- 1College of Fisheries, Guangdong Ocean University, Zhanjiang, China
- 2Department of Biological Sciences, University of Toronto Scarborough, Toronto, ON, Canada
- 3South China Sea Fisheries Research Institute, Chinese Academy of Fishery Sciences, Guangzhou, China
- 4Scientific Observing and Experimental Station of South China Sea Fishery Resources and Environment, Ministry of Agriculture and Rural Affairs, Guangzhou, China
- 5South China Sea Standards and Metrology Center, State Oceanic Administration, Guangzhou, China
Due to there being a lack of suitable approaches for evaluating the effectiveness of artificial reefs, two experiments were designed to examine the feasibility of acoustic telemetry, a rapidly developing method for tracking aquatic animals. The first experiment was conducted to understand the deployment procedures of an acoustic telemetry system and determine the appropriate deployment of receivers’ spacing, while the second experiment was conducted to quantify the site fidelity and habitat use of 11 reef fish in the Fangchenggang artificial reef area in the northern South China Sea, China. The results indicated that the logistic regression model was an effective way to balance the detection probability at different distances between the range test transmitter and receiver, with above 50% detection probability within 240 m and 80% detection probability within 110 m. The residency index, as a quantification of site fidelity, was 0.85 ± 0.24. The 100% minimum convex polygon, 95% kernel utilization distribution, and 50% kernel utilization distribution, which are the indicators of habitat use, were 34,522.94 ± 35,548.95, 1,467.52 ± 1,619.05, and 236.01 ± 294.59 m2, respectively. High site fidelity and the small spatial scale of habitat use for reef fish demonstrated that artificial reefs were an effective man-made structure to attract fish. Overall, this study supports the feasibility of the acoustic telemetry system, indicating that it provides a good approach for quantifying the associations between artificial reefs and fish.
1 Introduction
Global marine biodiversity and fishery resources have continuously decreased under the constant pressure of overfishing, water pollution, and climate change in recent years (Jackson et al., 2001; Worm et al., 2006; O′Hara et al., 2021). According to statistics from the Food and Agriculture Organization (FAO), the proportion of fish stocks within biologically sustainable levels decreased from 90% in 1974 to 64.6% in 2019 (Food and Agriculture Organization (FAO), 2020; FAO, 2022). Some active strategic management measures have been implemented worldwide to create more sustainable fisheries, including input-oriented control measures (such as fishing permits, closed fishing areas, and seasonal fishing restrictions) (FAO, 2003; Morison, 2004; Tang, 2011), output-oriented control measures (such as total allowable catches and fishing quotas) (Pope, 2009; Lyu et al., 2022), and technological control measures (such as marine conservation areas, marine life stocks enhancement, and artificial reefs construction) (FAO, 2011; Shen and Heino, 2014; Zhang et al., 2021). Among these measures, artificial reefs (ARs), man-made submarine structures, have the main purpose of increasing the diversity and biomass of fishery resources by providing additional favorable habitats for the organisms living in coastal waters (Sreekanth et al., 2017). Historically, Japan is the first country to research the ecological applications of ARs (OKA, 1962). Over the years, ARs have been widely deployed in coastal waters all over the world and have been studied in 56 countries (Lima et al., 2019). In China, the government invested a total of 5 billion RMB (approximately 0.75 billion USD) in marine ranching and AR construction from 2000 to 2016. As a result, more than 200 marine ranches in an area of 852.6 km2 had been built, where they had placed ARs of 60 million m3 in volume (Zhou et al., 2019). With the continuous investment in AR construction, there are increasing concerns about whether ARs can achieve the desired goals of enhancing stocks.
Evaluating the effectiveness of ARs is a challenge due to the complexity of topography in ARs. To our knowledge, methods for the effective evaluation of ARs can be divided into two categories: laboratory simulation and field monitoring. Laboratory simulation evaluates the effectiveness of ARs by observing the behavioral responses of reef fishes to the scaled-down AR models in experimental troughs (Zhou et al., 2011; Zhang et al., 2022). However, there are limitations of this ex situ approach in reflecting the actual situation of natural waters. In contrast, field monitoring includes traditional fishing gear, diving visual census, underwater video, and hydroacoustic detection (Lam, 2003; Liu et al., 2015; Yuan et al., 2021). However, these methods also have limitations. For instance, fishing gear (such as bottom trawl nets, gill nets, hooks and lines, and cage traps) have selectivity constraints for fishery targets. Bottom trawling, in particular, can only be used on the edge of an AR area and can easily cause habitat damage (Sun et al., 2020). Diving visual census and underwater video are limited due to factors such as light intensity, water depth, and turbidity (Zeng et al., 2021). Meanwhile, hydroacoustic detection may not detect benthic animals when they are in the acoustic blind zone, and it is difficult to separate the signal of animals from that of ARs (Ona and Mitson, 1996). Thus, other techniques need to be developed while implementing the approaches described above to fully understand the effectiveness of ARs.
Acoustic telemetry, also called ultrasonic telemetry, has become a popular in situ approach to studying the movement and habitat use of aquatic animals (Cooke et al., 2004; Hedger et al., 2009; Alós et al., 2012). The acoustic telemetry system is mainly composed of receivers and transmitters (Lyu et al., 2021). To obtain accurate information, the scientific deployment of the acoustic telemetry system (i.e., the receiver placement and transmitter attachment) is essential. Animals need to be individually tagged with transmitters that emit unique signals with position and environmental data (such as water temperature and depth), which are detected by the receivers placed underwater (i.e., the receiver array) (Brownscombe et al., 2020). Although numerous studies have quantified the movement of different species from migratory fishes (such as tunas and salmons) to sedentary fishes (such as rockfish and snappers) in the estuaries, bays, coral reef habitats, and open sea areas by acoustic telemetry (Welsh et al., 2012; Smith et al., 2015; Biggs and Nemeth, 2016; Block et al., 2019; Keller et al., 2020), few studies did that in AR area. Furthermore, for the AR effectiveness evaluation, most of the previous studies focused on species variation, quantity, and weight of fish assemblage in the AR area (Charbonnel et al., 2002; Cenci et al., 2011; Zeng et al., 2018). However, few studies have evaluated the effectiveness of ARs from the perspective of fish utilization in AR habitats by acoustic telemetry, meaning information regarding site fidelity and habitat use of reef fish in AR areas is limited.
The overall goal of the present study was to examine the feasibility of using acoustic telemetry to evaluate the fish attraction effectiveness of ARs. The specific objectives were to 1) understand the deployment procedures of acoustic equipment, 2) determine the appropriate receiver spacing, and 3) quantify the site fidelity and habitat use of reef fish in the Fangchenggang AR area, Beibu Gulf, northern South China Sea. The research results may enrich approaches to evaluating the effectiveness of ARs and contribute to the decision-making process of sustainable fisheries.
2 Materials and methods
2.1 Experiment I: deployment test of the acoustic telemetry system
2.2.1 Experimental design
To optimize the deployment of acoustic equipment and test the appropriate receiver spacing, a total of eight VR2Tx receivers and one range test transmitter with a 10-s fixed delay transmission interval (Vemco Ltd., Halifax, Nova Scotia, Canada) were used to deploy the acoustic telemetry system in the coastal waters with a depth averaging 12 m. The range test transmitter was moored in the front, followed by the receiver mooring every 50 m in a straight line. All of them were suspended at a depth of 7 m above the seabed (Figure 1).
