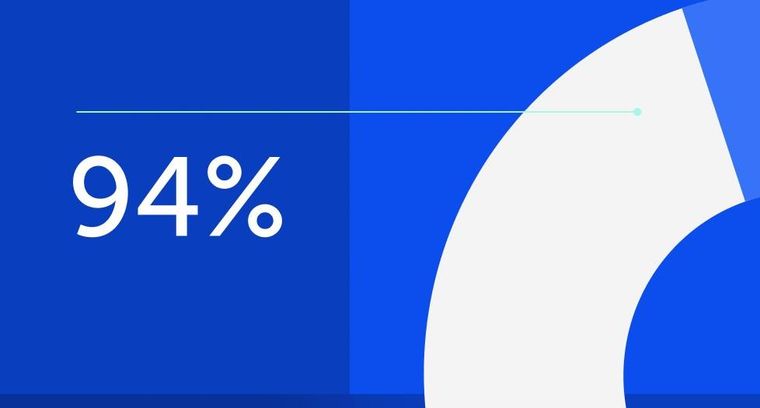
94% of researchers rate our articles as excellent or good
Learn more about the work of our research integrity team to safeguard the quality of each article we publish.
Find out more
ORIGINAL RESEARCH article
Front. Mar. Sci., 24 April 2023
Sec. Marine Pollution
Volume 10 - 2023 | https://doi.org/10.3389/fmars.2023.1111067
The study aimed to investigate the effect of hydrogen on nitrogen and phosphorus removal and microbial community composition in aquaculture water. Two groups were designed separated: Group H was charged with hydrogen and Group D was set as control. Water samples were collected at 0, 1, 2, 3, 4, 5, 6, and 7 days after hydrogen injection to detect not only the content of total nitrogen, ammonia nitrogen and phosphorus, but also microbial community composition in aquaculture water. Our findings have demonstrated that 7-days’ hydrogen treatment could effectively reduce ammonia nitrogen in aquaculture water. And in Day 7, the concentration of ammonia nitrogen in Group D was 1.66 times as that in Group H. Meanwhile, microbial structures in the water bodies of these two groups were significantly different. The abundance of bacteria such as Proteobacteria, Firmicutes, Bacteroidetes, and Desulphuria increased clearly in Group H, which means nitrification and denitrification occurred in the effect of hydrogen injection. These findings suggested that hydrogen could significantly improve aquaculture water health and reduce the production of ammonia nitrogen, which means hydrogen could be used as a functional external method to protect the aquaculture environment.
In recent years, affected by overfishing and environmental pollution in the natural environment, human beings began to rely on aquaculture to maintain the continuous supply of protein sources. Aquaculture industry has developed rapidly in the world, profoundly changing the global food consumption pattern, and bringing huge economic profits to fishery operators (Bohnes et al., 2022). In particularly, the intensive aquaculture mode developed rapidly, but its high input and high-yield aquaculture mode made the content of ammonia nitrogen, nitrite nitrogen, total nitrogen, total phosphorus, organic matter and other substances in the aquaculture water exceed the standard, and then it caused a large number of pathogenic microorganisms to multiply. It could further cause the eutrophication of the aquaculture water body to become increasingly serious (Becke et al., 2019), which not only puts great pressure on the ecological environment but also endangers the normal development and health of cultured animals, and this has seriously damaged the economic benefits of fishery practitioners (Ahmad et al., 2022).
As an important environmental factor in the aquaculture water body, ammonia nitrogen is an important indicator of water quality monitoring. It exists in the water body in the form of ionic ammonia and non-ionic ammonia (Camargo and Alonso, 2006). With the rapid development of high-density intensive aquaculture, excessive ammonia nitrogen in the aquaculture water body has become a common environmental problem. The main source of ammonia nitrogen in aquaculture water is usually from the feed and additives of aquatic animal (Zhou and Boyd, 2015; Boyd, 2018). During the breeding process, nitrogen containing organic substances in residual bait, residues, excreta and the carcasses of aquatic animal are released into the water body (Zhou and Boyd, 2015). It is worth noting that this kind of situation is more likely to occur in the high-density breeding mode (Anh et al., 2010), resulting in the accumulation of ammonia nitrogen. Studies have shown that non-ionic ammonia is the main form of toxicity that causes physiological stress on aquatic animals. Its toxicity is about 300 to 400 times that of ionic ammonia (Xu et al., 2021). Therefore, non-ionic ammonium is a pollutant which could pose great threat to the health of aquatic organisms (Zheng et al., 2021; Guo et al., 2022). Under ammonia nitrogen stress, fish will have acute and chronic toxic effects, and then significant oxidative stress and severe inflammation could be detected in fish. Studies have shown that the increase of non-ionic ammonia will inhibit the excretion of ammonia nitrogen in fish (Xu et al., 2021). In addition, because of good fat solubility of non-ionic ammonia, it is easy to diffuse into the body through the cell membrane, causing damage to important organs such as gill, reducing the oxygen carrying function of hemoglobin which could transport oxygen. As a result of tissue hypoxia, elevated blood CO2 concentration and pH value would lead fish dyspnea and decreasing feeding rate, inhibiting its growth and development (Jia et al., 2017; Liu et al., 2022). Ammonia nitrogen stimulates a series of toxic reactions, such as hypoxia convulsion, agitation or unresponsiveness of fish until death, which seriously damages the health status of breeding animals and the economic benefits of aquaculture practitioners (Camargo and Alonso, 2006). Therefore, there is a need to focus on the treatment of ammonia nitrogen and other pollutants when dealing with aquaculture water.
