, 2015).
The European short-snout seahorse H. hippocampus and long-snout seahorse H. guttulatus co-occur in sympatry in shallow European coastal habitats (Curtis et al., 2017). In Ria Formosa coastal lagoon (south of Portugal), the populations of these two species have suffered pronounced declines associated to multiple factors, such as habitat degradation, by-catch, natural phenomena (e.g., silting events) and illegal fishing; yet the direct causes for this decline are still being assessed (Caldwell and Vincent, 2012; Correia et al., 2018). Hippocampus hippocampus is classified, at global level, as Data Deficient by the IUCN Red List of Threatened Species (The IUCN Red List of Threatened Species, 2017a) and it is not extensively traded (Curtis et al., 2017), as other seahorse species that are targeted for TCM. Nevertheless, in the Mediterranean region, this species has been classified as Near Threatened (The IUCN Red List of Threatened Species, 2017b) and there are a few records of its illegal capture and trade to supply TCM (Planelles, 2017).
Nowadays, consumers are more concerned about environmental and food security matters and, as a result, are interested in knowing the origin and production processes of seafood (Leal et al., 2015; Doubleday et al., 2022). As such, seahorse aquaculture can potentially enhance consumers’ safety by providing a legal product to traditional and emerging markets. The FA profiles of edible muscles of farmed fish are influenced by the diet supplied during grow-out (Bordignon et al., 2020) and culture conditions, ultimately affecting the quality of the fillets that will reach the final consumer (Monge-Ortiz et al., 2018). As wild and cultured marine fish experience contrasting feeding regimes, their biochemical and geochemical profiles may be used to discriminate farmed seahorses from wild ones (Leal et al., 2015). Indeed, a geochemical tool has already been successfully applied to confirm the geographic origin of cultured H. guttulatus (Cabral et al., 2021), confirming the potential of these approaches to help fighting IUU fishing, as well as promoting the certification of origin for sustainably farmed seahorses.
The present study aimed to determine the FA profiles of cultured specimens of H. hippocampus and investigate the existence of intraspecific variation. While seahorses are usually consumed whole (muscle, viscera, bones and skin), the present study only analyzed the FA profile of trunk muscle. Muscle is an important lipid storage tissue in fish (Jobling et al., 1998) and while the FA profile of trunk muscle does not reflect the full FA pool of seahorses, it displays a more stable profile than that of gonads (e.g., ovaries) and reserve organs (e.g., liver) which are more susceptible to short-term shifts promoted by diet and abiotic factors (Planas et al., 2020; Zhang et al., 2022). Therefore, when aiming to perform intraspecific comparisons (e.g., male vs. female specimens), a more stable biological matrix will be less prone to bias in the pool of FA promoted by diet or different stages of sexual maturation (Zhang et al., 2022). The FA profiles of cultured short-snout seahorse’s trunk muscles are compared, from a nutritional point of view, to those of the whole-body of other cultured Hippocampus species described in the literature, acknowledging that the pool of FA of H. hippocampus is only partly represented by trunk muscle. Valuing the nutritional and functional potential of cultured seahorses is of main importance, as this may represent a potential pathway for relieving the ongoing fishing pressure over wild populations. This goal can be more easily achieved if a product with a more stable FA profile is presented to consumers, namely by encouraging the use of seahorse trunk muscle alone, rather than whole dried specimens featuring a much more variable FA profile.
Fourteen specimens of captive bred H. hippocampus were sourced from Centro de Ciências do Mar (CCMAR, University of Algarve, Faro, Portugal). The captive breeding program for H. hippocampus (Project HIPPONUTRE, reference 16-02-01-FMP-54) was approved by the ethics committee from the Veterinary Medicines Directorate, Ministry of Agriculture, Rural Development and Fisheries, Portugal. Under this approval, the program is conducted in accordance with the Guidelines of the European Union Council (86/609/EU) and Portuguese legislation for the use of laboratory animals. These short-snout seahorses (6 males and 8 females) were cultured using natural seawater from Ria Formosa coastal lagoon, located in Portugal’s southern Atlantic coast (36°59’ N; 7°51’ W). Temperature and salinity followed natural seasonal patterns with annual mean values of 20 °C and 35.7, respectively. During grow-out seahorses were fed on wild mysids Mesopodopsis slabberi and/or Leptomysis spp., depending on daily and/or seasonal availability.
The seahorse samples considered for FA analysis died from natural causes, during the same time frame (during the year of 2020). In the laboratory, frozen seahorse samples, maintained at -20 °C, were washed with distilled water and freeze-dried (Labogene CoolSafe 55-9L PRO), prior to the extraction of their trunk muscle. Seahorses’ whole body dry weight (DW) was registered, presenting mean values (± standard deviation, SD) of 1.41 ± 0.23 g in females and 1.49 ± 0.67 g in males. Seahorse sex was determined through the presence of brood pouch in the specimens, a trait exclusive to males.
Seahorses’ trunk was considered to be the extension from the cleithral ring to the last trunk ring, as described by Lourie (2003). This body part was separated from the rest of the whole body (98.34 ± 0.41% in females and 98.18 ± 0.53% in males) using ceramic coated blades, with dorsal trunk muscles (Figure 1) being removed with the help of stainless-steel tweezers. Tweezers and blades were cleaned with 99% (w/v) ethanol between the replicates of each sex group, to avoid cross contamination. Trunk muscle samples were stored at -20 °C until further analysis.
Figure 1 Trunk cross-section of a female short-snout seahorse Hippocampus hippocampus cultured at Centro de Ciências do Mar (CCMAR, University of Algarve, Faro, Portugal). Trunk muscles were extracted from the dorsal region highlighted by the yellow circle.
Freeze-dried samples with a mean DW of 23.99 ± 7.43 mg were macerated in the extraction tube using a stainless-steel spatula. Lipid extraction was performed using a modified Bligh and Dyer (1959) method. Briefly, 2500 µL of methanol (MeOH) (HPLC grade, Fisher Scientific, UK) and 1250 µL of dichloromethane (CH2Cl2) (HPLC grade, Fisher Scientific, UK) were added to each sample, which were then sonicated for 1 min and incubated on ice in an orbital shaker at 150 rpm (Stuart SSL2 Reciprocating Shaker) for 30 min. After a second addition of 1250 µL CH2Cl2, samples were centrifuged at 1207 g force for 10 min (Centurion Scientific Pro Analytical C4000R with a BRK5324 rotor, Stoughton, UK) and the organic phase was collected. Biomass residue was re-extracted by adding another 2500 µL of MeOH and 2500 µL of CH2Cl2, followed by centrifugation. A volume of 2250 µL of Mili Q water (Synergysup®, Millipore Corporation, Billerica, MA, USA) was added in the organic phases to promote phase separation and after a new centrifugation for 10 min at 537 g force, the organic phase was collected. The aqueous phase was re-extracted with 1880 µL of CH2Cl2 and centrifuged. The combined organic phases were dried under a nitrogen stream and total lipid content was determined by gravimetry.
