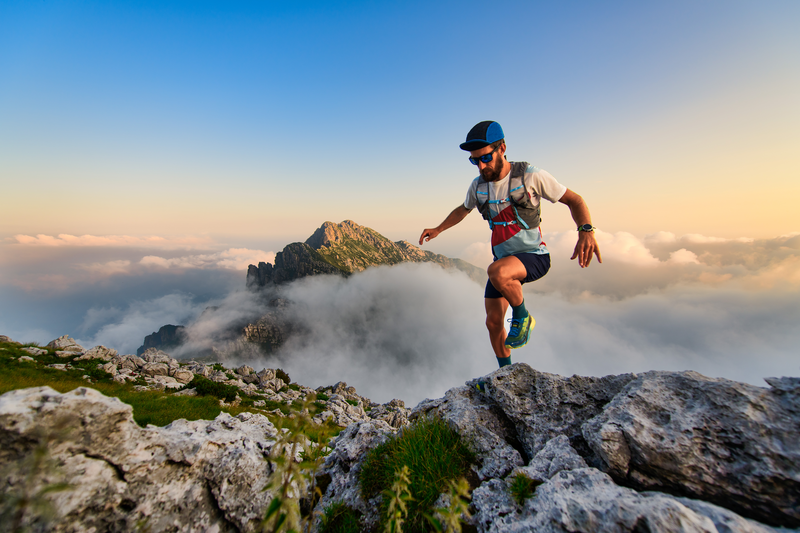
94% of researchers rate our articles as excellent or good
Learn more about the work of our research integrity team to safeguard the quality of each article we publish.
Find out more
EDITORIAL article
Front. Mar. Sci. , 20 January 2023
Sec. Deep-Sea Environments and Ecology
Volume 10 - 2023 | https://doi.org/10.3389/fmars.2023.1132451
This article is part of the Research Topic Deep-sea Sponge Ecosystems: Knowledge-based Approach Towards Sustainable Management and Conservation View all 29 articles
Editorial on the Research Topic
Deep-sea sponge ecosystems: Knowledge-based approach towards sustainable management and conservation
Sponge-dominated communities form a variety of habitats – sponge grounds, aggregations, gardens, and reefs – that are widespread throughout the world’s oceans (Maldonado et al., 2015). They are especially prevalent in upper bathyal areas within national jurisdictions (e.g. continental shelves and slopes, Klitgaard and Tendal, 2004), but also occur on seamounts, mid-ocean ridges, and canyons in areas beyond national jurisdictions (e.g. Murillo et al., 2012; Xavier et al., 2015). On account of their uniqueness, functional significance (Pham et al., 2019) and vulnerability to direct and indirect impacts from established (e.g. bottom fishing; Murillo et al., 2016) and emerging (e.g. deep-sea mining) anthropogenic activities, these habitats were listed by the Oslo-Paris Convention for the Protection of the Marine Environment of the North-East Atlantic as threatened and/or endangered (OSPAR Commission, 2008), and many are classified as Vulnerable Marine Ecosystems (VMEs – FAO, 2009). Ensuring these habitats’ long-term sustainability therefore requires a sound scientific knowledge base to be brought to the highest levels of the political and conservation agendas.
This Research Topic invited the international scientific community to address major knowledge gaps on deep-sea sponge habitats, with a particular focus on those in the North Atlantic and Arctic Ocean, resulting from the H2020 SponGES project1, but including also studies performed in the Mediterranean Sea and the Pacific Ocean. Collectively, these studies enabled:
- Strengthening the knowledge on species biology and ecology, for instance on species reproduction, growth rates, and symbioses (Busch et al., 2020; Idan et al., 2020; Koutsouveli et al., 2020; Prado et al., 2021);
- Unraveling biogeographic patterns and connectivity across spatial and temporal scales (Cárdenas, 2020; Pisera and Gerovasileiou, 2021; Ríos et al., 2021; Santín et al., 2021; Wang et al., 2021; Xavier et al., 2021);
- Enlightening the environmental drivers and enhancing the capacity to hind- and forecast changes in distribution using new modeling approaches (Culwick et al., 2020; Mitchell and Harris, 2020; Hanz et al., 2021; Liu et al., 2021; Rodríguez-Basalo et al., 2021; Samuelsen et al., 2022);
- Improving the understanding of ecological functioning and role of these ecosystems on energy transfer and biogeochemical cycling, as well as habitat provision (Bart et al., 2021; deKluijver et al., 2021; Goren et al., 2021; Maldonado et al., 2021; Manjón-Cabeza et al., 2021; Rios et al., 2021);
- Assessing the impact of anthropogenic activities and climate-driven changes, providing a better understanding of resilience and recovery to different stressors (Idan et al., 2020; Morrison et al., 2020; Wurz et al., 2021);
- Unlocking the biotechnological potential of these species and ecosystems particularly towards the development of sponge-derived tissue engineering scaffolds and new model cell systems (Hesp et al.; Dudik et al., 2021; Martins et al., 2021).
