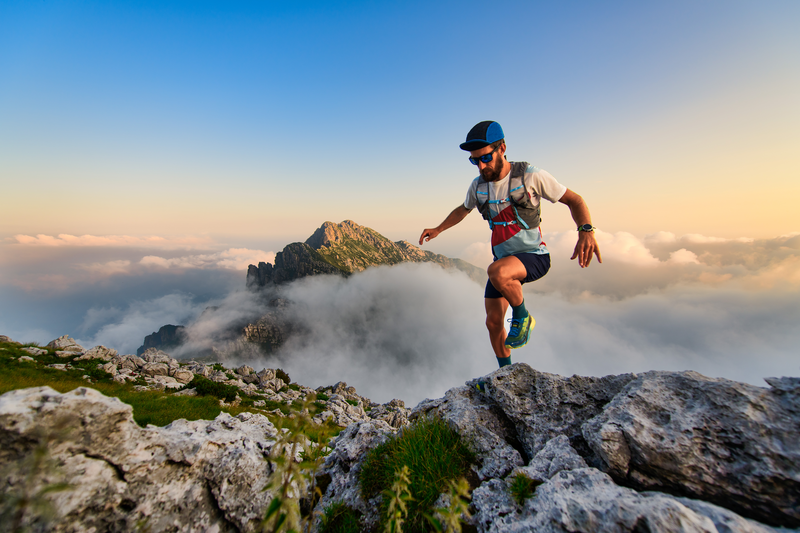
94% of researchers rate our articles as excellent or good
Learn more about the work of our research integrity team to safeguard the quality of each article we publish.
Find out more
ORIGINAL RESEARCH article
Front. Mar. Sci. , 20 April 2023
Sec. Marine Biology
Volume 10 - 2023 | https://doi.org/10.3389/fmars.2023.1127790
This article is part of the Research Topic Syngnathid Fishes: Biology, Ecology, Physiology, Conservation and Innovative Rearing Techniques View all 13 articles
Reliably quantifying the strength of visual sexual signals, such as iridescence, has been challenging across the field of evolutionary biology, but is critically important for studying biologically relevant trait variation. To address this issue, we present the Iridescence Detection and Isolation Algorithm (IDIA), which was designed to isolate the iridescent signal from photographs for quantification of ornamentation. The Gulf pipefish, Syngnathus scovelli, served as a model system for testing the limits of the algorithm, and was an ideal test case due to their female-specific iridescent bands on their abdomens with a large degree of among-individual variation. Specifically, we tested the repeatability of iridescence estimates in a variety of settings, including manual versus automated measurements, a gradient of lighting intensities, observational data from multiple populations, and in detecting exposure to synthetic estrogen. Using the IDIA, female iridescence was quantified in two ways with results indicating a manual measurement of each individual band may be more reliable than the automated measurement taken by drawing a polygon around all bands. However, the intensity of the lighting the photographs were taken in did not significantly affect repeatability of the measurement of iridescence no matter how it was taken. The IDIA was able to detect geographical variation in female ornamentation of S. scovelli, demonstrating that our automated approach can potentially replicate previously-described population-level variation. Differences in the iridescent signal were significant when comparing female pipefish from the Florida coast to females collected from the Texas coast, indicating the possibility that external factors, such as differing environmental conditions, could affect the strength of female visual signals. Lastly, the IDIA was applied in an ecotoxicology application to detect the development of iridescence in male pipefish exposed to synthetic estrogen. Exposed males began expressing banding patterns with iridescence levels within the range of females. The results from this study confirm the feasibility of using the IDIA for measuring iridescence in fish across a variety of applications.
The evolution of elaborate ornamentation in sexually mature organisms that are advantageous for mating success, rather than survival (Kirkpatrick, 1982), can be explained by the theory of sexual selection (Darwin, 1871; Clutton-Brock, 2007; Clutton-Brock, 2017). Darwin distinguished primary sexual characteristics, such as the organs for reproduction, from secondary sexual characters, which include morphological differences seen between sexes that are not directly involved in reproduction (Darwin, 1871; Jones and Ratterman, 2009). Exaggerated traits can be used for either intrasexual selection, where members of the same sex evolve weaponry used in mating competition for access to the other sex, or intersexual mate choice resulting in ornamentation to attract the choosy sex (Emlen and Oring, 1977; Moore, 1990; Clutton-Brock, 2007). Secondary sexual traits, such as bold coloration or horns and tusks in males, do not seem to fit into the theory of natural selection because they are costly to produce, maintain, and in many cases, make the organisms more evident to potential predators, decreasing survivorship (West-Eberhard, 1979; Muniz and Machado, 2018; Hare and Simmons, 2019). The evolution and persistence of secondary sexually selected traits emerge from the preference of large or elaborate ornaments; therefore increasing the reproductive success of individuals bearing these traits (Fisher, 1915; Kirkpatrick et al., 1990).
The study of sexual selection has focused predominantly on male ornamentation and the corresponding female mate choice; however, these studies do not reflect the diversity of the animal kingdom and may lead to biases in research and the development of evolutionary theory (Clutton-Brock, 2009; Számadó and Penn, 2015; Hare and Simmons, 2019). The presence of female secondary sexual characteristics raises important unanswered questions about the evolutionary mechanisms influencing sexual selection acting upon female ornamentation. Female intrasexual and intersexual selection exist across a wide variety of taxa, including fishes (Dijkstra et al., 2009; Flanagan et al., 2014; Rometsch et al., 2021), insects (Rillich et al., 2009), crabs (Baldwin and Johnsen, 2012), amphibians (Pröhl and Hödl, 1999), birds (Trail, 1990; Sæther, 2002; Lipshutz, 2017), and mammals (Bebié and McElligott, 2006; Powell, 2008), often resulting in the development of elaborate female traits. The investigation of female ornamentation in sex-role reversed systems creates the opportunity to understand sexual selection pressures more fully on females, but currently repeatable tools for quantifying variation in many ornaments are lacking.
Testing the measurement of female sexual signals should ideally be conducted within a group of organisms where the mating system and sexually selected traits are well understood. One of the most apparent cases of role reversal with higher sexual selection pressures on females is seen in the family Syngnathidae. Syngnathids, including seahorses, pipefishes, pipehorses, and seadragons, provide a unique platform to examine female ornamentation and male mate choice as they exhibit male pregnancy with several species possessing a sex-role-reversed mating system (Vincent et al., 1992; Jones et al., 2001). In this family of fishes, females transfer eggs to the males’ brooding structures following elaborate courtship displays, and the male then fertilizes the eggs and houses the embryos as they develop (Wilson et al., 2003; Silva et al., 2010). Although seahorses and some pipefish species display monogamous behaviors, pipefish exhibit a range of polyandrous to polygynandrous behaviors, with sexual dimorphism with more elaborate traits in females (Rosenqvist and Berglund, 2011). This role reversal results in females competing for access to mates and therefore experiencing stronger sexual selection pressures (Jones et al., 2005). Female ornamentation in pipefishes has been shown to predict mating success (Flanagan et al., 2014; Monteiro et al., 2017), reproductive success (Berglund et al., 1997), fecundity (Mobley et al., 2018), female-female competitiveness (Bernet et al., 1998), and female and offspring quality (Cunha et al., 2017).
Female signals have evolved across pipefish species, and due to the energetic costs of producing both eggs and ornamentation, are often honest indicators of a females’ quality (Berglund, 2000; Sogabe and Ahnesjö, 2011; Rose et al., 2013b). Sexually selected signals in female pipefish include dimorphic bodies (longer torsos, increased body width, ventral flaps, or skin folds), displays (temporary melanistic changes, dancing vertically in the water column, body twitches, or postures), and markings on the body (stripes, spots, banding patterns, or iridescence; reviewed in Rosenqvist and Berglund, 2011) and have been quantified in a variety of ways. Developing tools to measure the various sexually selected ornaments can therefore provide information about an individual’s fitness. For example, temporary female ornamentation expressed during courtship in the broadnose pipefish, Syngnathus typhle, has been shown to be strongly correlated with the number of eggs females have, indicating ornamentation is an honest signal of the female’s fertility (Berglund et al., 1997). Mobley and colleagues (Mobley et al., 2018) measured the thickness of the stripes found on the female torso of the sex-role-reversed Stigmatopora nigra, the wide-bodied pipefish, and found correlations between the ornament and fecundity. Band width was also used in the sex-role-reversed species Syngnathus abaster, the black-striped pipefish, in addition to a measurement of luminance, to show selection for wider stripes in females and a higher overall fertility and fecundity in females with darker stripes (Cunha et al., 2017). Additionally, the number of female secondary selected bands has been shown to be a good predictor of reproductive success in the Gulf pipefish, Syngnathus scovelli. A component of these previously-counted bands is their iridescence. While iridescence has been quantified in several organisms (reviewed in Gruson et al., 2019), this trait has not been commonly measured in fish.
