- 1Coastal and Marine Management Group, Leibniz-Institute for Baltic Sea Research, Rostock, Germany
- 2Marine Research Institute, Klaipeda University, Klaipėda, Lithuania
- 3Biological Station Hiddensee, University of Greifswald, Kloster, Germany
- 4Landesamt für Umwelt, Naturschutz und Geologie, Dezernat: Gewässergüte von Fließ- und Küstengewässern, Güstrow, Germany
We combine historical and recent monitoring data with modeling to get a better insight into water quality development of the large Oder/Szczecin Lagoon at the German/Polish border in the southern Baltic Sea region and especially of the role of macrophytes. Data indicates that the system is eutrophic for centuries and a naturally eutrophic system. During the last decades, external nutrient loads decreased but still keep the system in a eutrophic state. The systems primary production is limited by light and nitrogen and cannot be sufficiently managed by external nutrient load reductions. We consider 36% macrophyte coverage of the lagoon area as potential historical maximum. Despite its shallowness the lagoon was never a macrophyte dominated, clear water system. About 31% of the lagoon area would be covered by macrophytes in a good ecological status according to the European Water Framework Directive. However, the existing water transparency targets seem too ambitious and not realistic. Changes in macrophyte coverage on water quality are restricted to near shore areas and hardly affect the open lagoon. Existing models require an improved representation of water transparency and effects on macrophyte colonization depth. Presently the patchy macrophyte coverage is only about 12% of the lagoon area. This low coverage and a relatively poor species composition results in a non-satisfactory state classification. However, ecologically valuable angiosperms and charophytes seem to recover. A strict avoidance of mechanical disturbances could be a measure to support macrophyte re-colonization. A systematic improvement of piscivorous fish stocks may be a supporting measure to reduce eutrophication. Restoration perspectives and consequences for environmental policies are discussed.
1 Introduction
The shallow Oder or Szczecin Lagoon at the German/Polish border in the southern Baltic Sea belongs to the largest lagoons in Europe. The lagoon and its surrounding host important and valuable habitats. As a consequence, the entire lagoon belongs to the European Natura 2000 network for rare and threatened species, large parts are under landscape protection, the coastal area hosts two national parks and several areas are nature reserves. The high diversity of rare habitats results from a wide range of different soils and a diverse glacial morphology. For centuries, fishery was the dominating economic sector. Today, tourism at the lagoon is gaining more and more importance. However, the major problem is the poor ecological water quality in the lagoon that degrades its ecological functioning and hampers bathing tourism development. This situation is not only typical for other lagoons in Germany, such as the Darss-Zingst lagoons or the Schlei, or for other lagoons in the Baltic region, such as the Vistula and the Curonian lagoons, but is common for many lagoons world-wide. Therefore, lessons learned from the Oder Lagoon have a general relevance and can, with some adjustments, be transferred to other systems, as well.
Responsible for the poor water quality in the lagoon are very high riverine nutrient loads, especially with the Oder (Polish: Odra) river. With an average water discharge of about 500 m3/s and a drainage area of about 120,000 km2, the Oder/Odra River is one of the most important rivers in the Baltic Sea catchment. It contributes about 93% of the total nitrogen (TN) and 95% of the total phosphorus (TP) loads to the lagoon. According to Friedland et al. (2019a), the annual riverine TN and TP loads to the lagoon increased between the 1880’s and the maximum in the 1980’s from about 14,000 t TN (1,000 t TP) to 115,000 t TN (10,500 t TP). During recent decades the loads declined to 56,750 t TN (2,800 t TP) in 2010-2014. The fast load reduction resulted from political changes especially in Poland, causing a transformation of industries, reduced agricultural loads and improved waste water treatment. For reaching a good ecological status of the Baltic Sea, the HELCOM Baltic Sea Action Plan (HELCOM, 2013) demands a reduction of annual riverine nutrient inputs to the lagoon to about 48,850 t TN (1,570 t TP). The present Polish thresholds for a good ecological status of large lowland rivers (4.0 mg TN/l and 0.29 mg TP/l) (Garcia et al., 2012) would result in annual loads to the lagoon of about 65,000 t TN (4,900 t TP). The stricter German thresholds (2.6 mg TN/l and 0.1 mg TP/l) (BLANO, 2014) would end-up in loads of about 44,000 t TN (1,700 t TP). The German thresholds for rivers according to the European Water Framework Directive (WFD) are well in agreement with the demands from a Baltic Sea protection perspective (HELCOM, 2013). Questions are if these reduced loads would be sufficient to enable a good ecological quality in the lagoon, how a good ecological status would look like and/or if the lagoon is a naturally eutrophied system?
