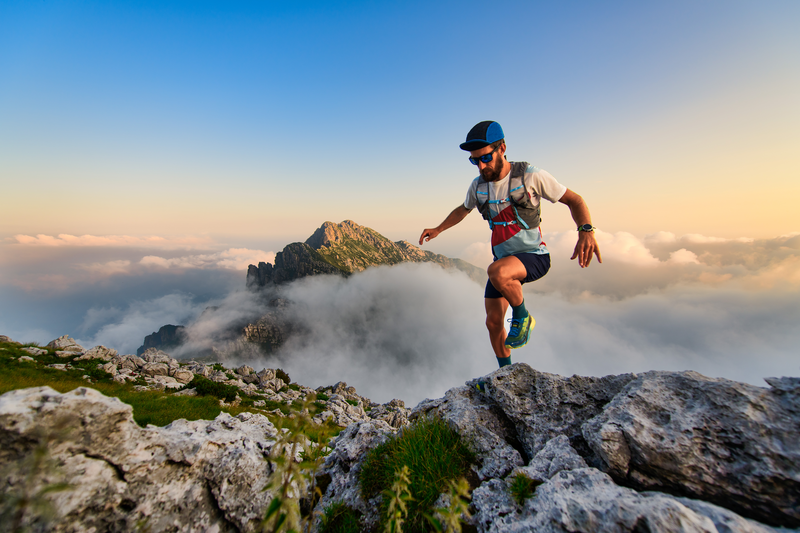
94% of researchers rate our articles as excellent or good
Learn more about the work of our research integrity team to safeguard the quality of each article we publish.
Find out more
ORIGINAL RESEARCH article
Front. Mar. Sci. , 09 February 2023
Sec. Marine Ecosystem Ecology
Volume 10 - 2023 | https://doi.org/10.3389/fmars.2023.1110621
This article is part of the Research Topic The Response of Microalgae and Plankton to Climate Change and Human Activities View all 12 articles
Diatoms are an important component of submarine biogenic sediments and often used for carrying out palaeoceanographical reconstructions. To understand the relationship between diatoms and environment in the western equatorial Pacific, diatoms from the Holocene sediments in the northern West Caroline Basin were selected for analysis. We made quantitative statistics on diatoms and divided diatom assemblages through cluster analysis. A total of 53 species or varieties of diatoms belonging to 22 genera were identified. The range of diatom abundance was 0-88,373 valves/g. The diatoms found were mainly oceanic warm-water species. Overall, Azpeitia nodulifera was the species with the highest contribution, followed by Hemidiscus cuneiformis and Thalassiosira pacifica with these three species together accounting for approximately 80% in this area. We identified four diatom groupings and divided the study area into three regions. Diatom assemblage I in the West Caroline Basin was mainly affected by the North Equatorial Counter Current (NECC), Antarctic Intermediate Water (AAIW) and upwelling, and the supply of nutrients was relatively high. Assemblage II in the West Caroline Ridge was less influenced by currents, and thus had lower abundance and species diversity than assemblage I. The complex diatom assemblages in the Yap Trench were probably controlled by Upper Circumpolar Deep Water (UCPW) and Lower Circumpolar Deep Water (LCPW).
The West Caroline Basin is located in the core of the Western Pacific Warm Pool, where the annual average sea-surface temperature is a minimum of 28°C (Wang et al., 2009; Qi et al., 2020; Chen et al., 2021). Sea temperature anomalies in the region influence climate change in the Asia-Pacific region and even the world by heating seawater and transporting radiant heat to the atmosphere (Long et al., 2021). Understanding the generation and burial of biological sediments in the region is crucial to understanding the global carbon cycle and changes in marine conditions (Lyle and Baldauf, 2015).
As one of the general primary producers, diatoms are an important component of submarine biogenic sediments. In sea areas where the water depth is greater than the carbonate compensation depth, fossil diatom frustules are relatively well preserved (Zhang et al., 2015). The distribution of diatoms in sediment deposits, and the factors that control their distributions, are often discussed by researchers when reconstructing both contemporary environments and paleoenvironments (Chen et al., 2019). Diatoms from upper sediments have been studied extensively in various regions (Esper and Gersonde, 2014; Ren et al., 2014), allowing for reliable paleoceanographic reconstructions. (Tsoy and Moiseenko, 2014; Shen et al., 2017b). However, only a few investigations were published in the western Pacific. In the West Philippine Basin, the relative abundance of seven tropical pelagic diatoms was greater than 20%, and their distribution pattern was influenced by the flow of the Kuroshio Current (Zhai et al., 2010; Chen et al., 2014; Shen et al., 2017a). In the southern Mariana Trench, Cai (2019) found a large number of Ethmodiscus rex (Rattray) Hendey and other small diatoms, dominated by warm-water and eurythermal species.
The purpose of this study was to investigate the species composition and abundance of diatoms in Holocene sediments of the northern West Caroline Basin. We analyzed the environmental factors that control the distribution of diatoms by considering a series of processes, including their production, sedimentation and burial, and explored the differences of diatom assemblages in different environments and their causes. This study enriched diatom research in the western Pacific and contributed to the establishment of a comprehensive diatom–based data set, which was necessary for carrying out future paleoceanographic reconstructions in the region.
