- 1Dipartimento di Scienze della Terra, dell’Ambiente e della Vita, Università degli Studi di Genova, Genoa, Italy
- 2Consorzio Nazionale Interuniversitario per le Scienze del Mare (CoNISMa), Rome, Italy
- 3National Biodiversity Future Center (NBFC), Palermo, Italy
- 4Fondazione AzioneMare, Lugano, Switzerland
- 5Centro di Fisica Applicata, Datazione e Diagnostica, Dipartimento di Matematica e Fisica “Ennio de Giorgi”, Università del Salento, Lecce, Italy
Several bathyal cold-water coral provinces, characterized by a lush growth of habitat-forming scleractinians, have been recognized in the Mediterranean Sea. However, the search for this biogenic habitat only marginally targeted the Italian coast of the Ligurian Sea (NW Mediterranean basin) despite historical and a few recent local studies in the region reporting the presence of corals. This study used bathymetry maps, side-scan sonar profiles, historical charts, and trawling routes to identify sites that could potentially host coral habitats in the eastern sector of the Ligurian Sea. Remotely operated vehicle video footage from various projects (2015-2021, 20 dives) was then used to characterize four sub-areas (Genoa Plateau, Portofino, Deiva Marina, and Monterosso) where corals were detected between 450 m and 750 m depth. Radiocarbon dating was used to trace back the geological history of the coral structures. A small coral mound, impacted by trawling activities, was found on the Genoa Plateau, while four massive coral structures were found in the other sub-areas, mainly located in a morphologically complex and highly energetic canyon region. High levels of megafaunal biodiversity, including rarely reported alcyonaceans as Placogorgia coronata, were observed together with moderate fishing impact. Overall, the identified coral areas potentially account for 9 km2 of both subfossil mounds (as old as 13300 years BP), dominated by Desmophyllum pertusum, and living reefs, dominated by Madrepora oculata, the latter representing up to 23% of the substrate coverage. The few living colonies of D. pertusum in the area represent the first documented records for the Ligurian Sea. These data support the presence of a distinct eastern Ligurian cold-water coral province.
Introduction
Cold-water coral (CWC) reefs and mounds are massive biogenic seafloor structures built by the continuous deposition of a complex aragonitic skeletal framework produced by scleractinian species and by the deposition of fine hemipelagic sediments filling the open spaces between the frameworks (Freiwald et al., 2004; Roberts et al., 2006; Freiwald et al., 2009; Roberts et al., 2009; Wang et al., 2021). They form one of the most important habitats of the deep-sea realm and a climax biocoenosis of the bathyal zone worldwide, including the Mediterranean Sea (SPA/RAC–UN Environment/MAP, 2019). This habitat holds a crucial role in bathyal Mediterranean environments representing a pole of attraction for the deep-sea fauna (Rueda et al., 2019). The associated macro- and megafauna exploit the complex framework of scleractinian branches for refuge, feeding or reproduction. They may as well live in the nearby coral rubble and soft bottom or in the tridimensional canopy of habitat-forming anthozoans and sponges settling on the dead coral framework and rubble (Rueda et al., 2019). The occurrence of this habitat also positively influences deep-sea biogeochemical cycles and pelagic-benthic coupling processes (van Oevelen et al., 2009; Cathalot et al., 2015; Rossi et al., 2017) and supports highly profitable fishing grounds for professional and recreational fishers (D’Onghia et al., 2017). CWCs are the engineers of one of the best studied vulnerable marine ecosystems (VMEs) in the deep sea because of their high sensitivity to mechanical impacts and slow recovery ability (e.g., slow growth rates, settlement limited by increased silting). These characteristics support the need for high protection levels, only marginally put in place in the Mediterranean basin (Otero and Marin, 2019).
Mediterranean bathyal habitat-forming scleractinians are generally considered a relic of the cold fauna of the Quaternary period (Plio-Pleistocene), and the majority of the sites host coral rubble or three-dimensional coral frameworks (Vertino et al., 2014; Taviani et al., 2019a). Single living colonies and dead and living frameworks of Madrepora oculata Linnaeus, 1758 and Desmophyllum pertusum (Linnaeus, 1758) (= Lophelia pertusa) are generally found on hard substrata with variable slope and seafloor heterogeneity conditions (Orejas et al., 2009; Fabri et al., 2014; Chimienti et al., 2019; Corbera et al., 2019; Fabri et al., 2019). Their growth is sustained by energetic hydrodynamics related to the flow of the cold and oxygen-enriched Levantine Intermediate Water (LIW) (Robinson et al., 2001; Millot and Taupier-Letage, 2005; Taviani et al., 2017; Chimienti et al., 2019; Vertino et al., 2019; Wienberg et al., 2022). Structuring colonial species such as D. pertusum and M. oculata have the capability to form reefs that alter as topographic seafloor structures the local hydrodynamic and sedimentary regimes and whose growth is balanced by bioerosion (Roberts et al., 2009). Successive periods of reef development supplemented by the deposition of fine sediments and bioerosion phenomena eventually result in the formation of coral mounds, which are covered by living reefs or by dead coral frameworks and coral rubble interspersed in mud (Roberts et al., 2006). Eight major Mediterranean provinces with flourishing scleractinian CWCs (sensu Taviani et al., 2011) have been identified in the last 20 years in the Alboran Sea, Gulf of Lion, Tyrrhenian Sea, Sicily Channel, Ionian and Adriatic seas (Chimienti et al., 2019; Angeletti et al., 2020). In the NW Mediterranean Sea, three provinces have been so far recognised: two located within the Gulf of Lion canyon system and nearby Spanish canyons (Orejas et al., 2009; Fabri et al., 2014; Fabri et al., 2017; Fabri et al., 2019; Lastras et al., 2019; Fabri et al., 2022), and the newly discovered one in the Corsica Channel (Angeletti et al., 2020; Angiolillo et al., 2023). Instead, our knowledge on the presence of living reefs has always been fragmentary along the continental slope of the Ligurian Sea, located between the Gulf of Lion and the Corsica Channel. In this area, historical information on the occurrence of scleractinian CWCs comes from fishing bycatch (Brunelli and Bini, 1934; Rossi, 1958; Relini-Orsi, 1974; Morri et al., 1986) and sedimentologic deep surveys (Blanc, 1959; Blanc et al., 1959). These data were included in a series of nautical charts (Fusco, 1967, 1968, 1972) reporting twelve historical coral areas (here referred to as Fusco coral areas, F1-F12), each approximately 4 km2. These were found along the entire Ligurian arc between 500 m and 700 m depth, with ten distributed in the eastern sector (F3-F12), from Genoa to Monterosso (Fusco, 1967, 1968, 1972) (Figure 1A). In recent times, bathyscaphe and remotely operated vehicle (ROV) surveys (Tunesi and Diviacco, 1997; Tunesi et al., 2001; Delbono et al., 2014; Fanelli et al., 2017; Enrichetti et al., 2018) reported findings of dead coral frameworks and living colonies, including a living reef of M. oculata off Monterosso (Figure 1A).
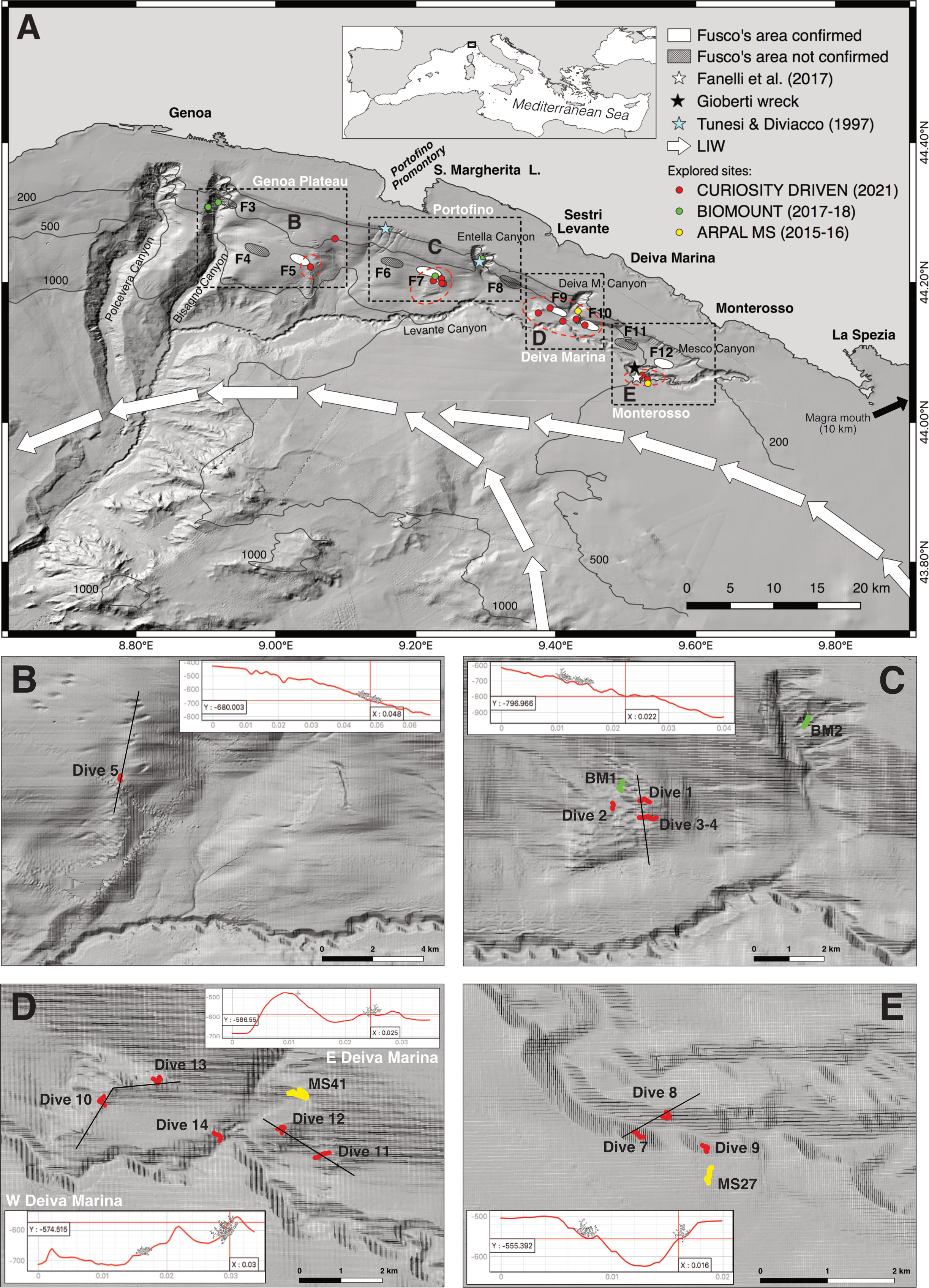
Figure 1 Bathymetric-morphological map (A) of the eastern shelf and slope of the Ligurian Sea (NW Mediterranean Sea), incised by numerous canyons. Bold white arrows mark the flow path of the Levantine Intermediate Water (LIW). Historical reports of areas with scleractinian cold-water coral occurrences based on Fusco charts (1967, 1968, 1972) are indicated by filled ovals and labelled as F3-F12 (areas confirmed as coral sites are marked in white). Recently obtained coral findings are also shown (marked by stars; see legend for references). Dots indicate the sites considered in this study (see code of the projects). The dashed black boxes delimit the four target sub-areas, while the labels (B-E) indicate the coral areas (delimited by dashed red ovals within each sub-area), whose enlargement is provided in the maps below. These maps report the position of the ROV tracks carried out in each coral area and the terrain profiles in the sites with coral occurrences (insets). All maps are based on Atlas MaGIC bathymetry, Chiocci et al., 2021 (datum and coordinate system WGS84).
Despite its undisclosed deep-sea biocoenotic potential (Enrichetti et al., 2018) and the high fishing pressure (Relini, 2007), nobody has ever attempted to investigate and assess the environmental status of the entire bathyal coral belt identified by Fusco. The large-scale mapping and characterization of the extent, structural complexity, ecological role and vulnerability of CWC habitats are nowadays considered fundamental studies. This is especially in light of the need to identify those marine ecosystems that, because of their high ecological value and the numerous ecosystem services they provide, have a high priority for conservation (Lo Iacono et al., 2019; Montefalcone et al., 2021). This approach is not only supported by the sustainable development goals of the UN 2030 Agenda regarding the conservation of the seas and their resources (Ryabinin et al., 2019; Koulouri et al., 2022) but is an integral part of the new EU Biodiversity Strategy for 2030 aiming at strengthening the protection of marine ecosystems and achieving or maintaining their “good environmental status” (Miu et al., 2020; Hermoso et al., 2022). This strategy stresses the need for an ecosystem-based approach to managing human activities at sea. It also aims to expand protected areas, establish strictly protected areas for habitats and fish stocks recovery, and tackle practices that disturb the seabed.
This study aims to present a novel large-scale characterization of scleractinian CWC occurrences in the eastern Ligurian Sea, integrating historical information and modern technological approaches to support the definition of a distinct Ligurian CWC province. The main goals are to i) report the current geographical and bathymetrical extent of the CWC areas, ii) quantify the structural complexity and attractiveness of the biogenic habitat through a characterization of the coral structures and the associated biodiversity with a particular focus on habitat-forming species, iii) quantify the anthropogenic impacts and assess the vulnerability levels to depict potential conservation areas and iv) trace the occurrence of the CWCs in the region over the past millennia through radiocarbon dating.
Materials and methods
Study area
The study area encompasses about 60 km of continental slope (from 350 m to 800 m depth) localized in the eastern part of the Ligurian Sea (Figure 1A). With respect to the western sector of the Ligurian Sea, the eastern one is characterized by a wider continental shelf breaking at about 120 m depth and a less pronounced slope, incised by three major canyons (Migeon et al., 2011; Soulet et al., 2016; Enrichetti et al., 2020). Two of these topographic structures (Polcevera Canyon and Bisagno Canyon) run perpendicularly to the coast in front of the city of Genoa, and one (Levante Canyon) runs EW almost parallel to the coast for the entire length of the region before converging into the Genoa canyon system (Cattaneo-Vietti et al., 2010; Würtz, 2012) (Figure 1A). Recent studies highlighted the role of this latter system in driving the prokaryotic and meiofaunal diversity, with both canyon structures being depocenters of primary organic matter (Carugati et al., 2019; Corinaldesi et al., 2019). The sinuous Levante Canyon receives the inputs of three other minor tributaries, running perpendicularly to the coast, namely the Entella Canyon, Deiva Marina Canyon and Punta Mesco Canyon (Figure 1A). These canyons do not have a clear connection to any continental river system, a widespread characteristic among Ligurian canyons (Harris and Whiteway, 2011). The Levante Canyon has steep slopes (Delbono et al., 2014; Fanelli et al., 2017), and it funnels a large amount of sediments partly deriving from the Magra River, the largest Ligurian river flowing in the basin about 10 km east of the city of La Spezia (Bassano et al., 2000) (Figure 1A). The study area is lashed by the LIW, which enters the Ligurian basin from the Tyrrhenian Sea through the Tuscan Archipelago and along the western coast of Corse, flowing westward along-slope between 300 and 700 m depth (Astraldi et al., 1994; Cattaneo-Vietti et al., 2010; Prieur et al., 2020) (Figure 1A). Above the LIW flow surface waters (MAW, Modified Atlantic Water) from 0 m to 100 m depth and the Winter Cold Water (WCW) from 100 m to 300 m, while Western Mediterranean Deep Water (WMDW) occupies the water column from 700 m depth down to the seafloor (Marty and Chiavérini, 2010; Prieur et al., 2020).
From the geographical point of view, the ten historical coral areas identified by Fusco (Fusco, 1967, 1968, 1972) in this region can be divided into four main sub-areas (Figure 1A), each with different environmental settings and different fishing pressures. (1) The first sub-area (Genoa Plateau) includes three Fusco areas: F3, located at around 500 m depth on the eastern flank of the Bisagno Canyon, and F4 and F5 located at around 700 m depth on the wide low-gradient bathyal slope extending between the city of Genoa and the Portofino Promontory. Among all Fusco areas, F4 and F5 are the furthest from both the continental shelf and the path of the Levante Canyon (Figure 1A). The relatively limited heterogeneity of the Genoa Plateau makes this an important epi- and mesobathyal trawling area for many species of commercial interest (Orsi Relini and Relini, 1998; Tudela et al., 2003).
(2) The second sub-area (Portofino) includes F6 and F7, located approximately 5 km off the Portofino Promontory between 600 m and 700 m depth, and F8, situated off the city of Sestri Levante in the proximities of the Entella Canyon at about 500 m depth (Figure 1A). These areas are located at 5-7 km from the path of the Levante Canyon. The fishing ground “Di Terra le Rame” runs parallel to the coast for about 25 km between Sestri Levante and the Portofino Promontory (Relini, 2007) and is one of the most famous red shrimp fishing areas of the Ligurian Sea with up to 12 trawling vessels from the town of Santa Margherita Ligure carrying out up to 60-120 hauls per year (Enrichetti et al., 2018). The trawling paths generally cross F6 and F7 along their northern border at about 500-650 m depth (Enrichetti et al., 2018).
(3) The third sub-area (Deiva Marina) includes F9 and F10, located W and E, respectively, of the southern margin of the Deiva Marina tributary (Figure 1A). These Fusco areas are located close to the Levante Canyon at the base of the continental slope, at around 600 m depth. F9 is marginally crossed by trawlers running the “Di Terra le Rame” fishing path towards Portofino (Enrichetti et al., 2018). Finally, (4) the fourth sub-area (Monterosso) includes F11 and F12, located in the easternmost sector, off the town of Monterosso and close to both the main path of the Levante Canyon and the Mesco tributary (Figure 1A). Previous investigations confirmed the occurrence of scattered coral rubble in F12 while reported living coral frameworks in its southern proximities, within the canyon (Fanelli et al., 2017). At least 19 trawling vessels from Santa Margherita Ligure and La Spezia are known to work at both epi- and mesobathyal depths in the easternmost area (Relini, 2007; Fanelli et al., 2017), with local commercial longliners frequenting the Levante Canyon (Fanelli et al., 2017), although no quantitative fishing effort data are available for this region.
