- 1Jiangsu Key Laboratory of Marine Bioresources and Environment, Jiangsu Ocean University, Lianyungang, China
- 2Co-Innovation Center of Jiangsu Marine Bio-industry Technology, Jiangsu Institute of Marine Resources Development, Lianyungang, China
- 3Jiangsu Institute of Marine Resources Development, Lianyungang, China
The clam Cyclina sinensis is one of the important economical aquaculture shellfish in China. However, the mechanisms of sex determination and differentiation in C. sinensis have not been fully studied. In this study, full-length cDNAs of DMRT3 and FOXL2 were cloned and functionally characterized. The ORF region of CsDMRT3 consists of 1137 nucleotides, which encode 378 amino acids contains a conserved DM domain of DMRT family. The ORF region of CsFOXL2 is 1245 bp, encodes 414 amino acids, and contains a conserved FH domain. Tissue-specific expression results showed that the higher expression level of CsDMRT3 and CsFOXL2 was found in the ovary and testis of C. sinensis. The expression levels of CsDMRT3 and CsFOXL2 also peaked at the maturation stage of male and female gonadal development, respectively. Moreover, the expression levels of CsDMRT3 and CsFOXL2 were significantly higher in the trochophore and D-larval stages than in other stages. The transcript levels of CsDMRT3 reached the highest level at 11 months of age, while the CsFOXL2 reached the highest level at 7 months of age. In estradiol-treated experiments, the expression levels of CsDMRT3 and CsFOXL2 in the gonads were highest at 5 µg/L estradiol treatment, and histologically, it was observed that the oocytes diameters became larger with increasing estradiol concentration. These results suggest that CsDMRT3 and CsFOXL2 play an important role in gonadal development and sex differentiation of C. sinensis.
1 Introduction
Sex differences are essential characteristics of sexually reproducing organisms. In aquatic animals, species of both sexes grow at different rates (Liao, 2022). Therefore, understanding the sex development of aquatic animals is important. Key sex-related genes have been identified in bivalves, including FOXL2, DMRT family, WNT4, FST, β-CATENIN, and SOXE, which are involved in the development, gonad maintenance and germ cell formation of individual organisms (Christelle et al., 2014; Shi et al., 2014; Tong et al., 2015).
The DMRT (double-sex and mab-3-related transcription factor) gene family was first detected from fruit fly Drosophila melanogaster, where all genes in the family are transcription factors. The DMRT family is involved in the sex determination and differentiation of organisms, as well as in embryonic development and organ formation (Hildreth, 1965). Shinseog et al. (2003) found that DMRT3, DMRT5, and DMRT7 exhibit sex specificity, suggesting their involvement in the gonadal development of mouse Mus culus. DMRT3 transcripts in Japanese pufferfish Takifugu rubripes begin to express at the larval stage and are highly expressed in adult testes (Akihiko et al., 2006). Similar results were found in experiments with Atlantic cod (Hanne and Øivind, 2012).
FOXL2, forkhead transcription factor gene 2, is a member of the forkhead (FOX) family. The FOXL2 coding region is highly conserved in Homo, Capra hircus, and M. culus. (Cocquet et al., 2002). FOXL2 represses the male gene pathway during female gonadal differentiation and regulates and maintains ovarian development in mice (Loffler et al., 2003; Chris et al., 2005). Previous experiments have shown that FOXL2 is expressed very differently in the testis and ovary of chicken Gallus gallus domesticus, and its testis expression is much lower than the ovary expression (Govoroun et al., 2004). Similar results were found during gonadal differentiation in frog Rana rugosa (Yuki et al., 2008). In invertebrates, FOXL2 is involved in regulating embryonic development in sea urchin Strongylocentrotus purpuratus (Tu et al., 2006). Liu et al. (2013) found that FOXL2 was expressed at a higher level in the D-larval stage of Zhikong scallop Chlamys farreri. The expression level of FOXL2 in the freshwater mussel Hyriopsis cumingii gonad gradually decreases with age (Wang et al., 2020).
Estradiol (E2) is a sex steroid hormone produced by cholesterol metabolism and plays an important role in gonadal development and sex differentiation in animals. Specifically, Ni et al. (2012) found that the injection of estradiol into oysters induces sex reversal from male to female. Yan et al. (2011) treated razor clam Sinonovacula constricta with estradiol, causing an increase in oocyte diameter, suggesting that estradiol plays an endogenous regulatory role in the gonadal development of razor clam. Estradiol affects on the expression levels of sex-related genes. Estradiol exposure decreases the expression levels of DMRT1 and SOX9, which are male key factors in Pelodiscus sinensis (Wang et al., 2014). The expression level of FOXL2 was elevated in the ovaries of sea urchins Mseocentrotus nudus after estradiol exposure (Hu et al., 2021). The same result was also found in blue tilapia Oreochromis aureus (Cao et al., 2010).
To date, studies on genes related to sexual differentiation and the mechanisms of sexual differentiation among mollusks have mainly focused on oyster C. gigas, scallop C. farreri, and freshwater mussel H. cumingii (Liu, 2012; Tian et al., 2012; Christelle et al., 2014). However, detailed findings on the sex determination and differentiation of C. sinensis have not been reported. Understanding the sex determination and differentiation of C. sinensis is important for the reproduction and breeding of clams (Wei et al., 2020; Dong et al., 2021; Liao et al., 2022). In the present study, the full-length cDNAs of DMRT3 and FOXL2 were cloned first. The changes in the expression patterns of DMRT3 and FOXL2 were analyzed in different tissues, gonadal development stages, larval developmental stages, and different month-old clams. Finally, the expression levels of DMRT3 and FOXL2 of C. sinensis in response to estradiol exposure were investigated. This study will provide a reference for subsequent studies on gonad development and sex differentiation of C. sinensis.
2 Materials and methods
2.1 Ethics statement
All animal experiments were conducted in accordance with the guidelines and approval of the Animal Experiment Ethics Committee at Jiangsu Ocean University.
2.2 Animals and sampling
All clams of this study were obtained from Lianyungang Comprehensive Experimental Station, the national shellfish industry system of China. The clams were acclimated in a seawater tank (salinity: 25‰) for a week before experimental processing. During the acclimatization, the clams were fed with microalgae Chaeroeeros moelleri twice in the 8:30am and 18:00pm. The microalgae Chaeroeeros moelleri was purchased from Wudi Zaocheng Biotechnology company (Shandong, China).
Past studies have shown that the gonadal development of C. sinensis can be divided into five stages: proliferation stage, growing stage, maturation stage, spawning stage, and spent stage (Racotta et al., 2003; Liao, 2022). Based on above gonadal staging system, eight male and eight female clams in the growing stage were selected and adductor, mantle, pipe, gill, foot, hepatopancreas and gonad were collected. Ovarian and testis samples were also collected from C. sinensis with different gonadal development stages, respectively. During the larval development of C. sinensis, the fertilized egg, trochophore, D-larvae, and umbo larvae were sampled in a 1.5 mL DNase/RNase-free centrifuge tube. In addition, the gonads of juvenile clams aged 3−12 months were also collected every month from October 2020 to September 2021. All tissues were immediately frozen in liquid nitrogen and stored at −80°C for RNA extraction.
2.3 RNA isolation, cDNA synthesis, and RACE PCR
Total RNA from the clam tissues was isolated using TRIzol reagent (TaKaRa, Japan), following the manufacturer’s instruction, and then stored at −80°C. PrimeScript RT reagent kit with gDNA Eraser (TaKaRa, Tokyo, Japan) was used for first-strand cDNA synthesis. Ready-cDNA, which was used as the template for RACE PCR, was synthesized using the SMART™ RACE cDNA Amplification Kit (Clontech, USA) in accordance with the manufacturer’s instructions.
The program of touchdown PCR was conducted in the 5′- and 3′-RACE PCR amplification as follows: 5 cycles of 95°C for 30 s, 72°C for 30 s, 72°C for 2 min; 5 cycles of 95°C for 30 s, 70°C for 30 s, 72°C for 2 min; 5 cycles of 95°C for 30 s, 68°C for 30 s, 72°C for 2 min; and 20 cycles of 95°C for 30 s, 66°C for 30 s, 72°C for 2 min, and then 72°C for 7 min for elongation. The PCR products were purified using a DNA Purification Kit (CWBiotech, Beijing, China), and the purified products were subcloned into a pEASY-T1 vector (Transgen, China) and sequenced by Ruibiotech Company (Beijing, China). The sequences of all the primers used are displayed in Table 1.
2.4 Sequence and phylogenetic analysis
All the sequence data were analyzed using DNAStar 11.1. The open reading frame (ORF) of genes was predicted by the ORF finder. The conserved domains and genomic structures were analyzed using the online software Simple Modular Architecture Research Tool (SMART) (http://smart.embl.de/) and Splign (https://www.ncbi.nlm.nih.gov/sutils/splign/splign.cgi), respectively. Phylogenetic trees were constructed using MEGA 5.0 with the Neighbor-joining (NJ) method. Amino acid sequences from other species were downloaded from the GenBank database.
2.5 Quantitative real-time PCR
Primer Premier 5.0 was used to design specific primers for qPCR (Table 1). The qPCR was then conducted using the SYBR Premix Ex Taq™ kit (Takara, Japan) on a StepOnePlus Real-Time PCR system (Applied Biosystems) according to the instructions. β-actin was selected as the reference gene in the qPCR reaction system. The 2−∆∆CT method was used for the analysis of the relative gene expression.
2.6 Estradiol exposure
Estradiol regent was obtained from Sigma (St. Louis, MO, USA). The stock solution (4 mg/mL) of estradiol was prepared in ethanol. Two-year-old C. sinensis samples in the growing stage were selected for the experiment. The average length and body weight of the clams were in the range of 3.2−3.4 cm and 13−15 g, respectively. First, 180 healthy clams were randomly divided into three groups of 60 with three duplicates in each group. Clams were exposed to estradiol (E2: control group, 5 µg/L, and 50 µg/L; Wu, 2019). The same feeding regime was kept during the exposure. Uneaten food and feces were removed before water renewal. The water was changed daily, and fresh hormones were added. After 21 days of exposure, the gonad tissues of the clam were frozen in liquid nitrogen and stored at −80°C for RNA extraction. The remaining ovaries were fixed in 4% paraformaldehyde (PFA) overnight. Then, paraffin-embedded ovary samples were sliced on a microtome, and hematoxylin-eosin staining was carried out. Finally, ovary sections of 12 clams were observed under a Nikon 90i microscope, and the oocyte diameter was recorded.
