- 1Bio-Resources Business Development Division, Riken Food Co., Ltd., Tagajyo, Japan
- 2Nishina Center for Accelerator-Based Science, RIKEN, Saitama, Japan
- 3Fisheries Promotion Division, Department of Agriculture, Forestry and Fisheries, Iwate Prefectural Government, Morioka, Japan
- 4Faculty of Fisheries, Kagoshima University, Kagoshima, Japan
- 5United Graduate School of Agricultural Sciences, Kagoshima University, Kagoshima, Japan
Aquaculture of marine macroalgae (i.e. seaweeds) such as the kelp Undaria pinnatifida is expected to contribute to future food and biomass production. Although macroalgal survival, biomass, and morphology are strongly affected by the density of individual plants in natural environments, little is known about the cultivation density (individuals per 1 m of cultivation rope) of macroalgae required to optimize aquaculture production, commercial profit (sales – labor expenses for processing), and quality as food. The present study examined the effect of increasing the cultivation density of U. pinnatifida from 10 to 200 individuals m-1 on survival rate, biomass production, profit, and morphological features related to quality as food. Survival rate was almost 100% in all treatments, indicating self-thinning did not occur. Biomass production increased with increasing density, suggesting that the maximum density possible is in excess of 200 individuals m-1. However, although profit rose with increasing density from 10 to 120 individuals m-1, it did not rise further if density was further increased. Moreover, some morphological features related to quality increased or decreased with increasing density. On balance, these results suggest that 80-120 individuals m-1 is an appropriate density range to optimize production of this species in terms of both profit and quality as food. However, only 10-30 individuals m-1 was the density best suited to enhance production of the sporophyll form, which is known to be a nutritious food both for humans and sea urchins.
Introduction
Large brown macroalgae, including kelp (Order Laminariales) and fucoids (Order Fucales), are dominant taxa in subpolar and temperate reef ecosystems and are known to be highly productive (Steneck et al., 2002). Moreover, increasing evidence suggests their potential for use in carbon sequestration and therefore contribution to mitigating climate change (Fillbee-Dexter and Wernberg, 2020; Watanabe et al., 2020; Sato et al., 2022). However, regional kelp forests dominated by perennial species are declining under ocean warming in temperate areas (Smale, 2020). In contrast, aquaculture production of annual or biennial kelp species, such as Undaria and Saccharina spp., has been increasing around the world, especially in China, Korea, and Japan, to meet the demand for these products as food for humans, feed for farmed animals, and as sources of various chemicals (Hurd et al., 2014; Chung et al., 2017). Hence, aquaculture techniques that enhance the biomass production of kelp species and improve their quality as food, also enhance carbon sequestration and commercial profit, thereby providing benefits both ecologically and economically.
Population density (number of individuals per m2) is an important factor affecting survival rate, individual size, and population biomass of marine macroalgae (Schiel and Choat, 1980; Causens and Hutchings, 1983; Reed, 1990; Creed et al., 1998; Arenas et al., 2002; Monro and Poore, 2005; Scrosati, 2005), as well as terrestrial plants (Schmitt and Wulff, 1993; Smith and Whitelam, 1997; Enquist et al., 1998; Deng et al., 2012). An increase in population density generally causes decreases in survival rate (i.e., self-thinning), individual size, and population biomass, as a result of intraspecific competition for resources such as light and nutrients (Enquist et al., 1998; Scrosati, 2005; Deng et al., 2012), although the biomass of kelp and fucoid brown algae can increase with increasing density of individuals within the range which occurs in nature (Schiel and Choat, 1980; Causens and Hutchings, 1983). These organisms have strategies to avoid self-thinning, such as physiological integration in clonal plants and low light acclimation under crowded conditions at high densities (e.g., Scrosati and DeWreede, 1997). Moreover, raised density results in an increase in stem or stipe length, which is described as a shade avoidance response (Reed, 1990; Creed et al., 1998; Arenas et al., 2002; Monro and Poore, 2005). Additionally, in terrestrial plants, shading spaces are minimized by expansion of leaves (Givnish, 1988; Smith, 1982) and regulation of petiole length (Takenaka, 1994; Pearcy and Yang, 1998). In aquaculture, too, an increase in the cultivation density of macroalgae (number of individuals per m of cultivation rope) results in a decrease in the size of individuals (Gao et al., 2014). Whereas light is limited in crowded populations of upright plants and macroalgae in natural environments, farmed macroalgae, which grow downwards or sideways from cultivation ropes, may be able to acquire adequate light to survive even at high densities. However, to date, there have been no reports concerning the effect of cultivation density on survival rate, production, profit, and quality as food in macroalgal species.
The annual kelp Undaria pinnatifida (Harvey) Suringar is native to Japan, China, and Korea, and has been cultivated as a major industrial species in these countries since the 1950s (Yamanaka and Akiyama, 1993). This species is now a cosmopolitan invasive species outside its original native Pacific East Asian distribution, introduced to other countries in ship ballast or with aquacultural seedlings (e.g. oyster; Crassostrea gigas), as documented in New Zealand (Hay and Luckens, 1987), Australia (Sanderson, 1990), Argentina (Casas and Piriz, 1996; Dellatorre et al., 2014), France (Castric-Fey et al., 1999), Italy (Cecere et al., 2000), and the USA (Silva et al., 2002). Although there were some attempts to remove this invasive species from newly settled coasts (Stuart, 2004; Wotton et al., 2004), several cultivation studies have been performed in France (Perez et al., 1984) and Spain (Pérez-Cirera et al., 1997; Peteiro and Freire, 2011; Peteiro and Freire, 2012; Peteiro et al., 2016).
Undaria pinnatifida has a life history of alternating heteromorphic generations, with a macroscopic (edible) sporophyte and a microscopic gametophyte (Peteiro et al., 2016). The sporophyte has a holdfast (analogous to a rhizoid), a stipe (analogous to a stem), edible blades (analogous to leaves), and an edible reproductive organ, the sporophyll (Peteiro et al., 2016). In Japan, sporophytes are generally cultivated on ropes at the sea surface from autumn to spring at a density of 50–300 individuals m-1 (Figure 1A, Saito, 1962; Hori, 1981; Ishikawa, 1991; Dan et al., 2015), and are harvested, boiled (Figures 1B,C), salted (Figures 1D, E), and processed (Figure 1F) before shipment (Yamanaka and Akiyama, 1993; Hasegawa and Suzuki, 2005). Increasing the cultivation density of this species is expected to enhance biomass production and sales, but it also may raise the labor expenses for processing (and thereby decrease the profit) because the manual effort required is the same, whatever the size of each individual kelp.
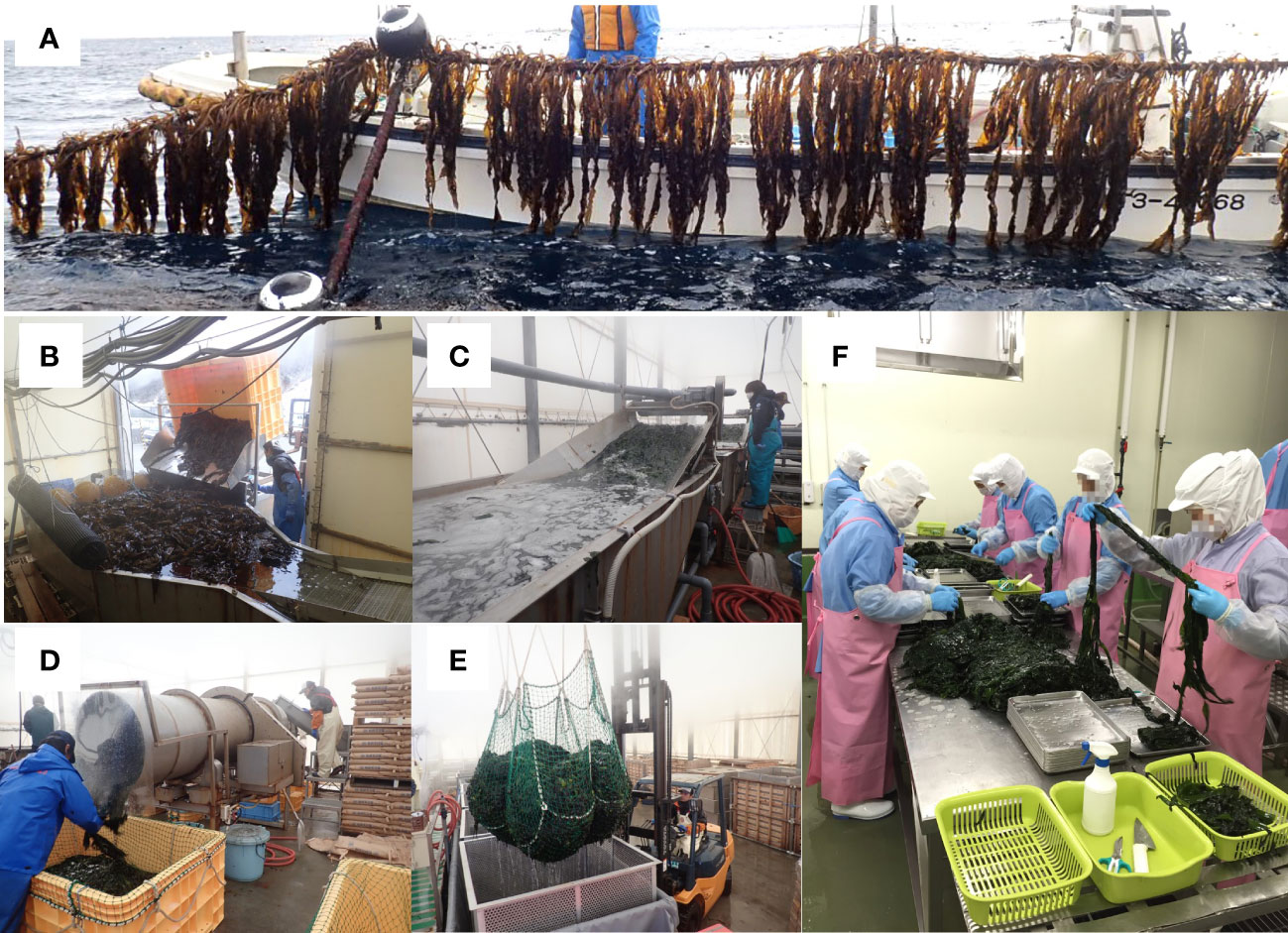
Figure 1 Cultivation and processing of Undaria pinnatifida (‘wakame’) on the Sanriku coast of Japan. (A), Sporophytes adjusted to approximately 100 individuals per 1 m of cultivation rope in January. (B, C), Boiling of post-harvest wakame using large vats. (D, E), Salting process: wakame is tossed with salt using a mixer (D) and removed from the soaking tank the following day (E). (F), separating blades and stipes by manual labour. Images from Ryori Bay (A), Utatsu Bay (B-E), and Okirai Bay (F) on the Sanriku coast.