Iridescence is found across a broad distribution of taxa ranging from ctenophores to cephalopods to various insects, fish, reptiles, amphibians, birds and even the fur of some small mammals (Doucet and Meadows, 2009). Within these taxa, the iridescent ornamentation fulfills a variety of roles such as species recognition (Silberglied and Taylor, 1978), intraspecies competition (Fitzstephens and Getty, 2000; Lim and Li, 2006), or age determination (Doucet et al., 2006; Bitton and Dawson, 2008). Similar to many other types of ornamentation, iridescent signals have been found to be costly to maintain as depleted environments result in organisms with lower levels of iridescence (McGraw et al., 2002; Kemp et al., 2006; Lim and Li, 2007). Iridescence has also been found to be an important component of mate choice. In male peacocks (Pavo cristatus), both the brightness of the eyespots and the degree of iridescence on the train was correlated to frequency of visitation by females and male mating success (Loyau et al., 2007). Among syngnathids, multiple species possess some variation of iridescent markings, including the weedy seadragon (Phyllopteryx taeniolatus), the African freshwater pipefish (Enneacampus ansorgii), the straightnose pipefish (Nerophis ophidion), the worm pipefish (Nerophis lumbriciformis), the Gulf pipefish (Syngnathus scovelli), the blue-stripe pipefish (Doryrhamphus excisus), and the sargassum pipefish (Syngnathus pelagicus). Measuring this observed iridescence has varied across different researchers, with iridescence being quantified as the area of the blue sexual markings (Berglund et al., 1986; Flanagan et al., 2014), in terms of presence/absence of iridescence (Partridge et al., 2010), and as a rating on a scale (Sundin et al., 2016). To collect data that can be compared across studies, and across species, a reliable and repeatable method for measuring iridescent signals in pipefishes is needed.
Syngnathus scovelli is commonly used as a model system for the understanding of sexual selection pressures on females due to their sexual dimorphism playing an important role in polyandrous mate choice decisions (Jones et al., 2001; Rose et al., 2013a; Partridge et al., 2013; Flanagan et al., 2014; Anderson and Jones, 2019). At sexual maturity, females of this species possess an intricate array of ornamentation along their abdomens of multiple components. The permanent light-colored bands located on the middle of the female’s torso are estrogen-responsive (Partridge et al., 2010; Anderson et al., 2020), and are overlaid by a bluish iridescent signal covering part or all of the band. Additionally, during courtship and other social behaviors, female pipefish develop alternating light-dark bars along the top of their abdomen and proceeding down the length of the tail. This melanistic change usually corresponds with the S-shaped display of S. scovelli courtship. Previous studies have shown that females are selected for by the number and area of these permanent light-colored bands, with females that possess either many intermediately sized bands, or a small number of large bands experiencing the greatest mating success (Flanagan et al., 2014), but the iridescent signal of these bands has not been investigated.
To fully understand the mechanisms involved with the evolution of secondary sexual traits, reliable measurements of elaborate ornaments are necessary to determine the relationship between a selected trait and an organism’s fitness. Identifying the strength of these visual sexual signals reliably and without human bias has been challenging across the field, and little is known about the extent of natural variation in many of these signals (Rosenqvist and Berglund, 2011). In this study, the Iridescence Detection and Isolation Algorithm (IDIA) was developed and tested as a quantification method for iridescent signals. This program was used on many individual Gulf pipefish to investigate the effect of lighting intensity on the strength of the iridescent signal, detect geographical variation in the female ornamentation, and identify morphological changes in male S. scovelli as a result of estrogen exposure.
Syngnathus scovelli used to test the effects of lighting intensity in this study were collected in Tampa Bay, under special activities license # SAL-18-0182-E granted by the State of Florida in June 2019. All animal use protocols were approved under The University of Tampa AUP #2018-1. Photos of animals used in the geographic variation portion of this study were new analyses conducted using data from Flanagan et al. (2016). For the study investigating the role of synthetic estrogen on band development and iridescence in male pipefish, animals were collected from Redfish Bay near Aransas Pass, Texas (N 27 53 39.07, W 97 7 51.69) in July 2013 under the Texas Parks and Wildlife permit number SPR-0808-307 with protocols approved by the Institutional Animal Care and Use Committee at Texas A&M University and outlined in AUP # 2013–0020 (Reference #001898).
The Iridescence Detection and Isolation Algorithm (IDIA) was designed as a macro for MS Access Database to isolate the iridescent signal into a false-color image from photographs. The false color image then allows for automation of the process of quantifying iridescent ornamentation based on the spectral characteristics of each pixel rather than solely human identification of the signal. To generate the equation for the Iridescence Detection and Isolation Algorithm (IDIA), we first explored parameters that could report iridescence from the brightness and color patterns from images of known iridescence. Images of pipefish with iridescent signals were linked to a Microsoft Access database and were visualized within the program using package modules mIntelJPEGLibrary.bas and cDIBSection.cls (from VB Accelerator) to convert images from JPEG to Device-Independent Bitmaps (DIB file formats). DIB formats store data as a color table by mapping RGB values to individual pixels in the image, while keeping original image size and pixel scaling consistent across digital platforms. Converted images were displayed and used to develop the algorithm and were initially visually assessed for specific pixels in the image that represented iridescence, in this case, on the torso of pipefish females. The corresponding RGB values from pixels we identified as iridescent were statistically analyzed for their parameters to create the optimal values. Equations (see below) were developed to consistently identify the pixels throughout the entire images that met the combination of criteria of known iridescent pixels. After this initial empirical development step, where trial and error were used to determine the visual fit to images and the appropriate thresholds (visually analyzing pixel by pixel by eye), coding in MSAccess used the equations described below to assign red to each pixel in the entire image where pixels with the spectral characteristics of iridescence was detected. Developing the correct placement of red signal replacing iridescence for quantification was not a completely automated process because the user was able to optimize the parameters for their specific image and iridescence or colors of the trait under review. Reflections and inconsistencies in images due to variation in lighting conditions caused some non-specific shading and false iridescent signals on the fish, therefore we generated multiple photos with the false coloration over the iridescent signals with different cutoff thresholds to better fit the iridescence profile for each individual image. The overall goal of defining the parameters was to be able to replace pixels that met the correct criteria with a false signal that could be quantified, replacing the need to make decisions on a pixel by pixel bases for images.
Optimization of the parameters that could best identify pixels with iridescence was empirically derived from images of pipefish with known iridescence across a range of color parameters. Pixels were assessed in ImageJ for their red, green, and blue hexadecimal channel intensity using a scale of 0 – 255 color units, where 0 represented an absence of that color and 255 indicated a maximum brightness, a parameter which is defined as , where i refers to the color (R for red, G for green, or B for blue) and j and k refer to the x and y coordinates of the pixel in the image, respectively. A minority of pixels were too dark to measure so were excluded from the analysis. A minimum value of brightness for each color () was determined using pixels with known iridescence and pixel lacking iridescence, which allowed us to identify the optimal iridescence values and optimal ratios of red to green, green to blue, and red to blue for the study species. This process of selecting pixels corresponding to blue hue iridescence in the bands on Gulf pipefish allowed us to develop the parameters for the equation supporting the IDIA program that could highlight all pixels within that range, removing human biases in the downstream process of iridescent selection for quantification. The particular criterions for these parameters listed below can be optimized for other iridescent traits on different species in the future.
Four main parameters were combined to generate the IDIA equation and get an overall iridescence score. The four parameters were chosen because they were identified as the most variable components in the iridescent pipefish bands, which are brightness and saturation of the blue hue. The parameters used were suggested by Gruson and colleagues (Gruson et al., 2019) as the best parameters for isolating these components of the blue hue. The first parameter addresses the difference between the color scores of the pixels () and a determined minimum brightness value () to detect the potential for iridescence and ensure pixels are not too dark (Equation 1). The optimal values for S. scovelli were set at > 80, > 90, and > 60.
