- 1Laboratory of Marine Organism Taxonomy and Phylogeny, Center for Ocean Mega-Science, Institute of Oceanology, Chinese Academy of Sciences, Qingdao, China
- 2Key Laboratory of Marine Sedimentology and Environmental Geology, First Institute of Oceanography, Ministry of Natural Resources, Qingdao, China
- 3Southern Marine Science and Engineering Guangdong Laboratory (Zhuhai), Zhuhai, China
- 4Pilot National Laboratory for Marine Science and Technology (Qingdao), Qingdao, China
- 5University of Chinese Academy of Sciences, Beijing, China
The Antarctic region plays a key role in regulating the Earth’s climate and contains a unique record of environmental change. Foraminifera, a group of shell-bearing protists, are widely used as paleoenvironmental proxies. However, core-based reconstructions of Antarctic paleoenvironments are often hindered by the lack of foraminiferal fossil record. Foraminiferal ancient DNA provides new avenues for understanding environmental change, but the correlation between molecular ecological features of foraminifera and environmental conditions remains poorly understood. Here, we obtained surface sediment samples from the Southern Ocean at water depths ranging from 50 to 4399 m and measured eight environmental variables. We generated a DNA metabarcoding dataset of foraminifera and presented the first assessment of relationships between foraminiferal molecular diversity and environmental variables in the Antarctic region. The results showed that the alpha diversity of whole community and abundant subcommunity was positively correlated with water depth and negatively correlated with temperature, chlorophyll a and pheophytin a, while the alpha diversity of rare subcommunity had no linear correlation with the above environmental variables. Both rare and abundant foraminiferal subcommunities exhibited distance-decay relationships, but only the beta diversity of rare subcommunity showed a significant positive correlation with water depth. This study reveals contrasting biogeographical patterns of abundant and rare foraminifera and their different correlations with Antarctic environmental variables, holding promise to provide more proxies for reconstructing past environments using foraminiferal ancient DNA and more information for predicting the impact of future environmental changes on polar biodiversity.
1. Introduction
The Antarctic region contains multiple extreme environmental conditions, including low temperatures, freeze-thaw cycles, ultra-oligotrophic conditions, wind abrasion and high radiation levels (Rosa et al., 2020). Its surrounding ocean current, the Antarctic Circumpolar Current, is the largest current in the world and interacts with the Pacific, Atlantic, and Indian Oceans through deep-water circulations (Barker and Thomas, 2004). Such a unique environment in the Antarctic region shapes its diverse and unusual marine fauna (Griffiths, 2010) and provides an exceptional field laboratory for studying the relationship between biological communities and environmental variability. However, research on Antarctic biodiversity has been largely limited by the relative inaccessibility of the region due to its remoteness, thick ice sheets and harsh weather conditions (Griffiths, 2010). The Southern Ocean seafloor covers an area of 34.8 million km², of which over 85% are deeper than 1000 m (Clarke and Johnston, 2003), but most benthic samples taken for investigations are from depths of less than 500 m (Griffiths, 2010). Compared with its shallow-water benthic communities, little is known about benthic biodiversity in the vast deep-sea area of the Southern Ocean (Janosik and Halanych, 2010; Brandt and Gutt, 2011).
Foraminifera, a group of shell-bearing protists, are significant components of the marine ecosystem in the Antarctic region (Mikhalevich, 2004). They can rapidly respond to environmental changes because of their unicellular organization and relatively short life cycles, and their hard shells can be preserved as fossils in marine sediments across geological timescales (Goldstein, 1999; Bouchet et al., 2012). For these reasons, foraminifera become efficient proxies for reconstructing past environmental conditions (Gooday, 2003). The study of foraminifera in the Antarctic region can date back to the nineteenth century, but ecological investigations of foraminifera are mostly based on the morphological characters of their calcareous, agglutinated, or organic tests (Majewski, 2010). Molecular techniques offer an alternative approach to assess foraminiferal diversity in marine environments (Pawlowski et al., 2002). Habura et al. (2004) revealed unexpected foraminiferal diversity in the sediment samples collected from shallow Antarctic stations using DNA sequencing method. With the advent of ultra-deep sequencing technology, Lecroq et al. (2011) assessed foraminiferal richness using Illumina sequencing technology and unveiled hidden diversity of early monothalamous lineage in deep-sea sediments of the Southern Ocean. These findings revealed the immense richness of unexplored foraminiferal phylotypes and improved understanding of foraminiferal diversity in the Antarctic region.
The application of foraminifera in previous paleoenvironmental studies was mainly based on their fossil tests. However, most calcareous foraminifera in the Antarctic region are limited to relatively shallow water depths, whereas below the carbonate compensation depth (CCD), foraminiferal assemblages are dominated by agglutinated taxa, which are poorly preserved in fossil record and are of little use for paleoenvironmental studies (Majewski et al., 2018).
Ancient DNA (aDNA) provides new ways to reconstruct past environmental changes using foraminifera (Pawłowska et al., 2016). Numerous studies report the preservation of foraminiferal aDNA in marine sediments over thousands of years (Lejzerowicz et al., 2013; Pawłowska et al., 2014; Szczuciński et al., 2016). Recently, Pawłowska et al. (2020) retrieved aDNA sequences of the planktonic foraminifera Neogloboquadrina pachyderma from a 140,000-year-old sediment core and found that the genomic variations of N. pachyderma could reflect the palaeoceanographic changes. Additionally, foraminiferal aDNA has been shown to be a useful complementary indicator of palaeotsunami deposits, especially in the absence of foraminiferal tests (Szczuciński et al., 2016). However, our knowledge of the biogeographical patterns and driving mechanisms of foraminifera and the environmental information documented in foraminiferal DNA data remains insufficient.
Biological communities are normally composed of abundant and rare species (Logares et al., 2014), but previous molecular ecological studies on foraminifera have mostly focused on the whole community and rarely compared the ecological properties of abundant and rare taxa. Increasing studies have shown that abundant and rare subcommunities tend to have contrasting biogeographical patterns and different environmental responses, which are seen in both prokaryotes and eukaryotes, such as marine microeukaryotes (Logares et al., 2014), pelagic bacteria (Liu et al., 2015), planktic eukaryotes (Xue et al., 2018), benthic bacteria (Jiao et al., 2017; Hou et al., 2020). In-depth analysis of the biogeographical patterns of abundant and rare foraminifera and their responses to environmental variables has the potential to provide more accurate proxies for future reconstruction of paleoenvironments using foraminiferal ancient DNA.
In this study, we collected surface sediment samples from ten sites between 50 to 4399 m in the Southern Ocean and obtained a DNA metabarcoding dataset of foraminifera using high-throughput sequencing technology. The specific goals of this study are listed as follows: (1) to analyze the ecological characteristics of foraminiferal assemblages in the Antarctic region based on DNA data; (2) to compare and determine the biogeographical patterns and driving mechanisms of abundant and rare foraminiferal subcommunities in the Antarctic region; (3) to separately assess the correlations of whole community, abundant and rare subcommunities with multiple environmental variables in the Antarctic region.
2. Materials and methods
2.1. Study sites and sediment collection
We collected sediment samples from ten sites between 50 to 4399 m in the Southern Ocean based on the 34th Chinese Antarctic Scientific Expedition from January to February 2018 onboard the R/V “Xiangyanghong 01” (Table S1). The study areas are in the convergence region of the Weddell Sea and the Scotia Sea, close to the Antarctic Peninsula (Figure 1). Four sites (ANT01 to ANT04) with water depths ranging from 50 m to 340 m are situated on the South Orkney Plateau. Three sites (ANT05, ANT06 and ANT07) with water depths ranging from 440 m to 1052 m are close to the King George Island, the largest island of the South Shetland Islands archipelago. ANT08 (2551 m) and ANT09 (3389) belong to the Weddell Sea, near the east side of the South Orkney Plateau. ANT10 is the deepest of all study sites, with a water depth of over 4300 m, and it is close to the north of the South Orkney Plateau, belonging to the Scotia Sea. At each site, one sediment sample was collected using a box corer (0.5 × 0.5 × 0.84 m3), and undisturbed 0-1 cm surface sediments were recovered from the box corer with a clean spoon. We took three subsamples from one sediment sample and separately transferred them to a sealable polyethylene bag. All samples were frozen at −80° C on board until further processing.
