- 1National Center for Scientific Research, Research and Service Units (USR) 3278 - Centre de Recherches Insulaires et Observatoire de l’environnement (CRIOBE) - École Pratique des Hautes Études (EPHE)-University of Perpignan Via Domitia (UPVD)-CNRS, Perpignan, France
- 2Department of Earth and Marine Sciences, University of Palermo, Palermo, Italy
In the Mediterranean Sea, vermetid reefs can modify coastal seascapes forming platforms in the intertidal zone of rocky coasts. With their three-dimensional and seaward-expanding structure, these bioconstructions support high biodiversity levels and provide important ecological functions and ecosystem services. In this study, we perform a first structural characterization of a vermetid reef seascape (hereafter, vermetid reefscape) and quantitatively assess the degree of their contribution to the structural complexity of the coastal seascape. Aerial images of a vermetid reef coast were acquired in the Marine Protected Area of Capo Gallo-Isola delle Femmine (Southern Tyrrhenian Sea) by means of unmanned aerial vehicle technology. In the seascape, the outer reef, platform, and coast classes were identified and digitized in GIS environment. The resulting vermetid reefscape was analysed by means of FRAGSTATS software using metrics belonging to the “area-edge”, “shape”, and “aggregation” categories. To quantitatively assess the structural complexity, the edge density, area-weighted perimeter area ratio, and landscape shape index metrics were applied to the seascapes with and without the vermetid reefs to simulate a sea level rise scenario. In addition, the effect of their presence in terms of coast length (i.e., total edge) was statistically tested using the Wilcoxon signed rank test. The outer reef had the highest total edge value (5,785.6 m) and, at the same time, the lowest class area (1,040 m2). It was also the patchiest, and the most fragmented and geometrically complex class in the seascape. Overall, the bioconstruction positively contributed to the structural complexity of the seascape with higher values of coastal area (12%), edge density (139%), area-weighted perimeter-area ratio (90%), and landscape shape index (66%). The Wilcoxon test revealed a statistically significant effect of the vermetid reefs presence on the coastal total edge (z = 3.9, p < 0.005), with a large effect size (r = 0.74). The results showed an overall higher structural complexity of the vermetid reefscape, indicating that its loss would lead to a significantly less complex seascape, entailing detrimental effects on the supported biodiversity levels and the valuable ecosystem services provided.
1 Introduction
In recent years, seascape ecology rapidly developed as the marine-centric counterpart of the landscape ecology (Turner, 1989; Ray, 1991; Wedding et al., 2011; Bell and Furman, 2017; Pittman et al., 2018), emerging as a new and interdisciplinary science for marine sustainability studies (Pittman et al., 2021). Its applications allow for the exploration of the multi-scale linkages between ecological structure, function, and change in marine environments using a spatially explicit and quantitative approach, with potential to inform management and conservation practices (Pittman et al., 2021). As in the case of the landscape ecology, main focuses are the study of seascape composition (i.e., what and how much is present of each habitat or cover type) and configuration (i.e., the spatial structure or the arrangement of the spatial elements). Changes in configuration are expected to cause changes in ecological functions (Turner, 1989; Turner and Gardner, 2015), hence the importance to assess the relationships between spatial patterns and ecological processes and/or services occurring in the seascape. To do so, seascape ecology has inherited numerous metrics designed to quantify spatial properties in landscapes and analyze spatial patterns (O’Neill et al., 1988; McGarigal, 2002; Cushman et al., 2008; Turner and Gardner, 2015). Their application has proven useful also in the marine realm (Boström et al., 2011; Wedding et al., 2011), especially in coastal seascape research (Bell and Furman, 2017).
So far, the research interest of seascape ecologists has mainly focused on benthic seascapes (Zajac et al., 2000; Brown et al., 2011; Proudfoot et al., 2020), with a number of applications in nearshore and shallow water areas (Pittman et al., 2004; Boström et al., 2011). The attention to coastal seascapes gained momentum along with the research on coastal and marine ecosystem services, which in most cases are provided and therefore more appropriately evaluated and managed at the entire seascape scale (Barbier, 2012; Barbier, 2017). Coastal seascape ecology has been investigating several subtidal and intertidal biogenic habitats across different spatial scales (Robbins and Bell, 1994; Garrabou et al., 1998; Fonseca et al., 2002; Boström et al., 2011; Pittman and Brown, 2011; Furman et al., 2015; Parnell, 2015; Staveley et al., 2017). Among these, marine bioconstructions are of peculiar interest as they modify the biophysical properties of the seascape through their long-lasting and three-dimensional structures that serve as a secondary substrate for the colonization by other marine organisms (Ingrosso et al., 2018). It could be expected that the higher the complexity, size, and stability of these formations, the greater the positive effects on species number and diversity (McCoy and Bell, 1991; Sebens, 1991). Complexity can be defined as “the absolute abundance (per unit area or per unit volume) of individual structural components” for a certain scale of investigation (McCoy and Bell, 1991) and is an important feature of seascape configuration. As such, complexity assessments are used in both two- and three-dimensional seascape analyses. In the case of marine bioconstructions, shape complexity is most commonly investigated relatively to their three-dimensional structure (Burns et al., 2019; Price et al., 2019; Pascoe et al., 2021; Ventura et al., 2021; Carlot et al., 2023), which influences the heterogeneity and therefore the number of microhabitats provided. Irregularities such as holes and crevices provide surfaces with different microclimatic conditions (e.g., humidity, light exposure) and/or shelter from predators compared to the surrounding environment, influencing the number of species that can coexist and resulting in characteristic assemblages of species.
In the Mediterranean Sea, seascape ecology studies on biogenic habitats focused mainly on seagrass meadows (Gatti et al., 2012; Pagès et al., 2014; Pace et al., 2017; Abadie et al., 2018; Rodil et al., 2021) and coralligenous formations (Garrabou et al., 1998; Bracchi et al., 2017; Sini et al., 2019) with many other marine habitats yet to be explored. It is the case of the vermetid reefs, a carbonatic bioconstruction built by the synergistic activity of the encrusting red alga Neogoniolithon brassica-florida and gregarious gastropods belonging to the genus Dendropoma (Safriel, 1966), namely D. cristatum, D. anguliferum, D. lebeche (Templado et al., 2015), and an undescribed one in the Ionian Sea (Calvo et al., 2009). These formed a cryptic complex of species (Templado et al., 2015) under the name of Dendropoma petraeum, which was recently disentangled by Templado et al. (2015).
Vermetid reefs are found at the tide level in rocky shores and can have different morphologies, from simple and thin crusts to broad platforms expanding seawards (Laborel, 1987; Antonioli et al., 1999). Other shapes can result from differential erosive processes, such as the mushroom-like pillars, micro-atolls, and islands (Safriel, 1966; Antonioli et al., 1999). Platforms represent the most complex among the vermetid reef structures (Milazzo et al., 2016), on which the characteristic outer reef, inner edge, and cuvette can be identified (Figure 1). The outer reef is the biologically active part of the reef, dense with living Dendropoma individuals. It grows upwards and seawards as a crevices-rich and complex structure, often exceeding 50 cm of thickness (Chemello and Silenzi, 2011). The inner edge is a small Dendropoma encrustation that can be found at the boundary between the platform and the rocky shore, acting as a superior delimiter of the reef. Compared to its outer counterpart, the inner edge is thinner, mostly vertically developed, and with less dense Dendropoma abundances. Enclosed by the two margins is the zone of the cuvettes, shallow depressions no deeper than 50 cm that form small pools in low tide and calm water conditions (Molinier and Picard, 1953).
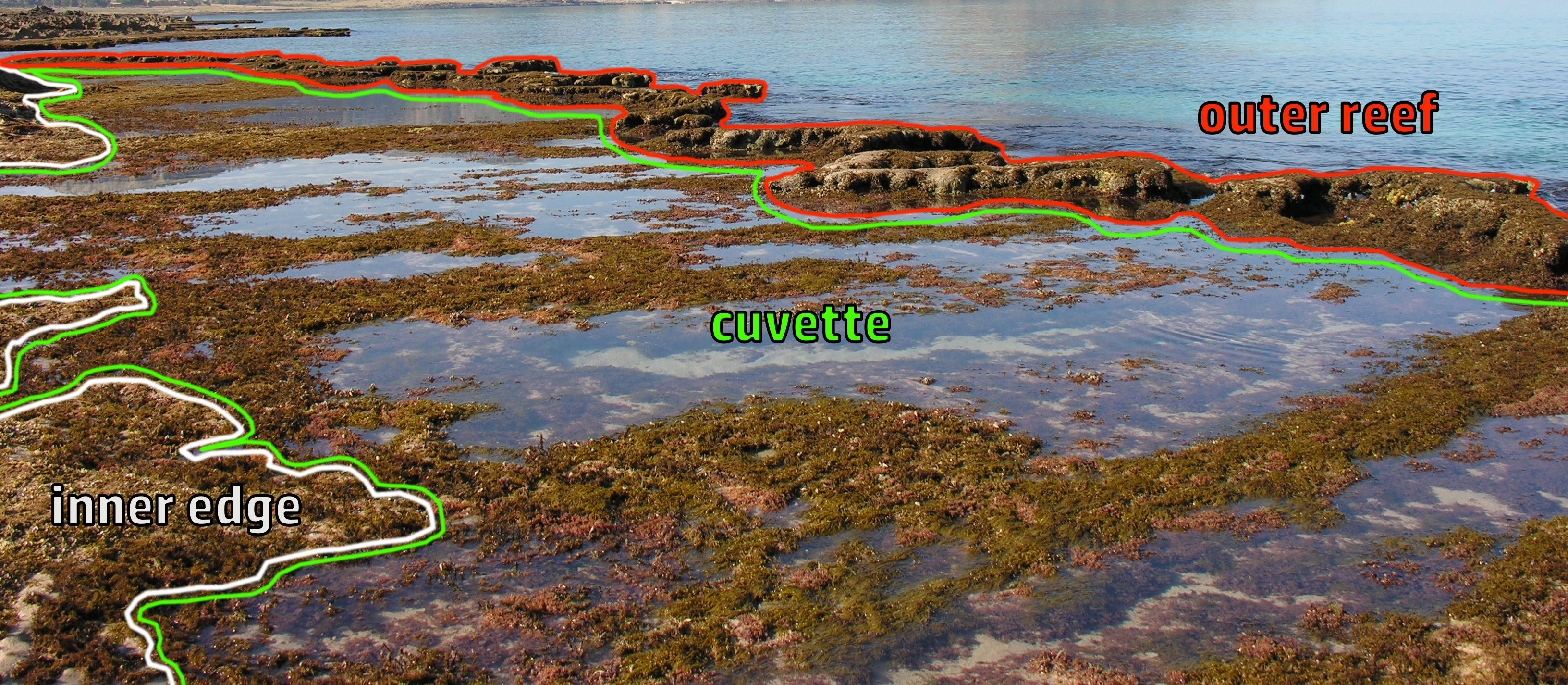
Figure 1 Picture of a vermetid reef platform in the northwestern coast of Sicily (Italy). The outer reef, cuvette, and inner edge parts are shown (Photo Credits: R.C.).