The data and knowledge produced in the course of these studies have and will continue to support the development and implementation of strategic policies and instruments towards the achievement of biodiversity and sustainability goals, core principles on the European Union Blue Growth strategy and the United Nations 2030 Agenda for Sustainable Development.
Fundamental knowledge on species biology and ecology is critical to understand their tolerances to both natural and anthropogenic stressors. Yet, aspects such as reproductive ecology, growth rates, and sponge-microbial symbioses are still poorly documented, especially in the deep sea.
Koutsouveli et al. studied the reproductive cycle and strategy of five Geodia species across the boreo-Arctic region. They found all species to be gonochoristic (separate males and females) and oviparous, and to have a similar reproductive season (from late spring to early autumn) during which a large proportion of the population (60–90%) is engaged in reproduction. The relatively large size and high lipid content found in the oocytes of these species suggest an adaptation to deep-sea and cold-water conditions, potentially allowing for a longer planktonic phase duration (and therefore dispersal) of the resulting lecitotrophic larvae. In another study, Idan et al. compared the reproductive strategy in two species - Chondrosia reniformis Nardo, 1847 and Axinella polypoides Schmidt, 1862 - found both in shallow and mesophotic habitats in the Levantine Sea. The reproductive patterns observed differed in the two habitats with presumably temperature-regulated seasonal sexual reproduction in both species in the shallow-water habitat vs year-round high fecundity sexual reproduction in A. polypoides, and low fecundity and asexual reproduction in C. reniformis in the mesophotic habitat. These observations suggest reproductive plasticity as an adaptation to different environmental conditions.
Using a novel and non-invasive approach, Prado et al. were able to estimate in-situ, the growth rate of the vase-shaped glass sponge Asconema setubalense Kent, 1870. Applying a Structure-from-Motion technique to underwater imagery collected in the same area over six years, they estimated an average annual growth rate of 2.2 cm year-1 (height) and 2.2 cm year-1 (diameter), with higher growth rates in smaller (younger) individuals, and no growth in the largest (older) individual. According to their model, A. setubalense reaches maximum size at approximately 98 years of age, thereby confirming the slow growth and high longevity of these deep-sea species.
Busch et al. examined the microbial communities in adults, embryos and recruits of two sponge species – Craniella zetlandica (Carter, 1872) and Craniella infrequens (Carter, 1876), combining 16S rRNA amplicon sequencing and advanced microscopy techniques such as transmission electron microscopy and Fluorescence in situ Hybridization. They found distinct prokaryotic communities associated with the two high microbial abundance species (and control seawater samples), largely dominated by Chloroflexi Amplicon Sequence Variants (ASVs), and with most Chloroflexi ASVs shared across the three life stages. These results indicate that the microbial consortia are likely to be vertically transmitted from parents to offspring. They also noted that a considerable proportion of core ASVs found belong to the SAR202 clade, a group thought to be involved in the degradation of recalcitrant organic matter, and therefore likely to be playing a nutritional role in these species’ holobiont.
Having a good understanding of the biogeographic and connectivity patterns of species and habitats distributions is a fundamental base for the development of area-based management tools.
Many sponge species occur associated with cold-water coral reefs (CWC). Santín et al. examined the sponge diversity in CWC provinces of the Mediterranean Sea. They found 46 species on CWC of the Catalan margin (two new to science), and at least 172 sponge species among all Mediterranean CWC provinces, therefore considered diversity hotspots at a basin scale. The biogeographic patterns observed indicate the presence of a unique Mediterranean CWC sponge fauna, distinct from the Atlantic CWC sponge fauna (despite low endemism), and suggest water masses as key drivers of present-day distribution and diversity within this enclosed basin.