The quality of this species as food is often evaluated from morphological features of the edible blades. For example, thicker blades are known to have better texture (Kusaka, 2004). Flat blades without wrinkles are considered more valuable (Ohno et al., 1999; Nanba et al., 2011). Individuals with blade lengths of >75, 55-75, and <55 cm are classified into 1st, 2nd, and short grades, respectively, by the Fishermen Cooperative Association of Iwate Prefecture, which is one of the three most productive regions for this species, the other two being Miyagi and Tokushima Prefectures. Additionally, sporophylls of this species are known to be healthy and nutritious food both for human consumption (Mak et al., 2013) and as feed for sea urchins (Takagi et al, 2020 , Takagi et al, 2020). However, the cultivation density optimizing biomass production, profit, edible blade quality, and sporophyll production have not been assessed.
In the present study, increasing the cultivation density of U. pinnatifida from 10 to 200 individuals m-1 was assessed for effects on biomass production, commercial profit, morphological features related to perceived quality as food, and sporophyll production. Sporophytes were cultivated from November and in January cultivation density was prepared at 10, 30, 50, 80, 100, 120, 200 individuals m-1. Sporophytes were harvested from these different density treatments on five occasions during the harvesting season between February and April to measure individual weight, blade width, blade thickness, total length, stipe length, sporophyll weight, biomass production, and profit. The relationships between cultivation density and these variables were evaluated using statistical modeling.
Materials and methods
Cultivation of Undaria pinnatifida and the study site
The Undaria pinnatifida seedlings utilized in the cultivation test were obtained using ordinary industrial methods from Iwate Prefecture, in the Sanriku region, Japan (Figure 2A). The protocol of the producing method was described as follows and in supplemental figure (Figures SA–G). In June 2008, mature sporophytes with sporophylls were collected from Touni Bay (Figure 2B). The sporophylls were derived from sporophytes and were shade-dried overnight. They were soaked for 30 minutes in a 100 L container filled with sterilized seawater to release the zoospores, and then seedling substrate of a palm-thread were soaked in the container to settle spores (Figures SA–C). After soaking the substrate in the seawater released zoospores for about 30 minutes, the substrate with spores was immediately transferred to the Undaria cultivation site in the sea at Touni Bay, where it was placed in a net bag and suspended from a horizontal cultivation rope to a depth of about 10 m (Figures SD,E). During the incubation period under the seas, zoospores on the substrate were germinated to immature gametophytes.
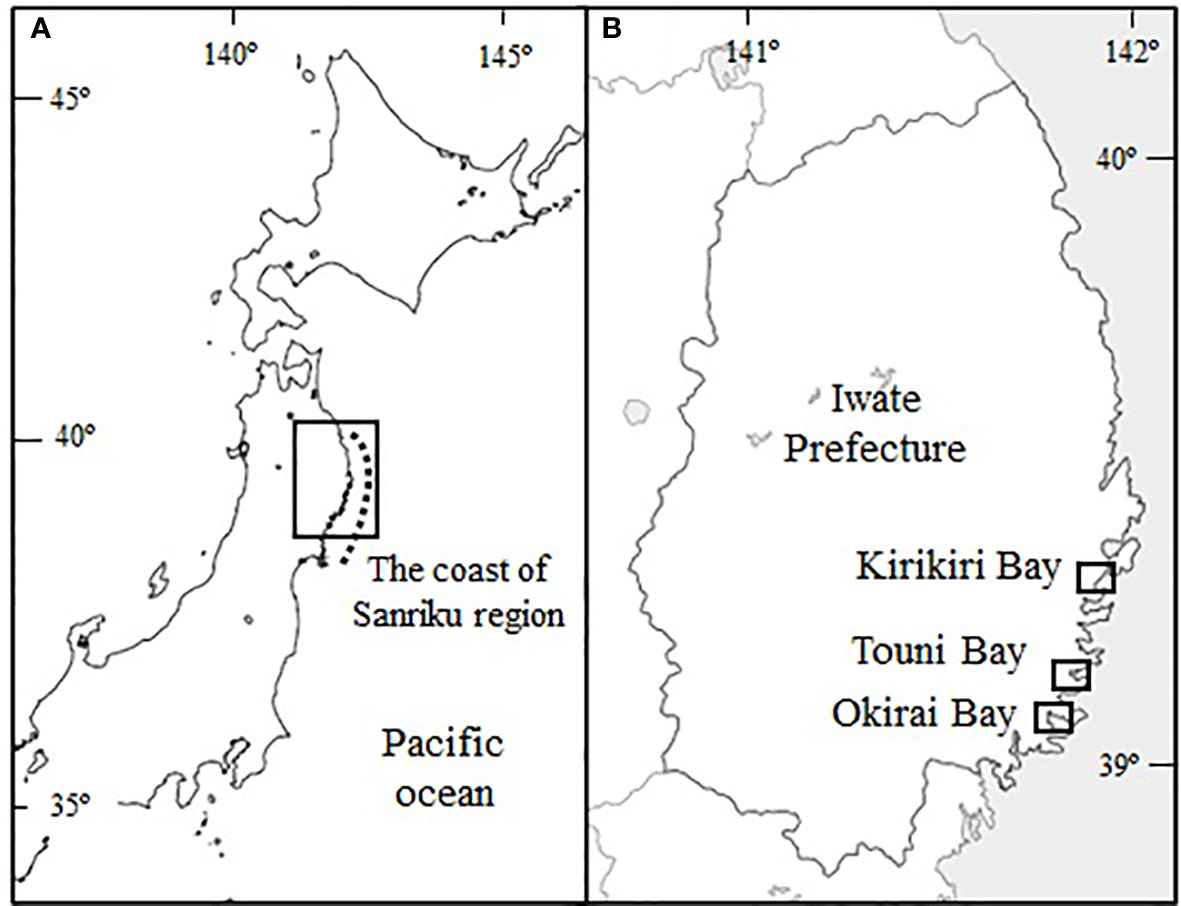
Figure 2 (A), Map of eastern Honshu and Hokkaido, Japan, showing the Sanriku coastal region. (B) Okirai Bay, on the coast of and Iwate Prefecture, which is the main cultivation site for U. pinnatifida in this study. Touni Bay is the seedling production site of this study. Kirikiri Bay is where Iwate Fisheries Technology Center collected the long-term water temperature data cited in this study.
In early September, the substrate with immature and mature gametophytes were withdrawn from the bag and spread out horizontally at the same depth in the sea (Figure SF). The substrate was gradually raised by 2 m every 2 weeks until late October, which adjusts the light intensity, encouraging the gametophytes growth, male and female gametophytes maturation, fertilization and production of embryos, and the initiation of sporophytes growth to obtain seedlings (Figure SG). The seedlings were transferred to Okirai Bay (Figure 2B), on October 31st, 2008 and suspended at 1 m depth for 10 days to acclimate to cultivation conditions, seawater temperature, wave motion, and nutrient concentration.
On November 10th, the seedlings were wound around a mother rope (100 m length, 20 mm diameter) and cultivation began. The number of floats attached to the mother rope was adjusted, depending on the Undaria sporophyte growth, to maintain the cultivation depth at 1 m. Previous studies have reported that morphological development of the Undaria sporophyte is complete when the total length exceeds 70 cm (Taniguchi et al., 1981; Ishikawa, 1994). Therefore, in the present study, surplus sporophytes were excised randomly from 300 sporophytes m-1 or more on January 10th, 2009, when the total length had reached about 50 cm, producing sporophyte growth densities of 10, 30, 50, 80, 100, 120, or 200 individuals m-1 of mother rope (treatments designated as D10, D30, D50, D80, D100, D120, and D200, respectively). The remaining sporophytes were almost evenly spaced with respect to the length of the mother rope. The D10 treatment had a cultivation rope length of 30 m, but in the other treatments it was 10 m. Small sporophytes that germinated on the cultivation rope after excision were all removed at bimonthly intervals so that the original cultivation density of each group was maintained.
