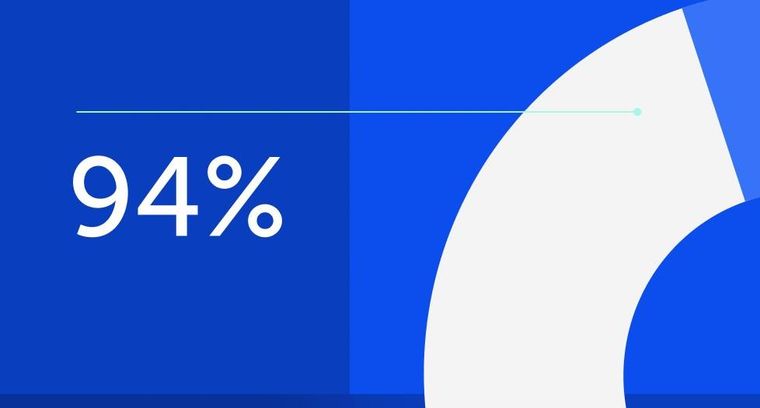
94% of researchers rate our articles as excellent or good
Learn more about the work of our research integrity team to safeguard the quality of each article we publish.
Find out more
ORIGINAL RESEARCH article
Front. Mar. Sci., 09 May 2023
Sec. Coastal Ocean Processes
Volume 10 - 2023 | https://doi.org/10.3389/fmars.2023.1101658
This article is part of the Research TopicProcesses and Management of Altered Estuaries and Deltas in the AnthropoceneView all 11 articles
The Nakdong Estuary of Korea is a dramatic example of an urban estuary that has been heavily developed with the installation of two estuarine dams and approximately 17 km2 of tidal flat reclamation. This study aims to understand the relative contribution of the different physical alterations by implementing four model simulations of the COAWST modeling system: (1) present condition, (2) pre-estuarine dam construction, (3) pre-tidal flat reclamation, (4) both pre-estuarine dam construction and pre-reclamation. In Cases 2 and 4, the model grid was modified to extend the Nakdong estuary upriver about 60 km and remove the estuarine dam. In Cases 3 and 4, the model grid was modified to restore the reclaimed tidal flats. All simulations shared the same initial conditions, boundary conditions, and forcing. Then, the simulation results for current velocity, suspended sediment concentration (SSC), and deposition were used to estimate the relative contribution of each alteration. The results showed that the annual, depth-averaged currents for the pre-estuarine dam (Case 2) were 80% faster than the present condition but were 10% slower for Case 3 (no land reclamation case). Likewise, the depth-averaged SSC was higher by about 25 mg/L in the estuary for Case 2, but it was reduced by about 9 mg/L for Case 3. The increase of velocity and SSC for Case 2 resulted from the removal of the estuarine dam, which changed both the tidal properties (tidal amplitude was reduced by about 40 cm) and estuarine circulation (factor of 5 reductions in the vertical salinity gradient), while the decrease for Case 3 was induced by the reduction in sediment accommodation space (15% of water volume) without a change in the circulation. These results also revealed that the estuarine dam enhanced deposition more than the land reclamation that showed very little change. The changes in current velocity, SSC, and deposition for Case 4 (no dam/reclamation) were very similar to Case 2. Overall, this study suggests that the influence of the estuarine dam was larger than that of the land reclamation in the altered Nakdong Estuary. Moreover, the combination of estuarine alterations acted to enhance the effects. These findings can serve as analogs that can be applied to other estuaries where various human alterations have occurred.
Estuaries are critical interfaces between rivers and the ocean, offering habitat for important species and acting as effective sediment traps (Guerry et al., 2012; Eidam et al., 2020). Because of their advantageous location at the land-ocean boundary, estuaries have undergone intensive development by humans to increase their utilization over the past centuries. The physical alterations of estuarine development have included land reclamation and dredging, as well as the construction of estuarine dams (French, 2001; Morris and Mitchell, 2013; Wolanksi and Elliott, 2015). Channel dredging has frequently been needed to facilitate ship navigation, and the dredged materials were often used to reclaim tidal flats to secure land for industrial, agricultural, and domestic purposes. Estuarine dams, while not as common as other alterations, have been constructed within the tidal limit to block salt intrusion and regulate freshwater discharge for agricultural, industrial, and domestic use (Williams et al., 2014).
Multiple human alterations have often occurred in a single estuary, each influencing the hydrodynamics and sediment dynamics. Previous studies have tried to characterize the relative influence of each of these combined human alterations. First, the influence of both land reclamation and dredging was revealed in The Ems River Estuary (Germany) which is an estuary that has undergone both alterations. Following those human changes, this estuary has experienced continuous siltation, necessitating additional dredging (de Jonge et al., 2014; van Maren et al., 2015a). The dredging contributed to this siltation by increasing tidal amplitude and reducing hydraulic drag, and then increasing the up-estuary sediment transport (van Maren et al., 2015b). On the other hand, land reclamation led to the reduction of sediment sinks by increasing suspended sediment concentration due to decreased accommodation space (van Maren et al., 2016). Similar processes were found in other European estuaries such as the Seine estuary (France) (Grasso et al., 2018; Grasso and Le Hir, 2019) and the Loire estuary (France) (Walther et al., 2012; Winterwerp and Wang, 2013). Second, dam construction and dredging occurred in the Brisbane River estuary (Australia), resulting in enhanced sediment trapping (Eyre et al., 1998). In other estuaries, it has been found that dam construction increased the trapping capacity of the estuary by reducing the freshwater flow, while dredging contributed to trapping by increasing the volume of the estuarine basin. Third, in the estuary where the dam construction and land reclamation were done, the dam construction reduced the tidal prism. This change reduced seaward transport but increased landward transport and deposition (Zhang et al., 2015; van der Spek and Elias, 2021). Meanwhile, land reclamation enhanced erosion by reducing flood dominance and decreasing landward transport caused by tidal pumping (Cheng et al., 2020). Additionally, both estuarine dam construction and land reclamation can influence wave reworking, causing the migration of sand shoals in front of the estuary (Barusseau et al., 1998; Gao et al., 2012; Jia et al., 2013).
Previous studies suggested that various human alterations have an influence on sediment transport with different intensities and effects depending on where the alterations occurred (Luan et al., 2016; Chu et al., 2022). These studies considered the responses that occurred after human alterations. Furthermore, despite its importance to not only the management of the estuarine environment but also the future restoration of the estuary, there has been no quantitative comparison of the influence of each alteration and the mechanism that caused the changes.
The Nakdong Estuary in South Korea provides a dramatic example of an estuary that has been significantly modified by human activities in its surrounding region, including dam construction and land reclamation. Over the last century, two major estuarine dams were built there to prevent saltwater intrusion. The first was the permanently closed Noksan Dam constructed in 1934 in the West Nakdong Estuary and the second was the Nakdong Estuarine Dam constructed between 1983-1987 in the East Nakdong Estuary to regulate river discharge. Approximately 17 km2 of the estuarine area was also reclaimed to create additional area for agricultural, industrial, and residential uses since 1927. Previous observational studies found that deposition in the West Nakdong Estuary and the East Nakdong Estuary differed significantly after those alterations. Deposition in the West Nakdong Estuary was gradual, at about 2-4 cm/yr, and surficial sediment was muddy; while in the East Nakdong Estuary deposition was rapid at 6 cm/yr and surficial sediment was sandy (Williams et al., 2013). Observations and a modeling study revealed that the East Nakdong Estuary was mostly affected by residual sediment flux convergence by river discharge, resulting in a rapid deposition during flood events, while the West Nakdong Estuary was affected by sediment flux convergence by tides and waves causing gradual deposition (Williams et al., 2015; Chang et al., 2020).
Although these studies have revealed sediment transport patterns that occurred after dam construction and land reclamation in the Nakdong Estuary, the relative contributions of the dam and land reclamation to the sediment transport and the quantitative comparison of sediment transport and morphological change between these alterations were still unclear due to the limitations of observations. Also, no studies have been conducted to understand the change in sediment transport mechanisms in response to those alterations in the Nakdong Estuary. To complement previous studies, this study aimed to quantitatively understand the relative contribution of the dam and land reclamation to increased sedimentation and changes to the sediment transport mechanisms throughout the Nakdong Estuary.
A numerical hydrodynamic and sediment transport model, the Coupled Ocean Atmosphere Wave Sediment Transport (COAWST) modeling system was implemented to achieve the objective by conducting and analyzing four model simulations: (1) present condition, (2) no estuarine dam construction, (3) no tidal flat reclamation, (4) both no estuarine dam construction and no tidal flat reclamation. The specific objectives to be answered by analyzing the model results are: (1) examine the relative intensity of change as a response to the estuarine dam construction and land reclamation by comparing hydrodynamics and sediment dynamics; (2) estimate the changes to sediment transport mechanism for each alteration by decomposing sediment flux; and (3) investigate the interaction between the dam and land reclamation.
The structure of this paper is as follows. Section 2 introduces the Nakdong Estuary in further detail, while Section 3 describes the four simulations. The model simulation results are presented in Section 4. Then, Section 5 discusses the model results in terms of different impacts of dam construction and land reclamation in sediment transport and mechanisms. Finally, the conclusions of this study are drawn in Section 6.
The Nakdong Estuary is the second longest river in Korea with a length of 510 km and a drainage basin area of approximately 23,384 km2 (Figure 1A). The Nakdong River bifurcates into two distributaries 20 km from the estuary mouth. The distributary that splits to the west flows about 22 km to form the West Nakdong and discharges to the offshore through the West Nakdong channel. The eastern distributary flows about 20 km to form the East Nakdong, and due to the Eulsuk Island, it leads to the offshore mainly through the East Eulsuk channel also known as the Main channel, with some split to the West Eulsuk channel (Figure 1B). There are eight sand shoals spread in front of the estuary blocking the external forcing from offshore.