Phospholipid (PL) amount in total lipid extracts were determined spectrophotometrically through the phosphorus assay, as described by Bartlett and Lewis (1970). Briefly, the lipid extracts were re-suspended in 300 µL of CH2Cl2 and 10 µL of the samples were transferred to glass tubes. The samples were dried under a nitrogen stream and 125 µL of perchloric acid (HClO4) (70% w/v) were posteriorly added. The tubes were then placed in a heating block (SBH200D/3, Stuart, Bibby Scientific Ltd., Stone, UK) at 180 °C for 60 min. A volume of 825 µL of Milli Q water and 125 µL sodium molybdate (NaMoO4.H2O) (2.5% w/v) were added to the tubes containing the samples. After homogenization, 125 µL of ascorbic acid (C6H8O6) (10% w/v) were added and the samples were incubated in a water bath (Precisterm, JP Selecta, Barcelona, Spain) at 100 °C for 10 min. The standard solutions of 0.1–2.0 µg of phosphate (solution of 100 µg. mL−1 NaH2PO4·2H2O), were subjected to the same treatment as the samples, except for the placement in the heating block. A volume of 200 µL of the samples and standards was transferred to a 96-well plate and the absorbance was measured at 797 nm, using a microplate ultraviolet-visible spectrophotometer (Multiskan GO, Thermo Fisher Scientific, Vantaa, Finland). The conversion factor 775/31 (25) was applied in order to obtain the amount of PL in the lipid extracts.
Fatty acid methyl esters (FAME) were prepared from total lipid extracts of the trunk muscle of H. hippocampus by alkaline transmethylation. An amount of total lipid extract corresponding to 10 µg of PL were transferred to tubes previously washed with n-hexane (95%). After drying the lipid extract under a nitrogen stream, 1 mL of internal standard 19:0 FA (1.08 µg mL−1, CAS number1731-94-8, Merck) in n-hexane (99%) was added to the tube containing the lipids, followed by the addition of 200 µL of a methanolic potassium hydroxide (KOH) solution (2 M). After sample homogenization, 2 mL of a saturated sodium chloride solution (NaCl) (10 mg mL−1) were added and the sample tubes were centrifuged for 5 min at 537 g force. A volume of 600 µL of the organic phase containing the FAME was extracted and dried under a nitrogen stream. For gas chromatography mass spectrometry (GC-MS) analysis, FAME were re-suspended in 100 µL of n-hexane (99%). A volume of 2.0 µL of the FAME solution was injected in the GC-MS equipment (Agilent Technologies 5977 B GC/MSD, Santa Clara, CA, USA) with a DB-FFAP column (123-3232, J and W Scientific, Folsom CA, USA) presenting the following specifications: 30 m in length, an internal diameter of 320 µm and a film thickness of 0.25 µm. The equipment was connected to a Mass Selective Detector operating with an electron impact mode at 70 eV and a scanning mass range of m/z 50–550 (1 s cycle in a full scan mode). Concerning the system conditions, helium was used as the carrier gas (constant flow 1.4 mL min−1), inlet temperature 220 °C and detector temperature 230 °C. Oven temperature was programmed as follows: initial temperature of 58 °C for 2 min; linear increase to 160 °C (25 °C min−1); linear increase to 210 °C (2 °C min−1); linear increase to 225 °C (20 °C min−1) and maintenance at 225 °C for 15 min. The data acquisition software employed was the GCMS 5977B/Enhanced MassHunter.
Fatty acid peaks were identified using Agilent MassHunter Qualitative Analysis 10.0 software, through retention time values and comparison of MS spectra with the NIST chemical database library and MS spectra of a FAME certified standard mixture (Supelco 37 Component FAME Mix, Sigma-Aldrich, Darmstadt, Germany). The absolute composition of FA (µg mg−1 DW) was determined using calibration curves of the FAME certified standard mixture under the same instrumental conditions and the FA 19:0 as the internal standard, while the relative abundances (%) were obtained from absolute values.
The assessment of nutritional quality of H. hippocampus trunk muscle, in terms of FA relative abundance (% of total fatty acids, TFA) was determined according to four lipid indexes. The atherogenic (AI), thrombogenic (TI) and hypocholesterolemic/hypercholesterolemic indexes (HH), were obtained from the formulas described by Ulbricht and Southgate (1991), while the polyene index (PI) was determined according to Lubis and Buckle (1990). The FA 12:0 and 14:0 were not included in the equations, as they were not detected in the trunk muscles of H. hippocampus. The equations for these lipid indexes are as follows (MUFA refers to monounsaturated FA and PUFA to polyunsaturated FA):
To assess the existence of significant differences (p-value ≤ 0.05) in the absolute abundance (μg mg−1 DW) of FA composition between H. hippocampus males and females, both groups were compared. Although the absolute abundance of some FA exhibited a normal distribution (determined by Shapiro-Wilk test), due to the low number of replicates, a non-parametric version of the t-test for unpaired samples (Mann -Whitney U) was performed. The same approach was applied to assess if both groups were significantly different, in terms of their pool of saturated FA (SFA), MUFA and PUFA, in terms of their lipid indexes and for lipid and PL contents. Bonferroni’s correction was applied whenever multiple comparisons were performed.
All statistical analyses were performed using RStudio v4.0.2 (RStudio Team, 2019), as well as the graphical representations which were obtained with ggplot2 package (Wickham, 2016).
The crude lipid content of H. hippocampus trunk muscles was 4.05 ± 2.15% DW in females and 2.82 ± 1.48% DW in males (Supplementary Table S1). Regarding PL content, the mean values were 8.23 ± 3.34 µg mg−1 DW in females and 7.91 ± 2.36 µg mg−1 DW in males. Lipid and PL contents did not present significant differences between females and males (Mann-Whitney U, p-value = 0.18 for lipid content and p-value = 0.95 for PL content).
