- 1Instituto de Investigaciones Marinas y Costeras (IIMyC), Facultad Ciencias Exactas y Naturales, Universidad Nacional de Mar del Plata-Consejo Nacional de Investigaciones Científicas y Técnicas, Mar del Plata, Provincia de Buenos Aires, Argentina
- 2Institute of Anthropic Impact and Sustainability in Marine Environment-National Research Council (IAS-CNR), Mazara del Vallo, Sicily, Italy
Introduction: Maternal care in marine decapods involves eggs caring in the brood compartment until the larvae hatch. This behavior mainly allows embryo mass oxygen supply, ensuring healthy embryonic development. The present study aimed to analyze the effect of different sound sources (anthropogenic and biologic) and their temporal patterns (low and high rate: 1 min of the sound stimulus + 5 min of silence and 1 min of the sound stimulus + 1 min of silence, respectively) on the maternal care of the key crab species, Neohelice granulata.
Methods: In the laboratory, three acoustic stimuli were played back: an artificial white noise (10 Hz – 20 kHz), and two sounds obtained from the crabs´ natural habitat, motorboat passages and biological signals from a crabs’ predator fish. Three behavioral variables were quantified: still position, and two maternal care behaviors: abdominal flapping and chelae probing.
Results: Results demonstrated that the high rate anthropogenic stimuli, white noise and motorboat, affected all behavioral variables, increasing the still position and diminishing the maternal care behaviors. Otherwise, the predatory stimulus did not affect the still position although diminished the maternal care behaviors (high rate).
Discussion: The different behavioral response depending on the sound stimuli may indicate that crabs distinguish sound sources. The anthropogenic noise is suggested to cause distraction that is linked to the increased still position, while the predator stimulus would be associated with an alert behavior not affecting the locomotion behavior. The sound stimuli effect on the maternal care behavior revealed a negative effect that potentially could affect offspring survival. This is important considering the ecosystem engineering function of the studied key crab species. The reduction of the noise emission pattern rate is suggested as a mitigation action to diminish sound impact effects in the crab’s natural habitat. The study contributes the first to assessing the effect of different sound sources on the maternal care behavior of a crustacean species.
Introduction
Aquatic ecosystems are composed of a combination of sounds produced by animals (biophonies), physical agents (geophonies) and human activities (anthropophonies) which defines the soundscape (Pijanowski et al., 2011). These particular mixtures of sounds reflect the ecological pattern and processes of specific aquatic environments (Matsinos et al., 2008; Ceraulo et al., 2018). The complexity of the biophony component is directly related to the structure of the animal community (Kennedy et al., 2010).
Among biophonies, the sound production in crustaceans is well known. Species of more than 20 families of aquatic, semiterrestrial and terrestrial crustaceans are described to produce sound through substrate-borne vibrations and air/water-borne sound (Nakamachi et al., 2021). Specifically, in marine decapods, sound production is related to different communicative behaviors such as defensive (Clayton, 2005; Patek et al., 2009; Buscaino et al., 2011; Goh et al., 2019; Roberts, 2021), mating (Buscaino et al., 2015; Filiciotto et al., 2019; Flood et al., 2019), orientation (Stanley et al., 2012; Sal Moyano et al., 2021) and agonistic interactions (Boon et al., 2009; Goh et al., 2019; Taylor et al., 2019; Ceraulo et al., 2022). Despite the diverse decapod sound signals described and their associated behaviors, much less known is the effect of biologic sound signals (e.g. from predators) on the behavior. Very few studies were conducted on this topic, in fact, three studies in vertebrates (fishes: Luczkovich et al., 2000; Remage-Healey et al., 2006; whales: Miller et al., 2022) and only two in invertebrates that tested the effect of predatory sounds on the feeding and locomotion behavior of crabs (Hughes et al., 2014; Snitman et al., 2022). Sound is characterized by the pressure variation and the displacement of the particles of the medium in which the same sound is propagating (i.e. particle motion). Regarding the sensitivity of decapod crustaceans, diverse sensory mechanoreceptors such as statocysts, chordotonal organs and setae were described to be involved in the detection of substrate vibrations and sound particle motion (Popper et al., 2001). A variety of studies demonstrated that decapods appear more sensitive to low frequency acoustic stimuli resulting from the particle motion (see Roberts and Elliott, 2017, and references therein).
Among anthropophonies, the sound caused by different human sources (e.g. shipping, pile driving, seismic surveys) is considered a global pollutant adding noise to ecosystems and masking natural sounds (Clark et al., 2009; Ceraulo et al., 2018). Impacts on marine animals are known to depend on noise intensity and temporal patterns of exposure (Popper et al., 2014; Blom et al., 2019). The effect of anthropogenic sound on marine mammals and fishes is well studied (e.g. see reviews: Slabbekoorn et al., 2010; Erbe et al., 2016), while much fewer studies were conducted in invertebrates and, especially, in crustaceans. Those studies focused on crustaceans include anthropogenic noise effects on behavioral (predatory: Chan et al., 2010; Nousek-McGregor and Mei, 2016; predatory and foraging: Wale et al., 2013; locomotion and activity: Solan et al., 2016; Snitman et al., 2022) and physiological (Celi et al., 2015; Filiciotto et al., 2016) traits. However, no previous studies were conducted on crustaceans to test the effect of anthropogenic sound on behaviors that involve a direct impact on fitness, such as maternal care with important consequences in the offspring survival. In contrast, the effect of noise on parental care behavior was demonstrated in fishes (Picciulin et al., 2010; Nedelec et al., 2016; Nedelec et al., 2017; McCloskey et al., 2020; Nedelec et al., 2022).
Parental or maternal care includes all parental traits that enhance offspring fitness (Trumbo, 2012). Maternal care is widespread among many animal taxa. These care traits are associated with an evolutionary response to physically harsh environments, involving a selective advantage (Clutton-Brock, 1991). Parental care controls for physicochemical stress produced by, for example, abiotic factors such as temperature, anoxia and salinity (Clutton-Brock, 1991). In crustacean marine decapods, maternal behaviors involve the care of the eggs that females carry in their brood compartment until the hatching of eggs and larvae is released (Diesel, 1992). This behavior is related to the supply of oxygen to the embryo mass, exhibiting active brooding comportment directed towards their ventilation (Fernández and Brante, 2003). Oxygen limitation in the center of the embryo mass usually occurs given the large number of eggs (Strathmann and Strathmann, 1995). Moreover, oxygen availability varies throughout embryonic development in response to embryo oxygen demands: low oxygen consumption in early stages while high in late stages (Naylor and Taylor, 1999; Fernández et al., 2000). Decapod females can assess the oxygen consumption of the embryos and modify the brooding behavior according to the embryos’ oxygen demands (Baeza & Fernández, 2002; Fernández and Brante, 2003). Since females provide the oxygen to the egg mass, their behavior is a critical factor during embryonic development, given that oxygen limitation was demonstrated to influence survival, growth rate and size of eggs and hatching larvae (Palumbi and Johnson, 1982; Strathmann and Strathmann, 1995; Baeza and Fernández, 2002).
In addition to ventilating and providing oxygen to the embryo mass, maternal behaviors allow cleaning and elimination of metabolites, avoiding microbial infections during egg development (Clutton-Brock, 1991). Besides, maternal care allows protection of eggs from predators or adverse abiotic conditions (i.e. temperature, salinity) (Strathmann, 1985; Thiel, 1999).
Decapod females show active maternal behaviors, being the abdominal flapping the most recognized behavior (Fernández and Brante, 2003; Silva et al., 2007). Other less frequent maternal care behaviors involve standing (raised body), chela and pereiopods probing (females introduced the chela/dactyls of the pereiopods into the embryo mass) (Baeza and Fernández, 2002). Abdominal flapping is currently related to increase the oxygen availability while chelae/pereiopod probing is associated with the assessment of oxygen conditions in the embryo mass (Baeza and Fernández, 2002; Fernández and Brante, 2003).
Neohelice granulata is a varunid semiterrestrial crab considered a key crab species in the intertidal zone of estuaries, salt marshes and mangroves of the South-western Atlantic Ocean, being distributed from San Jose Gulf, northern Patagonia, Argentina (42°82´S; 64°83´W), to Lagoa Araruama, Rio de Janeiro, Brazil (22°85´S; 42°85´W) (Spivak et al., 2019). The Mar Chiquita coastal lagoon is a wetland located in the Buenos Aires Province, Argentina (37°40′ S, 57°23′ W) declared as a Man and the Biosphere Reserve (MAB) by UNESCO, conforming to the UNESCO World Network of Biosphere Reserves. In this lagoon, N. granulata is a dominant species used as a model study given the great diversity of publications conducted on several topics of its physiology, ecology and behavior (see Luppi and Rodriguez, 2020; Rodriguez and Luppi, 2020). Moreover, this crab is considered a key species and an ecosystem engineer because of its burrowing activity that regulates the estuarine ecosystem functioning (Gutiérrez et al., 2006).