2.2. Measurement of physicochemical properties
Eight environmental variables were measured for each sample, including water depth, temperature, salinity, chlorophyll a (Chl a), pheophytin a (Pheo a), total nitrogen (TN), total carbon (TC) and total organic carbon (TOC). The water depth, in situ temperature and salinity were measured using a shipborne Sea-Bird Electronics 911plus Conductivity-Temperature-Depth (CTD) profiler. The obtained CTD data were processed using the standard processing procedure of SBE Company. The concentrations of Chl a and Pheo a in sediment samples were analyzed using a Turner Designs (Model II) fluorometer (Trilogy, USA). Total nitrogen, total carbon and total organic carbon in the samples were determined by a vario MACRO cube elemental analyzer (Elementar, Germany).
2.3. DNA extraction, PCR amplification and illumina sequencing
Total environmental DNA (eDNA) was extracted from ca. 0.25 g sediment using DNeasy PowerSoil kit (QIAGEN, Germany) and three eDNA extracts were required for each subsample. The foraminiferal-specific 37F hypervariable region of 18S rRNA gene was amplified using the foraminiferal-specific primers s14F3 and s17 following the amplification reaction volume and PCR program described by Li et al. (2020). For Illumina sequencing, tagged forward and reverse primers with 6-nt sequences appended at their 5′-end were used to multiplex multiple samples into a single library. A unique combination of tagged primers was designed for each subsample. PCR products were detected by agarose gel electrophoresis and purified with E.Z.N.A Gel Extraction Kit (Omega Bio-Tek, USA). The sequencing libraries were prepared with TruSeq DNA PCR-Free Sample Preparation Kit (Illumina, USA) following the manufacturer’s protocol and assessed using the Agilent Bioanalyzer 2100 system. The Illumina HiSeq 2500 platform was used to sequence libraries and generated 250 bp paired-end reads. The datasets presented in this study can be found in online repositories. The names of the repository/repositories and accession number(s) can be found below: BioProject, PRJNA908891.
2.4. Quality control and processing of sequencing data
After we obtained raw paired-end reads from the Illumina sequencing platform, we performed data processing as described by Li et al. (2020). We demultiplexed raw paired-end reads to samples based on their unique barcodes. Low-quality paired-end reads were discarded, and the barcode and primer sequences of the remaining reads were cut off. We merged paired-end reads using FLASH (Magoč and Salzberg, 2011), and filtered the spliced reads using QIIME (Caporaso et al., 2010) according to its quality-controlled process. Denoising on the retained reads was performed using the UNOISE3 algorithm in USEARCH (Edgar, 2016) and the correct biological sequences (representative sequences of OTUs) were picked out. All effective reads were clustered into OTUs with the otutab command in USEARCH according to the representative sequences of OTUs, and taxonomic assignment of OTUs was performed based on the Protist Ribosomal Reference (PR2) database (Guillou et al., 2013) using BLAST. Specific parameters and more detailed information on data processing can be found in Li et al. (2020). Planktonic foraminiferal OTUs and the OTUs that could not be assigned to foraminifera were discarded from the dataset. The OTUs that were assigned to foraminifera but had an identity value below 90% were placed into the group of Unknown Foram. The retained OTUs were classified as abundant or rare in relation to their relative abundance in all sites. The OTUs occupied >0.1% of total reads were defined as “abundant taxa” (Logares et al., 2014; Liu et al., 2015; Wang et al., 2021), those with reads proportions <0.01% were defined as “rare taxa” (Zhao et al., 2021), and the remaining OTUs were defined as “intermediate taxa”.
2.5. Statistical analysis
Proportions of OTUs and reads belonging to different taxonomic groups at the class level (Figure 2) and taxonomic composition at the order level in the ten sites (Figure S2) were visualized using the R package ggplot2 (Wickham, 2016). The variation trends of the seven environmental factors along water depth were shown in Figure 3, and their correlations with water depth were analyzed by the Spearman’s Rank Correlation Test. The phylotype richness was represented by the number of observed OTUs. Shannon index and phylotype richness were selected as indicators of alpha diversity (α-diversity) referring to previous studies (Zeng et al., 2019; Li et al., 2020; Kang et al., 2021). The correlations between Shannon index and phylotype richness and water depth (Figure 4A) and temperature (Figure 4B) were tested by Pearson correlation statistic and visualized using OriginPro 9.0 software packages (OriginLab Corporation, USA). Before calculating two α-diversity indices, we normalized the data of each sample corresponding to the sample with the least reads. A mantel test was conducted using the R package vegan to determine the relationship between the Bray-Curtis dissimilarity and water depth, geographic distance and environmental factors (Chl a and Pheo a) (Figure 5). Variation partitioning analysis (VPA) was used to evaluate the proportion of community variation explained by water depth and temperature in abundant and rare subcommunities (Figure S3). Beta diversity (β-diversity) of abundant and rare subcommunities was partitioned into two components: nestedness and species turnover by applying Baselga’s approach to assess their contribution to community dissimilarities (Table S3).
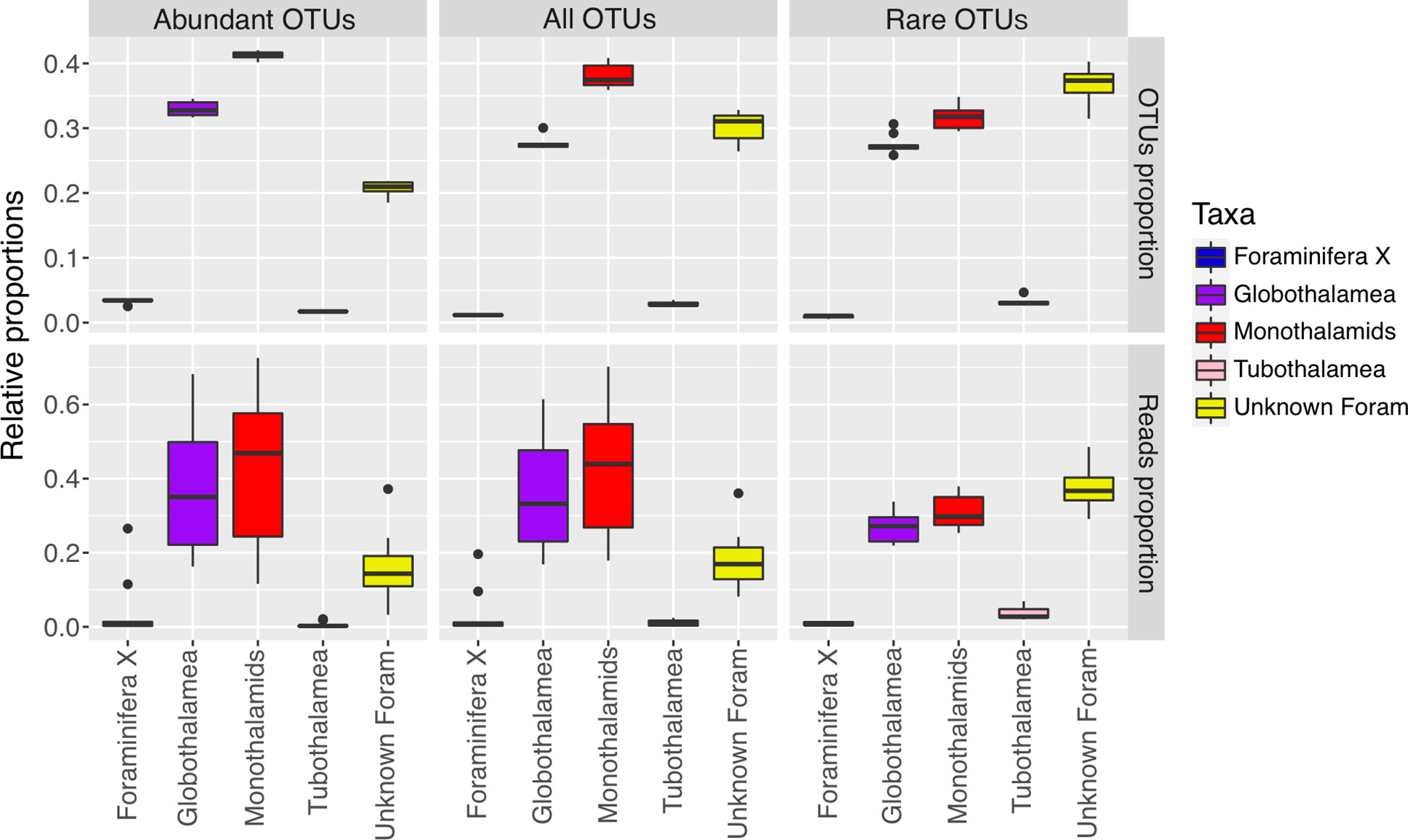
Figure 2 Proportions of OTUs and reads assigned to different taxonomic groups at the class level in abundant, rare and all taxa.