2.7 Statistical analyses
All experimental data are expressed as mean ± SD and were analyzed using SPASS 23.0. The homogeneity of variance for all data was tested using Levene’s method. When the homogeneity variance was unsatisfactory, the percentage data were processed by taking the arcsine or square root. Statistical analysis was conducted using one-way analysis of variance (ANOVA) with Duncan’s test on the gene expression levels, and statistical significance was defined at P value <0.05.
3 Results
3.1 Sequence analysis of DMRT3 and FOXL2 in C. sinensis
In the study, the cDNA sequences of CsDMRT3 (GenBank accession No: OP970557) and CsFOXL2 (GenBank accession No: OP970558) were obtained by partial cDNA cloning and RACE technique. The ORF region of CsDMRT3 was 1137 bp, which encoded 378 amino acids containing the DM domain (16−69 aa) and DMA domain (189−228 aa; Figure 1A). The ORF sequence of CsFOXL2 was 1245 bp. The deduced amino acid sequence was 414 aa and contains the FH domain (177−267 aa; Figure 1B).
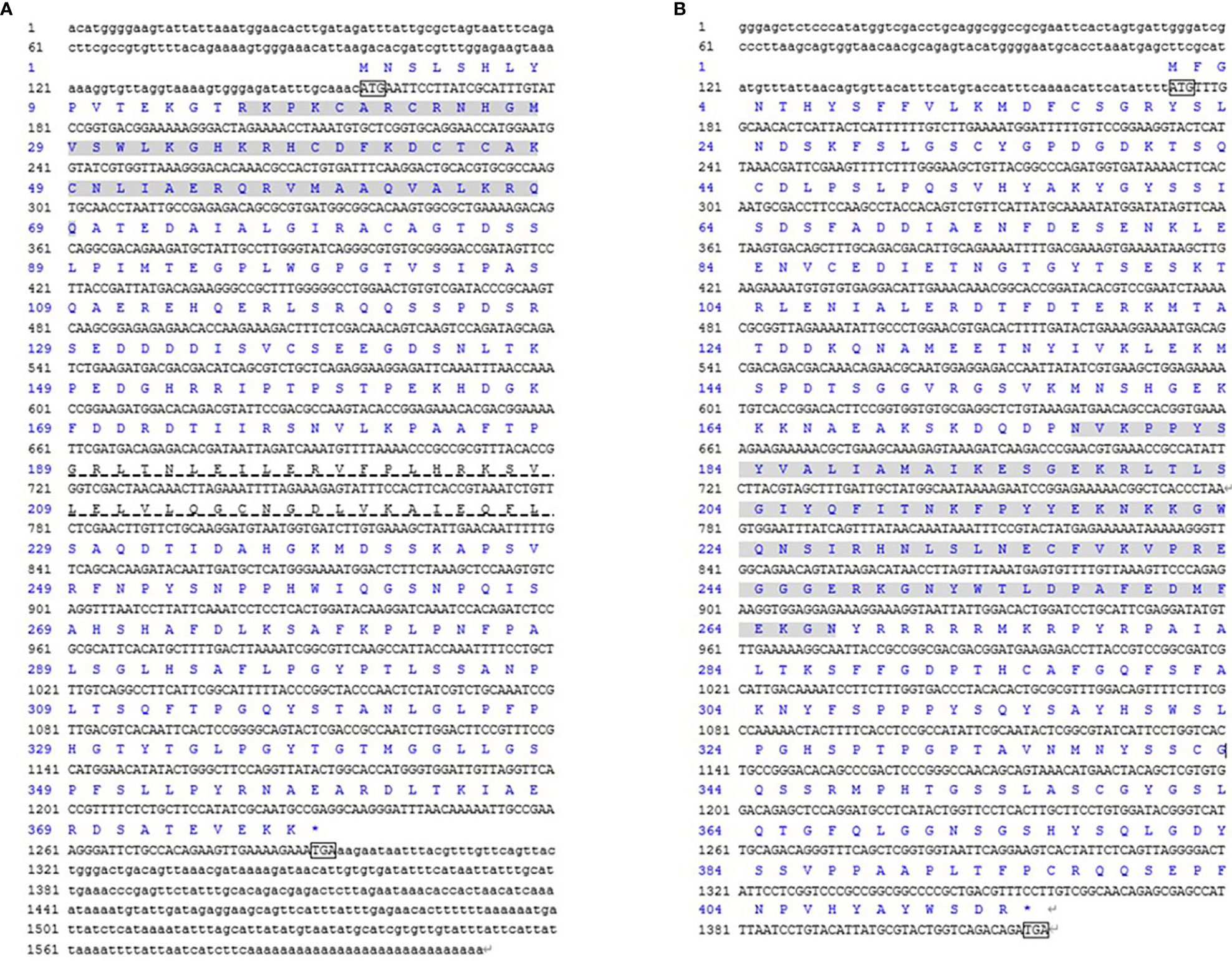
Figure 1 The DMRT3 (A) and FOXL2 (B) cDNA and deduced amino acid sequence of C. sinensis. The ATG/TGA indicated by the box starts codon/stop codon; shaded regions represent the DM domain and the FH domain, respectively. The DMA domain was indicated by virtual underline.
The predicted molecular weight of the CsDMRT3-encoded protein was 41.38 kDa, whereas the theoretical isoelectric point was 8.05. The α helix of the CsDMRT3 protein was 25.93%, the extended strand was 2.65%, the β strand was 3.17%, and the disordered was 68.25% (Figures 2A, C). Among the protein encoded by CsFOXL2, the proportions of α-helix, extended chain, β-turn, and irregular coiling were 27.54%, 8.94%, 5.80%, and 57.73%, respectively (Figures 2B, D). The predicted molecular weight of the CsFOXL2-encoded protein was 46.55 kDa, whereas the theoretical isoelectric point was 7.55.
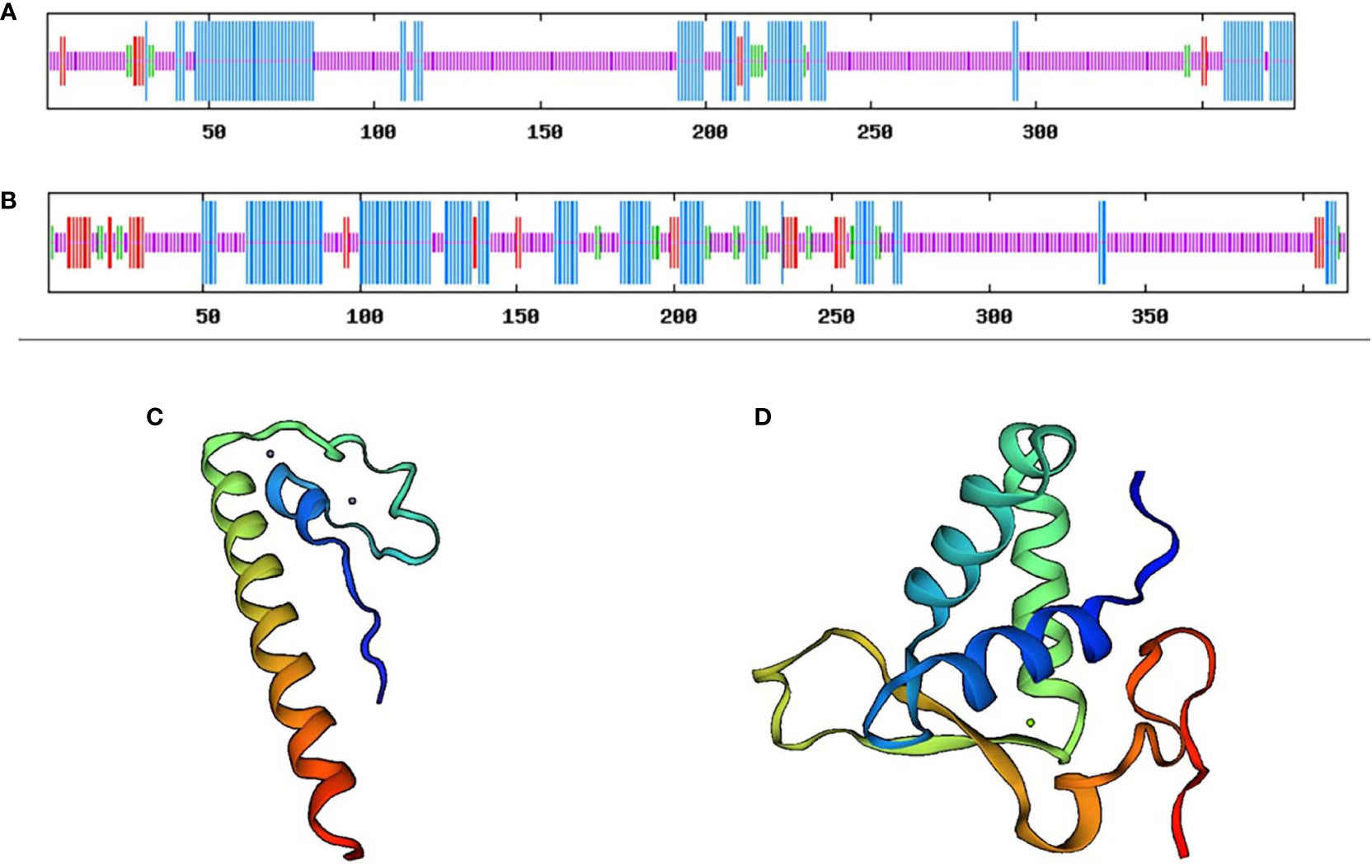
Figure 2 The secondary structure and tertiary structure prediction of DMRT3 (A) and FOXL2 (B) in C. sinensis. Blue represents the alpha helix, red represents the extended strand, green represents the beta-turn, and orange represents the random coil.
