- 1European Marine Biological Resource Centre (EMBRC-ERIC), Paris, France
- 2Institute of Marine Biology, Biotechnology and Aquaculture (IMBBC), Hellenic Centre for Marine Research (HCMR), Heraklion, Greece
- 3Genoscope, Institut François Jacob, Commissariat à l’Energie Atomique (CEA), Université Paris-Saclay, Evry, France
- 4Alfred Wegener Institute, Helmholtz Centre for Polar and Marine Research, Bremerhaven, Germany
- 5Helmholtz Metadata Collaboration, GEOMAR Helmholtz Centre for Ocean Research, Kiel, Germany
- 6Integrative Marine Ecology Department, Stazione Zoologica Anton Dorn, Naples, Italy
- 7Centro de Ciências do Mar, Universidade do Algarve, Faro, Portugal
- 8Marine Biological Association of the United Kingdom, Plymouth, United Kingdom
- 9School of Biological and Marine Sciences, University of Plymouth, Plymouth, United Kingdom
- 10Gump South Pacific Research Station, University of California Berkeley, Moorea, French Polynesia
- 11Department of Zoology and Animal Cell Biology, Faculty of Science and Technology and Research Centre for Experimental Marine Biology and Biotechnology Plentziako itsas Estazioa, University of the Basque Country (PiE-UPV/EHU), University of the Basque Country (UPV/EHU), Biscay, Spain
- 12Flanders Marine Institute [Vlaams Instituut voor de Zee (VLIZ)], Oostende, Belgium
- 13Adaptation and Diversity in Marine Environment laboratory (UMR7144), Station Biologique de Roscoff, Centre national de la recherche scientifique (CNRS) and Sorbonne University, Roscoff, France
- 14Department of Marine Sciences, University of Gothenburg, Göteborg, Sweden
- 15Department of Biological Sciences, The George Washington University, Washington, DC, United States
- 16Génomique Métabolique, Genoscope, Institut François Jacob, Commissariat à l'Energie Atomique et aux énergies alternatives (CEA), Centre national de la recherche scientifique (CNRS), Université Evry, Université Paris-Saclay, Evry, France
- 17Norwegian College of Fishery Science, UiT The Arctic University of Norway, Tromsø, Norway
- 18Marine Ecology and Management, Operational Directorate Natural Environment, Royal Belgian Institute of Natural Sciences, Brussels, Belgium
Marine ecosystems, ranging from coastal seas and wetlands to the open ocean, accommodate a wealth of biological diversity from small microorganisms to large mammals. This biodiversity and its associated ecosystem function occurs across complex spatial and temporal scales and is not yet fully understood. Given the wide range of external pressures on the marine environment, this knowledge is crucial for enabling effective conservation measures and defining the limits of sustainable use. The development and application of omics-based approaches to biodiversity research has helped overcome hurdles, such as allowing the previously hidden community of microbial life to be identified, thereby enabling a holistic view of an entire ecosystem’s biodiversity and functioning. The potential of omics-based approaches for marine ecosystems observation is enormous and their added value to ecosystem monitoring, management, and conservation is widely acknowledged. Despite these encouraging prospects, most omics-based studies are short-termed and typically cover only small spatial scales which therefore fail to include the full spatio-temporal complexity and dynamics of the system. To date, few attempts have been made to establish standardised, coordinated, broad scaled, and long-term omics observation networks. Here we outline the creation of an omics-based marine observation network at the European scale, the European Marine Omics Biodiversity Observation Network (EMO BON). We illustrate how linking multiple existing individual observation efforts increases the observational power in large-scale assessments of status and change in biodiversity in the oceans. Such large-scale observation efforts have the added value of cross-border cooperation, are characterised by shared costs through economies of scale, and produce structured, comparable data. The key components required to compile reference environmental datasets and how these should be linked are major challenges that we address.
1 Introduction
The Earth’s biosphere is under immense, multi-faceted, pressure from human activities. Greenhouse gas emissions driving rapid climate change, unprecedented rates of habitat destruction, and the intense pollution of the seas, air, and land, are causing complex changes to the ecosystems we rely on for survival (Harley et al., 2006; Nesmith et al., 2021). The European Union’s political will to combat environmental damage is growing and is reflected in ambitious plans to reduce environmental impact and mitigate climate change, and pledges to restore degraded ecosystems (Directorate-General for Environment European Commission, 2021). Societies require focused, high-quality, and reliable ecosystem assessments, with regional coverage, to rapidly detect the impact of management and policy decisions on biodiversity.
The ocean includes more than 96% of the Earth’s water resources (National Oceanic and Atmospheric Administration, 2021). The value of ocean-related activities was estimated to be $1.5 trillion in 2010 and was projected to reach $3 trillion by 2030 (Organization for Economic Co-operation and Development, 2016). A more recent estimation of the annual gross marine product value was at least $2.5 trillion per year (Hoegh-Guldberg, 2015; Sumaila et al., 2021). The importance of continuous ocean observation was highlighted at least 20 years ago (Dexter and Summerhayes, 2010 and references therein; Task Team for an Integrated Framework for Sustained Ocean Observing, 2012 and references therein) and several initiatives have been established since that time (Speich et al., 2019). Physico-chemical ocean observation has evolved over time, and today has become fully operational, standardised, and coordinated from the regional to the global level. The EU’s Copernicus Marine Service and the Euro-Argo European Research Infrastructure Consortium (ERIC) are outstanding examples of how the physics and chemistry of the ocean are monitored in Europe. Under the United Nations Educational, Scientific and Cultural Organisation (UNESCO) umbrella, the Global Ocean Observing System (GOOS) includes international coordinated networks for long-term ocean observation. One of the challenges of the United Nations Decade of Ocean Science for Sustainable Development (hereinafter UN Ocean Decade; Challenge 7) concerns the expansion and extension of capabilities of the GOOS and biological observation is one of the fields to focus on (Claudet et al., 2020; Heymans et al., 2020).
Marine ecosystems are rich and complex, hosting more than 480,000 described species according to the World Register of Marine Species (WoRMS), and it is estimated that there are at least 0.7 - 1 million eukaryotic marine species in total (Appeltans et al., 2012). Together, this biodiversity sustains marine ecosystem functioning and in turn the provision of marine ecosystem services for human society (Snelgrove et al., 2014). The health and status of marine biodiversity is becoming of increasing concern because of a range of impacts, including climate change and pollution (Directorate-General for Environment European Commission, 2021). Protecting and restoring biodiversity is critical as the wellbeing of society is tightly linked to animal, plant, and ecosystem health. The marine environment and its biodiversity are proving to be even more susceptible than previously believed, with various effects in community composition observed as ocean temperature, circulation, and chemistry changes (Harley et al., 2006; Antão et al., 2020). Biodiversity data are therefore an important tool for monitoring ecosystem health (Rodríguez-Ezpeleta et al., 2021).
Significant initiatives that organise and improve access to biodiversity data and information are the Ocean Biodiversity Information System (OBIS) and the Global Biodiversity Information Facility (GBIF). OBIS provides access to data and information for more than 120,000 marine species and supports numerous projects and initiatives studying biodiversity1. In addition to hosting biodiversity data and information, GBIF works on standards, best practices and tools that support global biodiversity research2. The demand for generation of concrete, well-described and reusable biodiversity data increases the need for marine biodiversity observations at a European level, especially from established, long-term, monitoring programmes (Balvanera et al., 2022). Spatially extensive and long-term time series will allow the understanding of how various human pressures affect marine communities and their biodiversity (Boero et al., 2015; Hughes et al., 2017). The physico-chemical parameters and the pressures to the marine environment are being measured effectively and data are broadly available, however, marine biodiversity observation remains uncoordinated, immature, and scattered across various initiatives (Canonico et al., 2019; Claudet et al., 2020; Satterthwaite et al., 2021). This weakness in measuring biodiversity as a minimum to access the biological communities makes it impossible to deduce and understand causalities.
To move towards a sustainable and environmentally conscious society, it is urgent that we rapidly develop and organise extensive and integrated biodiversity observation research to complement the sophistication and scope of the physico-chemical observation networks. Ultimately, characterising the extent and function of marine biodiversity is essential for fully understanding the ocean and enabling the sustainable use of natural marine resources. Here we describe how the European Marine Biological Resource Centre - European Research Infrastructure Consortium (see Supplementary Material 1 Text Box EMBRC-ERIC), a European ESFRI (European Strategy Forum on Research Infrastructures) research infrastructure, consolidates and further develops the omics-based marine observation efforts of Europe and provides a framework for marine stations and laboratories to integrate their existing biological observation efforts into a coordinated European genomic observatory (GO). To this end, the EMBRC has created a Europe-wide network of interoperating molecular biodiversity observatories using shared protocols and collectively developing best practices: called the European Marine Omics Biodiversity Observation Network (EMO BON). This network produces a steady stream of standardised, FAIR-compliant (Findable, Accessible, Interoperable, Reusable) base-line biodiversity data and information products tailored to the varied needs of European and international stakeholders. EMO BON contributes towards globally coordinated research in marine biodiversity, encompassing microbial communities to macro-organisms, by applying environmental omics approaches and integrating other forthcoming technologies.