Identification and investigation of the coral areas
Various approaches were employed to detect the sites that could potentially host the coral structures within the four sub-areas. First, multibeam GIS shapefiles based on the Atlas MaGIC bathymetry (Chiocci et al., 2021) and provided by the Marine Geology Laboratory (Università degli Studi di Genova) were studied to search for areas of seafloor heterogeneity within or in the proximities of target Fusco coral areas. Details on the acquisition and processing of the multibeam data are discussed in Romagnoli et al. (2013) and Bosman et al. (2015). A side-scan sonar (SSS, Gaymarine) mounted on the hull of the catamaran Daedalus (Fondazione AzioneMare), including a Simrad EA400 echo sounder and operating at 50 kHz frequency, was then run using a 2°×60° fan-shaped beam width for a useful bathymetric range of 1500 m to obtain two-dimensional seafloor profiles of the Fusco areas and their proximities (about 50 km2) with particular focus where the topographic reliefs were identified (Figure SM1). This allowed for the identification of the optimal sites where to deploy the ROV. The criteria used in this process included the height and dimension of the elevated seabed structures, slope angle, strength of the backscatter signal, and water depth. In addition, to identify areas avoided by fishing activities (such as outcropping rocks and coral structures), the trawling routes of the entire region were obtained from a free AIS (Automatic Identification System) vessel tracking online platform (https://www.vesselfinder.com/it). Routes were monitored daily from January 2020 to February 2021. Finally, previous literature data (e.g., Rossi, 1958; Tunesi and Diviacco, 1997; Fanelli et al., 2017; Enrichetti et al., 2018) and novel indications from fishers were taken into consideration. In particular, fishers were informally interviewed during the project to investigate if they knew the position of coral areas (based on entanglements and collection of coral bycatches) and reported coral occurrences near F9 and F10.
ROV footage acquisition
Twenty ROV dives were carried out in the region, of which 14 were made in the summer and fall of 2021 for the Curiosity Project to explore the sites where reliefs have been detected (Table 1 and Figure 1A). Six additional ROV dives, obtained from previous expeditions in the area, were also considered. They were carried out for the Biomount Project (four dives made in 2017 and 2018) and the Marine Strategy ARPAL Project (two dives made in 2015 and 2016) (Table 1 and Figure 1A). Dives were carried out from 350 m to about 800 m depth accounting for about 30 hours of video footage and 8.2 km of explored seafloor (about 16400 m2) (Table 1).
Curiosity and Biomount ROV surveys were carried out with the ROV Multipluto (GayMarine). The ROV, mainly travelling approximately 2 m above the seafloor at less than 0.5 m sec-1, was equipped with two strobe lights, a high‐definition video camera (Sony RX100 II), a depth sensor, a compass, and an acoustic tracking position system (USBL HDR Gaymarine). The video camera, with an integral 20-megapixel still camera, was positioned in a multisensory head capable of 360° pan, +90°-80° tilt and articulated neck elevation (50 cm). ARPAL ROV surveys were carried out with the ROV Pollux III equipped with a digital camera (Nikon D80), a strobe (Nikon SB 400), a HD video camera (Sony HDR-HC7), and underwater lights. The ROV also had a depth sensor, a compass and the underwater acoustic positioning system (Ultra-Short Baseline, USBL) LinkQuest TrackLink 1500 MA. Both ROVs were equipped with a three‐jaw grabber mounted on a manipulative arm dedicated to collecting specimens for taxonomical analyses and scleractinian corals for radiocarbon dating. Parallel laser beams (distanced 10 cm) provided a scale for dimensional reference. When unavailable, average biometric references of the target taxa were used to obtain demographic data.
Data processing
To evaluate the general environmental characteristics of the investigated sites and the complexity of the coral structures in each sub-area, 40 frames were extrapolated from each video using the free software VLC for a total of 800 frames. Unusable frames, those that were replicated, out-of-focus, or too far off the bottom, were removed from the dataset and replaced. The environment of each site was characterized in terms of the percentage composition of mud, rock, coral rubble and scleractinian framework. When a coral structure was detected, a percentage coverage of the dead and living portions in each frame was made to evaluate the status of the biogenic habitat. Coverages were measured by analyzing the frames with the software ImageJ (version 1.53a). Data were presented as an average value for each one of the reported five coral areas, identified considering only those dives carried out on coral structures (13 out of 20) and keeping W and E Deiva Marina separated. In addition, an estimation of the maximum height of the coral structures (considering the exposed dead and living portions together) was given for each coral area. Whenever possible, the dominant scleractinian species in the analyzed frames was identified for each coral area. Finally, to better evaluate the topography of the coral areas, terrain profiles were plotted using the Atlas MaGIC bathymetry (Chiocci et al., 2021) and the profile tool plugin available on QGIS software (version 3.22).
All megabenthic and demersal taxa visible in the entire ROV footage, both on hard and soft bottoms, were identified to the lowest possible taxonomic level. A list was provided for the four sub-areas indicating the relative abundance (four categories, from zero to three) of each taxon. An average species richness value was also given for each coral area, considering only those dives carried out on coral structures and keeping W and E Deiva Marina separated.
The occurrence and density (expressed as the average number of individuals or colonies m-2 ± Standard Error, SE) of non-scleractinian habitat-forming species (sponges, antipatharians and alcyonaceans) settled on dead coral frameworks and rubble were assessed by counting all visible and well-aligned specimens for each species in up to 50 frames targeting the coral structures per each dive. The species taken into consideration were: the sponge Pachastrella monilifera Schmidt, 1868, the antipatharians Parantipathes larix (Esper, 1788) and Parantipathes sp., and the alcyonaceans Acanthogorgia armata Verrill, 1878, Muriceides lepida Carpine and Grasshoff, 1975, Placogorgia coronata Carpine and Grasshoff, 1975, and Placogorgia massiliensis Carpine and Grasshoff, 1975. The taxonomic identification of these species was carried out in the Zoology Laboratory of the University of Genoa based on the collected material. An average value of density was given for each species in each site. Density data were also presented for the main structuring species, the scleractinians M. oculata and D. pertusum, considering only living colonies. In addition, height-percentage frequency distributions (8 size classes, from 5 to 70 cm) were made for M. oculata in the coral areas showing the highest densities of living colonies. Height data were provided for the living colonies of D. pertusum. Morphometric data were obtained with the software ImageJ.
The distribution and density (expressed as the number of items ha-1) of urban and fishing litter were assessed for each site considering the entire video footage and length of the ROV track to evaluate the extent and origin of the anthropogenic impact in the different sub-areas. In addition, an interpolation of AIS trawling vessel routes with the bathymetric-morphological map was used to detect areas of no fishing effort and the occurrence and average density of a soft-bottom fragile anthozoan belonging to an unidentified species of the genus Acanella (Keratoisididae) were used as a bioindicator of pristine environments.
All maps presented in this study were built with QGIS software (version 3.22) using Atlas MaGIC bathymetry (Chiocci et al., 2021).
Radiocarbon dating
Eight specimens of dead scleractinian corals belonging to three species [M. oculata, D. pertusum, and Desmophyllum dianthus (Esper, 1794)] were used for radiocarbon dating (Table 2 and Figure SM1). Six were selected from the four study sub-areas (Genoa Plateau, Portofino, Deiva Marina, and Monterosso). Of these, four samples were collected during the Curiosity surveys, one during the Biomount project and one was a bycatch sample collected during a fishing survey conducted in the 1980s (Table 2 and Figure SM2). Two additional samples were collected during the Biomount project on two offshore seamounts of the Ligurian Sea, namely Penelope Seamount (497 m depth) and Spinola Seamounts (1810 m depth). These latter were added since they provided further information on the coral ages of the region in the bathyal offshore zones.
Analyses were carried out by AMS (Accelerator Mass Spectrometry) at CEDAD (Centre for Dating and Diagnostics), University of Salento, Italy (Calcagnile et al., 2005; Calcagnile et al., 2019). Samples were submitted to standard processing procedures (Calcagnile et al., 2004; Hajdas et al., 2021; Quarta et al., 2021). The measurement of 14C/12C and 13C/12C isotopic ratios was used to calculate conventional radiocarbon ages, according to Stuiver and Polach (1977). Conventional radiocarbon ages were then calibrated to calendar years using the Marine20 calibration curve valid for marine data (Heaton et al., 2021) and the OxCal Vers. 4.4 Software (Ramsey, 2001). A local reservoir correction ΔR = -90 ± 80 years was used as the average value accounting for a deglacial to Holocene marine reservoir age of R = 370 ± 40 years (Siani et al., 2000; Reimer and McCormac, 2002; Siani et al., 2011).
Results
Localization, environmental characteristics and structural complexity of the coral areas
The preliminary investigations conducted with indirect tools (SSS surveys, multibeam bathymetry maps, bycatch records, and AIS trawling routes) confirmed the occurrence of reliefs in all four investigated sub-areas and specifically in five of the ten target Fusco coral areas or their proximities (Figure 1A). ROV investigations then reported scleractinian CWC occurrences to a different extent in the four sub-areas and five major coral areas were identified (Figures 1B–E).
(1) Multibeam bathymetry and SSS surveys identified no conspicuous topographic relief in the Genoa Plateau sub-area, except for a 20 m-large circular structure localized SE of F5 at 690 m depth (Figures 1A, SM1A). Here, the ROV exploration (Dive 5) confirmed the occurrence of a heavily fragmented rubble of D. pertusum (Figure 2A), extending on a sub-horizontal (about 10°) muddy bottom (Figures 1B, 2A). The exposed coral rubble represented 41% of the investigated seafloor (Figure 3A), reaching up to 15 cm in thickness (Figure 3B). Neither living nor dead scleractinian CWC occurrences were detected in the dives conducted in the Bisagno Canyon (BM41 and BM42) and NE of F5 (Dive 6) (Figure 1A).
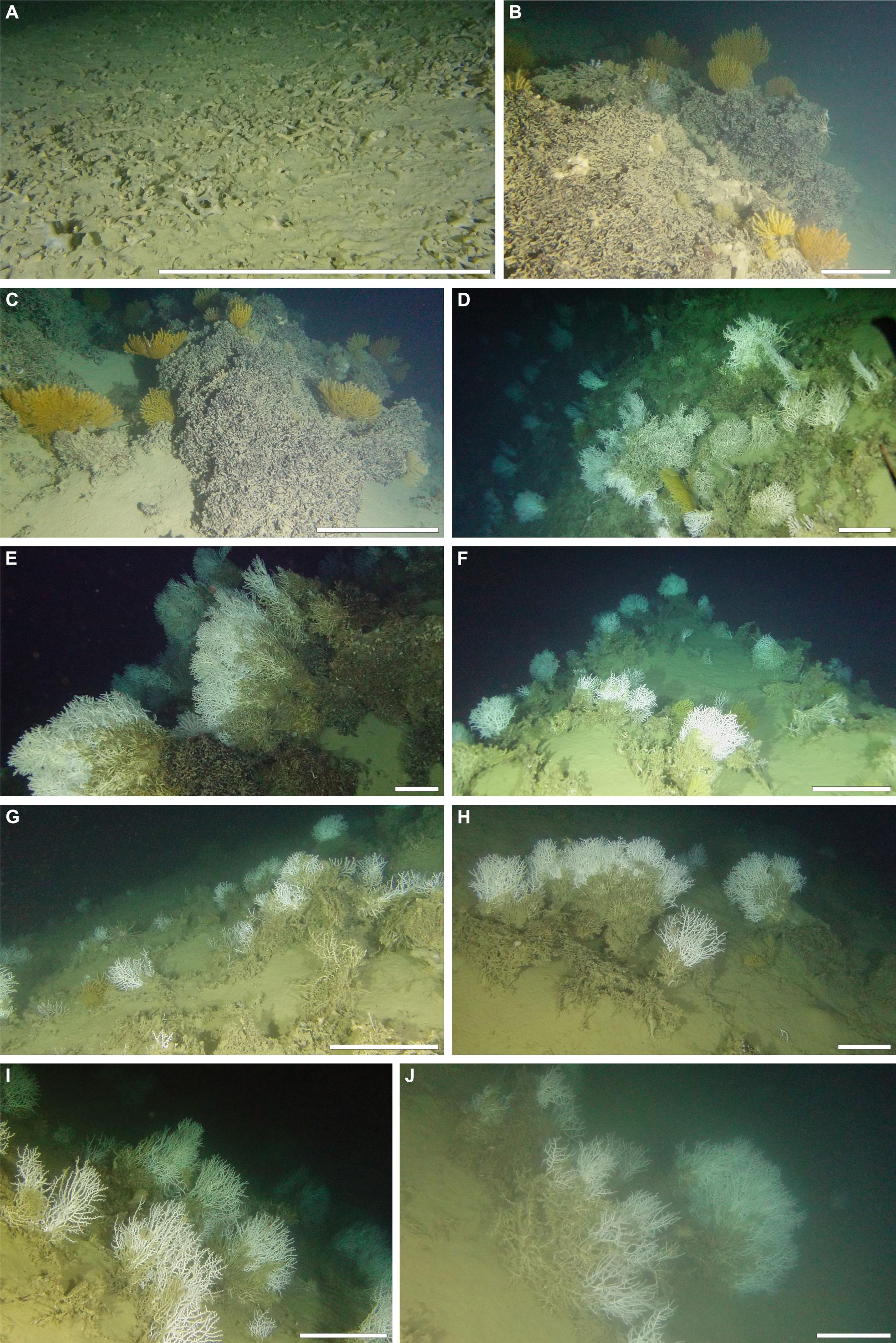
Figure 2 Representative ROV footage of the scleractinian frameworks for each coral area. (A) Accumulation of Desmophyllum pertusum fragments on mud (Genoa Plateau, Dive5), (B, C) dead framework dominated by D. pertusum and colonized by the alcyonacean Placogorgia coronata (Portofino) (Dive4), (D, E) living M. oculata frameworks developing on the sloping walls and (F) the sub-horizontal crest of the W Deiva Marina relief (Dive13), (G, H) living frameworks of M. oculata interspersed with mud on the E Deiva Marina relief (Dive11), (I, J) living frameworks of M. oculata developing on the silted terraces of the Levante Canyon (Monterosso, Dive8 and Dive7, respectively). Scale bar: 50 cm.
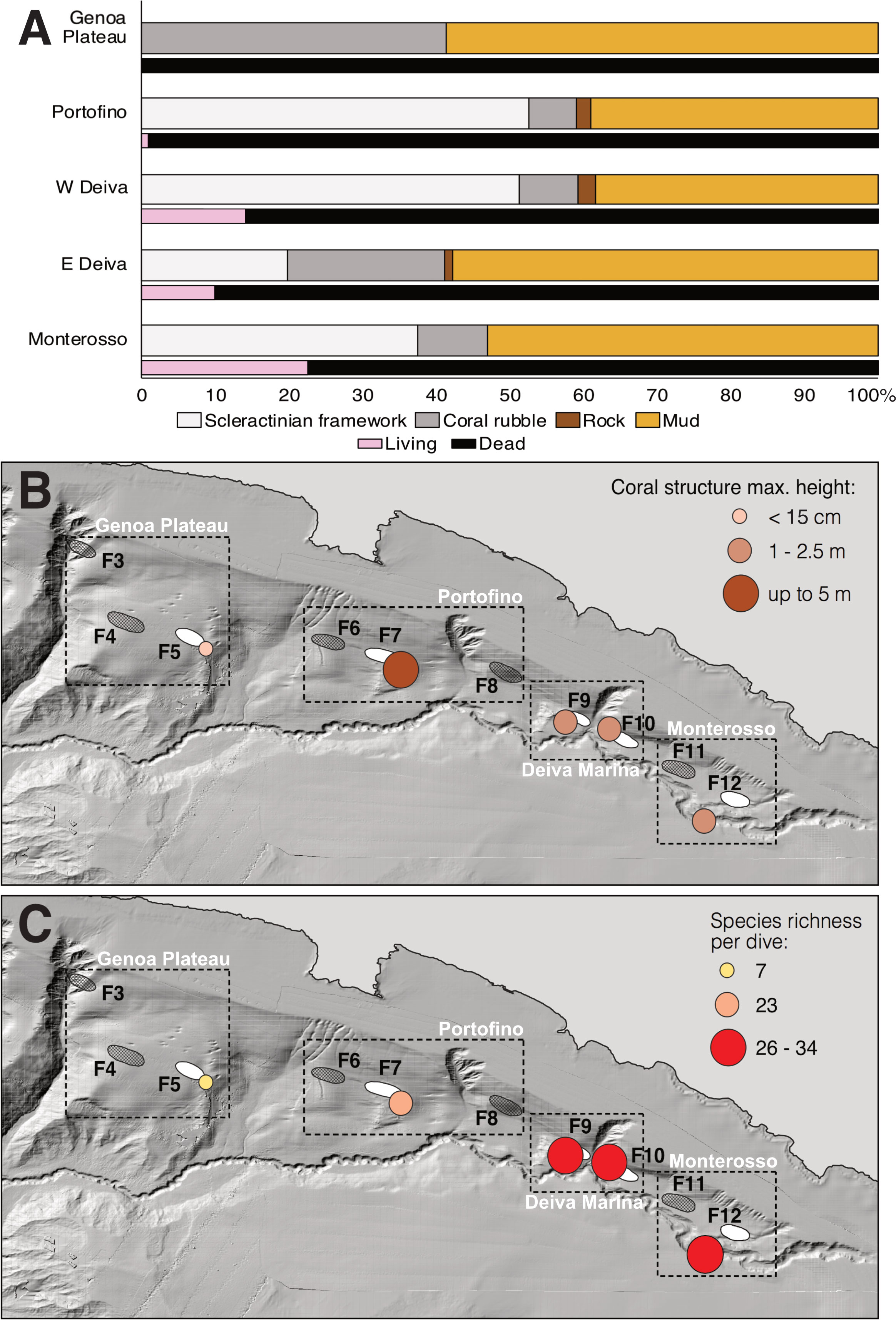
Figure 3 Average percentage coverage of substrate categories and living and dead portions of the observed scleractinian frameworks in each coral area of the eastern Ligurian Sea (A). Spatial distribution of the maximum heights of the coral structures and (B) of the average species richness (n° of species) per dive on each coral area (C) (maps based on Atlas MaGIC bathymetry, Chiocci et al., 2021).