Studies have showed that different aquatic organisms have different tolerance to ammonia nitrogen. For example, bluegill is more sensitive while channel catfish has better resistance (Huff et al., 2013a). Nitrogen forms are diverse and can be transformed into each other, so nitrogen cycle generally exists in the material cycle system composed of the earth’s air, water and soil environment (Canfield et al., 2010). As far as the aquaculture system is concerned, ammonia nitrogen, nitrate nitrogen, nitrite nitrogen and other nitrogen forms will transform and migrate mutually in these environments in the body of water, sediment and aquatic organisms (Zerkle and Mikhail, 2017; Rose et al., 2021). Their chemical processes are extremely complex and changeable, which could affected by physical, chemical, biological and other factors, and each process interacts and influences each other. It is generally believed that nitric acid nitrogen is non-toxic to aquatic organisms, ammonia nitrogen is toxic, and the intermediate product nitrite nitrogen is toxic, while nitrogen is stable and non-toxic which cannot be directly used by aquatic organisms, and does not participate in the nitrogen transformation process in water (Lou et al., 2016; Kim et al., 2019). It has always been a hot research field for researchers on how to transform toxic nitrogen in the aquaculture water into non-toxic nitrogen or low toxic nitrogen forms (Bucking, 2017). There have been many studies on how to prepare the ammonia nitrogen concentration in aquaculture water, among which the microbial action is the most widely used because of its high efficiency, green and low cost, such as adding microbial agents (Yang et al., 2019; Praveen et al., 2021). Microbial treatment refers to the transformation of ammonia nitrogen through the chemical reaction in the process of microbial growth and metabolism. Adjusting the composition of the microbial community in the water body to realize the biotechnology of using different microorganisms to transform nitrogen to regulate aquaculture water quality has become quite important in the field of aquaculture (Niu et al., 2021). For example, under the action of nitrifying bacteria, the nitrification reaction could take place, which converts the highly toxic ammonia nitrogen into less toxic nitrite or basically non-toxic nitrate. Some products are converted into nitrogen through the denitrification process, thus effectively reducing the concentration of ammonia nitrogen (Yasuda et al., 2017; Holmes et al., 2019). In recent years, there are many researches on nitrogen removal in wastewater. According to the principle of nitrate removal, it can be divided into physical method, chemical method and biological method. It is widely used in industrial wastewater, domestic sewage and aquaculture water. Compared to other sewage treatment, in terms of aquaculture water treatment, its demand for treatment methods is more economic, safe and simple. Autotrophic denitrification is a new biological denitrification technology to remove nitrate in water., Kurt first used hydrogen to carry out hydrogen autotrophic denitrification in a conical reactor (Kurt et al., 1987). The principle is that hydrogen is used as an electronic donor by autotrophic microorganisms to reduce the oxidizing pollutant NO3- in water to N2 (Karanasios et al., 2010). Compared with other physical and chemical methods such as adsorption method, chemical precipitation method and active metal reduction method, it has the characteristics of lower cost such as no addition of potentially toxic chemical reagents, harmless to cultured animals, simple operation and easy maintenance (Broman et al., 2017; Zha et al., 2020). It has achieved remarkable results in many water treatment fields such as high ammonia wastewater treatment and drinking water treatment, and most of these applications tested by the hydrogen nutrition process have achieved high efficiency and high denitrification rate (Karanasios et al., 2010; Shinoda et al., 2020). Considering that the aquaculture mode is generally medium and large intensive farms and individual farmers, it is more suitable for the aquaculture water treatment mode.
This study innovatively used the method of filling hydrogen into the aquaculture water body to demonstrate whether the removal of nitrogen and phosphorus were detected and the different pattern of microbial community composition and structure in the water body. And this work would provide the theoretical basis and technical support for removing ammonia nitrogen in aquaculture water.