2 Strategic development areas
Omics-based observation of marine ecosystems already occurs across the world, inspired by the Genomics Observation Network (GON; Davies et al., 2014). With EMO BON, EMBRC establishes a European component of the GON, ensuring adherence to the same high-standards and occupying the European space in marine biodiversity observation and ecosystem functioning. The EMBRC operators represent a critical mass in initiating a long-term biodiversity observation network, and this network acts as a catalyst for other regions to join this global effort. All EMO BON protocols, and data management procedures are published and available to facilitate adoption and generation of comparable data by any interested party. Furthermore, EMBRC will host training workshops, and develop online tutorials on the sampling and sample preparation protocols to increase the standardisation of monitoring capacity. As such, the nascent EMO BON omics observatory provides a long-term, regional programme to support the UN Ocean Decade Challenge 7 to Expand The GOOS.
2.1 Adding nodes to the genomic observatory network: from local to regional alignment
Some of the oldest marine institutes in the world, which operate long-term observation, biodiversity monitoring and research activities, are among the EMBRC operators. Several of these organisations have in recent years implemented environmental genomics, sensu lato, into their observations. However, until now this has been lacking international coordination, leading to fragmented and heterogeneous approaches and methodologies across Europe. To provide standardised and holistic information on biodiversity composition along the European coast, EMBRC coordinates these previously isolated monitoring efforts into the joint genomic observatory EMO BON. EMBRC also includes institutes that have a smaller focus on marine genomic observations where EMO BON provides the context and support their capacity to build and align their local omics observation efforts. EMO BON unifies the operating stations and, at the same time, creates new observation sites, bringing the European marine biodiversity observation from the current local level to a regional pan-European level.
Currently, the network consists of 19 marine sampling stations, extending from the Arctic to the Red Sea and distributed across European Regional Seas (Figure 1). The EMO BON observatory network covers three broad marine habitats to assess biodiversity: the water column, the soft substrates, and the hard substrates. The EMO BON sampling stations organise the local sampling efforts and select which habitats to investigate according to local capacity, and scientific interests (Figure 1). The sample processing and sequencing strategies, as well as the handling of the generated data, and the compliance to international frameworks are centrally coordinated by EMO BON (Figure 2).
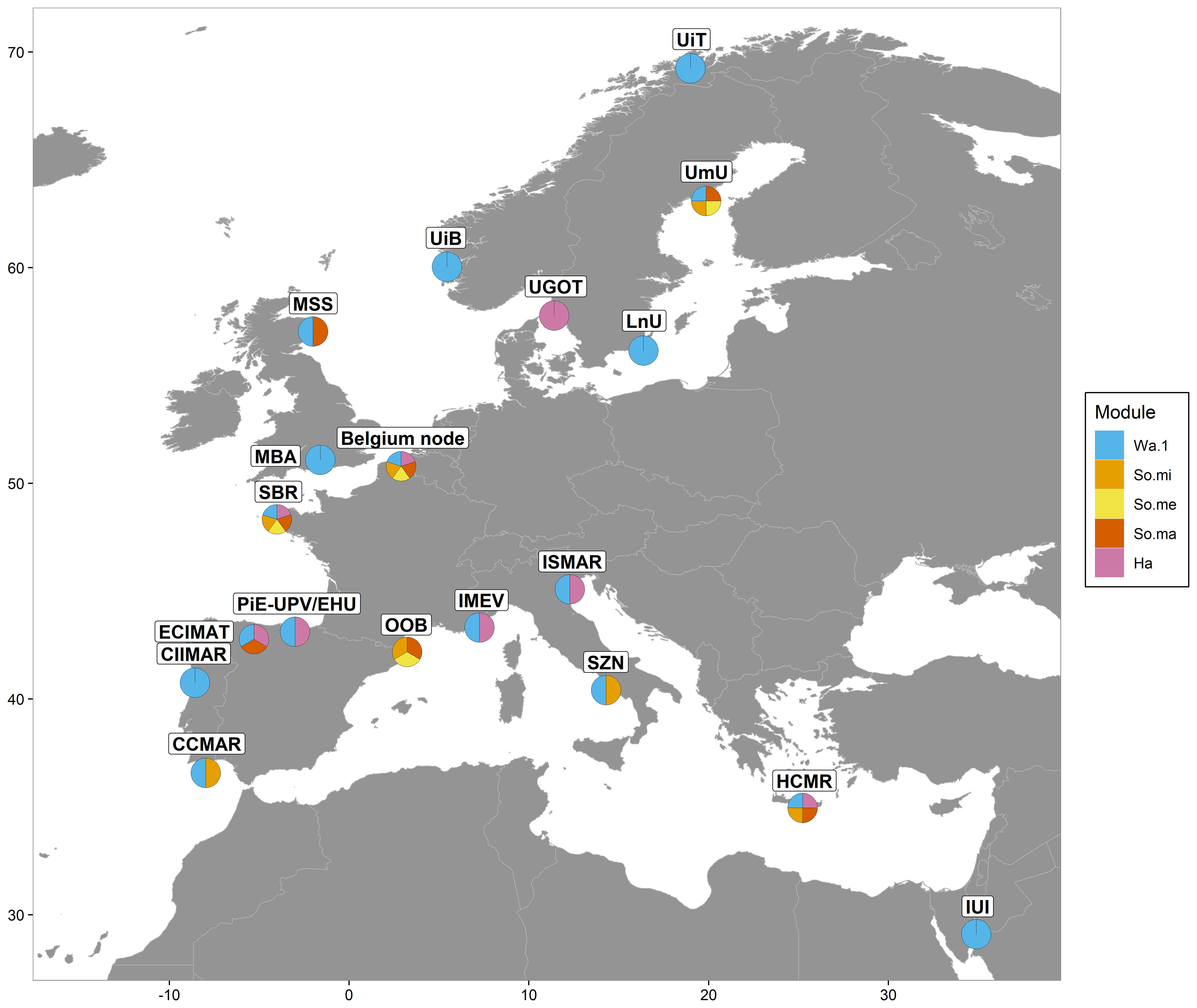
Figure 1 Map indicating the location of the 19 EMO BON (European Marine Omics Biodiversity Observation Network) observatory stations. The location of the labelled observatories is indicated by the pie charts. The type of sampling is shown in colour-coded pie charts below each observatory label. In case there was inadequate space, the pie charts were moved at the right-side of the label. Wa.1: Water column sampling (microorganisms module); So.mi: Soft substrate sampling (microorganisms module); So,me: Soft substrate sampling (meiobenthos module); So.ma: Soft substrate sampling (macrobenthos module); Ha: Hard substrate sampling (Autonomous Reef Monitoring Structure module). Observatories affiliations as indicated on the map in alphabetical order: Belgium node [Ghent University (UGENT), Flanders Marine Institute (VLIZ), Royal Belgian Institute of Natural Sciences (RBINS), Katholieke Universiteit Leuven (KULeuven)], Centre for Marine Sciences (CCMAR), Hellenic Centre for Marine Research (HCMR), Institut de la Mer de Villefranche (IMEV), Interdisciplinary Centre of Marine and Environmental Research (CIIMAR), Interuniversity Institute for Marine Sciences (IUI), Linnaeus University (LnU), National Research Council Institute of Marine Science (ISMAR), Observatoire Océanologique de Banyuls sur Mer (OOB), Plentzia Marine Station University of the Basque Country (PiE-UPV/EHU), Scottish Government – Marine Scotland Science (MSS), Station Biologique de Roscoff (SBR), Stazione Zoologica Anton Dohrn (SZN), The Arctic University of Norway (UiT), The Marine Biological Association (MBA), Umeå Marine Sciences Centre (UmU), University of Bergen (UiB), University of Gothenburg (UGOT), Vigo University Marine Research Centre (ECIMAT).
