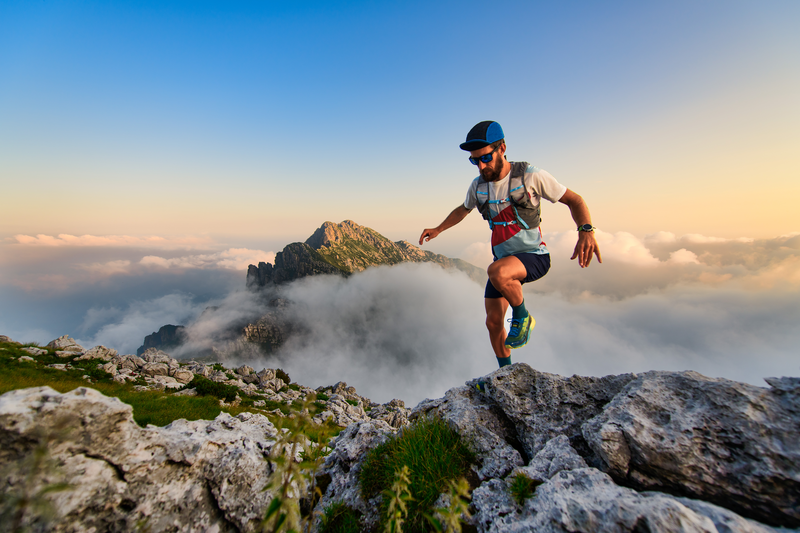
94% of researchers rate our articles as excellent or good
Learn more about the work of our research integrity team to safeguard the quality of each article we publish.
Find out more
ORIGINAL RESEARCH article
Front. Mar. Sci. , 23 March 2023
Sec. Marine Fisheries, Aquaculture and Living Resources
Volume 10 - 2023 | https://doi.org/10.3389/fmars.2023.1110980
The Philippine Sea, owing to its complicated circulation pattern and the presence of numerous seamounts, is considered to be a hotspot for biodiversity and an important fishing ground. However, to date, few studies on ichthyoplankton in the Philippine Sea have been conducted. During the summer, autumn, and winter of 2020–2021, a series of investigations were conducted to study the spatial and temporal structure of fish larvae in the Philippine Sea. A total of 65 samples were collected by vertical hauling from a depth of 200 m to the surface. A total of 882 fish larvae representing 81 distinct taxa, 49 genera, and 23 families were identified. A seasonal pattern was also observed based on the differences in abundance and dominant taxa. The abundance of fish larvae was 11.35 ± 12.46 ind./100 m3 (mean ± sd.) in summer, 5.83 ± 7.03 ind./100 m3 in autumn, and 2.57 ± 2.73 ind./100 m3 in winter. The dominant species were Vinciguerria nimbaria, Encrasicholina punctifer, and Cyclothone sp. in summer, V. nimbaria, and Ceratoscopelus warmingii in autumn, and Diaphus spp., D. garmani, and Sigmops sp. in winter. A significant latitudinal variation in fish larvae assemblages was found via cluster analysis, and two geographic groups were separated by the northern boundary of the North Equatorial Current. In addition, the fish larvae species diversity also showed a significant latitudinal gradient in autumn, which disappeared in summer. Canonical correspondence analysis indicated that the structure of fish larvae assemblages was affected by average temperature, salinity, and net primary productivity in the upper ocean and sea level anomaly. All dominant species were associated with specific niche environments. This study suggests that latitudinal zonation of fish larvae also occurs in the oligotrophic low latitude Pacific Ocean and is enhanced in autumn. These results provide multi-seasonal baseline data of fish larvae assemblage in the upper Philippine Sea, being an important reference for the planning of marine protected areas in the high seas.
Information concerning the ecology of ichthyoplankton is valuable for the protection and sustainable exploitation of fish resources (Houde, 2018),which can be used to assess population size (Van der Lingen and Huggett, 2003), as well as to identify spawning grounds (Tsukamoto, 2006; Kurogi et al., 2012; Kuroki et al., 2020) and spawning seasons (Sassa and Hirota, 2013). Ichthyoplankton are highly sensitive to changes in hydrological conditions. Because of their weak swimming ability, they are also vulnerable to starvation and predation (China and Holzman, 2014; Downie et al., 2020). Thus, environmental factors strongly influence the distribution of ichthyoplankton. In the oceanic ecosystem, ichthyoplanktons are an important component of marine plankton communities, playing a key role in the marine food webs through bottom-up control (Frederiksen et al., 2006). For example, shortages of post-larval sand eels drove seabird breeding failures during the chick-rearing period in the Orkney and Shetland Islands (Perkins et al., 2018). Long-term data on the ecology of ichthyoplankton are essential for the sustainability of fishery resources and for understanding the dynamics of marine ecosystems (FAO, 2003; Houde, 2018).
The Philippine Sea, located in the tropical and subtropical western Pacific Ocean, is strongly influenced by the western boundary current system, including the North Equatorial Current (NEC), the Kuroshio Current (KC), the Mindanao Current (MC), and the Subtropical Countercurrent (STCC) (Hu et al., 2015; Oka et al., 2018). The western boundary currents have been shown to affect the distribution patterns and transport processes of larval fish in the western Pacific. Sassa et al. (2004) separated myctophid larvae into four groups based on their species composition in the Philippine Sea: Kuroshio Axis, Kuroshio Countercurrent, Subtropical Countercurrent-North Equatorial Current, and Pan tropical-subtropical. The horizontal distributions of these assemblages are well-defined by the positions of the currents and are related to the oceanographic structure. Zenimoto et al. (2009) revealed that differences in Japanese eel larval transport might be due to the latitude of the NEC bifurcation, which fluctuates with ENSO events. The Philippine Sea is a typical oligotrophic sea with low concentrations of chlorophyll a (Chl a), nitrogen, and phosphorus. Low latitude oligotrophic oceans are the habitat of many important high trophic level predators (Itano, 2000). The Philippine Sea area is a hotspot for biodiversity and climatic impacts due to the complex ocean circulation and intensive multiscale air-sea interactions (Tittensor et al., 2010; Hu et al., 2020). Ecological research on the plankton of the Philippine Sea has largely focused on primary productivity (Chen et al., 2017; Kodama et al., 2021), the community and size spectra of zooplankton (Dai et al., 2016; Dai et al., 2017; Sun and Wang, 2017; Yang et al., 2017), and the distribution of economically important fish larvae (Tsukamoto, 2006; Kurogi et al., 2012). Studies on the ichthyoplankton in Philippine Sea are still relatively rare.
