- 1Arabian Center for Climate and Environmental Sciences, New York University Abu Dhabi, Abu Dhabi, United Arab Emirates
- 2Sorbonne Université (CNRS/IRD/MNHN), LOCEAN-IPSL, Paris, France
The Arabian Sea is an exceptionally complex system that hosts a highly productive marine ecosystem. This intense productivity leads to high oxygen consumption at depth that maintains, together with the sluggish circulation, the world’s thickest oxygen minimum zone (OMZ). While observations have been scarce in the region, evidence for a recent (1960-2020) decline in oxygen is emerging in the northern Arabian Sea. However, in the longer term (2050 to 2100) the future evolution of the OMZ is more uncertain, as the model projections that have been carried out are not consistent with each other. On the one hand, this reflects the limitations of current generation models that do not adequately represent key physical and biogeochemical processes, resulting in large O2 biases in the region under present-day conditions. On the other hand, the inherent difficulty of predicting future O2 conditions in the Arabian Sea is a consequence of the sensitivity of O2 supply and consumption to local and remote changes that evolve on different timescales. Here we aim to synthesize current knowledge of the Arabian Sea OMZ in relation to important factors controlling its intensity and review its recent change and potential future evolution. In particular, we explore potential causes of the differences in recent and future O2 trends in the region and identify key challenges to our ability to project future OMZ changes and discuss ideas for the way forward.
1 Introduction
Ocean currents and mixing supply dissolved oxygen to water masses at depth while organic matter degradation consumes it. In the Arabian Sea, as in other oceanic regions where biological productivity is high and ventilation is weak, the inefficient replenishment of oxygen depleted by intense remineralization results in the presence of a poorly oxygenated water body at depth, known as oxygen minimum zone (OMZ). While moderate oxygen deficits (hypoxia, usually defined as O2 lower than 60 mmol m-3) challenge the survival of higher trophic animals such as crustaceans and fishes (Vaquer-Sunyer and Duarte, 2008), near complete O2 depletion (suboxia, defined here as O2 lower than 4 mmol m-3) favors denitrification, a type of anaerobic respiration where nitrate is used as an electron acceptor. This not only depletes the inventory of bio-available nitrogen, critical for phytoplankton growth, but also releases N2O, a potent greenhouse gas (Codispoti et al., 2001; Bange et al., 2005; Gruber, 2008).
The warming of the ocean waters decreases dissolved O2 solubility, enhances respiration-induced oxygen consumption and strengthens vertical stratification, thus weakening the oxygen replenishment of the ocean interior (Oschlies et al., 2018). These changes together cause the ocean to lose oxygen as it warms up, a process known as ocean deoxygenation (Keeling et al., 2010; Robinson, 2019). The ocean lost 2% of its O2 between 1960 and 2010 (Ito et al., 2017; Schmidtko et al., 2017). Previous studies attribute most of this oxygen decline to changes in ocean ventilation and to a lesser extent reduced solubility, with biogeochemical processes playing a potentially significant role only in the low-latitude low O2 regions (Bindoff et al., 2019; Couespel et al., 2019; Oschlies, 2019; Buchanan and Tagliabue, 2021). Deoxygenation is expected to accelerate in the future with the ocean losing about 3-4% of its O2 inventory by 2100 under the high-emission scenario, with most of this loss concentrated in the upper 1000 m (Long et al., 2019). Even if the emissions were to stop, deoxygenation will continue over centuries in the deep ocean (Long et al., 2019) as the decline of O2 committed by historical emissions is estimated to be 3 times larger than current O2 loss (Oschlies, 2021). In the tropical thermocline, ocean deoxygenation has been shown to cause the expansion and shoaling of OMZs (Stramma et al., 2008; Stramma et al., 2010; Breitburg et al., 2018; Bindoff et al., 2019; Zhou et al., 2022). This can lead to a substantial reduction in habitat of sensitive marine organisms, including commercial fish species such as tunas and billfishes (Stramma et al., 2012b). Oxygen decline can also increase the frequency and severity of hypoxic conditions in the ocean, challenging the health of various ecosystems and causing important loss of marine biodiversity and shifts in the food web structure (Rabalais et al., 2002; Laffoley and Baxter, 2019; Hughes et al., 2020). Observational evidence suggests that even slight changes in dissolved oxygen can profoundly alter OMZ plankton community composition (Wishner et al., 2018; Goes et al., 2020).
In the Arabian Sea, observations as well as model simulations point toward a decline in oxygen in the region over the recent decades (Banse et al., 2014; Rixen et al., 2014; Piontkovski and Al-Oufi, 2015; Ito et al., 2017; Schmidtko et al., 2017; Queste et al., 2018; Buchanan and Tagliabue, 2021; Lachkar et al., 2021; Zhang et al., 2022; Hood et al., 2023). Yet, model-based future projections show insignificant future O2 changes (and even oxygenation in the central and southern sectors) by the end of the century (2080-2100) (Bopp et al., 2013; Kwiatkowski et al., 2020). Thus, recent and future trends in O2 in the region appear inconsistent for reasons that remain unclear. Here, we explore the important processes controlling the OMZ intensity as well as recent and future trends and discuss factors potentially contributing to this apparent conundrum. We also highlight and discuss the sources of major uncertainties tainting the future projections of the Arabian Sea OMZ. More specifically, in section 2, we overview the main factors controlling O2 demand and supply in the Arabian Sea, and use a Lagrangian analysis to guide our discussion of the sources and timescales of the ventilation of the OMZ. In section 3, we review the recent changes in the Arabian Sea OMZ as inferred from recent observational and modeling studies. In section 4, we discuss the potential future changes in the Arabian Sea OMZ, while focusing on the key mechanisms controlling these changes as well as their timescales and the uncertainties around their relative importance and interaction. Finally, in section 5, we discuss the differences between recent and future O2 trends and their potential causes and summarize the key challenges to our ability to predict future Arabian Sea OMZ changes as well as explore ideas for a way forward.
2 The Arabian Sea oxygen minimum zone
The Arabian Sea hosts the world’s thickest OMZ thanks to a combination of large remineralization fluxes associated with intense summer and winter blooms together with a sluggish circulation, particularly in the northern and eastern portions of the basin. Suboxia at the Arabian Sea OMZ core favors intense denitrification fluxes representing up to 20-30% of global pelagic nitrogen loss despite occupying less than 2% of the World Ocean area (Dueser et al., 1978; Naqvi et al., 1982; Gupta and Naqvi, 1984; Naqvi, 1987; Bange et al., 2005; Hood et al., 2023). As the intensity of the Arabian Sea OMZ is set by the magnitude of oxygen demand (remineralization) and supply (ventilation) in the region, we next explore the main factors controlling the two terms of this balance (Figure 1).
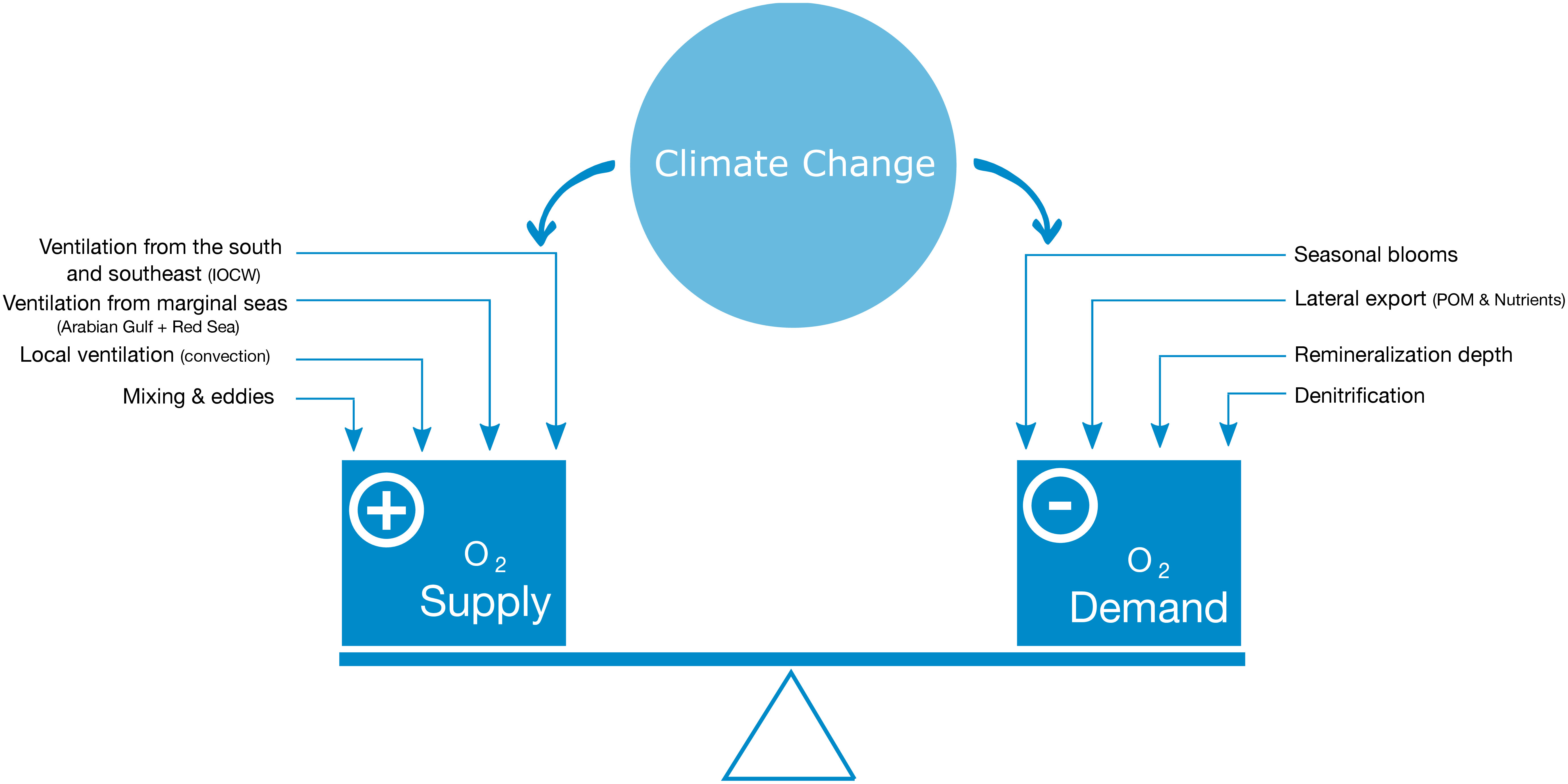
Figure 1 Factors controlling the Arabian Sea OMZ intensity. Main factors controlling the O2 supply (left pan of the balance) and demand (right pan of the balance) under the influence of climate change that may offset the balance, favoring deoxygenation or oxygenation.
2.1 Factors controlling O2 demand in the Arabian Sea OMZ
O2 consumption in the Arabian Sea OMZ is affected by various factors. Here we review the role of four major mechanisms, namely: (i) the magnitude of seasonal blooms, (ii) the lateral advection of organic matter and nutrients from the productive western Arabian Sea to the central and eastern sectors, (iii) the depth of remineralization and (iv) the intensity of denitrification (Figure 1).