The aquaculture water used in the experiment is from aquaculture pond of largemouth bass (Micropterus salmoides) in the National Agricultural Science and Technology Park’s aquaculture pond in Baiyun District, Guangzhou, China. Ammonium chloride (NH4Cl, GR, 99.8%) was purchased from Shanghai MACKLIN Biochemical Technology Co., LTD.
12 round plastic buckets of 300-L capacity were prepared and then they were filled with 200 L aquaculture water. Before the beginning of experiment, 2 g ammonium chloride were added into these buckets respectively, and then the 12 plastic buckets were divided into Group D and Group H separately. Group D was set as control which means no hydrogen filling into the water. Group H was charged 1000 ppm/ton/hour hydrogen for 15 minutes at 9:00 am and 3:00 pm everyday. Water samples were collected at 9:00 pm in 0, 1, 2, 3, 4, 5, 6, and 7 days after hydrogen injection to detect the content of total nitrogen, ammonia nitrogen, and phosphorus. Water samples after 7-days’ treatment were collected to analyze the microbial community composition.
PH of the water body is measured with a pH meter (Orion Research Inc., Beverly, MA, USA) and the content of total nitrogen, ammonia nitrogen, and phosphorus were determined with ultraviolet UV spectrometer (Youke Instrument Technology Co., Ltd., Shanghai, China).
The microbial community structure and diversity of Group D and Group H were analyzed by high-throughput sequencing technology. Microbial DNA from water sample per tank in Group D and Group H were extracted with EZNA Fecal DNA Kit (Omega Bio-tek, Inc., United States). The V3-V4 variable region of the 16S rRNA gene was amplified by PCR. Amplicons were separated on a 2% agarose gel, followed by purifying using the AxyPrep DNA Gel Extraction Kit (Axygen Biosciences, Union City, CA, USA), and quantifying using an ABI StepOnePlus real-time PCR instrument (Life Technologies, Carlsbad). Then, purified amplicons were mixed in equimolar amounts and paired-end sequencing (2×250) was conducted on an Illumina platform (HiSeq 2,500, Illumina, San Diego, CA, United States). After filtering for noisy sequences and checking for the presence of chimeras, data were assigned to the same operational taxonomic units (OTUs). Intergroup Venn analysis was conducted to identify endemic and shared OTUs using the microbial indicative analysis function of the Omicshare platform (https://www.omicshare.com/). Moreover, the overall microbial composition was conducted with beta analysis through partial least squares discriminant analysis in the Omicshare platform. It was using the RDP classifier software for microbial species annotation (version 2.2, http://sourceforge.net/projects/rdpclassifier/).
As shown in Figure 1, with the extension of treatment time, the concentration of ammonia nitrogen in the water body of Group H decreased significantly with the extension of treatment time, which was significantly lower than that of Group D from the fourth day of treatment (P<0.05). 7 days later, compared to initial concentration, the concentration of ammonia nitrogen in Group D (Control) increased by 45.21%, but the concentration of ammonia nitrogen in Group H decreased by 16.33%. And in Day 7, the concentration of ammonia nitrogen in Group D was 1.66 times as that of Group H. However, there was no significant difference in total nitrogen content and total phosphorus content between Group D and Group H (P>0.05).
Figure 1 Effect of hydrogen on (A) ammonia nitrogen content, (B) total nitrogen content and (C) total phosphorus content of aquaculture water. Different lowercase letters above each bar in each group represent significant differences (P < 0.05).
After 7 days of treatment, as indicated in Table 1, Sobs index, ACE index, Chao1 index, and Shannon Weiner index of microorganisms in aquaculture water in Group H were significantly higher than those in Group D (Control) (P <0.05), while Simpson index was significantly lower than that in Group D (P <0.05).
Figure 2, which showed that Group D (Control) and Group H were obviously separated. PCoA analysis demonstrated substantial differences between Group D (Control) and Group H, indicating that hydrogen had significant effects on the autochthonous microbiota compared to the control.
From Figure 3, we can know that after 7-days’ treatment, Group D and Group H has different pattern of microbial community composition. Compared with Group D, the abundance of Actinobaciota, Patescibacteria, Cyanobacteria, Chloroflexi, Planctomycota, Verrucomicrobiota decreased in Group H, while the abundance of Proteobacter, Firmicutes, Bacteroidota, Desulfobacter increased. As explained in Figure 4, on the phyla level, the abundance of Proteobacteria, Firmicutes, Bacteroides and Desulfurized bacteria in Group H was significantly higher than that in Group D (P <0.05), while the abundance of Actinomycetes, Patella bacteria, Cyanobacteria, Planctomycota and Verrucomicrobia in Group H was significantly lower than that in Group D (P<0.05).