The study area is located in the northern West Caroline Basin (136°0′-142°0′E, 4°0′-9°0′N; Figure 1). The bathymetric range of the study area is highly variable (2395-7837 m), with an average water depth of 4,015 m, including the Yap Trench (> 6500 m), the West Caroline Ridge (< 3000 m), and a flat topographic basin in the southern part (Qi et al., 2020; Chen et al., 2020a). In general, the water depth gradually decreases from southwest to northeast. Because the study area is located in the deep sea, it is influenced little by continental runoff and the seawater possesses a high transparency of 20-40 m throughout the year (Yue et al., 2018; Zhang, 2020).
Figure 1 (A) Study area (dashed boxes I and II indicate the study areas of Shen et al. 2017a and Cai (2019), respectively) and (B) Sampling stations. The red arrows represent surface and subsurface currents (Hu et al., 2015; Pujari et al., 2021), the yellow arrows represent deep currents (Kawano et al., 2006; Kender et al., 2018; Chen et al., 2020a; Liu et al., 2022), and the green arrows represent the Antarctic Intermediate Water (Bingham and Lukas, 1995). Abbreviations: NEC, North Equatorial Current; NECC, North Equatorial Counter Current; NEUC, North Equatorial Undercurrent; NGCC, New Guinea Coastal Current; NGCUC, New Guinea Coastal Undercurrent; LUC, Luzon Undercurrent; MC: Mindanao Current; ME, Mindanao Eddy; MUC, Mindanao Undercurrent; AAIW, Antarctic Intermediate Water; LCPW, Lower Circumpolar Deep Water; UCPW, Upper Circumpolar Deep Water.
The West Caroline Basin experiences a typical tropical marine climate with high temperature and rainfall all year round (Qi et al., 2020). The thermocline depth in the study area is 100-150 m. The surface seawater possesses high temperature, low salinity and low nutrient content, and is characterized by a two-layer system. A barrier layer separates the nutrient-rich deep water from the surface water (Vinogradov, 1981; Kobayashi and Takahashi, 2002; Chen et al., 2021).
The many currents in the western Pacific (Zhang, 2008) comprise water masses of different characteristics that have an important impact on phytoplankton including diatoms. Formed from the eastward diversion of Mindanao Current (MC), the North Equatorial Counter Current (NECC;1-8°N) crosses the West Caroline Basin from west to east and influences the study area (Christian et al., 2004). The Antarctic Intermediate Water (AAIW; 500-1200 m water depth) enters the equatorial western Pacific Ocean through the Vitiaz Channel and its northwestward branch flows to and affects the study area (Bingham and Lukas, 1995). The deep Upper Circumpolar Deep Water (UCPW) and Lower Circumpolar Deep Water (LCPW) currents do not directly affect diatoms in the euphotic zone, but, may play a role in the sedimentation and burial of diatom frustules.
The studied samples were obtained from the Global Change and Air-Sea Interaction Special-West Pacific Block PAC-CJ15 Submarine Sediment and Benthic Organisms Investigation Project. The samples were collected using a box corer from April to June 2017 (Zhang et al., 2021; Zhou et al., 2021). We selected 80 upper sediment samples (0-10 cm) for analysis, and the samples contained sediments since the Holocene referring to the sedimentation rate near the study area (Cai, 2019). The distribution of sampling stations is shown in Figure 1B.
All samples were processed in the diatom analysis laboratory of the Third Institute of Oceanography, Ministry of Natural Resources, Xiamen, China. We prepared samples following a modified version of the method of Hakansson (1984). Briefly, samples weighing ca. 5 g were dried in an oven at 60°C and weighed before processing. Samples were treated with 10% HCL and 30% H2O2 to remove carbonates and organic material, respectively, and washed in distilled water to remove these chemicals from the solution. Samples were then soaked in distilled water for 24 h and scattered using an ultrasonic dispersion instrument (120 Hz) for 2 min. Diatoms (>15 μm) were the main components, which indicates their relationship to the environment would be reliable. Small diatoms (<15 μm) have been shown to little effect on the results of this type of study, so were not considered (Chen et al., 2019). Therefore, samples were filtered through a 15-µm sieve to remove microdiatoms and other material finer than 15 µm, after which the suspension containing diatoms was concentrated to 2 ml. When completely homogeneous, the sub-sample of the suspension was transferred to a cover slip and air-dried. Finally, three permanent slides were made for each sample using Canadian balsam as a fixative (Chen et al., 2020b). The prepared slides were observed and identified under an optical microscope (Olympus BX51, objective lens 40×, ocular lens 20×), and at least 300 diatom valves were counted for each sample. For incomplete individuals, valves were counted if they were more than half complete. Diatom classification and identification mainly followed illustrations from published studies (Jin et al., 1982; Round et al., 1990; Jin et al., 1991; Cheng et al., 1996; Guo and Qian, 2003; Qi and Li, 2004; Smol and Stoermer, 2010; Cheng and Gao, 2012). Because Ethmodiscus rex specimens in the samples were fragmentary, we performed only area-based qualitative statistics.
The total abundance of diatoms is the total number of valves per gram of air-dried sediment (valves/g). Species diversity was calculated using the Shannon-Weaver index (Shannon and Weaver, 1949). The diatom assemblages were statistically analyzed using the R software environment and correlated with various environmental factors. Prior to statistical analysis, data were first standardized for all species. In the analysis, species with >2% relative abundance in at least one sample were included (Imbrie and Kipp, 1971), and rare species (those occurring at fewer than five stations) and data for station E13 (where no diatoms were found) were excluded. Diatom assemblages were differentiated using cluster analysis. Since detrended correspondence analysis showed a maximum gradient of 1.68 < 2, redundancy analysis (RDA) was used to determine whether differences in the assemblage vectors of each sample were statistically significant.
