- 1Centre for Planetary Health and Food Security, Griffith University, Nathan, QLD, Australia
- 2Port of Brisbane Pty. Ltd., Lytton, QLD, Australia
Humpback whales enter Moreton Bay, in southeast Queensland, Australia, each year during their annual migration. Little is known about the ecological significance of the bay for the humpback whale population. In a region characterised by rapid coastal and maritime development, as well as a growing humpback whale population, there is an urgent need to fill knowledge gaps surrounding the populations’ seasonal distribution and habitat use in these coastal waters. This study procured the first detailed information regarding humpback whale distribution, behaviour, and habitat use within Moreton Bay, relative to the main east coast migratory corridor. It was found that on average 42.41% of the individuals observed on the southern leg of the migration entered the bay. 76.78% of pods entering the bay had accompanying calves and 47.82% of these pods were found to be resting or logging, a behaviour often associated with nursing, at the time of observation. These findings provide strong evidence for a previously undocumented role of Moreton Bay as a resting stopover for migrating humpback whales.
1 Introduction
Southern hemisphere humpback whales (SHHW) (Megaptera novaeangliae) have evolved an extreme migratory life history that exploits the seasonal productivity of their principal prey item, Antarctic krill (Euphausia superba). Their annual migrations between the Southern Ocean and equatorial breeding grounds are among the longest migratory journeys of any mammal on Earth, covering c.a.10 000 km (Andriolo et al., 2006; Zerbini et al., 2006). The migration is associated with five to nine months of fasting, representing a period of prolonged negative energy balance, exacerbated among females that simultaneously bear the energetic demands of pregnancy, parturition, and lactation. Consequently, individuals may lose between one-third to one-half of their summer body mass during this time (Slijper, 1962; Dawbin, 1966; Lockyer and Brown, 1981; Baraff et al., 1991; Clapham et al., 1999). Understanding the species’ energy conserving behaviours and strategies is of increasing importance in a rapidly changing Antarctic sea-ice ecosystem.
Managing energy balance is of particular importance for capital breeders such as SHHWs that fund the energetic cost of reproduction via temporally constrained periods of hyperphagia (Frisch, 1984; Gittleman and Thompson, 1988; Jönsson, 1997; Stephens et al., 2009). A single sub-optimal feeding season can carry individual fitness consequences, such as exhaustion, immunosuppression, and excessive mobilisation of lipophilic environmental toxins (Jönsson, 1997; Bengtson Nash et al., 2013; Bengtson Nash, 2018; Bengtson Nash et al., 2018). For sexually mature females, single or repeated sub-optimal feeding seasons may further carry population consequences via larger inter-pregnancy intervals, sub-optimal maternal provisioning of foetus’ and dependent calves, or aborted pregnancies (Lockyer, 1986; Lockyer, 2007; Williams et al., 2013; Christiansen et al., 2014; Christiansen et al., 2016; Christiansen et al., 2018; Castrillon and Bengtson Nash, 2020; Christiansen et al., 2022).
Most SHHW populations are recovering from their post-whaling bottleneck, with some approaching pre-whaling numbers (Paterson et al., 2001; Noad et al., 2011). Concerns are, however, growing for many migratory species utilising increasingly impacted and fragmented habitats. Degradation of habitats through industrial, agricultural, and residential development, climate change, and pollution are some of the major threats that migratory species face (Robinson et al., 2005). Due to the extensive geographical range that these species occupy throughout their annual cycle (Runge et al., 2014; Schuster et al., 2019), conservation efforts are complicated by their migration across the jurisdictional limits of various nations (Ruckelshaus et al., 2008; Miller et al., 2018). The Convention on Conservation of Migratory Species of Wild Animals (CMS), also known as the Bonn Convention, was established in 1979 and seeks to conserve migratory species across their entire range. Conservation efforts primarily focus on breeding and feeding areas (Mehlman et al., 2005; Sheehy et al., 2011), with migratory corridors often neglected. Notably, many species of migratory ungulates, birds, and sea turtles (Shillinger et al., 2008; Harris et al., 2009; Murray et al., 2014; Runge et al., 2015), have dramatically declined in numbers despite well-established protected areas at both migration terminus points. For SHHWs that seasonally migrate between Antarctic feeding grounds, far removed from permanent human settlements, to urbanised coastal areas, the importance of safeguarding the entire migratory corridor of the species’ range is particularly well exemplified.
The major drivers behind the seasonal migration of SHHWs out of Antarctica remain under active theoretical debate. Aside from diminished winter food availability, avoidance of predation at high latitudes (Corkeron and Connor, 1999), calf winning mass (Norris, 1967), and more recently skin molting (Pitman et al., 2020), are all theories that have been proposed. Such uncertainty regarding the fundamental ecology of the species, translates to carry-over uncertainty when forecasting the response of populations to rapidly changing functional habitats. In extra-Antarctic waters SHHWs are known to travel in close proximity to the coastline, preferring routes less than 200 m in depth, and generally within 20 km of the coast (Peel et al., 2018). Travel from northern breeding grounds, with accompanying newborn calves, is characterised by slower travel close to shore, with frequent resting stops (Simmons and Marsh, 1986; Mattila et al., 1989; Ersts and Rosenbaum, 2003; Félix and Guzmán, 2014). A ‘stopover site’ for migratory species is a site where the migration is paused to rest, conserve energy, and refuel (Mehlman et al., 2005; Dingle and Drake, 2007). Such sites, therefore, carry a disproportionate impact on an individual’s mass gain and reproductive success, relative to time spent there (Drent et al., 2003; Smith and Moore, 2003; Schaub et al., 2008; Sheehy et al., 2011). The social and physiological drivers of humpback whale resting stopovers have previously been described (Chittleborough, 1953; Corkeron et al., 1994; Jenner et al., 2001; Braithwaite et al., 2012), and can summarised as a pause in migratory travel for mothers to engage in nursing and socialising with calves-of-the-year and older calves during the early stages of their southern migration (Franklin et al., 2011), contributing to the social development and high survival rates of calves and younger humpback whales (Franklin, 2014). Females with accompanying calves will favour lagoons with calm, shallow, and warm waters (Bruce et al., 2014; Ejrnæs and Sprogis, 2021) and utilise these areas as resting stopovers as the conditions facilitate coordination of nursing behaviour, and protect the calves from rough sea conditions, predators, and aggression from sexually active males (Craig et al., 2014; Bejder et al., 2019; Ejrnæs and Sprogis, 2021). Resting and nursing in these lagoon habitats, further plays a critical role in optimising energy balance of both mother and calf by minimising energy expenditure and maximising conversion of the mother’s lipid rich milk to calf body mass. Recently, Videsen et al. (2017) quantified calf nursing dynamics and found that mothers and young calves were engaged in resting and nursing 20% of the total time observed. Resting stopover sites are therefore of key functional significance for SHHWs and even a relatively small amount of habitat disturbance or loss could carry negative population impacts (Runge et al., 2014) through for example disrupted nursing behaviour, or increased travel distance between suitable resting stopovers.
By comparison to feeding and breeding grounds, the ecological importance of resting stopovers have received less attention (Possingham et al., 2001; Wilson et al., 2006), yet the importance of properly identifying, characterising, and effectively managing human interactions in such areas are clear. For Australia’s transient humpback whale populations, the Australian coastline offers numerous potential resting stopovers, yet only a few have been adequately characterised and formally recognized in the literature, notably Exmouth Gulf in Western Australia and Hervey Bay in Queensland (Jenner et al., 2001; Franklin et al., 2011; Franklin, 2014; Bejder et al., 2019).
Moreton Bay is located approximately 1700km south of the Great Barrier Reef, the breeding ground of the east coast of Australia migrating humpback whale population (Paterson and Paterson, 1984). It is a semi-enclosed embayment, sheltered by the large sand Islands, Moreton Island (Mulgumpin) and North Stradbroke Island (Minjerribah) to the east. The average winter sea surface temperature in the bay is 20°C with an average depth of 8m (Pantus and Dennison, 2005). The natural physical environment of Moreton Bay offers similar conditions to other well-established SHHW resting stopovers. The bay, however, it is also located adjacent to one of Australia’s fastest developing regions, and is the location of Australia’s fastest growing container port, the Port of Brisbane. Humpback whales are regularly sighted within Moreton Bay, however, little is known about the proportion of the population that utilises the bay, their distribution, or their habitat use; all necessary parameters for effective management of human-wildlife interactions. This study sought to quantify these through systematic species abundance and distribution surveys across five consecutive years (2017 – 2021). In addition, surveys covered the main migratory corridor as a reference for findings. Here we describe the migratory dynamics and behaviours observed which are of broad relevance for the management of Moreton Bay, and similar embayment along Australia’s migration corridors.