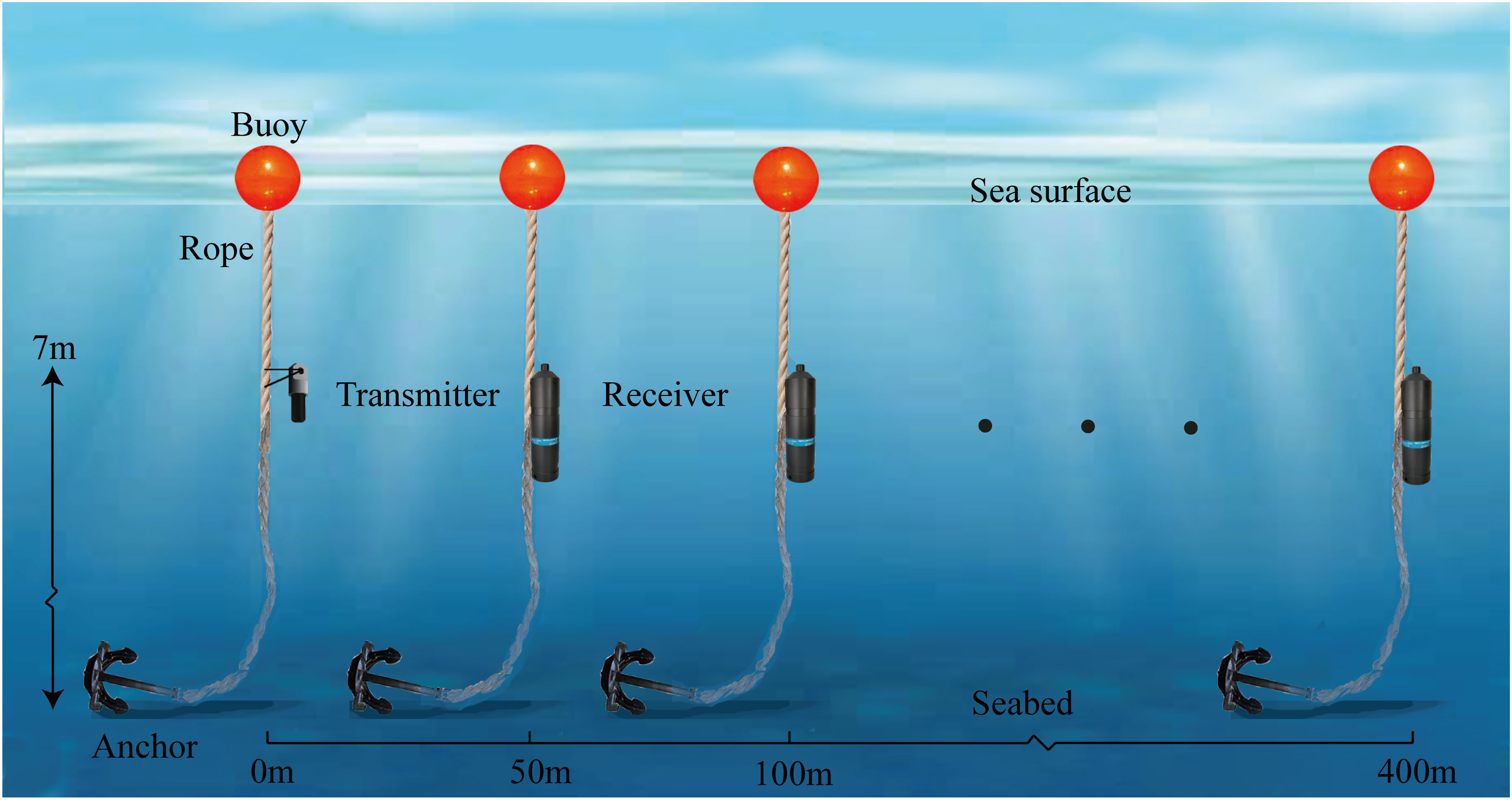
Figure 1 Schematic of range test experiment showing the deployment of the moored transmitter and receiver. The range test transmitter (not attached directly to the rope) was moored in the front (0 m), and eight receivers were moored at fixed distances from the transmitter (50, 100, 150, 200, 250, 300, 350, and 400 m).
2.2.2 Experimental procedure
Firstly, the position (longitude and latitude) of the transmitter and receiver were determined by Google Earth software (Google Inc., Mountain View, California, USA). Prior to placement, the transmitter and receiver were singly tied at a depth of 7 m from the bottom of the nylon rope using cable ties. An anchor and a buoy were tied to the two ends of the nylon rope, respectively (Figure 1). Some parameters such as the battery remaining capacity of the receiver were set in a computer installed with Vemco user environment (VUE) software (www.innovasea.com). Then, the transmitter and receiver were placed in the predetermined position with the help of a high-accuracy differential GPS instrument (Trimble Inc., Sunnyvale, California, USA). After 1 h, they were retrieved from the sea, and the experimental data in the receivers were downloaded by the VUE software.
2.2 Experiment II: fish tracking in the Fangchenggang AR area
2.2.1 Study area
The fish tracking experiment was conducted in the Fangchenggang AR area (21°25.278′–21°25.697′N, 108°12.924′–108°14.039′E) with a water depth averaging 16 m between July and September 2017. Since the first ARs were placed in 1979, about 2,190 ARs (3–6 m in height) with 123,760 m3 in volume have been placed here to date (Figure 2) (Jia et al., 2021).
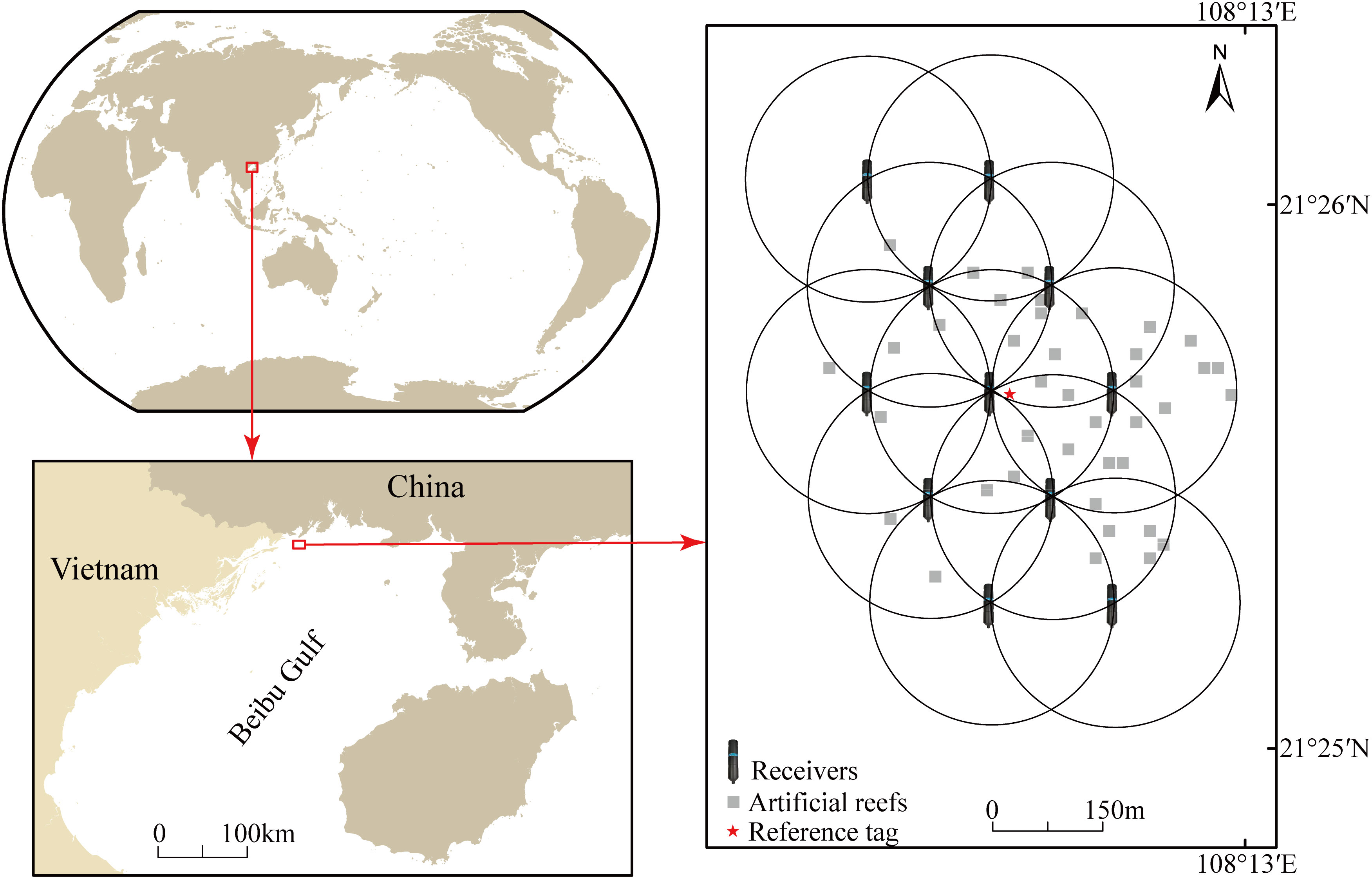
Figure 2 Map of the study area located in Beibu Gulf, northern South China Sea. The 11-receiver array was placed in polygons between July and September 2017. A reference tag was placed at the center of the array.