(2) SSS profiles in the Portofino sub-area identified a massive relief 5 km SE of the eastern cape of the Portofino Promontory, crossing F7 on its SE side (Figures 1A, SM1B). Based on the bathymetric-morphological map, the relief stretches for about 4 x 2 km from 620 m to 960 m depth (Figure 1C). It was explored on both the western (BM1 and Dive 2) and eastern sides (Dives 1, 3, and 4). The terrain profile along the E margin is overall sloping (around 30°) (Figure 1C), although steeper areas are present on a smaller scale. The relief was covered for about 53% of its investigated area by scleractinian frameworks, located between 642 m and 779 m depth (Figure 3A). Outcropping rock and rubble, the latter scattered in the mud among the corals and at the base of the topographic relief, contributed with a minor percentage (Figure 3A). The exposed coral structure, up to 5 m high (Figure 3B), was covered for 99% by dead D. pertusum (Figures 2B, C, 3A), with few living colonies of this species found between 642 m and 747 m depth. Neither living nor dead scleractinian CWC occurrences were detected in the Entella Canyon (BM2) (Figures 1A, C).
(3) Multibeam bathymetry data and SSS profiles in the sub-area of Deiva Marina revealed the occurrence of two large reliefs, each about 2 km long, in the proximities of both Fusco coral areas (F9 and F10) bordering the southern margins of the Deiva Marina Canyon (Figures 1A, SM1C–D). The western relief, situated at about 1-2 km from the Levante Canyon and about 2 km from the Deiva Marina Canyon in the proximities of F9, is a 2.5 m-high elevation (Figure 3B) emerging from a gently sloping bathyal plain at about 715 m depth and rapidly reaching the crest at about 570 m depth with an average slope of 45° (Dives 10 and 13) (Figure 1D). The scleractinian frameworks mainly occurred along the flanks of the relief in large incisures and along the sub-horizontal crest (Figures 2D–F) covering up to 51% of the investigated seafloor (Figure 3A). The coral structure was covered by living M. oculata colonies (14%) (between 570 m and 610 m depth) growing on massive dead M. oculata frameworks (Figure 3A). Large fan-like living and dead scleractinian frameworks were reported on the sloping substrate (Figure 2E), with colonies oriented eastward. Additionally, dead coral structures of M. oculata were observed at 730 m depth, where the Deiva Marina Canyon converges in the Levante Canyon (Dive 14) (Figure 1D). The eastern relief, situated along the continental slope closer to both canyons (in the proximities of F10), emerges from a bathyal plain at about 640 m depth and gently reaching the crest at about 445 m depth (Dive 11 and 12) (Figure 1D). The coral structure, as high as 1 m (Figure 3B), was covered by living M. oculata colonies (10%) growing (between 525 m and 640 m depth) on poorly developed dead frameworks of M. oculata (Figures 2G, H). These latter covered about 20% of the investigated seafloor (Figure 3A). Coral rubble was mainly found at the base of the reliefs in both W and E Deiva Marina coral areas, accounting for 8% and 21% of the investigated area, respectively (Figure 3A). The northern site (MS41), at 350-504 m depth, was dominated by sub-vertical rocky walls with neither living nor dead scleractinian CWC presence (Figure 1D).
(4) Lastly, multibeam bathymetry maps and SSS profiles confirmed the absence of reliefs in F11 and F12 in the sub-area of Monterosso. However, south of F12, in the Levante Canyon, two distinct coral structures (300 m and 500 m long) were detected between 502 m and 577 m depth beside the one already reported by Fanelli et al. (2017) (Dive 7) (Figures 1A, SM1E). One is located on the southern canyon wall (Dive 9), about 1 km east of the known one, and the other is located on the northern wall of the canyon (Dive 8) in front of the known one (Figure 1E). The coral frameworks were situated on silted, gently sloping terraces (about 30°) found along the steep walls of the canyon (Figure 1E). The coral structures, up to 1 m high, were covered by living M. oculata colonies (Figures 2I, J) growing on poorly developed dead frameworks of M. oculata covering up to 37% of the explored seafloor (Figures 3A, B). Overall, the living component was more significant than in any other coral area (23%) (Figure 3A). Colonies were eastward oriented also in these coral structures. No scleractinian CWC occurrences were detected on the muddy plain outside the canyon (MS27) (Figure 1E).
Associated biodiversity and habitat-forming species
One hundred four megabenthic and demersal taxa were identified in the entire region, largely attributed to the species level (Table SM1). Additionally, dead patches of Neopycnodonte zibrowii (Gofas, Salas and Taviani, 2009) were reported on outcropping sub-vertical rocks in the sub-areas of Portofino and Deiva Marina. Of the living taxa, cnidarian anthozoans corresponded to about 18% of the total diversity, crustaceans to 17%, and fishes to about 25%. The total species richness reported in the four sub-areas varied from 27 taxa in the Genoa Plateau to 72 in Deiva Marina (Table SM1). Considering only the dives carried out on coral structures, the lowest average value was found in the coral rubble of the Genoa Plateau (7 taxa per dive), followed by the coral structures of Portofino (23 taxa per dive), W Deiva Marina and Monterosso (26 and 27 taxa per dive, respectively), and E Deiva Marina showing the highest value (34 taxa per dive) (Figure 3C).
Nine hard-bottom habitat-forming species were recorded in the region, except for the Genoa Plateau coral area (Table SM1), for about 5600 counted specimens. Desmophyllum pertusum and M. oculata represented the dominant habitat formers of the studied coral areas. Living colonies of D. pertusum were found only in the deepest parts of the Portofino coral area with a maximal average density of 2 ± 0.5 colonies m-2 (Figure 4A), while living colonies of M. oculata were mainly found in the easternmost coral structures with maximal average densities of 13 ± 2 colonies m-2 (W Deiva Marina) and 8 ± 1 colonies m-2 (Monterosso) (Figure 4B). Besides these two species, small patches of living D. dianthus were recorded in all major coral areas. Habitat-forming alcyonaceans were abundantly represented on the dead coral frameworks or the nearby coral rubble. Acanthogorgia armata reached maximal average abundances of 7 ± 1 colonies m-2 on the coral rubble of the Portofino relief (Figure 4C). Muriceides lepida formed dense meadows on the Portofino dead coral framework (8 ± 3 colonies m-2) and the crest of the W Deiva Marina coral structure (20 ± 4 colonies m-2) (Figure 4D). The fan-like gorgonian P. coronata formed dense patches on the Portofino dead coral frameworks (5 ± 1 colonies m-2) and on the coral rubble of W Deiva Marina (2 ± 0.5 colonies m-2) (Figure 4E). The bushy colonies of P. massiliensis were mainly encountered on the Portofino and E Deiva Marina coral rubble (10 ± 2 colonies m-2 and 7 ± 2 colonies m-2, respectively) (Figure 4F). Habitat-forming species also included three other taxa, which were, however, overall poorly represented, namely the massive demosponge P. monilifera, reaching maximal average abundances of 2 ± 0.5 individuals m-2 in E Deiva Marina and the antipatharians P. larix and Parantipathes sp., reaching maximal average abundances of 2 ± 1 colonies m-2 and 2 ± 0.5 colonies m-2, in E Deiva Marina and Portofino, respectively. Also these species were mainly settled on the dead coral frameworks or the nearby coral rubble.
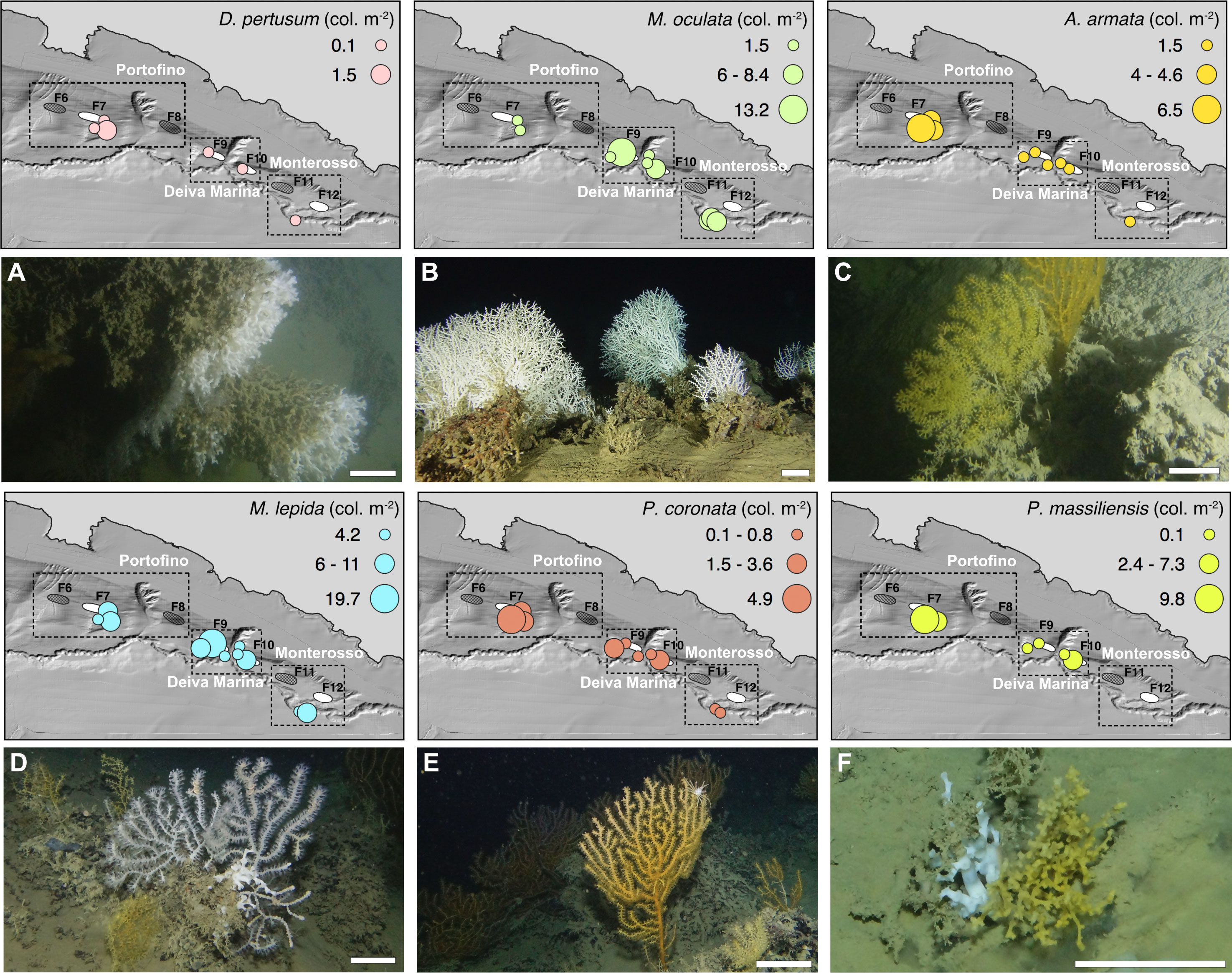
Figure 4 Distribution and average density (expressed as the number of living colonies m-2) of the main framework-building species and other habitat-formers in each dive. ROV footage of the considered species: (A) Desmophyllum pertusum (Portofino, Dive1), (B) Madrepora oculata (W Deiva Marina, Dive13), (C) Acanthogorgia armata (Portofino, Dive1), (D) Muriceides lepida (Portofino, Dive3), (E) Placogorgia coronata (Portofino, Dive4), (F) Placogorgia massiliensis (Deiva Marina, Dive11). Scale bar: 10 cm.
The percentage frequency distributions of the heights of the dominant habitat-forming scleractinian, M. oculata, showed that the modal class was around 20 cm in all three considered coral areas, namely W Deiva Marina (367 measured colonies, Dive 13), E Deiva Marina (320 measured colonies, Dive 11), and Monterosso (706 measured colonies, Dives 7, 8, 9). Despite this, both populations of Deiva Marina showed a lower percentage in the peak (about 38%) with respect to Monterosso (about 52%) (Figure 5). In addition, the structure of the E Deiva Marina population was marginally shifted towards the smaller size classes, while that of W Deiva Marina showed a slightly higher percentage of colonies above 50 cm high (Figure 5). The living colonies of D. pertusum reported in Portofino showed an average height of 10 ± 1.3 cm (up to 22 cm).
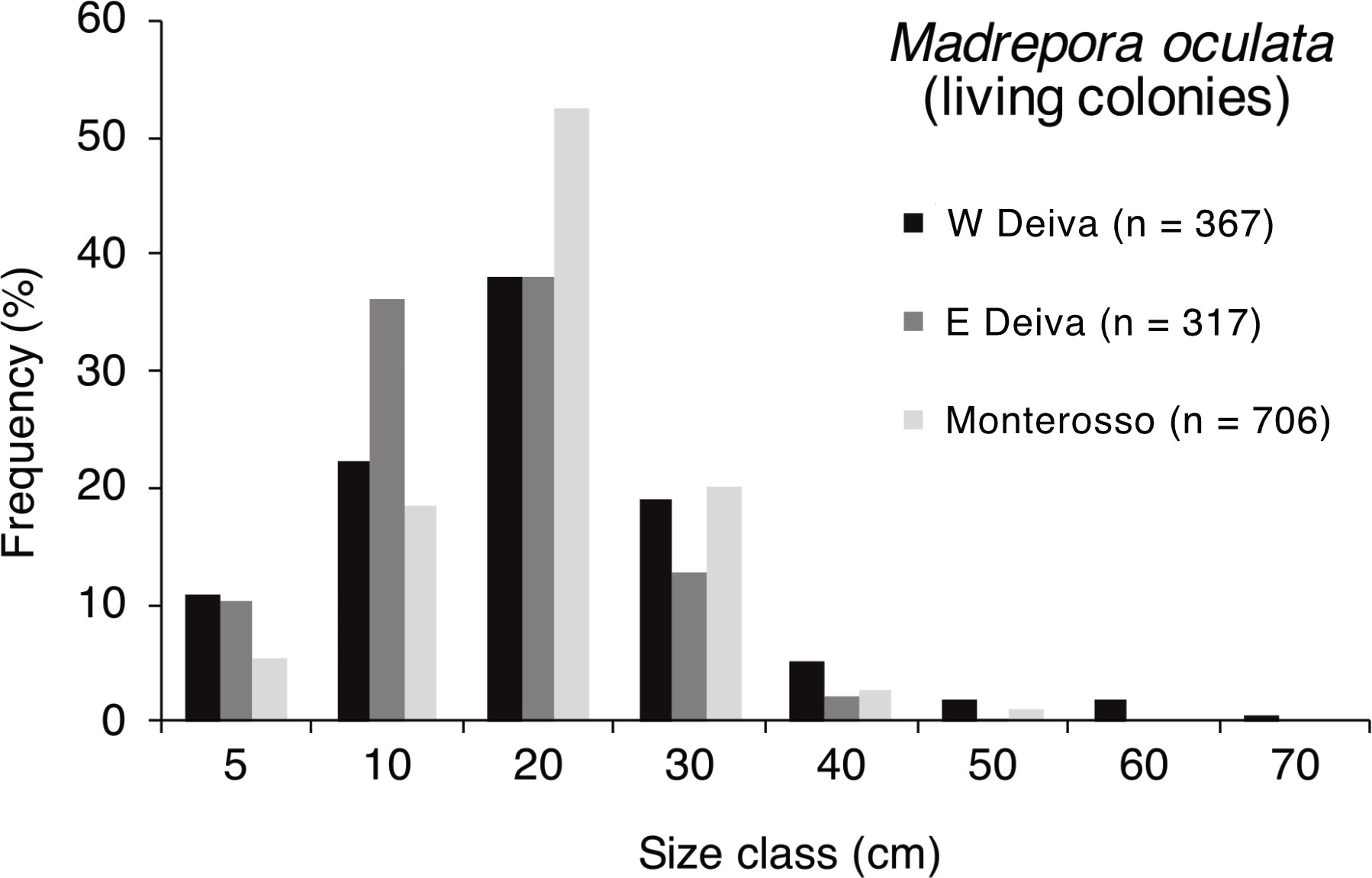
Figure 5 Height-frequency distribution of the main living populations of Madrepora oculata in the easternmost Ligurian coral areas.
Interesting sightings were also made on the soft bottoms nearby the coral occurrences (Table SM1). Among the most interesting or frequently observed taxa, it is worth mentioning the patches of large forams observed in Portofino and Monterosso, the demosponge Atergia corticata Stephens, 1915 (Deiva Marina), and one record of the large hydrozoan Branchiocerianthus italicus Stechow, 1921 (Monterosso, MS27). Among anthozoans, a yet unidentified keratoisidid species belonging to the genus Acanella was largely reported on the deep mud plain nearby the coral occurrences of W Deiva Marina (Figures 6A, B) and Monterosso. Soft-bottom isopods identified as Munnopsurus atlanticus (Bonnier, 1896) were commonly reported crawling on mud near the Monterosso coral sites.