2 Materials and methods
2.1 Survey areas
Two spatial areas to the east and west of North Stradbroke and Moreton Islands, respectively (Figure 1) were surveyed over 5 consecutive years (2017 -2021). The two survey areas corresponded to 1) the sheltered Moreton Bay waters, between the Australian mainland and North Stradbroke and Moreton Islands (Area 1), and 2) the main Coral Sea migratory corridor, along the east coast of North Stradbroke and Moreton Islands (Area 2). Moreton Bay (Area 1) covered an area of 2284.9 km², from Mooloolaba in the north, to Thornlands in the south (approximately 95 km). This area has a high level of commercial and recreational maritime traffic and encompasses the main shipping channel across the bay, that facilitates high levels of commercial traffic to and from the Port of Brisbane, as well as several commercial ferry operating routes. The main migratory corridor (Area 2) study area was reduced between 2017 and 2018 to dedicate greater focus to Area 1, the area of study interest. In 2017, A1 covered 834.7 km2 from the north of Moreton Island, south to the former Yarraman mine site on North Stradbroke Island (approximately 60 km). In 2018-2021 the northern boundary was moved south to the middle of Moreton Island (approximately 30 km), reducing the total survey area to 438 km² (Figure 1).
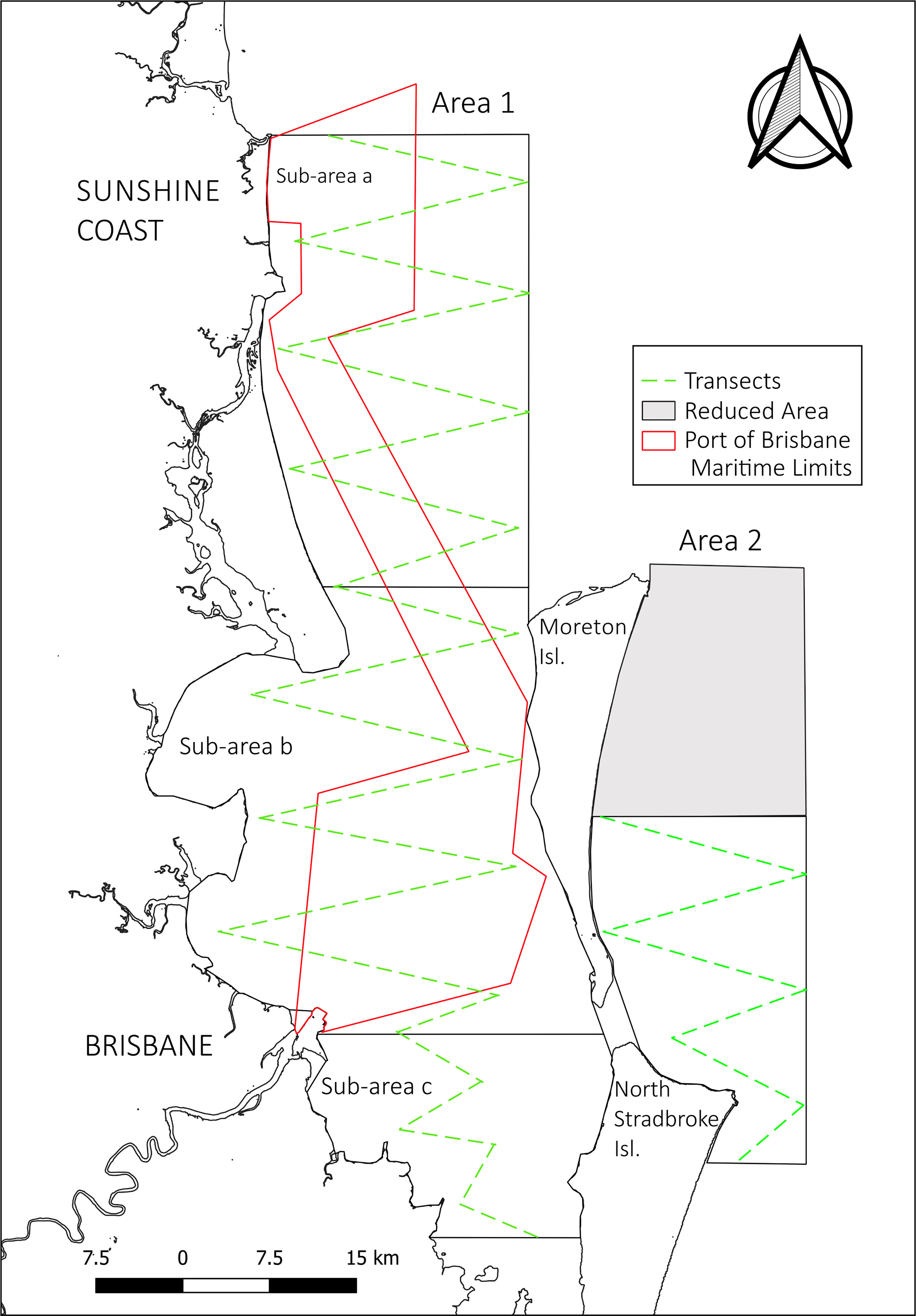
Figure 1 Survey Areas 1 (sub-areas a, b and c) and 2, depicted with the Port of Brisbane limits and transect design. The grey area depicts the survey area removed from 2017 to subsequent years.
In order to explore localised habitat use, Area 1 was divided into three sub-areas (Figure 1). Sub-area a, with an area of 853 km², represents the northern part of Area 1, stretching from Mooloolaba to Moreton Island. Although part of this area belongs to Moreton Bay Marine Park, it extends beyond the enclosed bay area into open water with an ocean depth of more than 30 m. The area also encompasses the northern part of the shipping channel which traverses the bay from Mooloolaba to the Brisbane River mouth (90 km) and is dredged to maintain a minimum depth of 14 m. Sub-area b with an area of 1,076 km², covers the northern part of the Moreton Bay embayment stretching from the top of Moreton Island to the Port of Brisbane. This area is characterized by shallow waters with a depth from 1 to 15 m and encompasses the southern part of the shipping channel. Sub-area c with an area of 357 km², represents the southern part of Moreton Bay, with very shallow waters ranging from 1 to 10 m. This area does not include any part of the shipping channel, although coincides with the ferry routes of several vehicle and passenger ferries and is also the area with greatest recreational boating activity.
2.2 Sampling design
Data collection was performed via boat-based line transect sampling methodology (Buckland et al., 1993). The transect design was mapped with an effective sampling band of 2.5 km in a zigzag formation (Figure 1). This systematic method incorporates observer effort thus allowing species presence and absence to be quantified. Transect sampling was performed at a travel speed of 8 knots, conducted against the direction of whales’ migration to minimise the possibility of animals being recorded twice. Within Area 1, where the whales’ direction of travel is less consistent, fluke and dorsal photos were obtained to exclude duplicate sighting recordings. Two observers positioned at the bow of the vessel at 2m above sea level, actively scanned with their naked eyes an angle of 100° each covering the starboard or port side from 90° to 10° beyond the bow, thus ensuring animals on the transect line were detected, and satisfying distance sampling assumptions that the detection probability g(0) was 1. Upon sighting an animal, observers used an angle board to measure the radial angle, and estimated the radial distance to the sighting. These were recorded, as well as the boat’s location using a Global Position System (GPS) and the sighting time. Depending on the pod distance, the decision to leave the transect and approach the pod to obtain detailed information on pod composition, size, behaviour, exact GPS position and photo-identification, was made. In these instances, when all the necessary information had been collected, the survey vessel returned to the point where the transect had been abandoned and the transect survey resumed. Depending on the number of trained personnel on the vessel the observers and the captain rotated each transect to rest. Surveys were only conducted under optimal sea conditions (Beaufort scale <4). Surveys were conducted for 5-6 weeks across the peak of each migration events at this latitude, i.e. during June – July (northward migration) and September – October (southward migration), when the whales are travelling to and from their winter breeding grounds respectively. Ancillary environmental data, such as sea state (Beaufort), wind direction and cloudiness were also collected at the start of each transect, as well as if conditions changed throughout. Whale sightings made whilst travelling along a transect were categorized as ‘on effort’ observations. When sightings were made whilst not travelling along a transect (e.g. from the launching point to the start of a transect point), these were categorized as ‘off-effort’ sightings.
2.2.1 Area correction
To account for both the change in the size of the reference area (Area 2) from 2017 to subsequent years, as well as the much larger survey area of Area 1 compared to Area 2, all gross sighting numbers were standardised by survey area covered. i.e.
Unless stated otherwise in the text, all calculations have been based on area corrected values.
Whale density is defined as the number of individuals/km2
2.2.2 Pod size and composition
Pod composition was categorised as outlined in Table 1 below.
2.2.3 Behaviour
For the purpose of this study, the different behaviours were categorised as outlined in Table 2.
To better understand the use of the Sub-areas of Area 1, a geographic information system (GIS) model of the study area was constructed using QGIS 3.22 (Quantum Geographical Information System) (QGIS, 2009) Australian coastal data were incorporated as a vector layer, and depth data were incorporated as a 5 m bathymetric grid. GPS coordinates of pods with accompanying calves resting and logging within the bay, were plotted to obtain these groups depth information.
2.3 Statistical analysis
A Chi-squared test of independence (p=0.05) was used to evaluate the significance of key pod composition and behavioural differences observed between study Areas 1 and 2.