The summer southwesterly winds drive upwelling along the coasts of Oman and Somalia whereas the winter northeasterly winds induce convective mixing in the northern Arabian Sea. Both mechanisms transport nutrients from the deep layers into the well-lit upper ocean, causing two seasonal phytoplankton blooms (Banse and McClain, 1986; Madhupratap et al., 1996; Wiggert et al., 2005; Lévy et al., 2007; Resplandy et al., 2012; Lachkar et al., 2018). Although previous studies suggest that nitrogen is generally the most limiting nutrient of biological productivity in the Arabian Sea, silicate and more importantly iron have also been suggested to limit productivity locally (Naqvi et al., 2002; Wiggert et al., 2006; Moffett et al., 2007; Wiggert and Murtugudde, 2007; Koné et al., 2009; Naqvi et al., 2010; Resplandy et al., 2011; Moffett et al., 2015; Chinni and Singh, 2022). Indeed, previous works indicate that the region off the coasts of Somalia and Oman is prone to iron limitation during both summer and winter monsoon seasons (Wiggert et al., 2006; Wiggert and Murtugudde, 2007; Moffett and Landry, 2020). A recent modeling study by Guieu et al. (2019) suggests that around half of summer primary production in the Arabian Sea is dependent on iron supply through aeolian dust deposition, confirming the critical role that iron may play in limiting biological production in the Arabian Sea. Iron limitation was also suggested to contribute to the weak and delayed response of diatoms to upwelling (Moffett and Landry, 2020). Finally, interannual variability in dust driven iron fluxes was hypothesized to contribute to interannual variability in Arabian Sea productivity (Moffett and Landry, 2020).
The lateral advection of organic matter and unutilized nutrients from the upwelling zone into the central sectors of the Arabian Sea have been shown to affect the intensity of the OMZ there (McCreary et al., 2013; Moffett and Landry, 2020). For instance, McCreary et al. (2013) investigated the role of lateral advection and transport of organic matter in controlling the oxygen distribution in the Arabian Sea. Their modeling work highlights the importance of lateral transport of organic matter from the western Arabian Sea into the central and eastern sectors of the Arabian Sea, where it enhances remineralization and contributes to lowering O2 levels to below suboxic thresholds. A recent analysis of back-scatter data in the northern Arabian Sea also supports that cross-shelf transport of organic matter is linked to the intensification of the OMZ in the eastern Arabian Sea (Sarma et al., 2020). This transport is likely dominated by eddies. Indeed, mesoscale eddies and submesoscale filaments have been shown to play a critical role in advecting nutrients from the coastal upwelling region into the oligotrophic central Arabian Sea (e.g. Koné et al., 2009; Resplandy et al., 2011).
The depth of remineralization, defined as the depth at which sinking organic matter is decomposed back to inorganic carbon and nutrients (Kwon et al., 2009; Cavan et al., 2017), is an additional factor modulating the Arabian Sea OMZ intensity. For instance, early observational studies showed evidence that the aggregation of organic matter with lithogenic particles from rivers increases the efficiency of the export fluxes in the Bay of Bengal relative to the Arabian Sea (Nair et al., 1989; Ittekkot et al., 1991; Rao et al., 1994). In a modeling study, Al Azhar et al. (2016) have demonstrated that the shallower remineralization depth in the Arabian Sea relative to the Bay of Bengal (driven by relatively slower particle sinking speeds in the former) contributes to enhancing the OMZ there. Indeed, the absence of a major source of ballast minerals similar to the one associated with the large riverine input in the Bay of Bengal decreases the particle sinking speed, thus increasing the organic matter residence time in the OMZ layer, and hence enhancing oxygen consumption there.
Finally, the biological consumption of O2 is reduced at low-O2 levels as anaerobic respiration (canonical denitrification and anaerobic ammonium oxidation) is favored over aerobic respiration (Oschlies et al., 2019; Rixen et al., 2020). Thus, increasing denitrification (in case of the expansion of the OMZ suboxic core) can act as a relative source of O2 that stabilizes the OMZ and limits its intensification (negative feedback) (Lachkar et al., 2016; Lachkar et al., 2019). An additional mechanism through which denitrification acts on O2 is via its impact on nitrogen inventory. Indeed, denitrification generates deficits in bio-available nitrogen that may not be compensated by N2 fixation on short timescales (Naqvi, 2008; Oschlies et al., 2019). This can lead to a reduction in biological productivity and respiration, thus limiting O2 consumption (Altabet et al., 1995). Under such conditions, an eventual expansion of the volume of suboxic waters in the OMZ core can be accompanied by a reduction of the volume of hypoxic waters (Deutsch et al., 2011; Lachkar et al., 2016).
2.2 O2 supply to the Arabian Sea OMZ: sources and timescales
The ventilation of the Arabian Sea OMZ is sensitive to both basin-scale circulation as well as to local mixing processes (Figure 1). Previous studies point to the Indian Ocean Central Water (IOCW) and the Persian (Arabian) Gulf Water (PGW) as two major sources of ventilation of the OMZ (Wyrtki et al., 1971; Gupta and Naqvi, 1984; Resplandy et al., 2012; McCreary et al., 2013; Acharya and Panigrahi, 2016; Lachkar et al., 2019). IOCW forms through convective mixing as Subantarctic Mode Water in the southern Indian Ocean and is advected northward into the OMZ as a part of the Somali Current. The relatively oxygen-rich PGW subducts in the northern Arabian Sea after its outflow from the Gulf and is exported into the Arabian Sea OMZ (Rixen et al., 2005; Lachkar et al., 2019; Schmidt et al., 2020; Schmidt et al., 2021). Moreover, waters from both the Red Sea and the Indonesian throughflow have been suggested to influence properties of the Arabian Sea thermocline (McCreary et al., 2013; Lachkar et al., 2019). Finally, winter convective mixing in the northern Arabian Sea is a well-known mechanism of local ventilation of the upper OMZ (Resplandy et al., 2012; McCreary et al., 2013).
Here we present the results of a quantitative analysis of the sources and timescales of the Arabian Sea OMZ ventilation based on a Lagrangian particle tracking approach (Supplementary Information). OMZ water particles are traced back to five ventilation source entries: namely the southern entry at 5°S (south), the south-eastern entry at 78°E (southeast), the Bab El Mendeb (Red Sea), the Strait of Hormuz (Arabian Gulf) and the base of the winter mixed layer (local ventilation) (Figure 2). This analysis confirms that the ventilation of the OMZ is dominated by the inflow of IOCW from the south as nearly of OMZ water parcels originate from the southern source (Figure 2A). The analysis of the east-west distribution of particles at the southern entry section (5°S) further indicates that they are mostly associated with waters advected along the western Arabian Sea (west of 42°E) (Figure S1 in Supplementary Information). The remaining sources (southeastern, local ventilation and marginal seas) play a substantially smaller role. However, when considering the upper OMZ only (100-300 m), the contribution of the southwestern source to the OMZ ventilation becomes considerably smaller (45%). Conversely, the contributions of the remaining ventilation sources are twice to three times larger (Figure 2A). The analysis of the depth profiles of the source waters at their original locations as well as when they enter the OMZ reveals that the dominance of the southwestern source is established mostly for the deeper layers of the OMZ (300-1500 m), whereas in the upper layer (100-300m) the contribution of the remaining sources is of comparable magnitude (Figures 2B, C). Furthermore, the analysis of ventilation timescales associated with the different sources reveal that despite its relatively smaller contribution in terms of water volume, the Arabian Gulf has a ventilation timescale (a few months) that is around two orders of magnitude shorter than that associated with the inflow of IOCW from the south (a few decades) (Figure 2D). Thus, despite its modest contribution to the ventilation on decadal timescales, the Arabian Gulf is a more efficient source of ventilation on shorter timescales (months to years). This implies that the Arabian Sea OMZ is likely to respond more rapidly to changes in the Arabian Gulf water properties (e.g., changes in O2 or water density) than to changes involving the transport of IOCW or its O2 content (Table 1).
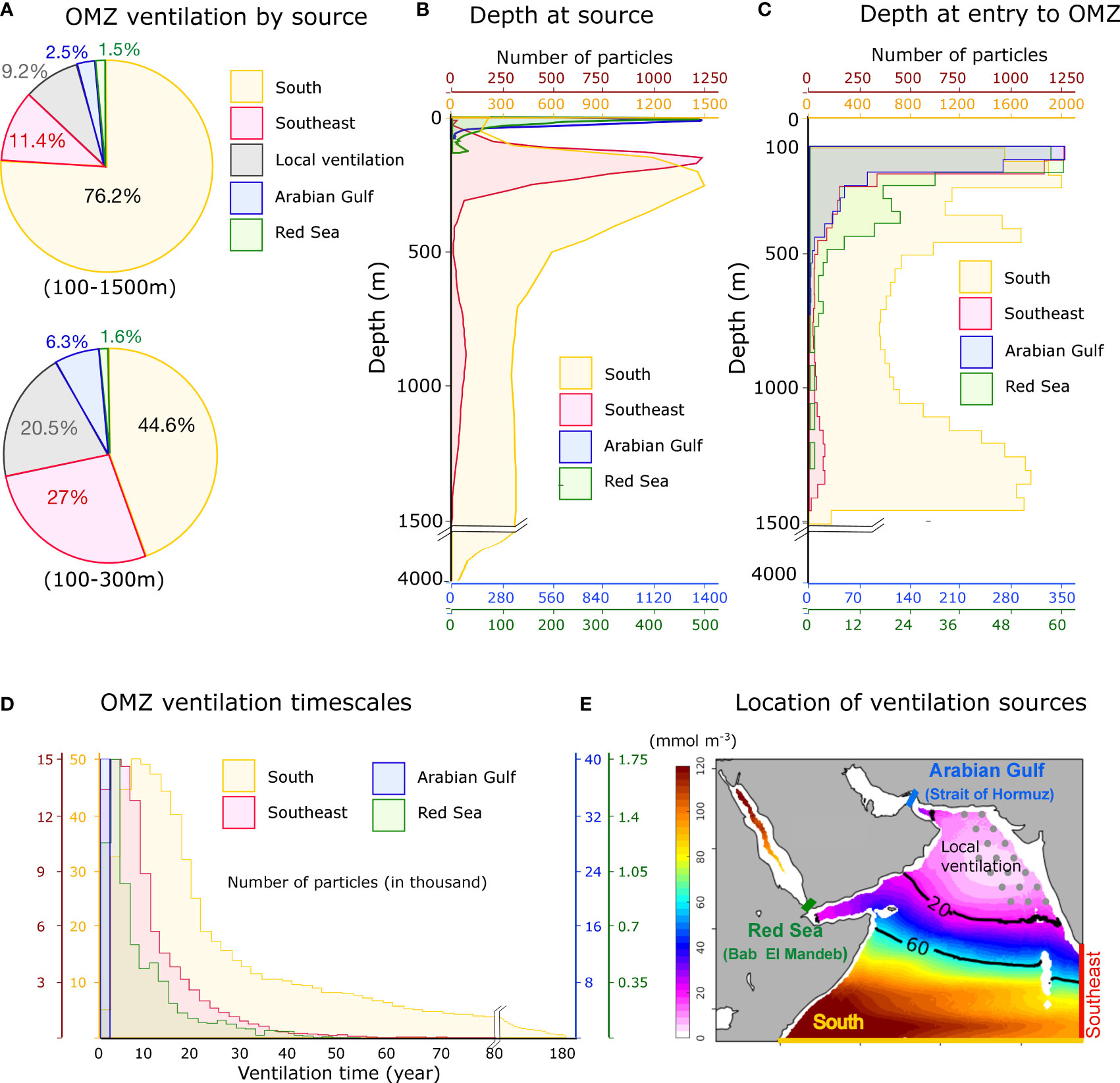
Figure 2 Arabian Sea OMZ ventilation sources and timescales. (A) Contributions of different source waters to the ventilation of the Arabian Sea OMZ (100-1500 m) and its upper part (100-300 m). The OMZ waters are linked through Lagrangian tracking to five potential sources associated with five sections shown in (E): south (at 5°N), southeast (at 78°E), the Arabian Gulf (Strait of Hormuz), the Red Sea (Bab El Mandeb) and the local ventilation (winter convection). (B, C) Particle depth at source (B) and at entry to the OMZ (C) associated with different remote sources. (D) Frequency distribution of OMZ ventilation timescales (in year) associated with different remote sources. Note that given the differences in the number of particles associated with each source, different sources are shown on different vertical axes in (B–D). (E) Location of the entry sections where source particles are intercepted. Grey circles indicate the area of local ventilation (where the OMZ core gets in contact with the winter mixed layer). Color shading shows annual mean O2 (in mmol m-3) averaged between 250 and 700 m.