The Mar Chiquita coastal lagoon soundscape was previously characterized, describing particular temporal and spatial patterns of anthropogenic (motorboat passages) and biologic (fish and crustaceans) sounds (Ceraulo et al., 2020). Particularly, a study revealed that motorboat passages affected the reproductive call rate of a fish species (Ceraulo et al., 2021). The sound production in N. granulata was recently reported, characterizing the specific signals and the associated reproductive behavior (Filiciotto et al., 2019; Sal Moyano et al., 2019). In addition, current studies demonstrated the effect of artificial and habitat anthropogenic and biological sounds on the physiological stress and locomotion behavior of this species (Filiciotto et al., 2018; Snitman et al., 2022). The maternal care behaviors in N. granulata were previously characterized (Silva et al., 2007). However, no earlier studies evaluated the effect of sound signals on the maternal care traits of this species.
In this context, the present study aimed to analyze the effect of different sound sources: anthropogenic (motorboat passages obtained from the crab habitat and an artificial white noise) and biologic from a crabs’ predator (fish), considering two temporal patterns of emission (low and high rate), on the maternal care behaviors of N. granulata.
Material and methods
Origin and collection of experimental subjects
Ovigerous female crabs were collected manually from the field, the Mar Chiquita Coastal Lagoon. Following Silva et al. (2007), ovigerous females in a late stage of embryonic development were selected given that the frequency of maternal care is increased as egg hatching is closer. Similar-sized females ranging from 22.5 to 26.5 mm of carapace width were used due to the brooding behavior is associated with body size (Fernández et al., 2006). Females were transported to the laboratory and acclimated in natural seawater aquaria (30 × 35 × 25 cm, 26 L capacity, filled with 3 L), at a density of four crabs/aquarium, under a controlled photoperiod of 14:10 h, and continuous aeration. The ambient room temperature was 23.5 ± 2°C. Individuals were fed daily with rabbit pellet food and water was changed after feeding. Crabs were maintained for a maximum of one week in the laboratory and then replaced by fresh animals.
Experimental system
A circular experimental PVC tank (1.2 m diameter and 1.5 m depth) filled with seawater at a depth of 1.2 m was used. A subaquatic video camera (Barlus, UW-S2Z-CX10 model, connected to an NVR IP 16 channels, Hikvision, DS-7616NI-Q1 model) was placed on the top and center of the tank to allow visualization of the entire tank’s bottom surface. An underwater loudspeaker (Model UW30, Lubell Labs Inc., USA, Rated Frequency Response between 100 Hz - 10 kHz) connected to a Power Amplifier (Model APXII-300, American Pro, 230V, 50 Hz, China) plugged into the stereo output of a laptop was located suspended 40 cm from the bottom and 10 cm from the tank lateral wall.
Acoustic stimuli selection
Three acoustic stimuli were used: white noise (bandwidth range of 10 Hz – 20 kHz), and two sound stimuli acquired from the natural habitat of the crab, Mar Chiquita Coastal Lagoon, obtained from a previous soundscape study of the lagoon (Ceraulo et al., 2020). The white noise stimulus was digitally created using the wgn Matlab-function “wgn”. The natural habitat acoustic stimuli belonged to biologic sounds produced by the black drum fish (Pogonias courbina) and anthropogenic sounds emitted by motorboat passages. The fish P. courbina is a predatory species of N. granulata (Blasina et al., 2010) and emits choruses during the reproductive period (Ceraulo et al., 2020). To isolate the fish signals and avoid the co-presence of diverse sources of soundscape components, a specific 1000 Hz low-pass filter was applied to the fish selected files, while no filters were applied to motorboat passage signals.
For the selection of motorboat passages files, only passes with burst broadband noise (frequency below and above 700 Hz, C type-class, see Ceraulo et al., 2021) were used given that this type of noise was demonstrated to be the most intense and frequent in the lagoon (Ceraulo et al., 2021). From the dataset, 30-100 sec duration files were selected from different days and hours (N total = 45). Playlists were constructed by randomly choosing four different files. All playlists had a similar total duration. For fish stimulus, black drum choruses containing a high number of signals (more than 200 in the original dataset, Ceraulo et al., 2020) were selected from the dataset and one-min duration files from different days and hours were chosen (N total = 10). Each playlist consisted of only one file.
For each stimulus (motorboat, white noise and fish), two patterns of emission were considered: low and high rates. In the high rate pattern, playlists contained 1 min of the sound stimulus + 1 min of silence; while in the low rate one, playlists contained 1 min of the sound stimulus + 5 min of silence. For the stimuli obtained from the habitat (motorboat and fish), both conditions of temporal patterns were selected as proxies of the lagoon mouth soundscape during the warm season: high rate motorboat passes on weekend days while low rate during weekdays; and high rate fish choruses during peak hours (sunset: 19:00 to 21:00 h) while low rate fish choruses in the rest of the day given that these signals showed a strong daily circadian pattern.
Ten different playlists for each stimulus (motorboat and fish) and pattern of emission (low and high rate) were constructed. A control without sound was used. Thus, six treatments were conducted: (1) low rate motorboat, (2) high rate motorboat, (3) low rate white noise, (4) high rate white noise, (5) low rate fish, (6) high rate fish, and a control without sound.
Acoustic analysis
To test the experimental system, a calibrated hydrophone (model Reson TC4013, with a sensitivity response of -211 ± 3 dB re 1V/μPa between a wide frequency range of 1 Hz and 150 kHz) coupled with a preamplifier (1-MHz bandwidth single-ended voltage and a high-pass filter set at 10 Hz, 20 dB gain, Avisoft Bioacoustics), connected to a digital acquisition card (Avisoft UltraSoundGate 116h) managed by the Avisoft Recorder USGH software (Avisoft Bioacoustics) was located in the center of the PVC tank at a depth of 20 cm from the bottom. The acoustic stimuli and tank background noise were acquired at the sampling frequency of 100 kHz with 16-bit resolution and analyzed by the Avisoft-SASLab Pro software (Avisoft Bioacoustics). Figure 1 shows the spectrogram and the sound pressure level (Lp,rms dB re 1µPa) of an example of a motorboat, white noise and fish playlist. The power spectrum of the playlists with a high rate emission temporal pattern for the three stimuli is shown in Figure 2. The peak frequency for motorboat, white noise and fish stimuli were 3442 Hz (amplitude 130 dB re 1µPa), 6177 Hz (126 dB re 1µPa) and 195 Hz (121 dB re 1µPa), respectively.
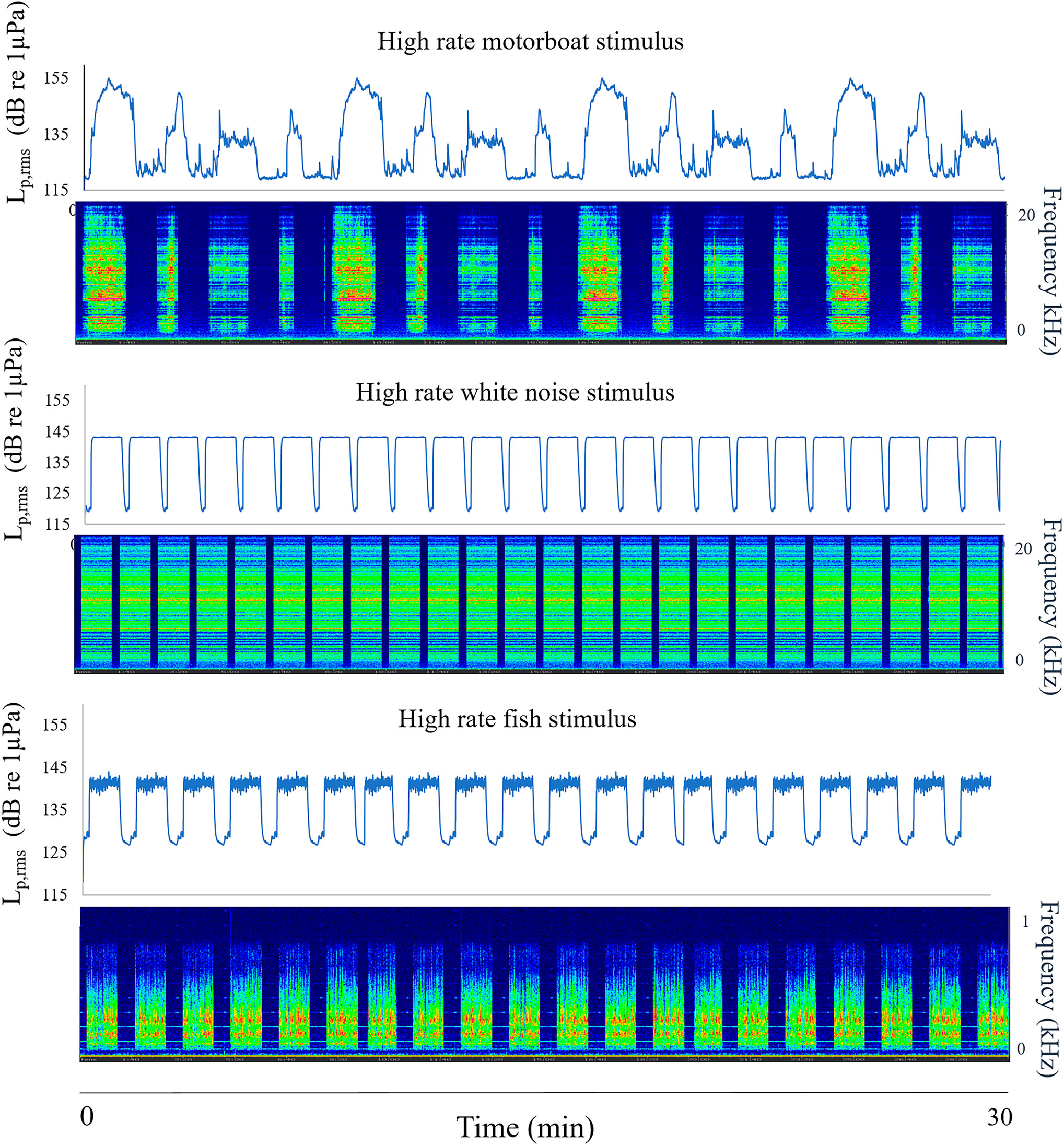
Figure 1 Spectrograms and sound pressure level (Lp,rms dB re 1µPa) of 30 min duration of a playlist example of the different stimuli: motorboat, white noise and fish (8192 samples of FFT size, Hann window and signal superposition of 50%, Linear frequency scale).
