- 1Crustacean Culture Division, Indian Council of Agricultural Research-Central Institute of Brackishwater Aquaculture, Chennai, India
- 2Crustacean Culture Division, Kakdwip Research Centre, Indian Council of Agricultural Research-Central Institute of Brackishwater Aquaculture Kakdwip, Kakdwip, India
- 3Aquatic Animal Health and Environment Division, Indian Council of Agricultural Research-Central Institute of Brackishwater Aquaculture, Chennai, India
- 4Crustacean Culture Division, Navsari Research Centre of Indian Council of Agricultural Research-Central Institute of Brackishwater Aquaculture, Navsari, India
- 5Nutrition Genetics and Biotechnology Division, Indian Council of Agricultural Research-Central Institute of Brackishwater Aquaculture, Chennai, India
Closing the complex life cycle of closed thelycum shrimp in captivity is one of the fundamental challenges in breeding programs. In the present study, we investigated the sexual maturity, broodstock development, and spawning performance of two generations of captive-reared Indian white shrimp, Penaeus indicus, over 36 months originated from a single stock of wild brooders. The post larvae (GN-1) produced from wild P. indicus (G0) and P.L. (GN-2) produced from captive-reared (GN-1) broodstocks were nursery (1000 PL m-3) and grow-out (12 shrimp m-2) reared, and subsequently raised in broodstock ponds (1 shrimp m-2). The annual salinity and photoperiod in broodstock ponds varied between 20 to 36 ppt, and 11.2 -12.5 L and 11.5-12.8 D hours, respectively. The light intensity varied between 91 ± 6 lux at dusk to 75,358 ± 1719 lux at noon. The size at first impregnation or mating was 16.45 ± 1.7 g (132 DOC) and 17.62 ± 1.9 g (90 DOC), respectively, in GN-1 and GN-2 females. By 220 DOC, 25% of the GN-1 females initiated gonad development, whereas 55% of the GN-2 females recorded developing ovaries or were in stage II at 150 DOC. The broodstock attained an average final body weight of 38.85 ± 1.5 g (GN-1) and 42.65 ± 1.8 g (GN-2) by 360 DOC. The highest (p<0.01) eggs per gram body weight (5137 ± 303 eggs g-1) and hatchability, H (83 ± 0.7%), was recorded in wild broodstocks (G0) followed by GN-2 (1,715 ± 162 eggs g-1; H: 69 ± 2%) and GN-1 (1,476 ± 151 eggs g-1; H: 75 ± 1%). However, captive-reared broodstock had better survival (89-92%) than wild broodstocks (71 ± 0.8%). Further, indoor maturation trial (21 days) using ablated broodstocks (GN-1) revealed 79% of the impregnated broodstocks undergo molting, resulting in the loss of sperm pack and subsequent reduction in mating efficacy to 29%. The average sperm count and percentage of normal sperm also recorded (p<0.05) reduction during the maturation cycle. The data generated in the present investigation can form the baseline information for developing the breeding strategy for the genetic improvement of Indian white shrimp in India.
Introduction
Penaeid shrimp continues to be one of the most traded seafood commodities, with an annual global production of 6.5 million tons (FAO, 2020). Being one of the global leaders in shrimp production, India contributes 0.84 million tons worth USD $ 5.8 billion to global shrimp export market1. Indian shrimp farming was initially dominated by native penaeids such as black tiger shrimp, Penaeus monodon, and Indian white shrimp, P. indicus. However, wild broodstock-dependent shrimp farming was severely hampered by viral epidemics such as the white spot syndrome virus (WSSV), which attacked the global shrimp industry in the early 1990s (Chou et al., 1995; Li et al., 2016). As wild-caught brooders were the common sources of pathogen introduction to the farming system (Coman et al., 2006; Hoa et al., 2011; Debnath et al., 2015), a new blueprint for shrimp farming based on genetically improved broodstock became the prerequisite to overcome these constraints. Thus, the industry shifted towards developing and producing domesticated and specific pathogen-free (SPF) lines (Browdy, 1998). Thus, the Pacific white shrimp, Litopenaeus vannamei (hereafter referred as P. vannamei), emerged as a favorable species owing to its relative ease in captive breeding, ability to tolerate high stocking density, tolerance to wide salinity regime, and requirement of a less proteinaceous diet (Wyban, 2007). These favorable traits led to the development of genetically selected specific pathogen-free lines and subsequent introduction into Taiwan, followed by several Asian countries (Briggs et al., 2004; Wyban, 2007). In India, SPF P. vannamei was introduced in 2009, which gave fresh momentum to the shrimp industry and resulted in an exponential escalation of shrimp production over the past two decades. Nonetheless, single species-oriented culture started to raise challenges such as over-dependence on imported broodstocks and their escalating prices, inbreeding depression due to the large-scale use of farm-raised broodstocks, fluctuation in market demand, and emerging new pathogens, etc. (Alavandi et al., 2019; Kumaran et al., 2021). These underscore the need to widen the shrimp basket through species diversification and the development of strategic approaches to explore complementary native penaeids for sustainability (Vijayan, 2019).
The Indian white shrimp, P. indicus, is an important brackishwater farmed species in the traditional culture systems in India and was the focus of earlier research and developmental programs (Muthu et al., 1979). The first successful seed production was carried out in this species in the early 1980s using wild-sourced broodstocks (Muthu and Laxminarayana, 1979; Emmerson, 1980). Later, many researchers worked on the reproductive biology of P. indicus in India, Southeast Asian, and African countries (Primavera, 1985; Makinouchi & Primavera, 1987; Mohamed and Diwan, 1991; Mosha and Gallardo, 2013; Tomy et al., 2016; Saikrithi et al., 2019). Although P. indicus was dominant in Indian shrimp farming in the early 1990s, the species lost importance after the WSSV outbreaks and subsequent SPF P. vannamei introduction. Recently, as a part of the indigenous penaeid revival, the Indian white shrimp was selected as a national priority species due to its growth and reproductive potential (Anand et al., 2019; Vijayan, 2019; Anand et al, 2023). The successful nursery and field trials recorded average productivity of 3-7 tons per ha based on stocking density and geographical conditions (CIBA, 2019; Christina et al., 2020; Anand et al., 2021; Panigrahi et al, 2021). Although sustainable production of native penaeids is greatly desired, penaeid shrimp seed-producing hatcheries still depend on wild broodstocks for native shrimp seed production. The wild broodstock sourcing, apart from creating the risk of pathogen entry (Hoa et al., 2011; Debnath et al., 2014) and predictability issues (Crocos and Coman, 1997), impedes the opportunity for genetic improvement programs (Preston et al., 2010). Hence, optimization of captive breeding protocols and domestication are prerequisites for the initiation of any successful breeding program.
The fundamental challenge of any selective breeding program is closing the complex life cycle of candidate/target species (Browdy, 1992; Arnold et al., 2013). The success in selective breeding programs for P. vannamei across the globe has primarily been attributed to its captive breeding and domestication (Wyban, 2019). However, the selective breeding program of closed thelycum shrimps is comparatively slow in progress. Considerable research work has been carried out on the domestication and selective breeding of closed thelycum species such as P. monodon (Coman et al., 2005; Preston et al., 2010; Arnold et al., 2013; Coman et al., 2013; Coman et al., 2013; Norman-Lόpez et al., 2016); P. japonicus (Preston et al., 2004), P. esculentus (Keys and Crocos, 2006; Wen et al., 2015), P. paulensis (Peixoto et al., 2004; Peixoto et al., 2008) and P. merguiensis (Hoang et al., 2002). Nevertheless, the selective breeding programs in closed thelycum species still remain a harsh challenge (Wilder, 2019) due to inherent reproductive constraints such as poor mating efficiency with limited success in artificial insemination and slow gonad development in captivity. For example, though the Government of India has permitted the import of SPF P. monodon, there is a dearth of large-scale seed production due to limited mating success in captivity (Personal communication). As the reproductive performance of the shrimp varies among the species, it is important to have in-depth knowledge on the captive broodstock development and breeding cycle of the shrimp to start any genetic improvement programme. In this context, the Indian white shrimp, P. indicus, being a complementary native closed thelycum penaeid with a shorter time gap between the generations (Muthu et al., 1986) and easy-to-spawn species, remains a potential native penaeid for domestication. Further, the species is recently identified as a national priority species for diversification of Indian shrimp farming due to its high growth potential (Vijayan, 2019). Indian white shrimp, P. indicus has also been selected as a species of choice for genetic improvement in Egypt (Megahed et al., 2019) and a candidate species for farming in the Philippines due to its growth attributes (Santander-Avancena et al., 2021). Recently, Megahed et al. (2019) estimated the survival heritability of four generations of P. indicus. The authors reported that the effect of environmental interaction is higher on survival heritability. However, the information on the reproductive performance, such as spawning, gonad development and mating of the broodstock in captivity over the time period was lacking. In another reproductive study, Santander-Avancena et al. (2022) reported that the mating efficacy of P. indicus significantly improved by monosex rearing (80%) compared to only 20% in the communal rearing group where a size group of 5-7 g P. indicus were only used. However, to the best of our knowledge, earlier studies have not explored the potential and constraints in the reproductive performance of the Indian white shrimp in captivity in detail. A lack of domesticated stocks of P. indicus challenges the genetic improvement of the species in India. Hence, a deeper understanding on the reproductive performance of captive-reared broodstock in relation to Indian tropical conditions is pertinent. Against this background, the current study investigates the reproductive performance of a single stock of P. indicus, which was reared under captivity over two generations. Further, mating efficiency, moulting interval, captive-induced reproductive dysfunctions, the role of environmental cues (photoperiod, salinity, and light intensity), etc., were also studied in detail. The spawning performance of the wild broodstock of P. indicus was also compared with captive-reared lines.
Materials and methods
Experimental animals
The captive-reared broodstocks (GN-1 and GN-2) used in the present trial originated from a single stock of wild P. indicus brooders (n=75) shore seined at 30-90 m depth off the coasts of Chennai, Tamil Nadu (13°02′N and 80°10′E; 12° 46’ N and 80° 18’ E) during 2018. The present investigation was grouped into two trials, Trial I and II. In the first part of the investigation (Trial I), primary sexual maturity, broodstock development, and reproductive performance of captive-reared P. indicus (GN-1 and GN-2) were compared in relation to existing tropical environmental conditions. Further, the spawning performance of the captive-reared broodstocks was compared with wild P. indicus brooders. In the second part of the investigation (Trial II), moulting interval, mating frequency, spermatophore quality, and oocyte development of captive-reared broodstocks subjected to eyestalk ablation were investigated in indoor maturation units.
TRIAL I
Captive broodstock development
The wild brooders were individually stocked in FRP tanks (100 L) in the primary quarantine unit after temperature and salinity acclimation at Muttukadu Experimental Station (MES) of ICAR-CIBA, Muttukadu, Tamil Nadu, India. The brooders (n=75) were screened for WSSV and other OIE-listed pathogens (Kimura et al., 1996). The healthy and disease-free gravid broodstocks, G0 (n=45), were allowed to spawn at MES Shrimp hatchery, Muttukadu. The broodstock management and larval rearing of P. indicus were carried out as per Anand et al. (2019). The post larvae produced from the wild gravid females (G0) were termed first-generation (GN-1), and the subsequent generation of the post larvae produced from the domesticated parents are called GN-2 and GN-3, respectively. The schematic diagram in Figure 1 depicts the summary of the broodstock generations under study I. The terminology G0, GN-1, GN-2, and GN-3 for generations were used in the present study as per Keys and Crocos (2006). A similar seed production strategy was used for estimating the spawning performance of G0, GN-1, and GN-2 lines.
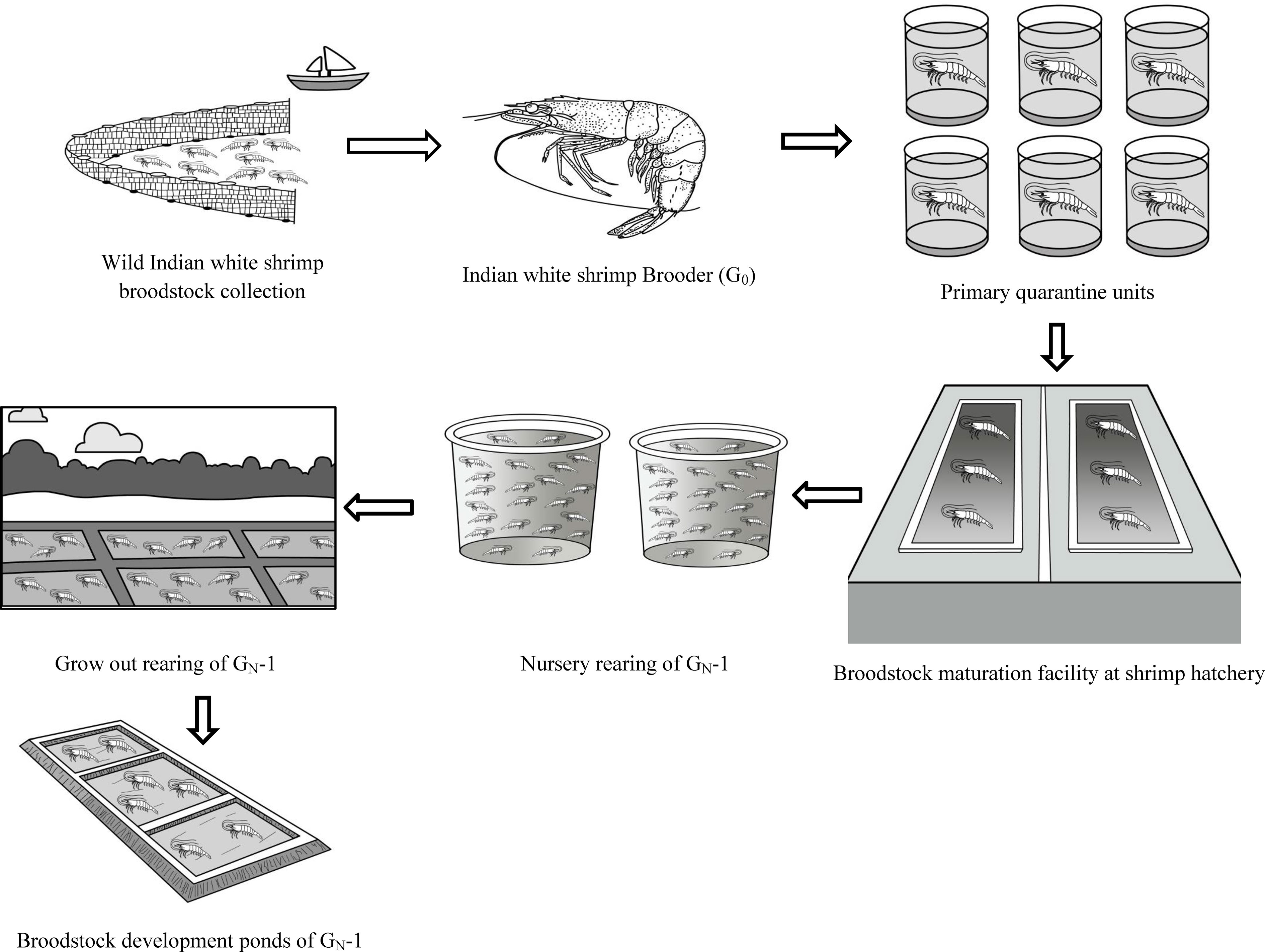
Figure 1 Schematic diagram of Trial I depicting the wild P. indicus broodstock (G0) sourcing to initiate captive broodstock development program during 2018. The Post larvae (GN-1) raised from the G0 stocks were nursery and grow out reared and subsequently raised in broodstock ponds. The matured broodstocks were spawned at the maturation facility at Muttukadu Experimental Station (MES) of ICAR-CIBA, Muttukadu, Tamil Nadu, India, and the life cycle was closed in captivity in 2019. The same procedure was followed for second-generation broodstock (GN-2) captive breeding in the year 2020.
The first (GN-1) and second (GN-2) generations were nursery reared for a period of 45 days in a mixotrophic nursery-rearing system at 1000 number m-3 in FRP tanks (5 ton). A mixotrophic system was developed using autotrophic microalgae (Thalassiosira sp. at 40,000 cells mL-1) and a heterotrophic-based biofloc system (Anand et al., 2023). The fermented carbohydrate was applied at the rate of 3-5 ppm twice a week to develop microbial floc in the nursery rearing system. The nursery-reared (45 DOC) first and second generations (GN-1 and GN-2) of P. indicus were stocked in grow-out ponds (500 m2) at 12 shrimp m-2 for a period of 4 months. Subsequently, the shrimps were partially harvested and restocked in broodstock ponds (300 m2) at 1 shrimp m-2. The parameters indicating primary sexual maturity, percentage of the mated or impregnated females, size at first impregnation, onset of gonad development, and size at male maturity were recorded during the broodstock rearing period at monthly intervals. Primary male sexual maturity and impregnated females were defined as the possession of spermatophores in male and inseminated spermatophores in thelycum of female shrimp, respectively. The length (total length/carapace length) and weight analysis were carried out to find the regression relationship among different life stages (nursery, grow out, and broodstock) of Indian white shrimp. No quantitative genetic selection procedure was carried out during the entire study period as the main objective of the study was to delineate the challenges and potential during the optimization of reproductive performance and breeding protocol for captive broodstock development in relation to existing tropical conditions in India. The entire broodstock, grow-out, and nursery system were based on zero water exchange systems.
Feed management
The shrimps were fed with experimental pellet feed having 36.65% crude protein during the nursery and grow-out period. The feed ingredient and proximate composition of the experimental feed are given in Table 1. The ablated broodstocks reared in the indoor maturation facility were fed with SPF polychaete (Topsy Bait, Netherlands) and maturation pellet feed (INVE Ltd, Thailand) at 1:1 ratio for six times a day at four hourly intervals. The fresh feeds were given at 15-20% of body weight, and pellet feed was given at 3% of body weight.