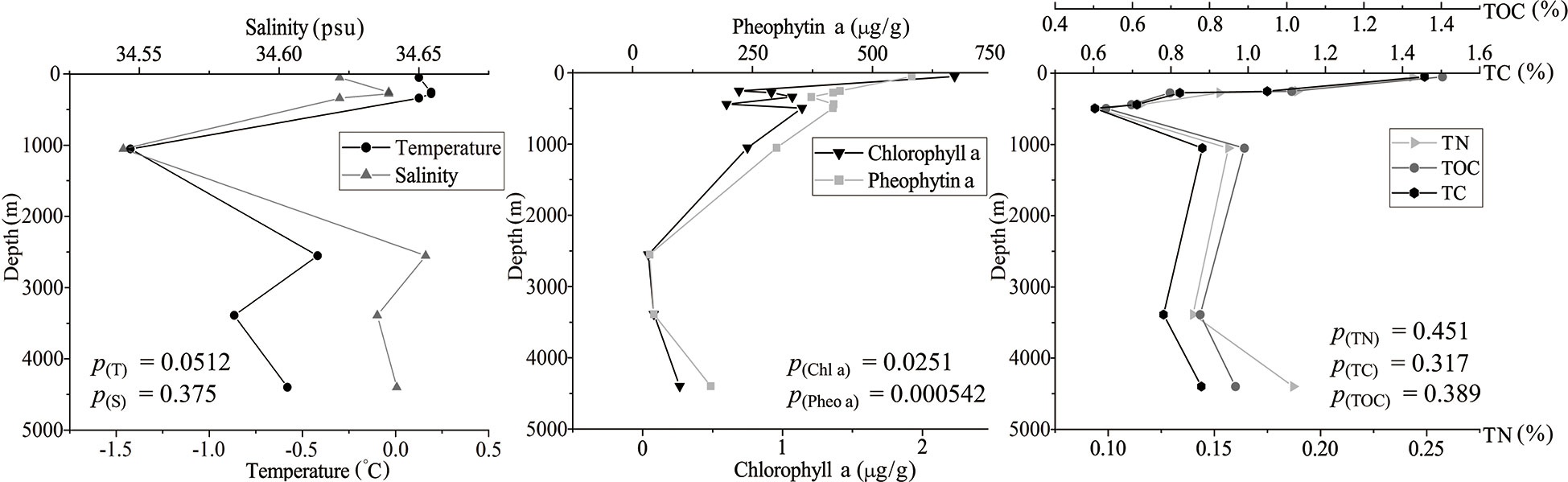
Figure 3 Profiles of bottom seawater temperature, salinity, and concentrations of chlorophyll a, pheophytin a, total nitrogen, total carbon and total organic carbon in the sediment samples. The correlations between the seven environmental variables with water depth were analyzed by the Spearman’s Rank Correlation Test. The results of statistical significance tests are shown at the bottom of each panel.
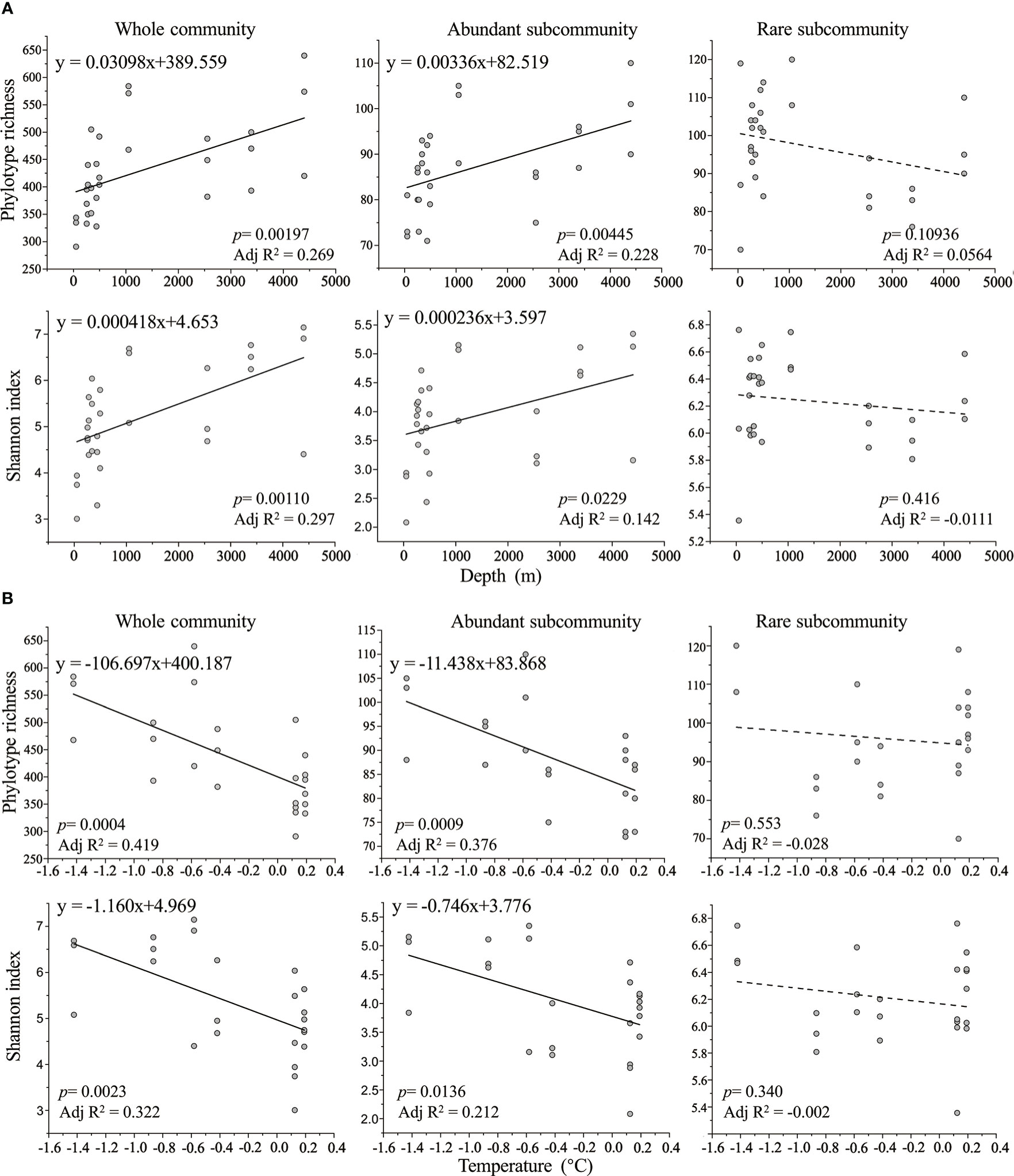
Figure 4 The correlations between foraminiferal alpha diversity estimated by phylotype richness and Shannon index and water depth (A) and temperature (B).