In the European WFD, nutrient concentrations in the water body still play an important role, but for the definition of a good ecological status of coastal waters, biological quality elements, namely phytoplankton, macrophytes and angiosperms, benthic invertebrate fauna as well as fish (in transitional water), are essential. It is well known, that submerged and emergent macrophytes are affected by water quality but at the same time have a strong influence on water quality, as well (Scheffer, 1998; Scheffer et al., 2001; Horppila and Nurminen, 2003; Hilt et al., 2006; Blindow et al., 2014). They reduce current velocities and waves, reduce sediment resuspension and increase water transparency (James and Barko, 1994; Van den Berg et al., 1999; Madsen et al., 2001; Hussner et al., 2010). Macrophytes interact with phytoplankton concentrations by reducing the light availability, competing for nutrients and by favoring zooplankton and its grazing (Balls et al., 1989; Jeppesen et al., 1999; Scheffer, 1998; Van Donk and van de Bund, 2002). They serve as sink for particulate matter but also as source for dissolved P, mobilized from sediments (Carpenter and Lodge, 1985; Jeppesen et al., 1999). Last not least, macrophytes provide habitats for a wide range of species, such as juvenile fish and invertebrates (Scheffer, 1998). These facts are known for decades. Despite that, the common model-based approaches in coastal waters for defining historic reference status for a water body, thresholds for a good water quality and external nutrient loads ensuring a good status usually do not take into account macrophytes (Schernewski et al., 2015; Friedland et al., 2019a). Therefore, a questions is whether the spatial macrophytes coverage and its long-term dynamic can be neglected, especially in shallow systems? Are the existing German thresholds describing the good ecological state for nutrients (0.07 mg/l TP; 0.53 mg/l TN), summer chlorophyll a (14.3 μg/l) (BLANO, 2014; Schernewski et al., 2015) and water transparency (Secchi depth of 1.7 m; Sagert et al., 2008) and suggested modified values (Friedland et al., 2019a) against this background reliable? If not, what are the general implications for defining water quality thresholds in shallow coastal waters?
Since macrophytes in itself are a core indicator for a good ecological status in the European WFD, additional questions are, what the best reachable state is and what the most suitable management options are? Is river basin management alone sufficient? Was this shallow lagoon ever dominated by macrophytes, faced a regime shift from a clear water to a turbid state and is its recovery hampered by hysteresis as suggested by Friedland et al. (2019a) or reported by Blindow and Meyer (2015) for other southern Baltic lagoons and bays?
To be able to answer these questions, we reconstruct the historic macrophytes coverage around 1890; document the present state of macrophyte species composition and spatial distribution in the lagoon; estimate the spatial coverage of emerse and submerse macrophyte species in a potential ‘good ecological status’ according to the WFD; carry out model scenario simulations on the potential effects of macrophytes on water quality, analyze the long-term development of water quality parameters that affect macrophytes and, last not least, assess the possible future states and management options. The paper focusses on restoration, management and policy implementation and less on biological aspects.