A total of 16 FA were identified in the trunk muscle of cultured adult males and females of H. hippocampus. The absolute FA compositions revealed high mean values for 16:0, 18:0, 18:1 n-9 and 22:6 n-3 (DHA), in both female (2.82 ± 1.11, 1.81 ± 0.89, 0.90 ± 0.41 and 0.93 ± 0.35 μg mg−1 DW, respectively) and male specimens (1.99 ± 0.95, 1.52 ± 0.78, 0.74 ± 0.44 and 0.80 ± 0.41 μg mg−1 DW, respectively) (Table 1). Concerning lipid classes, SFA displayed the highest absolute values in both sexes, with females tending to exhibit higher mean values (4.73 ± 1.94 μg mg−1 DW) than males (3.58 ± 1.76 μg mg−1 DW). The amount of PUFA followed SFA in terms of absolute composition (2.25 ± 0.98 μg mg−1 DW in females and 1.88 ± 0.98 μg mg−1 DW in males), with MUFA displaying the lowest values (1.15 ± 0.55 μg mg−1 DW in females and 0.85 ± 0.53 μg mg−1 DW in males). Concerning n-3 and n-6 PUFA, the former exhibited the highest mean absolute values (1.46 ± 0.62 μg mg−1 DW in females and 1.09 ± 0.49 μg mg−1 DW in males) (Table 1). The n-6/n-3 ratio exhibited values lower than 1, in both females and males (Table 1).
Table 1 Absolute fatty acid composition (µg mg-1 DW) of trunk muscle from cultured female (n = 8) and male (n = 6) short snout seahorses Hippocampus hippocampus.
Table 2 summarizes the lipid indexes (AI, TI, HH and PI) recorded for cultured females and males of H. hippocampus. Females tended to present higher AI (0.87 ± 0.18) and TI (0.91 ± 0.17) than males (AI: 0.75 ± 0.14; TI: 0.84 ± 0.18), while males showed a tendency for higher HH (1.24 ± 0.25) and PI (0.52 ± 0.13) than females (HH: 1.06 ± 0.23; PI: 0.40 ± 0.07). Females and males did not present significant differences (Mann-Whitney U, p-value > 0.05) in lipid indexes.
Marine fish are usually rich in highly unsaturated FA (HUFA), especially DHA and EPA (Woods, 2003; Lin et al., 2008; Huang et al., 2010). In the case of H. hippocampus specimens surveyed in the present study, both females and males displayed higher absolute values of 16:0, 18:0, 18:1 n-9 and DHA, in their trunk muscles. These findings are in accordance with available literature, in which 16:0 is the SFA with highest expression in fish tissues and 18:1 n-9 is one of the most abundant MUFA (Amoussou et al., 2022). The HUFA DHA, one of the FA displaying a higher absolute abundance in the trunk muscles of H. hippocampus females and males, is an important component of cell membranes involved in the synthesis of muscle fibers in fish (Planas et al., 2020; Sushchik et al., 2020). In the work developed by Planas et al. (2020), DHA and EPA were two of the most dominant FA in mysidaceans (Siriella armata and Leptomysis sp.) commonly used as prey for adult H. hippocampus and H. guttulatus. The absolute abundance of DHA in the trunk muscles of male and female H. hippocampus may therefore be a consequence of their diet in captivity. Nevertheless, in line with the work developed by Xu et al. (2018), the absolute value of EPA in trunk muscles apparently did not reflect as linearly the dietary regime experienced by seahorses in captivity.
Trunk muscles of both females and males demonstrated a higher content of n-3 PUFA than n-6 PUFA. A high content of n-3 PUFA in the muscles from males and females results in an n-6/n-3 ratio lower than 1, highlighting the nutritional potential of H. hippocampus (Table 1). Although it has been suggested a minimum n-6/n-3 ratio of 1:1 for balanced diets, since modern human diets are rich in n-6 PUFA, which increases the probability of developing health disorders, such as cardiovascular, inflammatory, and autoimmune diseases (Simopoulos, 2002; Scaioli et al., 2017), an increase in n-3 PUFA consumption will contribute to balance the n-6/n-3 ratio towards a healthier diet (Vallecillos et al., 2021).
The trunk muscles from H. hippocampus, which, to our best knowledge, had not yet been described in terms of the FA profile, presented higher absolute compositions of SFA in both sex groups (Table 1). While diet influences the FA composition of the fillets in cultured fish (Vallecillos et al., 2021), Ballester-Lozano et al. (2011) highlight that one must also account for the lipid content of the fillet (neutral and polar fractions), as this is also a source of variability in FA profiles. Triacylglycerols (TAG) are rich in MUFA and SFA, while polar lipids present a high proportion of PUFA (Cowey and Sargent, 1977; Rey et al., 2018; Sushchik et al., 2020; Vallecillos et al., 2021). An increase in fillet lipid content is related to an increase in fat deposits, usually rich in TAG, resulting in higher MUFA and lower PUFA relative abundances (%TFA) (Ballester-Lozano et al., 2011; Vallecillos et al., 2021). Additionally, the study developed by Osako et al. (2003) addressing horse-mackerel (Trachurus japonicus) described that the relative abundances of SFA in the muscle’s total lipid content decreased with starvation, which was metabolized for energy production. Faleiro and Narciso (2013) reported that SFA abundance was linearly transferred from prey to juvenile H. guttulatus, while the transferring of MUFA and PUFA was not straightforward. Since H. hippocampus were fed with mysidacea, one of the main prey consumed by seahorses in the wild and that is known to exhibit high relative abundances of PUFA and SFA (Planas et al., 2020), the predominance of these two FA classes in seahorse trunk muscles may reflect their dietary regime.
According to Jobling et al. (1998), the liver and skeletal muscles are important sites for lipid storage, with some differences between fish species. In terms of fat content, fish can be grouped into four categories: lean (< 2%), low fat (2-4%), medium fat (4-8%) and high fat (> 8%) (in terms of wet weight) (Tonial et al., 2014). Since the lipid value of H. hippocampus trunk muscle ranged between 4.05 ± 2.15% DW (females) and 2.82 ± 1.48% DW (males) (Supplementary Table S1), this species ranks in the lean category, as our data refer to dried specimens and average moisture content in seahorses represent 75% of their wet weight (Lin et al., 2008; Lin et al., 2009).
Differences in reproductive investment of male and female fish, may lead to different nutritional profiles (Lin et al., 2009). However, this likely depends on the type of breeding strategy exhibited by fishes. Capital breeding is based on the buildup of reserves by breeders, which are allocated for the spawning season, while in the income strategy breeders use the food ingested, directly for reproductive purposes (Sainmont et al., 2014). Studies carried out in the temperate H. guttulatus and the tropical H. reidi suggested that seahorses display a mixed capital-income strategy, which might be modulated by species-specific differences on inter-batch intervals and temperature thresholds (Planas et al., 2020; Planas et al., 2021). Accordingly, an initial mixed capital-income period is followed by an income breeding period with progressive exhaustion of body reserves. It is likely, however, that most lipid and FA changes on muscle tissue (i.e., a rather conservative tissue) due to dietary resources occur to a much lower extent than in gonads, especially in females. In the case of the trunk muscle, females and males of H. hippocampus did not exhibit significant differences between the absolute abundance of FA and FA classes (i.e., SFA, MUFA and PUFA). Lean fish store their lipids in the liver (Njinkoué et al., 2002; Calder, 2013) and, as such, the FA composition of trunk muscles of H. hippocampus may be more stable, between sexes. However, additional studies should be performed during the reproductive season, for a better understanding of how FA are mobilized from different tissues during this stage.
