- 1Laboratory for Marine Fisheries Science and Food Production Processes, Pilot National Laboratory for Marine Science and Technology (Qingdao), Key Laboratory of Maricultural Organism Disease Control, Ministry of Agriculture and Rural Affairs, Qingdao Key Laboratory of Mariculture Epidemiology and Biosecurity, Yellow Sea Fisheries Research Institute, Chinese Academy of Fishery Sciences, Qingdao, China
- 2Shanghai Ocean University, Shanghai, China
- 3Xinjiang Uygur Autonomous Region Institute of Fisheries Science, Urumqi, China
- 4College of Aquaculture, Tianjin Agricultural University, Tianjin, China
- 5Network of Aquaculture Centres in Asia-Pacific, Bangkok, Thailand
Infection with Decapod iridescent virus 1 (iDIV1), an important emerging disease of shrimps and crabs, has been included in the Quarterly Aquatic Animal Disease Report (QAAD) by the Network of Aquaculture Centres in Asia-Pacific (NACA) and listed by the World Organization for Animal Health (WOAH). China has classified iDIV1 as a Class II animal pandemic disease. In the present study, to determine the susceptibility of Penaeus japonicus to Decapod iridescent virus 1 (DIV1), healthy kuruma shrimp were artificially infected with DIV1 (isolate SHIV 20141215) by per os (the pathway that mimics natural transmission) and intramuscular injection (invasive pathway). The infected P. japonicus showed clinical signs such as anorexia, retardation, evident reddish body, swollen and whitish lymphoid organs, and mortalities of almost 100%. Real-time PCR showed that all the challenged individuals by per os or intramuscular routes were DIV1-positive with an average virus load between 10(9.09 ± 0.58) and 10(8.94 ± 0.45) copies/μg-DNA, respectively. Histological examination revealed karyopyknosis, and eosinophilic inclusions and minute basophilic stains were combined in lymphoid organs, hematopoietic tissue and gills of diseased individuals. In addition, lymphoid organs showed disorganization of the tubule matrix. In situ DIG-labeling loop-mediated isothermal amplification (ISDL) also demonstrated the presence of DIV1 signals existed in lymphoid organs, hemopoietic tissue, gills, epithelial tissue, hepatopancreas and muscle. Ultrathin sections examined using transmission electron microscopy (TEM) revealed the presence of DIV1 virions, the virogenic stroma, and the nucleocapsid production process in infected cells. In addition, pathogen surveillance of cultured samples showed that the DIV1 detection rate of farmed P. japonicus samples from five coastal provinces in China was 5.3% (9/157) in 2022. The results mentioned above support that P. japonicus is a newly confirmed susceptible host for DIV1, enhancing the pathogen ecological understanding of pathogens and giving more support for developing DIV1 preventive and control strategies.
Introduction
Large icosahedral viruses belonging to the Iridoviridae family have diameters between 120 and 350 nm, and some of them can reach 350 nm (e.g. genus Lymphocystivirus) (King et al., 2011). This family of viruses is distinguished by the broad range of vertebrate and invertebrate hosts it can infect, including fish, amphibians, and reptiles as well as invertebrates (such as insects) (King et al., 2011). Based on the particle size, host range, and the presence of methyltransferase, International Committee on Taxonomy of Viruses (ICTV) classifies the Iridoviridae family as Iridovirus, Chloriridovirus, Daphniairidovirus, Decapodiridovirus, Ranavirus, Lymphocystivirus, and Megalocytivirus, a total of 7 genera (ICTV, 2021). In farmed shrimps and crabs, a newly discovered virus called Decapod iridescent virus 1 (DIV1) has resulted in significant economic losses (Xu et al., 2016; Qiu et al., 2017; Qiu et al., 2019a; Qiu et al., 2021; Qiu et al., 2022) and was designated as the only species of the genus Decapodiridovirus within the Iridoviridae family by the ICTV (ICTV, 2019). DIV1 is a double-stranded DNA (dsDNA) virus with a 166 kbp-long genome (Li et al., 2017; Qiu et al., 2018b). It has two original isolations, Cherax quadricarinatus iridovirus (isolate CQIV CN01) and Shrimp hemocyte iridescent virus (isolate SHIV 20141215) (ICTV, 2019). According to the current database, only these two original isolates have completed whole-genome sequencing with very high sequence similarity. Previous studies have confirmed that DIV1 susceptible hosts meeting the World Organization for Animal Health (WOAH) criteria (WOAH, 2022) include P. vannamei, P. monodon, Macrobrachium rosenbergii, M. nipponense, Exopalaemon carinicauda, Procambarus clarkii, Cherax quadricarinatus, Portunus trituberculatus (Xu et al., 2016; Qiu et al., 2017; Qiu et al., 2019a; Chen et al., 2019; Srisala et al., 2021; Qiu et al., 2022). Eriocheir sinensis and Pachygrapsus crassipes, two species of crab, were intramuscularly injected with DIV1 in an invasive experimental challenge (Pan et al., 2017), rendering them unable to be designated as susceptible species just yet. P. chinensis, M. superbum, Nereis succinea, Pomacea canaliculata, Plexippus paykulli, and a few other cladocera can all have DIV1 detected by PCR (Qiu et al., 2017; Qiu et al., 2018c; Qiu et al., 2019a; Qiu et al., 2019b; Qiu et al., 2021), however the infection status has not been determined at the histology level. Therefore, more research is required to determine whether these species are susceptible hosts, vectors, or carriers.
Crustaceans provide nutritious and high-quality protein, unsaturated fatty acids, vitamin D, and other micronutrients for human beings. With the increase in market demand, the scale of shrimp and crab farming is expanding year by year. However, due to the lack of biosecurity awareness in the rapid rise of intensive aquaculture, disease has become one of the main factors restricting the increase of shrimp and crab production. The kuruma shrimp, Penaeus japonicus, is one of the most essential cultured and captured shrimps in the world and is farmed in Southeast Asian nations such as China, Japan, Korea, Australia, and others (Hewitt and Duncan, 2001; Ren et al., 2020; Cao et al., 2022). Due to its excellent desiccation resistance, tasty flavor, and abundant nutritional value, it is regarded as one of the most commercially important species of the Penaeidae family (Zhang et al., 2022). In 2021, China’s annual P. japonicus aquaculture production totaled 44,548 tons (Bureau of Fisheries et al., 2022). DIV1 was detected in samples of P. japonicus raised in China, according to target surveillance, in 2017 and 2018 (Qiu et al., 2018c; Qiu et al., 2019b). He et al. (2021) infected healthy P. japonicus with DIV1 by intramuscular injection as an invasive challenge. DIV1 had an inhibitory effect on the immune enzyme activity of shrimp, according to the enzyme activity study and transcriptomics analysis, and HSP70, C-type lectins, and caspase may be crucial defenses against DIV1 infection (He et al., 2021). The intramuscular injection, however, does not satisfy the WOAH requirements for listing species susceptible to a pathogen because it is not a technique that mimics natural transmission channels. Therefore, it is necessary to confirm further that P. japonicus is susceptible to DIV1.
It is necessary to identify a pathogen’s susceptible hosts for international trade and disease control (Qiu et al., 2022). Therefore, healthy kuruma shrimp were experimentally infected using invasive and non-invasive experimental techniques to test P. japonicus’ susceptibility to DIV1. In this investigation, clinical observation, pathogen detection, and histology were used to examine the infection status of the individuals. Additionally, we conducted the DIV1 analysis on the cultivated P. japonicus in a few Chinese coastal provinces.
Materials and methods
Shrimp
P. japonicus individuals from a farm in Jiaonan, Qingdao, Shandong Province of China were utilized for the experimental infection of DIV1. They were about 9 - 10 cm in body length and had been cultured for 80 days. The shrimp were healthy and showed good activity.
Prior to the challenge experiment, the samples of shrimp were tested by real-time PCR and confirmed to be free of white spot syndrome virus (WSSV), infectious hypodermal and hematopoietic necrosis virus (IHHNV), acute hepatopancreas necrosis disease-causing Vibrio (VAHPND), Enterocytozoon hepatopenaei (EHP) and DIV1. All detection techniques were created from previous research (Liu et al., 2018; Qiu et al., 2020) or were advised by the World Organization for Animal Health (WOAH) (WOAH, 2021).
