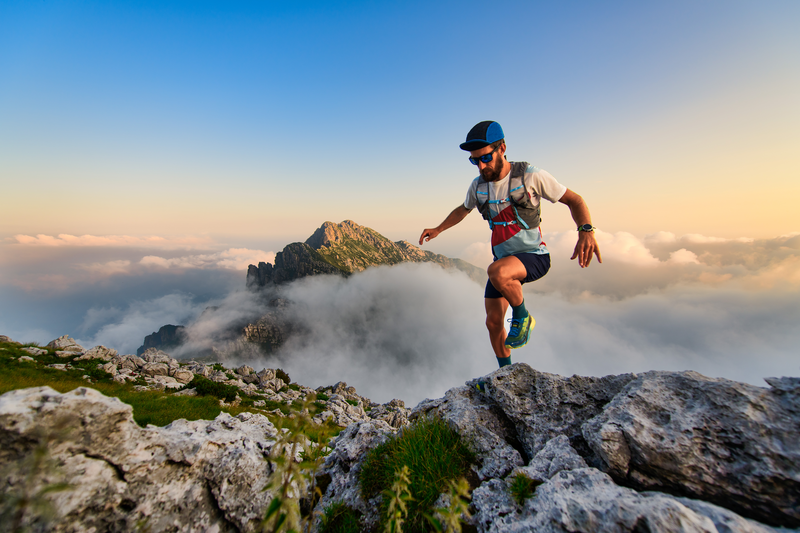
94% of researchers rate our articles as excellent or good
Learn more about the work of our research integrity team to safeguard the quality of each article we publish.
Find out more
ORIGINAL RESEARCH article
Front. Mar. Sci. , 27 February 2023
Sec. Marine Ecosystem Ecology
Volume 10 - 2023 | https://doi.org/10.3389/fmars.2023.1104528
This article is part of the Research Topic Biological Adaptations and Ecological Responses of Mangrove Ecosystem to Environmental Stresses and Changes View all 4 articles
Introduction: The waters surrounding the Wanshan Islands are important traditional fishing grounds in China, with rich habitat types. Acanthopagrus latus is an economically important species in this area; however, the distribution of its spawning grounds and habitat use patterns remain unknown.
Methods: Thus 100 otolith samples of A. latus were collected from three geographic areas (MW: Qi’ao Island Mangrove Water Habitat; OW: Yamen Estuary Oyster Farm Water Habitat; RW: Dong’ao-Guishan Island Reef Water Habitat), and the concentrations of Sr and Ca along the shortest axis of the vertical otolith annual or lunar rings were measured to span the entire life cycle of A. latus, with the core and edge areas corresponding to environmental characteristics at birth and capture, respectively.
Results and discussion: Analysis of covariance (ANCOVA) revealed that the ratios of Sr/Ca in otolith edges of RW samples are significantly higher than those of OW and MW samples; however, both the values of Sr/Ca ratio in otolith cores collected from OW and MW are comparable with those of RW samples. Cluster analysis and non-metric multidimensional scaling (nMDS) indicated that at the juvenile stage, RW and MW individuals in the two main clusters belonged to the same cluster. There was no significant difference between the cores of the RW samples and the edges of the MW and OW samples. Therefore, the spawning area of A. latus in the Wanshan Islands is thought to have originated from low to medium-salinity waters with mangroves and oyster farm habitats in the Pearl River Estuary. A. latus from RW was found to have three distinct habitat-use patterns: 1) Marine Resident (7.2% of sampled fish) fish that remain in marine habitats for life; 2) Marine Migrant (16.4% of sampled fish) juveniles inhabit low to moderate salinity habitats and migrate to marine habitats as they grow; 3) Estuarine Visitor (76.4% of sampled fish) repeated migration between low to moderate salinity and marine habitats. This suggests widespread migration between estuarine and marine habitats throughout the ontogeny. The plasticity of this habitat use and the protection of spawning grounds should be considered in future fisheries management because A. Latus in this area has been the victim of the overexploitation of resources.
The Wanshan Islands are coastal islands of China. It is located southeast of Zhuhai City in the Pearl River Estuary (21.8°N, 22.5°N, 113.5° 114.4°E). Rich nutrients were brought from the mainland and spread directly around the islands by the Pearl River, forming an important spawning and fattening ground for marine fish (Dai et al., 2019).
The yellowfin seabream Acanthopagrus latus (Houttuyn, 1782) is a eurythermal and euryhaline benthic reef fish that is an important economic species in the Wanshan Islands in the Pearl River Estuary (Liu et al., 2017). A. latus is a congenital hermaphrodite that spawns gradually and steadily (Eskandari et al., 2013). Females release eggs in small numbers during the spawning season (Eskandari et al., 2013) before becoming either a functional male or a functional female during the following spawning season. Once a fish becomes a functional female, it remains a female for the rest of its life. (Hesp et al., 2004). The spawning period of A. latus differed greatly between regions. The spawning season in the northern South China Sea last from October to January, with the maximum yield of juveniles occurring in late October (Jean et al., 2000). A.latus spawns in Shark Bay, Australia, in late winter and early spring. According to Liu et al. (2004) and Yang et al. (2004), the population of A. latus in the Pearl River Estuary appears to have genetic differentiation and belongs to an independent geographical group compared to those in the surrounding waters. Many reef fish, such as A. latus, experience habitat changes as they grow and develop (Moriniere et al., 2003; Chittaro et al., 2004; Menezes et al., 2021). They also move between habitats regularly to forage or lay eggs (Nagelkerken et al., 2000). However, not all fish migrate (Chapman et al., 2012a). Whether fish continue to inhabit or move relatively short distances movement within a watershed or migrate more widely between habitats depends on various factors, including the species and proximity of various habitats, and the ability to reproduce, forage, and avoid predators (Clarke et al., 2015). A. latus is a dominant species in the mangrove habitat of this area (Wu et al., 2018). Owing to overfishing and habitat destruction, the wild population has suffered a significant decline (Zhu et al., 2001; Xia et al., 2008; Chang et al., 2012). Exploring the migration movements and habitat utilization of A. latus is critical for developing and implementing resource conservation strategies.
However, the migration patterns of marine fish in open waters are difficult to study and traditionally rely on capturing, tagging, and recapturing individuals. Tracking multiple life-history stages of fish using conventional tagging techniques provides useful information for determining the timing and duration of habitat use; however, many tagging techniques are inherently biased. In addition, the early life stages of small and large fish are difficult to label, leading to a lack of information on some or all of their life cycles in many species (Bradford and Taylor, 1997; Clarke et al., 2015).