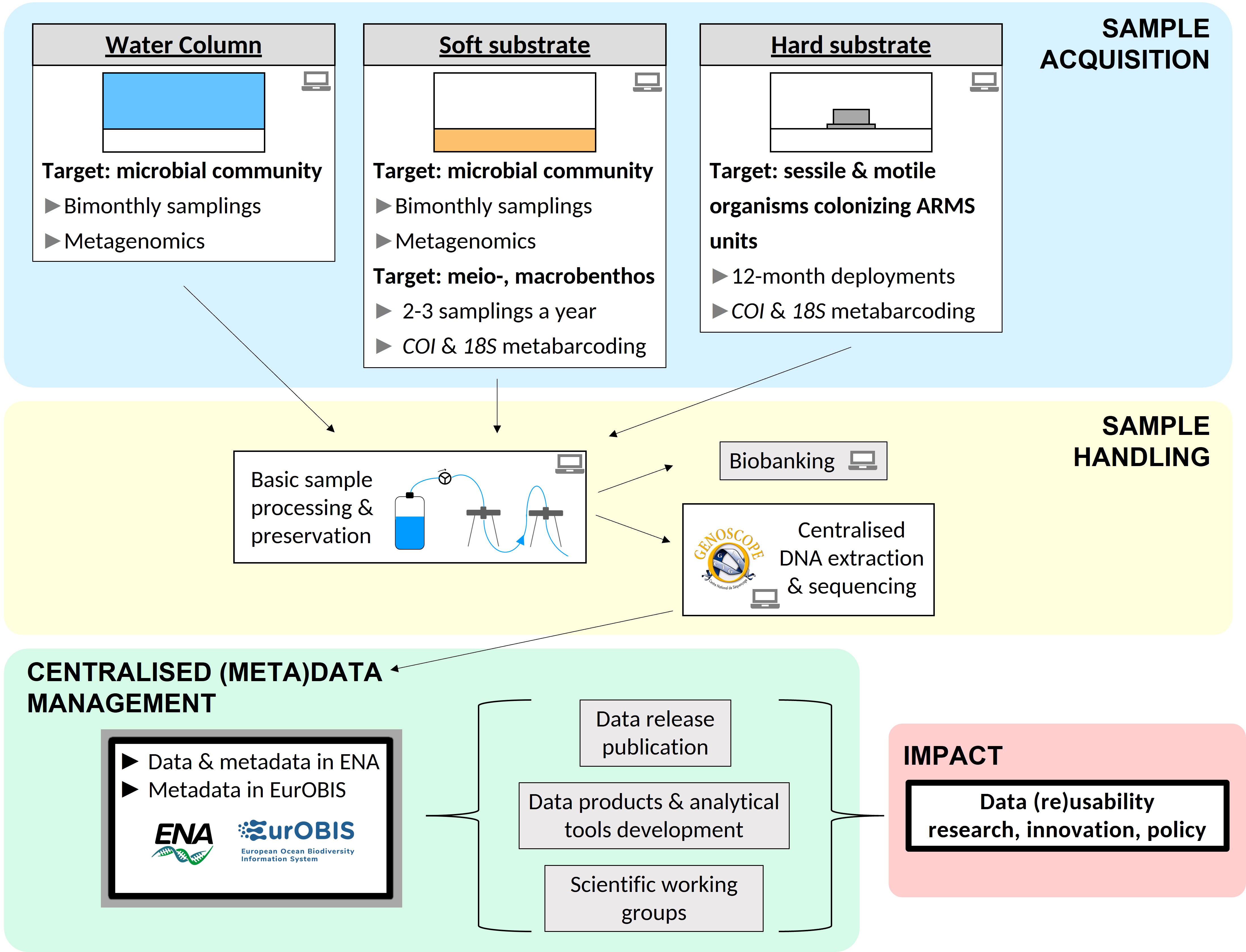
Figure 2 Flow chart overview of EMO BON (European Marine Omics Biodiversity Observation Network) strategy from sample acquisition to actions targeting data (re)usability. The computer symbol () indicates that metadata is being collected using electronic log-sheets at this step. ENA, European Nucleotide Archive; EuroOBIS, European node of the Ocean Biodiversity Information System.
2.2 Synchronising common and best practices
EMO BON faced the challenge of incorporating multiple sites with varying degrees of involvement in GO initiatives, ranging from occasional participants in the Ocean Sampling Day (OSD) initiative to fully operational augmented observatories. To accommodate such a wide range of capabilities, EMO BON implements a modular approach, with a module being a specific set of methodologies, protocols, and procedures valid for a specific habitat type (i.e. water column, soft substrate, and hard substrate). Each additional module represents an increase in observation capability and fine-scale sampling. The water column module is the core component and is shared among 90% of the stations, ensuring at least one set of comparable data across the network and baseline genomics data (Figure 1).
All EMO BON observatory stations are operating under the same well-described and publicly available strategies and workflows. This way, the delivered samples, datasets, and information are standardised and comparable, and provide high-level insights into regional biodiversity. Modules, protocols, and guidelines are included in the EMO BON Handbook which is published and openly shared on the EMBRC website3, and discoverable on the Ocean Best Practices System (OBPS) repository (Santi et al., 2021). The Handbook of EMO BON contains the genomic sampling Standard Operating Procedures (SOPs) and the network’s operational guidelines. The protocols are re-evaluated following feedback from the observatory stations and minor adjustments may be made to ensure that the dynamics of each station are appropriately accommodated. Additional protocols and guidelines will be incorporated as they emerge. New procedures are evaluated and standardised by the network before being added to the EMO BON Handbook. This will allow for the observatory to function as an innovation platform to test new methodologies and approaches.
The rigorous collection of metadata is imposed to ensure that the appropriate contextual information is available for all samples, allowing their reusability and harmonisation. The collected metadata describes the full provenance from sample collection to data generation. A fixed set of obligatory complementary (meta)data, including Essential Ocean Variables (EOVs), are collected at each sampling event. EMO BON uses the Minimum Information for any x Sequence (MIxS; Yilmaz et al., 2011) and Darwin Core (Wieczorek et al., 2012) metadata standards, to ensure compatibility with GON and the Global Omics Observatory Network (GLOMICON; Buttigieg et al., 2019). The data and metadata lifecycle from collection to storage and publication is described in the openly shared EMO BON Data Management Plan (DMP) that is also discoverable on the OBPS (Santi et al., 2022).
2.3 From in situ to in silico strategy
A standard sampling strategy is maintained throughout the EMO BON network with regular sampling events at fixed time intervals tailored to each habitat and targeted biological community (Figure 2). Sampling aspects that may be customised according to in situ conditions are seawater and sediment sampled volumes, and the means of reaching the sampling site. The GO is targeting different biological communities from each habitat. In water column sampling, emphasis is given to the plankton microbial community by sampling two different plankton size fractions (0.2-3 and 3-200 μm). In soft substrates samplings, the microorganisms, and the meiobenthic and macrobenthic communities, are targeted by applying different SOPs. The hard substrates are assessed using a passive sampling approach where Autonomous Reef Monitoring Structures4 (ARMS) units are deployed on the sea bottom to be colonised by motile and sessile macro-organisms (Figure 2) (Obst et al., 2020).
EMO BON samples are processed on site following described SOPs and targeting to reduce bias related to laboratory conditions, equipment, and operator handling. Following collection and short-time storage at the individual stations, all samples are transferred for centralised DNA extraction and sequencing at the highly experienced in molecular biology French National Sequencing Center (Genoscope) (Figure 2). DNA extraction is performed using commercially available kits, to minimise extraction deviations among samples. Water column and soft substrate microbial community samples are processed by metagenomics sequencing (Illumina short-read sequence technology), while the benthic organism samples (meio- and macro-benthos from soft substrates and macro-organisms from hard substrates) are targeted by metabarcoding approaches. The widely used nuclear 18S rRNA and mitochondrial COI markers were selected for metabarcoding biodiversity assessment to capture the eukaryotic diversity within both genomes (nucleic and mitochondrial).
For all samples collected, the generated raw sequences undergo a basic quality control step, and both the raw and the quality-controlled data are uploaded to the European Nucleotide Archive (ENA) (Cummins et al., 2022) and released to the public at regular intervals. The EMO BON DMP has been developed to ensure a unified approach to data management across EMO BON and harmonisation of dataset organisation including sample labelling, data publication, workflows, quality controls, storage, and data release (Santi et al., 2022). The first EMO BON sampling campaign took place in June 2021, and more than 500 seawater, 200 soft sediment and 100 hard substrate samples have been collected since then (Supplementary Material 2 Table S1). The first batch of samples analysed produced approximately 600 Gb of genomic data that are now in the process of being publicly released (Supplementary Material 2 Table S1).
To calibrate laboratory omics procedures and to ensure consistent, high quality, data analyses, mock community samples have been created and are included in the sequencing as positive controls. In addition, negative control samples are collected together with the samples to check for contamination during sampling and sample handling. Mock communities and negative controls are processed alongside the samples and generated data are treated and published together with the biodiversity data.