In November 2008, a temperature logger (Stowaway TidbiT, Onset Computer Corporation, Boume, MA, USA) was deployed near the sea surface (0.5 m deep) at the study site. Daily mean water temperatures were calculated from measurements made at 1 h intervals. The daily data were averaged for the beginning, middle, and end of each month. These were compared to the representative seawater temperature changes recorded at the Undaria cultivation farm at Kirikiri Bay (Figure 2B) in Iwate Prefecture. The daily seawater temperature data from 1979 to 2008 was averaged for the beginning, middle, and end of each month. Surface seawater samples were collected in 500 mL plastic bottles twice a month at the cultivation site. The samples were filtered through 0.8 µm cellulose acetate filters (DISMIC, 25CS080AS, Advantec, Japan) into 15 mL acid-washed plastic vials and frozen until analysis. The NO3−N concentrations in the water samples were measured with an auto-analyzer (QuAAtro 2-HR, BLTEC, Japan).
Measurements of morphology, biomass production, and commercial profit
All sporophytes in the D10 and D30–D200 treatments were collected from the 3 m and 1 m cultivation ropes, respectively, on five occasions between February and April 2009 (i.e. during the harvest season). On the collecting vessel, the sporophytes from each density treatment were placed in a separate net bag and in a plastic backet with seawater and transferred to the laboratory. The number of harvested individuals, individual weight, total length, and stipe length of all specimens were measured. The survival rate was calculated as a ratio of the final number of individuals harvested each season to the initial ones counted in January in each treatment.
The blade width, blade thickness, and sporophyll weight of the longest 30 individuals in each density treatment were measured because it was difficult to measure these variables of all individuals harvested from all treatments while the samples were fresh. Blade thickness was measured at the widest point of the intercalary region adjacent to the central stipe. The blade was folded to achieve four times its true thickness (Ishikawa, 1991; Sato et al., 2016) and the total thickness was measured with an electronic caliper (NTD13, Mitsutoyo Cooperation, Kawasaki, Kanagawa, Japan). Blade thickness was then calculated by dividing the thickness of the folded portion by four. The number of individuals with wrinkles was also counted.
Biomass production was calculated as the sum of individual sporophyte weights in each density treatment. Commercial profit was estimated by subtracting labor expenses from sales, only for the samples harvested in April 8th and 24th (i.e. the main harvest season). To estimate the sales value, the total biomass of first-grade sporophytes (kg/m), with a blade length of more than 75 cm, was multiplied by 1000 (m rope) to expand to an industrial scale; by 0.23 to convert to the amount of salt-boiled sporophytes; and by 13.64 (USD/kg) to convert to sale value (USD), based on company data at Riken Food. Co. Ltd. To estimate labor expenses, the cultivation density (individuals m-1) was multiplied by 1000 (m rope) to expand to industrial scale; by 30 (sec individual-1) to estimate the working time required for processing (i.e. separating the blades from their stipes, sec/m; Hasegawa and Suzuki, 2005); by 1/3600 to convert the time scale to hours; and by 6.51 (USD/h, the minimum wage in Iwate Prefecture, 2016) to convert to labor expense (USD).
Statistical analyses
Before the analysis, morphological data for 20 individuals were randomly sampled from each density treatment in order to unify the data numbers among treatments and to ensure randomness. Because survival rate, individual weight, sporophyll weight, blade width, blade thickness, and biomass production decreased or increased with increasing density, linear models were fitted to the relationship between density (as factor) and these variables, using lm function of the R software (R Development Core Team). These variables and the density factor were logarithmically transformed in some cases in order to ensure the assumption of normality and variance homogeneity of the data, and to improve the goodness of fit of the model based on Akaike information criterion (AIC). Differences between a null model and the model of interest were tested using F test. A relationship between fitted value and residuals of the model was visually checked using autoplot function in the package ‘ggfortify’. Since total length and stipe length peaked at a density between 10 and 200 individuals m-1, generalized additive models were fitted to the relationship between density and these variables using gam function in the package ‘mgcv’. These variables and the density factor were logarithmically transformed in some cases and the goodness of fit of the model was assessed based on AIC. Differences between a linear model and the model of interest were tested using F test. A relationship between fitted value and response of the model was visually checked using the function ‘gam.check’ in R. As number of individuals with wrinkles was count data ranging between 0 and 30, a generalized linear model was fitted to the relationship between density and the variable using glm function, with binomial distributions and logit link functions. Because profit increased with increasing density but saturated at a certain density, like a photosynthesis-light curve, a non-linear model based on a Michaelis-Menten plot was fitted to the relationship between density and profit using nls function in R by non-parametric bootstrapping (n=500 bootstrap simulations). A relationship between fitted value and residuals of the model was visually checked using the function ‘nlsResiduals’ in the package ‘nlstools’.
Results
The seawater temperature at Okirai Bay decreased from 15.6°C in November, 2008 to 6.1°C in early March, 2009, but then increased to 9.6°C by April, 2009 at the end of the cultivation period. These seawater temperature changes were similar to those in the Kirikiri data (1979–2008; Iwate Prefecture Fisheries Technology Center in-house data for 1979-2008, personal communication to YS and TF), but the values for Okirai were generally higher by 0.3–1.5°C. The NO3-N concentration at Okirai increased from 2.36 µM in November to a maximum of 7.34 µM at the end of February, but then decreased to a minimum value of 0.86 µM in April when cultivation ended.
Images of representative sporophytes cultivated at each density are shown in Figure 3. The shape differed depending on the cultivation density: sporophytes produced at lower density tended to have a shorter stipe length, larger sporophylls, and wrinkled blades; while those at higher density had a longer stipe length, smaller sporophylls, and fewer wrinkles on their blades.
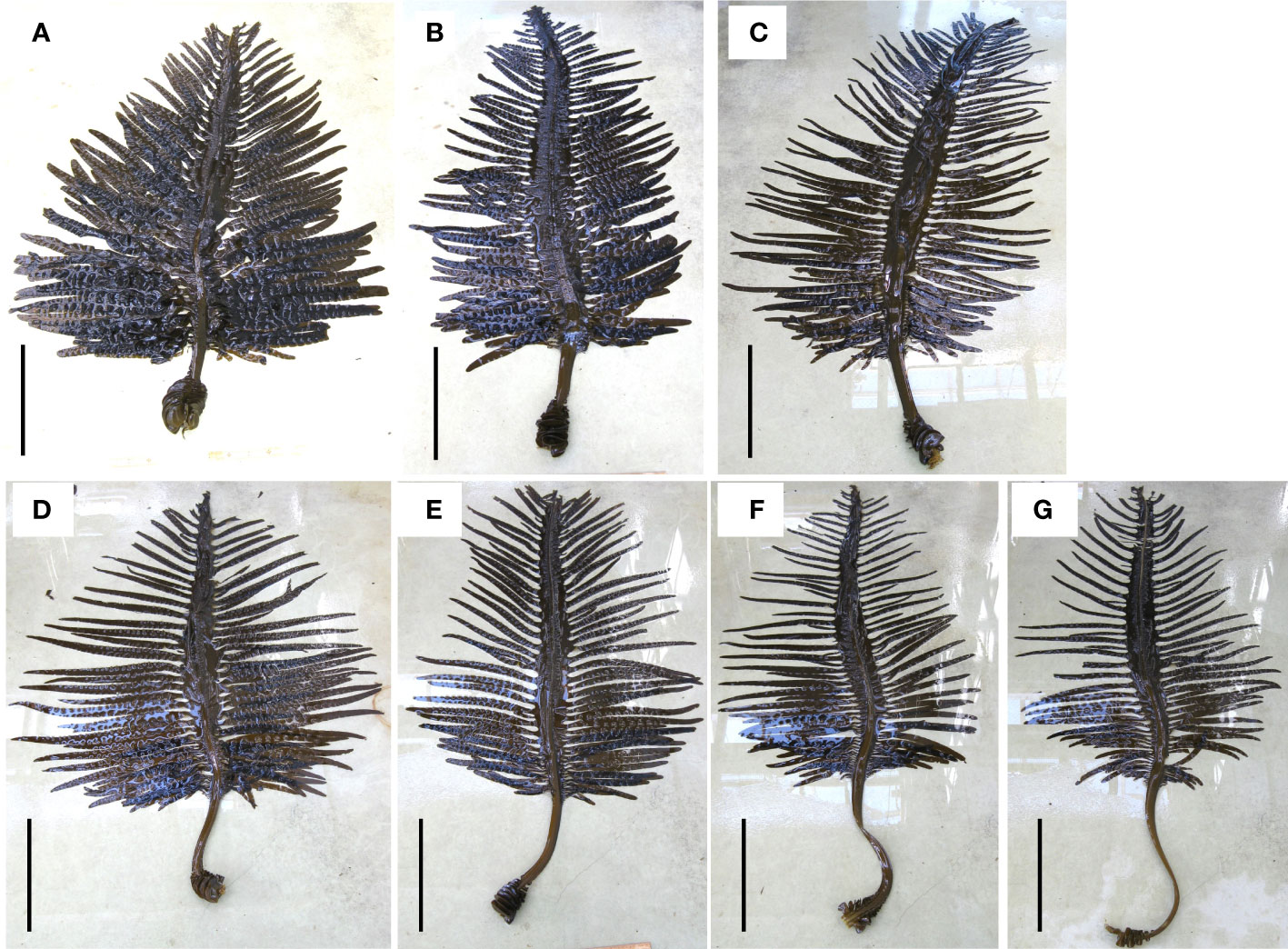
Figure 3 Photographs of representative U. pinnatifida sporophytes cultivated at different densities of individuals per 1 m cultivation rope: (A), D10; (B), D30; (C), D50; (D), D80; (E), D100; (F), D120; (G), D200. Scale bar 100 cm.