Considering the structural features of the vermetid reefs, a coastal seascape in which they are densely present is supposed to have higher structural complexity and heterogeneity levels compared to seascapes in which they are absent. With their three-dimensional and seaward-expanding structure, vermetid reefs support high biodiversity levels (Safriel and Ben-Eliahu, 1991), providing additional space and microhabitats, reduction of physical disturbances, refuge from predation, and a nursery habitat for many benthic and fish assemblages (Goren and Galil, 2001; Chemello and Silenzi, 2011). In addition to benefitting marine biodiversity, the presence of the vermetid reefs in the coastal seascape provides many valuable ecosystem services such as coastal protection, carbon sequestration, and sediment transport regulation (Milazzo et al., 2016).
Despite such ecological importance to Mediterranean coastal seascapes, vermetid reefs are a poorly known and neglected habitat (Picone et al., 2022), whose persistence is at risk. Threatened by a high anthropogenic pressure and the global climate change, local declines and even local losses have been observed and reported in the eastern and central areas of the Mediterranean Sea (Galil, 2013; Badreddine et al., 2019; Bisanti et al., 2022). Ocean warming and acidification negatively impact early life stages of D. cristatum, thus impairing the building of new reef surface (Alessi et al., 2019). In addition, considering the slow growth rate of Dendropoma, sea level rise is likely one of the most threatening pressures to these bioconstructions (Milazzo et al., 2016). The projected sea level rise under future emissions scenarios (Cooley et al., 2022) would likely lead to the submergence of the reefs, entailing the loss of their structures and key functions in the intertidal zone, with detrimental effects on biodiversity as well as ecosystem services provided. The non-living structures of the submerged reefs would likely continue playing a role in supporting biodiversity in deeper waters, but their original contribution to the structural complexity of the seascape as well as their unique ecological role would be lost.
To our knowledge, no study so far has characterized a coastal seascape with vermetid reefs (hereafter “vermetid reefscape”) through the landscape ecology lens. Such approach would provide new insights on the structural properties of the vermetid reefs and set a reference baseline for future studies. In this paper, we provide a first characterization of a vermetid reefscape using landscape ecology metrics, and quantitatively assess the degree of its contribution to the structural complexity of the coastal seascape.
2 Materials and methods
2.1 Study area and data collection
The characterization of the vermetid reefscape was performed in the “Capo Gallo – Isola delle Femmine” Marine Protected Area (CG - 38°C12′37.33”N – 13°C17′10.62”E), along the north-western coast of Sicily (South of Italy). The area is mainly characterized by limestone rocks and shallow rocky coasts along which large vermetid reef platforms can be found. A coast section of 830 m length with a dense presence of reefs was identified and chosen as the study site (Figure 2A). A high-resolution (0.05 m/px) georeferenced aerial image (GeoTIFF) of the study area was produced through unmanned aerial vehicle technology (UAV) by Donnarumma et al. (2021) (Figure 2B). After distributing 24 georeferenced Ground Control Points (GCPs), four flights were performed over the 830 m coastline of the study area and covering a total surface of about 5 ha, using a Hexarotor Skyrobotic SF6, equipped with the SONY DSC-QX100 camera, at 40 m AGL (Above Ground Level) (Figure S1). To make the orthophotomosaic, a total of 700 high quality image frames were produced and processed using the photogrammetric image elaboration software AGISoft Photoscan (Donnarumma et al., 2021).
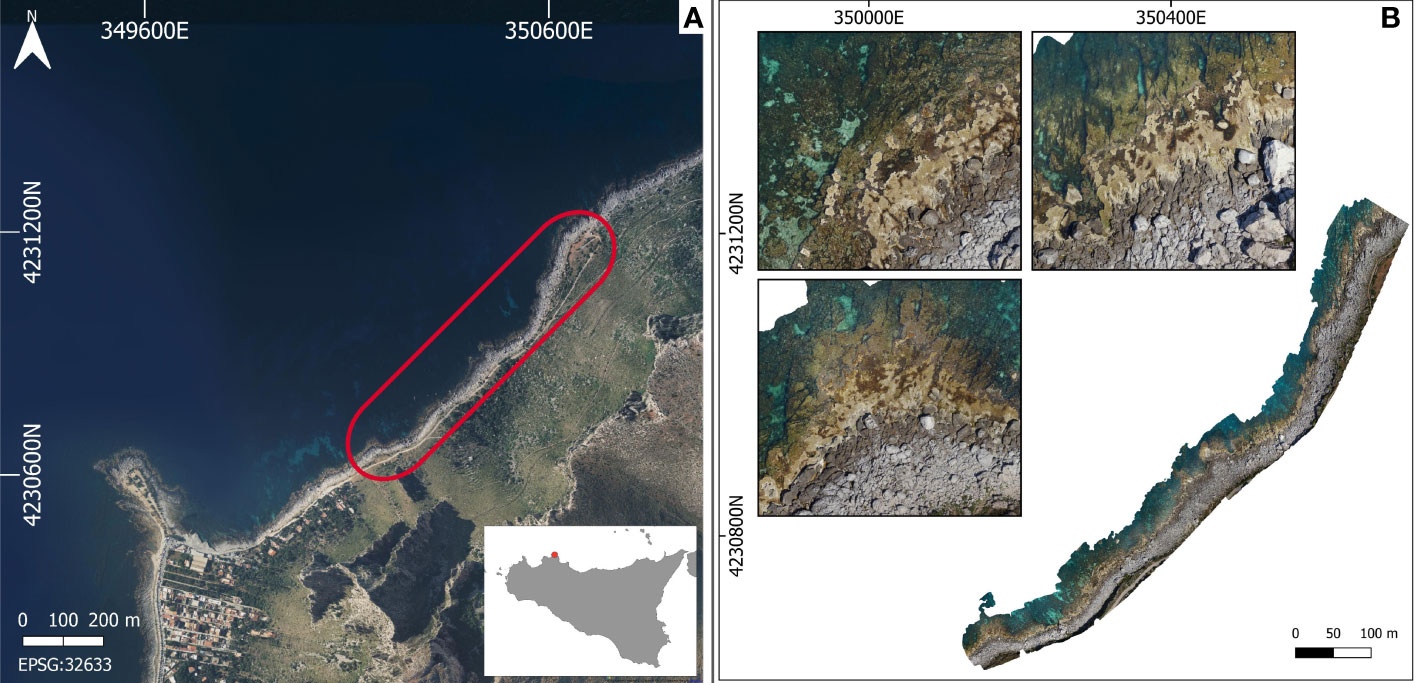
Figure 2 (A) Capo Gallo –Isola delle Femmine Marine Protected Area (Sicily, Italy). The red area encloses the investigated coastal seascape. (B) Aerial image of the study area, generated through unmanned aerial vehicle technology (Donnarumma et al., 2021).
To characterize the vermetid reefscape, the main classes composing the seascape were identified. Patches of the outer reef class were selected as the outer and clearer areas of the bioconstruction, in contrast to the usually adjacent and darker patches of the cuvette zone. Due of its main vertical component, the inner edge could not be correctly represented by a 2D-image. For this reason, this portion of the reef was identified together with the cuvette zone as single class named the platform. For the sake of comprehensiveness and boundaries calculations, the class sea was identified in the seascape too. Finally, the coast class was selected as the remaining areas between the abovementioned classes and the road bordering the inner parts of the seascape.
Starting from the aerial image of the investigated area, the four seascape classes were manually digitized in GIS environment (QGIS, version 3.16.14) as a polygon vector file. The resulting vermetid reefscape (VRS) was produced considering only those features falling in a 25 m buffer area developed around the coast edge (Figure 3). The produced vector seascape was finally validated through in situ measurements.