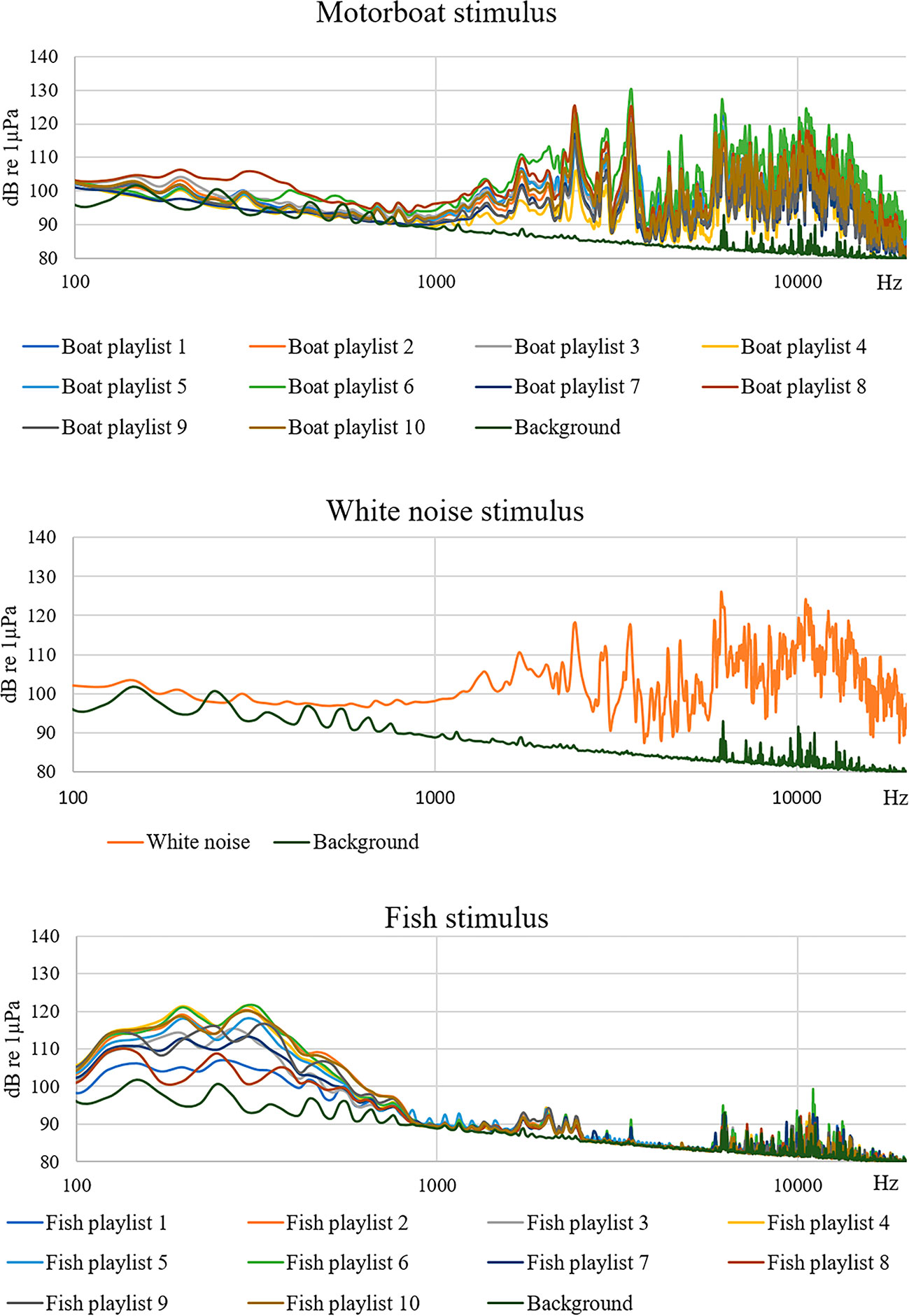
Figure 2 Power spectrum of all playlists with a high rate emission pattern of the motorboat, white noise and fish stimuli (FFT size 4096, hamming window, resolution 24.4 Hz).
Experimental protocol
A female was randomly taken from the maintenance aquaria and located in the center of the PVC tank using a net. After a 10 min habituation period in the experimental tank, the video recording started and the experiment began. The total experiment duration was 60 min, divided into two phases of 30 min each: the “before phase” without sound exposure, and the “test phase” with sound exposure when the different stimuli were played back. At the end of the experiment, both the transducer and video recording were turned off and the crab returned to different maintenance aquaria. Ten replicates for the control (no sound stimulus was emitted in any of the two phases) and per treatment (N = 6: low rate motorboat, high rate motorboat, low rate white noise, high rate white noise, low rate fish, high rate fish; without sound exposure in the before phase and under stimuli exposure in the test phase), were performed (N total = 70). For each replicate of the different treatments, a distinct playlist was randomly assigned and played back. Each female was used in only one trial to meet the assumption of experimental independence.
Behavioral observations
Following Silva et al. (2007), two maternal care behaviors were recognized: “abdominal flapping” (females moved the abdomen forwards and backwards beating rhythmically the egg mass) and “chela probing” (females used one or both chela to pierce the egg mass, sometimes taking and carrying particles to the mouth). The time duration (sec) of the two different maternal behaviors displayed by ovigerous females, flapping and probing, was considered. Both maternal behaviors were observed to occur while the female was walking or when she stopped locomotion. For the statistical analysis, the flapping was considered individually and, jointly with the probing and named generally “maternal care”. The time in which females were observed still (without walking or moving the pereiopods nor the chelae) for 5 sec or longer was also quantified and considered as a “still” position. The duration in which the three behavioral variables were displayed (flapping, flapping + probing = maternal care, still position) was quantified in each phase (without sound exposure and with sound exposure) for each of the six treatments and the control.
Statistical analysis
Models with Poisson error distribution were fitted given that the nature of the data were counts (in seconds) of different behavioral variables. The residuals patterns and overdispersion were examined using the function testUniformity() and testDispersion() from the DHARMa package (Hartig, 2018). In all the cases, model validation of the residuals was applied to verify that underlying statistical assumptions were not violated. When overdispersion was detected, it was corrected incorporating an extra overdispersion parameter using a quasi-Poisson distribution (Ver Hoef and Boveng, 2007). Thus, to each behavioral variable quantified (still position, maternal care and flapping) in both before and test phases, a quasi-Poisson generalized linear model (GLM) with log link (See Zuur et al., 2009) was used to test the effects of the diverse stimuli (levels: low and high rate motorboat, low and high rate white noise, low and high rate fish). Finally, in the test phase, posthoc mean comparisons between the control and the low and high rate levels of each behavioral variable were conducted using an interaction means test in the “emmeans” package (Lenth et al., 2018). All statistical analyses were performed in R 3.3.1 (R Core Team, 2016). A diagram of the experimental design used is shown in Figure 3.