Because of the enhanced ventilation of the western Arabian Sea from the IOCW and the PGW, the Arabian Sea OMZ is more intense in the eastern portion of the basin despite the productivity being highest along the western side (Morrison et al., 1999; Resplandy et al., 2012; McCreary et al., 2013; Rixen et al., 2014; Acharya and Panigrahi, 2016; Zhang et al., 2022). This feature, previously referred to as the eastward shift of the AS OMZ is also presumably aided by eddy-driven lateral transport of organic matter and unutilized nutrients from the western to the central Arabian Sea that contributes to enhancing respiration and lowering O2 concentrations there below suboxic thresholds (McCreary et al., 2013). Moreover, intense vertical and lateral eddy mixing in the western Arabian Sea (Resplandy et al., 2012; McCreary et al., 2013; Lachkar et al., 2016) play a crucial role in supplying O2 to the western Arabian Sea.
Finally, isopcynal and diapycnal mixing have been shown to strongly contribute to OMZ ventilation (Gnanadesikan et al., 2012; Lévy et al., 2022). In the Arabian Sea, model simulations suggest that in the absence of oxygen supplied by eddy mixing, the volume of the Arabian Sea OMZ would double (Lachkar et al., 2016). Changes in mixing have also been shown to dominate future projected deoxygenation in global models (Couespel et al., 2019; Lévy et al., 2022).
3 Past changes in the Arabian Sea OMZ
3.1 Past variability in the Arabian Sea OMZ from paleo-records
Paleo-reconstructions suggest important past changes in the intensity of the Arabian Sea OMZ over different periods and on timescales ranging from decades to millennia (Altabet et al., 1995; Altabet et al., 1999; Altabet et al., 2002; Gupta et al., 2003; Singh et al., 2011). For instance, Altabet et al. (1999) suggested that denitrification in the Arabian Sea was greatest during interglacial periods and probably ceased during most glacial phases. Additionally, evidence from paleo-records suggests an intensification of the OMZ core from the mid to late Holocene (Das et al., 2017). Previous studies attributed this to both large-scale ventilation changes as well as increased monsoon intensity and export fluxes (Schulz et al., 1998; Pichevin et al., 2007; Böning and Bard, 2009; Rixen et al., 2014; Das et al., 2017; Gaye et al., 2018; Joshi et al., 2021). On shorter timescales, Altabet et al. (2002) established a strong correspondence between changes in denitrification (and OMZ intensity) in the Arabian Sea and century-scale Dansgaard–Oeschger events during the last glacial period, with denitrification increasing during warm periods concurrently with the summer monsoon and productivity intensification, and decreases during cold phases. Other studies linked past OMZ intensity changes to changes in winter monsoon wind intensity y (Reichart et al., 1998; Reichart et al., 2004; Klöcker and Henrich, 2006). For instance, Reichart et al. (1998) reconstructed the fluctuations of the Arabian Sea OMZ over the past 225,000 years using multiple paleo-proxies and found the lowest O2 concentrations to correlate with maxima in productivity and weak winter mixing. On the basis of an analysis of sediment samples collected off Pakistan in the northern Arabian Sea, Klöcker and Henrich (2006) attributed the weakening (strengthening) of the OMZ during the stadials (interstadials, Late Holocene) to enhanced (reduced) winter monsoons resulting in an invigorated (weakened) ventilation at intermediate depths. Similarly, Reichart et al. (2004) linked the intensification of the Arabian Sea OMZ during interstadials to enhanced stratification in the northern Arabian Sea during these warm periods leading to reduced winter mixing.
3.2 Recent changes in the Arabian Sea OMZ
More recently, the analysis of historical O2 observations in the Arabian Sea over the last few decades documents trends dominated by a decline in most of its northern sector and inconsistent changes in the central and southern parts (Rixen et al., 2020) (Figure 3). For example, the analysis of global historical oxygen observations by Schmidtko et al. (2017) reveals a moderate O2 decline in the subsurface of the Arabian Sea between 1960 and 2010. Similarly, O2 trend analysis by (Schmidtko et al., 2017) indicates a drop in oxygen in the northern and western Arabian Sea as well as along the west coast of India over the same period. Using over 2000 O2 profiles collected between 1960 and 2008 off the coast of Oman, Piontkovski and Al-Oufi (2015) reported a decline in O2 in the upper 300 m in the northern and northwestern Arabian Sea, attributed to increased thermal stratification and a shoaling of the oxycline between the 1960s and 2000s. In an analysis of sea glider data and historical profiles, Queste et al. (2018) also reported an intensification of the suboxic conditions at depth in the Sea of Oman over the recent decades. Banse et al. (2014) analyzed historical O2 measurements collected in the Arabian Sea in the 150-500 m layer between 1959 and 2004. They found no clear systematic trend across the entire basin, although O2 was found to decline in most of the central Arabian Sea and slightly increase in the northeastern Arabian Sea and in the southern Arabian Sea (Figure 3). Nitrite (NO2-) is an intermediate product formed during nitrification and denitrification. Due to the lack of oxygen required for nitrification in the upper part of the Arabian Sea OMZ, the accumulation of nitrite known as the secondary nitrite maximum (SNM) is assumed to be caused primarily by denitrification (Gupta et al., 1976; Naqvi, 1991). Banse et al. (2014) also analyzed historical trends in nitrite (NO2-) concentrations in the subsurface. They found inconsistent trends in different locations with a dominance of profiles indicating an increase in nitrite over time, suggesting a potential intensification of denitrification over the observation period. Similarly, Rixen et al. (2014) analyzed trends in nitrite in the Arabian Sea from the Joint Global Ocean Flux Study (JGOFS) measurements in 1995 and from published data prior to 1993. They found an expansion of the SNM in 1995 relative to the pre-JGOFS data with an increase in western and southern sectors, indicating a potential increase in denitrification. However, these changes could also be linked to interannual variability characterizing denitrification in the region (Lachkar et al., 2021), rather than reflecting long-term trends (Naqvi, 2019). A recent analysis of historical observations by Zhou et al. (2022) has revealed an expansion of the area occupied by all major OMZs, including the Arabian Sea OMZ, over the last 3 to 4 decades. Goes et al. (2020) reported evidence of increased winter stratification (and reduced winter convection) in the northern Arabian Sea, together with a decrease in the N:P ratios, indicative of an increase in denitrification over the recent decades. These changes were suggested to create a niche favorable for the mixotroph Noctiluca scintillans in the northern Arabian Sea (do Rosário Gomes et al., 2014; Goes et al., 2020). Finally, preliminary observations suggest recent O2 decline in the Arabian marginal seas (i.e., the Red Sea and the Gulf) with the emergence of summertime hypoxia in the Gulf (Al-Ansari et al., 2015; Al-Yamani and Naqvi, 2019; Saleh et al., 2021; Lachkar et al., 2022) and ongoing deoxygenation in the northern Red Sea (Naqvi, 2019).
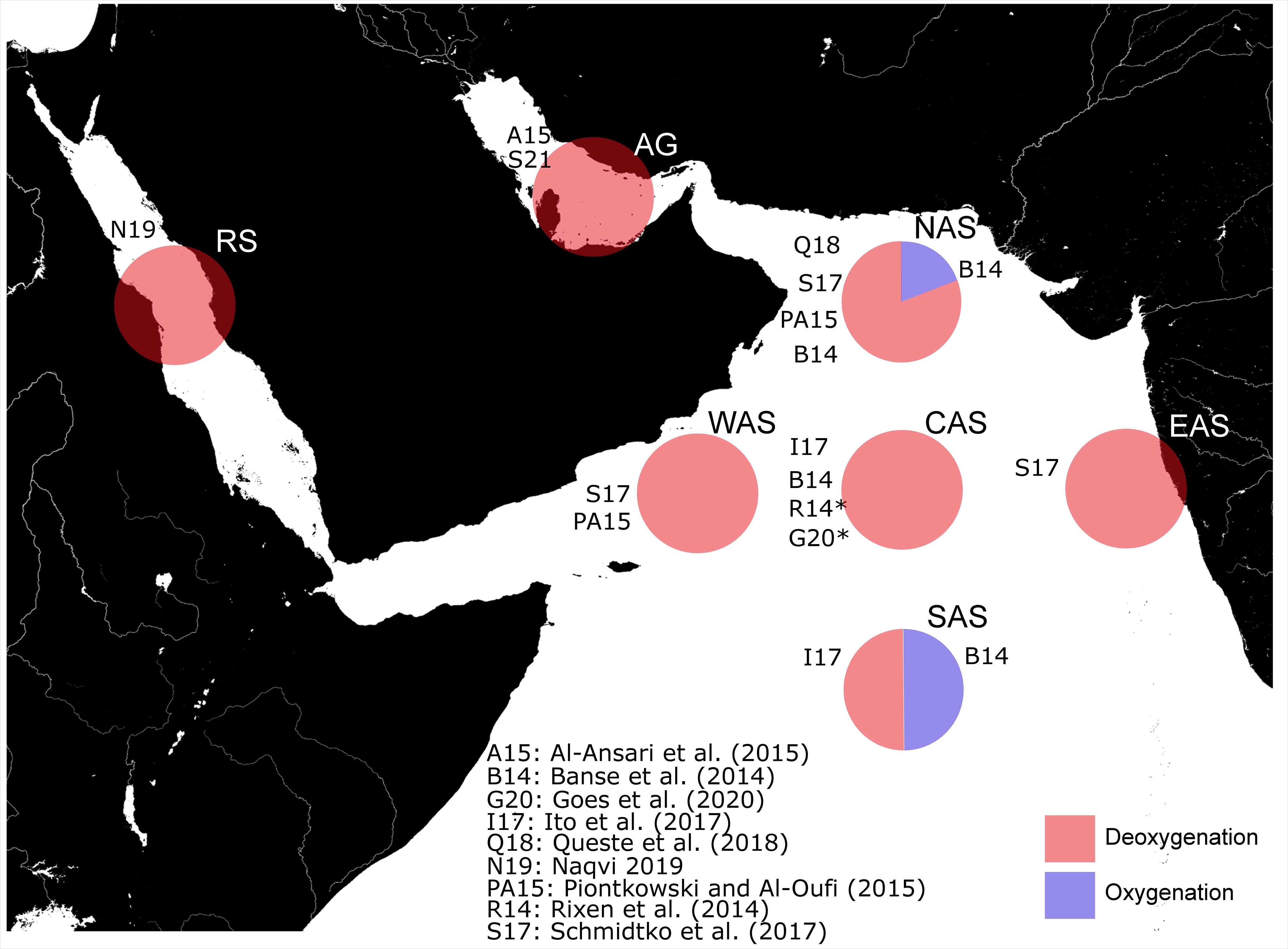
Figure 3 Observational studies reporting recent O2 changes in the Arabian Sea. Observational studies documenting recent O2 trends in the northern (NAS), central (CAS), southern (SAS), eastern (EAS) and western (WAS) Arabian Sea, as well as in the Arabian Gulf (AG) and Red Sea (RS). In each subregion, the proportion of studies suggesting deoxygenation (red) vs. oxygenation (blue) is indicated with a pie chart. Deoxygenation trends in studies labeled with (*) are inferred from relevant proxy data and are not based on direct measurements of oxygen.
