- 1Key Laboratory of Mariculture, Ministry of Education, Ocean University of China, Qingdao, China
- 2Function Laboratory for Marine Fisheries Science and Food Production Processes, Qingdao National Laboratory for Marine Science and Technology, Qingdao, China
The objective of this study was to investigate the effects of different rhythmic light spectra and intensities on growth performance and physiological and biochemical parameters of juvenile steelhead trout (Oncorhynchus mykiss). Seven treatments were randomly assigned to 21 tanks using a single-flow system for 13 weeks (N = 3), namely blue–purple–red light (BPR), red–purple–blue light (RPB), blue light (VB), and red light (VR). These light treatments alternated at 300, 900, and 1,200 lx, as well as a constant 900 lx of blue light (CB), red light (CR), and white light (CW). Results showed that the highest feed intake (FI), final body weight (FBW), and specific growth rate (SGR) were observed in the BPR treatment, which were significantly higher than those in the CW, CR, CB, and VB treatments. BPR treatment resulted in higher levels of insulin-like growth factor 1 (IGF-1), free triiodothyronine (FT3) and thyroxine (FT4), superoxide dismutase (SOD), and catalase (CAT), and total antioxidant capacity (T-AOC) activities were found. Fish exposed to BPR showed significantly enhanced lipase (LPS) and trypsin (Trp) activity in the stomach and gut tissues which promoted digestion. Trout exposed to a constant light spectra and intensity environment showed decreased activities of antioxidant and gastrointestinal digestive enzymes. Our results indicate the positive influence of BPR light conditions on the growth, stress response, digestion, and metabolism of juvenile steelhead trout, which is likely related to its similarity to the light rhythm in natural water environments, and can be used to improve growth and physiological status in the aquaculture trout.
1 Introduction
Light including light spectra, intensity, and photoperiod, is one of the most important factors in aquaculture, as it affects endocrine organs, especially the hypothalamus and hypophysis, through visual and central nervous systems. It ultimately affects feeding, growth, development, physiological status, and behavior (Handeland et al., 2013; Gao et al., 2021; Zou et al., 2022). Fish have a specific preference for certain light conditions because of evolutionary adaptation to the changing light environment. Light spectra and intensity may have different effects on growth performance among fish species and across development stages with positive or negative influences (Wei et al., 2019; Honryo et al., 2020; Wang et al., 2020; Mandario et al., 2021; Zou et al., 2022).
Light spectra and intensity play fundamental roles in the feeding and growth of fish. Different species exhibit specific preferences for certain light environments (Villamizar et al., 2009; Handeland et al., 2013; Wei et al., 2019; Wang et al., 2020; Khanjani and Sharifinia, 2021; Wu et al., 2021; Zou et al., 2022). Self-feeding of rainbow trout (Oncorhynchus mykiss) can be affected by light intensity (Mizusawa et al., 2007). Increased light intensity improves feeding efficiency in juvenile Atlantic salmon (Salmo salar) (Fraser and Metcalfe, 1997). Under high light intensity conditions, the specific growth rate (SGR) and feed conversion ratio (FCR) of rainbow trout are higher than those under low light intensities (Taylor et al., 2006). Low intensity red light is beneficial for promoting growth of rainbow trout, whereas blue light induces stress and other negative effects on growth (Karakatsouli et al., 2007; Karakatsouli et al., 2008). Chen et al. (2022b) found that blue and red light combinations instead of constant light spectra enhanced growth of steelhead trout (Oncorhynchus mykiss). However, to date, many studies have focused on the constant light spectra and intensity conditions of trout growth. Meanwhile, the influence of daily rhythmic light spectra and intensity changes has been limited.
The impacts of light spectra and intensity on fish growth involves endocrine hormones, circulating biochemical processes, antioxidant defenses, and digestion. The pineal gland interprets light signals and rhythmically synthesizes melatonin (MT) to maintain the body’s biological clock (Iigo et al., 2007). MT secretion is affected by light spectra, and simultaneous blue and green light exposure has shown a more pronounced inhibitory effect than red light in Atlantic salmon (Handeland et al., 2013). Growth hormone (GH), insulin-like growth factor 1 (IGF-1), and cortisol levels in olive flounder (Paralichthys olivaceus) were higher under green light than those under white light (Zou et al., 2022). Plasma free thyroxine (FT4) levels in Atlantic salmon significantly increased under high-intensity light (1000 lx) compared to those under low-intensity light (1 lx). Meanwhile, low-intensity light also led to abnormal development (Handeland et al., 2013). Various oxidative stress responses under different light spectrum and intensity have been highlighted in multiple fish species (Gao et al., 2016; Khanjani and Sharifinia, 2021; Wu et al., 2021; Chen et al., 2022b). Guller et al. (2020) found that blue light could enhance the antioxidant capacity and immune performance of rainbow trout. Digestive enzymes are crucial for nutrient absorption and synthesis, and are also significantly influenced by light spectra and intensity in steelhead trout (Chen et al., 2022a) and Nile tilapia (Oreochromis niloticus) (Khanjani and Sharifinia, 2021).
Light can be absorbed by water, and long-wavelength light spectra have a greater influence. As the height of the sun, namely the light incidence angle, changes regularly during the daytime, light spectra and intensity at a certain water depth simultaneously change rhythmically. Aquatic animals have evolved to adapt to these predictable changes and form rhythmic patterns of physiological and behavioral functions (Villamizar et al., 2011). In this study, we evaluated the growth, endocrine hormone levels, plasma biochemical parameters, antioxidant defense, and digestion of juvenile steelhead trout in response to different daily rhythmic light spectra and intensities. Our results provide more detailed insights into the influence of multi-level light conditions on fish growth and present novel evidence of optimal light conditions for trout aquaculture.
2 Materials and methods
2.1 Animal preparation
Steelhead trout eye eggs were purchased from Troutlodge Inc. (Washington, USA). Before the experiment commenced, the juvenile trout were acclimatized to fresh water for two weeks, when the fish were fed to apparent satiation twice a day at 08:00 and 16:00 with a commercial trout feed and maintained on a 24 h oxygen supply and 12 L:12D photoperiod.
2.2 Experimental design
Twenty-one fiberglass tanks (220 L volume, 0.60 m height × 0.84 m diameter) coated with white paint on the inner surface and blue paint on the outer surface were used during the experiment. Each tank was covered with shade cloth to avoid ambient light contamination. Customized LED lighting systems with the following spectra and intensities were used for seven treatments: red (λ = 660–670 nm, 0–100 W), blue (λ = 455–465 nm, 0–100 W), purple (λ = 400–435 nm, 0–100 W), and white (full spectrum, 0–100 W) (Wuxi Huazhaohong Optoelectronic Technology Co., Ltd., Wuxi, China). Light spectra and intensity were determined using a lighting analyzer (PLA-300; Everfine Inc., Hangzhou, China) and were adjusted every two days. The light intensity for different experimental requirements was determined by adjusting the power of the LED lamps and their height from the water surface. Each treatment had three replicates with 12 fish per tank.