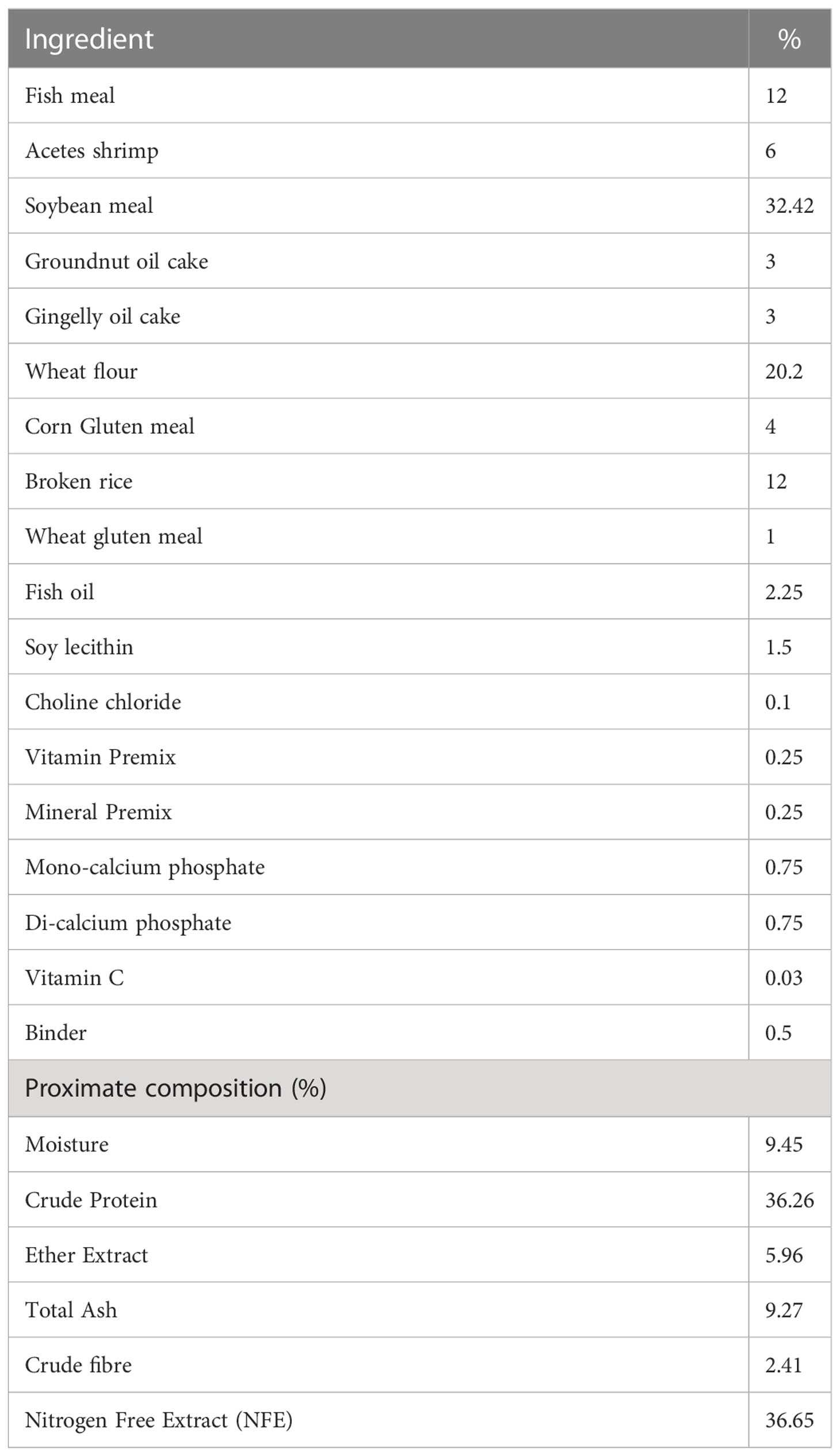
Table 1 Ingredient and proximate composition of the experimental feed provided during nursery and grow-out period of Indian white shrimp in captivity.
Length-weight relationship of captive-reared P. indicus at different life stages
To estimate the length-weight relationship and condition factor of different growth stages of male and female shrimp, individual total length, weight and carapace length were measured. Total length is measured between the tip of the rostrum to the tip of the telson, whereas postorbital notch to posterior carapace margin is measured as carapace length (CL). The stages between the initial and final length or final weight data for each phase are calculated to find a regression relationship among different growth stages. The regression analysis among body weight (B.W.) and total length (T.L.) or carapace length (CL) during different life stages was carried out using the power regression model, W=aLb, where W indicates body weight (g) and L indicates the total length or carapace length (cm) of the shrimp.
The exponent b was used to calculate the deviation from the isometry (b=3) or allometry (< or > 3). The fitness of the linear regression equations was determined based on the coefficient of determination (R2). A relative condition factor, Kn was calculated based on the ratio of body weight to length when the regression coefficient, b value is not equal to 3 or follows negative allometric growth as per the earlier methods (Emmerson, 1980; Palacios et al, 1999a; Arcos et al., 2003) based on the formula; Kn=W/aLb, where W and L are the body weight (BW) and total length (TL) respectively for each growth stage of the shrimp. The a is intercept and b is the slope of the regression line generated during the length-weight analysis of each growth stage. A Fulton’s condition factor (K) was calculated in accordance with Lalrinsanga et al. (2012) as per the formula, K=W/L3×100 when shrimps had the regression coefficient, b value 3 or closer.
Reproductive performance of wild and captive-reared broodstocks
The impregnated captive-reared (GN-1 and GN-2) and wild broodstocks (G0) with advanced stages of gonad development (n=45) were evaluated for reproductive performance parameters. The female and male broodstock were stocked at 6 shrimp m-2 in the indoor maturation tanks (5 ton) at 1:1 ratio. The female broodstock at intermoult/early premoult was unilaterally ablated and tagged. In the case of captive-reared stocks, first-time spawners which were not spawned already were used. However, in the case of wild brooders, as spawning history was not known, the first spawn was also considered from the day after eyestalk. The latency period in days was taken as the duration between the eyestalk and the development of gonad in broodstock (Palacios et al., 1999b). Spawning performance parameters such as latency period, fecundity, eggs per gram body weight, and spawning frequency were recorded. After spawning, fertilized eggs were collected in a 10 L bucket. Three 100 mL subsamples were randomly collected after thoroughly mixing to ensure eggs were homogenous within the water column for fecundity estimation. The collected eggs were subjected to treflan (0.01 ppm) and povidone-iodine (100 ppm) dip for 30-sec each and released into the hatching tank (500 L). The spawning was designated complete (spent) or partial (Stages I–III) based on the ovarian stage of the spawned brooder. The stages of the ovaries were examined visually through the dorsal side of the exoskeleton as per the methods described by Brown and Patlan (1974); Tan-Fermin and Pudadera (1989); Ceballos-Vázquez et al. (2003) and Ceballos-Vázquez et al., 2010. The spent spawners with transparent ovaries were recorded as completely spawned, while the spawners with part of their ovaries visible through the dorsal exoskeleton of the abdomen were recorded as partial spawners. Similar management procedures were followed during the reproductive performance evaluation in all three lines of broodstocks, G0, GN-1, and GN-2. The fecundity, eggs per gram body weight, percentage of fertilized eggs, and hatching rate were calculated as per the given formula below.
Fecundity = Total number of eggs released/number of brooders
Eggs per gram body weight = Total number of eggs released/body weight of the brooder (g)
Percentage of fertilized eggs = (Total number of fertilized eggs/total number of eggs) × 100
Hatching rate = (Total number of hatched nauplius/total number of eggs) × 100.
Evaluation of abiotic parameters
The physicochemical parameters in the broodstock ponds, indoor maturation tanks, and larval rearing units were monitored at regular intervals. The dissolved oxygen (D.O., mg L-1), temperature (°C), pH, and salinity (g L-1) were monitored through D.O. probe (YSI Environmental, USA), thermometer, pH meter (Horiba, Japan), and salinometer (Atago, Japan), respectively. The level of total ammonia-N (TAN), nitrite-N (NO2-N), nitrate-N (NO3-N), and phosphate-P (PO4-P) were measured spectrophotometrically, and alkalinity, total hardness, calcium level were measured by titrimetric methods as per APHA (2012). The light intensity at the water surface during the dawn, noon, and dusk period in the indoor maturation units and outdoor broodstock rearing ponds were recorded using lux meter (Lutron, India). The monthly natural photoperiod (day and night length cycle) during the captive broodstock development period was recorded from www.timeanddate.com.
Evaluation of microbial counts
Total bacterial count (TBC) and total Vibrio count (TVC) in broodstock ponds, larval rearing, and maturation units were determined as per the earlier described method (Kumar et al., 2019) with minor modifications. Briefly, the water samples were collected in a sterile bottle from the centre of the tank. The water (200 mL) was homogenized, and subsequently, 10-fold serial dilution was made in normal saline solution (NSS). A 0.1 mL of appropriate dilutions was applied in duplicate over the plates. Tryptone soy agar containing 1.0% w/v NaCl and thiosulfate citrate bile salts sucrose agar (HiMedia, Mumbai, India) were used for TBC, and TVC counts, respectively. Plates were incubated at 28°C for 24 h, and colonies in the range of 30–300 were counted and expressed as a bacterial colony-forming unit (cfu).
Principal component analysis
Principal component analysis (PCA) was carried out to find the association between the abiotic environmental parameter such as salinity, day length, night length, light intensity, and minimum and maximum temperature with gonad maturation and mating level of GN-1 and GN-2 populations. The first principal component is a linear combination of the environmental and reproductive parameters, which explains the possible variation. The second principal component explains as much of the remaining variation, and so on. The PCA analysis was carried out using Factoextra and FactoMineR packages in R4.0.2 (Kassambara and Mundt, 2017). Before analysis, all quantitative variables were auto-scaled to a unit variance allowing each variable to contribute equally to the PCA model. As the present data contained both categorical variable (month) and continuous quantitative variable (other parameters), hence factor analysis of mixed data (FAMD) function of FactoMiner package was used to compute the FAMD. Different function within Factoextra package was used to extract eigenvalues, contribution by individual parameter and visualization of the data. From the derived FAMD, the correlation circle was drawn for quantitative variables using fviz_famd_var function.
Trial II
The second part of the investigation, study II was conducted to evaluate moulting frequency, mating percentage, sperm quality analysis, and the histological architecture of ovarian developmental stages of captive-reared broodstock subsequent to eye stalk ablation for a period of 21 days maturation cycle in the indoor maturation units. The captive-reared broodstocks, GN-1 (28-35 g) of P. indicus, were stocked in the maturation units at 6 shrimp m-2. The broodstocks were fed with fresh and pellet feed as per the details given in Trial I.
Histological architecture of the ovary
The ovarian architecture of captive-reared broodstocks (GN-1) in trial 1 was studied using histological techniques. The different developmental stages of the ovary of the eyestalk ablated broodstocks (n=3) were selected as per earlier described methods (Brown and Patlan, 1974; Tan-Fermin and Pudadera, 1989; Ceballos-Vázquez et al., 2003), dissected out and preserved in 10% neutral buffered formalin for 24-48 h, followed by long-term preservation in 70% ethanol until further analysis. The histological procedures were conducted as per the standard procedure described by Bell and Lightner (1988). The tissues stored in 70% ethanol were subjected to dehydration in graded alcohol series (70%, 90%, and 100%) and clearing in xylene, followed by paraffin wax embedding in tissue embedder (Leica Biosystems, Germany). The tissue blocks were sectioned (4-5 µm) in microtome (Leica Biosystems, Germany) and further processed (deparaffinized and rehydrated) and stained with hematoxylin-eosin (HiMedia, India). The stained sections were mounted (DPX) and examined under a light microscope (Zeiss, Germany), and photographs were taken at 40 × magnification.
Evaluation of male reproductive performance
In indoor maturation units, the spermatophores of the intermoult male broodstock (moult stage C) were manually ejaculated to estimate the male reproductive performance parameters. The total sperm count and percentage of normal sperm were recorded before and end of the experimental trial. The cell density was recorded on a hemocytometer as per the methods described by Arnold et al. (2012). The number of normal sperm (spherical body with spike intact) and the number of abnormal sperm (malformed) were recorded.
Statistical analysis
The survival and hatching rate data are presented as percentages, and values were arcsine transformed prior to further statistical analyses. The water quality and microbial community parameters among the broodstock ponds, indoor maturation units, and larval rearing units were expressed as mean with standard error. One-way ANOVA was carried out to compare reproductive performance among G0, GN-1, and GN-2 broodstocks. All the analyses were carried out after examining the data for normality and homogeneity of variance, and the ANOVA was followed by Tukey’s post hoc means comparison. The level of statistical significance was set at P < 0.05. The type of spawning (completer or partial) among the broodstock was analyzed based on the chi-square analysis (X2). All the statistical analysis was performed using SPSS Version 20 (IBM SPSS Statistics for Windows, Version 20.0.). The correlation matrix was estimated using the corrplot, a multivariate correlation program to understand the correlation among fecundity, average body weight, eggs per gram body weight, total length, etc., using a statistical package in R4.0.2.
Results
Trial 1
During trial, I, broodstock development and closing of the life cycle of P. indicus were attempted in captivity, and the reproduction protocol was optimized. The nursery-reared GN-1 and GN-2 were subsequently stocked in grow-out and broodstock ponds. Three successive generations of P. indicus (GN-1 and GN-2, GN-3) were produced in captivity from a single stock of P. indicus.
Abiotic parameters during captive broodstock development
The physico-chemical parameters such as temperature, salinity, and photoperiod in the captive broodstock ponds were subjected to prevailing weather and seasonal fluctuation. The annual light intensity (lux), water temperature (minimum and maximum), salinity, and photoperiod (day and night length) during the captive broodstock development period is given in Figures 2A–D, respectively. The salinity and temperature in the broodstock ponds varied between 20-36 ppt and 25.7-30.8°C, respectively (Figure 2C). The day length or light cycle ranged between 11.90 to 12.09 h, with the highest day length recorded during May-August (correlated with a higher percentage of broodstock with advanced stage of gonad development), whereas the lowest day length (11.20 h) coincided with the winter period (Dec-Jan) (Figure 2). The light intensity at the water surface in the broodstock rearing ponds was subjected to the dawn and dusk effect, with the highest light intensity (75,358 ± 1719 lux) recorded at noon (12-2 PM), followed by dawn (556 ± 128) and dusk (91 ± 6 lux) (Figure 2). However, the light intensity in the indoor maturation units had a narrow range and varied between 36 ± 2.2 to 641 ± 167 lux, respectively (Figure 2).
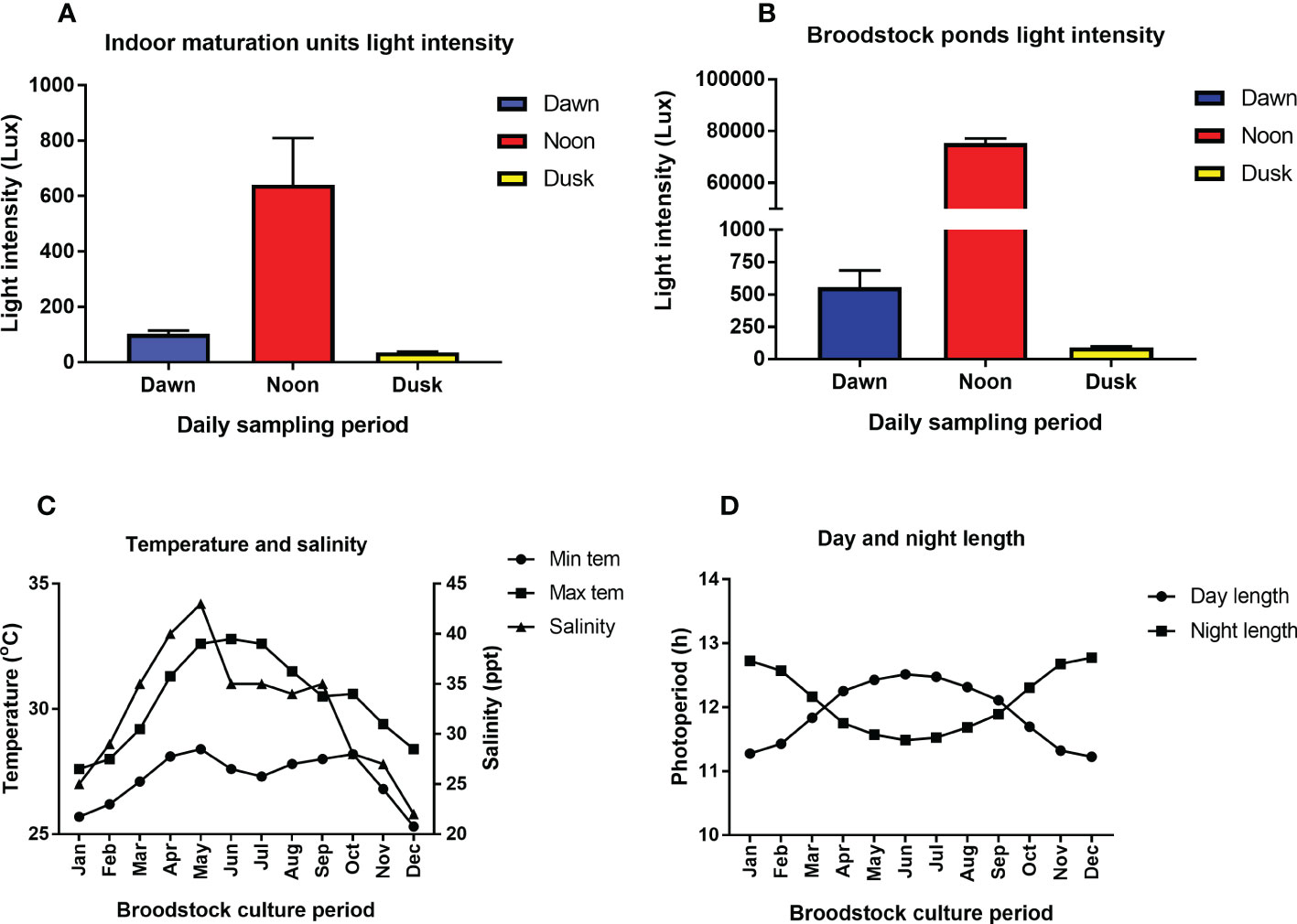
Figure 2 (A–D). The annual variation in environmental parameters such as light intensity (lux), water temperature (minimum and maximum), salinity, photoperiod (day and night length) during the captive broodstock development period of Penaeus indicus. (A): Indoor maturation units light intensity. (B): Broodstock ponds light intensity, (C): Temperature and salinity, (D): Day and night length.