Recent modelling works have reproduced declining O2 trends in the Arabian Sea region and investigated their potential drivers. For instance, historical deoxygenation trends were simulated in the mesopelagic zone (200-1000 m) in the northern and northwestern Arabian Sea in a global hindcast simulation covering the period between 1958 and 2014 (Buchanan and Tagliabue, 2021). The study further suggests that this O2 loss is driven by changes in apparent oxygen utilization (AOU), likely associated with ventilation changes. These conclusions were also supported by the analysis of O2 trends in the Arabian Sea region over the period from 1975 and 2014 using the latest Coupled Model Intercomparison Project (CMIP6) model simulations (Buchanan and Tagliabue, 2021). Finally, a strong deoxygenation in the northern Arabian Sea in the 100-1000 m subsurface layer was reported in a recent regional modeling study of the Indian Ocean covering the period from 1982 to 2010 (Lachkar et al., 2021). Causing an important intensification of the OMZ core and a significant increase in denitrification there, this O2 loss was attributed to increased stratification and reduced ventilation as a result of the recent fast warming in the northern Arabian Sea and the Gulf (Lachkar et al., 2021).
4 Future changes in the Arabian Sea OMZ
4.1 Mechanisms and timescales
The Arabian Sea OMZ is vulnerable to various potential future changes affecting O2 demand and supply terms (Table 1 and Figure 4). More specifically, increases in stratification are expected to lead to a significant reduction in productivity and export under future climate change (Bopp et al., 2013; Long et al., 2019; Kwiatkowski et al., 2020). While a few previous works suggested a potential future intensification of summer upwelling-favorable winds in the northern Arabian Sea (Sandeep and Ajayamohan, 2015; deCastro et al., 2016; Praveen et al., 2016), other studies indicate a future weakening of winter monsoon winds that could cause a reduction in convective mixing in the northern Arabian Sea and decrease productivity there (Vallivattathillam et al., 2017). Additionally, a weakening of the summer Walker circulation was also suggested to lead to summer productivity decline in the southern Arabian Sea (e.g., Vallivattathillam et al., submitted). Lachkar et al. (2018) highlighted that changes in OMZ intensity associated with productivity changes can develop over relatively short timescales (Table 1). Although both CMIP5 and CMIP6 multi-model averages point to a future reduction in productivity in the region, individual models show important discrepancies and project changes that go in both directions (Bopp et al., 2013; Kwiatkowski et al., 2020). Additionally, these models generally do not take into account potential changes in dust deposition or effects of changes in denitrification on productivity. For instance, Guieu et al. (2019) have shown that almost half of the Arabian Sea productivity is dependent on iron supply via dust deposition. Thus, changes in this source of iron due to wind changes for instance can have a dramatic impact on productivity and OMZ (Moffett and Landry, 2020). Lachkar et al., (2016) demonstrated that a weakening of denitrification can lead to a significant increase in productivity and export, with implications for the OMZ volume.
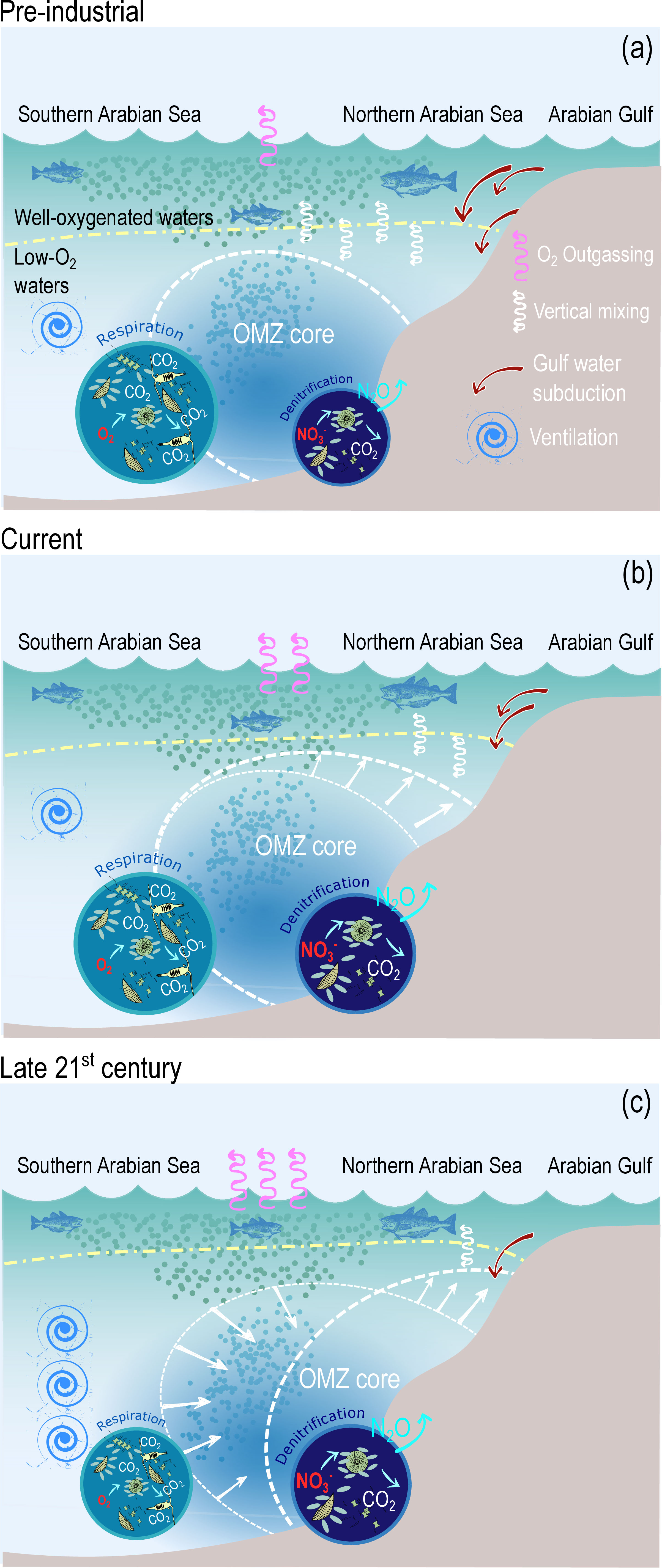
Figure 4 Summary of recent and potential future changes in Arabian Sea OMZ. This schematic summarizes the recent and main potential future changes in the Arabian Sea OMZ as well as their drivers and implications. (A) Pre-industrial conditions: cool conditions favor strong O2 solubility in the seawater and weak outgassing. Well-oxygenated waters (O2 > 100 mmol m-3) occupy a thick upper layer overlaying the OMZ. Strong winter convection and subduction of Gulf waters in the north ventilate the upper OMZ, thus maintaining its denitrifying suboxic core contained at depth. (B) Present-day conditions: weaker O2 solubility in the seawater causes moderate outgassing. A weaker winter convection and subduction of Gulf waters in the north cause an expansion of the upper OMZ and an increase of denitrification. (C) Future conditions: warmer conditions further lower O2 solubility in the water and enhance outgassing. Moreover, enhanced stratification limits the vertical penetration of well-oxygenated waters, contained in a thinner layer (compressing potential habitat). Reduction in winter convection and Gulf water subduction causes a weakening of the ventilation of the upper OMZ in the northern Arabian Sea and a shoaling of the OMZ core, potentially leading to enhanced denitrification there. However, in the central and southern Arabian Sea, enhanced ventilation as well as reduced productivity and respiration lead to important oxygenation at depth, causing a substantial shrinking of the OMZ core.
Additional mechanisms can affect the O2 demand in the Arabian Sea region such as the increase in nutrient loading associated with major river runoff and changes in the plankton community composition (Table 1). Early studies (e.g., Naqvi et al., 2000) suggested that eutrophication associated with increased nutrient loading resulting from agricultural and urban coastal pollution may have contributed to reported intensification of the hypoxic zone along the western continental shelf of India during summer and autumn. Nevertheless, more recent work by Gupta et al. (2021) tends to imply that the anoxic zone along the west coast of India is essentially formed through the upwelling of deoxygenated waters during the summer season and is little affected by riverine input of nutrients or sewage discharge from Indian megacities. These authors argue that acute oxygen deficiency was reported in areas where no major rivers exist, and far from the major cities on the west coast of India. They also noted that hypoxia intensity has remained relatively stable in the region despite a substantial increase in anthropogenic nutrient input over the last five decades (Gupta et al., 2016; Gupta et al., 2021). Finally, changes in the size and composition of phytoplankton dominant groups can affect the efficiency of particle export and hence alter the remineralization depth and the intensity of the OMZ. For instance, the reported recent shift in the composition of winter phytoplankton blooms in the northern Arabian Sea from diatoms to Noctiluca scintillans can have repercussions on the export fluxes, as these mixotrophs are essentially grazed on by salps, which typically produce large and rapidly sinking fecal pellets (do Rosário Gomes et al., 2014). Yet, predicting future changes in plankton community structure is challenging because of the complexity and the uncertainty around the mechanisms controlling the diversity within the plankton functional groups (Henson et al., 2021). Nevertheless, recent model projections suggest a future increase in the dominance of smaller phytoplankton groups, which could imply a reduced export efficiency and hence a shallower remineralization depth under warmer climate (Henson et al., 2021).
Potential future changes in the O2 supply terms entail changes in solubility or ventilation terms. Surface warming is expected to increase outgassing, causing a loss of O2 that is particularly important near the surface (Figure 4). In the ocean interior, changes in the intensity of ventilation can lead to changes in the OMZ intensity. For instance, an increase in winter stratification is predicted to inhibit convection and hence limit winter ventilation of the upper OMZ in the northern Arabian Sea (Figure 4). This mechanism has been suggested to contribute to recent OMZ intensification in the region (Goes et al., 2020; Lachkar et al., 2021). Furthermore, the warming of the Arabian Gulf is predicted to lead to a reduction in the subduction of the Gulf water in the northern Arabian Sea (Lachkar et al., 2019; Lachkar et al., 2021). Additionally, declining levels of O2 in the Gulf waters (Saleh et al., 2021; Lachkar et al., 2022) and potentially increasing semi-labile total organic carbon (TOC) in the Gulf may further contribute to lowering O2 in the upper layers of the OMZ in the northern Arabian Sea (Al-Yamani and Naqvi, 2019).
In the central and southern Arabian Sea, as well as in the deeper layers of the northern Arabian Sea, water ventilation is mostly associated with the inflow of the IOCW from the south along the western Arabian Sea (Figure 2; Schmidt et al., 2020; Schmidt et al., 2021). Thus, an increase in the O2 content of these water masses is expected to lead to a weakening of the OMZ, particularly in the southern Arabian Sea (Vallivattathillam et al., in review) (Table 1 and Figure 4). The enhanced O2 content of the equatorial Indian Ocean thermocline is relatively robust in most CMIP5 and CMIP6 models (Bopp et al., 2013; Kwiatkowski et al., 2020) and is likely associated with an increase in the ventilation of the tropical thermocline (Bopp et al., 2017; Oschlies et al., 2017; Oschlies et al., 2018) aided with a decrease in O2 biological consumption.
Finally, changes that affect O2 gradients without changing the regional O2 inventory can also lead to changes in the volume and intensity of the Arabian Sea OMZ. For instance, a change in mixing driven by changes in eddy activity (e.g., Beech et al., 2022) in the region could alter the intensity of the OMZ (Lachkar et al., 2016; Lévy et al., 2022). Furthermore, changes in the remineralization depth associated with changes in the particle sinking speed or remineralization rate (for instance due to warming or changes in the community composition) can also affect the depth and intensity of the Arabian Sea OMZ as has been demonstrated by previous studies (e.g., Al Azhar et al., 2016) (Table 1).