Preparing the DIV1 inoculum
This study prepared DIV1 inoculum from DIV1-infected (isolate SHIV 20141215) P. vannamei tissues previously, which tested DIV1 positive and common shrimp pathogens negative by real-time PCR (Chen et al., 2019). PPB-Tris was used to homogenize shell-off cephalothoraxes of DIV1-infected P. vannamei (Huang et al., 1999). A 0.45 μm filter was used to remove the supernatant after the homogenate had been centrifuged at 9,100 g for 10 min. Real-time PCR was utilized to determine the viral load of the filtered supernatant’s template (Qiu et al., 2020). Prior to usage, the inoculum was kept at -80°C.
The experimental infection of P. japonicus with DIV1
The operation of the experimental infection was similar to that of the host susceptibility studies previously published (Chen et al., 2019; Qiu et al., 2022), and was partially adjusted according to the characteristics of P. japonicus. A total of 200 individuals of P. japonicus were raised in 90-L plastic tanks (61×42.5×35 cm) filled with 45 L of sand-filtered seawater, which had a salinity of around 32 and a temperature of 23–24°C. Throughout the experiment, 90% of the seawater in plastic containers was changed daily and continually aerated. Glass windows and a natural light/dark cycle were provided in the wet laboratory. The shrimp were temporarily raised for 5 days while fed daily with minced oyster meat. Then 180 individuals were randomly divided into four groups, including the per os (PO), intramuscular injection (IM), intramuscular injection control (CIM), and per os control (CPO) groups (each group contained three 90-L plastic tanks and 15 individuals per tank). Each individual in the IM group received an injection of 50 μL of the DIV1 inoculum, which had been diluted to 103 copies/L using PPB-His (376.07 mM NaCl, 6.32 mM K2SO4, 6.4 mM MgSO4, 14.41 mM CaCl2, and 26.10 mM histidine hydrochloride, pH 6.5) (Huang et al., 1999). PPB-His was administered in the same amount to the CIM group. After a 24-hour starving period, shrimp in the PO group were fed minced DIV1-infected P. vannamei tissues mixed with equal amounts of minced oyster meat at 10% of their total body weight, while the CPO group was fed minced oyster meat at 10% of their total body weight in the same way.
During the experiment, the survival number of each group was counted every day, then used GraphPad Prism 8 to make the survival curve. The dying individuals were dissected and sampled daily during the experiment, and all surviving individuals were dissected and sampled after the experiment.
DNA extraction and detection of DIV1 by real-time PCR
In the artificial infection experiment, total DNA was extracted using the TIANamp Marine Animal DNA Kit (TIANGEN Biotech, Beijing, China) from 30 mg of each P. japonicus’s gills and hepatopancreas mixture. Utilizing the Nanodrop 2000c, the extracted DNA’s quality and concentration were assessed (Thermo Fisher Scientific). Real-time PCR was utilized to examine DNA samples as templates for quantitative detection (Qiu et al., 2020). To determine the geometric averages and standard deviations for each group, copies of DIV1 per μg of total DNA (copies/g-DNA) were converted to respective logarithms. The viral load data of the IM group and the PO group were used for one-way analysis of variance using Excel (Microsoft 365MSO).
Histopathological analysis
The cephalothoraxes of P. japonicus, sampled during the challenge experiment, were treated for 24 hours with Davison’s AFA fixative (DAFA) before being switched to 70% ethanol (Bell and Lightner, 1988). According to Bell & Lightner’s instructions, paraffin-embedded sections were produced and stained with hematoxylin and eosin (H&E) solutions (1988).
In situ DIG-labeling loop-mediated isothermal amplification (ISDL)
The ISDL assays targeting the gene of the second largest subunit of DNA-directed RNA polymerase II of DIV1 followed the method published by Chen et al., 2019. Paraffin sections were dewaxed and rehydrated according to previous literature (Bell and Lightner, 1988; Lightner, 1996). Rehydrated slides were added with ddH2O and denatured on a 100°C heating block for 2 min, then subsequently placed in a wet box. Total of 150 µL DIG-LAMP mixture, which has the same constituents as described in in Chen et al., 2019, were added dropwise to each slide. The slides were horizontally incubated at 65°C for 60 min, followed by 85°C for 5 min. Subsequent steps were performed in accordance with the post-hybridization steps of a normal in situ hybridization (Lightner, 1996). Tissue sections of healthy P. japonicus were used as the control.
TEM
For TEM examination, tissue samples from P. japonicus samples were cut into ultrathin sections. Minute tissue fragments of the lymphoid organ, nervous tissue, gills, heart, and stomach in less than 1 mm3 of P. japonicus were preserved for 24 hours at 4°C using TEM fixative, which contains 2% paraformaldehyde, 2.5% glutaraldehyde, 160 mM NaCl, and 4 mM CaCl2 in 200 mM PBS (pH 7.2). The tissues were secondarily fixed with 1% osmium tetroxide for 2 hours prior to ultrathin sectioning, after which they were embedded in Spurr’s resin and stained with uranyl acetate and lead citrate. In the Medical College of Qingdao University, ultrathin sections were placed on collodion-coated grids and studied using a JEOL JEM-1200 electron microscope (Jeol Solutions for Innovation, Japan) and HT7700 (Hitachi, Japan) operating at 80 to 100 kV.
Detection of DIV1 in clinical P. japonicus samples in some coastal provinces of China
A total of 153 farmed P. japonicus samples from 17 batches were gathered from five provinces of China in 2022. DNA was extracted from about 30 mg gills and hepatopancreas mixture of each shrimp, followed by the test of DIV1 using two real-time PCR methods targeting the fragment of MCP and ATPase genes, respectively (Qiu et al., 2018a; Qiu et al., 2020).
Results
Clinical signs of artificially infected P. japonicus
The infection experiment lasted for 10 days post-infection (dpi) of P. japonicus. The P. japonicus in the IM group was the earliest to show clinical signs of anorexia, retardation, and reddish body, and all died within 3 dpi (Figure 1). In the PO group, the overall onset was relatively slow. At 3 dpi, P. japonicus began to show clinical signs like those in the IM group, and at 5 dpi, significant death began to occur, and at 6 dpi, most of the individuals died. For the CIM and CPO groups, within 10 dpi, there was no large number of deaths except for accidental factors (Figure 1).