The tropical and subtropical waters of the western Pacific Ocean provide spawning and nursing grounds for some important commercial fish, e.g., bluefin tuna Thunnus orientalis, Japanese eel Anguilla japonica, and Japanese conger Conger myriaster. Tsukamoto (2006) proposed that the Japanese eel spawns near seamounts west of the Mariana Islands. Kurogi et al. (2012) discerned that the Japanese conger spawns in the area along the Kyushu-Palau Ridge around 17°N, 136°E. In the western Pacific, ichthyoplankton research has focused on coastal waters due to the logistical and economic considerations of investigations (Huang et al., 2017; Zhang et al., 2019a; Zhang et al., 2020; Hou et al., 2021). Surveys carried out in the open ocean are generally focused on the Kuroshio Current region (Su et al., 2011; Sassa and Takasuka, 2019), the Oyashio Current region (Shida et al., 2007), and their transitional waters (Aoki and Miyashita, 2000; Sassa et al., 2002). Except for descriptions by Sassa et al. (2004, 2007a) of the horizontal and vertical distribution patterns of myctophid larvae in the subtropical-tropical waters of the western North Pacific, few studies have been done on the spatial and temporal variation of ichthyoplankton assemblages in the tropical and subtropical western Pacific (Table S1). The lack of multi-seasonal baseline data limits our understanding of the ecological functions of fish larvae in pelagic ecosystems. To obtain such baseline data on fish larvae assemblages in the Philippine Sea, we conducted three cruises in 2020 and 2021 and analyzed the spatial and seasonal variation of ichthyoplankton based on 65 samples collected.
From July 6 to September 13, 2020, 28 stations were sampled along two transects by the R/V Dayanghao and Dayangyihao. The east transect was located along 135°E, between 13°N and 28.5°N, and the west transect was located along 129°E, between 13°N and 22°N. Another 15 and 22 stations were sampled by the R/V Shenhaiyihao from January 17 to January 28 and October 27 to November 27, 2021, respectively (Figure 1). Ichthyoplankton samples were collected using a WP2 plankton net (mesh size of 200 μm, mouth area 1 m2), towed vertically from a depth of 200 m to the surface at a speed of 1 m/s. Each sample was divided into two subsamples with a Folsom plankton splitter. The two stochastic sub-samples were preserved in 5% (v/v) buffered formalin-seawater solution and 95% alcohol. The larvae stored in formalin-seawater were counted and identified in the laboratory using morphological characteristics (Moser, 1996; Okiyama, 2014; Wan and Zhang, 2016). The specimens were identified to the lowest taxon possible. Damaged specimens were grouped as unidentified. Larvae stored in alcohol were used to aid the identification of the species. The counts were standardized to the density of individuals per 100 m3.
Figure 1 Hydrographic features of west North Pacific, with sampling stations of ichthyoplankton in oligotrophic water of the Philippine Sea. Currents: KC (Kuroshio Current), MC (Mindanao Current), NEC (North Equatorial Current), STCC (Subtropical Countercurrent). Stations sampled in summer are shown in red, stations sampled in autumn are shown in orange, and stations sampled in winter are shown in green. The annual chlorophyll data in the background was downloaded from the E.U. Copernicus Marine Service.
Temperature and salinity data were collected using the 911 PLUS CTD system. Content of Chlorophyll a (Chl a), net primary productivity (NPP), and suspended particulate matter concentration (SPM) (based on satellite remote sensing data with 4 km resolution) and sea level anomaly (SLA) (based on physics reanalysis data with 0.25 degrees resolution) and Mix layer depth (MLD) (based on physics reanalysis data with 0.083 degrees resolution) for August 2020, January 2021, and November 2021 were downloaded from the E.U. Copernicus Marine Service (https://marine.copernicus.eu/).
Dominant taxa were defined as those taxa for which the index of relative importance (IRI) was greater than 100 (Zhang et al., 2019a). The IRI value was calculated by the following formula:
where N% and F% represent the relative abundance and frequency of occurrence. Ichthyoplankton diversity was measured using the Margalef species richness index, Pielou’s evenness index, and the Shannon-Wiener index. The indices were calculated based on abundance data in Primer (Version 6.0, PRIMER-e, UK), and loge was used in diversity index calculations. All calculations were at the species level; samples not identified to the species level were treated as a species unless otherwise specified.
One-way ANOVA in R was used to analyze differences in abundance between summer, autumn, and winter in the same sampled areas (south of 18°N on the east transect). To clarify the spatial distribution of the assemblages in summer and autumn, cluster analysis based on Bray-Curtis similarity with log (x + 1) transformed abundance data was performed in Primer (Version 6.0, PRIMER-e, UK).
The environmental variables that were found to influence the fish larvae assemblages were selected to analyze the community-environment relationships in the Philippine Sea. These were the average temperature from 0 to 200 m (T0-200), average salinity from 0 to 200 m (S0-200), Chl a, NPP, MLD, SPM, and SLA. Canonical correspondence analysis (CCA) was used to explore the effects of spatial/environmental variables on ichthyoplankton assemblage based on the maximum gradient length of detrended correspondence analysis (DCA) being 5.04. CCA was operated in CANOCO software (Version 5.0, Wageningen University & Research, NL) based on the abundance of predominant species (abundance >1%). T0-200, S0-200, NPP, and SLA were chosen to be analyzed based on the forward selection results. The larval abundance was transformed by log (x + 1) to reduce dominance effects.
The oceanographic structures were determined by a temperature-salinity (T-S) diagram during the cruises. Stations south of 20°N were affected by the NEC, with a higher surface temperature and a higher salinity at 150–200 m (Figures 2, S1). The sea surface temperature (SST) in the Philippine Sea is warmer in summer compared to autumn and winter (Figure S2). The temperature of the southern part of the Philippine Sea is higher than in the northern part, but there is a small temperature difference in summer (Figure S2). The surface salinity (SSS) was relatively lower in the southern Philippine Sea, and the salinity was > 34.8 psu as deep as 100 to 200 meters (Figures 2, S2). Chl a in the surface Philippine Sea was low (< 0.15 mg/m3), and lower Chl a levels were observed in summer compared to autumn and winter (Figure S2).
A total of 882 fish larvae representing 81 distinct taxa in 23 families were recorded. Most specimens (91.5%) were identified to genus or species level. Less than 5% of the specimens were unidentified due to damage caused by the trawling process. Among the 23 families, only five exceeded 1% of the total abundance, Phosichthyidae (31.4%), Myctophidae (29.8%), Engraulidae (18.3%), Gonostomatidae (9.0%), and Paralepididae (1.6%). Among these families, 10 were represented by only one taxon and were only collected once. Most larvae belonged to the order Myctophiformes, followed by the order Stomiiformes (Table 1). These two orders dominate the global mesopelagic fish fauna. We collected myctophid larvae in 49 stations and identified 32 myctophid taxa (20 identified to species) belonging to 16 genera (Table 1). Genus Diaphus was the most abundant, accounting for 22.9% of all myctophid larvae, followed by the genera Lampanyctus (13.1%), Hygophum (12.8%), and Ceratoscopelus (12.1%). In addition, we collected stomiiformes fish larvae in 40 stations and identified 17 stomiiformes taxa (10 identified to species) belonging to seven genera (Table 1). Genus Vinciguerria was the most abundant, accounting for 74.7% of all stomiiformes fish larvae, followed by genus Cyclothone (16.8%).
Table 1 The information of ichthyoplankton in the summer, autumn, and winter in the Philippine Sea, the main taxa (A % >10, or F %>10) are shown in bold.