The environmental data obtained in this study included sea-surface temperature (SST), salinity (SSS), dissolved oxygen (DO), nutrients (silicate, phosphate and nitrate), chlorophyll a, net primary production (NPP), particulate organic carbon (POC) and water depth (WD). Data such as SST and SSS were obtained in situ using the SBE 911Plus Conductivity-Temperature-Depth system; nutrient and oxygen levels were derived from the CARS2009 dataset for 1930 to 2009 with 1/2°-resolution (Qu et al., 2022); chlorophyll a and POC concentrations for 1997 to 2009 at 9-km resolution were downloaded from https://oceancolor.gsfc.nasa.gov/l3/; NPP data for 1997 to 2009 with 1/6°-resolution were downloaded from http://www.science.oregonstate.edu/ocean.productivity/custom.php; and WD data in those figures from the GEBCO-2020 Grid at 15 arc-second intervals were downloaded from https://data.noaa.gov/onestop.
According to the data obtained from in situ measurements and websites (Figure 2), SST at each station varied relatively little, with a range of 28.90 to 29.27°C (average 29.12°C). The SSS variation was also small, from 33.94 to 34.07 (average 33.99) whereas the DO content varied from 4.52 to 4.56 ml/l (average 4.54 ml/l). Nitrate and phosphate contents varied little, ranging from 0.03 to 0.06 µmol/l and 0.04 to 0.11 µmol/l, respectively, whereas silicate content varied widely (1.11-2.97 µmol/l) and showed a trend of gradual increase from the northeast to the southwest. This increase occurs because the study area is located in the main pathway for the invasion of the silica-rich AAIW into the Northwest Pacific Ocean (Reid, 1973; Zhang, 2008; Zhang et al., 2016). In addition, NPP, POC and chlorophyll a content all showed a gradual increase from north to south, indicating higher phytoplankton biomass and primary production in the southern part of the study area.
A total of 53 species or varieties of diatoms belonging to 22 genera were identified at 80 stations. The relative percentages of each taxa were listed in the table of supplementary material. Diatom abundance varied from 0 to 88,373 valves/g, with an average of 14,319 valves/g. Some individual stations (E13, C03, B04, A01) yielded few diatoms, although diatom abundance was generally high, especially in the southern part of the study area where the abundance was generally greater than 20,000 valves/g (Figure 3). The diatom abundance and species diversity in the southern deep-sea basin of the study area were higher than those in the West Caroline Ridge and Yap Trench in the north. Correlation analysis showed a strong positive correlation between diatom abundance and the content of chlorophyll a and POC (Table 1). Silicate was the most significant environmental factor affecting the distribution of diatom abundance, and there was a negative correlation between phosphate and diatom abundance.
In this study, diatoms with relative percentages more than 10% were designated as dominant species, and those with percentages greater than 5% but less than 10% were designated as sub–dominant species (Pokras and Molfino, 1986). Therefore, the dominant species were Azpeitia africanus, Azpeitia nodulifera, Coscinodiscus sp., Hemidiscus cuneiformis, Synedra sp., Synedra tabulata, Thalassiosira pacifica and Thalassiosira leptopus, with the sub-dominant species being Coscinodiscus argus, Coscinodiscus decrescens, Roperia tesselata, Thalassionema nitzschioides and Thalassiosira excentrica (Table 2 and Figure 4). Azpeitia nodulifera (an oceanic warm-water species; Shen et al., 2017a) had the highest mean percentage content, followed by H. cuneiformis (an oceanic warm-water species; Shen et al., 2017a) and T. pacifica (a cosmopolitan species; Halse and Syvertsen, 1996). Overall, these three species together accounted for approximately 80% in this area.
All stations in the study area were dominated by warm-water diatoms (Figure 5), which constituted on average 79.81% of the diatom abundance. The most abundant species were A. nodulifera, H. cuneiformis and A. africanus, which made up 78.62% of the total. Other warm-water species included Asteromphalus heptactis, Asterolampra marylandica, Asterolampra sp., Coscinodiscus radiatus, Mastogloia cocconeiformis, Rhizosolenia bergonii, Roperia tesselata and Triceratium pentacrinus. This diversity of warm-water species was consistent with the environmental conditions of the Western Pacific Warm Pool, where the study area was located. In addition, because of the location in the deep sea, the diatoms were mainly planktonic taxa (average 99.67%) and benthic diatoms occurred only sporadically in the study area (Figure 5).
Ethmodiscus rex fragments were widely distributed in the West Caroline Basin (Figure 6). The valves of E. rex are huge and readily broken, so most of the individuals recorded were present as fragments. High concentrations of E. rex fragments were mainly recorded at stations in the deeper basins and troughs in the southern part of the study area, whereas the number of fragments in the shallow West Caroline Ridge was generally low. The correlation analysis showed that E. rex abundance had a weak positive correlation with WD. The study of E. rex in the southern Mariana Trench by Cai (2019) also found that this species was more abundant in deeper waters and less abundant in shallower waters, and that there was a positive correlation between E. rex abundance and WD, consistent with our findings.
Figure 6 Distribution of Ethmodiscus rex fragments in the study area. There are definitions for 'a few' (< 10,000 per g), 'many' (10,000–1,000,000 per g) and 'a great many' (> 1,000,000 per g).