A comparison of the amino acid sequence encoded by the CsDMRT3 with other species (Figure 3A) showed that DMRT3 contains a conserved DM domain. The highest identity of CsDMRT3 with Mercenaria mercenaria was 85.1%, and it shares 40.5%, 48.4%, 48.7%, 35.8%, and 36.2% identity with homologs in Pomacea canaliculate, Aplysia californica, Crassostrea virginica, Acanthaster planci, and Limulus polyphemus, respectively. Therefore, DMRT3 is relatively conserved in shellfish, especially in bivalves. The comparison of the sequence of FOXL2 (Figure 3B) showed that the FH domain is also present in other species. CsFOXL2 shares 84.7%, 62.3%, 58.1%, 57.9%, 51.4%, and 49.0% identity with homologs in Mercenaria mercenaria, Dreissena polymorpha, Haliotis rubra, Gigantopelta aegis, Octopus bimaculoides, and Owenia fusiformis, respectively.
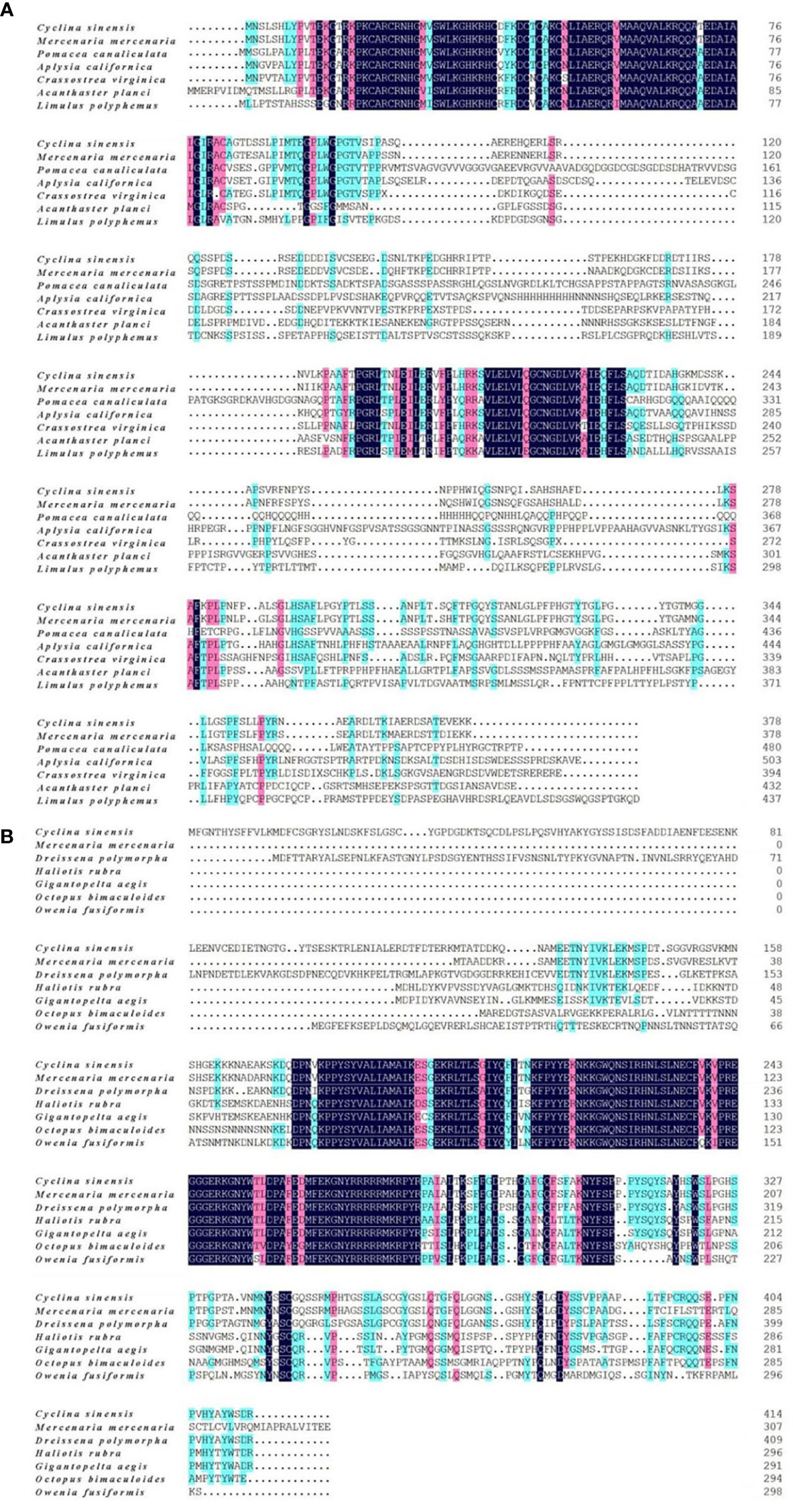
Figure 3 The amino acid alignment of DMRT3 (A) and FOXL2 (B) from C. sinensis and other species. Different colors represent residues sharing homology, cyan, pink and black regions indicate homology above 50%, above 75%, and equal to 100%, respectively.
Phylogenetic tree was constructed based on the sequence of the CsDMRT3 with 12 species by using the Neighbor-Joining method, and the results indicate that CsDMRT3 cluster with the other bivalves and stay farther away from humans and mice (Figure 4A). Phylogenetic analysis showed that CsFOXL2 formed a cluster with those of other bivalve shellfish species (Figure 4B).
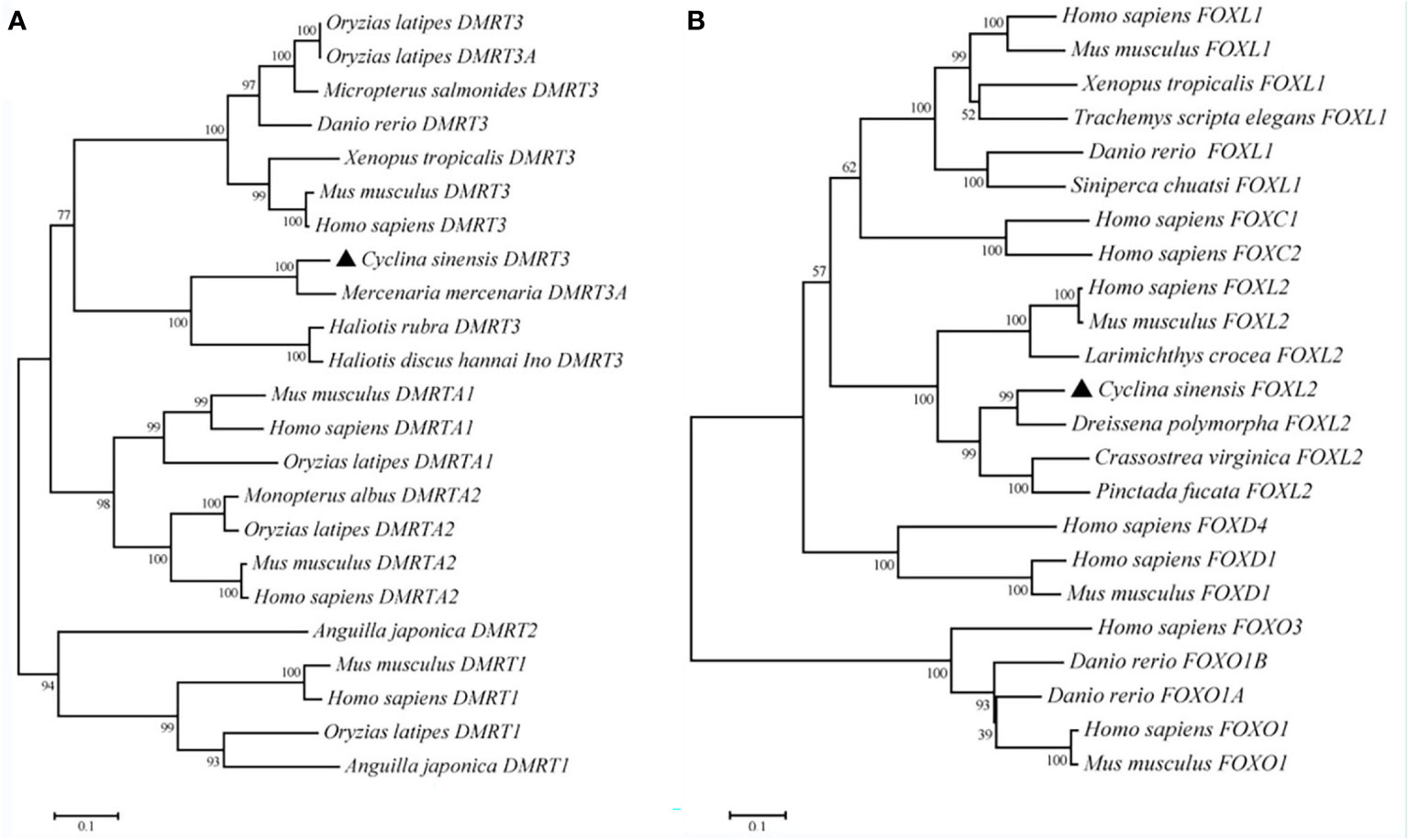
Figure 4 The phylogenetic tree of DMRT3 (A) and FOXL2 (B) from C. sinensis and other species. Note: C. sinensis were marked by a black triangle.
3.2 Expression levels of DMRT3 and FOXL2 in different tissues and gonadal development stages of C. sinensis
Tissue-specific expression results showed that the expression level of CsDMRT3 in the testes significantly higher than that of other tissues, including the hepatopancreas, ovary, and foot (Figure 5A). During the gonadal development of C. sinensis, the expression level of CsDMRT3 in the testis increased significantly from the proliferation stage to maturation stage, and reached the peak level at the maturation stage (Figure 6A). Subsequently, the expression of CsDMRT3 in the testis of C. sinensis continued to decline from spawning stage to spent stage (Figure 6A).