2.2.2 Receiver placement
Prior to receiver placement, a range test was performed in accordance with the procedures in Experiment I to know the appropriate receiver spacing in the study area; the only difference is that receivers were moored upside down. The results of the range test showed that hourly detection probability was above 80% within 150 m, so the receiver spacing was set as 150 m to guarantee high detection proportion. To cover the AR concentration zone, 11 Vemco VR2Tx receivers were moored upside down at a depth of 7 m above the seabed given the height of ARs and the bottom swimming of reef fish. The receivers were placed in polygons to increase the probability that transmissions could be detected by multiple receivers simultaneously (Figure 2). Given that biofouling might occlude signals, all the receivers were coated with an antifouling paint in advance (Heupel et al., 2008). In addition, a transmitter as the reference tag was moored at the center of the receiver array to validate the accuracy of positioning results presented by the acoustic telemetry system (Muñoz et al., 2020).
2.2.3 Fish capture, tagging, and release
The animal study was approved by the Animal Experimental Ethics Committee of Guangdong Ocean University, China. A previous study found that the released seabreams have homing behavior, as they tend to return to the site where they were captured (Mitamura et al., 2005). To test this, a total of 11 individuals of reef fish from six species, with total lengths ranging from 185 to 270 mm and weights ranging from 195 to 500 g, were captured in Fangchenggang coastal waters (not in the study AR area) using line angling by local fishermen. These fish were reared for 48 h at a conventional cage net to guarantee that they are in a normal state.
Prior to tagging, the nylon line was looped at the middle of the transmitter (Vemco V9, 69 kHz, 47 mm in length, 9 mm in diameter, 2.7 g in water, 60–120-s random delay transmission interval) and fixed by cyanoacrylate glue; then, the processed transmitters and a curved needle were immersed in a 75% ethanol solution for 5 min. After that, each individual was anesthetized by immersion in the seawater containing a dose of 100 mg/L of tricaine methane-sulfonate (MS 222) until loss of fish body equilibrium was observed (Neves et al., 2018). All the individuals were measured to the nearest millimeter in total length and weighed to the nearest gram. One end of the nylon line on the processed transmitter was threaded through the fish dorsal muscle using the curved needle and tied with another end of the line; 0.5% povidone-iodine solution was then sprayed around the wound to prevent infection. For tagging, the fish out of the water process was completed within 5 min. Then, the tagged fish were placed in a tank with fresh seawater to recover their normal behavior (Figure 3). Finally, they were held at the ballast tank of a fishing vessel and released at the center of the receiver array in the study area. The biological information and release date of 11 tagged fish are summarized in Table 1.
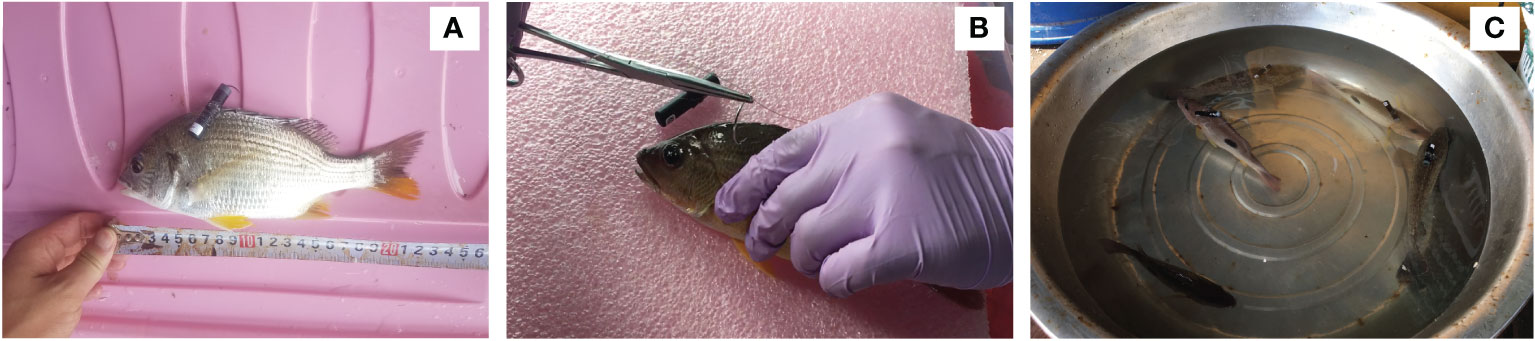
Figure 3 Digital images of fish tagging process: (A) Acanthopagrus latus tagged with a Vemco V9 acoustic transmitter, (B) a curved needle used for tagging, and (C) some tagged fish placed in the fresh seawater.
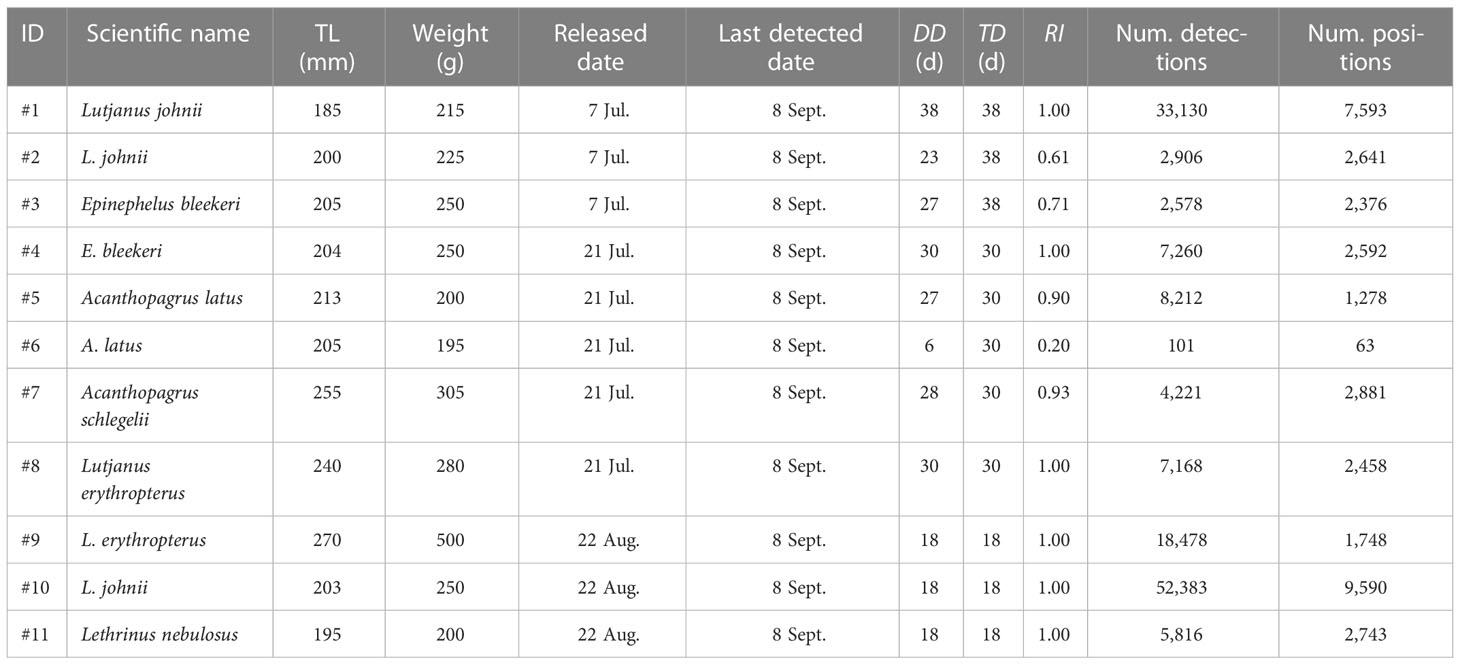
Table 1 Summary of the data for 11 reef fish tagged with acoustic transmitters between July and September 2017, including fish ID, species name, total length (TL), weight, released date, last detected date, detected days (DD), tracking days (TD), residency index (RI), number of (Num.) detections, and number of (Num.) positions.