Water quality parameters and microbial counts
The water quality parameters and microbial counts of the broodstock ponds, indoor maturation tanks, and larval rearing tanks are given in Table 2. The indoor larval rearing and maturation units had an average temperature of 31 ± 0.7 and 30 ± 0.2 °C, respectively. The average salinity also recorded a narrow fluctuation in the indoor maturation (34 ± 0.4 ppt) and larval rearing tanks (33 ± 0.3 ppt). The alkalinity and hardness in broodstock pond, maturation units, and larval rearing varied from 104 – 160 and 7043 – 7678 ppm, respectively. The total ammonia nitrogen (TAN) level was lowest in the broodstock ponds (0.19 ± 0.1 ppm) compared to maturation tanks (0.62 ± 0.1 ppm) and larval rearing tanks (0.27 ± 0.1 ppm). Similarly, the lowest nitrite-N was also recorded in broodstock ponds (0.03 ± 0.01 ppm). The microbial count analysis (Table 2) revealed the highest load in indoor maturation units (64 ± 37 × 105 cfu ml-1), followed by broodstock ponds (41 ± 14 × 105 cfu ml-1).
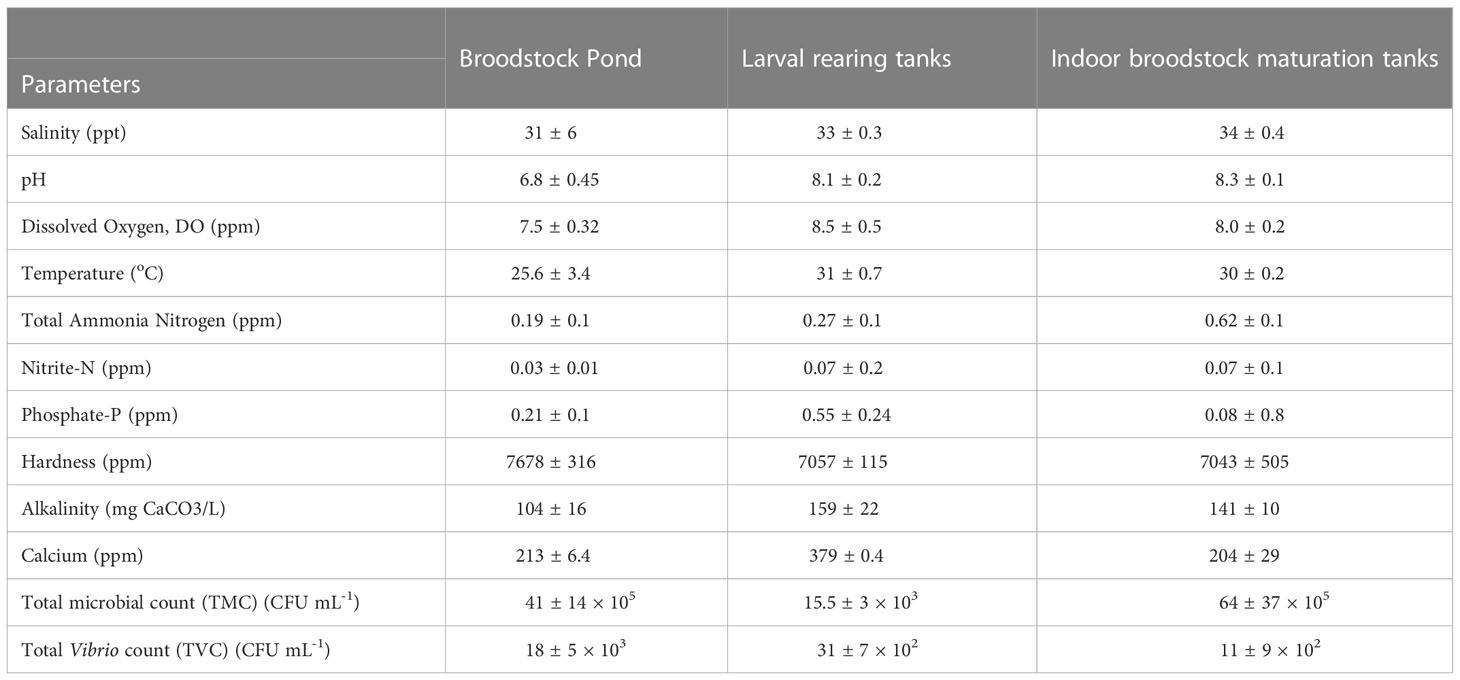
Table 2 Water quality and microbiological parameters (mean ±SE) in broodstock pond, larval rearing tanks, and indoor broodstock maturation tanks during the study period in Trial I.
Growth, length-weight relationship, and condition factor of captive-reared P. indicus at different life stages
The wild female and male broodstock had a size range of 14.5 to 90.0 g (11.9 – 23.4 cm) and 22.9 – 53.7 g (14.3 – 20.3 cm), respectively. The captive-reared male and female broodstocks ranged from 19.0 – 47.7 g and 16.0 – 36.6 g, respectively (Supplementary Table 1). The regression relationship between the body weight (BW) and total length (TL), and the relative condition factor of the different age groups of captive-reared and wild broodstock is given in Figures 3A–C. The length-weight analysis revealed that nursery-reared shrimp recorded positive allometric growth with the slope of the regression, b value close to 3 or above in both GN-1 and GN-2 populations. However, the shrimps in grow out and broodstock-rearing phases had negative allometric growth (b = 2.3 to 2.84), indicate as the shrimp growth advances towards maturity, the body weight gain is not proportional to the total length of the shrimp. As grow-out and broodstock rearing stages recorded negative allometric growth patterns, the relative condition factor (Kn) was calculated, which ranged from (1.00-1.04), indicating shrimps were in better rearing condition throughout the culture period. During the nursery stages, the shrimps recorded towards positive allometry with b value closer to 3 or slightly above indicated an increase in length was directly proportional to weight gain or shrimp grew faster in length than weight. As b value was closer to 3, Fulton condition factor (K) was calculated for nursery-reared shrimps, which ranged from 0.65-0.67 (Figures 3A, B). The sex-wise variation in length-weight analysis and condition factor during the grow-out and broodstock rearing period is given in Supplementary Table 1. The carapace length varied from 2.9-4.6 cm in wild female broodstock (G0), while in captive broodstock, it varied from 2.6-4.1 cm in GN-1 and 2.9-4.5 cm in GN-2 female broodstocks (Supplementary Table 2). The regression analysis among carapace length and body weight recorded a high negative allometric relationship with the slope of the regression or b values ranging from 1.20 to 2.
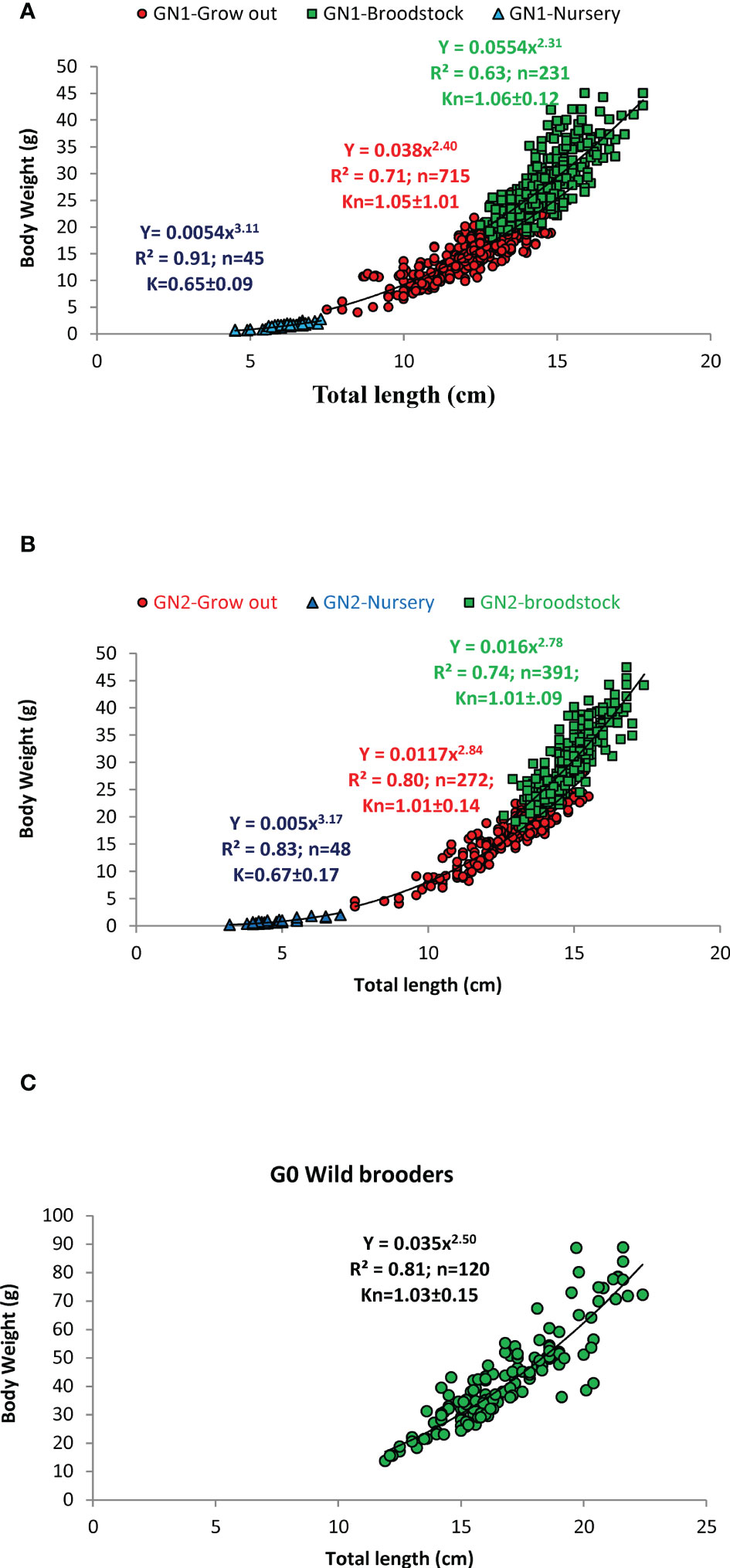
Figure 3 (A–C). The regression relationship among the body weight and total length (TL) at different growth stages of Indian white shrimp Penaeus indicus during nursery, grow-out, and broodstock rearing period of the captive-reared broodstocks. (A): GN-1 first-generation (B): GN-2: second generation, (C): G0: wild broodstock; Kn: relative condition factor, K: Fulton condition factor; n: sample size; R2: coefficient of determination.
The nursery-reared GN-1 and GN-2 shrimps attained an average final body weight of 0.55 ± 0.2 and 0.56 ± 0.2 g at the end of 45 days of nursery rearing with no difference in growth (p>0.05) among them. The average monthly growth rate of captive-reared P. indicus during the grow-out and broodstock-rearing period is given in Figure 4. The weight gain of the shrimp was not proportional to age and varied with different growth phases. At the end of 360 DOC, females and males of GN-2 in the broodstock ponds attained an average body weight of 42.7 ± 1.8 g and 34.3 ± 1.8 g, respectively, which were 9.8 and 7.0% non-significantly higher (p>0.05) compared to GN-1 (F: 38.9 ± 1.5 g; M: 32.0 ± 1.2 g). The growth performance of females was invariably higher compared to males in both generations.
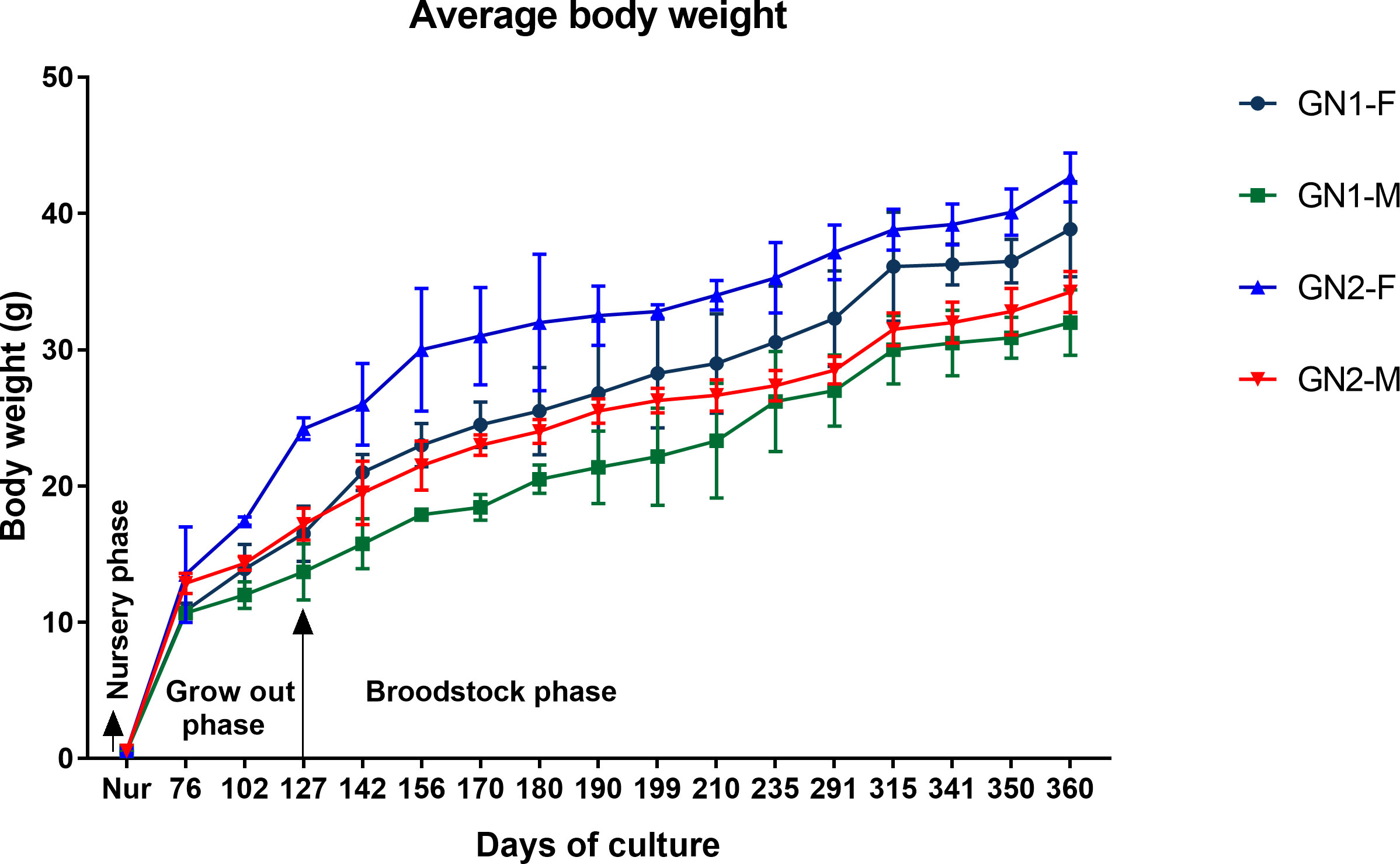
Figure 4 Average growth rate (mean ± SD) of captive-reared male and female GN-1 and GN-2 generations of Penaeus indicus during nursery, grow out, and broodstock rearing period.
Evaluation of mating and gonad development in captive-reared broodstocks
The percentage of impregnation and onset of gonad development in captive-reared broodstocks (GN-1 and GN-2) over a time period is given in Figures 5A, B. The captive-reared females, GN-1 attained size at first impregnation or mating at 16.5 ± 1.7 g (Age: 130 DOC), and 80% of the males reached primary sexual maturity (presence of whitish spermatophore mass) at 12.5 ± 1.2 g onwards. The captive-reared GN-2 started impregnated at 17.6 ± 1.9 g (Age: 100 DOC), and 80% of the male had milky whitish spermatophores. By the age of 150 DOC, 55% of the GN-2 females recorded the onset of gonad development compared to 25% of gonad development observed in GN-1 females at 220 DOC. The onset of gonad development was visually recorded through the dorsal part of the exoskeleton examination. The female shrimps with pale greenish ovaries visible on the cephalothoracic region of the shrimp (ovary with stage II) were grouped as brooders with developing ovaries or stage II brooders. In GN-1 females, the shrimps with developing ovaries (stage II) had a size range of 22-35 g with an average broodstock size of 29.67 ± 4.21 g (Figure 5), and 22 g as the lowest-sized shrimp. Similarly, at 150 DOC, the group of GN-2 females with developing ovaries had an average size of 30.2± 4.5 g (Figure 5) with a size range of 24.3-36.0 g. The smallest size of female shrimp with developing ovaries had a size of 24.3 g. By the age of 9 – 10 months, 70% of the captive-reared females (GN-2) recorded advanced gonad development (2 or 3rd stage) in the captive system.
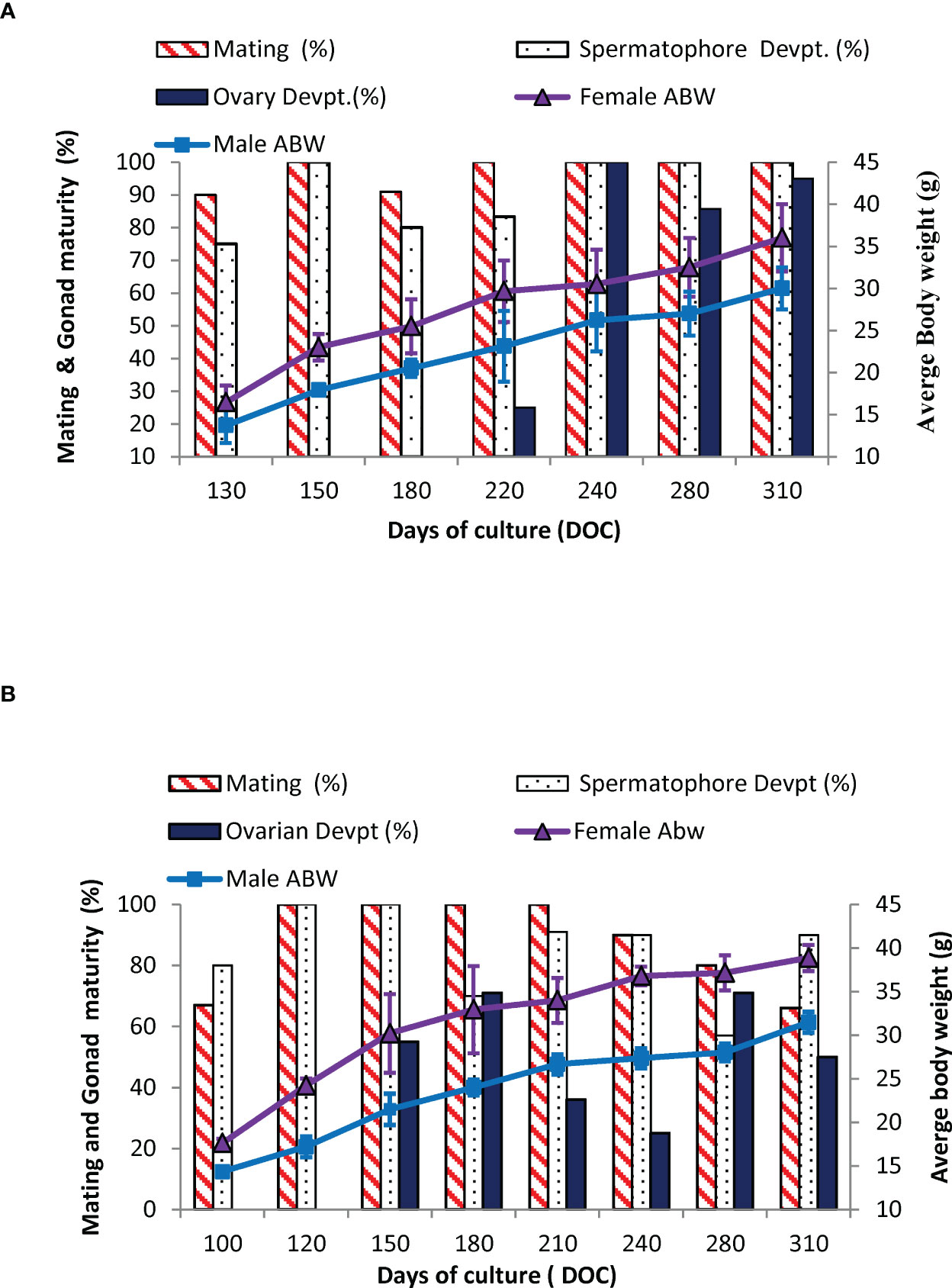
Figure 5 (A, B). Percentage of mating, gonad development (ovary and spermatophore), and average body weight, ABW (g) in captive-reared male and female Penaeus indicus during the broodstock development period. (A): GN-1 and (B): GN-2.