4.2 Emergence of climate change driven O2 changes and uncertainties around future trends
The northern sector of the Arabian Sea is among the few rare oceanic regions where forced O2 trends emerge from noise already in the present-day (2005-2014) (Rodgers et al., 2015). This is particularly true for the upper layers (200-300 m) of the northern Arabian Sea where forced negative O2 trends, associated with an increase in water age, can already be detected (Long et al., 2016). The time of emergence of the climate change driven signal varies spatially and for different depth layers and occurs later in the central Arabian Sea (Long et al., 2016; Gong et al., 2021). Furthermore, as hypoxic and suboxic water volumes display stronger interannual variability than O2 concentrations (Deutsch et al., 2011), the forced climate change signal is likely to emerge later for the OMZ volume and intensity.
Global model future projections suggest that while the northern Arabian Sea is likely to undergo significant deoxygenation, the central and southern parts will be affected by a weak oxygenation signal that has low detectability due to strong natural variability (Bopp et al., 2017). The deoxygenation in the northern Arabian Sea appears to be driven by an increase in AOU, induced by an important increase in the water age (Long et al., 2019). In contrast, a decrease in water age is expected in the southern Arabian Sea (as in most of the equatorial Indian Ocean). This factor, together with reduced export, is predicted to lead to an increase in O2 levels (Long et al., 2019) (Figure 4).
Nevertheless, these projections come with important uncertainties stemming from global models’ persistent misrepresentation of oxygen in the tropics in general (Oschlies, 2019), and in the Arabian Sea in particular (Schmidt et al., 2021). For instance, observations suggest a decline of O2 in the tropics over the last 50 years, whereas global models simulate non-significant (or even positive) oxygen trends (Stramma et al., 2012a; Oschlies et al., 2017). In addition to poorly constrained biogeochemical processes, different hypotheses were proposed to explain this mismatch such as excessive diapycnal mixing, ill-represented equatorial jets and natural variability in winds not properly represented in models (Stramma et al., 2012a; Oschlies et al., 2017). In the Arabian Sea, the inaccurate representation of the OMZ in global models was linked to several factors, including an imprecise representation of marginal seas (the Arabian Gulf and the Red Sea) and an overestimation of O2 in the IOCW source regions in the Southern Ocean (Schmidt et al., 2021). Despite an improvement in resolution and biogeochemical complexity, the biases reported in CMIP5 models are also present in CMIP6 models with the exception of two models that show significant improvement (Séférian et al., 2020). Furthermore, the projected O2 trends in the Arabian Sea show a strong sensitivity to the employed lateral mixing coefficient with low mixing models showing a strong O2 decline and strong mixing models displaying weak O2 trends (Bahl et al., 2019).
5 Summary and discussion
Dissolved oxygen is important for marine life and for structuring ecosystems and biogeochemistry. Climate change driven ocean warming leads to global deoxygenation through various mechanisms (i.e., reduced solubility, reduced ventilation, enhanced respiration). This has been consistently reproduced by models and is consistent with recent observations. Global deoxygenation will continue and amplify in the future in response to projected additional ocean warming. It is well established that declining dissolved O2 levels detrimentally affect marine life, alter microbial processes and biogeochemical cycling of key nutrients, potentially enhancing greenhouse gas emissions, thus affecting the Earth’s climate. However, deoxygenation effects on ecosystems and biogeochemistry strongly depend on crossing critical thresholds, and hence are a function of the local O2 levels, and more generally the local chemical (pH, pCO2, macro- and micro-nutrients) and physical (e.g., temperature) environment. Therefore, although driven by global warming, deoxygenation is most relevant at the local and regional scales, where its ecological, biogeochemical and climatic impacts are most prominent.
Future deoxygenation is robust across Earth system models in the surface and the deep oceans as well as in the thermocline of the mid and high latitudes (Bopp et al., 2017). Yet, there is no clear theoretical basis for projecting future deoxygenation in the tropical thermocline. Indeed, models show inconsistent trends in the tropics where OMZs are located as the thermal (solubility) and non-thermal (ventilation and respiration) effects oppose each other (Bopp et al., 2017; Long et al., 2019). Although O2 solubility is expected to decrease in response to future climate warming, models project a concomitant decrease in the AOU, mostly driven by a stronger ventilation at thermocline depth (Bopp et al., 2017), attributed to various mechanisms such as a shoaling and thinning of thermocline (Deutsch et al., 2011; Bopp et al., 2017), changes in equatorial undercurrents, and changes in relative contributions of surface and deep waters (Stramma et al., 2012a; Bopp et al., 2017). Projected future reduction in productivity may contribute to reduce oxygen utilization in the tropics. However, quantifying the role of respiration in future oxygen trends remains elusive due to the poor representation of biogeochemical processes in models (Oschlies et al., 2018). This is not only because the complexity of microbial respiration is often overly simplified in models (Laufkötter et al., 2017; Robinson, 2019), but also as key biogeochemical feedbacks involving iron, N2 fixation and denitrification (that can either accelerate O2 decline or oppose it) are still neither well understood nor properly represented in current generation models (Ulloa et al., 2012; Oschlies et al., 2019; Moffett and Landry, 2020; Wallmann et al., 2022). In the Arabian Sea, our ability to predict future changes is further hindered by additional uncertainties emanating from the poorly known and quantified role of marginal seas, as well as the uncertainty around the potential future changes in the Indian monsoon winds, and poorly understood regional processes such as the role of dust deposition, iron limitation or benthic processes.
The future (2050-2100) oxygenation projected by global models (Bopp et al., 2013; Long et al., 2019; Kwiatkowski et al., 2020) in the central and southern Arabian Sea contrasts with the observed recent (1960-2020) oxygen decline in the region (Zhou et al., 2022). We hypothesize that this apparent inconsistency is a consequence of the differences in timescales associated with local and remote perturbations driving recent and future O2 changes and their relative importance (Table 1). On the one hand, the reduction of upper OMZ ventilation associated with the impacts of local warming in the northern Arabian Sea (i.e., reduced winter convective mixing and weakened Gulf water subduction) involves shorter timescales than those associated with the slower ventilation of the deeper layers via the IOCW. On the other hand, the limited changes in biological productivity over the recent decades in the Arabian Sea in particular and the Indian Ocean in general contrast with the more significant decline projected by the end of the century (Roxy et al., 2016; Kwiatkowski et al., 2020). Thus, in the longer term, O2 increase in the equatorial thermocline or in the IOCW source waters in the Southern Ocean, combined with a more significant decline in O2 demand can act to reverse recent deoxygenation trends in the region.
The strong natural variability in O2 time series challenges the detection and attribution of observed trends to climate change (Bindoff et al., 2019). Furthermore, it remains unclear how low O2 waters will be impacted by deoxygenation, nor how the Arabian Sea ecosystem may respond to O2 changes. For instance, (Bahl et al., 2019) found an expansion of hypoxia in simulations showing the weakest global deoxygenation (low mixing models) and a contraction of the OMZ volume in simulations projecting the strongest global deoxygenation (high-mixing models). This is because O2 depends on a subtle balance between supply and consumption processes, both depending on local and global climate change, making it difficult to predict in which direction this balance may shift (Fu et al., 2018; Resplandy, 2018; Lévy et al., 2022). Therefore, studies addressing future deoxygenation need to go beyond assessing changes in O2 inventories and consider changes in O2 distributions. Moreover, suitable observational strategies ensuring an adequate sampling of the Arabian Sea are needed in order to enhance our ability to detect long-term O2 changes in the region and help with their attribution to the climate perturbation.
Finally, the representation of key physical and biogeochemical processes in models need to be improved in order to reduce inconsistencies among projected future O2 changes in the region. In this regard, regional downscaling of global model simulations can help reduce uncertainties associated with model misrepresentation of present-day condition (Figure 5). For instance, Vallivattathillam et al. (in review) have shown that downscaling CMIP5 simulations using a pseudo global warming approach (Xu et al., 2019) that corrects for systematic biases in the representation of physical circulation and biogeochemistry under current climate reduces inter-model discrepancies in future projections and yields more robust O2 trends in the Arabian Sea region under future climate change. More generally, improved representation of key processes in models through refined parameterizations and increased resolution, jointly with enhanced coverage of oxygen observations in space and time, is vital to detect ongoing oxygen changes in the Arabian Sea region and predict the future of its OMZ.
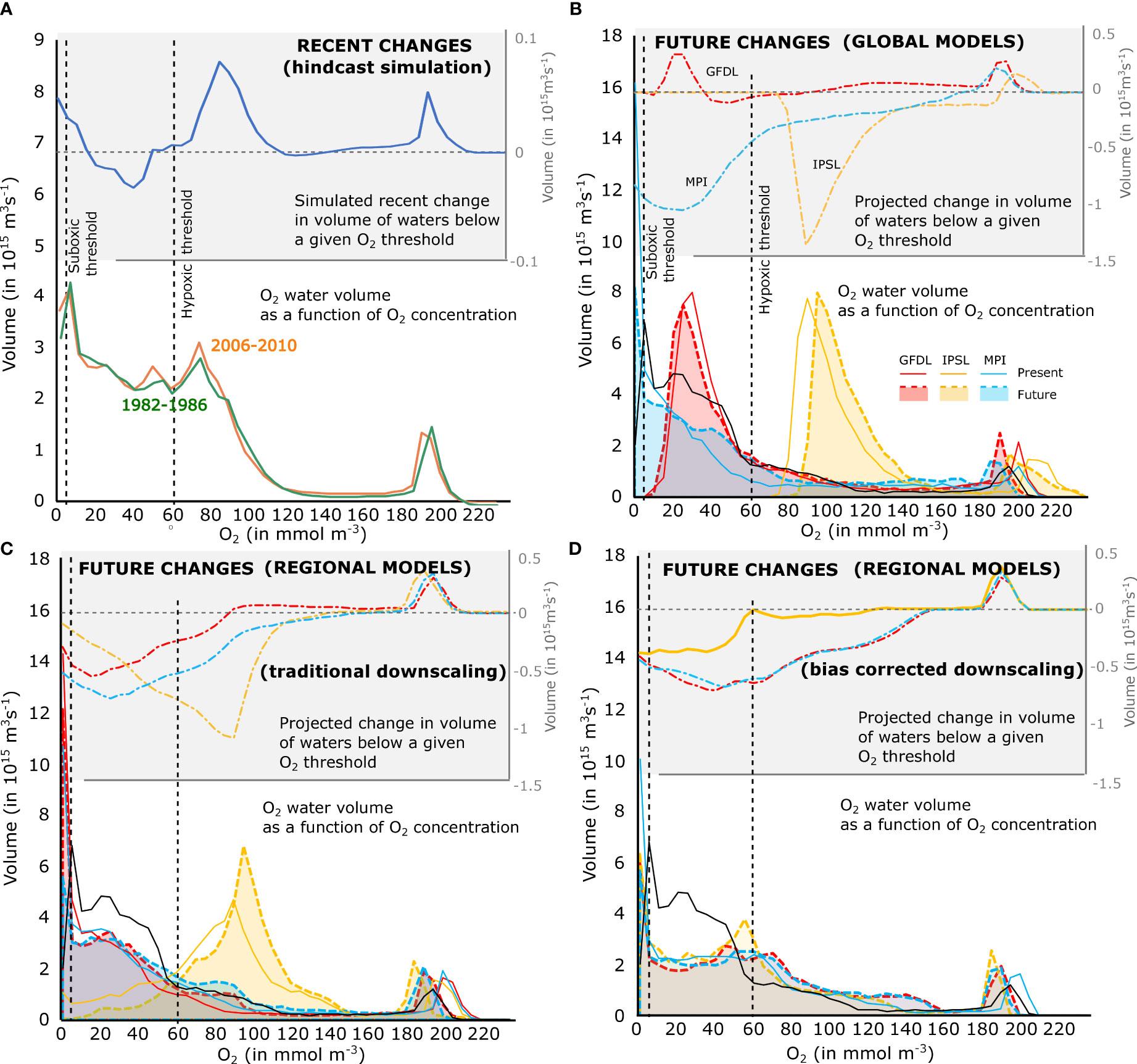
Figure 5 Recent and future changes in O2 frequency distribution in the Arabian Sea. (A) O2 frequency distribution in the Arabian Sea as simulated in the early 1980s (green) and the late 2000s (orange) as well as the cumulative frequency of simulated O2 change over the 1982-2010 period (blue, right axis) from (Lachkar et al., 2021). Note the expansion of poorly oxygenated waters (O2 < 100 mmol m-3) as well as waters with extreme O2 depletion (O2 < 10 mmol m-3). (B–D) O2 frequency distribution in the Arabian Sea in the present-day (solid line) and in the future (dashed filled area) as well as cumulative frequency of future O2 change (dash-dotted line, right axis) as simulated by (B) three global models (MPI, GFDL and IPSL) and a regional model (ROMS) forced with the three global models using traditional (C) and bias-corrected (D) downscaling approaches. Traditional downscaling consists in forcing the regional model with boundary conditions simulated by the global model, whereas bias-corrected downscaling (also known as pseudo global warming) consists in constructing regional model lateral boundary conditions by adding global model projected changes onto observational data. (B–D) Note the reduction in model discrepancies in future O2 projections in the downscaled experiments, particularly when using the bias-correction approach, relative to the global simulations. Adapted from Vallivattathillam et al. (in review).