In addition, samples targeting plankton of larger size fractions (>20 and >200 μm) are collected and stored independently at local observatories. The SOPs for larger plankton size fractions are also provided as a resource to support the standardisation of the sampling protocols and to assist observatories in their desire to align efforts. The EMO BON community will use these SOPs as references with the purpose of testing and improving them.
3 Preserving the molecular natural history
3.1 Material samples
Additional replicates of the collected material samples (biosamples) are placed in long-term storage at the participating observatories under the stewardship of EMBRC, thus creating a distributed marine biobank in Europe. There are some already operational biobanks for marine samples, for instance the Marine Sample Bank5 and the MarBank6, which EMO BON seeks to exemplify from. The long-term storage replicates are cryopreserved (-80°C), without using any preservative solutions to ensure the preservation of the genetic material (Pérez-Espona, 2021; Falco et al., 2022). One replicate of the stored samples will be re-analysed at the end of this decade using the best available technology at that time, thereby providing the opportunity to apply advanced techniques currently unavailable to us. This may lead to new insights into the biodiversity and ecosystem function of the present decade and allow methodological comparisons and calibrations. Additional sample replicates can be re-analysed with a different experimental focus, where alternative questions and additional complementary hypotheses may be explored. Consequently, a wide-scope of experimental possibilities will emerge to enable the application of novel ideas and near-future technological developments to the EMO BON samples.
The application of FAIR principles in sample collections is often neglected, even if the access to biosamples can result in data generation of additional value (Koster and Woutersen-Windhouwer, 2018; Jetz et al., 2019). The digitization of information accompanying biosamples is critical for allowing the reusability of a sample collection (Wilkinson et al., 2016). EMO BON ensures FAIR traceability of all the samples that allow the locating of any sample, and its accompanying metadata, in real time. In addition, EMBRC has performed due diligence procedures with the EMO BON samples providing countries and negotiated to facilitate use of the samples, in compliance with Access and Benefit Sharing (ABS) and the Nagoya Protocol.
3.2 FAIR data principles for data and metadata
EMO BON has adopted a detailed DMP that is openly shared through the OBPS and will ensure that FAIR data principles are followed (Santi et al., 2022). The lifecycle of data and metadata generated is thoroughly described in the DMP along with the quality assurance and quality control procedures. EMO BON centrally coordinates the submission and release of the raw sequencing data and the accompanying metadata.
The accompanying metadata follows the MIxS checklist v5.013 (Yilmaz et al., 2011) and Darwin Core (Wieczorek et al., 2012) metadata terms. The metadata list is rich and includes metadata that describe the full provenance of the samples and data. There are 146 metadata terms used in total, with 31 of these terms being project specific, i.e., refer to particular activities within this project that cannot be described with standardised terms, as for example date a sample was shipped for analyses. The full metadata list is available in the EMO BON DMP and the templates used can be found in the EMBRC website7.
Data and metadata are submitted to suitable public databases at frequent time intervals and become openly available. Sequencing raw and quality-controlled data are submitted to ENA and are attached with ENA accession numbers as digital identifiers. The collected sampling and analyses metadata, the associated environmental (meta)data, and the ENA accession numbers indicating the sequencing raw data are submitted to the European node of the international Ocean Biodiversity Information System (EurOBIS) and from there passed on to European Marine Observation and Data Network (EMODNet). EMO BON (meta)data entries destined for EurOBIS are provided in the standardised Darwin Core Archive (DwC-A) format. The data and metadata are assigned a Creative Commons licence with attribution (CC BY) with their release. A dataset publication follows shortly after the data and metadata release to describe the data, the metadata, and their online location.
These measures not only make the outputs from EMO BON FAIR and machine- and human- operable and understandable, but also speed up the process from sampling to data publishing. EMO BON is developing a data management system to be used throughout the project that handles the regular creation of omics (meta)data in a structured way. The system will use Research Object (RO) Crates (Soiland-Reyes et al., 2022) reflecting the structure of EMO BON, and is designed to function on existing data platforms. This semi-automated system will guide the rapid and consistent submission of (meta)data to marine databases, repositories, and data catalogues.
3.3 Compliance with international frameworks for the utilisation of marine genetic resources
Marine scientific research on biological material falls under several different legal regimes depending on where the research is performed, and/or how the material is collected and utilised. The activity of collecting biomaterial for science falls under the United Nations Convention on the Law of the Sea (UNCLOS) when performed within the national jurisdiction. The way in which biological resources may be Accessed, and how the Benefits that result from their utilisation in research and development are Shared with the country of origin refers to the ABS system. EMBRC undertook the due diligence for ABS (for access and use of genetic resources) and UNCLOS (for accessing the exclusive economic zone for biological sampling) on behalf of EMO BON to ensure compliance of activities. Since the EMO BON collection remains under its stewardship, when necessary EMBRC will centralise negotiations, providing countries that follow ABS regulatory frameworks to declare utilisation of samples that fall under ABS. In addition, EMBRC will take action to ensure that observatory stations comply with UNCLOS and have collected the appropriate sampling permits when necessary.
3.4 Policy coordination with partners, projects, and other infrastructures
EMBRC collaborates with its sister infrastructures within and outside Europe to develop opportunities to explore data in oceanographic and ecological contexts, microbiome research, and anthropogenic impacts assessment. Through collaboration with LifeWatch ERIC8, workflows are being developed to combine the metabarcoding data with physical and chemical data to produce complex marine system models, aiming to create valuable outputs for policy makers. Example of such workflow is the non-indigenous species detection workflow built around the hard substrate EMO BON data and the ARMS data9.
In addition, EMBRC partners are developing a metagenomic computational workflow, named metaGOflow (Zafeiropoulos et al., submitted manuscript), which enables standardised and timely analyses of the data producing taxonomic inventories and functional gene profiles of each EMO BON sample. The workflow will allow the release of these data products to the MGnify platform (Mitchell et al., 2020). The data products, and all sample and analytical metadata will be released as RO-Crates (Soiland-Reyes et al., 2022), and a Virtual Research Environment for data exploration, visualisation and analysis is under development.
An important aspect of the EMO BON initiative is to ensure coordination and collaboration between the existing omics-based observatories in Europe and beyond. To foster strong ties, EMBRC proposes to form a coalition of observatories that collaborate on the continued evolution of biological observation. Efforts should go beyond time-limited project-based funding, and towards establishing Memoranda of Understanding and Collaboration Agreements among research infrastructures, organisations, and institutions, to agree on a shared mission. Creating binding agreements covering areas such as minimum data and metadata standards, shared protocols, intercalibration, and cross-checking of samples, will empower the participants of the mission and ensure adherence to its objectives, while at the same time it will help avoid mission creep and shifting priorities. Implementing this shared mission will lead to wide-ranging benefits and added value for the science community and society. Ultimately, this will strengthen biological observation by allowing many parallel initiatives to come together under a shared vision, and emerge with a strong, organised, and federated biological observation network, on par with that of physical and chemical oceanography (Jetz et al., 2019).
4 Discussion
4.1 A European component of a global system
In the marine domain, the primary global mission for this decade is the UN Ocean Decade, which is strongly linked with the Sustainable Development Agenda for 2030 (Colglazier, 2015; Claudet et al., 2020; Heymans et al., 2020). National and regional strategies are aligned with implementation guidance created during a consultative preparatory phase (2018-2020), and many regional meetings have underscored the need to improve biological and ecological observation of the oceans to support sustainable development (Canonico et al., 2019; Satterthwaite et al., 2021; Balvanera et al., 2022). The UN Ocean Decade action Ocean Biomolecular Observing Network10 (OBON) focuses on marine biological observation and EMO BON alienates with OBON to standardise and build the capacity for marine biological observation in Europe. EMO BON is endorsed as a UN Ocean Decade project associated with the programme OBON and synergizes with other regional or thematic biomolecular observing activities. Therefore, EMO BON complements OBON and together aim to decipher coastal biodiversity and contribute to the building of a coastal ocean biomolecular observing system.
To participate in global observation missions, any regional or local observatory must align its strategies and operations to global scale frameworks and agree to accept data and metadata standards to ensure comparability and interoperability. EMO BON unites individual biological observation stations in Europe and provides the context and opportunity to participate in an advanced highly coordinated biodiversity observation framework. This network aims to become a paradigmatic observatory with its activities and procedures being able to be transposed to other regions and aspires to grow national observation capacities by engaging with national stakeholders and policy makers. This paradigm can accordingly contribute to expanding marine observation efforts and to overcoming the existing geographical, social, and economic biases in FAIR biodiversity data production (Ramírez et al., 2022). The geographic expansion of marine genomic observation is necessary to fill the existing gaps in the sampling effort in Europe, as for example in the Eastern Mediterranean and Eastern Baltic Sea.