Knowledge of the sponge fauna of the Orphan seamount located off the northeast coast of Newfoundland (Canada) was advanced by Ríos et al. with the description of a new species – Tedania (Tedaniopsis) rappi, and comparison with other congeneric species of the Atlantic Ocean.
Xavier et al. examined the patterns of spatial and bathymetric distribution as well as phylogenetic and biogeographic affinities of deep-sea lithistids of the Azores archipelago. Combining species occurrence data and phylogenetic reconstructions, the authors found this archipelago and its neighboring seamounts to be a lithistid hotspot in the central North Atlantic. This assemblage was characterized by mix of steno- and eurybathic species, with highest diversity in the upper bathyal (200–800 m depth, and greatest similarity and phylogenetic affinities to other areas of the northeast Atlantic and Mediterranean. On another study, Pisera and Gerovasileiou reported the occurrence of three lithistid species in shallow-water caves and upper bathyal habitats in the Aegean Sea. A particularly abundant population of Neophrissospongia endoumensis Pisera and Vacelet, 2011 with the largest individual estimated to be up to 900 years in age was found in one of the caves. Their occurrence in these special cryptic habitats is hypothesized to result from colonization from populations in deeper areas after the last glaciation.
Using three-dimensional particle tracking modelling, Wang et al. simulated the dispersal of larvae of the glass sponge Vazella pourtalesii (Schmidt, 1870), thereby estimating the connectivity of its populations throughout the northwestern Atlantic. The authors found a clear break around Cape Hatteras, with no larvae exchange between populations to the North and South of it. This suggests that the dense populations of the Canadian Scotian Shelf and those on the east coast of the US are largely isolated and therefore long-term conservation measures require an integrated regional approach.
The vast majority of sponges possess a biosilica-based skeleton, producing highly diverse skeletal structures (spicules) that constitute the basis of sponge taxonomy and systematics, and can be used to reconstruct the evolutionary history of the group. An example of this is the study by Cárdenas that found the patterns micro-ornamentation of sterrasters to match extant and fossil genera within the family Geodiidae. Mapping of these characters onto an updated Geodiidae phylogenetic tree, contested once again the monophyly of the family and suggested this spicule type to have evolved independently multiple times in this group.
The environmental factors underpinning the distribution of species and habitats in the deep sea are still poorly understood. However, integration of ecological and oceanographic data using modern analytical approaches offer unparalleled opportunities for closer integration across disciplines and enhancing of predictive capacity.
Hanz et al. and Hanz et al. used a benthic lander to investigate the natural near-bottom environmental conditions in Sambro Bank (Scotian Shelf) where a dense aggregation of the glass sponge Vazella pourtalesii occurs. With an array of lander-mounted sensors, they were able to record fluctuations in temperature (8.8–12° C), dissolved oxygen (4.9–8.9 mg l-1), current speed (average 0.12 m s-1), and vertical carbon flux (100 ± 72 mg C m-2 day-1) of the benthic boundary layer on hourly-seasonal time scales. They found V. pourtalesii to be resilient to the effects high levels of sediment resuspension caused by short-term episodical storm events, and to benefit from semi-diurnal tidal currents for food supply. However, high current peaks caused the dislodgement of some sponges from their upright position, potentially impacting their health in the longer term.
Using Maximum Entropy and Generalized Additive modelling techniques, Rodríguez-Basalo et al. developed high-resolution spatial models of two glass sponges – Pheronema carpenteri (Thomson, 1869) and Asconema setubalense - in a topographically complex area of the Cantabrian Sea. They found substrate type, depth, and current velocity to be the main environmental factors driving the distribution of A. setubalense, whereas silicates, eastness, slope, and current velocity where the main factors for P. carpenteri’s distribution in the area.
Using 3D reconstructions based on high-resolution imagery collected with a ROV, Mitchell and Harris assessed fine-scale community dynamics of a deep-sea community of the Ridge seamount (Pacific Ocean) dominated by glass sponges and octocorals. Through spatial point process analyses and Bayesian network inference, they found a random distribution of dead individuals amongst the living ones, suggesting density was not an underlying cause of death. They also observed that at different spatial scales there was evidence of competition between taxa sharing the same habitat. On another study area (Labrador Sea, Northwest Atlantic), and coupling field observations with mathematical (fluid flow) modeling, Culwick et al. investigated the patterns of distribution and density of four types of sponge grounds and their linkage to near-bottom flow conditions. By simulating the flow around idealized sponge morphologies (spherical and ellipsoid) and over sponge grounds topographic models, they identified a slowed benthic boundary layer caused by the wake of individual sponges and the community as a whole. This layer is hypothesized to play an important role both on larval recruitment and food uptake, and therefore critical for the maintenance of these habitats.