Ratios of the color hues were then used to identify the specific color patterns for Gulf pipefish bands compared to background color of the fish’s abdomen as the second parameter (Equation 2). The values 0.9, 1.3, and 1.4 were used for the red:green, red:blue, and green:blue ratios respectively to distinguish iridescence for S. scovelli, as described in the previous paragraph.
The third parameter measures the difference between green and blue channels (Equation 3), with a value of 40 being selected out of 0-255 range for the S. scovelli bands.
The fourth parameter adds an additional verification of iridescence based on the total size of the image. Here, we measure the value of blue intensity () for each pixel relative to the minimum and maximum values for the blue intensity in the surrounding pixels (Equation 4). The surrounding pixels that are incorporated into the calculation of the local range is determined by the photo size (# of pixels). For a 5 MP photo, a window of 3 pixels away in each direction was used, resulting in a 7x7 grid used to calculate the local range of blue intensity for the included pixels. The number of pixels in the image and size of this pixel window (n) is determined by calculating and rounding its product down to the nearest odd number. This value is then used to establish how many pixels in each direction surrounding the pixel of interest should be included in the local range (m) by calculating . With this local range now defined, the local minimum and maximum blue values ( and ) are used to define the contrast in iridescence between the focal pixel and its local range (Equation 4).
The size of this pixel window scales inversely with pixel dimension, as a higher resolution leads to a larger window. The pixel blue band value () for Equation 4 should be as close as possible to the high end (and far from the low) of the local range of the blue band, and the local range should be as wide as possible to assure the pixel is within a cluster of iridescent and not a false signal.
The IDIA equation creates a final value for each pixel to be used for replacing iridescence with a false-color signal that can be quantified in ImageJ. To calculate the IDIA, a series of squared residual functions for each parameter (Equation 1-4) are calculated for each pixel in the selected area of images were then added together to get an iridescence score (targeting the 0-255 range; Equation 5).
The final residuals penalization function is adjusted up by 10000, cut off below 0, and square root is taken, to get an IDIA score in the 0-255 range. Five images are created in MSAccess by red replacing the color for any pixel with an IDIA value of 140,150,160,170, and 180, which allows the user to set the sensitivity of the program by determining which IDIA value best represents the iridescence for each particular image to account for any variation in background signals. Once the user selects the best image, the corresponding IDIA value is used for quantifying the number of pixels that meet that threshold in a given area of the photo that the user selects in ImageJ.
All pipefish used in the study were photographed to include the entirety of the iridescent bands located on their torsos in a single image, with a scale included in every image. Olympus cameras used for photographs that ranged in model, but the photos taken fell into two categories, 1) 3968 X 2976 pixels (1.2 to 2.3MB), and 2) 4608 X 3456 pixels (5.85 to 6.12MB).
For quantification analysis of the iridescence area on the body, pixel-scale data containing the RGB values were extracted from photographs using ImageJ 1.8.0 for the location set by the user for measurement (Schneider et al., 2012). In ImageJ, the area of the torso that includes the colored bands was selected using the polygon tool, and the x/y coordinate system was used to identify specific pixel locations to analyze their spectral characteristics (using Analyze→Tools→Set XY Coordinates). Using the scale in ImageJ, the number of pixels per millimeter were measured and entered into the IDIA MSAccess macro in addition to the IDIA threshold previously selected from the 140-180 range by the user. The 140-180 threshold was chosen because those values produced the most reliable and repeatable identification of the iridescence found in the bands by the IDIA program, rather than background reflectance that could falsely identify non-iridescence in the photos. For example, since we are imaging fish that have a reflective surface, we aimed to identify just the iridescence in the given area that is associated with the bands, which was verified by human eye (similar to the butterfly example in Gruson et al., 2019). The final calculation of iridescence is generated by MSAccess by summing the total number of pixels within the area of the torso box that meet the IDIA threshold and converting the pixels to area using the scale in each image to provide the final measurement of the iridescent area in squared millimeters. In S. scovelli, iridescence is predominantly distributed on the torso, beginning just behind the operculum and usually extending to the urogenital opening (Figure 1B; although in some females, it extends onto the tail, Figures 1C, 2).
Figure 1 (A) Photos of the iridescent bands found on female S. scovelli and (B) the corresponding iridescent signal that is highlighted by the Iridescence Detection and Isolation Algorithm (IDIA). (C) represents the area of the torso that possesses the iridescent bands while (D, E) show the two ways of quantifying iridescence though using the IDIA and ImageJ (mean band iridescence and overall iridescence respectively).
Figure 2 A map of the four geographic sites chosen to analyze for this study from Flanagan et al. (2016). For each location, there is a photo of a female S. scovelli collected at that site (top) paired with that same photograph after being processed through the Iridescence Detection and Isolation Algorithm (IDIA) (bottom). The red colouration in these photographs represents areas distinguished as iridescent by the IDIA. Due to limitations in the field all photos were taken in various lighting conditions. However, we conducted experiments under varied lighting conditions and found that iridescence does not significantly differ in response to lighting (Figure 3).
This study used two approaches to quantify torso iridescence, 1) measuring all iridescence from the torso of pipefish and 2) isolating the signal from the bands themselves. This distinction allows us to determine the area of iridescence from the sexually-selected bands themselves relative to the overall iridescence shown in the torso area, which might be responding to different selective pressures, such as pre- and post-copulatory sexual selection, environmental conditions, or ecological competition. After the images were processed using the IDIA, iridescence was quantified and reported in two ways, overall iridescence, and mean band iridescence (Figure 1).
Overall iridescence was measured by outlining the focal area of iridescence on the pipefish using the polygon tool in ImageJ (Figure 1E), using a series of selected landmark locations around the area of interest on the torso of the pipefish, including the banding pattern. The selected x/y coordinates and pixel RGB values from the polygon area were used in the IDIA algorithm to sum up the pixels that contain the iridescent signal within the designated polygon, converting it to area using the metric scale in the image (mm2). The use of this tool allows for all of the iridescence to be measured on the fish without human decisions, therefore removing user bias associated with outlining the banding patterns. Overall iridescence measurements used in the analyses were not adjusted for by the number of bands.
To measure the contribution of the individual bands to the overall iridescence on the torso of the fish, the band iridescence method was calculated by replicating methods used in Flanagan et al. (2014). However, instead of measuring the physical area of the band via the permanent coloration located on the female torsos, the iridescent signal identified by the red from the IDIA false-color image was measured instead. The false-color images were exported to ImageJ where the scale was set, and the red coloration signified as iridescence for each individual band was outlined by hand (Figure 1D). With the outlines, area of iridescence (mm2) was generated for every band. For each pipefish, the individual band area measurements were summed and divided by the total number of iridescent bands resulting in a mean band iridescence.
Each pipefish whose image was measured for iridescence was also measured for length and dorso-ventral body depth. The length was measured from the tip of the snout to the end of the tail, and depth was measured from the point in front of the dorsal fin down the bottom of the torso. All statistical analyses were conducted in R Studio version 1.2.5 using packages MASS (Venables and Ripley, 2002) and car (Fox and Weisberg, 2019) for the generalized linear models.
Previous studies have indicated that color perception may be affected by additional components, including the background pattern and color of the organism, color sensitivity of the receiver, or environmental factors, such as ambient light (Gomez and Théry, 2007; Loyau et al., 2007). Because of this, the purpose of this initial study was to test the reliability of the IDIA and determine the effect of varying light intensities on the strength of the iridescent signal found on S. scovelli. Twenty-four sexually mature female S. scovelli were collected in seagrass beds located in Tampa Bay using a push net in June 2019 and transported to The University of Tampa in Tampa, FL. Pipefish were photographed in 2.5-gallon tanks filled with seawater made to 28 ppt using Instant Ocean® Sea Salt (http://www.instantocean.com) across three different lighting conditions previously used in other pipefish studies (Masonjones et al., 2010; Flanagan et al., 2014; Flanagan et al., 2016), including 9, 13, and 21 lumens using an Olympus ToughTG-5 digital camera placed 8.4 cm in front of the tank. White, waterproof paper was added to the back and bottom of all tanks to keep the background coloration constant and a PAR sensor was used to indicate the intensity of the light. A white photo box was utilized for the two lower range lighting levels (9 and 13 lumens) with an LED light bar placed on the top, and all other light sources were eliminated, and the highest lighting level was set-up outside of the photo box in regular fluorescent lighting (21 lumens). At each lighting level, three photos were taken of the individual pipefish to account for any inherent variation in the photo-taking process and test the repeatability of the program, resulting in nine photographs per pipefish. The entire photographing process took less than five minutes.