The lighting conditions for the seven treatments were as follows: constant white (900 Lux, CW), constant red (900 Lux, CR), constant blue (900 Lux, CB), the same daily rhythmic change (600 + 900 + 1200 + 900 + 600 Lux) of red (VR) and blue (VB), daily rhythmic changes of red, purple, and blue (600 Lux red + 900 Lux purple + 1200 Lux blue + 900 Lux purple + 600 Lux red, RPB), and daily rhythmic changes in blue, purple, and red (600 Lux blue + 900 Lux purple + 1200 Lux red + 900 Lux purple + 600 Lux blue, BPR) (Figure 1). The tested light intensity in the present study are in order to confer to the reported light intensity from rainbow trout and Atlantic salmon (Mizusawa et al., 2007; Handeland et al., 2013), and our previous study on steelhead trout (unpublished data). The photoperiod for all treatments was set at 15 L: 9 D between 4:30 and 19:30. The light duration for rhythmic change groups was divided into five periods, each of which lasted three hours.
At the beginning and end of the experiment, the trout were starved for 24 h to ensure complete emptying of the digestive tract. Prior to commencing the experiment, the fish were anesthetized in a 30 mg/L tricaine methanesulfonate (MS-222; Sigma-Aldrich, USA) solution, after which they were gently dried and weighed. During the experiment, all the fish (initial weight, 30.01 ± 2.95 g) were fed twice daily (at 08:00 and 16:00) with commercial trout feed. Uneaten surplus feed was collected after feeding for 30 min. The feed intake was calculated by measuring the feed moisture content and correcting the actual daily intake. Water exchange capacity during the experiment was maintained at 70 L/h using a flowing water system. Water temperature, dissolved oxygen level, and pH were measured three times per day using a YSI professional water quality tester (YSI Inc., Ohio, USA). Water samples were collected every three days to determine phosphate, total ammonia nitrogen, nitrite, and nitrate nitrogen using an automatic chemical analyzer (Cleverchem 380 cadmium, DeChem-Tech, Germany). During the 13-week experimental period, constant water temperature, dissolved oxygen, and pH were obtained at 16 ± 0.2°C, > 6.5 mg/L, and 7.2 ± 0.2, respectively. Phosphate, total ammonia nitrogen, nitrite, and nitrate nitrogen were maintained at 0.065 ± 0.003 mg/L, 0.127 ± 0.003 mg/L, 0.09 ± 0.05 mg/L, and 3.43 ± 1.9 mg/L, respectively.
At the end of the experiment, 15 fish from each treatment with five fish per treatment, were randomly selected and immediately anesthetized using 100 mg/L MS-222 and were then placed in an ice tray. Liver, midgut, and stomach tissues were collected using sterilized scissors and forceps, placed in polyethylene tubes (5 mL; Beijing Labgic Technology Co., Ltd., Beijing, China), and immediately snap-frozen in liquid nitrogen. Blood samples were collected from the tail vein using a 2.5-mL syringe and stored in 1.5-mL polyethylene tubes, centrifuged at 875 rpm and 4°C for 10 min. The serum was separated from the red blood cells, transferred into polyethylene tubes, and immediately placed in liquid nitrogen. All the samples were stored at −80 °C until further analysis.
2.3 Growth parameters
To calculate and compare feeding and growth, fish biometry, including total number and weight, was measured at the beginning and end of the culture period. The number of fish at the beginning of the experiment and the number of fish remaining at the end of the experiment were recorded respectively to calculate the survival rate. Feeding, growth parameters, and nutrition indices included initial body weight (IBW), final body weight (FBW), specific growth rate (SGR), feed intake (FI), feed conversion ratio (FCR), and survival rate (SR). The SGR, FCR, and SR are calculated as follows:
Where t is the number of experimental days, Ft is the number of fish at the end of the experiment, and F0 is the number of fish at the beginning of the experiment.
2.4 Serum hormones and biochemical analyses
Melatonin (MT), growth hormone (GH), insulin-like growth factor 1 (IGF-1), free triiodothyronine (FT3), and free thyroxine (FT4) levels in serum were determined using enzyme-linked immunosorbent assay kits (Nanjing Jiancheng Bioengineering Institute, Nanjing, China). Serum triglyceride (TG), total cholesterol (TC), and glucose (GLU) levels were measured using a Cobas C-311 automatic biochemical analyzer (Roche, Basel, Switzerland). Activities of alanine transferase (ALT), alkaline phosphatase (ALP), and lactate dehydrogenase (LDH) were analyzed using kits (Roche, Inc., Basel, Switzerland).
2.5 Antioxidant capacity analyses
Superoxide dismutase (SOD), catalase (CAT), total antioxidant capacity (T-AOC) activity, and malondialdehyde (MDA) levels were analyzed using kits (Nanjing Jiancheng Bioengineering Institute, Nanjing, China).
2.6 Digestive enzyme activity analyses
Amylase (AMS), lipase (LPS), pepsin (Pep) and trypsin (Trp) were analyzed using assay kits (Nanjing Jiancheng Bioengineering Institute, Nanjing, China)
2.7 Statistical analysis
All parameters are expressed as mean ± standard deviation. SAS version 9.4 (SAS Institute Inc., Cary, North Carolina, USA) was used for the statistical analysis. Differences among treatments were analyzed using one-way analysis of variance (ANOVA), followed by Tukey’s multiple comparison tests. The significance level was set at P = 0.05.
3 Results
3.1 Growth parameters
The growth parameters for juvenile steelhead trout are presented in Table 1. Different daily rhythmic light spectra and intensity changes significantly affected trout growth (P< 0.05). The FBW and SGR in the BPR group were the highest and were significantly higher than those in the CW, CB, CR, and VB groups (P< 0.05). The highest FI levels were observed in the BPR and RPB groups. The FI in the CW, CR, CB and VB groups were significantly lower than those in the BPR and RPB groups (P< 0.05). There were no significant differences in FCR and SR among the groups (P > 0.05).
3.2 Serum hormones levels
The serum hormone levels in juvenile steelhead trout are presented in Figure 2. There was no significant difference in GH levels between groups (P > 0.05). However, IGF-1 levels were significantly higher in the BPR group than those in the other groups (P< 0.05). No significant difference was observed in MT levels among the groups (P > 0.05). FT3 levels were significantly higher in the BPR group than those in the other groups, and FT4 levels were significantly higher in the BPR group than those in the CW, VR, VB, and RPB groups (P< 0.05).