Figure 1 Map of Nakdong Estuary with annotation of channels and cross-sections used for analysis. (A) Map of South Korea and location of Nakdong Estuary. (B) The major anthropogenic structures are referred to as the Noksan Dam, the Nakdong Estuarine Dam, and Noksan, Shinho, and Myungji reclamation. The three channels are referred to as the West Nakdong channel, the west Eulsuk channel, and the main channel following Williams et al. (2013). The main channel was used to reveal the responses of salinity distribution and stratification in Figure 4. (C) Cross-sections where the sediment fluxes were calculated. Positive means landward or eastward flux and the negative means seaward or westward flux.
The climate of the Nakdong Estuary is dominated by the East Asian monsoon. The summer climate is characterized by weak southeasterly winds and heavy precipitation, while relatively strong northwesterly winds and small precipitation occur during winter. Because of this monsoonal characteristic, about 68% of the annual precipitation occurs during the summer months between July and September, increasing freshwater discharge from the river (Park and Lee, 2016). The wave climate is also mainly controlled by the East Asian monsoon. Strong waves occur in summer when the wind blows into the estuary and weak waves occur in winter when the wind blows out from the estuary (Yoon et al., 2008). The Nakdong Estuary is classified as a microtidal, semi-diurnal system. The maximum tidal range reaches 1.5 m during a spring tide (Yoon and Lee, 2008).
The Nakdong Estuary has historically served as a habitat and as a resource for humans. The population living near this area increased from 5 million in 1966 to 7 million in 2017 (Kim, 2011). This increase has resulted in continuous development of the estuarine area. Estuarine dam construction and land reclamation are two significant anthropogenic alterations to the Nakdong Estuary within the last century.
Beginning in the 1930s, estuarine dams have been established in the Nakdong estuary to enable agricultural and industrial water usage. The Noksan Dam was the first to be built in 1934 in the West Nakdong River (Figure 1B) to save agricultural water by blocking the freshwater discharge, while the Nakdong Estuarine Dam was built in 1987 in the East Nakdong for agricultural and industrial purposes. The operation of these two estuarine dams showed significant differences, which led to different hydrodynamic environments on both sides of the Nakdong Estuary. The Noksan dam in the West Nakdong was permanently closed to save freshwater and discharged only when the water level inside of the dam was much higher than that of the sea to prevent flooding of farmland. On the other hand, the Nakdong Estuarine Dam in the East Nakdong consists of 10 radial-type main floodgates connected to the main channel to control freshwater discharge, and 5 floodgates connected to the West Eulsuk channel to support the main floodgates. Discharge primarily occurs through the main channel, and discharge through the West Eulsuk channel is only released when a large flood event occurs, such as the huge typhoon period that occurs less than twice a year, to support the main floodgates. During flood conditions, the floodgates are opened from the bottom to release freshwater, and the more the floodgates are opened from the bottom, the larger the amount of water discharged, containing both coarse sediments transported in bedload on the bottom and fine sediments transported in suspended load in the water column. These floodgates remain closed during dry conditions, and only a small amount of freshwater overflows from the top of the gates when the water level becomes higher than the dam gates; this freshwater carries only fine-grained sediment (Shin et al., 2019). As a result of the dam constructions, the West Nakdong became a low-energy regime lacking the influence of river discharge and the waves and tides became a major force to transport the sediment, causing the gradual deposition of surficial fine sediment. On the other hand, the East Nakdong was significantly controlled by river discharge from the Nakdong estuarine dam, causing dramatically rapid deposition during summer flood periods (Williams et al., 2013; Williams et al., 2015; Chang et al., 2020).
The other important human alteration which occurred in the Nakdong Estuary was land reclamation. Substantial areas of tidal flats were lost for industrial development and residential uses. Reclamation began in the 1990s in the Myungji and Noksan districts for developing residential and industrial complexes, respectively. The Myungji reclamation was conducted by solidifying the seaward edge of the island with approximately 3 km of seawalls. The Noksan reclamation was rapidly shifted from tidal flat to land by expanding the Shinho industrial port westward. As a result of reclamation, approximately 17 km2 of tidal flats (13 km2 in West Nakdong and 4 km2 in East Nakdong) had been lost since 1927, straightening the coastline.
A numerical hydrodynamic and sediment transport model, Coupled-Ocean-Atmosphere-Wave-Sediment Transport (COAWST) modelling system was applied to the Nakdong Estuary. In a previous study, COAWST was used to develop a model that reproduced the present-day sediment transport in the Nakdong Estuary and to reveal the mechanisms of sediment transport and morphological change (Chang et al., 2020). Here we expand on the previous study by designing three additional model scenarios to investigate the relative contributions of the estuarine dam and land reclamation to morphological change. In these additional model scenarios, the model configuration was modified to represent the Nakdong Estuary without the dam and/or land reclamation projects. This section provides the details of the designed model scenarios, briefly explains the model settings described more in Chang et al. (2020), and introduces how the model results were analyzed.
Four model scenarios were designed and implemented to investigate the relative contributions of dam construction and land reclamation. They included 1) present condition (Case 1: Present), 2) No estuarine dam construction (Case 2: No dam), 3) No-tidal flat reclamation (Case 3: No reclamation), 4) No-estuarine dam and No-reclamation (Case 4: No dam/reclamation).
Case 1 (Present; Figure 2A) represents the present-day condition of the Nakdong Estuary for which results are reported in Chang et al. (2020). For Case 2 (No dam; Figure 2B), the model grid was modified so that the estuarine grid in the main channel was removed, and the Nakdong Estuary was extended upstream by about 60 km to the Nakdong River (Figure 2C). The model grid retained the Noksan dam so that the West Nakdong was still blocked. This was because the Noksan dam has not been discharged to save agricultural water since its construction in 1934, while most human developments occurred in the late 1900s including the land reclamation of a massive tidal flat region and the construction of the Nakdong estuarine dam. The bathymetry of the Nakdong River was based on available bathymetric maps, while the bathymetry of the Nakdong Estuary was the same as in Case 1. The sediment sample data from a previous study was interpolated to derive the sediment fraction on the model’s riverbed (Kim et al., 2017). Bed sediment in the Nakdong River was dominated by sand (Figure 3A).
Figure 2 Description of the model domain of (A) Case 1 (Present), (B) The areas where the model results were mainly analyzed. (C) Case 2 (No dam) was extended to the Nakdong River without the Nakdong Estuarine dam while the Noksan dam was still closed, (D) Case 3 (No reclamation) without land reclamation of about 17 km2, (E) The areas where land reclamation occurred compared to Case 1. (F) Case 4 (No dam/reclamation) without both dam construction and land reclamation by combining the Case 2 and 3. The color is water depth for each scenario.
Figure 3 Reproduced sediment bed properties. (A) sand distribution of no dam scenarios (Cases 2 and 4) (top) and sediment fraction of designated points. (B) sand distribution of no reclamation cases (Case 1 and 3) (top) and sediment fraction of designated points. The area of the river added for the no-dam case was dominated by sand and the area of the flats added for the no-reclamation case contained similar fractions of sediment in the bed which were relatively equally distributed.
Case 3 (No reclamation) was designed to represent the system in the absence of the tidal flat reclamation projects in the Nakdong Estuary (Figure 2D). The reclaimed tidal flat was removed from the model grid based on the 1971 coastline (Figure 2E). Based on the historical records, about 13.4 km2 of tidal flats in the West Nakdong and only 3.6 km2 of tidal flats in the East Nakdong were restored in this scenario. The bathymetry of restored areas was recreated based on the bathymetric map from 1971 (Williams et al., 2015). Lacking observations, the sediment fraction in the restored area was obtained by interpolating the nearest sediment size distribution of the surrounding area (Figure 3B). Most of the restored tidal flats showed a similar sediment fraction with similar percentages of clay, silt, and sand.
Case 4 (No dam and no reclamation) was designed to reproduce pristine conditions that lack both the estuarine dam and reclamation (Figure 2F). The bathymetry and sediment fraction of the Nakdong River which was used for Case 2 and those of restored tidal flats for Case 3 were combined to represent hydrodynamic and sediment transport conditions prior to both estuarine dam construction and land reclamation.
The model grid included the Nakdong Estuary and the offshore region, and for Cases 2 and 4 the upstream Nakdong River was also included. The finest horizontal grid resolution was about 70 m in the estuary and grew coarser offshore. The resolution of the vertical grid increased within the estuary (0.1 m) and decreased at the open boundary (11 m). The model scenarios were run to represent sediment transport through one year (January 1 – December 31, 2011), and this period was selected because the observation and model validation was conducted in previous studies (Williams et al., 2015; Chang et al., 2020). All scenarios shared the same forcing including four different types: wind, river discharge, waves, and tides. The river discharge was concentrated during summer and occurred at the upstream river channel for Cases 2 and 4 without the estuarine dam construction, while the discharge was released at the location of the estuarine dam for Cases 1 and 3. The fluvial discharge data observed daily in the Nakdong estuarine dam for 2011 by the Korea Water Resources Corporation was used. The annual mean discharge was about 450 m3/s and the maximum reached 10,200 m3/s in July, accounting for 60% of discharge in summer. The sediment discharge increased corresponding to riverRechel discharge and the same amount of sediment was included for each scenario. The waves and tides for the scenarios were propagated from the boundary, sharing the same wave heightRechel and tidal range. All scenarios also shared the sameRechel initial conditions and boundary conditions to isolate the influence of estuarine dam construction and land reclamation. Like Chang et al. (2020), the model accounted for six sediment classes including two fluvial sediment classes (clay, silt) and fourRechel estuarine sediment classes (clay, silt, sand, washload sediment). Cohesive sediment processes such as flocculation and deflocculation were neglected in the sediment transport calculations following Warner et al. (2008).
The model was validated extensively by Chang et al. (2020) and performed reasonably well in reproducing the hydrodynamics and sediment dynamics in the Nakdong Estuary. The in site observation data for 2011 of tide, current velocity, and SSC were utilized to validate the model results (Williams et al., 2015). The model was validated at the point between the inlets and the point located in front of the Nakdong estuarine dam. The correlation coefficient reached about 0.96 for tide, 0.84 for current velocity, and 0.80 for SSC. And the skill value showed >0.91 for tide, >0.83 for current velocity, and >0.64 for SSC. For more details, readers can refer to Chang et al. (2020).