Author contributions
ZL conceived the review and wrote the manuscript with contributions from all authors. DH performed the Lagrangian analysis. All authors contributed to the article and approved the submitted version.
Acknowledgments
Support for this research has come from the Arabian Center for Climate and Environmental Sciences (ACCESS), through the New York University Abu Dhabi (NYUAD) Research Institute Grant CG009. Computations were performed at the High Performance cluster (HPC) of NYUAD, Dalma. We thank the NYUAD HPC team for technical support. The authors are also grateful to the editor Amal Jayakumar and to reviewers: Amit Sarkar, Helga Do Rosario Gomes, and Arun Deo Singh, for their constructive comments that helped improve the paper. The model code can be accessed online at http://www.crocoocean.org/.
Conflict of interest
The authors declare that the research was conducted in the absence of any commercial or financial relationships that could be construed as a potential conflict of interest.
Publisher’s note
All claims expressed in this article are solely those of the authors and do not necessarily represent those of their affiliated organizations, or those of the publisher, the editors and the reviewers. Any product that may be evaluated in this article, or claim that may be made by its manufacturer, is not guaranteed or endorsed by the publisher.
Supplementary material
The Supplementary Material for this article can be found online at: https://www.frontiersin.org/articles/10.3389/fmars.2023.1122043/full#supplementary-material
References
Acharya S. S., Panigrahi M. K. (2016). Eastward Shift and maintenance of Arabian Sea oxygen minimum zone: Understanding the paradox. Deep Sea Res. Part I: Oceanogr. Res. Papers 115, 240–252.
Al-Ansari E. M., Rowe G., Abdel-Moati M., Yigiterhan O., Al-Maslamani I., Al-Yafei M., et al. (2015). Hypoxia in the central Arabian Gulf Exclusive Economic Zone (EEZ) of Qatar during summer season. Estuarine Coast. Shelf Sci. 159, 60–68. doi: 10.1016/j.ecss.2015.03.022
Al Azhar M., Temimi M., Zhao J., Ghedira H. (2016). Modeling of circulation in the a rabian g ulf and the s ea of o man: Skill assessment and seasonal thermohaline structure. J. Geophys. Res.: Oceans 121, 1700–.
Altabet M. A., Francois R., Murray D. W., Prell W. L. (1995). Climate-related variations in denitrification in the Arabian Sea from sediment 15N/14N ratios. Nature 373, 506–509. doi: 10.1038/373506a0
Altabet M. A., Higginson M. J., Murray D. W. (2002). The effect of millennial-scale changes in Arabian Sea denitrification on atmospheric CO2. Nature 415, 159–162. doi: 10.1038/415159a
Altabet M. A., Murray D. W., Prell W. L. (1999). Climatically linked oscillations in Arabian Sea denitrification over the past 1 my: Implications for the marine n cycle. Paleoceanography 14, 732–743. doi: 10.1029/1999PA900035
Al-Yamani F., Naqvi S. (2019). Chemical oceanography of the Arabian Gulf. Deep Sea Res. Part II: Topical Stud. Oceanogr. 161, 72–80.
Bahl A., Gnanadesikan A., Pradal M.-A. (2019). Variations in ocean deoxygenation across earth system models: isolating the role of parameterized lateral mixing. Global Biogeochemical Cycles 33, 703–724. doi: 10.1029/2018GB006121
Bange H. W., Naqvi S. W. A., Codispoti L. (2005). The nitrogen cycle in the Arabian Sea. Prog. Oceanogr. 65, 145–158. doi: 10.1016/j.pocean.2005.03.002
Banse K., McClain C. R. (1986). Winter blooms of phytoplankton in the Arabian Sea as observed by the coastal zone color scanner. Mar. Ecol. Prog. Ser. 34, 201–211. doi: 10.3354/meps034201
Banse K., Naqvi S., Narvekar P., Postel J., Jayakumar D. (2014). Oxygen minimum zone of the open Arabian Sea: variability of oxygen and nitrite from daily to decadal timescales. Biogeosciences 11, 2237–2261. doi: 10.5194/bg-11-2237-2014
Beech N., Rackow T., Semmler T., Danilov S., Wang Q., Jung T. (2022). Long-term evolution of ocean eddy activity in a warming world. Nat. Climate Change 12, 1–8. doi: 10.1038/s41558-022-01478-3
Bindoff N. L., Cheung W. W., Kairo J. G., Arístegui J., Guinder V. A., Hallberg R., et al. (2019). “Changing ocean, marine ecosystems, and dependent communities,” in IPCC special report on the ocean and cryosphere in a changing climate (Cambridge, UK and New York, NY, USA: Cambridge University Press), 477–587.
Böning P., Bard E. (2009). Millennial/centennial-scale thermocline ventilation changes in the Indian Ocean as reflected by aragonite preservation and geochemical variations in Arabian Sea sediments. Geochimica Cosmochimica Acta 73, 6771–6788. doi: 10.1016/j.gca.2009.08.028
Bopp L., Resplandy L., Orr J. C., Doney S. C., Dunne J. P., Gehlen M., et al. (2013). Multiple stressors of ocean ecosystems in the 21st century: projections with CMIP5 models. Biogeosciences 10, 6225–6245. doi: 10.5194/bg-10-6225-2013
Bopp L., Resplandy L., Untersee A., Le Mezo P., Kageyama M. (2017). Ocean (de) oxygenation from the last glacial maximum to the twenty-first century: insights from earth system models. Philos. Trans. R. Soc. A: Mathematical Phys. Eng. Sci. 375, 20160323.
Breitburg D., Levin L. A., Oschlies A., Grégoire M., Chavez F. P., Conley D. J., et al. (2018). Declining oxygen in the global ocean and coastal waters. Science 359. doi: 10.1126/science.aam7240
Buchanan P. J., Tagliabue A. (2021). The regional importance of oxygen demand and supply for historical ocean oxygen trends. Geophys. Res. Lett. 48, e2021GL094797. doi: 10.1029/2021GL094797
Cavan E. L., Trimmer M., Shelley F., Sanders R. (2017). Remineralization of particulate organic carbon in an ocean oxygen minimum zone. Nat. Commun. 8, 14847. doi: 10.1038/ncomms14847
Chinni V., Singh S. K. (2022). Dissolved iron cycling in the Arabian Sea and sub-tropical gyre region of the Indian Ocean. Geochimica Cosmochimica Acta 317, 325–348. doi: 10.1016/j.gca.2021.10.026
Codispoti L., Brandes J. A., Christensen J., Devol A., Naqvi S., Paerl H. W., et al. (2001). The oceanic fixed nitrogen and nitrous oxide budgets: Moving targets as we enter the anthropocene? Scientia Marina 65, 85–105. doi: 10.3989/scimar.2001.65s285
Couespel D., Lévy M., Bopp L. (2019). Major contribution of reduced upper ocean oxygen mixing to global ocean deoxygenation in an earth system model. Geophys. Res. Lett. 46, 12239–12249. doi: 10.1029/2019GL084162
Das M., Singh R. K., Gupta A. K., Bhaumik A. K. (2017). Holocene Strengthening of the oxygen minimum zone in the northwestern Arabian Sea linked to changes in intermediate water circulation or indian monsoon intensity? Palaeogeogr. Palaeoclimatol. Palaeoecol. 483, 125–135. doi: 10.1016/j.palaeo.2016.10.035
deCastro M., Sousa M., Santos F., Dias J., Gómez-Gesteira M. (2016). How will somali coastal upwelling evolve under future warming scenarios? Sci. Rep. 6, 1–9.
Deutsch C., Brix H., Ito T., Frenzel H., Thompson L. (2011). Climate-forced variability of ocean hypoxia. science 333, 336–339. doi: 10.1126/science.1202422
do Rosário Gomes H., Goes J. I., Matondkar S. P., Buskey E. J., Basu S., Parab S., et al. (2014). Massive outbreaks of noctiluca scintillans blooms in the Arabian Sea due to spread of hypoxia. Nat. Commun. 5, 1–8. doi: 10.1038/ncomms5862
Dueser W., Ross E., Mlodzinska Z. (1978). Evidence for and rate of denitrification in the Arabian Sea. Deep Sea Res. 25, 431–445. doi: 10.1016/0146-6291(78)90551-9
Fu W., Primeau F., Keith Moore J., Lindsay K., Randerson J. T. (2018). Reversal of increasing tropical ocean hypoxia trends with sustained climate warming. Global Biogeochemical Cycles 32, 551–564. doi: 10.1002/2017GB005788
Gaye B., Böll A., Segschneider J., Burdanowitz N., Emeis K.-C., Ramaswamy V., et al. (2018). Glacial–interglacial changes and holocene variations in Arabian Sea denitrification. Biogeosciences 15, 507–527. doi: 10.5194/bg-15-507-2018
Gnanadesikan A., Dunne J., John J. (2012). Understanding why the volume of suboxic waters does not increase over centuries of global warming in an earth system model. Biogeosciences 9, 1159–1172. doi: 10.5194/bg-9-1159-2012
Goes J. I., Tian H., do Rosario Gomes H., Anderson O. R., Al-Hashmi K., deRada S., et al. (2020). Ecosystem state change in the Arabian Sea fuelled by the recent loss of snow over the himalayan-tibetan plateau region. Sci. Rep. 10, 1–8. doi: 10.1038/s41598-020-64360-2
Gong H., Li C., Zhou Y. (2021). Emerging global ocean deoxygenation across the 21st century. Geophys. Res. Lett. 48, e2021GL095370. doi: 10.1029/2021GL095370
Gruber N. (2008). The marine nitrogen cycle: overview and challenges. Nitrogen Mar. Environ. 2, 1–50.