New modelling approaches, also allowed to both hindcast (Samuelsen et al., 2022) and predict (Liu et al., 2021), at monthly to multi-decadal timescales, the variability of environmental factors underpinning the distribution of these habitats. Combined, the studies by Samuelsen et al., and Liu et al. greatly enhanced our capacity to predict effects of future anthropogenic and climate-driven changes at management-relevant scales.
Sponge habitats are increasingly acknowledged to play key functional roles, delivering various goods and services, from enhancing biodiversity locally to transferring of energy from lower to higher trophic levels, participating in biogeochemical cycling, and provisioning of habitat and nursery areas for many other organisms, from invertebrates to fishes. However, to date, few studies have attempted to quantify such functions and assess their relevance at the ecosystem level.
Using an experimental pulse-chase approach, Bart et al. traced the fate of carbon- and nitrogen-enriched dissolved and particulate organic matter (DOM/POM), and showed the transfer of the organic matter consumed by sponges to brittlestars, demonstrating the occurrence of a sponge-loop pathway. These results corroborate previous findings of the sponge loop in shallow-water (de Goeij et al., 2013), further highlighting the importance of this taxonomic group on the recycling of major nutrients, and sustaining of food-webs across various marine ecosystems. These findings further supported the development by de Kluijver et al. of a metabolic network model for one of the most common deep-sea habitat-forming species (Geodia barretti Bowerbank, 1858) and its microbial symbionts, with quantification of carbon, nitrogen, and oxygen fluxes. Such study found this holobiont to have a complex metabolism, consisting of aerobic and anaerobic processes, with lower (volume-specific) metabolic rates in larger individuals, a pattern characteristic of slow-growing organisms.
In another study, combining in-situ and laboratory incubations, Maldonado et al. investigated the nitrogen and phosphorus fluxes in two hexactinellid species - Vazella pourtalesii (Schmidt, 1870) and Schaudinnia rosea (Fristedt, 1887). The net fluxes of ammonium, nitrite, nitrate, and phosphate upscaled to the level of the habitat that V. pourtalesii forms on the Scotian Shelf, showed that 2.6 million kg of ammonium may be taken up annually from the bottom water by this species in the area. Such uptake is hypothesized to limit the bacterioplankton community and consequently the establishment of a more diverse sponge community feeding on it.
The role of sponges as “living hotels” was emphasized in the study by Goren et al., examining the macrofauna associated with massive sponges collected in two habitats (shallow vs mesophotic) on the Israeli Mediterranean Coast. They found over 1000 individuals representing 60 taxa across six major groups, and particularly within Polychaeta, Crustacea and Ophiuroidea, living within the sponge aquiferous system. Distinct sponge-associated communities were found in shallow and mesophotic habitats, with highest richness (and sponge diversity) in the latter, but higher densities (per sponge volume) in the former. In another study, Manjón-Cabeza et al. examined the echinoderm communities found in four types of sponge aggregations occurring in the Cantabrian Sea. They found a diverse assemblage with 42 echinoderm species (28 ophiuroids and 14 starfishes), occurring in the area. However, no evidence of association between the echinoderms and sponges was found, suggesting that other factors such as depth or substrate may be shaping the distribution of species in both groups.
To date, only a few studies have addressed the effects of direct and indirect anthropogenic pressures, such as bottom fisheries and climate change, on deep-sea sponge habitats (Murillo et al., 2016; Beazley et al., 2018; Beazley et al., 2020). Morrison et al. investigated the recovery of sponge ground communities of an Arctic seamount (Schulz Bank) four years after physical disturbance by (experimental) bottom trawling. The diversity and abundance of the epibenthic megafauna on the impacted sites were shown to be significantly lower than on control sites, suggesting that recovery to pre-impact conditions is likely to take long timeframes. In addition to physical removal as bycatch, plumes of suspended sediments during fishing operations may have detrimental effects on sponge physiology. Wurz et al. showed, using a mesocosm experiment, that the habitat-forming glass sponge Vazella pourtalesii was able to maintain normal physiological performance (respiration and clearing rates) for up to one week of natural sediment exposure (at concentrations up to 57 mg L-1). However, longer-term exposure (i.e., after 14 days) led to a decrease in bacterial clearing rates suggesting an arrest in pumping activity. Such response mechanism is thought to be energetically demanding and to compromise other physiological processes such as growth and reproduction, compromising overall sponge health, and therefore not sustainable for extended periods of time.