Figure 4 Effect of hydrogen on the difference in microbial community composition of phyla level in aquaculture water after 7 days. “*” means P < 0.05 and “**” means P < 0.01.
The genus level as shown in Figure 5, the abundance of norank_f:norank_o:Saccharimonadales, Desulfovibrio, Dechromonas, Paeniclostridium, Clostridium_sensu_stricto_1, Dechromobacter in Group H was significantly higher than that of Group D (P <0.05), and the abundance of TM7a, CL500-29_marine_group was significantly lower than that of Group D (P <0.05). The species level as shown in Figure 6, the abundance of Dechloromonas_sp._An3-5 and norank_o_Saccharimonadales in Group H was significantly higher than that of Group D (P <0.05).
Figure 5 Effect of hydrogen on the difference in microbial community composition of genus level in aquaculture water after 7 days. “*” means P < 0.05 and “**” means P < 0.01.
Figure 6 Effect of hydrogen on the difference in microbial community composition of species level in aquaculture water after 7 days. “*” means P < 0.05 and “**”means P < 0.01.
Considering that the aquaculture mode is generally medium and large intensive farms and individual farmers, it is more suitable for the aquaculture water treatment mode (Li et al., 2021). Relying only on the system of self-regulation can’t guarantee the stability and harmless of the nitrogen cycle in the breeding environment, it is necessary to use external factors to control and promote the transformation direction of the nitrogen form (Akaboci et al., 2018). Nitrification and denitrification process are the most important nitrogen absorption process and mechanism in aquaculture water body (Zhang et al., 2019; Martínez-Espinosa et al., 2020). The present study showed, the ammonia nitrogen content in the water body in Group H was gradually decreased from the initial concentration in the process of hydrogen injection. However, the content of ammonia nitrogen in Group D gradually increased. After 7 days’ treatment, the ammonia nitrogen content in Group H was significantly lower than that in Group D (P <0.05). This suggests that the ammonia nitrogen concentration was significantly reduced in the progression of nitrogen cycle in water. Compared with Group D with 2.4 mg/L ammonia nitrogen, the concentration of ammonia nitrogen in Group H is at a safer level of 1.4 mg/L. For the sensitive aquaculture animals, with a very positive significance, this indicates that water may be more conducive to the survival and development of breeding animals after hydrogen filling treatment. For example, previous study showed that under the environmental conditions of gradient ammonia nitrogen concentration, the three common cyprinid tissues such as liver and gills have a higher health level at low ammonia nitrogen concentration (Wang et al., 2017). In our research here, the results of physical and chemical analysis showed that during the 7-days’ experiment, the ammonia nitrogen content in Group D increased significantly, while the ammonia nitrogen content in Group H decreased significantly.
The microbial diversity of the fish aquaculture water body is closely related to the stability of the water quality environment (Woese, 1994). When the diversity of water microbial community increases, the probability of aquatic biological diseases can be reduced (Woese, 1994; Niu et al., 2019). This study showed that the microbial Sobs index, ACE index, Chao1 index and Shannon-Wiener index of Group H were significantly higher than Group D (P <0.05), while the Simpson index of Group H was significantly lower than Group D p (P <0.05), which showed that a diversity of the microbial community increased significantly, which was conducive to ensuring the circulation of carbon, nitrogen, phosphorus and other elements in the water body and increasing the stability of the water environment (Fuhrman and Jed, 2009). At present, the effect of hydrogen injection in large-scale aquaculture water bodies on microbial communities is not studied. Noting that the study of microbial community changes in large reservoirs by some researchers (Lymperopoulou et al., 2012), it is suggested that the microbial community composition will change with water quality in environments with different spatial sizes, and the influence of hydrogen injection in aquaculture water bodies of large spatial scale could be investigated next.