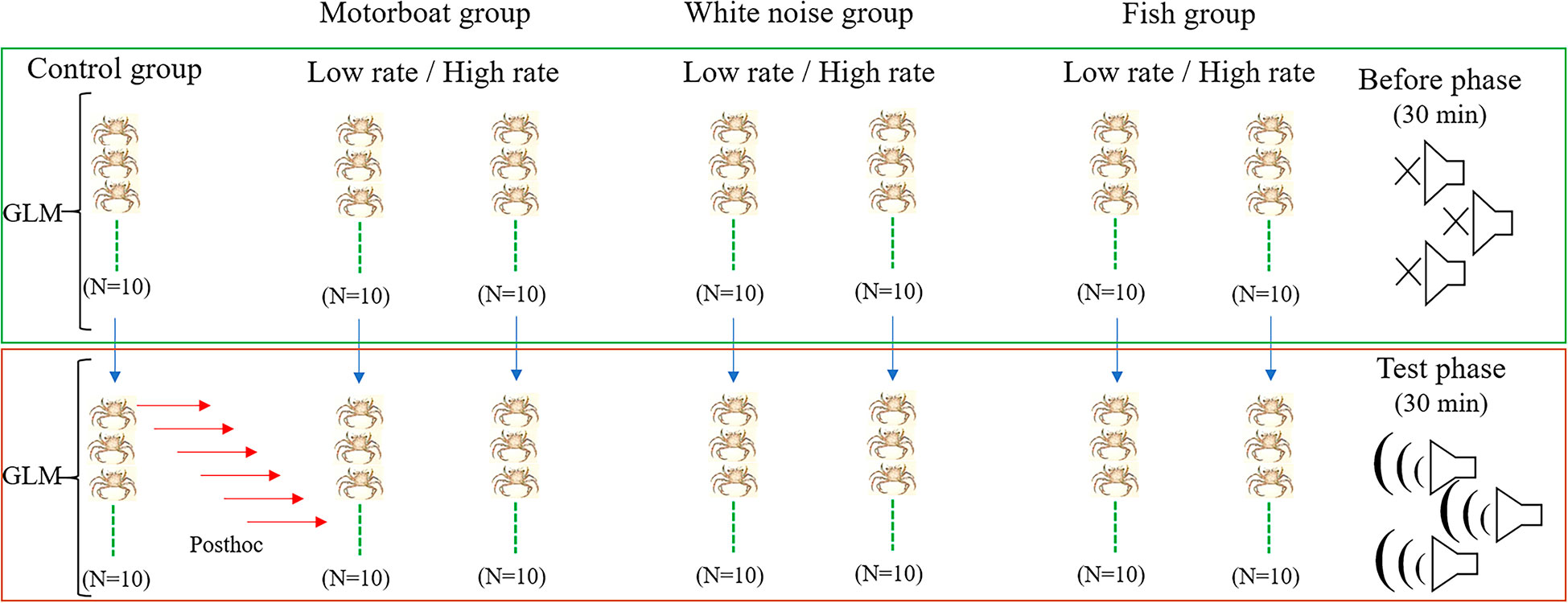
Figure 3 Diagram of the experimental design used showing both phases, before and test, with the control and six levels (low and high rate motorboat, low and high rate white noise, low and high rate fish) in each phase. For each phase, a GLM was performed. In the test phase, the six red arrows represent the posthoc comparisons between the control and levels. The same design was applied for the three behavioral variables (still position, maternal care (flapping + probing) and flapping).
Results
In the “before phase”, no differences were found between the control and the different treatments (group of animals intended to test the diverse stimuli in the test phase) for any of the three behavioral variables quantified (still position: χ2 = 8.52, df = 6, P = 0.2024; maternal care: χ2 = 11.397, df = 6, P = 0.07686; flapping: χ2 = 8.687, df = 6, P = 0.192).
When analyzing the “test phase”, differences were encountered in the crab’s response when exposed to the anthropogenic stimuli for all variables: the sound stimuli increased (both low and high rate motorboat, and high rate white noise) the still position while diminished the maternal care and flapping (high rate motorboat and white noise) compared to the control without sound stimuli (Table 1, Figure 4). In the case of the motorboat treatment, the effect on the still position was greater given that both patterns of emission boosted this behavioral variable. In the fish treatment, no differences were found in the still position (low and high rate), while the high rate pattern reduced the maternal care and flapping behaviors compared to the control (Table 1, Figure 4).
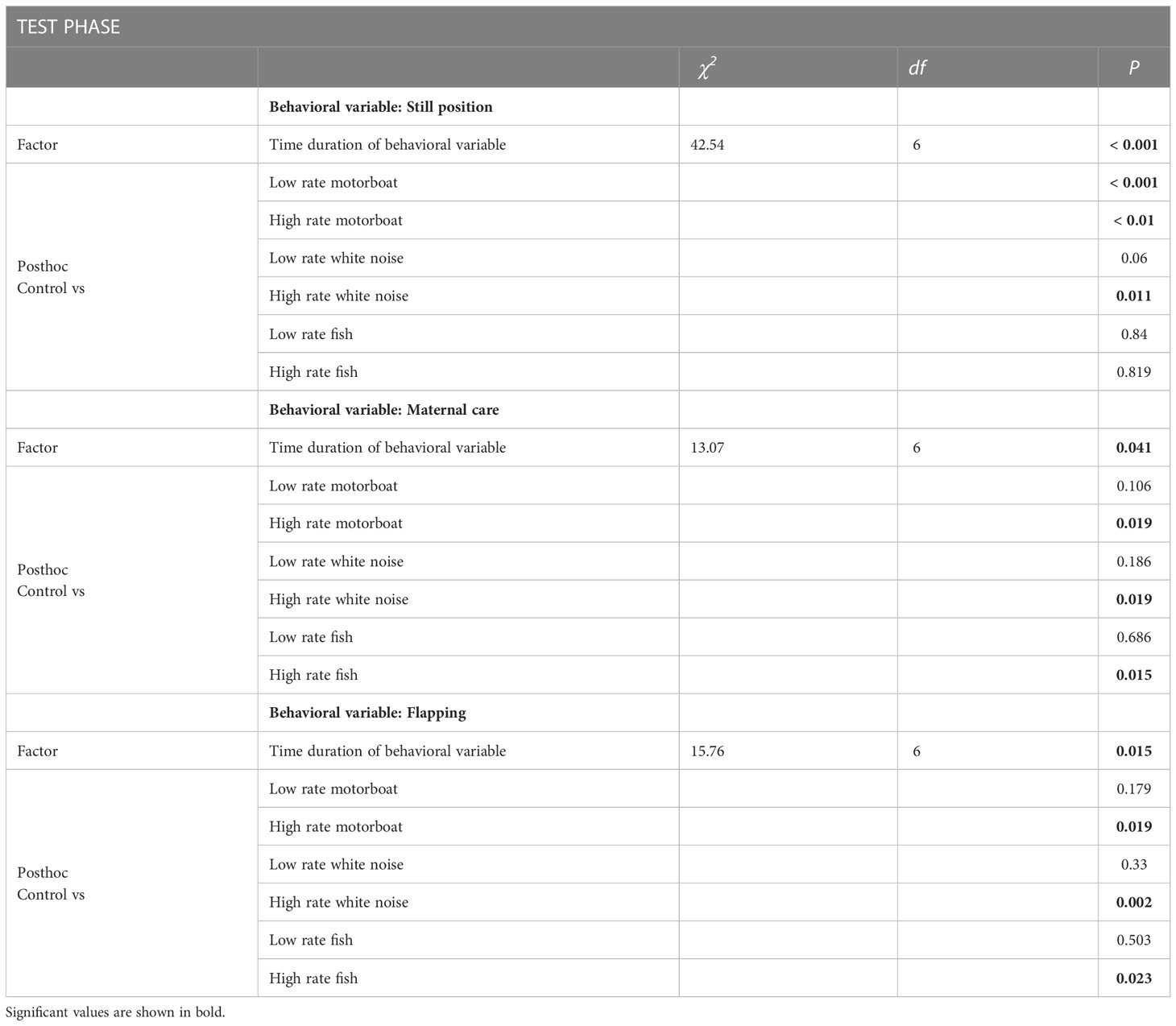
Table 1 Results of the GLM of the “Test phase”, showing the effects of the sound stimuli (motorboat, white noise, and fish) and both temporal emission patterns of sound (low and high rate) on the time duration (sec) of the different behavioral variables quantified (still position, maternal care (flapping + probing), and flapping).