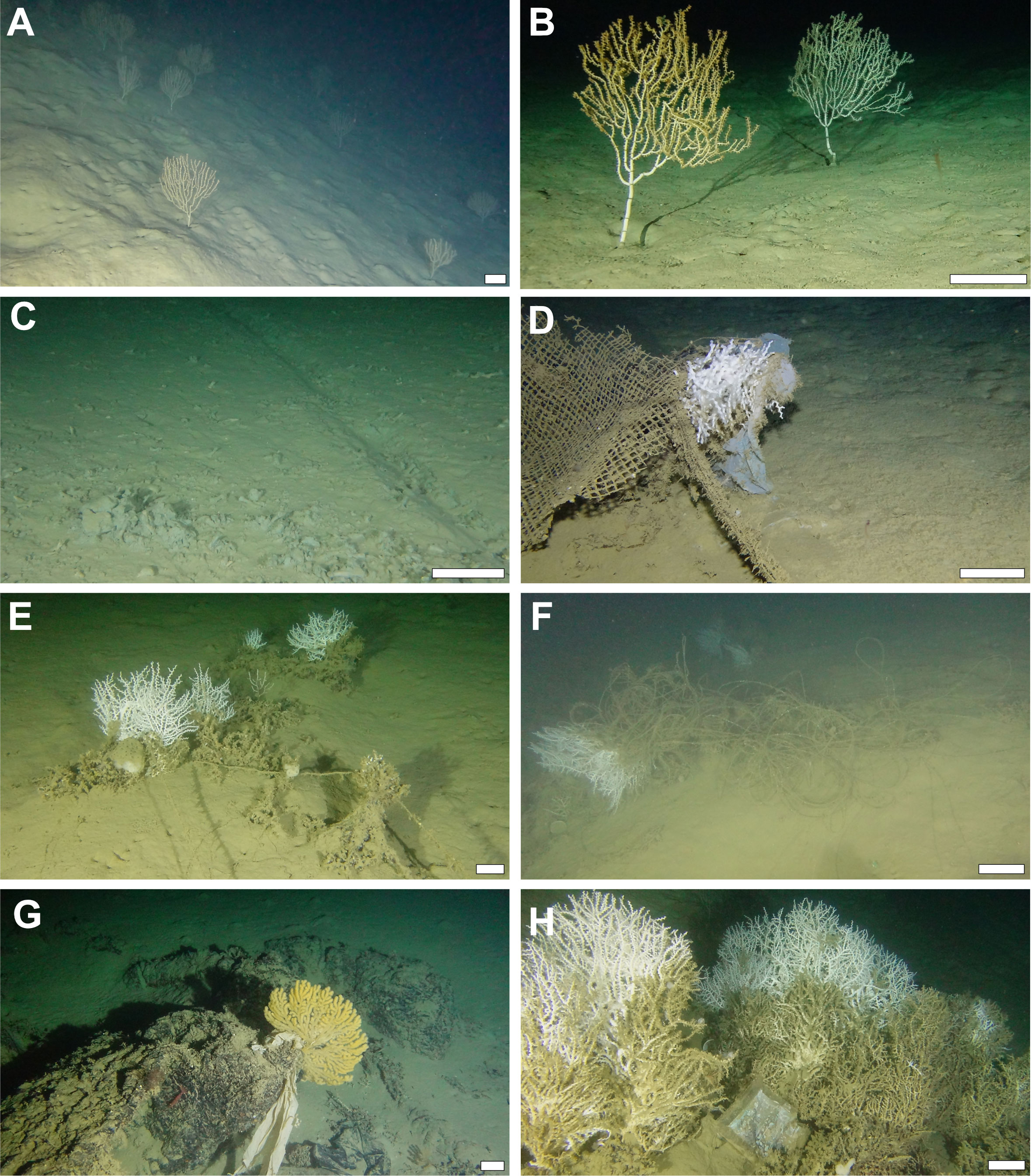
Figure 6 ROV footage of the anthropogenic impacts. (A, B) Forest of the keratoisidid Acanella sp. on the bathyal plain near the W Deiva Marina coral area (Dive14), (C) trawling track crossing the Genoa coral rubble accumulation (Dive5), (D, E) fragments of nets and lines entangling Madrepora oculata colonies in W and E Deiva Marina (Dive10 and Dive 11, respectively), (F) lost longline engulfing a coral colony in Monterosso (Dive7), (G) plastic fragment entangled on a colony of Placogorgia coronata in W Deiva Marina (Dive10), (H) plastic bag laying on M. oculata in Monterosso (Dive8). Scale bar: 10 cm.
Anthropogenic impacts
The anthropic impact was calculated on the entire investigated area, accounting for about 16400 m2. Overall, 502 items were recorded along the ROV tracks, attributed for 53.7% to abandoned, lost or otherwise discarded fishing gear (ALDFG), including longlines (62%), net fragments (27%), ropes (7%), and other items (e.g., buoys, moorings, bricks, boxes) (4%) related to professional or recreational fishing activities. The remaining 46.3% of the recorded items were attributed to general marine litter, including plastic items (e.g., fragments, glasses) (94%), metal fragments (5%) and glass bottles (1%). The normalized density distribution of these items among the sites was uneven (Figures 7A, B). Urban litter was recorded in all sub-areas, with densities as high as 1180 items ha-1 in W Deiva Marina (Dive 10), especially at the relief base. High values were also recorded on the Portofino (up to 246 items ha-1) (Dive 3) and Monterosso coral structures (up to 401 items ha-1) (Dive 8) (Figure 7A).
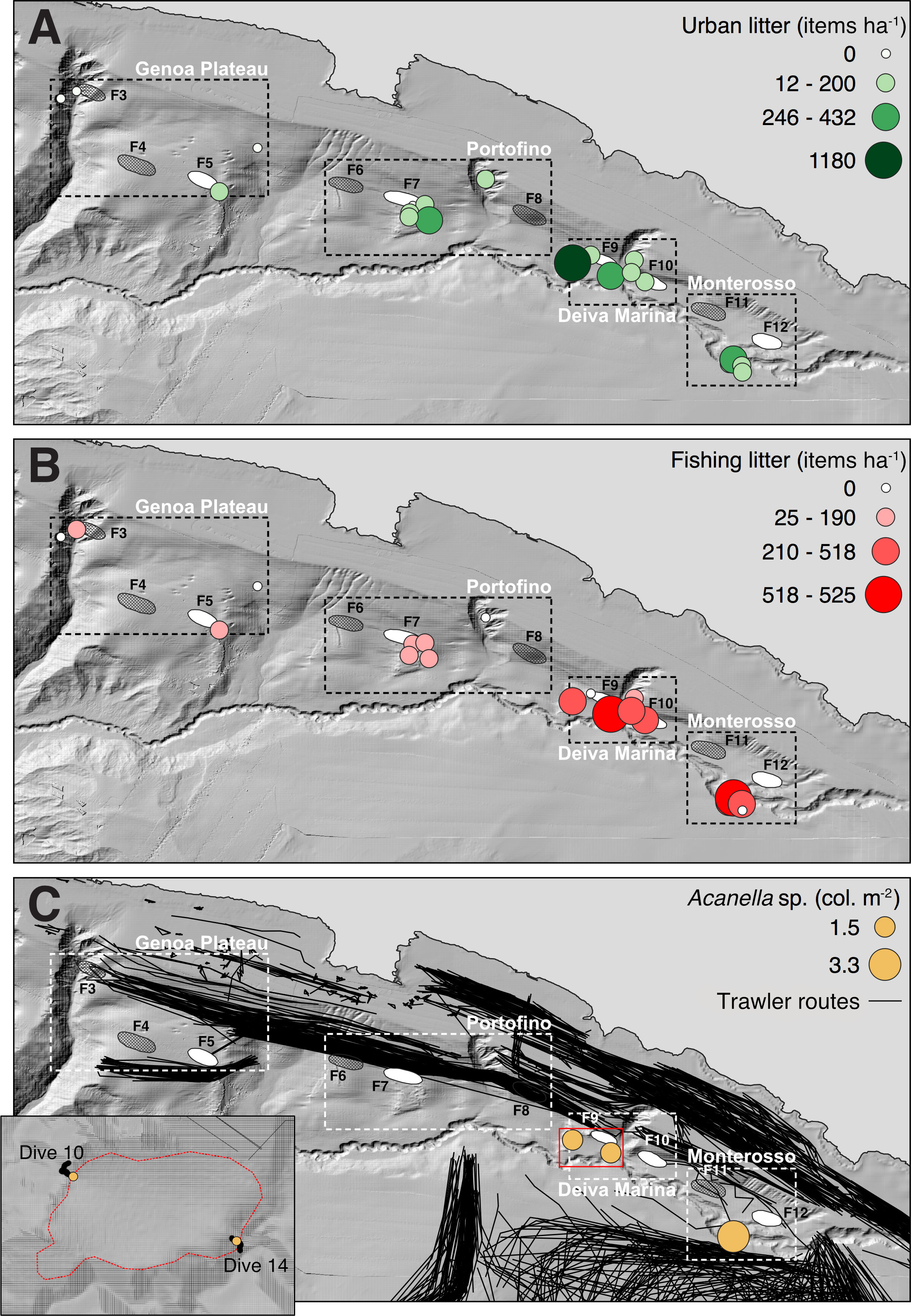
Figure 7 Anthropogenic impact. Distribution and average density (expressed as number of items ha-1) of (A) urban litter and (B) fishing litter. (C) Localization of the trawling routes (black lines) in the study area and identification of a trawling-free muddy plain near W Deiva Marina (F9) hosting high densities of the keratoisidid Acanella sp. (maps based on Atlas MaGIC bathymetry, Chiocci et al., 2021).
Low-density values of urban litter were reported from the Genoa Plateau coral rubble accumulation (100 items ha-1) (Figure 7A), which also showed low values of fishing litter density (50 items ha-1) (Dive 5) (Figure 7B). Here, the coral area was crossed by numerous deep trawling marks (Figure 6C) and imprints of trawling nets were observed. Dense AIS trawling routes crossed the entire Genoa Plateau, including the investigated area (Figure 7C) and numerous trawling vessels were encountered during the surveys. The other coral areas were subjected to a variable accumulation of fishing litter: the Portofino coral area was the one with the lowest density of fishing litter in all its explored sites (up to 188 items ha-1) (Dive 3) (Figure 7B). AIS trawling routes crossed the area along its northern border and vessels, observed during the surveys, marginally run over the F7 relief (Figure 7C). In Deiva Marina, the highest density values of fishing litter were recorded for the western coral area (up to 525 items ha-1) (Dive 14) (Figure 7B). In the entire sub-area, accumulations of fishing debris were commonly observed in all the investigated sites and overturned coral blocks were observed at the base of the reliefs in the sub-horizontal muddy areas. Here, AIS trawling routes mainly stopped nearby the flanks of the canyon (Figure 7C). Trawlers, commonly observed during the surveys, were reported to work very close to both reliefs. They start hauling the nets just before the rising of the relief in E Deiva Marina and they lower the nets nearby the western relief, at the beginning of the “Di Terra le Rame” trawling path towards Portofino. Finally, the Monterosso coral area showed density values of fishing litter as high as 518 items ha-1 (Dive 8) (Figure 7B). The sites were impacted by the crossing of trawling routes on the southern margin and only occasionally were run over (Figure 7C). Recreational fishing boats using longlines were observed for both coral areas of Deiva Marina.
A total of 352 entanglement events were reported in the region, excluding the Genoa Plateau coral rubble accumulation. Entanglements were caused both by fishing items (Figures 6D–F) and urban litter (Figures 6G, H). Considering the entire dataset, about 69% of the entanglements were reported on dead coral frameworks (especially in Portofino and W Deiva Marina), 22% on the living scleractinian CWCs (especially in E Deiva Marina and Monterosso), and 9% on erect alcyonaceans (especially in Portofino). Necrotic alcyonaceans were also reported in the area, mainly in Portofino (56.4%) and W Deiva Marina (23.1%).
Finally, three sites located nearby the coral structures of W Deiva Marina and Monterosso, characterized by sub-horizontal muddy planes, hosted dense populations of the keratoisidid Acanella sp. (Figures 6A, B, 7C). The analysis of the AIS trawling routes showed that the investigated bathyal muddy plateau of W Deiva Marina, with an estimated extension of about 54,000 km2, is not affected by trawling (Figure 7C).
Radiocarbon dating
The eight analyzed scleractinian coral samples comprised four specimens with ages ranging between 13365 and 7269 years before present (yrs BP). Two of these ages were obtained from D. pertusum specimens collected in the sub-areas of Genoa Plateau (F5) and Portofino (F7), one was obtained from a fragment of M. oculata collected on the Penelope Seamount, and one from a specimen of D. dianthus collected on the Spinola Seamount (Table 2 and Figures 8A, B, SM2). These samples were collected at approximately 500 m and 1800 m depth in the lower bathyal zone (Table 2). Four specimens of M. oculata, collected between 560 m and 650 m depth from Portofino (F7), W Deiva Marina (F9), and Monterosso (south of F12), revealed modern ages ranging from 229 to 76 yrs BP (Table 2 and Figures 8A, B, SM2).
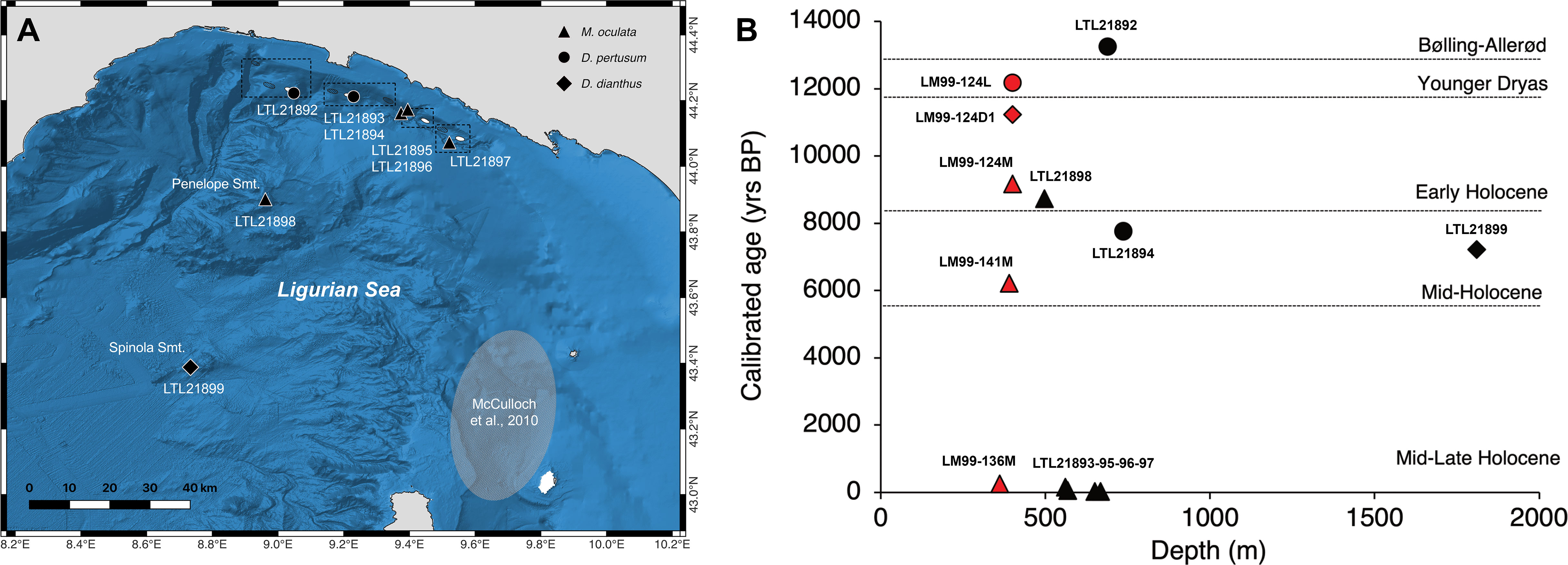
Figure 8 Radiocarbon dating. (A) Map of the sampling locations of the dated corals (black symbols). The grey oval indicates the sampling area of McCulloch et al., 2010. (B) Calibrated age (yrs BP) of the Ligurian coral samples plotted versus water depth. Legend of symbols and colours: circles refer to Desmophyllum pertusum, triangles refer to Madrepora oculata, and diamonds refer to Desmophyllum dianthus. Black is for the samples analyzed in the present study, while red is for Tuscan samples dated in McCulloch et al., 2010.
Discussion
Type and age of the Ligurian bathyal coral structures
The geomorphological features identified in the eastern sector of the Ligurian Sea include different types of coral structures (Figure 9), which have been collocated in the post-glacial timeline (Bølling-Allerød, Younger Dryas, Holocene) thanks to radiocarbon dating and following the most recent temporal distribution patterns of Mediterranean habitat-forming scleractinian CWCs (Figure 8B) (McCulloch et al., 2010; Fink et al., 2015; Stalder et al., 2015; Corbera et al., 2022a; Wienberg et al., 2022).
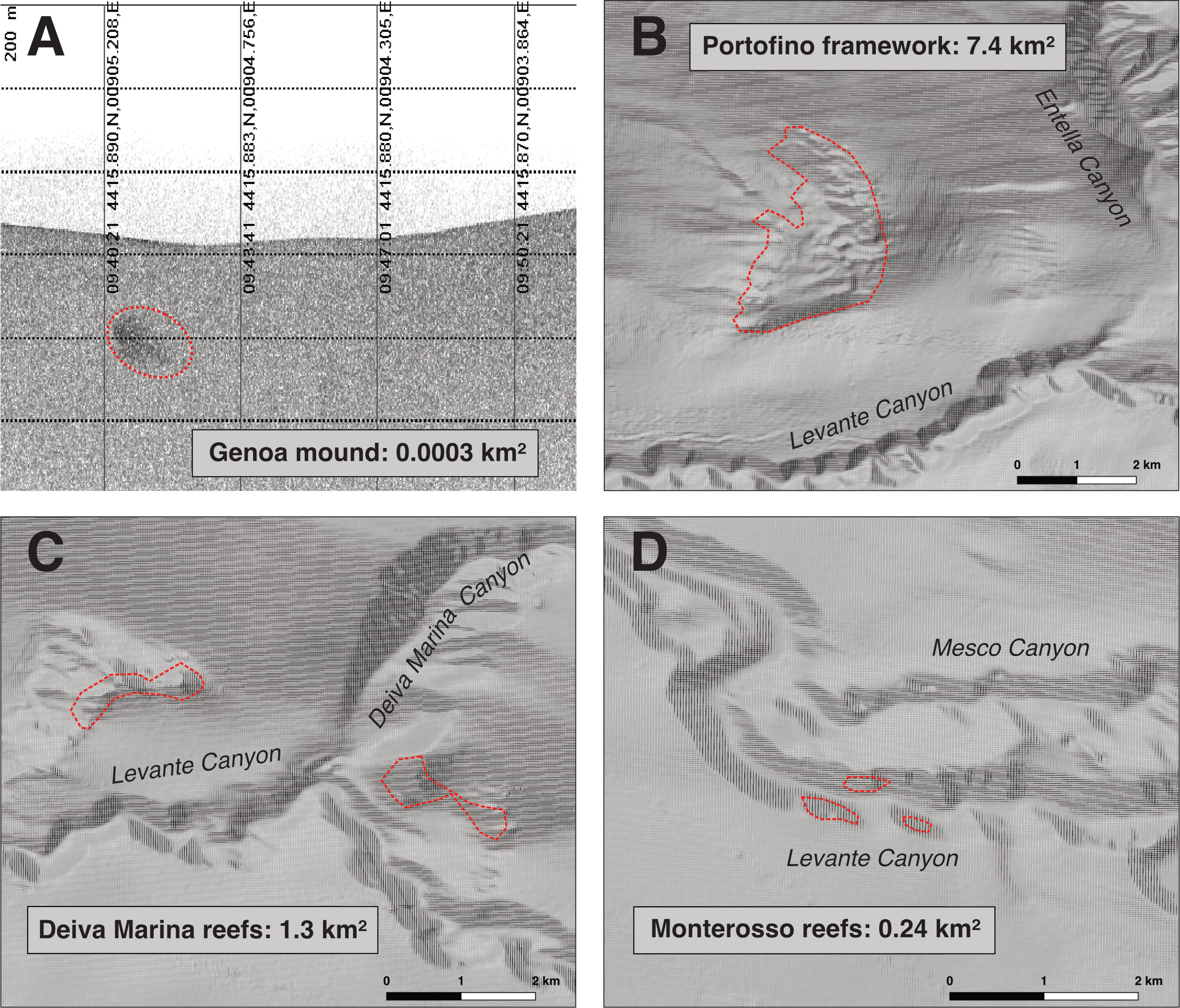
Figure 9 Putative extent of the coral areas in each sub-area (A, D) [(A) SSS image, (B–D) maps based on Atlas MaGIC bathymetry, Chiocci et al., 2021].