Teleost fish have three pairs of otoliths: sagittae, lapilli, and asterisci. Sagittae are usually chosen for elemental analysis because they are the largest of the three pairs (Yang et al., 2011). The growth of otoliths is the process of continuous adsorption of elements on the surface, and elemental buildup on the growth surface is permanently preserved and serves as an elemental record throughout the life history of the fish (Campana, 1999). Inorganic microelements in otoliths mainly come from water; therefore, the composition of microelements in otoliths of fish in different habitats varies. Strontium and calcium are some of the most commomly trace metals in otolith (Wang et al., 2009; Bounket et al., 2021). According to Farrell and Campana (1996) and Walther and Thorrold (2006), Sr and Ca in otoliths in freshwater and marine fish, respectively, are mainly from water (absorbed through gills). In fish migration studies, the Sr/Ca ratio is most commonly used because it is a better indicator of salinity in several species, the mean of which is positively correlated with the salinity of the surrounding water (Secor et al., 1995; Yang et al., 2011; Song et al., 2022; Vu et al., 2022). This method has been successfully applied to A. latus in other Chinese waters to determine population connectivity and birth origin (Chang et al., 2012) and to distinguish between farmed and wild individuals (Wang et al., 2018). At present, the Sr/Ca threshold value is widely used in migration research on various reef fish species, such as Haemulon flavolineatum in the Bahamas (Chittaro et al., 2004), Etelis coruscans and Etelis boweni in six Pacific Island countries (Sih et al., 2022), Diplodus sargus and Diplodus prayensis in the western Mediterranean (Bouchoucha et al., 2018), and Lutjanus jocu in eastern Brazil (Menezes et al., 2021).
In this study, the otolithic microelement ratios of A. latus in different habitats and life cycles were used to study their migration patterns in the Pearl River Estuary. First, we determined whether fish from different geographic areas spawned in similar environments. Second, research on A. latus growth and morphology revealed the dispersal patterns of individuals of different body sizes and ages in different habitats. Finally, the life history of A. latus in the Guishan-Dong’ao island reef habitat (RW) was investigated based on the three migratory movement patterns. This study aimed to test the hypothesis that A. latus, a reef habitat (marine), is capable of short-distance migration and that its larval stage is near mangrove (oligohaline) and oyster farm (mesohaline) habitats or inhabits places with similar water chemistry.
Overall 100 A. latus were collected, with 17 collected from the mangrove waters (MW) of Qi’ao Island in the winter of 2021, 55 collected from the waters of Guishan Island and Dong’ao Island (RW) in the autumn of 2021, and 28 tails collected from the oyster farm (OW) in the Yamen estuary in the winter of 2021 (Figure 1).
The fish samples collected are naturally dead catches caught by local fishermen, so we do not need to euthanize the fish. Immediately after collection, samples were stored in a refrigerator. After thawing the fish samples, a pair of otoliths were extracted from the cochlea. Soft tissue on the surface was removed, cleaned, and dried. Considering that otoliths were broken during the sampling process, intact otoliths were used consistently for elemental microchemical analysis.
The relationship between the age of an individual and morphological characteristics was divided into three stage groups, following Shi et al. (2012): age 1-year-old fish have a body length (BL) not less than 170 mm and 150 g weighs; age 2 years old fish have a BL not less than 220 mm and 330 g weighs; and age 3 years old fish have a BL not less than 260 mm and 560 g weighs. The largest individual can measure 350 mm in BL and 3350 g weigh.
Before chemical analyses, otoliths must first be cleaned, embedded, ground, and sliced. The selected otolith particles were fixed to double-sided adhesive tape, and the samples were arranged in designated positions. Then, epoxy resin is injected into the mold, vacuum, grind and polish the resin after curing, make the surface smooth, and then stick it to the 4.9 mm×2.6 mm×2 mm rectangle or other shaped ore nuggets. One side was ground with a 2200 W water cutter, then finely ground with 3000-grit sandpaper until the core of the otolith was exposed, and then washed in clean water. Next, a solid optical resin was used to adhere the fine polishing surface on the glass sheet, followed by rough grinding until the thickness was 300 μm, then polished on a polishing machine to make a laser sheet. This laser sheet can be used to investigate the interrelationship between opaque and transparent or translucent minerals on the same sheet. It is easy to observe changes in otolith textures by making laser sheets, and they will not be crushed if they are stored in a desiccator for a long time.
Trace-element mapping of the otolith was performed using an NWR 193 nm ArF Excimer laser-ablation system coupled to an iCAP RQ ICPMS at Guangzhou Tuoyan Analytical Technology Co., Ltd., Guangzhou, China. The advantages of LA-ICP-MS include the ability to observe patterns in the cross-section of the otolith, which corresponds to the longevity of the fish when sampled from the core to the edge; however, the disadvantage is that data post-processing takes a longer time (Sih et al., 2022).
The ICP MS was tuned using NIST 610 and NIST 612 standard glasses to yield low oxide production rates. The He carrier gas was fed into the cup at 0.7 l/min, and the aerosol was then mixed with 0.79 l/min of Ar make-up gas. For otoliths, the following 14 isotopes were measured (with their respective dwell times in milliseconds listed in parentheses):7Li, 23Na, 24Mg, 29Si, 43Ca, 55Mn, 57Fe, 59Co, 60Ni, 65Cu, 66Zn, 88Sr, 121Sb, and 137Ba, corresponding to a total dwell time of 140 ms. The laser fluence was 3.5 J/cm2, with a repetition rate of 20 Hz and a 10 um spot size corresponding to a scan speed of 5um/s. The raw isotope data were reduced using the “Baseline Subtract” and “Trace Elements Data Reduction Scheme”. The DRS runs within the IOLITE package of Paton et al. (2011). In IOLITE, user-defined time intervals were established for the baseline correction procedure to calculate session-wide baseline-corrected values for each isotope. Trace elements were calibrated using NIST612 and NIST 610 as external standards.
After the chemical analysis, the otolith sections were photographed under a microscope to observe the ablation lines. The edge and core region lengths (and their relationship with ablation lines) were determined on these images using Image-Pro Plus 4.5 software. As shown in supplementary materials (Appendix 1), the outermost 100 μm layer is defined as the edge region, and the innermost 100 μm layer is defined as the core region. The trace elements in the core area can reflect the environment of the hatching water. In contrast, those in the marginal otolith area can describe the water in the fish catchment area. Element/Ca ratios were averaged across the estimated area in the core and edge regions. To validate the Sr/Ca ratios as an indicator of A. latus habitat use and movement patterns, we used the edge of the values and the minimum and maximum values of the otolith elemental relations of intensity for each habitat. Only the edge of the otolith was examined, as this is the region of the otolith associated with the habitat of interest. The last 100 μm values refer to the edge of the otolith in individuals at different stages of life and represent the material deposited into the otolith most recently. Oligohaline signatures were based on otoliths from the northern waters of Qi’ao Island (mangrove habitat). Chemical signatures for the mesohaline areas were based on fish from the Yamen Estuary areas (oyster farm habitat). Marine signatures based on otoliths from the seas of Guishan Island and Dongao Island (reef habitat), the sites farthest from the coastline (approximately 70 km), were used (Table 1). Sr/Ca ratios (×1000) between 1 and 3 were considered oligohaline, 3 and 5 mesohaline, and > 5 marine (Figure 2).