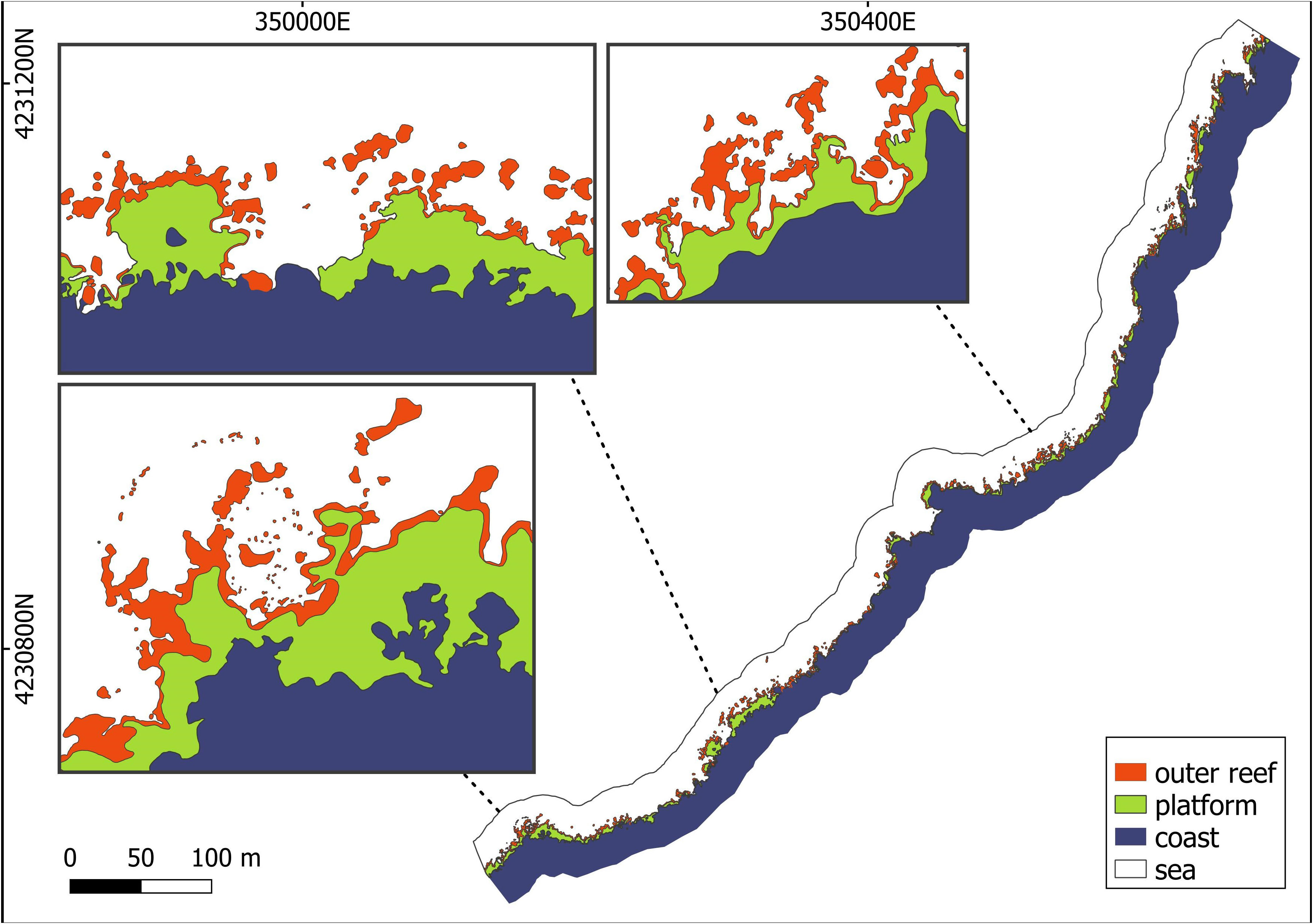
Figure 3 Vermetid reefscape (VRS) and its composing classes (i.e., outer reef, platform, coast, and sea) after the digitization of the aerial image of the studied area.
Starting from the vermetid reefscape, two additional seascape scenarios were produced:
- reef coast (RC), in which outer reef, platform, and coast classes were merged (Figure 4);
- no-reef coast (NRC), in which the outer reef and platform classes were removed and only the coast is present (Figure 4). The seascape represents a hypothetical scenario in which the vermetid reefs are not present as a result of their growth rate (Sisma-Ventura et al., 2020) and the local projected sea level rise rates (Lo Presti et al., 2022).
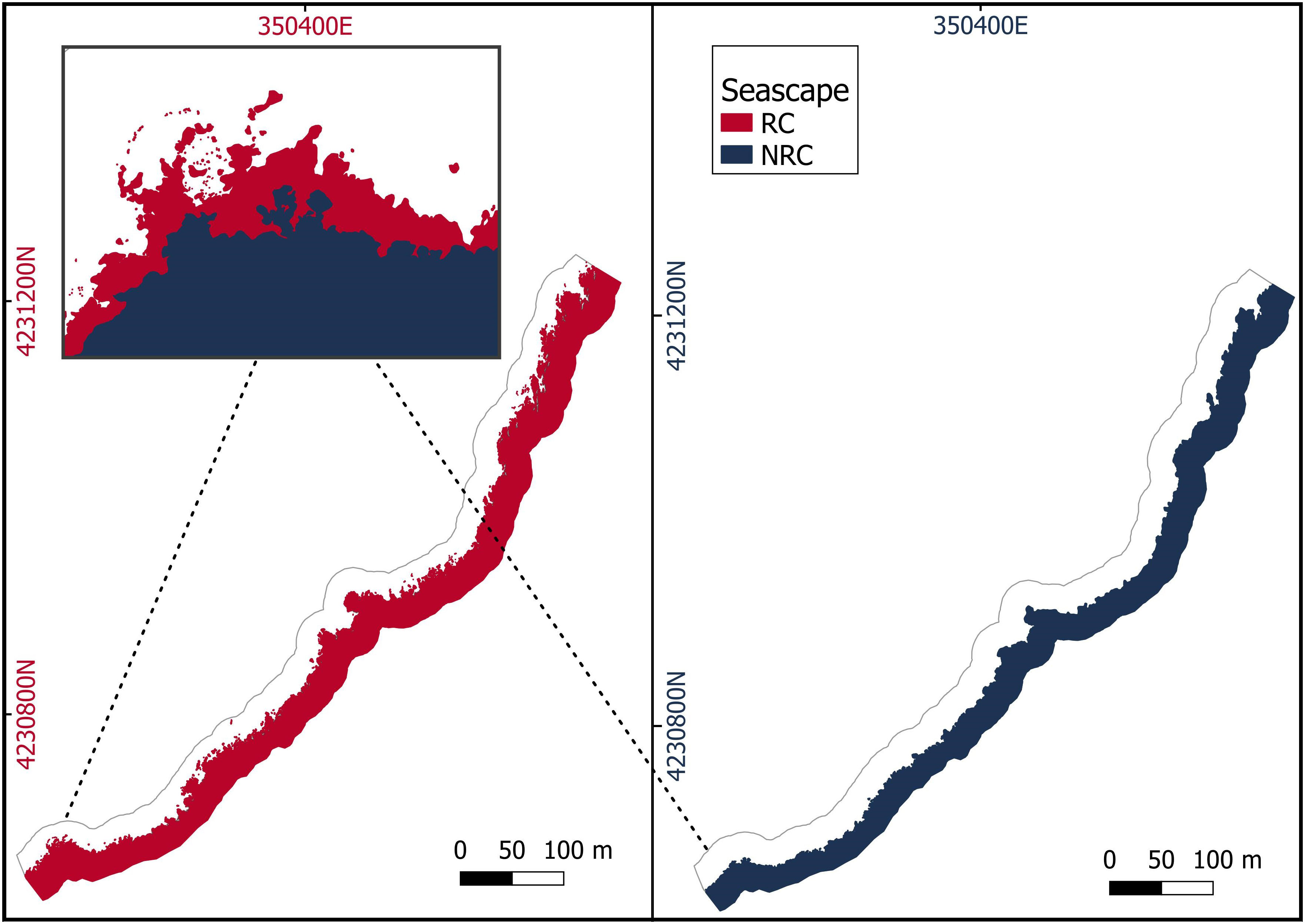
Figure 4 Reef coast (RC) seascape, in which the vermetid reef and the coast are merged in a single class, and a hypothetical scenario of the same seascape without the vermetid reefs (no-reef coast - NRC). The magnified image shows the overlapping of the two seascapes.
2.2 Characterization of seascape structure and composition
To characterize the structure and composition of the vermetid reefscape, landscape ecology metrics were applied at both the class and the landscape levels using Fragstats (version 4.2.1), a software tool designed to quantify landscape structure (McGarigal and Marks, 1995). The metrics used in this study belonged to the “area-edge”, “shape”, and “aggregation” categories included in the software tool. The class metrics “total edge” (TE), “class area” (CA), “edge density” (ED), “percentage of landscape” (PLAND), “area-weighted perimeter-area ratio” (PARA AM), “number of patches” (NP), “patch density” (PD), “splitting index” (SPLIT), and “landscape shape index” (LSI) were applied to the classes of the VRS seascape. The landscape version of the same metrics, except for CA, PLAND, NP, and SPLIT, were applied to the RC and NRC seascapes (Table 1).