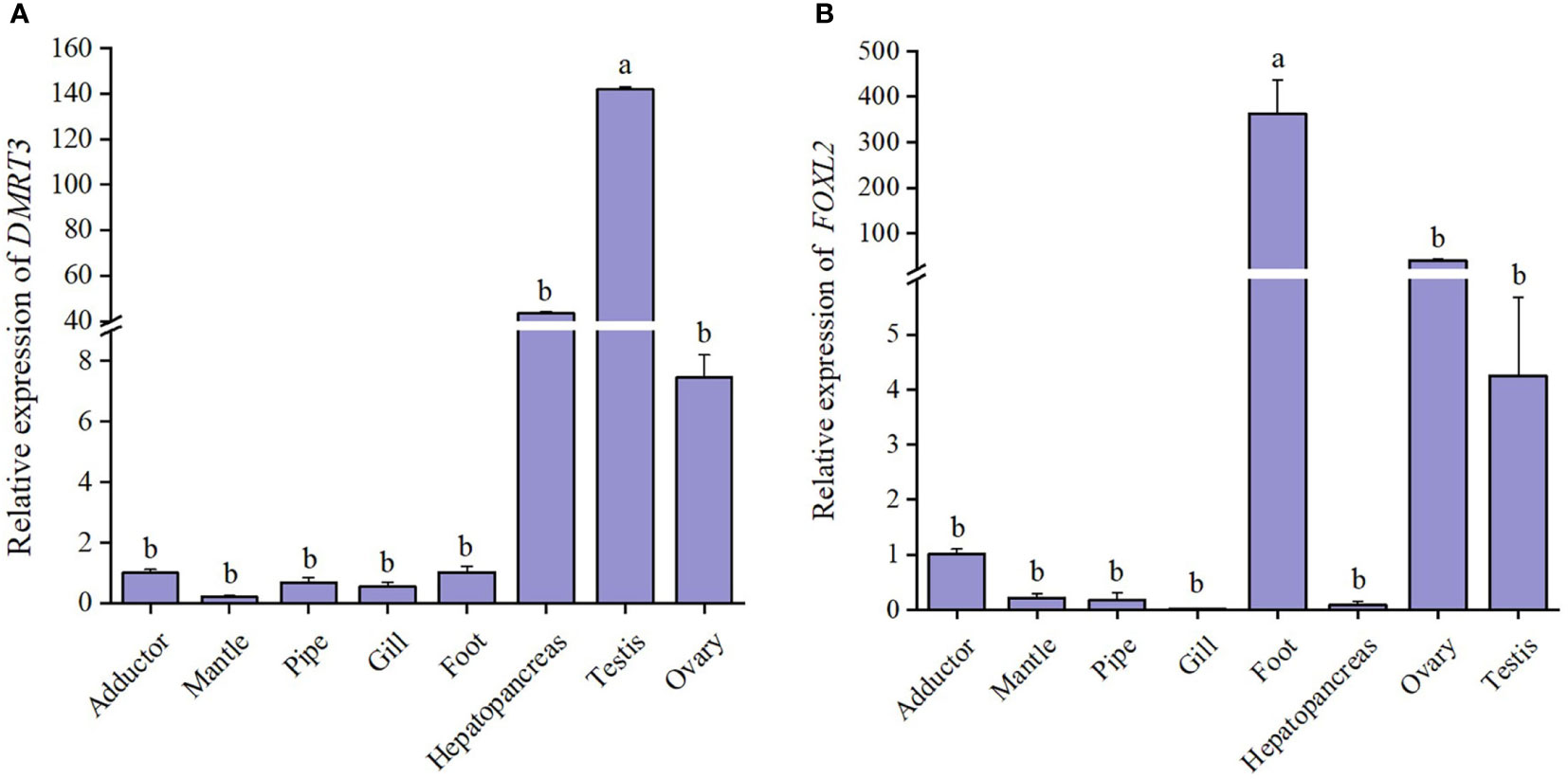
Figure 5 The relative expression levels of DMRT3 (A) and FOXL2 (B) in different tissues of C. sinensis. Different letters represent significant differences (P<0.05).
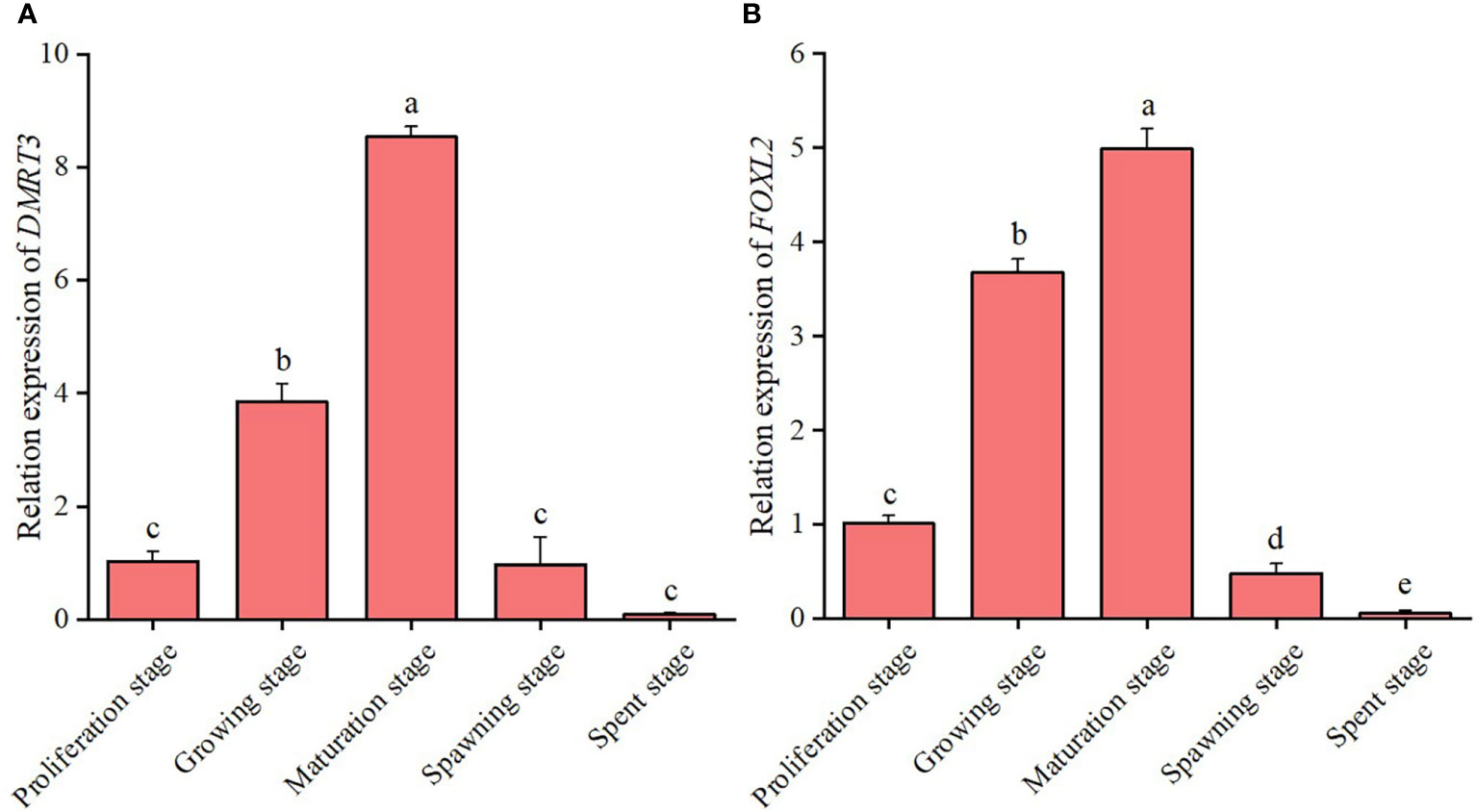
Figure 6 The relative expression levels of DMRT3 (A) and FOXL2 (B) in the different gonadal developmental stages of C. sinensis. The expression levels of DMRT3 and FOXL2 was only detected in testis and ovary, respectively. Different letters represent significant differences (P<0.05).
The present result showed that the highest expression level of CsFOXL2 was found in the foot of C. sinensis. Moreover, the results showed that the levels of CsFOXL2 in the ovaries of C. sinensis were higher than that in the testis (Figure 5B). Further analysis revealed that the expression level of CsFOXL2 in the ovary increased significantly from the proliferation stage to maturation stage, and reached the peak level at the maturation stage throughout the reproductive cycle of C. sinensis. Then, the expression level of CsFOXL2 in the ovary decreased significantly from spawning stage to spent stage. The lowest expression level of CsFOXL2 was found in the spent stage (Figure 6B).
3.3 Expression levels of DMRT3 and FOXL2 in larvae development and different months of C. sinensis
During the period of larval development of C. sinensis, CsDMRT3 had the lower expression level in fertilized egg and umbo larval stages. However, the expression level of CsDMRT3 in the trochophore and D-larval stages was significantly higher than that of other two stages. (Figure 7A). For the expression levels of CsDMRT3 in C. sinensis at different months of age, the results showed that the transcript level of CsDMRT3 in the gonads at different months of age showed a sharp increase from 5 months of age to 11 months of age. Moreover, the expression level of CsDMRT3 reached the lowest level in the gonads of 12-month-old C. sinensis (Figure 8A).