2.3 Statistical analysis
2.3.1 Experiment I
The detection proportion (DPi, %) for each receiver is calculated as follows (Mathies et al., 2014):
where ODi is the number of observed detections for a receiver i and EDi is the number of expected detections. One-way ANOVA was conducted to test for significant differences in the detection proportion at different distances between the range test transmitter and receiver; the data were checked for normality using the Shapiro–Wilk test and for homogeneity of variances with Levene’s test, which were performed in the SPSS 22.0 software (IBM Inc., Armonk, New York, USA). p < 0.05 was considered statistically significant.
Considering that the transmission detected or not detected was the binary response variable, a logistic regression model was fitted to the data to predict the detection probability at different distances (i.e., the appropriate receiver spacing) by using the “glm” function in the R version 4.2.0 (Kessel et al., 2014; Swadling et al., 2020). The model significance was examined by the Hosmer and Lemeshow goodness-of-fit test, and p > 0.05 means it provides a satisfactory fit to the data (Hosmer and Lemeshow, 2000).
2.3.2 Experiment II
The presence and absence of fish in the study area could be detected by an individual receiver, while the fish position was detected by three or more receivers simultaneously and calculated based on the time difference of transmission arrival at the receivers (Williams-Grove and Szedlmayer, 2017). We had originally intended to download the data at the end of the experiment. However, due to the typhoon, receivers were retrieved twice during the experiment, and tracking dates were 7 to 14 July, 21 July to 1 August, and 2 August to 8 September 2017. A residency index (RIj) was used to quantify the site fidelity of the reef fish to the AR area, which was defined as follows (March et al., 2010; Alós et al., 2011):
where DDj is the number of detected days for a tagged fish j and TDj is the tracking days between the released date and the last detected date within the receiver array. RIj varies from 0 (lowest, completely absent) to 1 (highest, completely present) (Moxham et al., 2019).
The home range was used to characterize the habitat use. Hence, three measures of the home range were estimated for the tagged fish: 100% minimum convex polygon (100% MCP, i.e., based on 100% of the observed fish positions), 95% kernel utilization distribution (95% KUD, i.e., general activity area), and 50% KUD (i.e., core activity area) (Alós et al., 2012), which were performed using the Arctoolbox in Arcgis 10.8 software (ESRI Inc., Redlands, California, USA).
3 Results
3.1 Receiver detection range
The detection proportion declined significantly with increasing distance between the range test transmitter and receiver (one-way ANOVA, p < 0.05). The mean detection proportion per 20 min (mean ± SD) varied from a high of 81.1% ± 0.7% at 50 m to a low of 19.2% ± 4.2% at 400 m. The largest incremental decrease in detection proportion was observed between 250 and 300 m. The logistic regression model provides a satisfactory fit to the data (Hosmer and Lemeshow test, p > 0.05), which indicated that 50% detection probability was achieved at approximately 240 m and 80% detection probability was achieved at approximately 110 m (Figure 4).
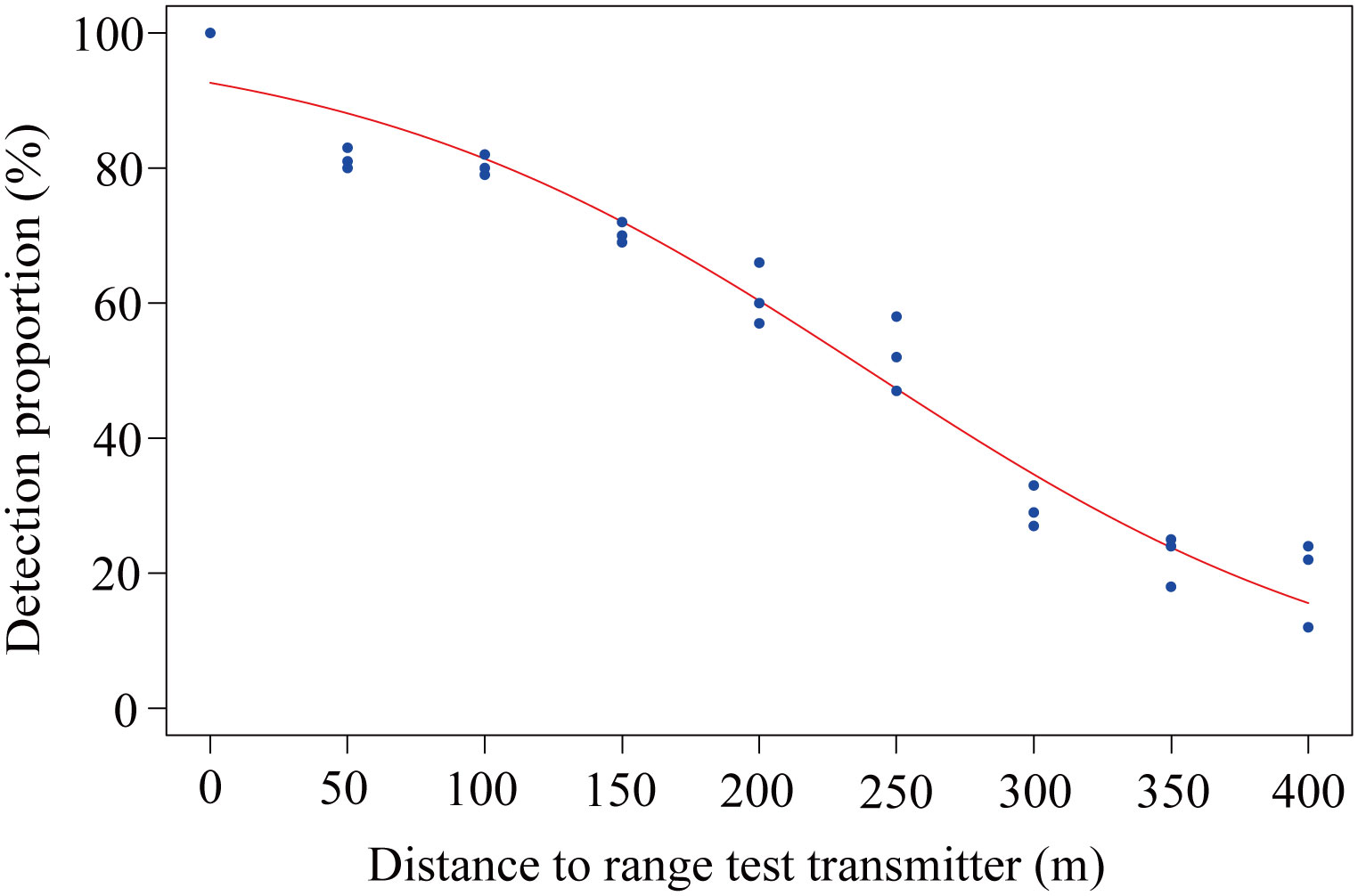
Figure 4 Detection proportion profiles of the 1-h range test in experiment I. Blue dots represent the observed detection proportion per 20 min (n = 3). Red line represents the logistic curve.
3.2 Site fidelity
During the tracking experiment, a total of 142,253 detections for 11 tagged fish were observed within the receiver array, ranging from 101 for fish #6 to 52,383 for #10 (Table 1). Fish showed a high site fidelity to the AR area, and the mean RI was 0.85 ± 0.24. Three out of 11 tagged fish expressed moving behaviors. Three individuals (#2, #6, and #7) left the study area but returned and stayed until the end of the experiment. Two individuals (#3 and #5) left and returned to the study area multiple times but ultimately stayed until the end of the experiment (Table 1). The remaining individuals stayed in the study area throughout the experiment (Figure 5).
