- 1National Oceanic and Atmospheric Administration (NOAA) Office of National Marine Sanctuaries, Flower Garden Banks National Marine Sanctuary, Galveston, TX, United States
- 2Rosenstiel School of Marine, Atmospheric, and Earth Science, Cooperative Institute for Marine and Atmospheric Studies (CIMAS), University of Miami, Miami, FL, United States
- 3Atlantic Oceanographic and Meteorological Laboratories (AOML), National Oceanic and Atmospheric Administration (NOAA), Miami, FL, United States
- 4Department of BioSciences, Rice University, Houston, TX, United States
- 5Harbor Branch Oceanographic Institute, Florida Atlantic University, Fort Pierce, FL, United States
- 6CSS Inc, Fairfax, VA, United States
- 7CPC Inc, Galveston, TX, United States
- 8Harte Research Institute for Gulf of Mexico Studies, Texas A&M University–Corpus Christi, Corpus Christi, TX, United States
- 9Department of Biology & Marine Biology, University of North Carolina Wilmington, Wilmington, NC, United States
East and West Flower Garden Bank (FGB) are part of Flower Garden Banks National Marine Sanctuary (FGBNMS) in the northwest Gulf of Mexico. This geographically-isolated reef system contains extensive coral communities with the highest coral cover (>50%) in the continental United States due, in part, to their remoteness and depth, and have historically exhibited low incidence of coral disease and bleaching despite ocean warming. Yet in late August 2022, disease-like lesions on seven coral species were reported during routine monitoring surveys on East and West FGB (2.1–2.6% prevalence). A series of rapid response cruises were conducted in September and October 2022 focused on 1) characterizing signs and epidemiological aspects of the disease across FGB and within long-term monitoring sites, 2) treating affected coral colonies with Base 2B plus amoxicillin, and 3) collecting baseline images through photostations and photomosaics. Marginal and/or multi-focal lesions and tissue loss were observed, often associated with substantial fish and invertebrate predation, affecting the dominant coral species Pseudodiploria strigosa (7–8% lesion prevalence), Colpophyllia natans (11–18%), and Orbicella spp. (1%). Characterizing this disease event during its early epidemic phase at East and West FGB provides a critical opportunity to observe how coral disease functions in a relatively healthy coral ecosystem versus on reefs chronically affected by various stressors (e.g., Caribbean reefs adjacent to urban centers). Insights into the etiology, spread, and impacts of the disease can ultimately inform efforts to mitigate its effects on coral communities.
Introduction
Coral reefs provide numerous ecosystem services including support of robust fisheries and tourism industries. Over the last half-century, however, natural and anthropogenic stressors including pollution, overfishing, disease, and ocean warming have contributed to widespread degradation of coral reefs throughout the Tropical Western Atlantic (Eakin et al., 2010; Jackson et al., 2014; Woodley et al., 2016; Gil-Agudelo et al., 2020; Cróquer et al., 2021). Flower Garden Banks National Marine Sanctuary (FGBNMS) is a high coral cover reef ecosystem in the northwest Gulf of Mexico that has shown remarkable resilience despite declines in coral reef health elsewhere (Aronson et al., 2005; Johnston et al., 2016). East and West Flower Garden Bank (FGB) are two of the 17 banks within the sanctuary, and are located along the outer continental shelf, approximately 190 km south of the Texas-Louisiana border (Figure 1). The banks range in depth from 16–150 m and support extensive coral reef communities to 46 m depths, including many Caribbean coral, sponge, and fish species. Portions of the reef have been monitored annually since 1989, with mean coral cover at East and West FGB remaining near or above 50%, dominated by reef-building species Pseudodiploria strigosa, Colpophyllia natans, and Orbicella spp. (Aronson et al., 2005; Johnston et al., 2016; Johnston et al., 2020).
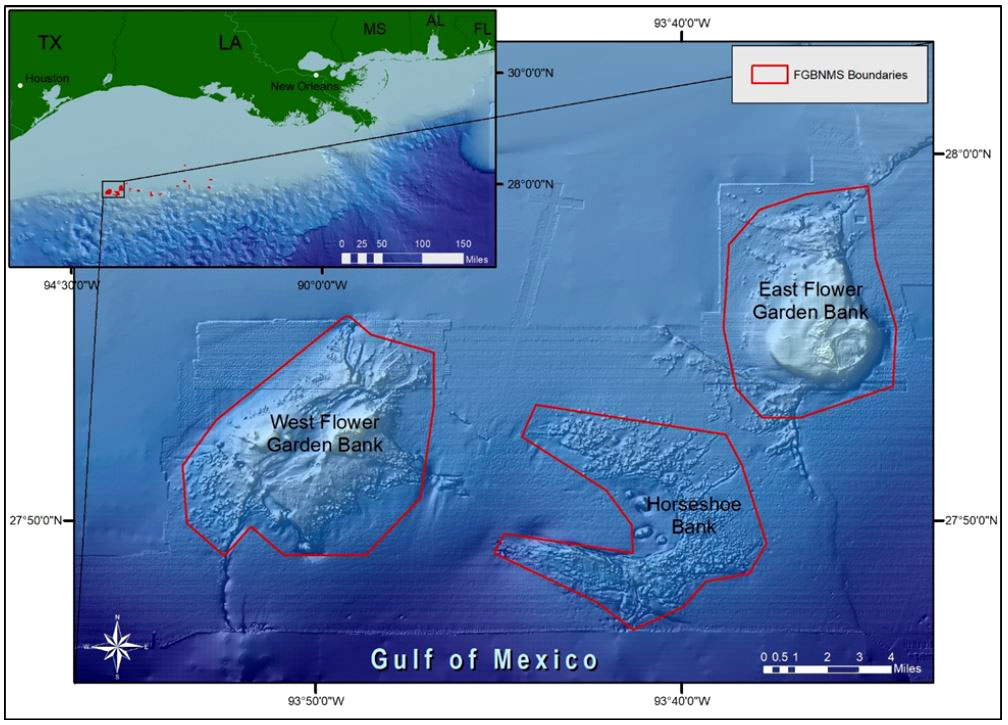
Figure 1 The Flower Garden Banks National Marine Sanctuary (northwest Gulf of Mexico) consists of 17 reefs and banks off the coasts of Texas and Louisiana (inset). East and West FGB boundaries are outlined in red.
It has been hypothesized that the depth and remoteness of East and West FGB have buffered them from extreme temperature, disease outbreaks, and other sources of mass mortality plaguing other shallower, coastal reef habitats throughout the region (Aronson et al., 2005; Johnston et al., 2016). There have been isolated instances of localized coral mortality events due to thermal stress-related bleaching (Johnston et al., 2019b), hurricanes and storms (Robbart et al., 2009; Wright et al., 2019; Shore et al., 2021), hypoxia (Johnston et al., 2019a; Kealoha et al., 2020; Doyle et al., 2022), and disease (Borneman and Wellington, 2005). Following these events, coral recovery has often been rapid, with few persistent changes in community composition or coral cover over time (2020; Johnston et al., 2016). During routine monitoring of both banks in late August 2022, disease lesions of unknown etiology were observed on brain and star corals, including P. strigosa, C. natans, O. faveolata, and O. franksi.