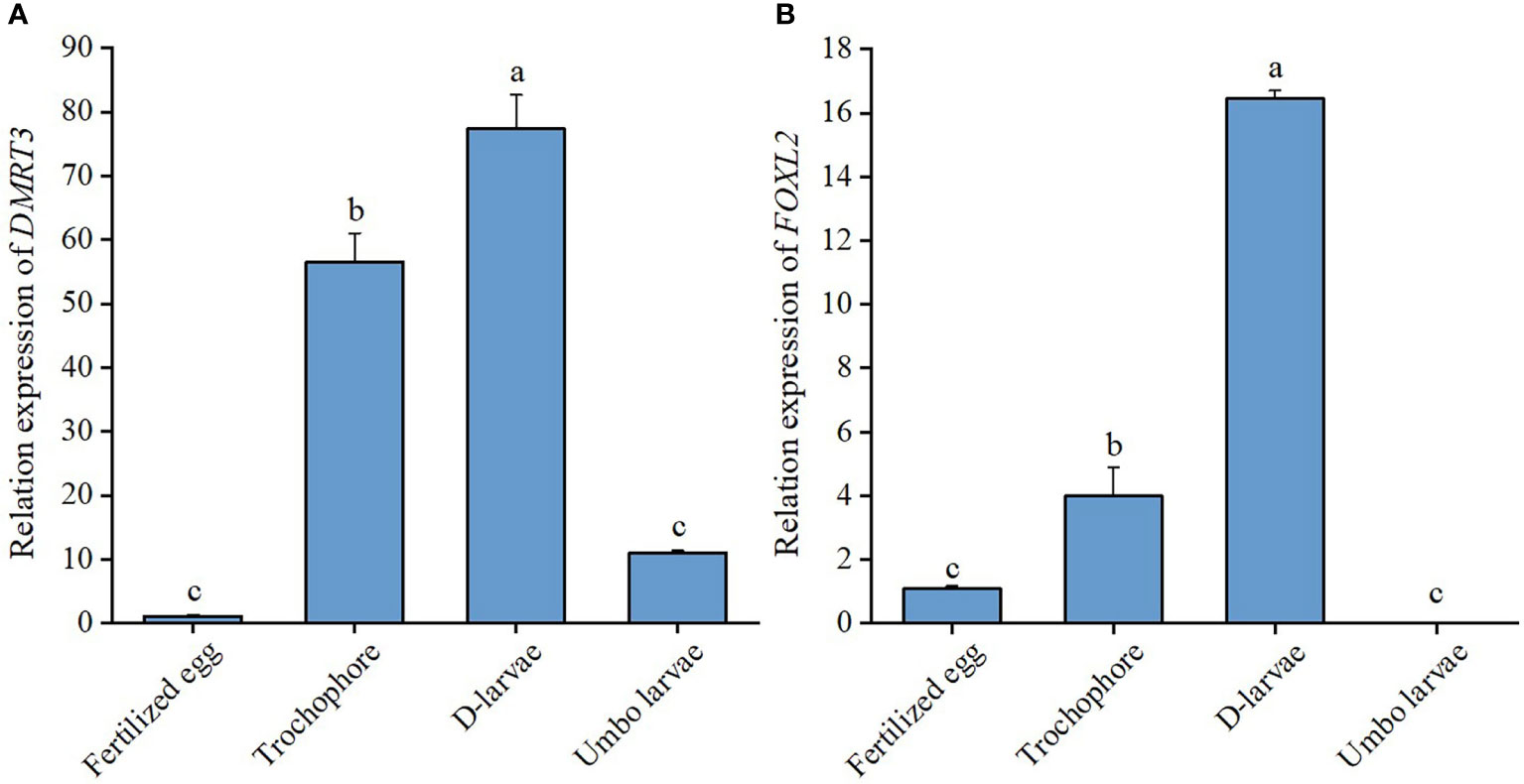
Figure 7 The relative expression levels of DMRT3 (A) and FOXL2 (B) in larval development stages of C. sinensis. Different letters represent significant differences (P<0.05).
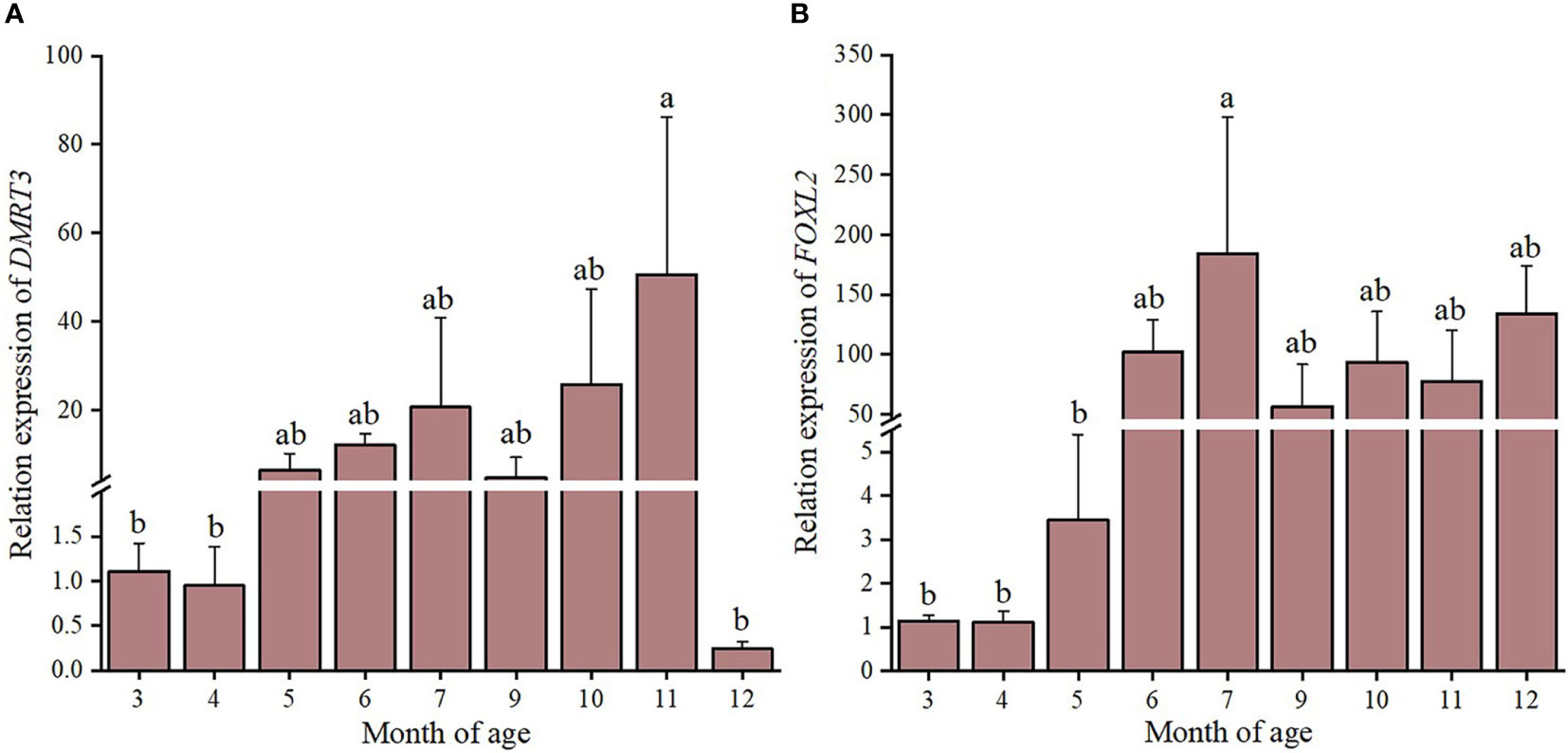
Figure 8 The relative expression levels of DMRT3 (A) and FOXL2 (B) in different months ages of C. sinensis. Different letters represent significant differences (P<0.05).
The expression level of CsFOXL2 increased significantly from the fertilized egg to D-larval stage, while the expression level of CsFOXL2 declined sharply in the umbo larval stage (Figure 7B). For the expression levels of CsFOXL2 in C. sinensis at different months of age, the results showed that the low transcript levels of CsFOXL2 were found in 3 to 5 months of age, increased at 6 months of age, and then dropped at 9 months of age (Figure 8B).
3.4 Expression levels of CsDMRT3 and CsFOXL2 in gonads after estradiol exposure
Results of estradiol exposure experiments showed that 5 µg/L estradiol treatment caused a significant increase in CsDMRT3 transcript level of male gonads. However, no significant changes in CsDMRT3 expression levels were observed at 50 µg/L estradiol treatment compared to the control group (Figure 9A). Moreover, estradiol treatment up-regulated the expression level of CsFOXL2 in female gonads compared to the control group (Figure 9B).
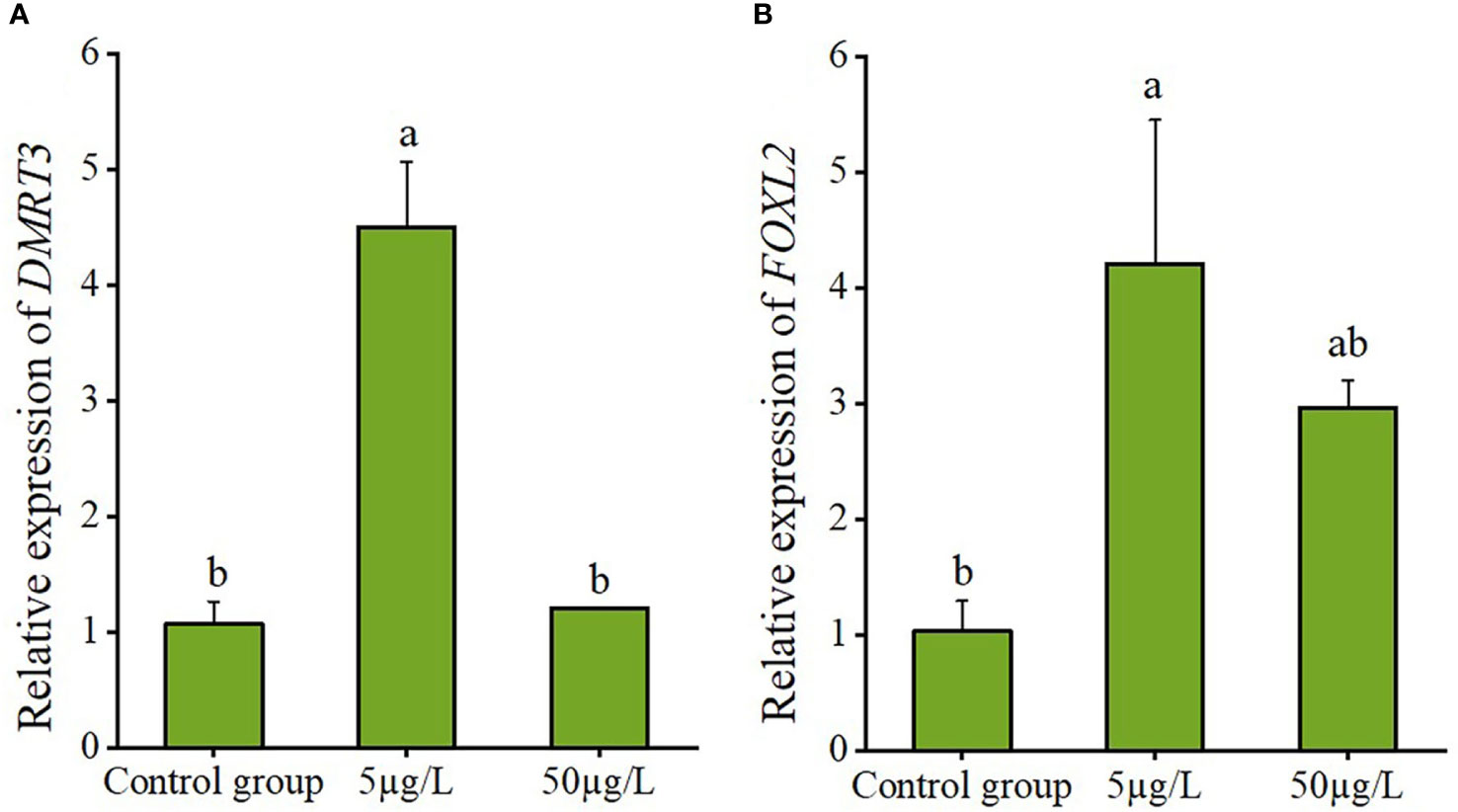
Figure 9 The relative expression levels of DMRT3 in the testis (A) and FOXL2 in the ovary (B) of C. sinensis under estradiol exposure treatments. Different letters represent significant differences (P<0.05).