Table 1 Descriptive statistics of A. latus and bottom seawater salinity and temperature at sampling sites.
Two approaches were used to assign samples as baseline. First, unknown sample-to-baseline comparisons were performed using Sr/Ca ratios and the averages for the various baseline (i.e., known salinity) groups. Then, transects across the otoliths for each individual were plotted and compared to the baseline data. In addition, discriminant function analysis of the Sr/Ca ratios was applied to the core and edge area average of each otolith from Guishan Island and Dongao Island (reef habitat) to ascertain the adherence of the samples to one of the suggested patterns of habitat use, as proposed by Franco et al. (2019): migrants, visitors, or permanent residents. Marine Migrant, that is, fish Sr/Ca ratios (<5) at the core showed a single increase to over five at the otolith edges. Estuarine Visitor (EV), that is, fish whose Sr/Ca ratios oscillate between estuarine (1–5) and marine (>5) ratios more than once. Marine Residents (MR), that is, fish with Sr/Ca ratios typical of being close to marine areas (>5).
Shapiro-Wilk and Levene tests were performed on all ratios to test the assumptions of normality and variance homogeneity, respectively. After logarithmic transformation, the Sr/Ca values of the edge and core satisfied normality and variance homogeneity (Shapiro-Wilk test and Levene test, p < 0.05). The potential effects of body length and weight on elemental ratios were examined using analysis of covariance (ANCOVA) and correlation tests (Pearson) to examine Sr/Ca at the margins and cores. No ratio was significantly correlated between body length or weight (ANCOVA and correlation test, p > 0.05). The Sr/Ca ratios of the three sampling points were analyzed using a univariate analysis of variance (ANOVA).
Ward’s hierarchical clustering analysis was used to investigate the similarity of the larval stages of the RW, OW, and MW samples based on Sr/Ca values corresponding to the core and marginal zones. Cluster analysis was performed without prior classification, and the number of clusters was determined using the visual aspects of the dendritic maps. Genetic correlation coefficients were used to assess whether dendrograms preserved pairwise distances between the original unmodeled datasets. Ward’s hierarchical clustering analysis was also confirmed using Euclidean distance-based non-metric multidimensional scaling (nMDS) analysis (Tanner et al., 2012). Finally, stress values are used to assess goodness of fit, with stress values < 0.1 providing a good representation of dimensionality reduction and stress values < 0.2 are good (Clarke, 1993).
Statistical analysis was performed using SPSS and Excel software, and cluster and nMDS analyses were performed using Primer software. Two-dimensional maps of Sr concentrations was drawn using iolite 4.0. The strontium content in otoliths is much lower than that of calcium; therefore, the ratio of strontium to calcium is standardized according to international practice. The ratio of calcium content to Sr and Ba contents ×103 was unified.
Table 1 shows the results of YSI measurements taken at the sampling points of the yellowfin seabream in the MW, RW, and OW regions. The average salinity (7.1 ppt) of the northern waters of Qi’ao Island in the MW area is lower than that of the southern waters (13.2 ppt); therefore, the salinity range is wider in this area. The otolith Sr/Ca threshold estimated for marine environment corresponds to the salinity of RW (31.8 ± 0.8, Table 1). A. latus from MW and OW were almost all less than 170 mm in BL, whereas those from RM were mostly greater than 170 mm in BL.
The ratios of Sr/Ca in otolith edges of RW samples are significantly higher than those of OW and MW samples (Figure 3B, p < 0.05); however, both the values of Sr/Ca ratio in otolith cores collected from OW and MW are comparable with those of RW samples (Figure 3A, p > 0.05). Therefore, it can be inferred that the waters inhabited by juvenile stages of A. latus from RW were similar in water chemistry to those inhabited by juvenile stages of A. latus from the other two locations. (Figure 3A). Furthermore, no significant difference existed between the core of the otolith from RW and the OW and MW otolith edges (p > 0.05). Therefore, it can be inferred that the juvenile stage of the yellowfin seabream from RW inhabited both OW and MW or areas with similar water chemistry to the two locations (Figure 3C).
Figure 3 Box plot of A. latus otolith edge and core elemental ratios. MW, mangrove habitat waters; OW, oyster farm habitat waters; RW, reef habitat waters. Different letters show significant differences between sampling sites (P < 0.05). (A) the core, (B) the edge, (C) the RW edge and MW and OW cores. a, b, ab represent differences in each other.
Ward’s hierarchical clustering analysis of elements/Ca from the otolith core region (infant stage) from RW, OW, and MW yielded two main clusters characterized by fish from the three habitats. The two clusters included 85% and 15% of the samples, with RW accounting for 61% and 54%, OW accounting for 6% and 32%, and MW accounting for 33% and 14% (Figure 4). The results of nMDS shows that some RW individuals were separated from the main group, MW was divided into two groups, and OW was mainly in one group. The results of nMDS and the cluster analysis are mutually supportive. (Figure 4) Therefore, it can be inferred that the RW and MW individuals in the two main groups belong to the same group at the juvenile stage.
All individuals in each region were grouped according to their body length. Most of the A. latus from RW were older than 1 year (Table 1); four body length groups were divided based on the relationship between age and BL (Figure 5B). To reduce the error caused by the difference in sample size between the body length groups, the samples from the other two locations were divided equally into three groups based on the body length range to ensure that the number of samples in each group was similar. The results showed that A. latus in the 150–171 mm MW group had the greatest change in Sr/Ca ratio, possibly due to a more obvious change trend in habitat salinity in its life history (Figure 5A). In A. latus from RW, the Sr/Ca ratio increased from the core to the edge. It can be inferred that there was a clear trend of moving from mid-low salinity to the ocean salinity region from birth to the present stage (Figure 5B). A. latus in the 88–117 mm and 134–164 mm groups from OW had Sr/Ca constantly lingered in the ocean and low to moderate salinity regions from the core to the edge (Figure 5C).
Figure 5 The Sr/Ca ratios (Mean±SE) along line transect from the core (0 μm) to the edge in the sagittal plane of A. latus otoliths from Wanshan islands. Grey areas are non-marine waters. The legend indicates the range of body lengths for each groups and n indicates the number of samples in group. (A) A. latus from MW, (B) A. latus from RW, (C) A. latus from OW.