Disease outbreaks are of increasing concern, especially as the Caribbean region is a hotspot of coral diseases (Weil et al., 2006; Ruiz-Moreno et al., 2012; van Woesik and Randall, 2017), experiencing recurring disease outbreaks such as white plague from the 1970s through the mid-2000s (reviewed in Cróquer et al., 2021). White plague produces lesions at colony margins leading to rapid tissue loss and mortality (Richardson et al., 1998; Bythell et al., 2004). White plague affects nearly 40 species and is now considered endemic to much of the Tropical Western Atlantic (Cróquer et al., 2021). Despite identification of Aurantimonas coralicida in white plague (Type II)-affected corals, a universal white plague pathogen has not been confirmed, and it has been hypothesized that white plague signs may be caused by multiple etiologies. The most recent coral disease described to date, stony coral tissue loss disease (SCTLD), was first reported in September 2014 off the coast of southeast Florida (Precht et al., 2016), and has become the most lethal coral disease ever recorded due to its swift spread across colonies and reefs, extensive tissue necrosis, and high mortality rates affecting at least 22 species (NOAA, 2018; Kramer et al., 2019). SCTLD is characterized by subacute to acute tissue loss resulting in the exposure of the coral skeleton below, and often begins as focal (single) or multifocal lesions. For both white plague and SCTLD, patterns of tissue loss typically radiate outward from focal lesions, or advance in a linear or crescent band from the margin or base of the colony, and often progress across the entire colony leading to complete colony mortality within weeks to months (Precht et al., 2016; Cróquer et al., 2021; Meiling et al., 2021).
SCTLD constitutes an ongoing epizootic event in the Caribbean, and is currently in an epidemic phase at some locations and an endemic phase in others (Aeby et al., 2021; Cróquer et al., 2021). SCTLD’s etiology remains unknown, yet both direct and waterborne transmission routes are hypothesized to occur (Aeby et al., 2019; Muller et al., 2020; Studivan et al., 2022a; Studivan et al., 2022b). While corals affected by SCTLD respond to antibiotic treatments (Neely et al., 2021; Shilling et al., 2021; Walker et al., 2021), it remains uncertain whether bacteria are primary causative agents or a secondary infection. Virus-like particles and genomic sequence signatures have been observed in infected samples and associated with algal symbionts of the family Symbiodiniaceae (Miller et al., 2020; Work et al., 2021; Veglia et al., 2022). SCTLD has spread continuously since 2014, affecting the entirety of Florida’s coral reefs from Martin County to the Dry Tortugas, and several locations in the Caribbean, including Belize, the Dominican Republic, Jamaica, Puerto Rico, Saint Martin, and the U.S. Virgin Islands (Kramer et al., 2019). Many coral species that are highly susceptible to SCTLD and sentinels of disease onset in Florida, such as Meandrina meandrites and Dichocoenia stokesii, are rarely observed at FGBNMS (Clark et al., 2014; NOAA, 2018). Thus, high cover species such as P. strigosa and C. natans constitute the highly susceptible species within the sanctuary.
While it was unknown if the observed lesions at FGBNMS were SCTLD, white plague, or another disease altogether, a series of rapid response cruises were conducted in September and October 2022 according to action items in the FGB SCTLD Preparedness Plan (Johnston, 2021). Monitoring and research activities focused on 1) characterizing the signs and epidemiological aspects of the disease across FGB and within long-term monitoring sites, 2) treating affected coral colonies with Base 2B plus amoxicillin, and 3) collecting baseline images through repetitive photostations and photomosaics. In this brief research report, we describe the initial signs of this new and unusual disease event observed at East and West FGB from August through October 2022.
Materials and methods
Study area
These observations were made on the coral reefs of East and West FGB in the northwestern Gulf of Mexico (Figure 1). East FGB (65.86 km2) ranges in depth from 17–130 m (27°54.5´N, 93°36.0´W) while West FGB (27°52.4´N, 93°48.8´W) is deeper (18–140 m) and larger in area (77.54 km2). Both banks are capped by well-developed coral reefs from 17–46 m covering 3.31 km2 at East FGB and 1.44 km2 at West FGB.
Initial disease observations were documented from August 30–September 2, 2022 across both coral-capped banks. Monitoring and response cruises thereafter were performed in long-term monitoring (LTM) study sites at East and West FGB during September and October 2022, beginning days after initial observations. Immediate response was possible via reprogramming of resources and partner engagement. The 10,000 m2 LTM sites (17–27 m depth) have been monitored annually since 1989 to characterize benthic cover and fish community trends on the central area of each bank (Johnston et al., 2020). Therefore, these well documented sites are designated as priority areas in the FGB SCTLD Preparedness Plan (Johnston, 2021) for monitoring and intervention.
Benthic surveys
From August 30–September 2, 2022, divers reported observations of diseased corals from East and West FGB during 30 stratified random National Coral Reef Monitoring Program (NCRMP) benthic surveys (2022b; CRCP, 2022a). Thereafter, three response-specific cruises were executed to conduct additional surveys from September 6–9, September 20–21, and October 4–7, 2022 following the FGB SCTLD Preparedness Plan (Johnston, 2021).
Within LTM study sites, diver propulsion vehicle (DPV) surveys were performed on September 6 and 8, 2022 (approximately 5 m above the reef) to count corals with lesions. Additionally, a total of 77 marked repetitive photostations (37 at East FGB and 40 at West FGB) were photographed to document prevalence and track disease progression of impacted colonies. These photostations which have been photographed annually since 1989 are both mapped and marked by permanent pins with numbered tags on the reef to aid in relocation by scuba divers. All stations were photographed using a D7000 DSLR (Nikon) at a distance of 2 m from the substrate, with photographs encompassing roughly 5 m2 (Johnston et al., 2020). The total number of colonies within each photostation were summed and colonies with lesions were recorded. As time permitted, divers treated lesions using Base 2B plus amoxicillin. The antibiotic treatment was mixed in an 8:1 base to antibiotics ratio, loaded into syringes, and applied directly to conspicuous lesion margins.
Water quality
A Sea-Bird SeaFET high-precision temperature and pH sensor was deployed from December 4, 2021–August 25, 2022 on the West FGB (27 m). The sensors recorded on an hourly basis. A Sea-Bird 56 temperature sensor was deployed from June 4, 2019–October 4, 2022 on the East FGB (21 m) and recorded every five minutes. Temperature readings were averaged to obtain a daily mean value. Satellite-derived surface salinity from the NOAA Environmental Research Division Data Access Program (ERDDAP; dataset Sea Surface Salinity, Near Real Time, Miras SMOS 3-Day Mean) was used to estimate benthic salinity, as little stratification occurs in the upper 20 m of the water column. During response cruises, salinity, dissolved oxygen (DO), and pH were measured at 20 m depth using a handheld YSI water quality sensor to complement data collected by the stationary water quality instruments on the seafloor.
Results
Lesion morphology
Initial observations from August 30–September 2, 2022 documented disease lesions on P. strigosa, C. natans, and O. faveolata colonies at East and West FGB (Figure 2), with lesions observed at every station on all NCRMP sampling locations across both banks. Lesions generally consisted of a stark border dividing healthy tissue from white, denuded coral skeleton, usually along colony margins. The lesion-affected areas were generally small in size (< 10 cm in border length, based on diver estimates) and largely restricted to corals on the central reef cap above 30 m depth. Lesions were often multifocal (Figure 2E), especially in the case of P. strigosa and O. faveolata. Divers also observed numerous incidents of predation on coral colonies with lesions (e.g., parrotfish, damselfish, fireworms, hermit crabs), with fish grazing often resulting in erosion of the underlying skeletons from several millimeters to one-centimeter depth (Figure 2B). The similar visual signs of corallivory and disease lesions often made differentiation between the two difficult. However, in some cases, it was clear that a coral had an active disease lesion (e.g., based on tissue sloughing) and corallivory was observed to be occurring on the active lesion. This has previously been observed on reefs in Florida affected by SCTLD (Noonan and Childress, 2020; Titus et al., 2022). Therefore, corallivores may have been attracted to feed on the diseased tissues of at least some corals, and the feeding behaviors of these corallivores may have ultimately obscured areas of lesion and/or associated denuded skeleton.