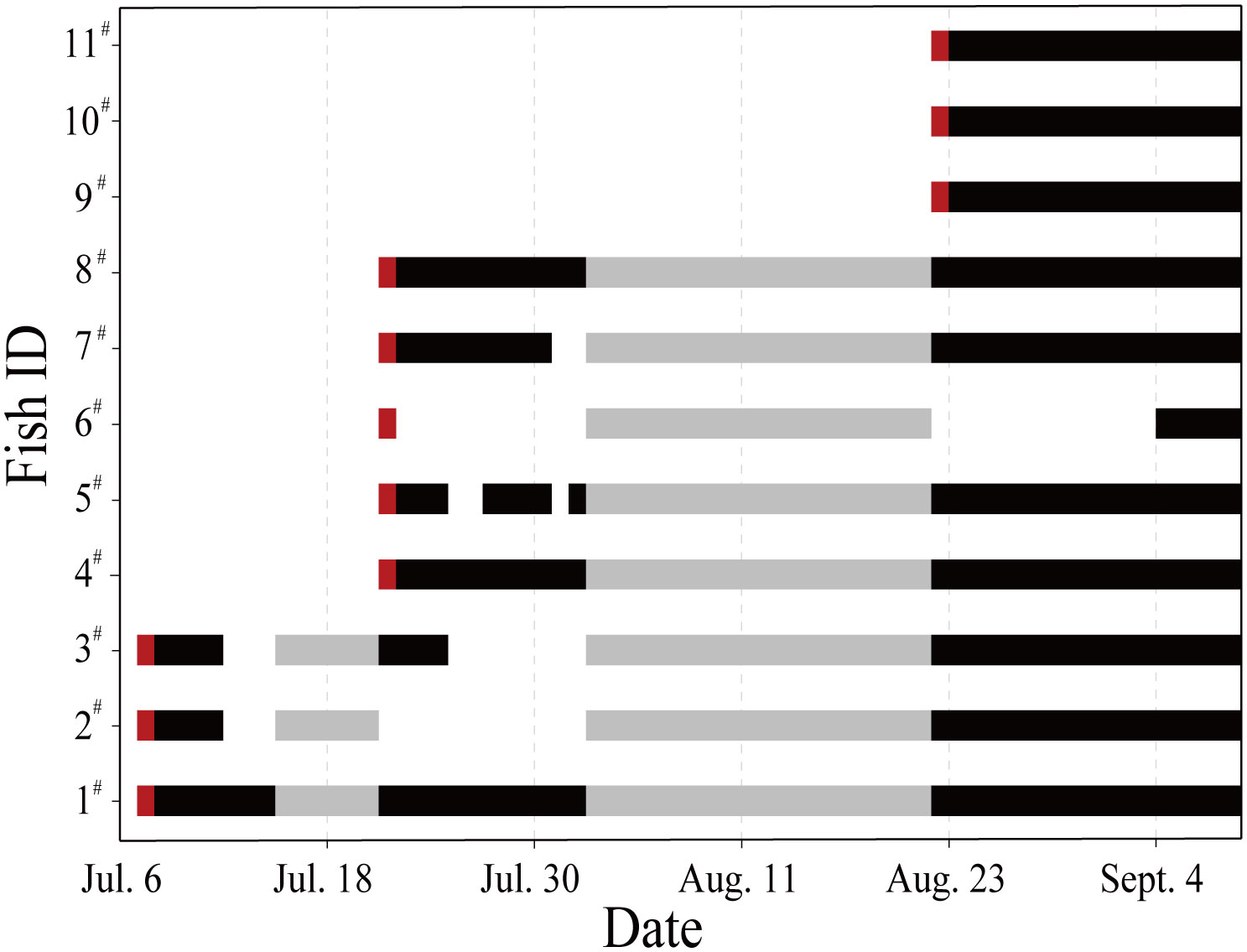
Figure 5 Plot of the daily presence–absence of 11 tagged reef fish between July and September 2017. Red represents the released date, black represents the detected date after the release, and gray represents the date without deploying the receiver array.
3.3 Habitat use range
A total of 35,963 positions were obtained for 11 tagged fish during the tracking experiment. Fish #10 had the maximum number of positions (9,590), but the positioning result of fish #6 was poor with the minimum number of positions (63) (Table 1). Each fish occupied a small range in the AR area (Figure 6). Habitat use range estimated using the 100% MCP was between 337.45 m2 for fish #6 and 133,527.08 m2 for fish #1, with a mean value of 34,522.94 ± 35,548.95 m2; the 95% KUD was between 50.54 m2 for fish #6 and 4,925.45 m2 for fish #9, with a mean value of 1,467.52 ± 1,619.05 m2; the 50% KUD was between 10.92 m2 for fish #7 and 1,009.64 m2 for fish #9, with a mean value of 236.01 ± 294.59 m2.
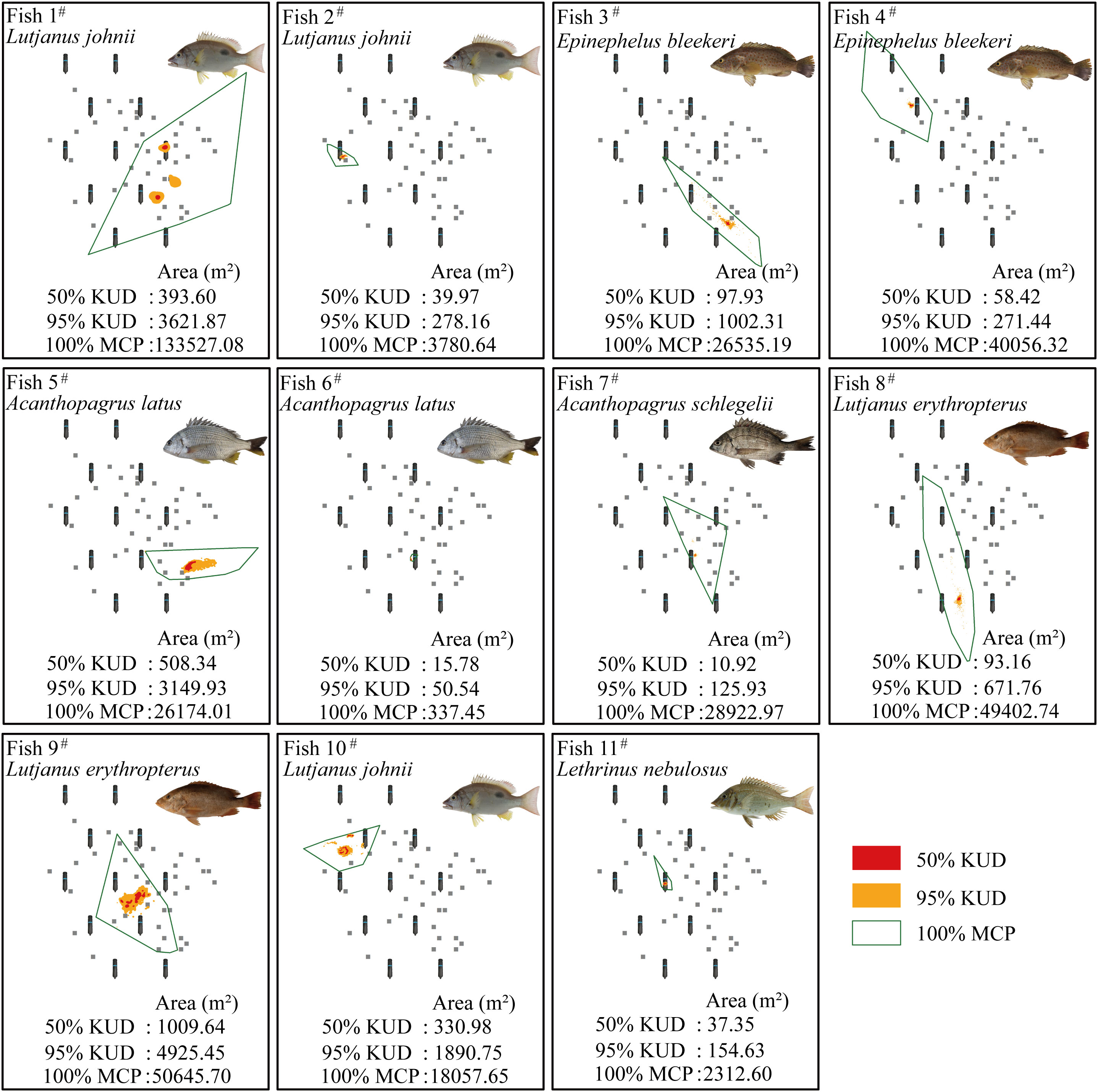
Figure 6 Spatial plot of the habitat use range with the minimum convex polygon (100% MCP) and kernel utilization distribution (95% and 50% KUD) for 11 tagged fish in the Fangchenggang artificial reef sea area. MCP, minimum convex polygon; KUD, kernel utilization distribution.