3.5 Ovaries histological analysis of estradiol exposure
Histological examination showed that estradiol exposure promoted the ovarian development of C. sinensis (Figure 10). Specifically, the main type of germ cells of C. sinensis was pre-vitellogenesis oocyte (POI) and the cytoplasm of POI was basophilic in the control group (Figure 10A). In the estradiol group (5 µg/L estradiol, 50 µg/L estradiol), the main type of germ cells of C. sinensis was post-vitellogenesis oocytes (POL) and the cytoplasm of POL is eosinophilic (Figures 10B, C). Further analysis revealed that the mean long diameters of the oocytes in control group, 5µg/L group and 50 µg/L group were 42.62 ± 1.50 µm, 59.56 ± 2.22 µm, and 69.05 ± 1.99 µm, respectively. The short diameters of oocytes after different estradiol treatments were 28.23 ± 1.01 µm, 38.30 ± 1.29 µm, and 46.82 ± 1.52 µm (Table 2).
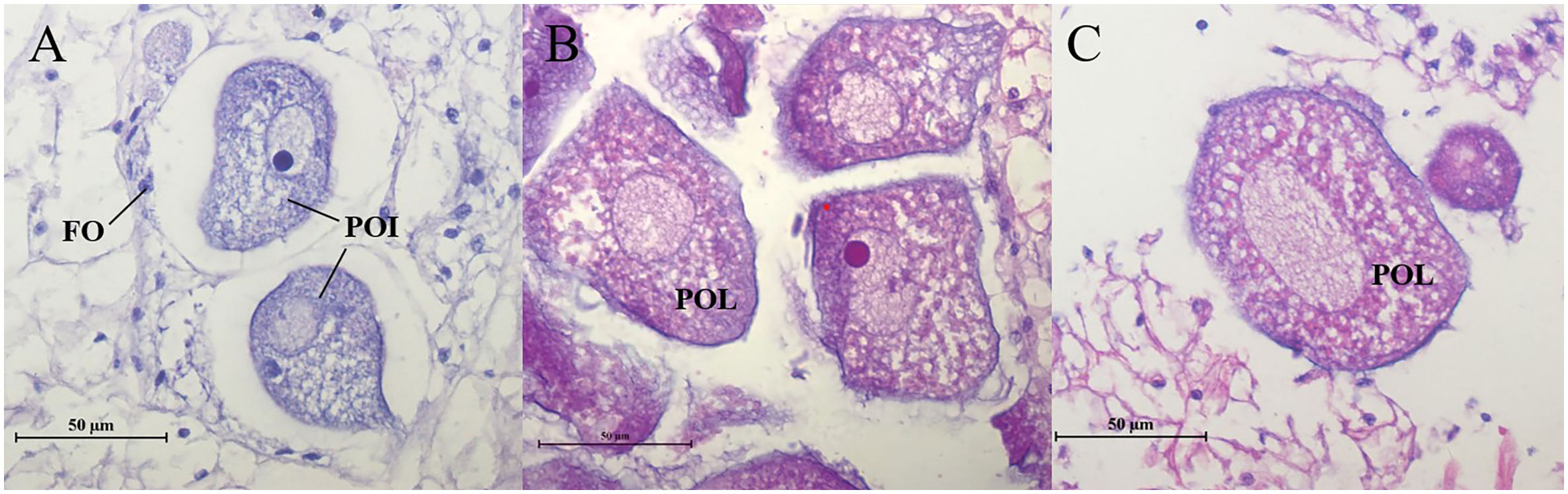
Figure 10 Ovaries histological characteristic of C. sinensis under estradiol exposure treatments. (A) control group, (B) 5 µg/L group, (C) 50 µg/L group. POI, pre-vitellogenesis oocyte; POL, post-vitellogenesis oocyte; FO, follicle cells.
4 Discussion
The DMRT family is a class of sex-determination and differentiation-related genes that are commonly found in organisms, and the genes in this family are highly conserved in invertebrates and vertebrates (Zhang et al., 2015; Craig et al., 2002). The DMRT family-specific DM domain, which binds specifically in a zinc finger-like manner, is the main functional domain in the gene sequence Guo et al. (2020). Sinclair et al. (1990) found that zinc finger proteins can bind to other regulated genes and then regulate downstream genes, ultimately affecting testis development. The present results showed that no transmembrane structure was detected for the CsDMRT3 protein, suggesting that the DMRT3 protein in C. sinensis is an intracellular protein. DMRT3 protein has also been reported in studies on largemouth bass Micropterus salmoides, mandarin fish Siniperca chuatsi, and swamp eel Monopterus albu. Furthermore, the DM and DMA domains were present in the protein sequence encoded by CsDMRT3, indicating that the structure of DMRT3 was conserved between vertebrate and invertebrates. Yu et al. (2007) found that 68% of the amino acid sequences in DM domains of DMRT were identical in 16 different evolutionary positions species.
The DMRT family is involved in sex determination and differentiation, gonadal development, and maintenance of organ function in organisms, and plays an important role in the development of individuals (Grandi et al., 2000; Loffler et al., 2003; Li et al., 2018). Previous experiments showed that DMRT3 was expressed only in the testes of Carassius auratus (Wang et al., 2014). However, DMRT3 transcripts have been detected in both testes and ovaries of zebrafish and Mandarin fish (Li et al., 2008; Han et al., 2021). Similar results were found in scallops and ctenophores (Feng et al., 2010; Zhao, 2021). Tissue distribution analysis showed that the expression level of CsDMRT3 in the testes significantly higher than in the ovary. The findings clarify that the expression level of CsDMRT3 shows sexual dimorphism. The expression level of CsDMRT3 in the testis reached the peak level at the maturation stage. The lowest expression level of CsDMRT3 was found in the spent stage. Studies on the oyster C. gigas and scallops Patinopecten yessoensis yielded similar results (Amine et al., 2009; Zhao, 2021). Therefore, it is hypothesized that DMRT3 is involved in the regulation of testis development and germ cell formation. The expression levels of CsDMRT3 in larvae of different developmental stages were examined by qPCR. Results show that the expression level of CsDMRT3 was the highest in the D-larval stage and low in the umbo larval stage, indicating that CsDMRT3 was mainly synthesized in the D-larval stage. Similar features were found in the embryonic development of zebrafish and mice, and this finding is presumably related to the formation of the nervous system (Shinseog et al., 2003; Li et al., 2008; Wang and Luo, 2014). The expression level of CsDMRT3 increased at 4 months of age and was highest at 11 months of age. Therefore, CsDMRT3 is involved in the growth and development of the testis.
FOXL2 has an important role in sex determination and differentiation, ovarian development and maintenance, embryonic development, and immune regulation in animals (He et al., 2020; Craig et al., 2005). Sequence analysis of the CsFOXL2 in the present study showed that CsFOXL2 contains an FH domain. The phylogenetic tree of the FOX family shows that C. gigas FOXL2 is clustered together with FOXL2. Therefore, FOXL2 has a highly conserved FH domain, which is typical of the fox family (Amine et al., 2009). The expression level of CsFOXL2 was highest in the foot. FOXL2 was highly expressed in the foot in the study of H. cumingii, indicating the possible existence of a mechanism in the foot that regulates sex determination and differentiation in clams (Wang et al., 2020). The transcript levels of CsFOXL2 were higher in the ovary than in the testis. The same result was observed in C. gigas, where the expression level of CsFOXL2 does not show sexual dimorphism (Amine et al., 2009). The expression level of CsFOXL2 during gonad development initially increased and then decreased from the proliferation stage to the spent stage. This finding is consistent with the expression characteristics of FOXL2 during scallop gonad development (Ning et al., 2021; Zhao, 2021). The involvement of CsFOXL2 in early ovarian development and oocyte differentiation can be hypothesized based on the trend of CsFOXL2 expression during ovarian development. In the present study, the expression level of CsFOXL2 during larval development was analyzed. The results showed that CsFOXL2 was transcribed in fertilized eggs, and Liu (2012) concluded that this phenomenon occurred, because FOXL2 was expressed maternally. CsFOXL2 was significantly highly expressed in the D-larval stage, but barely expressed in the umbo larvae stage. Similar results were observed for C. farreri and the bay scallop Argopecten irradians irradians (Liu et al., 2013). For the result, Ning et al. (2021) suggested that primordial germ cells (PGCs) may first appear in the D-larval stage. The D-larval stage is a period of rapid construction of larvae tissue in C. sinensis, including the initial formation of larval shells and visceral masses. Therefore, it is hypothesized that CsFOXL2 is involved in the formation of certain organs of the larvae. The expression level of CsFOXL2 reached its peak at 7 months of age. Therefore, CsFOXL2 is involved in ovarian growth and development.