2 Study area and methods
2.1 Study area – the Oder/Szczecin Lagoon
The Oder Lagoon (53°48’N,14°08’E) has a surface area of 687 km². With an average depth of 3.8 m, the oligohaline lagoon is shallow and brackish (salinity between 1 and 3 PSU). It is connected to the Baltic Sea via three outlets. About 40% of the lagoon surface belongs to the Kleines Haff, the smaller bay in Germany, and 60% to the Wielki Zalew, the larger Polish bay. The average depth is 3.8 m with a natural maximum depths of 8.5 m (Figure 1). The dredged shipping channel across the Wielki Zalew has a depth above 10 m. Central parts of the lagoon show a salinity between 0.5 and 2 PSU, but the Swina shipping channel enables temporal Baltic water intrusions that increase the salinity locally up to 6 PSU (Radziejewska and Schernewski, 2008).
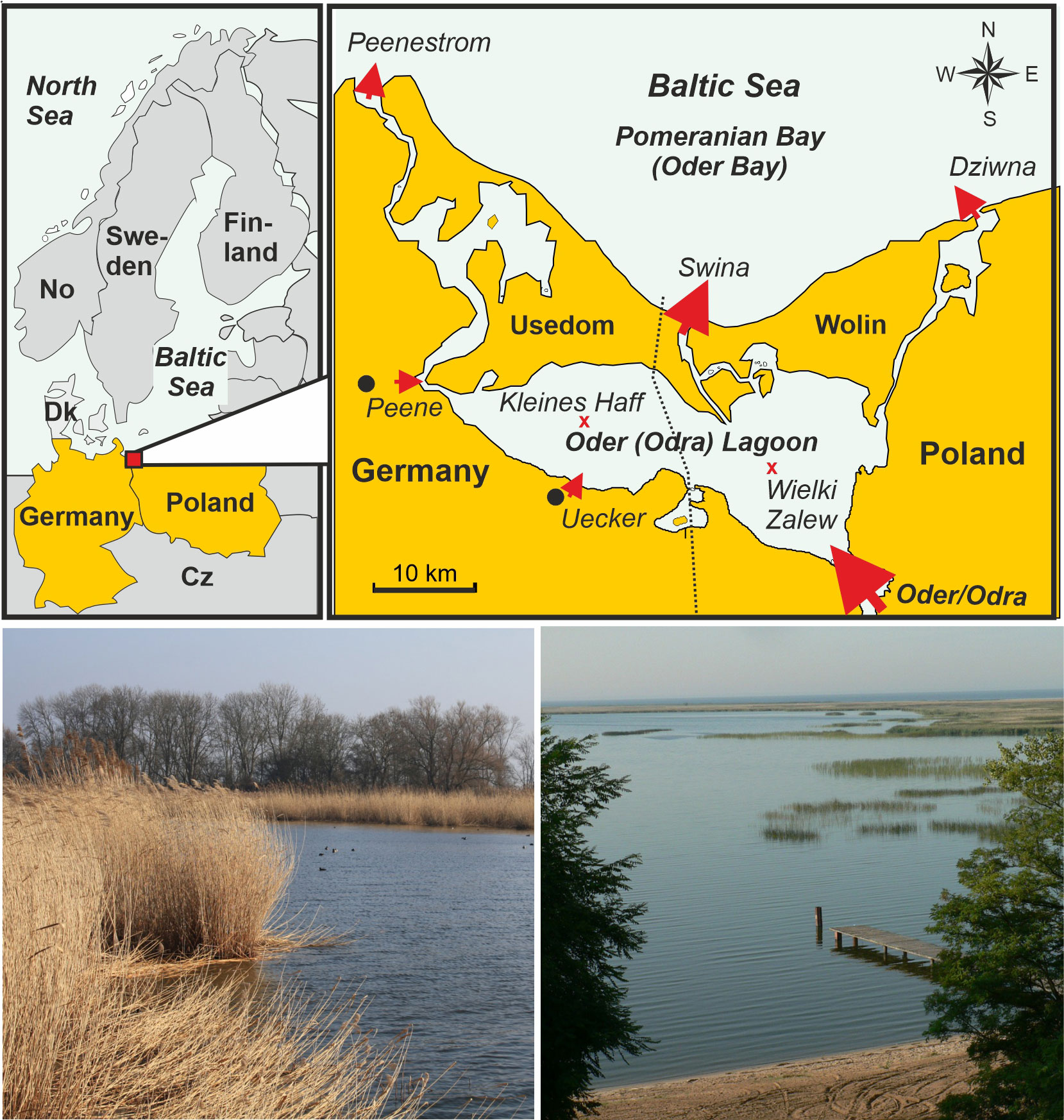
Figure 1 The Oder/Szczecin Lagoon at the German/Polish border, its subdivision into the western, German, Kleines Haff (small lagoon) and the eastern, Polish, Wielki Zalew (large lagoon) as well as major rivers and outlets to the Baltic Sea. The photos give an impression of the reed belt (Phragmites australis) in the western Kleines Haff (left) and bulrush (Schoenoplectus lacustris) areas in the northern Wielki Zalew (right). The red crosses indicate the central sampling stations in the two parts of the lagoon.