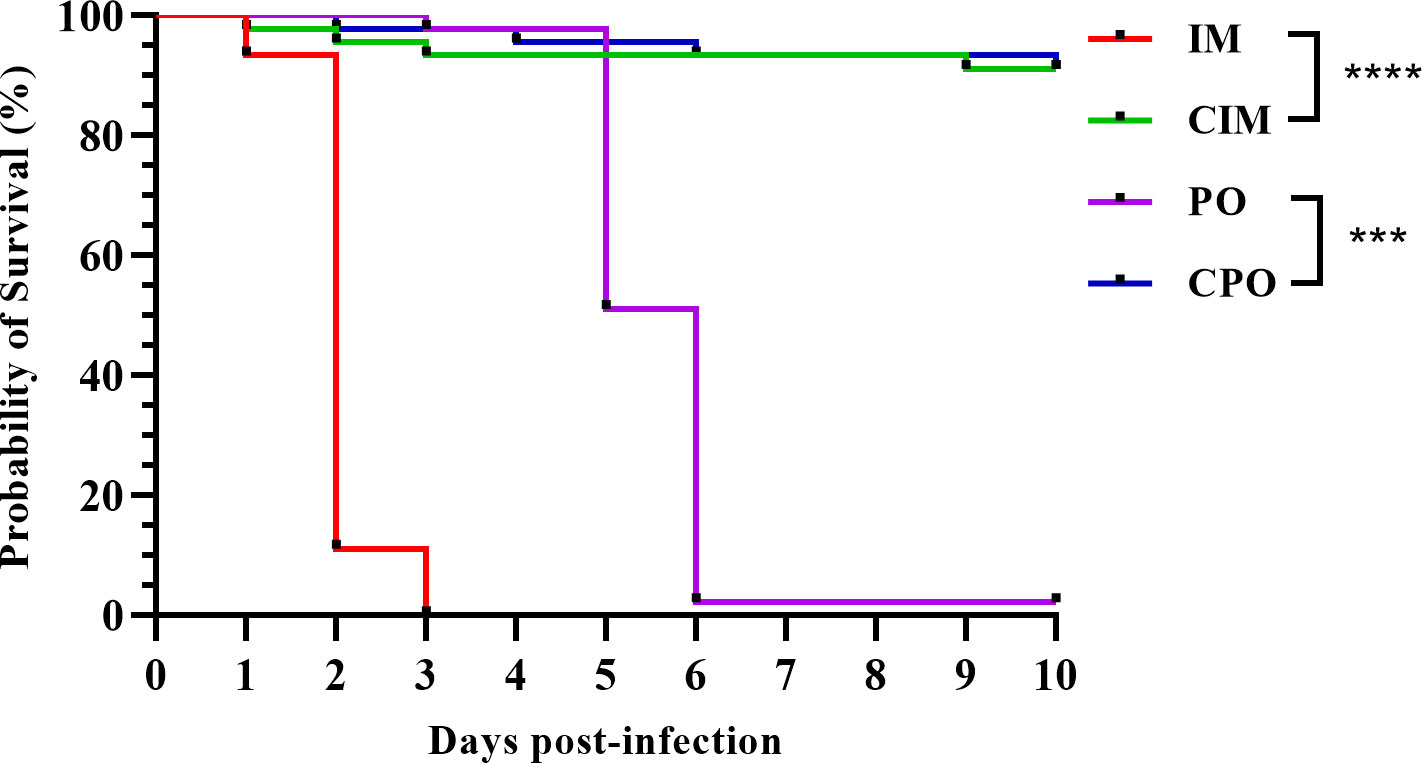
Figure 1 Survival curve of P. japonicus in each group of infection experiment; IM: intramuscular injection challenge group; CIM: intramuscular injection control group; PO: per os challenge group; CPO: per os control group; ***: P value was less than 0.001; ****: P value was less than 0.0001.
By comparing the clinical signs between the challenge groups and the control groups, it was found that in addition to anorexia and retardation, the most obvious appearance sign of the infected groups was the reddish body, and almost all infected individuals showed obvious red body color (Figures 2A, D). When central longitudinal dissection was performed, the infected individuals showed a swollen and whitish lymphoid organ in front of the hepatopancreas (Figures 2B, C), whereas this was not observed in control individuals (Figures 2E, F).
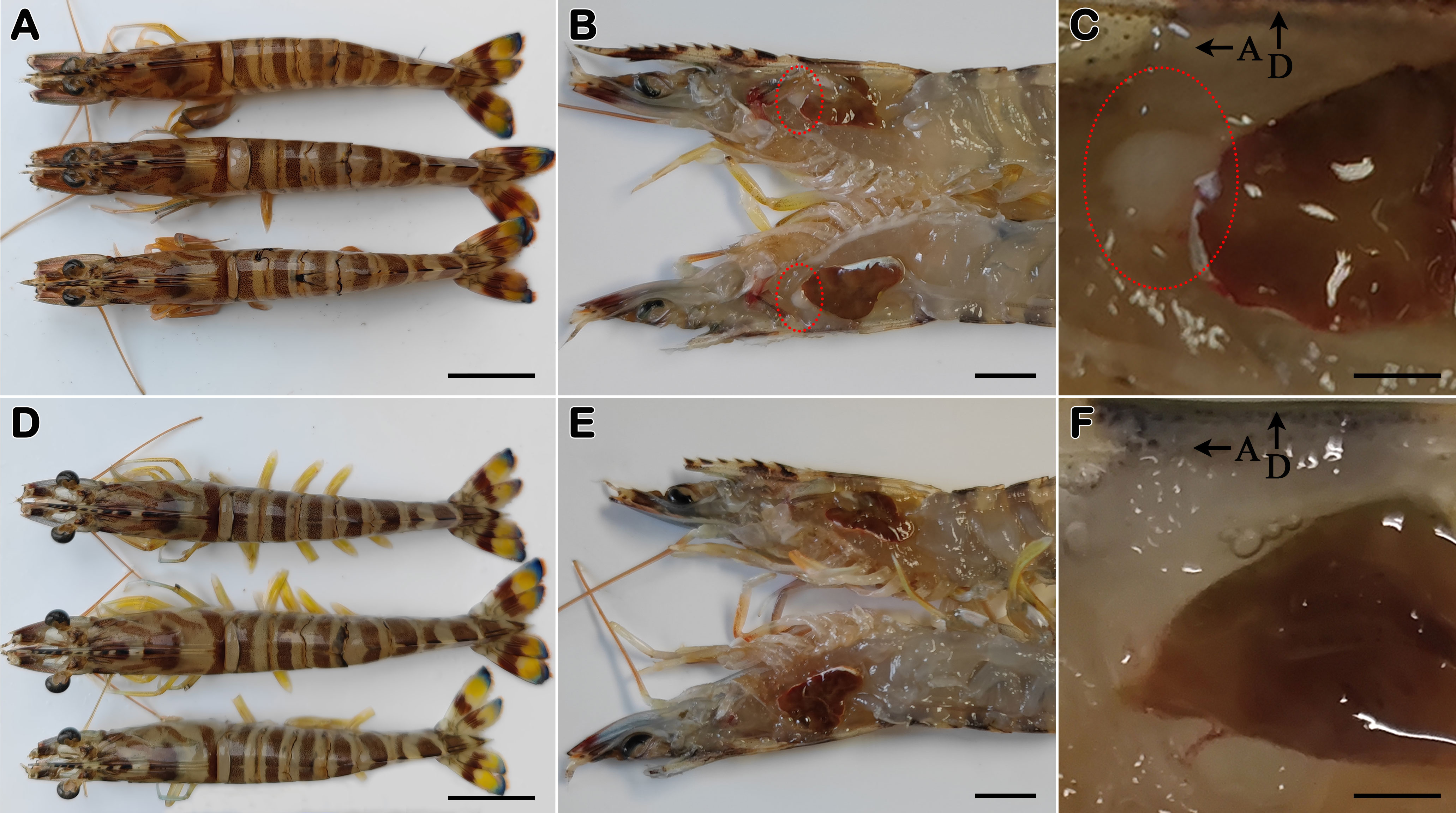
Figure 2 Clinical signs of Penaeus japonicus. (A–C) External appearance, central longitudinal section and close view of central longitudinal section of diseased individuals (from PO and IM groups); (D–F) External appearance, central longitudinal section and close view of central longitudinal section of healthy individuals (from CPO and CIM groups). Red circle: whitish lymphoid organ; A: toward the anterior region of the shrimp; D: toward the dorsal region of the shrimp. Bar, 20 mm (A, D); 10 mm (B, E); 2 mm (C, F).
DIV1 detection of experimental P. japonicus
A total of 28 DNA samples (seven individuals were randomly selected for each group of IM, CIM, PO, and CPO) were extracted and subjected to real-time PCR. With geometric average DIV1 loads of 10(9.09 ± 0.58) and 10(8.94 ± 0.45) copies/μg-DNA, respectively, all samples in the IM and PO groups were tested to be DIV1 positive (Table 1). All samples in the control group were negative for DIV1 (Table 1). One-way analysis of variance showed no significant difference in viral load between the IM and PO groups.
Histopathological examination
In the lymphoid organ, hematopoietic tissue, and gills of the moribund P. japonicus samples (from PO and IM groups), histopathological analysis revealed karyopyknosis and eosinophilic inclusions intermingled with minute basophilic stains (Figures 3A, C, E). In addition to the above unique lesions, lymphoid organs show disorganization of the lymphoid tubule matrix accompanied by abnormal morphology of the nuclei plus the presence of karyorrhectic and pyknotic nuclei. The muscle, hepatopancreas, and epithelial tissue did not exhibit any notable histological alterations (not shown). After H&E staining, the tissue samples from healthy individuals (from CPO and CIM groups) were shown as normal under the microscope (Figures 3B, D, F).