Based on the Sr/Ca ratios of otoliths, the life history of individuals of different ages from RW were analyzed, and a random individual in each age group was selected. It was found that the 3-year-old individual (BL:329 mm) inhabits low-salinity waters until 1 year of age, progressing to the middle-salinity area when it grows and develops at 2 years old and finally to the marine salinity area after the age of 3 years old (Figure 6A). The 2-year-old individual (BL:241 mm) inhabited medium-salinity waters from birth to the age of 1, had already inhabited the ocean salinity area before reaching the age of 2 years, returned to medium salinity after it grew and developed, and finally returned to the ocean salinity zone after reaching the age of 3 years old (Figure 6C). A 1-year-old individual (BL:183 mm) spent most of his life in the ocean salinity zone (Figure 6B). The under-1-year-old individuals moved back and forth between the meso-salinity and oceanic salinity zones throughout their life history (Figure 6D). Random sampling does not represent the entire age group; however, the randomness of the life histories of the four individuals of different ages can be observed.
Figure 6 Sr/Ca ratio profiles of A. latus of different ages from RW. Individual size (Body length, BL) and habitat are also included in each graph. The vertical red dashed lines indicate mean annual growth increments; the yellow line indicates the month age (ring) position and the grey area indicates that the individual lives in the low to middle salinity area. Y-axis is Sr/Ca ratio. X-axis is distance to the core (mm). (A) the 3-year-old individual (BL:329 mm), (B) the 1-year-old individual (BL:183 mm), (C) the 2-year-old individual (BL:241 mm), (D) the under-1-year-old individuals(BL:139 mm).
The patterns were formed by the Sr/Ca ratios (Figure 7). Three patterns of movement were found in individuals from the RW areas according to the Sr/Ca ratios. The individuals of the first pattern (Figure 7A), assigned as MM, had Sr/Ca ratios that increased more consistently after forming the first annual ring. Individuals with the second pattern (Figure 7B), assigned as Estuarine Visitors (EV), oscillated between the estuarine and marine bands. A third pattern (Figure 7C), named Marine Resident (MR), showed no evidence of movement between the marine and estuarine systems. Marine Resident, Marine Migrant, and Estuarine Visitor patterns can be distinguished from each other by (Figure 8A) a consistent reddish color (Figure 8B) alternating greenish to reddish colors, and (Figure 8C) alternating greenish and reddish colors, respectively.
Figure 7 Types of profiles for the Sr/Ca ratios in otoliths of individuals from the RW. The grey area indicates that the individual lives in the low to middle salinity area. (A) The individuals of the Marine Resident pattern of movement. (B) The individuals of the Marine Migrant pattern of movement. (C) The individuals of the Estuarine Visitor pattern of movement.
Figure 8 Two-dimensional maps of Sr concentrations in the otoliths of A. latus. (A) Marine Resident, (B) Marine Migrant, (C) Estuarine Visitor. Black transect lines from the core to the edge of otoliths indicate putative annuli.
Fish classified as EV were the most frequent, accounting for 76.4 % of all individuals (Table 2). Most individuals have a low-salinity life history.
Table 2 Percent of individuals of A. latus with each pattern of movements in the RW according to the Sr/Ca ratios in otoliths.
Elemental concentrations in otolith cores can reflect spawning area environments (Mu et al., 2021), while the otolith edge can reflect the current habitat environment where the fish are caught. Spawning grounds are critical for fish survival and reproduction and play a key role in supplementing fishery resources (Wang et al., 2021). The difference analysis showed no significant differences between the otolith cores of the three sampling areas. It could be inferred that A. latus from the three areas probably originated from spawning areas with similar environmental conditions. There was no significant difference between the core of the RW otolith and the edge of the OW and MW otoliths, indicating that the RW otolith spawning area was probably in OW, MW, or low-to medium-salinity areas with similar water chemistry between the two sites. Liu et al. (2004) and Yang et al. (2004) showed that the A. latus population in the Pearl River Estuary appears to have genetic differentiation and belongs to an independent geographical group. According to Song et al. (2021) and Wang et al. (2018), populations of wild A. latus in various parts of the southeast coast of China were distinguished from the genetic and otolith element characteristics, respectively, indicating that the individual differences in each sampling site were relatively large. Part of this may be because A. latus does not migrate long distances. This is because, unless extreme conditions such as habitat destruction or ecological niche squeeze occur, for reef-trending fish, moving away from islands and reefs during long-distance migration increases the risk of predation and fishing. Numerous studies have shown that shallow soft-bottomed habitats, including mangroves and tidal flats, are important juvenile nursery sites (Krumme, 2004; Ram et al., 2020; Fierro-Arcos et al., 2021; Plumlee et al., 2022). The diet of adult A. latus in different habitats mainly consists of fish and bivalves (oysters and mussels) (Pan et al., 2021; Platell et al., 2007; Sourinejad et al., 2015). Therefore, oyster farms can serve as foraging sites for adult fish with reproductive abilities. Accordingly, we infer that A. latus spawns in the Wanshan Islands in low-to medium-salinity waters with mangroves and oyster farm habitats in the Pearl River Estuary. Tran et al. (2019) studied the nursery of A. latus in the Tien Yen Estuary, northern Vietnam, and indicated that the nursery was mainly from the low-to-medium salinity area of the estuary, which was consistent with our findings.
Cluster analysis and nMDS revealed differences and associations among the three habitats of A. latus populations. It is mainly divided into two categories: 85% of the A. latus juveniles belong to the same population. These results were consistent with those of the difference analysis. Most A. latus came from different habitats; however, the juveniles in the estuary lived in the same water chemistry environment. The difference from previous results is that a small number of individuals from RW are dispersed far from the main categories, reflecting the complexity of fish habitats. Certainly, the elements accumulated in otoliths are determined not only by the salinity characteristics of the water environment in which the individual lives but by other factors, such as the temperature characteristics of the water environment in which the individual lives (Nelson et al., 2018; Mondal et al., 2022), type of food ingested (Vrdoljak et al., 2021), ontogeny, and physiological factors (Yamada and Baba, 2009).
Coupling analysis of individual fish otolith microchemistry with age or body length has become a widely used approach to explore relevant aspects of fish ecology, such as linking individuals to specific habitats throughout their life cycles and elucidating migration and population structure (Sturrock et al., 2012). A. latus from MW had the greatest variation in Sr/Ca, possibly due to a noticeable change in the changing trend in habitat salinity throughout its life history. This phenomenon may be because the MW area is closest to the inland river and is thus most affected by the diluting water of the river. Its salinity varies greatly depending on the season (Sheng et al., 2010). This may also be due to the formation of salinity gradients in tidal creeks at various shore distances within the mangroves (Shafique et al., 2022). Juveniles are not very good swimmers and usually move in and out of mangroves with tides (Zhang et al., 2021). The Sr/Ca ratios of the larvae in the remaining two body length groups were relatively stable, and it can be inferred that they did not spread far from the hatching area. This may have been caused by offshore larvae retention (Schiemer et al., 2001) and the active components of larval dispersal, as demonstrated by Schludermann et al. (2012) in the Danube. The Sr/Ca ratio in A. latus from RW increased from the core to the edge, and there was no obvious difference in the changing trend of Sr/Ca between the different body length groups. It can be inferred that there is a clear trend of movement from low-medium to oceanic salinity regions from birth to the extant, which may not be related to body length. Sr/Ca changes were more stable in OW juvenile. Avigliano et al. (2021) indicated that in species inhabiting salinities of 20–35%, the Sr/Ca ratio had a limited ability to discriminate movement between habitats. Nevertheless, some individuals will experience a period of higher salinity habitats in the early developmental stage. Generally, marine fish may have different salinity requirements during reproduction, hatching, and development. Salinity changes often lead to corresponding changes in fish migration and clustering behavior (Xiong et al., 2014). The otolith Sr and Ca microchemical results of A. latus individuals from four age groups from RW show not only the common characteristics of the migration pattern of A. latus; however, they also show the differences and characteristics between individuals. A. latus requires a lower salinity water environment during the incubation period and mainly lives in higher salinity waters in the later growth stage.