3 Results
3.1 Survey effort
During the five-year sampling period, 41 survey days were conducted during the northern migration, between the 14th of June and 12th of July. Survey effort corresponded to 23 days in Area 1 and 18 days in Area 2. A total of 81 transects in Area 1 (1420.25 km) and 29 transects in Area 2 (425.21 km) were completed. A total of 49 survey days were conducted during the southward migration, between the 12th of September and 19th of October. Here, 31 days were dedicated to Area 1 and 18 days to survey Area 2. A total of 100.5 transects in Area 1 were completed (1851.1km) and 35 transects in Area 2 (534.94 km), (Table 3). No surveys were carried out during the northward migration of 2021. All Area 2 transects were covered across the five years surveyed. By contrast, consecutive stretches of poor weather, combined with a high level of whale encounters, prevented completion of the transect network in Area 1 during the northward migration 2019, with approximately 16.72% of the transect distance in Area 1 not completed (3 transects). Similarly, the transect network in Area 1 was not completed during the southward migration of 2017 and 2018, with approximately 20% (4.5 transects) and 6% (1 transect) of the transect distance not completed, respectively.
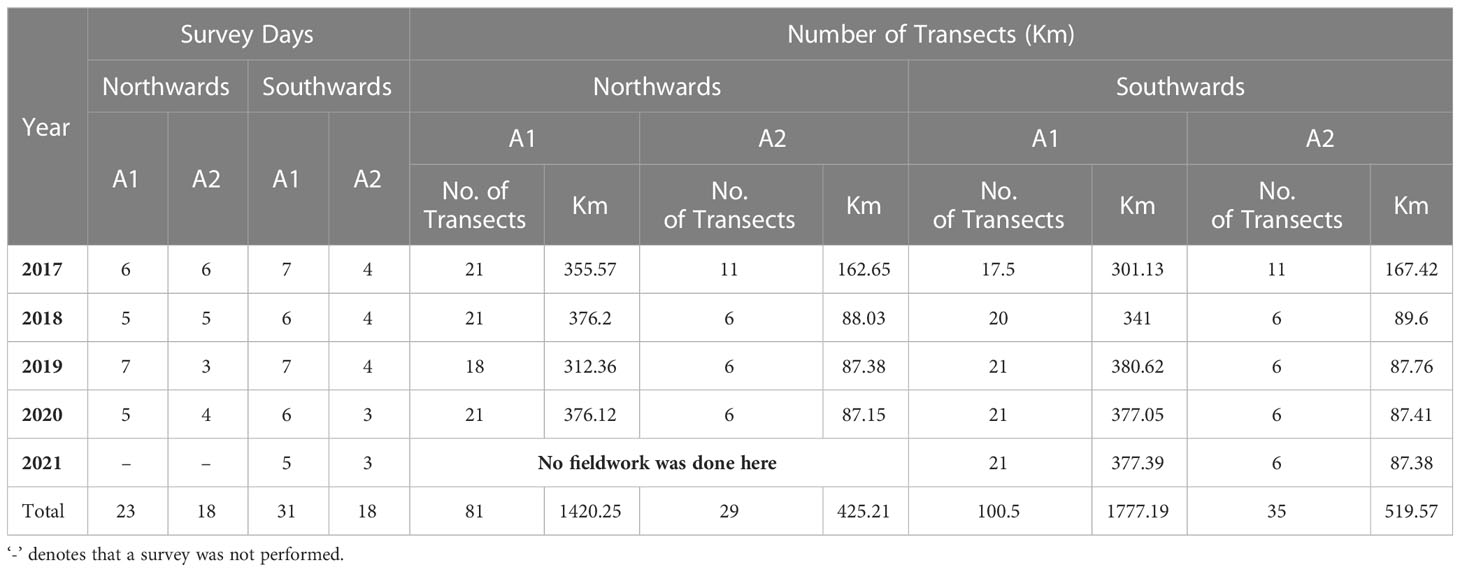
Table 3 Summary of survey effort, including survey days and transect distance covered in each respective area during the northward and southward migrations of the five years survey.
3.2 Whale numbers and density
A total of 813 individual humpback whales were sighted during transect surveys associated with both the northward and southward field campaigns during 2017-2021 (Table 4). A further 536 individuals were sighted off-effort, whilst travelling to or from transects. Off-effort individuals were included only for the evaluation of whale distribution and habitat use, but not for abundance and density calculations.
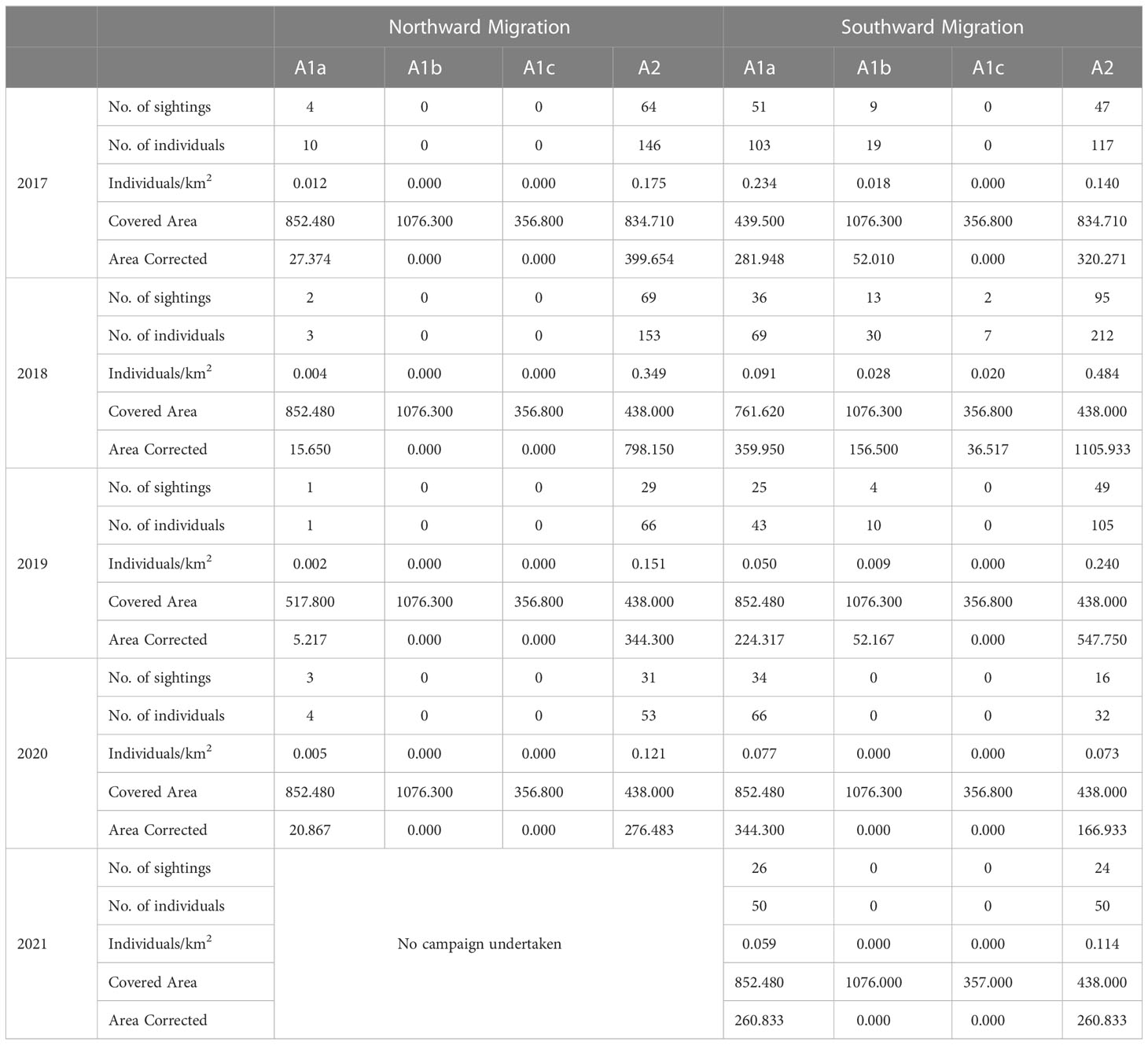
Table 4 Humpback whale numbers and density in allocated areas per year, using on and off effort data.
During the northward migration, a total of 18 individuals across 10 sightings were observed in Area 1. This number rose to 397 individuals across 200 sightings on the southward migration in gross number terms.
On an area-corrected basis, the proportion of individuals observed in each area, during the five-year survey period, were as follows: on the northward migration, Area 1 animals corresponding to 3.66% of individuals observed during this period. By contrast, on the southward migration, individuals observed in Area 1 rose to 42.41% of individuals recorded during the migration.