4 Discussion
This was the first study to conduct a range test and fish tracking using an acoustic telemetry system in the AR area of the northern South China Sea. Through two experiments, we mastered the usage of acoustic equipment and quantified the site fidelity and habitat use of fish. Our results showed the high site fidelity and small spatial scale of habitat use for reef fish, which demonstrated that ARs were an effective man-made structure for attracting fish. The present study finally proved that acoustic telemetry can be successfully used to evaluate the fish attraction effectiveness of ARs.
The key to the deployment of the acoustic telemetry system was the receiver placement and transmitter attachment. Before the receiver is placed, two common methods can be chosen for receiver mooring. One is the concrete mooring (i.e., a concrete block base with an embedded metal bar or polyvinyl chloride pipe), and another is what the present study did. The receiver is moored at a depth of about 1 m from the bottom and needs to be retrieved by diving using the first method (Whoriskey et al., 2019), whereas physical barriers can obstruct the transmission of signals (Welsh et al., 2012; Mathies et al., 2014). Given the height of ARs and the convenience of retrieval, our study chose the second method. In addition, determining appropriate receiver spacing by range test is essential prior to receiver placement. Previous publications have highlighted that the receiver’s detection range varies significantly over space and time and recommend that acoustic telemetry studies need to perform in situ range tests (Kessel et al., 2014). In our study, the range test results were different in Experiment I (80% detection probability within 110 m) and Experiment II (80% detection probability within 150 m), which supports the above recommendation. This is likely due to the bottom topography like ARs, but long-term range test with different environmental factors (such as water depth, tied, and ambient noise) needs to be further studied for obtaining optimal receiver spacing. For transmitter attachment, three main methods for tagging fish with transmitters have been reported. Transmitters can be secured externally, inserted into the stomach, and surgically implanted in fish (Hussey et al., 2015). The study results from Dance et al. (2016) indicated that external attachment of transmitters in fish was detected significantly more than doing so for internal attachment and pointed out that internal attachments may weaken the detection range. In contrast, external attachments are easily performed and minimize handling time. Therefore, our study used external attachment. The result with no transmitter loss and no fish death in our study suggested that external attachment of transmitters may be a successful method for reef fish.
Fish site fidelity and habitat use range are two important indicators to characterize the effectiveness of ARs. In this study, most of the fish showed strong fidelity to the ARs with the mean RI of 0.85 ± 0.24, except that of fish #6 (with RI of 0.2) was not observed until 5 days before the end of the tracking experiment. Although some fish left and returned to the study area during the experiment (it is possible that they were exploring the surroundings and searching for a place to live in), 11 fish (not captured from the study area) stayed in the AR area at the end of the experiment. However, on the contrary, Mitamura et al. (2005) found that the released seabreams have homing behavior, as they tend to return to the site where they were captured. The reason for this difference is that it is possible that the AR area in this study provides a favorable habitat for fish feeding and hiding. For habitat use range, our results indicated that each fish occupied a range in the AR area for living, but in general, it was a small spatial range. This is similar to the findings in an army tank reef from Topping and Szedlmayer (2011). Moreover, to avoid the effect of a typhoon, the receivers were retrieved and connected to the computer to obtain data. It is also advisable to download the data regularly throughout the study to prevent data loss due to boat traffic or bad weather.
The current approaches for evaluating the effectiveness of ARs have limitations and represent only a snapshot of the complex AR ecosystem (Brownscombe et al., 2022). Luckily, the acoustic telemetry system can provide more continuous tracking for the organisms in the AR area. Furthermore, the current approaches require the contrast area for evaluating the effectiveness of ARs. However, it is a challenge to identify a suitable contrast area; so, to date, there is no scientific way to determine the spacing between the AR area and the contrast area (Roni et al., 2018). The MCP in the present study includes all the observed activity positions for each fish in the AR area; the longest distance between the MCP points may be the spacing between the AR area and contrast area, which needs more kinds of reef fish to verify due to the significant MCP differences. In fact, it is still a debate whether the ARs enhance fish population or simply temporally attract fish aggregation (Pickering and Whitmarsh, 1997; Brickhill et al., 2005). Even though the capability of ARs in enhancing fish populations requires long-term study, our study demonstrated that ARs can play a role in attracting fish, including fish that did not originate from the ARs. This information provides valuable contributions to stock enhancement projects in selecting fish species and releasing sites; i.e., priority should be given to the reef fish and AR area.
5 Conclusions
AR construction is expected to become more widely used for maintaining sustainable coastal fisheries. However, it will be challenging to evaluate the effectiveness of ARs, especially with current evaluation approaches. Our study demonstrates that acoustic telemetry can be used as a supplemental approach for evaluating the fish attraction effectiveness of ARs. Meanwhile, this approach is also applicable to studies of other organisms such as shrimp and crabs in the AR area. To better guide AR construction and management, further research is needed to understand the preferences of different reef fish for different AR types (concrete reefs or boat reefs), shapes (cubic or cylindrical), sizes, and depths in the future.
Data availability statement
The original contributions presented in this study are included in the article/supplementary material. Further inquiries can be directed to the corresponding author.
Ethics statement
The animal study was reviewed and approved by the Animal Experimental Ethics Committee of Guangdong Ocean University, China.
Author contributions
SL, GC, and XW conceptualized and designed the study. SL, LW, ZW, and KL conducted the fieldwork and participated in the data analysis. SL drafted the manuscript. HL, LW, GC, and XW wrote sections of the manuscript and revised the manuscript. All authors contributed to the article and approved the submitted version.
Funding
This study was supported by the special funds for Sino-Indonesian Technical Cooperation in Coastal Marine Ranching (12500101200021002), the Science and Technology Infrastructure Project of the Department of Science and Technology of Guangdong Province (2021B1212110005), and the Postgraduate Education Innovation Project of Guangdong Ocean University (202245).
Acknowledgments
We thank Bingnan Li and Xiaowen Chen for assisting with figure drawing and Lei Zeng for assisting with the deployment of equipment in the field.
Conflict of interest
The authors declare that the research was conducted in the absence of any commercial or financial relationships that could be construed as a potential conflict of interest.
Publisher’s note
All claims expressed in this article are solely those of the authors and do not necessarily represent those of their affiliated organizations, or those of the publisher, the editors and the reviewers. Any product that may be evaluated in this article, or claim that may be made by its manufacturer, is not guaranteed or endorsed by the publisher.