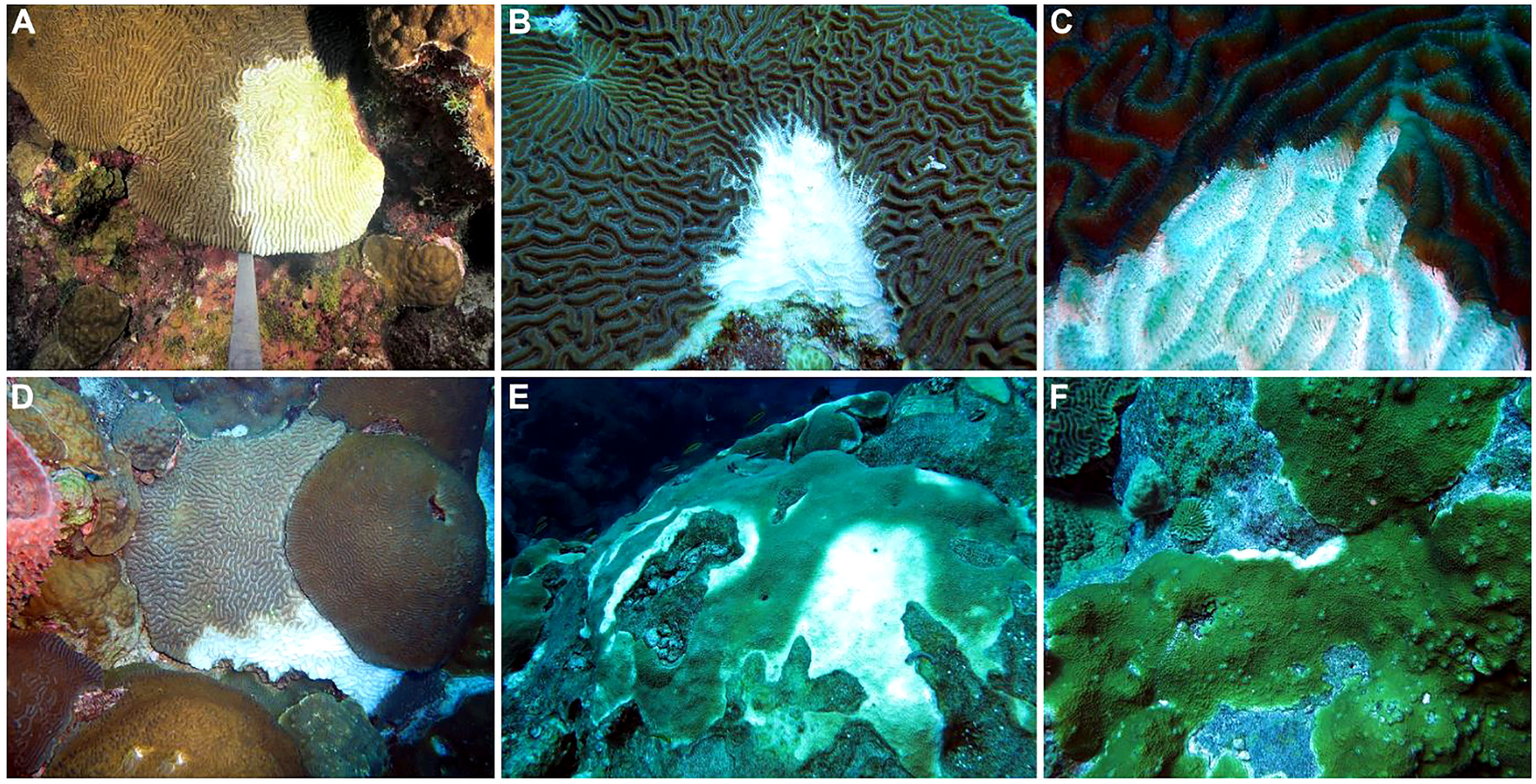
Figure 2 Examples of disease lesions on primary reef-building coral species from Flower Garden Banks (northwest Gulf of Mexico). (A) Focal lesion starting from the margin of a Pseudodiploria strigosa colony, (B) focal lesion on P. strigosa colony with evidence of skeletal damage due to predation (bottom), (C) rapid tissue loss and necrosis at the lesion front on P. strigosa colony, (D) active lesion spreading across Colpophyllia natans colony, (E) multifocal infection on Orbicella faveolata colony, (F) focal lesion beginning on Orbicella franksi colony.
Disease prevalence
During the first response cruise, 16 coral colonies with lesions (7 O. faveolata, 5 P. strigosa, 4 C. natans) were identified during DPV surveys within the one-hectare study site at East FGB (Supplementary Table S1). Subsequently, 21 colonies with lesions (12 P. strigosa, 5 O. faveolata, 4 C. natans) were identified within the study site while treating colonies with Base2B. Within the West FGB study site, a total of 23 colonies with lesions (12 O. faveolata, 7 P. strigosa, 2 C. natans, 1 O. annularis, and 1 Siderastrea siderea) were identified during DPV surveys; however, a total of 57 colonies with active lesions (11 O. faveolata, 35 P. strigosa, 5 C. natans, 1 O. annularis, 1 O. franksi, and 4 S. siderea) were identified and treated by divers within the area (Supplementary Table S1). Differences in observed lesions indicate that DPV surveys were not as effective at counting lesions as diver surveys, despite their utility for surveying large areas of the reef. Based on diver observations, colonies with lesions exhibited patchy distribution. Often, corals tightly clustered together and/or touching would have multiple small (<10 cm) lesions (Figure 3B).