The density of whales, as calculated by area and sub-areas, is presented in Table 4. Area 2 presented the highest density of whales during all northward migration campaigns, which was > ten times higher than the density in Area 1 during the same period. The low density of whales found in Area 1 during the northward migration, was concentrated entirely in Sub-area a (Figure 2). During the southward migration, the density of whales in Area 1 increased substantially (from 0.021 Individuals/km² to 1.113) with a maximum density across the survey period found in Sub-area a in 2017 (0.234), exceeding the density of whales in Area 2 during this same year (0.140).
3.3 Distribution
The spatial distribution of individuals changed markedly between the northward and southward migration timepoints. During the northward migration, distribution was highly biased toward Area 2. Only 3.66% (n=18, area corrected(ac)=69.10) of the observed northward migrating individuals were observed in Area 1, all of which were within Sub-area a (Figure 3). During the southward migration, the presence of individuals increased at least 10-fold in Area 1, corresponding to an average 42.41% (n=397, ac=1768.54) of the total individuals migrating south (35.28%, n=331, ac=1471.34 in Sub-area a; 6.25%, n=59, ac=260.67 in Sub-area b; and 0.87%, n=7, ac=36.51 in Sub-area c) (Figure 4). Although most of the sightings within Area 1 were concentrated in the northern part of the area, the whales also aggregate throughout the bay from the middle of Bribie Island, south to Moreton and North Stradbroke Islands. Sightings were made as far south as Cleveland, at the bottom of Area 1, decreasing in frequency from north to south. In the southern section of Moreton Bay, sightings were biased toward the east, in line with the sheltered coasts of Moreton Island. No sightings were made along the greater Brisbane coastline.
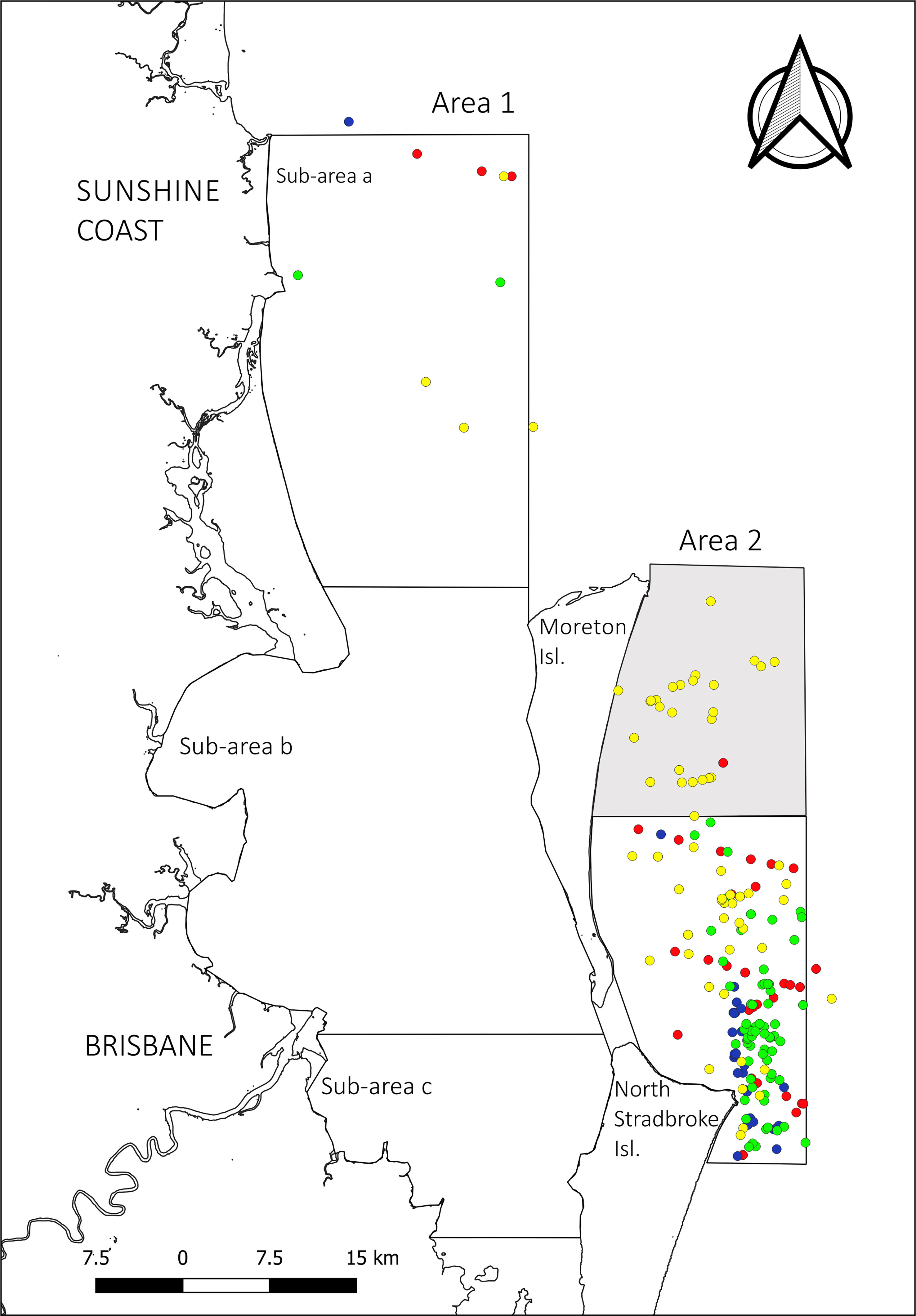
Figure 3 Compilation of humpback whale sightings during the northward migration over the five-year study period. In yellow 2017, in green 2018, in blue 2019, in red 2020 (no fieldwork occurred during 2021 northward migration). Shaded portion of A2 denotes the area covered in 2017 but not in subsequent years.
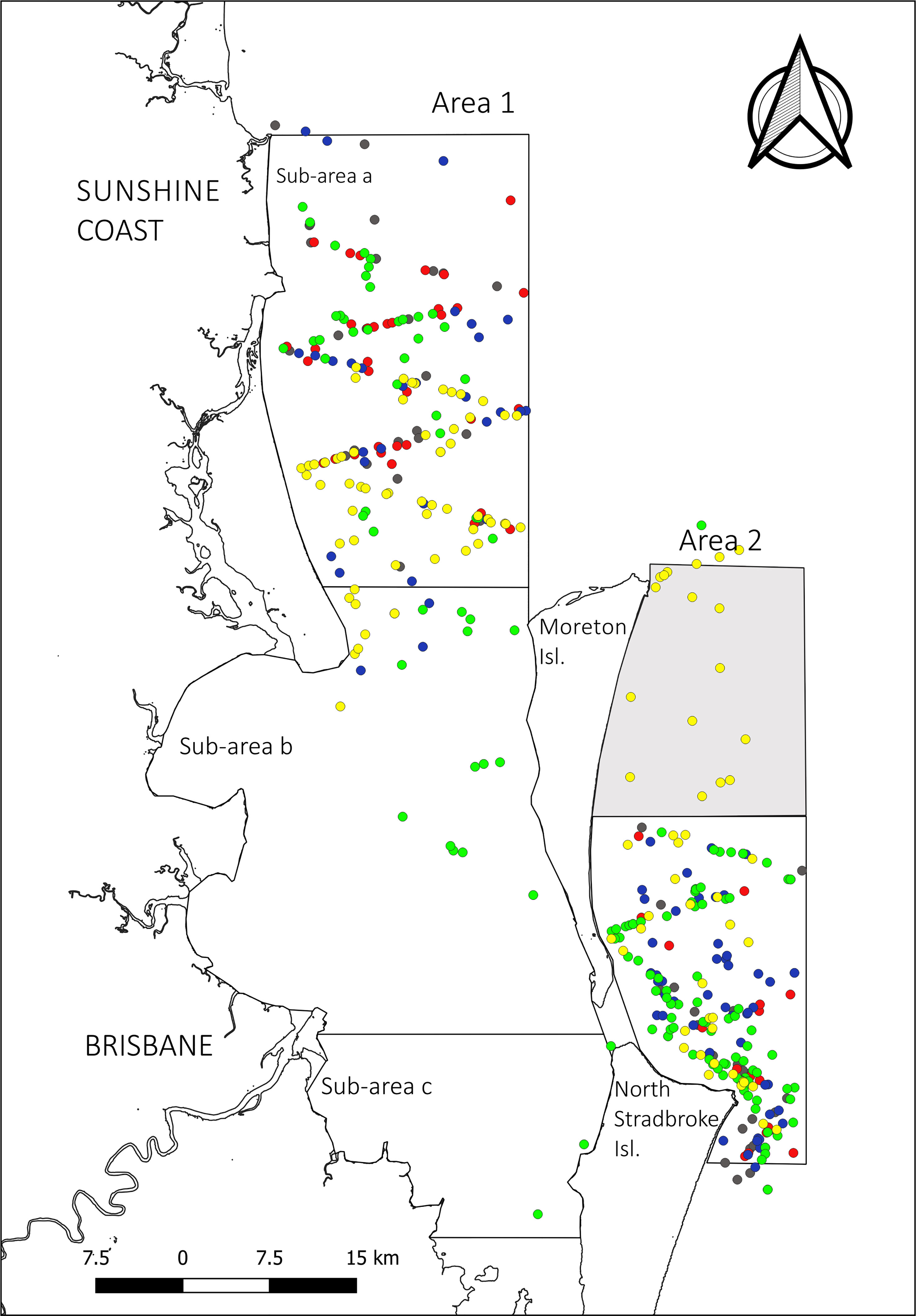
Figure 4 Compilation of humpback whale sightings during the southward migration across the five-year study period. In yellow 2017, in green 2018, in blue 2019, in red 2020 and in grey 2021. Shaded portion of A2 denotes the area covered in 2017 but not in subsequent years.