The microbial community structure of the aquaculture water body is very complex and changeable, which not only affects the water environmental indicators, but also may be closely related to the gut microbial community of the aquatic organisms (Jin et al., 2017). Proteobacteria and bacteroidetes are dominant species of bacteria widely distributed in freshwater bodies. (Henne et al., 2012; Vaz-Moreira et al., 2013; Vaz-Moreira et al., 2017).In Group H of this study, proteobacteria replaced actinomycetes as the first dominant species compared with Group D. At the species level, Dechloromonas_sp._An3-5 and norank_o_Saccharimonadales abundances of Group H were significantly higher than these of Group D (P <0.05). Dechloromonas which is commonly used in the denitrification treatment system, is a denitrifier which belongs to the family Proteobacteria. And Dechloromonas always has participated in the denitrification process of all the enzyme line, which involved in the metabolism of nitrogen such as reducing NO3- to N2 (Coates et al., 2001; Pieper and Santos, 2004; Yang et al., 2021). In addition, some bacteria in Proteobacteria, Firmicutes, Bacteroidetes, and Desulphuria all have the function of denitrification (He et al., 2016; Wu et al., 2020; Wang et al., 2021). Not all denitrifiers could undergo complete denitrification, and these microorganisms acted together on the denitrification chain, indicating positive implications for NO2- and NO3- in many wastewater treatment systems. Norank_o_Saccharimonadales is related to the metabolism of sugar, and it is the main microorganism of the hydrolysis and fermentation of macromolecular organic matter in the system (Liu et al., 2020). It is closely related to the degradation of organic matter in the water body, which is conducive to the improvement of water quality. Our results also suggest that the above two bacteria might be related to hydrogen autotrophic denitrifying bacteria. The groups of bacteria with nitrification and denitrification in Group H, such as Proteus, Desulfurization bacteria, abundance of Dechloromonas_sp._An3-5 and norank_o_Saccharimonadales was increased, which shows that there are huge differences in the nitrogen cycle process between Group D and Group H. Ammonia nitrogen changes to nitrite nitrogen and nitrate nitrogen which was with lower toxicity, and then obvious nitrification and denitrification occur in the water. As nitrate is the highest valence compound of nitrogen element, it can be reduced to low valence nitrogen. In this experiment, the process is accelerated by filling the reducing gas hydrogen. At this stage, microorganisms with nitrification and denitrification functions could become the dominant bacteria in the system, which might also imply that some strains belong to hydrogen autotrophic denitrifying bacteria. And we believe that it requires further experiments to explore the denitrifying microbial population suitable for aquaculture water.
In conclusion, our findings have demonstrated that 7-days’ hydrogen treatment could effectively reduce ammonia nitrogen concentration in aquaculture water. Meanwhile, hydrogen significantly changed the microbial structures and distributions in the water bodies. The abundance of bacteria such as Proteobacteria, Firmicutes, Bacteroidetes, and Desulphuria increased clearly in Group H, which means nitrification and denitrification occurred in the effect of hydrogen injection. These findings suggested that hydrogen could significantly improve aquaculture water health, reduce the production of ammonia nitrogen, which means hydrogen could be used as a functional external method to protect the aquaculture environment.
The datasets presented in this study can be found in online repositories. The names of the repository/repositories and accession number(s) can be found below: BioProject, PRJNA915221.
JH and LW: conceptualization.SN, JH, LH, ML and XL: methodology. SN,WZ and JY: formal analysis and writing –original draft. LH, ML, YL and XL: data curation. SN,JH, and LW: writing – review and editing. SN,WZ and JY: visualization. LW: supervision. All authors contributed to the article and approved the submitted version.
This research was supported by Foshan Agricultural Special Project and Guangzhou Science and Technology Program project (202102020234).
YL is employed by Guangdong Cawolo Hydrogen Technology Company Ltd. The remaining authors declare that the research was conducted in the absence of any commercial or financial relationships that could be construed as a potential conflict of interest.
All claims expressed in this article are solely those of the authors and do not necessarily represent those of their affiliated organizations, or those of the publisher, the editors and the reviewers. Any product that may be evaluated in this article, or claim that may be made by its manufacturer, is not guaranteed or endorsed by the publisher.