With a precipitation of about 550 mm/a, the climate is humid at the border between oceanic to continental. As a consequence, the rivers discharge large amounts of freshwater, with an average of 536 m³/s. Because of its large river basin of 120.000 km², the Oder/Odra river alone contributes 504 m³/a (Friedland et al., 2019a). The average water residence time is about 3 months in the Kleines Haff and around 1 month in Wielki Zalew. A winter ice cover lasting several weeks is still common and the water temperature exceeds 20°C during summer. In about 15% of the time, wind speed above 6 m/s prevails. This wind speed is usually sufficient to cause vertical mixing in the lagoon. With about 60%, wind-directions between south and west dominate (Radziejewska and Schernewski, 2008).
According to the OECD (1982) classification the lagoon is hypertrophic. According to the European WFD classification the chemical quality is classified as ‘not good’ (IKSO, 2022a). This is true for both parts of the lagoon, the eastern Wielki Zalew in Poland and the western Kleines Haff in Germany. Both form separate WFD assessment water bodies. In the Kleines Haff, for example, the concentrations (compared to the thresholds for a good ecological status) are for summerly water transparency (Secchi depth) 0.7 m (1.7 m), for summerly chlorophyll-a concentrations 70 μg/l (14.3 μg/l), for average annual total phosphorus concentrations 5 μmol/l (2.3 μmol/l) and for average annual total nitrogen concentrations 100 μmol/l (38.1 μmol/l) (Schernewski et al., 2015; Friedland et al., 2019a). The ecological quality according to the WFD, taking into account benthic flora and fauna, as well, is classified as ‘insufficient’ (IKSO, 2022a). An updated plan of measures in the river basin has recently been published (IKSO, 2022b).
2.2 Methods
The WFD requires a regular monitoring of macrophytes and an assessment of the ecological state. In the German Baltic, the official tool PHYBIBCO (PHYtoBenthic Index for Baltic inner COastal waters) is applied for quality assessments within the WFD. Elements are angiosperms and macrophytes (e.g. characean/charophytes). The ecological value of species, the percentage spatial coverage per water depth and the loss of colonization depth are criteria, as well. Emerse vegetation, such as reed and bulrush, is not taken into account (Nickel et al., 2019).
In the Kleines Haff, the assessment is presently based on seven transects sampled in three year intervals. Commissioned by the State Agency for Environment, Nature Conservation and Geology Mecklenburg-Vorpommern (LUNG), transect data was gathered and reported by company MariLim for the years 2007, 2008, 2015, 2018 and 2021. Earlier data for the Kleines Haff is reported in Gosselck and Schabelon (2007) for the year 1997/1998, Selig et al. (2006) for the year 2005 as well as Dumke (2001) and Porsche et al. (2008). To complement the transect data and to get a better overview about the spatial coverage and distribution of macrophytes in 2016, several long sidescan sonar profiles were taken by boat in different parts of the Kleines Haff. As soon as the sidescan sonar data indicated submerse macrophytes, samples were taken, and the species determined.