In previous studies, A. latus was generally considered a reef-oriented fish that would not typically choose long-distance migration or migration in the wild (Shi et al., 2012); little is known about the migratory movement patterns of A. latus. The possibility of migration between habitats with different salinities is also discussed based on changes in the Sr/Ca time series of A. latus otoliths. Three migration patterns were inferred based on the cross-section of A. latus otoliths from the RW (n = 55), indicating individual differences in habitat use. For Marine Residents (7.2 % of all fish), the otolith growth axis showed constant Sr/Ca ratios, as they were captured in high salinity habitats. This chemical signature can be explained by individuals who did not enter the estuary as expected during infancy. Such habitats may be tide pools or shallow reefs that shelter juvenile fish from predators (Horn et al., 1998). Marine Migrants (16.4% of all fish) are fish that develop early in estuaries and migrate to marine habitats after a year or two. During the sampling, it was found that the body length of A. latus caught in RW was almost all >170 mm in adult fish, and the body length of A. latus caught in OW and MW was almost all <170 mm in juvenile fish (Table 1). There were obvious differences in the population characteristics between the different habitats. This pattern is present in many fish species and may be related to the high food availability, shelter, and protection from predators typically provided by saltwater habitats (Menezes et al., 2021). Fish classified as estuarine visitors were the most common, accounting for 76.4% of all individuals, and movement between estuarine and marine systems occurred mainly in fish measuring 210±49 mm in BL. Pollock (1982) used the marker-recapture method to study A.latus and discovered that on the east coast of Australia, smaller individuals were only active on a small scale within 6 km of the release site. In contrast, larger individuals have small-scale and large-scale activity records ranging from 10 to 90 km in length. This massive movement appears to be associated with traveling to and from the spawning areas. Our findings are consistent with those of Franco et al. (2019), confirming the theory of partial migration, which describes a population that includes migratory and resident individuals (Chapman et al., 2012a). Factors contributing to this phenomenon may be related to the environmental constraints or ecological interactions experienced by each fish, such as food resources (Brodersen et al., 2008), levels of predation stress (Skov et al., 2013), levels of fishing stress, and intraspecific competition (Chapman et al., 2012b). This may also be related to changes in feeding habits as A. latus grows. Nagelkerken et al. (2000) used underwater observation to study the food-providing function of Caribbean mangroves and explained how dietary changes in ontogeny were related to mangroves and associated with migration between nurseries and reef areas.
No researchers have conducted a comprehensive study on the migration pattern and habitat utilization of Acanthopagrus fish, such as A. latus, based on trace element characteristics in otoliths, especially in estuarine areas with complex water environments. This shows the significance of this study for future research on Acanthopagrus fish in estuaries.
Future studies can combine the distribution characteristics of trace elements in A. latus otoliths with their isotopic changes to further analyze the distribution characteristics of otolith elements further. Analyzing the relationship between the changes in otolith elements and changes in the water environment can provide critical insight for further study of the life history of reef-oriented fish, such as A. latus, and provide a detailed scientific basis and theoretical support for the assessment of estuarine reef fishery resources and management of fishery resource development and utilization in the Pearl River Estuary.
Based on the analysis of otolith microchemistry, A. latus was found to have three distinct habitat use patterns, and to be classified as estuarine visitors are the most common, accounting for 76.4% of all individuals. A. latus needs to live in low-salinity waters during the incubation period in the wild. Juvenile stages of A. latus from the three locations inhabit in the waters with a similar water chemistry. As adults, they mainly live in higher salinity waters, but some individuals can live in high-salinity waters for a period of time during their early development. Spawning areas in Wanshan Islands may originate from low salinity water in OW or MW. Our results indicate that A. latus in surveyed areas should be considered as a single management unit. Studying of the migration and life history may provide clues for resources conservation of A. latus. Meanwhile, the exact locations of spawning and migratory routes remain unclear and still need further examination.
The original contributions presented in the study are included in the article/Supplementary Material. Further inquiries can be directed to the corresponding authors.
The animal study protocol was approved by the committee on Laboratory Animal Welfare and Ethics of South China Sea Fisheries Research Institute, Chinese Academy of Fishery Sciences (nhdf2022-16 and 5th Dec. 2022).
GT: collected the data, analyzed the data, prepared figures, drafted the original manuscript. YL: funding acquisition, review and editing. ZH, SB, JY, JL, YS: collected the samples and investigation. TW, YX, PW, CL: conceived the ideas and designed the methodology. All authors contributed to the article and approved the submitted version.
This work was supported by National Key R&D Program of China (No. 2019YFD0901205, No. 2019YFD0901204, and No. 2019YFD0901201), Guangdong Basic and Applied Basic Research Foundation (No. 2019B1515120065), Science and Technology Planning Project of Guangdong Province (No. 2019B121201001), Central Public-interest Scientific Institution Basal Research Fund, CAFS (No. 2020TD16), and Central Public-interest Scientific Institution Basal Research Fund, South China Sea Fisheries Research Institute, CAFS (No. 2021SD04).
The authors appreciate all scientific staff and students for their assistance with sample collection and experiment implementation. We are grateful to the members of Guangdong Provincial Key Laboratory of Aquatic Economic Animal Breeding from College of life sciences in Sun Yat-sen University of China for a part of sample collections.
The authors declare that the research was conducted in the absence of any commercial or financial relationships that could be construed as a potential conflict of interest.
All claims expressed in this article are solely those of the authors and do not necessarily represent those of their affiliated organizations, or those of the publisher, the editors and the reviewers. Any product that may be evaluated in this article, or claim that may be made by its manufacturer, is not guaranteed or endorsed by the publisher.