The lipid indexes considered in the present study, AI, TI, PI and HH, provide important information on the nutritional value and suitability of nutritional resources for human health (Marques et al., 2019; Conde et al., 2021). Low values of AI and TI are predictors of a low risk for developing cardiovascular diseases in humans (Tonial et al., 2014; Conde et al., 2021). The AI and TI of H. hippocampus trunk muscles were higher than those recorded for the muscles of other marine fish species (Rueda et al., 2001). However, male seahorses tended to present lower values for both indexes than females (Table 2). In the case of HH, the higher the value, the more adequate is the fish fat for human nutrition (Tonial et al., 2014). In the present study, males exhibited a tendency for higher HH values, than females. The PI is considered a proxy of lipid oxidation (Marques et al., 2019), expressing the retention of PUFA in relation to more stable FA 16:0 (Chaula et al., 2019). The higher mean values in HH and PI, as well as lower values for AI and TI in males, may suggest that trunk muscles of male seahorses are more suitable for human consumption than those from females. Nonetheless, it is worth highlighting that there were no significant differences between the lipid indexes recorded for both males and females H. hippocampus and future analysis should be performed to support these findings.
Fish present different lipid contents according to species-specific factors, their geographical origin and diet, as well as variable intra-specific features, such as size and sexual maturity (Rueda et al., 2001). The relative abundances (%) of FA common to the trunk muscles of H. hippocampus and to the whole body of cultured adults H. kuda, H. trimaculatus, H. comes and H. erectus described in the literature, were compared. As no significant differences were observed between the FA profiles recorded in trunk muscles of female and male H. hippocampus, data from the two sexes were pooled. The seahorse species considered for the interspecific comparison performed in the present study are amongst the most valued syngnathids in TCM (Lin et al., 2008; Chang et al., 2013). Furthermore, they are believed to have several beneficial effects on human health, such as in reducing fatigue and caducity or enhancing immunity (Lin et al., 2008; Lin et al., 2009; Kang et al., 2017), even though there are few scientific studies that have investigated the nutritional value and healthy benefits of Hippocampus spp. (Shen et al., 2016).
The essential DHA has been described to play a relevant role in humans’ nervous tissues (Crawford et al., 1999; Ahmmed et al., 2020). This FA was amongst the most abundant FA in the whole body of cultured H. kuda (27.2% TFA) and H. trimaculatus (22.4% TFA) (Figures 2A, B, respectively and Supplementary Table S1). In line with these two species, the DHA levels in H. hippocampus trunk muscles (12% TFA) were amongst those of FA with a higher relative abundance, which may indicate that cultured specimens of this species could indeed be an interesting source of DHA for humans. The other two seahorse species considered, revealed lower relative abundances of DHA (5.5% TFA for H. comes and 5.6% TFA for H. erectus) than H. hippocampus trunk muscles (Figures 2C, D, respectively and Supplementary Table S1). Additionally, 18:1 n-9 was also one of the most abundant FA, in H. hippocampus (11% TFA), H. comes (11.4% TFA) and H. erectus (20.4% TFA) (Figures 2C, D, respectively and Supplementary Table S1). This FA has modulatory effects in human physiological activities and has been suggested to have a protective role in autoimmune and inflammatory diseases (Sales-Campos et al., 2013). Other important FA to human health that were common in the FA pool of H. hippocampus, as well as other seahorse species already farmed, were EPA and ARA (information only available to date for H. comes (Buen-Ursua et al., 2015) and H. erectus (Vargas-Abúndez et al., 2021)) (Figures 2C, D, respectively and Supplementary Table S1). The relative abundance of EPA was always higher in the four species of seahorses considered than in H. hippocampus trunk muscles, while ARA was higher in H. erectus (7.5% TFA). Eicosapentaenoic acid has been pointed as potentially having a preventive effect in cardiac diseases (Nestel, 2000; Schunck et al., 2018), while ARA has an important role in the fostering of immunity (Sonnweber et al., 2018).
Figure 2 Mean fatty acid relative abundances (% of total fatty acids) of trunk muscle of cultured adult short snout seahorses Hippocampus hippocampus (n = 14) and whole body of cultured adults of H. kuda (n = 8, as described by Lin et al., 2009) (A), H. trimaculatus (n = 8, as described by Lin et al., 2009) (B), H. comes (n = 6, as described by Buen-Ursua et al., 2015) (C) and H. erectus (n = 16, as described by Vargas-Abúndez et al., 2021) (D). The mean relative values of fatty acids from H. kuda and H. trimaculatus, with the exception of 22:6 n-3, are estimated values retrieved from bar graphs.
Total SFA was the most abundant FA class in H. hippocampus trunk muscles (58.5% TFA), as well as in three of the four species identified in the literature (H. kuda, with 47.8% TFA; H. trimaculatus, 47.8% TFA and H. erectus, 45.2% TFA). Polyunsaturated fatty acids followed SFA in terms of their mean relative abundances (H. hippocampus, 28.1% TFA; H. kuda, 33.7% TFA and H. trimaculatus, 31.9% TFA), with the exception of H. erectus, which presented a high relative abundance of MUFA (31.0% TFA) (Figure 3; Supplementary Table S1). Four of the species considered, including H. hippocampus from the present study, were fed with mysids, while H. erectus was fed with amphipods. The higher relative abundance of MUFA than PUFA in H. erectus, may be related to the diet in captivity, since the fatty acid profile of captured amphipods was richer in SFA, followed by MUFA (Vargas-Abúndez et al., 2021). There were no data for FA classes (%TFA) of H. comes. Some of these inter-specific differences might rely, however, on both the effect of temperature and the reproductive stage of the specimens analyzed, factor that deserve further investigation.
Figure 3 Mean relative abundance (% of total fatty acids) of saturated fatty acids (SFA), monounsaturated fatty acids (MUFA) and polyunsaturated fatty acids (PUFA) of trunk muscle of cultured adult short snout seahorses Hippocampus hippocampus (n = 14) and whole body of cultured adults of H. kuda (n = 8, as described by Lin et al., 2009), H. trimaculatus (n = 8, as described by Lin et al., 2009) and H. erectus (n = 16, as described by Vargas-Abúndez et al., 2021). Hippocampus kuda and H. trimaculaus SFA relative compositions are estimated values retrieved from the data available in Lin et al. (2009).