The model results were analyzed to quantify the influence of estuarine dam construction and land reclamation on hydrodynamics and sediment transport. The model results from the initial 14 days were excluded from the analysis to allow for the model to stabilize. To quantitatively evaluate the roles of estuarine dam construction and land reclamation, the annual and depth-averaged current velocity and suspended sediment concentration (SSC) were calculated and compared between scenarios (Figure 1B). Sediment flux was calculated as the product of velocity (u(z)) and SSC (c(z)). This sediment flux was integrated through the cross-sections representing the boundaries between the East and West Nakdong, offshore, and river (Figure 1C), and then time-integrated to estimate the net sediment transport through boundaries (Scully and Friedrichs, 2007; Sommerfield and Wong, 2011). The bed level change was calculated as the difference between the bed level after one year to that after the model was stabilized (14 days). The deposited sediment mass was also compared to estimate the depositional pattern of each scenario. The sediment mass deposited or eroded from the sediment bed was analyzed using the same procedure with bed level change and integrated spatially.
Another method was applied to compare the processes related to sediment transport and deposition. The water column stability determined by stratification and vertical mixing is an important factor that significantly affects the vertical diffusion of suspended sediment within the water column. The Richardson number (Ri) was calculated to compare water column stability:
This number was suggested by Richardson (1920) based on the relative magnitudes of vertical shear (du/dz) and vertical buoyancy frequency (N) where g is gravitational acceleration, ρ is water density, and u is current velocity. Previous studies provide the stability threshold number of 0.25 (Miles, 1961). Ri<0.25 is generally a sufficient condition for instability while Ri>0.25 insures stability. By multiplying the Richardson number by 4 and taking the log, positive values can be interpreted to indicate the presence of stratification, and negative values indicate a well-mixed water column.
To understand the underlying processes driving fluxes, many researchers have applied decompositions of flux (Dyer, 1997; Díez-Minguito et al., 2014; Becherer et al., 2016). This study used more advanced sediment flux decomposition suggested by Burchard et al. (2018), which consists of five components to estimate the relative importance of sediment transport mechanisms for each modeled scenario. The decomposition takes the following form:
Here, the prime (‘) and a tilde (~) denote the deviation of a tidal average and a vertical mean respectively. The []z represents depth-averaged values and < > the tidal-averaged values. Also []y represents the lateral-averaged values and []A the cross-sectional averaged values. The first term on the right-hand side is called the mean flux and represents the average transport which is usually down-estuary transport driven by river runoff, but can be influenced by other processes such as wind. The second term is referred to as the tidal pumping flux and is calculated as the tidal covariance of tidally averaged transport. This flux represents the depth-averaged sediment transport difference between flood and ebb tides. The third term was referred to as the estuarine circulation flux and represents the vertical covariance of tidally averaged transport. The fourth term was vertical and temporal covariance transport. This flux represents the difference in sediment transport between flood and ebb by tidal straining resulting from the destabilization of the water column. The last term was temporal depth covariance induced by Stokes transport.
The sediment flux decomposition analysis was completed to evaluate how anthropogenic modifications (land reclamation and damming) may have shifted the balance among the sediment flux mechanisms. All the model scenarios applied this decomposition to compare and examine the relative influence of estuarine dam construction and land reclamation on the sediment transport mechanism. For each model scenario, the decomposition was applied to three locations (the main channel, the West Nakdong channel, and the West Eulsuk channel) and during two different modeled events (tide and discharge events).
To evaluate the response of estuarine circulation and water column stability to estuarine dam construction and land reclamation, the salinity, Richardson Number, and vertical SSC distribution were compared between scenarios. Figure 4 shows the vertical salinity distributions along the main channel that were averaged over cross-sections and one tidal cycle on July 9, 2011, which represents the typical condition of freshwater discharge. The presence of the estuarine dam significantly influenced the salinity distribution, exhibiting similar distributions of salinity for Cases 1 and 3 (Figures 4B, D). The water column showed strong stratification with a large salinity gradient between the surface and bottom because of freshwater discharge from the estuarine dam. An exception was that the water column was well-mixed in front of the dam where the freshwater discharge occurred from the bottom. On the other hand, Cases 2 and 4 showed higher salinity in the main channel, ranging from 29 to 33 psu (Figures 4C, E). This increased salinity without vertical salinity gradient resulted from enhanced saltwater intrusion in the absence of the estuarine dam as well as enhanced mixing due to the increased current velocity.
Figure 4 The distribution of tidally averaged salinity (B–E), the Richardson Number (F-I), and suspended sediment concentration (SSC) (J–M) in the main channel for Case 1 (B, F, J), Case 2 (C, G, K), Case 3 (D, H, I) and Case 4 (E, I, M). The results were averaged on July 9, 2011, when there was river discharge corresponding to the annual mean value and mean tidal range (A). The x-axis indicates the distance up the main channel, with 6.5 km being the location of the present-day Nakdong Estuarine Dam. The blue in salinity (B–E) and SSC (F–I) means low salinity and SSC, while red means high salinity and SSC. The Richardson Number was scaled as (log10(4Ri)) so that positive values indicate stratified conditions, and negative values indicate well-mixed conditions.
Water column stability is determined by the density gradient and current velocity and is an important factor that controls both the vertical mixing and the vertical diffusion of suspended sediment from the bed. Figure 4 also shows the Richardson number, averaged over cross-sections and one tidal cycle, for model scenarios (Cases 1 to 4), and the presence or absence of the estuarine dam played an important role in water column stability. For scenarios with the estuarine dam (Cases 1 and 3), the Richardson number exceeded the critical value of 0.25 throughout the channel, implying that the water was strongly stratified due to the influence of freshwater discharge from the estuarine dam (Figures 4F, H). On the other hand, Cases 2 and 4 showed the Richardson number less than 0.25 in the channel especially in the mid-water column and surface waters, indicating that the layers were well-mixed (Figures 4G, I). This mixing resulted from increased saltwater intrusion and faster current velocities in the absence of the estuarine dam.
Figure 4J to 4M show the vertical distribution of SSC in the main channel, averaged over cross-sections and one tidal cycle, for Cases 1 to 4, respectively. The distribution of SSC was also significantly influenced by the estuarine dam construction. The SSC for Cases 1 and 3 was limited to the bottom of the water column due to the stratification (Figures 4J, L). In front of the dam (5-6.5 km), the suspended sediment was diffused to the water surface from the bottom (Figures 4J, L). In contrast, the scenarios without the estuarine dam (Cases 2 and 4) showed slightly higher SSC in the water column owing to the enhanced mixing in the water column (Figures 4K, M).
The current velocity and suspended sediment concentration were averaged over the depth and a year to quantitively compare the response of the hydrodynamics and sediment dynamics. Figure 5 shows the mean current velocities (arrows) and suspended sediment concentrations (pseudocolor) for 4 scenarios (Cases 1 to 4) and their values for the East and West Nakdong, and the whole estuary are tabulated in Table 1. The estuarine dam had a greater influence on the annual mean current velocity than land reclamation. The overall pattern of currents of Case 2 was similar to that of Case 1, but the magnitude increased from 0.088 m/s for Case 1 to 0.158 m/s for Case 2 (Figure 5B). This significant change in current speed mostly occurred in the East Nakdong, but the current speed remained similar in the West Nakdong. The current speeds for Case 4 were the fastest among the scenarios, but they were only slightly faster than those for Case 2 (Figure 5D; Table 1). It is noted that the velocity turned landward in the West Eulsuk Channel while the seaward velocity increased in the main channel, unlike the current directed seaward in both channels for Case 2. The current velocity for Case 3 didn’t change significantly, implying that the land reclamation had a weaker influence than the estuarine dam (Figure 5C; Table 1).
Figure 5 Depth-averaged and annual mean velocity (arrows) and suspended sediment concentration (SSC, pseudocolor) depending on the presence of the estuarine dam and land reclamation for (A) Case 1, (B) Case 2, (C) Case 3, and (D) Case 4.
Table 1 Comparison of hydrodynamics and sediment dynamics for each case. East and west denote the East Nakdong and West Nakdong estuary, respectively.
The suspended sediment concentration changed significantly by both the estuarine dam and the land reclamation. The SSC increased from 0.036 kg/m3 for Case 1 to 0.052 kg/m3 (44%) for Case 2 in the entire Nakdong estuary (Figure 5B; Table 1). The concentration on both sides of the Nakdong Estuary increased, though it was higher (88%) in the East Nakdong compared to a slight increase (10%) in the West Nakdong. A greater increase of SSC in the East Nakdong was consistent with the increase in the current velocity that occurred in the East Nakdong for the scenarios without the estuarine dam (Cases 2 and 4). On the other hand, the SSC of the entire estuarine area decreased by 42% from 0.036 to 0.021 kg/m3 for Case 3 (Figure 5C). This decrease in SSC occurred mainly in the West Nakdong (43% from 0.046 to 0.026 kg/m3). The SSC was the most enhanced for Case 4, which was about 10% larger than Case 2 (Figure 5D; Table 1). The increase in SSC occurred in both the East and West Nakdong and the response of SSC corresponded to the enhanced current velocity seen in Case 4.
The cross-sectionally integrated, time cumulative sediment flux was calculated through the five cross-sectional transects (shown in Figure 1C) to characterize the transport of sediment (Figure 6). The sediment fluxes for M4 and J2 appeared similar to other cross-sections in the East Nakdong and the West Nakdong, respectively, and they were excluded from Figure 6. See the supplementary material for all cross-sections (Figure S1). The sediment flux of M1 in front of the Nakdong estuarine dam was mainly directed seaward about 600 kt at the end of the year for Cases 1-3, but it increased to about 900 kt for Case 4 (Figure 6C). The increase in sediment flux was gradual most of the time, but the large sediment flux occurred during the large discharge events. M3 near the tidal inlet showed similar behavior to M1 but had a reduced magnitude of sediment flux (220 kt for Cases 1-3 and 400 kt for Case 4), indicating the convergence of sediment flux (Figure 6E). The cross-section WE, located in the West Eulsuk channel, exhibited different behavior in sediment flux (Figure 6D). No sediment flux occurred for Cases 1 and 3 because no discharge was allowed through the estuarine dam in the West Eulsuk channel. By removing the estuarine dam (Case 2), WE showed a similar pattern of sediment flux as shown in the East Nakdong, but at a reduced magnitude. On the other hand, the sediment flux was directed landward with the total integrated sediment flux of about 400 kt for Case 4. The change in the sediment flux coincided with the change in currents (Figure 5D). Overall, the removal of the dam appears to have a larger impact than the land reclamation in the East Nakdong estuary.