EMO BON becomes part of the existing marine biological observation system and aspires to set the basis for the regular and standardised production of genomic data. EMO BON’s openly shared protocols and guidelines contribute to building the capacity for marine biological observation beyond this network. The discoverable and FAIR observation data produced can promote the full integration of omics data into OBIS and GBIF, therefore expanding their marine omics component. This would promote the reusability of the data and relevant information and add the biological component in environmental policy making in a systematic and standardized way.
Outstanding examples of similar observation initiatives are the National Ecological Observatory Network (NEON11) and Australia’s Integrated Marine Observing System (IMOS12). Both initiatives are considerably contributing to their related fields and are proving the value biological observation networks can provide. NEON is covering freshwater and terrestrial ecosystems in the US and produces ecological data for over 20 years; their data have fuelled different research areas such as litter patterns in forests (Jevon et al., 2022) and plant biodiversity research (Liang et al., 2022). The focus of IMOS is the monitoring of marine coastal communities by employing different methodologies such as animal tracking and genomics; one of their focus areas is food security wherein marine data are directly integrated to decision-making regarding for instance highly productive coastal zones. EMO BON is motivated by such well-established initiatives and aspires to increase its direct impact in a similar way.
Through the large-scale, and long-term context of biodiversity observation, EMO BON will improve our understanding of the oceans, the impact of climate change and anthropogenic stressors, and provide the necessary resources to make informed decisions about sustainable actions for the European waters. It will facilitate the European Union’s Missions, such as Healthy Oceans, Seas, Coastal, and Inland Waters13, Adaptation to Climate Change14 and Mission Starfish 2030: Restore our Ocean and Waters15. Moreover, environmental genomic data can be integrated with data generated through experimental research linking genomes to phenotypes under different environmental conditions. Such traversal research is expected to be synergistic and deliver new, and unexpected, insights into the potential contribution of the marine ecosphere for the improvement of human health and well-being, and improve our understanding how anthropogenically induced changes can affect the functioning of marine ecosystems.
4.2 The role of omics in marine biological observation
Omics is an umbrella term for the wide range of high-throughput approaches used to analyse biomolecules; it includes, but is not limited to, genomics, transcriptomics, proteomics, and metabolomics (see also Supplementary Material 3 Text Box eDNA). Environmental omics approaches, where DNA is sampled from an environmental medium, provide a means of capturing the most representative amount of biodiversity information, and the data needed to implement reliable services to monitor ocean life (Pawlowski et al., 2018; Bush et al., 2019). This observation network applies biomolecular omics methodologies, such as metagenomics and metabarcoding, to produce maximal information on community biodiversity and to provide means to access the pool of genes present in an ecosystem. The EMO BON omics data will reveal the biodiversity of different communities from microorganisms to Metazoa, their temporal trends and shifts, the ecological relationships among species, and their responses to environmental changes. This information can be subsequently translated to the function and role of taxa in the environment and used to other applied research fields. Omics data can support the conservation of ecosystems and the sustainable use of marine resources (for example Bowser et al., 2020; Turunen et al., 2021), as well as the monitoring of wild animal populations such as fish (Andruszkiewicz et al., 2017) or mammals (Suarez-Bregua et al., 2022). In addition, omics data can be integrated into ecosystem models to develop innovative investigations in food security (for example Grützke et al., 2019; Sequino et al., 2022), biodiscovery (for example Mahapatra et al., 2020; Shaaban et al., 2022), novel treatments in biofuels (Sartaj et al., 2022) and bioremediation (Rodríguez et al., 2022), and other services such as disease detection in the environment (Wurtzer et al., 2022) or clinical diagnostics (Forbes et al., 2017). In the last decade, omics methods have matured into viable solutions to support efficient biodiversity observation from microbes to whales, reorienting our understanding of ecology and evolution, as well as the ways humans are embedded in the physical world (Canonico et al., 2019; Rodríguez-Ezpeleta et al., 2021).
Omics biodiversity data is not only important for monitoring and managing the natural environment (Makiola et al., 2020; Rodríguez-Ezpeleta et al., 2021), but it also allows us to identify the least-considered component of the sea, the marine microbiome (Abreu et al., 2022). As noted in the Atlantic Ocean Research Alliance (AORA) Marine Microbiome Roadmap, with omics we now have the capabilities for mainstreaming microbiome observation, which will be a game-changer in complementing current observation efforts (Bolhuis et al., 2020). EMO BON integrates elements and recommendations from the AORA Roadmap to ensure that it responds to the needs of the Atlantic Ocean research communities, as well as serving as a basis for expanding compatible and interoperable observation of biodiversity between marine regions, both in the northern and southern hemispheres.
4.3 Standardisation of ocean observation efforts
The Essential Ocean Variables (EOVs) defined by GOOS offer an interface to UN-led efforts to improve and standardise planetary-scale marine observation (Miloslavich et al., 2018). The EOVs dedicated to biology and ecosystems (Bio-Eco) are relatively novel. Organisms are the smallest units considered by the Bio-Eco EOVs; however, they overlook information on single genomes and gene pools, on species demographic history, functional role, potential to respond to change, types of interactions and coevolution, and therefore EOVs alone cannot be integrated to modelling and predictions. On the other hand, the Essential Biodiversity Variables (EBVs) described by the Group on Earth Observation Biodiversity Observation Network (GEO BON), focus on representing biological entities in the environment and organise information across processes, traits and groups of organisms including information on genetic composition, taxonomic diversity, and functional type composition (Pereira et al., 2013; Muller-Karger et al., 2018). EBVs were defined under the scope of a comprehensive description of biodiversity and can serve as a list of variables to be considered in monitoring programmes (Pereira et al., 2013; Balvanera et al., 2022).
GOs, such as EMO BON, can have multiple and pivotal roles in sustaining and evolving the use and value of EOVs and EBVs. Omics data are necessary for defining the emerging EOVs for microorganisms and can be used to complement the existing EOVs for non-microbial organisms (for example, EOV Zooplankton biomass and diversity) (Miloslavich et al., 2018). The systematic generation of omics data by GOs can serve as the data collection used to calibrate and operationalize EOVs and EBVs by attaining omics related variables from a variety of standardised datasets (Kissling et al., 2018; Jetz et al., 2019). The temporal resolution of GOs data is necessary for defining the functional biodiversity that can rapidly change with time, and for identifying the critical time intervals during which the dynamically changing biodiversity can be safely accessed, i.e., within which time span can biodiversity of a given community be described. EMO BON produces omics biodiversity and functional data for numerous taxa simultaneously; this can similarly serve as the data collection to define the aggregation level useful for EOVs and EBVs, i.e., which functional (e.g., predatory-plankton, grazer meiobenthos) or taxonomic levels (e.g. microorganisms including bacteria, archaea, microbial eukaryotes) can be grouped and considered as one variable.
EMO BON measures physical and biochemical EOVs as a common metric to connect biodiversity to its environment. GOs such as EMO BON can promote the collection of EOVs and other EBVs together with omics data and therefore help make biological observation interoperable and comparable to a higher level while adding multidisciplinarity into the system. This would allow a parallel assessment of diverse essential and standardised values and thus contribute to predicting ecosystem changes and responses. This structured approach to EOVs and EBVs measurement can help escaping Data-Rich-Information-Poor (DRIP) datasets and move towards hypothesis-driven data collection.
Omics technologies can apply to all the Bio-Eco EOVs and several EBVs, however, specialised methods need to be developed and agreed upon to measure them. The desirable high-level coordination of EMO BON and other GOs can contribute to, on one hand, testing methodologies, and, on the other hand, applying the suggested best practices across a broad spatial and temporal scale. EMO BON can assist in spreading the information on EOVs and EBVs best practices through training events, shared protocols, and promoting public discussions. GOs have the potential to increase the significance and quality of EOVs monitoring globally (Muller-Karger et al., 2018).
4.4 Challenges in setting up and operating a biodiversity observation network
During the preparation and initial operation of the EMO BON network, different challenges and obstacles had to be overcome to warrant its sustainability, high-quality and significant impact. Securing prolonged funding is possibly the biggest challenge for long-term observation. Biodiversity research is largely funded by short-term projects; this makes the continuance of efforts almost impossible after a project’s completion. As a result, many initiatives fade soon after a dynamic launch. EU RIs were established to support research activities at the EU level, to provide equipment, resources, data, e-services, and communication networks. EU RIs are part of the ESFRI Roadmap which sustains their existence in time. Hence, EU RIs such as EMBRC are in the position to provide the needed prolongment, to reinforce and reassure the future of observation networks. However, EMBRC is not an RI with observation as its primary role, therefore, it had to reassure internal and external stakeholders that EMO BON is and will be an impactful initiative producing useful data. The effort to sustain the network is continuous and EMO BON needs to remain relevant to the global observation landscape and increase its impact to conserve the support and funding it receives.