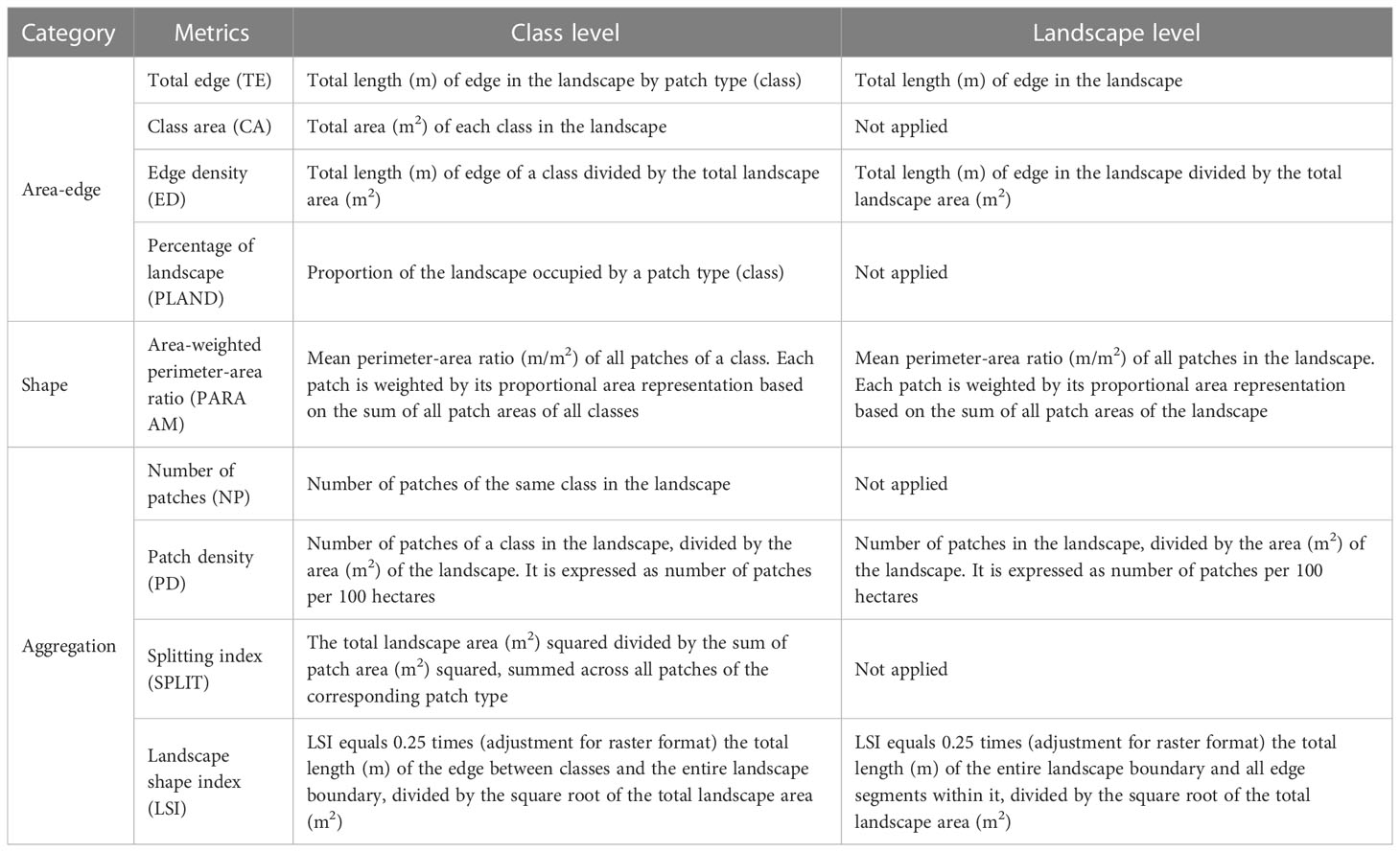
Table 1 Fragstats metrics applied for the characterization of the seascape structure and composition at class and landscape level.
To use Fragstats, the vector files of the three seascapes (VRS, RC, and NRC) were converted to raster files (GeoTIFF) using a 0.1 m/px resolution. In computing area-edge metrics, no background/boundary interface was counted as edge. The sea class was disabled and no metric was applied to it.
2.3 Structural complexity comparison in seascape scenarios
To account for the presence of the vermetid reefs in the coastal seascape in terms of added structural complexity, seascapes RC and NRC (i.e., with and without the reef) were compared. As a first explorative approach, a set of landscape metrics previously applied for the seascape analysis was used. The effect of the vermetid reefs on the seascape focused on metrics based on length-area ratios to account for the structural modifications caused by the presence of the bioconstruction in the seascape. The metrics selected for such comparison were edge density (ED), area-weighted perimeter-area ratio (PARA AM), and landscape shape index (LSI). The area (CA) metric was also included as a reference measure. The contribution was calculated as the natural logarithm of the ratios between the RC and the NRC values for each metric, so that a positive value would indicate an increase in the metric output provided by the presence of the vermetid reefs.
Following, a statistical approach was used to test the effect of the “presence of vermetid reefs” factor on the total edge of the coast. The quantity of total edge per unit area was used as a proxy of the overall coast complexity, being it one of the building components of all the used complexity metrics. To do so, RC and NRC coasts were both sampled using a total of 195 adjacent rectangles of 5 m length and 30 m width. The rectangles were created as vector polygons in QGIS and placed continuously along the coast perpendicularly to its profile to avoid the influence of changes in coast orientation on the samples. Once placed, rectangles were then positioned so to include both the RC and the NRC coastal edges. To make the RC samples representative of the reef structure along the coast, different width classes were identified (Figures S2, S3). Reef width was accounted for using reef area in the samples as a proxy (i.e., the greater the area of the reef in the rectangle, the longer its width). Based on the value range obtained from all the 195 RC samples, five classes were produced (Table 2).
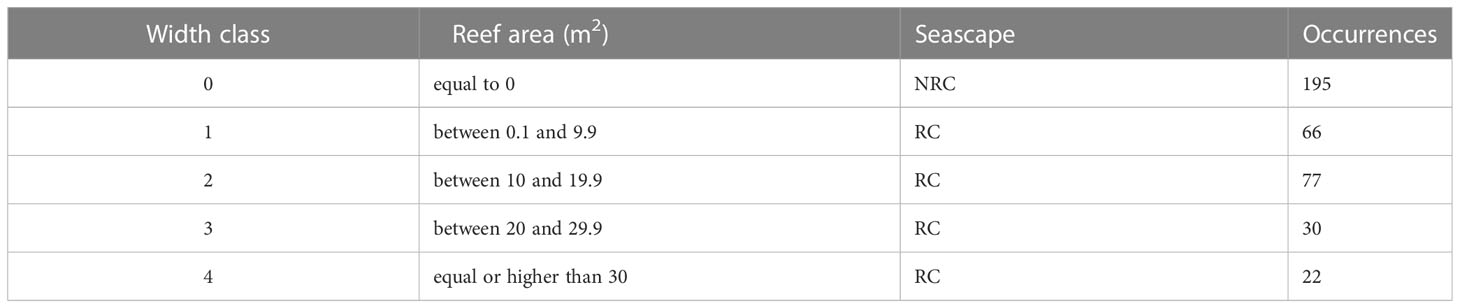
Table 2 Classification of reef width based on the reef area occupied in the rectangle samples of the RC and NRC seascapes.
For each of the reef classes from 1 to 4, 7 rectangles were randomly sampled, providing that a distance of at least 5 m was guaranteed from each other to respect independence of observations (Figure S1). The corresponding 28 sampling rectangles from the NRC scenario were then selected for the 0 width class, reaching an overall sample size of 56. To test the effect of the “presence of vermetid reefs” factor on the “total edge” variable, the values in the RC and NRC scenarios were paired by sampling rectangle and their difference was tested using a paired statistical test. To choose the appropriate statistical method, the distribution of the total edge data was determined using the Shapiro-Wilk test, which indicated a significant departure from normality (W = 0.76, p < 0.005). Based on this, the non-parametric Wilcoxon signed rank test was performed. To provide a measure of the magnitude of the factor’s effect, the “effect size” (r) was calculated as the ratio between the “z” statistic and the square root of the sample size (N). Ranging from 0 to 1, the effect size value can be considered small (0.10 - < 0.30), moderate (0.30 - < 0.5), or large (≥ 0.5) according to Cohen’s classification of effect sizes. In the presence of a significant effect and therefore rejection of the null hypothesis (i.e., no effect of vermetid reefs presence on the total edge of the coast), the effect size would express the degree to which the vermetid reefs contribute to increasing the seascape coastal complexity in terms of added total edge.
3 Results
Among the three classes in the seascape, the outer reef was the one with the highest total edge value (5,785.6 m) and, at the same time, the lowest class area (1,040 m2). Excluding the sea class, the outer reef accounted for only 3.9% of the total seascape. Opposite are the results of the edge-area metrics for the coast class, which had the lowest total edge (2,248.6), but the highest area (23,710 m2), representing 89.3% of the seascape. The platform class was, therefore, the second highest class in terms of both total edge (3,511.8 m) and area (1,803 m2, 6.8%). Accordingly, the highest edge density value was reached by the outer reef (0.12 m/m2), followed by the platform (0.07 m/m2) and the coast (0.05 m/m2) classes (Table 3). In line with these results, the value of the area-weighted perimeter-area ratio for the outer reef class was the highest (55,618), almost three times the platform value (19,544), and more than thirty times compared to the coast (1,479). Aggregation metrics showed the outer reef as the patchiest (NP = 679; PD = 14,193) as well as the most fragmented (i.e., reduced area and smaller patches) class in the seascape (SPLIT = 208,449), followed again by the platform (NP = 103; PD = 2,153; SPLIT = 9,615) and the coast (NP = 7; PD = 146; SPLIT = 4.1). Finally, the same pattern occurred in the case of the landscape shape index, which identified the outer reef as the most geometrically complex among the classes in the seascape (LSI = 44.8), followed by the platform, and the coast (Table 3). The analysis at the landscape level revealed the reef coast seascape (RC) to have higher values than the no-reef coast (NRC) in all the area-edge, shape, and aggregation metrics applied (Table 3). The natural logarithm ratios of the metrics selected for the comparison of the RC and NRC seascapes were all positive, ranging from 0.1 (CA) to 0.87 (ED) (Figure 5). In particular, compared to the NRC scenario, the RC seascape had 12% more coastal area, while the edge density, area-weighted perimeter-area ratio, and landscape shape index were 139%, 90%, and 66% higher, respectively. The Wilcoxon signed rank test revealed a statistically significant effect of the vermetid reefs presence on the coastal total edge (z = 3.9, p < 0.005), with a large effect size (r = 0.74). Total edge values in the RC seascape were significantly higher than in the NRC scenario, with the median score for the RC seascape being 21.9 compared to 8.5 for the same seascape without the vermetid reefs (Figure 6).