Survival rate (Figure 4A) was almost 100% in all treatments and was not significantly affected by density (P = 0.657) and season (P = 0.962). No significant difference was found between a null model and the linear model (P = 0.7382), indicating that the survival rate was a constant value.
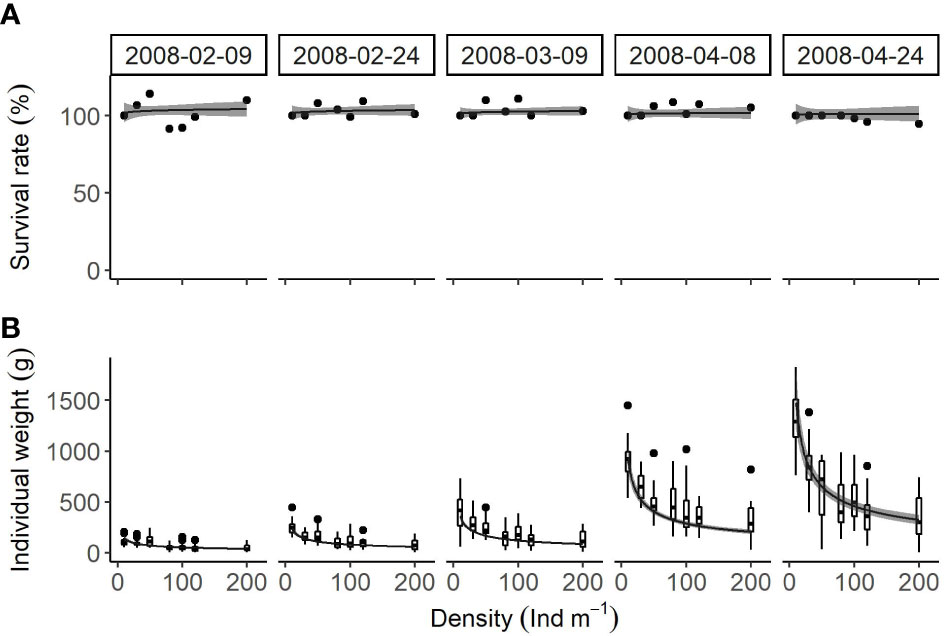
Figure 4 Relationships between cultivation density and (A) survival rate and (B) individual weight (box plots) of U. pinnatifida, harvested on five different dates. Solid lines and grey zones indicate mean and confidence intervals, respectively, estimated using linear models.
Individual weight (Figure 4B) was significantly affected by density (P < 0.001) and season (P < 0.001) but there was no significant interaction between these two factors (P = 0.541). An increased density from 10 to 200 individual/m resulted in significant decrease in this variable: 148.9 ± 24.5 g to 37.5 ± 4.7 g on February 9th; 235.6 ± 30.3 g to 57.7 ± 5.7 g on February 24th; 361.4 ± 38.8 g to 86.3 ± 7.1 g on March 9th; 904.5 ± 121.7 g to 204.4 ± 21.0 g on April 8th; and 1475.2 ± 257.8 g to 323.7 ± 43.4 g on April 24th.
Total length and stipe length peaked at densities between 10 and 200 individuals m-1: the density resulting in maximum lengths varying according to season (based on the general additive models used; Figures 5A, B). Total length was highest at 36 individuals m-1 and lowest at 89 individuals m-1 between February and March but highest at 89 individuals m-1 and lowest at 36 individuals m-1 in April. Similarly, stipe length was highest at 52 individuals m-1 and lowest at 84 individuals m-1 between February and March but highest at 84 individuals m-1 and lowest at 52 individuals m-1 in April.
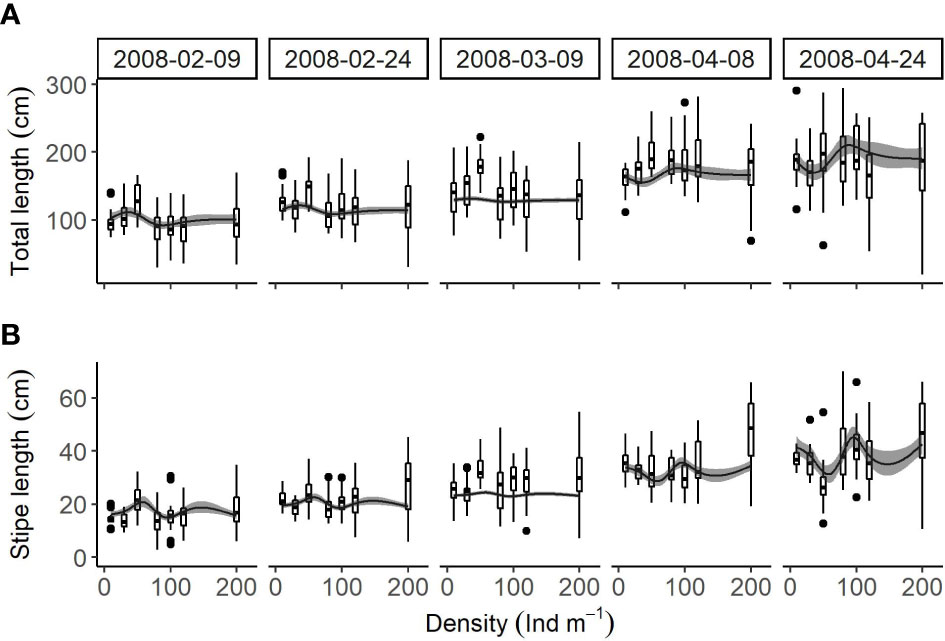
Figure 5 Relationships (box plots) between cultivation density and (A) total length and (B) stipe length of U. pinnatifida, harvested on five different dates. Solid lines and grey zones indicate mean and confidence intervals, respectively, estimated using generalized additive models.
Blade width and thickness (Figures 6A, B) were significantly affected by density (P < 0.001) and season (P < 0.001). There was no significant interaction between these two factors (P = 0.078 for blade width, P = 0.318 for blade thickness). The increased density resulted in moderate decreases in blade width from 64.5 ± 2.4 cm to 55.1 ± 2.7 cm on February 9th, from 72.3 ± 2.1 cm to 62.1 ± 2.4 cm on February 24th, from 83.0 ± 2.0 cm to 69.4 ± 2.2 cm on March 9th, from 110.0 ± 3.3 cm to 88.2 ± 3.5 cm on April 8th, and from 131.4 ± 5.1 cm to 100.2 ± 5.3 cm on April 24th. Similarly, it caused moderate decreases in blade thickness from 0.26 ± 0.01 mm to 0.24 ± 0.01 mm on February 9th, from 0.29 ± 0.01 mm to 0.26 ± 0.01 mm on February 24th, from 0.31 ± 0.01 mm to 0.28 ± 0.01 mm on March 9th, from 0.35 ± 0.01 mm to 0.32 ± 0.01 mm on April 8th, and from 0.38 ± 0.01 mm to 0.34 ± 0.01 mm on April 24th.
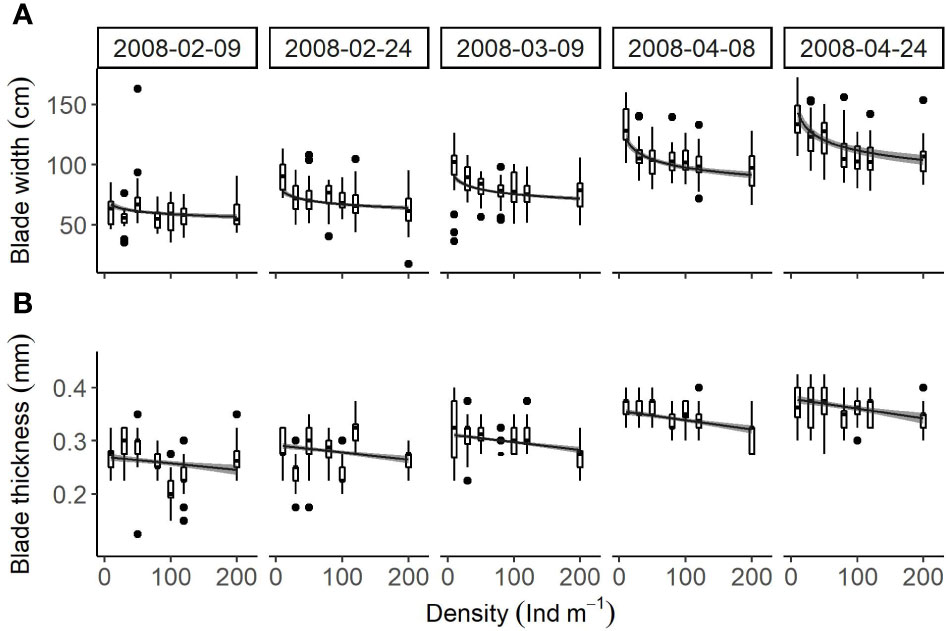
Figure 6 Relationships (box plots) between cultivation density and (A) blade width and (B) blade thickness of U. pinnatifida, harvested on five different dates. Solid lines and grey zones indicate mean and confidence intervals, respectively, estimated using linear models.
