- 1Department of Biological Sciences, University of Chester, Chester, United Kingdom
- 2The Thresher Shark Research and Conservation Project, Cebu, Philippines
- 3Department of Marine and Coastal Sciences, Rutgers University Marine Field Station, Tuckerton, NJ, United States
- 4School of Environmental Sciences, University of Liverpool, Liverpool, United Kingdom
- 5College of Natural Science and Mathematics, Mindanao State University, General Santos City, South Cotabato, Philippines
- 6Divelink Cebu, Daanbantayan, Philippines
We used acoustic telemetry to investigate the roles of depth and temperature in the daytime foraging behaviours of 13 tagged pelagic thresher sharks by monitoring their fine scale vertical movements in the Philippines. Cumulatively, pelagic thresher shark dives traversed the entire water column where they encountered temperatures that ranged from 33°C at the surface to 12°C at 250 m depths throughout the day, but the movements of individuals varied in the extent of both their deep and shallow water limits. Dives were not synchronized to diurnal cycles, and periodicity reflected cycles of similar dives, the dives themselves, deviations, cruising, and individuality. Pelagic thresher shark movements between the warm surface layer and cooler waters below the thermocline (155 – 175 m) may reflect a common Alopiid strategy that balances maintaining tolerable ambient water temperatures with opportunities to search for and forage on spatially patchy distributions of prey.
Introduction
Sharks are ancestrally poikilothermic (Compagno, 2002), but some species have evolved unique physiological and morphological characteristics to respond to disconnects between essential resources that partition habitats in the pelagic environment (prey availability, warmth, dissolved oxygen, light), and exploit its vertical structure (Compagno, 2002; Sutton, 2013; Schlaff et al., 2014). Variations of these characteristics, which may differ among species of the same genus, have manifested in cranial endothermy (to buffer heat exchange for the brain and eyes) (Bres, 1993; Weng and Block, 2004), and/or endothermy for swimming (Klimley et al., 2002; Meekan et al., 2015; Harding et al., 2021). Other species have adapted behavioural traits that manifest in vertical oscillations through the water column to mediate unfavourable ambient water temperatures and exposure to hypoxic conditions that occur at depth where prey are located (Carey and Scharold, 1990; Gleiss et al., 2019; Arostegui et al., 2020; Andrzejaczek et al., 2022). Diving to access prey in deep dark water cools muscles and eyes that are needed for wide-ranging searches. If a physiological solution is not evolved, timely returns to warm surface waters devoid of prey are required (Lawson et al., 2010; Sutton, 2013; Gleiss et al., 2019).
End points of vertical movement for foraging are defined as diel vertical migration (DVM) on one end and rapid but short aperiodic excursions on the other (Gleiss et al., 2019). This spectrum is seen in a broad range of predatory taxa in the pelagic zone including istiophorids, scombrids, and lamniform elasmobranchs (Lawson et al., 2010; Bernal et al., 2017; Gleiss et al., 2019). Although oscillatory dives might be expected to be similar within a species, they may be modified by local cues and individual traits learned from experience within spatially and temporally well-defined bodies of water (Bres, 1993; Weng and Block, 2004; Schlaff et al., 2014; Andrzejaczek et al., 2022). Understanding the variation (periodicity) in oscillatory diving in such conditions is important to quantifying the range of traits on which selection acts (Lawson et al., 2010; Bernal et al., 2017; Gleiss et al., 2019; Andrzejaczek et al., 2022).
The pelagic thresher shark (Alopias pelagicus) is an Indo-Pacific lamniform that is reported to frequent coastal and offshore waters from the surface to at least 632 m (Stevens et al., 2009; Sutton, 2013; Oliver et al., 2019; Arostegui et al., 2020). Pelagic thresher sharks are likely to be migratory (Baum et al., 2003; Nakano et al., 2003; Reardon et al., 2004; Oliver, 2012; Sutton, 2013; Oliver et al., 2019), but little is known of their vertical movements in the open ocean (Oliver et al., 2019; Arostegui et al., 2020). Since pelagic thresher sharks prey on food items that use DVM (which is a widespread phenomenon in marine and freshwater species) as an evasion strategy (Hays, 2003; Oliver et al., 2013), we can predict that their diving behaviours will be challenged by depth gradients in temperature and dissolved oxygen. Here we used acoustic telemetry to quantify the periodicity of pelagic thresher shark dives in the Visayan Sea and investigate the role that depth and temperature play in their daytime foraging behaviour.
Method
Monad Shoal is a shallow coastal seamount situated in the Central Visayan Sea, eight kilometers east of Malapascua Island, Cebu, in the Philippines (N 11° 19’ 06.7”, E 124° 11’ 31.9”) (Oliver, 2012; Oliver et al., 2019). The seamount rises 250 m from the sea floor to 15 - 25 m depths where it plateaus with a surface area of approximately 4.5 km2. Cleaner fish occupy stations along the mount’s southern fringe where they provide essential parasite removal services that benefit thresher shark health and fitness (Oliver et al., 2011; Cadwallader et al., 2015; Oliver and Bicskos Kaszo, 2015). The predictable and repetitive occurrence of these interactions offered a unique opportunity to tag pelagic thresher sharks without catching them, and to monitor their movements near and away from the seamount (Figure 1A) (Oliver et al., 2019).