Values of PUFA/SFA ratio below 0.45 have been described as not ideal for human health, as they express the potential of increasing cholesterol levels in the blood (Tonial et al., 2014). The trunk muscles of H. hippocampus presented a PUFA/SFA ratio of 0.49, while cultured H. kuda and H. trimaculatus presented values of 0.91 and 0.87, respectively (Supplementary Table S1). Nevertheless, according to Chen and Liu (2020), the protective role of some MUFA, such as 18:1 n-9, should also be taken into account, as they contribute to the activity of protein receptors that decrease cholesterol in the serum.
The absolute and relative compositions of H. hippocampus trunk muscles may indicate that cultured specimens of this species have the potential to be an interesting source of health-promoting FA, such as DHA, a feature shared with other seahorse species already valued by TCM. While seahorses FA profiles composition may be of interest in terms of their nutritional value, it is important to underline the need to develop sustainable seahorse production practices and foster the conservation of wild populations.
Hippocampus hippocampus is a species, for which aquaculture practices are still being established (Planas et al., 2013) and, although it is apparently not targeted for human consumption, there are some reports of illegal capture in the southern coast of mainland Portugal (Planelles, 2017). This preliminary study provided some clues concerning the nutritional potential of the trunk muscles of cultured H. hippocampus, with male specimens tending to present the best results in terms of the lipid indexes considered (AI, TI, HH and PI), even though there were no significant differences between the two sexes. Furthermore, this species exhibited some trends already highlighted for other cultured seahorse species valued in TCM, such as high relative abundances of DHA and 18:1 n-9 and, to a smaller extent, of EPA and ARA. Cultured H. hippocampus may be a sustainable component of functional foods, as long as its production is framed within strict good practices and can be reliably traced from farm to fork to contribute towards the conservation of wild populations of these flagship marine species.
The original contributions presented in the study are included in the article/Supplementary Material. Further inquiries can be directed to the corresponding authors.
Ethical review and approval was not required for the animal study because The seahorses considered in this study were not euthanized and died from natural causes. The captive breeding program for H. hippocampus (Project HIPPONUTRE, reference 16-02-01-FMP-54) was approved by the ethics committee from the Veterinary Medicines Directorate, Ministry of Agriculture, Rural Development and Fisheries, Portugal. Under this approval, the program is conducted in accordance with the Guidelines of the European Union Council (86/609/EU) and Portuguese legislation for the use of laboratory animals.
Conceptualization, FR, MRD, and RC; methodology, FR, MRD, and MC; validation, FR, MRD, and MC; formal analysis, AEC, FR, and MC; investigation, AEC, FR, and RC; resources, MRD, JP, MP, and RC; data curation AEC, FR, and MC; writing—original draft preparation, AEC; writing—review and editing, All authors; visualization, AEC; supervision, FR, MP, MRD, and RC; funding acquisition, MRD and RC. All authors have read and agreed to the published version of the manuscript.
This study was financially supported by project CITAQUA, “Desenvolvimento do Projeto de Reforço do Polo de Aveiro (H4)”, framed within Measure 10 of Investment TC-C10-i01 - Hub Azul - Rede de Infraestruturas para a Economia Azul, financed by the Recovery and Resilience Plan (RRP) and supported by Fundo Azul of the Portuguese Government. This work was also supported by national funds through Fundação para a Ciência e Tecnologia (FCT) under a PhD grant to AEC (UI/BD/153063/2022) and within the scope of the Individual Call to Scientific Employment Stimulus 2017 with a Junior Researcher contract [CEECIND/00580/2017 to FR]. Additionally, this study received Portuguese national funds from FCT through projects UIDB/04326/2020, UIDP/04326/2020 and LA/P/0101/2020 and was financed by the scientific project HIPPONUTRE – “Cultivo do cavalo marinho de focinho comprido, Hippocampus guttulatus: Optimização zootécnica e avaliação de requisitos nutricionais” (Ref. proj. 16-02-01-FMP-54), financed by the Programa Operacional MAR2020. We also acknowledge financial support to CESAM by FCT/MCTES (UIDP/50017/2020 + UIDB/50017/2020 + LA/P/0094/2020) and LAQV-REQUIMTE (UIDP/50006/2020 + UIDB/50006/2020) through national funds and, where applicable, co-funded by ERDF, within Portugal 2020 Partnership Agreement and Compete 2020, and to the Portuguese Mass Spectrometry Network (RNEM, LISBOA-01-0145-FEDER-402-022125).
We acknowledge Fernando Ricardo for sourcing the seahorse samples from CCMAR and transporting them to the University of Aveiro.
The authors declare that the research was conducted in the absence of any commercial or financial relationships that could be construed as a potential conflict of interest.
All claims expressed in this article are solely those of the authors and do not necessarily represent those of their affiliated organizations, or those of the publisher, the editors and the reviewers. Any product that may be evaluated in this article, or claim that may be made by its manufacturer, is not guaranteed or endorsed by the publisher.
The Supplementary Material for this article can be found online at: https://www.frontiersin.org/articles/10.3389/fmars.2023.1135250/full#supplementary-material
Ahmmed M. K., Ahmmed F., Tian H., Carne A., Bekhit A. E. D. (2020). Marine omega-3 (n-3) phospholipids: a comprehensive review of their properties, sources, bioavailability, and relation to brain health. Compr. Rev. Food Sci. Food Saf. 19 (1), 64–123. doi: 10.1111/1541-4337.12510
Amoussou N., Marengo M., Iko Afé O. H., Lejeune P., Durieux É.D.H., Douny C., et al. (2022). Comparison of fatty acid profiles of two cultivated and wild marine fish from Mediterranean Sea. Aquaculture. Int. 30, 1435–1452. doi: 10.1007/s10499-022-00861-3
Ballester-Lozano G. F., Benedito-Palos L., Navarro J. C., Kaushik S., Pérez-Sánchez J. (2011). Prediction of fillet fatty acid composition of market-size gilthead sea bream (Sparus aurata) using a regression modelling approach. Aquaculture 319 (1-2), 81–88. doi: 10.1016/j.aquaculture.2011.06.015
Bartlett E. M., Lewis D. H. (1970). Spectrophotometric determination of phosphate esters in the presence and absence of orthophosphate. Anal. Biochem. 36, 159–167. doi: 10.1016/0003-2697(70)90343-X
Bligh E. G., Dyer W. J. (1959). A rapid method of total lipid extraction and purification. Can. J. Biochem. Physiol. 37, 911–917. doi: 10.1139/o59-099
Bordignon F., Tomás-Vidal A., Trocino A., Milián Sorribes M. C., Jover-Cerdá M., Martínez-Llorens S. (2020). Fatty acid signatures in different tissues of mediterranean yellowtail, Seriola dumerili (Risso 1810), fed diets containing different levels of vegetable and fish oils. Animals 10 (2), 198. doi: 10.3390/ani10020198
Buen-Ursua S. M. A., Azuma T., Arai K., Coloso R. M. (2015). Improved reproductive performance of tiger tail seahorse, Hippocampus comes, by mysid shrimp fed singly or in combination with other natural food. Aquacult. Int. 23 (1), 29–43. doi: 10.1007/s10499-014-9795-1
Burdge G. C., Calder P. C. (2005). Conversion of α linolenic acid to longer-chain polyunsaturated fatty acids in human adults. Reprod. Nutr. Dev. 45 (5), 581–597. doi: 10.1051/rnd:2005047
Cabral A. E., Ricardo F., Patinha C., Silva E. F. D., Correia M., Palma J., et al. (2021). Successful use of geochemical tools to trace the geographic origin of long-snouted seahorse Hippocampus guttulatus raised in captivity. Animals 11 (6), 1534. doi: 10.3390/ani11061534
Calder P. C. (2013). “Nutritional benefits of omega-3 fatty acids,” in Food enrichment with omega-3 fatty acids. Eds. Jacobsen C., Nielsen N. S., Horn A. F., Sørensen A. D. M. (Cambridge, GB: Woodhead Publishing), 3–26.