Number of individuals with wrinkles (Figure 7A) was significantly affected by density (P < 0.001) and season (P < 0.001). There was a significant interaction between these two factors (P = 0.046). The increased density resulted in gradual decreases in this variable from 0.969 ± 0.336 to 0.001 ± 0.001 on February 9th, from 1.646 ± 0.451 to 0.001 ± <0.001 on February 24th, from 2.700 ± 0.657 to 0.001 ± <0.001 on March 9th, from 7.797 ± 2.726 to <0.001 ± <0.001 on April 8th, and from 13.726 ± 6.007 to <0.001 ± <0.001 on April 24th.
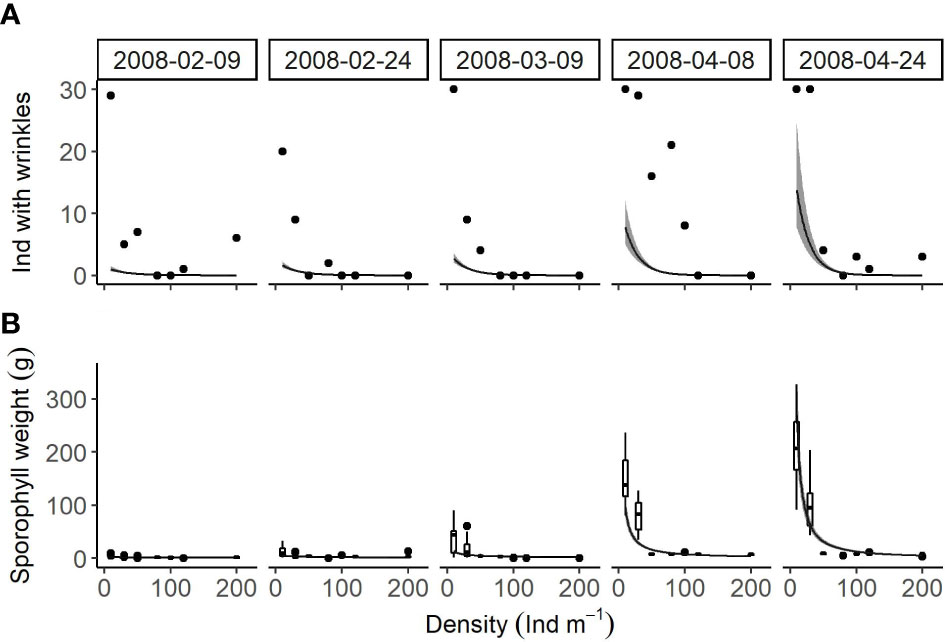
Figure 7 Relationships between cultivation density and (A) number of individuals with wrinkles and (B) sporophyll weight (box plots) of U. pinnatifida, harvested on five different dates. Solid lines and grey zones indicate mean and confidence intervals, respectively, estimated using generalized linear model.
Sporophyll weight (Figure 7B) was significantly affected by density (P < 0.001) and season (P < 0.001). There was a significant interaction between these two factors (P < 0.001), indicating that the effect of density varied among seasons. The increased density resulted in slight decreases in this variable from 2.2 ± 0.5 g to 1.0 ± 0.2 g on February 9th, from 5.8 ± 1.1 g to 1.4 ± 0.2 g on February 24th, and from 14.1 ± 2.2 g to 1.9 ± 0.2 g on March 9th, while it caused significant decreases in the value from 96.7 ± 19.1 g to 3.7 ± 0.6 g on April 8th and from 269.7 ± 68.5 g to 5.2 ± 1.0 g on April 24th.
Biomass production (Figure 8) was significantly affected by density (P < 0.001) and season (P < 0.001). There was no significant interaction between these two factors (P = 0.561). The increased density resulted in gradual increases in this variable from 2.48 ± 0.62 kg to 12.58 ± 4.12 kg on February 9th, from 3.85 ± 0.77 kg to 19.29 ± 5.04 kg on February 24th, from 5.82 ± 0.97 kg to 28.76 ± 6.33 kg on March 9th, from 14.09 ± 2.93 kg to 67.65 ± 18.44 kg on April 8th, and from 22.58 ± 6.02 kg to 106.75 ± 36.91 kg on April 24th.
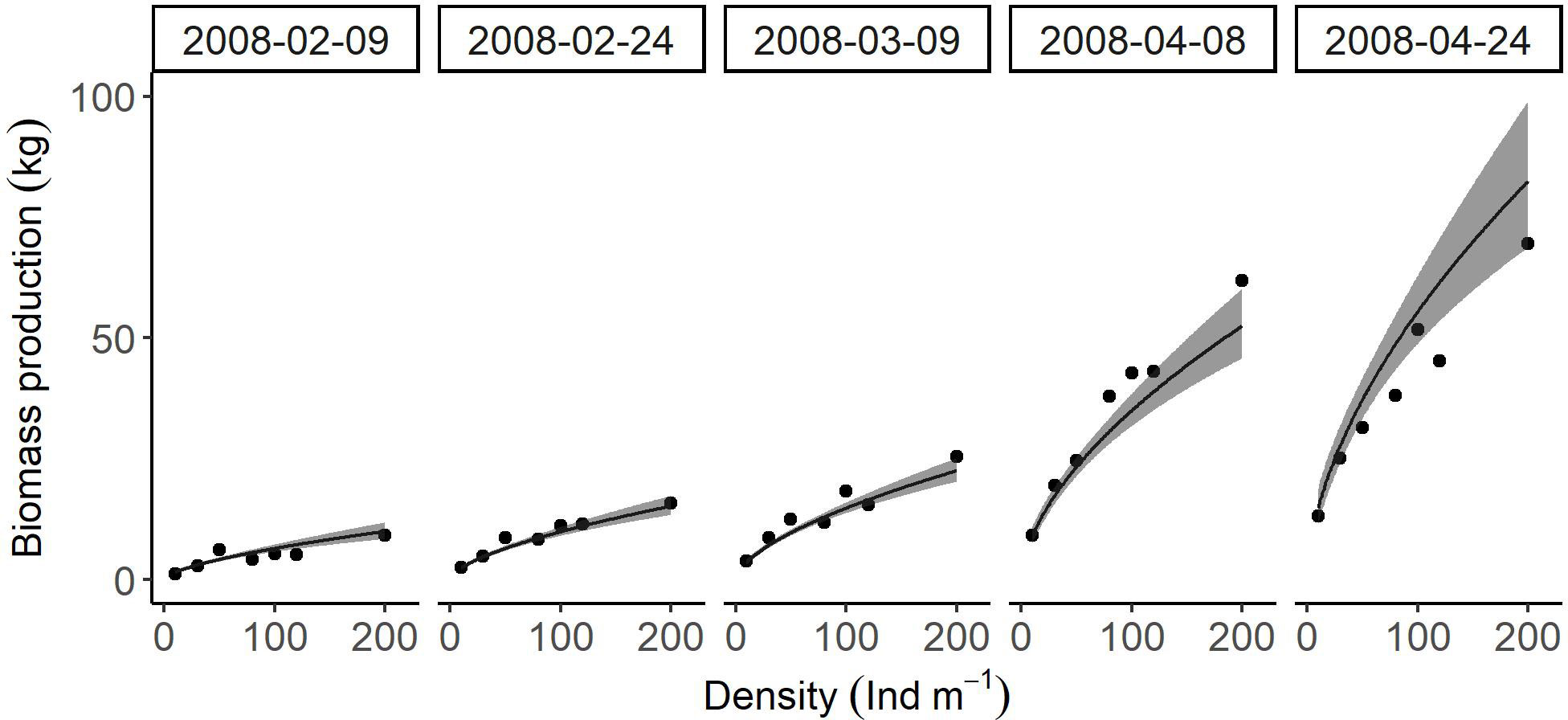
Figure 8 Relationships between cultivation density and biomass production of U. pinnatifida, harvested on five different dates. Solid lines and grey zones indicate mean and confidence intervals, respectively, estimated using a linear model.
Profit (Figure 9), estimated from the pooled data for April 8th and 24th, rose from US$ 41,662 ± 12,669 to 132,066 ± 9,399 with increasing density from 10 to 152 individuals m-1. However, the change in profit was relatively small between 80 and 200 individuals m-1. The values at densities of 80, 100, 120, and 200 individuals m-1 were: US$ 111,132 ± 8,494; 122,157 ± 9,106; 128,696 ± 9,050; and 127,934 ± 15,888, respectively.