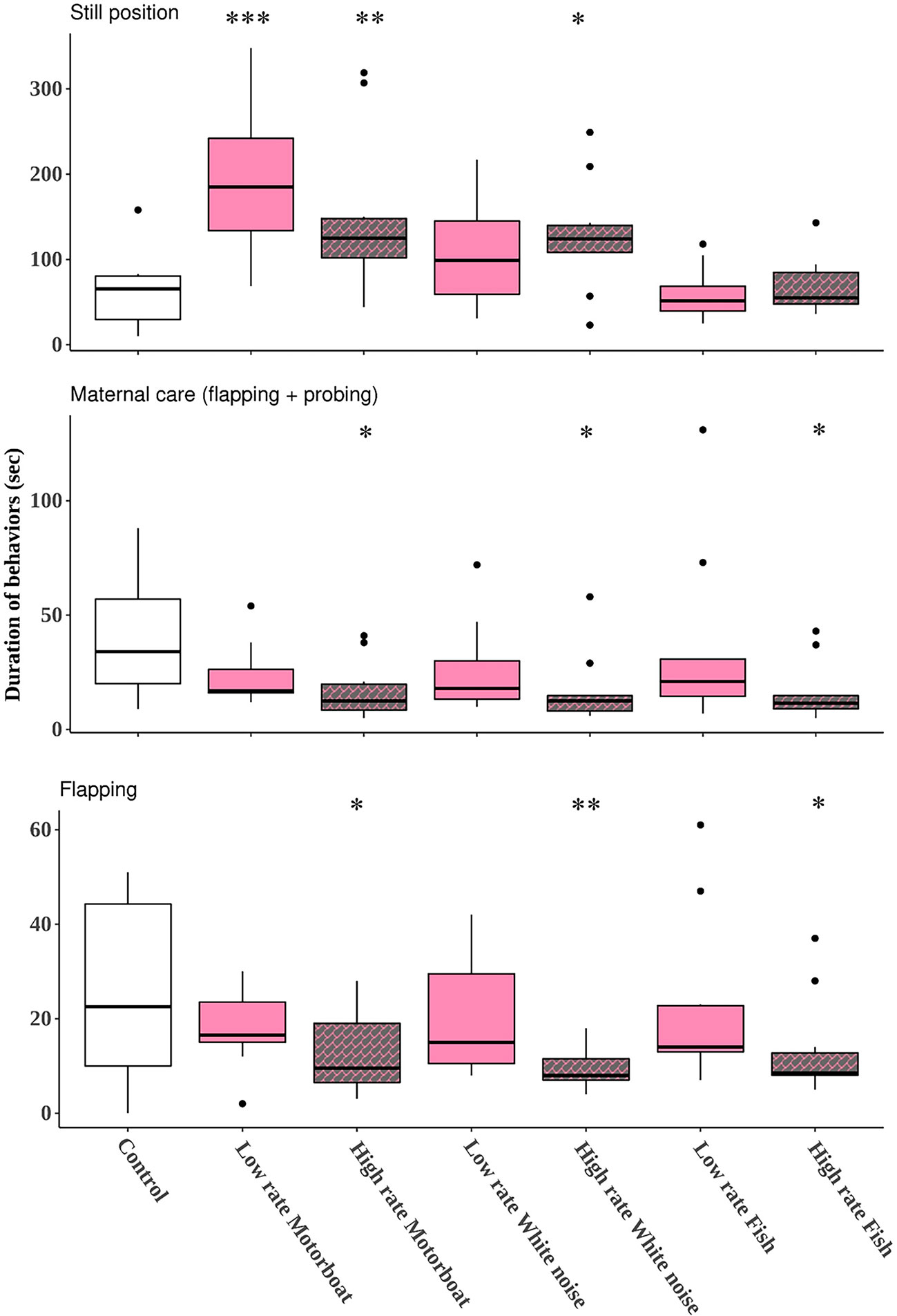
Figure 4 Results of the “test phase” showing the duration (sec) of the behavioral variables quantified: still position, maternal care (flapping + probing), and flapping, for each stimuli and patterns of emission (low rate motorboat, high rate motorboat, low rate white noise, high rate white noise, low rate fish, high rate fish) and the control without sound. High rate temporal emission patterns of the stimuli are represented with grey color, low rate temporal emission patterns of the stimuli with pink color and the control with white color. GLM, significant results: *** P < 0.001, ** P < 0.01, * P < 0.05. The asterisks represent the posthoc comparisons between the control and each treatment for the three behavioral variables.
Consequently, differences among the behavioral response depending on the sound stimuli source were found: both anthropogenic stimuli increased the still position and diminished the frequency of the maternal behaviors; while fish predatory stimulus only reduced the maternal care behaviors in the high rate emission pattern but not the still position. Behavioral observations demonstrated that when the predatory stimulus started, the crab locomotion was interrupted for a few seconds (2-3) and immediately restored (75% of the cases considering the total number of replicates, low and high rate, N = 20); while when anthropogenic stimuli began, the crabs stopped walking and stayed for several seconds (>5) in still position (motorboat: 85%, white noise: 90%).
Discussion
Maternal care in decapods is directly related to the egg mass oxygen provision and healthy embryonic development having vital ecological consequences on the offspring’s fitness. This study is the first to assess the effect of different artificial and habitat sound sources (anthropogenic and biologic) on the maternal care behavior of a crustacean species, the key crab Neohelice granulata. Results revealed a negative effect of sounds on maternal care traits. The high rate pattern of sound emission showed negative effects on the studied behaviors compared to the low rate one. Besides, the study demonstrates that this crab species is behaviorally responding differently according to the diverse sound stimuli, thus, it may be distinguishing sound sources. In this sense, only anthropogenic stimuli boosted the still position, suggesting a distraction effect, while the predatory stimulus may elicit an alert behavior without affecting the locomotion pattern. In addition, results are discussed in the context of the ecological importance of the crab species and the habitat.
The diverse sound stimuli emitted in the present study demonstrated that distinct sound sources elicited different behavioral responses: all stimuli, anthropogenic (motorboat passages, both high and low rate temporal patterns, and high rate white noise) and biological from a crab´s predator (high rate) reduced the maternal care behaviors, but only the first ones (white noise and motorboat passages) increased the still position of crabs. The similar response of crabs to white noise and motorboat stimuli (although the motorboat stimulus had a greater effect given that the low rate pattern also reduced the still position) could be due to the non-discrimination between them given that their peak frequency is higher than the known crustacean’s sensitivity (see Roberts and Elliott, 2017). The reduced locomotion in N. granulata, when exposed to different anthropogenic sound sources, was previously described (Filiciotto et al., 2018; Snitman et al., 2022). Similarly, several studies conducted on decapods found reduced locomotion, a resting time increased or a response behavior (antipredator) diminished, in the presence of motorboat noise (Chan et al., 2010; Wale et al., 2013; Filiciotto et al., 2016; Nousek-McGregor and Mei, 2016; Solan et al., 2016). Considering the effect of biological sounds from predators, a study conducted in crabs demonstrated that reduced the feeding behavior (Hughes et al., 2014), in cetaceans diminished the foraging behavior (Miller et al., 2022), and in fishes affected the mating choruses and calling rates (Luczkovich et al., 2000; Remage-Healey et al., 2006). A study performed in N. granulata, showed reduced locomotion in the presence of predatory sounds from a fish and a crab (Snitman et al., 2022). In contrast, in the present study, the still position was not affected by predatory fish stimulus, although diminished the frequency of the maternal care behaviors displayed. The fact that distinct sound sources, anthropogenic and biologic, affected differently the still position (increased when exposed to anthropogenic stimuli and with no effect under fish stimulus exposure) may indicate that each stimulus promote a diverse behavioral response.
On one hand, anthropogenic stimuli may be associated with a distraction or confusion effect that would be linked with the increased still position (and reduced maternal care): immediately after hearing these stimuli, crabs were observed to stop locomotion for several seconds, retarding their return to the previous locomotion pattern. This retarded response or distracted behavior might imply an ecological disadvantage given crabs may be exposed to risks such as a predator attack. The distraction effect of ship noise disrupting the information gathering ability of animals was previously proposed to occur in hermit crabs (Yim-Hol Chan et al., 2010; Tidau and Briffa, 2019). On the other hand, predatory fish sounds might be related to elicit an alert behavior that could be explained by the observed behavioral response of stop walking for few seconds (2-3) when hearing the stimulus started and, immediately after, restoring the locomotion behavior. In its natural habitat, this species walks around the burrows showing a fast-running behavior to them in the presence of risk (del Valle Fathala and Maldonado, 2011). Thus, the potential alert behavior elicited immediately after hearing the predator sound would favor the fast response to return and hide in the burrow allowing survival against risk rather than causing a distraction (Snitman et al., 2022). Likewise, an alert behavior was previously described in the lobster Palinurus elephas in the presence of a predator (Buscaino et al., 2011).
The results above discussed that show different behavioral responses depending on the sound stimuli (considering their diverse band frequency ranges) are novel and interesting given that support the idea about crabs may be discerning diverse sound sources. Behavioral experiments demonstrating how animal react are useful to assess their hearing capability (Popper and Hawkins, 2021). In this sense, it is important to consider that the experiment was conducted in a tank, thus, conditions such as environmental variables were completely controlled which is important given the high variability of the natural habitat of the crab (coastal lagoon). However, it also may be highlighted that the sound properties of acoustic stimuli can get modified by the surrounding environment (reflexions of the tank), thus, implying differences in tanks sound propagation compared to habitat sound propagation (Akamatsu et al., 2002). Besides, it was highly reported that crustaceans might only detect the particle motion component of the sound because the lacking of gas-filled organs inside the exoskeleton (Popper et al., 2001; but see Radford et al., 2022). A limitation of the present study was the lack of measurements of the particle motion sound component. To obtain results that are more representative of what occurs in nature and taking into account the fact that the description of the pressure variation alone is not exhaustive when studying the reactions to sound stimuli in crustaceans, future studies should be conducted in nature (or in bigger tanks) and with systems able to characterize also the particles motion.
Regarding the different temporal sequences of the sound stimuli emission pattern used in the present study, low and high rate, significant differences were found between patterns: the high rate ones showed an effect on the behavioral variables. In the Mar Chiquita coastal lagoon, both emission patterns are commonly represented by motorboat passages and fish choruses, mainly the high rate ones in the lagoon mouth, during the warmer months (spring and summer) (Ceraulo et al., 2020). Thus, the results found, demonstrate the importance of the potential effects of the high rate emission pattern of sound on this crabs species in its natural habitat. Similarly, a previous study conducted on a marine fish with parental care found that only a continuous noise (high rate temporal pattern) negatively affected nest inspection and spawning compared to the intermittent (low rate temporal pattern) treatment, thus, affecting reproductive success and offspring fitness (Blom et al., 2019). Also in a reef fish, it was demonstrated that motorboats affected parental behavior and offspring survival under a long-term exposure study (Nedelec et al., 2017).