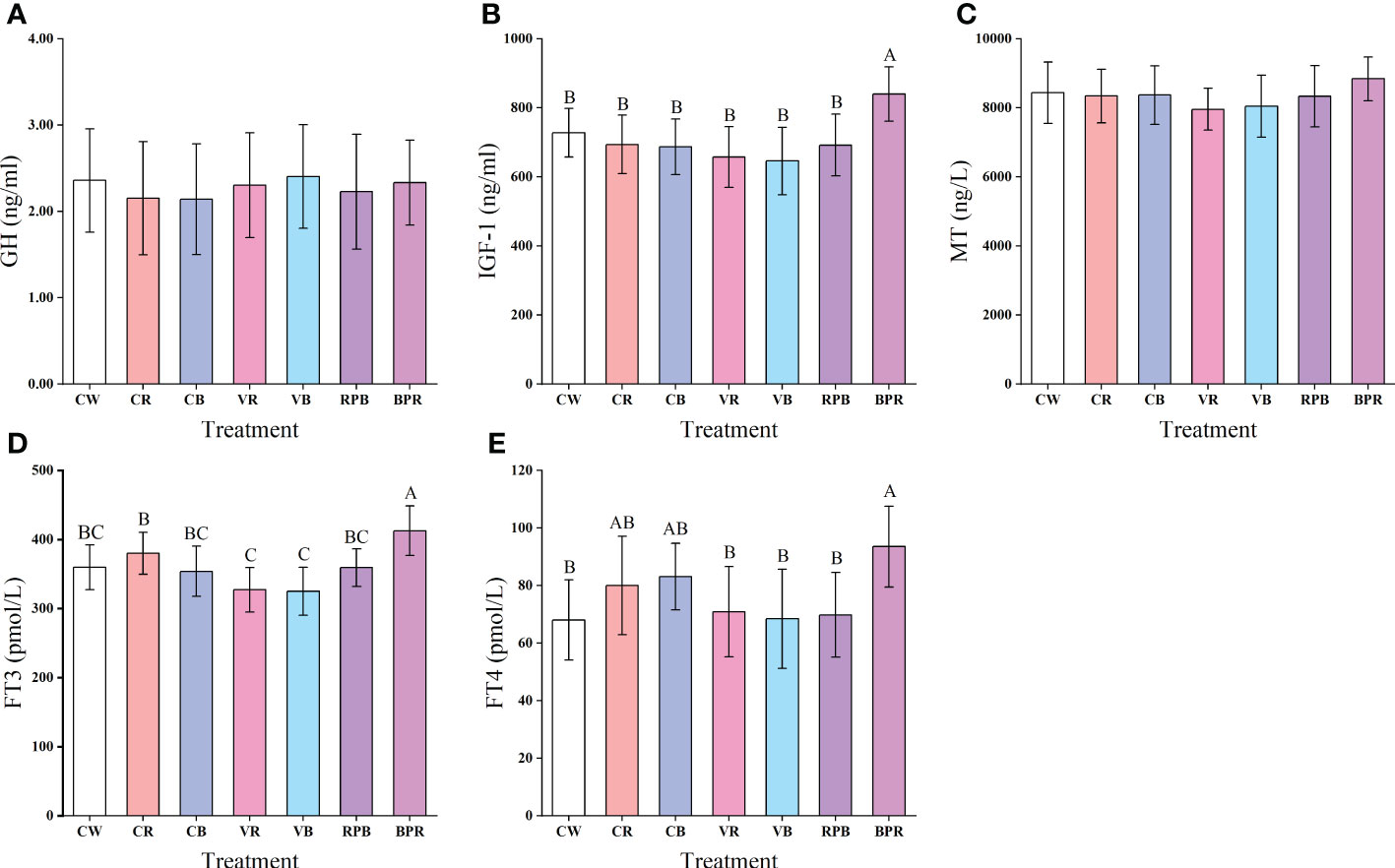
Figure 2 Serum hormones levels of GH (A), IGF-1 (B), MT (C), FT3 (D) and FT4 (E) in juvenile steelhead trout reared under different light conditions. Different capital letters denote significant differences among the steelhead trout treatments. Results are expressed as mean ± standard deviation (n=15).
3.3 Serum biochemical parameters
Serum levels of TG, TC, and GLU and activities of ALT, ALP, and LDH are presented in Table 2. There were no statistically significant differences in serum TG, TC, and GLU levels (P > 0.05). ALT activity was significantly higher in the CW group than those in the CB, VR, RPB and BPR groups (P< 0.05). ALT activity was significantly lower in the VR and BPR groups than those in the CW, CR and VB groups (P< 0.05). ALP activity was significantly higher in the CW group than those in the CR, CB, VR, and VB groups (P< 0.05). Statistically higher LDH activity was observed in the CW group than those in the other groups (P< 0.05). The lowest LDH activity were observed in the BPR and RPB groups.
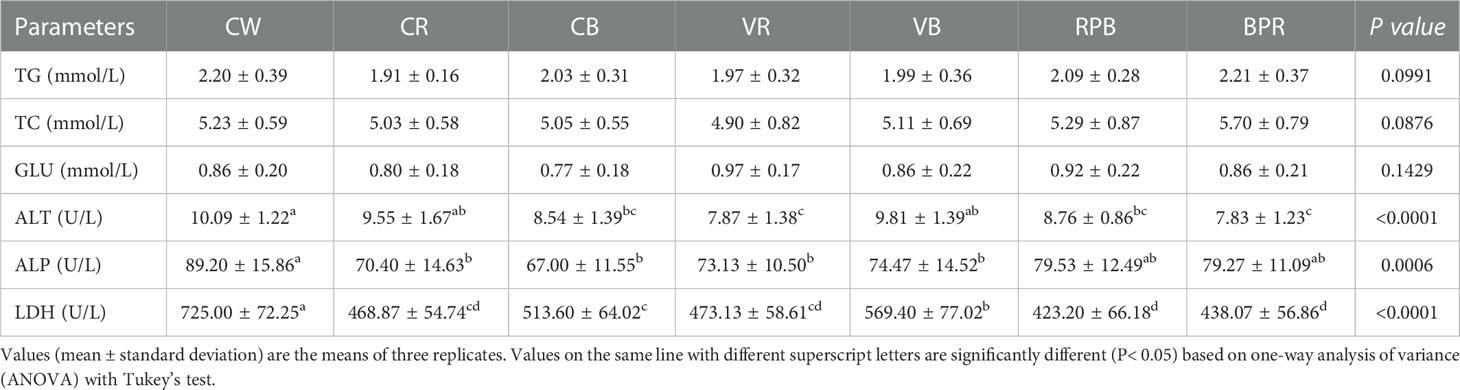
Table 2 The serum biochemical parameters of steelhead trout reared under different light conditions.
3.4 Antioxidant capacity
Antioxidant capacity was significantly affected by the daily rhythmic light spectra and intensity changes (Figure 3). CAT and T-AOC activities in the RPB and BPR groups were significantly higher than those in the other groups (P< 0.05). SOD activity in the BPR group was significantly higher than those in the CW, CR, CB, VR, and VB groups (P< 0.05), but not significantly higher than those in the RPB group (P > 0.05). The lowest SOD activity was observed in the CW and VR groups. T-AOC activity in the CW, CR and VR groups was significantly lower than those in the CB, VB, BPR and RPB groups (P< 0.05). MDA levels in the CR and VR groups were significantly higher than those in the CB, VB, RPB, and BPR groups (P< 0.05).