The accumulation of D. pertusum coral rubble found on the Genoa Plateau is most likely the exposed top of a small CWC mound (Figure 9A). However, only sediment cores could confirm this assumption as they are indispensable to tracing the recurring periods of coral reef proliferation and sedimentation of a mound and to delineating the paleoenvironmental factors that drove, over geological times, its formation (Huvenne et al., 2009; Roberts et al., 2009; Wang et al., 2021; Corbera et al., 2022a; Wienberg et al., 2022). Nevertheless, a mound is highly probable, given the high density of the rubble mixed with mud (Figure 2A). Although multibeam echosounders and photogrammetric techniques are now preferred to obtain high-definition maps of these structures, 2D mosaics have been broadly used to localize them, especially on flat seafloors (Remia and Taviani, 2005; Lim et al., 2021; Fabri et al., 2022), such as that of the Genoa Plateau. It has also been demonstrated that small-sized CWC mounds, which are impacted by trawling, appear with a reduced backscatter in the SSS (Roberts et al., 2006), as observed here. Small, scattered coral frameworks dominated by coral rubble may also result from not optimal environmental conditions exposing the mound to natural bioerosion and fragmentation and, ultimately, to burial (Wang et al., 2021). The size of the Genoa mound is significantly smaller than what is reported for other Mediterranean mounds, particularly the large mounds of Santa Maria di Leuca (up to 300 m wide) (Savini and Corselli, 2010), the subfossil mounds of Pantelleria (up to 10 m high and 90 m wide) (Martorelli et al., 2011), the Corsica Channel mounds (70-330 m wide) (Angeletti et al., 2020), and the km-long belts observed in the Alboran coral mounds provinces (Lo Iacono et al., 2012; Lo Iacono et al., 2014; Corbera et al., 2019; Wienberg et al., 2022) (Table SM2). On the other hand, the shallow-buried subfossil mounds of the Tuscan archipelago are more comparable in size (10 m wide and 3-4 m high) (Remia and Taviani, 2005).
The age of the coral sample obtained from the surface of the Genoa mound (13365 yrs BP) falls in the Bølling-Allerød warm interval (14.6-12.9 ka), and represents, so far, the oldest datum for this region. It is closely followed by the age datum (12180 yrs BP) obtained from a coral sample coming from another bathyal subfossil mound off the Tuscan Archipelago (355-441 m depth) (Figure 8B) (Remia and Taviani, 2005; McCulloch et al., 2010). The recorded age of the Genoa surface coral sample coincides, in the NW Mediterranean Sea, with a peak of meltwater discharge, which caused a significant reorganization of the thermohaline circulation leading to increased productivity and invigoration of the LIW, and, ultimately, to a proliferation of the corals (Fink et al., 2015; Wang et al., 2019; Corbera et al., 2021; Wienberg et al., 2022). The reported ages obtained from surface samples of D. pertusum in the Ligurian Sea are in accordance with numerous other stratigraphic records suggesting that, with a few exceptions (e.g., Corbera et al., 2022a), most Mediterranean CWC mounds dominated by this species showed maximum vertical growth or aggradation rates during the last deglaciation (Bølling-Allerød warm interval and the Younger Dryas cooling event), and during the early Holocene, in relation to climate-paced environmental changes (McCulloch et al., 2010; Fink et al., 2015; Stalder et al., 2015; Vertino and Corselli, 2019; Vertino et al., 2019; Wienberg, 2019; Chapron et al., 2020; Le Houedec et al., 2021; Wienberg et al., 2022).
The Portofino coral structure is a massive D. pertusum framework localized on a low-gradient slope, accounting for an estimated surface of about 7.4 km2 (Figure 9B) and possibly ascribable to a large CWC mound. Only the Alboran Sea hosts elongated ridge-like biogenic structures more extensive than the one reported here (Corbera et al., 2019; Hebbeln, 2019; Corbera et al., 2021; Wienberg et al., 2022) (Table SM2). Based on the radiocarbon dating of surface samples, the Portofino framework is at least 7838 years old (Figure 8B), falling close to the boundary between Early Holocene (11.7-8.2 ka) and Mid-Holocene (8.2-5.9 ka), similarly to the surface coral samples collected on the Penelope and Spinola seamounts (8.7 ka and 7.2 ka, respectively) and on the Tuscan CWC mounds (McCulloch et al., 2010) (Figure 8B). Prolific coral growth was supported in the western basin during the Early Holocene thanks to the formation of winter intermediate waters, which sustained high productivity despite a general reduction of LIW (Fink et al., 2015). On the other hand, the onset of marked oligotrophic conditions characterized the western areas in the Mid-Holocene, a period in which a widespread decline of corals was observed (Fink et al., 2015; Stalder et al., 2018), putatively also affecting bathyal offshore sites such as seamounts. Further geomorphological and sedimentological studies are needed to fully understand the geological development of the Portofino coral structure, but the reported age is consistent with the Mid-Holocene coral demise.
Lastly, the Deiva Marina and the Monterosso coral structures are ascribable to active reefs (Roberts et al., 2009). The two topographic reliefs of Deiva Marina cover at least 1.3 km2, while the fragmentary patches of Monterosso cover altogether about 0.24 km2 (Figure 9C, D). The reefs found on the Santa Maria di Leuca (Savini and Corselli, 2010) and Cabliers mounds (Corbera et al., 2019; Corbera et al., 2021) have a more extensive structural development (Table SM2); however, the Deiva Marina reefs show a more considerable continuous growth of the living portions similarly to what was observed in the flourishing sites of the Gulf of Lion (Fabri et al., 2022), Corsica Channel (Angeletti et al., 2020), and the Gulf of Naples (Taviani et al., 2019b; Angiolillo et al., 2023) (Table SM2). Based on the radiocarbon dating, the easternmost coral frameworks (W Deiva Marina and Monterosso) resulted younger than the westernmost ones (Genoa and Portofino) (Figure 8B). Sediment cores, however, are needed to investigate the age of the easternmost reefs further, since only modern rubble was collected in these localities.
Overall, despite more regional paleoenvironmental data and samplings being needed to obtain a complete picture of the proliferation and demise of scleractinian CWCs in the Ligurian Sea and nearby basins, this study provides a significant contribution to the understanding of the temporal occurrence of CW scleractinian corals in the region.
Spatial distribution and characteristics of the Ligurian bathyal coral structures
Of the five bathyal coral occurrences identified in this study, only the Portofino coral area is partially well enclosed in the historical boundaries specified in the nautical charts of Fusco (Fusco, 1967, 1968, 1972), while the others are located nearby. The historical coral areas were, indeed, mainly depicted based on fishers’ indications of retrieval of coral rubble, which could have been dragged from nearby structures by nets moving accidentally too close to the reliefs (Rossi, 1958; Enrichetti et al., 2018), as also reported around the subfossil Tuscan mounds (Remia and Taviani, 2005). Modified trawl nets with rubber rollers were also suggested for the easternmost areas (F11 and F12) (Fanelli et al., 2017), supporting the possibility of highly destructive dragging actions. Accidental impacts are confirmed in this study based on the trawling routes and the in-situ observation of trawling vessels. The Portofino framework was better enclosed in the historical boundaries of Fusco thanks to information coming from previous investigations (Blanc, 1959; Blanc et al., 1959) and the fact that, being a massive relief situated on a gentle slope, it was more exposed to trawling activities, hence to localization, especially along its northern boundary, closer to the fishing routes. Although found on a plateau, the Genoa mound was far too small to be precisely identified on the Fusco charts. The Deiva Marina and Monterosso reefs, located in canyon areas less accessible to trawling, were not precisely located too. The drag of scattered coral rubble miles from the source coral sites under the action of trawlers may also explain the historical identification of those Fusco coral areas lacking distinct biogenic reliefs within or nearby their boundaries (F3, F4, F6, F8, F11). However, it must be considered that seismostratigraphic analyses, not used here, may be able to identify buried topographic coral features, as carried out along the Tunisian slope (Camafort et al., 2020) and in the Tuscan Archipelago (Remia and Taviani, 2005). Overall, the Fusco charts (1967, 1968, 1972) proved reliable, suggesting that they represent some of the oldest maps available for the Mediterranean Sea regarding the distribution of scleractinian CWC frameworks, hence holding a particular historical value.
The localization of the Ligurian CWC mounds on muddy, low-gradient slope areas is in accordance with what was reported in other Mediterranean mound provinces, such as Santa Maria di Leuca (Savini and Corselli, 2010; Savini et al., 2014), Corsica Channel (Angeletti et al., 2020), Tuscan Archipelago (Remia and Taviani, 2005), East and West Melilla and Cabliers (Lo Iacono et al., 2014; Corbera et al., 2019; Corbera et al., 2021; Wienberg et al., 2022), where, however, the number of recorded mounds is significantly higher (from tens to hundreds) (Table SM2). The Deiva Marina and Monterosso reefs localized in canyons are, instead, unique for the Mediterranean Sea, showing 10% to 23% of living colonies thriving on complex dead frameworks for an overall seafloor coral coverage varying between 20% and 51%. Living reefs, in fact, although smaller and more fragmentary, are reported only in the Sardinian canyons (Taviani et al., 2017; Moccia et al., 2019), while the flourishing populations of the S Adriatic canyons (Angeletti et al., 2014), Dohrn Canyon (Taviani et al., 2019b; Angiolillo et al., 2023), and Gulf of Lion canyons (Orejas et al., 2009; Fabri et al., 2019; Fabri et al., 2022) thrive on rocks with no dead biogenic framework (Table SM2). A significant percentage of outcropping rock is reported in the study area only on the Deiva Marina reliefs, while no hardgrounds are visible in the Monterosso coral area. Considering the inclination of the substrate, the Monterosso reefs, thriving on muddy, sub-horizontal terraces exposed to strong currents, are similar to the Corse ones (Angeletti et al., 2020; Angiolillo et al., 2023). The W Deiva Marina reefs, on the other hand, thriving on sub-vertical substrata are similar to the populations observed in the Cap de Creus, Lacaze-Duthiers, Cassidaigne and Dohrn canyons (Orejas et al., 2009; Fabri et al., 2019; Taviani et al., 2019b; Dominguez-Carrió et al., 2022; Fabri et al., 2022; Angiolillo et al., 2023). However, the dense populations in the Lacaze-Duthiers Canyon are unique since they are located on the outer rim of outcropping lithified sedimentary strata (Fabri et al., 2014; Fabri et al., 2022).
The dominance of D. pertusum on the Genoa mound (690 m depth) and the Portofino framework (640-780 m depth), even if mainly subfossil, is in accordance with the general bathymetric distribution of this species in the Mediterranean Sea (Chimienti et al., 2019) and with most of the previous Mediterranean findings regarding mounds (Corbera et al., 2019). The Portofino framework is nowadays experiencing minimal growth, with only a few living colonies of D. pertusum observed in the area (up to 2 colonies m-2) (642-747 m depth). Yet, their existence is significant as it is the first documented report for the Ligurian Sea. Desmophyllum pertusum is also reported for the easternmost reefs, but its abundance is negligible. Significantly higher densities for this species have been reported in the Lacaze-Duthiers Canyon (Fabri et al., 2022), Corsica Channel (Angiolillo et al., 2023), Dohrn Canyon (Angiolillo et al., 2023), and on the Cabliers coral mound (Corbera et al., 2019) (up to 4-6 colonies m-2) (Table SM2). In the eastern Ligurian Sea, M. oculata is the main living reef-building species, found in a depth range (500-640 m depth) consistent with the main bathymetric distribution of this species in the Mediterranean basin (Chimienti et al., 2019). Among the known provinces, M. oculata is as deep as 1100 m in Santa Maria di Leuca and as shallow as 180-200 m depth in the Gulf of Lion and Alboran Sea (Chimienti et al., 2019) (with a minimum of 129 m depth in La Fonera Canyon) (Lastras et al., 2016) (Table SM2). The average density values of M. oculata range from 6 to 13 colonies m-2, similar to what is reported in Cassidaigne Canyon (Fabri et al., 2019), Dohrn Canyon (Angiolillo et al., 2023), Corsica Channel (Angiolillo et al., 2023), and on the Cabliers coral mound (Corbera et al., 2019) (up to 4-13 colonies m-2) (Table SM2).
The Ligurian living coral structures develop in a turbulent hydrodynamic LIW regime, reaching a speed of about 5 cm s-1 at 400 m (Poulain et al., 2020), and in a high turbidity environment (Fanelli et al., 2017), as also documented here in the ROV footage. The colonies and the dead biogenic framework underneath are oriented perpendicularly to the EW-flowing current in the Levante Canyon and its Deiva Marina tributary and reach considerable heights (up to 100 cm and 22 cm, respectively, for living M. oculata and D. pertusum) supporting the existence of favourable environmental conditions for their proliferation and growth (Gori et al., 2013; Fanelli et al., 2017; Fabri et al., 2019; Angeletti et al., 2020; Corbera et al., 2022b; Fabri et al., 2022). The deeper coral frameworks of Genoa and Portofino, located far from the Levante Canyon in areas subjected to higher sedimentation, show no or only modest living portions. In contrast, the highest percentage of living frameworks (23%) is reported in the Monterosso reefs, located within a narrow sector of the canyon. At the same time, the larger colonies are found on the W Deiva Marina elevation. These observations support both the canyon influence in sustaining the coral occurrence and the existence of local strong hydrodynamic regimes, coupled with the morphology of the reliefs, influencing their differential growth. In the Ligurian Sea, M. oculata reaches heights comparable to those reported for the Cabliers coral mound (up to 130 cm) (Corbera et al., 2019), Corsica Channel (up to 107 cm) (Angiolillo et al., 2023), Nora and Tavolara canyons (Sardinia) (more than 1 m) (Taviani et al., 2017; Moccia et al., 2019), Cassidaigne Canyon (78 cm) (Fabri et al., 2019), and Urania Bank in the Sicily Channel (up to 70 cm) (Freiwald et al., 2009) (Table SM2). Desmophyllum pertusum colonies are significantly more developed in most of the known provinces, particularly on the Cabliers coral mound (bushes 3 m high) (Corbera et al., 2019), Lacaze-Duthiers Canyon (up to 88 cm, with bushes as high as 2.5 m) (Fabri et al., 2022), Corsica Channel (up to 80 cm) (Angiolillo et al., 2023), and Dohrn Canyon (up to 72 cm) (Angiolillo et al., 2023) (Table SM2).
Associated biodiversity and habitat-forming species
The visual census carried out in the coral areas of the eastern Ligurian Sea accounts for 104 megabenthic and demersal taxa, of which 89 are found on the coral frameworks and coral rubble (and partially on rocks), while the remaining ones on the nearby soft bottoms. Among the identified taxa, ten are habitat-forming species (including Acanella sp. on soft bottoms), of which four (D. dianthus, D. pertusum, M. oculata, and P. larix) are included in the vulnerable categories of the IUCN Anthozoa Red List (Table SM1) (Otero et al., 2017). Besides sessile and sedentary fauna, the overall biodiversity also includes a large number of mobile visiting species, nine of which are of commercial interest, particularly Eledone cirrhosa (Lamarck, 1798), Aristeus antennatus (Risso, 1816), Lepidopus caudatus (Euphrasen 1788), and Pagellus bogaraveo, the latter considered Near Threatened (Table SM1). Indeed, much evidence has been provided on the utilization of CWC-dominated habitats and canyons by fishes as areas for refuge, growth, and reproduction, supporting the classification of these areas as Essential Fish Habitats (EFH) (D’Onghia et al., 2012; Capezzuto et al., 2018). These sites also attract numerous planktonic species, some already highlighted as possible preferred prey for CWCs (Fanelli et al., 2017).
The highly tridimensional structures (here expressed in height and substrate coverage) of Portofino, W and E Deiva Marina, and Monterosso show a significant attractiveness for megabenthic and demersal species (23-34 taxa per dive). On the other hand, the Genoa mound, dominated by coral rubble, presents the lowest richness of associated megafauna (7 taxa per dive). This datum is consistent with the low habitat complexity and high level of degradation of the coral area; however, although the site was well explored due to its limited size, it should be considered that longer ROV tracks were carried out in the other coral areas. The overall diversity found in the sub-areas shows the same pattern, with 27 species recorded on the Genoa Plateau (and no habitat-forming taxa) and 53-72 in the other three sub-areas (including 7-9 habitat-forming species), without clear differences in terms of megafauna, between areas hosting only dead frameworks (Portofino, 60 taxa) or living reefs on dead frameworks (W Deiva Marina, E Deiva Marina, and Monterosso, 42, 64 and 53 taxa, respectively).
The visual census confirms the prominent role of this tridimensional biogenic habitat in attracting a rich associated bathyal diversity (Chimienti et al., 2019; Rueda et al., 2019). The different sampling efforts and the different dimensional targets of the taxonomic analyses partially bias comparisons with other Mediterranean areas dominated by scleractinian CWCs. Nevertheless, it is possible to recognize the eastern Ligurian coral region among the richest provinces, including the S Adriatic one (111 species) (Angeletti et al., 2014) and Santa Maria di Leuca (257 species) (Mastrototaro et al., 2010) (Table SM2). Differences in species diversity can be attributed to various environmental factors, such as substrate inclination, local hydrodynamic conditions, organic inputs, and the complexity of the biogenic structures (Fabri et al., 2019). Including meiofauna and macrofauna in the census boosts diversity levels (Oceana, 2014) and may show more distinct differences between the rubble, dead frameworks and living portions (Mastrototaro et al., 2010).