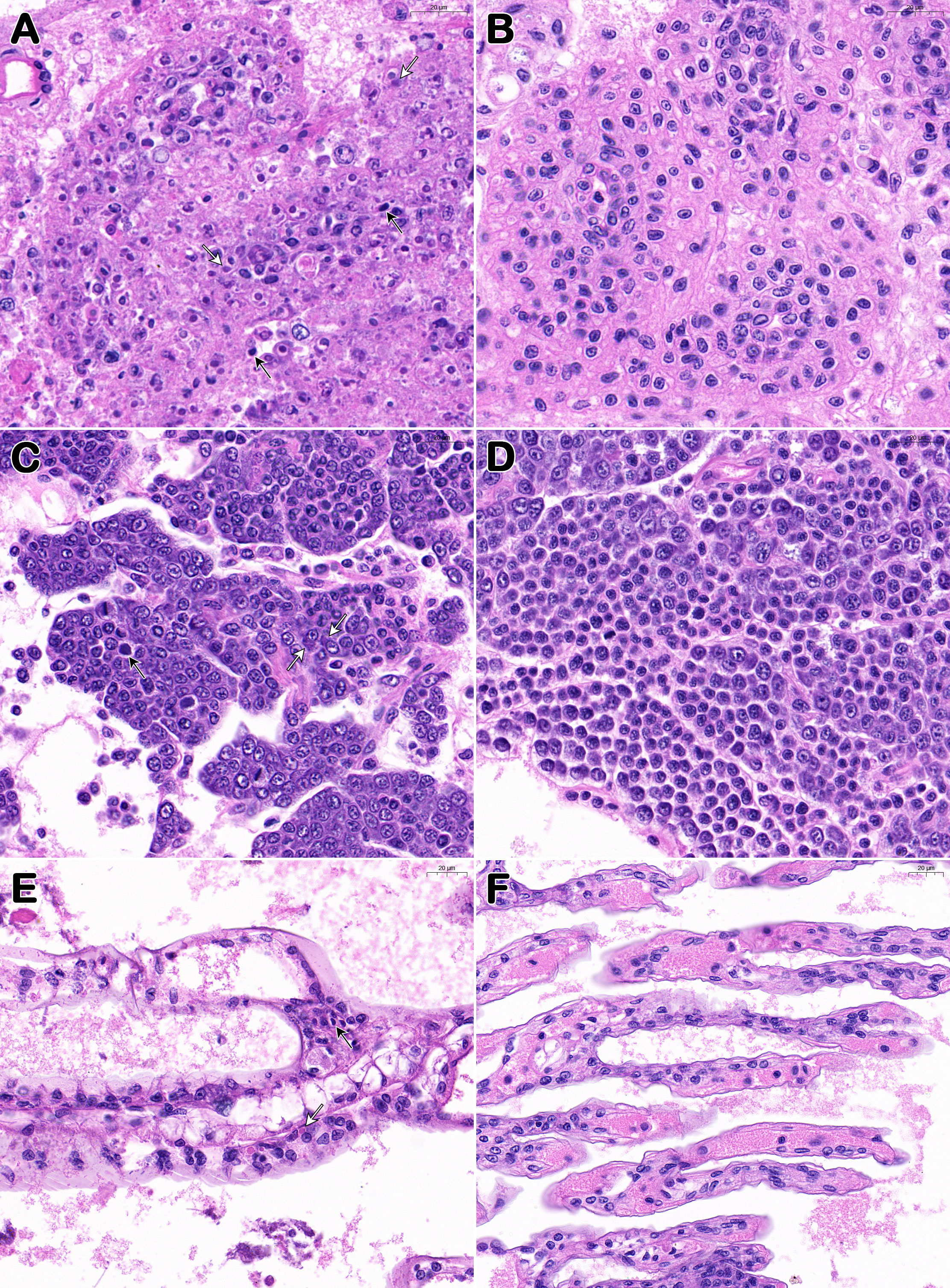
Figure 3 Histopathological features of Davison’s AFA fixative fixed P. japonicus. (A, C, E) Lymphoid organ, hemopoietic tissue, and gills of diseased individuals (from PO and IM groups); (B, D, F) Lymphoid organ, hemopoietic tissue, and gills of healthy individuals (from CPO and CIM groups). White arrows show the eosinophilic inclusions and black arrows show the karyopyknotic nuclei. Bar, 20 μm (A–F).
ISDL
For ISDL, a LAMP system was applied to in situ amplification to produce DIG-labeled products and subsequently detected with anti-DIG antibody on histological sections. Consistent with the histopathological findings, ISDL results of diseased P. japonicus (from PO and IM groups) revealed the presence of positive blue signals in lymphoid organs, hemopoietic tissue and gills(Figures 4A, C, E). These positive signals are concentrated and scattered, both intracellular and extracellular. In addition, blue signals were also observed in epithelial tissue, hepatopancreas, and muscle, and most of these signals were concentrated in the nucleus (Figures 4G, I, K). In contrast, there was no positive signal in the tissues above from healthy P. japonicus (from CPO and CIM groups) (Figures 4B, D, F, H, J, L).
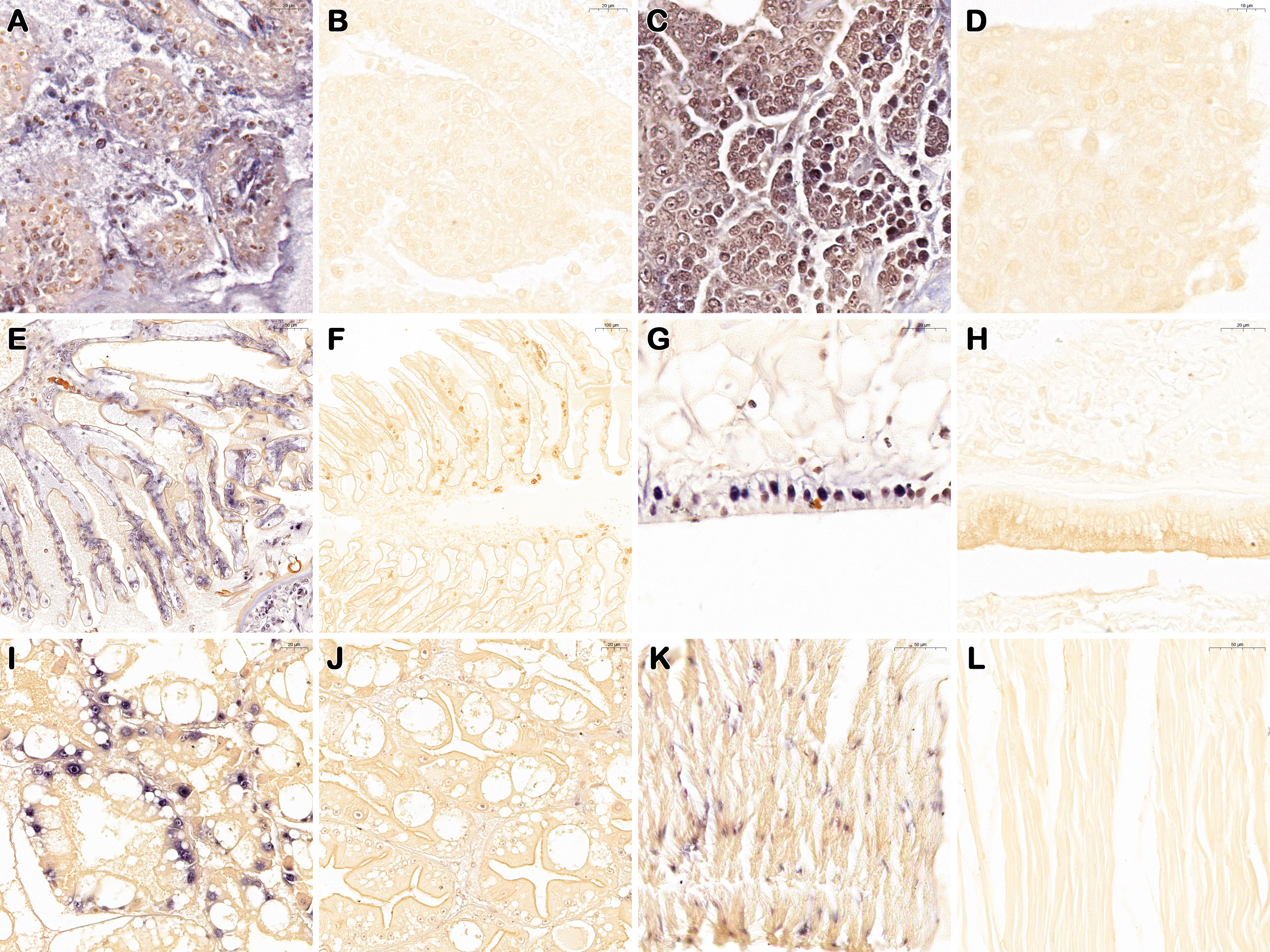
Figure 4 In situ DIG-labeling loop-mediated isothermal amplification targeting DIV1 on histological sections of P. japonicus. Lymphoid organ, hemopoietic tissue, gills, epithelial tissue, hepatopancreas, and muscle of DIV1 infected individuals (from PO and IM groups) (A, C, E, G, I, K); Lymphoid organ, hemopoietic tissue, gills, epithelial tissue, hepatopancreas, and muscle of healthy individuals (from CPO and CIM groups) (B, D, F, H, J, L). Bar, 20 μm (A–C, G–J); 10 μm (D); 50 μm (E, K, L); 100 μm (F).