Caldwell I. R., Vincent A. C. J. (2012). Revisiting two sympatric European seahorse species: apparent decline in the absence of exploitation. Aquat. Conserv: Mar. Freshw. Ecosyst. 22, 427–435. doi: 10.1002/aqc.2238
Chang C. H., Jang-Liaw N. H., Lin Y. S., Fang Y. C., Shao K. T. (2013). Authenticating the use of dried seahorses in the traditional Chinese medicine market in Taiwan using molecular forensics. J. Food Drug Anal. 21 (3), 310–316. doi: 10.1016/j.jfda.2013.07.010
Chaula D., Laswai H., Chove B., Dalsgaard A., Mdegela R., Hyldig G. (2019). Fatty acid profiles and lipid oxidation status of sun dried, deep fried, and smoked sardine (Rastrineobola argentea) from lake Victoria, tanzania. J. Aquat. Food Prod. Technol. 28 (2), 165–176. doi: 10.1080/10498850.2019.1570992
Chen J., Liu H. (2020). Nutritional indices for assessing fatty acids: A mini-review. Int. J. Mol. Sci. 21 (16), 5695. doi: 10.3390/ijms21165695
Cohen F. P., Valenti W. C., Planas M., Calado R. (2017). Seahorse aquaculture, biology and conservation: knowledge gaps and research opportunities. Rev. Fish. Sci. Aquac. 25 (1), 100–111. doi: 10.1080/23308249.2016.1237469
Conde T. A., Neves B. F., Couto D., Melo T., Neves B., Costa M., et al. (2021). Microalgae as sustainable bio-factories of healthy lipids: evaluating fatty acid content and antioxidant activity. Mar. Drugs 19 (7), 357. doi: 10.3390/md19070357
Correia M., Koldewey H. J., Andrade J. P., Esteves E., Palma J. (2018). Identifying key environmental variables of two seahorse species (Hippocampus guttulatus and Hippocampus hippocampus) in the ria Formosa lagoon, south Portugal. Environ. Biol. Fishes 101 (9), 1357–1367. doi: 10.1007/s10641-018-0782-7
Cowey C. B., Sargent J. R. (1977). Lipid nutrition in fish. Comp. Biochem. Physiol. 57 (4), 269–273. doi: 10.1016/0305-0491(77)90051-7
Crawford M. A., Bloom M., Broadhurst C. L., Schmidt W. F., Cunnane S. C., Galli C., et al. (1999). Evidence for the unique function of docosahexaenoic acid during the evolution of the modern hominid brain. Lipids 34, S39–S47. doi: 10.1007/BF02562227
Curtis J. M. R., Santos S. V., Nadeau J. L., Gunn B., Bigney Wilner K., Balasubramanian H., et al. (2017). Life history and ecology of the elusive European short-snouted seahorse Hippocampus hippocampus. J. Fish Biol. 91 (6), 1603–1622. doi: 10.1111/jfb.13473
Doubleday Z. A., Martino J. C., Trueman C. (2022). Harnessing universal chemical markers to trace the provenance of marine animals. Ecol. Indic. 144, 109481. doi: 10.1016/j.ecolind.2022.109481
Faleiro F., Narciso L. (2013). Prey–predator dynamics in seahorses (Hippocampus guttulatus): deciphering fatty acid clues. Aquac. Res. 44 (4), 618–633. doi: 10.1111/j.1365-2109.2011.03067.x
Foster S. J., Kuo T. C., Wan A. K. Y., Vincent A. C. (2019). Global seahorse trade defies export bans under CITES action and national legislation. Mar. Policy 103, 33–41. doi: 10.1016/j.marpol.2019.01.014
Ghasemi A., Ghavimi R., Momenzadeh N., Hajian S., Mohammadi M. (2021). Characterization of antitumor activity of a synthetic moronecidin-like peptide computationally predicted from the tiger tail seahorse Hippocampus comes in tumor-bearing mice. Int. J. Pept. Res. Ther. 27, 2391–2401. doi: 10.1007/s10989-021-10260-6
Himaya S. W. A., Ryu B., Qian Z. J., Kim S. K. (2012). Paeonol from Hippocampus kuda bleeler suppressed the neuro-inflammatory responses in vitro via NF-κB and MAPK signaling pathways. toxicol. In Vitro 26 (6), 878–887. doi: 10.1016/j.tiv.2012.04.022
Huang X., Yin Y., Shi Z., Li W., Zhou H., Lv W. (2010). Lipid content and fatty acid composition in wild-caught silver pomfret (Pampus argenteus) broodstocks: effects on gonad development. Aquaculture 310 (1-2), 192–199. doi: 10.1016/j.aquaculture.2010.10.015
Jobling M., Johansen S. J. S., Foshaug H., Burkow I. C., Jørgensen E. H. (1998). Lipid dynamics in anadromous Arctic charr, Salvelinus alpinus (L.): seasonal variations in lipid storage depots and lipid class composition. Fish. Physiol. Biochem. 18 (3), 225–240. doi: 10.1023A:1007747201521
Johnston T. A., Wiegand M. D., Szmadyla R. L., Porteous L. R., Casselman J. M., Leggett W. C. (2020). Sex-based differences in fatty acid composition of adult walleye. Ecol. Freshw. Fish 29 (4), 654–664. doi: 10.1111/eff.12541
Kang N., Kim S. Y., Rho S., Ko J. Y., Jeon Y. J. (2017). Anti-fatigue activity of a mixture of seahorse (Hippocampus abdominalis) hydrolysate and red ginseng. Fish Aquat. Sci. 20, 1–8. doi: 10.1186/s41240-017-0048-x
Kim H. S., Kim S. Y., Fernando I. S., Sanjeewa K. A., Wang L., Lee S. H., et al. (2019). Free radical scavenging activity of the peptide from the alcalase hydrolysate of the edible aquacultural seahorse (Hippocampus abdominalis). J. Food Biochem. 43 (7), e12833. doi: 10.1111/jfbc.12833
Koldewey H. J., Martin-Smith K. M. (2010). A global review of seahorse aquaculture. Aquaculture 302 (3-4), 131–152. doi: 10.1016/j.aquaculture.2009.11.010
Leal M. C., Pimentel T., Ricardo F., Rosa R., Calado R. (2015). Seafood traceability: current needs, available tools, and biotechnological challenges for origin certification. Trends Biotechnol. 33 (6), 331–336. doi: 10.1016/j.tibtech.2015.03.003
Lin Q., Lin J., Lu J., Li B. (2008). Biochemical composition of six seahorse species, hippocampus sp., from the Chinese coast. J. World Aquac. Soc 39 (2), 225–234. doi: 10.1111/j.1749-7345.2008.00159.x
Lin Q., Lin J., Wang C. (2009). Biochemical composition of the wild and cultured seahorses, Hippocampus kuda bleeker and Hippocampus trimaculatus leach. Aquac. Res. 40 (6), 710–719. doi: 10.1111/j.1365-2109.2008.02149.x
Lourie S. (2003). “Measuring seahorses,” in [Project seahorse technical report No.4], vol. 15. (University of British Columbia Fisheries Centre).