The Supplementary Material for this article can be found online at: https://www.frontiersin.org/articles/10.3389/fmars.2023.1104528/full#supplementary-material
BL, Body length; EV, Estuarine Visitor; MW, Qi’ao Island Mangrove Water Habitat; MR, Marine Residents; nMDS, Non-metric multidimensional scaling; MW, Mangrove Water; OW, Yamen Estuary Oyster Farm Water Habitat; RW, Dong’ao-Guishan Island Reef Water Habitat.
Avigliano E., Ibañez A., Fabré N., Fortunato R. C., Méndez A., Pisonero J., et al. (2021). Unravelling the complex habitat use of the white mullet, Mugil curema, in several coastal environments from Neotropical pacific and Atlantic waters. Aquat. Conserv. 31 (4), 789–801. doi: 10.1002/aqc.3486
Bouchoucha M., Darnaude A. M., Brach-Papa C., Gonzalez J.-L, Lenfant P (2018). Growth, condition and metal concentration in juveniles of two diplodus species in ports. Mar. Pollut. Bull. 126, 31–42. doi: 10.1016/j.marpolbul.2017.10.086
Bounket B., Tabouret H., Gibert P., Bareille G., Pecheyran C., Carrel G., et al. (2021). Spawning areas and migration patterns in the early life history of Squalius cephalus (Linnaeus 1758): Use of otolith microchemistry for conservation and sustainable management. Aquat. Conservation: Mar. Freshw. Ecosystems. 31 (10), 2772–2787. doi: 10.1002/aqc.3682
Bradford M. J., Taylor G. C. (1997). Individual variation in dispersal behaviour of newly emerged chinook salmon (Oncorhynchus tshawytscha) from the upper fraser river, british columbia. Can. J. Fisheries Aquat. Sci. 54 (7), 1585. doi: 10.1139/f97-065
Brodersen J., Ådahl E., Brönmark C., Hansson L. A. (2008). Ecosystem effects of partial fish migration in lakes. Oikos. 117 (1), 40–46. doi: 10.1111/j.2007.0030-1299.16118.x
Campana S. E., Chouinard G. A., Hanson J. M., Frechet A. (1999). Mixing and migration of overwintering atlantic cod (gadus morhua) stocks near the mouth of the gulf of st. lawrence. Can. J. Fisheries Aquat. Sci. 56 (10), 1873–1881.
Chang M. Y., Tzeng W. N., You C. F. (2012). Using otolith trace elements as biological tracer for tracking larval dispersal of black porgy, Acanthopagrus schlegeli and yellowfin seabream, a. latus among estuaries of western Taiwan. Environ. Biol. Fishes. 95 (4), 491–502. doi: 10.1007/s10641-012-0081-7
Chapman B. B., Hulthén K., Brodersen J., Nilsson P. A., Skov C., Hansson L., et al. (2012b). Partial migration in fishes: Causes and consequences. J. Fish Biol. 81 (2), 456–478. doi: 10.1111/j.1095-8649.2012.03342.x
Chapman B. B., Skov C., Hulthen K., Brodersen J., Nilsson P. A., Hansson L. A., et al. (2012a). Partial migration in fishes: definitions, methodologies and taxonomic distribution. J. Fish Biol. 81 (2), 479–499. doi: 10.1111/j.1095-8649.2012.03349.x
Chittaro P. M., Fryer B. J., Sale P. F. (2004). Discrimination of french grunts (Haemulon flavolineatum, desmarest 1823) from mangrove and coral reef habitats using otolith microchemistry. J. Exp. Mar. Biol. Ecology. 308 (2), 169–183. doi: 10.1016/j.jembe.2004.02.021
Clarke K. R. (1993). Non-parametric multivariate analyses of changes in community structure. Austral Ecology. 18 (1), 117–143. doi: 10.1111/j.1442-9993.1993.tb00438.x
Clarke A. D., Telmer K. H., Shrimpton J. M. (2015). Movement patterns of fish revealed by otolith microchemistry: A comparison of putative migratory and resident species. Environ. Biol. Fishes. 98 (6), 1583–1597. doi: 10.1007/s10641-015-0384-6
Dai M., Liu H., Wu F., Gong X., Liao X., Huang H. (2019). Net-phytoplankton community and its relationship with environmental factors in the wanshan islands adjacent sea. Mar. Environ. Sci. 4, 540. doi: 10.13634/j.cnki.mes.2019.04.009
Eskandari G., Saghavi H., Najafabadi M. Z., Madiseh S. D., Koochaknejad E. (2013). Effect of salinity on reproductive performance of Acanthopagrus latus (Houttuyn) in spawning tanks. Aquaculture Res. 44 (4), 588–595. doi: 10.1111/j.1365-2109.2011.03062.x
Farrell J., Campana S. E. (1996). Regulation of calcium and strontium deposition on the otoliths of juvenile tilapia, Oreochromis niloticus. Comp. Biochem. Physiol. 115 (2), 103–109. doi: 10.1016/0300-9629(96)00015-1
Fierro-Arcos D., Jarrín J. R. M., Aburto-Oropeza O., Harvey E. S., Rastoin-Laplane E., Salinas-de-León P. (2021). Mangrove fish assemblages reflect the environmental diversity of the Galapagos islands. Mar. Ecol. Prog. Series. 664, 183–205. doi: 10.3354/meps13628
Franco T. P., Albuquerque C. Q., Santos R. S., Saint'Pierre T. D., Araujo F. G. (2019). Leave forever or return home? the case of the whitemouth croaker Micropogonias furnieri in coastal systems of southeastern Brazil indicated by otolith microchemistry. Mar. Environ. Res. 144, 28–35. doi: 10.1016/j.marenvres.2018.11.015
Hesp S. A., Potter I. C., Hall N. G. (2004). Reproductive biology and protandrous hermaphroditism in Acanthopagrus latus. Environ. Biol. Fishes. 70 (3), 257–272. doi: 10.1023/B:EBFI.0000033344.21383.00
Horn M. H., Martin K. L. M., Chotkowski M. A. (1998). Intertidal fishes: Life in two worlds Vol. 399 (California: Academic Press).
Jean C. T., Lee S. C., Chen C. T. (2000). Population structure of yellowfin seabream, Acanthopagrus latus, from the waters surrounding Taiwan, based on mtDNA sequences. Ichthyological Res. 47 (2), 187–192. doi: 10.1007/BF02684240
Krumme U. (2004). Patterns in tidal migration of fish in a Brazilian mangrove channel as revealed by a split-beam echosounder. Fisheries Res. 70 (1), 1–15. doi: 10.1016/j.fishres.2004.07.004
Liu H. Y., Jiang S. G., Su T. F., Gong S. Y. (2004). Polymorphism study of the mitochondrial DNA d-loop gene sequences from Sparus latus. J. Fisheries China. 28 (4), 371–374.