Figure 6 Cross-sectionally integrated cumulative sediment flux for estuarine cross-sections. (A) Tide range and wave height during 2011. (B) River discharge during 2011. Cross-sectionally integrated cumulative sediment fluxes are shown for cross-sections (C) M1, (D) WE, (E) M3, (F) J1, and (G) J3. For M1, WE, M3, and J1, positive sediment flux is directed up-estuary. For J3, positive sediment flux is directed eastward. The M4 cross-section appeared similar to other cross-sections in the East Nakdong (M1, M3, and WE), and J2 appeared to be similar to J1, so these cross-sections were omitted. For all cases and channels, the reader is referred to the supplementary material (Figure S1).
The cumulative sediment flux for the year was less in the West Nakdong than in the East Nakdong. Since no freshwater discharge was allowed from the estuarine dam in the West Nakdong, sediment was transported into the estuary through the inlet (J1) for all scenarios (Figure 6F). Of the four scenarios, the largest cumulative sediment flux (about 100 kt) occurred for Case 4. Cases 2 and 3 had smaller sediment fluxes about 50 kt and the smallest sediment flux was shown for Case 1. The cross-section at the western boundary of the West Nakdong (J3) had positive sediment flux except for Case 2, implying the gradual inflow of sediment (Figure 6G). Cases 1 and 3 delivered about the same amount of sediment across this transect, while this sediment flux decreased for Case 2 at about 10 kt. Notably, the inflow of sediment was amplified for Case 4, delivering 120 kt across transect J3. The responses of sediment flux in the West Nakdong indicated the influence of both the estuarine dam construction and land reclamation.
The presence and absence of the land reclamation and estuarine dam led to changes in hydrodynamics and sediment transport, which then changed depositional patterns. Figure 7 shows the bed level change over a year for all scenarios (Figures 7A–D) and the difference in bed level change between Case 1 and other scenarios (Figures 7E–G). The most significant bed level changes due to both erosion and accretion occurred in the tidal flats and the main channel for Case 1 (Figure 7A). The major bed level change in the West Nakdong resulted mainly from deposition, but the magnitude was smaller. The removal of the estuarine dam (Cases 2 and 4) significantly changed the bed level in the main channel. The overall pattern for Case 2 was similar to that for Case 1 (Figure 7B), but the erosion and accretion were intensified (Figures 7B, E). On the other hand, there was no noticeable change in the bed level in the West Nakdong between Cases 1 and 2. The bed level change due to the land reclamation was less than due to the estuarine dam construction. The patterns for the bed level change were quite similar between Cases 1 and 3 (Figures 7A, C), but different in the tidal inlets in general and most significant in the West Nakdong (Figure 7F). The pattern for the bed level change for Case 4 had combined features for both Cases 2 and 3 (Figure 7D). The resemblance in the main channel between Cases 2 and 4 and in the West Nakdong between Cases 3 and 4 is striking (Figure 7). However, the bed level change in the West Eulsuk channel for Case 4 didn’t correspond to any other cases. More erosion with a spatial mean of 15 cm appeared in the West Eulsuk channel for Case 4 in response to the combined effects of the estuarine dam and land reclamation.
Figure 7 The annual change of bed level and the difference between the present (Case 1) and each case. Top: Bed level change over a year for (A) Case 1, (B) Case 2, (C) Case 3, and (D) Case 4. Positive is bed deposition and negative is bed erosion. Bottom: (E) The difference in the annual bed level change between Case 1 and Case 2, (F) Case 1 and Case 3, and (G) Case 1 and Case 4. Positive represents greater deposition and negative represents greater erosion compared with the present case.
These changes in bed level in response to the presence/absence of the dam and land reclamation can be seen in the amount of sediment mass deposited. Table 1 tabulated the net amount of deposited sediment for each scenario in the West Nakdong, East Nakdong, and entire estuary. With the removal of the estuarine dam for Case 2, the decrease in deposited sediment was notable in the East Nakdong, amounting to a 91% reduction compared to Case 1. In the West Nakdong, the reduction also occurred, but it was smaller by 70%. In contrast, about 50% of deposition occurred in the West Nakdong for Case 3, while the East Nakdong estuary underwent a 12% reduction from Case 1. These changes were less significant than the changes for Case 2, reflecting that land reclamation had less impact on the deposition. The sediment mass for Case 4 showed the combined effects of both alterations. The deposited sediment mass decreased by about 80% in the East Nakdong, indicating the effect of the estuarine dam removal. On the other hand, about 70% of the sediment mass decreased in the West Nakdong, representing the restoration of the reclaimed tidal flats (Table 1).
Next, the depositional patterns for the river-derived sediment were analyzed for each model scenario. The percentage of river-derived sediment was calculated for 10 areas (1: Upstream, 1: Estuary, 8: Coastal/shelf) and shown in Figure 8 for each scenario. The washload sediment passed the river section without deposition and the majority of washload sediment (40-57%) settled in the inner estuary (Figure 8A). The remaining washload sediment was transported to the west and further offshore. As for Cases 1 and 3, more than 90% of silt and clay were deposited in the estuary and nearby coastal region (Figures 8B, C) and only a small amount of silt and clay escaped the estuary and dispersed to all other model domains. Without the estuarine dam (Cases 2 and 4), about 35% of clay was deposited in the river, and 25 and 19% of clay were deposited in the estuary and nearshore, respectively. The remaining 20% of clay was dispersed in all other coastal and shelf areas. The heavier silt was deposited in the river and estuary, totaling about 80%. About 10% of the silt was deposited in the nearshore and the remaining 10% of silt was dispersed in the coastal and shelf area (Figure 8C). These results suggested that the presence of the estuarine dam resulted in the increased retention of riverine sediment in the estuary and that the river sediment was more widely dispersed offshore in the absence of the estuarine dam.
Figure 8 Fraction of deposited (A) washload, (B) clay, and (C) silt discharged from the river (%) for the four scenarios for the domain divided into one river, one estuary, and eight coastal/shelf sub-environments. The percent of deposited river sediment in each sub-environment was represented by color for Case 1 (black), Case 2 (blue), Case 3 (red), and Case 4 (magenta).
The model results indicated that the estuarine dam construction and land reclamation caused different magnitudes of changes to current velocity, SSC, and depositional mass (Table 1). In general, the estuarine dam produced more significant impacts than the land reclamation. The model results showed that the influence of the estuarine dam was more significant in the averaged current speed in the Nakdong Estuary. The mean current velocity doubled when the estuarine dam was removed in the East Nakdong (Figure 9; Table 1). Similar changes in current speed were reported elsewhere when an estuarine dam was constructed (Kim et al., 2006; Zhu et al, 2011). On the other hand, land reclamation had a weak impact on current speed (Figure 9B). Our results correspond to the findings of Yang and Chui (2017) that the reclamation of about 14% of the total area reduced the current speed by only 9%.
Figure 9 The difference of annual and depth-averaged velocity, suspended sediment concentration (SSC), sediment flux, and bed level change over a year between (A) Case 1 (present) and Case 2 (no dam), (B) Case 1 and Case 3 (no reclamation), and (C) Case 1 and Case 4 (no dam/reclamation). Differences between velocity (arrows) and SSC (pseudocolor) are shown on the top row (A–C). Differences between sediment flux (arrows) and bed level change over a year (pseudocolor) are shown on the bottom row (D–F).
SSC was sensitive to the presence of the estuarine dam and land reclamation and the estuarine dam construction mainly impacted the East Nakdong, while impacts of reclamation were more significant in the West Nakdong (Figures 9A, B). The SSC change due to estuarine dam construction and reclamation had a similar magnitude of change, but with opposite trends. The increased SSC for the case that did not include the estuarine dam resulted from the change of hydrodynamics and sediment dynamics in the estuary in two main aspects. First was the enhanced vertical mixing and shear stress due to the increased current velocity, causing the resuspension of sediment from the bottom (Li et al., 2012; Burchard et al., 2019). The other important aspect was that the increased saltwater intrusion in the estuary decreased the vertical salinity gradient, resulting in well-mixed conditions and increased vertical dispersion of suspended sediment. On the other hand, the SSC decreased for the model run that did not include land reclamation even though the velocity was slightly increased (Table 1). This corresponded to previous studies that looked at the impact of land reclamation in other estuaries (van Maren et al., 2016; Gao et al., 2018; Cheng et al., 2020). The land reclamation affected sediment resuspension by changing the sediment sinks, resulting in a loss of accommodation space (Fountoukis and Nenes, 2005; van Maren et al., 2016).
The amount of sediment deposited indicated that the presence of the estuarine dam has had more influence than land reclamation on the increased sedimentation in the Nakdong Estuary. On the other hand, the depositional patterns in the East and West Nakdong responded differently to the combined effects of the estuarine dam and land reclamation (Case 4). In the East Nakdong, deposition was reduced, and the amplitude of reduction was larger than the result of the model scenario that only removed the estuarine dam (Table 1). Deposition in the West Nakdong was slightly increased for Case 4 relative to the scenario that only removed land reclamation (Case 3), implying the significant influence of land reclamation there. These results suggested that the location of the human alterations is important to the response of sediment dynamics, and also that the impact of these human alterations can be amplified by their interaction.