3.4 Pod size and composition
3.4.1 Pod size
The modal pod size in Area 1 increased from 2 (range 1-3) to 3 (range from 1-6) between the northward and southward migrations. In Area 2, the modal pod size was 2 (range 1-10) during both (Figure 5).
3.4.2 Pod composition
The predominant pod composition types during the northward migration by area were solo whales and non-competitive pods for Area 1, and non-competitive pods followed by pairs for Area 2. This dynamic changed during the southward migration, particularly for Area 1 where pods with calves made up 76.78% of all sightings, comprised of 47.32% mother-calf pairs (MC) and 25.89% mother-calf pairs accompanied by an escort (MCE). By contrast, MC pairs represented just 12.6% of observations for Area 2 on the southward migration, a defining difference between the two areas (X2 (1, N=130) = 13.569; p=0.001). Instead, the predominant pod composition in Area 2 during the southward migration was pairs, representing 25.8% of observed pods in the area (Figure 6).
3.4.3 Calf pods
Of the pods observed with accompanying calves on the southward migration, more than half (61.6%) in Area 1 were MC pairs alone (Table 1), whereas mother-calf pairs were accompanied by an escort (Table 1) in 33.7% of the sightings. In a small percentage (4%) of sightings in Area 1 during the southward migration, a mother-calf pair were accompanied by a non-competitive pod (NCP+C) (Table 1) (Figure 7). By comparison to Area 1, MC pods made up 43% of calf pods in Area 2 during the same period, 34% had an accompanying escort, whilst 22% were mother calves travelling as part of a non-competitive pod (NCP+C).
3.5 Behaviour
Behavioural data was recorded on 276 pods in total, 99 during the northward migration and 177 during the southward migration. Travelling was the most common behaviour observed in Area 2 throughout the entire five-year study period (57.9% of individuals on the northward migration and 57.3% of individuals on the southward migration) (Table 2). By contrast, behaviours observed most frequently in Area 1 changed markedly between the two timepoints, coinciding with the influx of greater whale numbers into the area. During the northward migration, the dominant behaviours among the few whales entering Area 1 were breaching, tail and fin slapping and traveling. During the southward migration, the main behaviour observed was breaching (33.3%) followed by resting (28.8%) (Figure 8). Notably, resting whales made up only 4.45% of whales in A2 during the southward migration, compared to 28.88% of observed behaviours in A1, a highly significant (p<0.00001) difference between the two study areas.
3.5.1 Whale behaviours in area 1 sub-areas a, b, and c during the southward migration
In an attempt to better understand the nature and extent of habitat use by migrating humpback whales in Moreton Bay, whale behaviours were explored according to Sub-areas in Area 1 (Figure 9). Sub-areas a and b presented all coded behaviours. The predominant behaviour in Sub-area a was breaching (36.4%), followed by resting (24.2%), while resting (46.1%) was the most common behaviour in Sub-area b. Only two sightings were made in Sub-area c with traveling and resting as the recorded behaviours respectively.
3.5.2 Calf pods
When behaviours were further separated by pods with calves, it was found that 42.4% (n=39) of the pods with accompanying calves were observed resting and a further 5.4% (n=5) logging (Figure 10) at the time of observation. 76% (n = 38) of these sightings were made in water between 5 and 20 m (median 12.3m), and the remaining 24% (n=12) in a water depth greater than 20m (median 37.1m).
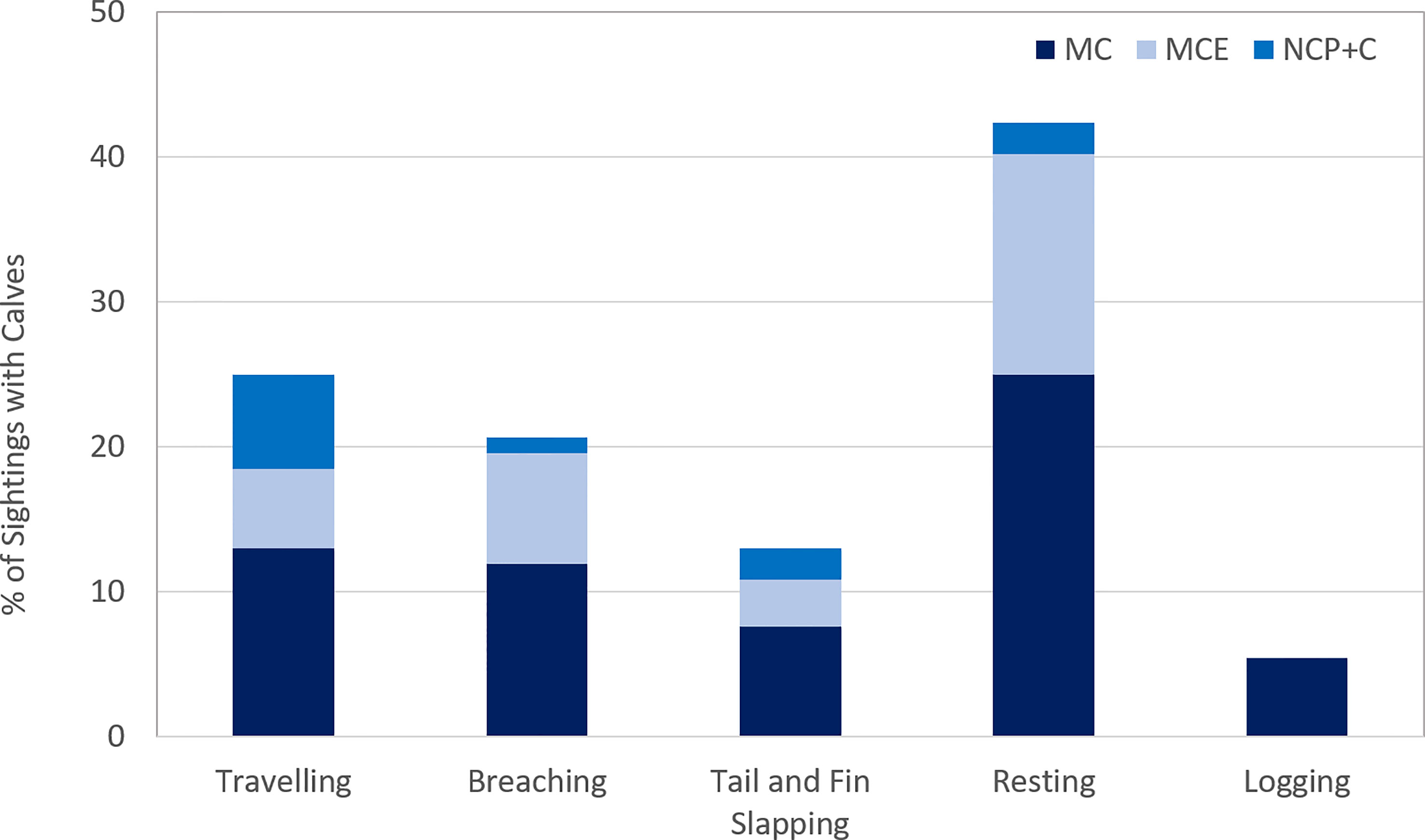
Figure 10 Behaviours recorded in Area 1, during the southward migration, in groups with calves. MC: mother and calf; MCE: mother, calf and escort; NCP+C: non-competitive pod and calf.
4 Discussion
Quantification of distribution, relative abundance, behaviour, and habitat of migrating humpback whales in Moreton Bay, over a five-year period, revealed substantive evidence that Moreton Bay is not only visited by a significant proportion of the annual migratory cohort, but that it also that it plays a functional role as a resting stopover for this population.
The unique significance of Moreton Bay, particularly to mothers and calves, was demonstrated by the marked change in spatial habitat use between the two migration time-points. Regardless of the survey year, there was a substantial increase in the number of whales entering the bay between the northward and southward migrations. The most notable feature of whale utilisation of Area 1 on the southward migration was the influx of pods with accompanying calves. Pods with calves made up 76.7% of sightings in Area 1 during the southward migration, whereas pods with calves amounted to less than half of the sightings in Area 2. By comparison to Area 1, consistent whale numbers were observed in Area 2 across both time periods, supporting the well-known role of Area 2 as a main migratory corridor of this population.