For the statistical analysis of species data, species with relative percentages > 2% were selected and rare species (those occurring at fewer than five stations) were excluded. The environmental data included WD, SST, SSS, silicate, nitrate, phosphate, DO, POC, NPP and chlorophyll a. The RDA results for environmental variables and samples, and for environmental variables and diatom species, are provided in Table 3 and Figures 7, 8. The first two RDA axes explained 35.8% and 23.2% of the total variation, respectively. Correlation between environmental variables and RDA axes are shown by both length and angle of arrows. Using cluster analysis, the 80 samples could be divided into four groupings based on the composition of the diatom species and diatom abundance (Figures 7, 9).
Figure 7 RDA biplot of environmental variables and samples. The eigenvalues for RDA axis 1 and 2 are 0.010 and 0.007, respectively. SST, sea-surface temperature; SSS, sea-surface salinity; DO, dissolved oxygen; Si, silicate; P, phosphate; N, nitrate; POC, particulate organic carbon; Chlor, chlorophyll a; NPP, net primary production; WD, water depth.
Figure 8 RDA biplot of diatom taxa and environmental factors. The eigenvalues for RDA axis 1 and 2 are 0.010 and 0.007, respectively. SST, sea-surface temperature; SSS, sea-surface salinity; DO, dissolved oxygen; Si, silicate; P, phosphate; N, nitrate; POC, particulate organic carbon; Chlor, chlorophyll a; NPP, net primary production; WD, water depth.
Figure 9 Distribution of diatom assemblages in the study area. The red arrow represents the NECC, the green arrow represents the AAIW and the yellow arrows represent deep currents.
Assemblage I occurred at most stations, particularly in the deep-sea basins in the central and southern part of the study area. The diatoms were mainly oceanic warm-water species A. nodulifera and H. cuneiformis, with some coastal species (e.g., S. tabulata, C. argus, and Coscinodiscus divisus) and a few benthic species, such as A. undulatus. The percentage abundances of the species in the assemblage were as follows: A. nodulifera 34.19% to 90.70% (average 68.16%); H. cuneiformis 0% to 15.16% (average 5.70%); S. tabulata 0% to 29.06% (average 2.87%); and A. undulatus 0% to 2.04% (average 0.32%). The total diatom abundance in this assemblage was generally high (3,696-88,373 valves/g), with an average of 17,826 valves/g. In addition, warm-water species only accounted for 78.37% of the assemblage, because of the relatively high number of eurythermal species. Assemblage I was positively correlated with SST and silicate and negatively correlated with phosphate and represents a tropical oceanic environment.
Assemblage II was mainly detected in the northern part of the study area at the West Caroline Ridge. This assemblage has a species composition similar to Assemblage I, dominated by A. nodulifera, H. cuneiformis and T. pacifica. The relative percentages were: A. nodulifera 63.16% to 91.3% (average 76.89%); H. cuneiformis 0% to 19.05% (average 8.52%); T. pacifica 0% to 8.11% (average 2.41%); and the coastal species C. argus 0% to 5.41% (average 1.53%). Compared to Assemblage I, the total diatom abundance of this assemblage was markedly lower (640-2483 valves/g), with an average of 1,705 valves/g, and warm-water species accounted for 88.04% of the assemblage. Assemblage II was positively correlated with phosphate and negatively correlated with SST and silicate. The water depth of its tropical oceanic environment is shallower than Assemblage I.
Assemblage III was detected at two stations, A01 and B04, located in the waters near the Yap Trench. This assemblage exhibited both a low total diatom abundance (average 132 valves/g) and a relatively homogeneous species composition, with only three diatom species identified. The average abundances of A. nodulifera, H. cuneiformis and C. argus were 87.5%, 9.375% and 3.125%, respectively. Warm-water species represented a maximum of 96.875% of the total. Assemblage III was positively correlated with phosphate and DO.
The fourth assemblage only included station C03, located in the southern Yap Trench. The total abundance of diatoms at this station was only 76 valves/g, of which A. nodulifera constituted 50%, T. leptopus made up 25%, and the remainder were Coscinodiscus sp. The proportion of warm-water species was 50%. Assemblage IV was negatively correlated with DO and WD.
In the study area, warm-water diatoms possessed an absolute advantage, with the lowest percentage abundance of these diatoms being 44.44% at station I06, indicating a tropical oceanic environment. The tropical pelagic species A. nodulifera was the most abundant diatom at each station, consistent with the view that A. nodulifera is the main component of diatom assemblages in the surface sediments of the tropical Pacific (Jousé et al., 1971). E. rex fragments are also widely distributed in this area, corresponding with the nutrient-poor environment in the Western Pacific Warm Pool (Abrantes et al., 2007). Compared with study area I in the West Philippine Basin (Figure 1), there are the same four dominant species, A. africanus, A. nodulifera, H. cuneiformis and T. leptopus, indicating that the two areas possess similar growth conditions for diatoms. However, the proportion of warm-water diatoms, especially A. nodulifera, in the West Caroline Basin was obviously greater than in the West Philippine Basin as a result of latitudinal differences and a more typical pelagic environment.
T. nitzschioides is widely distributed at low latitudes, and is often used as an indicator of upwelling and increased production in coastal areas; however, the species is as abundant in nutrient-poor open oceans as in coastal areas, and is described as a cosmopolitan species (Kobayashi and Takahashi, 2002; Ren et al., 2014; Lyle and Baldauf, 2015). In this study, T. nitzschioides was mainly detected in the high-productivity area in the southern West Caroline Basin (Figure 10). Considerable upwelling (3-10°N, strongest at 6-7°N) is observed in this area (Long et al., 2021), suggesting that T. nitzschioides is also good proxy for upwelling and increased production in the open ocean. Similarly, Kobayashi and Takahashi (2002) found T. nitzschioides may reflect weak upwelling in the equatorial western Pacific.