A distributed observatory includes multiple stations in different areas. The stations themselves are diverse in terms of sampling, laboratory or data expertise, ecosystem type and available resources. Therefore, it is challenging to integrate this mixture of different characteristics into one network. It is necessary to take advantage of each station’s strengths, respect their history as well as the time and experience of individual participants. However, a network has to operate under agreed procedures, standards and protocols. To achieve this, EMO BON went through a preparatory non-operational phase during which several consultation events and open discussion rounds took place to determine the operational procedures to be followed. During the preparatory phase, the diversity of the stations was taken into account and voice was given to any individual researcher who wanted to contribute. The protocols used among different station were analysed and examined before the current protocols were finalised. In addition, EMBRC took advantage of the expertise available in its working groups such as the ABS and the e-Infrastructure working group.
Another often neglected challenge is data management following FAIR and open science principles. Data management needs to be initiated at the beginning of any project, and it is necessary to decide on the procedures for data and metadata collection, storing, handling, and sharing early on. EMO BON gave emphasis on FAIR data management from its preparatory phase, recognized the experts in the EMBRC network and worked directly with them and allocated resources to support the data management. Furthermore, the connection to the global level is significant here as it is important to adopt global standards and use public databases to ensure the interoperability and reusability of the data.
5 Final remarks
The overarching objectives of the newly formed EMBRC genomic observatory network, EMO BON, are to support, coordinate and maintain a pan-European biological observation effort to obtain long-term, high-quality omics-based marine biodiversity information. The data generated by the network will contribute to understanding the dynamics of marine life. Taking into account different temporal scales, scientific conclusions will be drawn concerning temporal biodiversity trends and short- and long-term variability. Such information and conclusions can in turn be related to climate change, pollution, and other stressors. Applied marine biological research will be invigorated by data and information produced within the EMO BON and aid bioprospecting efforts that may target specific marine areas or seasons. The identification of non-indigenous species or pathogens could also be facilitated, and their spreading in an ecosystem will be traceable. Under an interannual or decadal perspective, this effort will illuminate the links between biodiversity and the changing ocean and help predict the reciprocal relation between biology and ocean health.
EMBRC aims to maintain this initiative as open and accessible to the whole scientific community. All actions within the network are described and displayed online and the consortium will remain receptive to feedback and external contributions. Training activities will be directed not only to participants within EMBRC, but also to the broader community, and will engage with potential future contributors. Academics from other scientific fields, such as bioinformatics and biotechnology, will also be able to employ the FAIR data generated within EMO BON to advance their research. Dissemination actions will target scientists, stakeholders, and the public, and will contribute to the UN Decade of the Ocean plan to create inclusive science initiatives, encourage dialogue between scientists and policy makers, and improve science communication to the public.
EMO BON network encourages contributions and supporting actions towards a unified approach to marine biological observation. In particular, we support researchers and institutes working together using open community standards and protocols described within EMBRC EMO BON to enable a global network of genomic observatories. We advocate for a rigorous, standardised and structured biological observation universal system that maintains high-quality, trusted, FAIR data capable of feeding informed decision making. Through this organised and coordinated approach we can progress further through collaborative efforts to explore the data aiming for a deeper understanding of the ocean, to develop further applied uses of marine omics observation data and to support multidisciplinarity to investigate novel approaches for the data.
Author contributions
IS, PLB, RC, MC, ND, GK, FN, MO, JV and NP conceived the idea and co-wrote the paper. IS led the writing of the paper. All other authors contributed significant ideas and wrote the paper. All authors contributed to the article and approved the submitted version.
Funding
The EMO BON genomic observatory is funded and financed by the European Marine Biological Resource Centre - European Research Infrastructure Consortium (EMBRC-ERIC), which is part of the European Strategy Forum on Research Infrastructures (ESFRI). The following organizations contribute to EMO BON: Ghent University (UGENT), Flanders Marine Institute (VLIZ), Royal Belgian Institute of Natural Sciences (RBINS), Katholieke Universiteit Leuven (KULeuven), Centre for Marine Sciences (CCMAR), Hellenic Centre for Marine Research (HCMR), Institut de la Mer de Villefranche (IMEV), Interdisciplinary Centre of Marine and Environmental Research (CIIMAR), Interuniversity Institute for Marine Sciences (IUI), Linnaeus University (LnU), National Research Council Institute of Marine Science (CNR-ISMAR), Observatoire Océanologique de Banyuls sur Mer (OOB), Plentzia Marine Station University of the Basque Country (PiE-UPV/EHU), Scottish Government – Marine Scotland Science (MSS), Station Biologique de Roscoff (SBR), Stazione Zoologica Anton Dohrn (SZN), The Arctic University of Norway (UiT), The Marine Biological Association (MBA), Umeå Marine Sciences Centre (UmU), University of Bergen (UiB), University of Gothenburg (UGOT), Vigo University Marine Research Centre (ECIMAT). This work is also supported by the France Genomique National infrastructure, funded as part of the «Investissements d’Avenir» program managed by the Agence Nationale pour la Recherche (contract ANR-10-INBS-09). The development of metagenomic computational workflow (metaGOflow) received funding from programme EOSC Life (EU Horizon 2020 research and innovation programme under grant agreement No 824087). Prerequisite work on the ARMS sampling campaigns was supported by the infrastructure programs ASSEMBLE Plus (grant no. 730984). RC would like to recognise the support of programmes TechOceanS (Horizon 2020 research and innovation programme under Grant Agreement No 101000858) and EuroSea (Horizon 2020 research and innovation programme under grant agreement No 862626). RL would like to recognise the support of Research Foundation - Flanders (FWO), grant numbers I002021N and I001621N. CJC and BL received Portuguese national funds from FCT - Foundation for Science and Technology through projects UIDB/04326/2020, UIDP/04326/2020 and LA/P/0101/2020. MO would like to recognise the support of the Swedish Agency for Marine and Water Management (grant no 3181- 2019).
Acknowledgments
We are grateful to all individuals from the operational stations contributing to EMO BON. We wish to thank the EMBRC e-Infrastructure Working Group and the EMBRC Traceability Working Group for insightful discussions. We are especially grateful to Guillaume Duspara for assistance with graphics. RC would like to acknowledge the contribution of NEREA group at SZN and especially Anna Chiara Trano.
Conflict of interest
The authors declare that the research was conducted in the absence of any commercial or financial relationships that could be construed as a potential conflict of interest.
Publisher’s note
All claims expressed in this article are solely those of the authors and do not necessarily represent those of their affiliated organizations, or those of the publisher, the editors and the reviewers. Any product that may be evaluated in this article, or claim that may be made by its manufacturer, is not guaranteed or endorsed by the publisher.