Comprehensively examining the above results, it was found that the change in sediment dynamic and morphological change due to the construction of the estuarine dam was relatively stronger than the change due to the land reclamation. Two major factors seem to have played a role in showing these results. The first is the type and location of the estuarine dam. The Nakdong estuary consists of the Noksan Dam, which does not discharge in the west, and the Nakdong estuarine dam, which controls the discharge in the east. In previous studies, this difference was reported to cause a change in sediment transport between the East and West Nakdong (Williams et al., 2013), and the effect of dam discharge was significant in the East Nakdong (Chang et al., 2020). The location of the dam also seems to have a significant impact. Figueroa et al. (2022a) showed that tidal currents vanish adjacent to estuarine dams due to the dead-end channel. For estuarine dams located less than the resonance length from the mouth, the effect of the dead-end channel will tend to reduce the tidal currents over a significant portion of the remnant estuary. The Nakdong estuarine dam is very close to the estuary mouth, only about 6 km upstream. This contributes to this region being greatly affected by the estuarine dam. The second is the location and extent of land reclamation. The land reclamation in the Nakdong Estuary is mainly focused in the West Nakdong and occurred at the estuary mouth. This reclamation at the mouth of the estuary leads to channel straightening to the effect that its shape more closely resembles a wave- or river-dominated estuary (Jung et al., 2021). This environmental change from a tide-dominated to a wave- or river-dominated estuary is corresponding to previous studies (Williams et al., 2013; Chang et al., 2020) and caused the increased influence of land reclamation in the West Nakdong compared to the East Nakdong.
To understand the changing sediment transport mechanisms due to the estuarine dam and land reclamation, the sediment fluxes were decomposed for the model scenarios. Figure 10 shows the decomposed sediment fluxes for tide, discharge, and wave events in the main channel. Here, only four decomposed sediment fluxes (mean, tidal pumping, estuarine circulation, and Stokes flux) along with the total flux are shown for Cases 1 and 2 because the tidal straining was very small, and Cases 3 and 4 were essentially the same as Cases 1 and 2, respectively. The full data can be found in the supplementary material (Figure S2). During the discharge event, the seaward mean flux due to the freshwater discharge is the only dominant mechanism, regardless of the presence of the estuarine dam, along the main channel (Figure 10). During the non-discharge period (tide event), the seaward mean flux was dominant with the presence of the estuarine dam (Case 1). With the removal of the estuarine dam (Case 2), the mean flux and the tidal pumping flux were dominant and directed seaward. The landward estuarine circulation flux increased and the Stokes flux also existed. The magnitude of these landward fluxes was smaller than the seaward mean and tidal pumping fluxes.
Figure 10 Time-averaged, cross-sectionally integrated sediment flux along-channel profiles in the main channel during tide and discharge events. Each panel shows the decomposed sediment fluxes (mean (A, F), Tidal pumping (B, G), Estuarine circulation (C, H), Stokes (D, I)) and total flux (E, J) for Case 1 (black) and Case 2 (blue) to represent the influence of the estuarine dam. For all cases and flux components, the reader is referred to the supplementary material (Figure S2).
The previous studies revealed that the change in the tidal pumping flux and the Stokes flux can be related to the change in tidal properties (e.g., Gao et al., 2014). To estimate the change in tidal properties, the tide amplitude was compared in the three channels and the tidal distortion was calculated by the ratio of M2 amplitude and M4 amplitude (Figure 11). The tidal asymmetry was calculated as log(uF/uE)3 where uF is the tidal current velocity during flood and uE is the velocity during ebb. The change in tidal properties after the construction of an estuarine dam affected sediment transport in two aspects.
Figure 11 Tidal properties in the three channels for different cases. Tide amplitude, distortion, and asymmetry are shown for (A) the main channel with Cases 1 and 2, (B) the West Nakdong channel with Cases 1, 2, and 4, and (C) the West Eulsuk channel with Cases 1 and 2. Tidal distortion was calculated as the ratio between M2 amplitude and M4 amplitude, and tidal asymmetry was calculated based on the tidal current during flood and ebb. For all cases and channels, the reader is referred to the supplementary material (Figure S3).
First, the change in tidal properties modified the Stokes transport. With the construction of the estuarine dam, the tide amplitude increased, especially the M2 amplitude was more amplified than M4. The distortion of the tidal wave was less pronounced due to this increased tidal amplitude (Zhu et al., 2021). The decreased tidal distortion resulted in the decrease of the phase difference between tidal current and sea surface height (Buschman et al., 2010). Therefore, the landward Stokes transport primarily generated by the shift from the mixed to standing wave was decreased with the tidal distortion. The reduction of tidal distortion also caused reduced asymmetry of tidal waves, causing reduced landward Stokes transport (Stanev et al., 2007; Guo et al., 2014; Burchard et al., 2018).
Second, this change in Stokes transport affected the tidal pumping flux. Without the estuarine dam, the landward Stokes transport caused landward water and momentum accumulation, generating a negative seaward water level gradient. This water level gradient induces a seaward return flow (Buschman et al., 2010; Guo et al., 2014), which in turn resulted in seaward tidal pumping (Guo et al., 2014; Moftakhari et al., 2016). The decreased landward Stokes drift after the construction of the estuarine dam caused the removal of seaward Stokes return flow, resulting in a decrease in the tidal pumping flux (Figueroa et al., 2022b). Unlike the tidal pumping and Stokes fluxes related to tidal properties, the estuarine circulation flux was directly related to the balance between baroclinic pressure gradient and friction (Geyer and MacCready, 2014; Hansen and Rattray Jr, 1966). This flux decreased with the estuarine dam construction due to the restricted landward bottom density gradient, resulting in decreased baroclinic pressure gradient (Shin et al., 2019). Therefore, the construction of the estuarine dam simplified the sediment transport mechanisms from a tide- and discharge-driven environment to a discharge-dominated environment.
To investigate the change in sediment transport mechanisms in the West Nakdong channel where the most land reclamation occurred, but no freshwater discharge was allowed, Figure 12 shows the decomposed sediment fluxes for tide and discharge events. Here, only three decomposed sediment fluxes (mean, tidal pumping, and Stokes flux) along with the total flux are shown for Cases 1 and 3 because the sediment fluxes of other components were insignificant, and Cases 2 and 4 were essentially the same as Cases 1 and 3, respectively. The total sediment flux in the West Nakdong channel was smaller than in the main channel and directed landward. The tidal pumping flux was the dominant component of the total sediment flux regardless of the land reclamation but was higher without the land reclamation (Figure 12b). Moreover, the tidal pumping flux was greatest at the inlet and decreased landward. The mean and Stokes fluxes also existed, but the magnitude was small. Because the West Nakdong had no river discharge and was far away from the main channel, the sediment flux during the discharge event was similar to the tide event but weaker.
Figure 12 Time-averaged, cross-sectionally integrated sediment flux along-channel profiles in the West Nakdong Channel during tide and discharge events. Each panel shows the decomposed sediment fluxes (mean (A, E), tidal pumping (B, F) and Stokes fluxes (C, G)) and total flux (D, H) for Case 1 (black) and Case 3 (red) to represent the influence of the land reclamation. For all cases and flux components, the reader is referred to the supplementary material (Figure S4).
In the West Nakdong where the river discharge was blocked in all model scenarios, the change in tidal properties was the main sediment transport mechanism, resulting in the change in the tidal pumping flux (Figure 11). Basically, the land reclamation caused the change in tidal properties by reducing the tidal storage (van Maren et al., 2023). The land reclamation of the shallow tidal flats resulted in a decrease in the estuarine volume and thus a change in tidal propagation pattern (Chu et al., 2022). Especially, the tidal propagation was accelerated, and the tidal amplitude was increased by increased convergence (Savenije et al., 2008; Van Rijn, 2011; Song et al., 2013; Zhu et al., 2016). The elimination of the tidal flat, where shallow water tidal components such as M4 tide were generated, resulted in more increase in the tidal amplitude of M2 than M4 (Li et al., 2018). The reduction of tidal distortion due to this decreased shallow water tidal components caused the decreased flood dominance in the estuary (Aubrey et al., 1988), which decreased the tidal pumping from offshore (Song et al., 2013; Liang et al., 2018). In addition, it is known that the response of the sediment transport can be different depending on the location of the reclaimed area (Liang et al., 2018). When the reclamation occurred near the inlet, the reduction of the surface area near the inlet promoted the transfer of energy from astronomical tide (such as M2) to shallow water tide (such as M4), enhancing flood dominance. On the other hand, the reclamation of the tidal flats inside the estuary restrained the tidal energy of shallow water tide and thus reduced the flood dominance, which corresponds to the case of the West Nakdong (Liang et al., 2018). This study implies that the location of the land reclamation is an important factor in estuarine sediment transport.
The hydrodynamic and sedimentary changes for Case 4 resulted from the combined effects of Cases 1 and 2. Case 4 showed similar characteristics of the removal of the land reclamation in hydrodynamic and sediment dynamics as Case 3 in the West Nakdong. On the other hand, the characteristics in the change of current velocity, SSC, and bel level for Case 4 were similar to those of Case 1 in the East Nakdong where the estuarine dam was removed. However, the amplitude of change in velocity and SSC was enhanced in the West Eulsuk channel and the cumulative sediment flux was directed landward with both alterations because of combined impacts by both alterations. Figure 13 shows the sediment flux of three components (mean, tidal pumping, and estuarine circulation) for two events (tide and discharge events) along the West Eulsuk channel for Cases 2 and 4. The mean flux was dominant and directed seaward for Case 2 during the discharge event, but the magnitude was smaller than the sediment flux in the main channel. However, the sediment flux was insignificant for all components for Case 4, except for the seaward mean flux at the estuarine dam (Figure 13E). For the tide event, the sediment fluxes were very small for all decomposed components for Case 2. However, both the mean flux and the tidal pumping components were a similar magnitude and directed landward during the tide event. The estuarine circulation was weak for Cases 2 and 4. Thus, the sediment flux for Case 2 showed a similar behavior in the main channel, but the sediment flux for Case 4 revealed a similar behavior in the West Nakdong.
Figure 13 Time-averaged, cross-sectionally integrated sediment flux along-channel profiles in the West Eulsuk Channel during tide and discharge events. Each panel shows the decomposed sediment fluxes (mean (A, E), tidal pumping (B, F) and estuarine circulation fluxed (C, G)) and total flux (D, H) for Case 2 (blue) and Case 4 (magenta) to represent the interaction between the estuarine dam construction and the land reclamation. For all cases and flux components, the reader is referred to the supplementary material (Figure S5).