According to the IRI index, Vinciguerria nimbaria, Encrasicholina punctifer, and Cyclothone sp. were the dominant taxa in the summer, accounting for 63.6% of the abundance. In the autumn, V. nimbaria and Ceratoscopelus warmingii were the dominant species accounting for 50.8% of the abundance. In the winter, Diaphus spp., D. garmani, and Sigmops sp. were the dominant taxa accounting for 43.4% of the abundance (Tables 1, S2).
The Shannon–Wiener index for the fish larvae ranged from 0–2.32 in summer, 0–2.14 in autumn, and 0–1.59 in winter. The Shannon–Wiener index for 15 stations was zero because only one species was caught in seven stations during the summer, five stations during autumn, and three stations during winter. The Shannon–Wiener index did not differ significantly among the summer, autumn, and winter based on the Wilcoxon test of the same stations sampled during the three seasons (Figure 3A).
Figure 3 Temporal variation of the Shannon-Wiener index (A) and larvae abundance (B) from summer to winter (*, significant; ns, no significant).
The abundance of ichthyoplankton in the tropical and subtropical Philippine Sea changed between August 2020, January 2021, and November 2021. We compared the same stations during summer, autumn, and winter. Our results showed that ichthyoplankton abundance in the Philippine Sea was higher in summer than in autumn and winter, but there was no significant difference between autumn and winter (Figure 3B). The fish larval abundance of most stations was low (0 –45.60 ind./100 m3), and no ichthyoplankton were collected in two stations in winter (Figure 4). The mean abundance of the fish larvae was 11.35 ind./100 m3 (0.68–45.60 ind./100 m3) in summer, 5.83 ind./100 m3 (0.83–29.97 ind./100 m3) in autumn, and 2.57 ind./100 m3 (0–11.85 ind./100 m3) in winter.
Figure 4 Seasonal and spatial variations of fish larvae assemblages in the Philippine Sea. The fish larvae abundance during the 3 seasons (A, summer; B, autumn; C, winter), and abundance of dominant species Vincigueriia nimbaria (D), Encasicholina punctifer (E), and Ceratoscopelus warmingii (F) in the Philippine Sea, stations sampled in summer are shown in red, stations sampled in autumn are shown in orange, and stations sampled in winter are shown in green.
At the species level, V. nimbaria was the dominant fish larva in both summer and autumn. In addition, strong seasonal shifts in community structure were observed. In summer, we collected 15 species that were not recorded in autumn, for example the dominant species E. punctifer. Meanwhile, in autumn we collected 22 species that were not recorded in summer, for example, the dominant species C. warmingii. (Figures 4, 5).
Figure 5 Latitudinal distribution patterns of fish larvae abundance and species numbers in the 135°E transect of the Philippine Sea during the summer (A, B) and autumn (C, D). The Larvae abundance showed by the size of the circle. The latitudinal trends of species diversity were analyzed based on the linear regression model, significance by F-test.
The components of fish larvae assemblages changed with the seasons, and their abundances had a definite latitudinal distribution (Figure 5). Totals of 98.7% and 87.5% of the larvae of V. nimbaria were collected north of 20°N during the summer and autumn, respectively, indicating that the distribution range was limited in the Philippine Sea. In summer, the relationship between the fish larval species diversity and latitude was not significant (P = 0.7686), but larval species diversity showed a significant positive correlation with latitude in autumn (P< 0.001) (Figure 5). The species diversity increased along with latitude in autumn.
Significant spatial structure was recognized based on the cluster analysis. The larval assemblages corresponded well with stations sampled in the northern and southern parts of the Philippine Sea. In the summer, the cluster analysis of Bray-Curtis similarity based on the predominant species (abundance > 1%) divided the larval assemblage into three spatial groups (Figures 6A, B). Group NPSS I (northern part of the Philippine Sea in the summer I) and NPSS II (northern part of the Philippine Sea in the summer II) consisted of seven and two stations sampled from the northern region of the Philippine Sea, respectively. The group SPSS (southern part of the Philippine Sea in the summer) consisted of six stations sampled from the southern region of the Philippine Sea. The dominant characteristic taxa of group NPSS I were V. nimbaria and Cyclothone sp., and Cyclothone sp. was dominant in group NPSS II. The group SPSS was defined by the dominant taxa E. punctifer and D. garmani. In the autumn, the cluster analysis of Bray-Curtis similarity divided the larval assemblage into three spatial groups (Figures 6C, D). Group NPSA I (northern part of the Philippine Sea in autumn I) and NPSA II (northern part of the Philippine Sea in autumn II) each consisted of four stations sampled from the northern region of the Philippine Sea. The group SPSA (southern part of the Philippine Sea in autumn) consisted of five stations sampled from the southern region of the Philippine Sea. The dominant characteristic taxa of group NPSA I were V. nimbaria, and Hygohum reinhardtii of group NPSA II. The group SPSA was defined by the dominant taxa Ceratoscopelus warmingii, Diaphus sp., and Lestidium orirhtale.
Figure 6 Dendrograms showing the stations groups defined by the Bray-Curits similarity and their spatial locations in the Philippine Sea during the summer (A, B) and autumn (C, D). NPSS (northern of the Philippine Sea in the summer), SPSS (southern of the Philippine Sea in the summer), NPSA (northern of the Philippine Sea in the autumn), SPSA (southern of the Philippine Sea in the autumn).
The CCA analysis revealed the relationship between fish larvae and environmental factors. The first two axes explained 15.9% of the variation in species and 74.5% of the variation in species-environment (Table S3). T0-200, S0-200, and NPP were environmental factors affecting ichthyoplankton assemblages based on the Monte Carlo tests employed in this study (Table 2), and the factors T0-200, S0-200, and NPP explained 9.0%, 5.1%, and 4.2% variation in the ichthyoplankton assemblage, respectively. T0-200 and S0-200 were strongly correlated with the first axis and the second axis, respectively. T0-200 was positively correlated with the first axis, and S0-200 was negatively correlated with the second axis. In addition, NPP was negatively correlated with the first axis (Figure 7 and Table 2).
Table 2 Conditional effects and the intra-set correlations of environmental factors with the first two axes of CCA.
Figure 7 CCA biplot of fish larvae and sampling stations with environmental factors in the Philippine Sea. The dominant species are shown in a bold and filled triangle, Ben_sp (Benthosema sp.), Cer_war (Ceratoscopelus warmingii), Cyc_abla (Cyclothone alba), Cyc_sp (Cyclothone sp.), Dia_gar (Diaphus garmani), Dia_spp Diaphus spp.), Enc_pun (Encrasicholina punctifer), Gon_atl (Gonostoma atlanticum), Hyg_rei (Hygophum reinhardtii), Hyg_sp (Hygophum sp.), Lam_ala (Lampanyctus alatus), Lam_sp (Lampanyctus sp.), Vin_nim (Vinciguerria nimbaria). Stations sampled in summer are shown in red, stations sampled in autumn are shown in orange, and stations sampled in winter are shown in green.