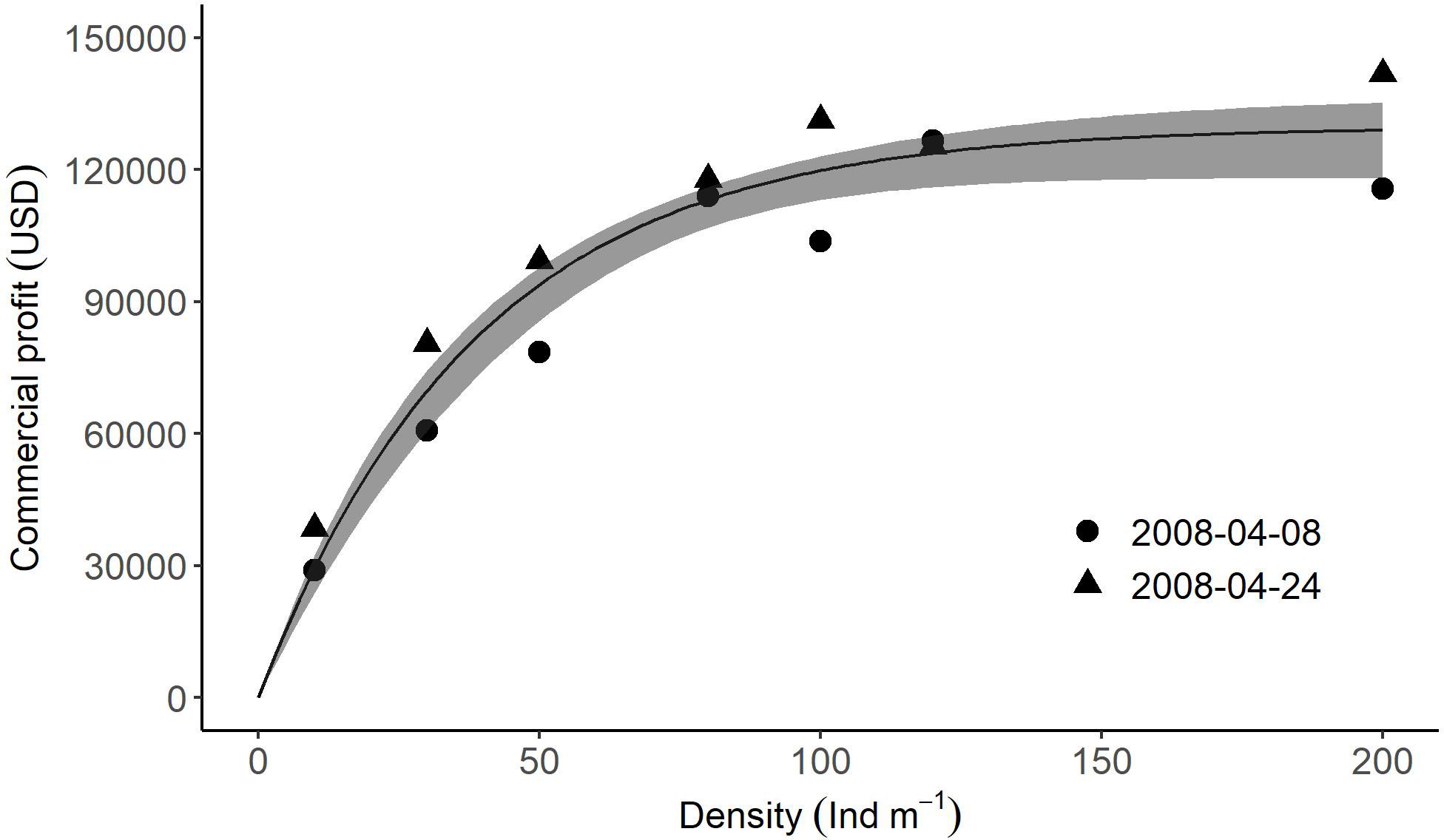
Figure 9 Relationships between cultivation density of U. pinnatifida and profit estimated from data obtained during the main harvest season (on April 8th and 24th). Solid lines and grey zones indicate mean and confidence intervals, respectively, estimated using a non-linear model with bootstrap.
Discussion
Previous studies have shown that increases in the population density of plants and macroalgae result in decreased survival rates, which is described as self-thinning (Enquist et al., 1998; Scrosati, 2005; Deng et al., 2012). However, in the present study, survival rates of the macroalga U. pinnatifida cultivated at different densities between 10 and 200 individuals per m were almost 100%. Because the farmed algae grow downwards or sideways from cultivation ropes, they may be able to acquire adequate light to survive even at high densities. Moreover, the strategies to avoid self-thinning is reported to include the physiological integration of clonal plants and low light acclimation at high densities (Scrosati and DeWreede, 1997). U. pinnatifida is not a clonal plant, but its compensation irradiance is very low (2.1-5.9 μmol photons m-2 s-1), especially at winter temperature of 8 °C (2.1 μmol photons m-2 s-1, Sato et al., 2021), which occurs during cultivation at the present study site. Moreover, the strain of U. pinnatifida used in the present study has a relatively high tolerance to nutrient-poor conditions, based on the comparison of nutrient uptake kinetics among six strains derived from six different localities in Japan (Sato et al., 2016). These traits may also contribute to avoiding self-thinning under resource limitations at the higher densities in the present study.
In general, an increase in population density causes a decrease in individual size of plants and macroalgae, as a result of intraspecific competition for resources such as light and nutrients (Enquist et al., 1998; Scrosati, 2005; Deng et al., 2012); and an increase in stipe or plant length, which is known as the shade-avoidance response (Reed, 1990; Creed et al., 1998; Arenas et al., 2002; Monro and Poore, 2005; Scrosati, 2005). Gao et al. (2014) reported that increasing the cultivation density from 5-10 to 16-20 individuals m-1 resulted in decreases in the size of blades, stipes, and sporophylls of the macroalga U. pinnatifida. The present study showed that increasing cultivation density at an industrial scale from 10 to 200 individuals m-1 results in decreases in individual weight, blade width, blade thickness, and sporophyll weight. However, in contrast to previous studies, the present study found that total length and stipe length of U. pinnatifida peaked at the cultivation density between 10 and 200 individuals m-1. These variables peaked at ca. 50 individuals m-1 (where individual size was 71.0 ± 5.7 g) on February 9th and, on April 24th, at ca. 80 individuals m-1 (where individual size was 514.7 ± 45.2 g). These results indicate that the shade avoidance response occurred at these densities, while wider space for growth at the lower densities and small individual size at higher densities suppressed intraspecific competition and shade avoidance response.
In terrestrial plant species, population biomass is known to decrease with increasing population density across the scale of 0.01 to 1000 individuals m-2 (Enquist et al., 1998). In contrast, Schiel and Choat (1980) showed that the population biomass of the kelp Ecklonia radiata and the fucoid brown alga Sargassum sinclairii increased with increasing population density across the range that occurs in nature (ca. 40 individuals m-2 in Ecklonia and ca. 200 individuals m-2 in S. sinclairii). Similarly, the present study showed that biomass production of the kelp U. pinnatifida increased with increasing cultivation density from 10 to 200 individuals m-1. However, the commercial profit from U. pinnatifida aquaculture rose with increasing cultivation density from 10 to ca. 80-120 individuals m-1 but did not rise any further in response to further increases in density because of the raised labor expense required to process a larger number of smaller macroalgae thalli at increasing density.
It was also found in the present study that increasing the cultivation density from 10 to 200 individuals m-1 produced an increase in the number of individual blades without wrinkles, which is a highly valued feature (Ohno et al., 1999; Nanba et al., 2011). However, increasing density also led to a decrease in blade thickness, which results in weak product texture (Kusaka, 2004). On balance, these results suggest that 80-120 individuals m-1 is the most appropriate cultivation density of U. pinnatifida to optimize both profit and quality as food.
In practice, U. pinnatifida should be cultivated at a density of 10-30 individuals m-1 in order to enhance sporophyll production, because the sporophyte weight of this species decreased drastically with increasing density from 10 to 200 individuals m-1 in the present study. The fresh sporophyll of this cosmopolitan species has been recognized recently as an excellent feed for sea urchins (Takagi et al, 2020; Takagi et al, 2020), which are known to induce phase shifts from highly-productive kelp forests to barren grounds by their destructive grazing because of increased population size and/or lowered food availability in subpolar and temperate reef ecosystems (Steneck et al., 2002; Filbee-Dexter and Scheibling, 2014). The adverse effects of grazing sea urchins have been ameliorated by their removal from barren areas in order to enable kelp forests to recover (Eger et al., 2022), and by raising these sea urchins in aquaculture. Aquaculture of sea urchins fed on U. pinnatifida sporophylls, produced by low-density cultivation, may contribute to both restoration of kelp forests and raised income for fishermen (Takagi et al, 2020; Takagi et al, 2020).
In Japan, U. pinnatifida sporophytes have been commonly cultivated from autumn (between October and December) to spring (April) (Peteiro et al., 2016). However, recent ocean warming may shorten the cultivation period by a month or more, because an increase in autumn temperature is predicted to decrease survival and growth rates of the juvenile sporophytes of this species (Gao et al., 2013) and increase the risk of herbivory by small isopods (Endo et al., 2021). In this context, the present study showed that the biomass production of U. pinnatifida was higher on April 24th than on April 8th but the profit was almost same for these two dates of harvesting, suggesting that a reduction of 16 days in the cultivation period did not significantly affect profit. This finding provides new insight to maintaining the aquaculture industry of this species in a warming world.