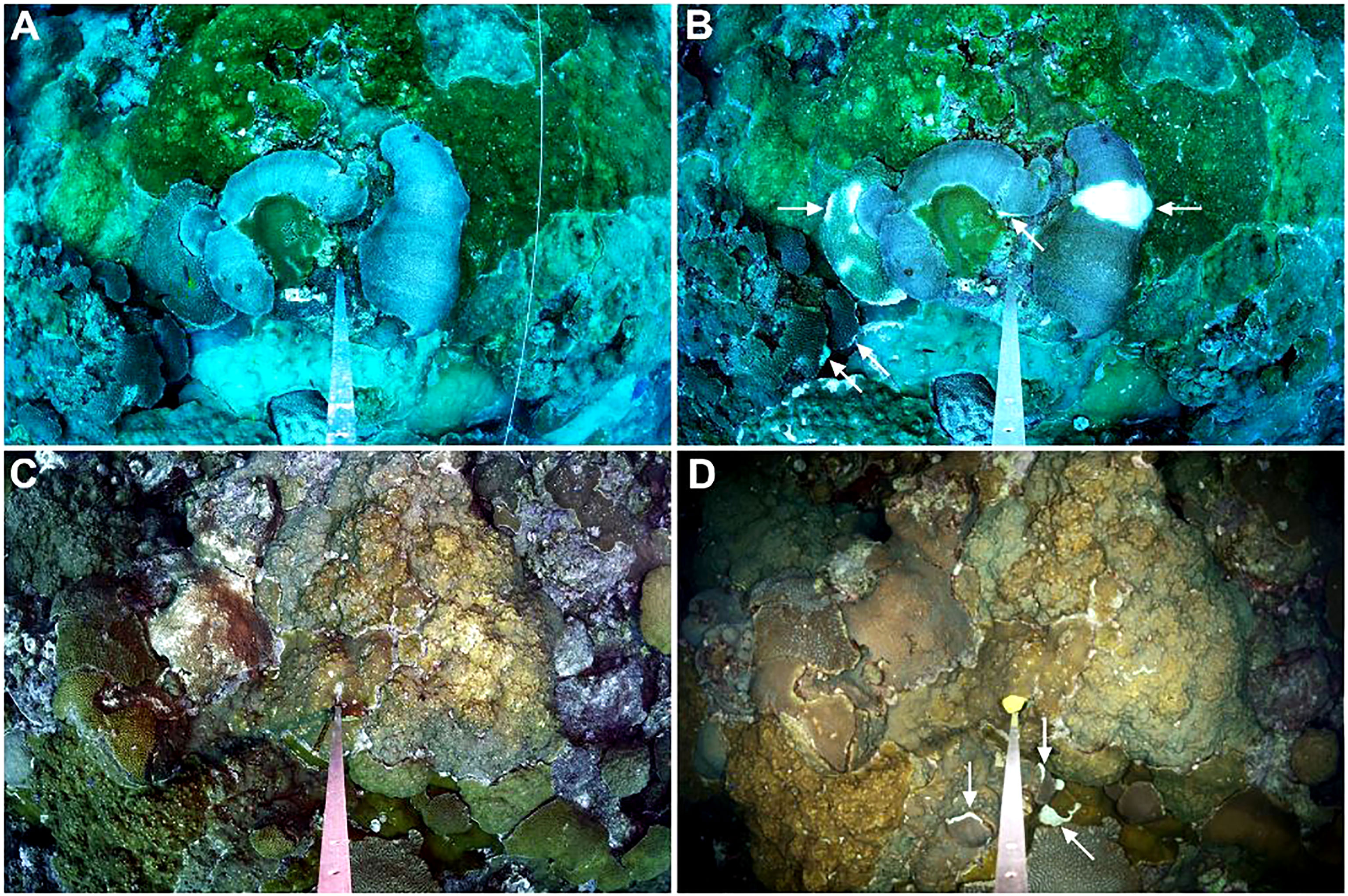
Figure 3 Repetitive photostations in 2021 and 2022 at East and West Flower Garden Bank. (A) Repetitive photostation 301 from East Flower Garden Bank (FGB, northwest Gulf of Mexico) with no lesions in 2021 and (B) the same photostation in 2022 with lesions on two Colpophyllia natans colonies, two Pseudodiploria strigosa colonies (larger lesion with fish biting), and a C. natans colony with multifocal lesions and bleaching; (C) repetitive photostation 810 from West FGB with no lesions in 2021 and (D) the same photostation in 2022 with lesions on two P. strigosa colonies and an Orbicella franksi colony.
A total of 1,586 coral colonies representing 14 coral species were identified in the 37 repetitive photostations at East FGB. Disease lesions were observed on half of the coral species recorded (7 of 14). Overall disease prevalence within East FGB monitoring stations was 2.6%, 95% CI [1.86, 3.49] (41 of 1,586 corals photographed; Table 1), and ranged in prevalence from 0-12% per station. Pseudodiploria strigosa and C. natans were the most impacted coral species, with 8% and 11% of colonies being afflicted with disease lesions, respectively (Supplementary Table S2). In the WFGB photostations (n = 40), a total of 2,001 coral colonies representing 12 species were identified. Disease lesions were observed on 66.7% of species (8 of 12; Table 1). Overall disease prevalence within monitoring stations was 2.1%, 95% CI [1.56, 2.88] (43 of 2,001 corals photographed), and ranged from 0-14% per station. Pseudodiploria strigosa and C. natans were the most impacted coral species, with 7% and 18% of colonies exhibiting disease lesions, respectively (Supplementary Table S2). No disease lesions were observed on corals in these photostations taken the year prior in 2021 (Figure 3).
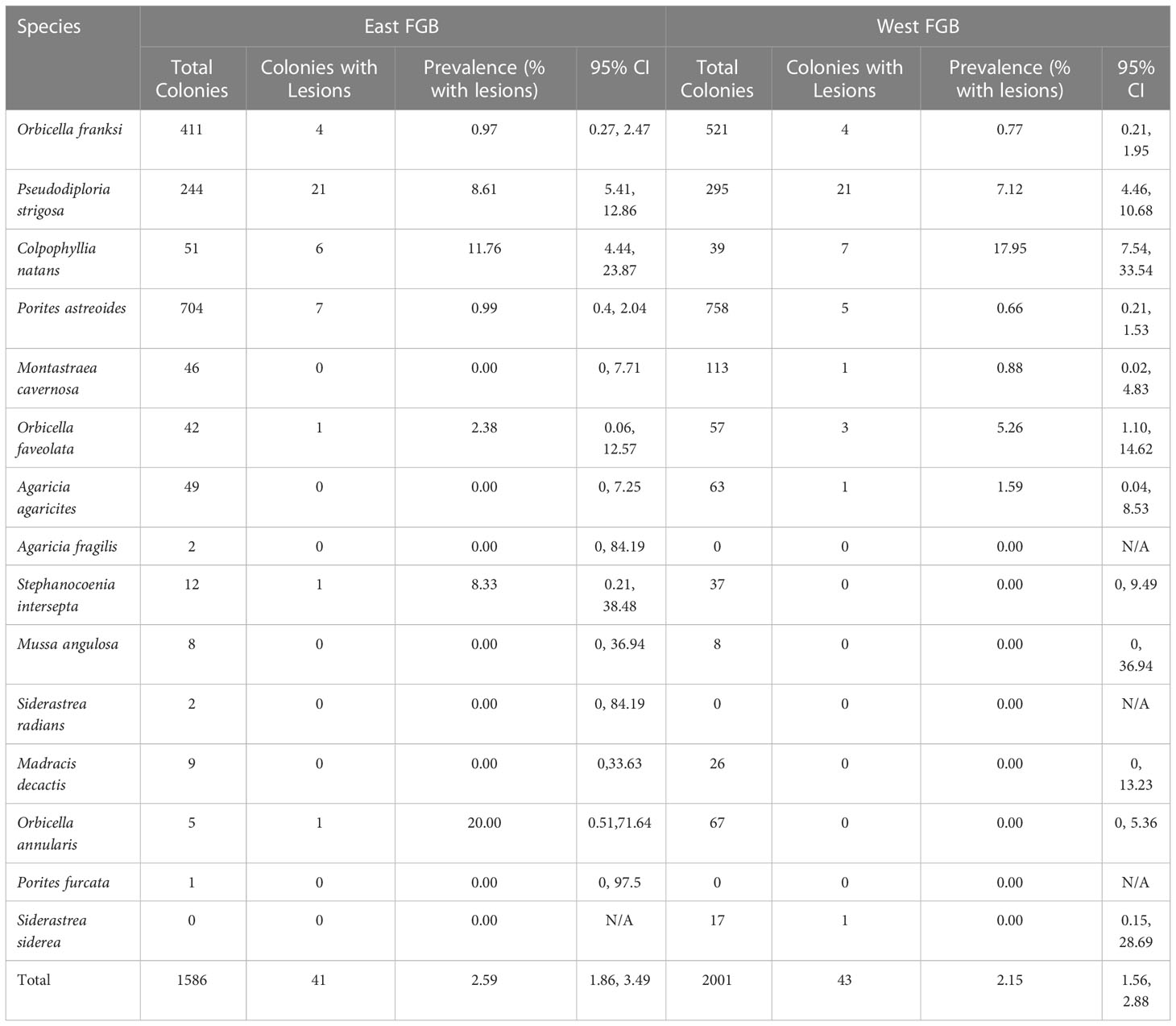
Table 1 Total number of coral colonies observed, number with disease lesions, and lesion prevalence by species with upper and lower 95% confidence intervals for East and West Flower Garden Bank (FGB, northwest Gulf of Mexico) repetitive monitoring stations.