As shown in the CCA ordination plot, the dominant larvae were mainly located at the ends of the two axes (Figure 7), indicating that these species were niche specialists. The dominant larvae V. nimbaria showed a positive relationship with NPP but a negative relationship with T0-200. The abundant larvae Diaphus garmani, and Diaphus spp. were positively related to T0-200. Cyclothone sp. was positively related to S0-200, and C. warmingii was negatively related to S0-200 (Figure 7). By contrast, the common species were largely situated close to the origin of the ordination plot, indicating that these species were generalists.
The abundance of fish larvae was in the range of values from the Philippine Sea (Sassa et al., 2004, Sassa et al., 2007a), and lower than the abundance in the Kuroshio waters (Su et al., 2011; Hsieh and Lo, 2019) (Table S1). The dominant species V. nimbaria, E. punctifer, C. warmingii, D. garmani, Cyclothone sp., Sigmops sp., and Diaphus spp. have also been reported to have high abundance in the Philippine Sea and tropical and subtropical western Pacific (Sassa et al., 2004; Sassa et al., 2007a; Huang et al., 2017; Hsieh and Lo, 2019). Myctophid and stomiiform fish dominate fish assemblages in global mesopelagic fish fauna (Sutton et al., 2020). These fish spend their larval stages in the epipelagic zone. Myctophid fish are preyed upon by gadiform fish, squid, tuna, dolphins, and whales (Ohizumi et al., 1998; Phillips et al., 2001; Yamamura and Inada, 2001), and as such, they play a key ecological role in the western Pacific marine food web (Sassa et al., 2002; Catul et al., 2011). In this study, myctophids had the highest species occurrence in the study area. We collected myctophid larvae from 49 stations and identified 32 myctophid taxa. Taxa and numbers of myctophid larvae in this study were less than those found in the investigation in 1994 (Sassa et al., 2004; Sassa et al., 2007a). We believe this is because we sampled at a 0–200 m depth, while Sassa et al. towed in deeper waters. We collected stomiiform fish larvae from 40 stations. We identified 18 stomiiform taxa belonging to seven genera (Table 1). Vinciguerria was the most abundant genus, accounting for 74.7% of all stomiiform fish larvae, followed by Cyclothone (16.8%). The genus Vinciguerria has always been considered to be abundant in global oceans (Dove et al., 2021). In the California Current region, the main species was V. lucetia (Moser and Smith, 1993; Gerardo et al., 2018), whereas the main species of genus Vinciguerra in the Philippine Sea in this study was V. nimbaria. V. nimbaria was reported as a dominant species in the Kuroshio region and in the eastern central Atlantic (Sassa, 2019; Dove et al., 2021).
Engraulidae was third in abundance despite being represented by only two taxa, because Engraulidae including Encrasicholina punctifer constituted 18.7% of the larvae. E. punctifer plays an important ecological role in the western Pacific as a major forage source for yellowfin tuna (Itano, 2000). We collected the E. punctifer larvae mainly in the NEC region (Figure 4E), and the distribution patterns of E. punctifer were generally consistent with the previous research (Ozawa and Tsukahara, 1973).
The NEC (between about 10°N and 20°N) is driven by the easterly trade winds and Ekman transport (Lukas, 2001; Hu et al., 2015), and it is one of the main currents flowing westward over the upper ocean in the western North Pacific. Based on the cluster analysis, the fish larvae assemblages were perennially divided into two spatial groups in the Philippine Sea. In this study, the latitudinal distributions of fish larvae assemblages showed distinct latitudinal variations. The boundary between the southern and northern fish larvae assemblages was around 20°N latitude, which corresponded well with the northern boundary of the NEC current. The larvae of E. punctifer, and C. warmingii are abundant in the NEC region, while V. nimbaria, and H. reinhardtii are largely distributed on the northern side of the NEC. Along the same transect, (Sassa et al., 2004) found similar distribution patterns in myctophia larvae assemblages. The myctophid larvae were divided into a Kuroshio Countercurrent assemblage and a Subtropical Countercurrent-North Equatorial Current assemblage, with a boundary of 23°N during June in the tropical Philippine Sea (Sassa et al., 2004). The same distribution patterns of latitudinal variation were also found in the phytoplankton (Han et al., 2004) and zooplankton communities (Nagai et al., 2015; Yang et al., 2017). A significant latitudinal variation of phytoplankton and zooplankton is always correlated with main water masses in the subtropical and tropical Western Pacific. Zooplanktons were reported as important prey and predators for larval fish (McClatchie et al., 2012; Nunn et al., 2012). Furthermore, Yang et al. (2017) reported the spatial patterns in zooplankton communities associated with currents and divided the zooplankton community into three assemblages related to the STCC, NEC, and North Equatorial Countercurrent in the Philippine Sea. Nagai et al. (2015) also found that chaetognaths had significant differences between subtropical areas and the NEC. The same spatial patterns indicated that fish larvae may be determined by prey availability and predation pressure from zooplankton.
In autumn, species diversity showed a significant positive correlation with latitude (P< 0.001), but the latitudinal trend was not found in summer. One possible explanation of seasonal differences in the latitudinal trends of the species richness is that along the latitude the lower surface sea temperature weakens thermal stratification in the autumn, thereby allowing upwelling to move nutrients into the upper ocean. In terms of myctophid larvae assemblages, the abundance was significantly higher in the north of the Philippine Sea than those in the southern region, and the numbers of species did not clearly differentiate between the northern region and the NEC in the summer (Sassa et al., 2004). The latitudinal pattern was different from the fish larvae in the Atlantic, which showed a decreasing trend from the equator to the temperate regions (Dove et al., 2021). More similar distribution patterns were found in NPP and zooplankton; the NPP showed an increasing trend from the equator to the temperate zones in the Philippine Sea. Sun and Wang (2017) reported that both the biomass and species number of zooplankton were significantly higher in the subtropical zones than in tropical areas. This suggests that the latitudinal gradient pattern of pelagic communities in the tropical and subtropical western Pacific Ocean may differ from that of the Atlantic Ocean at the same latitude.