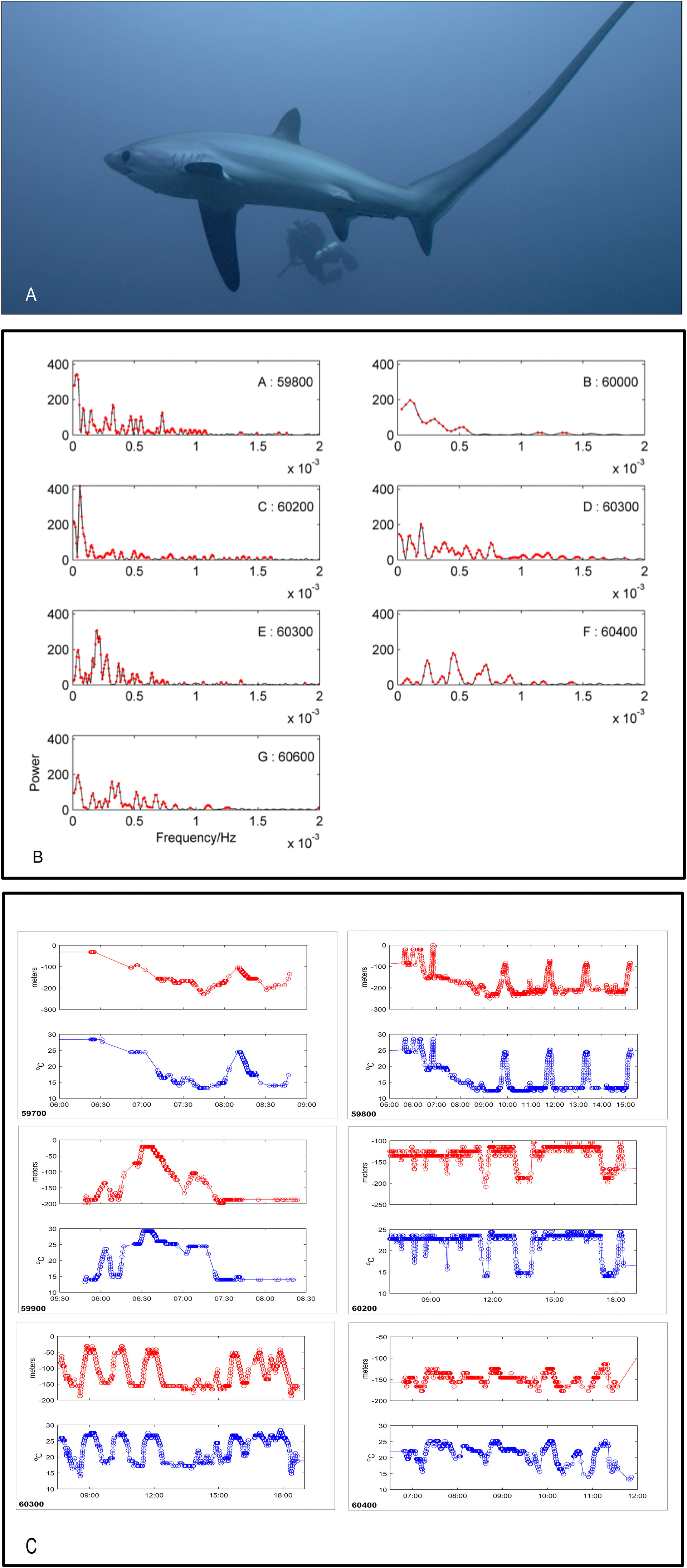
Figure 1 (A) Tags were deployed into the sharks’ interdorsal musculature using closed-circuit rebreathers and a modified speargun. (B) Lomb-Scargle periodograms of depth for six acoustically-tagged pelagic thresher sharks (shark 60300 has two separate time series, in sub-panels D, E). Red dots correspond to P-values below 0.05 (representing frequencies with higher power than would be expected under white noise). (C) Examples of depth (m) and temperature profiles (°C) against time of day (x-axis) expanded from full records. Shark tag ID is indicated in the bottom left of each sub-panel.
Divers tagged thresher sharks with external sensored acoustic tags (Lotek Wireless Inc. model MM-M-16-25-TP) darted into the interdorsal musculature from 7 to 28 June 2014 (see methods in Oliver et al., 2019). Transmitters broadcasted unique CDMA-encoded identifiers every 3 seconds (Grothues, 2009). A second channel on each tag alternately (6 s repeat) transmitted pressure (psi) or temperature (°C). In the days after tagging we tracked sharks leaving the seamount from a skiff equipped with stereo directional hydrophones (LPH_1, Lotek Wireless, Inc.) connected to a logging/processing system (MAP RT-A Lotek Wireless Inc.) (Oliver et al., 2019). A RayMarine (model CP100) CHIRP down-vision sonar, broadcasting at 200 kHz with Time Varying Gain surveyed the water column for reflective targets and measured depth to provide environmental context for the interpretation of the dive patterns.
Temperature and pressure data were filtered using methods described in Oliver et al. (2019), and then scaled into 50 bins according to preprogramed ranges of -6 °C to 34 °C and 0 and 750 psi (~510 m) respectively. To investigate the temperature profile of the water column from scatter plots, the alternating temperature and depth data were discretized to the nearest 5 s timestamp, after which co-occurring values from a given shark were plotted against each other.
Three of the tag sensors (two temperature and one pressure) failed during the tracking period, but failures did not occur over the entire detection period and were independent of each other (pressure or temperature). Sensor failures were identifiable as either high frequency (~12 s) oscillations between reporting real data and false floors (raw value = 0) when the underlying dive behaviours could be observed, or when they went to 0 for ensuing durations. Sensor failures also decoupled the temperature/depth relationship for part of the record. Descriptive statistics of dispersion were therefore limited to data set fragments prior to sensor failure (independently for depth and temperature). We used the Lomb-Scargle method to quantify the periodicity of thresher sharks’ vertical movements because the time series of depth for the tagged sharks contained unequally spaced observations resulting from different tracking encounters of a given shark (Lomb, 1976; Scargle, 1982). All of the analyses applied MATLAB (The MathWorks, Inc., Natick, Massachusetts, USA) native functions.