Lubis Z., Buckle K. A. (1990). Rancidity and lipid oxidation of dried-salted sardines. Int. J. Food Sci. Technol. 25, 295–303. doi: 10.1111/j.1365-2621.1990.tb01085.x
Marques B., Lillebø A. I., Domingues M. D. R. M., Saraiva J. A., Calado R. (2019). Effect of high-pressure processing (HPP) on the fatty acid profile of different sized ragworms (Hediste diversicolor) cultured in an integrated multi-trophic aquaculture (IMTA) system. Molecules 24 (24), 4503. doi: 10.3390/molecules24244503
Monge-Ortiz R., Tomás-Vidal A., Rodriguez-Barreto D., Martínez-Llorens S., Pérez J. A., Jover-Cerdá M., et al. (2018). Replacement of fish oil with vegetable oil blends in feeds for greater amberjack (Seriola dumerili) juveniles: effect on growth performance, feed efficiency, tissue fatty acid composition and flesh nutritional value. Aquac. Nutr. 24 (1), 605–615. doi: 10.1111/anu.12595
Nestel P. J. (2000). Fish oil and cardiovascular disease: lipids and arterial function. Am. J. Clin. Nutr. 71 (1), 228S–231S. doi: 10.1093/ajcn/71.1.228S
Njinkoué J. M., Barnathan G., Miralles J., Gaydou E. M., Samb A. (2002). Lipids and fatty acids in muscle, liver and skin of three edible fish from the Senegalese coast: Sardinella maderensis, Sardinella aurita and cephalopholis taeniops. comp. Biochem. Physiol. - B Biochem. Mol. Biol. 131 (3), 395–402. doi: 10.1016/S1096-4959(01)00506-1
Olivotto I., Planas M., Simões N., Holt G. J., Avella M. A., R. Calado R. (2011). Advances in breeding and rearing marine ornamentals. J. World Aquacult. Soc 42, 135–166. doi: 10.1111/j.1749-7345.2011.00453.x
Osako K., Kuwahara K., Saito H., Hossain M. A., Nozaki Y. (2003). Effect of starvation on lipid metabolism and stability of DHA content of lipids in horse mackerel (Trachurus japonicus) tissues. Lipids 38 (12), 1263–1267. doi: 10.1007/s11745-003-1187-5
Planas M., Burhans R., Simões N. (2017). “Seahorses and pipefish,” in Marine ornamental species aquaculture. Eds. Calado R., Olivotto I., Planas M., Holt G. J. (West Sussex, UK: Wiley Blackwell), 299–326.
Planas M., Olivotto I., González M. J., Laurà R., Angeletti C., Amici A., et al. (2021). Pre-breeding diets in the seahorse Hippocampus reidi: how do they affect fatty acid profiles, energetic status and histological features in newborn? Front. Mar. Sci. 8, 688058. doi: 10.3389/fmars.2021.688058
Planas M., Olivotto I., González M. J., Laurà R., Zarantoniello M. (2020). A multidisciplinary experimental study on the effects of breeders diet on newborn seahorses (Hippocampus guttulatus). Front. Mar. Sci. 7, 638. doi: 10.3389/fmars.2020.00638
Planas M., Quintas P., Chamorro A. (2013). Maturation of Hippocampus guttulatus and Hippocampus hippocampus females by manipulation of temperature and photoperiod regimes. Aquaculture 388, 147–152. doi: 10.1016/j.aquaculture.2013.01.030
Planelles M. (2017). El Lucrativo contrabando con caballitos de mar (El País). Available at: https://elpais.com/politica/2017/01/16/actualidad/1484595017_603568.html.
Rey F., Alves E., Domingues P., Domingues M. R. M., Calado R. (2018). A lipidomic perspective on the embryogenesis of two commercially important crabs, Carcinus maenas and Necora puber. bull. Mar. Sci. 94 (4), 1395–1411. doi: 10.5343/bms.2017.1140
RStudio Team (2019). RStudio: integrated development for r. Ed. Boston M. A. (RStudio, Inc.). Available at: http://www.rstudio.com/.