Environmental context
Prior to initial observations, daily mean seawater temperature ranged from 26.4–29.8°C from June through September at EFGB (21 m) and 25.6–29.9°C at West FGB (27 m) throughout June, July, and August 2022 (Supplementary Figure S1). Temperatures on the reef never exceeded 30°C from June through September. Satellite-derived surface salinity ranged from 32.7–38.2 psu at East and West FGB in 2022 (Supplementary Figure S2). While salinity values decreased in summer months, indicating a freshwater presence that peaked in late June, surface salinity values were within the expected range of salinity for coral reefs located in the Western Atlantic (31–38 psu; Coles and Jokiel, 1992). Preliminary pH data (hourly) showed diel variation of as much as 0.16 as recorded by the SeaFET (May–August, 2022). During response cruises, salinity ranged from 35.8–36 ppt, pH ranged from 8.05–8.12, and DO 5.43–5.47 (mg/L) at both banks at 20 m depth.
Discussion
Coral diseases in the Tropical Western Atlantic have increased significantly during the past 40 years, dramatically impacting foundational reef-building species (Acropora spp., Orbicella spp., Pseudodiploria spp., C. natans, S. siderea, Montastraea cavernosa, etc.; Woodley et al., 2016; Cróquer et al., 2021). Despite widespread coral reef declines in recent decades throughout the Tropical Western Atlantic (Jackson et al., 2014), East and West FGB corals have changed very little since they were first assessed in the early 1970s, have recovered from coral bleaching events when they occurred, and showed minimal signs of disease (2019a; 2019b; Borneman and Wellington, 2005; Johnston et al., 2016; Gil-Agudelo et al., 2020). In 2003 and 2004, Borneman and Wellington (2005) conducted baseline surveys to document the extent of coral disease at East and West FGB, as they reported previous disease observations from FGB were unreliable due to confusion with predation, competition, and other factors including lack of sampling and histological examination. Results of this study found that few known Caribbean diseases were documented at East and West FGB, and that disease signs that were observed were often inconsistent with established coral disease pathologies. Of the 1,220 coral colonies surveyed for tissue loss diseases by Borneman and Wellington (2005), only one putative case of white plague was observed (0.08% prevalence). Since 2009, disease observations in annual benthic surveys within East and West FGB monitoring sites have ranged from 0–0.3% (Johnston et al., 2020).
During the summer of 2022, daily seawater temperature at depth (21 m and 27 m) was typical for summer months at FGBNMS (Johnston et al., 2019b). Bleaching threshold curves for East and West FGB suggest that more than 50 days above 29.5°C would initiate bleaching in these locations (Johnston et al., 2019b); however, only 27 days at East FGB (between June and September 2022) and 21 days at West FGB (June and August 2022) were above this threshold, suggesting that the observed tissue loss was not likely due to thermal stress in 2022. It is noted, however, that over the past decade, a significant seawater warming trend both at depth (~ 20 m) and in surface waters has been observed at FGB (Johnston et al., 2019b; Manzello et al., 2021). Other water quality parameters measured during the response, including pH, salinity, and DO, were within limits considered typical for coral reefs located in the Western Atlantic; however, lowered salinity following early summer peak in river discharge in combination with warm summer water temperatures may have contributed to compounded stress on the reef.
The recent disease outbreak at East and West FGB is unusual in terms of prevalence on this near-pristine reef system; however, at the time of this report, many uncertainties remain. Based on photography and diver observations alone, it is difficult to determine if the recent disease observed is SCTLD or a type of white plague, as these diseases manifest in a similar manner (Gintert et al., 2019; Cróquer et al., 2021), or whether the event described here is driven by a disease state of some other etiology. Examinations of histology, gross morphology, microbial and ‘omics signatures, quantification of lesion progression rates, as well as transmission experiments (to assess susceptibility) are among the diagnostic information urgently needed for stony coral species to facilitate comparison of the disease signs observed at FGB with the case definitions for white plague and SCTLD to help determine the nature of the ongoing event. A concerning observation was that the most SCTLD-susceptible species at FGBNMS (P. strigosa and C. natans) were the most affected; however, the overall prevalence of lesions at FGB was lower than at epidemic sites in the Caribbean region (Precht et al., 2016; NOAA, 2018). In addition, few observations of diseases and their progression on colony surfaces exist for the upper mesophotic reef habitats (~ 50 m), which are fairly extensive and well developed at FGBNMS (but see Brandt et al., 2021; Chaves-Fonnegra et al., 2021; Williams et al., 2021). Lesions could manifest differently at mesophotic depths as a function of light availability, depth-adapted algal symbionts (Correa et al., 2009) and/or lower temperatures than are typical of lesions described in case studies from shallow reefs (Miller and Richardson, 2015; Chaves-Fonnegra et al., 2021).
The factors influencing transmission of the ongoing disease at FGB require assessment. There is evidence that both SCTLD and white plague can be transmitted directly through tissue contact and the water column (Aeby et al., 2019; Muller et al., 2020; Cróquer et al., 2021). Biotic vectors may also be affecting the spread of this disease among colonies within East and West FGB. The high density of corals at FGB may itself facilitate disease transmission. Many colonies are space limited by the high cover on the banks (nearly 50%), and many colony margins are immediately adjacent to one another. In addition, divers on disease response cruises observed corallivory from parrotfish and fireworms, with the former removing relatively large areas of coral tissue and skeleton at lesion areas and colony margins. Recent reviews of predator behavior in coral disease dynamics found that corallivores may target lesion-affected coral tissue with often-unknown ecological consequences, but in several cases, predators may exacerbate disease impacts through the transportation or hosting of pathogens (Nicolet et al., 2018; Renzi et al., 2022). Parrotfish and/or butterflyfish corallivory may suppress lesion progression through the partial or complete removal of disease-associated tissue (Titus et al., 2022), or contrarily, exacerbate spread through oral or fecal deposition (but see Nicolet et al., 2018). Regardless, additional observations and more research over time are needed to discern potential mechanisms of coral disease spread in FGBNMS.
The resilience of FGBNMS coral reefs, often thought to result from their depth, remote location, and relatively stable environment from surrounding deep water, may be challenged as coral disease outbreaks and climate change effects intensify (Dee et al., 2019; Manzello et al., 2021; Lawman et al., 2022). For example, the dominant coral species at FGBNMS are considered to be highly- to moderately-susceptible to SCTLD, white plague, and other coral diseases. These species comprise 47% of the absolute benthic cover in the long-term monitoring sites (O. franksi: 30%; P. strigosa: 8%; C. natans: 2%; O. faveolata: 3%; O. annularis: 1%; and M. cavernosa: 3%; Johnston et al., 2020). While it is presently unclear which disease is affecting corals at FGB, an outbreak of a highly-virulent disease presents a substantial threat to the persistence of this system. Both SCTLD and white plague have been shown to have major impacts on coral reef ecosystems in the wider Caribbean (reviewed in Cróquer et al., 2021). Disease risk in FGBNMS is amplified by the difficulty in accessing, monitoring, and treating corals at these sites due to their remoteness, particularly during the winter months when weather conditions in the northwest Gulf of Mexico make dive operations challenging.