Results
We tagged 13 pelagic thresher sharks overall, and actively tracked ten of these during 21 days of field operations (Oliver et al., 2019). Six of the corresponding time series provided sufficient data (>900 observations each) for the Lomb-Scargle analyses to provide clear pictures of diving behaviour. Although the mean ( ± SD) water temperature was 24 ± 3 °C for all sharks combined, the ambient water temperature that individual thresher sharks were exposed to during their vertical movements ranged from 12 °C to 33 °C and was highly variable (Table 1). The depth profiles of the tagged thresher sharks were also highly variable and ranged from the surface of the water column (0 m) to the bottom of the water column (250 m at the deepest point in the valley below). All of the sharks travelled to depths in excess of 175 m, but the mean depths were much shallower and varied among individuals (Table 1). Although there was some spatial and temporal variation, the water temperature gradually declined from 30 °C at the surface to approximately 24 °C at 155 m (Figure 2). It then declined rapidly to 15 °C over the next 20 m in the thermocline. Below 180 m, the water appeared to be well mixed and was generally between 13 and 14 °C with a minimum of 12 °C(Figure 2).
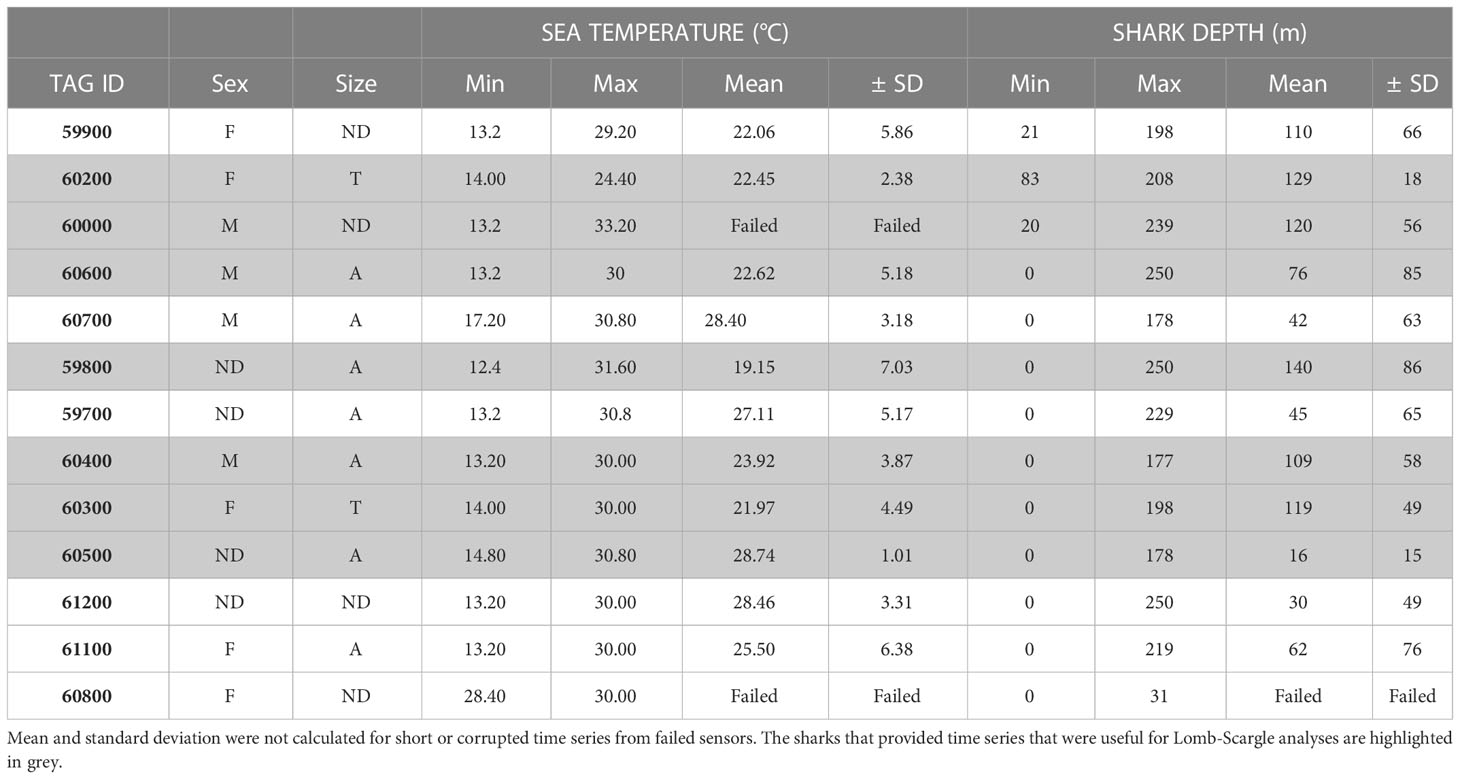
Table 1 Summary data of tagged and tracked male (M), female (F), juvenile (J), transitional (T), adult (A), and sex/maturity undetermined (ND) pelagic thresher sharks.