The distribution of diatoms in sediments is directly related to the different temperature and salinity conditions in the euphotic zone; however, diatom frustules experience dissolution in the water column, current transport and various geological processes during sinking and after deposition on the seafloor. As a result, there are many factors affecting the distribution of diatoms in sediments (Zhang et al., 2013; Astakhov et al., 2015). Using the diatom assemblage distribution (Figure 9), the study area was divided into three regions: West Caroline Basin, West Caroline Ridge and Yap Trench. The West Caroline Basin mainly contains diatom assemblage I, the West Caroline Ridge is dominated by diatom assemblage II, and the Yap Trench has a mixed assemblage and more complex diatom distribution.
Compared with the northern part of the study area, the deep-sea basin has higher primary production. Correspondingly, the total abundance of assemblage I is obviously higher than other assemblages. Sufficient nutrients are needed for diatoms to flourish in this area, and the supply of nutrients is likely from horizontal and vertical current transport.
Previous studies have shown that the NECC is the main cause of high chlorophyll a in the surface water of the Western Pacific Warm Pool and the New Guinea Coastal Current transports nutrients that fertilize the southern waters of the NECC region (Christian et al., 2004; Chen et al., 2017). In general, the west-to-east NECC delivers warm, nutrient-rich water, which is conducive to the growth and reproduction of diatoms in the study area. In addition, the AAIW exhibits low temperatures and high silicon and low phosphorus contents (Bostock et al., 2004; Zhang, 2008; Zhang, 2016; Kashino et al., 2020). The current brings silicon-rich water and increases the silicate content of surface water in the West Caroline Basin through upwelling, which also weakens the dissolution of siliceous fossils. Because of the strong influence of NECC, AAIW and upwelling in the West Caroline Basin, diatom assemblage I has higher abundance and species diversity than other assemblages.
Different diatom species have different nutrient requirements and the ratio of nutrients in water has a significant effect on diatom species diversity and abundance (Owen and Crossley, 1992). RDA analysis shows that A. nodulifera and H. cuneiformis are positively correlated with phosphate, S. tabulate is positively correlated with nitrate, whereas T. leptopus and T. pacifica are not sensitive to nutrient changes (Figure 8). Therefore, lower phosphate concentrations in the West Caroline Basin are responsible for the lower content of A. nodulifera and H. cuneiformis in assemblage I. There are also some coastal species such as S. tabulata, C. argus and C. divisus, and even the benthic species A. undulatus in assemblage I. We assume that the presence of these species is the result of NECC transport from the shallow coastal waters of the Palau Islands.
The low total abundance of assemblage II (640-2,483 valves/g) in the West Caroline Ridge is caused by the weak influence of NECC and AAIW in this region, resulting in limited supply of nutrients. The absence of T. nitzschioides in assemblage II also suggests that this region is hardly influenced by upwelling. Meanwhile, sediments on the ridge are easily scoured by currents (Sun et al., 2013; Shen et al., 2017a). The lack of nutrients (especially silicate) and upwelling, combined with an unfavorable topography for sediment accumulation, gives rise to the low abundance and species diversity of this assemblage.
As can be seen in Figure 2, the phosphate levels were relatively high in the West Caroline Ridge, and assemblage II was positively correlated with phosphate. Accordingly, the relative abundance of A. nodulifera and H. cuneiformis was very high (85.42%). The presence of coastal species such as C. argus, Actinocyclus ehrenbergii and Bacteriastrum hyalinum in assemblage II indicates an input of terrestrial material from the northern islands.
Similar to the West Caroline Ridge, the Yap Trench is located at the margin of the NECC and is less affected by this current, causing diatom abundance to be generally low. In addition, the diatom species composition varied greatly among stations in this area, and the distribution of diatom assemblages was complex. Previous studies have shown that UCPW and LCPW (deeper than UCPW) are the main deep water masses in the Yap Trench and form a counterclockwise circulation in the south of the trench (Liu et al., 2022). The mixing of diatom assemblages in the region is assumed to be caused by the large topographic variations and migration of diatom frustules via abyssal flow.
A total of 53 diatom species or varieties belonging to 22 genera were identified in Holocene sediments of the northern West Caroline Basin. Diatom abundance varied from 0 to 88,373 valves/g, with an average of 14,319 valves/g. The dominant diatom species were A. africanus, A. nodulifera, Coscinodiscus sp., H. cuneiformis, Synedra sp., S. tabulata, T. leptopus and T. pacifica. Warm-water diatom species were overwhelmingly dominant, with the tropical oceanic species A. nodulifera being the dominant component in the diatom assemblages. T. nitzschioides reflected the upwelling and increased production in the West Caroline Basin.
Four groupings of diatoms were identified by cluster analysis and the study area could be divided into three regions. Diatom assemblage I in the West Caroline Basin was mainly affected by NECC, AAIW and upwelling, and the supply of nutrients was sufficient to maintain relatively high productivity. Assemblage II in the West Caroline Ridge was less influenced by currents, and the diatom species were dominated by A. nodulifera and H. cuneiformis, with less species diversity. The complex diatom assemblages in the Yap Trench were probably controlled by the deep UCPW and LCPW currents.
This study is the first to investigate Holocene diatom assemblage distributions and reveal their reflection on the environment in the West Caroline Basin, providing fundamental new information for paleoceanographic reconstruction in the western Pacific based on fossil diatom records.
The raw data supporting the conclusions of this article will be made available by the authors, without undue reservation.