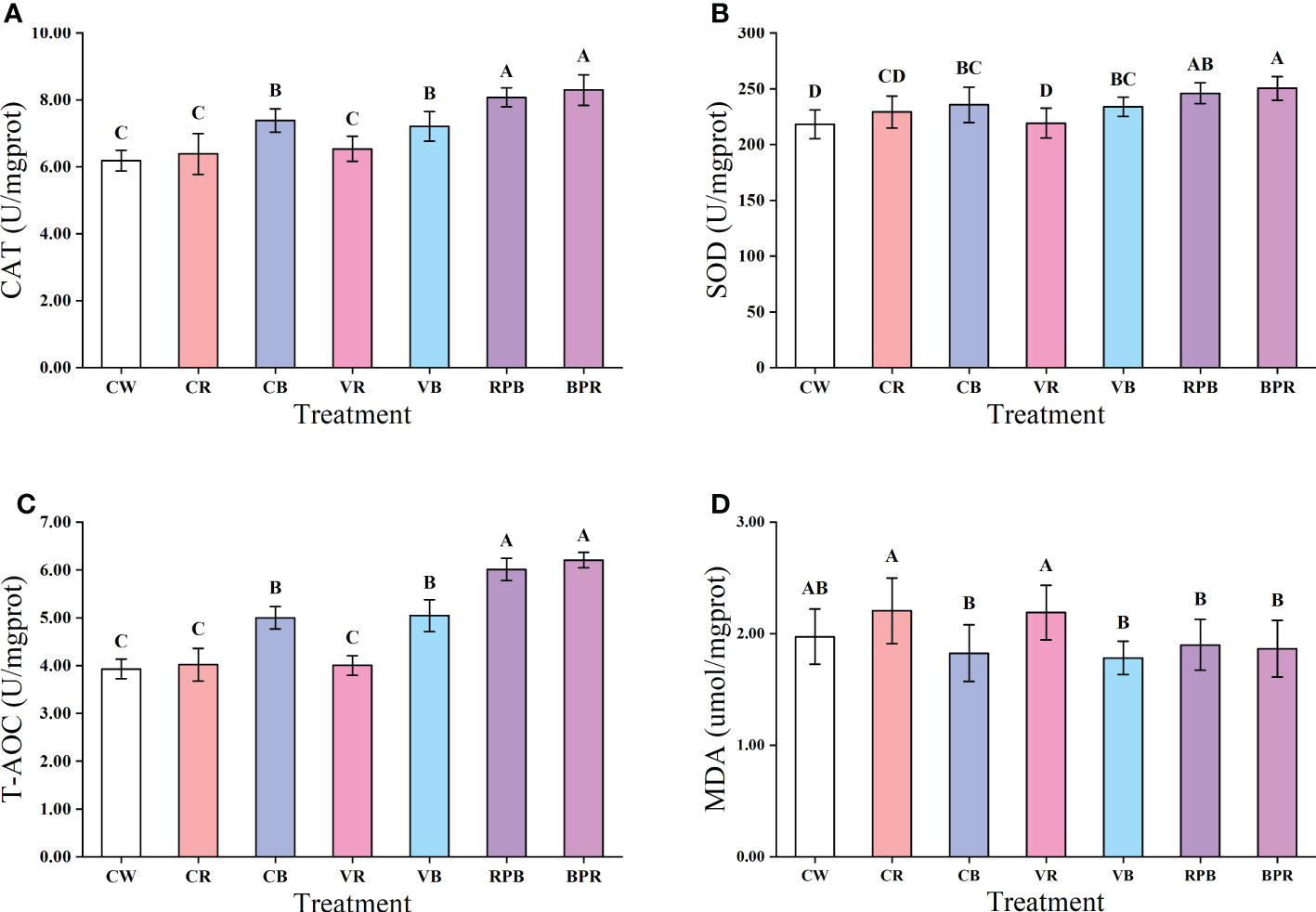
Figure 3 Antioxidant enzymes activities of the liver in juvenile steelhead trout reared under different light color conditions. (A) CAT activity; (B) SOD activity; (C) T-AOC activity; (D) MDA level. Different capital letters denote significant differences among the steelhead trout treatments. Results are expressed as mean ± standard deviation (n=15).
3.5 Digestive enzyme activity
Activities of AMS, LPS, Pep, and Trp in the midgut and stomach are shown in Figure 4. Midgut and gastric LPS activities were significantly higher in the BPR group than those in the other groups (P< 0.05). Statistically higher midgut Trp activity was observed in the BPR group than those in the other groups (P< 0.05). Trp activity in the CW and VB groups was significantly lower than those in the other groups (P< 0.05). No statistical differences were detected in AMS and gastric Pep among the groups (P > 0.05).
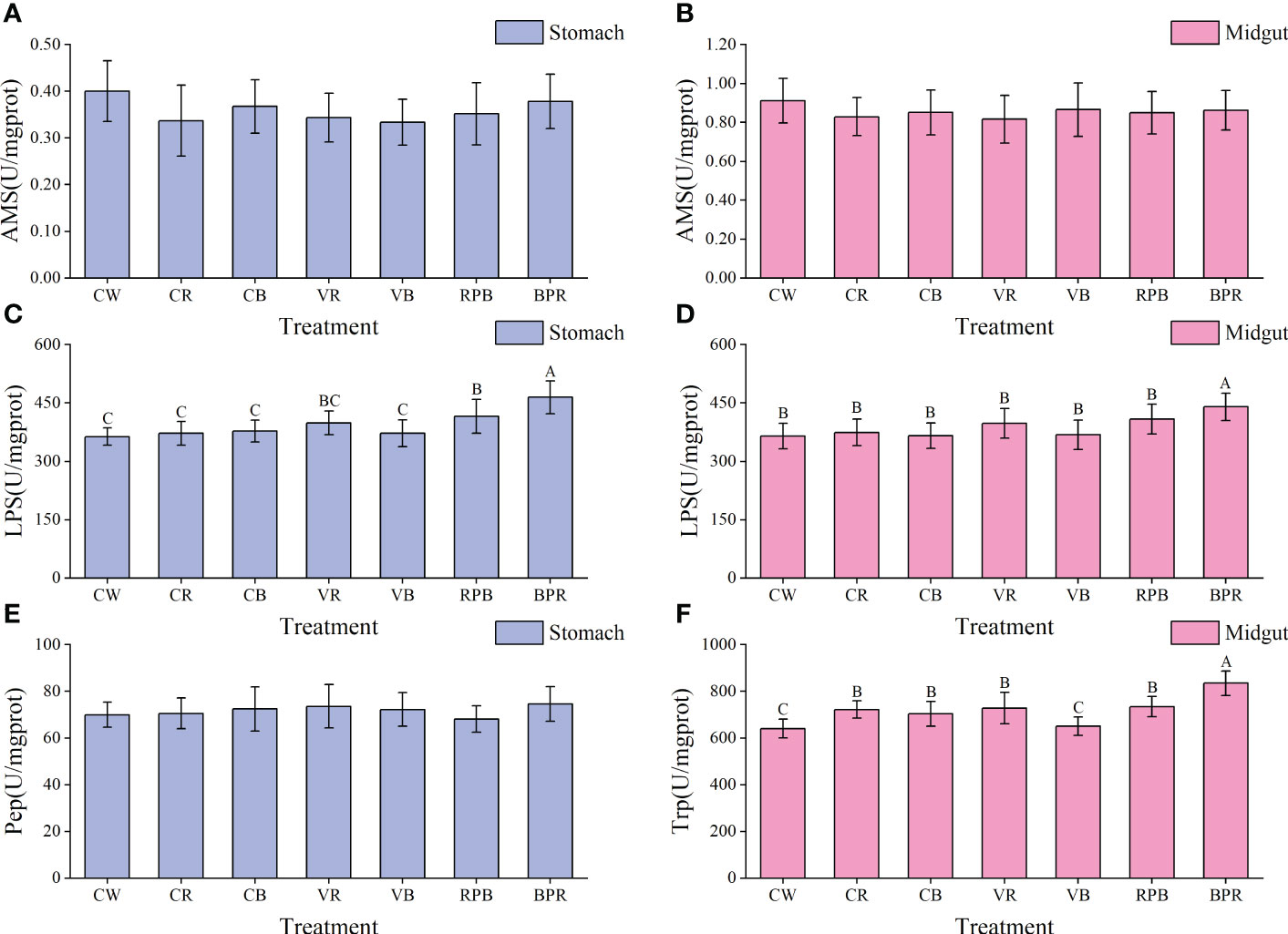
Figure 4 Digestive enzymes activities of the midgut and stomach in juvenile steelhead trout reared under different light color conditions. (A) AMS activity in the stomach; (B) AMS activity in the midgut; (C) LPS activity in the stomach; (D) LPS activity in the midgut; (E) Pep activity in the stomach; (F) Trp activity in the midgut. Different capital letters denote significant differences among the steelhead trout treatments. Results are expressed as mean ± standard deviation (n=15).