Considering the effects of the diverse stimuli, all high rate patterns affected the maternal care quantified as probing + flapping, and the flapping behavior. Thus, flapping was the greatest behavior affected by the different stimuli. The flapping is the most frequent maternal care behavior displayed in this species (Silva et al., 2007), and the greatest related to oxygen provision to the embryo mass (Fernández et al., 2000). Consequently, this result may indicate a potential negative effect on the eggs oxygen supply. Mating behavior and maternal care, proxies for reproductive success, are behavioral traits conforming to important components of an individual’s fitness given that reflect the survival capacity (Andersson, 1994). As well demonstrated in previous studies, brooding care in marine decapods is directly associated with the oxygen supply to the embryo mass (see Baeza and Fernández, 2002). Although we did not conduct a long-term study to evaluate costs on offspring, the negative effect of sound sources on the frequency of the maternal behaviors displayed may imply a reduction in oxygen supply, potentially affecting embryonic development, and consequently, offspring survival. Estuaries are changeable and vulnerable environments, characterized by high fluctuations in chemical and physical parameters, such as salinity, temperature and dissolved oxygen (Viaroli et al., 2007). The effects of salinity on embryonic development were previously reported in N. granulata through in vitro experiments (Bas and Spivak, 2000). The laboratory experiments performed in the present study contained oxygen-saturated seawater. However, given the variable conditions of the Mar Chiquita coastal lagoon estuary, e.g. dissolved oxygen (Luppi et al., 2013), the effect of sound on maternal behavior, and the potential consequent reduction in the oxygen supply, might be considered since it could add negative effects to the natural low dissolved oxygen concentrations of the habitat, impairing embryonic development. It was demonstrated that oxygen limitation retarded the development of inner embryos in gastropods (Cohen and Strathmann, 1996), and increased the risk of egg predation in a fish with parental care (Olsson et al., 2016). Specifically on aquatic invertebrates, the adverse impacts of hypoxia were widely assessed (reviewed by Galic et al., 2019). The physiological constraint of oxygen provision in marine invertebrates may have important ecological and evolutionary consequences at the population level (Baeza and Fernández, 2002). Future long-term studies conducted in N. granulata testing the effect of sound throughout the embryonic development and assessing direct traits associated to brood survival (e.g. number of hatched larvae, larvae weight and size, etc.) would help to better understand the effect of sound on offspring fitness and, consequently, the potential effects at a population level.
In this context, it is important to highlight that, although some studies evaluated the effects of anthropogenic sounds on invertebrates (e.g. Morley et al., 2018; Solé et al., 2018), very few have focused on the impacts of noise on marine ecosystem services considering how affects species that mediate ecosystem functioning (for an exception see Solan et al., 2016). The responses of marine invertebrates to anthropogenic noise are still little known, hindering the understanding of ecosystem impacts and the development of mitigation plans (Wale et al., 2019). In this sense, the present study contributes to a great extent to the knowledge of the anthropogenic sound effect on an ecosystem engineering key crab species in a coastal lagoon that provides important ecosystem services. Besides, the results demonstrating no negative effects on behavior of the low rate anthropogenic stimuli may suggest potential mitigation actions such as the reduction of the noise emission pattern rate. Thus, the present study provides important data to be used in the development of management plans and sustainable use in the Mar Chiquita coastal lagoon.
Data availability statement
The original contributions presented in the study are included in the article/supplementary material. Further inquiries can be directed to the corresponding author.
Author contributions
MM, MC, TL and GB designed the experiment. MM conducted the experiment. MC and GB performed the acoustical analysis. MM wrote the draft of the manuscript. All authors reviewed and edited the manuscript. MM, MG, TL and GB provided resources for this study. All authors contributed to the article and approved the submitted version.
Funding
This project was funded by the Agencia Nacional de Promoción Científica y Tecnológica (PICT-2019-668, PICT-2017-373) and Universidad Nacional de Mar del Plata (EXA 984/20). Financial support was also provided by the CAIMAR Joint Laboratory Italy-Argentina (Laboratori Congiunti Bilaterali Internazionali) of the Italian National Research Council (2017–2019) and the project BOSS: Study of bioacoustics and applications for the sustainable exploitation of marine resources (Projects of major importance in the Scientific and Technological Collaboration Executive Programs, funded by the Italian Ministry of Foreign Affairs and International Cooperation and the Argentinean Ministry of Science, Technology and Innovative Productivity).
Acknowledgments
The authors thank Jesús Nuñez for his help with the statistical analysis.
Conflict of interest
The authors declare that the research was conducted in the absence of any commercial or financial relationships that could be construed as a potential conflict of interest.
Publisher’s note
All claims expressed in this article are solely those of the authors and do not necessarily represent those of their affiliated organizations, or those of the publisher, the editors and the reviewers. Any product that may be evaluated in this article, or claim that may be made by its manufacturer, is not guaranteed or endorsed by the publisher.
References
Akamatsu T., Okumura T., Novarini N., Yan Y. H. (2002). Empirical refinements applicable to the recording of fish sounds in small tanks. J. Acoust. Soc Am. 112, 3073–3082. doi: 10.1121/1.1515799
Baeza J. A., Fernández M. (2002). Active brood care in Cancer setosus (Crustacea: Decapoda): the relationship between female behaviour, embryo oxygen consumption and the cost of brooding. Funct. Ecol. 16, 241–251. doi: 10.1046/j.1365-2435.2002.00616.x
Bas C. C., Spivak E. D. (2000). Effect of salinity on embryos of two southwestern atlantic estuarine grapsid crab species cultured in vitro. J. Crust. Biol. 20, 647–656. doi: 10.1163/20021975-99990088
Blasina G. E., Barbini S. A., Díaz de Astarloa J. M. (2010). Trophic ecology of the black drum, Pogonias cromis (Sciaenidae), in mar chiquita coastal lagoon (Argentina). J. Appl. Ichthyol. 26, 528–534. doi: 10.1111/j.1439-0426.2010.01459.x
Blom E., Kvarnemo C., Dekhla I., Schöld S., Andersson M. H., Svensson O., et al. (2019). Continuous but not intermittent noise has a negative impact on mating success in a marine fish with paternal care. Sci. Rep. 9, 5494. doi: 10.1038/s41598-019-41786-x
Boon P. Y., Yeo D. C. J., Todd P. A. (2009). Sound production and reception in mangrove crabs Perisesarma spp. (Brachyura: Sesarmidae). Aquat. Biol. 5, 107–116. doi: 10.3354/ab00136
Buscaino G., Filiciotto F., Gristina M., Buffa G., Bellante A., Maccarrone V., et al. (2011). Defensive strategies of European spiny lobster Palinurus elephas during predator attack. Mar. Ecol. Prog. Ser. 423, 143–154. doi: 10.3354/meps08957
Buscaino G., Gavio A., Galvan D., Filiciotto F., Maccarrone V., de Vincenzi G., et al. (2015). Acoustic signals and behaviour of Ovalipes trimaculatus in the context of reproduction. Aquat. Biol. 24, 61–73. doi: 10.3354/ab00636
Celi M., Filiciotto F., Vazzana M., Arizza V., Maccarrone V., Ceraulo M., et al. (2015). Shipping noise affecting immune responses of european spiny lobster Palinurus elephas (Fabricius 1787). Can. J. Zool. 93, 113–121. doi: 10.1139/cjz-2014-0219
Ceraulo M., Papale E., Caruso F., Filiciotto F., Grammauta R., Parisi I., et al. (2018). Acoustic comparison of a patchy Mediterranean shallow water seascape: Posidonia oceanica meadow and sandy bottom habitats. Ecol. Indic. 85, 1030–1043. doi: 10.1016/j.ecolind.2017.08.066
Ceraulo M., Sal Moyano M. P., Bazterrica M. C., Hidalgo F. J., Papale E., Grammauta R., et al. (2020). Spatial and temporal variability of the soundscape in a southwestern Atlantic coastal lagoon. Hydrobiologia 847, 2255–2277. doi: 10.1007/s10750-020-04252-8
Ceraulo M., Sal Moyano M. P., Bazterrica M. C., Hidalgo F., Snitman S., Papale E., et al. (2022). Agonistic behaviour and sound production during male-male varunid crabs (Cyrtograpsus angulatus, Dana 1851) encounters. ). J. Mar. Sci. Eng. 10, 1370. doi: 10.3390/jmse10101370
Ceraulo M., Sal Moyano M. P., Hidalgo F. J., Bazterrica M. C., Mazzola S., Gavio M. A., et al. (2021). Boat noise and black drum vocalizations in mar chiquita coastal lagoon (Argentina). J. Mar. Sci. Eng. 9, 44. doi: 10.3390/jmse9010044
Chan A. A. Y. H., Giraldo-Perez P., Smith S., Blumstein D. T. (2010). Anthropogenic noise affects risk assessment and attention: the distracted prey hypothesis. Biol. Lett. 6, 458–461. doi: 10.1098/rsbl.2009.1081
Clark C. W., Ellison W. T., Southall B. L., Hatch L., Van Parijs S. M., Frankel A., et al. (2009). Acoustic masking in marine ecosystems: intuitions, analysis, and implication. Mar. Ecol. Progr. Ser. 395, 201–222. doi: 10.3354/meps08402
Clayton D. (2005). Substrate (acoustic/vibrational) communication and ecology of the ghost crab Ocypode jousseaumei (Brachyura: Ocypodidae), Mar. Freshw. Behav. Physiol. 38, 53–70. doi: 10.1080/10236240500057952
Clutton-Brock T. H. (1991). The evolution of parental care (Princeton, New Jersey: Princeton University Press).