Principal component analysis of abiotic and reproductive parameters
To understand the interaction effect between the monthly variation in environmental factors and reproductive parameters such as mating and gonad development of captive-reared broodstocks, the principal component analysis was carried out. The analysis suggests that reproductive parameters such as mating, spermatophore, and ovary development, along with environmental parameters like months, maximum and minimum temperature, day-night cycle, salinity, and light intensity at noon and dusk were the major contributor to the first principal component and accounted for 40.2% variances (Figure 6C). The day-length period had a positive influence on the mating of the shrimp and gonad development (Figure 6). Further, the result suggests that the effect of environmental conditions was more pronounced on mating and spermatophore development than ovarian development. Among the light intensity measured at different time points, the light intensity at dusk had the maximum negative influence on the reproductive development of the shrimp. Annual temperature and salinity also tend to have a strong influence on the reproductive performance of the shrimp, as both these parameters follow the same directions. The second principal component was mainly contributed by month and light intensity at dawn (Figure 6).
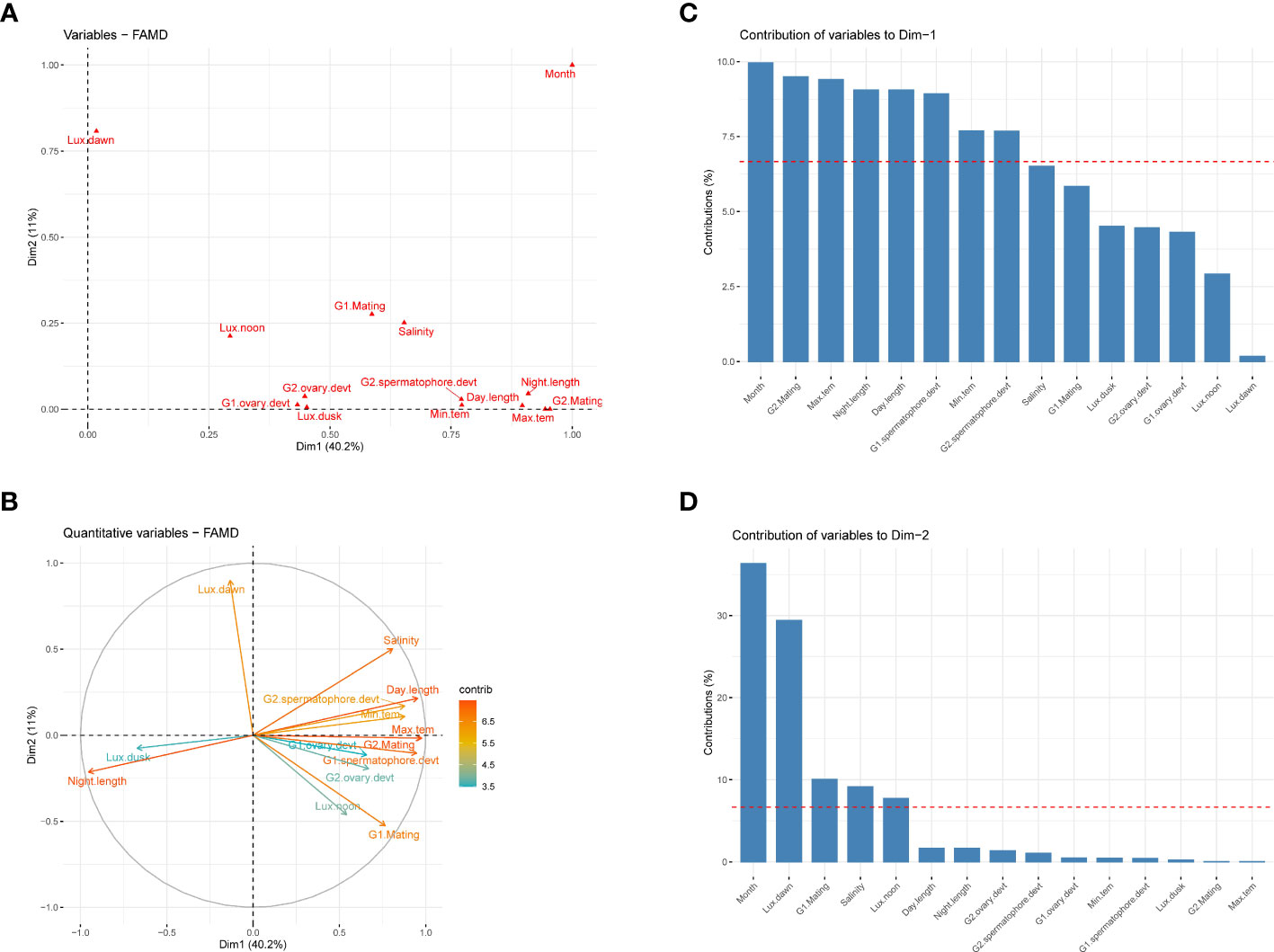
Figure 6 Principal component analysis of environmental and reproductive parameters of captive-reared Penaeus indicus. (A): The PCA of quantitative and qualitative variables by Factor Analysis of Mixed Data (FAMD), (B): Correlation circle of quantitative variables, (C): Relative contribution of variables in first dimension, (D): Relative contribution of variables in second dimension.
Spawning performance of captive-reared vs wild broodstocks of P. indicus
The spawning population of wild broodstock (G0) had a wide size range from 25 g to 90 g, whereas captive-reared broodstock size ranged from 25 to 45 g. The percentage frequency distribution of the spawners (G0, GN-1, and GN-2) size groups (g) contributed to successful spawning depicted in Figures 7A, B. Among the wild spawners (G0), 35 – 40 g size groups contributed to maximum (21.6%) spawning, followed by 40 – 45 g (17.1%) size groups, while the maximum size group contributed by captive-reared spawners were 30 – 35 g (32.0%) in GN-1 groups and 35 – 40 g (53.8%) in GN-2 groups. Partial spawning or complete spawning was estimated visually based on the ovarian colour through the dorsal exoskeleton after spawning. The type of spawning (complete or partial) among the broodstocks was calculated based on the chi-square analysis (X2) is given in Figure 8 While 80.0% of the spawning by the wild broodstock G0 was complete, the percentage of partial spawning was higher in GN-1 (62.9%) and GN-2 (53.8%), and only 37.2 and 46.2% of GN-1 and GN-2 broodstocks, respectively recorded complete spawning.
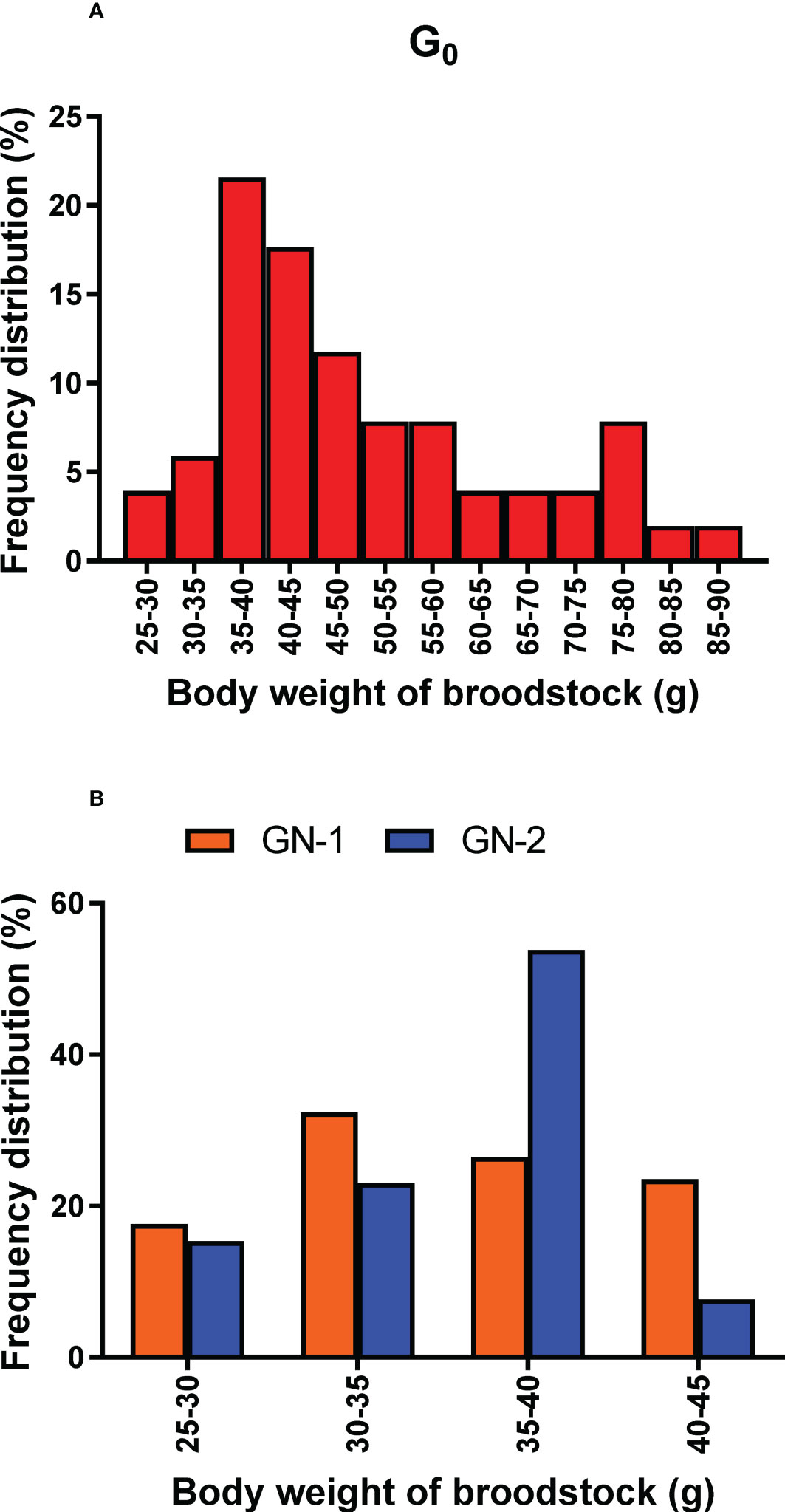
Figure 7 (A, B). Histogram depicts frequency distribution percentage of different size groups (g) of Penaeus indicus broodstocks contributed to successful spawning. (A): wild broodstock, G0. (B): First (GN-1) and second-generation (GN-2) captive-reared broodstocks.
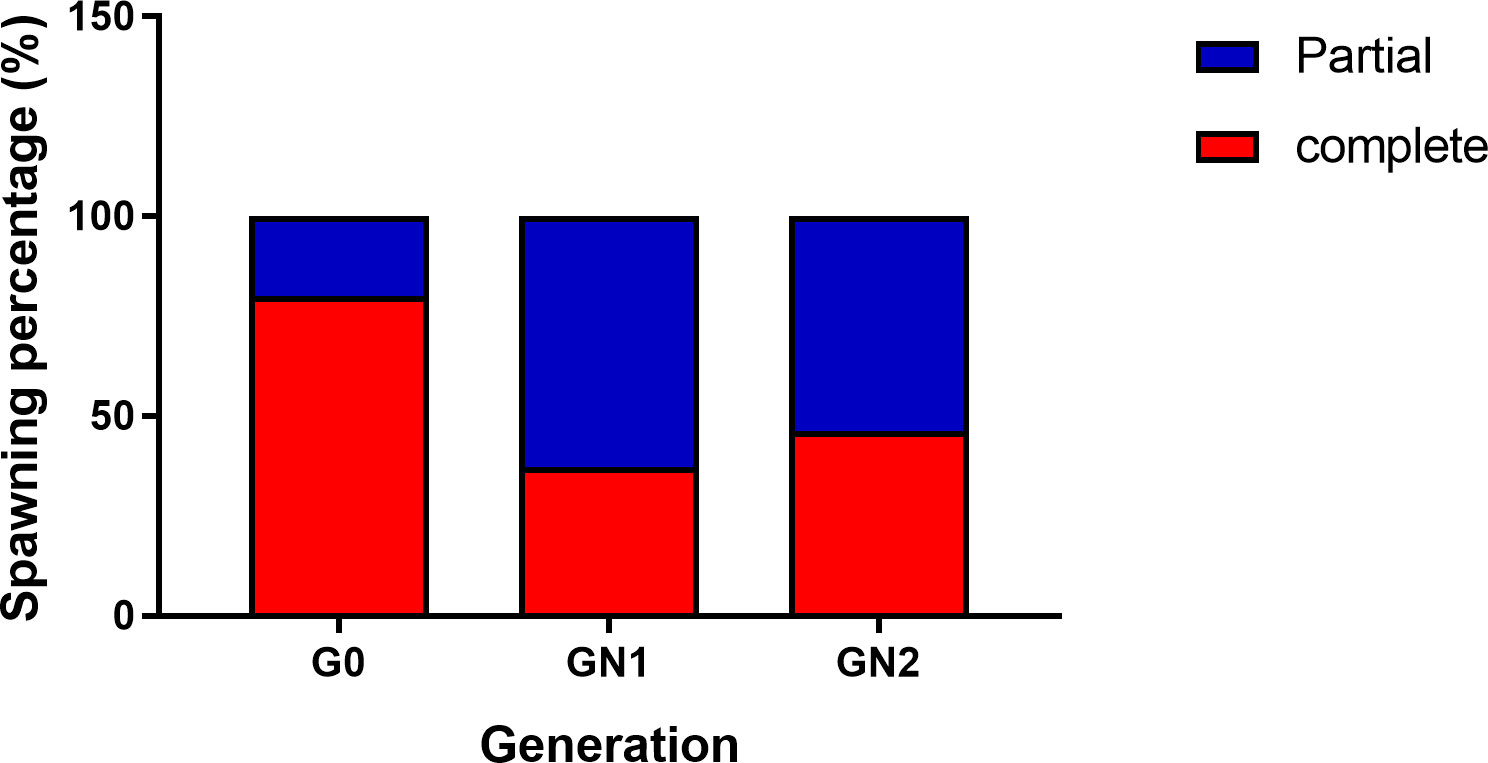
Figure 8 The type of spawning (complete or partial) among Penaeus indicus broodstock, G0 (wild), GN-1 (First generation), GN-2 (Second generation) based on the chi-square analysis (X2).
The spawning performance of wild (G0) and captive-reared broodstock (GN-1 and GN-2) is presented in Table 3. There was a significant difference (p<0.01) in fecundity among the broodstocks, with the highest fecundity recorded in wild broodstock, G0 (2,25,110 ± 11,173 eggs), followed by captive-reared broodstocks, GN-2 (59,923 ± 6,512 eggs) and GN-1 (52,612 ± 5,874 eggs). Similarly, the wild broodstock also had the highest (p<0.01) eggs per gram body weight (5,137 ± 303 eggs g-1) compared to captive-reared broodstocks, GN-2 (1,715 ± 162 eggs g-1) and GN-1 (1,476 ± 151 eggs g-1). Among the captive-reared broodstocks, 2nd generation lines (GN-2) had 16.2 and 13.9% higher (p>0.05) eggs per gram body weight and fecundity compared to GN-1. Based on the egg hatchability or fertilization rate, the spawners were divided into two groups; Group I, with above 60% egg hatchability, and Group II, with a reproductive cycle below 60% egg hatchability. In the first group (Group I), G0 recorded the highest (p<0.01) hatchability (83 ± 0.7%), followed by GN-2 (77 ± 2%) and GN-1 (75 ± 1%) groups. In the second group (Group II), 18.3 ± 2.9, 16.5 ± 2.5, and 6.3 ± 1.3% spawn of GN-1 GN-2 and G0, respectively, recorded unfertilized eggs or poor egg hatchability. Nevertheless, the captive-reared GN-2 had the highest contribution of ablated broodstock to successful spawning (69%), followed by GN-1 (47%). In contrast, only 18% of ablated wild broodstocks contributed to successful spawning, as the majority of the wild broodstock spawning was contributed from unablated wild gravid spawners. Similarly, after eyestalk ablation, captive-reared broodstocks recorded higher survival (89-92%) compared with wild broodstock (71 ± 0.8%).