Seven habitat-forming species, besides M. oculata and D. pertusum, are reported in the study area settled on dead coral frameworks and coral rubble, supporting the importance of these substrates for the development of animal forests, which, ultimately, increase the tridimensionality of the habitat (Rueda et al., 2019). The co-occurrence of animal forests (dominated by sponges, anthozoans, bivalves, or barnacles) and habitat-forming scleractinian CWCs has been observed in all the known provinces of the Mediterranean basin (Table SM2) on coral remains or rocks with the highest diversity in the Cassidaigne Canyon (13 species) (Fabri et al., 2014), Santa Maria di Leuca (11 species) (Mastrototaro et al., 2010), and Corsica Channel (9 species) (Angeletti et al., 2020; Angiolillo et al., 2023) (Table SM2). In the explored region, many habitat-forming anthozoan species, including the rare alcyonaceans P. coronata (primarily reported in the NW Mediterranean Sea) (Carpine and Grasshoff, 1975; Enrichetti et al., 2018) and A. armata (mainly found in the Alboran Sea) (Otero et al., 2017; Rueda et al., 2021), are also known from the NE Atlantic Ocean. The high occurrence of northern Atlantic species in the Ligurian Sea is a biogeographic pattern already observed in other bathyal habitats, probably related to the cold deep waters of this region (Bo et al., 2020). Worth mentioning is also the occurrence of large meadows of the endemic bathyal species P. massiliensis (Carpine and Grasshoff, 1975; Sartoretto and Zibrowius, 2018) thriving on coral rubble.
Anthropogenic impacts
Finally, this study quantifies the anthropogenic impacts on the coral ecosystems along the eastern Ligurian slope. Both urban litter and ALDFG are pervasive in all sub-areas. The two components contribute almost equally to the observed seafloor litter, with 46% of the recorded items attributed to urban litter, of which 94% are plastic items. The remaining 54% of the recorded items are attributed to ALDFG, mainly longlines (62%). General plastic items and longlines are commonly the dominant components of bathyal seafloor litter (Angeletti et al., 2014; Fabri et al., 2014; Savini et al., 2014; Tubau et al., 2015; Lastras et al., 2016; Angiolillo and Fortibuoni, 2020; Bo et al., 2020; Dominguez-Carrió et al., 2020; Grinyó et al., 2020; Angiolillo et al., 2021a; Bo et al., 2021; Grinyó et al., 2021; Fabri et al., 2022).
The high occurrence of urban litter is consistent with the heavily urbanized Ligurian coastline, the heavy commercial and recreative maritime traffic of the region, and its narrow continental shelf (Enrichetti et al., 2020). The occurrence of urban items was already reported in the western Ligurian canyons (up to 12 items km-1 and about 200 items ha-1 for French and Italian ones, respectively) (Fabri et al., 2014; Giusti et al., 2019). The accumulation of land-originated litter and vessel-discarded litter in canyons reflects the funnelling effect of these typographic structures (Tubau et al., 2015; Lastras et al., 2016; Cau et al., 2017; Pierdomenico et al., 2020) and may be associated with energetic coastal hydrodynamic processes such as storms (Tubau et al., 2015). This explains why the distance of the canyon head from the coast is generally known to have a significant influence on litter distribution (Fabri et al., 2014; Tubau et al., 2015; Gerigny et al., 2019; Angiolillo and Fortibuoni, 2020; Fabri et al., 2022; Angiolillo et al., 2021b). When considering the coral areas separately, urban litter shows higher densities than ALDFG on the W Deiva Marina reef (up to 1180 items ha-1), at the base of the relief, and on the Portofino framework (up to 246 items ha-1). The first area possibly receives litter funnelled by the Deiva Marina Canyon, whose head is only 4 km from densely populated cities and harbours. The Portofino framework is further from the influence of the canyons system but is located in an area crossed by numerous commercial and touristic vessels. Urban items are reported in multiple CWC provinces, although with lower values than what is found in the eastern Ligurian Sea, including Lacaze-Duthiers and Cassidaigne canyons (1-2 items km-1, respectively) (Fabri et al., 2014), Cap de Creus Canyon (up to 160 items ha-1) (Dominguez-Carrió et al., 2020), and Santa Maria di Leuca (600 items ha-1) (Savini et al., 2014) (Table SM2).
The high occurrence and typologies of ALDFG support a significant presence of artisanal and recreational fishing activities in the studied coral areas, confirmed by in situ boats observed during the surveys. As urban litter, fishing gear were also reported in the western Ligurian canyons (up to 100 items km-1 and about 700 items ha-1, for French and Italian ones, respectively) (Fabri et al., 2014; Giusti et al., 2019). The eastern Ligurian deep continental shelf and shelf break show higher values of ALDFG (about 600-1400 items ha-1) (Enrichetti et al., 2020) with respect to the investigated slope region (up to 525 items ha-1), suggesting a more moderate impact at higher depths, probably related to a more limited number of fishers exploiting these areas. The abundance of fishing items is overall higher on the tridimensional reef frameworks compared to what observed on the coral rubble of the Genoa mound, supporting a high catchability to longlines of the complex coral frameworks (Mytilineou et al., 2014). When considering the coral areas separately, ALDFG show high densities, especially on the E Deiva Marina reef (up to 398 items ha-1) and on the Monterosso reefs (up to 518 items ha-1). Concomitantly, these areas also show a lower percentage of large M. oculata colonies and a high percentage of entanglements on this species, supporting the high vulnerability of these reefs. A similar impact was also reported in the Bari Canyon (Angeletti et al., 2014), Dohrn Canyon and Corsica Channel (Angiolillo et al., 2023). ALDFG density was modest on the Portofino framework and the W Deiva Marina reef; here, entanglements were mainly observed on the dead coral frameworks and habitat-forming anthozoans. These areas are probably more frequented by trawlers, therefore, are less accessible to artisanal and recreational fishers. ALDFG are reported in most CWC provinces, except for the Cabliers coral mound, which is far away from the coastline (Corbera et al., 2019; Corbera et al., 2021). Significant density values are found in the Cap de Creus Canyon (over 500 items ha-1) (Dominguez-Carrió et al., 2020), Dohrn Canyon (5.3 ALDFG per 100 m) (Angiolillo et al., 2023), French canyons (0.3 m of longlines m-2) (Fabri et al., 2022), and Santa Maria di Leuca (0.1 items m-2) (Savini et al., 2014) (Table SM2). Significant impact is also reported on the Chella Bank (Lo Iacono et al., 2012).
Besides small-scale fisheries, trawling posed and still poses a severe threat to the studied coral areas, especially exposed mounds and regions adjacent to reefs. This is suggested by the analysis of the trawling routes and the in situ observation of vessels and is evidenced by the observed coral rubble (ranging between 6% and 21% in the areas with coral reliefs), trawling scars, overturned frameworks, and imprints of trawling nets supporting past and recent traffic. This is not an unusual impact in CWC-dominated areas (Tursi et al., 2004; Lo Iacono et al., 2012; Lastras et al., 2016; D’Onghia et al., 2017; Fanelli et al., 2017; Moccia et al., 2019; Angiolillo et al., 2023). In this regard, the occurrence of forests of a fragile species, such as the keratoisidid Acanella sp., is a good indicator of pristine environments, as already pointed out for the far better-known species Isidella elongata (Esper, 1788) (Carbonara et al., 2022) especially in areas that result less accessible to trawling and therefore turn into indirectly protected enclaves (Bo et al., 2015). Based on the extent of the area and the average density of the colonies, the bathyal low-gradient slope of W Deiva Marina potentially hosts up to 81,000 colonies, making this area one of the most valuable VMEs of the region (FAO, 2009). Considering the high sensitivity of soft-bottom bathyal keratoisidid species such as I. elongata (Otero et al., 2017) and the historical decline of the populations in the NW Mediterranean region (Relini et al., 1986; Gonzalez-Irusta et al., 2022), management initiatives targeting the seafloor integrity in these poorly exploited and confined areas would have a substantial effect on the conservation of this habitat and its associated resources.
Conclusions
A broad knowledge gap in terms of the large-scale distribution of deep-sea benthic habitats characterized the Ligurian Sea. While a significant effort was recently conducted to characterize the deep circalittoral and shelf break megabenthic biocoenoses of the Ligurian coast (Enrichetti et al., 2019), only historical data based on bycatch (Rossi, 1958; Relini-Orsi, 1974) and point-like modern surveys (Fanelli et al., 2017; Enrichetti et al., 2018; Giusti et al., 2019; Bo et al., 2020; Bo et al., 2021) were available at bathyal depths. The present study, therefore, provides novel information regarding the localization and characteristics of the bathyal CWC ecosystems of the eastern Ligurian Sea. It would be interesting in the future to explore the genetic connectivity levels among the four main NW bathyal coral sites (NE Sardinia, Corsica Channel, eastern Ligurian Sea, and Gulf of Lion), which, together, represent one of the most flourishing scleractinian CWC regions of the Mediterranean Sea.
The distribution of the investigated bathyal coral structures suggests the existence of a CWC belt. This latter is sustained by the strong bottom currents related to the along-slope flow of the LIW and flux of particulate organic material moving into the Levante Canyon along the entire eastern Ligurian basin, mainly between 450 and 750 m depth. Further studies will help to fill the knowledge gap in the western sector of the basin; however, so far, the putative area of the coral occurrences based on the extension of the topographic reliefs and the ROV surveys accounts for about 9 km2 of dead coral frameworks, coral rubble and flourishing living reefs, the latter represented by up to 23% of living M. oculata colonies (Figure 9). Besides the vast provinces of Santa Maria di Leuca (68 km2) (Savini et al., 2014) and Alboran Sea (Wienberg et al., 2022), the eastern Ligurian CWC belt is comparable to the Corsica Channel (5.3 km2) and Gulf of Lion provinces (Table SM2).
Present data support the existence of a distinct eastern Ligurian CWC province. The overall area is likely to increase because the coral occurrence in the Entella Canyon is still to be verified, and the calculations in both Deiva Marina areas need to better take into account the extension of the reefs along the slopes of the sites, only marginally considered here due to the steep inclination and strong currents. In addition, other interesting areas in the Levante Canyon have been identified. The implementation of high-resolution bottom mapping technologies, modern acoustic systems, and the recent deployment of a long-term deep observatory (Levante Canyon Mooring) (Ciuffardi et al., 2020; Ciuffardi et al., 2023) will prove fundamental to monitoring the environmental parameters and define predictive habitat models in the region. So far, based on the data regarding coral framework extent and complexity, associated diversity, density of habitat-forming species, occurrence of rare species, and vulnerability to anthropogenic impacts, the Portofino framework, the Deiva Marina reefs with the nearby bathyal low-gradient slope, and the Monterosso reefs represent top priority sites eligible for specific conservation actions, including offshore fisheries restricted areas (FRAs) as already suggested or put in action for other sensitive habitats (de Juan and Lleonart, 2010; Fabri et al., 2014; Angeletti et al., 2021).
Data availability statement
The original contributions presented in the study are included in the article/Supplementary Material. Further inquiries can be directed to the corresponding author.
Author contributions
Conceptualization, MB, GB. Methodology, validation, formal analysis, MB, FE, FB, GG, GQ, LC, GB. Investigation, resources, data curation, writing-original draft preparation, MB, FE, FB, GG, GQ, LC, GB. Writing-review and editing, MB, FE, FB, GQ, LC, GB. Visualization, supervision, project administration, MB, FE. All authors contributed to the article and approved the submitted version.
Funding
This work was supported by Università degli Studi di Genova, Curiosity Driven Project “Lost coral reefs: ancient deep-sea bioherms in a modern world” (2021-2022) (including open access publication fees), Agenzia Regionale per la Protezione dell’Ambiente Ligure (grant no. 127/2015, 109/2016, 110/2017, within the Marine Strategy Framework Monitoring Program, ARPAL n. 177/2014), and the SIR-MIUR_BIOMOUNT Project “Biodiversity Patterns of the Tyrrhenian Seamounts” [grant number RBSI14HC9O].
Acknowledgments
The authors would like to thank the members of the Fondazione Azione Mare for their help, dedication, experience, and technical expertise during all field surveys. The authors would like to thank also Prof. Danilo Morelli and Prof. Nicola Corradi (Università degli Studi di Genova) for their help with the bathymetric dataset and Mr Alfonso Costantini for the information on coral bycatch.
Conflict of interest
The authors declare that the research was conducted in the absence of any commercial or financial relationships that could be construed as a potential conflict of interest.
Publisher’s note
All claims expressed in this article are solely those of the authors and do not necessarily represent those of their affiliated organizations, or those of the publisher, the editors and the reviewers. Any product that may be evaluated in this article, or claim that may be made by its manufacturer, is not guaranteed or endorsed by the publisher.
Supplementary material
The Supplementary Material for this article can be found online at: https://www.frontiersin.org/articles/10.3389/fmars.2023.1114417/full#supplementary-material
Supplementary Figure 1 | Side-scan sonar profiles of the five coral areas. (A) Genoa Plateau, (B) Portofino, (C) W Deiva Marina, (D) E Deiva Marina, (E) Monterosso.
Supplementary Figure 2 | Pictures of the specimens submitted to AMS radiocarbon dating. (A) Desmophyllum pertusum from the Genoa Plateau (F5), (B, C) Madrepora oculata and D. pertusum from the Portofino framework (F7), (D, E) M. oculata from W Deiva Marina (F9), (F) M. oculata from Monterosso (F12), (G) M. oculata from the Penelope Seamount, and (H) Desmophyllum dianthus from the Spinola Seamount.
References
Angeletti L., Castellan G., Montagna P., Remia A., Taviani M. (2020). The “Corsica channel cold-water coral province” (Mediterranean Sea). Front. Mar. Sci. 7. doi: 10.3389/fmars.2020.00661
Angeletti L., D’Onghia G., Otero M. D. M., Settanni A., Spedicato M. T., Taviani M. (2021). A perspective for best governance of the Bari canyon deep-Sea ecosystems. Water 13, 1646. doi: 10.3390/w13121646
Angeletti L., Taviani M., Canese S., Foglini F., Mastrototaro F., Argnani A., et al. (2014). New deep-water cnidarian sites in the southern Adriatic Sea. Mediterr. Mar. Sci. 15, 263–273. doi: 10.12681/mms.558
Angiolillo M., Bo M., Toma M., Giusti M., Salvati E., Giova A., et al. (2023). A baseline for the monitoring of Mediterranean upper bathyal biogenic reefs within the marine strategy framework directive objectives. Deep Sea Res. I 194, 103963. doi: 10.1016/j.dsr.2023.103963
Angiolillo M., Fortibuoni T. (2020). Impacts of marine litter on Mediterranean reef systems: from shallow to deep waters. Front. Mar. Sci. 7. doi: 10.3389/fmars.2020.581966
Angiolillo M., Gérigny O., Valente T., Fabri M. C., Tambute E., Rouanet E., et al. (2021b). Distribution of seafloor litter and its interaction with benthic organisms in deep waters of the Ligurian Sea (Northwestern Mediterranean). Sci. Total Environ. 788, 147745. doi: 10.1016/j.scitotenv.2021.147745
Angiolillo M., La Mesa G., Giusti M., Salvati E., Di Lorenzo B., Rossi L., et al. (2021a). New records of scleractinian cold-water coral (CWC) assemblages in the southern Tyrrhenian Sea (western Mediterranean sea): human impacts and conservation prospects. Prog. Ocean. 197, 102656. doi: 10.1016/j.pocean.2021.102656
Astraldi M., Gasparini G. P., Sparnocchia S. (1994). “The seasonal and interannual variability in the Ligurian‐Provençal basin,” in Seasonal and interannual variability of the Western Mediterranean Sea. Ed. La Viollette P. E. (Washington, DC: American Geophysical Union), 93–113. doi: 10.1029/CE046p0093
Bassano E., Boniforti R., Pezzani A. (2000). “Parametri meteorologici e idrologici,” in Sistema informativo e di monitoraggio marino costiero della provincia della spezia. Ed. Peroni C. (La Spezia, Italy: ENEA), 17–55.
Blanc J. J. (1959). Recherches sur les vases du Golfe de Génes (Région de Portofino). Ann. Inst. Océan. 37, 274–287.
Blanc J. J., Pérès J. M., Picard J. (1959). Coraux profonds et thanatocoenoses quaternaires en Méditerranée. Coll. Intern. Sci. Terr. CNRS 83, 185–192.
Bo M., Bavestrello G., Angiolillo M., Calcagnile L., Canese S., Cannas R., et al. (2015). Persistence of pristine deep-sea coral gardens in the Mediterranean Sea (SW Sardinia). PloS One 10, e0119393. doi: 10.1371/journal.pone.0119393
Bo M., Coppari M., Betti F., Enrichetti F., Bertolino M., Massa F., et al. (2021). The high biodiversity and vulnerability of two Mediterranean bathyal seamounts support the need for creating offshore protected areas. Aq. Cons. Mar. Fresh. Ecosys. 31, 543–566. doi: 10.1002/aqc.3456
Bo M., Coppari M., Betti F., Massa F., Gay G., Cattaneo-Vietti R., et al. (2020). Unveiling the deep biodiversity of the Janua Seamount (Ligurian sea): first Mediterranean sighting of the rare Atlantic bamboo coral Chelidonisis aurantiaca studer 1890. Deep Sea Res. Part I 156, 103186. doi: 10.1016/j.dsr.2019.103186
Bosman A., Casalbore D., Anzidei M., Muccini F., Carmisciano C., Latino F. C. (2015). The first ultra-high resolution digital terrain model of the shallow-water sector around Lipari island (Aeolian islands, Italy). Ann. Geophys. 58, 1–21. doi: 10.4401/ag-6746
Brunelli G., Bini G. (1934). Ricerche comparative sulle pesche profonde di diversi mari italiani. Boll. Pes. Pisc. Idrob. 10, 733–744.