4 Discussion
In the natural aquatic environment, because of the strong absorption capacity of water for long-wavelength light and the regular height changes of the sun (light incidence angle) during the day, the light intensity and spectra change rhythmically at a certain water depth (Sanchez-Vazquez et al., 2019; Ruchin, 2021). Teleost fish can experience reduced stress and maintain homeostasis because of evolutionary adaptation to their specific living light environment. This is conducive to growth, development, and reproduction (Kusmic and Gualtieri, 2000; Cheng and Flamarique, 2007; Villamizar et al., 2011; Grossman, 2014; Eilertsen et al., 2018). Different light conditions can affect fish feeding (Kraaij et al., 1998; Cobcroft et al., 2001; Kestemont and Baras, 2001; Marchand et al., 2002; Puvanendran and Brown, 2002). Feed intake by rainbow trout is positively correlated with light intensity within a tolerable range (Mizusawa et al., 2007). Karakatsouli et al. (2008) and Karakatsouli et al. (2007) showed that growth performance of rainbow trout exposed to a low intensity of red light was enhanced compared to blue light, and blue light induced an increased stress response.
In the present study, rhythmic rather than constant light intensity and spectra in the BPR group were more conducive to growth, feeding, and metabolism of juvenile steelhead trout (Table 1). Our results are consistent with those of Chen et al. (2022b). Chen et al. (2022b) reported that blue and red light combinations improved feeding conversion efficiency and enhanced growth of steelhead trout. Our data showed that the light variation pattern of the BPR group was closer to that of natural water than the constant light spectra and constant/rhythmic light intensity. Low light intensity in the morning and evening was akin to a small light incidence angle, long irradiation distance, and high proportion of short-wavelength light. Meanwhile, high light intensity and a large proportion of long-wavelength light were in line with the natural light conditions at noon. LED lights have been widely used in aquaculture as a controllable artificial light source (Song et al., 2018). Our results suggest that an LED lighting system closely simulating the light conditions of the natural habitat of steelhead trout, such as the daily rhythmic change in BPR lighting, can improve fish growth.
The pineal gland and retina of teleost fish are photoreceptors and the photosensitive rod photoreceptor and cone cells help them to identify specific wavelengths (Kraaij et al., 1998). Mediated by the hypothalamus-pituitary-growth hormone (HPGH) pathway, GH and IGF-1 have a significant influence on the growth of fish directly or indirectly (Bjornsson, 1997; Mommsen, 2001; Perez-Sanchez et al., 2002; Zou et al., 2022). However, fish metabolism and the external environment affect the levels of GH and IGF-1 (Bertucci et al., 2019; Triantaphyllopoulos et al., 2020). Some studies have shown that IGF-1 content is positively correlated with fish growth (Wang et al., 2020; Perello-Amoros et al., 2021; Chen et al., 2022b). Our findings show that daily rhythmic light spectra and intensity changes in the BPR group significantly increased IGF-1 concentrations through indirect stimulation of the visual system, which promoted the growth of steelhead trout (Figure 2). Our data showed that the serum GH content of steelhead trout was much lower than that of IGF, with no significant difference among the groups, which had no significant effect on the growth. A signal molecule that acts as a receptor of the external light environment, MT is predominantly involved in the circadian rhythm and gonad development of fish. It can also regulate synthesis of related hormones (Bromage et al., 2001; Falcon et al., 2007; Zou et al., 2022). Thyroid hormones (THs) play an important role in regulating growth, development, reproduction, migration, metabolism, and osmotic pressure of fish. They mainly exist in the form of FT3 and FT4 in blood (Johnson and Lema, 2011; Peter, 2011; Mullur et al., 2014; Abdalla and Bianco, 2014). In this study, there was no significant difference in MT levels among groups. However, serum FT3 and FT4 levels in steelhead trout were significantly higher in the BPR group than in the other groups. Our results indicate that rhythmic BPR lighting exerted a positive effect on growth-related hormones in steelhead trout.
A uncomfortable light environment stimulates the visual system of fish and results in a stress response. This can subsequently disrupt the immune system and resulting in adverse outcomes (Song and Choi, 2019). Several biochemical parameters, including ALT, ALP, and LDH, reflect oxidative stress levels. In this study, liver and myocardium functions of juvenile steelhead trout were likely disrupted under constant 900 lx white light. This resulted in high levels of circulating ALT, ALP, and LDH, and consequently low growth rates. Our results indicate that conventional light sources that use constant white light in aquaculture are potentially harmful to trout.
The antioxidant defense system of fish can be disrupted by external environmental changes and produces reactive oxygen species (ROS).This can negatively impact fish health (Nyska and Kohen, 2002; Nishida, 2011). The appropriate light intensity and spectra can enhance the antioxidant enzymes activities of fish, including SOD, CAT, and T-AOC, comprising the antioxidant defense system and playing a crucial role in decreasing intracellular ROS, and reducing cell damage (Shin et al., 2011; Gao et al., 2016; Guller et al., 2020; Akhtar et al., 2022; Chen et al., 2022b). MDA can cause cell membrane damage and function loss, resulting in negative effects on other physiological functions. Therefore, it is used as an important index reflecting the oxidative damage level (Martinez-Alvarez et al., 2002). Long-wavelength red light emitted from LED lights can cause oxidative stress in fish. Meanwhile, short-wavelength light can effectively reduce oxidative stress and improve immunity. Our findings have shown that there has been an apparent decrease in the antioxidant capacity of juvenile steelhead trout exposed to red light. The MDA level in the constant and daily rhythmic change of red light groups was significantly higher than that in other groups, except for constant white light. Our results are in line with those of Guller et al. (2020), who reported that the activities of liver antioxidant enzymes in rainbow trout were higher when exposed to blue light than when exposed to red light. In this study, the CAT, SOD, and T-AOC activities of juvenile steelhead trout in the BPR and RPB groups exposed to daily rhythmic light spectra and intensity changes were significantly higher than those in the other groups. In a recent study on blue–red light combinations of juvenile steelhead trout, this combination improved antioxidant capacity by increasing activities of liver antioxidant enzymes, while constant red light increased MDA levels This led to intense stress and decreased immunity (Chen et al., 2022b). This phenomenon indicates that changes in light conditions induce a response in the antioxidant defense system via the visual system. This can contribute to preventing physiological damage to the changing environment.