Ahmad A. L., Chin J. Y., Harun M. H. Z. M., Low S. C. (2022). Environmental impacts and imperative technologies towards sustainable treatment of aquaculture wastewater: a review. J. Water Process Engineering. 46, 102553. doi: 10.1016/j.jwpe.2021.102553
Akaboci T. R. V., Gich F., Ruscalleda M., Balaguer M. D., Colprim J. (2018). Assessment of operational conditions towards mainstream partial nitritation-anammox stability at moderate to low temperature: reactor performance and bacterial community. Chem. Eng. J. 350, 192–200. doi: 10.1016/j.cej.2018.05.115
Anh P. T., Kroeze C., Bush S. R., Mol A. P. (2010). Water pollution by intensive brackish shrimp farming in south-east Vietnam: causes and options for control. Agric. Water Manage. 97 (6), 872–882. doi: 10.1016/j.agwat.2010.01.018
Becke C., Schumann M., Geist J., Brinker A. (2020). Shape characteristics of suspended solids and implications in different salmonid aquaculture production systems. Aquaculture 516, 734631. doi: 10.1016/j.aquaculture.2019.734631
Bohnes F. A., Hauschild M. Z., Schlundt J., Nielsen M., Laurent A. (2022). Environmental sustainability of future aquaculture production: analysis of Singaporean and Norwegian policies. Aquacult. 549, 737717. doi: 10.1016/j.aquaculture.2021.737717
Broman E., Jawad A., Wu X., Christel S., Ni G., Lopez-Fernandez M., et al. (2017). Low temperature, autotrophic microbial denitrification using thiosulfate or thiocyanate as electron donor. Biodegradation 28 (4), 287–301. doi: 10.1007/s10532-017-9796-7
Bucking C. (2017). A broader look at ammonia production, excretion, and transport in fish: a review of impacts of feeding and the environment. J. Comp. Physiol. B 187 (1), 1–18. doi: 10.1007/s00360-016-1026-9
Camargo J. A., Alonso Á. (2006). Ecological and toxicological effects of inorganic nitrogen pollution in aquatic ecosystems: a global assessment. Environ. Int. 32 (6), 831–849. doi: 10.1016/j.envint.2006.05.002
Canfield D. E., Glazer A. N., Falkowski P. G. (2010). The evolution and future of earth's nitrogen cycle. Science 330, 192–196. doi: 10.1126/science.1186120
Coates J. D., Chakraborty R., Lack J. G., O'Connor S. M., Cole K. A., Bender K. S., et al. (2001). Anaerobic benzene oxidation coupled to nitrate reduction in pure culture by two strains of dechloromonas. Nature 411 (6841), 1039–1043. doi: 10.1038/35082545
Fuhrman J. A. (2009). Microbial community structure and its functional implications. Nature 459 (7244), 193–199. doi: 10.1038/nature08058
Guo H., Chen S., Ouyang K., Kuang Y., Yang H., Wang Y., et al. (2022). Evaluation of ammonia nitrogen exposure in immune defenses present on spleen and head-kidney of wuchang bream (Megalobrama amblycephala). Int. J. Mol. Sci. 23 (6), 3129. doi: 10.3390/ijms23063129
He Q., Feng C., Peng T., Chen N., Hu Q., Hao C. (2016). Denitrification of synthetic nitrate-contaminated groundwater combined with rice washing drainage treatment. Ecol. Eng. 95, 152–159. doi: 10.1016/j.ecoleng.2016.06.043
Henne K., Kahlisch L., Brettar I., Hofle M. G. (2012). Analysis of structure and composition of bacterial core communities in mature drinking water biofilms and bulk water of a citywide network in Germany. Appl. Environ. Microbiol. 78 (10), 3530–3538. doi: 10.1128/AEM.06373-11
Holmes D. E., Dang Y., Smith J. A. (2019). Nitrogen cycling during wastewater treatment. Adv. Appl. Microbiol. 106, 113–192. doi: 10.1016/bs.aambs.2018.10.003
Huff L., Delos C., Gallagher K., Beaman J. (2013). Aquatic life ambient water quality criteria for ammonia-freshwater (Washington DC: US Environmental Protection Agency).
Jia X., Zhang D., Wang F., Dong S. (2017). Immune responses of litopenaeus vannamei to non-ionic ammonia stress: a comparative study on shrimps in freshwater and seawater conditions. Aquacult Res. 48 (1), 177–188. doi: 10.1111/are.12872
Jin Y., Wu S., Zeng Z., Fu Z. (2017). Effects of environmental pollutants on gut microbiota. Environ. pollut. 222, 1–9. doi: 10.1016/j.envpol.2016.11.045
Karanasios K., Vasiliadou I., Pavlou S., Vayenas D. (2010). Hydrogenotrophic denitrification of potable water: a review. J. Hazardous Materials 180 (1-3), 20–37. doi: 10.1016/j.jhazmat.2010.04.090