Sponge communities, particularly those in shallower waters have been experiencing episodical mass mortality as a result of anomalously-high water temperature events (Garrabou et al., 2009). However, under more gradual changes as those sponges have been considered “winners”, when compared to calcifying organisms such as, for instance, scleractinian corals (Bell et al., 2013; Beazley et al., 2018; Beazley et al., 2020). Through an in-situ transplantation experiment, Idan et al. showed that individuals of the sponge Agelas oroides (Schmidt, 1864) collected at mesophotic depths (100-200 m) and transplanted in a shallow habitat at 10 m depth, experienced overall mortality when water temperature had risen to over 28°C; unlike sponges in the control group that were transplanted at the same mesophotic depths where temperature never rose above 20°C. Taking into account these results they hypothesized that the 3°C increase in water temperature observed in the Israeli coast over the past 60 years to be one of the main factors leading to the disappearance of A. oroides from shallow-waters in the area.
Sponges and the sponge holobiont have long been recognized as prolific sources of marine natural products with human health applications (Calado et al., 2022), having attracted great interest from the pharmaceutical industry. In more recent years, there is also an increasing interest on sponge-derived biomaterials such as biosilica and collagen, as these present enormous potential for the development of tissue engineering scaffolds and drug-delivery applications (e.g., Kaya et al., 2021). In their study, Martins et al. characterized the morphological, mechanical, and chemical properties of biosilicas extracted from several deep-sea habitat-forming sponges that are widely distributed across the boreo-Arctic region - Geodia barretti, Geodia atlantica, Stelletta normani, Phakellia ventilabrum, and Axinella infundibuliformis. The high skeletal interconnectivity and porosity observed revealed these species as interesting biomimetic models for tissue engineering applications. Dudik et al. further explored the bioactivity and osteogenic capacity of the bioceramics of the same species. Their results showed these were non-cytotoxic, and that the biosilica of Geodia atlantica in particular promoted the deposition of calcium phosphate, therefore representing a promising avenue for bone tissue regeneration.
The latest development of a sponge cell line (Conkling et al., 2019), and the demonstrated possibility to genetically modify cultured cells using a CRISPR/Cas12a gene editing system as shown by Hesp et al., represents a major scientific breakthrough which further allows the establishment of new models to understand not only evolutionary processes but also upscale the production of bioactive compounds for clinical trials.
Designed the study: JX, SP, EK; edited the contributions: JX, SP, EK; wrote the draft of the manuscript: JX; contributed text and reviewed the manuscript: JX, SP, EK. All authors contributed to the article and approved the submitted version.
This Research Topic, and much of the research herein reported, has been performed in scope of the SponGES project, which received funding from the European Union’s Horizon 2020 research and innovation programme under grant agreement No. 679849. This document reflects only the authors’ views and the Executive Agency for Small and Medium-sized Enterprises (EASME) is not responsible for any use that may be made of the information it contains. JX research is further supported by national funds through FCT Foundation for Science and Technology within the scope of UIDB/04423/2020 and UIDP/04423/2020, and CEECIND/00577/2018.
We thank all scientists contributing to this Research Topic. A special thanks to the SponGES consortium, from principal investigators, to technicians, early career researchers and undergraduate students for their commitment in producing knowledge on so many aspects of sponge (and deep-sea) biology and ecology. We are greatly indebted to all experts that acted as additional manuscript handling editors (Daniela Zeppilli, Daphne Cuvelier, Chiara Romano, Clara Rodrigues, Cristina Gambi, Lorenzo Angeletti, Jose Angel Alvarez Perez) or as reviewers of the manuscripts herein published. We would also like to thank the editorial staff at Frontiers in Marine Sciences for the invitation and support throughout the development of this volume. We dedicate this Research Topic to our dear friend and coordinator of the SponGES project, Hans Tore Rapp, Professor at the University of Bergen (Norway), who passed away so prematurely, and before this volume was completed. This is both a result of and a tribute to his exceptional contributions to sponge and deep-sea science. You will be missed for many years to come.
The authors declare that the research was conducted in the absence of any commercial or financial relationships that could be construed as a potential conflict of interest.