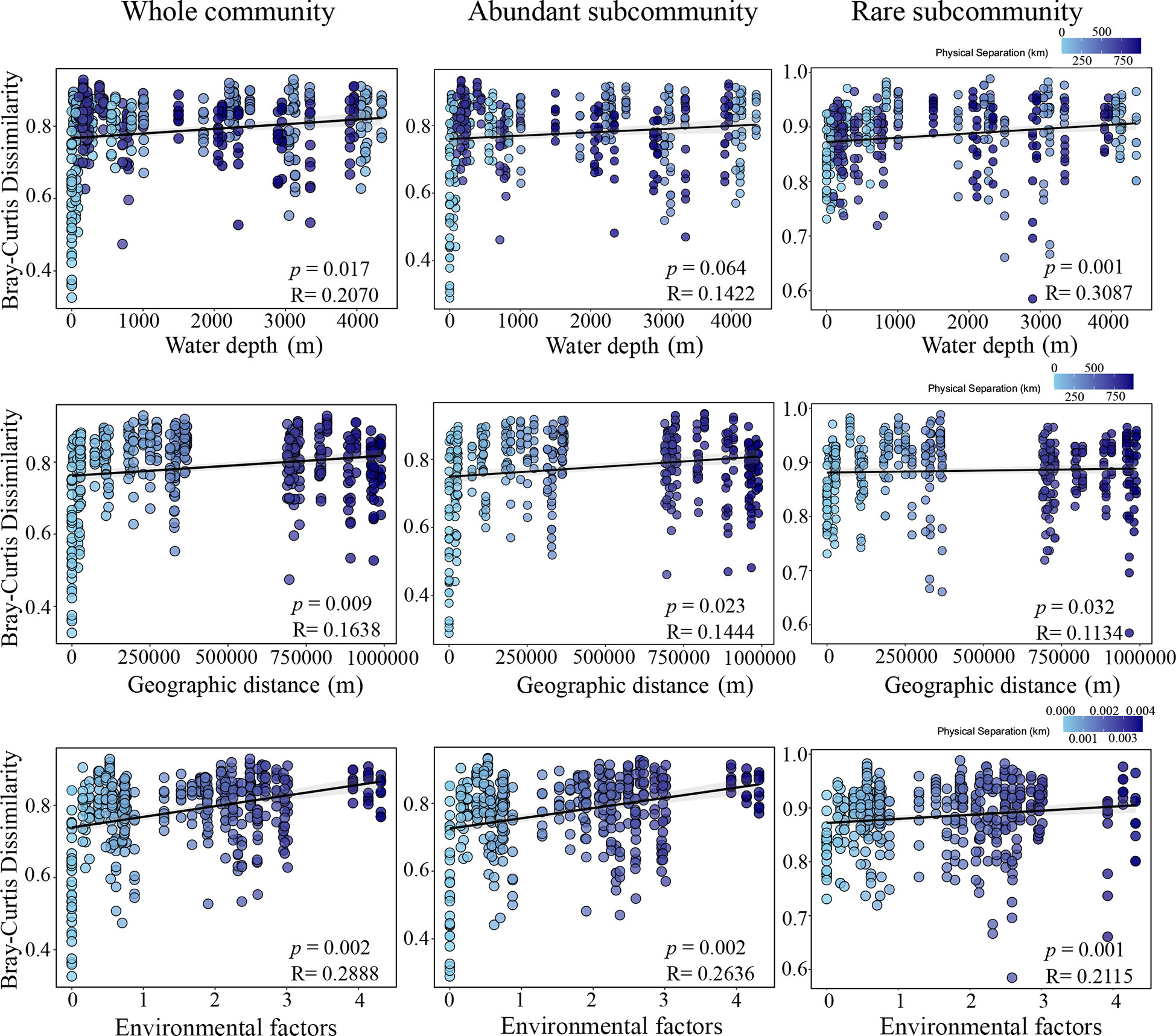
Figure 5 Mantel test results between Bray-Curtis dissimilarity of whole community, abundant and rare subcommunities with water depth, geographic distance and environmental factors (chlorophyll a and pheophytin a).
3. Results
3.1. Overview of sequencing data
In our study, a total of 898,437 effective reads were clustered into 1322 initial OTUs. After taxonomic assignment, 35 OTUs that could not find a hit in the PR2 database and 14 OTUs which were attributed to planktonic foraminifera were removed (Table S2). Finally, 1273 OTUs representing 894,160 reads were retained for downstream analysis. Among the 1273 OTUs that were assigned to benthic foraminifera, 410 OTUs had a sequence similarity of less than 90% to the reference sequences in the PR2 database and were placed to the group of Unknown Foram, 471 OTUs were assigned to the paraphyletic clade monothalamiids, 344 OTUs were assigned to the class Globothalamea, 35 OTUs were assigned to the class Tubothalamea, and 13 OTUs were a part of Foraminifera-X.
3.2. General composition and distribution patterns of foraminiferal assemblages
Among the 1273 OTUs, 121, 541 and 611 OTUs were placed to the abundant taxa, intermediate taxa and rare taxa, representing 724650, 139798 and 29712 reads, respectively. The abundant taxa accounted for 9.5% of all OTUs but occupied 81.0% of reads. Conversely, the rare taxa constituted the highest proportion of OTUs (48.0%), while their reads contributed to only 3.3% of total reads (Figure S1). Abundant and rare foraminiferal taxa exhibited distinct compositional differences at the class level (Figure 2). For abundant taxa, soft-shelled monothalamous foraminifera occupied the highest proportion of both OTUs (41%) and reads (42%), with only 20% of OTUs and 16% of reads belonging to the group of Unknown Foram. However, for rare taxa, 37% of OTUs and 37% of reads were placed to the group of Unknown Foram.
Abundant and rare foraminiferal subcommunities showed different distribution patterns. For abundant subcommunity, the ten sites had obviously different taxonomic composition at the order level (Figure S2). In ANT01, ANT04, ANT05, ANT08 and ANT09, monothalamiids accounted for the highest proportion of reads (over 55%), while in ANT02, ANT03, ANT06 and ANT10, most reads were assigned to the order Rotaliida. For rare subcommunity, the ten sites showed similar taxonomic composition at the order level. The Unknown Foram occupied the highest proportion of reads (37%), followed by monothalamous foraminifera (31%), and only about 18% of reads were assigned to the order Rotaliida in rare taxa.
3.3. Correlations between foraminiferal diversity and environmental variables
In our study area, the water temperature varied between -1.43°C and 0.20°C, and the salinity varied between 34.54 psu and 34.64 psu. The water temperature and salinity showed complex and similar trends along water depth from 50 to 4399 m, reaching the lowest value at the depth of about 1000 m. The concentrations of sediment Chl a and Pheo a were negatively correlated with water depth. Concentrations of TN, TC and TOC first decreased sharply with water depth from 50 m to 495 m, and then slowly increased with water depth (Figure 3).
Alpha diversity of foraminiferal communities was estimated by phylotype richness and Shannon index. For whole community and abundant subcommunity, their two α-diversity indices had positive correlations with water depth (Figure 4A) and negative correlations with temperature (Figure 4B). In addition, the correlations between the two α-diversity indices of whole community and water depth and temperature were significant (p < 0.01), whilst only phylotype richness of abundant subcommunity showed significant correlations with the above two environmental variables (p < 0.01). No linear correlations were observed between the two α-diversity indices of rare subcommunity with water depth and temperature. Except for water depth and temperature, Phylotype richness and Shannon index of whole community and abundant subcommunity were also negatively correlated with Chl a and Pheo a (Table 1). There was no linear correlation between foraminiferal alpha diversity and TN, TOC, and TC (Table 1).