Digestive enzyme activity is an important index for measuring fish digestion and metabolism ability (Perez-Sirkin et al., 2020; Yu et al., 2020a). This can be affected by feeding habits (Castro-Ruiz et al., 2019; Yu et al., 2020b) and light environment (Khanjani and Sharifinia, 2021; Chen et al., 2022a). In this study, activities of LPS and Trp in the stomach and midgut of juvenile steelhead trout were higher than those of AMS. This is consistent with the characteristics of steelhead trout as carnivorous fish. Our data showed no significant difference in FI between the BPR and RPB groups. However, LPS and Trp activities in the BPR group were significantly higher than those in the RPB group. FCR was also higher in the BPR group, suggesting improved digestion ability. Results of this study indicate that the positive influence of the BPR light environment improves feeding, IGF-1 level, antioxidant capacity, digestion and metabolism abilities of juvenile steelhead trout, which promote its growth. This may be explained by evolutionary adaptation of fish to their natural living light environment.
5 Conclusion
In this study, compared with other constant or rhythmic light treatments, the BPR light environment, which is akin to the light rhythm in natural waters, was beneficial to the feeding, growth, stress resistance, digestion, and metabolism of juvenile steelhead trout. These findings are worth considering in aquaculture lighting applications as an effective means of improving fish growth.
Data availability statement
The original contributions presented in the study are included in the article/Supplementary Material. Further inquiries can be directed to the corresponding authors.
Ethics statement
The animal study was reviewed and approved by Regulations of the Administration of Affairs Concerning Experimental Animals of China Regulations of the Administration of Affairs Concerning Experimental Animals of Shandong Province. Written informed consent was obtained from the owners for the participation of their animals in this study.
Author contributions
SM performed the trial and data analysis, wrote the original draft, and proposed the research methods and ideas. YZ and SD supervised research progress, proposed research methods and research ideas, and edited manuscript. XC and SC performed the trial. LL, YD, and QG reviewed and edited the original draft. All authors contributed to the article and approved the submitted version.
Funding
This publication was supported by the National Natural Science Foundation of China (NSFC) (Nos. U1906206 and 31872575), the National Key Research and Development Program of China (No. 2019YFD0901000), and the Scientific and Technological Demonstration for Offshore Facilities Fisheries of Shandong Province (2021SFGC0701).
Acknowledgments
The authors of this study would like to thank everyone who critically reviewed this manuscript and helped with the sample collection. This study was supported by the National Natural Science Foundation of China (NSFC) (Nos. U1906206 and 31872575), the National Key Research and Development Program of China (No. 2019YFD0901000), and the Scientific and Technological Demonstration for Offshore Facilities Fisheries of Shandong Province (2021SFGC0701), and the OUC-AUBURN Joint Research Center for Aquaculture and Environmental Sciences.
Conflict of interest
The authors declare that the research was conducted in the absence of any commercial or financial relationships that could be construed as a potential conflict of interest.
Publisher’s note
All claims expressed in this article are solely those of the authors and do not necessarily represent those of their affiliated organizations, or those of the publisher, the editors and the reviewers. Any product that may be evaluated in this article, or claim that may be made by its manufacturer, is not guaranteed or endorsed by the publisher.
References
Abdalla S. M., Bianco A. C. (2014). Defending plasma T3 is a biological priority. Clin. Endocrinol. 81 (5), 633–641. doi: 10.1111/cen.12538
Akhtar M. S., Tripathi P. H., Ciji A. (2022). Light spectra influence the reproductive performance and expression of immune and anti-oxidative defense genes in endangered golden mahseer (Tor putitora) female brooders. Aquaculture 547, 737355. doi: 10.1016/j.aquaculture.2021.737355
Bertucci J. I., Blanco A. M., Sundarrajan L., Rajeswari J. J., Velasco C., Unniappan S. (2019). Nutrient regulation of endocrine factors influencing feeding and growth in fish. Front. Endocrinol. 10. doi: 10.3389/fendo.2019.00083
Bjornsson B. T. (1997). The biology of salmon growth hormone: from daylight to dominance. Fish Physiol. Biochem. 17 (1-6), 9–24. doi: 10.1023/a:1007712413908
Bromage N., Porter M., Randall C. (2001). The environmental regulation of maturation in farmed finfish with special reference to the role of photoperiod and melatonin. Aquaculture 197 (1-4), 63–98. doi: 10.1016/s0044-8486(01)00583-x
Castro-Ruiz D., Mozanzadeh M. T., Fernandez-Mendez C., Andree K. B., Garcia-Davila C., Cahu C., et al. (2019). Ontogeny of the digestive enzyme activity of the Amazonian pimelodid catfish Pseudoplatystoma punctifer (Castelna 1855). Aquaculture 504, 210–218. doi: 10.1016/j.aquaculture.2019.01.059
Cheng C. L., Flamarique I. N. (2007). Chromatic organization of cone photoreceptors in the retina of rainbow trout: single cones irreversibly switch from UV (SWS1) to blue (SWS2) light sensitive opsin during natural development. J. Exp. Biol. 210 (23), 4123–4135. doi: 10.1242/jeb.009217
Chen X., Zhou Y., Huang J., An D., Li L., Dong Y., et al. (2022a). Blue and red light color combinations can enhance certain aspects of digestive and anabolic performance in juvenile steelhead trout Oncorhynchus mykiss. Front. Mar. Sci. 9. doi: 10.3389/fmars.2022.853327
Chen X., Zhou Y., Huang J., An D., Li L., Dong Y., et al. (2022b). The effects of blue and red light color combinations on the growth and immune performance of juvenile steelhead trout, Oncorhynchus mykiss. Aquacul. Rep. 24, 101156. doi: 10.1016/j.aqrep.2022.101156