The role of Moreton Bay as a resting stopover for the east coast of Australia migrating humpback whale population was further supported by the observation that of the pods with accompanying calves observed within the bay, 47.82% were resting or logging at the time of observation. These findings suggest a unique functional role of Moreton Bay, particularly for mother-calf pairs. The shallow, and sheltered, lagoon-like habitat present in Moreton Bay match those described for resting stopovers in other regions in Australia, such as Hervey Bay (Franklin, 2014), Jervis Bay (Bruce et al., 2014), and Exmouth Gulf (Jenner et al., 2001; Bejder et al., 2019). These shallow lagoon-type habitats offer protection to calves from turbulent offshore conditions, predators, or aggression from sexually active males (Whitehead and Moore, 1982; Glockner and Venus, 1983; Smultea, 1994; Ersts and Rosenbaum, 2003; Craig et al., 2014). It has been suggested that premature conspecific social encounters increase the risk of elevated energy expenditure, interruption of nursing bouts, mistaken imprinting, or nursing attempts, potential separation of calves from mums, and calf injury or death (Jones and Swartz, 1984; Thomas and Taber, 1984; Smultea, 1994; Craig et al., 2014; Ransome et al., 2022). In addition, these waters facilitate effective coordination of nursing behaviour (Whitehead and Moore, 1982; Glockner and Venus, 1983; Smultea, 1994; Ersts and Rosenbaum, 2003). Stationary nursing, is energetically advantageous for both mother and calf; as it allows the mother to provide energy to her newborn while conserving as much of her own limited energy stores, and at the same time allows the calf to allocate energy for growth rather than movement (Harrison, 1969; Herman and Tavolga, 1980). While nursing behaviour is challenging to study in cetaceans since suckling occurs below the surface and milk transfer is difficult to verify, much of the literature describes that nursing behaviour occurs at a depth of 10 to 20 m (Glockner and Venus, 1983; Cartwright, 2005). In the current study, 76% of the pods with calves observed to be resting or logging were in waters between 5 and 20m in depth at the time of observation. Although this study did not attempt to detect or quantify nursing behaviour, the prevalent observations of resting and logging whales with calves in shallow waters, commensurate with nursing activity, provide strong indication that nursing occurs within the bay. The large proportion of time that mother-calf pairs dedicate to nursing and resting (Videsen et al., 2017; Bejder et al., 2019; Ejrnæs and Sprogis, 2021), is reflective of the critical importance of energy balance in this extreme migrating species, and the implications for individual fitness. For example, bigger calf size has been observed to be associated with better breath-holding ability, reduced vulnerability to predators, reduced heat loss during migration to high latitudes, and greater resilience to environmental variability (Christiansen et al., 2018).
Given the significant functional importance of resting stopovers for humpback whale energy balance, the discovery of Moreton Bay as a previously undocumented resting stopover is of significant conservation and management importance. Moreton Bay exhibits high levels of species diversity and richness but is also a region of high levels of all-year maritime traffic (Maritime Safety Queensland, 2005). As such, whales entering the bay must increasingly navigate anthropogenic stressors and threats such as disturbance and ship strike (Mayaud et al., 2022). Vessel proximity and underwater noise may disrupt critical nursing and resting behaviours (Pirotta et al., 2019), whilst whales engaged in resting and nursing are known to be less responsive to approaching vessels, and therefore more susceptible to ship strike (Laist et al., 2001). Ship strike risk is further exacerbated among calves and young that spend a greater amount of time at the surface (Laist et al., 2001; Lammers et al., 2013). Finally, vulnerability of this demographic group is further compounded by the low detectability of resting animals, and the restriction of avoidance by both whales and ships at shallow depths. Whilst further work is needed to understand the risk of humpback whale ship-strike from a range of vessel-categories in Moreton Bay (Mayaud et al., 2022), the change in vessel traffic across Area 1 sub-areas a, b and c, likely provide clear clues as to the major risks associated with each sub-area. Sub-area a, which forms the entrance to the main Port of Brisbane shipping channel, is dominated by larger commercial vessels aggregating from deeper offshore waters to enter into the bay (Mayaud et al., 2022). The absence of the shipping channel in sub-area c results in a lack of large commercial vessels further south in the bay. By contrast, the protected inshore waters of sub-areas b and c, coincide with numerous islands, reefs, and fishing grounds making these areas particularly popular for smaller recreational vessels, as well as regular passenger ferry traffic.
5 Conclusions
Systematic abundance and distribution surveys of Moreton Bay, and the adjacent main migratory corridor, over the five-year period provided substantive evidence for the previously undocumented role of Moreton Bay as a resting stopover for the east coast of Australia migrating humpback whale population. Notably, the current study revealed a temporally constrained influx of whales into the bay during the southward migration, particularly by pods with accompanying calves. Pods with accompanying calves were further reported to be resting or logging 47.82% of the time, indicative of nursing behaviour. These findings present a local environmental management challenge for the sustainable use of this rapidly developing region. Further, they bring focus to the need for identifying similar resting stopover sites of these extreme migratory populations across their species range as continued degradation of key stopover sites will carry a disproportionate impact to species’ energetic budgets.
Data availability statement
The raw data supporting the conclusions of this article will be made available by the authors, without undue reservation.
Ethics statement
The animal study was reviewed and approved by Griffith University Animal Ethics Committee.
Author contributions
JC and SB conceived and designed the study. JC, GD, JA and RM Performed fieldwork and data collection. JC and SB analysed the data. JC took the lead in writing the manuscript with substantial support from SB. All authors contributed to the article and approved the submitted version.
Funding
This work was funded by The Port of Brisbane Pty Ltd.
Acknowledgments
The authors acknowledge the Traditional Owners the Quandamooka People, traditional custodians of the lands, waters and seas where this study was conducted. The authors pay respects to elders, past, present and future.
Conflict of interest
The authors declare that this study received funding from Port of Brisbane Pty Ltd. The funder contributed to late stage editing of the manuscript.
Publisher’s note
All claims expressed in this article are solely those of the authors and do not necessarily represent those of their affiliated organizations, or those of the publisher, the editors and the reviewers. Any product that may be evaluated in this article, or claim that may be made by its manufacturer, is not guaranteed or endorsed by the publisher.
References
Andriolo A., Martins C., Engel M., Pizzorno J., Más-Rosa S., Freitas A., et al. (2006). The first aerial survey to estimate abundance of humpback whales (Megaptera movaeangliae) in the breeding ground off Brazil (Breeding stock a). J. Cetacean Res. Manag. 8, 307. doi: 10.47536/jcrm.v8i3.728
Baker C. S., Herman L. M. (1984). Aggressive behavior between humpback whales (Megaptera novaeangliae) wintering in Hawaiian waters. Can. J. Zool. 62, 1922–1937. doi: 10.1139/z84-282
Baraff L. S., Clapham P. J., Mattila D. K., Bowman R. S. (1991). Feeding behaviour of humpback whales in low-latitude water. Mar. Mammal Sci. 7, 197–202. doi: 10.1111/j.1748-7692.1991.tb00567.x
Bejder L., Videsen S., Hermannsen L., Simon M., Hanf D., Madsen P. (2019). Low energy expenditure and resting behaviour of humpback whale mother-calf pairs highlights conservation importance of sheltered breeding areas. Sci. Rep. 9, 1–11. doi: 10.1038/s41598-018-36870-7
Bengtson Nash S. M. (2018). Toxicological risks and considerations associated with lipophilic contaminant burdens of southern ocean mysticetes. Fossi C, Panti C editors. Marine Mammal Ecotoxicology: impacts of multiple stressors on population health 1st Edition (Elsevier). doi: 10.1016/B978-0-12-812144-3.00014-0
Bengtson Nash S., Castrillon J., Eisenmann P., Fry B., Shuker J., Cropp R., et al. (2018). Signals from the south; humpback whales carry messages of Antarctic Sea-ice ecosystem variability. Global Change Biol. 24, 1500–1510. doi: 10.1111/gcb.14035
Bengtson Nash S. M., Waugh C. A., Schlabach M. (2013). Metabolic concentration of lipid soluble organochlorine burdens in the blubber of southern hemisphere humpback whales through migration and fasting. Environ. Sci. Technol. 47, 9404–9413. doi: 10.1021/es401441n
Braithwaite J. E., Meeuwig J. J., Jenner K. C. S. (2012). Estimating cetacean carrying capacity based on spacing behaviour. PloS One 7, e51347. doi: 10.1371/journal.pone.0051347
Bruce E., Albright L., Sheehan S., Blewitt M. (2014). Distribution patterns of migrating humpback whales (Megaptera novaeangliae) in Jervis bay, Australia: A spatial analysis using geographical citizen science data. Appl. Geogr. 54, 83–95. doi: 10.1016/j.apgeog.2014.06.014
Buckland S. T., Anderson D. R., Burnham K. P., Laake J. L. (1993). Distance sampling: Estimating abundance of biological populations (New York: Chapman & Hall).
Cartwright R. (2005). A comparative study of the behaviour and dynamics of humpback whale (Megaptera novaeangliae) mother and calf pairs during their residence in nursery waters (Manchester Metropolitan University).