The hydrochemical and biological data for the Oder Lagoon covers the German and the Polish parts and was provided by the national authorities, the LUNG and the Pomeranian Voivodeship Inspectorate, in joint data reports accessible under https://www.wasserblick.net/. The monitoring follows the WFD requirements and includes a transnational inter-calibration to ensure data comparability. Presently, the physico-chemical and phytoplankton monitoring takes place at three locations on the German and three on the Polish side and includes a monthly sampling, at least during the ice-free period. LUNG (2016) provides more details on the monitoring program.
For the simulations, the well-established and validated model setup for Oder Lagoon was applied (Friedland et al., 2019b; Schernewski et al., 2019), which is based on the coupled model GETM-FABM-ERGOM (Neumann, 2000) with a horizontal resolution of 150m and 20 vertical layers that adjust their thickness to the density gradients (Gräwe et al., 2015). The model setup covers Oder Lagoon and the southern part of Pomeranian Bight. The hydrodynamic model is based on GETM (General Estuarine Transport Model; www.getm.eu; Burchard and Bolding, 2002) and computes temperatures, stratification, the current fields and resuspension dynamics. Wave induced resuspension is calculated using a simplified approach, which is still not fully validated, because of a lack of data. Via FABM (Framework for Aquatic Biogeochemical Models; Bruggeman and Bolding, 2014), IOW´s biogeochemical model ERGOM (Neumann, 2000; Neumann et al., 2002; Radtke et al., 2012) was incorporated to the model system. ERGOM is a NPZD model in its core, capable to simulate the nitrogen and phosphorus dynamics in the lower trophic food web. Therefore, three functional phytoplankton groups are considered (large phytoplankton mainly responsible for spring bloom, small phytoplankton growing mainly in summer season and nitrogen-fixing cyanobacteria, which are not depending on dissolved inorganic nitrogen). The growth of all three phytoplankton groups is limited by nutrient and light availability, as well as zooplankton grazing. The underwater light attenuation is computed dynamically, considering a constant background attenuation, as well as shading by phytoplankton and resuspended organic material. The light model was validated using Secchi Depth data by Friedland et al. (2019b). Dead phyto- and zooplankton is pooled as fast-sinking detritus, which gets re-mineralized into the dissolved nutrients, if oxygen is available. Oxygen consumption and production are included in all model processes via stoichiometric factors. A basic model for the nutrient dynamics in the sediment is included, capable to model key processes like denitrification and phosphate release under anoxic condition. While ERGOM was initially developed to model the nutrient and phytoplankton dynamics in the open Baltic Sea, key parameters were adjusted in the used setup to improve the model quality, using the available observations from the regular WFD monitoring stations (Friedland et al., 2019a). For details on the model, its validation and a critical discussion of the performance please refer to (Neumann, 2000; Friedland et al., 2019a; Friedland et al., 2019b; Schernewski et al., 2019).
The biogeochemical model ERGOM does not explicitly represent macrophytes in the form of state variables. Therefore, the model simulations have the character of a sensitivity study and only the model calculated relative changes between the scenarios can be regarded as sufficiently reliable for interpretation. As a consequence, the scenarios related to the effects of macrophytes were kept simple, as well. We consider the reduction of sediment resuspension and water transparency resulting from reduced current velocities and waves (James and Barko, 1994; Barko and James, 1998; Scheffer, 1998; Van den Berg et al., 1999; Madsen et al., 2001), the reduced light availability resulting from shading and the consequences of increased zooplankton on phytoplankton (Balls et al., 1989; Schriever et al., 1995; Jeppesen et al., 1999; Van Donk and van de Bund, 2002).