Regardless of the etiology of the current disease event, the response framework outlined in the FGB SCTLD Preparedness Plan completed the year prior (Johnston, 2021) allowed resource managers and research partners to mobilize quickly and respond efficiently to collect observational data and conduct sampling for disease diagnostic analyses. This included the collection of 386 discrete samples from 182 coral colonies to investigate causative agents and cellular manifestation of lesions. Tissue samples of disease-affected and visually healthy coral colonies were collected for downstream analyses with partner institutions, including transmission electron microscopy (TEM), histopathology, dsRNA-Immunofluorescence (Coy et al., 2022) and ‘omics (algal symbiont, bacteria and virus profiling, metagenomics/metatranscriptomics) from C. natans, P. strigosa, and/or O. faveolata. In addition, 50 mucus samples were collected from C. natans, P. strigosa, M. cavernosa, O. franksi, and O. faveolata species for probiotic screening to better understand how some bacterial symbionts can potentially serve as an alternative treatment option for coral hosts. As disease monitoring continues at the banks, additional samples from other species will be collected when possible if signs of disease are documented.
Disease progression will also be documented on future monitoring cruises, including additional images at repetitive monitoring stations and additional samples for microbial and ‘omics time series (Martindale et al., 2021; Shore et al., 2021). In addition to photostations surveyed during the initial disease response cruises, high-resolution photomosaics were collected using a custom rig consisting of a single SLR (EOS Rebel SL3, Canon) and two GoPro (HERO6) cameras. These photomosaics will be analyzed as part of NCRMP efforts to provide a landscape view of disease patterns and monitoring of large reef areas through time.
Whether the putative disease signs documented at East and West FGB do in fact indicate the presence of SCTLD, a type of white plague, or another disease, epidemic-level rapid coral tissue loss of any kind is likely to be detrimental to the FGB reef ecosystem. This is especially true given that intervention methods developed thus far have not shown success as a preventative treatment and are labor-intensive (Neely et al., 2021; Shilling et al., 2021; Walker et al., 2021). While antibiotic treatments may in some cases be used to promote recovery of individual lesions, the effort, time, and resources to repeatedly treat a remote, high-coral cover reef system are not presently feasible, especially since treatments do not necessarily mitigate disease spread, emergence of new lesions on treated colonies, or transport of pathogens. To what extent the arrival of this putative disease may threaten the health of the FGB reef system is unknown at this time, and the question remains whether the inherent resilience and remoteness of this reef ecosystem will buffer the impact severity and potential recovery. Through continued monitoring, research, and response, this question will be addressed, and collaborative analysis of samples collected will hopefully allow for a better understanding of the etiology of this disease outbreak at FGB.
Data availability statement
The raw data supporting the conclusions of this article will be made available by the authors, without undue reservation.
Author contributions
All authors contributed to the study conception and design. Data collection was performed by MJ, MS, IE, AC, NB, RE, KE, RH, XH, MN, KO’C, AP-C, and AS. Data analysis, tables, and graphics were prepared by MJ and MS. The manuscript was written by MJ, MS, IE, and AC. Authors contributing to sample analyses were MS, IE, AC, XH, NB, and BU. Funding was provided by GS, JV, XH, and AC. All authors provided input on previous versions of the manuscript. All authors contributed to the article and approved the submitted version.
Acknowledgments
We thank the United States Department of Interior’s Bureau of Ocean Energy Management (BOEM) for supporting the Flower Garden Banks National Marine Sanctuary long-term monitoring program. We thank the National Coral Reef Monitoring Program (NCRMP) biological team divers for initial observations. NCRMP is funded by NOAA Coral Reef Conservation Project #743. Work on this project was also supported by NSF award #2109622 (to AC), NOAA Ocean Acidification Program award #NA19OAR0170354 (to XH), and the National Marine Sanctuary Foundation. Response cruises were conducted in tandem with BOEM and FGBNMS Monitoring Programs, as well as the NCRMP Climate Cruise led by Ian Enochs at NOAA AOML. We thank the National Marine Sanctuary Foundation and Moody Gardens Aquarium for rapid response assistance. We thank scientific divers from the NOAA dive center, partnering aquariums (Moody Gardens, Texas State Aquarium, and Audubon Aquarium), and partnering academic Institutions (Texas A&M Galveston, Texas A&M Corpus Christi, University of Miami CIMAS, and NOAA AOML). We thank Marilyn E. Brandt (University of the Virgin Islands) for sharing her input and expertise related to the potential etiology of this disease event. Fieldwork and sampling were conducted under permit: FGBNMS-2019-001.
Conflict of interest
Author KE is employed by CSS, Inc., Authors RH, MN, and KO’C are employed by CPC Inc.
The remaining authors declare that the research was conducted in the absence of any commercial or financial relationships that could be construed as a potential conflict of interest.
Publisher’s note
All claims expressed in this article are solely those of the authors and do not necessarily represent those of their affiliated organizations, or those of the publisher, the editors and the reviewers. Any product that may be evaluated in this article, or claim that may be made by its manufacturer, is not guaranteed or endorsed by the publisher.
Supplementary material
The Supplementary Material for this article can be found online at: https://www.frontiersin.org/articles/10.3389/fmars.2023.1111749/full#supplementary-material
Supplementary Table 1 | Combined data for all coral species with lesions within East and West Flower Garden Bank Long Term Monitoring (FGB LTM, northwest Gulf of Mexico) sites from Diver Propulsion Vehicle (DPV) and diver surveys.
Supplementary Table 2 | Percent cover, coral species, total number of coral colonies observed and number with disease lesions, and prevalence with 95% upper and lower confidence intervals for East and West Flower Garden Bank (FGB, northwest Gulf of Mexico) repetitive monitoring stations.
Supplementary Figure 1 | Daily mean seawater temperature (°C) at East Flower Garden Bank (FGB, 21 m) and West FGB (27 m) from June to August 2022. The threshold known to trigger bleaching at FGB is 50 days above 29.5 (°C); however, temperatures did not exceed this threshold in 2022 (27 days at EFGB and 21 days at WFGB).
Supplementary Figure 2 | Satellite derived daily mean surface salinity (psu) at East Flower Garden Bank (FGB) and West FGB in 2022.
References
Aeby G., Ushijima B., Bartels E., Walter C., Kuehl J., Jones S., et al. (2021). Changing stony coral tissue loss disease dynamics through time in Montastraea cavernosa. Front. Mar. Sci. 8. doi: 10.3389/fmars.2021.699075
Aeby G. S., Ushijima B., Campbell J. E., Jones S., Williams G. J., Meyer J. L., et al. (2019). Pathogenesis of a tissue loss disease affecting multiple species of corals along the Florida reef tract. Front. Mar. Sci. 6. doi: 10.3389/fmars.2019.00678
Aronson R. B., Precht W. F., Murdoch T. J., Robbart M. L. (2005). Long-term persistence of coral assemblages on the flower garden banks, northwestern gulf of Mexico: implications for science and management. Gulf Mexico Sci. 23 (1), 84–94. doi: 10.18785/goms.2301.06
Borneman E., Wellington G. (2005). Pathologies affecting reef corals at the flower garden banks, northwestern gulf of Mexico. Gulf Mexico Sci. 23, 95–106. doi: 10.18785/goms.2301.07
Brandt M. E., Ennis R. S., Meiling S. S., Townsend J., Cobleigh K., Glahn A., et al. (2021). The emergence and initial impact of stony coral tissue loss disease (SCTLD) in the united states virgin islands. Front. Mar. Sci. 8. doi: 10.3389/fmars.2021.715329
Bythell J., Pantos O., Richardson L. (2004). “White plague, white band, and other ‘White’ diseases,” in Coral health and disease. Eds. Rosenberg E., Loya Y. (Berlin, Heidelberg: Springer), 351–365. doi: 10.1007/978-3-662-06414-6_20
Chaves-Fonnegra A., Panassiti B., Smith T. B., Brown E., Clemens E., Sevier M., et al. (2021). Environmental and biological drivers of white plague disease on shallow and mesophotic coral reefs. Ecography 44, 1071–1085. doi: 10.1111/ecog.05527
Clark R., Taylor J. C., Buckel C. A., Kracker L. M. (2014). “Fish and benthic communities of the flower garden banks national marine sanctuary: Science to support sanctuary management,” in NOAA Technical memorandum NOS NCCOS 179 (MD: Silver Spring), 317 pp.