Castrillon J., Bengtson Nash S. (2020). Evaluating cetacean body condition; a review of traditional approaches and new developments. Ecol. Evol. 10, 6144–6162. doi: 10.1002/ece3.6301
Chittleborough R. (1953). Aerial observations on the humpback whale, megaptera nodosa (Bonnaterre), with notes on other species. Mar. Freshw. Res. 4, 219–226. doi: 10.1071/MF9530219
Christiansen F., Bejder L., Burnell S., Ward R., Charlton C. (2022). Estimating the cost of growth in southern right whales from drone photogrammetry data and long-term sighting histories. Mar. Ecol. Prog. Ser. 687, 173–194. doi: 10.3354/meps14009
Christiansen F., Dujon A. M., Sprogis K. R., Arnould J. P. Y., Bejder L. (2016). Noninvasive unmanned aerial vehicle provides estimates of the energetic cost of reproduction in humpback whales. Ecosphere 7, e01468–e01n/a. doi: 10.1002/ecs2.1468
Christiansen F., Víkingsson G. A., Rasmussen M. H., Lusseau D. (2014). Female body condition affects foetal growth in a capital breeding mysticete. Funct. Ecol. 28, 579–588. doi: 10.1111/1365-2435.12200
Christiansen F., Vivier F., Charlton C., Ward R., Amerson A., Burnell S., et al. (2018). Maternal body size and condition determine calf growth rates in southern right whales. Mar. Ecol. Prog. Ser. 592, 267–281. doi: 10.3354/meps12522
Clapham P. J. (1992). Age at attainment of sexual maturity in humpback whales, megaptera novaeangliae. Can. J. Zool. 70, 1470–1472. doi: 10.1139/z92-202
Clapham P. J., Young S. B., Brownell R. L. Jr (1999). Baleen whales: conservation issues and the status of the most endangered populations. Mammal Rev. 29, 37–62. doi: 10.1046/j.1365-2907.1999.00035.x
Corkeron P. J. (1995). Humpback whales (Megaptera novaeangliae) in hervey bay, Queensland: behaviour and responses to whale-watching vessels. Can. J. Zool. 73, 1290–1299. doi: 10.1139/z95-153
Corkeron P. J., Brown M., Slade R. W., Bryden M. M. (1994). Humpback whales, megaptera novaeangliae (Cetaceaa: Balaenopteridae), in hervey bay, Queensland. Wildlife Res. 21, 293–205. doi: 10.1071/WR9940293
Corkeron P. J., Connor R. C. (1999). Why do baleen whales migrate? Mar. Mammal Sci. 15, 1228–1245. doi: 10.1111/j.1748-7692.1999.tb00887.x
Craig A. S., Herman L. M., Pack A. A., Waterman J. O. (2014). Habitat segregation by female humpback whales in Hawaiian waters: avoidance of males? Behaviour 151, 613–631. doi: 10.1163/1568539X-00003151
Dawbin W. H. (1966). “The seasonal migratory cycle of humpback whales,” in Whales, dolphins, and porpoises (Berkeley and Los Angeles: University of California Press), 145–170. xv+ 789.
Drent R., Both C., Green M., Madsen J., Piersma T. (2003). Pay-offs and penalties of competing migratory schedules. Oikos 103, 274–292. doi: 10.1034/j.1600-0706.2003.12274.x
Ejrnæs D. D., Sprogis K. R. (2022). Ontogenetic changes in energy expenditure and resting behaviour of humpback whale mother–calf pairs examined using unmanned aerial vehicles. Wildlife Res 49 (1), 34–45. doi: 10.1071/WR20186
Ersts P. J., Rosenbaum H. C. (2003). Habitat preference reflects social organization of humpback whales (Megaptera novaeangliae) on a wintering ground. J. Zool. 260, 337–345. doi: 10.1017/S0952836903003807
Félix F., Guzmán H. M. (2014). Satellite tracking and sighting data analyses of southeast pacific humpback whales (Megaptera novaeangliae): is the migratory route coastal or oceanic? Aquat. Mammals 40 (4), 329–340. doi: 10.1578/AM.40.4.2014.329
Franklin W. (2014). Abundance, population dynamics, reproduction, rates of population increase and migration linkages of eastern Australian humpback whales (Megaptera novaeangliae) utilising hervey bay, Queensland (Doctor of Philosophy, Southern Cross University).
Franklin T., Franklin W., Brooks L., Harrison P., Baverstock P., Clapham P. (2011). Seasonal changes in pod characteristics of eastern Australian humpback whales (Megaptera novaeangliae), hervey bay 1992–2005. Mar. Mammal Sci. 27, E134–E152. doi: 10.1111/j.1748-7692.2010.00430.x
Frisch R. E. (1984). Body fat, puberty and fertility. Biol. Rev. 59, 161–188. doi: 10.1111/j.1469-185X.1984.tb00406.x
Gittleman J. L., Thompson S. D. (1988). Energy allocation in mammalian reproduction. Am. Zoologist 28, 863–875. doi: 10.1093/icb/28.3.863
Glockner D. A., Venus S. C. (1983). “Identification, growth rate, and behavior of humpback whale (Megaptera novaeangliae) cows and calves in the waters off Maui, hawaii 1977–1979,” in Communication and behavior of whales. Ed. Payne R. (Boulder, Colo: Westview Press), 223–258.
Harris G., Thirgood S., Hopcraft J. G. C., Cromsigt J. P., Berger J. (2009). Global decline in aggregated migrations of large terrestrial mammals. Endangered Species Res. 7, 55–76. doi: 10.3354/esr00173
Herman L. M., Tavolga W. N. (1980). The communication systems of cetaceans. In L. M. Herman (Ed.) Cetacean Behavior: Mechanisms and Functions. (NY: Wiley & Sons, Inc.), 149–209.
Herman L. M., Antinoja R. C. (1977). Humpback whales in the Hawaiian breeding waters: population and pod characteristics. Sci. Rep. Whales Res. Institute 29, 59–85.
Jenner K. C. S., Jenner M.-N. M., McCabe K. A. (2001). Geographic and temporal movements of humpback whales in Western Australia waters. APPEA J. 41, 749–765. doi: 10.1071/AJ00044
Jones M. L., Swartz S. L. (1984). Demography and phenology of gray whales and evaluation of whale-watching activities in Laguna San ignacio, Baja California sur, Mexico. IN Jones ML, Swartz SL, Leatherwood S editors. The Gray Whale: Eschrichtius Robustus. (San Diego: Academic Press) 309-374. doi: 10.1016/B978-0-08-092372-7.50020-0
Jönsson K. I. (1997). Capital and income breeding as alternative tactics of resource use in reproduction. Oikos 78, 57–66. doi: 10.2307/3545800
Laist D. W., Knowlton A. R., Mead J. G., Collet A. S., Podesta M. (2001). Collision between ships and whales. Mar. Mammal Sci. 17, 35–75. doi: 10.1111/j.1748-7692.2001.tb00980.x
Lammers M., Pack A., Lyman E., Espiritu L. (2013). Trends in collisions between vessels and north pacific humpback whales (Megaptera novaeangliae) in Hawaiian waters, (1975–2011). J. Cetacean Res. Manage. 13, 73–80. doi: 10.47536/jcrm.v13i1.557
Lockyer C. (1986). Body fat condition in northeast Atlantic fin whales, balaenoptera physalus, and its relationship with reproduction and food resource. Can. J. Fisheries Aquat. Sci. 43, 142–147. doi: 10.1139/f86-015
Lockyer C. (2007). All creatures great and smaller: a study in cetacean life history energetics. J. Mar. Biol. Assoc. United Kingdom 87, 1035–1045. doi: 10.1017/S0025315407054720
Lockyer C., Brown S. (1981). “The migration of whales,” in Animal migration (Cambridge: Cambridge University Press), 105–137.
Maritime Safety Queensland (2005). Marine incidents annual report 2004 (Brisbane: Marketing and Education Unit, Maritime Safety Queensland).
Mattila D. K., Clapham P. J., Katona S. K., Stone G. S. (1989). Population composition of humpback whales, megaptera novaeangliae, on silver bank 1984. Can. J. Zool. 67, 281–285. doi: 10.1139/z89-041
Mayaud R., Castrillon J., Wilson C., Peel D., Smith J. N., Luche G. D., et al. (2022). Traffic in a nursery: Ship strike risk from commercial vessels to migrating humpback whales (Megaptera novaeangliae) in a rapidly developing Australian urban embayment. Mar. Policy 146, 105332. doi: 10.1016/j.marpol.2022.105332
Mehlman D. W., Mabey S. E., Ewert D. N., Duncan C., Abel B., Cimprich D., et al. (2005). Conserving stopover sites for forest-dwelling migratory landbirds. Auk 122, 1281–1290. doi: 10.1093/auk/122.4.1281
Miller R. L., Marsh H., Cottrell A., Hamann M. (2018). Protecting migratory species in the Australian marine environment: A cross-jurisdictional analysis of policy and management plans. Front. Mar. Sci. 5. doi: 10.3389/fmars.2018.00229
Mobley J. R., Herman L. M. (1985). Transience of social affiliations among humpback whales (Megaptera novaeangliae) on the Hawaiian wintering grounds. Can. J. Zool. 63, 762–772. doi: 10.1139/z85-111
Morete M. E., Freitas A., Engel M. H., Pace R. M. III, Clapham P. J. (2003). A novel behavior observed in humpback whales on wintering grounds abrolhos bank (Brazil). Mar. Mammal Sci. 19, 694–707. doi: 10.1111/j.1748-7692.2003.tb01125.x
Müeller M., Boutiere H., Weaver A., Candelon N. (1998). Ethogram of the bottlenose dolphin (Tursiops truncatus), with special reference to solitary and sociable dolphins.