In addition, while aquaculture of marine macroalgae is expected to contribute to carbon sequestration, it is pointed out that there are significant uncertainties related to carbon flow by farmed and wild seaweeds (Hurd et al., 2022; Troell et al., 2022). Therefore, before getting into discussions like industrial use of carbon sequestration by seaweeds (e.g., carbon emission trading), it is first necessary to accumulate knowledge on how to improve the productivity of seaweed farming and optimize the benefit of seaweed resources. The present study showed that U. pinnatifida farming could provide various benefits by regulation of the cultivation density; for feed (10-30 individuals m-1), for edible food with higher profit and quality (80-120 individuals m-1), for biomass (more than 200 individuals m-1). Therefore, for future development, it is necessary to clarify the cultivation density of U. pinnatifida that matches the environment and industrial needs in each region. Furthermore, it is also necessary to estimate the concise density that maximizes biomass production and carbon sequestration as general knowledge.
Conclusion
Morphology and biomass of Undaria pinnatifida were affected by the density of individual plants on the rope in aquaculture conditions, and the variables have the potential to impact the industrial application as follows:
1. The maximum biomass production was estimated at more than 200 individuals m-1 since increased value with increasing density.
2. The optimum condition of both the commercial profit and the quality as the food was estimated at 80–120 individuals m-1.
3. The best density to obtain the sporophylls, which are available for nutritious food for humans and sea urchins, was at 10–30 individuals m-1.
The cultivation density adjustment can be reliably controlled artificially; hence it would be a valuable aquaculture technology to make the seaweed aquaculture industry more effective, which is expected to play various roles, including from edible food to carbon sequestration in the future.
Data availability statement
The raw data supporting the conclusions of this article will be made available by the authors, without undue reservation.
Author contributions
YS conceptualized the research, YS and TF conducted the experiment and collected data, HE analyzed the data, YS prepared the original draft of the manuscript, YS and HE reviewed and edited the manuscript. All authors contributed to the article and approved the submitted version.
Acknowledgments
We are very grateful to Hitoshi Hashimoto (Okirai Bay Fisheries Cooperative) for his generous support during the field surveys and for providing the cultivated U. pinnatifida. We also thank Dr. Nobuyoshi Nanba (Kitasato University) for his kind advice and cooperation in the measurement of nutrients; Prof. Gregory N. Nishihara (Nagasaki University) for advice on statistical modeling; and Tadashi Takahashi (Iwate Prefectural Fisheries Technology Centre), Hironobu Watanabe and Masakazu Honda (Riken Food Co., Ltd.) for their valuable advice and cooperation. This study was conducted before the Great East Japan Earthquake of March 11th, 2011, and we received the support of many fishermen in Iwate Prefecture who subsequently suffered huge losses because of the earthquake and tsunami. We here express our gratitude to all of them and empathize with the terrible losses suffered by them and their families.
Conflict of interest
Author YS was employed by the company Riken Food Co., Ltd.
The remaining authors declare that the research was conducted in the absence of any commercial or financial relationships that could be construed as a potential conflict of interest.
Publisher’s note
All claims expressed in this article are solely those of the authors and do not necessarily represent those of their affiliated organizations, or those of the publisher, the editors and the reviewers. Any product that may be evaluated in this article, or claim that may be made by its manufacturer, is not guaranteed or endorsed by the publisher.
Supplementary material
The Supplementary Material for this article can be found online at: https://www.frontiersin.org/articles/10.3389/fmars.2023.1085054/full#supplementary-material
Supplementary Figure | Photographs of seedling production. (A), Sporophylls were soaked for 30 minutes in a 100L container filled with sterilized seawater to release the zoospores. (B,C), Seedling substrate of a palm-thread were soaked in the container to settle zoospores for about 30 minutes. (D,E), The substrate with zoospores was placed in a net bag and suspended from a horizontal cultivation rope to a depth of about 10m in June. (F), In early September, the substrate with zoospores and germinated gametophytes was withdrawn from the bag, settles at a horizontal cultivation rope, and spread out horizontally at the same depth. (G), The substrate with juvenile sporophytes to use cultivate as seedlings. The substrate was gradually risen by 2 m every 2 weeks until late October, which adjusts the light intensity, encouraging the gametophytes growth, male and female gametophytes maturation, fertilizing and appearing embryo, and starting growth of sporophytes.
References
Arenas F., Viejo R. M., Fernández C. (2002). Density-dependent regulation in an invasive seaweed: Responses at plant and modular levels. J. Ecol. 90, 820–829.
Casas G. N., Piriz M. L. (1996). Surveys of Undaria pinnatifida (Laminariales phaeophyta) in golfo nuevo, Argentina. Hydrobiologia 326/327, 213–215.
Castric-Fey A., Beaupoil C., Bouchain J., Pradier E., L’Hardy-Halos M. T. (1999). The introduced alga Undaria pinnatifida (Laminariales, alariaceae) in the rocky shore ecosystem of the st. malo area: Growth rates and longevity of the sporophyte. Bot. Mar. 42, 83–96.
Causens R., Hutchings M. J. (1983). The relationship between density and mean frond weight in monospecific seaweed stands. Nature 301, 240–241.
Cecere E., Petroccelli A., Saracino O. D. (2000). Undaria pinnatifida (Fucophyceae, laminariales) spread in the central Mediterranean: Its occurrence in the mar piccolo of taranto (Ionian Sea, southern Italy). Cryptogam. Algol. 21, 305–309.
Chung I. K., Sondak C. F. A., Beardall J. (2017). The future of seaweed aquaculture in a rapidly changing world. eur. J. Phycol. 52, 495–505. doi: 10.1080/09670262.2017.1359678
Creed J. C., Kain J. M., Norton T. A. (1998). An experimental evaluation of density and plant size in two Large brown seaweeds. J. Phycol. 34, 39–52.
Dan A., Ohno M., Matsuoka M. (2015). Changes of the research and development on the resources of Undaria and Laminaria in the culture ground of tokushima coasts. Bull. Tokushima Pref. Fish. Res. Ins. 10, 25–48.
Dellatorre F. G., Amoroso R. O., Saravia J., Orensanz J. M. (2014). Rapid expansion and potential range of the invasive kelp Undaria pinnatifida in the southwestern Atlantic. Aquat Invasions 9, 467–478.
Deng J., Ran J., Wang Z., Brown J. H. (2012). Models and tests of optical density and maximal yield for crop plants. Proc. Nat. Acad. Sci. 109, 15823–15828. doi: 10.1073/pnas.1210955109
Eger A. M., Marzinelli E. M., Christie H., Fagerli C. W., Fujita D., Gonzales A. P., et al. (2022). Global kelp forest restoration: Past lessons, present status, and future directions. Biol. Rev 97, 1449–1475. doi: 10.1111/brv.12850
Endo H., Sato Y., Kaneko K., Takahashi D., Nagasawa K., Okumura Y., et al. (2021). Ocean warming combined with nutrient enrichment increases the risk of herbivory during cultivation of the marine macroalga Undaria pinnatifida. ICES. J. Mar. Sci. 78, 402–409. doi: 10.1093/icesjms/fsaa069
Enquist B. J., Brown J. H., West G. B. (1998). Allometric scaling of plant energetics and population density. Nature 395, 163–165.
Filbee-Dexter K., Scheibling R. (2014). Sea Urchin barrens as alternative stable states of collapsed kelp ecosystems. Mar. Ecol. Prog. Ser. 495, 1–25.
Fillbee-Dexter K., Wernberg T. (2020). Substantial blue carbon in overlooked Australian kelp forests. Sci. Rep. 10, 1–6. doi: 10.1038/s41598-020-69258-7
Gao X., Endo H., Taniguchi K., Agatsuma Y. (2013). Genetic differentiation of high-temperature tolerance in the kelp Undaria pinnatifida sporophytes from geographically separated populations along the pacific coast of Japan. J. Appl. Phycol. 25, 567–574.
Gao X., Endo H., Taniguchi K., Agatsuma Y. (2014). Effects of experimental thinning on the growth and maturation of the brown alga Undaria pinnatifida (Laminariales; phaeophyta) cultivates in matsushima bay, northern Japan. J. Appl. Phycol. 26, 529–535.
Givnish T. J. (1988). Adaptation to sun and shade: A whole-plant perspective. Aust. J. Plant Physiol. 15, 63–92.
Hasegawa K., Suzuki S. (2005). Work analysis of harvesting and salt-preserved wakame seaweed (Undaria pinnatifida) processing. Tech. Rep. Nat. Res. Inst. Fish. Eng. 27, 61–80.
Hay C. H., Luckens P. A. (1987). The Asian kelp Undaria pinnatifida (Phaeophyta: Laminariales) found in a new Zealand harbour. N. Z. J. Bot. 25, 364–366.
Hori T. (1981). Influence of warm drainage from nuclear power plants on living things (Cultivation test of Undaria pinnatifida at uchiura bay, fukui prefecture, Japan). Aquacul. Sci. 29, 88–97. doi: 10.11233/aquaculturesci1953.29.88
Hurd C. L., Harrison P. J., Bischof K., Lobban C. S. (2014). Seaweed ecology and physiology, second edition (Cambridge: Cambridge University Press).