For all images, both band and overall iridescence were measured. To remove any biases, a double-blind procedure was implemented when the photos were read into the IDIA. The first researcher sorted images into their own folders and created a key that linked the photo ID to the specific pipefish and lighting condition. These folders were then given to a second researcher who used a random number generator to assign each folder a new number. These new numbers were added to the original key and given back to the first researcher. The randomized photos were used when collecting iridescence measurements and at the end, the measurements were matched to the original pipefish ID and lighting condition using the key. As there was no interest in the interaction between the different lighting conditions and the two measurement methods, mean band and overall iridescence were analyzed separately. Overall and mean band iridescence expressed normality and equal variances, therefore, one-way ANOVAs between the two iridescent measurements and lighting conditions were performed.
Iridescence was quantified for female pipefish from four geographic locations that were previously collected for population genomics analyses (Flanagan et al., 2016). Females were selected from two Texas populations (South Padre and Port Aransas) and two Florida populations (Fort De Soto and Anne’s Beach) and the IDIA was used to detect potential variation in iridescence (Figure 2). In addition to the mean band and overall iridescence measurement, the iridescence on the torso that was not on the banding patterns was calculated. Torso iridescence was calculated by subtracting the mean band iridescence from the overall iridescence.
Seventeen pipefish were randomly selected from each location to create equal sample sizes for the analysis. An initial analysis into the morphometrics of the female S. scovelli indicated body length and depth significantly varied across the four geographic locations (One-way ANOVA: F3,64 = 27.29, p< 0.0001; One-way ANOVA: F3,64 = 48.43, p< 0.0001 respectively). Overall, the Florida pipefish were longer and deeper than the Texas populations. Additionally, the females from the Port Aransas TX population expressed significantly greater length and depth than the South Padre location (Figure S2A, One-Way ANOVA: F3, 64 = 11.79, p< 0.0001). Due to the strong relationship between length and depth (Pearson’s correlation: r = 0.87, df = 66, p< 0.0001), only one was incorporated into our models. The iridescent ornament is largely located on the torso of the females and stops before or just after the urogenital pore; therefore the depth of the female is more likely to affect the amount of iridescence present. All iridescence measurements were divided by body depth to adjust for depth variation prior to the analysis of band and overall iridescence. The depth-adjusted overall iridescence expressed normality and equal variances therefore a one-way ANOVA between overall iridescence and location was performed. Mean band and torso iridescence residuals were not normally distributed and log-transforming the data did not achieve normality. These two iridescence measurements were analyzed separately using a GLM with an underlying quasi-Poisson distribution comparing the iridescence measurements across locations.
The relationship between the permanent band area, measured by Flanagan et al. (2016), and the iridescent signal of the band, measured using the IDIA in this study, was analyzed. Additionally, we tested whether this relationship was consistent across three of the different populations of S. scovelli. Mean band area measurements were obtained from Flanagan et al. (2016) for the South Padre, Texas population and both Florida locations (Anne’s Beach and Fort De Soto) to compare against corresponding mean band iridescence calculations. Seventeen pipefish were randomly selected for each of the three locations from the original dataset to obtain equal sample sizes for the analysis. Pearson correlation coefficients were first determined between mean band area and mean band iridescence independent of location. In addition, three subsets were created for each of the individual populations and Pearson correlation coefficients were found between mean band area and mean band iridescence for each location.
Non-pregnant male S. scovelli were collected from Redfish Bay near Aransas Pass, Texas (N 27 53 39.07, W 97 7 51.69) in July 2013 under the Texas Parks and Wildlife permit number SPR-0808-307. Fish were housed for 18 days and exposed to 5-10 ng/l 17α-ethinylestradiol (EE2) (n=8) using a solution of EE2 dissolved in ethanol described in Rose et al. (2015), or non-exposed in EE2-free saltwater with trace amounts of ethanol for the control (n=5). The males used in the control and experimental groups were sized matched, with no significant difference in length or depth, to ensure size was not impacting any reaction to the EE2. Water changes were conducted every other day replacing 10% of the water to maintain the EE2 levels, following protocols approved by the Institutional Animal Care and Use Committee at Texas A&M University and outlined in AUP # 2013–0020 (Reference #001898). The polygon tool approach was used to measure the overall iridescence on the first and last day of the study to detect morphological changes resulting from environmental contaminants. The overall iridescence value from Day 1 was subtracted from the final overall iridescence measurement to calculate an overall change in iridescence for males in both the estrogen-exposed and unexposed treatments. Overall iridescence measurements from the day 18 photographs of male S. scovelli exposed to estrogen were also compared to the overall iridescence measurements from unexposed female S. scovelli collected from the same location in Port Aransas, Texas. Due to a violation of normality, a Wilcoxon rank sum test was used to compare the difference in the iridescent signal expressed between the unexposed and exposed males as well as exposed males and unexposed females.
Twenty-four pipefish were originally caught and photographed; however, three presented too dark of a background coloration on the torso for the IDIA to confidently detect any iridescence and were thus removed from the analysis. Mean band and overall iridescence did not vary between the different lighting conditions (9 lumens = 0.099 ± 0.0033, 13 lumens = 0.099 ± 0.0028, 21 lumens = 0.107 ± 0.0022; One-way ANOVA: F2,60 = 2.29, p= 0.109); however, the highest level of lighting (21 lumens) was shown to detect greater overall iridescence compared to the others, but was not statistically significant (9 lumens = 3.93 ± 0.297, 13 lumens = 4.00 ± 0.314, 21 lumens = 4.89 ± 0.312; One-way ANOVA: F2,60 = 3.01, p= 0.057) (Figure 3). Further post hoc analysis of the overall iridescence showed that none of the pairwise comparisons were significantly different (Tukey HSD all p> 0.05). Variation of overall iridescence was highlighted through looking at the three iridescent measurements per lighting condition for each individual pipefish (Figure S1). Within each fish there was inherent variation of the signal as the boxplots representing the different lighting conditions were not always in line, with some cases more extreme than others (Figure S1B).
Figure 3 (A) Mean band iridescence does not significantly differ across the different lighting conditions (n=21), however, the (B) overall iridescence is nearly significant, likely due to the slightly higher levels measured at 21 lumens.
Using the IDIA, both overall iridescence (One-way ANOVA: F3,64 = 10.43, p< 0.0001) and torso iridescence (GLM: χ23 = 44.64, p< 0.0001) were found to be significantly different across the four geographic locations, however, mean band iridescence was not significantly different (GLM: χ23 = 7.61, p = 0.055) (Table 1; Figure 4A). For both the overall iridescence and torso iridescence, pipefish from Anne’s Beach, Florida expressed the highest levels of iridescence (Figures 4B, C respectively). Post hoc analysis via Tukey’s HSD test (Table S1) showed the overall iridescence on pipefish from Anne’s Beach was not significantly higher than the population from South Padre, Texas, but both populations had significantly higher overall iridescence than the other Florida population from Fort De Soto. The pipefish collected from Port Aransas, Texas showed no significant difference in overall iridescence when compared to any of the other populations (Table 1; Figure 4B). Torso iridescence did differ among populations, with the Anne’s Beach population having the highest expression levels compared to the other populations with overwhelmingly positive estimates from the GLMM (2.07 ± 0.096) while iridescence from the other populations had estimates that were substantially negative (FL Fort de Soto: -0.98 ± 0.18, TX South padre: -0.87 ± 0.18, TX Port Aransas: -0.54 ± 0.16). This confirmed initial visual observations made from photographs of pipefish (Figure 2).
Table 1 Summary of morphometrics (mean ± S.E.) including body depth, number of bands, mean band iridescence, overall iridescence, and torso iridescence for female S. scovelli across the four geographic locations and results of statistical analyses conducted.
Figure 4 The variation in (A) depth-adjusted mean band iridescence, (B) depth-adjusted overall iridescence, and (C) torso iridescence across four distinct populations of female S. scovelli. These populations include two from Florida [Anne’s Beach (FLAB) and Fort DeSoto (FLFD)] and two from the coast of Texas [Port Aransas (TXPA) and South Padre (TXSP)]. Mean band iridescence was not significant across the geographic locations and both overall iridescence and torso iridescence differed significantly between the different populations. Letters denote results from Tukey HSD post-hoc analysis; different letters represent significantly different populations (P<0.05). The asterisk above FLAB in (C) denotes the overwhelmingly positive estimates from the GLMM compared to the substantially negative estimates corresponding to the other three populations.