Supplementary material
The Supplementary Material for this article can be found online at: https://www.frontiersin.org/articles/10.3389/fmars.2023.1118120/full#supplementary-material
Glossary
Footnotes
- ^ https://obis.org/
- ^ https://www.gbif.org/
- ^ http://www.embrc.eu/emobon
- ^ https://www.protocols.io/workspaces/global-arms-program
- ^ https://ropme.org/?page_id=362
- ^ https://www.hi.no/en/hi/forskning/research-groups-1/marbank
- ^ www.embrc.eu/emo-bon
- ^ http://www.lifewatch.eu
- ^ https://www.lifewatch.eu/internal-joint-initiative/validation-cases/long-term-monitoring-of-invasive-marine-species/
- ^ https://www.oceandecade.org/actions/ocean-biomolecular-observing-network-obon/
- ^ https://www.neonscience.org/
- ^ https://imos.org.au/
- ^ https://ec.europa.eu/info/research-and-innovation/funding/funding-opportunities/funding-programmes-and-open-calls/horizon-europe/eu-missions-horizon-europe/healthy-oceans-seas-coastal-and-inland-waters_en
- ^ https://ec.europa.eu/clima/eu-action/adaptation-climate-change_en
- ^ https://ec.europa.eu/info/publications/mission-starfish-2030-restore-our-ocean-and-waters_en
References
Abreu A., Bourgois E., Gristwood A., Troublé R., Acinas S. G., Bork P., et al. (2022). Priorities for ocean microbiome research. Nat. Microbiol. 7, 937–947. doi: 10.1038/s41564-022-01145-5
Andruszkiewicz E. A., Starks H. A., Chavez F. P., Sassoubre L. M., Block B. A., Boehm A. B. (2017). Biomonitoring of marine vertebrates in Monterey bay using eDNA metabarcoding. PloS One 12, e0176343. doi: 10.1371/journal.pone.0176343
Antão L. H., Bates A. E., Blowes S. A., Waldock C., Supp S. R., Magurran A. E., et al. (2020). Temperature-related biodiversity change across temperate marine and terrestrial systems. Nat. Ecol. Evol. 4, 927–933. doi: 10.1038/s41559-020-1185-7
Appeltans W., Ahyong S. T., Anderson G., Angel M. V., Artois T., Bailly N., et al. (2012). The magnitude of global marine species diversity. Curr. Biol. 22, 2189–2202. doi: 10.1016/j.cub.2012.09.036
Balvanera P., Brauman K. A., Cord A. F., Drakou E. G., Geijzendorffer I. R., Karp D. S., et al. (2022). Essential ecosystem service variables for monitoring progress towards sustainability. Curr. Opin. Environ. Sustain. 54, 101152. doi: 10.1016/j.cosust.2022.101152
Boero F., Kraberg A. C., Krause G., Wiltshire K. H. (2015). Time is an affliction: why ecology cannot be as predictive as physics and why it needs time series. J. Sea Res. 101, 12–18. doi: 10.1016/j.seares.2014.07.008
Bolhuis H., Buttigieg P. L., Goodwin K., Groben R., Iudicone D., Lacoursière-Roussel A., et al. (2020). Atlantic Ocean research alliance - marine microbiome roadmap. Zenodo. doi: 10.5281/zenodo.3632526
Bowser M., Brassfield R., Dziergowski A., Eskelin T., Hester J., Magness D., et al. (2020). Towards conserving natural diversity: a biotic inventory by observations, specimens, DNA barcoding and high-throughput sequencing methods. Biodivers. Data J. 8, e50124. doi: 10.3897/BDJ.8.e50124
Bush A., Compson Z. G., Monk W. A., Porter T. M., Steeves R., Emilson E., et al. (2019). Studying ecosystems with DNA metabarcoding: lessons from biomonitoring of aquatic macroinvertebrates. Front. Ecol. Evol. 7. doi: 10.3389/fevo.2019.00434
Buttigieg P. L., Janssen F., Macklin J., Pitz K. (2019). “The global omics observatory network: shaping standards for long-term molecular observation,” in Biodiversity information science and standards(Leiden, Netherlands) 3, e36712. doi: 10.3897/biss.3.36712
Canonico G., Buttigieg P. L., Montes E., Muller-Karger F. E., Stepien C., Wright D., et al. (2019). Global observational needs and resources for marine biodiversity. Front. Mar. Sci. 6. doi: 10.3389/fmars.2019.00367
Claudet J., Bopp L., Cheung W. W. L., Devillers R., Escobar-Briones E., Haugan P., et al. (2020). A roadmap for using the UN decade of ocean science for sustainable development in support of science, policy, and action. One Earth 2, 34–42. doi: 10.1016/j.oneear.2019.10.012
Colglazier W. (2015). Sustainable development agenda: 2030. Science 349, 1048–1050. doi: 10.1126/science.aad2333
Cummins C., Ahamed A., Aslam R., Burgin J., Devraj R., Edbali O., et al. (2022). The European nucleotide archive in 2021. Nucleic Acids Res. 50, D106–D110. doi: 10.1093/nar/gkab1051
Davies N., Field D., Amaral-Zettler L., Clark M. S., Deck J., Drummond A., et al. (2014). The founding charter of the genomic observatories network. GigaScience 3, 2. doi: 10.1186/2047-217X-3-2
Dexter P., Summerhayes C. (2010). “Ocean observations: the global ocean observing system (GOOS),” in Troubled waters: ocean science and governance (Cambridge: Cambridge University Press), 161–178.
Directorate-General for Environment European Commission (2021) EU Biodiversity strategy for 2030: bringing nature back into our lives. LU: publications office of the European union. Available at: https://data.europa.eu/doi/10.2779/677548.
Falco R., Appiah-Madson H. J., Distel D. L. (2022). The ocean genome legacy: a genomic resource repository for marine life. Biopreservation Biobanking 20, 104–106. doi: 10.1089/bio.2021.0148
Forbes J. D., Knox N. C., Ronholm J., Pagotto F., Reimer A. (2017). Metagenomics: the next culture-independent game changer. Front. Microbiol. 8. doi: 10.3389/fmicb.2017.01069
Grützke J., Malorny B., Hammerl J. A., Busch A., Tausch S. H., Tomaso H., et al. (2019). Fishing in the soup – pathogen detection in food safety using metabarcoding and metagenomic sequencing. Front. Microbiol. 10. doi: 10.3389/fmicb.2019.01805
Harley C. D. G., Randall Hughes A., Hultgren K. M., Miner B. G., Sorte C. J. B., Thornber C. S., et al. (2006). The impacts of climate change in coastal marine systems. Ecol. Lett. 9, 228–241. doi: 10.1111/j.1461-0248.2005.00871.x
Heymans J. J., Bundy A., Christensen V., Coll M., de Mutsert K., Fulton E. A., et al. (2020). The ocean decade: a true ecosystem modeling challenge. Front. Mar. Sci. 7. doi: 10.3389/fmars.2020.554573
Hoegh-Guldberg O. (2015) Reviving the oceans economy: the case for action (Boston Consulting Group, WWF, Global Change Institute, The University of Queensland). Available at: https://www.worldwildlife.org/publications/reviving-the-oceans-economy-the-case-for-action-2015 (Accessed September 20, 2022).
Hughes B. B., Beas-Luna R., Barner A. K., Brewitt K., Brumbaugh D. R., Cerny-Chipman E. B., et al. (2017). Long-term studies contribute disproportionately to ecology and policy. BioScience 67, 271–281. doi: 10.1093/biosci/biw185
Jetz W., McGeoch M. A., Guralnick R., Ferrier S., Beck J., Costello M. J., et al. (2019). Essential biodiversity variables for mapping and monitoring species populations. Nat. Ecol. Evol. 3, 539–551. doi: 10.1038/s41559-019-0826-1
Jevon F. V., Polussa A., Lang A. K., William Munger J., Wood S. A., Wieder W. R., et al. (2022). Patterns and controls of aboveground litter inputs to temperate forests. Biogeochemistry 161, 335–352. doi: 10.1007/s10533-022-00988-8
Kissling W. D., Walls R., Bowser A., Jones M. O., Kattge J., Agosti D., et al. (2018). Towards global data products of essential biodiversity variables on species traits. Nat. Ecol. Evol. 2, 1531–1540. doi: 10.1038/s41559-018-0667-3
Koster L., Woutersen-Windhouwer S. (2018) FAIR principles for library, archive and museum collections: a proposal for standards for reusable collections. Code4Lib J. Available at: https://journal.code4lib.org/articles/13427 (Accessed November 14, 2022).
Liang M., Baiser B., Hallett L. M., Hautier Y., Jiang L., Loreau M., et al. (2022). Consistent stabilizing effects of plant diversity across spatial scales and climatic gradients. Nat. Ecol. Evol. 6, 1669–1675. doi: 10.1038/s41559-022-01868-y
Mahapatra G. P., Raman S., Nayak S., Gouda S., Das G., Patra J. K. (2020). Metagenomics approaches in discovery and development of new bioactive compounds from marine actinomycetes. Curr. Microbiol. 77, 645–656. doi: 10.1007/s00284-019-01698-5
Makiola A., Compson Z. G., Baird D. J., Barnes M. A., Boerlijst S. P., Bouchez A., et al. (2020). Key questions for next-generation biomonitoring. Front. Environ. Sci. 7. doi: 10.3389/fenvs.2019.00197
Miloslavich P., Bax N. J., Simmons S. E., Klein E., Appeltans W., Aburto-Oropeza O., et al. (2018). Essential ocean variables for global sustained observations of biodiversity and ecosystem changes. Glob. Change Biol. 24, 2416–2433. doi: 10.1111/gcb.14108
Mitchell A. L., Almeida A., Beracochea M., Boland M., Burgin J., Cochrane G., et al. (2020). MGnify: The microbiome analysis resource in 2020. Nucleic Acids Research 48, D570–D578. doi: 10.1093/nar/gkz1035
Muller-Karger F. E., Miloslavich P., Bax N. J., Simmons S., Costello M. J., Sousa Pinto I., et al. (2018). Advancing marine biological observations and data requirements of the complementary essential ocean variables (EOVs) and essential biodiversity variables (EBVs) frameworks. Front. Mar. Sci. 5. doi: 10.3389/fmars.2018.00211
National Oceanic and Atmospheric Administration (2021) How much water is in the ocean? Available at: https://oceanservice.noaa.gov/facts/oceanwater.html (Accessed September 13, 2022).