Guieu C., Al Azhar M., Aumont O., Mahowald N., Lévy M., Éthé C., et al. (2019). Major impact of dust deposition on the productivity of the Arabian Sea. Geophys. Res. Lett. 46, 6736–6744. doi: 10.1029/2019GL082770
Gupta A. K., Anderson D. M., Overpeck J. T. (2003). Abrupt changes in the asian southwest monsoon during the holocene and their links to the north atlantic ocean. Nature 421, 354–357. doi: 10.1038/nature01340
Gupta G., Jyothibabu R., Ramu C. V., Reddy A. Y., Balachandran K., Sudheesh V., et al. (2021). The world’s largest coastal deoxygenation zone is not anthropogenically driven. Environ. Res. Lett. 16, 054009. doi: 10.1088/1748-9326/abe9eb
Gupta R. S., Naqvi S. (1984). Chemical oceanography of the Indian Ocean, north of the equator. Deep Sea Res. Part A. Oceanogr. Res. Papers 31, 671–706. doi: 10.1016/0198-0149(84)90035-9
Gupta R. S., Rajagopal M., Qasim S. (1976). Relationship between dissolved oxygen and nutrients in the north-western Indian Ocean. J Mar. Sci 5, 201–211.
Gupta G., Sudheesh V., Sudharma K., Saravanane N., Dhanya V., Dhanya K., et al. (2016). Evolution to decay of upwelling and associated biogeochemistry over the southeastern Arabian Sea shelf. J. Geophys. Res.: Biogeosciences 121, 159–175. doi: 10.1002/2015JG003163
Henson S. A., Cael B., Allen S. R., Dutkiewicz S. (2021). Future phytoplankton diversity in a changing climate. Nat. Commun. 12, 5372. doi: 10.1038/s41467-021-25699-w
Hood R. R., Rixen T., Levy M., Hansell D. A., Coles V. J., Lachkar Z. (2023). Oxygen, carbon and ph variability in the Indian Ocean (Elsevier).
Hughes D. J., Alderdice R., Cooney C., Kühl M., Pernice M., Voolstra C. R., et al. (2020). Coral reef survival under accelerating ocean deoxygenation. Nat. Climate Change 10, 296–307. doi: 10.1038/s41558-020-0737-9
Ito T., Minobe S., Long M. C., Deutsch C. (2017). Upper ocean o2 trends: 1958–2015. Geophys. Res. Lett. 44, 4214–4223. doi: 10.1002/2017GL073613
Ito T., Nenes A., Johnson M., Meskhidze N., Deutsch C. (2016). Acceleration of oxygen decline in the tropical pacific over the past decades by aerosol pollutants. Nat. Geosci. 9, 443–447. doi: 10.1038/ngeo2717
Ittekkot V., Nair R., Honjo S., Ramaswamy V., Bartsch M., Manganini S., et al. (1991). Enhanced particle fluxes in Bay of Bengal induced by injection of fresh water. Nature 351, 385–387. doi: 10.1038/351385a0
Joshi G. P., Naik S. S., Banakar V. (2021). Last 10000 years variation in the intensity of OMZ-core reconstructed from sediment of the eastern Arabian Sea. J. Geological Soc. India 97, 243–248. doi: 10.1007/s12594-021-1673-7
Keeling R. F., Körtzinger A., Gruber N.. (2010). Ocean deoxygenation in a warming world. Annu. Rev. Mar. Sci. 2, 199–229. doi: 10.1146/annurev.marine.010908.163855
Klöcker R., Henrich R. (2006). Recent and late quaternary pteropod preservation on the pakistan shelf and continental slope. Mar. Geol. 231, 103–111. doi: 10.1016/j.margeo.2006.05.014
Koné V., Aumont O., Lévy M., Resplandy L. (2009). Physical and biogeochemical controls of the phytoplankton seasonal cycle in the Indian Ocean: A modeling study. Indian Ocean Biogeochemical Processes Ecol. Variability 185, 350. doi: 10.1029/2008GM000700
Kwiatkowski L., Torres O., Bopp L., Aumont O., Chamberlain M., Christian J. R., et al. (2020). Twenty-first century ocean warming, acidification, deoxygenation, and upper-ocean nutrient and primary production decline from cmip6 model projections. Biogeosciences 17, 3439–3470. doi: 10.5194/bg-17-3439-2020
Kwon E. Y., Primeau F., Sarmiento J. L. (2009). The impact of remineralization depth on the air–sea carbon balance. Nat. Geosci. 2, 630–635. doi: 10.1038/ngeo612
Lachkar Z., Lévy M., Smith S. (2018). Intensification and deepening of the Arabian Sea oxygen minimum zone in response to increase in indian monsoon wind intensity. Biogeosciences 15, 159–186. doi: 10.5194/bg-15-159-2018
Lachkar Z., Lévy M., Smith K. (2019). Strong intensification of the Arabian Sea oxygen minimum zone in response to Arabian Gulf warming. Geophys. Res. Lett. 46, 5420–5429. doi: 10.1029/2018GL081631
Lachkar Z., Mehari M., Al Azhar M., Lévy M., Smith S. (2021). Fast local warming is the main driver of recent deoxygenation in the northern Arabian Sea. Biogeosciences 18, 5831–5849. doi: 10.5194/bg-18-5831-2021
Lachkar Z., Mehari M., Lévy M., Paparella F., Burt J. A. (2022). Recent expansion and intensification of hypoxia in the Arabian Gulf and its drivers. Front. Mar. Sci. 9, 1616. doi: 10.3389/fmars.2022.891378
Lachkar Z., Smith S., Lévy M., Pauluis O. (2016). Eddies reduce denitrification and compress habitats in the Arabian Sea. Geophys. Res. Lett. 43, 9148–9156. doi: 10.1002/2016GL069876
Laffoley D. D., Baxter J. (2019). Ocean deoxygenation: Everyone’s problem-causes, impacts, consequences and solutions (Gland, Switzerland: IUCN).
Laufkötter C., John J. G., Stock C. A., Dunne J. P. (2017). Temperature and oxygen dependence of the remineralization of organic matter. Global Biogeochemical Cycles 31, 1038–1050. doi: 10.1002/2017GB005643
Lévy M., Resplandy L., Palter J. B., Couespel D., Lachkar Z. (2022). The crucial contribution of mixing to present and future ocean oxygen distribution. Ocean mixing, 329–344. doi: 10.1016/B978-0-12-821512-8.00020-7
Lévy M., Shankar D., André J.-M., Shenoi S., Durand F., de Boyer Montégut C. (2007). Basin-wide seasonal evolution of the Indian Ocean’s phytoplankton blooms. J. Geophys. Res.: Oceans 112.
Long M. C., Deutsch C., Ito T. (2016). Finding forced trends in oceanic oxygen. Global Biogeochemical Cycles 30, 381–397. doi: 10.1002/2015GB005310
Long M., Ito T., Deutsch C. (2019). Oxygen projections for the future. ocean deoxygenation: Everyone’s problem Vol. 562 (Gland, Switzerland: IUCN).
Madhupratap M., Kumar S. P., Bhattathiri P., Kumar M. D., Raghukumar S., Nair K., et al. (1996). Mechanism of the biological response to winter cooling in the northeastern Arabian Sea. Nature 384, 549–552. doi: 10.1038/384549a0
McCreary J. P., Yu Z., Hood R. R., Vinaychandran P., Furue R., Ishida A., et al. (2013). Dynamics of the indian-ocean oxygen minimum zones. Prog. Oceanogr. 112, 15–37. doi: 10.1016/j.pocean.2013.03.002
Moffett J. W., Goepfert T. J., Naqvi S. W. A. (2007). Reduced iron associated with secondary nitrite maxima in the Arabian Sea. Deep Sea Res. Part I: Oceanogr. Res. Papers 54, 1341–1349.
Moffett J. W., Landry M. R. (2020). Grazing control and iron limitation of primary production in the Arabian Sea: Implications for anticipated shifts in southwest monsoon intensity. Deep Sea Res. Part II: Topical Stud. Oceanogr. 179, 104687.
Moffett J. W., Vedamati J., Goepfert T. J., Pratihary A., Gauns M., Naqvi S. (2015). Biogeochemistry of iron in the a rabian s ea. Limnol. Oceanogr. 60, 1671–1688. doi: 10.1002/lno.10132
Morrison J. M., Codispoti L., Smith S. L., Wishner K., Flagg C., Gardner W. D., et al. (1999). The oxygen minimum zone in the Arabian Sea during 1995. Deep Sea Res. Part II: Topical Stud. Oceanogr. 46, 1903–1931.
Nair R., Ittekkot V., Manganini S., Ramaswamy V., Haake B., Degens E., et al. (1989). Increased particle flux to the deep ocean related to monsoons. Nature 338, 749–751. doi: 10.1038/338749a0
Naqvi S. (1987). Some aspects of the oxygen-deficient conditions and denitrification in the Arabian Sea. J. Mar. Res. 45, 1049–1072. doi: 10.1357/002224087788327118
Naqvi W. (1991). Geographical extent of denitrification in the Arabian Sea in relation to some physical processes. Oceanologica Acta 14, 281–290.
Naqvi S. (2008). The Indian Ocean. Nitrogen Mar. Environ. 2, 631–681. doi: 10.1016/B978-0-12-372522-6.00014-1
Naqvi S. (2019). “Evidence for ocean deoxygenation and its patterns: Indian ocean,” in Ocean deoxygenation: Everyone’s problem. Eds. Laffoley D., Baxter J. M. (Gland, Switzerland: IUCN).
Naqvi S., Jayakumar D., Narvekar P., Naik H., Sarma V., D’souza W., et al. (2000). Increased marine production of n2o due to intensifying anoxia on the indian continental shelf. Nature 408, 346–349. doi: 10.1038/35042551
Naqvi S., Moffett J., Gauns M., Narvekar P., Pratihary A., Naik H., et al. (2010). The Arabian Sea as a high-nutrient, low-chlorophyll region during the late southwest monsoon. Biogeosciences 7, 2091–2100. doi: 10.5194/bg-7-2091-2010
Naqvi S. W. A., Noronha R. J., Reddy C. G. (1982). Denitrification in the Arabian Sea. Deep Sea Res. Part A. Oceanogr. Res. Papers 29, 459–469. doi: 10.1016/0198-0149(82)90070-X
Naqvi S., Sarma V., Jayakumar D. (2002). Carbon cycling in the northern Arabian Sea during the northeast monsoon: Significance of salps. Mar. Ecol. Prog. Ser. 226, 35–44. doi: 10.3354/meps226035
Oschlies A. (2021). A committed fourfold increase in ocean oxygen loss. Nat. Commun. 12, 1–8. doi: 10.1038/s41467-021-22584-4
Oschlies A., Brandt P., Stramma L., Schmidtko S. (2018). Drivers and mechanisms of ocean deoxygenation. Nat. Geosci. 11, 467–473. doi: 10.1038/s41561-018-0152-2
Oschlies A., Duteil O., Getzlaff J., Koeve W., Landolfi A., Schmidtko S. (2017). Patterns of deoxygenation: sensitivity to natural and anthropogenic drivers. Philos. Trans. R. Soc. A: Mathematical Phys. Eng. Sci. 375, 20160325.