The maximum current speed of the NEC at times reaches 30 cm/s near the surface (Wang et al., 2019), and the NEC bifurcates into the KC and MC on the Philippine coast (Hu et al., 2015). In this study, larvae of E. punctifer and C. warmingii, the two predominant larvae of the NEC assemblages, have also been reported as the most abundant larvae in the Kuroshio waters off eastern Taiwan island (Hsieh and Lo, 2019) and the South China Sea (Hsieh et al., 2011; Huang et al., 2017). We proposed that these fish larvae were probably transported by the NEC westward to the Kuroshio waters off eastern Taiwan island and the South China Sea. The fish larvae transported by ocean currents were common; the Kuroshio Current carried the fish larvae to the Kuroshio-Oyashio transition region (Sassa et al., 2002; Sassa and Kawaguchi, 2004), and the Japanese eel larvae were transported by the NEC and KC to east Asia (Tsukamoto, 2006). Meanwhile, larvae of V. nimbaria were collected in the Kuroshio waters off eastern Taiwan island (Hsieh and Lo, 2019) and the South China Sea (Hsieh et al., 2011). However, in the Philippine Sea, larvae of V. nimbaria were distributed on the northern side of the NEC. This suggests that V. nimbaria in the Kuroshio waters off eastern Taiwan island and the South China Sea were not transported from the NEC, and V. nimbaria as a cosmopolitan species may spawn in their own waters (Dove et al., 2021). Based on the abundance difference and the predominant fish larvae, a significant seasonal difference in the Philippine Sea fish larvae assemblages was observed in this study. These seasonal changes for most taxa suggest that each species may have a fixed spawning period in the Philippine Sea. Seasonal changes in ichthyoplankton assemblages in coastal and estuarine water are relatively common (Korsman, 2013; Zhang et al., 2019a; Zhang et al., 2019b; Jiang et al., 2021). Seasonal differences were also found in the California Current (Loeb et al., 1983; Moser and Smith, 1993; Peiro-Alcantar et al., 2016) and Kuroshio Current regions (Moku et al., 2003; Sassa et al., 2007b; Sassa and Hirota, 2013). Gonadal maturation and spawning of fish depend on the appropriate external environment to ensure that larvae are produced when conditions are most suitable for their survival.
Because the distribution of ichthyoplankton assemblages is affected by environmental factors, most research has shown that the temperature, salinity, content of Chl a, zooplankton, currents, and water mass are correlated with the distribution and structure of ichthyoplankton (Zhang et al., 2019a; Dove et al., 2021; Hou et al., 2021; Jiang et al., 2021). We built the relationship between ichthyoplankton assemblage structure with environmental factors by CCA analysis. Our results revelated that ichthyoplankton in the Philippine Sea were correlated with T0-200, S0-200, NPP, and SLA.
Temperature affects the development and metabolism of larvae; larval daily growth rates increased by approximately 0.01 per °C increase in temperature (Houde, 1989). The optimum temperature range for fish larval survival is narrow, and the lethal limits are broad (Ehrlich and Muszynski, 1982; Dove et al., 2021). Therefore, the distribution of larvae is associated with temperature changes. In this study, the temperature variation was associated with large-scale latitude change. As shown in the CCA ordination plot, larvae of D. garmani and E. punctifer showed a positive relationship with T0-200, indicating that the two fish larvae showed a preference for warmer oceans. Ocean warming as the greatest impact of climate change has been reported to influence the ichthyoplankton in abundance, metabolism, and geographical distributions (Hsieh et al., 2009; Asch, 2015; Walsh et al., 2015; Zhang et al., 2019c). For example, in the southern California region, both warm and cold-water taxa showed a significant increase in abundance from the cold to warm period based on 50-year-long ichthyoplankton investigation surveys (Hsieh et al., 2009). In the present study, temperature was a key factor in structuring the fish larvae assemblages in the Philippine Sea. Accordingly, the temperature-dependent fish larvae would migrate toward higher latitudes as global warming.
The mesoscale eddy modulates the abundance and distribution of marine organisms (Bertrand et al., 2014). Eddy processes play an important role in controlling ocean productivity and the timing of phytoplankton blooms (Mahadevan et al., 2012). Godø et al. (2012) studied the impact of mesoscale eddies on the patchiness of biomass and found that they provided rich feeding habitats for higher trophic marine life. Meanwhile, pelagic predators increased inside anticyclonic eddies along with the mesopelagic prey abundance in anticyclone cores (Arostegui et al., 2022). Mesoscale eddies could move nutrient-rich deep water into the surface layer, promoting primary productivity and supporting more fish larvae (Falkowski et al., 1991). The mesoscale eddy could create greater prey availability for Hygophum reinhardtii, as it has a positive relationship with SLA. In addition, the mesoscale eddy is an important dispersal pathway and as such determines the distribution of fish larvae (Tiedemann et al., 2018). The Philippine Sea is one of the most mesoscale eddy-energetic regions (Yang et al., 2013). Most eddies propagate westward, and the westward speed can reach 19.2 cm/s (Yang et al., 2013). SLA as an explicit expression of a mesoscale eddy, was another factor determining the distribution of fish larvae in this study. Mesoscale eddies may be involved in the transport of the fish larvae and the determination of the distribution.
The common species had a broader degree of environmental adaptation than the dominant species in the present study. Previous studies have generally focused on the effects of environmental factors on the fish larvae community, but environmental adaptation was often ignored (Hsieh and Lo, 2019; Zhang et al., 2019a; Dove et al., 2021; Hou et al., 2021). The dominant larvae were largely located at the ends of the two axes. By contrast, the common species were primarily situated close to the origin of the ordination plot. This suggested that dominant species had more response to the environmental factors than common species. Meanwhile, larvae of V. nimbaria showed a negative relationship with T0-200 but a positive relationship with NPP. This pattern indicated that as a cosmopolitan species, V. nimbaria was mainly distributed in subtropical rich feeding water. Larvae of E. punctifer, Diaphus garmani, and Diaphus spp. were positively related to T0-200, and these larvae were largely distributed in lower latitudes.
The four selected environmental factors only explained 21.3% of the variability of ichthyoplankton (Table 2), indicating that more factors should be considered. Because of the dynamics of fish larvae assemblages and marine ecosystems, the relationship between the environment and fish larvae is extremely complex. More environmental data and fish larvae samples are needed to improve our understanding of ichthyoplankton in the Philippine Sea.
In this study, a total of 882 fish larvae were collected from 65 stations in the Philippine Sea. The samples contained 81 distinct taxa belonging to 49 genera and 23 families. The predominant taxa in the summer were V. nimbaria, E. punctifer, and Cyclothone sp.; the predominant taxa were V. nimbaria and C. warmingii in the autumn, and the predominant taxa in the winter were Diaphus spp., D. garmani, and Sigmops sp. Cluster analysis identified two main spatial groups closely related to the NEC in the Philippine Sea, and the northern and southern fish larvae assemblages were separated by the northern boundary of the NEC. In addition, there was a clear seasonal shift in species diversity. The species diversity showed a significant positive correlation with latitude (P< 0.001). The abundance of fish larvae in the southern Philippine Sea was higher during summer than during autumn and winter, but there was no significant difference between autumn and winter. T0-200, S0-200, NPP, and SLA were the key environmental factors affecting the distribution of ichthyoplankton in the Philippine Sea. In addition, the common species had a broader degree of environmental adaptation than the dominant species. The dominant larvae were specialists, while the common species were generalists. More surveys of the Philippine Sea should be considered to collect additional information on ichthyoplankton and inform fisheries management.
The datasets presented in this study can be found in online repositories. The names of the repository/repositories and accession number(s) can be found in the article/Supplementary Material.
CF, DS, and CW conceptualized the study and designed the experiment. CF, XW, and GL performed the experiment and data visualization. CF, HZ, and DZ participated the investigation cruises and collected the samples. CF write the original draft. DS and CW review and edit the draft. All authors contributed to the article and approved the submitted version.