Murray N. J., Clemens R. S., Phinn S. R., Possingham H. P., Fuller R. A. (2014). Tracking the rapid loss of tidal wetlands in the yellow Sea. Front. Ecol. Environ. 12, 267–272. doi: 10.1890/130260
Noad M., Dunlop R., Paton D., Kniest H. (2011). Abundance estimates of the east Australian humpback whale population: 2010 survey and update Vol. 30 (Tromsø, Norway: Paper to the International Whaling Commission Scientific Committee).
Norris K. (1967). Some observations on the migration and orientation of marine mammals. In: Storm RM (ed.) Animal Orientation and Migration. (Corvallis, OR: Oregon State University Press), 101–125.
Pack A. A., Herman L. M., Spitz S. S., Craig A. S., Hakala S., Deakos M. H., et al. (2012). Size-assortative pairing and discrimination of potential mates by humpback whales in the Hawaiian breeding grounds. Anim. Behav. 84, 983–993. doi: 10.1016/j.anbehav.2012.07.024
Pantus F. J., Dennison W. C. (2005). Quantifying and evaluating ecosystem health: A case study from moreton bay, Australia. Environ. Manage. 36, 757–771. doi: 10.1007/s00267-003-0110-6
Paterson R., Paterson P. (1984). A study of the past and present status of humpback whales in east Australian waters. Biol. Conserv. 29, 321–343. doi: 10.1016/0006-3207(84)90003-X
Paterson R., Paterson P., Cato D. (2001). Status of humpback whales, megaptera novaeangliae, in east Australia at the end of the 20th century Vol. 47 (Memoirs-Queensland Museum), 579–586.
Peel D., Smith J. N., Childerhouse S. (2018). Vessel strike of whales in Australia: The challenges of analysis of historical incident data. Front. Mar. Sci. 5, 69. doi: 10.3389/fmars.2018.00069
Pirotta E., Mangel M., Costa D. P., Goldbogen J., Harwood J., Hin V., et al. (2019). Anthropogenic disturbance in a changing environment: modelling lifetime reproductive success to predict the consequences of multiple stressors on a migratory population. Oikos 128, 1340–1357. doi: 10.1111/oik.06146
Pitman R. L., Durban J. W., Joyce T., Fearnbach H., Panigada S., Lauriano G. (2020). Skin in the game: Epidermal molt as a driver of long-distance migration in whales. Mar. Mammal Sci. 36, 565–594. doi: 10.1111/mms.12661
Possingham H. P., Andelman S., Noon B., Trombulak S., Pulliam H. (2001). Making smart conservation decisions. Conserv. Biol.: Res. Priorities Next Decade. Edited by M.E. Soule and G.H. Orians. (Washington: Island Press). 23, 225–244.
Ransome N., Kew A., Duque E., Morais M., Wright W., Smith J. N. (2022). Escorting of a mother humpback whale (Megaptera novaeangliae) and the death of her calf during aggressive mating behavior. Mar. Mammal Sci 38 (4), 1643–1653. doi: 10.1111/mms.12922
Robinson R. A., Learmonth J. A., Hutson A. M., Macleod C. D., Sparks T. H., Leech D. I., et al. (2005). Climate change and migratory species (Thetford, Norfolk: British Trust for Ornithology The Nunnery).
Ruckelshaus M., Klinger T., Knowlton N., Demaster D. P. (2008). Marine ecosystem-based management in practice: Scientific and governance challenges. BioScience 58, 53–63. doi: 10.1641/B580110
Runge C. A., Martin T. G., Possingham H. P., Willis S. G., Fuller R. A. (2014). Conserving mobile species. Front. Ecol. Environ. 12, 395–402. doi: 10.1890/130237
Runge C. A., Watson J. E. M., Butchart S. H. M., Hanson J. O., Possingham H. P., Fuller R. A. (2015). Protected areas and global conservation of migratory birds. Science 350, 1255–1258. doi: 10.1126/science.aac9180
Schaub M., Jenni L., Bairlein F. (2008). Fuel stores, fuel accumulation, and the decision to depart from a migration stopover site. Behav. Ecol. 19, 657–666. doi: 10.1093/beheco/arn023
Schuster R., Wilson S., Rodewald A. D., Arcese P., Fink D., Auer T., et al. (2019). Optimizing the conservation of migratory species over their full annual cycle. Nat. Commun. 10, 1754. doi: 10.1038/s41467-019-09723-8
Sheehy J., Taylor C. M., Norris D. R. (2011). The importance of stopover habitat for developing effective conservation strategies for migratory animals. J. Ornithol. 152, 161–168. doi: 10.1007/s10336-011-0682-5
Shillinger G. L., Palacios D. M., Bailey H., Bograd S. J., Swithenbank A. M., Gaspar P., et al. (2008). Persistent leatherback turtle migrations present opportunities for conservation. PloS Biol. 6, e171. doi: 10.1371/journal.pbio.0060171
Smith R. J., Moore F. R. (2003). Arrival fat and reproductive performance in a long-distance passerine migrant. Oecologia 134, 325–331. doi: 10.1007/s00442-002-1152-9
Smultea M. A. (1994). Segregation by humpback whale (Megaptera novaeangliae) cows with a calf in coastal habitat near the island of Hawaii. Can. J. Zool. 72, 805–811. doi: 10.1139/z94-109
Stephens P. A., Boyd I. L., McNamara J. M., Houston A. I. (2009). Capital breeding and income breeding: their meaning, measurement, and worth. Ecology 90, 2057–2067. doi: 10.1890/08-1369.1
Thomas P. O., Taber S. M. (1984). Mother-infant interaction and behavioral development in southern right whales, eubalaena australis. Behaviour 88, 42–60. doi: 10.1163/156853984X00470
Tyack P., Whitehead H. (1983). Male Competition in Large groups of wintering humpback whales. Behaviour 83, 132–154. doi: 10.1163/156853982X00067
Videsen S. K. A., Bejder L., Johnson M., Madsen P. T. (2017). High suckling rates and acoustic crypsis of humpback whale neonates maximise potential for mother–calf energy transfer. Funct. Ecol. 31, 1561–1573. doi: 10.1111/1365-2435.12871
Whitehead H., Moore M. J. (1982). Distribution and movements of West Indian humpback whales in winter. Can. J. Zool. 60, 2203–2211. doi: 10.1139/z82-282
Williams R., Vikingsson G. A., Gislason A., Lockyer C., New L., Thomas L., et al. (2013). Evidence for density-dependent changes in body condition and pregnancy rate of north Atlantic fin whales over four decades of varying environmental conditions. ICES J. Mar. Sci. 70 (6), 1273–1280. doi: 10.1093/icesjms/fst059
Wilson K. A., McBride M. F., Bode M., Possingham H. P. (2006). Prioritizing global conservation efforts. Nature 440, 337–340. doi: 10.1038/nature04366
Würsig B., Whitehead H. (2009). “Aerial behavior,” in Encyclopedia of marine mammals (Second edition). Eds. Perrin W. F., Würsig B., Thewissen J. G. M. (London: Academic Press).
Keywords: migratory species, resting stopovers, Southern hemisphere humpback whales, habitat use, energy balance, Moreton Bay, Antarctic climate change, sustainable development
Citation: Castrillon J, Mayaud R, Wilson C, Dalle Luche G, Allen J and Bengtson Nash S (2023) Moreton Bay; A previously unrecognized resting stopover for east-coast of Australia migrating humpback whales. Front. Mar. Sci. 10:1063197. doi: 10.3389/fmars.2023.1063197
Received: 06 October 2022; Accepted: 23 March 2023;
Published: 19 April 2023.
Edited by:
Lars Bejder, University of Hawaii at Manoa, United StatesReviewed by:
Adam Ari Pack, University of Hawaii at Hilo, United StatesJorge Acevedo, Fuego-Patagonia y Antártica, Chile
Copyright © 2023 Castrillon, Mayaud, Wilson, Dalle Luche, Allen and Bengtson Nash. This is an open-access article distributed under the terms of the Creative Commons Attribution License (CC BY). The use, distribution or reproduction in other forums is permitted, provided the original author(s) and the copyright owner(s) are credited and that the original publication in this journal is cited, in accordance with accepted academic practice. No use, distribution or reproduction is permitted which does not comply with these terms.
*Correspondence: Susan Bengtson Nash, cy5iZW5ndHNvbm5hc2hAZ3JpZmZpdGguZWR1LmF1