Oschlies A., Koeve W., Landolfi A., Kähler P. (2019). Loss of fixed nitrogen causes net oxygen gain in a warmer future ocean. Nat. Commun. 10, 1–7. doi: 10.1038/s41467-019-10813-w
Pichevin L., Bard E., Martinez P., Billy I. (2007). Evidence of ventilation changes in the Arabian Sea during the late quaternary: Implication for denitrification and nitrous oxide emission. Global Biogeochemical Cycles 21. doi: 10.1029/2006GB002852
Piontkovski S., Al-Oufi H. (2015). The omani shelf hypoxia and the warming Arabian Sea. Int. J. Environ. Stud. 72, 256–264. doi: 10.1080/00207233.2015.1012361
Praveen V., Ajayamohan R., Valsala V., Sandeep S. (2016). Intensification of upwelling along oman coast in a warming scenario. Geophys. Res. Lett. 43, 7581–7589. doi: 10.1002/2016GL069638
Queste B. Y., Vic C., Heywood K. J., Piontkovski S. A. (2018). Physical controls on oxygen distribution and denitrification potential in the north west Arabian Sea. Geophys. Res. Lett. 45, 4143–4152. doi: 10.1029/2017GL076666
Rabalais N. N., Turner R. E., Wiseman ,. W. J. Jr (2002). Gulf of mexico hypoxia, aka “the dead zone”. Annu. Rev. Ecol. Systematics 33, 235–263. doi: 10.1146/annurev.ecolsys.33.010802.150513
Rao C., Naqvi S., Kumar M. D., Varaprasad S., Jayakumar D., George M., et al. (1994). Hydrochemistry of the bay of bengal: possible reasons for a different water-column cycling of carbon and nitrogen from the Arabian Sea. Mar. Chem. 47, 279–290. doi: 10.1016/0304-4203(94)90026-4
Reichart G.-J., Brinkhuis H., Huiskamp F., Zachariasse W. J. (2004). Hyperstratification following glacial overturning events in the northern Arabian Sea. Paleoceanography 19. doi: 10.1029/2003PA000900
Reichart G.-J., Lourens L., Zachariasse W. (1998). Temporal variability in the northern Arabian Sea oxygen minimum zone (OMZ) during the last 225,000 years. Paleoceanography 13, 607–621. doi: 10.1029/98PA02203
Resplandy L. (2018). Climate change and oxygen in the ocean. Nature: Int. Weekly J. Sci 557, 314–315.
Resplandy L., Lévy M., Bopp L., Echevin V., Pous S., Sarma V., et al. (2012). Controlling factors of the oxygen balance in the Arabian Sea’s omz. Biogeosciences 9, 5095–5109. doi: 10.5194/bg-9-5095-2012
Resplandy L., Lévy M., Madec G., Pous S., Aumont O., Kumar D. (2011). Contribution of mesoscale processes to nutrient budgets in the Arabian Sea. J. Geophys. Res.: Oceans 116.
Rixen T., Baum A., Gaye B., Nagel B. (2014). Seasonal and interannual variations in the nitrogen cycle in the Arabian Sea. Biogeosciences 11, 5733–5747. doi: 10.5194/bg-11-5733-2014
Rixen T., Cowie G., Gaye B., Goes J., do Rosário Gomes H., Hood R. R., et al. (2020). Reviews and syntheses: Present, past, and future of the oxygen minimum zone in the Northern Indian Ocean. Biogeosciences 17, 6051–6080. doi: 10.5194/bg-17-6051-2020
Rixen T., Guptha M., Ittekkot V. (2005). Deep ocean fluxes and their link to surface ocean processes and the biological pump. Prog. Oceanogr. 65, 240–259. doi: 10.1016/j.pocean.2005.03.006
Robinson C. (2019). Microbial respiration, the engine of ocean deoxygenation. Front. Mar. Sci. 5, 533. doi: 10.3389/fmars.2018.00533
Rodgers K. B., Lin J., Frölicher T. L. (2015). Emergence of multiple ocean ecosystem drivers in a large ensemble suite with an earth system model. Biogeosciences 12, 3301–3320. doi: 10.5194/bg-12-3301-2015
Roxy M. K., Modi A., Murtugudde R., Valsala V., Panickal S., Prasanna Kumar S., et al. (2016). A reduction in marine primary productivity driven by rapid warming over the tropical Indian Ocean. Geophys. Res. Lett. 43, 826–833. doi: 10.1002/2015GL066979
Saleh A., Abtahi B., Mirzaei N., Chen C.-T. A., Ershadifar H., Ghaemi M., et al. (2021). Hypoxia in the persian Gulf and the strait of hormuz. Mar. pollut. Bull. 167, 112354. doi: 10.1016/j.marpolbul.2021.112354
Sandeep S., Ajayamohan R. (2015). Poleward shift in indian summer monsoon low level jetstream under global warming. Climate Dynamics 45, 337–351. doi: 10.1007/s00382-014-2261-y
Sarma V., Bhaskar T. U., Kumar J. P., Chakraborty K. (2020). Potential mechanisms responsible for occurrence of core oxygen minimum zone in the north-eastern Arabian Sea. Deep Sea Res. Part I: Oceanogr. Res. Papers 165, 103393.
Schmidt H., Czeschel R., Visbeck M. (2020). Seasonal variability of the Arabian Sea intermediate circulation and its impact on seasonal changes of the upper oxygen minimum zone. Ocean Sci. 16, 1459–1474. doi: 10.5194/os-16-1459-2020
Schmidt H., Getzlaff J., Löptien U., Oschlies A. (2021). Causes of uncertainties in the representation of the Arabian Sea oxygen minimum zone in CMIP5 models. Ocean Sci. 17, 1303–1320. doi: 10.5194/os-17-1303-2021
Schmidtko S., Stramma L., Visbeck M. (2017). Decline in global oceanic oxygen content during the past five decades. Nature 542, 335–339. doi: 10.1038/nature21399
Schulz H., von Rad U., Erlenkeuser H., von Rad U. (1998). Correlation between Arabian Sea and greenland climate oscillations of the past 110,000 years. Nature 393, 54–57. doi: 10.1038/31750
Séférian R., Berthet S., Yool A., Palmieri J., Bopp L., Tagliabue A., et al. (2020). Tracking improvement in simulated marine biogeochemistry between cmip5 and CMIP6. Curr. Climate Change Rep. 6, 95–119. doi: 10.1007/s40641-020-00160-0
Singh A. D., Jung S. J., Darling K., Ganeshram R., Ivanochko T., Kroon D. (2011). Productivity collapses in the Arabian Sea during glacial cold phases. Paleoceanography 26. doi: 10.1029/2009PA001923
Stramma L., Johnson G. C., Sprintall J., Mohrholz V. (2008). Expanding oxygen-minimum zones in the tropical oceans. science 320, 655–658. doi: 10.1126/science.1153847
Stramma L., Oschlies A., Schmidtko S. (2012a). Mismatch between observed and modeled trends in dissolved upper-ocean oxygen over the last 50 yr. Biogeosciences 9, 4045–4057. doi: 10.5194/bg-9-4045-2012
Stramma L., Prince E. D., Schmidtko S., Luo J., Hoolihan J. P., Visbeck M., et al. (2012b). Expansion of oxygen minimum zones may reduce available habitat for tropical pelagic fishes. Nat. Climate Change 2, 33–37. doi: 10.1038/nclimate1304
Stramma L., Schmidtko S., Levin L. A., Johnson G. C. (2010). Ocean oxygen minima expansions and their biological impacts. Deep Sea Res. Part I: Oceanogr. Res. Papers 57, 587–595.
Ulloa O., Canfield D. E., DeLong E. F., Letelier R. M., Stewart F. J. (2012). Microbial oceanography of anoxic oxygen minimum zones. Proc. Natl. Acad. Sci. 109, 15996–16003. doi: 10.1073/pnas.1205009109
Vallivattathillam P., Iyyappan S., Lengaigne M., Ethé C., Vialard J., Levy M., et al. (2017). Positive Indian Ocean dipole events prevent anoxia off the west coast of india. Biogeosciences 14, 1541–1559. doi: 10.5194/bg-14-1541-2017
Vallivattathillam P., Lachkar Z., Levy M. Robust shrinking of the Arabian Sea oxygen minimum zone with climate change projected with a downscaled model. Front. Mar. Sci.
Vallivattathillam P., Suresh I., Lengaigne M., Vialard J., Izumo T., Sadhvi K., et al. (2019). Mechanisms of the Southern Arabian Sea summer primary productivity weakening in response to anthropogenic forcing in CMIP5 models. J. Geophys. Res. Biogeosciences. 21:1-1. 1p.
Vaquer-Sunyer R., Duarte C. M. (2008). Thresholds of hypoxia for marine biodiversity. Proc. Natl. Acad. Sci. 105, 15452–15457. doi: 10.1073/pnas.0803833105
Wallmann K., José Y. S., Hopwood M. J., Somes C. J., Dale A. W., Scholz F., et al. (2022). Biogeochemical feedbacks may amplify ongoing and future ocean deoxygenation: a case study from the peruvian oxygen minimum zone. Biogeochemistry 159, 45–67. doi: 10.1007/s10533-022-00908-w
Wiggert J. D., Hood R., Banse K., Kindle J. (2005). Monsoon-driven biogeochemical processes in the Arabian Sea. Prog. Oceanogr. 65, 176–213. doi: 10.1016/j.pocean.2005.03.008
Wiggert J. D., Murtugudde R. G. (2007). The sensitivity of the southwest monsoon phytoplankton bloom to variations in aeolian iron deposition over the Arabian Sea. J. Geophys. Res.: Oceans 112.
Wiggert J., Murtugudde R., Christian J. (2006). Annual ecosystem variability in the tropical Indian Ocean: Results of a coupled bio-physical ocean general circulation model. Deep Sea Res. Part II: Topical Stud. Oceanogr. 53, 644–676. doi: 10.1016/j.dsr2.2006.01.027
Wishner K. F., Seibel B. A., Roman C., Deutsch C., Outram D., Shaw C. T., et al. (2018). Ocean deoxygenation and zooplankton: Very small oxygen differences matter. Sci. Adv. 4, eaau5180. doi: 10.1126/sciadv.aau5180
Wyrtki K., Bennett E. B., Rochford D. J.. (1971). Oceanographic atlas of the international Indian ocean expedition Vol. 531 (Washington, DC: National Science Foundation).
Xu Z., Han Y., Yang Z. (2019). Dynamical downscaling of regional climate: A review of methods and limitations. Sci. China Earth Sci. 62, 365–375. doi: 10.1007/s11430-018-9261-5
Zhang Z., Ma W., Chai F. (2022). Role of ocean circulation and settling of particulate organic matter in the decoupling between the oxygen minimum zone and the phytoplankton productive zone in the Arabian Sea: A modeling study. Front. Mar. Sci. 9, 1960. doi: 10.3389/fmars.2022.927921
Keywords: Arabian Sea oxygen minimum zone, ocean deoxygenation, oxygen trends, ocean ventilation and oxygenation, denitrification
Citation: Lachkar Z, Lévy M, Hailegeorgis D and Vallivattathillam P (2023) Differences in recent and future trends in the Arabian Sea oxygen minimum zone: processes and uncertainties. Front. Mar. Sci. 10:1122043. doi: 10.3389/fmars.2023.1122043
Received: 12 December 2022; Accepted: 16 March 2023;
Published: 27 April 2023.
Edited by:
Amal Jayakumar, Princeton University, United StatesReviewed by:
Amit Sarkar, Kuwait Institute for Scientific Research, KuwaitHelga Do Rosario Gomes, Columbia University, United States
Arun Deo Singh, Banaras Hindu University, India
Copyright © 2023 Lachkar, Lévy, Hailegeorgis and Vallivattathillam. This is an open-access article distributed under the terms of the Creative Commons Attribution License (CC BY). The use, distribution or reproduction in other forums is permitted, provided the original author(s) and the copyright owner(s) are credited and that the original publication in this journal is cited, in accordance with accepted academic practice. No use, distribution or reproduction is permitted which does not comply with these terms.
*Correspondence: Zouhair Lachkar, em91aGFpci5sYWNoa2FyQG55dS5lZHU=