Nesmith A. A., Schmitz C. L., Machado-Escudero Y., Billiot S., Forbes R. A., Powers M. C. F., et al. (2021). “Climate change, ecology, and justice,” in The intersection of environmental justice, climate change, community, and the ecology of life. Eds. Nesmith A. A., Schmitz C. L., Machado-Escudero Y., Billiot S., Forbes R. A., Powers M. C. F., et al (Cham: Springer International Publishing), 1–12. doi: 10.1007/978-3-030-55951-9_1
Obst M., Exter K., Allcock A. L., Arvanitidis C., Axberg A., Bustamante M., et al. (2020). A marine biodiversity observation network for genetic monitoring of hard-bottom communities (ARMS-MBON). Front. Mar. Sci. 7. doi: 10.3389/fmars.2020.572680
Organization for Economic Co-operation and Development (2016) The ocean economy in 2030 (Paris: OECD Publishing). Available at: https://www.oecd-ilibrary.org/economics/the-ocean-economy-in-2030_9789264251724-en (Accessed September 20, 2022).
Pawlowski J., Kelly-Quinn M., Altermatt F., Apothéloz-Perret-Gentil L., Beja P., Boggero A., et al. (2018). The future of biotic indices in the ecogenomic era: integrating (e)DNA metabarcoding in biological assessment of aquatic ecosystems. Sci. Total Environ. 637–638, 1295–1310. doi: 10.1016/j.scitotenv.2018.05.002
Pereira H. M., Ferrier S., Walters M., Geller G. N., Jongman R. H. G., Scholes R. J., et al. (2013). Essential biodiversity variables. Science 339, 277–278. doi: 10.1126/science.1229931
Pérez-Espona S. (2021). Conservation-focused biobanks: a valuable resource for wildlife DNA forensics. Forensic Sci. Int. Anim. Environ. 1, 100017. doi: 10.1016/j.fsiae.2021.100017
Ramírez F., Sbragaglia V., Soacha K., Coll M., Piera J. (2022). Challenges for marine ecological assessments: completeness of findable, accessible, interoperable, and reusable biodiversity data in European seas. Front. Mar. Sci. 8. doi: 10.3389/fmars.2021.802235
Rodríguez A., Castrejón-Godínez M. L., Sánchez-Salinas E., Mussali-Galante P., Tovar-Sánchez E., Ma. L. (2022). “Pesticide bioremediation: OMICs technologies for understanding the processes,” in Pesticides bioremediation. Eds. Siddiqui S., Meghvansi M. K., Chaudhary K. K. (Cham: Springer International Publishing), 197–242. doi: 10.1007/978-3-030-97000-0_8
Rodríguez-Ezpeleta N., Zinger L., Kinziger A., Bik H. M., Bonin A., Coissac E., et al. (2021). Biodiversity monitoring using environmental DNA. Mol. Ecol. Resour. 21, 1405–1409. doi: 10.1111/1755-0998.13399
Santi I., Cancio I., Corre E., Cox C., Exter K., Hoebeke M., et al. (2022). European Marine omics biodiversity observation network (EMO BON) – data management plan. EMBRC-ERIC. doi: 10.25607/OBP-1743
Santi I., Casotti R., Comtet T., Cunliffe M., Koulouri Y., Macheriotou L., et al. (2021). European Marine omics biodiversity observation network (EMO BON) handbook (Paris: EMBRC-ERIC). doi: 10.25607/OBP-1653
Sartaj K., Patel A., Matsakas L., Prasad R. (2022). Unravelling metagenomics approach for microbial biofuel production. Genes 13, 1942. doi: 10.3390/genes13111942
Satterthwaite E. V., Bax N. J., Miloslavich P., Ratnarajah L., Canonico G., Dunn D., et al. (2021). Establishing the foundation for the global observing system for marine life. Front. Mar. Sci. 8. doi: 10.3389/fmars.2021.737416
Sequino G., Valentino V., Villani F., De Filippis F. (2022). Omics-based monitoring of microbial dynamics across the food chain for the improvement of food safety and quality. Food Res. Int. 157, 111242. doi: 10.1016/j.foodres.2022.111242
Shaaban M. T., Abdelhamid R. M., Zayed M., Ali S. M. (2022). Evaluation of a new antimicrobial agent production (RSMM C3) by using metagenomics approaches from Egyptian marine biota. Biotechnol. Rep. 34, e00706. doi: 10.1016/j.btre.2022.e00706
Snelgrove P. V. R., Thrush S. F., Wall D. H., Norkko A. (2014). Real world biodiversity–ecosystem functioning: a seafloor perspective. Trends Ecol. Evol. 29, 398–405. doi: 10.1016/j.tree.2014.05.002
Soiland-Reyes S., Sefton P., Crosas M., Castro L. J., Coppens F., Fernández J. M., et al. (2022). Packaging research artefacts with RO-crate. Data Sci. 5, 97–138. doi: 10.3233/DS-210053
Speich S., Lee T., Muller-Karger F., Lorenzoni L., Pascual A., Jin D., et al. (2019). Editorial: Oceanobs’19: an ocean of opportunity. Front. Mar. Sci. 6. doi: 10.3389/fmars.2019.00570
Suarez-Bregua P., Álvarez-González M., Parsons K. M., Rotllant J., Pierce G. J., Saavedra C. (2022). Environmental DNA (eDNA) for monitoring marine mammals: challenges and opportunities. Front. Mar. Sci. 9. doi: 10.3389/fmars.2022.987774
Sumaila U. R., Walsh M., Hoareau K., Cox A., Teh L., Abdallah P., et al. (2021). Financing a sustainable ocean economy. Nat. Commun. 12, 3259. doi: 10.1038/s41467-021-23168-y
Task Team for an Integrated Framework for Sustained Ocean Observing (2012). A framework for ocean observing (Paris: UNESCO). Available at: http://unesdoc.unesco.org/images/0021/002112/211260e.pdf.
Turunen J., Mykrä H., Elbrecht V., Steinke D., Braukmann T., Aroviita J. (2021). The power of metabarcoding: can we improve bioassessment and biodiversity surveys of stream macroinvertebrate communities? Metabarcoding Metagenomics 5, e68938. doi: 10.3897/mbmg.5.68938
Wieczorek J., Bloom D., Guralnick R., Blum S., Döring M., Giovanni R., et al. (2012). Darwin Core: an evolving community-developed biodiversity data standard. PloS One 7, e29715. doi: 10.1371/journal.pone.0029715
Wilkinson M. D., Dumontier M., Aalbersberg I., Appleton G., Axton M., Baak A., et al. (2016). The FAIR guiding principles for scientific data management and stewardship. Sci. Data 3, 1–9. doi: 10.1038/sdata.2016.18
Wurtzer S., Waldman P., Levert M., Cluzel N., Almayrac J. L., Charpentier C., et al. (2022). SARS-CoV-2 genome quantification in wastewaters at regional and city scale allows precise monitoring of the whole outbreaks dynamics and variants spreading in the population. Sci. Total Environ. 810, 152213. doi: 10.1016/j.scitotenv.2021.152213
Keywords: omics, marine biodiversity, ocean observation, water column, soft substrate, hard substrate, metagenomics, metabarcoding
Citation: Santi I, Beluche O, Beraud M, Buttigieg PL, Casotti R, Cox CJ, Cunliffe M, Davies N, de Cerio OD, Exter K, Kervella AE, Kotoulas G, Lagaisse R, Laroquette A, Louro B, Not F, Obst M, Pavloudi C, Poulain J, Præbel K, Vanaverbeke J and Pade N (2023) European marine omics biodiversity observation network: a strategic outline for the implementation of omics approaches in ocean observation. Front. Mar. Sci. 10:1118120. doi: 10.3389/fmars.2023.1118120
Received: 07 December 2022; Accepted: 02 May 2023;
Published: 18 May 2023.
Edited by:
Marta Coll, Spanish National Research Council (CSIC), SpainReviewed by:
Francisco Ramírez, Spanish National Research Council (CSIC), SpainJoan Giménez, Spanish National Research Council (CSIC), Spain
Copyright © 2023 Santi, Beluche, Beraud, Buttigieg, Casotti, Cox, Cunliffe, Davies, de Cerio, Exter, Kervella, Kotoulas, Lagaisse, Laroquette, Louro, Not, Obst, Pavloudi, Poulain, Præbel, Vanaverbeke and Pade. This is an open-access article distributed under the terms of the Creative Commons Attribution License (CC BY). The use, distribution or reproduction in other forums is permitted, provided the original author(s) and the copyright owner(s) are credited and that the original publication in this journal is cited, in accordance with accepted academic practice. No use, distribution or reproduction is permitted which does not comply with these terms.
*Correspondence: Nicolas Pade, nicolas.pade@embrc.eu