Independent of location, a weak correlation was found between mean permanent band area from Flanagan et al. (2014) and mean band iridescence measured using the IDIA, with the general positive trend of band area increasing as the iridescence increased (Pearson’s correlation: r = 0.37, df = 49, p = 0.0068). When the three populations were analyzed separately, the strongest relationships between mean band area and mean band iridescence were found in the South Padre, Texas population (Pearson’s correlation: r = 0.42, df = 15, p = 0.095) and the Fort de Soto, Florida population (Pearson’s correlation: r = 0.40, df = 15, p = 0.12), but neither of the relationships were statistically significant (Figure 5). Female S. scovelli from Anne’s Beach, Florida demonstrated the weakest relationship between the mean band area and mean band iridescence (Pearson’s correlation: r = 0.31, df = 15, p = 0.23) (Figure 5).
Figure 5 The relationship between mean band area and mean band iridescence for the two Florida populations (Anne’s Beach and Fort De Soto) and the South Padre, Texas population. The strongest relationships between band area and iridescence were found for South Padre and Fort de Soto, while female pipefish from Anne’s Beach showed the weakest correlation. Overall, none of the relationships were statistically significant (p>0.05).
The change in iridescence after an eighteen-day period was significantly higher for male pipefish exposed to synthetic estrogen compared to the control (Wilcoxon rank sum test: W=1, P= 0.0031) (Figure 6A). The larger change in iridescence observed for S. scovelli exposed to estrogen was due to the high values of iridescence detected in exposed males on day eighteen compared with their initial measurements on day one (Figures 6B, C), while the unexposed males did not develop iridescence. Although the overall iridescence detected on exposed males is significantly lower than the overall iridescence reported for females collected in the same Port Aransas location (Wilcoxon rank sum test: W = 59, p = 0.0030), many of the iridescence values for exposed males (0.82-6.0) fall within the range of iridescence levels (2.3-13.0) of wild caught, non-exposed females.
Figure 6 (A) Change in area of iridescence (± S.E.) for male pipefish exposed to synthetic estrogen (n=8) and male pipefish unexposed to estrogen (n=5). Exposed males showed a significant increase in iridescence. (B) Example of a male S. scovelli on Day 1 of the study and (C) that same male after 18 days of exposure to 5 ng/L of EE2.
This study highlighted the effectiveness of the Iridescence Detection and Isolation Algorithm (IDIA) as a method to quantify iridescent signaling. After testing the validity of the program, IDIA was used to highlight geographic variation in the expression of the iridescent signal and to detect the development of iridescence resulting from exposure to contaminants. The use of the program successfully across several applications indicates the potential for this program to be evaluated in other systems. While Syngnathus scovelli served as a model system for testing the algorithm in the current study, the IDIA can be adapted to measure iridescence in a variety of taxa by optimizing the ratios of blue, red, and green that the IDIA distinguishes as iridescent for additional species.
To address the reliability of the IDIA individual pipefish were photographed at three different lighting levels and both the overall and mean band iridescence was measured. The different light intensities did not significantly affect the amount of the mean band iridescence that was recognized by the IDIA; however, when calculating overall iridescence, the results were nearly significant. Although using the polygon tool eliminates human biases, the program will acknowledge any iridescence not located on the bands within the area of interest, regardless of if it is true iridescence located on the pipefish or slight imperfections of the photo. This increases the sensitivity of the polygon tool and may be the leading cause of the nearly significant values for overall iridescence measurements. Therefore, users of the IDIA should determine the amount of background noise that could possibly be detected by the polygon tool before choosing between using the mean versus overall measurement methods. For example, in the current study, the photographs were taken through a glass aquarium where glare was detected in some of the images, resulting in false iridescence. Any photographs that contained a glare overlaying a significant portion of the torso were eliminated from the study as accurate measurements could not be taken.
The repeatability of the IDIA was additionally tested to ensure the program is a reliable resource. At each level of lighting three photos were taken of the same pipefish. The nine photos were then analyzed for measurements of iridescence for each individual pipefish. To investigate the overall effect of lighting on iridescence, the iridescence measurements for the three photos were averaged, however, when looking at them individually it helps to highlight the strength of repeatability for the IDIA (Figure S1). We detected variation in the iridescent measurements within each pipefish, but the magnitude of that variation differs considerably across the pipefish. Aside from a few cases, the degree of variation in the iridescent measurements was minimal, indicating the proximity of the three measurements and thus the repeatability of the IDIA (Figure S1). Additionally, the variation between the lighting conditions is highlighted with the majority of the three boxplots for each individual clustering together emphasizing through visual analysis the reliability of the program. The fish that show the highest magnitudes of variation have a light-colored background. It is possible that it is not the iridescence changing at the different lighting conditions but the background of the fish that is altering slightly therefore indirectly affecting the iridescence measurement that is calculated.
The IDIA was shown to be a reliable way to confidently assess iridescence allowing for it to be used in more applied methods. In terms of the different ways in which iridescence was quantified, physically tracing the iridescence showed more consistency than using the polygon tool in ImageJ to draw around the area of interest (Figures 3, S1). However, the different methods measure different aspects of the ornamentation that may be biologically significant and therefore both must be kept in mind for further analyses. When outside of a laboratory setting, ensuring fully stable conditions is near impossible. To increase confidence and consistency of iridescent measurements it is recommended that all photographs are taken in consistent conditions when possible, to prevent issues such as reflection of the camera or the sheen on containers, whether it be a glass container or plastic bags containing water. Additionally, images of lower resolution, or taken in considerably lower light conditions, will not work with the algorithm because of increased pixelation of the bands themselves, as the ornaments of interest are so narrow. If the IDIA is to be applied for analyses using photos with a wider range of lighting or pixel sizes a reoptimization of the program would be required.
After verifying the capability of the IDIA, it was successfully used to measure variation in band, overall, and torso iridescence in female S. scovelli across several geographic locations. Investigating the mean band iridescence, overall iridescence, and torso iridescence of female pipefish from the four geographic locations highlighted differences in the way the iridescent signal may be used within each population. These differences were notably emphasized through the overall iridescence and torso iridescence as the mean band iridescence did not vary drastically across the distinct populations. For both the overall and torso iridescence the highest levels were found in the Anne’s Beach populations, as expected from initial observations of the photos (Figure 2). The high overall iridescence found in South Padre pipefish was initially puzzling as they do not contain the significant background iridescence found of Anne’s Beach pipefish. However, this can be explained by the fact that they have significantly more bands (Figure S2B), which in turn increases the overall iridescence values.
Both the iridescent signal detected through the IDIA and the physical area of the permanent bands contribute to the representation of the ornament on female S. scovelli. To further understand the role that ornamentation plays, both individual components and any interactions between them must be considered. Prior to measurements, it was observed that some of the bands located on the female pipefish were either not distinguished as iridescent through the IDIA or were found to be only partially iridescent. Therefore, just because the physical bands are present does not mean that just as much iridescence will be present. This was supported through investigating the strength of the relationship between mean band area and mean band iridescence. Overall, there is a positive trend, as the band area increases the iridescence also increases, however, there is a weak, nonsignificant correlation. Geographically, the strongest correlations were seen in pipefish from South Padre, Texas and Fort De Soto, Florida. Both populations possess females with iridescence primarily on the bands. Female pipefish from Anne’s Beach, Florida demonstrated the weakest relationship between the physical area of the bands and the iridescent signal, likely due to the high amount of torso iridescence they have.
Band area and band iridescence are represented differently in the ornamentation highlighting the potential for band area and iridescence to be responsible for different roles in the mating system of S. scovelli and for these roles to change and fluctuate geographically. To fully understand the ornamentation found on S. scovelli, all components, the geographic differences, the physical area of the permanent bands, and the iridescent signal, must be considered. Overall, the variability of this sexually selected signal is illustrated and prompts further questions about what is driving this variation. One large factor tied to the variation in this ornamentation is the difference in the environments of these pipefish. Components such as the density of the seagrass beds they reside in or turbidity levels and depth of the water may be affecting the signal expression. If the pipefish reside in particularly dense beds, such as the seagrass sampled in Anne’s Beach, a higher level of iridescence may be beneficial for the females to be seen by potential mates through the blades of seagrass. Contrastingly, pipefish residing in areas of low visibility, such as the turbid waters of the Texas coast, may reallocate energy from visual sexual signals into something such as chemical signals (Ratterman et al., 2009; Sundin et al., 2010; Sundin et al., 2016; Sundin et al., 2017). While sampling depth did not vary greatly across the locations sampled for pipefish, previous studies have shown that the different wavelengths available at a certain depth may also impact iridescent ornamentation (Chae and Nishida, 1995). Additionally, sexual selection pressures themselves have been shown to vary geographically in other species of pipefish (Mobley and Jones, 2007; Monteiro and Lyons, 2012), providing another possible explanation for the variation in the iridescent signal.