This work was supported by the National Natural Science Foundation of China (42076122) and the China Ocean Mineral Resources Research and Development Association Program (DY-XZ-02 and DY135-E2-3-04).
We are grateful to the members of R/V Dayanghao, Dayangyihao and Shenhaiyihao for their help during the investigations. We also thank the editor and two reviewers for their valuable comments on earlier versions. We thank LetPub (www.letpub.com) for its linguistic assistance during the preparation of this manuscript.
The authors declare that the research was conducted in the absence of any commercial or financial relationships that could be construed as a potential conflict of interest.
All claims expressed in this article are solely those of the authors and do not necessarily represent those of their affiliated organizations, or those of the publisher, the editors and the reviewers. Any product that may be evaluated in this article, or claim that may be made by its manufacturer, is not guaranteed or endorsed by the publisher.
The Supplementary Material for this article can be found online at: https://www.frontiersin.org/articles/10.3389/fmars.2023.1110980/full#supplementary-material
Aoki I., Miyashita K. (2000). Dispersal of larvae and juveniles of Japanese anchovy Engraulis japonicus in the kuroshio extension and kuroshio-oyashio transition regions, western north pacific ocean. Fish Res. 49, 155–164. doi: 10.1016/S0165-7836(00)00197-1
Arostegui M. C., Gaube P., Woodworth-Jefcoats P. A., Kobayashi D. R., Braun C. D. (2022). Anticyclonic eddies aggregate pelagic predators in a subtropical gyre. Nature 609, 535–540. doi: 10.1038/s41586-022-05162-6
Asch R. G. (2015). Climate change and decadal shifts in the phenology of larval fishes in the California current ecosystem. Proc. Natl. Acad. Sci. U.S.A. 112, e4065–e4074. doi: 10.1073/pnas.1421946112
Bertrand A., Grados D., Colas F., Bertrand S., Capet X., Chaigneau A., et al. (2014). Broad impacts of fine-scale dynamics on seascape structure from zooplankton to seabirds. Nat. Commun. 5, 5239. doi: 10.1038/ncomms6239
Catul V., Gauns M., Karuppasamy P. K. (2011). A review on mesopelagic fishes belonging to family myctophidae. Rev. Fish Biol. Fish. 21, 339–354. doi: 10.1007/s11160-010-9176-4
Chen Y., Sun X., Zhu M., Zheng S., Yuan Y., Denis M. (2017). Spatial variability of phytoplankton in the pacific western boundary currents during summer 2014. Mar. Freshw. Res. 68, 1887. doi: 10.1071/MF16297
China V., Holzman R. (2014). Hydrodynamic starvation in first-feeding larval fishes. Proc. Natl. Acad. Sci. U.S.A. 111, 8083–8088. doi: 10.1073/pnas.1323205111
Dai L., Li C., Tao Z., Yang G., Wang X., Zhu M. (2017). Zooplankton abundance, biovolume and size spectra down to 3000 m depth in the western tropical north pacific during autumn 2014. Deep Sea Res. Part I: Oceanogr. Res. Pap. 121, 1–13. doi: 10.1016/j.dsr.2016.12.015
Dai L., Li C., Yang G., Sun X. (2016). Zooplankton abundance, biovolume and size spectra at western boundary currents in the subtropical north pacific during winter 2012. J. Mar. Syst. 155, 73–83. doi: 10.1016/j.jmarsys.2015.11.004
Dove S., Tiedemann M., Fock H. O. (2021). Latitudinal transition of mesopelagic larval fish assemblages in the eastern central Atlantic. Deep Sea Res. Part I: Oceanogr. Res. Pap. 168, 103446. doi: 10.1016/j.dsr.2020.103446
Downie A. T., Illing B., Faria A. M., Rummer J. L. (2020). Swimming performance of marine fish larvae: Review of a universal trait under ecological and environmental pressure. Rev. Fish Biol. Fish. 30, 93–108. doi: 10.1007/s11160-019-09592-w
Ehrlich K. F., Muszynski G. (1982). Effects of temperature on interactions of physiological and behavioural capacities of larval California grunion: Adaptations to the planktonic environment. J. Exp. Mar. Biol. Ecol. 60, 223–244. doi: 10.1016/0022-0981(82)90161-7
Falkowski P. G., Ziemann D., Kolber Z., Bienfang P. K. (1991). Role of eddy pumping in enhancing primary production in the ocean. Nature 352, 55–58. doi: 10.1038/352055a0
FAO, F.D (2003). The ecosystem approach to fisheries (Rome: FAO Technical Guidelines for Responsible Fisheries).
Frederiksen M., Edwards M., Richardson A. J., Halliday N. C., Wanless S. (2006). From plankton to top predators: Bottom-up control of a marine food web across four trophic levels. J. Anim. Ecol. 75, 1259–1268. doi: 10.1111/j.1365-2656.2006.01148.x
Gerardo A., Sylvia J., Ricardo S., Reginaldo D., Alejandro H., Martin H., et al. (2018). Distribution and abundance of the ichthyoplankton assemblages and its relationships with the geostrophic flow along the southern region of the California current. Lat. Am. J. Aquat. Res. 46, 104–119. doi: 10.3856/vol46-issue1-fulltext-12
Godø O. R., Samuelsen A., Macaulay G. J., Patel R., Hjøllo S.Sætre, Horne J., et al. (2012). Mesoscale eddies are oases for higher trophic marine life. PloS One 1, e30161. doi: 10.1371/journal.pone.0030161
Han D., Ikeya T., Takahashi M. M. (2004). Netplankton chlorophyll biomass along 175°E in the pacific ocean. J. Oceanogr. 60, 543–551. doi: 10.1023/B:JOCE.0000038347.66564.a5
Hou G., Wang J., Liu L., Chen Y., Pan C., Lin J., et al. (2021). Assemblage structure of the ichthyoplankton and its relationship with environmental factors in spring and autumn off the pearl river estuary. Front. Mari. Sci. 8. doi: 10.3389/fmars.2021.732970
Houde E. D. (1989). Comparative growth, mortality, and energetics of marine fish larvae: Temperature and implied latitudinal effects. Fish. Bull. 87, 471–495.
Hsieh C., Kim H. J., Watson W., Di Lorenzo E., Sugihara G. (2009). Climate-driven changes in abundance and distribution of larvae of oceanic fishes in the southern California region. Glob. Change Biol. 15, 2137–2152. doi: 10.1111/j.1365-2486.2009.01875.x
Hsieh H., Lo W. (2019). Mesoscale distribution and assemblage structure of fish larvae in the kuroshio waters off eastern Taiwan. Mar. Biodivers. 49, 1971–1986. doi: 10.1007/s12526-019-00958-8
Hsieh H., Lo W., Wu L., Liu D., Su W. (2011). Comparison of distribution patterns of larval fish assemblages in the Taiwan strait between the northeasterly and southwesterly monsoons. Zool. Stud. 50, 491–505.