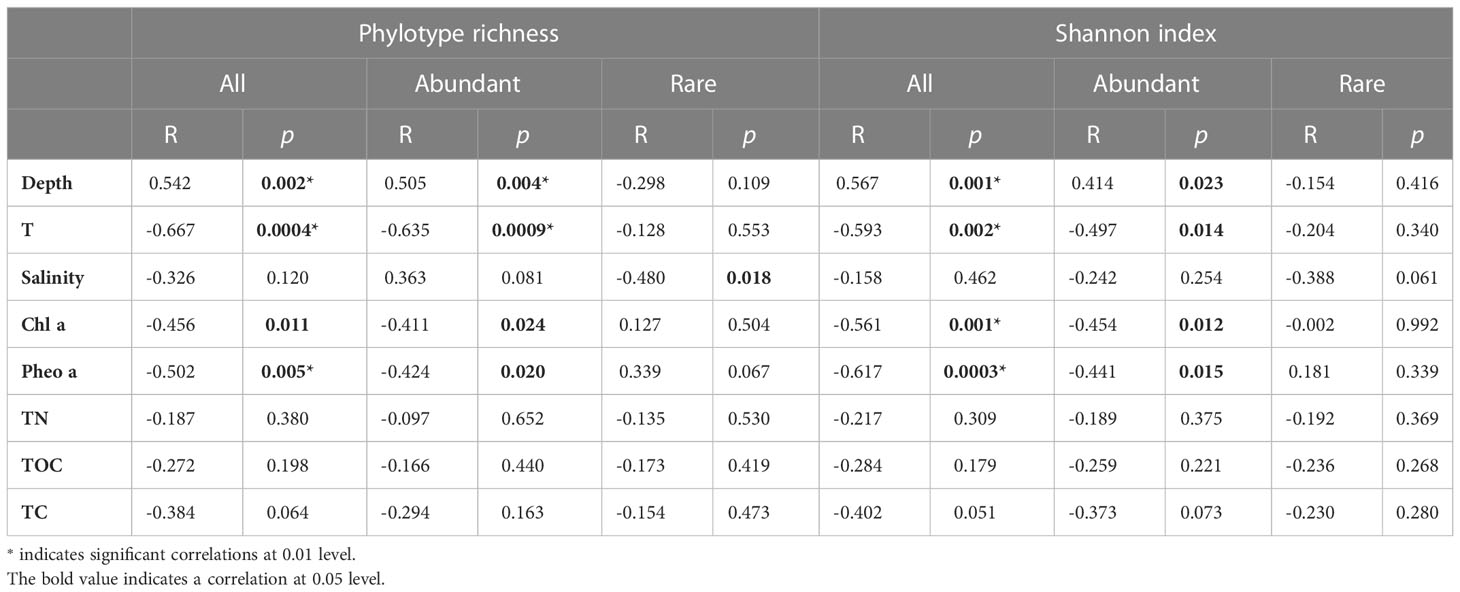
Table 1 Pearson correlations between two α-diversity indices (phylotype richness and Shannon index) and eight environmental variables.
3.4. Effects of environmental variables on foraminiferal community structure
The results of mantel test showed that the beta diversity of whole community, abundant and rare subcommunities had different relationships with geographic distance, water depth, and environmental factors (Figure 5). The Bray-Curtis dissimilarity of whole community was positively correlated with water depth (R = 0.207, p = 0.017), geographic distance (R = 0.1638, p < 0.01) and environmental factors (R = 0.2888, p < 0.01). The Bray-Curtis dissimilarity of abundant subcommunity was significantly correlated with environmental factors (R = 0.2636, p < 0.01), weakly correlated with geographic distance (R = 0.1444, p = 0.023), but not correlated with water depth (R = 0.1422, p = 0.064). The Bray-Curtis dissimilarity of rare subcommunity had significant correlations with water depth (R = 0.3087, p < 0.01) and environmental factors (R = 0.2115, p < 0.01), and had a weak correlation with geographic distance (R = 0.1134, p = 0.032).
The results of VPA showed that water depth and temperature had a greater impact on the abundant subcommunity than on the rare subcommunity (Figure S3). More than 7% of the community variations in the abundant subcommunity could be explained by water depth and temperature, while only 2.4% of community variations in the rare subcommunity could be explained by these two environmental variables. Water depth explained 2.3% and 0.2% of the community variations for abundant subcommunity and rare subcommunity, respectively. Temperature contributed 1.9% and 0.9% of the community variations for abundant subcommunity and rare subcommunity, respectively. Partitioning of β-diversity showed that the β-diversity (Beta.SOR) of abundant and rare subcommunities was shaped by different components (Table S3). The contribution of nestedness (Beta.NES = 0.4747) was two times more than that of spatial turnover (Beta.SIM = 0.2470) to β-diversity in abundant subcommunity, while nestedness component accounted for less than 1% of β-diversity for rare subcommunity and spatial turnover accounted for a great percentage (98.06%).
4. Discussion
4.1. Contrasting biogeographical patterns of abundant and rare foraminiferal subcommunities
Numerous studies have shown that abundant and rare bacterial subcommunities differ remarkably in diversity (Mo et al., 2018), community composition (Wang et al., 2020), distribution patterns (Jiao et al., 2017), driving mechanisms (Liu et al., 2015) and relationships with key environmental factors (Hou et al., 2020; Wang et al., 2021). Likewise, some studies have also confirmed the differences between abundant and rare eukaryotic subcommunities in structuring patterns (Logares et al., 2014) and ecological mechanisms (Xue et al., 2018).
Although the ecological study of foraminifera has a long history, the associated differences in diversity patterns and driving forces between abundant and rare foraminifera are still unclear. In this study, we compared the molecular diversity, taxonomic composition, distribution patterns and driving mechanisms of abundant and rare foraminifera in the Antarctic region based on a DNA metabarcoding dataset for the first time. Our results showed that the abundant taxa accounted for 9.5% of all OTUs representing 81.0% of all reads and the rare taxa accounted for 48.0% of all OTUs representing 3.3% of all reads (Figure S1), which indicated that rare taxa comprised a majority of foraminiferal community diversity in the Antarctic region. Abundant and rare foraminiferal subcommunities exhibited distinct differences in taxonomic composition, with the former dominated by soft-shelled monothalamous foraminifera and the latter by unclassified foraminifera (Figure 2), suggesting that a large proportion of rare foraminifera remain unexplored or that their DNA sequences are highly divergent from the reference sequences in the PR2 database. This result is not surprising since the Antarctic region is known for having a high rate of new species discoveries (Kaiser et al., 2009; Holzmann et al., 2022) and foraminifera are notorious for their high intragenomic variability (Weber and Pawlowski, 2014; Majewski et al., 2021). We also found that abundant and rare foraminiferal subcommunities had obviously different distribution patterns in the Antarctic region. Abundant foraminiferal taxa were more unevenly distributed across all sites than the rare taxa at the order level (Figure S2). Furthermore, our findings suggested that the mechanisms shaping β-diversity of abundant and rare foraminiferal subcommunities were different (Table S3). For abundant subcommunity, its β-diversity was driven by nestedness component, which is normally caused by species loss because of passive sampling, selective extinction, selective colonization, or habitat nestedness (Wang et al., 2010). However, the β-diversity of rare subcommunity was driven by spatial turnover, which usually results from species replacement because of environmental sorting or spatial and historical constraints (Baselga, 2010). Our study is the first attempt to synthetically analyze the community characteristics of abundant and rare foraminifera in the Antarctic region and reveals their differences in biogeographical patterns. Our findings suggest that abundant and rare foraminiferal taxa may occupy distinct ecological niches and play different roles in community stability. Distinguishing these two subcommunities in future studies may help to expand our understanding of foraminiferal community dynamics.
4.2. Different responses of abundant and rare subcommunities to environmental variables
Our results showed that both water depth and temperature had clearly different effects on abundant and rare foraminiferal subcommunities in the Southern Ocean (Figures 4, 5). The foraminiferal alpha diversity of abundant subcommunity was positively correlated with water depth (Figure 4A) and negatively correlated with temperature (Figure 4B), whilst no linear correlation existed between alpha diversity of rare subcommunity and these two environmental variables (Figure 4). In addition, the results of mantel test showed that the Bray-Curtis dissimilarity of abundant subcommunity was independent of water depth (p = 0.064), but the Bray-Curtis dissimilarity of rare subcommunity had a significant correlation with water depth (p < 0.01).