In order to estimate the combined effect, the cross-sectionally averaged residual current and the cross-sectionally integrated water flux were calculated (Figure 14). During the tide event, the residual current flowed from the West Nakdong to the East Nakdong and the velocity was increased without the estuarine dam construction (Figures 14A, B). This enhanced residual current without the dam carried more water from the West Nakdong to the East Nakdong (Figures 14B, C). On the other hand, the reclamation reduced the residual velocity but the magnitude of change was smaller than the change without estuarine dam construction (Figures 14A, B). It is important that the amount of water flux without the land reclamation increased, even though the residual velocity was decreased, due to the expansion of the cross-section (Figures 14B, C). Therefore, the combined impact between the enhanced residual current without the dam and the increased water flux without land reclamation resulted in the dramatically increased westward mean flux, which continued to become a landward flux in the West Eulsuk channel. During the discharge event, the mean flux due to river discharge was offset with the mean flux from the West Nakdong without both estuarine dam and land reclamation, while the high mean flux occurred without only estuarine dam (Figure 14C–E). Therefore, the land reclamation amplified the mean flux enhanced by the estuarine dam construction during the tide event and offset during it during the discharge event.
Figure 14 Velocity and water flux in the Central Nakdong channel. (A) The area where velocity and water flux were calculated. The cross-section averaged velocity and integrated water flux were calculated along the longitude (line A-B). Cross-section averaged velocity from A to B during the tide event from the Nakdong Estuarine dam (B) and during the dam discharge event (D). Negative is westward velocity. Cross-section integrated water flux from A to B during the dam discharge event from the Nakdong Estuarine dam during the tide event (C) and the with dam discharge (E). Negative is westward water flux.
Figure 11 shows the change in tidal properties along the West Eulsuk channel. The tidal amplitude decreased for Case 4 and the tidal distortion and asymmetry were enhanced by the interaction of both alterations compared to either the estuarine dam or the land reclamation alone. Thus, the landward tidal pumping flux increased, as explained in the previous section, by the generation of the shallow water tide (section 5.2). With the removal of the estuarine dam, the tidal deformation induced a decrease in the tidal pumping by enhancing the seaward return flow due to Stokes drift (section 5.1). In the West Eulsuk channel, the Stokes drift was weak, and the tidal pumping was dominantly landward. These results imply that while the West Eulsuk channel was affected by both the estuarine dam and land reclamation, it was more influenced by the land reclamation during the tide event.
Unlike the mechanism of tidal pumping flux in the estuary without dam construction, the flux due to Stokes transport was not changed in the estuary without estuarine dam construction and reclamation (Figure 13). Previous studies have suggested that the process of changing the current velocity by the construction of the estuarine dam is also important (Zhu et al, 2011; Ralston et al., 2019). Without the estuarine dam, the increased current velocity feels more friction, especially with the extended bottom area without land reclamation (Helaire et al., 2019). The enhanced bottom stresses generate shallow water tides causing a decreased flood dominant asymmetry (Figure 11). Therefore, the hydrodynamic change due to the estuarine dam construction interacted with the change of estuary area due to land reclamation, resulting in landward tidal pumping flux.
Estuaries around the world have undergone significant human developments due to population growth and economic growth. Accordingly, many researchers have studied the effect of such human developments on sediment transport and morphological changes. In particular, the mean current velocity after the estuarine dam construction dramatically decreased (Kim et al., 2006; Zhu et al., 2011), resulting in a decrease in SSC (Li et al., 2012; Burchard et al., 2019). This change was mainly caused by reduced tidal prism (Zhang et al., 2015; van der Spek and Elias, 2021). After the land reclamation, the current velocity increased slightly (Yang and Qi, 2017) and tidal pumping decreased (Cheng et al., 2020). The decrease in SSC occurred dominantly due to a loss in accommodation space (Fountoukis and Nenes, 2005; van Maren et al., 2016). This study not only reflects the results of previous studies in other estuaries but also revealed new findings to improve knowledge about the influence of human developments on altered estuaries. This study suggests that even a single estuary was able to have different effects from different types of human developments depending on the location where they occur dominantly. In addition, the magnitude of changes caused by the presence of an estuarine dam and reclamation was quantitively compared, revealing that the influence of the presence of the dam had a more significant influence than that of the reclamation in the Nakdong Estuary, and the mechanisms of these changes were revealed by decomposing sediment flux. It was also found that the two human developments interacted with each other and caused intensified impacts.
These results can provide an analog for the impacts of development activities on similar estuaries of the world such as micro-tidal estuaries that has been significantly altered by human development. In particular, our results can be used in two areas: environmental impact assessment for the alteration of an estuary for industrial and residential purposes and for the restoration of altered estuaries. First, estuaries are under pressure for development in underdeveloped and developing countries. It is important to assess the impacts of development on sediment transport and morphological change in advance. Various studies have been used for these purposes (Kidd, 1995; Ballinger and Stojanovic, 2010) and this study can contribute to understanding not only the response of sediment transport but also the interaction between multiple alterations and sediment transport processes. Second, this study can provide considerable knowledge about the sedimentary responses for the restoration of an estuary where multiple human alterations already occurred. Although numerous studies have been conducted for the restoration of altered estuaries, many of them were focused on the improvement of fisheries (Bergstrom et al., 2004; Blomberg et al., 2018) and water quality problems (Smit et al., 1997; Balkis et al., 2010). On the other hand, studies on sedimentary changes for the restoration of an estuary were rare (George et al., 2012) because sedimentary and morphological responses take a relatively long period of time compared to chemical and biological responses. Numerical modeling is a suitable method to compensate for this limitation, and this study enabled us to predict the sedimentary changes that may occur after the restoration. Therefore, it is expected to contribute to efficient decision-making in restoring an estuary by quantitatively investigating the relative contribution of these alterations.
The goal of this study was to investigate the relative impacts of sediment transport on the estuarine dam and land reclamation in an altered Nakdong Estuary by using a numerical model and determine the change in sediment transport mechanisms by using sediment flux decomposition. Several important conclusions can be summarized.
1. The estuarine dam construction built in the East Nakdong to control the river discharge decreased by 80% of current velocity and increased vertical stratification by limiting the saltwater intrusion. These changes caused approximately 25 mg/L of suspended sediment reduction. The sediment from river discharge was settled in the Nakdong River and spread to the estuary and offshore. On the other hand, the land reclamation mainly occurred in the West Nakdong and was responsible for relatively smaller changes to current velocity with only a 10% increase, with an increase of 9 mg/L of suspended sediment due to a decrease in sediment accommodation space (15% of water volume). The sediment included in river discharge mostly settled in the inner estuary. These changes in current velocity and SSC resulted in a large change in deposition with the estuarine dams increasing deposition 8 times more compared to land reclamation, while land reclamation reduced deposition by a factor of 1.04 times. The result of this study suggested that the presence of the estuarine dam had more influence on the increased sedimentation in the whole estuary, while the influence of land reclamation was focused to the West Nakdong where the tidal flats mainly were converted to land.
2. A five-component sediment flux decomposition was used to understand the underlying mechanisms responsible for changes to sediment transport due to the estuarine dam and land reclamation. In absence of high river discharge, the sediment flux without the estuarine dam consisted of fluxes due to mean flow, tidal pumping, estuarine circulation, and Stokes transport. After dam construction, sediment flux was simplified into only mean flow due to the change in both tidal properties and stratification, resulting in other mechanisms being diminished. The tidal pumping flux was dominant in the West Nakdong, where most of the land reclamation occurred, without influence from the river discharge. Land reclamation decreased the tidal pumping due to the change in tidal properties, while it didn’t change the sediment transport mechanism in the East Nakdong. The Central Nakdong which was influenced by both the estuarine dam construction and land reclamation showed dramatically increased tidal pumping and mean flow suggesting the interaction between those alterations.
3. The sediment dynamics for the case that did not include either the estuarine dam or land reclamation was similar to that for the case that only removed the estuarine dam because the presence of the dam has a stronger impact than the land reclamation. However, the magnitude of the response of sediment dynamics and changes to the mechanisms were more amplified, indicating the importance of interactions between the estuarine dam construction and land reclamation. Especially, the high sediment flux occurred without the estuarine dam in the Central Nakdong, and the expanded Central Nakdong without land reclamation transported more sediment from the West Nakdong to the East Nakdong. The East Nakdong was mostly influenced by the estuarine dam construction and the West Nakdong was influenced more by the land reclamation ii.
The raw data supporting the conclusions of this article will be made available by the authors, without undue reservation.
JC: conceptualization, data curation, formal analysis, investigation, methodology, validation, visualization, writing-original draft preparation. G-HL: conceptualization, data curation, funding acquisition, investigation, project administration, supervision, validation, writing-review and editing. CH: investigation, methodology, validation, writing-review and editing. SF: investigation, validation, visualization, writing-review and editing. NJ: data curation, investigation, visualization. All authors contributed to the article and approved the submitted version.
This research was supported by Basic Science Research Program (2017R1D1A1B05033162) and Center for Anthropocene Studies (2018R1A5A7025409) through the National Research Foundation of Korea (NRF).
The authors declare that the research was conducted in the absence of any commercial or financial relationships that could be construed as a potential conflict of interest.
All claims expressed in this article are solely those of the authors and do not necessarily represent those of their affiliated organizations, or those of the publisher, the editors and the reviewers. Any product that may be evaluated in this article, or claim that may be made by its manufacturer, is not guaranteed or endorsed by the publisher.