Hu D., Wang F., Sprintall J., Wu L., Riser S., Cravatte S., et al. (2020). Review on observational studies of western tropical pacific ocean circulation and climate. J. Oceanol. Limnol. 38, 906–929. doi: 10.1007/s00343-020-0240-1
Hu D., Wu L., Cai W., Gupta A. S., Ganachaud A., Qiu B., et al. (2015). Pacific western boundary currents and their roles in climate. Nature 522, 299–308. doi: 10.1038/nature14504
Huang D., Zhang X., Jiang Z., Zhang J., Arbi I., Jiang X., et al. (2017). Seasonal fluctuations of ichthyoplankton assemblage in the northeastern south China Sea influenced by the kuroshio intrusion. J. Geophys. Res. Oceans 122, 7253–7266. doi: 10.1002/2017JC012906
Itano G. D. (2000). The reproductive biology of yellowfin tuna (Thunnus albacares) in Hawaiian waters and the Western tropical pacific ocean: Project summary. Joint Institute Mar. Atmospheric Res.
Jiang Y., Lin B., He H., Ding G., Yan L., Zhang G., et al. (2021). Species composition and assemblages of ichthyoplankton in sansha bay, fujian province, China. Front. Mar. Sci. 8. doi: 10.3389/fmars.2021.758089
Kodama T., Watanabe T., Taniuchi Y., Kuwata A., Hasegawa D. (2021). Micro-size plankton abundance and assemblages in the western north pacific subtropical gyre under microscopic observation. PloS One 16, e250604. doi: 10.1371/journal.pone.0250604
Korsman B. (2013). The spacial and temporal community structure of ichthyoplankton in a northeast Florida estuary: A study of ingress at a faunal boundary (Gainesville: University of North Florida).
Kurogi H., Mochioka N., Okazaki M., Takahashi M., Miller M. J., Tsukamoto K., et al. (2012). Discovery of a spawning area of the common Japanese conger Conger myriaster along the Kyushu-Palau ridge in the western north pacific. Fish. Sci. 78, 525–532. doi: 10.1007/s12562-012-0468-6
Kuroki M., Miller M. J., Feunteun E., Sasal P., Pikering T., Han Y., et al. (2020). Distribution of anguillid leptocephali and possible spawning areas in the south pacific ocean. Prog. Oceanogr. 180, 102234. doi: 10.1016/j.pocean.2019.102234
Loeb V. J., Smith P. E., Moser H. G. (1983). Geographical and seasonal patterns of larval fish species structure in the California current are (California: CalCOFI).
Lukas R. (2001). Pacific ocean equatorial currents, encyclopedia of ocean sciences, 3rd Edn. (London: Elsevier) 429–436.
Mahadevan A., Asaro E. D., Lee C., Perry M. J. (2012). Eddy-driven stratification initiates north Atlantic spring phytoplankton blooms. Science 337, 54–58. doi: 10.1126/science.1218740
McClatchie S., Cowen R., Nieto K., Greer A., Luo J. Y., Guigand C., et al. (2012). Resolution of fine biological structure including small narcomedusae across a front in the southern California bight. J. Geophys. Res. Oceans 117, C04020. doi: 10.1029/2011JC007565
Moku M., Tsuda A., Kawaguchi K. (2003). Spawning season and migration of the myctophid fish Diaphus theta in the western north pacific. Ichthyol. Res. 50, 52–58. doi: 10.1007/s102280300007
Moser H. G., Smith P. E. (1993). Larval fish assemblages of the California current region and their horizontal and vertical distributions across a front. B. Mar. Sci. 53, 645–691.
Nagai N., Tadokoro K., Kuroda K., Sugimoto T. (2015). Latitudinal distribution of chaetognaths in winter along the 137°E meridian in the Philippine Sea. Plankton. Benthos. Res. 10, 141–153. doi: 10.3800/pbr.10.141
Nunn A. D., Tewson L. H., Cowx I. G. (2012). The foraging ecology of larval and juvenile fishes. Rev. Fish Biol. Fish. 22, 377–408. doi: 10.1007/s11160-011-9240-8
Ohizumi H., Yoshioka M., Mori K. (1998). Stomach contents of common dolphins (Delphinus delphis) in the pelagic Western north pacific. Mar. Mammal Sci. 14, 835–844. doi: 10.1111/j.1748-7692.1998.tb00767.x
Oka E., Ishii M., Nakano T., Suga T., Kouketsu S., Miyamoto M., et al. (2018). Fifty years of the 137°E repeat hydrographic section in the western north pacific ocean. J. Oceanogr. 74, 115–145. doi: 10.1007/s10872-017-0461-x
Okiyama M. (2014). An atlas of early stage fishes in Japan second edition (Hadano: Tokai University Press).
Ozawa T., Tsukahara H. (1973). On the occurrence of the engraulid fish, stolephorus buccaneeri strasburg, in the oceanic region of the equatorial Western pacific: Life history and distribution Vol. 17 (Kagoshima University: Laboratory of Fisheries Resources, Faculty of Fisheries), 151–171.
Peiro-Alcantar M. T., Funes-Rodríguez R., González-Armas R., Durazo R., Del Monte Luna P. (2016). Spatiotemporal variability of demersal fish larvae assemblages in the southern region of the California current. Mar. Biol. Res. 12, 524–540. doi: 10.1080/17451000.2016.1164319
Perkins A., Ratcliffe N., Suddaby D., Ribbands B., Smith C., Ellis P., et al. (2018). Combined bottom-up and top-down pressures drive catastrophic population declines of Arctic skuas in Scotland. J. Anim. Ecol. 87, 1573–1586. doi: 10.1111/1365-2656.12890
Phillips K. L., Jackson G. D., Nichols P. D. (2001). Predation on myctophids by the squid Moroteuthis ingens around macquarie and heard islands: Stomach contents and fatty acid analyses. Mar. Ecol. Prog. Ser. 215, 179–189. doi: 10.3354/meps215179
Sassa C. (2019). “Reproduction and early life history of mesopelagic fishes in the kuroshio region: A review of recent advances,” in Geophysical monograph series. Eds. Nagai T., Saito H., Suzuki K., Takahashi M. (Hoboken: John Wiley &Sons, Inc.), 273–294.