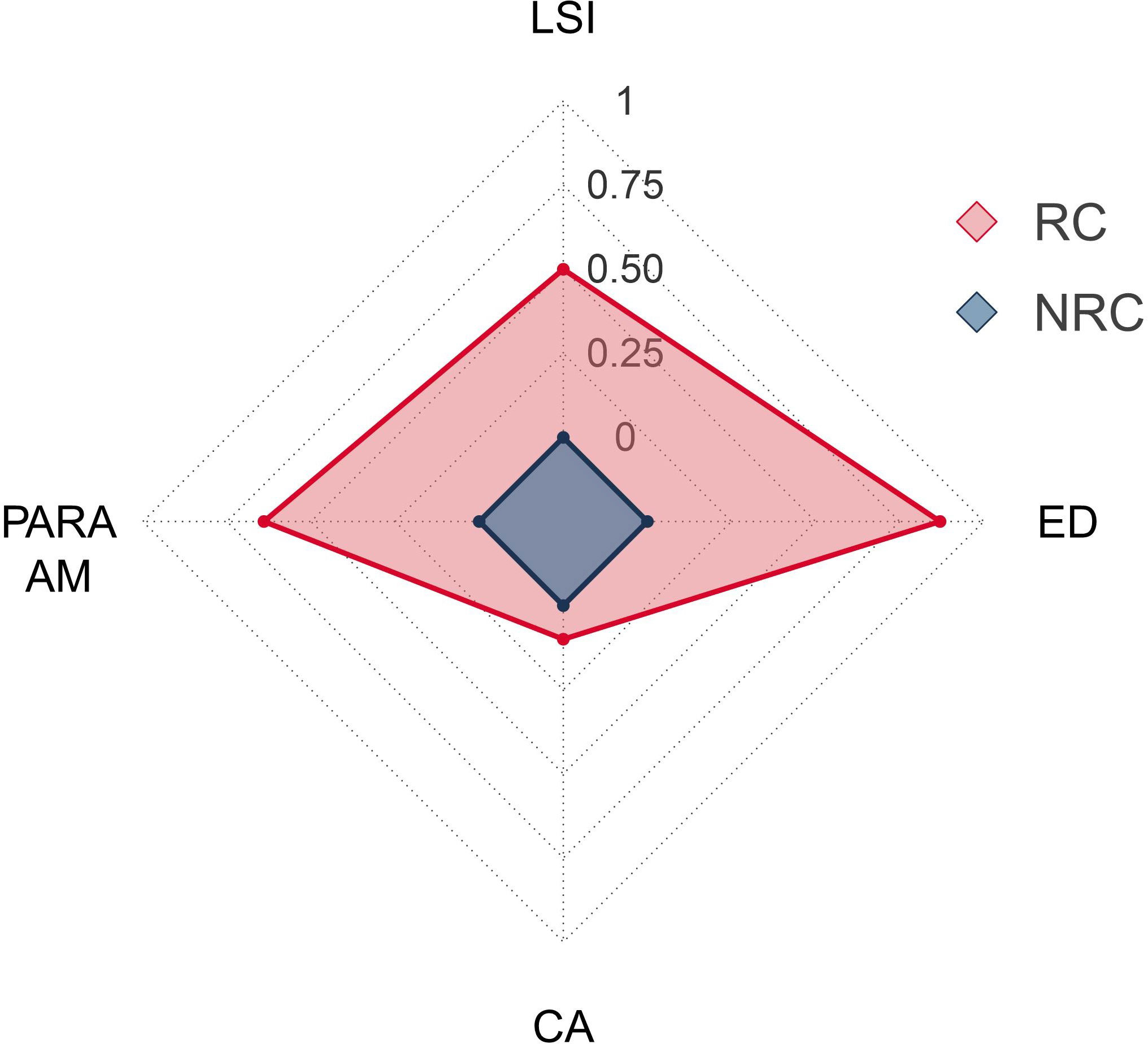
Figure 5 Contributions to the structural complexity provided by the presence of the vermetid reefs in the seascape. Values are calculated as the natural logarithm ratios of the area-weighted perimeter-area ratio (PARA AM), landscape shape index (LSI), and edge density (ED) metrics in the seascapes with (RC) and without (NRC) the vermetid reefs. The class area (CA) metric is also included as a reference.
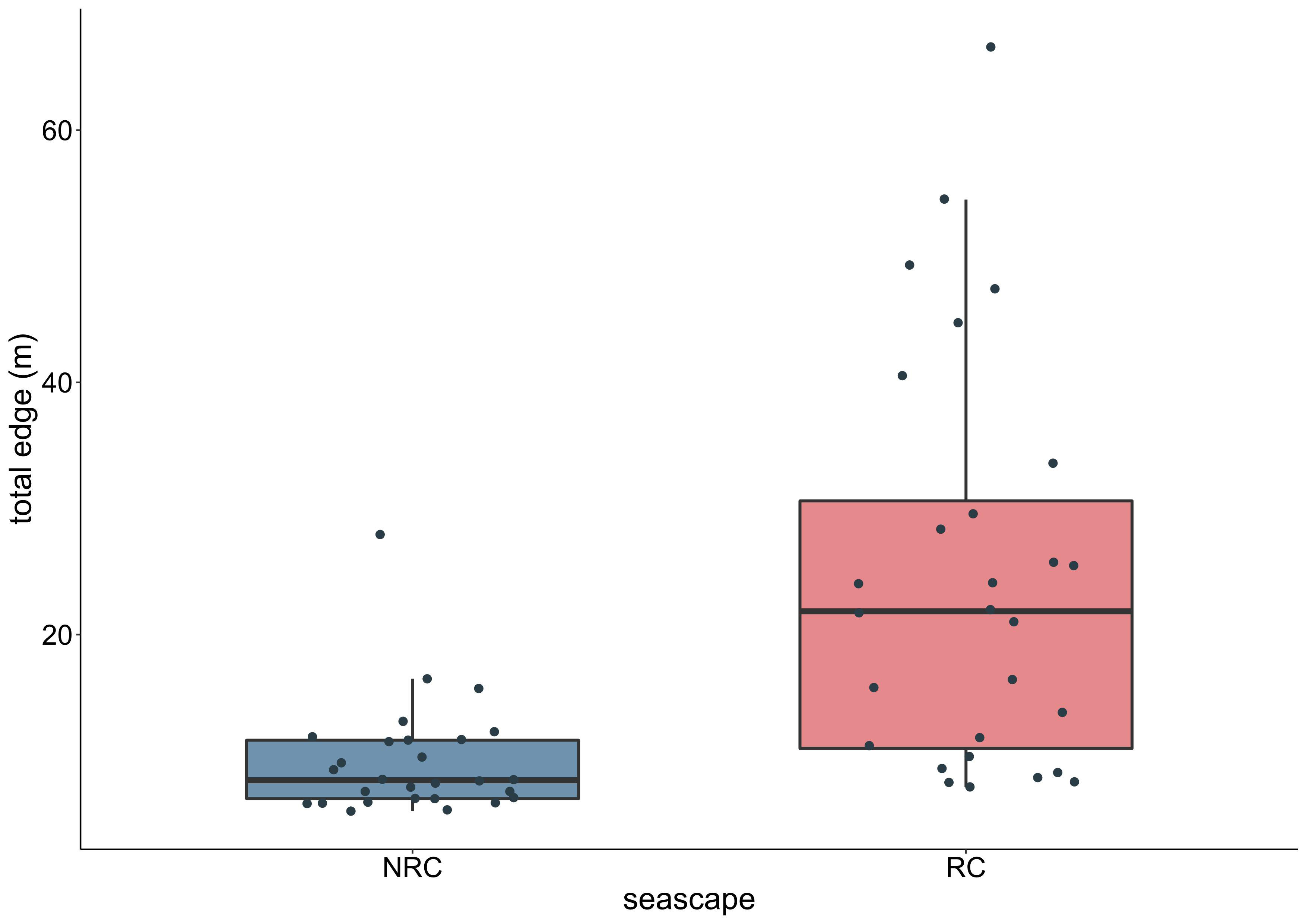
Figure 6 Total edge values of the coast samples in the seascapes without (NRC) and with (RC) the vermetid reefs.