TEM of ultrathin sections
In the lymphoid organ (Figures 5A, B), nervous tissue (Figure 5C) and stomach (Figure 5I), and in hemocytes of gills (Figures 5D, E, F) and heart (Figures 5G, H) of diseased P. japonicus (from PO and IM groups), TEM of ultrathin sections revealed agminate or dispersive icosahedral particles with the usual iridescent virus structure. Moreover, the paracrystalline arrays of mature virions were observed in the stromal matrix cells of the lymphoid organ (Figures 5A, B) and the hemocytes of gills (Figure 5F). On the other hand, healthy P. japonicus (from CPO and CIM groups) tissue showed no signs of virus (data not shown).
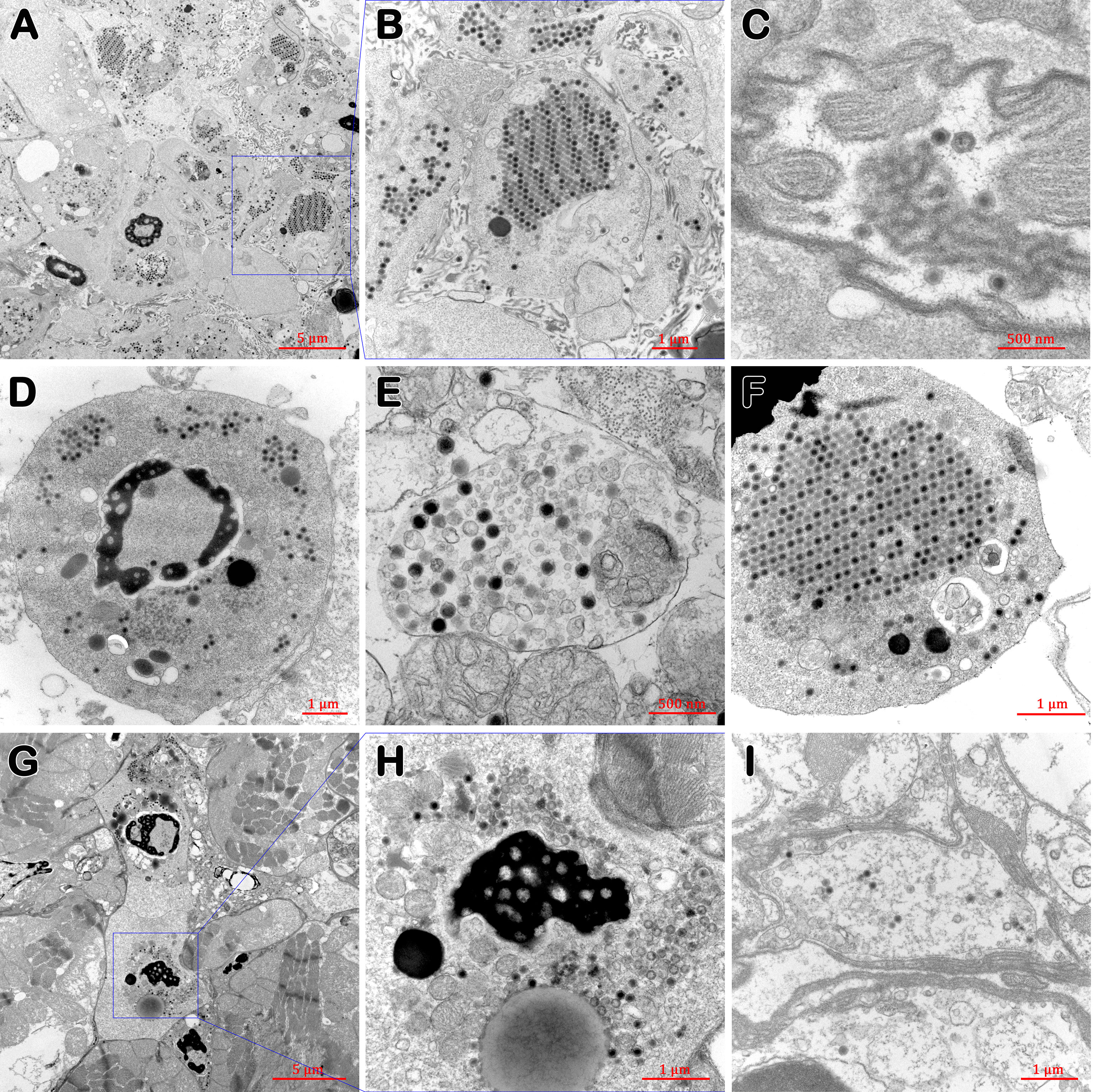
Figure 5 TEM of tissues of diseased P. japonicus (from PO and IM groups). (A, B) lymphoid organ; (C) nervous tissue; (D–F) gills; (G, H) heart; (I) stomach. (A, B), and (F) show the paracrystalline arrays of mature virions; H show the assembling virions. Bar: 5 µm (A, G); 1 µm (B, D, F, H, I); 500 nm (C, E).
DIV1 detection of epidemiological samples
DNA samples extracted from 17 batches of farmed P. japonicus in five provinces were tested with two real-time PCR methods targeting the fragment of ATPase and MCP genes, respectively. The results (Table 2) showed that 4 samples were positive for both MCP and ATPase genes of DIV1, with a total positive rate of 2.6% (4/153). Regarding the sampling area, all 4 positive samples were from 3 out of 4 farms in Zhejiang Province.
The positive rates (MCP & ATPase positive) of these three farms of P. japonicus samples were 20% (2/10), 10% (1/10) and 10% (1/10), respectively, and the total positive rate (MCP & ATPase positive) of samples in Zhejiang Province was 13.3% (4/30). Comparing the results of the two real-time PCR methods, the number of positive samples detected by the method targeting the MCP gene (25 samples) was significantly higher than that targeting the ATPase gene (6 samples). A total of 21 samples were positive by the MCP method but negative by the ATPase method. However, only 2 samples were positive for the ATPase method and negative for the MCP method.
Discussion
International Committee on Taxonomy of Viruses (ICTV) now classifies the Iridoviridae family comprising 7 genera, including Chloriridovirus, Daphniairidovirus, Decapodiridovirus and Iridovirus, belonging to Betairidovirinae, which mainly infecting insects and crustaceans, while Lymphocystivirus, Megalocytivirus and Ranavirus, belong to the Alphairidovirinae, which mainly infecting amphibians, reptiles and fish (ICTV, 2021). The infection with Decapod iridescent virus 1 (iDIV1) is an important emerging disease, often causing mortalities higher than 80%. It has been included in the Quarterly Aquatic Animal Disease Report (QAAD) by the Network of Aquaculture Centres in Asia-Pacific (NACA) in 2016 and listed by the World Organization for Animal Health (WOAH) in 2021. China has classified iDIV1 as a Class II animal pandemic disease in 2022.