Cobcroft J. M., Pankhurst P. M., Hart P. R., Battaglene S. C. (2001). The effects of light intensity and algae-induced turbidity on feeding behaviour of larval striped trumpeter. J. Fish Biol. 59 (5), 1181–1197. doi: 10.1111/j.1095-8649.2001.tb00185.x
Eilertsen M., Valen R., Drivenes O., Ebbesson L. O. E., Helvik J. V. (2018). Transient photoreception in the hindbrain is permissive to the life history transition of hatching in Atlantic halibut. Dev. Biol. 444 (2), 129–138. doi: 10.1016/j.ydbio.2018.10.006
Falcon J., Besseau L., Sauzet S., Boeuf G. (2007). Melatonin effects on the hypothalamo-pituitary axis in fish. Trends Endocrinol. Metab. 18 (2), 81–88. doi: 10.1016/j.tem.2007.01.002
Fraser N. H. C., Metcalfe N. B. (1997). The costs of becoming nocturnal: Feeding efficiency in relation to light intensity in juvenile Atlantic salmon. Funct. Ecol. 11 (3), 385–391. doi: 10.1046/j.1365-2435.1997.00098.x
Gao X. L., Li X., Li M. J., Song C. B., Liu Y. (2016). Effects of light intensity on metabolism and antioxidant defense in Haliotis discus hannai ino. Aquaculture 465, 78–87. doi: 10.1016/j.aquaculture.2016.08.010
Gao X. L., Pang G., Luo X., You W., Ke C. (2021). Effects of light cycle on motion behaviour and melatonin secretion in Haliotis discus hannai. Aquaculture 532, 735981. doi: 10.1016/j.aquaculture.2020.735981
Grossman G. D. (2014). Not all drift feeders are trout: a short review of fitness-based habitat selection models for fishes. Environ. Biol. Fish. 97 (5), 465–473. doi: 10.1007/s10641-013-0198-3
Guller U., Onalan S., Arabaci M., Karatas B., Yasar M., Kufrevioglu O. I. (2020). Effects of different LED light spectra on rainbow trout (Oncorhynchus mykiss): in vivo evaluation of the antioxidant status. Fish Physiol. Biochem. 46 (6), 2169–2180. doi: 10.1007/s10695-020-00865-x
Handeland S. O., Imsland A. K., Ebbesson L. O. E., Nilsen T. O., Hosfeld C. D., Baeverfjord G., et al. (2013). Low light intensity can reduce Atlantic salmon smolt quality. Aquaculture 384-387, 19–24. doi: 10.1016/j.aquaculture.2012.12.016
Honryo T., Okada T., Kawahara M., Sawada Y., Kurata M., Ishibashi Y. (2020). Effects of night-time light intensity and area of illumination in the sea cage culture of pacific bluefin tuna (Thunnus orientalis) juveniles. Aquaculture 521, 735046. doi: 10.1016/j.aquaculture.2020.735046
Iigo M., Abe T., Kambayashi S., Oikawa K., Masuda T., Mizusawa K., et al. (2007). Lack of circadian regulation of in vitro melatonin release from the pineal organ of salmonid teleosts. Gen. Comp. Endocrinol. 154 (1-3), 91–97. doi: 10.1016/j.ygcen.2007.06.013
Johnson K. M., Lema S. C. (2011). Tissue-specific thyroid hormone regulation of gene transcripts encoding iodothyronine deiodinases and thyroid hormone receptors in striped parrotfish (Scarus iseri). Gen. Comp. Endocrinol. 172 (3), 505–517. doi: 10.1016/j.ygcen.2011.04.022
Karakatsouli N., Papoutsoglou S. E., Panopoulos G., Papoutsoglou E. S., Chadio S., Kalogiannis D. (2008). Effects of light spectrum on growth and stress response of rainbow trout Oncorhynchus mykiss reared under recirculating system conditions. Aquacul. Eng. 38 (1), 36–42. doi: 10.1016/j.aquaeng.2007.10.006
Karakatsouli N., Papoutsoglou S. E., Pizzonia G., Tsatsos G., Tsopelakos A., Chadio S., et al. (2007). Effects of light spectrum on growth and physiological status of gilthead seabream Sparus aurata and rainbow trout Oncorhynchus mykiss reared under recirculating system conditions. Aquacul. Eng. 36 (3), 302–309. doi: 10.1016/j.aquaeng.2007.01.005
Kestemont P., Baras E. (2001). Environmental factors and feed intake: Mechanisms and interactions. Food Intake Fish 6, 131–156. doi: 10.1002/9780470999516.ch6
Khanjani M. H., Sharifinia M. (2021). Production of Nile tilapia Oreochromis niloticus reared in a limited water exchange system: The effect of different light levels. Aquaculture 542, 736912. doi: 10.1016/j.aquaculture.2021.736912
Kraaij D. A., Kamermans M., Spekreijse H. (1998). Spectral sensitivity of the feedback signal from horizontal cells to cones in goldfish retina. Visual Neurosci. 15 (5), 799–808. doi: 10.1017/s0952523898154184
Kusmic C., Gualtieri P. (2000). Morphology and spectral sensitivities of retinal and extraretinal photoreceptors in freshwater teleosts. Micron 31 (2), 183–200. doi: 10.1016/s0968-4328(99)00081-5
Mandario M. A. E., Castor N. J. T., Balinas V. T. (2021). Interaction effect of light intensity and photoperiod on egg hatchability, survival and growth of polychaete Marphysa iloiloensis from larva to juvenile. Aquaculture 531, 735890. doi: 10.1016/j.aquaculture.2020.735890
Marchand F., Magnan P., Boisclair D. (2002). Water temperature, light intensity and zooplankton density and the feeding activity of juvenile brook charr (Salvelinus fontinalis). Freshw. Biol. 47 (11), 2153–2162. doi: 10.1046/j.1365-2427.2002.00961.x
Martinez-Alvarez R. M., Hidalgo M. C., Domezain A., Morales A. E., Garcia-Gallego M., Sanz A. (2002). Physiological changes of sturgeon Acipenser naccarii caused by increasing environmental salinity. J. Exp. Biol. 205 (23), 3699–3706. doi: 10.1242/jeb.205.23.3699
Mizusawa K., Noble C., Suzuki K., Tabata M. (2007). Effect of light intensity on self-feeding of rainbow trout Oncorhynchus mykiss reared individually. Fish. Sci. 73 (5), 1001–1006. doi: 10.1111/j.1444-2906.2007.01429.x
Mommsen T. P. (2001). Paradigms of growth in fish. Comp. Biochem. Physiol. B-Biochem0 Mol. Biol. 129 (2-3), 207–219. doi: 10.1016/s1096-4959(01)00312-8
Mullur R., Liu Y. Y., Brent G. A. (2014). Thyroid hormone regulation of metabolism. Physiol. Rev. 94 (2), 355–382. doi: 10.1152/physrev.00030.2013
Nishida Y. (2011). The chemical process of oxidative stress by copper(II) and iron(III) ions in several neurodegenerative disorders. Monatshefte Fur Chemie 142 (4), 375–384. doi: 10.1007/s00706-010-0444-8
Nyska A., Kohen R. (2002). Oxidation of biological systems: Oxidative stress phenomena, antioxidants, redox reactions, and methods for their quantification. Toxicol. Pathol. 30 (6), 620–650. doi: 10.1080/01926230290166724