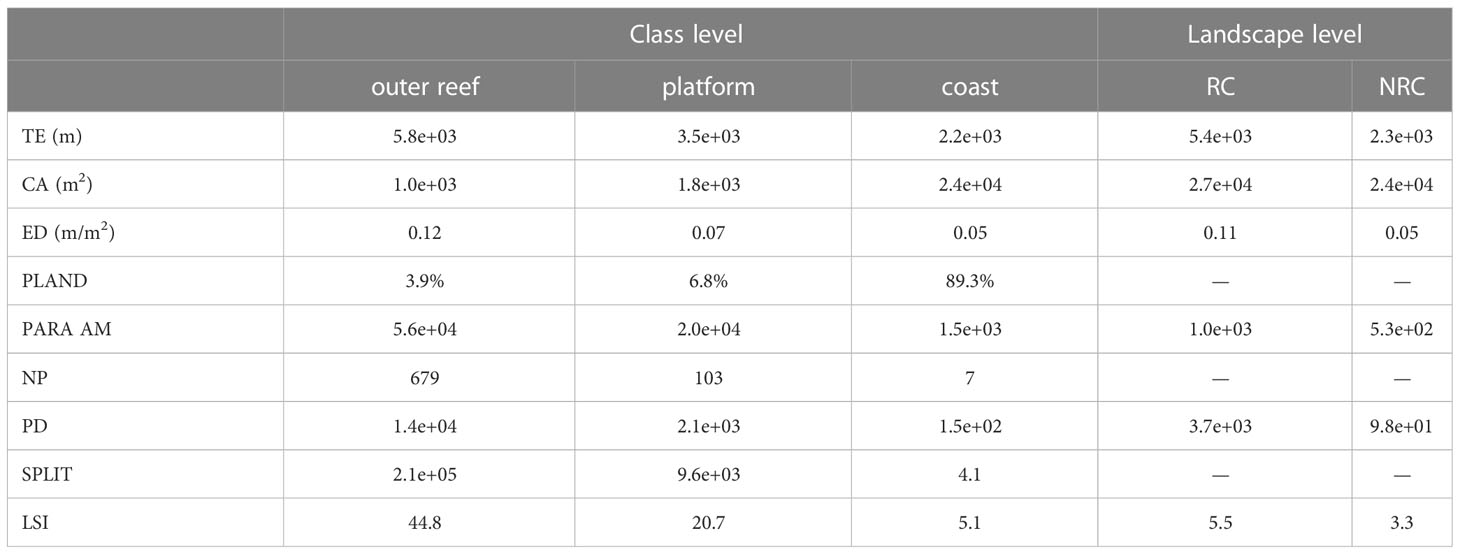
Table 3 Results of the metrics used for the characterization of the seascapes: total edge (TE); class area (CA); edge density (ED); percentage of landscape (PLAND); area-weighted perimeter-area ratio (PARA AM); number of patches (NP); patch density (PD); SPLIT; landscape shape index (LSI).
4 Discussion
The use of a UAV remote sensing sampling technique proved a useful tool to investigate the vermetid reefs from a structural perspective, as in the case of other drone-based applications to marine and coastal habitats, including bioconstructions (Collin et al., 2018; Ventura et al., 2018; Jackson-Bué et al., 2021; Tait et al., 2021; Brunier et al., 2022). Such approaches play an increasingly important role not only in detecting and monitoring changes in and impacts to marine and coastal bioconstructions, especially in the face of the threats posed by climate change, but offer also efficient tools to assess the degree to which such impacts affect the generation of many ecosystem services depending on the structural properties of the biogenic formations.
The methodology used in this study allowed a cost-efficient acquisition of high-resolution spatial data, suitable for the analysis of the role played by the vermetid reefs in modifying the structure of the coastal seascape. The visual identification and manual digitization of the seascape classes resulted in a low-cost and time saving method for the classification of a small-scale seascape characterized by a low number of visually discernible classes. If future studies were to be conducted, for instance, on the algal communities living on the vermetid reefs, different techniques would have to be implemented (e.g., Tait et al., 2021). Spectral information and classification algorithms (e.g., support vector machines (SVM), maximum likelihood) would be required to accurately and efficiently perform image classification, especially in the case of highly diverse seascapes with higher number of classes. These automated approaches would require specific training procedures to reach high levels of accuracy, potentially presenting limitations in their applications in the case of an environment characterized by spectral complexity (Brunier et al., 2022).
The analysis of the vermetid reefscape at the class level showed a clear pattern of increasing edge density, perimeter-area ratio of patches, and patchiness from the coast seaward. These features underpin a higher structural complexity of the vermetid reefs and its two constituting classes, the platform and the outer reef. The latter in particular accounts for only about 4% of the seascape surface, which makes it the least represented class, and is configured as a discontinuous, fragmented, and thin stripe between the platform and the sea. Compared to the platform, the outer edge has a six times higher patch density and a more than twenty times higher splitting index, a metric whose value increases as the class area decreases and is subdivided into smaller patches. The disaggregation of the outer reef was also captured by the high value of the landscape shape index that, together with the area-weighted perimeter-area ratio, indicated the outer reef as the most geometrically complex class overall.
The fragmented nature of the outer reef is a peculiar feature of this class of the vermetid reefscape, whose scattered structure results from the interaction of different factors such as hydrodynamics, type of rocky substrate, and reef growth (Safriel, 1966; Antonioli et al., 1999). Anthropogenic stressors acting on this already but naturally fragmented class could have severe impacts on it, drastically lowering the overall complexity and heterogeneity of the vermetid reef. The high perimeter-area ratio makes this class more exposed to potential threats negatively affecting the vitality of the Dendropoma individuals. Such scenario would be particularly concerning considering the functional role the outer reef plays in building new reef surface and maintaining the whole bioconstruction alive and expanding. An erosion rate not compensated by a sufficient growth of the outer reef would ultimately lead to the collapse of the entire structure as already reported for the Eastern Mediterranean (Galil, 2013).
The outcomes of the analysis performed at the landscape level were consistent to the ones at the class level, showing overall the same patterns. The presence of the bioconstruction in the RC seascape resulted in a longer coastal edge, a more complex geometric shape, and a patchier configuration of the seascape compared to the NRC scenario in which the vermetid reefs are absent. In the RC seascape, the total edge length was more than doubled compared to the NRC scenario, going from 2.3 to 5.4 km, with an increase in coastal area of about only 11%. The role played by the vermetid reefs in increasing the two-dimensional structural complexity of the seascape stems from their high total edge value per unit area, which positively affects metrics based on a length-area ratio such as perimeter-area ratio, edge density, and landscape shape index.
The comparison of the two seascape scenarios through the logarithmic ratios produced positive values indeed, providing a measure of the degree to which the vermetid reefs contribute to the overall structural complexity of the coastal seascape. The logarithmic responses of edge density, perimeter to area ratio, and landscape shape index were from five to almost nine times higher compared to the area increase. These results once again stress the important role played by the vermetid reef formation in shaping the coastal structure, pointing to features other than just the surface of the reef. The additional area provided by the platform in the intertidal zone is indeed pivotal for the ecological role played by the vermetid reefs, offering a diversified substrate made of crests and cuvettes with peculiar microhabitats, which allow the settlement of specific reef communities and the support of high biodiversity levels. Even though the link between platform area and the harboured biodiversity is acknowledged in the literature as the main emergent outcome of the presence of the vermetid reefs, our results showed that the additional area is not their main contribution to the coastal seascape structure. The relatively low coastal area increase (0.1 times) in the RC seascape entailed a 1.4-fold higher coastal total edge, resulting in a seascape characterized by a higher edge density value (Figure 6). The higher values of the perimeter to area ratio and the landscape shape index resulted also from differences in the shape of the vermetid reefscape. The perimeter to area ratio increases as a landscape has more patches with irregular perimeter, while high values of the landscape shape index result from landscapes deviating from a circular shape, with an increase in the amount of internal edges. The two metrics conveyed therefore additional information compared to the edge density, showing that the presence of the vermetid reefs underpinned also higher levels of shape complexity. The results of the Wilcoxon signed rank test confirmed the difference between the two seascapes, showing a statistically significant effect of the presence of the vermetid reefs on the coastal total edge. Moreover, the large effect size (r = 0.74) was consistent with the logarithmic ratio results, showing the high degree to which the vermetid reefs contribute to shaping the coastal seascape.
The loss of the vermetid reefscape as a result of anthropogenic fragmentation and degradation, or even the potential inundation of the reefs according to the sea level rise projections for the next decades (Cooley et al., 2022), would lead to a significantly simpler coastline shape, with a likely less degree of heterogeneity provided to the intertidal zone. Considering the ratio between the current reef growth rate of 0.19 mm/year (Sisma-Ventura et al., 2020) and the sea level rise rate of 1.4 mm/year estimated for the North-Western Sicily (Lo Presti et al., 2022), a scenario of complete inundation of the vermetid reefs is expected to occur between 2060 and 2080. Such changes would increase the competition for space and resources and ultimately lead to lower biodiversity levels (Rilov et al., 2021). In addition, the degradation of the biophysical structures and the ecological functions provided by the vermetid reefscape would involve the loss of many valuable ecosystem services. For instance, simpler, smaller, and more disaggregated patches of vermetid reefs would be less capable of preventing coastal erosion and regulating sediment transport, or acting as seawalls or breakwaters, protecting the coast from currents, waves, and extreme events (Milazzo et al., 2016). Finally, the loss of the outer reef and its living Dendropoma individuals would impair reef growth and prevent their CO2 sequestration from the atmosphere to build new carbonate structures.
The characterization of marine bioconstructions through their structural properties has been increasing in the recent literature, laying the foundations for the investigation of the links between structure and biodiversity, functions, and services in the marine realm. The seascape approach together with the metrics inherited from the landscape ecology proved a useful set of tools to investigate the role played by the vermetid reefs in shaping the coastal seascape structure, potentially paving the way to applications on other marine bioconstructions.
In conclusion, this paper showed the value of the vermetid reefscape from a structural perspective, pointing to the complexity that would be irreversibly lost at a seascape scale due to the anthropogenic pressures the vermetid reefs will continue facing for the next decades. The study provides a reference for future monitoring and impact assessments on the structure and status of the investigated seascape, representing also a starting point for environmental accounting applications. Future studies on the vermetid reefscape will need to focus on the relationship between the structural complexity and the composition of species assemblages colonizing the reef, investigating the effects of structural changes on the supported biodiversity at different scales. Such investigations would contribute to improving the knowledge on this neglected bioconstruction and its important role in shaping Mediterranean seascapes.
Data availability statement
The raw data supporting the conclusions of this article will be made available by the authors, without undue reservation.
Author contributions
FP: Conceptualization, Methodology, Software, Formal Analysis, Investigation, Data Curation, Writing – Original Draft, Visualization. RC: Conceptualization, Writing – Review & Editing, Supervision. All authors contributed to the article and approved the submitted version.