Coles S. L., Jokiel P. L. (1992). “Effects of salinity on coral reefs,” in Pollution in tropical aquatic systems. Eds. Connell D. W., Hawker D. W. (Boca Raton, FL: CRC Press), 147–166.
Correa A. M. S., Brandt M. E., Smith T. B., Thornhill D. J., Baker A. C. (2009). Symbiodinium associations with diseased and healthy scleractinian corals. Coral Reefs 28, 437–448. doi: 10.1007/s00338-008-0464-6
Coy S., Utama B., Spurlin J., Kim J., Deshmukh H., Lwigale P., et al. (2022). Visualization of RNA virus infection in a marine protist with a universal biomarker. Res. Square [preprint] 24. doi: 10.21203/rs.3.rs-2227466/v1
CRCP (2022a). “National coral reef monitoring program (NCRMP) benthic community assessment survey field protocols for U.S. Atlantic: Florida, flower garden banks, Puerto Rico, U.S. virgin islands 2022,” in NOAA Coral reef conservation program, vol. 29. (Silver Spring, MD: National Oceanic and (NOAA) Atmospheric Administration). doi: 10.25923/0708-8333
CRCP (2022b). “National coral reef monitoring program (NCRMP) coral demographics survey field protocols for U.S. Atlantic: Florida, flower garden banks, Puerto Rico, U.S. virgin islands 2022,” in NOAA Coral reef conservation program, vol. 27. (Silver Spring, MD: National Oceanic and (NOAA) Atmospheric Administration). doi: 10.25923/9A1R-M911
Cróquer A., Weil E., Rogers C. S. (2021). Similarities and differences between two deadly Caribbean coral diseases: white plague and stony coral tissue loss disease. Front. Mar. Sci. 8. doi: 10.3389/fmars.2021.709544
Dee S. G., Torres M. A., Martindale R. C., Weiss A. M., DeLong K. L. (2019). The future of reef ecosystems in the gulf of Mexico: insights from coupled climate model simulations and ancient hot-house reefs. Front. Mar. Sci. 6. doi: 10.3389/FMARS.2019.00691
Doyle S. M., Self M. J., Hayes J., Shamberger K. E. F., Correa A. M. S., Davies S. W., et al. (2022). Microbial community dynamics provide evidence for hypoxia during a coral reef mortality event. Appl. Environ. Microbiol. 88, e0034722. doi: 10.1128/aem.00347-22
Eakin C. M., Morgan J. A., Heron S. F., Smith T. B., Liu G., Alvarez-Filip L., et al. (2010). Caribbean Corals in crisis: record thermal stress, bleaching, and mortality in 2005. PloS One 5, e13969. doi: 10.1371/journal.pone.0013969
Gil-Agudelo D. L., Cintra-Buenrostro C. E., Brenner J., González-Díaz P., Kiene W., Lustic C., et al. (2020). Coral reefs in the gulf of Mexico Large marine ecosystem: conservation status, challenges, and opportunities. Front. Mar. Sci. 6. doi: 10.3389/fmars.2019.00807
Gintert B. E., Precht W. F., Fura R., Rogers F., Rice M., Precht L. L., et al. (2019). Regional coral disease outbreak overwhelms impacts from a local dredge project. Environ. Monit. Assess. 191, 630. doi: 10.1007/s10661-019-7767-7
Jackson J. B., Donovan M. K., Cramer K., Lam V. (2014). “Status and trends of Caribbean coral reefs: 1970-2012,” in IUCN, gland (Global Coral Reef Monitoring Network), 306.
Jackson J. B. C., Donovan M. K., Donovan K. L., Lam V. V. (editors) (2014). Status and Trends of Caribbean Coral Reefs: 1970-2012. Global Coral Reef Monitoring Network, IUCN, Gland, Switzerland.
Johnston M. A. (2021). Strategy for stony coral tissue loss disease prevention and response at flower garden banks national marine sanctuary Vol. 30 (Galveston, TX: U.S. Department of Commerce, National Oceanic and Atmospheric Administration, Flower Garden Banks National Marine Sanctuary). doi: 10.25923/5MBM-EQ74
Johnston M. A., Eckert R. J., Hickerson E. L., Nuttall M. F., Embesi J. A., Sterne T. K., et al. (2019a). Localized coral reef mortality event at East flower garden bank, gulf of Mexico. Bull. Mar. Sci. 95, 239–250. doi: 10.5343/bms.2018.0057
Johnston M. A., Embesi J. A., Eckert R. J., Nuttall M. F., Hickerson E. L., Schmahl G. P. (2016). Persistence of coral assemblages at East and West flower garden banks, gulf of Mexico. Coral Reefs 35, 821–826. doi: 10.1007/s00338-016-1452-x
Johnston M. A., Hickerson E. L., Nuttall M. F., Blakeway R. D., Sterne T. K., Eckert R. J., et al. (2019b). Coral bleaching and recovery from 2016 to 2017 at East and West flower garden banks, gulf of Mexico. Coral Reefs 38, 787–799. doi: 10.1007/s00338-019-01788-7
Johnston M. A., O’Connell K. O., Blakeway R. D., MacMillan J., Nuttall M. F., Hu X., et al. (2020). Long-term monitoring at East and West flower garden banks: 2019 annual report Vol. 88 (Galveston, TX: U.S. Department of Commerce, National Oceanic and Atmospheric Administration, Flower Garden Banks National Marine Sanctuary).
Kealoha A. K., Doyle S. M., Shamberger K. E. F., Sylvan J. B., Hetland R. D., DiMarco S. F. (2020). Localized hypoxia may have caused coral reef mortality at the flower garden banks. Coral Reefs 39, 119–132. doi: 10.1007/s00338-019-01883-9
Kramer P. R., Roth L., Lang J. (2019) Map of stony coral tissue loss disease outbreak in the Caribbean (ArcGIS Online). Available at: www.agrra.org (Accessed 14 Oct 2022).
Lawman A. E., Dee S. G., DeLong K. L., Correa A. M. S. (2022). Rates of future climate change in the gulf of Mexico and the Caribbean Sea: implications for coral reef ecosystems. J. Geophys. Res.: Biogeosci. 127, e2022JG006999. doi: 10.1029/2022JG006999
Manzello D. P., Kolodziej G., Kirkland A., Besemer N., Enochs I. C. (2021). Increasing coral calcification in Orbicella faveolata and Pseudodiploria strigosa at flower garden banks, gulf of Mexico. Coral Reefs 40, 1097–1111. doi: 10.1007/s00338-021-02108-8
Martindale R. C., Holstein D., Knowlton N., Voss J. D., Weiss A. M., Correa A. M. S. (2021). Editorial: Gulf of Mexico reefs: past, present and future. Front. Mar. Sci. 8. doi: 10.3389/fmars.2021.713058
Meiling S. S., Muller E. M., Lasseigne D., Rossin A., Veglia A. J., MacKnight N., et al. (2021). Variable species responses to experimental stony coral tissue loss disease (SCTLD) exposure. Front. Mar. Sci. 8. doi: 10.3389/fmars.2021.670829
Miller A. W., Richardson L. L. (2015). Emerging coral diseases: a temperature-driven process? Mar. Ecol. 36, 278–291. doi: 10.1111/maec.12142
Miller C. V., May L. A., Moffitt Z. J., Woodley C. M. (2020). Exploratory treatments for stony coral tissue loss disease: Pillar coral (Dendrogyra cylindrus) Vol. 78 (Charleston, SC: National Centers for Coastal Ocean Science, National Ocean Service National Oceanic and Atmospheric Administration). NOAA Technical Memorandum NOS NCCOS 245 and CRCP 37. doi: 10.25923/D632-JC82
Muller E. M., Sartor C., Alcaraz N. I., van Woesik R. (2020). Spatial epidemiology of the stony-coral-tissue-loss disease in Florida. Front. Mar. Sci. 7. doi: 10.3389/fmars.2020.00163
Neely K. L., Shea C. P., Macaulay K. A., Hower E. K., Dobler M. A. (2021). Short- and long-term effectiveness of coral disease treatments. Front. Mar. Sci. 8. doi: 10.3389/fmars.2021.675349
Nicolet K. J., Chong-Seng K. M., Pratchett M. S., Willis B. L., Hoogenboom M. O. (2018). Predation scars may influence host susceptibility to pathogens: evaluating the role of corallivores as vectors of coral disease. Sci. Rep. 8, 5258. doi: 10.1038/s41598-018-23361-y
NOAA (2018). “Stony coral tissue loss disease case definition,” in NOAA, vol. 10. (MD: Silver Spring).