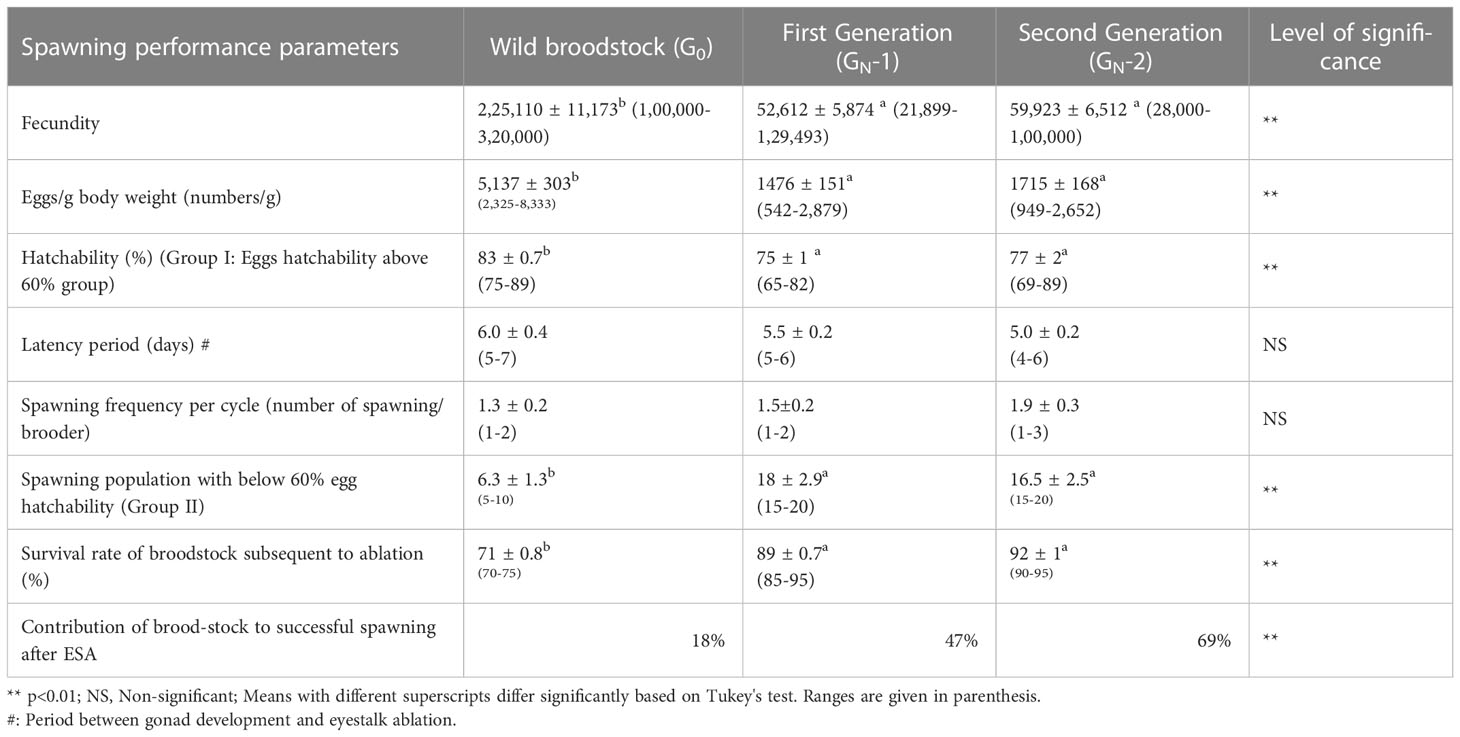
Table 3 The average (mean ± SE) and range of spawning performance parameters of wild (G0) and captive-reared Penaeus indicus first (GN-1) and second-generation (GN-2) broodstocks are based on one way ANOVA.
The correlation matrix depicts the relationship between fecundity and spawner’s body weight, carapace length, eggs per gram body weight, and total nauplius counts are given in Supplementary Figures 1A–C. The fecundity had a significant positive (p<0.05) correlation with eggs per gram body weight in broodstocks G0 (0.48, p<0.05), GN-1 (0.94, p<0.05) and GN-2 (0.89; p<0.01) lines. Similarly, the egg hatchability (GN-1: 0.85, p<0.05 and GN-2: 0.86, p<0.05) and total nauplius count (G0: 0.64, p<0.05; GN-1: 1, p<0.05 and GN-2: 1, p<0.05) were also positively correlated with the fecundity of broodstocks. However, wild broodstock fecundity did not have any positive correlation with egg hatchability. Likewise, although non-significant (p>0.05), the body weight of all three lines of spawners (G0, GN-1, and GN-1) had a positive correlation with fecundity.
The average length of the different larval stages produced from wild and captive-reared broodstocks during the study cycle is given in Supplementary Figure 2. The fertilized eggs had an average size of 256 ± 3 µm, 259 ± 8, and 240 ± 13 µm, respectively, in GN-1, GN-2, and GN-3 lines, and nauplius stage 1 had a size of 347 – 402 µm length. The size of the zoea larval stages (Z1 to Z3) ranged from 840 – 2502 µm, and the mysis stages (M1 to M3) ranged from 2628 to 4300 µm. The average length of post-larvae 1 of GN-1, GN-2, and GN-3 lines were 4.5 ± 0.6, 4.8 ± 0.1, and 4.8 ± 0.2 mm, respectively.
Trial II
In trial II, a detailed investigation of the moulting frequency, mating percentage, male reproductive performance parameters, and histological architecture of different ovarian stages of captive-reared broodstock subjected to eyestalk ablation was evaluated in indoor maturation units for a period of 21 days. At the end of the reproductive performance trial, 79% of the impregnated broodstock recorded moulting subsequent to eyestalk ablation. This corresponds to spermatophore loss with a decrease in impregnated females and a subsequent reduction in mating efficacy to 29%. Similarly, 59% of the broodstock recorded gonad development, and 52% of the broodstock responded to spawning successfully (Figure 9). The daily percentage of spawning, mating, and moulting in indoor maturation units is given in Figure 9. The average daily moulting of the broodstock post-eyestalk ablation period varied from 11 to 22%. The highest spawning was recorded during 3rd and 12th day of the post-eyestalk ablation. The histological architecture of ovarian developmental stages of the ablated captive-reared broodstocks is given in Figure 10, and comparative gonad development of wild brooders, G0 is also provided (Figure 10) to have an understanding of the variation in ovarian stages among wild gravid and eyestalk ablated captive brooders. Gravid ovaries with heterogeneous distribution of cortical oocytes along with previtellogenic oocytes were noticed in ablated captive-reared brooders in the final stage of maturity, while in wild gravid ovaries, a homogenous distribution of cortical oocytes surrounded by oogonia cells was only noticed.
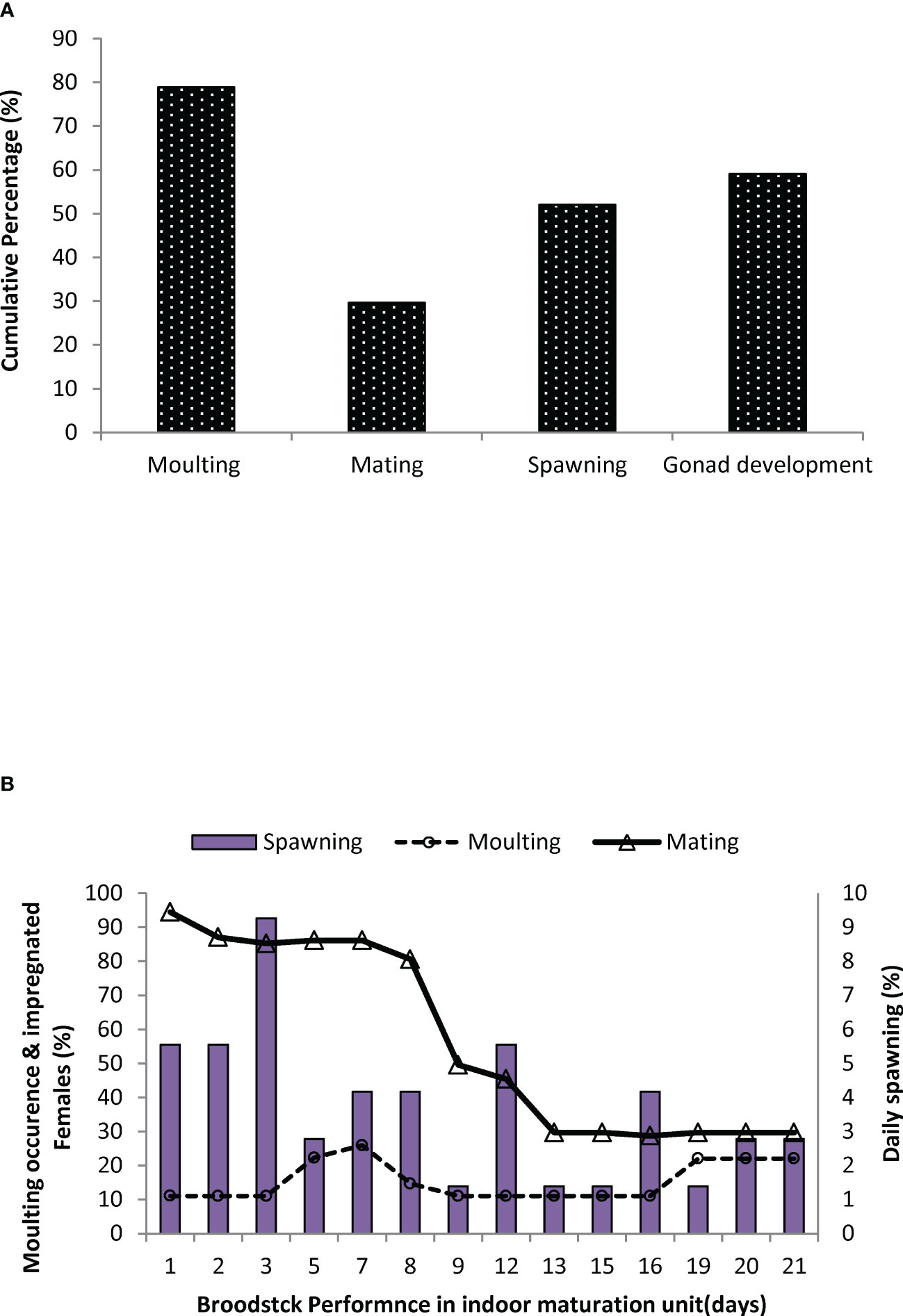
Figure 9 Reproductive performance of Penaeus indicus after eye-stalk ablation in indoor maturation cycle in Trial II. (A) Cumulative percentage of mating, moulting, spawning, and advanced gonad development in Penaeus indicus after eye-stalk ablation (B). Daily percentage of mating, moulting, and spawning of captive-reared Penaeus indicus broodstocks after eye-stalk ablation.
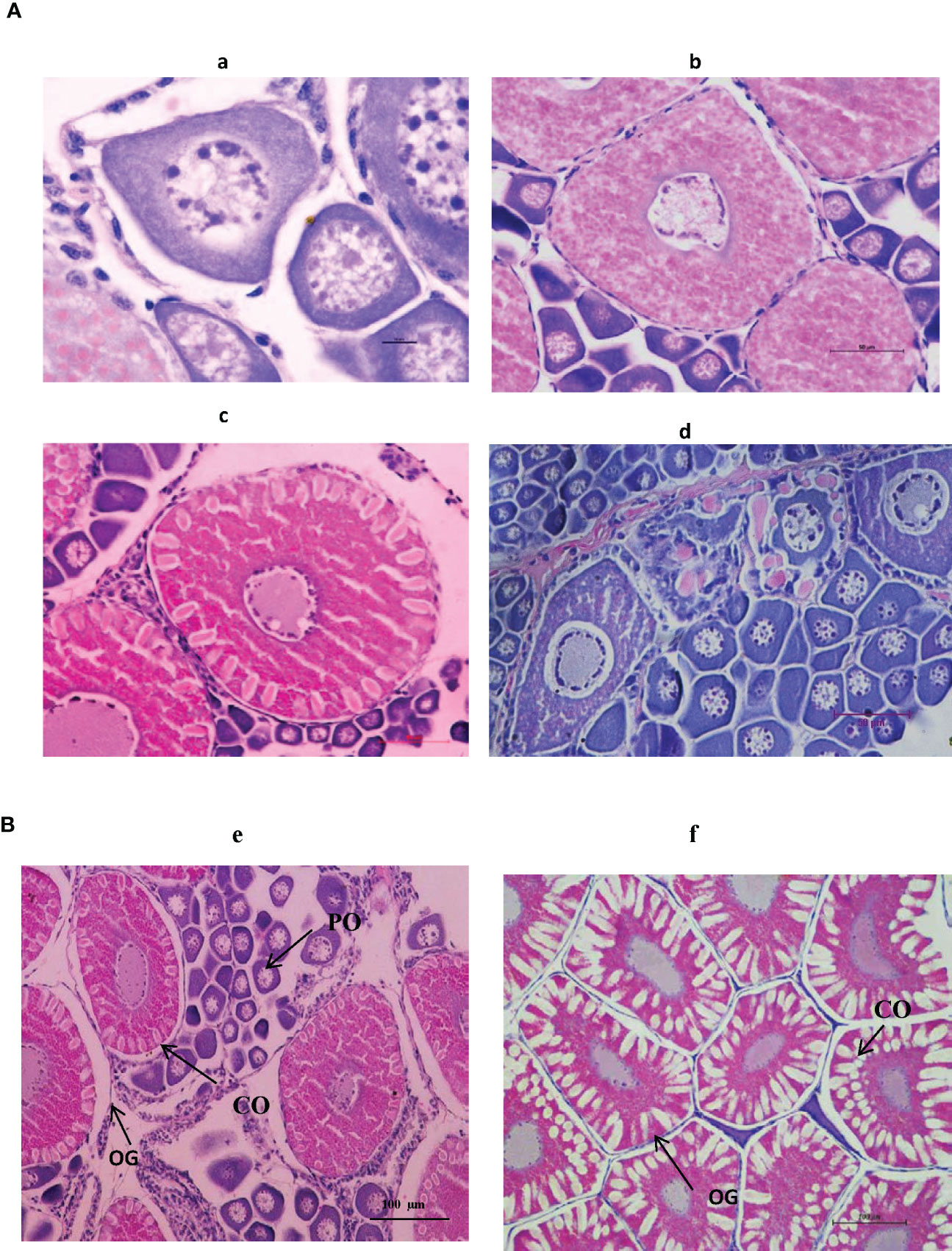
Figure 10 (A) The histological architecture of different ovarian stages of captive-reared Indian white shrimp Penaeus indicus. a). Previtellogenic oocyte (Scale bar: 100 × 10 × objective) b). Vitellogenic oocyte (Scale bar 40 × 10 × objective) c). Cortical oocyte (Scale bar 40 × 10 × objective) d). Spent/atretic oocyte (Scale bar 40 × 10 × objective) (B). The histological architecture of gravid ovarian stages of Indian white shrimp Penaeus indicus. e). Captive-reared broodstock f).Wild broodstock (Scale bar: 20 × 10 × objective) PO, Previtellogenic oocyte; CO, Cortical oocyte; OG, oogonial cells.
The total sperm count and percentage of normal and abnormal sperm of the captive-reared male broodstock before and after 21 days maturation cycle in the indoor maturation unit are given in Table 4. The male broodstock had an initial total sperm count of 4 ± 1 × 106 spermatophore ampoule-1, which reduced (p>0.05) to 3.5 ± 0.5 × 106 spermatophore ampoule-1 at the end of the trial. The percentage of the sperm with normal morphology was 83.9 ± 1.6% at the start of the experiment, which reduced to 79.6 ± 2.8% at the end of 21 days maturation cycle.
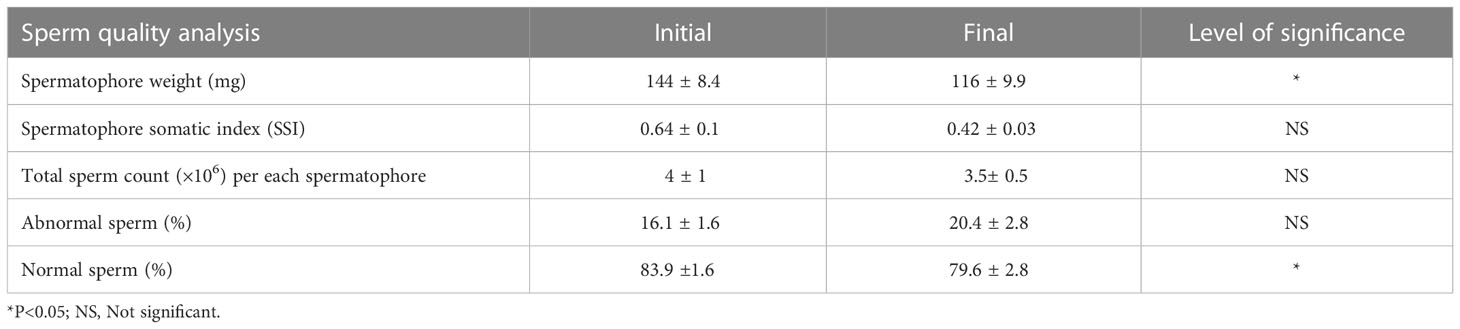
Table 4 Total sperm counts and percentage of normal and abnormal sperm (mean ± SE) in captive-reared male Penaeus indicus in the indoor maturation units before and after maturation cycle during trial II based on independent sample-T test.
Discussion
Closing the life cycle in captivity and domestication are the most critical prerequisites for the initiation of any genetic improvement programs. The present study discusses the reproductive potential and inherent reproductive constraints in the domestication of closed thelycum Indian white shrimp.
Reproductive performance
The reproductive performance of the shrimp can be evaluated through mating efficiency, spawning frequency, fecundity, hatching rate, etc. (Browdy, 1992; Wouters et al., 2001; Hoa et al., 2009; Coman et al., 2011; Goodall et al., 2016). The size of the individual spawner also plays a vital role in shrimp reproduction (Ibarra et al., 2007), as it is positively correlated with fecundity (Menasveta et al., 1994; Arcos et al., 2004; Debnath et al., 2014) and spawning frequencies (Andriantahina et al., 2012; Wen et al., 2015). In the present trial too, although non-significant, a positive correlation between fecundity and body weight was noticed among wild (G0) and captive-reared broodstocks (GN-1 and GN-2). In the present study, although the size ranges of the P. indicus broodstock were 25 – 90 g (wild) and 25 to 45 g (captive-reared) (Figure 7), the maximum spawning was contributed from 30 – 45 g size groups spawners. While the size-related maturity is species-specific, above 35 grams and below two years of age of Farfantepenaeus paulensis (Peixoto et al, 2005) and P. vannamei (Ren et al., 2020) are reported as ideal for seed production. Likewise, many commercial P. vannamei hatcheries prefer 30 – 45 g broodstock for nauplius production (Marsden et al., 2013; Wen et al., 2015; Ren et al., 2020). Ren et al. (2020) reported that females in different size classes employ different strategies when allocating energy to reproduction. The authors noted that medium-size group females generally invest more energy in reproduction than growth, resulting in a slower growth rate and multiple spawning than large brooders with a faster growth rate and less frequent spawning.