Cohen C. S., Strathmann R. R. (1996). Embryos at the edge of tolerance: effects of environment and structure of egg masses on supply of oxygen to embryos. Biol. Bull. 190, 8–15. doi: 10.2307/1542671
del Valle Fathala M., Maldonado H. (2011). Shelter use during exploratory and escape behaviour of the crab Chasmagnathus granulatus: a field study. J. Ethol. 29, 263–273. doi: 10.1007/s10164-010-0253-x
Diesel R. (1992). Maternal care in the bromeliad crab, Metopaulias depressus: protection of larvae from predation by damselfly nymphs. Anim. Behav. 43, 803–812. doi: 10.1016/S0003-3472(05)80203-9
Erbe C., Reichmuth C., Cunningham K., Lucke K., Dooling R. (2016). Communication masking in marine mammals: a review and research strategy. Mar. Poll. Bull. 103, 15–38. doi: 10.1016/j.marpolbul.2015.12.007
Fernández M., Bock C., Pörtner H. (2000). The cost of being a caring mother: the ignored factor in the reproduction of marine invertebrates. Ecol. Lett. 3, 487–494. doi: 10.1046/j.1461-0248.2000.00172.x
Fernández M., Brante A. (2003). Brood care in brachyuran crabs: the effect of oxygen provision on reproductive costs. Rev. Chil. Hist. Nat. 76, 157–168. doi: 10.4067/S0716-078X2003000200003
Fernández M., Calderón R., Cifuentes M., Pappalardo P. (2006). Brooding behaviour and cost of brooding in small body size brachyuran crabs. Mar. Ecol. Prog. Ser. 309, 213–220. doi: 10.3354/meps309213
Filiciotto F., Sal Moyano M. P., de Vincenzi G., Hidalgo F., Sciacca V., Bazterrica M. C., et al. (2018). Are semiterrestrial crabs threatened by human noise? assessment of behavioural and biochemical responses of Neohelice granulata (Brachyura, varunidae) in tank. Mar. Poll. Bull. 137, 24–34. doi: 10.1016/j.marpolbul.2018.07.023
Filiciotto F., Sal Moyano M. P., Hidalgo F., de Vincenzi G., Bazterrica M. C., Ceraulo M., et al. (2019). Underwater acoustic communication during the mating behaviour of the semi-terrestrial crab Neohelice granulata. Sci. Nat. 106, 35. doi: 10.1007/s00114-019-1633-x
Filiciotto F., Vazzana M., Celi M., Maccarrone V., Ceraulo M., Buffa G., et al. (2016). Underwater noise from boats: measurement of its influence on the behavior and biochemistry of the common prawn (Palaemon serratus, pennant 1777). J. Exp. Mar. Biol. Ecol. 478, 24–33. doi: 10.1016/j.jembe.2016.01.014
Flood A. S., Goeritz M. L., Radford C. A. (2019). Sound production and associated behaviours in the new Zealand paddle crab Ovalipes catharus. Mar. Biol. 166, 162. doi: 10.1007/s00227-019-3598-x
Galic N., Hawkins T., Forbes V. E. (2019). Adverse impacts of hypoxia on aquatic invertebrates: A meta-analysis. Sci. Total Environ. 652, 736–743. doi: 10.1016/j.scitotenv.2018.10.225
Goh S. L. R., Vishnu H., Ng N. K. (2019). The sounds of fighting: contests between violet vinegar crabs, Episesarma versicolor (Tweedie 1940) (Decapoda: Brachyura: Sesarmidae), are resolved through acoustic communication. J. Crust. Biol. 39, 331–341. doi: 10.1093/jcbiol/ruz023
Gutiérrez J. L., Jones C. G., Groffman P. M., Findlay S. E. G., Iribarne O. O., Ribeiro P. D., et al. (2006). The contribution of crab burrow excavation to carbon availability in surficial salt-marsh sediments. Ecosystems 9, 647–658. doi: 10.1007/s10021-006-0135-9
Hartig F. (2018). DHARMa: residual diagnostics for hierarchical (multi-level/mixed) regression models. r package v. 0.2. 0 (Regensburg: University of Regensburg).
Hughes A. R., Mann D. A., Kimbro D. L. (2014). Predatory fish sounds can alter crab foraging behaviour and influence bivalve abundance. Proc. R. Soc B 281, 20140715. doi: 10.1098/rspb.2014.0715
Kennedy E. V., Holderied M. W., Mair J. M., Guzman H. M., Simpson S. D. (2010). Spatial patterns in reef-generated noise relate to habitats and communities: evidence from a Panamanian case study. J. Exp. Mar. Biol. Ecol. 395, 85–92. doi: 10.1016/j.jembe.2010.08.017
Lenth R., Singmann H., Love J., Buerkner P., Herve M. (2018). “Emmeans: Estimated marginal means, aka least-squares means,” in (R package version 1.1). Available at: https://CRAN.R-project.org/package¼emmeans.
Luczkovich J. J., Daniel III H. J., Hutchinson M., Jenkins T., Johnson S. E., Pullinger R. C., et al. (2000). Sounds of sex and death in the sea: bottlenose dolphin whistles suppress mating choruses of silver perch. Bioacoustics 10, 323–334. doi: 10.1080/09524622.2000.9753441
Luppi T., Bas C., Mendez Casariego A., Albano M., Lancia J., Kittlein M., et al. (2013). The influence of habitat, season and tidal regime in the activity of the intertidal crab Neohelice (=Chasmagnathus) granulata. Helgol. Mar. Res. 67, 1–15. doi: 10.1007/s10152-012-0300-9
Luppi T., Rodriguez E. (2020). Neohelice granulata: a model species for the study of decapod crustaceans. vol 1 life history and ecology (Cambridge: Cambridge Scholar Publishing).
Matsinos Y. G., Mazaris A. D., Papadimitriou K. D., Mniestris A., Hatzigiannidis G., Maioglou D., et al. (2008). Spatio-temporal variability in human and natural sounds in a rural landscape. Landscape Ecol. 23, 945–959. doi: 10.1007/s10980-008-9250-7
McCloskey K. P., Chapman K. E., Chapuis L., McCormick M. I., Radford A. N., Simpson S. D. (2020). Assessing and mitigating impacts of motorboat noise on nesting damselfish. Environ. Poll. 266, 115376. doi: 10.1016/j.envpol.2020.115376
Miller P. J. O., Isojunno S., Siegal E., Lamb F. P. A., Kvadsheimc P. H., Curé C. (2022). Behavioral responses to predatory sounds predict sensitivity of cetaceans to anthropogenic noise within a soundscape of fear. PNAS 119, e2114932119. doi: 10.1073/pnas.2114932119
Morley E. L., Jones G., Radford A. N. (2018). The importance of invertebrates when considering the impacts of anthropogenic noise. Proc. R. Soc B 281, 20132683. doi: 10.1098/rspb.2013.2683
Nakamachi T., Asakura A., Hirohashi N. (2021). “Acoustic/vibration behaviors in crustaceans,” in Neuroendocrine regulation of animal vocalization. Eds. Rosenfeld C. S., Hoffmann F. (Elsevier, London: Academic Press), 39–54.
Naylor J. K., Taylor E. W. (1999). Heart rate and gill ventilation in ovigerous and non-ovigerous edible crabs, Cancer pagurus: the effect of disturbance, substrate and starvation. Mar. Behav. Physiol. 32, 129–145. doi: 10.1080/10236249909379044
Nedelec S. L., Mills S. C., Lecchini D., Nedelec B., Simpson S. D., Radford A. N. (2016). Repeated exposure to noise increases tolerance in a coral reef fish. Environ. Pollut. 216, 428–436. doi: 10.1016/j.envpol.2016.05.058
Nedelec S. L., Radford A. N., Gatenby P., Keesje Davidson I., Velasquez Jimenez L., Travis M., et al. (2022). Limiting motorboat noise on coral reefs boosts fish reproductive success. Nat. Commun. 13, 2822. doi: 10.1038/s41467-022-30332-5
Nedelec S. L., Radford A. N., Pearl L., Nedelec B., McCormick M. I., Meekan M. G., et al. (2017). Motorboat noise impacts parental behaviour and offspring survival in a reef fish. Proc. R. Soc B. 284, 20170143. doi: 10.1098/rspb.2017.0143
Nousek-McGregor A. E., Mei F. T. L. (2016). “Does noise from shipping and boat traffic affect predator vigilance in the European common hermit crab?,” in The effects of noise on aquatic life II, advances in experimental medicine and biology 875. Eds. Popper A. N., Hawkins A. (New York: Springer), 767–774.
Olsson K. H., Kvarnemo C., Andrén M. N., Larsson T. (2016). Hypoxia increases the risk of egg predation in a nest-guarding fish. R. Soc Open Sci. 3, 160326. doi: 10.1098/rsos.160326
Palumbi S. R., Johnson B. A. (1982). “A note on the influence of life-history stage on metabolic adaptation: the responses of limulus eggs and larvae to hypoxia,” in Physiology and biology of horseshoe crabs. Eds. Bonaventura J. C., Tesh S. (New York), 115–124.