According to the Criteria for Listing Species as Susceptible to Infection with a Specific Pathogen of WOAH, species of aquatic animals are considered susceptible to infection with a pathogenic agent when the presence of a multiplying or developing pathogenic agent has been demonstrated by the occurrence of natural cases or by experimental exposure that mimics natural transmission pathways (WOAH, 2022). Therefore, Pr. clarkii, M. rosenbergii, P. vannamei, P. monodon, M. nipponense and C. quadricarinatus have been shown to be susceptible hosts of DIV1 by the occurrence of natural cases (Xu et al., 2016; Qiu et al., 2017; Qiu et al., 2019a; Srisala et al., 2021), and E. carinicauda and Po. trituberculatus has been confirmed by experimental exposure that mimics natural transmission pathways (Chen et al., 2019; Qiu et al., 2022).
China’s targeted surveillance detected DIV1 in farmed P. japonicus samples in 2017 and 2018 (Qiu et al., 2018c; Qiu et al., 2019b). Ren et al. (2022) also detected DIV1 in cultured P. japonicus samples collected from Dalian, Liaoning Province of China, in 2020 through epidemiological investigation. He et al. (2021) challenged P. japonicus with DIV1 by intramuscular injection, which can lead to infection in healthy individuals. Although the above reports add to the evidence that P. japonicus may be susceptible to DIV1, there is no complete confirmation that P. japonicus is a susceptible host of DIV1. In this study, healthy P. japonicus were artificially infected with SHIV (an original isolate of DIV1) by per os (the pathway that mimics natural transmission) and intramuscular injection (invasive pathway). A variety of methods, nucleic acid detection, histopathological analyses, TEM, and ISDL, were then used to confirm whether P. japonicus was successfully infected with DIV1 and whether DIV1 is multiplying or developing in the cells. Through the present research, P. japonicus could be verified as a susceptible host of DIV1 because it fully complied with the Criteria for Listing Species as Susceptible to Infection with a Specific Pathogen of WOAH (WOAH, 2022).
Compared with the typical ‘white head’ of M. rosenbergii infected with DIV1, the penaeid shrimp is often thought to present with some atypical clinical signs, including an empty guts and stomach, softshell, expanded and deeper pigmentation spots on the epithelium, and reddish body (Qiu et al., 2017; Qiu et al., 2019a; Qiu et al., 2021). In this study, in addition to anorexia and retardation, the most obvious appearance symptom of DIV1-infected P. japonicus was that almost all of the challenged individuals showed the evident reddish body, consistent with the clinical signs published by He et al. (2021). Similarly, previous reports have shown that many individuals of P. vannamei infected with DIV1 exhibit an evident reddish body (Qiu et al., 2017; Qiu et al., 2021; Guo et al., 2022). The clinical sign of reddish body have been reported in many cases of shrimp disease in China and other Asian countries, but the earlier etiology is thought to be mainly due to infection with V. parahaemolyticus or WSSV (Huang et al., 1995; Alapide-Tendencia and Dureza, 1997; Liu et al., 1999; Fan et al., 2006; Jayasree et al., 2006; Zheng et al., 2011; Felix et al., 2011; Cao et al., 2014). The clinical sign of reddish body is a general sign indicating an imbalance in shrimp, but it may be caused by several pathogens and other uninfectious factors. In addition, the hepatopancreas and digestive tract of the infected P. japonicus did not show significant abnormalities compared with the healthy individuals in appearance and dissection. It is worth noting that the lymphoid organ of infected P. japonicus showed a swollen and whitish appearance, which could be clearly observed in front of the hepatopancreas in the central longitudinal dissection of the shrimp. Therefore, like the ‘white head’ of M. rosenbergii, the swollen and whitish lymphoid organs of P. japonicus may also be regarded as the typical symptom of iDIV1, which can be used to assist the clinical diagnosis. Unlike prawns, species such as crabs or crayfish infected with DIV1 cannot easily observe signs in their internal organs from the outside due to their thick and opaque carapace (Xu et al., 2017; Qiu et al., 2019a).
At the histopathological level, unique characteristics of karyopyknosis and eosinophilic inclusions and minute basophilic stains were combined in lymphoid organs, hematopoietic tissues, and gills of diseased P. japonicus, which was additionally confirmed by the ISDL blue signals at the same site in these tissues. In contrast to hematopoietic tissues and gills, lymphoid organs indicate disorder of the lymphoid tubule matrix followed by aberrant morphology of the nucleus plus the presence of karyorrhectic and pyknotic nuclei, and such lesions are more easily observed at low magnification. These lesions in lymphoid organs have also been reported previously and can be used as a preliminary marker to speed up screening for DIV1 infections (Sanguanrut et al., 2021). The eosinophilic inclusions were also present in cytoplasm in hemocytes, the cells of hematopoietic tissues and lymphoid organs of DIV1-infected shrimp, crayfish and crabs (Qiu et al., 2019a; Qiu et al., 2021; Qiu et al., 2022), which is very similar to some reported cases caused by the putative iridovirus in penaeid shrimp Protrachypene precipua (Lightner and Redman, 1993). The karyopyknosis is similar to some fish cases caused by grouper sleepy disease iridovirus (GSDIV) (Mahardika et al., 2004) and iridovirus in African lampeye and dwarf gourami (Sudthongkong et al., 2002). For ISDL results, in addition to lymphoid organs, hemopoietic tissues, and gills, DIV1 positive signals were also detected in epithelial tissues, hepatopancreas tubules, and muscle, but the signals in these three tissues were more concentrated in the nucleus. However, upon closer inspection, no obvious abnormalities were found in any of the three tissues. Therefore, for penaeid shrimp samples, lymphoid organs and hematopoietic tissues are the most suitable tissues for the pathological diagnosis of iDIV1. Sanguanrut et al. (2021) reported that a preliminary diagnosis could be made through lymphoid organs, and a further comprehensive diagnosis could be made combined with hematopoietic tissues. From TEM, the agminate or dispersive DIV1 virions were observed in lymphoid organs, the nervous tissue, the stomach, and hemocytes of gills and the heart of diseased P. japonicus. In addition, the virogenic stroma and the process of nucleocapsid formation could be observed in the cytoplasm. Xu et al. (2016), also observed clearly icosahedral DIV1 particles in the cytoplasm of hematopoietic tissue cells of diseased C. quadricarinatus by TEM (Xu et al., 2016; Qiu et al., 2017; Qiu et al., 2019a; Qiu et al., 2021; Qiu et al., 2022). In this study, hematopoietic tissues of prawn individuals were difficult to be identified by naked eye, so hematopoietic tissues could not be obtained during TEM sampling. In TEM observation of target tissue of P. vannamei, M. rosenbergii and Po. tritomatus, virion formation took place in the cytoplasmic morphologically distinct regions, termed virogenic stromata and the assembly of nucleocapsid can be observed, which was consistent with the results in this paper (Qiu et al., 2017; Qiu et al., 2019a; Qiu et al., 2022). Notably, some dispersive DIV1 particles were also observed in neural tissues, but virus replication and assembly were not observed. In terms of lymphoid organs, histopathological observation showed disorganization of the lymphoid tubule matrix accompanied by abnormal morphology of the nuclei plus the presence of karyorrhectic and pyknotic nuclei after DIV1 infection, while TEM also showed a large number of viruses in and out of lymphoid organ cells and a large number of abnormal cells, which also explained the swollen and whitish appearance of lymphoid organs from the histopathological and cytopathological levels.
China’s targeted surveillance showed that DIV1 was detected in farmed P. japonicus samples in 2017 and 2018, and the positive rate was 7.7% (1/13 in samples) in both years (Qiu et al., 2018b; Qiu et al., 2019b). Ren et al. (2022) showed that the positive rate of DIV1 in cultured P. japonicus collected from Liaoning Province of China in 2020 was 5.3% (9/157 in samples). In this study, DIV1 test results of epidemiological P. japonicus samples from five provinces in 2022 showed that a total of 4 samples were DIV1 (MCP & ATPase) positive, with a total positive rate of 2.6% (4/153 in samples). According to the above test results of collected samples, the positive rate of DIV1 in the farmed P. japonicus samples was relatively low. However, considering that the above surveys are active surveillance and the samples with disease onset are not investigated, the prevalence of DIV1 in the farmed P. japonicus cannot be ignored. In the present study, considering 17 batches of cultured samples investigated were all healthy asymptomatic shrimp, to improve the accuracy of detection, two published real-time PCR assays (Qiu et al., 2018a; Qiu et al., 2020) targeting two different genes (MCP and ATPase) were selected to detect DIV1. Samples were judged to be DIV1 (MCP & ATPase) positive only if both assays were positive. The results of the two methods were significantly different, which showed that the positive rate of the real-time PCR method for the MCP gene was significantly higher than that of the method for ATPase. Since the P. japonicus samples collected in this paper were all healthy and asymptomatic prawns, the DIV1 in the samples may be in a state of low load carrying rather than infection. Therefore, the difference in sensitivity and the instability in the detection of low-load samples may be the main reason for the different detection results of the two real-time PCR methods. Besides, the above results suggest that at least two gene fragments of DIV1 genome should be detected in the monitoring investigation of healthy samples, and only when two DIV1 gene fragments are detected positive can a positive result be given. In the Aquatic Manual of WOAH, the definition of confirmed case of pathogens such as WSSV and IHHNV is based on the detection of two gene fragments of pathogens at the same time (WOAH, 2021). Since the WOAH manual of iDIV1 has not yet been formulated, such double gene detection criteria should also be used in the identification of confirmed cases.