All claims expressed in this article are solely those of the authors and do not necessarily represent those of their affiliated organizations, or those of the publisher, the editors and the reviewers. Any product that may be evaluated in this article, or claim that may be made by its manufacturer, is not guaranteed or endorsed by the publisher.
Beazley L., Kenchington E., Murillo F. J., Brickman D., Wang Z., Davies A. J., et al. (2020). Climate change winner in the deep sea: Predicting the impacts of climate change on the distribution of the glass sponge Vazella pourtalesii. Mar. Ecol. Prog. Ser. 657, 1–23. doi: 10.3354/meps13566
Beazley L., Wang Z., Kenchington E., Yashayaev I., Rapp H. T., Xavier J. R., et al. (2018). Predicted distribution of the glass sponge Vazella pourtalesi on the scotian shelf and its persistence in the face of climatic variability. PloS One 13 (10), e0205505. doi: 10.1371/journal.pone.0205505
Bell J. J., Davy S. K., Jones T., Taylor M. W., Webster N. S. (2013). Could some coral reefs become sponge reefs as our climate changes? Global Change Biol. 19, 2613–2624. doi: 10.1111/gcb.12212
Bowerbank J. S. (1858). On the anatomy and physiology of the spongiadae. part i. on the spicula. Philos. Trans. R. Soc. 148 (2), 279–332.
Calado R., Mamede R., Cruz S., Leal M. C. (2022). Updated trends on the biodiscovery of new marine natural products from invertebrates. Mar. Drugs 20, 389. doi: 10.3390/md20060389
Carter H. J. (1872). On two new sponges from the Antarctic Sea, and on a new species of Tethya from shetland; together with observations on the reproduction of sponges commencing from zygosis of the sponge animal. Ann. Mag. Natural Hist. 9 (54), 409–435. doi: 10.1080/00222937208696612
Carter H. J. (1876). Descriptions and figures of deep-Sea sponges and their spicules, from the Atlantic ocean, dredged up on board H.M.S.’Porcupine’, chiefly in 1869 (concluded). Ann. Mag. Natural Hist. 18 (105), 226–240. (107): 388-410;(108): 458-479.
Conkling M., Hesp K., Munroe S., Sandoval K., Martens D. E., Sipkema D., et al. (2019). Breakthrough in marine invertebrate cell culture: Sponge cells divide rapidly in improved nutrient medium. Sci. Rep. 9, 17321. doi: 10.1038/s41598-019-53643-y
Culwick T., Phillips J., Goodwin C., Rayfield E. J., Hendry K. R. (2020). Sponge density and distribution constrained by fluid forcing in the deep Sea. Front. Mar. Sci. 7. doi: 10.3389/fmars.2020.00395
de Goeij J. M., Van Oevelen D., Vermeij M. J., Osinga R., Middelburg J. J., De Goeij A. F., et al. (2013). Surviving in a marine desert: the sponge loop retains resources within coral reefs. Science 342, 108–110. doi: 10.1126/science.1241981
FAO (2009). International guidelines for the management of deep-sea fisheries in the high seas. directives internationales sur la gestion de la pêche profonde en haute mer. directrices internacionales para la ordenación de las pesquerías de aguas profundas en alta mar (Rome: Food and Agriculture Organization of the United Nations), 73.
Fristedt K. (1887). Sponges from the Atlantic and Arctic oceans and the behring Sea. Vega-Expeditionens Vetenskap. Iakttagelser (Nordenskiöld) 4, 401–471.
Garrabou J., Coma R., Bensoussan N., Bally M., Chevaldonné P., Cigliano M., et al. (2009). Mass mortality in northwestern mediterranean rocky benthic communities: effects of the 2003 heat wave. Global Change Biol. 15, 1090–1103. doi: 10.1111/j.1365-2486.2008.01823.x
Kaya M., Bilican I., Mutjaba M., Sargin I., Haskoylu M. E., Ones E. T., et al. (2021). Sponge-derived natural bioactive glass microspheres with self-assembled surface channel arrays opening into a hollow core for bone tissue and controlled drug release applications. Chem. Eng. J. 407, 126667. doi: 10.1016/j.cej.2020.126667
Kent W.S. (1870). On the ‘Hexactinellidae’ or hexradiate spiculed silicious sponges taken in the ‘Norna’ expedition off the coast of Spain and portugal. with description of new species, and revision of the order. Mon. Microsc. J. 4, 241–252.