Hatchability is another important factor that limits the successful seed production of captive-reared shrimps. In the current study, wild broodstocks recorded higher hatchability (83 ± 1.7%) compared to captive-reared stocks GN-1 (75 ± 3%) and GN-2 (77 ± 3.7%) stocks. This matches with earlier reports where wild broodstocks were also reported to have better fecundity and hatchability compared to domesticated ones (Browdy et al., 1986; Keys & Crocos, 2006; Emerenciano et al., 2012; Arnold et al., 2013; Marsden et al., 2013; Wen et al., 2015). Likewise, Coman et al. (2006) reported below 40% and often below 10% hatching rate from domesticated P. monodon. Similar to this line, in the present trial, 16.5 to 18% of the captive-reared spawning population recorded hatchability below 60% compared to wild spawners (6 ± 1.2%). Further, the fecundity or eggs per gram body weight of captive-reared broodstocks (GN-1 and GN-2) were significantly lower (p<0.05) than the wild broodstocks (Table 3), which can be attributed to a higher percentage of partial spawning in captive-reared stocks (Figure 8) compared to wild gravid spawners. However, it is worth noting here that the reproductive performance of domesticated broodstocks increases over successive generations (Preston et al, 2010; Coman et al., 2013). For instance, the 5th generation line (G5) of P. monodon recorded an increase in spawning percentage and hatching rate from 52.0 to 77.1% and 21.5 to 31.6%, respectively, compared to G1 stocks (Coman et al., 2013). In the present study, too, GN-2 of P. indicus recorded 16.2 and 13.9% higher (P>0.05) eggs per gram body weight and fecundity, respectively, compared to first-generation (GN-1). Besides, Coman et al. (2013) reported significant improvements in nauplius count from the first two generations to the later generation of P. monodon (G5 and G8) stocks. As wild broodstock of P. indicus is reported to be a highly fecund animal with a fecundity up to 4,00,000 (Jayawardane et al., 2002; Anand et al., 2019), it is reasonable to assume that domestication and genetic improvement of the species through the selective breeding program can definitely improve the fecundity, breeding efficacy and reproductive size group of the captive-reared broodstock.
It is also important to note that, unlike open thelycum shrimp, closed thelycum shrimp possesses inherent reproductive constraints in captivity (Browdy et al., 1986; Hetzel et al., 2000; Coman et al., 2007; Wilder, 2019). Lack of proper mating in the confined indoor system ultimately results in poor fertilization rates. This is supported by the frequent moulting (79%) of broodstock subsequent to eyestalk ablation in the indoor maturation units (Trial II), which resulted in a loss of sperm pack resulting in poor impregnation percentage (29%). Further, a decline in total sperm count and an increase in the rate of abnormal sperm (Table 4) also point toward the fact that sperm quality deterioration in indoor captive maturation units can also lead to poor mating percentage or fertilization rate. Although it is reported that spermatophores from single mating can fertilize up to three successive spawns (Primavera, 1985), sperm quality deterioration and blackening of sperm pack in thelycum are also reported from closed thelycum shrimps (Pratoomchaat et al., 1993; Jiang et al., 2009; Alfaro-Montoya et al., 2018).
Furthermore, the delay between impregnation and gonad development of closed thelycum shrimps, unlike open thelycum shrimp, where shrimp freshly mate just before spawning (Palacios and Racotta, 2003; Arcos et al., 2004; Ibarra et al., 2007) also remain another challenge in successful spawning performance of closed thelycum shrimp in captivity. These remain major reasons behind the fact that despite the advancement in reproduction, domestication, and selective breeding of closed thelycum shrimp lag far behind the selective breeding of open thelycum shrimps such as P. vannamei. Even though artificial inseminations are successful in open thelycum shrimp, a few with limited success are reported in closed thelycum shrimps (Alfaro-Montoya et al., 2018). In fact, there is a dearth of scientific data on how to improve the mating efficacy of closed thelycum shrimps in captive systems. Recently, Santander-Avancena et al. (2022) reported monosex rearing of P. indicus (5-7 g) potentially increases mating success up to 80% compared to 20% mating recorded in communal rearing. This suggests the scope for exploring a single-pair mating system in P. indicus indoor captive systems. Further, as shrimps reared in outdoor broodstock ponds recorded 100% impregnation or mating, a multipronged strategy with manipulation of conducive environmental cues can enhance the mating efficacy in the indoor confined maturation units and is worth investigating.
The wild broodstocks used in the trial had a higher latency period and lower post-eye stalk ablation survival (71%) compared to domesticated broodstocks (89 – 92%). This matches with a recent historical review on the reproductive performance of wild P. monodon brooders in Bangladesh shrimp hatcheries, where authors reported wild broodstock quality deterioration and decline in survival after eyestalk ablation (Debnath et al., 2015). According to the authors, higher viral load in coastal water, poor post-capture handling practices, and transportation stress might be plausible explanations for the low survival of wild broodstocks in captivity (Debnath et al., 2014; Debnath et al., 2015). In fact, the latency period ranges from three days up to two months depending on the age and source of broodstock, stage of the moult cycle, etc. (Primavera, 1985; Palacios and Racotta, 2003; Arcos et al., 2004). It is worth noting that a lower latency period and higher survival recorded in the captive-reared broodstocks in the present study emphasize better broodstock condition and reproductive readiness of the captive-reared shrimp.
The oocyte size groups in different ovarian developmental stages of captive-reared broodstock in the present trial (Trial II) match the ovarian histological architecture of wild P. indicus broodstocks (Mohamed and Diwan, 1994; Anand et al., 2019). However, matured ovaries of the captive-reared broodstock recorded asynchronous ovarian development with cortical oocyte and previtellogenic oocytes, unlike gravid ovaries of wild broodstock with homogenous development of cortical oocytes throughout the ripe ovaries (Figure 10). In contrast, Palacios et al. (1999c) could not find any differences in relation to the proportion of late vitellogenic or cortical oocyte stages in pond-reared P. vannamei, unlike differences recorded in previtellogenic and early-vitellogenic oocyte distribution. A heterogeneous distribution of vitellogenin in the ovaries of ablated pond-reared broodstocks may be a plausible reason for the lower proportion of cortical oocytes in captive broodstock compared to wild gravid ovaries. Therefore, further investigation is needed to understand the vitellogenin pathways in ablated captive stocks compared to wild gravid spawners. The lower viability of the spawners immediately after eyestalk ablation was recorded (Primavera, 1985) and is reported to be due to due to the stress associated with the eyestalk ablation procedure (Palacios et al., 1999b).
The differential oocyte development in gravid ovaries of captive-reared brooders might have resulted in the release of only matured ova and a subsequent higher percentage of partial spawning in captive-reared stocks compared to wild gravid spawners. Further, unlike earlier reports where a lack of cortical rods was reported in gravid oocytes of pond-reared P. japonicus broodstock (Yano, 1998), well-distinguished cortical oocytes were recorded in the gravid ovaries of captive-reared P. indicus. The complete development of oocytes into its final cortical oocyte stages suggests the broodstock development potential of P. indicus captivity. The size of the fertilized eggs and larval stages (Supplementary Figure 2) also matches with earlier observations in wild P. indicus (Muthu et al., 1979; Anand et al., 2019).
Spawning frequency or multiple spawning is another crucial factor in breeding programs (Ibarra et al., 2007). It is reported that the majority of the nauplius production is contributed by a smaller population of multiple spawners (Arcos et al., 2003; Racotta et al., 2003). For instance, a 36-day study using P. vannamei broodstocks revealed only 19% population had multiple spawning with 18, and 15% population spawned once or twice, whereas 48% of females did not spawn at all (Arcos et al., 2003). Equally, a 29 days broodstock rearing study revealed only 14% of the population recorded multiple spawning (Arcos et al., 2004). Our results are consistent with earlier reports where only 20% of the captive-reared broodstock reported multiple spawning. However, selectively bred P. vannamei are reported to spawn up to 10 to 12 times per cycle in commercial shrimp hatcheries (Kannan et al., 2015; Ren et al., 2020). This indicates apart from phenotypic parameters (Hoang et al., 2002; Arcos et al., 2003; Palacios and Racotta, 2003), genetic (Ibarra et al., 2007) or endocrine parameters (Tsutsui et al., 2005; Treerattrakool et al., 2014; Huerlimann et al., 2018) also can play a significant role in shrimp broodstock performance. This further suggests that elucidating the endocrine mechanism behind the productive and non-productive domesticated shrimp is worth exploring. Additionally, though the broodstock performance of P. indicus cannot be compared with the reproductive performance of selectively bred P. vannamei, the reproductive potential of Indian white shrimp is on par with the optimal ranges reported for other closed thelycum shrimps (Keys et al., 2004; Preston et al., 2010). As the reproductive output of the species increases over successive generations, genetic improvement of spawning frequency, which is reported to have moderate heritability, h2 = 0.15 (Ren et al., 2020), may also be aimed at genetic improvement and selective breeding programs of Indian white shrimp.
Length-weight relationship
Length-weight relationship and the condition factor of the shrimp is influenced by both biotic and abiotic parameters (Gopalakrishnan et al., 2014). It is reported that crustaceans exhibit a bimodal growth pattern where one sex grows larger in size than the other (Hartnoll, 1982). This sigmoidal growth pattern becomes more conspicuous as the shrimp attain sexual maturity (Dall et al., 1990). In the present study, females were bigger than males as they grew faster toward maturity (Hoang et al., 2003). The L-W of P. indicus during the grow-out, and reproductive phase recorded negative allometric growth. It is recorded that body weight in proportion to length is higher in older shrimps. This is in accordance with earlier reports on P. monodon (Primavera et al., 1998); P. vannamei (Chow and Sandifer, 1991), and M. rosenbergii (Kurup et al., 2000; Lalrinsanga et al., 2012). This can be attributed to the fact that as shrimp grows towards maturity, the weight gain is not proportional to the length of the shrimp, unlike nursery stages where a positive allometric growth is observed due to increases in length with proportional body weight gain. Similarly, a negative allometric was also reported in pond-reared (b = 2.72) and wild shrimp (b = 2.49) by Gopalakrishnan et al. (2014). Though it is reported that wild P. monodon from natural water was heavier than the shrimps from culture ponds (Cheng and Chen, 1990), similar trends were not applicable in the present studies as only broodstock from wild or cultured ponds were available.
Relative condition index, a ratio of body weight to length, suggests that shrimp in good condition has a factor 1 or above (high body weight/length ratio), compared to poor condition factor (below 1) with a low body weight to length ratio (Emmerson, 1980; Palacios et al, 1999a; Arcos et al., 2003). In the present study, all the shrimps at different growth stages had a relative condition index (Kn) above 1, indicating shrimps were in good rearing condition. In contrast, a lower Fulton condition factor, K value, is reported in the early life stages (Kunda et al., 2008; Lalrinsanga et al, 2012; Mane et al., 2019). This matches with present reports where nursery-reared shrimps had b value closer to 3, so the Fulton condition factor (K) was calculated (Lalrinsanga et al, 2012), which ranged from 0.65-0.67, and was lower compared to the relative condition factor (Kn) calculated for grow out or adult brooders (1.02-1.04).This can be attributed to the fact that in early life stages, shrimp grew faster in length than weight (Mane et al., 2019). Similarly, a lower K value (0.70 ± 0.1) was recorded during the early culture phase of M. rosenbergii compared to a higher K value (1.05 ± 0.1) towards the end of the culture (Kunda et al., 2008; Lalrinsanga et al, 2012) Among the broodstocks, the condition factors of the female broodstocks were higher than the male irrespective of the generation (Udoinyang et al., 2016). On the contrary, Kumari et al. (2013) recorded higher K values for males. However, in the present study, no sex-wise variation in the relative condition index was recorded, which is in line with Choe (1971), who observed no difference in the body length and weight between sexes in P. japonicus. It is also important to note that environmental or cultural conditions (stocking density, feed management) can also play a direct role in the growth and condition factor of the penaeid shrimp.
Environmental parameters and broodstock rearing condition
Although the reproductive performance of the shrimp is under the control of additive genetic composition, farm management regime and culture environment also play a crucial role in shrimp reproduction (Ibarra et al., 2007). Environmental cues are critical modulators of gonad or vitellogenic stimulating hormones in penaeids shrimps. The PCA analysis revealed a strong influence of monthly variation in environmental parameters such as temperature, day-night cycle, and salinity in mating and gonad development of captive-reared Indian white shrimp (Figure 6). Abiotic parameters such as salinity, temperature, tank size and depth, light intensity and photoperiod, and lunar periodicity are also reported to play a prominent role in the reproductive performance of the shrimp (Hoang et al., 2002). In the present trial, although mating and maturation were not major constraints in outdoor broodstock ponds, a lack of predictability over critical parameters such as salinity and temperature was recorded due to seasonal fluctuations. For example, although gonad development of captive-reared P. indicus was recorded at 28 – 36 ppt salinity, 100% gonad absorption was recorded when salinity was reduced to below 25 ppt during the rainy season. Similarly, the earlier reports on the resorption of ovaries below the optimum level of salinity (Ituarte et al., 2010) also suggest the vital role of salinity in shrimp reproduction. Photoperiod (light and dark cycle) and light intensity were also reported to play a critical role in ovarian maturation and spawning of P. monodon (Primavera, 1985), P. stylirostris, P. vannamei (Chamberlain and Lawrence, 1987, Wyban et al., 1987), P. indicus (Emmerson et al., 1983), and P. merguiensis (Hoang et al., 2003). For example, in the present trial, successful mating was recorded in broodstocks reared under natural photoperiod compared to indoor maturation units with limited light intensity (Figures 2A, B). However, the gonad development was also not hampered even at higher light intensity during the natural photoperiod regime (Figure 2B) in broodstock ponds. In contrast, it is reported that dim light is an important prerequisite for maturation (Primavera, 1985), and bright light (above 1100 lux) or continuous dark conditions can inhibit the ovarian maturation of brooders (Hoang et al., 2003). This suggests light intensity requirement of the species varies depending on the mating or maturation stages of the shrimp. Natural ecosystems where shrimp mature and spawn in deep waters have weak light intensity, unlike complete darkness (Hoang et al., 2002). Unlike 100% mating or impregnation recorded in outdoor rearing units, the lack of subdued dawn and dusk effect and insufficient light intensity gradation over time might have resulted in poor mating efficiency in indoor maturation units (Trial II). The PCA analysis also revealed a strong influence of water temperature on the gonad development of captive-reared P.indicus (Figure 6). The present findings are in agreement with earlier studies where temperature is reported as one of the vital abiotic factors in shrimp reproduction (Primavera, 1985; Staples and Heales, 1991). For example, an increase in temperature from winter to spring was reported to enhance spawning in natural conditions (Rothlisberg et al., 1987; Dall et al., 1990; Yano, 1993). Further, a combined effect of day length and temperature is also found to stimulate ovarian maturation and spawning in P. duorarum (Cripe, 1994) and P. stylirostris (Robertson et al., 1991). These reinforce the importance of environmental cue manipulation to achieve better mating efficiency and reproductive performance of closed thelycum shrimps in captivity.
The rearing system is also reported to play a vital role in shrimp broodstock development. It is reported that the reproductive performance of domesticated P. vannamei was much better when raised in earthen ponds compared to the clear water recirculatory system (Ren et al., 2020). This finding corroborates our personal observation that mating efficiency and body weight gain were much inferior in indoor systems compared to outdoor broodstock ponds. Likewise, various studies also report the poor performance of indoor tank-raised broodstock compared to pond-reared broodstock irrespective of similar husbandry techniques (Preston et al., 2004; Coman et al., 2006; Andriantahina et al., 2012; Arnold et al., 2013). This urges many hatchery operators to depend on broodstocks raised in earthen broodstock ponds compared to SPF stocks reared in indoor maturation tanks (Ren et al., 2020). Conversely, outdoor ponds are subjected to seasonal fluctuation, natural calamities, biosecurity breaches, and subsequent pathogen entries. These necessitate the development of controlled semi-outdoor broodstock rearing units to achieve optimum positive conducive cues for shrimp gonad maturation under stringent biosecurity.
Further, suboptimal maturation conditions can also definitely affect the performance of broodstocks. Hence, it is also important to develop strategies to compensate for nutritional loss through well-managed nutritional supplements (Bray et al., 1990; Palacios et al., 2001; Wyban, 2007; Goodall et al., 2016). Broodstock ponds are conducive ecosystems that can enhance broodstock performance by harnessing natural productivity, whereas tank-based confined systems need natural microbial community manipulation. For example, a biofloc-based tank system reared P. duorarum broodstock recorded better reproductive performance compared to clear water system (Emerenciano et al., 2013). However, most hatchery owners prefer clear water indoor broodstock rearing systems for better broodstock visibility. The development of outdoor mixotrophic broodstock rearing units based on the integration of autotrophic and heterotrophic communities can have dual advantages as it can mimic the natural ecosystem through conducive environmental cues and can provide the scope for nutritional intervention based on microbial enrichment under stringent biosecurity in confinement.
Growth performance
The domesticated shrimps are also reported to have better feed utilization efficiency (Glencross et al., 2013) and disease resistance (Knibb et al., 2015), and enhanced farm productivity (Preston et al., 2010; Norman-Lόpez et al, 2016) over successive generations. Further, improvement in growth and metabolic advantages of closed thelycum P. monodon (Preston et al., 2010; Glencross et al., 2013), P. japonicus (Preston et al., 2004), P. esculentus (Keys and Crocos, 2006) were also recorded over generations. Further, it is reported that significant improvement in growth can be achieved from the 4th or 5th generation onwards (Norman-Lόpez et al, 2016). Although no genetic selection procedures were followed in the present study, the improved growth rate or reproductive performance of GN-2 compared with GN-1 might be due to unintended selection that happened during the domestication procedure (Figure 4). These further indicate the scope for potential growth improvement during the genetic selection-based breeding program of Indian white shrimp.