MC: conceptualization, methodology, writing - review and editing, supervision. GH: data curation, drawing, writing - original draft. JisX: resources, investigation, background information collection. CW: data curation. JizX: resources, investigation. HQ: supervision, writing - references editing. AZ: Chinese to English. All authors contributed to the article and approved the submitted version.
We acknowledge financial support from the National Key Research and Development Program of China (Grant No. 2019YFE0124700), the Global Change and Air-Sea Interaction Project granted by the Ministry of Natural Resources of China (Grant No. GASI-02-PACCJ15), and the National Natural Science Foundation of China (41976198, 91858203).
We are grateful to the crew and scientists on the GASI-02-PAC-CJ15 cruise, who collected the samples analyzed in this study. We thank Sev Kender, from Liwen Bianji (Edanz) (www.liwenbianji.cn) for editing the English text of a draft of this manuscript.
The authors declare that the research was conducted in the absence of any commercial or financial relationships that could be construed as a potential conflict of interest.
All claims expressed in this article are solely those of the authors and do not necessarily represent those of their affiliated organizations, or those of the publisher, the editors and the reviewers. Any product that may be evaluated in this article, or claim that may be made by its manufacturer, is not guaranteed or endorsed by the publisher.
The Supplementary Material for this article can be found online at: https://www.frontiersin.org/articles/10.3389/fmars.2023.1110621/full#supplementary-material
Abrantes F., Lopes C., Mix A., Pisias N. (2007). Diatoms in southeast pacific surface sediments reflect environmental properties. Quat. Sci. Rev. 26 (1-2), 155–169. doi: 10.1016/j.quascirev.2006.02.022
Astakhov A. S., Bosin A. A., Kolesnik A. N., Obrezkova M. S. (2015). Sediment geochemistry and diatom distribution in the chukchi Sea: application for bioproductivity and paleoceanography. Oceanography 28 (3), 190–201. doi: 10.5670/oceanog.2015.65
Bingham F. M., Lukas R. (1995). The distribution of intermediate water in the western equatorial pacific during January-February 1986. Deep-Sea Res. Part I 42 (9), 1545–1573. doi: 10.1016/0967-0637(95)00064-D
Bostock H. C., Opdyke B. N., Gagan M. K., Fifield L. K. (2004). Carbon isotope evidence for changes in Antarctic intermediate water circulation and ocean ventilation in the southwest pacific during the last deglaciation. Paleoceanography 19 (4), 4013. doi: 10.1029/2004PA001047
Cai Y. (2019). Sedimentary diatoms records and their paleoenvironmental indications in the southern Mariana trench and its adjacent areas (Xiamen, China: Xiamen University).
Cheng Z., Gao Y. (2012). Marine bacillariophyta pennatae (I) flora of China seas (Beijing: Science Press).
Chen M., Lan B. B., Shen L. N., Lan D. Z., Fang Q., Qi H. S. (2014). Characteristics of diatom distribution in the surface sediments of the Western Philippine basin. Acta Micropalaeontol. Sin. 31 (4), 321–334.
Chen M., Li Y. H., Qi H. S., Wang L., Zhang A. M., Shen L. N., et al. (2019). The influence of season and typhoon morakot on the distribution of diatoms in surface sediments on the inner shelf of the East China Sea. Mar. Micropaleontol. 146 (2019), 59–74. doi: 10.1016/j.marmicro.2019.01.003
Chen M., Qi H. S., Intasen W., Kanchanapant A., Wang C. T., Zhang A. M. (2020b). Distributions of diatoms in surface sediments from the chanthaburi coast, gulf of Thailand, and correlations with environmental factors. Reg. Stud. Mar. Sci. 34, 100991. doi: 10.1016/j.rsma.2019.100991
Chen Z., Sun J., Chen D. W., Wang S. H., Yu H., Chen H. T., et al. (2021). Effects of ocean currents in the Western pacific ocean on net-phytoplankton community compositions. Diversity-Basel 13 (9), 428. doi: 10.3390/d13090428
Chen Y. Y., Sun X. X., Zhu M. L., Zheng S., Yuan Y. Q., Denis M. (2017). Spatial variability of phytoplankton in the pacific western boundary currents during summer 2014. Mar. Freshw. Res. 68 (10), 1887–1900. doi: 10.1071/MF16297
Chen K., Xu J. S., Li G. X., Tian J., Yang J. C., Zhou S., et al. (2020a). Grain-size characteristics of the surface clastic sediments on the southern margin of the yap trench and their provenance implications. Mar. Geol. Quat. Geol. 40 (5), 46–57. doi: 10.16562/j.cnki.0256-1492.2019110601
Christian J. R., Murtugudde R., Ballabrera-Poy J., Mcclain C. R. (2004). A ribbon of dark water: phytoplankton blooms in the meanders of the pacific north equatorial countercurrent. Topical Stud. Oceanogr. 51 (1-3), 209–228. doi: 10.1016/j.dsr2.2003.06.002
Esper O., Gersonde R. (2014). New tools for the reconstruction of pleistocene Antarctic sea ice. Palaeogeogr. Palaeoclimatol. Palaeoecol. 399, 260–283. doi: 10.1016/j.palaeo.2014.01.019
Guo Y., Qian S. (2003). Marine bacillariophyta centricae flora of China seas (Beijing: Science Press).
Håkansson H. (1984). The recent diatom succession of Lake HavgårdssjÖn, south Sweden. In: Mann D. G. Ed. Proceedings of the Seventh International Diatom Symposium (Otto Koeltz: Philadelphia), 411–429.