Acknowledgments
We would like to thank C. Fazio for her contribution to the digitization of the seascape.
Conflict of interest
The authors declare that the research was conducted in the absence of any commercial or financial relationships that could be construed as a potential conflict of interest.
Publisher’s note
All claims expressed in this article are solely those of the authors and do not necessarily represent those of their affiliated organizations, or those of the publisher, the editors and the reviewers. Any product that may be evaluated in this article, or claim that may be made by its manufacturer, is not guaranteed or endorsed by the publisher.
Supplementary material
The Supplementary Material for this article can be found online at: https://www.frontiersin.org/articles/10.3389/fmars.2023.1134385/full#supplementary-material
Abbreviations
CA, Class Area; ED, Edge Density; LSI, Landscape Shape Index; NP, Number of Patches; NRC, No-Reef Coast; PARA AM, Area-Weighted Perimeter-Area Ratio; PD, Patch Density; PLAND, Percentage of Landscape; RC, Reef Coast; SPLIT, Splitting Index; TE, Total Edge; VRS, Vermetid Reef Seascape.
References
Abadie A., Pace M., Gobert S., Borg J. A. (2018). Seascape ecology in Posidonia oceanica seagrass meadows: linking structure and ecological processes for management. Ecol. Indic. 87, 1–13. doi: 10.1016/j.ecolind.2017.12.029
Alessi C., Giomi F., Furnari F., Sarà G., Chemello R., Milazzo M. (2019). Ocean acidification and elevated temperature negatively affect recruitment, oxygen consumption and calcification of the reef-building Dendropoma cristatum early life stages: evidence from a manipulative field study. Sci. Total. Environ. 693, 1–10. doi: 10.1016/j.scitotenv.2019.07.282
Antonioli F., Chemello R., Improta S., Riggio S. (1999). Dendropoma Lower intertidal reef formations and their palaeoclimatological significance (NW Sicily). Mar. Geol. 161, 155–170. doi: 10.1016/S0025-3227(99)00038-9
Badreddine A., Milazzo M., Abboud-Abi Saab M., Bitar G., Mangialajo L. (2019). Threatened biogenic formations of the Mediterranean: current status and assessment of the vermetid reefs along the Lebanese coastline (Levant basin). Ocean. Coast. Manage. 169, 137–146. doi: 10.1016/j.ocecoaman.2018.12.019
Barbier E. B. (2012). Progress and challenges in valuing coastal and marine ecosystem services. Rev. Environ. Econ. Policy 6, 1–19. doi: 10.1093/reep/rer017
Barbier E. B. (2017). Marine ecosystem services. Curr. Biol. 27, R507–R510. doi: 10.1016/j.cub.2017.03.020
Bell S. S., Furman B. T. (2017). Seascapes are landscapes after all; comment on Manderson, (2016): seascapes are not landscapes: an analysis performed using Bernhard riemann’s rules. ICES Journal of Marine Science 73, 1831–1838. ICES. J. Mar. Sci. 74 (8), 2276–2279. doi: 10.1093/icesjms/fsx070
Bisanti L., Visconti G., Scotti G., Chemello R. (2022). Signals of loss: local collapse of neglected vermetid reefs in the western Mediterranean Sea. Mar. pollut. Bull. 185, 114383. doi: 10.1016/j.marpolbul.2022.114383
Boström C., Pittman S. J., Simenstad C., Kneib R. T. (2011). Seascape ecology of coastal biogenic habitats: advances, gaps, and challenges. Mar. Ecol. Prog. Ser. 427, 191–217. doi: 10.3354/meps09051
Bracchi V. A., Basso D., Marchese F., Corselli C., Savini A. (2017). Coralligenous morphotypes on subhorizontal substrate: a new categorization. Cont. Shelf. Res. 144, 10–20. doi: 10.1016/j.csr.2017.06.005
Brown C. J., Smith S. J., Lawton P., Anderson J. T. (2011). Benthic habitat mapping: A review of progress towards improved understanding of the spatial ecology of the seafloor using acoustic techniques. Estuar. Coast. Shelf Sci. 92, 502–520. doi: 10.1016/j.ecss.2011.02.007
Brunier G., Oiry S., Gruet Y., F. Dubois S., Barillé L. (2022). Topographic analysis of intertidal polychaete reefs (Sabellaria alveolata) at a very high spatial resolution. Remote Sens. 14, 307. doi: 10.3390/rs14020307
Burns J. H. R., Fukunaga A., Pascoe K. H., Runyan A., Craig B. K., Talbot J., et al. (2019). “3D habitat complexity of coral reefs in the northwestern Hawaiian islands is driven by coral assemblage structure,” in The international archives of the photogrammetry, remote sensing and spatial information sciences XLII-2/W10, 61–67. doi: 10.5194/isprs-archives-XLII-2-W10-61-2019
Calvo M., Templado J., Oliverio M., MacHordom A. (2009). Hidden Mediterranean biodiversity: molecular evidence for a cryptic species complex within the reef-building vermetid gastropod Dendropoma petraeum (Mollusca: caenogastropoda). Biol. J. Linn. Soc Lond. 96, 898–912. doi: 10.1111/j.1095-8312.2008.01167.x
Carlot J., Vousdoukas M., Rovere A., Karambas T., Lenihan H. S., Kayal M., et al. (2023). Coral reef structural complexity loss exposes coastlines to waves. Sci. Rep. 13, 1683. doi: 10.1038/s41598-023-28945-x
Chemello R., Silenzi S. (2011). Vermetid reefs in the Mediterranean Sea as archives of sea-level and surface temperature changes. Chem. Ecol. 27, 121–127. doi: 10.1080/02757540.2011.554405
Collin A., Dubois S., Ramambason C., Etienne S. (2018). Very high-resolution mapping of emerging biogenic reefs using airborne optical imagery and neural network: the honeycomb worm (Sabellaria alveolata) case study. Int. J. Remote Sens. 39, 5660–5675. doi: 10.1080/01431161.2018.1484964
Cooley S., Schoeman D., Bopp L., Boyd P., Donner S., Ghebrehiwet D. Y., et al. (2022). “Oceans and coastal ecosystems and their services,” in Climate change 2022: impacts, adaptation and vulnerability. contribution of working group II to the sixth assessment report of the intergovernmental panel on climate change. Eds. Pörtner H. O., Roberts D. C., Tignor M., Poloczanska E. S., Mintenbeck K., Alegría A., et al (Cambridge, UK and New York, NY, USA: Cambridge University Press), 379–550. doi: 10.1017/9781009325844.005
Cushman S. A., McGarigal K., Neel M. C. (2008). Parsimony in landscape metrics: strength, universality, and consistency. Ecol. Indic. 8, 691–703. doi: 10.1016/j.ecolind.2007.12.002
Donnarumma L., D’Argenio A., Sandulli R., Russo G. F., Chemello R. (2021). Unmanned aerial vehicle technology to assess the state of threatened biogenic formations: the vermetid reefs of mediterranean intertidal rocky coasts. Estuar. Coast. Shelf. Sci. 251. doi: 10.1016/j.ecss.2021.107228
Fonseca M. S., Whitfield P. E., Kelly N. M., Bell S. S. (2002). Modeling seagrass landscape pattern and associated ecological attributes. Ecol. Appl. 12, 218–230. doi: 10.1890/1051-0761(2002)012[0218:MSLPAA]2.0.CO;2
Furman B. T., Jackson L. J., Bricker E., Peterson B. J. (2015). Sexual recruitment in Zostera marina: a patch to landscape-scale investigation. Limnol. Oceanogr. 60, 584–599. doi: 10.1002/lno.10043
Galil B. S. (2013). Going going gone: the loss of a reef building gastropod (Mollusca: caenogastropoda: vermetidae) in the southeast Mediterranean Sea. Zool. Middle. East. 59 (2), 179–182. doi: 10.1080/09397140.2013.810885
Garrabou J., Riera J., Zabala M. (1998). Landscape pattern indices applied to mediterranean subtidal rocky benthic communities. Landsc. Ecol. 13, 225–247. doi: 10.1023/A:1007952701795
Gatti G., Montefalcone M., Rovere A., Parravicini V., Morri C., Albertelli G., et al. (2012). Seafloor integrity down the harbor waterfront: the coralligenous shoals off vado ligure (NW Mediterranean). Adv. Oceanogr. Limnol. 3, 51–67. doi: 10.1080/19475721.2012.671190
Goren M., Galil B. S. (2001). Fish biodiversity in the vermetid reef of shiqmona (Israel). Mar. Ecol. 22, 369–378. doi: 10.1046/j.1439-0485.2001.01750.x
Ingrosso G., Abbiati M., Badalamenti F., Bavestrello G., Belmonte G., Cannas R., et al. (2018). Mediterranean bioconstructions along the Italian coast . Adv. Mar. Biol. (Elsevier), 61–136. doi: 10.1016/bs.amb.2018.05.001
Jackson-Bué T., Williams G. J., Walker-Springett G., Rowlands S. J., Davies A. J. (2021). Three-dimensional mapping reveals scale-dependent dynamics in biogenic reef habitat structure. Remote Sen. Ecol. Conserv. 7, 621–637. doi: 10.1002/rse2.213
Laborel J. (1987). Marine biogenic constructions in the mediterranean. a review. Sci. Rep. Port-Cros. Natl. Park. 13, 97–126.