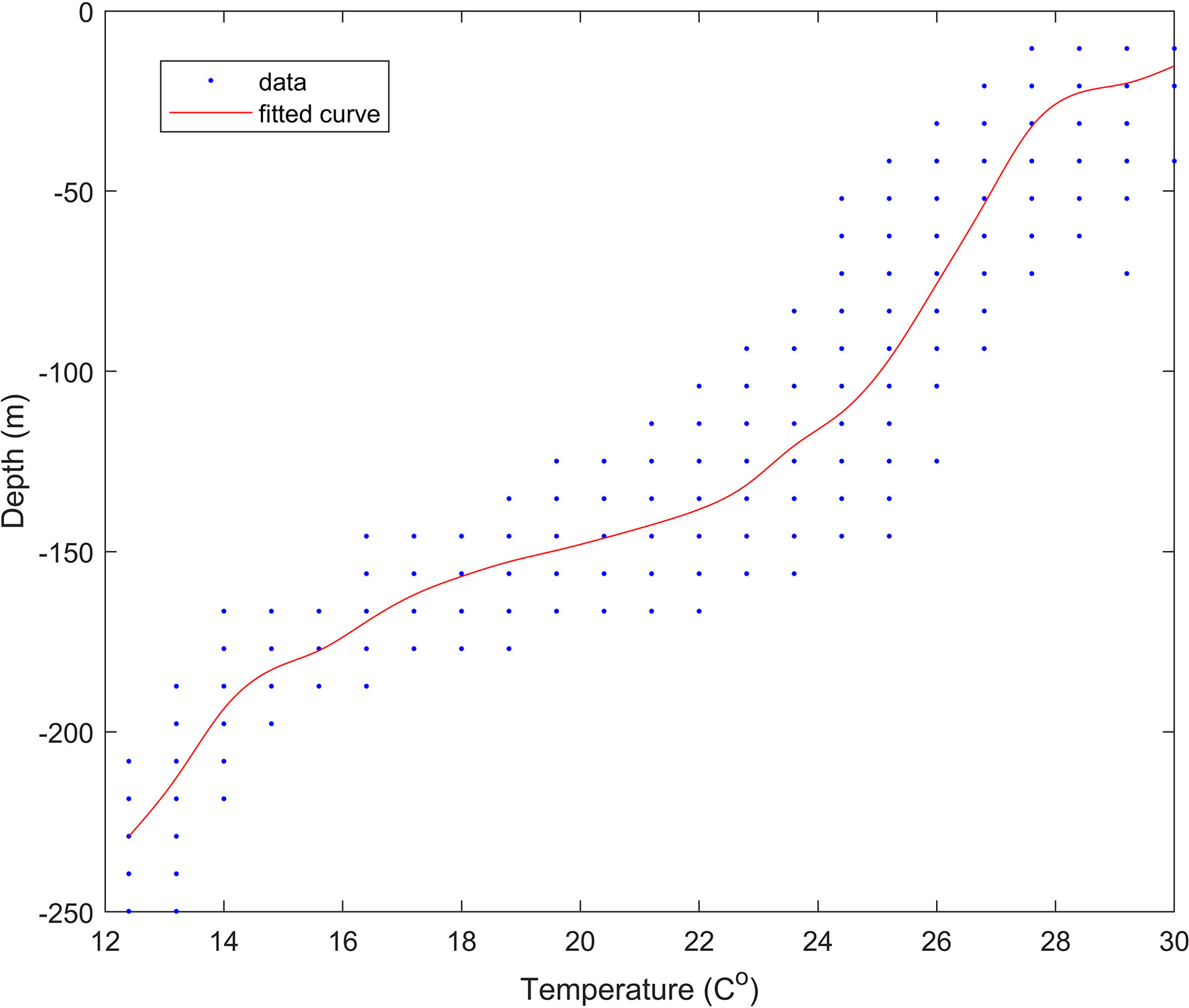
Figure 2 Depth versus temperature data for 1,146 records where depth and temperature data for a given shark (amongst all sharks combined) were both logged in a common 5 sec discretized time stamp. The red line shows the best fit model using a smoothed spline.
Sonar targets consisted of several types of structures including highly localized dense aggregations consistent with schools of fish or squids, intermittent scattering layers (narrowly constrained in depth), and possibly the sharks themselves (Figure 3). A deep scattering layer was apparent just above the sea floor during daytime and crepuscular observations (Figure 3).
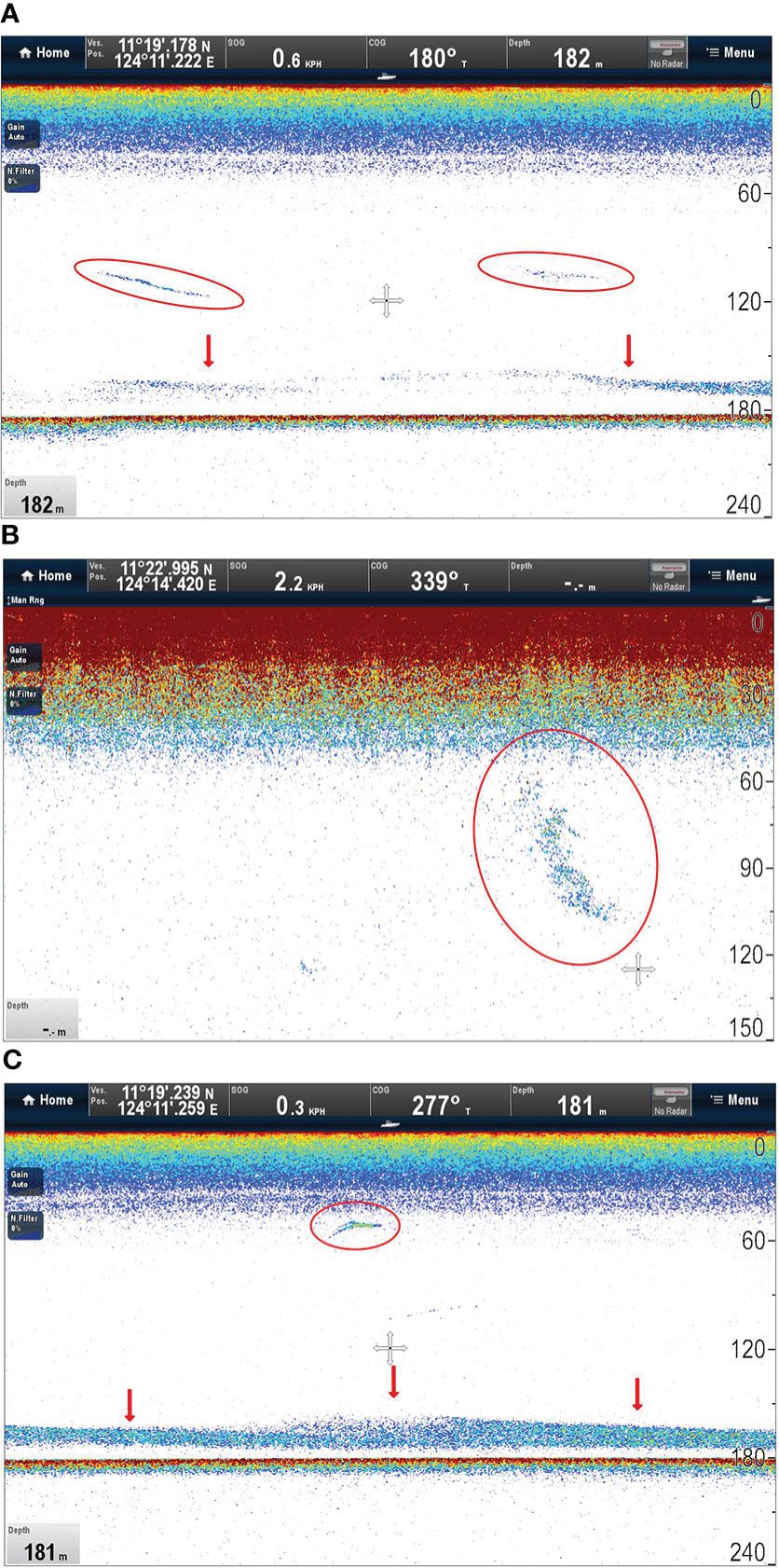
Figure 3 Ray-Marine sonograms showing (A) patchiness in the mid layer (circles) and continuity in the deep scattering layer (arrows); (B) a highly localised dense aggregation consistent with a school of fish (circle); and (C) intermittent scattering layers (arrows) and possibly the tracked shark itself (circle).
When comparing periodograms between sharks there were no obvious matching peaks for different individuals (Figure 1B). Inspection of the dive profiles revealed the mechanism behind the important spectrum bands. Low frequency peaks for some sharks were composed not of individual dive oscillations but periods of similar repetitive dives (e.g. shallow dives within the upper mixed layer of 5 to 10 minutes each) that gave way to a period of other cyclical dives (e.g. predominantly staying on the bottom and rising sharply and briefly through the thermocline to mid water before returning to the bottom with a single dive cycle having a period of about 1.2-1.6 hours) (Figure 1C: Shark ID no.s 59800, 6300). In another example there was a period of no diving followed by a period of diving (Figure 1C: Shark ID no. 6200). Occasionally, the longer dives had short deviations up or down superimposed on them with periods of 1-10 minutes, which were irregular among dives within a set of otherwise similar dives (Figure 1C: Shark ID no.s 59700, 59800). The highest frequency cycles were an artefact of sharks swimming level at a depth corresponding