Although it is currently unknown which of the iridescent traits may be used by male S. scovelli in mate selection, previous work has established that the number of permanent light-colored bands and their size increases the reproductive success of the female (Flanagan et al., 2014). Therefore, each of the three iridescent measurements could play an important role in mate selection, and these roles may differ between populations. However, there is the possibility that the iridescent ornamentation acts more as an amplifier trait. Amplifier traits are ones that do not serve to signify quality themselves, but rather to enhance the assessment of other traits that do signal quality (Doucet and Meadows, 2009). A study done by Anderson and Jones (2019) demonstrated the tight relationship between the area of the permanent bands and body depth, with deeper bodied females possessing larger bands, but found that males choose mates based on their depth rather than the ornamentation. The permanent light-colored bands and bluish iridescent signal may then be responsible for emphasizing the size of the females and therefore indirectly signaling the quality of potential mates as larger females have the capacity for more or larger eggs.
The IDIA was successfully used to detect morphological changes in male S. scovelli as a response to environmental contaminants, specifically synthetic estrogen (17α-ethinylestradiol, EE2). The most prominent source for this contamination is discharge from wastewater treatment plants into bodies of water. Syngnathus scovelli can potentially reside in seagrass beds located near the wastewater treatment plants discharge making them vulnerable to the negative effects of estrogen exposure. Previous laboratory studies have shown levels of EE2 exposure as low as 5 ng/L leads to the feminization of male pipefish, including the development of iridescent bands that are typically only found in sexually mature females, production of egg precursor proteins, and difficulty reproducing (Rose et al., 2013b, 2015). With the IDIA, the development of those iridescent bands was used as a morphological indicator of exposure to levels of 5-10 ng/L of sythetic estrogen.
Iridescence measurements calculated from the IDIA for the exposed male S. scovelli resulted in numbers within the range of the quantity of iridescence found on female S. scovelli. It should be noted that pipefish were exposed to higher concentrations of EE2 (5-10 ng/L) for the purpose of showing the development of iridescent bands. Lower levels of EE2 (<5 ng/L) do not induce as drastic of a change morphologically in male S. scovelli (Rose et al., 2013b, 2015). In aquatic systems EE2 has been detected in concentrations as high as 820 ng/L in US rivers and 35 ng/L in certain European locations (Kolpin et al., 2002; Pojana et al., 2007) and lower ranges of 0.5 to 10 ng/L in locations worldwide (Allen et al., 1999; Johnson et al., 2000). The IDIA can detect changes in morphology at levels of 5-10 ng/L, suggesting that S. scovelli has potential as an ecotoxicology model system for monitoring estrogen contamination in aquatic environments. By developing a standard curve with known levels of EE2 and corresponding levels of iridescence measured with the IDIA, future studies can link morphological changes in iridescence on fish to specific levels of synthetic estrogen exposure. This approach offers a simple and non-invasive way to estimate levels of estrogen in aquatic systems from iridescence quantified in photographs of male pipefish, rather than more invasive and time-consuming methods, such as sacrificing the animals to measure hormone levels using blood plasma or RNA-sequencing or testing the surrounding waters. However, further work is necessary to determine the efficacy of this technique for monitoring the presence of environmental contaminants, such as synthetic estrogen. It is worth noting that synthetic estrogen is not the only environmental contaminant found in aquatic environments, and other contaminants may also elicit morphological changes in iridescence. Therefore, additional research is required to evaluate the usefulness of iridescence-based monitoring for detecting a range of contaminants in aquatic systems.
The combined applications of the Iridescence Detection and Isolation Algorithm (IDIA) in this study provide support that this program is a successful tool for measuring iridescence but highlight issues relating to measuring changing signals in live animals and in producing reliable photographs. S. scovelli have the ability to change their background coloration (i.e. increase or decrease contrast of torso color to the iridescent bands) in response to different circumstances. Changes in the contrast have been observed for the S. scovelli following death, in response to stress, and throughout courtship, as seen in other syngnathids (Lin et al., 2009; Sundin et al., 2016). Linked to this phenomenon is the possibility that these changes in background coloration are influencing the magnitude of the iridescent signal detected by the IDIA. While still unknown and unresearched, examples of this have been observed scattered throughout multiple laboratory experiments (Figure S3). The reproductive timing of the pipefish may also influence the quality of photographs taken in the aspect that observations of females across different reproductive stages highlight an increase in torso size and robustness at the later reproductive stages, likely due to the development of ovaries. This increase in size may influence the manner in which the pipefish lays against the material while photographing, possibly influencing the quality of the iridescent measurement.
Iridescence has been shown to play a multitude of important roles within an immense diversity of organisms. Because of this, it is important to understand why iridescence would have evolved as a signal to begin with. It can serve as a sexual signal to increase an organism’s fitness, as a “weapon” for intraspecies competition, or a method for a species to find its own kind. However, to ever understand fully any of the functionality, there must be a way in which it can be quantified. The Iridescence Detection and Isolation Algorithm has the potential to be adapted and utilized across a variety of taxa. To do so, the specific biology of the organism of interest must be well understood and the program re-optimized in response. With programs such as the IDIA, fine-scale variation in iridescent signal is picked up, deepening the comprehension of why iridescence is present and how it is potentially evolving in populations experiencing selection.
All code used to conduct the analysis and produce the figures is available on github (https://github.com/coley-tosto/Sscov_band_iri) and archived on zenodo (https://doi.org/10.5281/zenodo.7793993). Data are available at https://doi.org/10.5281/zenodo.7796404.
NT, ER, and HM contributed to study design and SF provided intellectual support. HM was involved in the original development and optimization of the IDIA with Gulf pipefish. All measurements of iridescence and subsequent analyses were performed by NT. Writing of the manuscript was done by NT, ER, SF, HM. All authors contributed to the article and approved the submitted version.
Funding for the experiment surrounding iridescence across lighting conditions was provided by the University of Tampa Office of Undergraduate Research and Inquiry to NT for her Summer Undergraduate Research Fellowship.
The authors would like to acknowledge Michael Masonjones for help with the development of the IDIA procedure combining MSAccess macros and pre-existing features of ImageJ, Angelica Vega-Higgins for help with the optimization of the IDIA, Bridgette Froeschke for her assistance with running the statistical models, and all of the undergraduate students from the University of Tampa that helped with field collection of the pipefish.
The authors declare that the research was conducted in the absence of any commercial or financial relationships that could be construed as a potential conflict of interest.
All claims expressed in this article are solely those of the authors and do not necessarily represent those of their affiliated organizations, or those of the publisher, the editors and the reviewers. Any product that may be evaluated in this article, or claim that may be made by its manufacturer, is not guaranteed or endorsed by the publisher.
The Supplementary Material for this article can be found online at: https://www.frontiersin.org/articles/10.3389/fmars.2023.1127790/full#supplementary-material
Allen Y., Scott A. P., Matthiessen P., Haworth S., Thain J. E., Feist S. (1999). Survey of estrogenic activity in united kingdom estuarine and coastal waters and its effects on gonadal development of the flounder Platichthys flesus. Environ. Toxicol. Chem. 18, 1791–1800. doi: 10.1002/etc.5620180827
Anderson A. P., Jones A. G. (2019). Choosy gulf pipefish males ignore age but prefer active females with deeply keeled bodies. Anim. Behav. 155, 37–44. doi: 10.1016/j.anbehav.2019.05.018
Anderson A. P., Rose E., Flanagan S. P., Jones A. G. (2020). The estrogen-responsive transcriptome of female secondary sexual traits in the gulf pipefish. J. Hered. 111, 294–306. doi: 10.1093/jhered/esaa008
Baldwin J., Johnsen S. (2012). The male blue crab, Callinectes sapidus, uses both chromatic and achromatic cues during mate choice. J. Exp. Biol. 215, 1184–1191. doi: 10.1242/jeb.067512
Bebié N., McElligott A. G. (2006). Female aggression in red deer: Does it indicate competition for mates? Mamm. Biol. 71, 347–355. doi: 10.1016/j.mambio.2006.02.008
Berglund A. (2000). Sex role reversal in a pipefish: female ornaments as amplifying handicaps. Ann. Zool. Fennici 37, 1–13.