Lo Presti V., Antonioli F., Casalbore D., Chiocci F. L., Lanza S., Sulli A., et al. (2022). Geohazard assessment of the north-eastern Sicily continental margin (SW mediterranean): coastal erosion, sea-level rise and retrogressive canyon head dynamics. Mar. Geophys. Res. 43 (1), 1–18. doi: 10.1007/s11001-021-09463-9
McCoy E. D., Bell S. S. (1991). “Habitat structure: the evolution and diversification of a complex topic,” in Habitat structure. Eds. Bell S. S., McCoy E. D., Mushinsky H. R. (Dordrecht: Springer Netherlands), 3–27. doi: 10.1007/978-94-011-3076-9_1
McGarigal K. (2002). “Landscape pattern metrics,” in Encyclopedia of environmetrics. Eds. El-Shaarawi A. H., Piegorsch W. W. (Chichester, England: John Wiley & Sons), 1135–1142.
McGarigal K., Marks B. J. (1995). “FRAGSTATS: spatial pattern analysis program for quantifying landscape structure,” in General technical report PNW-GTR-351 (Portland, OR: U.S. Department of Agriculture, Forest Service, Pacific Northwest Research Station). doi: 10.2737/PNW-GTR-351
Milazzo M., Fine M., Claudia E., Marca L., Chemello R. (2016). Marine animal forests. Eds. Rossi S., Bramanti L., Gori A., Orejas C. (Cham: Springer International Publishing). doi: 10.1007/978-3-319-17001-5
Molinier R., Picard J. (1953). Notes biologiques à propos d’un voyage d’étude sur les côtes de sicile. Ann. Inst. Océanogr. Monaco. 28, 163–188.
O’Neill R. V., Krummel J. R., Gardner R. H., Sugihara G., Jackson B., DeAngelis D. L., et al. (1988). Indices of landscape pattern. Landsc. Ecol. 1, 153–162. doi: 10.1007/BF00162741
Pace M., Borg J. A., Galdies C., Malhotra A. (2017). Influence of wave climate on architecture and landscape characteristics of Posidonia oceanica meadows. Mar. Ecol. 38, 1–14. doi: 10.1111/maec.12387
Pagès J. F., Gera A., Romero A., Alcoverro T. (2014). Matrix composition and patch edges influence plant–herbivore interactions in marine landscapes. Funct. Ecol. 28, 1440–1448. doi: 10.1111/1365-2435.12286
Parnell P. E. (2015). The effects of seascape pattern on algal patch structure, sea urchin barrens, and ecological processes. J. Exp. Mar. Biol. Ecol. 465, 64–76. doi: 10.1016/j.jembe.2015.01.010
Pascoe K. H., Fukunaga A., Kosaki R. K., Burns J. H. R. (2021). 3D assessment of a coral reef at Lalo atoll reveals varying responses of habitat metrics following a catastrophic hurricane. Sci. Rep. 11, 12050. doi: 10.1038/s41598-021-91509-4
Picone F., Sottile G., Fazio C., Chemello R. (2022). The neglected status of the vermetid reefs in the Mediterranean Sea: a systematic map. Ecol. Indic. 143, 109358. doi: 10.1016/j.ecolind.2022.109358
Pittman S. J., Brown K. A. (2011). Multi-scale approach for predicting fish species distributions across coral reef seascapes. PloS One 6, e20583. doi: 10.1371/journal.pone.0020583
Pittman S. J., Lepczyk C. A., Wedding L. M., Parrain C. (2018). “Advancing a holistic systems approach in applied seascape ecology,” in Seascape ecology. Ed. Pittman S. J. (Hoboken, NJ: Wiley & Sons), 367–389.
Pittman S. J., McAlpine C. A., Pittman K. M. (2004). Linking fish and prawns to their environment: a hierarchical landscape approach. Mar. Ecol. Prog. Ser. 283, 233–254. doi: 10.3354/meps283233
Pittman S., Yates K., Bouchet P., Alvarez-Berastegui D., Andréfouët S., Bell S., et al. (2021). Seascape ecology: identifying research priorities for an emerging ocean sustainability science. Mar. Ecol. Prog. Ser. 663, 1–29. doi: 10.3354/meps13661
Price D. M., Robert K., Callaway A., Lo lacono C., Hall R. A., Huvenne V. A. I. (2019). Using 3D photogrammetry from ROV video to quantify cold-water coral reef structural complexity and investigate its influence on biodiversity and community assemblage. Coral. Reefs. 38, 1007–1021. doi: 10.1007/s00338-019-01827-3
Proudfoot B., Devillers R., Brown C. J. (2020). Integrating fine-scale seafloor mapping and spatial pattern metrics into marine conservation prioritization. Aquat. Conserv.: Mar. Freshw. Ecosyst. 30, 1613–1625. doi: 10.1002/aqc.3360
Rilov G., David N., Guy-Haim T., Golomb D., Arav R., Filin S. (2021). Sea Level rise can severely reduce biodiversity and community net production on rocky shores. Sci. Total. Environ. 791, 148377. doi: 10.1016/j.scitotenv.2021.148377
Robbins B. D., Bell S. S. (1994). Seagrass landscapes: a terrestrial approach to the marine subtidal environment. Trends Ecol. Evol. 9, 301–304. doi: 10.1016/0169-5347(94)90041-8
Rodil I. F., Lohrer A. M., Attard K. M., Hewitt J. E., Thrush S. F., Norkko A. (2021). Macrofauna communities across a seascape of seagrass meadows: environmental drivers, biodiversity patterns and conservation implications. Biodivers. Conserv. 30, 3023–3043. doi: 10.1007/s10531-021-02234-3
Safriel U. N. (1966). Recent vermetid formation on the Mediterranean shore of Israel. Proc. Malacol. Soc Lond. 37, 27–34.
Safriel U. N., Ben-Eliahu M. N. (1991). “The influence of habitat structure and environmental stability on the species diversity of polychaetes in vermetid reefs,” in Habitat structure. population and community biology series. Eds. Bell S. S., McCoy D. E., Mushinsy H. R. (Dordrecht: Springer Netherlands), 349–369. doi: 10.1007/978-94-011-3076-9_17
Sebens K. P. (1991). Habitat structure and community dynamics in marine benthic systems. Habitat Struct. 211–234. doi: 10.1007/978-94-011-3076-9_11
Sini M., Garrabou J., Trygonis V., Koutsoubas D. (2019). Coralligenous formations dominated by Eunicella cavolini (Koch 1887) in the NE Mediterranean: biodiversity and structure. Mediterr. Mar. Sci. 20, 174–188. doi: 10.12681/mms.18590
Sisma-Ventura G., Antonioli F., Silenzi S., Devoti S., Montagna P., Chemello R., et al. (2020). Assessing vermetid reefs as indicators of past Sea levels in the Mediterranean. Mar. Geol. 429, 106313. doi: 10.1016/j.margeo.2020.106313
Staveley T. A. B., Perry D., Lindborg R., Gullström M. (2017). Seascape structure and complexity influence temperate seagrass fish assemblage composition. Ecography. (Cop.). 40, 936–946. doi: 10.1111/ecog.02745
Tait L. W., Orchard S., Schiel D. R. (2021). Missing the forest and the trees: utility, limits and caveats for drone imaging of coastal marine ecosystems. Remote Sens. 13, 3136. doi: 10.3390/rs13163136
Templado J., Richter A., Calvo M. (2015). Reef building Mediterranean vermetid gastropods: disentangling the Dendropoma petraeum species complex. Mediterr. Mar. Sci. 17 (1), 13–31. doi: 10.12681/mms.1333
Turner M. G. (1989). Landscape ecology: the effect of pattern on process. Annu. Rev. Ecol. Syst. 20, 171–197. doi: 10.1146/annurev.es.20.110189.001131
Turner M. G., Gardner R. H. (2015). Landscape ecology in theory and practice (New York: Springer New York). doi: 10.1007/978-1-4939-2794-4
Ventura D., Bonifazi A., Gravina M. F., Belluscio A., Ardizzone G. (2018). Mapping and classification of ecologically sensitive marine habitats using unmanned aerial vehicle (UAV) imagery and object-based image analysis (OBIA). Remote Sens. 10, 1331. doi: 10.3390/rs10091331
Ventura D., Dubois S. F., Bonifazi A., Lasinio G. J., Seminara M., Gravina M. F., et al. (2021). Integration of close-range underwater photogrammetry with inspection and mesh processing software: a novel approach for quantifying ecological dynamics of temperate biogenic reefs. Remote Sens. Ecol. Conserv. 7, 169–186. doi: 10.1002/rse2.178
Wedding L. M., Lepczyk C. A., Pittman S. J., Friedlander A. M., Jorgensen S. (2011). Quantifying seascape structure: extending terrestrial spatial pattern metrics to the marine realm. Mar. Ecol. Prog. Ser. 427, 219–232. doi: 10.3354/meps09119
Keywords: Dendropoma, bioconstruction, neglected habitat, habitat structure, seascape ecology, landscape metrics, vermetid reefscape, Mediterranean Sea
Citation: Picone F and Chemello R (2023) Seascape characterization of a Mediterranean vermetid reef: a structural complexity assessment. Front. Mar. Sci. 10:1134385. doi: 10.3389/fmars.2023.1134385
Received: 30 December 2022; Accepted: 18 April 2023;
Published: 08 May 2023.
Edited by:
Monica Montefalcone, University of Genoa, ItalyReviewed by:
Ali Badreddine, Tyre Coast Nature Reserve, LebanonDaniele Ventura, Sapienza University of Rome, Italy
Copyright © 2023 Picone and Chemello. This is an open-access article distributed under the terms of the Creative Commons Attribution License (CC BY). The use, distribution or reproduction in other forums is permitted, provided the original author(s) and the copyright owner(s) are credited and that the original publication in this journal is cited, in accordance with accepted academic practice. No use, distribution or reproduction is permitted which does not comply with these terms.
*Correspondence: Flavio Picone, flaviopicone@hotmail.com