The Supplementary Material for this article can be found online at: https://www.frontiersin.org/articles/10.3389/fmars.2023.1101658/full#supplementary-material
Aubrey D. G., Friedrichs C. T., Aubrey D. G., Weishar L. (1988). “Hydrodynamics and sediment dynamics of tidal inlets. lecture notes on coastal and estuarine studies,” in Seasonal climatology of tidal non-linearities in a shallow estuary, vol. 29. (New York, NY: Springer). doi: 10.1007/978-1-4757-4057-8_6
Balkis N., Müftüoğlu E., Aksu A., Sur H. I., Apak R. (2010). The chemical oceanographic consequences of environmental restoration projects in the golden horn estuary (Marmara Sea, Turkey). Environmen Monit. Assess 164 (1), 67–79. doi: 10.1007/s10661-009-0875-z
Ballinger R., Stojanovic T. (2010). Policy development and the estuary environment: a Severn estuary case study. Mar. pollut. Bull. 61 (1-3), 132–145. doi: 10.1016/j.marpolbul.2009.12.020
Barusseau J. P., Bǎ M., Descamps C., Diop E. S., Diouf B., Kane A., et al. (1998). Morphological and sedimentological changes in the Senegal river estuary after the constuction of the diama dam. J. Afr Earth Sci. 26 (2), 317–326. doi: 10.1016/S0899-5362(98)00014-1
Becherer J., Flöser G., Umlauf L., Burchard H. (2016). Estuarine circulation vs tidal pumping: sediment transport in a well-mixed tidal inlet. J. Geophys. Res. Oceans. 121(8), 6251–6270. doi: 10.1002/2016JC011640
Bergstrom J. C., Dorfman J. H., Loomis J. B. (2004). Estuary management and recreational fishing benefits. Coast. Manage. 32 (4), 417–432. doi: 10.1080/08920750490487430
Blomberg B. N., Pollack J. B., Montagna P. A., Yoskowitz D. W. (2018). Evaluating the US estuary restoration act to inform restoration policy implementation: a case study focusing on oyster reef projects. Mar. Policy 91, 161–166. doi: 10.1016/j.marpol.2018.02.014
Burchard H., Lange X., Klingbeil K., MacCready P. (2019). Mixing estimates for estuaries. J. Phys. Oceanogr 49, 10.1175/JPO–D-18-0147.1. doi: 10.1175/JPO-D-18-0147.1
Burchard H., Schuttelaars H. M., Ralston D. K. (2018). Sediment trapping in estuaries. Ann. Rev. Mar. Sci. Annu. Rev. Mar. Sci. 10, 371–395. doi: 10.1146/annurev-marine-010816-060535
Buschman F. A., Hoitink A. J. F., van der Vegt M., Hoekstra P. (2010). Subtidal flow division at a shallow tidal junction. Water Resour. Res. 46, W12515. doi: 10.1029/2010WR009266
Chang J., Lee G., Harris C. K., Song Y., Figueroa S. M., Schieder N. W., et al. (2020). Sediment transport mechanisms in altered depositional environments of the anthropocene nakdong estuary: a numerical modeling study. Mar. Geol. 430, 106364. doi: 10.1016/j.margeo.2020.106364
Cheng Z., Jalon-Rójas I., Wang X. H., Liu Y. (2020). Impacts of land reclamation on sediment transport and sedimentary environment in a macro-tidal estuary. Estuar. Coast. Shelf Sci. 242, 106861. doi: 10.1016/j.ecss.2020.106861
Chu N., Yao P., Ou S., Wang H., Yang H., Yang Q. (2022). Response of tidal dynamics to successive land reclamation in the lingding bay over the last century. Coast. Eng 173, 104095. doi: 10.1016/j.coastaleng.2022.104095
de Jonge V. N., Schuttelaars H. M., van Beusekom J. E. E., Talke S. A., de Swart H. E. (2014). The influence of channel deepening on estuarine turbidity levels and dynamics, as exemplified by the ems estuary. Estuarine Coast. Shelf Sci. 139, 46–59. doi: 10.1016/j.ecss
Díez-Minguito M., Baquerizo A., de Swart H. E., Losada M. A. (2014). Structure of the turbidity field in the guadalquivir estuary: analysis of observations and a box model approach. J. Geophys. Res. Oceans 119 (10), 7190–7204. doi: 10.1002/2014JC010210
Eidam E., Sutherland D. A., Ralston D. K., Conroy T., Dye B. (2020). Shifting sediment dynamics in the coos bay estuary in response to 150 years of modification. J. Geophys. Res. Oceans 126, 10.1029/2020JC016771. doi: 10.1029/2020JC016771
Eyre B., Hossain S., Mckee L. (1998). A suspended sediment budget for the modified subtropical Brisbane river estuary, Australia. Estuarine Coast. Shelf Sci. 47 (4), 513–522. doi: 10.1006/ecss.1998.0371
Figueroa S. M., Lee G., Chang J., Jung N. W. (2022b). Impact of estuarine dams on the estuarine parameter space and sediment flux decomposition: idealized numerical modeling study. J. Geophys. Res. Oceans 127 (5), e2021JC017829. doi: 10.1029/2021JC017829
Figueroa S. M., Son M., Lee G. (2022a). Effect of estuarine dam location and discharge interval on estuarine hydrodynamics, sediment dynamics, and morphodynamics. Front. Mar. Sci., 2168. doi: 10.3389/fmars.2022.1035501
Fountoukis C., Nenes A. (2005). Continued development of a cloud droplet formation parameterization for global climate models. J. Geophys. Res. 110, D11212. doi: 10.1029/2004JD005591
French P. W. (2001). Coastal defenses: processes, problems and solutions (Oxfordshire, UK: Routledge). doi: 10.4324/9780203187630
Gao J., Li J., Wang H., Bai F., Cheng Y., Wang Y. (2012). Rapid changes of sediment dynamic processes in yalu river estuary under anthropogenic impacts. Int. J. Sediment Res. 27 (1), 37–49. doi: 10.1016/S1001-6279(12)60014-6
Gao G. D., Wang H. W., Bao X. W. (2018). The impacts of land reclamation on suspended-sediment dynamics in jiaozhou bay, qingdao, China. Estuar. Coast. Shelf Sci. 206, 61–75. doi: 10.1016/j.ecss.2017.01.012
Gao G. D., Wang H. W., Bao X. W., Song D., Lin X. P., Qiao L. L. (2014). Land reclamation and its impact on tidal dynamics in jiaozhou bay, qingdao, China. Estuar. Coast. Shelf Sci. 151, 285–294. doi: 10.1016/j.ecss.2014.07.017
George D. A., Gelfenbaum G., Stevens A. W. (2012). Modeling the hydrodynamic and morphologic response of an estuary restoration. Estuaries coasts 35 (6), 1510–1529. doi: 10.1007/s12237-012-9541-8
Geyer W. R., MacCready P. (2014). The estuarine circulation. Annu. Rev. Fluid Mech. 46 (1), 175–197. doi: 10.1146/annurev-fluid-010313-141302
Grasso F., Le Hir P. (2019). Influence of morphological changes on suspended sediment dynamics in a macrotidal estuary: diachronic analysis in the seine estuary (France) from 1960 to 2010. Ocean Dyn 69, 83–100. doi: 10.1007/s10236-018-1233-x
Grasso F., Verney R., Le Hir P., Thouvenin B., Schulz E., Kervella Y., et al. (2018). Suspended sediment dynamics in the macro tidal seine estuary (France): 1. numerical modeling of turbidity maximum dynamics. J. Geophys. Res. Oceans 123, 558–577. doi: 10.1002/2016JC012638
Guerry A. D., Ruckelshaus M. H., Arkema K. K., Bernhardt J. R., Guannel G., Kim C.-K., et al. (2012). Modeling benefits from nature: using ecosystem services to inform coastal and marine spatial planning. Intl J. Biodivers. Sci Ecosys Serv Manag. 8(1-2), 107–121. doi: 10.1080/21513732.2011.647835
Guo L., van der Wegen M., Roelvink J. A., He Q. (2014). The role of river flow and tidal asymmetry on 1-d estuarine morphodynamics. J. Geophys. Res. Earth Surf 119 (11), 2315–2334. doi: 10.1002/2014JF003110
Hansen D. V., Rattray M. Jr. (1966). Gravitational circulation in straits and estuaries (Washington: University of Washington, Department of Oceanography, Seattle).
Helaire L. T., Talke S. A., Jay D. A., Mahedy D. (2019). Historical changes in lower Columbia river and estuary floods: a numerical study. J. Geophys. Res. Oceans 124 (11), 7926–7946. doi: 10.1029/2019JC015055
Jia L., Pan S., Wu C. (2013). Effects of the anthropogenic activities on the morphological evolution of the modaomen estuary, pearl river delta, China. China Ocean Eng.27 6), 795–808. doi: 10.1007/s13344-013-0065-1
Jung N. W., Lee G., Jung Y., Figueroa S. M., Lagamayo K. D., Jo T.-C., et al. (2021). MorphEst: an automated toolbox for measuring estuarine planform geometry from remotely sensed imagery and its application to the south Korean coast. Remote Sens 13 (330), 33. doi: 10.3390/rs13020330
Kidd S. (1995). Planning for estuary resources: the Mersey estuary management plan. J. Environ. Plan Manage. 38 (3), 435–442. doi: 10.1080/09640569512968
Kim J. (2011). The history of nakdong river development and the foundation of folklore. J. Kor Folk Soc 54, 99–128. doi: G704-000983.2011.54.009
Kim S., Ahn J., Jung K., Lee K., Kwon H., Shin D., et al. (2017). Contamination assessment of heavy metals in river sediments (For the surface sediments from nakdong river). J. Korean Soc Environ. Eng 33 (4), 460–473. doi: 10.15681/KSWE.2017.33.4.460
Kim T. I., Choi B. H., Lee S. W. (2006). Hydrodynamics and sedimentation induced by large-scale coastal developments in the keum river estuary, Korea. Estuar. Coast. Shelf Sci. 68 (3-4), 515–528. doi: 10.1016/j.ecss.2006.03.003
Li L., Guan W., Hu J., Cheng P., Wang X. (2018). Responses of water environment to tidal flat reduction in xiangshan bay: part I hydrodynamics. Estuar. Coast. Shelf Sci. 206, 10.1016/j.ecss.2017.11.003. doi: 10.1016/j.ecss.2017.11.003
Li P., Yang S. L., Milliman J. D., Xu K. H., Qin W. H., Wu C. S., et al. (2012). Spatial, temporal, and human-induced variations in suspended sediment concentration in the surface waters of the Yangtze estuary and adjacent coastal areas. Estuar. Coast. Shelf Sci. 35 (5), 1316–1327. doi: 10.1007/s12237-012-9523-x
Liang H., Kuang C., Olabarrieta M., Song H., Ma Y., Dong Z., et al. (2018). Morphodynamic responses of caofeidian channel-shoal system to sequential large-scale land reclamation. Cont Shelf Res. 165, 10.1016/j.csr.2018.06.004. doi: 10.1016/j.csr.2018.06.004
Luan H. L., Ding P. X., Wang Z. B., Ge J. Z., Yang S. L. (2016). Decadal morphological evolution of the Yangtze estuary in response to river input changes and estuarine engineering projects. Geomorphology 265, 21–23. doi: 10.1016/j.geomorph.2016.04.022
Miles J. (1961). On the stability of heterogeneous shear flows. J. Fluid Mech. 10, 496–508. doi: 10.1017/S0022112061000305
Moftakhari R. H., Jay D., Talke S. A. (2016). Estimating river discharge using multiple-tide gauges distributed along a channel. J. Geophys. Res. Oceans 121, n/a–n/a. doi: 10.1002/2015JC010983
Morris R., Mitchell S. (2013). Has loss of accommodation space in the Humber estuary led to elevated suspended sediment concentrations? J. Front. Constr Eng 2, 1–9.