Fluctuations in sex hormone levels in the organism as the sexual maturation cycle changes, suggest that sex hormones may participate in gonadal development (Reis-Henriques and Coimbra, 1990; Matsumoto et al., 1997; Shangguan et al., 2022). Estradiol injection promoted the growth of sea scallop oocytes, demonstrating that estrogen is involved in the reproductive process in invertebrates (Wang and Croll, 2004). The expression level of CsDMRT3 was significantly higher at estradiol: 5 µg/L compared with the control group. Combined with the specific expression of DMRT3 in the male gonads, it is hypothesized that DMRT3 is involved in the development and the maintenance of gonadal function in C. sinensis (Wu et al., 2019). After estradiol treatment, the expression level of CsFOXL2 peaked at estradiol concentration of 5 µg/L, which was significantly higher compared with the control group. The experimental results suggest that estradiol treatment induced the upregulation of CsFOXL2. It indicates that estradiol may play a role in ovarian development by stimulating FOXL2 to induce estrogenic feedback mechanism (Narisato et al., 2006; Li et al., 2014; Banh Quyen et al., 2021). However, the expression level of CsFOXL2 was decreased at estradiol concentration of 50 µg/L compared with estradiol concentration of 5 µg/L. Similar results were observed in C. elegans. Zhu et al. (2019) concluded that the uptake of exogenous estrogen by fry leads to high estradiol concentrations in the body, resulting in a negative feedback mechanism that inhibits the production of endogenous estrogens. In the present experiment, the diameter of oocytes in the ovaries of C. sinensis remarkably increased with increasing estradiol concentration. The findings of this study are consistent with the study of Wang and Croll, 2004.
The results showed that DMRT3 and FOXL2 were involved in the development and maintenance of gonad in different tissues and gonad stages of C. sinensis. CsDMRT3 may be a candidate regulator of male development. In addition, FOXL2 may interact with ESR in oyster gonads to inhibit the expression of genes related to male development (Sun et al., 2022). CsFOXL2 was barely expressed in the umbo larvae stage, while CsDMRT3 was expressed at different stages of larval development, suggesting that the broader function of CsDMRT3 in early development.
5 Conclusion
The present study showed that the expression level of DMRT3 in C. sinensis was sexually dimorphic, indicating that DMRT3 is likely to be involved in male gonad development and germ cell formation. FOXL2 is closely associated with early organogenesis and ovarian development in C. sinensis. In addition, estradiol exposure stimulated ovarian development and oocyte growth. The results of this study provide valuable information for the subsequent study of sex determination and gonadal development of clam C. sinensis.
Data availability statement
The datasets presented in this study can be found in online repositories. The the cDNA sequences of CsDMRT3 can be found at GenBank, https://www.ncbi.nlm.nih.gov/genbank/, accession No: OP970557 and the sequences of CsFOXL2 can be found at GenBank, accession No: OP970558. Further inquiries should be directed to the corresponding author.
Ethics statement
The animal study was reviewed and approved by the Animal Experiment Ethics Committee at Jiangsu Ocean University.
Author contributions
SY: experimental design, formal analysis, and writing - original draft. MX: data curation, and validation. JX: data curation. XL: experimental design and data curation. ML: experimental design and formal analysis. SW: formal analysis. SF: experimental design. ZD: writing-editing and funding acquisition. All authors contributed to the article and approved the submitted version.
Funding
The work was financially supported by Jiangsu Natural Resources Development Special-Marine Science and Technology Innovation Project (JSZRHYKJ202008); Modern Agro-industry Technology Research System (CARS-49); The ‘JBGS’ Project of Seed Industry Revitalization in Jiangsu Province (JBGS[2021]034); Postgraduate Research & Practice Innovation Program of Jiangsu Province (SJCX201267); Jiangsu Graduate Research and Practice Innovation Program (KYCX2021-035).
Conflict of interest
The authors declare that the research was conducted in the absence of any commercial or financial relationships that could be construed as a potential conflict of interest.
Publisher’s note
All claims expressed in this article are solely those of the authors and do not necessarily represent those of their affiliated organizations, or those of the publisher, the editors and the reviewers. Any product that may be evaluated in this article, or claim that may be made by its manufacturer, is not guaranteed or endorsed by the publisher.
References
Akihiko Y., Kyung H. L., Hiromi F., Kazushi K., Susumu Y., Michiya M. (2006). Expression of the DMRT gene and its roles in early gonadal development of the Japanese pufferfish Takifugu rubripes. Comp. Biochem. Physiol. D. 1 (1), 59–68. doi: 10.1016/j.cbd.2005.08.003
Amine N., Anne-Sophie M., Marie-Laure S., Abdellah M., Blandine D., Michel M., et al. (2009). Identification and expression of a factor of the DM family in the oyster Crassostrea gigas. Comp. Biochem. Physiol. A. 152 (2), 189–196. doi: 10.1016/j.cbpa.2008.09.019
Banh Quyen Q. T., Guppy Jarrod L., Domingos Jose A., Budd Alyssa M., Pinto Ricardo C. C., Marc Adrien F. (2021). Induction of precocious females in the protandrous barramundi (Lates calcarifer) through implants containing 17β-estradiol-effects on gonadal morphology, gene expression and DNA methylation of key sex genes. Aquaculture 539 (1), 736601. doi: 10.1016/j.aquaculture.2021.736601
Cao J. L., Chen J. J., Wu T. T., Gan X., Luo Y. J. (2010). Molecular cloning and sexually dimorphic expression of DMRT4 gene in Oreochromis aureus. Mol. Biol. Rep. 37 (6), 2781–2788. doi: 10.1007/s11033-009-9820-z
Chris O., Shakib O., J Elias G., Manuela U., Laura C., Antonino F., et al. (2005). Foxl2 is required for commitment to ovary differentiation. Hu. Mol. Genet. 14 (14), 2053–2062. doi: 10.1093/hmg/ddi210
Christelle S., Pascal S., Béatrice A., Anne-Sophie M. (2014). Cg-SoxE and cg-β-catenin, two new potential actors of the sex-determining pathway in a hermaphrodite lophotrochozoan, the pacific oyster Crassostrea gigas. Comp. Biochem. Physiol. A. 167, 68–76. doi: 10.1016/j.cbpa.2013.09.018
Cocquet J., Pailhoux E., Jaubert F., Servel N., Xia X., Pannetier M., et al. (2002). Evolution and expression of FOXL2. J. Med. Genet. 39 (12), 916–921. doi: 10.1136/jmg.39.12.916
Craig R. M., Kevin P., Mark Q. M. (2005). Genomic inventory and expression of sox and fox genes in the cnidarian Nematostella vectensis. Dev. Genes. Evol. 215 (12), 618–630. doi: 10.1007/s00427-005-0022-y
Craig A. S., Tanya M. H., Peter J M., Andrew H S. (2002). Retracted: restricted expression of dmrt3 in chicken and mouse embryos. Gene. Expr. Patterns. 2 (1-2), 69–72. doi: 10.1016/S0925-4773(02)00360-X
Dong Z. G., Duan H. B., Zheng H. F., Ge H. X., Wei M., Liu M. M., et al. (2021). Research progress in genetic resources assessment, culture technique and exploration utilization of Cyclina sinensis. J. Fish. China. 45 (12), 2083–2098. doi: 10.11964/jfc.20201212545
Feng Z. F., Shao M. Y., Sun D. P., Zhang Z. F. (2010). Cloning, characterization and expression analysis of cf-dmrt4-like gene in Chlamys farrei. J. Fish. Sci. China. 17 (05), 930–940.
Govoroun M. S., Pannetier M., Pailhoux E., Cocquet J., Brillard J., Couty I., et al. (2004). Isolation of chicken homolog of the FOXL2 gene and comparison of its expression patterns with those of aromatase during ovarian development. Dev. Dynam. 231 (4), 859–870. doi: 10.1002/dvdy.20189
Grandi A. D., Calvari V., Bertini V., Bulfone A., Peverali G., Camerino G., et al. (2000). The expression pattern of a mouse doublesex -related gene is consistent with a role in gonadal differentiation. Mech. Dev. 90 (2), 323–326. doi: 10.1016/s0925-4773(99)00282-8
Guo P. F., Duan S. H., Dong S. S., Wu C. D., Wang G. L. (2020). Molecular characterization and expression analysis of Dmrt1 gene in Hyriopsis cumingii. GAB 39 (5), 2033–2041. doi: 10.13417/j.gab.039.002033
Han C., Wang C. W., Ouyang H. F., Zhu Q. Y., Huang J. J., Han L. Q. (2021). Characterization of dmrts and their potential role in gonadal development of mandarin fish (Siniperca chuatsi). Aquacult. Rep. 21. doi: 10.1016/j.aqrep.2021.100802
Hanne J., Øivind A. (2012). Sex dimorphic expression of five dmrt genes identified in the Atlantic cod genome. the fish-specific dmrt2b diverged from dmrt2a before the fish whole-genome duplication. Gene 505 (2), 221–232. doi: 10.1016/j.gene.2012.06.021
He J. Q., Zhang H., She Z. C., Zhen W. Q., You S. Y., Wang P. L. (2020). Cloning and expression pattern investigation of crassostrea hongkongensis Foxl2 gene. GAB 39 (04), 1519–1528. doi: 10.13417/j.gab.039.001519
Hildreth P. E. (1965). Doublesex, recessive gene that transforms both males and females of Drosophilid into intersexes. Genetics 51 (4), 659–678. doi: 10.1093/genetics/51.4.659
Hu B., Cui Z. P., Wei J. L., Zhang J., Wang Y., Zhang W. J., et al. (2021). Effects of exogeneous hormone on expression of dmrt1, foxl2, and boule genes in gonads of Sea urchin Mseocentrotus nudus. Chin. J. Fish. 34 (04), 1–6. doi: 10.3969/j.issn.1005-3832.2021.04.001
Li H. L., Liu J. G., Huang X. T., Wang D., Zhang Z. F. (2014). Characterization, expression and function analysis of DAX1 gene of scallop (Chlamys farreri jones and preston 1904) during its gametogenesis. J. Ocean. U. China 13 (4), 696–704. doi: 10.1007/s11802-014-2299-9
Li R. J., Zhang L. L., Li W. R., Zhang Y., Li Y. P., Zhang M. W., et al. (2018). FOXL2 and DMRT1L are yin and yang genes for determining timing of sex differentiation in the bivalve mollusk Patinopecten yessoensis. Front. Physiol. 9. doi: 10.3389/fphys.2018.01166
Li Q., Zhou X., Guo Y. Q., Shang X., Chen H., Lu H., et al. (2008). Nuclear localization, DNA binding and restricted expression in neural and germ cells of zebrafish Dmrt3. Biol. Cell. 100 (8), 453–463. doi: 10.1042/bc20070114
Liao X. T. (2022). Study on the gonadal development and expression of sex related genes Dmrt1 and Foxl2 in clam Cyclina sinensis. Master’s thesis of Jiangsu Ocean University. 1, 1–63. doi: 10.44354/d.cnki.gjsuy.2022.000148
Liao X. T., Sun Z. P., Cui Z. Q., Yan S. S., Fan S. S., Xia Q., et al. (2022). Effects of different sources of diet on the growth, survival, biochemical composition and physiological metabolism of clam (Cyclina sinensis). Aquac. Res. 10, 53. doi: 10.1111/are.15886
Liu X. L. (2012). Molecular cloning, expression pattern and function analysis of cf-foxl2 in the scallop Chlamys farreri. Doctoral Thesis of Ocean University of China. 1, 1–103.