Klitgaard A. B., Tendal O. S. (2004). Distribution and species composition of mass occurrences of large-sized sponges in the northeast Atlantic. Prog. Oceanogr. 61, 57–98. doi: 10.1016/j.pocean.2004.06.002
Maldonado M., Aguilar R., Bannister R. J., Bell J. J., Conway K. W., Dayton P. K., et al. (2015). “Sponge grounds as key marine habitats: A synthetic review of types, structure, functional roles, and conservation concerns,” in Marine animal forests. Eds. Bramanti L., Gori A., Orejas Saco del Valle C. (Cham: Springer). doi: 10.1007/978-3-319-17001-5_24-1
Murillo F. J., Muñoz P. D., Cristobo J., Rios P., González C., Kenchington E., et al. (2012). Deep-sea sponge grounds of the Flemish cap, Flemish pass and the grand banks of Newfoundland (northwest Atlantic ocean): distribution and species composition. Mar. Biol. Res. 8, 842–854. doi: 10.1080/17451000.2012.682583
Murillo F. J., Serrano A., Kenchington E., Mora J. (2016). Epibenthic assemblages of the tail of the grand bank and Flemish cap (northwest Atlantic) in relation to environmental parameters and trawling intensity. Deep Sea Res. I 109, 99–122. doi: 10.1016/j.dsr.2015.08.006
Nardo G. D. (1847). Osservazioni anatomiche sopra l’animale marino detto volgarmente rognone di mare. atti delle adunanze dell’I. r. Istituto Veneto di Sci. Lett. ed Arti. 6, 267–276.
OSPAR Commission (2008). Descriptions of habitats on the OSPAR list of threatened and/or declining species and habitats (Reference Number: 2008-07).
Pham C. K., Murillo F. J., Lirette C., Maldonado M., Colaco A., Ottaviani D., et al. (2019). Removal of deep-sea sponges by bottom trawling in the Flemish cap area: conservation, ecology and economic assessment. Sci. Rep. 9, 15843. doi: 10.1038/s41598-019-52250-1
Pisera A., Vacelet J. (2011). Lithistid sponges from submarine caves in the Mediterranean: taxonomy and affinities. Sci. Marina 75 (1), 17–40. doi: 10.3989/scsimar.2011.75n1017
Schmidt O. (1864). Supplement der Spongien des adriatischen Meeres. Enthaltend die Histologie und systematische Ergänzungen. (Wilhelm Engelmann:Leipzig) 1-4, 1–48.
Schmidt O. (1870). Grundzüge einer spongien-fauna des atlantischen gebietes Vol. iii-iv (Leipzig: Wilhelm Engelmann), 1–88.
Thomson C. W. (1869). On Holtenia, a genus of vitreous sponges. Proc. R. Soc. London 18, 32–35. doi: 10.1111/j.1365-2818.1869.tb01857.x
Keywords: Porifera, habitats - conservation, biodiversity and connectivity, ecological function, blue biotechnology, threats and impacts, management tools
Citation: Xavier JR, Pomponi SA and Kenchington EL (2023) Editorial: Deep-sea sponge ecosystems: Knowledge-based approach towards sustainable management and conservation. Front. Mar. Sci. 10:1132451. doi: 10.3389/fmars.2023.1132451
Received: 27 December 2022; Accepted: 12 January 2023;
Published: 20 January 2023.
Edited and Reviewed by:
Eva Ramirez-Llodra, REV Ocean, NorwayCopyright © 2023 Xavier, Pomponi and Kenchington. This is an open-access article distributed under the terms of the Creative Commons Attribution License (CC BY). The use, distribution or reproduction in other forums is permitted, provided the original author(s) and the copyright owner(s) are credited and that the original publication in this journal is cited, in accordance with accepted academic practice. No use, distribution or reproduction is permitted which does not comply with these terms.
*Correspondence: Joana R. Xavier, anhhdmllckBjaWltYXIudXAucHQ=
Disclaimer: All claims expressed in this article are solely those of the authors and do not necessarily represent those of their affiliated organizations, or those of the publisher, the editors and the reviewers. Any product that may be evaluated in this article or claim that may be made by its manufacturer is not guaranteed or endorsed by the publisher.
Research integrity at Frontiers
Learn more about the work of our research integrity team to safeguard the quality of each article we publish.