to the boundary between an upper or lower sensor reporting bin, so that one or the other depth bin might be reported every 6 s (Figure 1C: 6200). Some sharks made use of the entire water column throughout the day, while others appeared to be more selective in their depth profiles (Figure 1C). The total depth range appeared to influence the time period since dives over greater depth ranges took longer to traverse (Figure 1C).
Discussion
The longest dive oscillation phase (~10 hours) was consistent with our prediction that pelagic thresher sharks would respond to the DVM of their prey (Hays, 2003). DVM is typically characterised by prey species remaining in deep water during the day to avoid visually orientating predators and moving into shallow water at night where they feed before returning to depths at dawn (Bollens and Frost, 1989; Andrzejaczek et al., 2022). Our daytime sonargrams showed an intermittent sound scattering layer (SSL) close to the seafloor that was consistent with small schooling fish (Sameoto, 1982; Arostegui et al., 2020). While pelagic thresher sharks made no single dives that lasted a day, they appeared to respond to DVM patterns in their prey by performing series of deep-water dives in the early hours of the morning (Josse et al., 1998; Stevens et al., 2009; Musyl et al., 2011; Bernal et al., 2017). Similar patterns have been observed in a variety of shark species including bigeye thresher sharks, Alopias superciliosus, (Musyl et al., 2011), common thresher sharks, Alopias vulpinus (Cartamil et al., 2010; Cartamil et al., 2011), blue sharks, Prionace glauca (Carey and Scharold, 1990), white sharks, Carcharhinus carcharias (Dewar et al., 2004), basking sharks, Cetorhinus maximus (Shepard et al., 2006), and oceanic white tip sharks, Carcharhinus longimanus (Andrzejaczek et al., 2018). Yet the dominant periodicity in the vertical movements of the pelagic thresher sharks that we tagged varied for each individual in spite of having strong spectral periodicities at periods of less than 1 cycle per day, which indicated localized and idiosyncratic modifications in response to other factors (Musyl et al., 2011).