Halse G. R., Syvertsen E. E. (1996). “Marine diatoms,” in Identifying marine diatoms and dinoflagellates, 5–385. Academic Press, The United States. doi: 10.1016/B978-012693015-3/50005-X
Hu D. X., Wu L. X., Cai W. J., Gupta A. S., Ganachaud A., Qiu B., et al. (2015). Pacific western boundary currents and their roles in climate. Nature 522 (7556), 299. doi: 10.1038/nature14504
Imbrie J., Kipp N. G. (1971). “A new micropaleontological method for quantitative paleoclimatology: Application to a late pleistocene Caribbean core,” in The late Cenozoic glacial ages. Ed. Turekian K. (New Haven, Conn: Yale Univ. Press), 71–181.
Jin D., Cheng Z., Lin J., Liu S. (1982). Benthic diatoms of China seas (Beijing: China Ocean Press).
Jousé A. P., Kozlova O. G., Muhina V. V. (1971). Distribution of diatoms in the surface layer of sediment from the pacific ocean. Micropaleontol. Oceans, 263–269.
Kashino Y., Hasegawa T., Syamsudin F., Ueki I. (2020). Temperature and salinity variability at intermediate depths in the western equatorial pacific revealed by TRITON buoy data. J. Oceanogr. 76 (2), 121–139. doi: 10.1007/s10872-019-00530-9
Kawano T., Fukasawa M., Kouketsu S., Uchida H., Doi T., Kaneko I., et al. (2006). Bottom water warming along the pathway of lower circumpolar deep water in the pacific ocean. Geophys. Res. Lett. 33 (23), 23613–1-236. doi: 10.1029/2006GL027933
Kender S., Bogus K. A., Cobb T. D., Thomas D. J. (2018). Neodymium evidence for increased circumpolar deep water flow to the north pacific during the middle Miocene climate transition. Paleoceanogr. Paleoclimatol. 33 (7), 672–682. doi: 10.1029/2017PA003309
Kobayashi F., Takahashi K. (2002). Distribution of diatoms along the equatorial transect in the western and central pacific during the 1999 la niña conditions. Deep Sea Res. Part II: Topical Stud. Oceanogr. 49 (13-14), 2801–2821. doi: 10.1016/S0967-0645(02)00059-0
Liu X. H., Liu Y. Z., Cao W., Li D. (2022). Flow pathways of abyssal water in the yap trench and adjacent channels and basins. Front. Mar. Sci. 9. doi: 10.3389/fmars.2022.910941
Long Y., Noman M. A., Chen D. W., Wang S. H., Yu H., Chen H. T., et al. (2021). Western Pacific zooplankton community along latitudinal and equatorial transects in autumn 2017 (northern hemisphere). Diversity 13 (2), 58. doi: 10.3390/d13020058
Lyle M., Baldauf J. (2015). Biogenic sediment regimes in the neogene equatorial pacific, IODP site U1338: Burial, production, and diatom community. Palaeogeogr. Palaeocl. 433, 106–128. doi: 10.1016/j.palaeo.2015.04.001
Owen R. B., Crossley R. (1992). Spatial and temporal distribution of diatoms in sediments of lake Malawi, central Africa, and ecological implications. J. Paleolimnol. 7 (1), 55–71. doi: 10.1007/BF00197031
Pokras E., Molfino B. (1986). Oceanographic control of diatom abundances and species distributions in surface sediments of the tropical and southeast Atlantic. Mar. Micropaleontol. 10 (1-3), 165–188. doi: 10.1016/0377-8398(86)90028-9
Pujari L., Narale D., Kan J. J., Wu C., Zhang G. C., Ding C. L., et al. (2021). Distribution of chromophytic phytoplankton in the eddy-induced upwelling region of the West pacific ocean revealed using rbcL genes. Front. Microbiol. 12. doi: 10.3389/fmicb.2021.596015
Qi Z., Xu J., Li G., Zhao M., Zhai K., Long H., et al. (2020). The variation of carbonate lysocline depth in the western tropical pacific ocean. Acta Geol. Sin. 94 (12), 3852–3863. doi: 10.19762/j.cnki.dizhixuebao.2020048
Qu H. X., Wang J. B., Xu Y., Xu J. S., Li X. Z. (2022). Radiolarian assemblages controlled by ocean production in the western equatorial pacific. Deep-Sea Res. Part I 182, 103721. doi: 10.1016/j.dsr.2022.103721
Reid J. L. (1973). The shallow salinity minima of the pacific ocean. Deep Sea Res. Oceanogr. Abstr. 20 (1), 51–68. doi: 10.1016/0011-7471(73)90042-9
Ren J., Gersonde R., Esper O., Sancetta C. (2014). Diatom distributions in northern north pacific surface sediments and their relationship to modern environmental variables. Palaeogeogr. Palaeocl. 402, 81–103. doi: 10.1016/j.palaeo.2014.03.008
Round F. E., Crawford R. M., Mann D. G. (1990). Diatoms: biology and morphology of the genera (Cambridge, UK.: Cambridge university press).
Shannon C. E., Weaver W. (1949). The mathematical theory of communication (Urbana, IL) (Illinois, USA: University of illinois Press IL).