Liu X. L., Liu J. G., Wang D., Zhang Z. Y., Zhang Z. F. (2013). Expression pattern of the foxl2 gene in the scallop Chlamys farreri during development. J. Fish. Sci. China 20 (01), 206–211. doi: 10.3724/SP.J.1118.2013.00205
Loffler K. L., Zarkower D., Koopman P. (2003). Etiology of ovarian failure in blepharophimosis ptosis epicanthus inversus syndrome: FOXL2 is a conserved, early-acting gene in vertebrate ovarian development. Endocrinology 144 (7), 3237–3243. doi: 10.1210/en.2002-0095
Matsumoto T., Osada M., Osawa Y., Mori K. (1997). Gonadal estrogen profile and immunohistochemical localization of steroidogenic enzymes in the oyster and scallop during sexual maturation. Comp. Biochem. Physiol. B. 118 (4), 811–817. doi: 10.1016/S0305-0491(97)00233-2
Narisato H., Ayumi N., Masaaki K., Takuya K., Masatoshi M., Norihisa T. (2006). Feminization of Japanese medaka (Oryzias latipes) exposed to 17beta-estradiol: formation of testis-ova and sex-transformation during early-ontogeny. Aquat. Toxicol. 77 (1), 78–86. doi: 10.1016/j.aquatox.2005.11.001
Ni J. B., Zeng Z., Ke C. H. (2012). Sex steroid levels and expression patterns of estrogen receptor gene in the oyster Crassostrea angulata during reproductive cycle. Aquaculture 376-379, 105–116. doi: 10.1016/j.aquaculture.2012.11.023
Ning J. H., Cao W. A., Lu X., Chen M., Liu B., Wang C. D. (2021). Identification and functional analysis of a sex-biased transcriptional factor Foxl2 in the bay scallop Argopecten irradians irradians. Comp. Biochem. Physiol. B. 256, 11068. doi: 10.1016/j.cbpb.2021.110638
Racotta I. S., Ramirez J. L., Ibarra A. M., Rodrıguez-Jaramillo M. C., Carreño D., Palacios E. (2003). Growth and gametogenesis in the lion-paw scallop Nodipecten (Lyropecten) subnodosus. Aquaculture 217 (1-4), 0–349. doi: 10.1016/s0044-8486(02)00366-6
Reis-Henriques M. A., Coimbra J. (1990). Variations in the levels of progesterone in Mytilus edulis during the annual reproductive cycle. Comp. Biochem. Physiol. A. 95 (3), 343–348. doi: 10.1016/0300-9629(90)90230-P
Shangguan X. Z., Mao Y. R., Wang X. Q., Liu M. L., Wang Y. Y., Wang J. L., et al. (2022). Cyp17a effected by endocrine disruptors and its function in gonadal development of Hyriopsis cumingii. Gen. Comp. Endocrinol. 323-324, 114028. doi: 10.1016/j.ygcen.2022.114028
Shi Y., Wang Q., He M. X. (2014). Molecular identification of dmrt2 and dmrt5 and effect of sex steroids on their expressions in Chlamys nobili. Aquaculture 426, 21–30. doi: 10.1016/j.aquaculture.2014.01.021
Shinseog K., Jae R K., Robert C A., Vivian J B., David Z. (2003). Sexually dimorphic expression of multiple doublesex -related genes in the embryonic mouse gonad. Gene. Expr. Patterns 3 (1), 77–82. doi: 10.1016/S1567-133X(02)00071-6
Sinclair A. H., Berta P., Palmer M. S., Hawkins J. R., Griffiths B. L., Smith M. J. (1990). A gene from the human sex-determining region encodes a protein with homology to a conserved DNA-binding motif. Nature 346 (6281), 240–244. doi: 10.1038/346240a0
Sun D. F., Yu H., Li Q. (2022). Examination of the roles of foxl2 and dmrt1 in sex differentiation and gonadal development of oysters by using RNA interference. Aquaculture 548, 737732. doi: 10.1016/J.AQUACULTURE.2021.737732
Tian Y., Zhang B. L., Chang Y. Q. (2012). Cloning and bioinformatics analysis of aromatization gene P450c17 in sea cucumber Apostichopus japonicus (Selenka). J. Fish. Sci. China 19 (01), 22–32. doi: 10.3724/SP.J.1118.2012.00022
Tong Y., Zhang Y., Huang J. M., Xiao S., Zhang Y. H., Li J., et al. (2015). Transcriptomics analysis of Crassostrea hongkongensis for the discovery of reproduction-related genes. PloS. One 10 (8), e0134280. doi: 10.1371/journal.pone.0134280
Tu Q., Brown C. T., Davidson E. H., Paola O. (2006). Sea Urchin forkhead gene family: phylogeny and embryonic expression. Dev. Biol. 300 (1), 49–62. doi: 10.1016/j.ydbio.2006.09.031
Wang C. D., Croll R. P. (2004). Effects of sex steroids on gonadal development and gender determination in the sea scallop, Placopecten magellanicus. Aquaculture 238 (1–4), 0–498. doi: 10.1016/j.aquaculture.2004.05.024
Wang G. L., Dong S. S., Guo P. F., Cui X. Y., Duan S. H., Li J. L. (2020). Identification of Foxl2 in freshwater mussel Hyriopsis cumingii and its involvement in sex differentiation. Gene 754, 144853. doi: 10.1016/j.gene.2020.144853
Wang L., Hu T., Fu M. D., Yang K. Z., Qian G. Y., Ge C. T. (2014). The effects of estrogen on gonadal differentiation and expression of dmrt1 and sox9 in pelodiscus sinensis. Acta Hydrobiol. Sin. 38 (03), 467–473. doi: 10.7541/2014.66
Wang J., Luo C. (2014). Molecular cloning and expression analysis of dmrt3 in goldfish, Carassius auratus. Acta Hydrobiol. Sin. 38 (03), 548–555. doi: 10.7541/2014.77
Wei M., Ge H. X., Shao C. W., Yan X. W., Nie H. T., Duan H. B., et al. (2020). Chromosome-level genome assembly of the Venus clam, Cyclina sinensis, helps to elucidate the molecular basis of the adaptation of buried life. iScience 23 (6), 101148. doi: 10.1016/j.isci.2020.101148
Wu Q. D. (2019). Molecular mechanism of sex differentiation of Ruditapes philippinarum under the action of estradiol. Master’s thesis of Dalian Ocean University. 3, 1–159. doi: 10.27821/d.cnki.gdlhy.2019.000196
Wu Q. D., Tan Y., Wang J. T., Xie Q. Y., Huo M. Z., Fang L. (2019). Effect of estradiol stimulation on dmrt gene expression in Manila clam Ruditapes philippinarum. J. Dalian. Univ. 34 (03), 362–369. doi: 10.16535/j.cnki.dlhyxb.2019.03.009
Yan H. W., Li Q., Liu W. G., Ke Q. Z., Yu R. H., Kong L. F. (2011). Seasonal changes of oestradiol-17 and testosterone concentrations in the gonad of the razor clam Sinonovacula constricta (Lamarck 1818). J. Mollus. Stud. 77 (2), 116–122. doi: 10.1093/mollus/eyq045
Yu F. F., Zhou L., Wang M. F., Yu X. Y., Gui J. F. (2007). Cloning and sequence analysis of three DM domain in Pinctada martensii. J. Agric. Biotechnol. 2007 (05), 905–906. doi: 10.3969/j.issn.1674-7968.2007.05.034
Yuki O., Yoshinobu U., Yoichi M., Tohru K., Masahisa N. (2008). Molecular cloning and gene expression of Foxl2 in the frog Rana rugosa. Gen. Comp. Endocrinol. 159 (2-3), 170–177. doi: 10.1016/j.ygcen.2008.08.013
Zhang N., Huang W., Xu F., Li L., Zhang G. F., Guo X. M. (2015). Expression of two dmrt family genes in the pacific oyster Crassostrea gigas. Oceanol. Limnol. Sin. 46 (03), 717–724. doi: 10.11693/hyhz20140900249
Zhao D. (2021). Expression characteristics and function of genes Dmrt1 and Foxl2 in the scallop Patinopecten yessoensis. Master’s thesis of Shanghai Ocean University. 1, 1–73. doi: 10.27314/d.cnki.gsscu.2021.000103
Keywords: Cyclina sinensis, DMRT3, FOXL2, expression analysis, estradiol response
Citation: Yan S, Xu M, Xie J, Liao X, Liu M, Wang S, Fan S and Dong Z (2023) Characterization, expression profiling, and estradiol response analysis of DMRT3 and FOXL2 in clam Cyclina sinensis. Front. Mar. Sci. 10:1120015. doi: 10.3389/fmars.2023.1120015
Received: 09 December 2022; Accepted: 06 February 2023;
Published: 17 February 2023.
Edited by:
Yafei Duan, South China Sea Fisheries Research Institute, ChinaReviewed by:
Yongbo Bao, Zhejiang Wanli University, ChinaYuehuan Zhang, South China Sea Institute of Oceanology (CAS), China
Zhiyi Bai, Shanghai Ocean University, China
Copyright © 2023 Yan, Xu, Xie, Liao, Liu, Wang, Fan and Dong. This is an open-access article distributed under the terms of the Creative Commons Attribution License (CC BY). The use, distribution or reproduction in other forums is permitted, provided the original author(s) and the copyright owner(s) are credited and that the original publication in this journal is cited, in accordance with accepted academic practice. No use, distribution or reproduction is permitted which does not comply with these terms.
*Correspondence: Zhiguo Dong, dzg7712@163.com