Similar to the results of surveillance surveys in previous reports (Qiu et al., 2018b; Qiu et al., 2019b; Ren et al., 2022), the detection rate of DIV1 in cultivated P. japonicus samples investigated in this paper was also relatively low. However, since these surveys did not fully cover the breeding ponds with clinical signs, and P. japonicus has also been confirmed to be susceptible hosts of DIV1, which can be infected through natural ingestion, therefore, the potential threat of DIV1 to P. japonicus aquaculture should raise alarm.
Data availability statement
The raw data supporting the conclusions of this article will be made available by the authors, without undue reservation.
Author contributions
LQ and JH designed the experiments. X-MG, Y-HF, and J-YX carried out the experiments. X-YR contributed to the shrimp collection and sampling. LQ contributed to the funding of research. LQ, X-MG, and JH wrote the manuscript. All authors contributed to the article and approved the submitted version.
Funding
This work was supported by the projects under Shandong Provincial Natural Science Foundation, China (grant number ZR2021QC144); National Key R&D Program of China (grant number 2019YFD0900101); Central Public-interest Scientific Institution Basal Research Fund, YSFRI, CAFS (grant number 20603022022023); Central Public-interest Scientific Institution Basal Research Fund, CAFS (grant number 2020TD39); Central Public-interest Scientific Institution Basal Research Fund, CAFS (grant number 2022GH01) and the earmarked fund for CARS-48.
Conflict of interest
The authors declare that the research was conducted in the absence of any commercial or financial relationships that could be construed as a potential conflict of interest.
Publisher’s note
All claims expressed in this article are solely those of the authors and do not necessarily represent those of their affiliated organizations, or those of the publisher, the editors and the reviewers. Any product that may be evaluated in this article, or claim that may be made by its manufacturer, is not guaranteed or endorsed by the publisher.
References
Alapide-Tendencia E. V., Dureza L. A. (1997). ). isolation of vibrio spp. from Penaeus monodon (Fabricius) with red disease syndrome. Aquaculture 154 (2), 107–114.
Bell T. A., Lightner D. V. (1988). A handbook of normal penaeid shrimp histology (Tucson, AZ, USA: World Aquaculture Society).
Bureau of Fisheries, Ministry of Agriculture and Rural Affairs, National Fisheries Technology Extension Center, China Society of Fisheries (2022). China Fishery statistics yearbook (Beijing, China: China Agriculture Press).
Cao H.-P., Gu Y., Diao J., Xu L., Xu T.-Y., Jin T.-T., et al. (2022). Phenotypic and genomic characterization of pathogenic Providencia rettgeri from kuruma shrimp marsupenaeus japonicus. Transbound Emerg. Dis. 2022 69 (5), e2967–e2977. doi: 10.1111/tbed.14647
Cao H.-P., He S., Lu L.-Q., Yang X.-L., Chen B.-Y. (2014). Identification of a Proteus penneri isolate as the causal agent of red body disease of the cultured white shrimp Penaeus vannamei and its control with Bdellovibrio bacteriovorus. Antonie van Leeuwenhoek 105, 423–430. doi: 10.1007/s10482-013-0079-y
Chen X., Qiu L., Wang. H.-L., Zou P.-Z., Dong X., Li F.-H., et al. (2019). Susceptibility of Exopalaemon carinicauda to the infection with shrimp hemocyte iridescent virus (SHIV 20141215), a strain of decapod iridescent virus 1 (DIV1). Viruses 11 (4), 387. doi: 10.3390/v11040387
Fan J.-F., Song L.-C., Wang B., Zang H.-M., Liang Y.-B. (2006). Preliminary study on one pathogenic bacterium – Vibrio parahaemolyticus associated with red body disease in Litopenaeus vannamei. Mar. Sci. 4, 40–44.
Felix F., Nugroho T., Silalahi S., Octavia Y. (2011). Molecular characteristics of vibrio sp. causing black tiger prawn (Penaeus monodon) disease in Sumatra and Java shrimp ponds by 16S rDNA sequencing. J. Agr. Tech. 7 (3), 679–694. doi: 10.1016/j.fsi.2019.09.046
Guo X.-M., Qiu L., Gao W., Wang G.-H., Chen X., Huang J. (2022). Radical thermal therapy against infection with decapod iridescent virus 1 (DIV1). Aquaculture 561 (5), 738636. doi: 10.1016/j.aquaculture.2022.738636
Hewitt D. R., Duncan P. F. (2001). Effect of high water temperature on the survival, moulting and food consumption of Penaeus (Marsupenaeus) japonicus (Bate 1888). Aquaculture Res. 32, 305–313. doi: 10.1046/j.1365-2109.2001.00560.x
He Z.-H., Zhao J.-C., Chen X.-Y., Liao M.-Z., Xue Y., Zhou J.-N., et al. (2021). The molecular mechanism of hemocyte immune response in Marsupenaeus japonicus infected with decapod iridescent virus 1. Front. Microbiol. 12. doi: 10.3389/fmicb.2021.710845
Huang J., Song X.-L., Yu J., Yang C.-H. (1995). Baculoviral hypodermal and hematopoietic necrosis – study on the pathogen and pathology of the explosive epidemic disease of shrimp. Mar. Fisheries Res. 16 (1), 1–10.
Huang J., Song X.-L., Yu J., Zhang L.-J. (1999). The components of an inorganic physiological buffer for Penaeus chinensis. Methods Cell. Sci. 21 (4), 225–230. doi: 10.1023/A:1009876528852
ICTV (2019) One new genus with one new species in the subfamily betairidovirinae. international committee on taxonomy of viruses. Available at: https://talk.ictvonline.org/files/ictv_official_taxonomy_updates_since_the_8th_report/m/animal-dnaviruses-and-retroviruses/8051 (Accessed 20 April 2021).
ICTV (2021) Current ICTV taxonomy release. international committee on taxonomy of viruses. Available at: https://ictv.global/taxonomy (Accessed January 2023).
Jayasree L., Janakiram P., Madhavi R. (2006). Characterization of vibrio spp. associated with diseased shrimp from culture ponds of andhra pradesh (India). J. World Aquacult. Soc 37 (4), 523–532. doi: 10.1111/j.1749-7345.2006.00066.x
King A. M. Q., Adams M. J., Carstens E. B. (2011). Virus taxonomy: Ninth report of the international committee on taxonomy of viruses (London, UK: Elsevier).
Lightner D. V. (1996). A handbook of shrimp pathology and diagnostic procedures for disease of cultured penaeid shrimp (Baton Rouge, LA, USA: World Aquaculture Society).
Lightner D. V., Redman R. M. (1993). A putative iridovirus from the penaeid shrimp Protrachypene precipua burkenroad (Crustacea: Decapoda). J. Invertebr. Pathol. 62, 107–109. doi: 10.1006/jipa.1993.1084
Liu Y.-M., Qiu L., Sheng A.-Z., Wan X.-Y., Cheng D.-Y., Huang J. (2018). Quantitative detection method of Enterocytozoon hepatopenaei using TaqMan probe real-time PCR. J. Invertebr. Pathol. 151, 191–196. doi: 10.1016/j.jip.2017.12.006
Liu H., Wang K., Peng J.-X., Zhao J.-H., Zhang X.-Q. (1999). Study on bacterial pathogen of " red body disease” in Penaeus monodon. Fisheries Sci. Technol. Inf. 26 (1), 7–9.