Kim J. H., Kang Y. J., Kim K. I., Kim S. K., Kim J. H. (2019). Toxic effects of nitrogenous compounds (ammonia, nitrite, and nitrate) on acute toxicity and antioxidant responses of juvenile olive flounder, paralichthys olivaceus. Environ. Toxicol. Pharmacol. 67, 73–78. doi: 10.1016/j.etap.2019.02.001
Kurt M., Dunn I. J., Bourne J. R. (1987). Biological denitrification of drinking water using autotrophic organisms with H(2) in a fluidized-bed biofilm reactor. Biotechnol. Bioengineering 29 (4), 493–501. doi: 10.1002/bit.260290414
Li L., Tan L., Yang W., Xu X., Li J. (2021). Conjoint applications of meta-analysis and bioinformatic data toward understanding the effect of nitrate on fish. Sci. Total Environ. 794 (142777), 148645. doi: 10.1016/j.scitotenv.2021.148645
Liu X., Du M., Yang J., Wu Y., Xu Q., Wang D., et al. (2020). Sulfite serving as a pretreatment method for alkaline fermentation to enhance short-chain fatty acid production from waste activated sludge. Chem. Eng. J. 385, 123991. doi: 10.1016/j.cej.2019.123991
Liu G., Zhao L., Guo Z., Lin L. (2022). Bacillus velezensis LG37 absorbs and utilizes ammonia nitrogen from aquaculture water and enhances the toxicity tolerance of grass carp (Ctenopharyngodon idella) to ammonia (non-ionic ammonium). Israeli J. Aquaculture-Bamidgeh 74, 1–12. doi: 10.46989/001c.35763
Luo S., Wu B., Xiong X., Wang J.. (2016). Short-term toxicity of ammonia, nitrite, and nitrate to early life stages of the rare minnow (Gobiocypris rarus). Environ. Toxicol. Chem. 35 (6), 1422–1427. doi: 10.1264/jsme2.ME11253
Lymperopoulou D. S., Kormas K. A., Karagouni A. D. (2012). Variability of prokaryotic community structure in a drinking water reservoir (Marathonas, Greece). Microbes Environments 27 (1), 1–8. doi: 10.1264/jsme2.ME11253
Martínez-Espinosa C., Sauvage S., Bitar A. A., Green P., Sánchez-Pérez J. (2020). Denitrification in wetlands: a review towards a quantification at global scale. Sci. Total Environ. 754, 142398. doi: 10.1016/j.scitotenv.2020.142398
Niu A., Song L. Y., Xiong Y. H., Lu C. J., Junaid M., Pei D. S. (2019). Impact of water quality on the microbial diversity in the surface water along the three gorge reservoir (TGR), China. Ecotoxicol Environ. Saf. 20, 412–418. doi: 10.1016/j.ecoenv.2019.06.023
Niu X., Zhou S., Deng Y. (2021). Advances in denitrification microorganisms and processes. Sheng wu Gong Cheng xue bao= Chin. J. Biotechnol. 37 (10), 3505–3519. doi: 10.13345/j.cjb.210407
Pieper D. H., dos Santos V.t.A.M, Golyshin P.N (2004). Genomic and mechanistic insights into the biodegradation of organic pollutants. Curr. Opin. Biotechnol. 15 (3), 215–224. doi: 10.1016/j.copbio.2004.03.008
Praveen P., Vaidya A., Tutt K., Andrews J. (2021). Assessing the potential of purple phototrophic microbial community for nitrogen recycling from ammonia-rich medium and anaerobic digestate. Bioresource Technol. 320, 124436. doi: 10.1016/j.biortech.2020.124436
Rose A., Padovan A., Christian K., d.K. J., Kaestli M., Tsoukalis S., et al. (2021). The diversity of nitrogen-cycling microbial genes in a waste stabilization pond reveals changes over space and time that is uncoupled to changing nitrogen chemistry. Microbial Ecol. 81 (4), 1029–1041. doi: 10.1007/s00248-020-01639-x
Shinoda K., Eamrat R., Tsutsumi Y., Rujakom S., Singhopon T., Kamei T., et al. (2020). Newly established process combining partial hydrogenotrophic denitrification and anammox for nitrogen removal. Water Sci. Technol. 82 (7), 1272–1284. doi: 10.2166/wst.2020.406
Vaz-Moreira I., Nunes O. C., Manaia C. M. (2017). Ubiquitous and persistent proteobacteria and other gram-negative bacteria in drinking water. Sci. Total Environ. 586, 1141–1149. doi: 10.1016/j.scitotenv.2017.02.104
Vaz-Moreira I., Egas C., Nunes O. C., Manaia C. M. (2013). Bacterial diversity from the source to the tap: a comparative study based on 16S rRNA gene-DGGE and culture-dependent methods. FEMS Microbiol. Ecol. 83 (2), 361–374. doi: 10.1111/1574-6941.12002
Wang H. J., Xiao X. C., Wang H. Z., Li Y., Yu Q., Liang X. M., et al. (2017). Effects of high ammonia concentrations on three cyprinid fish: acute and whole-ecosystem chronic tests. Sci. Total Environ. 598, 900. doi: 10.1016/j.scitotenv.2017.04.070