Sassa C., Hirota Y. (2013). Seasonal occurrence of mesopelagic fish larvae on the onshore side of the kuroshio off southern Japan. Deep Sea Res. Part I: Oceanogr. Res. Pap. 81, 49–61. doi: 10.1016/j.dsr.2013.07.008
Sassa C., Kawaguchi K. (2004). Larval feeding habits of Diaphus garmani and Myctophum asperum (Pisces: Myctophidae) in the transition region of the western north pacific. Mar. Ecol. Prog. Ser. 278, 279–290. doi: 10.3354/meps278279
Sassa C., Kawaguchi K., Hirota Y., Ishida M. (2004). Distribution patterns of larval myctophid fish assemblages in the subtropical-tropical waters of the western north pacific. Fish Oceanogr 13, 267–282. doi: 10.1111/j.1365-2419.2004.00289.x
Sassa C., Kawaguchi K., Hirota Y., Ishida M. (2007a). Distribution depth of the transforming stage larvae of myctophid fishes in the subtropical-tropical waters of the western north pacific. Deep Sea Res. Part I: Oceanogr. Res. Pap. 54, 2181–2193. doi: 10.1016/j.dsr.2007.09.006
Sassa C., Kawaguchi K., Kinoshita T., Watanabe C. (2002). Assemblages of vertical migratory mesopelagic fish in the transitional region of the western north pacific. Fish Oceanogr. 11, 193–204. doi: 10.1046/j.1365-2419.2002.00199.x
Sassa C., Kawaguchi K., Taki K. (2007b). Larval mesopelagic fish assemblages in the kuroshio-oyashio transition region of the western north pacific. Mar. Biol. 150, 1403–1415. doi: 10.1007/s00227-006-0434-x
Sassa C., Takasuka A. (2019). Distribution of Symbolophorus californiensis (Teleostei: Myctophidae) in the kuroshio region during late winter: Evidence of a southward spawning migration. Deep Sea Res. Part I: Oceanogr. Res. Pap. 150, 103053. doi: 10.1016/j.dsr.2019.05.013
Shida O., Hamatsu T., Nishimura A., Suzaki A., Yamamoto J., Miyashita K., et al. (2007). Interannual fluctuations in recruitment of walleye pollock in the oyashio region related to environmental changes. Deep Sea Res. Part II Top. Stud. Oceanogr. 54, 2822–2831. doi: 10.1016/j.dsr2.2007.09.001
Su W., Lo W., Liu D., Wu L., Hsieh H. (2011). Larval fish assemblages in the kuroshio waters east of Taiwan during two distinct monsoon seasons. B. Mar. Sci. 87, 13–29. doi: 10.5343/bms.2010.1010
Sun D., Wang C. (2017). Latitudinal distribution of zooplankton communities in the Western pacific along 160°E during summer 2014. J. Mar. Syst. 169, 52–60. doi: 10.1016/j.jmarsys.2017.01.011
Sutton T. T., Hulley P. A., Wienerroither R., Zaera-Perez D., Paxton J. R. (2020). Identification guide to the mescopelagic fishes of the central and south east Atlantic ocean. FAO species identification guide for fishery purposes (Rome: FAO).
Tiedemann M., Fock H. O., Döring J., Badji L. B., Möllmann C. (2018). Water masses and oceanic eddy regulation of larval fish assemblages along the cape Verde frontal zone. J. Mar. Syst. 183, 42–55. doi: 10.1016/j.jmarsys.2018.03.004
Tittensor D. P., Mora C., Jetz W., Lotze H. K., Ricard D., Berghe E. V., et al. (2010). Global patterns and predictors of marine biodiversity across taxa. Nature 466, 1098–1101. doi: 10.1038/nature09329
Van der Lingen C., Huggett J. A. (2003). “The role of ichthyoplankton surveys in recruitment research and management of south African anchovy and sardine,” in Proceedings of the 26th annual larval fish conference. Eds. Browman H. I., Skiftesvik A. B.(Norway: Institute of Marine Research), 303–343.
Walsh H. J., Richardson D. E., Marancik K. E., Hare J. A. (2015). Long-term changes in the distributions of larval and adult fish in the northeast U.S. Shelf Ecosystem. PloS One 10, e137382. doi: 10.1371/journal.pone.0137382
Wan R., Zhang R. (2016). Fish eggs, larvae and juveniles in the offshore waters of China and their adjacent waters (Shanghai: Shanghai Scientific & Technical Publishers).
Wang F., Wang Q., Zhang L., Hu D., Hu S., Feng J. (2019). Spatial distribution of the seasonal variability of the north equatorial current. Deep Sea Res. Part I Oceanogr. Res. Pap. 144, 63–74. doi: 10.1016/j.dsr.2019.01.001
Yamamura O., Inada T. (2001). Importance of micronekton as food of demersal fish assemblages. B. Mar. Sci. 68, 13–25.
Yang G., Li C., Wang Y., Wang X., Dai L., Tao Z., et al. (2017). Spatial variation of the zooplankton community in the western tropical pacific ocean during the summer of 2014. Cont. Shelf Res. 135, 14–22. doi: 10.1016/j.csr.2017.01.009
Yang G., Wang F., Li Y., Lin P. (2013). Mesoscale eddies in the northwestern subtropical pacific ocean: Statistical characteristics and three-dimensional structures. J. Geophys. Res. Oceans 118, 1906–1925. doi: 10.1002/jgrc.20164
Zenimoto K., Kitagawa T., Miyazaki S., Sasai Y., Sasaki H., Kimura S. (2009). The effects of seasonal and interannual variability of oceanic structure in the western pacific north equatorial current on larval transport of the Japanese eel Anguilla japonica. J. Fish Biol. 74, 1878–1890. doi: 10.1111/j.1095-8649.2009.02295.x
Zhang H., Mammola S., Xian W., Zhang Z. (2019c). Modelling the potential impacts of climate change on the distribution of ichthyoplankton in the Yangtze estuary, China. Divers. Distrib. 26, 126–137. doi: 10.1111/ddi.13002
Zhang Z., Mammola S., Zhang H. (2020). Does weighting presence records improve the performance of species distribution models? A test using fish larval stages in the Yangtze estuary. Sci. Total Environ. 741, 140393. doi: 10.1016/j.scitotenv.2020.140393
Zhang H., Xian W., Liu S. (2019a). Seasonal variations of the ichthyoplankton assemblage in the Yangtze estuary and its relationship with environmental factors. PeerJ 7, e6482. doi: 10.7717/peerj.6482
Keywords: fish larvae, ichthyoplankton, Philippine Sea, seasonal change, spatial distribution, pelagic fish
Citation: Fang C, Wang X, Sun D, Zhai H, Zhang D, Liao G and Wang C (2023) Spatial distribution and seasonal variation of fish larvae in the upper 200 m of the Philippine Sea. Front. Mar. Sci. 10:1110980. doi: 10.3389/fmars.2023.1110980
Received: 29 November 2022; Accepted: 08 March 2023;
Published: 23 March 2023.
Edited by:
Bernardo Patti, National Research Council (CNR), ItalyReviewed by:
Marianna Musco, National Research Council (CNR), ItalyCopyright © 2023 Fang, Wang, Sun, Zhai, Zhang, Liao and Wang. This is an open-access article distributed under the terms of the Creative Commons Attribution License (CC BY). The use, distribution or reproduction in other forums is permitted, provided the original author(s) and the copyright owner(s) are credited and that the original publication in this journal is cited, in accordance with accepted academic practice. No use, distribution or reproduction is permitted which does not comply with these terms.
*Correspondence: Chunsheng Wang, d2FuZ3Npb0BzaW8ub3JnLmNu
Disclaimer: All claims expressed in this article are solely those of the authors and do not necessarily represent those of their affiliated organizations, or those of the publisher, the editors and the reviewers. Any product that may be evaluated in this article or claim that may be made by its manufacturer is not guaranteed or endorsed by the publisher.
Research integrity at Frontiers
Learn more about the work of our research integrity team to safeguard the quality of each article we publish.