Park H. B., Lee G. (2016). Evaluation of ADCP backscatter inversion to suspended sediment concentration in estuarine environment. Ocean Sci. J. 51, 1–17. doi: 10.1007/s12601-016-0010-3
Ralston D. K., Talke S., Geyer W. R., Al-Zubaidi H. A., Sommerfield C. K. (2019). Bigger tides, less flooding: effects of dredging on barotropic dynamics in a highly modified estuary. J. Geophys. Res. Oceans 124 (1), 196–211. doi: 10.1029/2018JC014313
Richardson L. F. (1920). The supply of energy from and to atmospheric eddies. Proc. R. Soc 97, 354–373. doi: 10.1098/rspa.1920.0039
Savenije H. H. G., Toffolon M., Haas J., Veling E. J. M. (2008). Analytical description of tidal dynamics in convergent estuaries. J. Geophys. Res. Oceans 113, https://doi. doi: 10.1029/2007JC004408
Scully M., Friedrichs C. (2007). Sediment pumping by tidal asymmetry in a partially mixed estuary. J. Geophys. Res. 112, 10.1029/2006JC003784. doi: 10.1029/2006JC003784
Shin H. J., Lee G., Kang K., Park K. (2019). Shift of estuarine type in altered estuaries. Anthropocene Coasts 2 (1), 145–170. doi: 10.1139/anc-2018-0013
Smit H., van der Velde G., Smits R., Coops H. (1997). Ecosystem responses in the Rhine-meuse delta during two decades after enclosure and steps toward estuary restoration. Estuaries 20 (3), 504–520. doi: 10.2307/1352610
Sommerfield C., Wong K. (2011). Mechanisms of sediment flux and turbidity maintenance in the Delaware estuary. J. Geophys. Res. 116, 10. doi: 10.1029/2010JC006462
Song D., Wang X., Zhu X., Bao X. (2013). Modeling studies of the far-field effects of tidal flat reclamation on tidal dynamics in the East China seas. Estuar. Coast. Shelf Sci. 133, 147–160. doi: 10.1016/j.ecss.2013.08.023
Stanev E. V., Brink-Spalink G., Wolff J. O. (2007). Sediment dynamics in tidally dominated environments controlled by transport and turbulence: a case study for the East Frisian wadden Sea. J. Geophys. Res. 112, C04018. doi: 10.1029/2005jc003045
van der Spek A. J. F., Elias E. P. L. (2021). Half a century of morphological change in the haringvliet and grevelingen ebb-tidal deltas (SW Netherlands) - impacts of large-scale engineering 1964-2015. Mar. Geol 432, 106404. doi: 10.1016/j.margeo.2020.106404
van Maren D. S., Beemster J. G. W., Wang Z. B., Khan Z. H., Schrijvershof R. A., Hoitink A. J. F. (2023). Tidal amplification and river capture in response to land reclamation in the Ganges-Brahmaputra delta. Catena 220, 106651. doi: 10.1016/j.catena.2022.106651
van Maren D. S., Oost A., Wang Z. B., Vos P. (2016). The effect of land reclamations and sediment extraction on the suspended sediment concentration in the ems estuary. Mar. Geol. . 376, 147–157. doi: 10.1016/j.margeo.2016.03.007
van Maren D. S., van Kessel T., Cronin K., Sittoni L. (2015b). The impact of channel deepening and dredging on estuarine sediment concentration. Cont Shelf Res. 95, 1–14. doi: 10.1016/j.csr.2014.12.010
van Maren D. S., Winterwerp J. C., Vroom J. (2015a). Fine sediment transport into the hyper-turbid lower ems river: the role of channel deepening and sediment-induced drag reduction. Ocean Dyn 65, 589–605.
Van Rijn L. C. (2011). Analytical and numerical analysis of tides and salinities in estuaries; part I: tidal wave propagation in convergent estuaries. Ocean Dyn 61, 1719–1741. doi: 10.1007/s10236-011-0453-0
Walther R., Schaguene J., Hamm L., David E. (2012). COUPLED 3D MODELING OF TURBIDITY MAXIMUM DYNAMICS IN THE LOIRE ESTUARY, FRANCE. Coast. Eng Proc. 1 (33), 22. doi: 10.9753/icce.v33.sediment.22
Warner J. C., Sherwood C. R., Signell R. P., Harris C., Arango H. G. (2008). Development of a three-dimensional, regional, coupled wave, current, and sediment-transport model. Comput. Geosci 34, 1284–1306. doi: 10.1016/j.cageo.2008.02.012
Williams J. R., Dellapenna T. M., Lee G. (2013). Shifts in depositional environments as a natural response to anthropogenic alterations: nakdong estuary, south Korea. Mar. Geol. 343, 47–61. doi: 10.1016/j.margeo.2013.05.010
Williams J. R., Dellapenna T. M., Lee G., Louchouarn P. (2014). Sedimentary impacts of anthropogenic alterations on the yeongsan estuary, south Korea. Mar. Geol. 357, 256–271. doi: 10.1016/j.margeo.2014.08.004
Williams J. R., Lee G., Shin H. J., Dellapenna T. M. (2015). Mechanism for sediment convergence in the anthropogenically altered microtidal nakdong estuary, south Korea. Mar. Geol. 369, 79–90. doi: 10.1016/j.margeo.2015.08.004
Winterwerp J. C., Wang Z. B. (2013). Man-induced regime shifts in small estuaries–I: theory. Ocean Dyn 63 (11-12), 1279–1292. doi: 10.1007/s10236-013-0662-9
Wolanksi E., Elliott M. (2015). Estuarine ecohydology: an introduction (Amsterdam: Elsevier Science).
Yang Y., Chui T. F. M. (2017). Hydrodynamic and transport responses to land reclamation in different areas of semi-enclosed subtropical bay. Cont Shelf Res. 143, 54–66. doi: 10.1016/j.csr.2017.06.008
Yoon E. C., Lee J. S. (2008). Characteristics of seasonal variation to sedimentary environment at the estuary area of the nakdong. J. Korean Soc Coast. Ocean Eng 20 (4), 372–389.
Yoon H., Park S., Lee I., Kim H. (2008). Spatiotemporal variations of seawater quality due to the inflow of discharge from nakdong river barrage. J. Korean Soc Mar. Environ. Energy 11, 78–85.
Zhang L., Wu B., Yin K., Li X., Kia K., Zhu L. (2015). Impacts of human activities on the evolution of estuarine wetland in the Yangtze delta from 2000 to 2010. Environ. Earth Sci. 73 (1), 435–447. doi: 10.1007/s12665-014-3565-2
Zhu C., Guo L., van Maren D. S., Wang Z. B., He Q. (2021). Exploration of decadal tidal evolution in response to morphological and sedimentary changes in the Yangtze estuary. J. Geophys. Res. Oceans 126 (9), e2020JC017019. doi: 10.1029/2020JC017019
Zhu Q., Wang Y. P., Ni W., Gao J., Li M., Yang L., et al. (2016). Effects of intertidal reclamation on tides and potential environmental risks: a numerical study for the southern yellow Sea. Environ. Earth Sci. 75 (23), 1472. doi: 10.1007/s12665-016-6275-0
Keywords: sediment transport mechanism, land reclamation, estuarine dam, relative contribution, the Nakdong Estuary, numerical model
Citation: Chang J, Lee G, Harris CK, Figueroa SM and Jung NW (2023) Relative contribution of the presence of an estuarine dam and land reclamation to sediment dynamics of the Nakdong Estuary. Front. Mar. Sci. 10:1101658. doi: 10.3389/fmars.2023.1101658
Received: 18 November 2022; Accepted: 05 April 2023;
Published: 09 May 2023.
Edited by:
Achilleas G. Samaras, Democritus University of Thrace, GreeceCopyright © 2023 Chang, Lee, Harris, Figueroa and Jung. This is an open-access article distributed under the terms of the Creative Commons Attribution License (CC BY). The use, distribution or reproduction in other forums is permitted, provided the original author(s) and the copyright owner(s) are credited and that the original publication in this journal is cited, in accordance with accepted academic practice. No use, distribution or reproduction is permitted which does not comply with these terms.
*Correspondence: Guan-hong Lee, Z2hsZWVAaW5oYS5hYy5rcg==
Disclaimer: All claims expressed in this article are solely those of the authors and do not necessarily represent those of their affiliated organizations, or those of the publisher, the editors and the reviewers. Any product that may be evaluated in this article or claim that may be made by its manufacturer is not guaranteed or endorsed by the publisher.
Research integrity at Frontiers
Learn more about the work of our research integrity team to safeguard the quality of each article we publish.