Conclusion
In the present study, the onset of mating, gonad development, and reproductive performance over two generations of captive-reared P. indicus in existing tropical environmental conditions in India was discussed. Further, its spawning performance in relation to wild broodstock revealed that it later had higher fecundity and hatchability, while captive-reared brooders recorded higher survival after eyestalk ablation and a better latency period. The indoor maturation cycle of eyestalk-ablated captive-reared broodstock revealed increased molting post-eyestalk ablation, resulting in a decline in mating percentage and spermatophore quality towards the end of the maturation cycle. As selective breeding programs in closed thelycum species are challenging due to inherent reproductive constraints such as poor mating efficiency with limited success in artificial insemination and slow gonad development in captivity, concerted efforts are needed to enhance natural mating through conducive environmental cue manipulation and functional broodstock diet for gonad development in captivity. The output from the current research findings forms baseline data for future genetic improvement and selective breeding of the native Indian white shrimp with zero pressure on wild stock. This can further generate a renewed interest among the shrimp industry and various stakeholders where the current exclusive dependence on single species Pacific white shrimp P. vannamei calls for species diversification using native penaeids.
Data availability statement
The original contributions presented in the study are included in the article/Supplementary Material. Further inquiries can be directed to the corresponding author.
Author contributions
PA: Conceptualization, conducting the experiment, investigation, methodology validation, visualization, data analysis, writing - original draft, review & editing. CB: Funding acquisition, conceptualization, project administration, methodology validation, resources distribution, supervision. writing -review & editing. RA: Conducting the larval and nursery experiments, investigation of water quality parameters, and analysis. IB: Male reproductive performance parameters analysis. RR: Growth and reproductive performance data collection and seed production. TV: Growth and reproductive performance data collection and larval rearing, review, and editing. AP: Resources, methodology, validation, review & editing. NS: Disease screening of broodstock. SR: Data collection on growth parameters during grow-out and nursery rearing. SK: Investigation of microbial analysis, correlation matrix and statistical analysis, data analysis and validation, review & editing. JA: Review & editing the manuscript. KA: Experimental diet formulation, investigation on proximate analysis. KJ: Review and editing, resources. KV: Project administration, funding acquisition, resources, monitoring and editing the manuscript. All authors contributed to the article and approved the submitted version.
Funding
The authors are grateful for the financial support and facility provided by the Indian Council of Agricultural Research and ICAR-Central Institute of Brackishwater Aquaculture (ICAR-CIBA) under the in-house project FISHCIBASIL201800200130 to carry out the research work.
Acknowledgments
The field and lab assistance received from the technical and field staff at Muttukadu Research Station of ICAR-CIBA, Muttukadu, Tamil Nadu, India, is duly acknowledged.
Conflict of interest
The authors declare that the research was conducted in the absence of any commercial or financial relationships that could be construed as a potential conflict of interest.
Publisher’s note
All claims expressed in this article are solely those of the authors and do not necessarily represent those of their affiliated organizations, or those of the publisher, the editors and the reviewers. Any product that may be evaluated in this article, or claim that may be made by its manufacturer, is not guaranteed or endorsed by the publisher.
Supplementary material
The Supplementary Material for this article can be found online at: https://www.frontiersin.org/articles/10.3389/fmars.2023.1101806/full#supplementary-material
Supplementary Figure 1 | (A–C). The correlation matrix depicts the relationship between fecundity and spawners body weight, carapace length, eggs per gram body weight, and total nauplius counts.
Supplementary Figure 2 | Larval length (Mean ± SE) of first (GN-1), second (GN-2), and third (GN-3) generation of larvae produced from the Penaeus indicus broodstock G0, GN-1, and GN-2, respectively.
Footnotes
References
Alavandi S. V., Muralidhar. M., Dayal S., Rajan J. S., Ezhil Praveena P., Bhuvaneswari T., et al. (2019). Investigation on the infectious nature of running mortality syndrome (RMS) of farmed pacific white leg shrimp, Penaeus vannamei, in shrimp farms of India. Aquaculture 500, 278–289. doi: 10.1016/j.aquaculture.2018.10.027
Alfaro-Montoya J., Braga A., and Umaña-Castro R. (2018). Research frontiers in penaeid shrimp reproduction: Future trends to improve commercial production. Aquaculture 503, 70–87. doi: 10.1016/j.aquaculture.2018.12.068
Anand P. S. S., Aravind R., Balasubramanian C. P., Kumar S., Jose A., Biju I. F., et al. (2022). Growth, survival, and osmo-ionic regulation in post larval and juvenile Indian white shrimp, penaeus indicus, reared under three levels of salinity in a semifloc system. Aquaculture 739042. doi: 10.1016/j.aquaculture.2022.739042
Anand P. S. S., Aravind R., Biju I. F., Balasubramanian C. P., Jose A., Christina L., et al. (2021). Nursery rearing of Indian white shrimp, Penaeus indicus: Optimization of dietary protein levels and stocking densities under different management regimes. Aquaculture 542 736807. doi: 10.1016/j.aquaculture.2021.736807
Anand P. S. S., Balasubramanian C. P., Francis B., Panigrahi A., Aravind R., Das R., et al. (2019). Reproductive performance of wild brooders of Indian white shrimp, Penaeus indicus: Potential and challenges for selective breeding program. J. Coast. Res. 86, 65–72. doi: 10.2112/SI86-010.1
Andriantahina F., Liu X., Huang H., Xiang J., Yang C. (2012). Comparison of reproductive performance and offspring quality of domesticated pacific white shrimp. Litopenaeus vannamei. Aquaculture 324, 194–200. doi: 10.1016/j.aquaculture.2011
APHA (American Public Health Association) (2012). Standard method for Examination of Water and Wastewater. 22nd ed. (Washington, DC: American Public Health Association).
Arcos F. G., Ibarra A. M., Palacios E., Vazquez-Boucard C., Racotta I. S. (2003). Feasible predictive criteria for reproductive performance of white shrimp Litopenaeus vannamei: egg quality and female physiological condition. Aquaculture 228, 335–349. doi: 10.1016/s0044-8486(03)00313-2
Arcos F. G., Racotta I. S., Ibarra A. M. (2004). Genetic parameter estimates for reproductive traits and egg composition in pacific white shrimp Penaeus(Litopenaeus) vannamei. Aquaculture 236, 151–165. doi: 10.1016/j.aquaculture.2004.03.003
Arnold S. J., Coman G. J., Burridge C., Rao M. (2012). A novel approach to evaluate the relationship between measures of male fertility and egg fertilization in Penaeus monodon. Aquaculture 338, 181–189. doi: 10.1016/j.aquaculture.2012.01.013
Arnold S. J., Coman G. J., Emerenciano M. (2013). Constraints on seed stock production in eighth-generation domesticated Penaeus monodon broodstock. Aquaculture 410, pp. doi: 10.1016/j.aquaculture.2013.06.023
Bell T. A., Lightner D. V. (1988). A handbook of normal penaeid shrimp histology. World Aquaculture Society Baton Rouge, 114.
Bray W. A., Lawrence A. L., Lester L. J. (1990). Reproduction of eyestalk ablated Penaeus stylirostris fed various levels of total dietary lipid. J. World Aquacult. Soc 21pp (1), 41–52. doi: 10.1111/j.1749-7345.1990.tb00952.x
Briggs M., Funge-Smith S., Subasinghe R., Phillips M. (2004). Introductions and movement of penaeus vannamei and penaeus stylirostris in Asia and the pacific. (Food and Agriculture Organization of the United Nations, Regional Office for Asia and the Pacific, Bangkok: RAP Publ. 2004/10), 92.
Browdy C. L. (1992). “A review of the reproductive biology of penaeus species: perspectives on controlled shrimp maturation systems for high quality nauplii production,” in Proceedings of the special session on shrimp farming, 22–25 may 1992, vol. 1992) . Ed. Wyban J. (Orlando, FL, USA: World Aquaculture Society, LA, USA), 22–51.
Browdy C. L. (1998). Recent developments in penaeid broodstock and seed production technologies: improving the outlook for superior captive stocks. Aquaculture 164, 3–21. doi: 10.1016/S0044-8486(98)00174-4
Browdy C. L., Hadani A., Samocha T. M., Loya Y. (1986). The reproductive performance of wild and pond-reared Penaeus semisulcatus de haan. Aquaculture 59, 251–258. doi: 10.1016/0044-8486(86)90007-4
Brown A. Jr., Patlan D. (1974). Color changes in the ovaries of penaeid shrimp as determinant of their maturity. Mar. Fish. Rev. 36 (7), 23–26.
Ceballos-Vázquez B. P., Palacios E., Aguilar-Villavicencio J., Racotta I. S. (2010). Gonadal development in male and female domesticated whiteleg shrimp, Litopenaeus vannamei, in relation to age and weight. Aquaculture 308, 116–123. doi: 10.1016/j.aquaculture.2010.08.020
Ceballos-Vázquez B. P., Racotta I. S., Elorduy-Garay J. F. (2003). Qualitative and quantitative analysis of the ovarian maturation process of penaeus vannamei after a production cycle. Invertebr. Reprod. Dev. 43 (1), 9–18. doi: 10.1080/07924259.2003.9652516
Chamberlain G. W., Lawrence A. L. (1987). Effect of light intensity and male and female eyestalk ablation on reproduction of and P. vannamei. World Maric. Soc 12, 357–372. doi: 10.1111/j.1749-7345.1981.tb00308.x
Cheng C. S., Chen L. C. (1990). Growth characteristics and relationships among body length, body weight and tail weight of Penaeus monodon from a culture environment in Taiwan. Aquaculture 91 (3-4), 253–263. doi: 10.1016/0044-8486(90)90192-P
Choe S. (1971). Body increases during molt and molting cycle of the oriental brown shrimp Penaeus japonicus. Mar. Biol. 9, 31–37.
Chou H. Y., Huang C. Y., Wang C. H., Chiang H. C., Lo C. F. (1995). Pathogenicity of a baculovirus infection causing white spot syndrome in cultured penaeid shrimps in Taiwan. Dis. Aquat. Organ. 23, 165–173.
Chow S., Sandifer P. A. (1991). Differences in growth, morphometric traits, and male sexual maturity among pacific white shrimp, Penaeus vannamei, from different commercial hatcheries. Aquaculture 92, 165–178. doi: 10.1016/0044-8486(91)90018-3
Christina L., Panigrahi A., Shyne Anand P. S., Das S., Ghoshal T. K., Ambasankar K., et al. (2020). Effect of varying levels of dietary protein on the growth performances of Indian white shrimp Penaeus indicus (H. Milne Edwards). Aquaculture 519, 734736. doi: 10.1016/j.aquaculture.2019.734736
CIBA. (2019). “Annual report 2018-19,” (Institute of Brackishwater Aquaculture: Chennai, Tamil Nadu, India), 240.
Coman G. J., Arnold S. J., Barclay M., Smith D. M. (2011). Effect of arachidonic acid supplementation on reproductive performance of tank-domesticated Penaeus monodon. Aquac. Nutr. 17, 141–151. doi: 10.1111/j.1365-2095.2009.00716.x
Coman G. J., Arnold S. J., Jones M. J., Preston N. P. (2007). Effect of rearing density on growth, survival and reproductive performance of domesticated Penaeus monodon. Aquaculture 264, 175–183. doi: 10.1016/j.aquaculture.2006.12.014
Coman G. J., Arnold S. J., Peixoto S., Crocos P. J., Coman F. E., Preston N. P. (2006). Reproductive performance of reciprocally crossed wild-caught and tank-reared Penaeus monodon broodstock. Aquaculture 252, 372–384. doi: 10.1016/j.aquaculture.2005.07.028
Coman G. J., Arnold S. J., Wood A. T., Preston N. P. (2013). Evaluation of egg and nauplii production parameters of a single stock of domesticated Penaeus monodon (giant tiger shrimp) across generations. Aquaculture 400–401, 125–128. doi: 10.1016/j.aquaculture.2013.03.015
Coman G. J., Crocos P. J., Arnold S. J., Keys S. J., Preston N. P., Murphy B. (2005). Growth, survival and reproductive performance of domesticated Australian stocks of theGiant tiger prawn, Penaeus monodon, reared in tanks and raceways. World Aquacult. 36pp, 464–479. doi: 10.1111/j.1749-7345.2005.tb00394.x
Cripe G. M. (1994). Induction of maturation and spawning of pink shrimp, by changing water temperature, and survival and growth of the young. Aquaculture 128, 255–260. doi: 10.1016/0044-8486(94)90314-X
Crocos P. J., Coman G. J. (1997). Seasonal and age variability in the reproductive performance of Penaeus semisulcatus: optimizing broodstock selection. Aquaculture 155, 55–67. doi: 10.1016/S0044-8486(97)00109-9
Dall W., Hill B. J., Rothlisberg P. C., Sharples D. J., Blaxter J, H, S., Southward A. J. (1990). “The biology of penaeidae,” in Advances in marine biology, vol. 27. (United sates: Academic Press), 1–489.
Debnath P., Karim M., Belton B. (2014). Comparative study of the reproductive performance and white spot syndrome virus (WSSV) status of black tiger shrimp (Penaeus monodon) collected from the bay of Bengal. Aquaculture 424-425, 71–77.
Debnath P., Khan S. H., Karim M., Belto N. B., Mohan C. V., Phillips M. (2015). Review of the history, status and prospects of the black tiger shrimp (Penaeus monodon) hatchery sector in Bangladesh. . Rev.Aquac 8 (3), 301–313.
Emerenciano M., Cuzon G., Arévalo M., Miquelajauregui M. M., Gaxiola G. (2013). Effect of short-term fresh food supplementation on reproductive performance, biochemical composition, and fatty acid profile of Litopenaeus vannamei (Boone) reared under biofloc conditions. Aquac. Int. 21pp (5), 987–1007. doi: 10.1007/s10499-012-9607-4
Emerenciano M., Cuzon G., Goguenheim J., Gaxiola G., Aquacop (2012). Floc contribution on spawning performance of blue shrimp Litopenaeus stylirostris. Aquac. Res. 44 (1), 75–85. doi: 10.1111/j.1365-2109.2011.03012.x
Emmerson W. D. (1980). Induced maturation of prawn Penaeus indicus. mar. Ecol. Prog. Ser. 2 pp, 121–131.
Emmerson W. D., Hayes D. P., Ngonyame M. (1983). Growth and maturation of Penaeus indicus under blue and green light.S. Afr. J. Zool. 18, 71–75.
FAO (2020). Sustainability in action (Food and Agriculture Organization: Rome), 206.Available at: http://www.fao.org/3/ca9229en/ca9229en.pdf.
Glencross B., Tabrett S., Irvin S., Wade N., Anderson M., Blyth D., et al. (2013). An analysis of the effect of diet and genotype on protein and energy utilization by the black tiger shrimp, Penaeus monodon why do genetically selected shrimp grow faster? Aquac. Nutr.19 pp, 128–138. doi: 10.1111/j.1365-2095.2012.00941.x
Goodall J. D., Wade N. M., Merritt D. J., Sellars M. J., Salee K., Coman G. J. (2016). The effects of adding microbial biomass to grow-out and maturation feeds on the reproductive performance of black tiger shrimp, Penaeus monodon. Aquaculture 450, pp. doi: 10.1016/j.aquaculture.2015.07.036
Gopalakrishnan A., Rajkumar M., Rahman M. M., Sun J., Antony P. J., Venmathimaran B. A., et al. (2014). Length-weight relationship and condition factor of wild, grow-out and’loose-shell affected’ giant tiger shrimp, Penaeus monodon (Fabriciu)(Decapoda: Penaeidae). J. Appl. Ichthyol. 30, 251–225. doi: 10.1111/jai.12269
Hartnoll R. G. (1982). “Growth. the biology of crustacea. vol 2,” in Embryology, morphology and genetics. Eds. Bliss D. E., Abele L. G. (New York, New York: Academic Press), Pp. 111–185.
Hetzel D. J. S., Crocos P. J., Davis G. P., Moore S. S., Preston N. P. (2000). Response to selection for growth in the kuruma shrimp, Penaeus japonicus. Aquaculture 181, pp. doi: 10.1016/S0044-8486(99)00237-9
Hoa T. T.T., Zwart M. P., Phuong N. T., Vlak J. M., de Jong M. C. (2011). Transmission of white spot syndrome virus in improved-extensive and semi-intensive shrimp production systems: A molecular epidemiology study. Aquaculture 313 (1-4), 7–14. doi: 10.1016/j.aquaculture.2011.01.013
Hoa N. D., Wouters R., Wille M., Thanh V., Dong T. K., Van Hao N., et al. (2009). A fresh-food maturation diet with an adequate HUFA composition for broodstock nutrition studies in black tiger shrimp Penaeus monodon (Fabriciu). Aquaculture 297 (1-4), pp.116–pp.121. doi: 10.1016/j.aquaculture.2009.09.005
Hoang T., Barchiesis M., Lee S. Y., Keenan C. P., Marsden G. E. (2003). Influences of light intensity and photoperiod on moulting and growth of Penaeus merguiensis cultured under laboratory conditions. Aquaculture 216 (1-4), pp. doi: 10.1016/S0044-8486(02)00460-X
Hoang T., Lee S. Y., Keenan C. P., Marsden G. E. (2002). Effect of temperature on the spawning of Penaeus merguiensis. J. Thermal Biol. 27pp (5), 433–437. doi: 10.1016/S0306-4565(02)00013-X
Huerlimann R., Wade N. M., Gordon L., Montenegro J. D., Goodall J., McWilliam S., et al. (2018). De novo assembly, characterization, functional annotation and expression patterns of the black tiger shrimp (Penaeus monodon) transcriptome. Sci. Rep. 8, ) pp. 1–14. doi: 10.1038/s41598-018-31148-4
Ibarra A. M., Racotta I. S., Arcos F. G., Palacios E. (2007). Progress on the genetics of reproductive performance in penaeid shrimp. Aquaculture 268, 23–43. doi: 10.1016/j.aquaculture.2007.04.028
Ituarte R. B., Spivak E. D., Camiolo M., Anger K. (2010). Effects of salinity on the reproductive cycle of female freshwater shrimp, Palaemonetes Argentinus.J.Crustac. Biol. 30 (2), 186–193.
Jayawardane P. A. A. T., McLusky D. S., Tytler P. (2002). Estimation of population parameters and stock assessment of Penaeus indicus (H. Milne edwards) in the western coastal waters of Sri Lanka. Asian Fish Sci. 15 (2), 155–166. doi: 10.33997/j.afs.2002.15.2.007
Jiang S. G., Huang J. H., Zhou F. L., Chen X., Yang Q. B., Wen W. G., et al. (2009). Observations of reproductive development and maturation of male Penaeus monodon reared in tidal and earthen ponds. Aquaculture 292, 121–128. doi: 10.1016/j.aquaculture.2009.03.054
Kannan D., Thirunavukkarasu P., Jagadeesan K., Shettu N., Kumar A. (2015). Procedure for maturation and spawning of imported shrimp Litopenaeus vannamei in commercial hatchery, south East coast of India. Fish. Aquacult J. 6, 14–16. doi: 10.4172/2150-3508.1000146
Kassambara A., Mundt F. (2017). “Factoextra: Extract and visualize the results of multivariate DataAnalyses,” in R package version. 1 (5), 337–354. Available at: https://CRAN.R-project.org/package=factoextra.