Patek S. N., Shipp L. E., Staaterman E. R. (2009). The acoustics and acoustic behavior of the California spiny lobster (Panulirus interruptus). J. Acoust. Soc Am. 125, 3434–3443. doi: 10.1121/1.3097760
Picciulin M., Sebastianutto L., Codarin A., Farina A., Ferrero E. A. (2010). In situ behavioural responses to boat noise exposure of Gobius cruentatus (Gmelin 1789; fam. gobiidae) and Chromis chromis (Linnaeus 1758; fam. pomacentridae) living in a marine protected area. J. Exp. Mar. Biol. Ecol. 386, 125–132. doi: 10.1016/j.jembe.2010.02.012
Pijanowski B. C., Villanueva-Rivera L. J., Dumyahn S. L., Farina A., Krause B. L., Napoletano B. M., et al. (2011). Soundscape ecology: the science of sound in the landscape. Bioscience 61, 203–216. doi: 10.1525/bio.2011.61.3.6
Popper A. N., Hawkins A. D. (2021). Fish hearing and how it is best determined. ICES J. Mar. Sci. 78, 2325–2336. doi: 10.1093/icesjms/fsab115
Popper A. N., Hawkins A. D., Fay R. R., Mann D. A., Bartol S., Carlson T. J., et al. (2014). “Effects on sound exposure,” in Sound exposure guidelines for fishes and sea turtles: a technical report prepared by ANSI-accredited standards committee S3/SC1 and registered with ANSI. Ed. Popper A. N., et al (New York: Springer and ASA press), 17–21.
Popper A. N., Salmon M., Horch K. W. (2001). Acoustic detection and communication by decapod crustaceans. J. Comp. Physiol. A 187, 83–89. doi: 10.1007/s003590100184
Radford C. A., Tay K., Goeritz M. L. (2022). Comparative sound detection abilities of four decapod crustaceans. J. Exp. Biol. 225, jeb243314. doi: 10.1242/jeb.243314
R Core Team (2016). R: A language and environment for statistical computing. R Foundation for Statistical Computing (Vienna, Austria). Available at: https://www.R-project.org/
Remage-Healey L., Nowacek D. P., Bass A. H. (2006). Dolphin foraging sounds suppress calling and elevate stress hormone levels in a prey species, the gulf toadfish. J. Exp. Biol. 209, 4444–4451. doi: 10.1242/jeb.02525
Roberts L. (2021). Substrate-borne vibration and sound production by the land hermit crab Coenobita compressus during social interactions. J. Acoust. Soc Am. 149, 3261–3272. doi: 10.1121/10.0004988
Roberts L., Elliott M. (2017). Good or bad vibrations? impacts of anthropogenic vibration on the marine epibenthos. Sci. Total Environ. 595, 255–268. doi: 10.1016/j.scitotenv.2017.03.117
Rodriguez E., Luppi T. (2020). “Neohelice granulata: a model species for the study of decapod crustaceans,” in Vol 2 anatomy and physiology (Cambridge: Cambridge Scholar Publishing).
Sal Moyano M. P., Ceraulo M., Hidalgo F., Luppi T., Nuñez J., Radford C. A., et al. (2021). Effect of natural and anthropogenic sound on the orientation of larvae and juveniles of estuarine crabs. Mar. Ecol. Progr. Ser. 669, 107–120. doi: 10.3354/meps13739
Sal Moyano M. P., Ceraulo M., Mazzola S., Buscaino G., Gavio M. A. (2019). Sound production mechanism in the semiterrestrial crab Neohelice granulata (Brachyura, varunidae). J. Acoust. Soc Am. 146, 3466–3474. doi: 10.1121/1.5128473
Silva P. V., Luppi T. A., Spivak E. D. (2007). Epibiosis on eggs and brooding care in the burrowing crab Chasmagnathus granulatus (Brachyura:Varunidae): comparison between mudflats and salt marshes. J. Mar. Biol. Ass. U. K. 87, 893–901. doi: 10.1017/S0025315407056068
Slabbekoorn H., Bouton N., van Opzeeland I., Coers A., ten Cate C., Popper A. N. (2010). A noisy spring: the impact of globally rising underwater sound levels on fish. Trends Ecol. Evol. 25, 419–427. doi: 10.1016/j.tree.2010.04.005
Snitman S. M., Mitton F., Provenzal M., Ceraulo M., Buscaino G., Gavio M. A., et al. (2022). Effect of biological and anthropogenic habitat sounds on oxidative stress biomarkers and behavior in a key crab species. Comp. Biochem. Physiol. C 257, 109344. doi: 10.1016/j.cbpc.2022.109344
Solan M., Hauton C., Godbold J. A., Wood C. L., Leighton T. G., White P. (2016). Anthropogenic sources of underwater sound can modify how sediment-dwelling invertebrates mediate ecosystem properties. Sci. Rep. 6, 1–9. doi: 10.1038/srep20540
Solé M., Lenoir M., Fortuño J. M., van der Schaar M., André M. (2018). A critical period of susceptibility to sound in the sensory cells of cephalopod hatchlings. Biol. Open 7, bio033860. doi: 10.1242/bio.033860
Spivak E. D., Lovrich G., Luppi T., Farias N., Ocampo E. (2019). Annotated checklist and bibliography of shrimps, lobsters, crabs and their allies (Crustacea: Decapoda) of the southwestern Atlantic ocean (Argentina and Uruguay). Frente Marítimo 26, 1–164.
Stanley J. A., Radford C. A., Jeffs A. G. (2012). Location, location, location: finding a suitable home among the noise. Proc. R. Soc Lond. B Biol. Sci. 279, 3622–3631. doi: 10.1098/rspb.2012.0697
Strathmann R. R. (1985). Feeding and nonfeeding larval development and life-history evolution in marine invertebrates. Ann. Rev. Ecol. Syst. 16, 339–361. doi: 10.1146/annurev.es.16.110185.002011
Strathmann R. R., Strathmann M. F. (1995). Oxygen supply and limits on aggregation of embryos. J. Mar. Biol. Ass. U. K. 75, 413–428. doi: 10.1017/S0025315400018270
Taylor J. R. A., DeVries M. S., Elias D. O. (2019). Growling from the gut: Co-option of the gastric mill for acoustic communication in ghost crabs. Proc. R. Soc B Biol. Sci. 286, 20191161. doi: 10.1098/rspb.2019.1161
Thiel M. (1999). “Extended parental care behavior in crustaceans –a comparative overview,” in The biodiversity crisis and Crustacea: Proceedings of the fourth international crustacean congress. Eds. Klein J.C.V. V., Schram F. R. (Balkema, Rotterdam: CRC Press), 221–226.
Tidau S., Briffa M. (2019). Distracted decision makers: ship noise and predation risk change shell choice in hermit crabs. Behav. Ecol. 30, 1157–1167. doi: 10.1093/beheco/arz064
Trumbo S. T. (2012). “Pattern of paternal care in invertebrates,” in The evolution of parental care. Eds. Royle N. J., Smiseth P. T., Kölliker M. (Oxford: Oxford Scholarship Online), 81–100.
Ver Hoef J. M., Boveng P. L. (2007). Quasi-poisson vs. negative binomial regression: how should we model overdispersed count data? Ecology 88, 2766–2772. doi: 10.1890/07-0043.1
Viaroli P., Laserre P., Campostrini P. (2007). Lagoons and coastal wetlands. Hidrobiologia 577, 1–3. doi: 10.1007/s10750-006-0412-9
Wale M. A., Briers R. A., Hartl M. G. J., Brysona D., Diele K. (2019). From DNA to ecological performance: effects of anthropogenic noise on a reef-building mussel. Sci. Total Environ. 689, 126–132. doi: 10.1016/j.scitotenv.2019.06.380
Wale M. A., Simpson S. D., Radford A. N. (2013). Noise negatively affects foraging and antipredator behaviour in shore crabs. Anim. Behav. 86, 111–118. doi: 10.1016/j.anbehav.2013.05.001
Yim-Hol Chan A. A., David Stahlman W., Garlick D., Fast C. D., Blumstein D. T., Blaisdell A. P. (2010). Increased amplitude and duration of acoustic stimuli enhance distraction. Anim. Behav. 80, 1075–1079. doi: 10.1016/j.anbehav.2010.09.025
Keywords: maternal care, anthropogenic sounds, biologic sounds, negative effects, crustaceans
Citation: Sal Moyano MP, Ceraulo M, Luppi T, Gavio MA and Buscaino G (2023) Anthropogenic and biological sound effects on the maternal care behavior of a key crab species. Front. Mar. Sci. 10:1050148. doi: 10.3389/fmars.2023.1050148
Received: 21 September 2022; Accepted: 09 January 2023;
Published: 23 January 2023.
Edited by:
Bronwyn M. Gillanders, University of Adelaide, AustraliaReviewed by:
Lucille Chapuis, University of Bristol, United KingdomMaite Mascaro, National Autonomous University of Mexico, Mexico
Copyright © 2023 Sal Moyano, Ceraulo, Luppi, Gavio and Buscaino. This is an open-access article distributed under the terms of the Creative Commons Attribution License (CC BY). The use, distribution or reproduction in other forums is permitted, provided the original author(s) and the copyright owner(s) are credited and that the original publication in this journal is cited, in accordance with accepted academic practice. No use, distribution or reproduction is permitted which does not comply with these terms.
*Correspondence: María Paz Sal Moyano, salmoyan@mdp.edu.ar