Liu J. L., Li H. L., Tang Y. J., Xie L. N., Zhong J. Y., Huang Q. Y., et al. (2017). Heavy metal pollution and risk analysis to human in economic fish of mangrove wetland in qi'ao island, zhuhai. Ecol. Science. 36 (05), 186–195. doi: 10.14108/j.cnki.1008-8873.2017.05.024
Menezes R., Moura P., Santos A., Moraes L. E., Condini M. V., Rosa R. S., et al. (2021). Habitat use plasticity by the dog snapper (Lutjanus jocu) across the abrolhos bank shelf, eastern Brazil, inferred from otolith chemistry. Estuarine Coast. Estuar. Coast. Shelf Sci. 263, 107637. doi: 10.1016/j.ecss.2021.107637
Mondal S., Chakrabarti R., Ghosh P. (2022). Multi-proxy (δ44/40Ca, Sr/Ca, and Δ47) study of fish otoliths for determination of seawater temperature. Chem. Geol. 605, 120950. doi: 10.1016/j.chemgeo.2022.120950
Moriniere E. C. D. L., Pollux B. J. A., Nagelkerken I., Hemminga M. A., Huiskes A. H. L., Velde G. V. D. (2003). Ontogenetic dietary changes of coral reef fishes in the mangrove-seagrass-reef continuum: Stable isotopes and gut-content analysis. Mar. Ecol. Prog. Series. 246, 279–289. doi: 10.3354/meps246279
Mu X. X., Zhang C., Zhang C. L., Yang J., Ren Y. P. (2021). Age-structured otolith chemistry profiles revealing the migration of conger myriaster in China seas. Fisheries Res. 239, 105938. doi: 10.1016/j.fishres.2021.105938
Nagelkerken I., Velde G. V. D., Gorissen M. W., Meijer G. J., Van't hof T., Den hartog C. (2000). Importance of mangroves, seagrass beds and the shallow coral reef as a nursery for important coral reef fishes, using a visual census technique. Estuar. Coast. Shelf Science. 51 (1), 31–44. doi: 10.1006/ecss.2000.0617
Nelson T. R., Devries D. R., Wright R. A. (2018). Salinity and temperature effects on element incorporation of gulf killifish fundulus grandis otoliths. Estuaries Coasts. 41 (4), 1164–1177. doi: 10.1007/s12237-017-0341-z
Pan W. N., Qin C. X., Zuo T., Yu G., Zhu W. T., Ma H. M., et al. (2021). Is metagenomic analysis an effective way to analyze fish feeding habits? a case of the yellowfin Sea bream Acanthopagrus latus (Houttuyn) in daya bay. Front. Mar. Sci. 8. doi: 10.3389/fmars.2021.634651
Paton C., Hellstrom J., Paul B., Woodhead J., Hergt J. (2011). Iolite: freeware for the visualisation and processing of mass spectrometric data. J. Anal. At. Spectrom. 26 (12), 2508–2518. doi: 10.1039/c1ja10172b
Platell M. E., Ang H. P., Hesp S. A., Potter I. C. (2007). Comparisons between the influences of habitat, body size and season on the dietary composition of the sparid Acanthopagrus latus in a large marine embayment. Estuarine Coast. Shelf Science. 72 (4), 626–634. doi: 10.1016/j.ecss.2006.11.026
Plumlee J. D., Kenworthy M. D., Gittman R. K., Keller D. A., Garnett E. E., Vaca-Pita L., et al. (2022). Remarkable euryhalinity of a marine fish lutjanus novemfasciatus in mangrove nurseries. Ecology. 103 (2), 1. doi: 10.1002/ecy.3582
Pollock B. R. (1982). Movements and migrations of yellowfin bream, Acanthopagrus australis (Guenther), in moreton bay, queensland as determined by tag recoveries. J. Fish Biol. 20 (3), 245–252. doi: 10.1111/j.1095-8649.1982.tb04705.x
Ram R., Pavan-Kumar A., Jaiswar A. K., Gireesh-Babu P., Krishna G., Chaudhari A. (2020). Identification of fish and shellfish larvae from mangroves using DNA barcodes. J. Coast. Res. 36 (5), 1106–1110. doi: 10.2112/JCOASTRES-D-19-00172.1
Schiemer F., Keckeis H., Reckendorfer W., Winkler G. (2001). The "inshore retention concept" and its significance for large rivers. Archiv fur Hydrobiologie 135 (2), 509–516. doi: 10.1127/lr/12/2001/509
Schludermann E., Tritthart M., Humphries P., Keckeis H. (2012). Dispersal and retention of larval fish in a potential nursery habitat of a large temperate river: An experimental study. Can. J. Fisheries Aquat. Sci. 69 (8), 1302–1315. doi: 10.1139/f2012-061
Secor D. H., Henderson-Arzapalo A., Piccoli P. M. (1995). Can otolith microchemistry chart patterns of migration and habitat utilization in anadromous fishes? J. Exp. Mar. Biol. Ecol. 192 (1), 15–33.
Shafique T., Khan M. A., Fatima S. U., Alamgir A. (2022). Multivariate and geospatial monitoring of water and soil quality impact on planted mangroves growth pattern at indus delta: A pilot study. J. Coast. Conserv. 26 (4), 1–19. doi: 10.1007/s11852-022-00873-4
Sheng J. Y., Tang L. Q., Ji X. M., Liu D. B. (2010). An examination of seasonal mean circulation and salinity distributions in the pearl river estuary of China using a nested-grid coastal ocean circulation model. Estuarine and Coastal Modeling 108–127. doi: 10.1061/41121(388)7
Sih T. L., Williams A. J., Hu Y., Kingsford M. J. (2022). High-resolution otolith elemental signatures in eteline snappers from valuable deepwater tropical fisheries. J. Fish Biol. 100 (6), 1475–1496. doi: 10.1111/jfb.15059
Shi X. F., Shi H. L., Lou B., Mao G. M. (2012). Biological characteristics and artificial propagation, culture technique for Sparus latus(Houttuyn). Hebei Fishery. 217 (1), 52–55.
Skov C., Chapman B. B., Baktoft H., Brodersen J., Brönmark C., Hansson L., et al. (2013). Migration confers survival benefits against avian predators for partially migratory freshwater fish. Biol. Letters. 9 (2), 20121178. doi: 10.1098/rsbl.2012.1178
Song P. Q., Liu C., Cai Z. Z., Liu S. G., Xiang J. L., Shan B. B., et al. (2021). Genetic characteristics of yellow seabream Acanthopagrus latus (Houttuyn 1782) (Teleostei: Sparidae) after stock enhancement in southeastern China coastal waters. Regional Stud. Mar. Science. 48 (2), 102065. doi: 10.1016/j.rsma.2021.102065
Song D. D., Xiong Y., Jiang T., Yang J., Zhong X. M., Tang J. H., et al. (2022). Early life migration and population discrimination of the small yellow croaker Larimichthys polyactis from the yellow Sea: inferences from otolith Sr/Ca ratios. J. Oceanology Limnology. 40 (2), 818–829. doi: 10.1007/s00343-021-1041-x
Sourinejad I., Ataei S. N., Kamrani E., Shojaei M. G. (2015). Feeding habits of yellowfin seabream, Acanthopagrus latus in the northern region of the Persian gulf. Caspian J. Environ. Sci. 13 (1), 31.