Rueda F. M., Hernández M. D., Egea M. A., Aguado F., García B., Martínez F. J. (2001). Differences in tissue fatty acid composition between reared and wild sharpsnout sea bream, Diplodus puntazzo (Cetti 1777). Brit. J. Nutr. 86 (5), 617–622. doi: 10.1079/BJN2001438
Sainmont J., Andersen K. H., Varpe Ø., Visser A. W. (2014). Capital versus income breeding in a seasonal environment. Am. Nat. 184 (4), 466–476. doi: 10.1086/677926
Sales-Campos H., Reis de Souza P., Crema Peghini B., Santana da Silva J., Ribeiro Cardoso C. (2013). An overview of the modulatory effects of oleic acid in health and disease. mini-rev. Med. Chem. 13 (2), 201–210. doi: 10.2174/1389557511313020003
Scaioli E., Liverani E., Belluzzi A. (2017). The imbalance between n-6/n-3 polyunsaturated fatty acids and inflammatory bowel disease: a comprehensive review and future therapeutic perspectives. Int. J. Mol. Sci. 18 (12), 2619. doi: 10.3390/ijms18122619
Schunck W. H., Konkel A., Fischer R., Weylandt K. H. (2018). Therapeutic potential of omega-3 fatty acid-derived epoxyeicosanoids in cardiovascular and inflammatory diseases. Pharmacol. Ther. 183, 177–204. doi: 10.1016/j.pharmthera.2017.10.016
Shen Q., Dai Z., Huang Y. W., Cheung H. Y. (2016). Lipidomic profiling of dried seahorses by hydrophilic interaction chromatography coupled to mass spectrometry. Food Chem. 205, 89–96. doi: 10.1016/j.foodchem.2016.02.151
Simopoulos A. P. (2002). The importance of the ratio of omega-6/omega-3 essential fatty acids. Biomed. Pharmacother. 56 (8), 365–379. doi: 10.1016/S0753-3322(02)00253-6
Sonnweber T., Pizzini A., Nairz M., Weiss G., Tancevski I. (2018). Arachidonic acid metabolites in cardiovascular and metabolic diseases. Int. J. Mol. Sci. 19 (11), 3285. doi: 10.3390/ijms19113285
Sushchik N. N., Makhutova O. N., Rudchenko A. E., Glushchenko L. A., Shulepina S. P., Kolmakova A. A., et al. (2020). Comparison of fatty acid contents in major lipid classes of seven salmonid species from Siberian Arctic lakes. Biomolecules 10 (3), 419. doi: 10.3390/biom10030419
The IUCN Red List of Threatened Species. (2017a). Hippocampus hippocampus (Global assessment). Available at: https://www.iucnredlist.org/species/10069/67618259 (Accessed November 14, 2022).
The IUCN Red List of Threatened Species. (2017b). Hippocampus hippocampus (Mediterranean assessment). Available at: https://www.iucnredlist.org/species/10069/90866381 (Accessed December 1, 2022).
Tocher D. R. (2015). Omega-3 long-chain polyunsaturated fatty acids and aquaculture in perspective. Aquaculture 449, 94–107. doi: 10.1016/j.aquaculture.2015.01.010
Tonial I. B., Oliveira D. F., Coelho A. R., Matsushita M., Coró F. A. G., De Souza N. E., et al. (2014). Quantification of essential fatty acids and assessment of the nutritional quality indexes of lipids in tilapia alevins and juvenile tilapia fish (Oreochromis niloticus). J. Food Res. 3 (3), 105–114. doi: 10.5539/jfr.v3n3p105
Ulbricht T. L. V., Southgate D. A. T. (1991). Coronary heart disease: seven dietary factors. Lancet 338, 985–992. doi: 10.1016/0140-6736(91)91846-M
Vaidyanathan T., Zhang X., Balakrishnan R., Vincent A. (2021). Catch and trade bans for seahorses can be negated by non-selective fisheries. Aquat. Conserv.: Mar. Freshw. Ecosyst. 31 (1), 43–59. doi: 10.1002/aqc.3419
Vallecillos A., Marín M., Bortoletti M., López J., Afonso J. M., Ramis G., et al. (2021). Genetic analysis of the fatty acid profile in gilthead seabream (Sparus aurata l.). Animals 11 (10), 2889. doi: 10.3390/ani11102889
Vargas-Abúndez J. A., Martínez-Moreno G. L., Simões N., Noreña-Barroso E., Mascaró M. (2021). Marine amphipods (Parhyale hawaiensis) as an alternative feed for the lined seahorse (Hippocampus erectus, perri 1810): nutritional value and feeding trial. PeerJ 9, e12288. doi: 10.7717/peerj.12288
Vincent A. C. (1996). “The international trade in seahorses,” in [Traffic network report] (Cambridge, UK: Traffic International).
Vincent A. C., Foster S. J., Koldewey H. J. (2011). Conservation and management of seahorses and other syngnathidae. J. Fish Biol. 78 (6), 1681–1724. doi: 10.1111/j.1095-8649.2011.03003.x
Vincent A. C., Meeuwig J. J., Pajaro M. G., Perante N. C. (2007). Characterizing a small-scale, data-poor, artisanal fishery: seahorses in the central Philippines. Fish. Res. 86 (2-3), 207–215. doi: 10.1016/j.fishres.2007.06.006
Wickham H. (2016). Ggplot2: elegant graphics for data analysis. 2nd ed (New York, USA: Springer International Publishing).
Woods C. M. (2003). Effects of varying artemia enrichment on growth and survival of juvenile seahorses, Hippocampus abdominalis. Aquaculture 220 (1-4), 537–548. doi: 10.1016/S0044-8486(02)00639-7
Xu H., Cao L., Wei Y., Zhang Y., Liang M. (2018). Lipid contents in farmed fish are influenced by dietary DHA/EPA ratio: a study with the marine flatfish, tongue sole (Cynoglossus semilaevis). Aquaculture 485, 183–190. doi: 10.1016/j.aquaculture.2017.11.054
Xu Y., Ryu S., Lee Y. K., Lee H. J. (2020). Brassicasterol from edible aquacultural Hippocampus abdominalis exerts an anti-cancer effect by dual-targeting AKT and AR signaling in prostate cancer. Biomedicines 8 (9), 370. doi: 10.3390/biomedicines8090370
Keywords: DHA, GC-MS, lipids, nutritional value, omega-3 fatty acids, syngnathids, seahorse
Citation: Cabral AE, Rey F, Domingues MR, Cabral M, Planas M, Palma J and Calado R (2023) Fatty acid profiles of cultured Hippocampus hippocampus trunk muscles and potential nutritional value. Front. Mar. Sci. 10:1135250. doi: 10.3389/fmars.2023.1135250
Received: 31 December 2022; Accepted: 25 April 2023;
Published: 12 May 2023.
Edited by:
Demin Cai, Yangzhou University, ChinaReviewed by:
Ivan Viegas, University of Coimbra, PortugalCopyright © 2023 Cabral, Rey, Domingues, Cabral, Planas, Palma and Calado. This is an open-access article distributed under the terms of the Creative Commons Attribution License (CC BY). The use, distribution or reproduction in other forums is permitted, provided the original author(s) and the copyright owner(s) are credited and that the original publication in this journal is cited, in accordance with accepted academic practice. No use, distribution or reproduction is permitted which does not comply with these terms.
*Correspondence: Ricardo Calado, cmpjYWxhZG9AdWEucHQ=; Felisa Rey, ZmVsaXNhLnJleUB1YS5wdA==
Disclaimer: All claims expressed in this article are solely those of the authors and do not necessarily represent those of their affiliated organizations, or those of the publisher, the editors and the reviewers. Any product that may be evaluated in this article or claim that may be made by its manufacturer is not guaranteed or endorsed by the publisher.
Research integrity at Frontiers
Learn more about the work of our research integrity team to safeguard the quality of each article we publish.