Calcagnile L., Maruccio L., Scrimieri L., delle Side D., Braione E., D'Elia M., et al. (2019). Development and application of facilities at the centre for applied physics, dating and diagnostics (CEDAD) at the university of salento during the last 15 years. Nucl. Instrum. Methods Phys. Res. B 456, 252–256. doi: 10.1016/j.nimb.2019.03.031
Calcagnile L., Quarta G., D’Elia M., Gottdang A., Klein M., Mous D. J. W. (2004). Radiocarbon precision tests at the Lecce AMS facility using a sequential injection system. Nucl. Instrum. Methods Phys. Res. B 215, 561–564. doi: 10.1016/j.nimb.2003.07.004
Calcagnile L., Quarta G., D’Elia M., Muscogiuri D., Maruccio L., Butalag K., et al. (2005). Instrumental developments at the IBA-AMS dating facility at the university of Lecce. Nucl. Instrum. Methods Phys. Res. B 240, 22–25. doi: 10.1016/j.nimb.2005.06.081
Camafort M., Gràcia E., Ranero C. R. (2020). Quaternary seismostratigraphy and tectonosedimentary evolution of the north Tunisian continental margin. Tectonics 39, e2020TC006243. doi: 10.1029/2020TC006243
Capezzuto F., Sion L., Ancona F., Carlucci R., Carluccio A., Cornacchia L., et al. (2018). Cold-water coral habitats and canyons as essential fish habitats in the southern Adriatic and northern Ionian Sea (central Mediterranean). Ecol. Quest. 29, 9–23. doi: 10.12775/EQ.2018.019
Carbonara P., Zupa W., Follesa M. C., Cau A., Donnaloia M., Alfonso S., et al. (2022). Spatio-temporal distribution of Isidella elongata, a vulnerable marine ecosystem indicator species, in the southern Adriatic Sea. Hydro 849, 4837–4855. doi: 10.1007/s10750-022-05022-4
Carpine C., Grasshoff M. (1975). Les Gorgonaires de la Méditerranée. Bull. Inst. Océanogr. (Monaco) 71, 1–140.
Carugati L., Martire M. L., Danovaro R. (2019). Patterns and drivers of meiofaunal assemblages in the canyons Polcevera and Bisagno of the Ligurian Sea (NW Mediterranean Sea). Prog. Oceanogr. 175, 81–91. doi: 10.1016/j.pocean.2019.03.010
Cathalot C., Van Oevelen D., Cox T. J. S., Kutti T., Lavaleye M., Duineveld G., et al. (2015). Cold-water coral reefs and adjacent sponge grounds: hotspots of benthic respiration and organic carbon cycling in the deep sea. Front. Mar. Sci. 2. doi: 10.3389/fmars.2015.00037
Cattaneo-Vietti R., Albertelli G., Aliani S., Bava S., Bavestrello G., Benedetti-Cecchi L., et al. (2010). The Ligurian Sea: state of the art, problems and perspectives. Chem. Ecol. 26, 319–340. doi: 10.1080/02757541003689845
Cau A., Alvito A., Moccia D., Canese S., Pusceddu A., Cannas R., et al. (2017). Submarine canyons along the upper Sardinian slope (Central Western Mediterranean) as repositories for derelict fishing gears. Mar. Pollut. Bull. 123, 357–364. doi: 10.1016/j.marpolbul.2017.09.010
Chapron L., Le Bris N., de Madron X. D., Peru E., Galand P. E., Lartaud F. (2020). Long term monitoring of cold-water coral growth shows response to episodic meteorological events in the NW Mediterranean. Deep Sea Res. Part I 160, 103255. doi: 10.1016/j.dsr.2020.103255
Chimienti G., Bo M., Taviani M., Mastrototaro F. (2019). “19 occurrence and biogeography of Mediterranean cold-water corals,” in Mediterranean Cold-water corals: past, present and future, vol. 9 . Eds. Orejas C., Jiménez C. (Cham: Springer), 213–243. doi: 10.1007/978-3-319-91608-8_19
Chiocci F. I., Budillon F., Ceramicola S., Gamberi F., Orrù P. (2021). “Atlante dei lineamenti di pericolosità geologica dei mari italiani,” in Risultati del progetto MaGIC (Rome: CNR Edizioni).
Ciuffardi T., Berta M., Bordone A., Borghini M., Celentano P., Cocchi L., et al. (2020). A new multidisciplinary observatory in the Eastern Ligurian Sea (NW Mediterranean sea): a combination of deep-sea and coastal measurements (EGU General Assembly 2020). doi: 10.5194/egusphere-egu2020-16533
Ciuffardi T., Kokkini Z., Berta M., Locritani M., Bordone A., Delbono I., et al. (2023). Deep water hydrodynamic observations around a cold-water coral habitat in a submarine canyon in the Eastern Ligurian Sea (Mediterranean Sea). Earth Sys. Sci. Data Disc. 15, 1933–1946. doi: 10.5194/essd-15-1933-2023
Corbera G., Iacono C. L., Gràcia E., Grinyó J., Pierdomenico M., Huvenne V. A., et al. (2019). Ecological characterisation of a Mediterranean cold-water coral reef: Cabliers coral mound province (Alboran Sea, western Mediterranean). Prog. Oceanogr. 175, 245–262. doi: 10.1016/j.pocean.2019.04.010
Corbera G., Lo Iacono C., Simarro G., Grinyó J., Ambroso S., Huvenne V. A., et al. (2022b). Local-scale feedbacks influencing cold-water coral growth and subsequent reef formation. Sci. Rep. 12, 20389. doi: 10.1038/s41598-022-24711-7
Corbera G., Lo Iacono C., Standish C. D., Anagnostou E., Titschack J., Katsamenis O., et al. (2021). Glacio-eustatic variations and sapropel events as main controls on the middle Pleistocene-Holocene evolution of the Cabliers coral mound province (W Mediterranean). Quat. Sci. Rev. 253, 106783. doi: 10.1016/j.quascirev.2020.106783
Corbera G., Lo Iacono C., Standish C. D., Gràcia E., Ranero C., Huvenne V. A., et al. (2022a). Glacial-aged development of the Tunisian coral mound province controlled by glacio-eustatic oscillations and changes in surface productivity. Mar. Geol. 446, 106772. doi: 10.1016/j.margeo.2022.106772
Corinaldesi C., Rastelli E., Canensi S., Tangherlini M., Danovaro R., Dell'Anno A. (2019). High rates of viral lysis stimulate prokaryotic turnover and c recycling in bathypelagic waters of a Ligurian canyon (Mediterranean Sea). Prog. Oceanogr. 171, 70–75. doi: 10.1016/j.pocean.2018.12.017
de Juan S., Lleonart J., and (2010). A conceptual framework for the protection of vulnerable habitats impacted by fishing activities in the Mediterranean high seas. Ocean Coast. Manage. 53, 717–723. doi: 10.1016/j.ocecoaman.2010.10.005
Delbono I., Ivaldi R., Pratellesi M., Fanelli E., Peirano A., Cocito S., et al. (2014). “Seafloor morphology: nature of the seabed and the cold water corals of the Levante canyon (eastern Ligurian Sea, NW Mediterranean),” in EGU General Assembly Conference Abstracts, Wien, Austria. 14732.
Dominguez-Carrió C., Riera J. L., Robert K., Zabala M., Requena S., Gori A., et al. (2022). Diversity, structure and spatial distribution of megabenthic communities in Cap de Creus continental shelf and submarine canyon (NW Mediterranean). Prog. Oceanogr. 208, 102877. doi: 10.1016/j.pocean.2022.102877
Dominguez-Carrió C., Sanchez-Vidal A., Estournel C., Corbera G., Riera J. L., Orejas C., et al. (2020). Seafloor litter sorting in different domains of Cap de Creus continental shelf and submarine canyon (NW Mediterranean Sea). Mar. Pollut. Bull. 161, 111744. doi: 10.1016/j.marpolbul.2020.111744
D’Onghia G., Calculli C., Capezzuto F., Carlucci R., Carluccio A., Grehan A., et al. (2017). Anthropogenic impact in the Santa Maria di Leuca cold-water coral province (Mediterranean Sea): observations and conservation straits. Deep Sea Res. Part II 145, 87–101. doi: 10.1016/j.dsr2.2016.02.012
D’Onghia G., Maiorano P., Carlucci R., Capezzuto F., Carluccio A., Tursi A., et al. (2012). Comparing deep-sea fish fauna between coral and non-coral “megahabitat” in the Santa Maria di Leuca cold-water coral province (Mediterranean Sea). PloS One 7, e44509. doi: 10.1371/journal.pone.0044509
Enrichetti F., Bavestrello G., Coppari M., Betti F., Bo M. (2018). Placogorgia coronata first documented record in Italian waters: use of trawl bycatch to unveil vulnerable deep-sea ecosystems. Aquat. Conserv.: Mar. Freshw. Ecosyst. 28, 1123–1138. doi: 10.1002/aqc.2930
Enrichetti F., Dominguez-Carrió C., Toma M., Bavestrello G., Betti F., Canese S., et al. (2019). Megabenthic communities of the Ligurian deep continental shelf and shelf break (NW Mediterranean Sea). PloS One 14, e0223949. doi: 10.1371/journal.pone.0223949
Enrichetti F., Dominguez-Carrió C., Toma M., Bavestrello G., Canese S., Bo M. (2020). Assessment and distribution of seafloor litter on the deep Ligurian continental shelf and shelf break (NW Mediterranean Sea). Mar. Poll. Bull. 151, 110872. doi: 10.1016/j.marpolbul.2019.110872
Evans J., Aguilar R., Alvarez H., Borg J. A., Garcia S., Knittweis L., et al. (2016). “Recent evidence that the deep sea around Malta is a biodiversity hotspot,” in Rapport du Congrès de la Commission Internationale pour l'Exploration Scientifique de la Mer Méditerranée (Kiel, Germany), Vol. 41. 463.
Fabri M. C., Bargain A., Pairaud I., Pedel L., Taupier-Letage I. (2017). Cold-water coral ecosystems in Cassidaigne canyon: an assessment of their environmental living conditions. Deep Sea Res. II 137, 436–453. doi: 10.1016/j.dsr2.2016.06.006
Fabri M. C., Dugornay O., de la Bernardie X., Guerin C., Sanchez P., Arnaubec A., et al. (2022). 3D-representations for studying deep-sea coral habitats in the Lacaze-Duthiers canyon, from geological settings to individual specimens. Deep Sea Res. I 187, 103831. doi: 10.1016/j.dsr.2022.103831
Fabri M., Pedel L., Beuck L., Galgani F., Hebbeln D., Freiwald A. (2014). Megafauna of vulnerable marine ecosystems in French Mediterranean submarine canyons: spatial distribution and anthropogenic impacts. Deep Sea Res. Part II 104, 184–207. doi: 10.1016/j.dsr2.2013.06.016
Fabri M. C., Vinha B., Allais A. G., Bouhier M. E., Dugornay O., Gaillot A., et al. (2019). Evaluating the ecological status of cold-water coral habitats using non-invasive methods: an example from Cassidaigne canyon, northwestern Mediterranean Sea. Prog. Oceanogr. 178, 102172. doi: 10.1016/j.pocean.2019.102172
Fanelli E., Delbono I., Ivaldi R., Pratellesi M., Cocito S., Peirano A. (2017). Cold-water coral Madrepora oculata in the eastern Ligurian Sea (NW Mediterranean): historical and recent findings. Aquat. Conserv.: Mar. Freshw. Ecosyst. 27, 965–975. doi: 10.1002/aqc.2751
FAO (2009). Report of the technical consultation on international guidelines for the management of deep-sea fisheries in the high seas, rome. 4–8 February and 25–29 august 2008. Food Agric. Organ. U.N. Fish. Aquacult. Rep. (Rome) 881, 86.
Fink H. G., Wienberg C., De Pol-Holz R., Hebbeln D., and (2015). Spatio-temporal distribution patterns of Mediterranean cold-water corals (Lophelia pertusa and Madrepora oculata) during the past 14,000 years. Deep Sea Res. Part I 103, 37–48. doi: 10.1016/j.dsr.2015.05.006
Freiwald A., Beuck L., Rüggerberg A., Taviani M., Hebblen D. (2009). The white coral community in the central Mediterranean Sea revealed by ROV surveys. Oceanography 22, 36–52. doi: 10.5670/oceanog.2009.06
Freiwald A., Fosså J. H., Grehan A., Koslow T., Roberts J. M. (2004). Cold-water coral reefs: out of sight-no longer out of mind. UNEP-WCMC (UK: Cambridge), 86.
Fusco N. (1967, 1968, 1972). “Il fondo del mare da sestri levante alla foce dell'Arno, da Capo di Noli a Sestri Levante, dal confine francese a capo di noli con annesse carte di pesca n 6, n. 7 e n. 8,” Ministero Marina mercantile, direzione generale della pesca marittima(Rome).
Gerigny O., Brun M., Fabri M. C., Tomasino C., Le Moigne M., Jadaud A., et al. (2019). Seafloor litter from the continental shelf and canyons in French Mediterranean water: distribution, typologies and trends. Mar. Pollut. Bull. 146, 653–666. doi: 10.1016/j.marpolbul.2019.07.030
Giusti M., Canese S., Fourt M., Bo M., Innocenti C., Goujard A., et al. (2019). Coral forests and derelict fishing gears in submarine canyon systems of the Ligurian Sea. Prog. Oceanogr. 178, 102186. doi: 10.1016/j.pocean.2019.102186
González-Irusta J. M., Cartes J. E., Punzón A., Díaz D., de Sola L. G., Serrano A. (2022). Mapping habitat loss in the deep-sea using current and past presences of Isidella elongata (Cnidaria: Alcyonacea). ICES J. Mar. Sci. 79, 1888–1901. doi: 10.1093/icesjms/fsac123
Gori A., Orejas C., Madurell T., Bramanti L., Martins M., Quintanilla E., et al. (2013). Bathymetrical distribution and size structure of cold-water coral populations in the Cap de Creus and Lacaze-Duthiers canyons (northwestern Mediterranean). Biogeosciences 10, 2049–2060. doi: 10.5194/bg-10-2049-2013
Grinyó J., Chevaldonné P., Schohn T., Le Bris N. (2021). Megabenthic assemblages on bathyal escarpments off the west Corsican margin (Western Mediterranean). Deep Sea Res. Part I 171, 103475. doi: 10.1016/j.dsr.2021.103475
Grinyó J., Lo Iacono C., Pierdomenico M., Conlon S., Corbera G., Gràcia E. (2020). Evidences of human impact on megabenthic assemblages of bathyal sediments in the Alboran Sea (western Mediterranean). Deep Sea Res. Part I 165, 103369. doi: 10.1016/j.dsr.2020.103369
Hajdas I., Ascough P., Garnett M. H., Fallon S., Pearson C. L., Quarta G., et al. (2021). Radiocarbon dating. Nat. Rev. Methods Primers 1, 62. doi: 10.1038/s43586-021-00058-7
Harris P. T., Whiteway T. (2011). Global distribution of large submarine canyons: geomorphic differences between active and passive continental margins. Mar. Geol. 285, 69–86. doi: 10.1016/j.margeo.2011.05.008
Heaton T., Köheler P., Butzin M., Bard E., Reimer R. W., Austin W. E. N., et al. (2021). Marine20 - the marine radiocarbon age calibration curve (0–55,000 cal BP). Radiocarbon 62, 779–820. doi: 10.1017/rdc.2020.68
Hebbeln D. (2019). “8 highly variable submarine landscapes in the Alboran Sea created by cold-water corals,” in Mediterranean Cold-water corals: past, present and future. Eds. Orejas C., Jiménez C. (Cham: Springer), 61–65. doi: 10.1007/978-3-319-91608-8_8
Hermoso V., Carvalho S. B., Giakoumi S., Goldsborough D., Katsanevakis S., Leontiou S., et al. (2022). The EU biodiversity strategy for 2030: opportunities and challenges on the path towards biodiversity recovery. Environ. Sci. Policy 127, 263–271. doi: 10.1016/j.envsci.2021.10.028
Huvenne V. A. I., Van Rooij D., De Mol B., Thierens M., O’Donnell R., Foubert A. (2009). Sediment dynamics and palaeo-environmental context at key stages in the Challenger cold-water coral mound formation: clues from sediment deposits at the mound base. Deep Sea Res. Part I 56, 2263–2280. doi: 10.1016/j.dsr.2009.08.003
Koulouri P., Mogias A., Gerovasileiou V. (2022). Ocean literacy across the Mediterranean Sea region in the era of 2030 agenda and the decade of ocean science for sustainable development, (2021-2030). Medit. Mar. Sci. 23, 266–269. doi: 10.12681/mms.30099
Lastras G., Canals M., Ballesteros E., Gili J. M., Sanchez-Vidal A. (2016). Cold-water corals and anthropogenic impacts in la Fonera submarine canyon head, northwestern Mediterranean Sea. PloS One 11, e0155729. doi: 10.1371/journal.pone.0155729
Lastras G., Sanchez-Vidal A., Canals M. (2019). “A cold-water coral habitat in la Fonera submarine canyon, northwestern Mediterranean Sea,” in Mediterranean Cold-water corals: past, present and future. Eds. Orejas C., Jiménez C. (Cham: Springer), 291–293. doi: 10.1007/978-3-319-91608-8_28
Le Houedec S., Mojtahid M., Ciobanu M., Jorry S. J., Bouhdayad F. Z., Guyonneau E., et al. (2021). Deglacial to Holocene environmental changes in the northern Ligurian Sea: the dual influence of regional climate variability and large-scale intermediate Mediterranean circulation. Palaeogeogr. Palaeoclimatol. Palaeoecol. 576, 110500. doi: 10.1016/j.palaeo.2021.110500
Lim A., Wheeler A. J., Conti L. (2021). Cold-water coral habitat mapping: trends and developments in acquisition and processing methods. Geosciences 11, 9. doi: 10.3390/geosciences11010009
Lo Iacono C., Gràcia E., Bartolomé R., Coiras E., Dañobeitia J. J., Acosta J. (2012). “Habitats of the Chella Bank , Eastern Alboran Sea (Western Mediterranean),” in Seafloor geomorphology as benthic habitat. Eds. Harris P. T., Baker E. K. (Elsevier), 681–690. doi: 10.1016/B978-0-12-385140-6.00049-9
Lo Iacono C., Gràcia E., Ranero C. R., Emelianov M., Huvenne V. A., Bartolomé R., et al. (2014). The West Melilla cold water coral mounds, Eastern Alboran Sea: morphological characterization and environmental context. Deep Sea Res. Part II 99, 316–326. doi: 10.1016/j.dsr2.2013.07.006
Lo Iacono C., Savini A., Huvenne V. A., Gràcia E. (2019). “15 habitat mapping of cold-water corals in the Mediterranean Sea,” in Mediterranean Cold-water corals: past, present and future. Eds. Orejas C., Jiménez C. (Cham: Springer), 157–171. doi: 10.1007/978-3-319-91608-8_15
Martorelli E., Petroni G., Chiocci F. L., Pantelleria Scientific Party (2011). Contourites offshore Pantelleria island (Sicily channel, Mediterranean sea): depositional, erosional and biogenic elements. Geo-Marine Lett. 31, 481–493. doi: 10.1007/s00367-011-0244-0
Marty J. C., Chiavérini J. (2010). Hydrological changes in the Ligurian Sea (NW Mediterranean, DYFAMED site) during 1995-2007 and biogeochemical consequences. Biogeosciences 7, 2117–2128. doi: 10.5194/bg-7-2117-2010
Mastrototaro F., d’Onghia G., Corriero G., Matarrese A., Maiorano P., Panetta P., et al. (2010). Biodiversity of the white coral bank off cape Santa Maria di Leuca (Mediterranean Sea): an update. Deep Sea Res. Part II 57, 412–430. doi: 10.1016/j.dsr2.2009.08.021
McCulloch M., Taviani M., Montagna P., Correa M. L., Remia A., Mortimer G. (2010). Proliferation and demise of deep-sea corals in the Mediterranean during the Younger Dryas. Earth Planet. Sci. Lett. 298, 143–152. doi: 10.1016/j.epsl.2010.07.036
Migeon S., Cattaneo A., Hassoun V., Larroque C., Corradi N., Fanucci F., et al. (2011). Morphology, distribution and origin of recent submarine landslides of the Ligurian margin (North-western Mediterranean): some insights into geohazard assessment. Mar. Geophys. Res. 32, 225–243. doi: 10.1007/s11001-011-9123-3
Millot C., Taupier-Letage I. (2005). “Circulation in the Mediterranean Sea,” in The Mediterranean sea. handbook of environmental chemistry, vol. 5K . Ed. Saliot A. (Berlin, Heidelberg: Springer), 29–66. doi: 10.1007/b107143
Miu I. V., Rozylowicz L., Popescu V. D., Anastasiu P. (2020). Identification of areas of very high biodiversity value to achieve the EU biodiversity strategy for 2030 key commitments. PeerJ 8, e10067. doi: 10.7717/peerj.10067
Moccia D., Cau A., Alvito A., Canese S., Cannas R., Bo M., et al. (2019). New sites expanding the “Sardinian cold-water coral province” extension: a new potential cold-water coral network? Aquat. Conserv. 29, 153–160. doi: 10.1002/aqc.2975
Montefalcone M., Tunesi L., Ouerghi A.. (2021). A review of the classification systems for marine benthic habitats and the new updated Barcelona Convention classification for the Mediterranean. Mar. Environ. Res. 169, 105387. doi: 10.1016/j.marenvres.2021.105387
Morri C., Bianchi C. N., Damiani V., Peirano A., Romeo G., Tunesi L. (1986). L'ambiente marino tra Punta della Chiappa e Sestri Levante (Mar Ligure): profilo ecotipologico e proposta di carta bionomica. Boll. Mus. Ist. Biol. Univ. Genova 52, 213–231.