Antarctica and the surrounding Southern Ocean are facing complex environmental changes, and their native biota are now challenged by environmental changes (Convey and Peck, 2019). The most immediate threats to Antarctic biota are consequences of increased temperature and altered sea ice (Chown et al., 2012). Although the Intergovernmental Panel on Climate Change (IPCC) aims to limit average global warming to 2°C by the end of this century, current trends of global temperature increase, sea level rise, and ice loss are at the upper end of the IPCC’s more pessimistic scenarios (Peters et al., 2013; Siegert et al., 2020). The Antarctic Peninsula has already warmed by more than 2°C in the 20th century, with some areas experiencing average annual temperature increases of 3°C or more between 1951 and 2011 (Turner et al., 2014). The native biota in the Antarctic region has adapted to the region’s extreme conditions over many millions of years (Convey and Peck, 2019) and they are more sensitive to temperature variation and much less able to survive elevated temperatures than marine groups elsewhere (Peck and Conway, 2000). Our results showed that the foraminiferal alpha diversity of whole community and abundant subcommunity decreased significantly (p < 0.01) with increasing temperature despite the temperature separation between ten sites being less than 2°C (Figure 3). Antarctic temperature is closely coupled with global sea level (Rohling et al., 2009; Pattyn and Morlighem, 2020). Our results demonstrated that water depth had significant correlations with the beta diversity of rare subcommunity, as well as the alpha diversity of whole community and abundant subcommunity, implying that sea level rise may have an impact on the diversity and community composition of native Antarctic foraminifera, especially those living in shallow waters. This study reveals the different effects of environmental variables on abundant and rare foraminiferal subcommunities and suggests that these two subcommunities may respond differently to future environmental changes, which could lead to subversive changes in Antarctic foraminifera assemblages.
4.3. Effects of environmental variables on foraminiferal community
In this study, we collected surface sediment samples from the Southern Ocean at water depths ranging from 50 to 4399 m and comprehensively analyzed the relationships between foraminiferal diversity and eight measured environmental variables. Our results showed that the foraminiferal alpha diversity was positively correlated with water depth (Figure 4A) and negatively correlated with temperature, chlorophyll a and pheophytin a (Figure 4B; Table 1). Foraminiferal diversity and species distribution are influenced by a variety of environmental factors, such as temperature, salinity, sediment type, primary productivity, organic matter fluxes to the sea floor, water currents and water masses (Murray, 1991). The deep sea contains different environmental settings and the parameters affecting benthic foraminifera tend to vary with bathymetry, therefore the bathymetric distribution of deep-sea foraminifera is considered to reflect the combined effects of various environmental factors closely related to water depth, rather than water depth itself (Gooday, 2003). In our study, temperature, chlorophyll a, pheophytin a, total organic carbon, total carbon and total nitrogen all varied with increasing water depth (Figure 3), so it is hard to quantify the direct effect of individual parameters on foraminiferal diversity.
The organic matter flux to the seafloor is thought to be a crucial parameter for benthic foraminiferal assemblages (Morigi et al., 2001). Although our analysis showed no linear correlation between foraminiferal alpha diversity and total organic carbon, total carbon and total nitrogen (Table 1), it does not mean that TOC, TC and TN had no effect on foraminiferal alpha diversity. Species diversity is believed to have a parabolic relationship with productivity and food supply in deep-sea and other ecosystems (Levin et al., 2001). This may explain why no linear correlations were found between foraminiferal alpha diversity and TOC, TC and TN. The VPA results of our study suggested that the proportion of community variation explained by a single environmental factor was low under the condition of constraining other environmental variables. For example, temperature alone contributed only 1.9% and 0.9% of the community variations for abundant subcommunity and rare subcommunity, respectively (Figure S3). The complex patterns of bathymetry, sea-ice cover, sediment types, surface productivity and water mass characteristics in the Antarctic region make it difficult to untangle the factors underlying the foraminiferal biogeographical patterns, which may be the result of multiple interrelated drivers.
4.4. Future application of foraminiferal DNA for polar paleoenvironmental reconstruction
Polar regions play a key role in maintaining the stability of the global climate system (Anisimov et al., 2001). Meanwhile, climate change in the polar regions is projected to be one of the largest and fastest on the Earth, and will lead to continuing increases in surface temperatures, losses of sea ice and tundra, and warming of permafrost in the Arctica and Antarctica (Turner et al., 2007). These changes will have significant impacts on global climate system and human society. Long-time-scale reconstruction of polar environmental changes will greatly improve our understanding of future climate and environmental changes, and thus better predict and respond to these changes.
Previous paleoenvironmental analyses have been largely based on the fossil record of foraminifera, ignoring the vast amount of foraminiferal DNA accumulated on the deep-sea floor (Lejzerowicz et al., 2013). Foraminiferal aDNA opens new avenues for understanding and reconstructing past environments using foraminifera (Pawłowska et al., 2020). Pawłowska et al. (2016) reported that the analysis of foraminiferal aDNA, with a focus on the non-fossilized monothalamous species, well supported the reconstruction of climate-driven environmental changes over the last millennium in Hornsund Fjord (Svalbard) based on sedimentological and micropaleontological records. The Antarctic region is dominated by cold conditions with thick ice and snow, which is conducive to the long-term preservation of DNA and provides good materials for foraminiferal aDNA study. However, the molecular ecology of benthic foraminifera in the Antarctic region is still poorly studied. In this study, we analyzed the biogeographical patterns of foraminifera based on the obtained DNA metabarcoding dataset and presented the first comprehensive assessment of relationships between ecological diversity of foraminifera and environmental conditions in the Antarctic region. Furthermore, we demonstrated that abundant and rare foraminiferal subcommunities had significantly different correlations with water depth and temperature, and we established equations between α-diversity indices of whole community and abundant subcommunity and water depth and temperature, which may provide additional proxies for future polar paleoceanography using foraminiferal aDNA.
5. Conclusions
In summary, this study was the first attempt to comprehensively analyze the ecological characteristics of foraminifera in the Antarctic region and their correlations with key environmental variables based on the eDNA metabarcoding dataset. The Antarctic foraminiferal community consists of a small number of abundant taxa and a high proportion of rare taxa, the former dominated by monothalamous foraminifera and the latter dominated by unclassified foraminifera. The alpha diversity of whole community and abundant subcommunity had positive correlations with water depth and negative correlations with temperature, Chl a and Pheo a, while no linear correlation was observed between the alpha diversity of rare subcommunity and these environmental variables. Both abundant and rare subcommunities showed distance-decay patterns, but the effect of water depth on the beta diversity of rare subcommunity was more significant than that of abundant subcommunity. Partitioning of beta diversity suggested that abundant and rare subcommunities were shaped by nestedness and turnover components, corresponding to two antithetic processes: species loss and species replacement, respectively. This study clearly reveals the contrasting biogeographical patterns of abundant and rare foraminifera in the Antarctic region and their different correlations with environmental variables, which may provide useful information and more accurate proxies for future reconstruction of polar paleoenvironments using foraminifera aDNA.
Data availability statement
The datasets presented in this study can be found in online repositories. The names of the repository/repositories and accession number(s) can be found below: BioProject, PRJNA908891.
Author contributions
QL, YL and TL conceived and designed the study. TL collected the samples. QL and HL performed the molecular experiments and bioinformatics analysis. QL, YL, HL and TL interpreted the data and wrote the manuscript. All authors contributed to the article and approved the submitted version.
Funding
This work received financial supports from the following projects: the Strategic Priority Research Program of the Chinese Academy of Sciences (XDB42000000); the Marine S&T Fund of Shandong Province for Pilot National Laboratory for Marine Science and Technology (Qingdao) (No.2022QNLM050203; 2021WHZZB0804); National Natural Science Foundation of China (Grant No. 41976058).
Acknowledgments
Special thanks are due to Prof. Dr. Zhimin Jian (Tongji University, China) for many supports and instructions to the first corresponding author in foraminiferal research.