Keys S. J., Crocos P. J. (2006). Domestication, growth and reproductive performance of wild, pond and tank-reared brown tiger shrimp Penaeus esculentus. Aquaculture 257 (1-4), 232–240. doi: 10.1016/j.aquaculture.2006.02.044
Keys S. J., Crocos P. J., Burridge C. Y., Coman G. J., Davis G. P., Preston N. P. (2004). Comparative growth and survival of inbred and outbred Penaeus (marsupenaeus) japonicus, reared under controlled environment conditions: indications of inbreeding depression. Aquaculture 241 (1-4), 151–168. doi: 10.1016/j.aquaculture.2004.08.039
Kimura T., Yamano K., Nakano H., Momoyama K., Hiraoka M., Inouye. K. (1996). Detection of penaeid rod-shaped DNA virus (PRDV) by PCR. Fish Pathol. 31, 93–98. doi: 10.3147/jsfp.31.93
Knibb W., Quinn J., Kuballa A., Powell D., Remilton C., Nguyen N. H. (2015). Yearly, pond, lineage and family variation of hepatopancreatic parvo-like virus (HPV) copy number in banana shrimp Fenneropenaeus merguiensis. J. Invertebr. Pathol. 128, 73–79. doi: 10.1016/j.jip.2015.05.002
Kumaran M., Geetha R., Antony J., Vasagam K. P. K., Anand P. R., Ravisankar T., et al. (2021). Prospective impact of coronavirus disease (COVID-19) related lockdown on shrimp aquaculture sector in India - a sectoral assessment. Aquaculture 531, 735922. doi: 10.1016/j.aquaculture.2020.735922
Kumar S., Shyne Anand P. S., De D., Ghoshal T. K., Alavandi S., Vijayan K. K. (2019). Integration of substrate in biofloc based system: Effects on growth performance, water quality and immune responses in black tiger shrimp, Penaeus monodon culture. Aquac. Res. 50 (10), 2986–2999. doi: 10.1111/are.14256
Kumari M. S., Sucharita M. S., Dash Bisnu P., Debanshu Sekhar P. (2013). Length-Weight relationships and condition factor of Penaeus monodon Fabricius, 1798 in Northern Odisha, India. Int. J. Fish. Aquat. 4, 2319–7064.
Kunda M., Azim M. E., Wahab M. A., Dewan S., Roos N., Thilsted S. H. (2008). Potential of mixed culture of freshwater prawn (Macrobrachium rosenbergii) and self-recruiting small species mola (Amblypharyngodon mola) in rotational rice–fish/prawn culture systems in Bangladesh. Aquac. Res. 39 (5), 506–517. doi: 10.1111/j.1365-2109.2008.01905.x
Kurup B. M., Harikrishnan M., Sureshkumar S. (2000). Length-weight relationship of male morphotypes of Macrobrachium rosenbergii (De Man, 1879) as a valid index for differentiating their developmental pathway and growth phases. Indian J. Fish. 47 (4), 283–290.
Lalrinsanga P. L., Pillai B. R., Patra G., Mohanty S., Naik N. K., Sovan S. A. H. U. (2012). Length-weight relationship and condition factor of giant freshwater prawn Macrobrachium rosenbergii (De Man, 1879) based on developmental stages, culture stages and sex. Turkish J. Fish. Aquat. Sci. 12 (4), 917–924. https://dergipark.org.tr/en/pub/trjfas-ayrildi/issue/13267/160221.
Li K., Liu L., Clausen J. H., Lu M., Dalsgaard A. (2016). Management measures to control diseases reported by tilapia (Oreochromis spp.) and whiteleg shrimp (Litopenaeus vannamei) farmers in Guangdong, China. Aquaculture 457, 91–99. doi: 10.1016/j.aquaculture.2016.02.008
Makinouchi S., Primavera M. H. (1987). Maturation and spawning of Using different ablation methods. Aquaculture 62 1), 73–81. doi: 10.1016/0044-8486(87)90186-4
Mane S., Sundaram S., Hule A., Sawant M., VD D. (2019). Length weight relationship of commercially important penaeid prawns of maharashtra, india. international research. J. Sci. Eng. 7, 35–40. http://creativecommons.org/licenses/by/4.0/.
Marsden G., Richardson N., Mather P., Knibb W. (2013). Reproductive behavioural differences between wild-caught and pond-reared Penaeus monodon prawn broodstock. Aquaculture 402, .141–.145. doi: 10.1016/j.aquaculture.2013.03.019
Megahed M. E., Elmesiry G., Mohamed K., Dhar A. K. (2019). Genetic parameters of survival in four generations of Indian white shrimp (Fenneropeneaus indicus). journal of applied. Aquaculture 31 (2), 121–139. doi: 10.1080/10454438.2018.1551169
Menasveta P., Sangpradub S., Piyatiratitivorakul S., Fast A. W. (1994). Effect of broodstock size and source on ovarian maturationand spawning of Penaeus monodon fabricius from the gulf of Thailand. J. World Aquac. Soc 25, 41–49. doi: 10.1111/j.1749-7345.1994.tb00802.x
Mohamed S. K., Diwan A. D. (1991). Neuroendocrine regulation of ovarian maturation in the Indian white prawn Penaeus indicus h. Milne edwards. Aquaculture 98 (4), 381–393. doi: 10.1016/0044-8486(91)90320-7
Mohamed S. ,. K., Diwan A. D. (1994). Vitellogenesis in the Indian white prawn Penaeus indicus (Crustacea: Decapoda: Penaeidae). J. Aqua. Trop. 9, 157–172.
Mosha E. J., Gallardo W. (2013). Distribution and size composition of penaeid prawns, Penaeus monodon and Penaeus indicus in saadan estuarine area, Tanzania. Ocean Coast. Manage. 82, 51–63. doi: 10.1016/j.ocecoaman.2013.05.003
Muthu M. S., Laxminarayana A. (1979). Induced breeding of the Indian white prawn Penaeus Indicus. Marine Fisheries Information Service, Technical and Extension Series 9, 6–6.
Muthu M. S., Laxminarayana A., Mohamed K. H. (1986). Induced maturation and spawning of Penaeus indicus without eyestalk ablation. Indian J.Fish. 33 (2), 246–250. http://eprints.cmfri.org.in/id/eprint/388.
Muthu M. S., Pillai N. N., George K. V. (1979). Larval development—Penaeus indicus H. Milne Edwards. CMFRI Bulletin 28, 12–21.
Norman-Lόpez A., Sellars M. J., Pascoe S., Coman G. J., Murphy B., Moore N., et al. (2016). Productivity benefits of selectively breeding black tiger shrimp (Penaeus monodon) in Australia. Aquac. Res. 47 (10), 3287–3296. doi: 10.1111/are.12782
Palacios E., Darren˜o D., Rodrı´guez-Jaramillo M. C., Racotta I. S. (1999b). Effect of eyestalk ablation on maturation, larval performance and biochemistry of white pacific shrimp, Penaeus vannamei, broodstock. J. Appl. Aquac. 9, 1–23. doi: 10.1300/J028v09n03_01
Palacios E., Perez-Rostro C. I., Ramirez J. L., Ibarra A. M., Racotta I. S. (1999a). Reproductive exhaustion in shrimp (Penaeus vannamei) reflected in larval biochemical composition, survival and growth. Aquaculture 171 (3-4), 309–321. doi: 10.1016/S0044-8486(98)00393-7
Palacios E., Racotta I. S. (2003). Effect of a number of spawns on the resulting spawn quality of 1-year old pond reared Penaeus vannamei (Boone) broodstock. Aqua. Res. 34, 427–435. doi: 10.1046/j.1365-2109.2003.00826.x
Palacios E., Racotta I. S., Heras H., Marty Y., Moal J., Samain J. F. (2001). Relation between lipid and fatty acid composition of eggs and larval survival in white pacific shrimp (Penaeus vannamei, boon). Aquac. Int.9 531–, 543. doi: 10.1023/A:1020589924810
Palacios E., Rodri´guez-Jaramillo M. C., Racotta I. S. (1999c). Comparison of ovary histology between different size wild and pond-reared shrimp Litopenaeus vannamei (=Penaeus vannamei). Invertebr. Reprod. Dev. 35, 251–259. doi: 10.1080/07924259.1999.9652391
Panigrahi A., Das R. R., Sundaram M., Sivakumar M. R., Jannathulla R., Lalramchhani C., et al. (2021). Cellular and molecular immune response and production performance of Indian white shrimp Penaeus indicus (H. Milne-Edwards, 1837), reared in a biofloc-based system with different protein levels of feed. Fish. Shellfish Immunol. 119, 31–41. doi: 10.1016/j.fsi.2021.09.002
Peixoto S., Cavalli R. O., Wasielesky W. (2005). Recent developments on broodstock maturation and reproduction of Farfantepenaeus paulensis. Braz. Arch. Biol. Technol. 48 (6), 997–1006. doi: 10.1590/S1516-89132005000800016
Peixoto S., Cavalli R. O., Wasielesky W., D`Incao F., Krummenauer D., Milach Â. (2004). Effects of age and size on reproductive performance of captive Farfantepenaeus paulensis broodstock. Aquaculture 238, 173–182. doi: 10.1016/j.aquaculture.2004.04.024
Peixoto S., Wasielesky Jr W., Martino R.C., Milach Â., Soares R., Cavalli R.O., et al (2008). Comparison of reproductive output, offspring quality, ovarian histology and fatty acid composition between similarly-sized wild and domesticated Farfantepenaeus paulensis. Aquaculture. 285(1-4), 201–206. doi: 10.1016/j.aquaculture.2008.08.021
Pratoomchaat B., Piyatiratitivorakul S., Menasveta P., Fast A. W. (1993). Sperm quality of pond-reared and wild-caught Penaeus monodon in Thailand 1. J. World Aquacult. Soc 24 (4), 530–540. doi: 10.1111/j.1749-7345.1993.tb00584.x
Preston N.P., Crocos P.J., Keys S.J., Coman G.J., Koenig R. (2004). Comparative growth of selected and non-selected Kuruma shrimp Penaeus (Marsupenaeus) japonicus in commercial farm ponds; implications for broodstock production. Aquaculture, 231 (1-4), 73–82. doi: 10.1016/j.aquaculture.2003.09.039
Preston N., Coman G., Moore N. (2010). Black tiger breeding program yields record shrimp harvests in Australia. Global Aquacult. Advocate 13 (5), 95. https://www.aquaculturealliance.org/.
Primavera J. H. (1985). “A review of maturation and reproduction in closed thelycum penaeids,” in Proceedings of the first international conference on the culture of penaeid Prawns/Shrimps, (Iloilo city, Philippines), 47–64. Aquaculture Department, Southeast Asian Fisheries Development Center. Available at: http://hdl.handle.net/10862/255.
Primavera J. H., Parado-Estepa F. D., Lebata J. L. (1998). Morphometric relationship of length and weight of giant tiger prawn Penaeus monodon according to life stage, sex and source. Aquaculture 164 (1-4), 67–75. doi: 10.1016/S0044-8486(98)00177-X
Racotta I. S., Palacios E., Ibarra A. M. (2003). Shrimp larval quality in relation to broodstock condition. Aquaculture 227, pp.107–pp.130. doi: 10.1016/s0044-8486(03)00498-8
Ren S., Mather P. B., Prentis P., Li Y., Tang B., Hurwood D. A. (2020). Quantitative genetic assessment of female reproductive traits in a domesticated pacific white shrimp (Penaeus vannamei) line in China. Sci. Rep. 10 (1), pp.1–pp10. doi: 10.1038/s41598-020-64597-x
Robertson L., Bray W., Lawrence A. (1991). Reproductive response ofto temperature manipulation. J. World Aquacult. Soc 22, 109–117. doi: 10.1111/j.1749-7345.1991.tb00723.x
Rothlisberg P. C., Jackson C. J., Pendrey R. C. (1987). Larval ecology of penaeis of the gulf of carpentaria, australia. i. assessing the reproductive activity of five species of from the distribution and abundance of the zoeal stages. Aust. J. Mar. Freshw. Res. 38, 1–1. doi: 10.1071/MF9870001
Saikrithi P., Balasubramanian C. P., Otta S. K., Tomy S. (2019). Characterization and expression profile of farnesoic acid O-methyltransferase gene from Indian white shrimp, Penaeus indicus. Comp. Biochem. Physiol. B, Biochem. Mol. Biol. 232, 79–86. doi: 10.1016/j.cbpb.2019.03.004
Santander-Avanceña S. S., Traifalgar R. F. M., Laureta L. V., Monteclaro H. M., Quinitio G. F. (2021). Interactive influence of dietary protein and lipid on maturation of Indian white prawn, Penaeus indicus broodstock. Aquaculture Res. 52 (5), 2243–2253. doi: 10.1111/are.15076
Santander-Avanceña S. S., Monteclaro H. M., Estante-Superio Erish G., Catedral D. D., Ferdin R., Traifalgar M. (2022). The influence of monosex rearing on gonad maturation and reproductive behavior of Indian white prawn, Penaeus indicus broodstock. Aquaculture 552 (5), 738030. doi: 10.1016/j.aquaculture.2022.73803
Staples D. J., Heales D. S. (1991). Temperature and salinity optima for growth and survival of juvenile banana prawns Penaeus merguiensis. J. Exp. Mar. Biol. Ecol. 154, 251–274. doi: 10.1016/0022-0981(91)90168-V
Tan-Fermin J. D., Pudadera R. A. (1989). Ovarian maturation stages of the wild giant tiger prawn, Penaeus monodon fabricius. Aquaculture 77 (2–3), 229–242. doi: 10.1016/0044-8486(89)90205-6
Tomy S., Saikrithi P., James N., Balasubramanian C. P., Panigrahi A., Otta S. K., et al. (2016). Serotonin induced changes in the expression of ovarian gene network in the Indian white shrimp, Penaeus indicus. Aquaculture 452, 239–246. doi: 10.1016/j.aquaculture.2015.11.003
Treerattrakool S., Boonchoy C., Urtgam S., Panyim S., Udomkit A. (2014). Functional characterization of recombinant gonad-inhibiting hormone (GIH) and implication of antibody neutralization on induction of ovarian maturation in marine shrimp. Aquaculture 428, 166–173. doi: 10.1016/j.aquaculture.2014.03.009
Tsutsui N., Katayama H., Ohira T., Nagasawa H., Wilder M. N., Aida K. (2005). The effects of crustacean hyperglycemic hormone-family peptides on vitellogenin gene expression in the kuruma prawn, Marsupenaeus japonicus. Gen. Comp. Endocr. 144, 232–239. doi: 10.1016/j.ygcen.2005.06.001
Udoinyang E. P., Amali O., Iheukwumere C. C., Ukpatu J. E. (2016). Length-weight relationship and condition factor of seven shrimp species in the artisanal shrimp fishery of Iko river estuary, southeastern Nigeria. Int. J. Fish. Aquat. Stud. 4 (2), 109–114.
Vijayan K. K. (2019). Domestication and genetic improvement of Indian white shrimp, Penaeus indicus: A complimentary native option to exotic pacific white shrimp, Penaeus vannamei. J. Coast. Res. 86, 270–276. doi: 10.2112/SI86-040.1
Wen W., Yang Q., Ma Z., Jiang S., Qiu L., Huang J., et al. (2015). Comparison of ovarian maturation and spawning after unilateral eyestalk ablation of wild-caught and pond-reared penaeus monodon. Spanish J. Agric. Res. 13 (3), 8. doi: 10.5424/sjar/2015133-7860
Wilder M. N. (2019). Advances in the science of crustacean reproductive physiology and potential applications to new seed production technology. J. Coast. Res. 86), 6–10. doi: 10.2112/SI86-002.1
Wouters R., Lavens P., Nieto J., Sorgeloos P. (2001). Penaeid shrimp broodstock nutrition: an updated review on research and development. Aquaculture 202, 1–21. doi: 10.1016/S0044-8486(01)00570-1
Wyban J. (2007). Domestication of pacific white shrimp revolutionizes aquaculture. . Global Aquacult. Advocate 10 (4), 42–44. https://www.aquaculturealliance.org/.
Wyban J. (2019). Selective breeding of Penaeus vannamei: Impact on world aquaculture and lessons for future. J. Coast. Res. 86 (sp1), 1–5. doi: 10.2112/SI86-001.1
Yano I. (1993). “Ultraintensive culture and maturation in captivity of penaeid shrimp,” in CRC Handbook of mariculture, vol. 1. crustacean aquaculture. Ed. McVey J. P. (USA: CRC Press), 289–314.
Keywords: breeding, closed thelycum, domestication, environmental cue, Indian white shrimp, Penaeus indicus, pond-reared broodstock
Citation: Anand PSS, Balasubramanian CP, Aravind R, Biju IF, Rajan RV, Vinay TN, Panigrahi A, Sudheer NS, Rajamanickam S, Kumar S, Antony J, Ambasankar K, Jithendran KP and Vijayan KK (2023) Reproductive performance of captive-reared Indian white shrimp, Penaeus indicus, broodstocks over two generations. Front. Mar. Sci. 10:1101806. doi: 10.3389/fmars.2023.1101806
Received: 18 November 2022; Accepted: 03 February 2023;
Published: 07 March 2023.
Edited by:
Johan Verreth, Wageningen University and Research, NetherlandsReviewed by:
Asaduzzaman Md, Chattogram Veterinary and Animal Sciences University, BangladeshJorge Alfaro Montoya, National University of Costa Rica, Costa Rica
Ilie S. Racotta, Centro de Investigación Biológica del Noroeste (CIBNOR), Mexico
Copyright © 2023 Anand, Balasubramanian, Aravind, Biju, Rajan, Vinay, Panigrahi, Sudheer, Rajamanickam, Kumar, Antony, Ambasankar, Jithendran and Vijayan. This is an open-access article distributed under the terms of the Creative Commons Attribution License (CC BY). The use, distribution or reproduction in other forums is permitted, provided the original author(s) and the copyright owner(s) are credited and that the original publication in this journal is cited, in accordance with accepted academic practice. No use, distribution or reproduction is permitted which does not comply with these terms.
*Correspondence: P. S. Shyne Anand, c2h5bmUuYW5hbmRAZ21haWwuY29tOw==; U2h5bmUuQW5hbmRAaWNhci5nb3YuaW4=