Berglund A., Rosenqvist G., Bernet P. (1997). Ornamentation predicts reproductive success in female pipefish. Behav. Ecol. Sociobiol. 40, 145–150. doi: 10.1007/s002650050327
Berglund A., Rosenqvist G., Svensson I. (1986). Mate choice, fecundity and sexual dimorphism in two pipefish species (Syngnathidae). Behav. Ecol. Sociobiol. 19, 301–307. doi: 10.1007/BF00300646
Bernet P., Berglund A., Rosenqvist G. (1998). Female-female competition affects female ornamentation in the sex-role reversed pipefish syngnathus typhle. Behaviour 135, 535–550. doi: 10.1163/156853998792897923
Bitton P.-P., Dawson R. D. (2008). Age-related differences in plumage characteristics of male tree swallowsTachycineta bicolor: hue and brightness signal different aspects of individual quality. J. Avian Biol. 39, 446–452. doi: 10.1111/j.0908-8857.2008.04283.x
Chae J., Nishida S. (1995). Vertical distribution and die1 migration in the iridescent copepods of the family sapphirinidae: a unique example of reverse migration? Mar. Ecol. Prog. Ser. 119, 111–124. doi: 10.3354/meps119111
Clutton-Brock T. (2007). Sexual selection in males and females. Science 318, 1882–1885. doi: 10.1126/science.1133311
Clutton-Brock T. (2009). Sexual selection in females. Anim. Behav. 77, 3–11. doi: 10.1016/j.anbehav.2008.08.026
Clutton-Brock T. (2017). Reproductive competition and sexual selection. Philos. Trans. R. Soc Lond. B Biol. Sci. 372. doi: 10.1098/rstb.2016.0310
Cunha M. A. B., Berglund A., Monteiro N. M. (2017). Female ornaments signal own and offspring quality in a sex-role-reversed fish with extreme male parental care. Mar. Ecol. 38, e12461. doi: 10.1111/maec.12461
Darwin C. (1871). The descent of man, and selection in relation to sex (London, England: Murray) Vol. 1.
Dijkstra P. D., van Dijk S., Groothuis T. G. G., Pierotti M. E. R., Seehausen O. (2009). Behavioral dominance between female color morphs of a lake Victoria cichlid fish. Behav. Ecol. 20, 593–600. doi: 10.1093/beheco/arp036
Doucet S. M., Meadows M. G. (2009). Iridescence: a functional perspective. J. R. Soc. Interface 6 (Suppl 2), S115–S132. doi: 10.1098/rsif.2008.0395.focus
Doucet S. M., Shawkey M. D., Hill G. E., Montgomerie R. (2006). Iridescent plumage in satin bowerbirds: structure, mechanisms and nanostructural predictors of individual variation in colour. J. Exp. Biol. 209, 380–390. doi: 10.1242/jeb.01988
Emlen S. T., Oring L. W. (1977). Ecology, sexual selection, and the evolution of mating systems. Science 197, 215–223. doi: 10.1126/science.327542
Fitzstephens D. M., Getty T. (2000). Colour, fat and social status in male damselflies, Calopteryx maculata. Anim. Behav. 60, 851–855. doi: 10.1006/anbe.2000.1548
Flanagan S. P., Johnson J. B., Rose E., Jones A. G. (2014). Sexual selection on female ornaments in the sex-role-reversed gulf pipefish (Syngnathus scovelli). J. Evol. Biol. 27, 2457–2467. doi: 10.1111/jeb.12487
Flanagan S. P., Rose E., Jones A. G. (2016). Population genomics reveals multiple drivers of population differentiation in a sex-role-reversed pipefish. Mol. Ecol. 25, 5043–5072. doi: 10.1111/mec.13794
Gomez D., Théry M. (2007). Simultaneous crypsis and conspicuousness in color patterns: Comparative analysis of a Neotropical rainforest bird community. Am. Nat. 169, S42–S61. doi: 10.1086/510138
Gruson H., Andraud C., Daney de Marcillac W., Berthier S., Elias M., Gomez D., et al (2019). Quantitative characterization of iridescent colours in biological studies: a novel method using optical theory. J. R. Soc. Interface 9 (1). doi: 10.1098/rsfs.2018.0049
Hare R. M., Simmons L. W. (2019). Sexual selection and its evolutionary consequences in female animals. Biol. Rev. Camb. Philos. Soc 94, 929–956. doi: 10.1111/brv.12484
Johnson A. C., Belfroid A., Di Corcia A. D. (2000). Estimating steroid oestrogen inputs into activated sludge treatment works and observations on their removal from the effluent. Sci. Total Environ. 256, 163–173. doi: 10.1016/S0048-9697(00)00481-2
Jones A. G., Ratterman N. L. (2009). Mate choice and sexual selection: what have we learned since Darwin? Proc. Natl. Acad. Sci. U. S. A. 106 (Suppl 1), 10001–10008. doi: 10.1073/pnas.0901129106
Jones A. G., Rosenqvist G., Berglund A., Avise J. C. (2005). The measurement of sexual selection using bateman’s principles: an experimental test in the sex-role-reversed pipefish Syngnathus typhle. Integr. Comp. Biol. 45, 874–884. doi: 10.1093/icb/45.5.874
Jones A. G., Walker D., Avise J. C. (2001). Genetic evidence for extreme polyandry and extraordinary sex-role reversal in a pipefish. Proc. Biol. Sci. 268, 2531–2535. doi: 10.1098/rspb.2001.1841
Kemp D. J., Vukusic P., Rutowski R. L. (2006). Stress-mediated covariance between nano-structural architecture and ultraviolet butterfly coloration. Funct. Ecol. 20, 282–289. doi: 10.1111/j.1365-2435.2006.01100.x
Kirkpatrick M. (1982). Sexual selection and the evolution of female choice. Evolution 36, 1–12. doi: 10.2307/2407961
Kirkpatrick M., Price T., Arnold S. J. (1990). The Darwin-Fisher theory of sexual selection in monogamous birds. Evolution 44, 180–193. doi: 10.2307/2409533
Kolpin D. W., Furlong E. T., Meyer M. T., Thurman E. M., Zaugg S. D., Barber L. B., et al. (2002). Pharmaceuticals, hormones, and other organic wastewater contaminants in U.S. streams 1999-2000: a national reconnaissance. Environ. Sci. Technol. 36, 1202–1211. doi: 10.1021/es011055j
Lim M. L. M., Li D. (2006). Behavioural evidence of UV sensitivity in jumping spiders (Araneae: Salticidae). J. Comp. Physiol. A Neuroethol. Sens. Neural Behav. Physiol. 192, 871–878. doi: 10.1007/s00359-006-0126-5
Lim M. L. M., Li D. (2007). Effects of age and feeding history on structure-based UV ornaments of a jumping spider (Araneae: Salticidae). Proc. Biol. Sci. 274, 569–575. doi: 10.1098/rspb.2006.0020
Lin Q., Lin J., Huang L. (2009). Effects of substrate color, light intensity and temperature on survival and skin color change of juvenile seahorses, Hippocampus erectus. Aquaculture 298, 157–161. doi: 10.1016/j.aquaculture.2009.10.015
Lipshutz S. E. (2017). Divergent competitive phenotypes between females of two sex-role-reversed species. Behav. Ecol. Sociobiol. 71, 106. doi: 10.1007/s00265-017-2334-0
Loyau A., Gomez D., Moureau B., Théry M., Hart N. S., Jalme M. S. (2007). Iridescent structurally based coloration of eyespots correlates with mating success in the peacock. Behav. Ecol. 18, 1123–1131. doi: 10.1093/beheco/arm088
Masonjones H. D., Rose E., McRae L. B., Dixson D. L. (2010). An examination of the population dynamics of syngnathid fishes within Tampa bay, Florida,USA. Curr. Zool. 56, 118–133. doi: 10.1093/czoolo/56.1.118
McGraw K. J., Mackillop E. A., Dale J., Hauber M. E. (2002). Different colors reveal different information: how nutritional stress affects the expression of melanin- and structurally based ornamental plumage. J. Exp. Biol. 205, 3747–3755. doi: 10.1242/jeb.205.23.3747
Mobley K. B., Jones A. G. (2007). Geographical variation in the mating system of the dusky pipefish (Syngnathus floridae). Mol. Ecol. 16, 2596–2606. doi: 10.1111/j.1365-294X.2007.03337.x
Mobley K. B., Morrongiello J. R., Warr M., Bray D. J., Wong B. B. M. (2018). Female ornamentation and the fecundity trade-off in a sex-role reversed pipefish. Ecol. Evol. 8, 9516–9525. doi: 10.1002/ece3.4459