Conflict of interest
The authors declare that the research was conducted in the absence of any commercial or financial relationships that could be construed as a potential conflict of interest.
Publisher’s note
All claims expressed in this article are solely those of the authors and do not necessarily represent those of their affiliated organizations, or those of the publisher, the editors and the reviewers. Any product that may be evaluated in this article, or claim that may be made by its manufacturer, is not guaranteed or endorsed by the publisher.
Supplementary material
The Supplementary Material for this article can be found online at: https://www.frontiersin.org/articles/10.3389/fmars.2023.1089482/full#supplementary-material
References
Anisimov O., Fitzharris B., Hagen J. O., Jefferies R., Marchant H., Nelson F., et al. (2001). “Polar regions (Arctic and Antarctic).” in Climate Change: Impacts, Adaptation and Vulnerability. The Contribution of Working Group II of the Intergovernmental Panel on Climate Change, Third Assessment Review, Eds. McCarthy J. J., Canziani O., Leary N., Dokken D. J., White K. (Cambridge: Cambridge University Press), 801–841.
Barker P. F., Thomas E. (2004). Origin, signature and palaeoclimatic influence of the Antarctic circumpolar current. Earth Sci. Rev. 66, 143–162. doi: 10.1016/j.earscirev.2003.10.003
Baselga A. (2010). Partitioning the turnover and nestedness components of beta diversity. Glob. Ecol. Biogeogr. 19, 134–143. doi: 10.1111/j.1466-8238.2009.00490.x
Bouchet V. M. P., Alve E., Rygg B., Telford R. J. (2012). Benthic foraminifera provide a promising tool for ecological quality assessment of marine waters. Ecol. Indic. 23, 66–75. doi: 10.1016/j.ecolind.2012.03.011
Brandt A., Gutt J. (2011). “Biodiversity of a unique environment: The southern ocean benthos shaped and threatened by climate change,” in Biodiversity hotspots. Eds. Zachos F., Habel J. (Berlin, Heidelberg: Springer), 503–526.
Caporaso J. G., Kuczynski J., Stombaugh J., Bittinger K., Bushman F. D., Costello E. K., et al. (2010). QIIME allows analysis of high-throughput community sequencing data. Nat. Methods 7, 335–336. doi: 10.1038/nmeth.f.303
Chown S. L., Lee J. E., Hughes K. A., Barnes J., Barrett P. J., Bergstrom D. M., et al. (2012). Challenges to the future conservation of the Antarctic. Science 337, 158–159. doi: 10.1126/science.1222821
Clarke A., Johnston N. M. (2003). Antarctic Marine benthic diversity. Oceanogr. Mar. Biol. 41, 47–114.
Convey P., Peck L. S. (2019). Antarctic Environmental change and biological responses. Sci. Adv. 5, eaaz0888. doi: 10.1126/sciadv.aaz0888
Edgar R. C. (2016). UNOISE2: improved error-correction for illumina 16S and ITS amplicon sequencing. BioRxiv. 081257. doi: 10.1101/081257
Goldstein S. T. (1999). “Foraminifera: a biological overview,” in Modern foraminifera. Eds. Sen Gupta B. K. (Dordrecht: Springer), 37–55.
Gooday A. J. (2003). Benthic foraminifera (Protista) as tools in deep-water palaeoceanography: environmental influences on faunal characteristics. Adv. Mar. Biol. 46, 1–90. doi: 10.1016/S0065-2881(03)46002-1
Griffiths H. J. (2010). Antarctic Marine biodiversity – what do we know about the distribution of life in the southern ocean? PLoS One 5, e11683. doi: 10.1371/journal.pone.0011683
Guillou L., Bachar D., Audic S., Bass D., Berney C., Bittner L., et al. (2013). The protist ribosomal reference database (PR2): A catalog of unicellular eukaryote small Sub-unit rRNA sequences with curated taxonomy. Nucleic Acids Res. 41 (Database issue), D597–D604. doi: 10.1093/nar/gks1160
Habura A., Pawlowski J., Hanes S. D., Bowser S. S. (2004). Unexpected foraminiferal diversity revealed by small-subunit rDNA analysis of Antarctic sediment. J. Eukaryot Microbiol. 51, 173–179. doi: 10.1111/j.1550-7408.2004.tb00542.x
Holzmann M., Gooday A. J., Majewski W., Pawlowski J. (2022). Molecular and morphological diversity of monothalamous foraminifera from south Georgia and the Falkland islands: Description of four new species. Eur. J. Protistol. 85, 125909. doi: 10.1016/j.ejop.2022.125909
Hou J., Wu L., Liu W., Ge Y., Mu T., Zhou T., et al. (2020). Biogeography and diversity patterns of abundant and rare bacterial communities in rice paddy soils across China. Sci. Total Environ. 730, 139116. doi: 10.1016/j.scitotenv.2020.139116
Janosik A. M., Halanych K. M. (2010). Unrecognized Antarctic biodiversity: A case study of the genus odontaster (Odontasteridae; asteroidea). Integr. Comp. Biol. 50, 981–992. doi: 10.1093/icb/icq119
Jiao S., Chen W., Wei G. (2017). Biogeography and ecological diversity patterns of rare and abundant bacteria in oil-contaminated soils. Mol. Ecol. 26, 5305–5317. doi: 10.1111/mec.14218
Kaiser S., Barnes D. K. A., Sands C. J., Brandt A. (2009). Biodiversity of an unknown Antarctic Sea: Assessing isopod richness and abundance in the first benthic survey of the amundsen continental shelf. Mar. Biodiv 39, 27–43. doi: 10.1007/s12526-009-0004-9
Kang E., Li Y., Zhang X., Yan Z., Wu H., Li M., et al. (2021). Soil pH and nutrients shape the vertical distribution of microbial communities in an alpine wetland. Sci. Total Environ. 774, 145780. doi: 10.1016/j.scitotenv.2021.145780
Lecroq B., Lejzerowicz F., Bachar D., Christen R., Esling P., Baerlocher L., et al. (2011). Ultra-deep sequencing of foraminiferal microbarcodes unveils hidden richness of early monothalamous lineages in deep-sea sediments. PNAS 108, 13177–13182. doi: 10.1073/pnas.1018426108
Lejzerowicz F., Esling P., Majewski W., Szczuciński W., Decelle J., Obadia C., et al. (2013). Ancient DNA complements microfossil record in deep-sea subsurface sediments. Biol. Lett. 9, 20130283. doi: 10.1098/rsbl.2013.0283
Levin L. A., Etter R. J., Rex M. A., Gooday A. J., Smith C. R., Pineda J., et al. (2001). Environmental influences on regional deep-sea species diversity. Annu. Rev. Ecol. Syst. 32, 51–93. doi: 10.1146/annurev.ecolsys.32.081501.114002
Li Q., Lei Y., Morard R., Li T., Wang B. (2020). Diversity hotspot and unique community structure of foraminifera in the world’s deepest marine blue hole – Sansha Yongle Blue Hole. Sci. Rep. 10, 10257. doi: 10.1038/s41598-020-67221-0
Liu L., Yang J., Yu Z., Wilkinson D. M. (2015). The biogeography of abundant and rare bacterioplankton in the lakes and reservoirs of China. ISME J. 9, 2068–2077. doi: 10.1038/ismej.2015.29
Logares R., Audic S., Bass D., Bittner L., Boutte C., Christen R., et al. (2014). Patterns of rare and abundant marine microbial eukaryotes. Curr. Biol. 24, 813–821. doi: 10.1016/j.cub.2014.02.050
Magoč T., Salzberg S. L. (2011). FLASH: fast length adjustment of short reads to improve genome assemblies. Bioinformatics 27, 2957–2963. doi: 10.1093/bioinformatics/btr507
Majewski W. (2010). Benthic foraminifera from West Antarctic fiord environments: An overview. Pol. Polar Res. 31, 61–82. doi: 10.4202/ppres.2010.05
Majewski W., Bart P. J., McGlannan A. J. (2018). Foraminiferal assemblages from ice-proximal paleo-settings in the Whales Deep Basin, eastern Ross Sea, Antarctica. Palaeogeogr. Palaeoclimatol. Palaeoecol. 493, 64–81. doi: 10.1016/j.palaeo.2017.12.041
Majewski W., Holzmann M., Gooday A. J., Majda A., Mamos T., Pawlowski J. (2021). Cenozoic climatic changes drive evolution and dispersal of coastal benthic foraminifera in the Southern Ocean. Sci. Rep. 11, 19869. doi: 10.1038/s41598-021-99155-6
Mikhalevich V. I. (2004). The general aspects of the distribution of Antarctic foraminifera. Micropaleontology 50, 179–194. doi: 10.2113/50.2.179
Mo Y., Zhang W., Yang J., Lin Y., Yu Z., Lin S. (2018). Biogeographic patterns of abundant and rare bacterioplankton in three subtropical bays resulting from selective and neutral processes. ISME J. 12, 2198–2210. doi: 10.1038/s41396-018-0153-6
Morigi C., Jorissen E. J., Gervais A., Guichard S., Borsetti A. M. (2001). Benthic foraminiferal faunas in surface sediments off NW Africa: Relationship with organic flux to the ocean floor. J. Foraminiferal Res. 31, 350–368. doi: 10.2113/0310350
Murray J. W. (1991). Ecology and palaeoecology of benthic foraminifera (Harlow: Longman Scientific and Technical).