Hurd C. L., Law C. S., Bach L. T., Britton D., Hovenden M., Paine E. R., et al. (2022). Forensic carbon accounting: Assessing the role of seaweeds for carbon sequestration. J. Phycol. 58, 347–363.
Ishikawa Y. (1991). Analysis of quantitative traits in cultured wakame for breeding. Fish. Genet. Breed. Sci. 16, 19–24.
Ishikawa Y. (1994). Quantitative traits in cultivated wakame Undaria pinnatifida. Fish. Genet. Breed. Sci. 20, 9–16.
Kusaka K. (2004). Breaking strength and thickness of stipe blade on matured Undaria pinnatifida collected in northern coast of pref. miyagi. Miyagi. Pref. Rep. Fish. Sci. 4, 61–66.
Mak W., Hamid N., Liu T., Lu J., White W. L. (2013). Fucoidan from new Zealand Undaria pinnatifida: Monthly variations and determination of antioxidant activities. Car. Polym. 95, 606–614.
Monro K., Poore A. G. B. (2005). Light quantity and quality induce shade-avoiding plasticity in a marine macroalga. J. Evol. Biol. 18, 426–435.
Nanba N., Fujiwara T., Kuwano K., Ishikawa Y., Ogawa H., Kado R. (2011). Effect of water flow velocity on growth and morphology of cultured Undaria pinnatifida sporophytes (Laminariales, phaeophyceae) in okirai bay on the sanriku coast, northeast Japan. J. Appl. Phycol. 23, 1023–1030.
Ohno M., Matsuoka M., Dan A., Pang S., Chaoyuan W. (1999). Morphological characteristics of the wild Undaria pinnatifida strain from qindao, China, growth in naruto, Japan, as compared with the wild plants in naruto. Aquacult. Sci. 47, 61–64.
Pearcy R. W., Yang W. (1998). The functional morphology of light capture and carbon gain in the redwood forest understorey plant Adenocaulon bicolor hook. Func. Ecol. 12, 543–552.
Pérez-Cirera J. L., Salinas J. M., Cremades J., Bárbara I., Granja A., Veiga A. J., et al. (1997). Cultivo de Undaria pinnatifida (Laminariales, phaeophyta) en Galicia. Nova Acta Cient Compostelana (Biol) 7, 3–28.
Perez R., Kass R., Barbaroux O. (1984). Culture expérimentales de l’algue Undaria pinnatifida sur les côtes de France. Sci. Pêche. 343, 3–16.
Peteiro C., Freire O. (2011). Effect of water motion on the cultivation of the commercial seaweed Undaria pinnatifida in a coastal bay of Galicia, Northwest Spain. Aquaculture. 314, 269–276.
Peteiro C., Freire O. (2012). Outplanting time and methodologies related to mariculture of the edible kelp Undaria pinnatifida in the Atlantic coast of Spain. J. Appl. Phycol. 24, 1361–1372.
Peteiro C., Sanchez N., Martinez B. (2016). Mariculture of the Asian kelp Undaria pinnatifida and the native kelp Saccharina latissima along the Atlantic coast of southern Europe: An overview. Algal. Res. 15, 9–23.
Reed D. C. (1990). An experimental evaluation of density dependence in a subtidal algal population. Ecology 71, 2286–2296.
Saito Y. (1962). Fundamental study of propagation of Undaria pinnatifida. Perform. Rep. Fish. Lab. Tokyo. Univ. 3, 1–101.
Sanderson J. C. (1990). A preliminary survey of the distribution of the introduced macroalga, Undaria pinnatifida (Harvey) suringar on the East coast of Tasmania. Bot. Mar. 33, 153–157.
Sato Y., Hirano T., Ichida H., Fukunishi N., Abe T., Kawano S. (2021). Extending the cultivation period of Undaria pinnatifida by using regional strains with phenotypic differentiation along the sanriku coast in northern Japan. Phycology. 1, 129–142. doi: 10.3390/phycology1020010
Sato Y., Hirano T., Niwa K., Suzuki T., Fukunishi N., Abe T., et al. (2016). Phenotypic differentiation in the morphology and nutrient uptake kinetics among Undaria pinnatifida cultivated at six sites in Japan. J. Appl. Phycol. 28, 3447–3458.
Sato Y., Nishihara G. N., Tanaka A., Belleza D. F. C., Kawate A., Inoue Y., et al. (2022). Variability in the net ecosystem productivity (NEP) of seaweed farms. Front. Mar. Sci. 9. doi: 10.3389/fmars.2022.861932
Schiel D. R., Choat J. H. (1980). Effects of density on monospecific stands of marine algae. Nature 285, 324–326.
Schmitt J., Wulff R. D. (1993). Light spectral quality, phytochrome and plant competition. Trends Ecol. Evol. 8, 47–51.
Scrosati R. (2005). Review of studies on biomass-density relationships (including self-thinning lines) in seaweeds: Main contributions and persisting misconceptions. Phycol. Res. 53, 224–233.
Scrosati R., DeWreede R. E. (1997). Dynamics of the biomass–density relationship and frond biomass inequality for Mazzaella cornucopiae (Gigartinaceae, rhodophyta): implications for the understanding of frond interactions. Phycologia 36, 506–516.
Silva P. C., Woodfield R. A., Cohen A. N., Harris L. H., Goddard J. H. R. (2002). First report of the Asian kelp Undaria pinnatifida in the northeastern pacific ocean. Biol. Invasions. 4, 333–338.
Smale D. A. (2020). Impacts of ocean warming on kelp forest ecosystems. New Phytol. 225, 1447–1454. doi: 10.1111/nph.16107
Smith H. (1982). Light quality, photoperception, and plant strategy. Ann. Rev. Plant Physiol. 33, 481–518.
Smith H., Whitelam G. C. (1997). The shade avoidance syndrome: Multiple responses mediated by multiple phytochromes. Plant Cell Env. 20, 840–844.
Steneck R. S., Graham M. H., Bourque B. J., Corbett D., Erlandson J. M., Estes J. A., et al. (2002). Kelp forest ecosystems: Biodiversity, stability, resilience and future. Environ. Conserv. 29, 436–459. doi: 10.1017/S0376892902000322
Stuart M. D. (2004). Review of research on undaria pinnatifida in new Zealand and its potential impact on the Eastern coast of south island (Wellington, New Zealand: Department of Conservation), 40.
Takagi S., Murata Y., Agatsuma Y. (2020). Feeding the sporophyll of Undaria pinnatifida kelp shortens the culture duration for the production of high-quality gonads of Mesocentrotus nudus sea urchins from a barren. Aquaculture 528, 735503.
Takagi S., Murata Y., Inomata E., Agatsuma Y., et al. (2020). Sporophyll of Undaria pinnatifida: A potential feed for the production of high-quality gonads in the sea urchin Mesocentrotus nudus (A. Agassiz, 1864). J. Appl. Phycol 32, 1467-1475.
Takenaka A. (1994). Effects of leaf blade narrowness and petiole length on the light capture efficiency of a ahoot. Ecol. Res. 9, 109–114.
Taniguchi K., Kito H., Akiyama K. (1981). Morphological variation of Undaria pinnatifida (Harvey) suringar -1. on the difference of growth and morphological characteristics of two types at matsushima bay, Japan. Bull. Tohoku. Reg. Fish. Res. Lab. 42, 1–9.
Troell M., Henriksson P. J. D., Buschmann A. H., Chopin T., Quahe S. (2022). “Farming the ocean – seaweeds as a quick fix for the climate?,” in Rev. Fish. Sci. Aquacult. doi: 10.1080/23308249.2022.2048792
Watanabe K., Yoshida G., Hori M., Umezawa Y., Moki H., Kuwae T. (2020). Macroalgal metabolism and lateral carbon flows can create significant carbon sinks. Biogeosciences 17, 2425–2440. doi: 10.5194/bg-17-2425-2020
Wotton D. M., O’Brien C., Stuart M. D., Fergus D. J. (2004). Eradication success down under: Heat treatment of a sunken trawler to kill the invasive seaweed Undaria pinnatifida. Mar. Pol. Bul. 49, 844–849.
Keywords: climate change, invasive species, intraspecific competition, shade avoidance response, Undaria pinnatifida, commercial profit, aquaculture
Citation: Sato Y, Fujiwara T and Endo H (2023) Density regulation of aquaculture production and its effects on commercial profit and quality as food in the cosmopolitan edible seaweed Undaria pinnatifida. Front. Mar. Sci. 10:1085054. doi: 10.3389/fmars.2023.1085054
Received: 31 October 2022; Accepted: 30 January 2023;
Published: 10 February 2023.
Edited by:
Wenlei Wang, Jimei University, ChinaReviewed by:
Pablo P. Leal, Instituto de Fomento Pesquero (IFOP), ChileTifeng Shan, Institute of Oceanology (CAS), China
Copyright © 2023 Sato, Fujiwara and Endo. This is an open-access article distributed under the terms of the Creative Commons Attribution License (CC BY). The use, distribution or reproduction in other forums is permitted, provided the original author(s) and the copyright owner(s) are credited and that the original publication in this journal is cited, in accordance with accepted academic practice. No use, distribution or reproduction is permitted which does not comply with these terms.
*Correspondence: Hikaru Endo, aC1lbmRvQGZpc2gua2Fnb3NoaW1hLXUuYWMuanA=