Monteiro N. M., Carneiro D., Antunes A., Queiroz N., Vieira M. N., Jones A. G. (2017). The lek mating system of the worm pipefish (Nerophis lumbriciformis): a molecular maternity analysis and test of the phenotype-linked fertility hypothesis. Mol. Ecol. 26, 1371–1385. doi: 10.1111/mec.13931
Monteiro N. M., Lyons D. O. (2012). Stronger sexual selection in warmer waters: the case of a sex role reversed pipefish. PloS One 7, e44251. doi: 10.1371/journal.pone.0044251
Moore A. J. (1990). The evolution of sexual dimorphism by sexual selection: the separate effects of intrasexual selection and intersexual selection. Evolution 44, 315–331. doi: 10.2307/2409410
Muniz D. G., Machado G. (2018). Mate sampling influences the intensity of sexual selection and the evolution of costly sexual ornaments. J. Theor. Biol. 447, 74–83. doi: 10.1016/j.jtbi.2018.03.026
Partridge C., Boettcher A., Jones A. G. (2010). Short-term exposure to a synthetic estrogen disrupts mating dynamics in a pipefish. Horm. Behav. 58, 800–807. doi: 10.1016/j.yhbeh.2010.08.002
Partridge C., Boettcher A., Jones A. G. (2013). The role of courtship behavior and size in mate preference in the sex-role-reversed gulf pipefish, Syngnathus scovelli. Ethology 119, 692–701. doi: 10.1111/eth.12110
Pojana G., Gomiero A., Jonkers N., Marcomini A. (2007). Natural and synthetic endocrine disrupting compounds (EDCs) in water, sediment and biota of a coastal lagoon. Environ. Int. 33, 929–936. doi: 10.1016/j.envint.2007.05.003
Powell D. M. (2008). Female–female competition or male mate choice? patterns of courtship and breeding behavior among feral horses (Equus caballus) on assateague island. J. Ethol. 26, 137–144. doi: 10.1007/s10164-007-0043-2
Pröhl H., Hödl W. (1999). Parental investment, potential reproductive rates, and mating system in the strawberry dart-poison frog, dendrobates pumilio. Behav. Ecol. Sociobiol. 46, 215–220. doi: 10.1007/s002650050612
Ratterman N. L., Rosenthal G. G., Jones A. G. (2009). Sex recognition via chemical cues in the sex-role-reversed gulf pipefish (Syngnathus scovelli). Ethology 115, 339–346. doi: 10.1111/j.1439-0310.2009.01619.x
Rillich J., Buhl E., Schildberger K., Stevenson P. A. (2009). Female crickets are driven to fight by the male courting and calling songs. Anim. Behav. 77, 737–742. doi: 10.1016/j.anbehav.2008.12.009
Rometsch S. J., Torres-Dowdall J., Machado-Schiaffino G., Karagic N., Meyer A. (2021). Dual function and associated costs of a highly exaggerated trait in a cichlid fish. Ecol. Evol. 11, 17496–17508. doi: 10.1002/ece3.8383
Rose E., Flanagan S. P., Jones A. G. (2015). The effects of synthetic estrogen exposure on the sexually dimorphic liver transcriptome of the sex-Role-Reversed gulf pipefish. PloS One 10, e0139401. doi: 10.1371/journal.pone.0139401
Rose E., Paczolt K. A., Jones A. G. (2013a). The contributions of premating and postmating selection episodes to total selection in sex-role-reversed gulf pipefish. Am. Nat. 182, 410–420. doi: 10.1086/671233
Rose E., Paczolt K. A., Jones A. G. (2013b). The effects of synthetic estrogen exposure on premating and postmating episodes of selection in sex-role-reversed gulf pipefish. Evol. Appl. 6, 1160–1170. doi: 10.1111/eva.12093
Rosenqvist G., Berglund A. (2011). Sexual signals and mating patterns in syngnathidae. J. Fish Biol. 78, 1647–1661. doi: 10.1111/j.1095-8649.2011.02972.x
Sæther S. A. (2002). Female calls in lek-mating birds: indirect mate choice, female competition for mates, or direct mate choice? Behav. Ecol. 13, 344–352. doi: 10.1093/beheco/13.3.344
Schneider C. A., Rasband W. S., Eliceiri K. W. (2012). NIH Image to ImageJ: 25 years of image analysis. Nat. Methods 9, 671–675. doi: 10.1038/nmeth.2089
Silberglied R. E., Taylor O. R. (1978). Ultraviolet reflection and its behavioral role in the courtship of the sulfur butterflies Colias eurytheme and C. philodice (Lepidoptera, pieridae). Behav. Ecol. Sociobiol. 3, 203–243. doi: 10.1007/BF00296311
Silva K., Vieira M. N., Almada V. C., Monteiro N. M. (2010). Reversing sex role reversal: compete only when you must. Anim. Behav. 79, 885–893. doi: 10.1016/j.anbehav.2010.01.001
Sogabe A., Ahnesjö I. (2011). The ovarian structure and mode of egg production in two polygamous pipefishes: a link to mating pattern. J. Fish Biol. 78, 1833–1846. doi: 10.1111/j.1095-8649.2011.02973.x
Sundin J., Aronsen T., Rosenqvist G., Berglund A. (2017). Sex in murky waters: algal-induced turbidity increases sexual selection in pipefish. Behav. Ecol. Sociobiol. 71, 1–8. doi: 10.1007/s00265-017-2310-8
Sundin J., Berglund A., Rosenqvist G. (2010). Turbidity hampers mate choice in a pipefish. Ethology 8, 713–721. doi: 10.1111/j.1439-0310.2010.01787.x
Sundin J., Rosenqvist G., Myhren S., Berglund A. (2016). Algal turbidity hampers ornament perception, but not expression, in a sex-Role-Reversed pipefish. Ethology 122, 215–225. doi: 10.1111/eth.12461
Számadó S., Penn D. J. (2015). Why does costly signalling evolve? challenges with testing the handicap hypothesis. Anim. Behav. 110, e9–e12. doi: 10.1016/j.anbehav.2015.06.005
Trail P. W. (1990). Why should lek-breeders be monomorphic? Evolution 44, 1837–1852. doi: 10.1111/j.1558-5646.1990.tb05254.x
Venables W. N., Ripley B. D. (2002). Modern applied statistics with s, 4th ed. (New York: Springer).
Vincent A., Ahnesjö I., Berglund A., Rosenqvist G. (1992). Pipefishes and seahorses: Are they all sex role reversed? Trends Ecol. Evol. 7, 237–241. doi: 10.1016/0169-5347(92)90052-D
West-Eberhard M. J. (1979). Sexual selection, social competition, and evolution. Proc. Am. Philos. Soc 123, 222–234.
Keywords: iridescence, sexual selection, ornamentation, pipefish, secondary sexual traits, Syngnathus scovelli, ecotoxicology
Citation: Tosto NM, Rose E, Flanagan SP and Mason HD (2023) The development of a quantification method for measuring iridescence using sexually selected traits in the Gulf pipefish (Syngnathus scovelli). Front. Mar. Sci. 10:1127790. doi: 10.3389/fmars.2023.1127790
Received: 20 December 2022; Accepted: 28 March 2023;
Published: 20 April 2023.
Edited by:
Douglas H. Adams, Florida Fish and Wildlife Conservation Commission, United StatesReviewed by:
José Pedro Andrade, University of Algarve, PortugalCopyright © 2023 Tosto, Rose, Flanagan and Mason. This is an open-access article distributed under the terms of the Creative Commons Attribution License (CC BY). The use, distribution or reproduction in other forums is permitted, provided the original author(s) and the copyright owner(s) are credited and that the original publication in this journal is cited, in accordance with accepted academic practice. No use, distribution or reproduction is permitted which does not comply with these terms.
*Correspondence: Heather D. Mason, aG1hc29uQHV0LmVkdQ==
Disclaimer: All claims expressed in this article are solely those of the authors and do not necessarily represent those of their affiliated organizations, or those of the publisher, the editors and the reviewers. Any product that may be evaluated in this article or claim that may be made by its manufacturer is not guaranteed or endorsed by the publisher.
Research integrity at Frontiers
Learn more about the work of our research integrity team to safeguard the quality of each article we publish.