The quantitative effect of macrophytes depend on parameters such as species composition, density and water depth. A reliable and transferable model parametrization for the Oder Lagoon can hardly be derived and has been estimated based on the literature. Therefore, the model scenario simulations represent hardly more than a sensitivity analysis. We assume that macrophytes cover the lagoon fully down to a water depth of two meters. Within macrophyte stands we assume that no resuspension of organic matter from the sediment takes place, that macrophytes reduce the light availability in the water body by 70% (down to 1 m water depth) and 30% in the 1-2 m interval and an increased zooplankton grazing by 20%. The model simulations are carried out separately for every changed parameter. One simulation combined all parameter changes. All scenario results are compared to present model simulations without an explicit consideration of macrophytes.
3 Results
3.1 Macrophytes in the Oder/Szczecin Lagoon – the historic state
In the 1890’s, Brandt (1896) carried out a field survey and mapping of macrophytes in the eastern part of the lagoon, the Wielki Zalew. He reported bulrush (Juncus l.), Potamogeton species and other macrophytes down to a colonization depth of at least 2 m and mentioned a rich and diverse fauna in emerse macrophytes stands. Based on comments by Neuhaus (1933), data of Neubaur (1927) and Holtz (1892) and conclusions by Gosselck and Schabelon (2007) it can be assumed that charophytes were present in the 1890’s in different parts of the lagoon, as well. Studies of Schubert et al. (2003) indicate that the following species were present in the lagoon a century ago: Chara contraria, Chara hispida, Chara tomentosa, Chara globularis, Nitellopsis obtuse, Potamogeton lucens and Ranunculus reptans.
Figure 2 extrapolates the field data to the entire lagoon assuming a maximum colonization depth of 2.5 m and that no gradients between different parts of the lagoon exist. This colonization depth shows the best agreement with the map of Brandt (1896). We consider the resulting 36% macrophyte coverage as the likely maximum historical coverage with macrophytes and as reference for the WFD (40% of the Wielki Zalew and 32% of the Kleines Haff). In comparison, assuming a maximum colonization depth of 2 m would