Shen L. N., Chen M., Lan B. B., Qi H. S., Zhang A. M., Lan D. Z., et al. (2017a). Diatom distribution as an environmental indicator in surface sediments of the West Philippine basin. Chin. J. Oceanol. Limn. 35 (2), 431–443. doi: 10.1007/s00343-016-5306-8
Shen L. N., Gao A. G., Li C., Chen M. (2017b). Diatom distribution and its relationship to sediment property in the minjiang estuary, southeast China. Acta Oceanol. Sin. 36 (10), 20–30. doi: 10.1007/s13131-017-1070-7
Smol J. P., Stoermer E. F. (2010). The diatoms: applications for the environmental and earth sciences (Cambridge, UK: Cambridge University Press).
Sun M. Q., Lan D. Z., Fu P., Chen M., Ye Y. Q. (2013). Diatom distribution in surface sediment and its relation with environment factors in the south China Sea. J. Appl. Oceanogr. 32 (1), 46–51. doi: 10.3969/J.ISSN.2095-4972.2013.01.006
Tsoy I. B., Moiseenko I. A. (2014). Diatoms from surface sediments of amurskiy bay, Sea of Japan. Russ. J. Mar. Biol. 40 (1), 10–23. doi: 10.1134/S106307401401009X
Vinogradov M. (1981). “Ecosystems of equatorial upwellings,” in Analysis of marine ecosystems. Ed. Longhurst A. (London: Academic Press), 69–93.
Wang H. N., Chen J. N., Lv X. Y. (2009). Spatial and temporal variability in ocean temperature in West pacific warm pool and its effect in ENSO cycle. Oceanol. Et Limnol. Sin. 40 (1), 1–7. doi: 10.11693/hyhz200901001001
Yue X. A., Yan Y. X., Ding H. B., Sun C. J., Yang J. P. (2018). Biological geochemical characteristics of the sediments in the yap trench and its oceanographic significance. Periodical Ocean Univ. China 48 (3), 88–96. doi: 10.16441/j.cnki.hdxb.20170145
Zhai B., Li T. G., Chang F. M., Cao Q. Y. (2010). Vast laminated diatom mat deposits from the west low-latitude pacific ocean in the last glacial period. Chin. Sci. Bull. 55 (2), 171–175. doi: 10.1007/s11434-009-0447-1
Zhang Y. H. (2008). The analyses of the subsurface water masses and intermediate water masses of the tropical Western pacific and their decadal variation (Shandong Province, China: Institute of Oceanology, Chinese Academy of Sciences).
Zhang S. (2016). The upper water body structure evolution in the core region of the Western pacific warm pool and its correlation to the ENSO-like process and ITCZ shift since MIS10 (Shandong Province, China: Institute of Oceanology, Chinese Academy of Sciences), 172.
Zhang Z. Y. (2020). Geomorphological characteristics and its influence to surface sedimentation in the junction of yap trench and Mariana trench, Western pacific ocean (Shandong Province, China: Shandong University of Science and Technology).
Zhang J. P., Deng X. G., Yang S. X., He G. W., Zhu B. D. (2015). Diatom ooze found in 7000 m submarine area of challenger depth in Mariana trench. Geol. Bull. Of China 34 (12), 2352–2354.
Zhang J. P., Deng X. G., Zhu B. D., Yang S. X., Guo L. H., Chen C. X., et al. (2016). Diatom ooze from the surface sediments in the challenger deep of the Western pacific ocean. Acta Micropalaeontol. Sin. 33 (1), 1–8. doi: 10.16087/j.cnki.1000-0674.2016.01.001
Zhang Y., Xu J. S., Li G. X., Liu Y. (2021). ENSO-like patterns and its driving mechanism in Western pacific warm pool during the glacial cycles. Earth Sci. Front. 29 (4), 168–178. doi: 10.13745/j.esf.sf.2022.1.5
Zhang J. P., Zhu B. D., Chen C. X., Zhang J. Y. (2013). Distribution pattern of diatom in surface sediments of the northwestern south China Sea and its environmental implications. Mar. Geol. Front. 29 (11), 8–13. doi: 10.16028/j.1009-2722.2013.11.003
Keywords: diatom, western equatorial Pacific, environmental significance, West Caroline Basin, Holocene sediment
Citation: Chen M, Huang G, Xu J, Wang C, Xu J, Qi H and Zhang A (2023) Diatom distribution in Holocene sediments from the northern West Caroline Basin (western equatorial Pacific) and their environmental significance. Front. Mar. Sci. 10:1110621. doi: 10.3389/fmars.2023.1110621
Received: 29 November 2022; Accepted: 20 January 2023;
Published: 09 February 2023.
Edited by:
Yanpei Zhuang, Jimei University, ChinaReviewed by:
Jinpeng Zhang, Guangzhou Marine Geological Survey, ChinaCopyright © 2023 Chen, Huang, Xu, Wang, Xu, Qi and Zhang. This is an open-access article distributed under the terms of the Creative Commons Attribution License (CC BY). The use, distribution or reproduction in other forums is permitted, provided the original author(s) and the copyright owner(s) are credited and that the original publication in this journal is cited, in accordance with accepted academic practice. No use, distribution or reproduction is permitted which does not comply with these terms.
*Correspondence: Min Chen, Y2hlbm1pbkB0aW8ub3JnLmNu; Jishang Xu, amlzaGFuZ3h1QG91Yy5lZHUuY24=
Disclaimer: All claims expressed in this article are solely those of the authors and do not necessarily represent those of their affiliated organizations, or those of the publisher, the editors and the reviewers. Any product that may be evaluated in this article or claim that may be made by its manufacturer is not guaranteed or endorsed by the publisher.
Research integrity at Frontiers
Learn more about the work of our research integrity team to safeguard the quality of each article we publish.