One of the most widespread behaviours associated with the movements of marine vertebrates in the open ocean is oscillatory swimming in which ectotherms optimize their body temperature by recovering heat lost when deep diving (Klimley et al., 2002; Harding et al., 2021), or by transferring heat to the water column to reduce heat stress (Kitagawa et al., 2006; Bernal et al., 2017). Shark species that exhibit this behavioural pattern include the bigeye thresher shark, Alopias superciliosus (Musyl et al., 2011), the scalloped hammerhead shark, Sphyrna lewini (Klimley, 1993), and the blue shark, Prionace glauca (Carey and Scharold, 1990). Oscillatory movements by pelagic thresher sharks in this study reflect this type of behaviour, and pelagic thresher sharks could be returning to surface waters to warm-up at the limit of their thermal inertia, after performing a deep dive (Andrzejaczek et al., 2022). The depth limits of the dive oscillations could also reflect a behavioural adaptation to avoid increasingly hypoxic conditions that occur below 150 m (Bernal et al., 2017; Arostegui et al., 2020; Harding et al., 2021; Andrzejaczek et al., 2022). Although swimming in oscillations may be efficient as a thermoregulation and/or respiration strategy for pelagic thresher sharks, it risks temporarily decoupling them from access to their prey (Klimley et al., 2002; Musyl et al., 2011).
The thermal gradient encountered by tagged pelagic thresher sharks was characterised by a strong thermocline, indicating a pycnocline, and the dives of several sharks were focused in corresponding depths. While such limits may have met a shark’s need to thermoregulate without additional travel, it is possible that pelagic thresher shark dive oscillations may also have intercepted the scent plumes of their prey which typically disperse along isopycnals (rather than spherically) in stratified water (Carey and Scharold, 1990). Our sonargrams showed that targets consistent with prey were found throughout the water column. DVM in thresher shark dives may therefore not have been a response to DVM in prey if a win-stay-lose-shift or backlighting strategy was favoured (Figure 3) (Carey and Scharold, 1990; Bonnet-Lebrun et al., 2021). These pressures could be working in tandem to drive the evolution of physiological solutions to thermoregulation in pelagic thresher sharks.
When investigating the vertical movements of a single pelagic thresher shark in the Red Sea, Arostegui et al. (2020) showed that the shark’s daytime diving behaviour avoided hypoxic conditions that occur below 300 m in relatively cool (mean 22.1 °C) water (even though that is where the daytime distribution of its prey was most likely to be located) in favour of nighttime overlaps with small epipelagic prey fishes that occur in relatively warm (mean 24.2 °C) surface waters (50-150 m). They concluded that the shark’s diving behaviour was not constrained by the Red Sea’s ambient water temperature which ranged from 21 to 26 °C over a 24-hour (day and nighttime) cycle. Conversely, the ambient water temperature that the 13 sharks that we tagged encountered in the Visayan Sea ranged from 12 to 33 °C over a 12-hour daytime cycle. The daytime dive profiles and 21 °C maximum temperature exchange that we recorded resemble those reported for closely related bigeye thresher sharks (Compagno, 2002; Musyl et al., 2011) and support other assertions that pelagic thresher sharks are physiologically and behaviourally adapted for thermotolerance (Weng and Block, 2004; Harding et al., 2021). Unlike the shark that was monitored in the Red Sea where depth was not limited by the seafloor and where the minimum temperature at maximum depth (632 m) was 21.6 °C, our observed maximum recorded diving depth of 250 m was constrained by the depth of the water column. Since most of the 13 sharks that we tagged only went to the bottom of the thermocline where the water temperature was 12 °C, data collected from pelagic thresher sharks in deeper, mixed, truly pelagic environments, will further knowledge of their ecology in the future.
Data availability statement
The raw data supporting the conclusions of this article will be made available by the authors, without undue reservation.
Ethics statement
The animal study was reviewed and approved by the Department of Tourism, the Governor of Cebu, and the Municipality of Daanbantayan, and adhered to the Philippine ‘Wildlife Resources Conservation and Protection Act’. Tagging was performed under IACUC compliant protocol No. 04-020 (Rutgers University).
Author contributions
SO and TG conceived and designed the study. ZM and TG organized the database and performed the statistical analysis. SO and TG wrote the first draft of the manuscript. SO, TG, AW, MS and GC collected the field data. All of the authors contributed to manuscript revision, read, and approved the submitted version.
Funding
This work would not have been possible without generous funding from Discovery Communications® and the allocation of essential resources by S. Christopher, D. Allen, Scubazoo and Passion Pictures. The research was additionally supported by a University of Chester International Research Excellence Award (to SPO), funded under the Santander Universities scheme. The funders were not involved in the study design, collection, analysis, interpretation of data, the writing of this article, or the decision to submit it for publication.
Acknowledgments
We are particularly grateful to the Philippines Department of Tourism, the Governor of the Province of Cebu, and the Municipal Mayor of DaanBantayan for their field support and guidance. We thank V. Cerna, M. Reed, S. Enderby, J. Isley, and R. Munns, M. Healey, A. Fritz, M. Schwarzmeier, M. Jenson, S. Zudnick, staff and crew from M/Y Vasco, TSRCP, Divelink Cebu, and Evolution Dive Resort for their assistance in the field, and we thank Matthew Spencer for contributing to the design of the analyses and reviewing the manuscript. The manuscript was enhanced by input from three reviewers whom we thank.
Conflict of interest
Author GC was employed by company Divelink Cebu.
The remaining authors declare that the research was conducted in the absence of any commercial or financial relationships that could be construed as a potential conflict of interest.
Publisher’s note
All claims expressed in this article are solely those of the authors and do not necessarily represent those of their affiliated organizations, or those of the publisher, the editors and the reviewers. Any product that may be evaluated in this article, or claim that may be made by its manufacturer, is not guaranteed or endorsed by the publisher.