Mytilineou C., Smith C. J., Anastasopoulou A., Papadopoulou K. N., Christidis G., Bekas P., et al. (2014). New cold-water coral occurrences in the Eastern Ionian Sea: results from experimental long line fishing. Deep Sea Res. Part II 99, 146–157. doi: 10.1016/j.dsr2.2013.07.007
Oceana (2014). Informe de síntesis para proceder a la elaboración del borrador del plan de gestión del LIC “Sur de almería-seco de los olivos”: Seco de los Olivos. proyecto LIFE + INDEMARES (LIFE07/NAT/E/00732). OCEANA – Istituto Espanol de Oceanografia (Madrid: Coordinación: Fundación Biodiversidad), 123.
Orejas C., Gori A., Lo Iacono C., Puig P., Gili J. M., Dale M. R. (2009). Cold-water corals in the Cap de Creus canyon, northwestern Mediterranean: spatial distribution, density and anthropogenic impact. Mar. Ecol. Prog. Ser. 397, 37–51. doi: 10.3354/meps08314
Orsi Relini L., Relini G. (1998). Seventeen instars of adult life in female Aristeus antennatus (Crustacea: decapoda: aristeidae). a new interpretation of life span and growth. J. Nat. Hist. 32, 1719–1734. doi: 10.1080/00222939800771231
Otero M. D. M., Marin P. (2019). “46 conservation of cold-water corals in the Mediterranean: current status and future prospects for improvement,” in Mediterranean Cold-water corals: past, present and future. Eds. Orejas C., Jiménez C. (Cham: Springer), 535–545. doi: 10.1007/978-3-319-91608-8_46
Otero M. D. M., Numa C., Bo M., Orejas C., Garrabou J., Cerrano C., et al. (2017). Overview of the conservation status of Mediterranean anthozoa (Malaga, Spain: International Union for Conservation of Nature), 73. doi: 10.2305/IUCN.CH.2017.RA.2.en
Pierdomenico M., Casalbore D., Chiocci F. L. (2020). The key role of canyons in funnelling litter to the deep sea: a study of the Gioia canyon (Southern Tyrrhenian Sea). Anthropocene 30, 100237. doi: 10.1016/j.ancene.2020.100237
Poulain P. M., Mauri E., Gerin R., Chiggiato J., Schroeder K., Griffa A., et al. (2020). On the dynamics in the southeastern Ligurian Sea in summer 2010. Cont. Shelf Res. 196, 104083. doi: 10.1016/j.csr.2020.104083
Prieur L., D'Ortenzio F., Taillandier V., Testor P. (2020). “Physical oceanography of the Ligurian Sea,” in The Mediterranean Sea in the era of global change 1: 30 years of multidisciplinary study of the Ligurian Sea. Eds. Migon C., Nival P., Sciandra A. (London: Wiley), 49–78. doi: 10.1002/9781119706960.ch3
Quarta G., Maruccio L., D’Elia M., Calcagnile L. (2021). Radiocarbon dating of marine samples: methodological aspects, applications and case studies. Water 13, 986. doi: 10.3390/w13070986
Reimer P. J., McCormac F. G. (2002). Marine radiocarbon reservoir corrections for the Mediterranean and Aegean seas. Radiocarbon 44, 159–166. doi: 10.1017/S0033822200064766
Relini G., Peirano A., Tunesi L., Associato-Lavagna C. S. P. (1986). Osservazioni sulle comunità dei fondi strascicabili del Mar Ligure centro-orientale. Boll. Mus. Ist. Biol. Univ. Genova 52, 139–116.
Relini-Orsi L. (1974). Un ambiente marino di grande interesse naturalistico: i fondi batiali al largo del promontorio di Portofino. Atti del IV Simposio Nazionale sulla Conservazione della Natura 1, 142–148.
Remia A., Taviani M. (2005). Shallow-buried pleistocene Madrepora-dominated coral mounds on a muddy continental slope, Tuscan archipelago, NE Tyrrhenian Sea. Facies 50, 419–425. doi: 10.1007/s10347-004-0029-2
Roberts J. M., Wheeler A. J., Freiwald A. (2006). Reefs of the deep: the biology and geology of cold-water coral ecosystems. Science 312, 543–547. doi: 10.1126/science.11198
Roberts J. M., Wheeler A., Freiwald A., Cairns S. (2009). Cold-water corals: the biology and geology of deep-Sea coral habitats (Cambridge: Cambridge University Press), 334. doi: 10.1017/CBO9780511581588
Robinson A. R., Leslie W. G., Theocharis A., Lascaratos A. (2001). “Mediterranean Sea circulation,” in Encyclopedia of ocean science. Eds. Steele J. H., Thorpe S. A., Turekian K. K. (San Diego: Academic), 1689–1705. doi: 10.1006/rwos.2001.0376
Romagnoli C., Casalbore D., Bortoluzzi G., Bosman A., Chiocci F. L., D’Oriano F., et al. (2013). Chapter 4 bathy-morphological setting of the Aeolian islands. Geol. Soc Lond. Mem 37, 27–36. doi: 10.1144/M37.4
Rossi L. (1958). Contributo allo studio della fauna di profondità vivente presso la Riviera Ligure di Levante. Doriana 2, 1–13.
Rossi S., Bramanti L., Gori A., Orejas C. (2017). Marine animal forests: the ecology of benthic biodiversity hotspots (Cham: Springer International Publishing), 1–1366. doi: 10.1007/978-3-319-17001-5
Rueda J. L., Gofas S., Aguilar R., Torriente A. D. L., García Raso J. E., Lo Iacono C., et al. (2021). “Benthic fauna of littoral and deep-sea habitats of the Alboran Sea: a hotspot of biodiversity,” in Alboran Sea-ecosystems and marine resources. Eds. Baez J. C., Vazquez J.-T., Caminas J. A., Idrissi M. M. (Cham: Springer), 285–358.
Rueda J. L., Urra J., Aguilar R., Angeletti L., Bo M., Garcia-Ruiz C., et al. (2019). “29 cold-water coral associated fauna in the Mediterranean Sea and adjacent areas,” in Mediterranean Cold-water corals: past, present and future. Eds. Orejas C., Jiménez. C. (Cham: Springer), 295–333. doi: 10.1007/978-3-319-91608-8_29
Ryabinin V., Barbière J., Haugan P., Kullenberg G., Smith N., McLean C., et al. (2019). The UN decade of ocean science for sustainable development. Front. Mar. Sci. 6. doi: 10.3389/fmars.2019.00470
Sartoretto S., Zibrowius H. (2018). Note on new records of living Scleractinia and Gorgonaria between 1700 and 2200 m depth in the western Mediterranean Sea. Mar. Biod. 48, 689–694. doi: 10.1007/s12526-017-0829-6
Savini A., Corselli C. (2010). High-resolution bathymetry and acoustic geophysical data from Santa Maria di Leuca cold water coral province (Northern Ionian Sea-Apulian continental slope). Deep Sea Res. Part II 57, 326–344. doi: 10.1016/j.dsr2.2009.08.014
Savini A., Vertino A., Marchese F., Beuck L., Freiwald A. (2014). Mapping cold-water coral habitats at different scales within the northern Ionian Sea (Central Mediterranean): an assessment of coral coverage and associated vulnerability. PloS One 9, e87108. doi: 10.1371/journal.pone.0087108
Schembri P. J., Dimech M., Camilleri M., Page R. (2007). Living deep-water Lophelia and Madrepora corals in Maltese waters (Strait of Sicily, Mediterranean Sea). Cah. Biol. Mar. 48, 77–83.
Siani G., Paterne M., Arnold M., Bard E., Métivier B., Tisnerat N., et al. (2000). Radiocarbon reservoir ages in the Mediterranean Sea and Black Sea. Radiocarbon 42, 271–280. doi: 10.1017/S0033822200059075
Siani G., Paterne M., Michel E., Sulpizio R., Sbrana A., Arnold M., et al. (2011). Mediterranean Sea Surface radiocarbon reservoir age changes since the last glacial maximum. Science 294, 1917–1920. doi: 10.1126/science.1063649
Soulet Q., Migeon S., Gorini C., Rubino J. L., Raisson F., Bourges P. (2016). Erosional versus aggradational canyons along a tectonically-active margin: the northeastern Ligurian margin (western Mediterranean Sea). Mar. Geol. 382, 17–36. doi: 10.1016/j.margeo.2016.09.015
SPA/RAC–UN Environment/MAP (2019) Updated classification of benthic marine habitat types for the Mediterranean region. Available at: https://www.rac-spa.org/sites/default/files/doc_fsd/habitats_list_en.pdf.
Stalder C., El Kateb A., Vertino A., Rüggeberg A., Camozzi O., Pirkenseer C. M., et al. (2018). Large-Scale paleoceanographic variations in the western Mediterranean Sea during the last 34,000 years: from enhanced cold-water coral growth to declining mounds. Mar. Micropaleontol. 143, 46–62. doi: 10.1016/j.marmicro.2018.07.007
Stalder C., Vertino A., Rosso A., Rüggeberg A., Pirkenseer C., Spangenberg J. E., et al. (2015). Microfossils, a key to unravel cold-water carbonate mound evolution through time: evidence from the eastern Alboran Sea. PloS One 10, e0140223. doi: 10.1371/journal.pone.0140223
Stuiver M., Polach H. A. (1977). Discussion reporting of 14C data. Radiocarbon 19, 355–363. doi: 10.1017/S0033822200003672
Taviani M., Angeletti L., Antolini B., Ceregato A., Froglia C., Lopez Correa M., et al. (2011). Geo-biology of Mediterranean deep-water coral ecosystems. Mar. Res. CNR 6, 705–719.
Taviani M., Angeletti L., Canese S., Cannas R., Cardone F., Cau A., et al. (2017). The “Sardinian cold-water coral province” in the context of the Mediterranean coral ecosystems. Deep Sea Res. Part II 145, 61–78. doi: 10.1016/j.dsr2.2015.12.008
Taviani M., Angeletti L., Cardone F., Montagna P., Danovaro R. (2019b). A unique and threatened deep water coral-bivalve biotope new to the Mediterranean Sea offshore the Naples megalopolis. Sci. Rep. 9, 1–12. doi: 10.1038/s41598-019-39655-8
Taviani M., Vertino A., Angeletti L., Montagna P., Remia A. (2019a). “Paleoecology of Mediterranean cold-water corals,” in Mediterranean Cold-water corals: past, present and future. Eds. Orejas C., Jiménez C. (Cham: Springer), 15–30. doi: 10.1007/978-3-319-91608-8_2
Tubau X., Canals M., Lastras G., Rayo X., Rivera J., Amblas D. (2015). Marine litter on the floor of deep submarine canyons of the northwestern Mediterranean Sea: the role of hydrodynamic processes. Prog. Oceanogr. 134, 379–403. doi: 10.1016/j.pocean.2015.03.013
Tudela S., Sardà F., Maynou F., Demestre M. (2003). Influence of submarine canyons on the distribution of the deep-water shrimp, Aristeus antennatus (Risso 1816) in the NW Mediterranean. Crustaceana 76, 217–225. doi: 10.1163/156854003321824567
Tunesi L., Diviacco G. (1997). “Observations by submersible on the bottoms off shore Portofino promontory (Ligurian Sea),” in Atti del 12° congresso AIOL. Ed. Piccazzo M. (Italy: Genova), 61–74.
Tunesi L., Diviacco G., Mo G. (2001). “Observations by submersible on the biocoenosis of the deep-sea corals off Portofino promontory (northwestern Mediterranean Sea),” in Proceedings of the first international symposium on deep-sea corals. Ed. Willison J. H. M., et al (Canada: Halifax), 76–87.
Tursi A., Mastrototaro F., Matarrese A., Maiorano P., D'Onghia G. (2004). Biodiversity of the white coral reefs in the Ionian Sea (Central Mediterranean). Chem. Ecol. 20 (sup1), 107–116. doi: 10.1080/02757540310001629170
van Oevelen D., Duineveld G., Lavaleye M., Mienis F., Soetaert K., Heip C. H. R. (2009). The cold-water coral community as hotspot of carbon cycling on continental margins: a foodweb analysis from Rockall Bank (northeast Atlantic). Limn. Ocean. 54, 1829–1844. doi: 10.4319/lo.2009.54.6.1829
Vertino A., Corselli C. (2019). “6 did quaternary climate fluctuations affect Mediterranean deep-Sea coral communities?,” in Mediterranean Cold-water corals: past, present and future. Eds. Orejas C., Jiménez C. (Cham: Springer), 51–55. doi: 10.1007/978-3-319-91608-8_6
Vertino A., Stolarski J., Bosellini F. R., Taviani M. (2014). “Mediterranean Corals through time: from Miocene to present,” in The Mediterranean Sea. Eds. Goffredo S., Dubinsky Z. (Dordrecht: Springer), 257–274. doi: 10.1007/978-94-007-6704-1_14
Vertino A., Taviani M., Corselli C. (2019). “9 spatio-temporal distribution of Mediterranean cold-water corals,” in Mediterranean Cold-water corals: past, present and future. Eds. Orejas C., Jiménez C. (Cham: Springer), 67–83. doi: 10.1007/978-3-319-91608-8_9
Wang H., Titschack J., Wienberg C., Korpanty C., Hebbeln D. (2021). The importance of ecological accommodation space and sediment supply for cold-water coral mound formation, a case study from the western Mediterranean Sea. Front. Mar. Sci. 8. doi: 10.3389/fmars.2021.760909
Wienberg C. (2019). “7 a deglacial cold-water coral boom in the alborán Sea: from coral mounds and species dominance,” in Mediterranean Cold-water corals: past, present and future. Eds. Orejas C., Jiménez C. (Cham: Springer), 57–60. doi: 10.1007/978-3-319-91608-8_7
Wienberg C., Krengel T., Frank N., Wang H., Van Rooij D., Hebbeln D. (2022). Cold-water coral mounds in the western Mediterranean Sea: new insights into their initiation and development since the mid-Pleistocene in response to changes of African hydroclimate. Quat. Sci. Rev. 293, 107723. doi: 10.1016/j.quascirev.2022.107723
Keywords: Mediterranean Sea, Ligurian Sea, scleractinian frameworks, remotely operated vehicles, mapping, fishing impact, vulnerability
Citation: Bo M, Enrichetti F, Betti F, Gay G, Quarta G, Calcagnile L and Bavestrello G (2023) The cold-water coral province of the eastern Ligurian Sea (NW Mediterranean Sea): historical and novel evidences. Front. Mar. Sci. 10:1114417. doi: 10.3389/fmars.2023.1114417
Received: 02 December 2022; Accepted: 27 June 2023;
Published: 25 July 2023.
Edited by:
Lorenzo Angeletti, IRBIM-CNR, ItalyReviewed by:
Claudia Wienberg, University of Bremen, GermanyMarie-Claire Fabri, Ifremer Centre de Méditerranée, France
Copyright © 2023 Bo, Enrichetti, Betti, Gay, Quarta, Calcagnile and Bavestrello. This is an open-access article distributed under the terms of the Creative Commons Attribution License (CC BY). The use, distribution or reproduction in other forums is permitted, provided the original author(s) and the copyright owner(s) are credited and that the original publication in this journal is cited, in accordance with accepted academic practice. No use, distribution or reproduction is permitted which does not comply with these terms.
*Correspondence: Marzia Bo, bWFyemlhLmJvQHVuaWdlLml0
†ORCID: Marzia Bo, orcid.org/0000-0001-8400-6722
Francesco Enrichetti, orcid.org/0000-0003-3256-4540
Federico Betti, orcid.org/0000-0002-5492-2655
Gianluca Quarta, orcid.org/0000-0002-3648-1411
Lucio Calcagnile, orcid.org/0000-0003-3572-6154
Giorgio Bavestrello, orcid.org/0000-0002-5428-2344