Noonan K. R., Childress M. J. (2020). Association of butterflyfishes and stony coral tissue loss disease in the Florida keys. Coral Reefs 39, 1581–1590. doi: 10.1007/s00338-020-01986-8
Precht W. F., Gintert B. E., Robbart M. L., Fura R., Van Woesik R. (2016). Unprecedented disease-related coral mortality in southeastern Florida. Sci. Rep. 6, 31374. doi: 10.1038/srep31374
Richardson L. L., Goldberg W. M., Kuta K. G., Aronson R. B., Smith G. W., Ritchie K. B. (1998). Florida’s mystery coral-killer identified. Nature 392, 557–558. doi: 10.1038/33302
Renzi J. J., Shaver E. C., Burkepile D. E., Silliman B. R. (2022). The role of predators in coral disease dynamics. Coral Reefs 41, 405–422. doi: 10.1038/33302
Robbart M. L., Aronson R. B., Deslarzes K. J., Precht W. F., Duncan L., Zimmer B., et al. (2009). “Post-hurricane assessment of sensitive habitats of the flower garden banks vicinity,” in United states department of the interior. minerals management service, gulf of Mexico OCS region (New Orleans, Louisiana: OCS Study MMS), 160 pp.
Ruiz-Moreno D., Willis B. L., Page A. C., Weil E., Cróquer A., Vargas-Angel B., et al. (2012). Global coral disease prevalence associated with sea temperature anomalies and local factors. Dis. Aquat. Org. 2012100 (3), 249–261. doi: 10.3354/dao02488
Shilling E. N., Combs I. R., Voss J. D. (2021). Assessing the effectiveness of two intervention methods for stony coral tissue loss disease on Montastraea cavernosa. Sci. Rep. 11, 8566. doi: 10.1038/s41598-021-86926-4
Shore A., Sims J. A., Grimes M., Howe-Kerr L. I., Grupstra C. G. B., Doyle S. M., et al. (2021). On a reef far, far away: anthropogenic impacts following extreme storms affect sponge health and bacterial communities. Front. Mar. Sci. 8. doi: 10.3389/fmars.2021.608036
Studivan M. S., Baptist M., Molina V., Riley S., First M., Soderberg N., et al. (2022a). Transmission of stony coral tissue loss disease (SCTLD) in simulated ballast water confirms the potential for ship-born spread. Sci. Rep. 12, 19248. doi: 10.1038/s41598-022-21868-z
Studivan M. S., Rossin A. M., Rubin E., Soderberg N., Holstein D. M., Enochs I. C. (2022b). Reef sediments can act as a stony coral tissue loss disease vector. Front. Mar. Sci. 8. doi: 10.3389/fmars.2021.815698
Titus K., O’Connell L., Matthee K., Childress M. (2022). The influence of foureye butterflyfish (Chaetodon capistratus) and symbiodiniaceae on the transmission of stony coral tissue loss disease. Front. Mar. Sci. 9. doi: 10.3389/fmars.2022.800423
van Woesik R., Randall C. J. (2017). Coral disease hotspots in the Caribbean. Ecosphere 8 (5), e01814. doi: 10.1002/ecs2.1814
Veglia A. J., Beavers K., Buren E. W. V., Meiling S. S., Muller E. M., Smith T. B., et al. (2022). Alphaflexivirus genomes in stony coral tissue loss disease-affected, disease-exposed, and disease-unexposed coral colonies in the U.S. virgin islands. Microbiol. Res. Announc. 11, e01199–e01121. doi: 10.1128/MRA.01199-21
Walker B. K., Turner N. R., Noren H. K. G., Buckley S. F., Pitts K. A. (2021). Optimizing stony coral tissue loss disease (SCTLD) intervention treatments on Montastraea cavernosa in an endemic zone. Front. Mar. Sci. 8. doi: 10.3389/fmars.2021.666224
Weil E., Smith G., Gil-Agudelo D. L. (2006). Status and progress in coral reef disease research. Dis. Aquat. Org. 69, 1–7. doi: 10.3354/dao069001
Williams S., García-Sais J., Sabater-Clavell J. (2021). Prevalence of stony coral tissue loss disease at El seco, a mesophotic reef system off vieques island. Front. Mar. Sci. 8. doi: 10.3389/fmars.2021.668669
Woodley C. M., Downs C. A., Bruckner A. W., Porter J. W., Galloway S. B. (2016). Diseases of coral (Hoboken, NJ: John Wiley & Sons).
Work T. M., Weatherby T. M., Landsberg J. H., Kiryu Y., Cook S. M., Peters E. C. (2021). Viral-like particles are associated with endosymbiont pathology in Florida corals affected by stony coral tissue loss disease. Front. Mar. Sci. 8. doi: 10.3389/fmars.2021.750658
Keywords: coral disease, Gulf of Mexico, marine sanctuary, stony coral tissue loss disease, white plague disease, disease intervention
Citation: Johnston MA, Studivan MS, Enochs IC, Correa AMS, Besemer N, Eckert RJ, Edwards K, Hannum R, Hu X, Nuttall M, O’Connell K, Palacio-Castro AM, Schmahl GP, Sturm AB, Ushijima B and Voss JD (2023) Coral disease outbreak at the remote Flower Garden Banks, Gulf of Mexico. Front. Mar. Sci. 10:1111749. doi: 10.3389/fmars.2023.1111749
Received: 30 November 2022; Accepted: 11 January 2023;
Published: 26 January 2023.
Edited by:
William F. Precht, Dial Cordy and Associates, Inc., United StatesReviewed by:
Adán Guillermo Jordán-Garza, Universidad Veracruzana, MexicoSteven Miller, Nova Southeastern University, United States
Copyright © 2023 Johnston, Studivan, Enochs, Correa, Besemer, Eckert, Edwards, Hannum, Hu, Nuttall, O’Connell, Palacio-Castro, Schmahl, Sturm, Ushijima and Voss. This is an open-access article distributed under the terms of the Creative Commons Attribution License (CC BY). The use, distribution or reproduction in other forums is permitted, provided the original author(s) and the copyright owner(s) are credited and that the original publication in this journal is cited, in accordance with accepted academic practice. No use, distribution or reproduction is permitted which does not comply with these terms.
*Correspondence: Michelle A. Johnston, bWljaGVsbGUuYS5qb2huc3RvbkBub2FhLmdvdg==