Perello-Amoros M., Garcia-Perez I., Sanchez-Moya A., Innamorati A., Velez E. J., Achaerandio I., et al. (2021). Diet and exercise modulate GH-IGFs axis, proteolytic markers and myogenic regulatory factors in juveniles of gilthead Sea bream (Sparus aurata). Animals 11 (8). doi: 10.3390/ani11082182
Perez-Sanchez J., Calduch-Giner J. A., Mingarro M., de Celis S. V. R., Gomez-Requeni P., Saera-Vila A., et al. (2002). Overview of fish growth hormone family. new insights in genomic organization and heterogeneity of growth hormone receptors. . Fish Physiol. Biochem 27 (3-4), 243–258. doi: 10.1023/B:FISH.0000032729.72746.c8
Perez-Sirkin D. I., Solovyev M., Delgadin T. H., Herdman J. E., Miranda L. A., Somoza G. M., et al. (2020). Digestive enzyme activities during pejerrey (Odontesthes bonariensis) ontogeny. Aquaculture 524, 735151. doi: 10.1016/j.aquaculture.2020.735151
Peter M. C. S. (2011). The role of thyroid hormones in stress response of fish. Gen. Comp. Endocrinol. 172 (2), 198–210. doi: 10.1016/j.ygcen.2011.02.023
Puvanendran V., Brown J. A. (2002). Foraging, growth and survival of Atlantic cod larvae reared in different light intensities and photoperiods. Aquaculture 214 (1-4), 131–151. doi: 10.1016/s0044-8486(02)00045-5
Ruchin A. B. (2021). Effect of illumination on fish and amphibian: development, growth, physiological and biochemical processes. Rev. Aquacul. 13 (1), 567–600. doi: 10.1111/raq.12487
Sanchez-Vazquez F. J., Lopez-Olmeda J. F., Vera L. M., Migaud H., Lopez-Patino M. A., Miguez J. M. (2019). Environmental cycles, melatonin, and circadian control of stress response in fish. Front. Endocrinol. 10. doi: 10.3389/fendo.2019.00279
Shin H. S., Lee J., Choi C. Y. (2011). Effects of LED light spectra on oxidative stress and the protective role of melatonin in relation to the daily rhythm of the yellowtail clownfish, Amphiprion clarkii. Comp. Biochem. Physiol. A Mol. Integr. Physiol. 160 (2), 221–228. doi: 10.1016/j.cbpa.2011.06.002
Song J. A., Choi C. Y. (2019). Effects of blue light spectra on retinal stress and damage in goldfish (Carassius auratus). Fish Physiol. Biochem. 45 (1), 391–400. doi: 10.1007/s10695-018-0571-4
Song C., Liu L., Lu P., Yang H., Li X., Gao X., et al. (2018). Design of artificial LED lighting in aquaculture workshop. J. Dalian Ocean Univ. 33 (2), 145–150. doi: 10.16535/j.cnki.dlhyxb.2018.02.002
Taylor J. F., North B. P., Porter M. J. R., Bromage N. R., Migaud H. (2006). Photoperiod can be used to enhance growth and improve feeding efficiency in farmed rainbow trout, Oncorhynchus mykiss. Aquaculture 256 (1-4), 216–234. doi: 10.1016/j.aquaculture.2006.02.027
Triantaphyllopoulos K. A., Cartas D., Miliou H. (2020). Factors influencing GH and IGF-I gene expression on growth in teleost fish: how can aquaculture industry benefit? Rev. Aquacul. 12 (3), 1637–1662. doi: 10.1111/raq.12402
Villamizar N., Blanco-Vives B., Migaud H., Davie A., Carboni S., Sánchez-Vázquez F. J. (2011). Effects of light during early larval development of some aquacultured teleosts: A review. Aquaculture 315 (1-2), 86–94. doi: 10.1016/j.aquaculture.2010.10.036
Villamizar N., García-Alcazar A., Sánchez-Vázquez F. J. (2009). Effect of light spectrum and photoperiod on the growth, development and survival of European sea bass (Dicentrarchus labrax) larvae. Aquaculture 292 (1-2), 80–86. doi: 10.1016/j.aquaculture.2009.03.045
Wang K., Li K., Liu L., Tanase C., Mols R., van der Meer M. (2020). Effects of light intensity and photoperiod on the growth and stress response of juvenile Nile tilapia (Oreochromis niloticus) in a recirculating aquaculture system. Aquacul. Fish 8 (1), 85–90. doi: 10.1016/j.aaf.2020.03.001
Wang T. T., Xu M. M., Wang J. T., Wan W. J., Guan D. Y., Han H. J., et al. (2020). A combination of rapeseed, cottonseed and peanut meal as a substitute of soybean meal in diets of yellow river carp Cyprinus carpio var. Aquacul. Nutr. 26 (5), 1520–1532. doi: 10.1111/anu.13099
Wei H., Li H.-D., Xia Y., Liu H.-K., Han D., Zhu X.-M., et al. (2019). Effects of light intensity on phototaxis, growth, antioxidant and stress of juvenile gibel carp (Carassius auratus gibelio). Aquaculture 501, 39–47. doi: 10.1016/j.aquaculture.2018.10.055
Wu L., Wang Y., Li J., Song Z., Xu S., Song C., et al. (2021). Influence of light spectra on the performance of juvenile turbot (Scophthalmus maximus). Aquaculture 533, 736191. doi: 10.1016/j.aquaculture.2020.736191
Yu Z., Li L., Zhu R., Li M., Duan J., Wang J. Y., et al. (2020a). Monitoring of growth, digestive enzyme activity, immune response and water quality parameters of golden crucian carp (Carassius auratus) in zero-water exchange tanks of biofloc systems. Aquacul. Rep. 16. doi: 10.1016/j.aqrep.2020.100283
Yu Z., Wu X. Q., Zheng L. J., Dai Z. Y., Wu L. F. (2020b). Effect of acute exposure to ammonia and BFT alterations on Rhynchocypris lagowski: Digestive enzyme, inflammation response, oxidative stress and immunological parameters. Environ. Toxicol. Pharmacol. 78. doi: 10.1016/j.etap.2020.103380
Keywords: daily rhythmic light spectra, light intensity, growth, physiological status, steelhead trout
Citation: Ma S, Li L, Chen X, Chen S, Dong Y, Gao Q, Zhou Y and Dong S (2023) Influence of daily rhythmic light spectra and intensity changes on the growth and physiological status of juvenile steelhead trout (Oncorhynchus mykiss). Front. Mar. Sci. 10:1116719. doi: 10.3389/fmars.2023.1116719
Received: 05 December 2022; Accepted: 04 January 2023;
Published: 19 January 2023.
Edited by:
Ce Shi, Ningbo University, ChinaReviewed by:
Qian Ma, Guangdong Ocean University, ChinaBaoliang Liu, Yellow Sea Fisheries Research Institute (CAFS), China
Copyright © 2023 Ma, Li, Chen, Chen, Dong, Gao, Zhou and Dong. This is an open-access article distributed under the terms of the Creative Commons Attribution License (CC BY). The use, distribution or reproduction in other forums is permitted, provided the original author(s) and the copyright owner(s) are credited and that the original publication in this journal is cited, in accordance with accepted academic practice. No use, distribution or reproduction is permitted which does not comply with these terms.
*Correspondence: Yangen Zhou, zhouyg@ouc.edu.cn; Shuanglin Dong, dongsl@ouc.edu.cn