Wang X., Zhu H., Yan B., Shutes B., Bañuelos G., Wen H., et al. (2021). Improving denitrification efficiency in constructed wetlands integrated with immobilized bacteria under high saline conditions. Environ. pollut. 287, 117592. doi: 10.1016/j.envpol.2021.117592
Woese C. (1994). There must be a prokaryote somewhere : microbiology's search for itself. Microbiol. Rev. 58 (1), 1–9. doi: 10.1128/mr.58.1.1-9.1994
Wu G., Li Z., Huang Y., Zan F., Dai J., Yao J., et al. (2020). Electrochemically assisted sulfate reduction autotrophic denitrification nitrification integrated (e-SANI®) process for high-strength ammonium industrial wastewater treatment. Chem. Eng. J. 381, 122707. doi: 10.1016/j.cej.2019.122707
Xu Z., Cao J., Qin X., Qiu W., Mei J., Xie J. (2021). Toxic effects on bioaccumulation, hematological parameters, oxidative stress, immune responses and tissue structure in fish exposed to ammonia nitrogen: a review. Animals 11 (11), 3304. doi: 10.3390/ani11113304
Yang X., Song Z., Zhou S., Guo H., Geng B., Peng X., et al. (2019). Insights into functional microbial succession during nitrogen transformation in an ectopic fermentation system. Bioresource Technol. 284, 266–275. doi: 10.1016/j.biortech.2019.03.135
Yang Z., Zhou Q., Sun H., Jia L., Zhao L., Wu W. (2021). Metagenomic analyses of microbial structure and metabolic pathway in solid-phase denitrification systems for advanced nitrogen removal of wastewater treatment plant effluent: a pilot-scale study. Water Res. 196, 117067. doi: 10.1016/j.watres.2021.117067
Yasuda T., Waki M., Fukumoto Y., Hanajima D., Kuroda K., Suzuki K., et al. (2017). Community structure of denitrifying and total bacteria during nitrogen accumulation in an ammonia-loaded biofilter. J. Appl. Microbiol. 123 (6), 1498–1511. doi: 10.1111/jam.13603
Zerkle A. L., Mikhail S. (2017). The geobiological nitrogen cycle: from microbes to the mantle. Geobiology 15 (3), 343–352. doi: 10.1111/gbi.12228
Zha X., Ma J., Lu X. (2020). Use of a low-cost and energy-efficient device for treating low-strength wastewater at low temperatures focusing on nitrogen removal and microbial community. Sci. Total Environ. 722, 137916. doi: 10.1016/j.scitotenv.2020.137916
Zhang Z., Zhang Y., Chen Y. (2019). Recent advances in partial denitrification in biological nitrogen removal: from enrichment to application. Bioresource Technol. 298, 122444. doi: 10.1016/j.biortech.2019.122444
Zheng P. H., Zhang X. X., Wang D. M., Li J. T., Zhang Z. L., Lu Y. P., et al. (2021). Molecular characterization and expression analysis of a novel glutaredoxin 3 gene in pacific white shrimp (Litopenaeus vannamei). Front. Mar. Sci. 8. doi: 10.3389/fmars.2021.687377
Keywords: hydrogen, aquaculture water, nitrogen and phosphorus removal, ammonia nitrogen removal, microbial community
Citation: Ning S, Hu J, Yu J, Huang L, Lai M, Li X, Lin Y, Zhong W and Wang L (2023) Hydrogen injection reduces ammonia nitrogen and changes microbial community composition in aquaculture water. Front. Mar. Sci. 10:1111067. doi: 10.3389/fmars.2023.1111067
Received: 29 November 2022; Accepted: 03 April 2023;
Published: 24 April 2023.
Edited by:
Jun Wang, South China Agricultural University, ChinaReviewed by:
Mingzhu Huang, Jiangxi Normal University, ChinaCopyright © 2023 Ning, Hu, Yu, Huang, Lai, Li, Lin, Zhong and Wang. This is an open-access article distributed under the terms of the Creative Commons Attribution License (CC BY). The use, distribution or reproduction in other forums is permitted, provided the original author(s) and the copyright owner(s) are credited and that the original publication in this journal is cited, in accordance with accepted academic practice. No use, distribution or reproduction is permitted which does not comply with these terms.
*Correspondence: Junru Hu, aHVqdW5ydTEwMjVAMTYzLmNvbQ==; Lei Wang, d2FuZ2xlaUBzY251LmVkdS5jbg==
Disclaimer: All claims expressed in this article are solely those of the authors and do not necessarily represent those of their affiliated organizations, or those of the publisher, the editors and the reviewers. Any product that may be evaluated in this article or claim that may be made by its manufacturer is not guaranteed or endorsed by the publisher.
Research integrity at Frontiers
Learn more about the work of our research integrity team to safeguard the quality of each article we publish.