References
Alós J., Cabanellas-Reboredo M., Lowerre-Barbieri S. (2012). Diel behaviour and habitat utilisation by the pearly razorfish during the spawning season. Mar. Ecol. Prog. Ser. 460, 207–220. doi: 10.3354/meps09755
Alós J., March D., Palmer M., Grau A., Morales-Nin B. (2011). Spatial and temporal patterns in Serranus cabrilla habitat use in the NW Mediterranean revealed by acoustic telemetry. Mar. Ecol. Prog. Ser. 427, 173–186. doi: 10.3354/meps09042
Biggs C. R., Nemeth R. S. (2016). Spatial and temporal movement patterns of two snapper species at a multi-species spawning aggregation. Mar. Ecol. Prog. Ser. 558, 129–142. doi: 10.3354/meps11846
Block B. A., Whitlock R., Schallert R. J., Wilson S., Stokesbury M. J. W., Castleton M., et al. (2019). Estimating natural mortality of Atlantic bluefin tuna using acoustic telemetry. Sci. Rep-UK. 9, 4918. doi: 10.1038/s41598-019-40065-z
Brickhill M. J., Lee S. Y., Connolly R. M. (2005). Fishes associated with artificial reefs: attributing changes to attraction or production using novel approaches. J. Fish. Biol. 67, 53–71. doi: 10.1111/j.0022-1112.2005.00915.x
Brownscombe J. W., Griffin L. P., Brooks J. L., Danylchuk A. J., Cooke S. J., Midwood J. D. (2022). Applications of telemetry to fish habitat science and management. Can. J. Fish. Aquat. Sci. 79, 1347–1359. doi: 10.1139/cjfas-2021-0101
Brownscombe J. W., Griffin L. P., Chapman J. M., Morley D., Acosta A., Crossin G. T., et al. (2020). A practical method to account for variation in detection range in acoustic telemetry arrays to accurately quantify the spatial ecology of aquatic animals. Methods Ecol. Evol. 11, 82–94. doi: 10.1111/2041-210X.13322
Cenci E., Pizzolon M., Chimento N., Mazzoldi C. (2011). The influence of a new artificial structure on fish assemblages of adjacent hard substrata. Estuar. Coast. Shelf s. 91, 133–149. doi: 10.1016/j.ecss.2010.10.009
Charbonnel E., Serre C., Ruitton S., Harmelin J.-G., Jensen A. (2002). Effects of increased habitat complexity on fish assemblages associated with large artificial reef units (French Mediterranean coast). ICES J. Mar. Sci. 59, S208–S213. doi: 10.1006/jmsc.2002.1263
Cooke S. J., Hinch S. G., Wikelski M., Andrews R. D., Kuchel L. J., Wolcott T. G., et al. (2004). Biotelemetry: a mechanistic approach to ecology. Trends Ecol. Evol. 19, 334–343. doi: 10.1016/j.tree.2004.04.003
Dance M. A., Moulton D. L., Furey N. B., Rooker J. R. (2016). Does transmitter placement or species affect detection efficiency of tagged animals in biotelemetry research? Fish. Res. 183, 80–85. doi: 10.1016/j.fishres.2016.05.009
Food and Agriculture Organization (FAO) (2003). “The ecosystem approach to fisheries (No. 4, suppl. 2),” in FAO technical guidelines for responsible fisheries (Rome: FAO Fisheries and Aquaculture Department). Available at: https://www.fao.org/3/y4470e/y4470e.pdf.
Food and Agriculture Organization (FAO) (2011). “Marine protected areas and fisheries (No. 4, suppl. 4),” FAO Technical Guidelines for Responsible Fisheries (Rome: FAO Fisheries and Aquaculture Department). Available at: https://www.fao.org/3/i2090e/i2090e.pdf.
Food and Agriculture Organization (FAO) (2020). The state of world fisheries and aquaculture 2020 (Rome: FAO Fisheries and Aquaculture Department). Available at: https://www.fao.org/3/ca9229en/CA9229EN.pdf.
Food and Agriculture Organization (FAO) (2022). The state of world fisheries and aquaculture 2022 (Rome: FAO Fisheries and Aquaculture Department). Available at: https://www.fao.org/3/cc0461en/cc0461en.pdf.
Hedger R. D., Hatin D., Dodson J. J., Martin F., Fournier D., Caron F., et al. (2009). Migration and swimming depth of Atlantic salmon kelts Salmo salar in coastal zone and marine habitats. Mar. Ecol. Prog. Ser. 392, 179–192. doi: 10.3354/meps08227
Heupel M. R., Reiss K. L., Yeiser B. G., Simpfendorfer C. A. (2008). Effects of biofouling on performance of moored data logging acoustic receivers. Limnol. Oceanogr-Meth. 6, 327–335. doi: 10.4319/lom.2008.6.327
Hosmer D. W., Lemeshow S. (2000). Applied logistic regression. 2nd ed. (New York: John Wiley & Sons, Inc). doi: 10.1002/0471722146
Hussey N. E., Kessel S. T., Aarestrup K., Cooke S. J., Cowley P. D., Fisk A. T., et al. (2015). Aquatic animal telemetry: A panoramic window into the underwater world. Science 348, 1255642. doi: 10.1126/science.1255642
Jackson J. B. C., Kirby M. X., Berger W. H., Bjorndal K. A., Botsford L. W., Bourque B. J., et al. (2001). Historical overfishing and the recent collapse of coastal ecosystems. Science 293, 629–637. doi: 10.1126/science.1059199
Jia X., Chen P., Tang Z., Chen H., Qin C., Du F. (2021). Construction and demonstration of marine ranching type of artificial reefs placement in subtropical waters: A case study of bailong pearl bay in fangchenggang (Beijing: China Agriculture Press).
Keller J. A., Herbig J. L., Morley D., Wile A., Barbera P., Acosta A. (2020). Grouper tales: use of acoustic telemetry to evaluate grouper movements at Western dry rocks in the Florida keys. Mar. Coast. Fish. 12, 290–307. doi: 10.1002/mcf2.10109
Kessel S. T., Cooke S. J., Heupel M. R., Hussey N. E., Simpfendorfer C. A., Vagle S., et al. (2014). A review of detection range testing in aquatic passive acoustic telemetry studies. Rev. Fish Biol. Fisher. 24, 199–218. doi: 10.1007/s11160-013-9328-4
Lam K. K. Y. (2003). Coral recruitment onto an experimental pulverised fuel ash-concrete artificial reef. Mar. pollut. Bull. 46, 642–653. doi: 10.1016/S0025-326X(02)00482-4
Lima J. S., Zalmon I. R., Love M. (2019). Overview and trends of ecological and socioeconomic research on artificial reefs. Mar. Environ. Res. 145, 81–96. doi: 10.1016/j.marenvres.2019.01.010
Liu H., Xu Q., Xu Q., Zhang Y., Yang H. (2015). The application of stereo-video technology for the assessment on population change of black rockfish Sebastes schlegeli in a vessel reef area in haizhou bay, China. J. oceanol. Limnol. 33, 107–113. doi: 10.1007/s00343-015-4049-2
Lyu S., Deng S., Lin K., Zeng J., Wang X. (2022). Designing and the pilot trial of bivalve molluscan fishing quotas on maoming coastal waters of China, northern south China Sea. Front. Mar. Sci. 9. doi: 10.3389/fmars.2022.863376
Lyu S., Lin K., Zeng J., Liu Y., Chen Z., Wang X. (2021). Fin-spines attachment, a novel external attachment method for the ultrasonic transmitters on hard fin-spines fish (Sparidae). J. Appl. Ichthyol. 37, 227–234. doi: 10.1111/jai.14164
March D., Palmer M., Alós J., Grau A., Cardona F. (2010). Short-term residence, home range size and diel patterns of the painted comber Serranus scriba in a temperate marine reserve. Mar. Ecol. Prog. Ser. 400, 195–206. doi: 10.3354/meps08410
Mathies N. H., Ogburn M. B., McFall G., Fangman S. (2014). Environmental interference factors affecting detection range in acoustic telemetry studies using fixed receiver arrays. Mar. Ecol. Prog. Ser. 495, 27–38. doi: 10.3354/meps10582
Mitamura H., Arai N., Sakamoto W., Mitsunaga Y., Tanaka H., Mukai Y., et al. (2005). Role of olfaction and vision in homing behaviour of black rockfish Sebastes inermis. J. Exp. Mar. Biol. Ecol. 322, 123–134. doi: 10.1016/j.jembe.2005.02.010
Morison A. K. (2004). Input and output controls in fisheries management: a plea for more consistency in terminology. Fisheries Manage. Ecol. 11, 411–413. doi: 10.1111/j.1365-2400.2004.00414.x
Moxham E. J., Cowley P. D., Bennett R. H., von Brandis R. G. (2019). Movement and predation: a catch-and-release study on the acoustic tracking of bonefish in the Indian ocean. Environ. Biol. Fish. 102, 365–381. doi: 10.1007/s10641-019-00850-1
Muñoz L., Aspillaga E., Palmer M., Saraiva J. L., Arechavala-Lopez P. (2020). Acoustic telemetry: a tool to monitor fish swimming behavior in sea-cage aquaculture. Front. Mar. Sci. 7. doi: 10.3389/fmars.2020.00645
Neves V., Silva D., Martinho F., Antunes C., Ramos S., Freitas V. (2018). Assessing the effects of internal and external acoustic tagging methods on European flounder Platichthys flesus. Fish. Res. 206, 202–208. doi: 10.1016/j.fishres.2018.05.015
O′Hara C. C., Frazier M., Halpern B. S. (2021). At-Risk marine biodiversity faces extensive, expanding, and intensifying human impacts. Science 372, 84–87. doi: 10.1126/science.abe6731
OKA M. (1962). Studies on the estimation of the stock capacity of “Gyoshō”. Bull. Jpn. Soc Sci. Fish. 28, 477–483. doi: 10.2331/suisan.28.477
Ona E., Mitson R. B. (1996). Acoustic sampling and signal processing near the seabed: the deadzone revisited. ICES J. Mar. Sci. 53, 677–690. doi: 10.1006/jmsc.1996.0087
Pickering H., Whitmarsh D. (1997). Artificial reefs and fisheries exploitation: a review of the a’ttraction versus production’ debate, the influence of design and its significance for policy. Fish. Res. 31, 39–59. doi: 10.1016/S0165-7836(97)00019-2
Pope J. G. (2009). “Input and output controls: the practice of fishing effort and catch management in responsible fisheries,” in A fishery manager’s guidebook, 2nd ed. Eds. Cochrane K. L., Garcia S. M. (Oxford: Wiley-Blackwell), 220–252. Available at: https://www.fao.org/3/Y3427E/y3427e06.htm.