Pattyn F., Morlighem M. (2020). The uncertain future of the Antarctic ice sheet. Science 367, 1331–1335. doi: 10.1126/science.aaz5487
Pawłowska J., Lejzerowicz F., Esling P., Szczuciński W., Zajączkowski M., Pawlowski J. (2014). Ancient DNA sheds new light on the Svalbard foraminiferal fossil record of the last millennium. Geobiology 12, 277–288. doi: 10.1111/gbi.12087
Pawłowska J., Wollenburg J. E., Zajączkowski M., Pawlowski J. (2020). Planktonic foraminifera genomic variations reflect paleoceanographic changes in the Arctic: evidence from sedimentary ancient DNA. Sci. Rep. 10, 15102. doi: 10.1038/s41598-020-72146-9
Pawłowska J., Zajączkowski M., Łącka M., Lejzerowicz F., Esling P., Pawlowski J. (2016). Palaeoceanographic changes in Hornsund Fjord (Spitsbergen, Svalbard) over the last millennium: new insights from ancient DNA. Clim. Past 12, 1459–1472. doi: 10.5194/cp-12-1459-2016
Pawlowski J., Fahrni J. F., Brykczynska U., Habura A., Bowser S. S. (2002). Molecular data reveal high taxonomic diversity of allogromiid foraminifera in Explorers Cove (McMurdo Sound, Antarctica). Polar Biol. 25, 96–105. doi: 10.1007/s003000100317
Peck L. S., Conway L. Z. (2000). The myth of metabolic cold adaptation: oxygen consumption in stenothermal Antarctic bivalves. Geological Society London Special Publications 177, 441–450. doi: 10.1144/GSL.SP.2000.177.01.29
Peters G. P., Andrew R. M., Boden T., Canadell J. G., Ciais P., Le Quéré C., et al. (2013). The challenge to keep global warming below 2 °C. Nat. Clim Chang 3, 4–6. doi: 10.1038/nclimate1783
Rohling E., Grant K., Bolshaw M., Roberts A. P., Siddall M., Hemleben Ch., et al. (2009). Antarctic temperature and global sea level closely coupled over the past five glacial cycles. Nat. Geosci. 2, 500–504. doi: 10.1038/ngeo557
Rosa L. H., da Silva T. H., Ogaki M. B., Pinto O. H. B., Stech M., Convey P., et al. (2020). DNA metabarcoding uncovers fungal diversity in soils of protected and non-protected areas on Deception Island, Antarctica. Sci. Rep. 10, 21986. doi: 10.1038/s41598-020-78934-7
Siegert M., Alley R. B., Rignot E., Englander J., Corell R. (2020). Twenty-first century sea-level rise could exceed IPCC projections for strong-warming futures. One Earth 3, 691–703. doi: 10.1016/j.oneear.2020.11.002
Szczuciński W., Pawłowska J., Lejzerowicz F., Nishimura Y., Kokociński M., Majewski W., et al. (2016). Ancient sedimentary DNA reveals past tsunami deposits. Mar. Geol. 381, 29–33. doi: 10.1016/j.margeo.2016.08.006
Turner J., Barrand N. E., Bracegirdle T. J., Convey P., Hodgson D. A., Jarvis M., et al. (2014). Antarctic climate change and the environment: an update. Polar Rec. 50, 237–259. doi: 10.1017/S0032247413000296
Turner J., Overland J. E., Walsh J. E. (2007). An Arctic and Antarctic perspective on recent climate change. Int. J. Climatol. 27, 277–293. doi: 10.1002/joc.1406
Wang J., Wang Y., Li M., Xu L., He N., Yan P., et al. (2021). Differential response of abundant and rare bacterial subcommunities to abiotic and biotic gradients across temperate deserts. Sci. Total Environ. 763, 142942. doi: 10.1016/j.scitotenv.2020.142942
Wang Y., Bao Y., Yu M., Xu G., Ding P. (2010). BIODIVERSITY RESEARCH: Nestedness for different reasons: the distributions of birds, lizards and small mammals on islands of an inundated lake. Divers. Distrib. 16, 862–873. doi: 10.1111/j.1472-4642.2010.00682.x
Wang Y., Ye F., Wu S., Wu J., Yan J., Xu K., et al. (2020). Biogeographic pattern of bacterioplanktonic community and potential function in the Yangtze river: Roles of abundant and rare taxa. Sci. Total Environ. 747, 141335. doi: 10.1016/j.scitotenv.2020.141335
Weber A. A.-T., Pawlowski J. (2014). Wide occurrence of SSU rDNA intragenomic polymorphism in foraminifera and its implications for molecular species identification. Protist 165, 645–661. doi: 10.1016/j.protis.2014.07.006
Wickham H. (2016). ggplot2 – elegant graphics for data analysis. 2nd ed. (Springer-Verlag New York: Media).
Xue Y., Chen H., Yang J. R., Liu M., Huang B., Yang J. (2018). Distinct patterns and processes of abundant and rare eukaryotic plankton communities following a reservoir cyanobacterial bloom. ISME J. 12, 2263–2277. doi: 10.1038/s41396-018-0159-0
Zeng Q., An S., Liu Y., Wang H., Wang Y. (2019). Biogeography and the driving factors affecting forest soil bacteria in an arid area. Sci. Total Environ. 680, 124–131. doi: 10.1016/j.scitotenv.2019.04.184
Keywords: foraminifera, polar region, environmental change, paleoenvironmental reconstruction, biogeographical pattern
Citation: Li Q, Lei Y, Li H and Li T (2023) Distinct responses of abundant and rare foraminifera to environmental variables in the Antarctic region revealed by DNA metabarcoding. Front. Mar. Sci. 10:1089482. doi: 10.3389/fmars.2023.1089482
Received: 04 November 2022; Accepted: 24 January 2023;
Published: 03 February 2023.
Edited by:
Juan D. Gaitan-Espitia, The University of Hong Kong, Hong Kong SAR ChinaReviewed by:
Petra Heinz, University of Vienna, AustriaWojciech Majewski, Institute of Paleobiology (PAS), Poland
Copyright © 2023 Li, Lei, Li and Li. This is an open-access article distributed under the terms of the Creative Commons Attribution License (CC BY). The use, distribution or reproduction in other forums is permitted, provided the original author(s) and the copyright owner(s) are credited and that the original publication in this journal is cited, in accordance with accepted academic practice. No use, distribution or reproduction is permitted which does not comply with these terms.
*Correspondence: Yanli Lei, leiyanli@qdio.ac.cn; Tiegang Li, tgli@fio.org.cn