Li F., Xu L., Yang F. (2017). Genomic characterization of a novel iridovirus from redclaw crayfish Cherax quadricarinatus: Evidence for a new genus within the family Iridoviridae. J. Gen. Virol. 98 (10), 2589–2595. doi: 10.1099/jgv.0.000904
Mahardika K., Yamamoto A., Miyazaki T. (2004). Susceptibility of juvenile humpback grouper cromileptes altivelis to grouper sleepy disease iridovirus (GSDIV). Dis. Aquat. Org 59, 1–9. doi: 10.3354/dao059001
Pan C. K., Yuan H. F., Wang T. T., Yang F., Chen J. M. (2017). Study of cherax quadricarinatus iridovirus in two crab. J. Appl. Oceanography 36 (1), 82–86. doi: 10.3969/J.ISSN.2095-4972.2017.01.010
Qiu L., Chen X., Gao W., Guo X.-M., Xie G.-S., Gong M., et al. (2022). Confirmation of susceptibility of swimming crab to infection with decapod iridescent virus 1. Aquaculture 548, 737607. doi: 10.1016/j.aquaculture.2021.737607
Qiu L., Chen X., Gao W., Li C., Guo X.-M., Zhang Q.-L., et al. (2021). Molecular epidemiology and histopathological study of a natural infection with decapod iridescent virus 1 in farmed white leg shrimp, Penaeus vannamei. Aquaculture 533, 736105. doi: 10.1016/j.aquaculture.2020.736105
Qiu L., Chen X., Guo X.-M., Gao W., Zhao R.-H., Zhang Q.-L., et al. (2020). A TaqMan probe based real-time PCR for the detection of decapod iridescent virus 1. J. Invertebr. Pathol. 173, 107367. doi: 10.1016/j.jip.2020.107367
Qiu L., Chen M.-M., Wang R.-Y., Wan X.-Y., Li C., Zhang Q.-L., et al. (2018b). Complete genome sequence of shrimp hemocyte iridescent virus (SHIV) isolated from white leg shrimp, Litopenaeus vannamei. Arch. Virol. 163 (3), 781–785. doi: 10.1007/s00705-017-3642-4
Qiu L., Chen M.-M., Wan X.-Y., Li C., Zhang Q.-L., Wang R.-Y., et al. (2017). Characterization of a new member of Iridoviridae, shrimp hemocyte iridescent virus (SHIV), found in white leg shrimp (Litopenaeus vannamei). Sci. Rep. 7 (1), 11834. doi: 10.1038/s41598-017-10738-8
Qiu L., Chen M.-M., Wan X.-Y., Zhang Q.-L., Li C., Dong X., et al. (2018a). Detection and quantification of shrimp hemocyte iridescent virus by TaqMan probe based real-time PCR. J. Invertebr. Pathol. 154, 95–101. doi: 10.1016/j.jip.2018.04.005
Qiu L., Chen X., Zhao R.-H., Li C., Gao W., Zhang Q.-L., et al. (2019a). Description of a natural infection with decapod iridescent virus 1 in farmed giant freshwater prawn, Macrobrachium rosenbergii. Viruses 11, 354. doi: 10.3390/v11040354
Qiu L., Dong X., Wan X.-Y., Huang J. (2018c). “Analysis of iridescent viral disease of shrimp (SHID) in 2017,” in Analysis of important diseases of aquatic animals in China in 2017 (Beijing: China Agriculture Press), 187–204. Bureau of Fisheries, Ministry of Agriculture and Rural Affairs, National Fisheries Technology Extension Center.
Qiu L., Dong X., Wan X.-Y., Huang J. (2019b). “Analysis of iridescent viral disease of shrimp (SHID) in 2018,” in 2019 Analysis of Important Diseases of Aquatic Animals in China (Beijing: China Agriculture Press), 189–207. Bureau of Fisheries, Ministry of Agriculture and Rural Affairs, National Fisheries Technology Extension Center, China Society of Fisheries.
Ren X., Chen W.-B., Ding Y., Zhang S.-S., Zeng F.-S., Han Y.-Z., et al. (2022). Investigation of five epidemic pathogens from Penaeus japonicus in dalian. journal of. Aquaculture 4, 23–39. doi: 10.3969/j.issn.1004-2091.2022.04.005
Ren X., Xu Y., Zhang Y., Wang X., Liu P., Li J. (2020). Comparative accumulation and transcriptomic analysis of juvenile Marsupenaeus japonicus under cadmium or copper exposure. Chemosphere 249, 126157. doi: 10.1016/j.chemosphere.2020.126157
Sanguanrut P., Thaiue D., Thawonsuwan J., Aldama-Cano D. J., Flegel T. W., Sritunyalucksana K. (2021). The lymphoid organ (LO) is an additional, prime target for decapod iridescent virus 1 (DIV1) in Penaeus vannamei. Aquaculture 547, 737482. doi: 10.1016/j.aquaculture.2021.737482
Srisala J., Sanguanrut P., Thaiue D., Laiphrom S., Siriwattano J., Khudet J., et al. (2021). Infectious myonecrosis virus (IMNV) and decapod iridescent virus 1 (DIV1) detected in captured, wild Penaeus monodon. Aquaculture 545, 737262. doi: 10.1016/j.aquaculture.2021.737262
Sudthongkong C., Miyata M., Miyazaki T. (2002). Iridovirus disease in two ornamental tropical freshwater fishes: African lampeye and dwarf gourami. Dis. Aquat. Org 48, 163–173. doi: 10.3354/dao048163
WOAH (2021) Manual of diagnostic tests for aquatic animals (World Organization for Animal Health). Available at: https://www.woah.org/en/what-we-do/standards/codes-and-manuals/aquatic-manual-online-access/ (Accessed 10 July 2022).
WOAH (2022)Chapter 1.5 criteria for listing species as susceptible to infection with a specific pathogen. In: Aquatic animal health code (World Organisation for Animal Health). Available at: https://www.woah.org/en/what-we-do/standards/codes-and-manuals/aquatic-code-online-access/?id=169&L=1&htmfile=chapitre_criteria_species.htm (Accessed 15 August 2022).
Xu L.-M., Wang T.-T., Li F., Yang F. (2016). Isolation and preliminary characterization of a new pathogenic iridovirus from redclaw crayfish Cherax quadricarinatus. Dis. Aquat. Organ. 120 (1), 17–26. doi: 10.3354/dao03007
Zhang Y.-Q., Yao N., Zhang C.-T., Sun X.-S., Huang J.-X., Zhao B.-R., et al. (2022). LncRNA-mRNA integrated profiling analysis in response to white spot syndrome virus in hepatopancreas in penaeus japonicus. Fish. Shellfish. Immunol 129, 251–262. doi: 10.1016/j.fsi.2022.08.061
Keywords: Decapod iridescent virus 1, susceptible species, Penaeus japonicus, lymphoid organ, clinical diagnosis
Citation: Qiu L, Guo X-M, Feng Y-H, Xing J-Y, Ren X-Y and Huang J (2023) Susceptibility of kuruma shrimp to the infection with Decapod iridescent virus 1. Front. Mar. Sci. 10:1114123. doi: 10.3389/fmars.2023.1114123
Received: 02 December 2022; Accepted: 10 January 2023;
Published: 23 January 2023.
Edited by:
Zhaoqun Liu, Hainan University, ChinaReviewed by:
Qilin Yi, Dalian Ocean University, ChinaCesar Marcial Escobedo-Bonilla, Unidad Sinaloa (IPN), Mexico
Najiah Musa, University of Malaysia Terengganu, Malaysia
Copyright © 2023 Qiu, Guo, Feng, Xing, Ren and Huang. This is an open-access article distributed under the terms of the Creative Commons Attribution License (CC BY). The use, distribution or reproduction in other forums is permitted, provided the original author(s) and the copyright owner(s) are credited and that the original publication in this journal is cited, in accordance with accepted academic practice. No use, distribution or reproduction is permitted which does not comply with these terms.
*Correspondence: Jie Huang, huangjie@ysfri.ac.cn
†These authors have contributed equally to this work