Roni P., Åberg U., Weber C. (2018). A review of approaches for monitoring the effectiveness of regional river habitat restoration programs. N. Am. J. Fish. Manage. 38, 1170–1186. doi: 10.1002/nafm.10222
Shen G., Heino M. (2014). An overview of marine fisheries management in China. Mar. Policy 44, 265–272. doi: 10.1016/j.marpol.2013.09.012
Smith J. M., Fresh K. L., Kagley A. N., Quinn T. P. (2015). Ultrasonic telemetry reveals seasonal variation in depth distribution and diel vertical migrations of sub-adult Chinook and coho salmon in puget sound. Mar. Ecol. Prog. Ser. 532, 227–242. doi: 10.3354/meps11360
Sreekanth G. B., Lekshmi N. M., Singh N. P. (2017). Can artificial reefs really enhance the inshore fishery resources along Indian coast? a critical review. P. Natl. A. Sci. India B. 89, 13–25. doi: 10.1007/s40011-017-0861-0
Sun J., Peng J. H., Tan Z. Y., Chen J. M., She S. S., Liu M. (2020). Impact of trawling on demersal fishery resources of the pearl river estuary near Hong Kong waters. J. Guangdong Ocean Univ. 40, 64–68. doi: 10.3969/j.issn.1673-9159.2020.01.009
Swadling D. S., Knott N. A., Rees M. J., Pederson H., Adams K. R., Taylor M. D., et al. (2020). Seagrass canopies and the performance of acoustic telemetry: implications for the interpretation of fish movements. Anim. Biotelem. 8, 8. doi: 10.1186/s40317-020-00197-w
Tang J. Y. (2011). Analysis on the practice of the limited entry system in foreign fisheries. J. Guangdong Ocean Univ. 31, 1–6. doi: 10.3969/j.issn.1673-9159.2011.02.001
Topping D. T., Szedlmayer S. T. (2011). Home range and movement patterns of red snapper (Lutjanus campechanus) on artificial reefs. Fish. Res. 112, 77–84. doi: 10.1016/j.fishres.2011.08.013
Welsh J. Q., Fox R. J., Webber D. M., Bellwood D. R. (2012). Performance of remote acoustic receivers within a coral reef habitat: implications for array design. Coral reefs 31, 693–702. doi: 10.1007/s00338-012-0892-1
Whoriskey K., Martins E. G., Auger-Méthé M., Gutowsky L. F. G., Lennox R. J., Cooke S. J., et al. (2019). Current and emerging statistical techniques for aquatic telemetry data: A guide to analysing spatially discrete animal detections. Methods Ecol. Evol. 10, 935–948. doi: 10.1007/s00338-012-0892-1
Williams-Grove L. J., Szedlmayer S. T. (2017). Depth preferences and three-dimensional movements of red snapper, Lutjanus campechanus, on an artificial reef in the northern gulf of Mexico. Fish. Res. 190, 61–70. doi: 10.1016/j.fishres.2017.01.003
Worm B., Barbier E. B., Beaumont N., Duffy J. E., Folke C., Halpern B. S., et al. (2006). Impacts of biodiversity loss on ocean ecosystem services. Science 314, 787–790. doi: 10.1126/science.1132294
Yuan X., Jiang Y., Zhang H., Jin Y., Ling J. (2021). Quantitative assessment of fish assemblages on artificial reefs using acoustic and conventional netting methods, in xiangshan bay, zhejiang province, China. J. Appl. Ichthyol. 37, 389–399. doi: 10.1111/jai.14157
Zeng L., Tang Z., Chen P., Hou C., Chen G. (2018). Bioacoustic application on fisheries management in an artificial reefs′ ecological reserve of bohai gulf China. Environ. Earth Sci. 77, 728. doi: 10.1007/s12665-018-7912-6
Zeng L., Tang Z., Chen P., Yu J., Chen G. (2021). Optimization of fishery resources assessment methods and ecological effects evaluation of artificial reefs. Mar. Biol. Res. 17, 72–85. doi: 10.1080/17451000.2021.1887497
Zhang S., Lin L., Wang X. (2021). Optimization of a marine fish release strategy: a case study of black sea bream Acanthopagrus schlegelii in the zhanjiang estuary, northern south China Sea. Front. Env. Sci-Switz. 9. doi: 10.3389/fenvs.2021.779544
Zhang H., Xie X., Chen P., Yuan H., Feng X., Tong F., et al. (2022). Study on attraction effect of artificial reefs vertical plate with different square apertures on Sparus macrocephalus. South China Fish. Sci. 18, 52–58. doi: 10.12131/20210103
Zhou Y., Cai W., Chen H., Chen P., Lv G., Jia X. (2011). Attraction effect of various artificial reef models on Sparus macrocephalus. J. Fish. Sci. China 35, 711–718. doi: 10.3724/SP.J.1231.2011.17324
Keywords: acoustic telemetry, artificial reefs, fish attraction, site fidelity, habitat use
Citation: Lyu S, Lauden HN, Wang L, Chen G, Wang Z, Lin K and Wang X (2023) Acoustic telemetry system as a novel approach for evaluating the effective attraction of fish to artificial reefs. Front. Mar. Sci. 10:1130547. doi: 10.3389/fmars.2023.1130547
Received: 23 December 2022; Accepted: 23 January 2023;
Published: 07 February 2023.
Edited by:
Kit Yue KWAN, Beibu Gulf University, ChinaReviewed by:
Liangliang Huang, Guilin University of Technology, ChinaBilin Liu, Shanghai Ocean University, China
Copyright © 2023 Lyu, Lauden, Wang, Chen, Wang, Lin and Wang. This is an open-access article distributed under the terms of the Creative Commons Attribution License (CC BY). The use, distribution or reproduction in other forums is permitted, provided the original author(s) and the copyright owner(s) are credited and that the original publication in this journal is cited, in accordance with accepted academic practice. No use, distribution or reproduction is permitted which does not comply with these terms.
*Correspondence: Xuefeng Wang, eHVlZmVuZzE5OTlAMTI2LmNvbQ==