References
Andrzejaczek S., Gleiss A. C., Jordan L. K. B., Pattiaratchi C. B., Howey L. A., Brooks E. J., et al. (2018). Temperature and the vertical movements of oceanic whitetip sharks, Carcharhinus longimanus. Sci. Rep. 8, 8351. doi: 10.1038/s41598-018-26485-3
Andrzejaczek S., Lucas T. C. D., Goodman M. C., Hussey N. E., Armstrong A. J., Carlisle A., et al. (2022). Diving into the vertical dimension of elasmobranch movement ecology. Sci. Adv. 8, eabo1754. doi: 10.1126/sciadv.abo1754
Arostegui M., Gaube P., Berumen M., Digiulian A., Jones B., Røstad A., et al. (2020). Vertical movements of a pelagic thresher shark (Alopias pelagicus): Insights into the species’ physiological limitations and trophic ecology in the red Sea. Endanger Species Res. 43, 387–394. doi: 10.3354/esr01079
Baum J. K., Myers R. A., Kehler D. G., Worm B., Harley S. J. (2003). Collapse and conservation of shark populations in the Northwest Atlantic. Science 299, 389–392. doi: 10.1126/science.1079777
Bernal D., Brill R. W., Dickson K. A., Shiels H. A. (2017). Sharing the water column: physiological mechanisms underlying species-specific habitat use in tunas. Rev. Fish Biol. Fisher 27, 843–880. doi: 10.1007/s11160-017-9497-7
Bollens S., Frost B. (1989). Predator-induced diel vertical migration in a planktonic copepod. J. Plankton Res. 11, 1047–1065. doi: 10.1093/plankt/11.5.1047
Bonnet-Lebrun A.-S., Collet J., Phillips R. A. (2021). A test of the win-stay–lose-shift foraging strategy and its adaptive value in albatrosses. Anim. Behav. 182, 145–151. doi: 10.1016/j.anbehav.2021.10.010
Cadwallader H. F., Turner J. R., Oliver S. P. (2015). Cleaner wrasse forage on ectoparasitic digeneans (phylum Platyhelminthes) that infect pelagic thresher sharks (Alopias pelagicus). Mar. Biodiv. Rec 45, 613. doi: 10.1007/s12526-014-0290-8
Carey F. G., Scharold J. V. (1990). Movements of blue sharks (Prionace glauca) in depth and course. J. Mar. Biol. 106, 329–342. doi: 10.1007/BF01344309
Cartamil D. P., Sepulveda C. A., Wegner N. C., Aalbers S. A., Baquero A., Graham J. B. (2011). Archival tagging of subadult and adult common thresher sharks (Alopias vulpinus) off the coast of southern California. J. Mar. Biol. 158, 935–944. doi: 10.1007/s00227-010-1620-4
Cartamil D., Wegner N. C., Kacev D., Ben-aderet N. B., Kohin S., Graham J. B. (2010). Movement patterns and nursery habitat of juvenile thresher sharks Alopias vulpinus in the southern California bight. Mar. Ecol. Prog. Ser. 404, 249–258. doi: 10.3354/meps08495
Compagno L. J. V. (2002). “Sharks of the world,” in An annotated and illustrated catalogue of shark species known to date, vol. 125. (United Nations Development Programme, Food and Agriculture Organization of the United Nations, Rome: FAO Fisheries Synopsis).
Dewar H., Domeier M., Nasby-Lucas N. (2004). Insights into young of the year white shark, Carcharodon carcharias, behavior in the southern California bight. Environ. Biol. Fishes 70, 133–143. doi: 10.1023/B:EBFI.0000029343.54027.6a
Gleiss A. C., Pattiaratchi C. B., Meekan M. G. (2019). Patterns and drivers of vertical movements of the large fishes of the epipelagic. Rev. Fish Biol. Fish 29 (2), 335–354. doi: 10.1007/s11160-019-09555-1
Grothues T. M. (2009). “A review of acoustic telemetry technology and a perspective on its diversification relative to coastal tracking arrays,” in Tagging and tracking of marine animals with electronic devices. Eds. Nielsen J. L., Arrizabalaga H., Fragoso N., Hobday A., Lutcavage M., Sibert J. (Netherlands: Springer), 77–90.
Harding L., Jackson A., Barnett A., Donohue I., Halsey L., Huveneers C., et al. (2021). Endothermy makes fishes faster but does not expand their thermal niche. Funct. Ecol 35” 1951–, 1959. doi: 10.1111/1365-2435.13869
Hays G. C. (2003). A review of the adaptive significance and ecosystem consequences of zooplankton diel vertical migrations. Hydrobiologia 503, 1630170. doi: 10.1023/B:HYDR.0000008476.23617.b0
Josse E., Bach P., Dagorn L. (1998). Simultaneous observations of tuna movements and their prey by sonic tracking and acoustic surveys. Hydrobiologia 371, 61–69. doi: 10.1023/A:1017065709190
Kitagawa T., Sartimbul A., Nakata H., Kimura S., Yamada H., Nitta A. (2006). The effect of water temperature on habitat use of young pacific bluefin tuna Thunnus orientalis in the East China Sea. Fish Sci. 72, 1166–1176. doi: 10.1111/j.1444-2906.2006.01273.x
Klimley A. (1993). Highly directional swimming by scalloped hammerhead sharks, Sphyrna lewini, and subsurface irradiance, temperature, bathymetry, and geomagnetic field. Mar. Biol. 117, 1–22. doi: 10.1007/BF00346421
Klimley A. P., Beavers S. C., Curtis T. H., Jorgensen S. J. (2002). Movements and swimming behavior of three species of sharks in la Jolla canyon, California. Environ. Biol. Fishes 63, 117–135. doi: 10.1023/A:1014200301213
Lawson G. L., Castleton M. R., Block B. A. (2010). Movements and diving behavior of Atlantic bluefin tuna Thunnus thynnus in relation to water column structure in the northwestern Atlantic. Mar. Ecol. Prog. Ser. 400, 245–265. doi: 10.3354/meps08394
Lomb N. R. (1976). Least-squares frequency analysis of unequally spaced data. Astrophys Space Sci. 39, 447–462. doi: 10.1007/BF00648343
Meekan M. G., Fuiman L., Davis R., Berger Y., Thums M. (2015). Swimming strategy and body plan of the world's largest fish: Implications for foraging efficiency and thermoregulation. Front. Mar. Sci. 2. doi: 10.3389/fmars.2015.00064
Musyl M. K., Brill R. W., Curran D. S., Fragoso N. M., McNaughton L. M., Nielsen A., et al. (2011). Postrelease survival, vertical and horizontal movements, and thermal habitats of five species of pe.lagic sharks in the central pacific ocean. Fish. Bull. 109 (4), 341–368.