Sturrock A. M., Trueman C. N., Darnaude A. M., Hunter E. (2012). Can otolith elemental chemistry retrospectively track migrations in fully marine fishes? J. Fish Biol. 81 (2), 766–795. doi: 10.1111/j.1095-8649.2012.03372.x
Tanner S. E., Vasconcelos R. P., Cabral H. N., Thorrold S. R. (2012). Testing an otolith geochemistry approach to determine population structure and movements of European hake in the northeast Atlantic ocean and Mediterranean Sea. Fisheries Res. 125-126, 198–205. doi: 10.1016/j.fishres.2012.02.013
Tran T. T., Tran H. D., Kinoshita I. (2019). Simultaneous and sympatric occurrence of early juveniles of Acanthopagrus latus and A.schlegelii (Sparidae) in the estuary of northern Vietnam. Limnology. 20 (3), 321–326. doi: 10.1007/s10201-019-00581-3
Vrdoljak D., Matić-Skoko S., Peharda M., Uvanović H., Markulin K., Mertz-Kraus R., et al. (2021). Otolith fingerprints and tissue stable isotope information enable allocation of juvenile fishes to different nursery areas. Water. 13 (9), 1293. doi: 10.3390/w13091293
Vu A. V., Baumgartner L. J., Limburg K. E., Doran G. S., Mallen-Cooper M., Gillanders B. M., et al. (2022). Life history strategies of Mekong pangasiid catfishes revealed by otolith microchemistry. Fisheries Res. 249, 106239. doi: 10.1016/j.fishres.2022.106239
Walther B. D., Thorrold S. R. (2006). Water, not food, contributes the majority of strontium and barium deposited in the otoliths of a marine fish. Mar. Ecol. Prog. Series. 311, 125–130. doi: 10.3354/meps311125
Wang C. H., Lin Y. T., Shiao J. C., You C. F., Tzeng W. N. (2009). Spatio-temporal variation in the elemental compositions of otoliths of southern bluefin tuna Thunnus maccoyii in the Indian ocean and its ecological implication. J. Fish Biol. 75 (6), 1173–1193. doi: 10.1111/j.1095-8649.2009.02336.x
Wang D., Yao L., Yu J., Chen P., Hu R. (2021). Response to environmental factors of spawning ground in the pearl river estuary, china. J. Mar. Sci. Eng. 9 (7), 763. doi: 10.3390/jmse9070763
Wang X., Wang L., Lv S., Li T. (2018). Stock discrimination and connectivity assessment of yellowfin seabream (Acanthopagrus latus) in northern south China Sea using otolith elemental fingerprints. Saudi J. Biol. Sci. 25 (6), 1163–1169. doi: 10.1016/j.sjbs.2017.09.006
Wu Y. M., Zheng P. S., Liu N., Tang Y. J., Cui Z. M., Huang P. P., et al. (2018). Diversity of fish species in guangdong mangrove areas. Acta Scientiarum Natralium Universitatis Sunyatseni. 57 (5), 104–114. doi: 10.13471/j.cnki.acta.snus.2018.05.013
Xia J. H., Huang J. H., Gong J. B., Jiang S. G. (2008). Significant population genetic structure of yellowfin seabream Acanthopagrus latus in China. J. Fish Biol. 73 (8), 1979–1992. doi: 10.1111/j.1095-8649.2008.02010.x
Xiong Y., Liu H. B., Liu P. T., Tang J. H., Yang J., Jiang T., et al. (2014). Reconstructing habitat history of larimichthys polyactis in lüsi coastal waters of jiangsu province, China based on otolith microchemistry. J. Appl. Ecology. 25 (3), 836–842. doi: 10.13287/j.1001-9332.2013.0017
Yamada H., Baba K. (2009). Ontogenetic changes of trace elements in otoliths and their spatial variations in rabbitfish, Siganus fuscescens. Bull. Japanese Soc. Fisheries Oceanography. 73 (1), 8–15.
Yang J., Jiang T., Liu H. B. (2011). Are there habitat salinity markers of the Sr:Ca ratio in the otolith of wild diadromous fishes? a literature survey. Ichthyological Res. 58 (3), 291–294. doi: 10.1007/s10228-011-0220-8
Yang H. R., Jiang S. G., Su T. F., Lv J. L. (2004). Genetic diversity of two wild populations of Sparus latus by RAPD. J. Trop. oceanography. 23 (2), 55–61. doi: 10.1117/12.528072
Zhang Y. M., Yan S. Z., Wang W. Q., Wang M. (2021). Habitat use by fish across tidal cycles in a tropical estuarine mangrove ecosystem (Dongzhaigang bay, hainan, China). J. Coast. Res. 37 (1), 156–167. doi: 10.2112/JCOASTRES-D-20-00046.1
Keywords: Acanthopagrus latus, habitat, migration, otolith microelement, LA-ICP-MS
Citation: Tang G, He Z, Liu Y, Xiao Y, Bi S, Sun Y, Liang J, Wu P, Wang T, Ye J and Li C (2023) Acanthopagrus latus migration patterns and habitat use in Wanshan Islands, Pearl River Estuary, determined using otolith microchemical analysis. Front. Mar. Sci. 10:1104528. doi: 10.3389/fmars.2023.1104528
Received: 21 November 2022; Accepted: 14 February 2023;
Published: 27 February 2023.
Edited by:
Hao Cheng, South China Sea Institute of Oceanology (CAS), ChinaReviewed by:
Zhimao Mai, South China Sea Institute of Oceanology (CAS), ChinaCopyright © 2023 Tang, He, Liu, Xiao, Bi, Sun, Liang, Wu, Wang, Ye and Li. This is an open-access article distributed under the terms of the Creative Commons Attribution License (CC BY). The use, distribution or reproduction in other forums is permitted, provided the original author(s) and the copyright owner(s) are credited and that the original publication in this journal is cited, in accordance with accepted academic practice. No use, distribution or reproduction is permitted which does not comply with these terms.
*Correspondence: Yong Liu, bGl1eW9uZ0BzY3NmcmkuYWMuY24=; Chunhou Li, c2NzbGNoQHZpcC4xNjMuY29t
Disclaimer: All claims expressed in this article are solely those of the authors and do not necessarily represent those of their affiliated organizations, or those of the publisher, the editors and the reviewers. Any product that may be evaluated in this article or claim that may be made by its manufacturer is not guaranteed or endorsed by the publisher.
Research integrity at Frontiers
Learn more about the work of our research integrity team to safeguard the quality of each article we publish.