Nakano H., Matsunaga H., Okamoto H., Okazaki M. (2003). Acoustic tracking of bigeye thresher shark alopias superciliosus in the eastern pacific ocean. Mar. Ecol-Prog Ser. 265, 255–261. doi: 10.3354/meps265255
Oliver S. P. (2012). The behaviour and biology of pelagic thresher sharks (Alopias pelagicus) in the Philippines (Bangor University Repository: PhD thesis. Bangor University Press).
Oliver S. P., Bicskos Kaszo A. E. (2015). Pelagic thresher shark (Alopias pelagicus) gives birth at a cleaning station in the Philippines. Coral Reefs 34, 17–18. doi: 10.1007/s00338-014-1249-8
Oliver S. P., Grothues T. M., Williams A. L., Cerna V., Silvosa M., Cases G., et al. (2019). Risk and resilience: High stakes for sharks making transjurisdictional movements to use a conservation area. Biol. Conserv. 230, 58–66. doi: 10.1016/j.biocon.2018.11.013
Oliver S. P., Hussey N. E., Turner J. R., Beckett A. J. (2011). Oceanic sharks clean at coastal seamount. PloS One 6 (3), e14755. doi: 10.1371/journal.pone.0014755
Oliver S. P., Turner J. R., Gann K., Silvosa M., Jackson T. D. (2013). PloS One, 8(7), e67380. doi: 10.1371/journal.pone.0067380
Reardon M., Márquez F., Trejo T., Clarke S. C. (2004). Alopias pelagicus. in: IUCN 2010. IUCN Red List Threatened Species.Version 2010 (1).
Sameoto D. (1982). Zooplankton and micronekton abundance in acoustic scattering layers on the Nova scotian slope. Can. J. Fish Aquat Sci. 39, 760–777. doi: 10.1139/f82-104
Scargle J. D. (1982). Studies in astronomical time series analysis. II-statistical aspects of spectral analysis of unevenly spaced data. ApJ 263, 835–853. doi: 10.1086/160554
Schlaff A. M., Heupel M. R., Simpfendorfer C. A. (2014). Influence of environmental factors on shark and ray movement, behaviour and habitat use: A review. Rev. Fish Biol. Fish 24 (4), 1089–1103. doi: 10.1007/s11160-014-9364-8
Shepard E. L., Ahmed M. Z., Southall E. J., Witt M. J., Metcalfe J. D., Sims D. W. (2006). Diel and tidal rhythms in diving behaviour of pelagic sharks identified by signal processing of archival tagging data. Mar. Ecol. Prog. Ser. 328, 205–213. doi: 10.3354/meps328205
Stevens J. D., Bradford R. W., West G. J. (2009). Satellite tagging of blue sharks (Prionace glauca) and other pelagic sharks off eastern Australia: Depth behaviour, temperature experience and movements. Mar. Biol. 157, 575–591. doi: 10.1007/s00227-009-1343-6
Sutton T. T. (2013). Vertical ecology of the pelagic ocean: Classical patterns and new perspectives. J. Fish Biol. 83 (6), 1508–1527. doi: 10.1111/jfb.12263
Keywords: pelagic thresher shark, vertical movements, thermoregulation, foraging, acoustic telemetry
Citation: Oliver SP, Grothues TM, Mayo ZJ, Williams AL, Silvosa M and Cases G (2023) Depth and temperature profiles reflect individual differences in the daytime diving behaviours of pelagic thresher sharks. Front. Mar. Sci. 10:1128473. doi: 10.3389/fmars.2023.1128473
Received: 20 December 2022; Accepted: 19 January 2023;
Published: 09 February 2023.
Edited by:
Molly E. Lutcavage, University of Massachusetts Boston, United StatesReviewed by:
Richard Brill, College of William & Mary, United StatesRussell Bradford, Commonwealth Scientific and Industrial Research Organisation (CSIRO), Australia
Chi Hin Lam, University of Massachusetts Dartmouth, United States
Copyright © 2023 Oliver, Grothues, Mayo, Williams, Silvosa and Cases. This is an open-access article distributed under the terms of the Creative Commons Attribution License (CC BY). The use, distribution or reproduction in other forums is permitted, provided the original author(s) and the copyright owner(s) are credited and that the original publication in this journal is cited, in accordance with accepted academic practice. No use, distribution or reproduction is permitted which does not comply with these terms.
*Correspondence: Simon P. Oliver, cy5vbGl2ZXJAY2hlc3Rlci5hYy51aw==
†ORCID: Simon P. Oliver, orcid.org/0000-0002-2975-1233
Thomas M. Grothues, orcid.org/0000-0003-2095-8286