result in a total macrophyte covered area of 27% of the total lagoon surface area. It is likely that the existing gradients in water transparency between both parts of the lagoon (Friedland et al., 2019a) existed a century ago, as well. This means that the past spatial macrophyte coverage in the Kleines Haff (Figure 2) is possibly overestimated, but data that would allow an estimation of the maximum colonization depths 130 years ago is lacking. Transferring the present relative transparency gradient to the past would result in past maximum macrophyte coverage in the Kleines Haff of only about 20%. These facts suggest that the lagoon was never a macrophyte dominated, clear water system. However, it does not mean that macrophytes do not play an important role in the lagoon’s ecology.
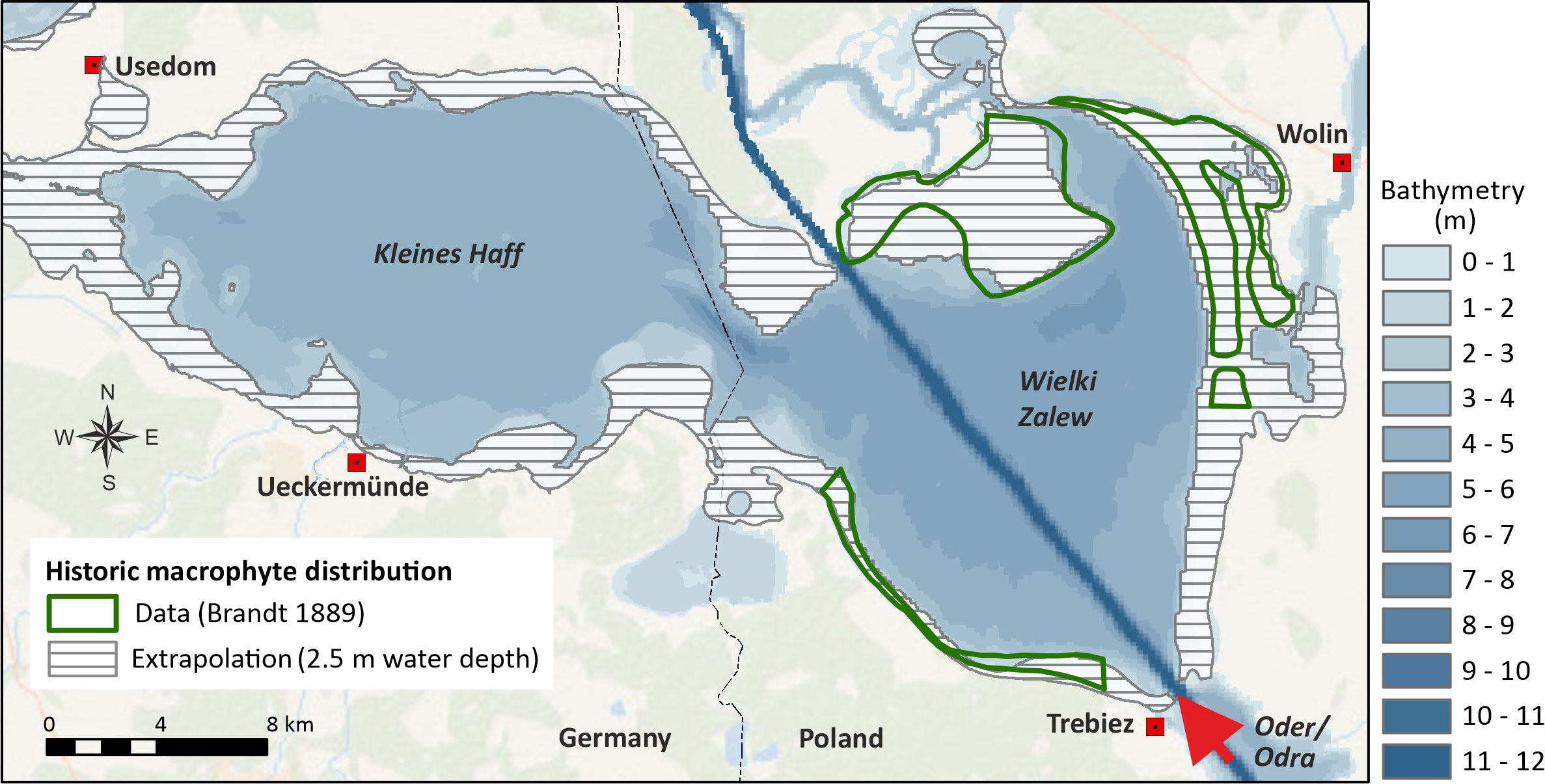
Figure 2 Macrophytes coverage around 1890 based on data (Brandt, 1896) and extrapolation to the entire lagoon assuming 2.5 m water depth as maximum colonization depth.
3.2 Present state of macrophyte coverage and composition
The results combine own data on spatial macrophyte coverages and colonization depths, with a literature analysis and transect data obtained from WFD monitoring. Focus is on the Kleines Haff. Reed (Phragmites australis) and bulrush (Schoenoplectus lacustris), littoral helophytes, are the dominant species and are abundant at the entire lagoon coastline. During the sampling campaign in 2016, reed was observed down to a water depth of 1.5 m and bulrush down to 2.6 m. These emerse macrophytes compete with submerged vegetation for space. The reed belts in the lagoon are dense. Three meters inside the reed belt (from the sea front) near the town Bellin, an average number of up to 312 reed stems/m2 with an average diameter of 7 mm was counted.
Only in sheltered areas of the Kleines Haff, submerse macrophytes are abundant and diverse. In front of emerse macrophyte belts and in shallow exposed areas the coverage is patchy with low densities (Dumke, 2001; Gosselck and Schabelon, 2007). Species and their share are compiled in Table 1. In the Kleines Haff, Potamogeton species are most abundant and cover plots of 5-50 m² (Gosselck and Schabelon, 2007) followed by Ceratophyllum demersum. The recent monitoring shows a significant coverage with Myriophyllum spicatum, as well.
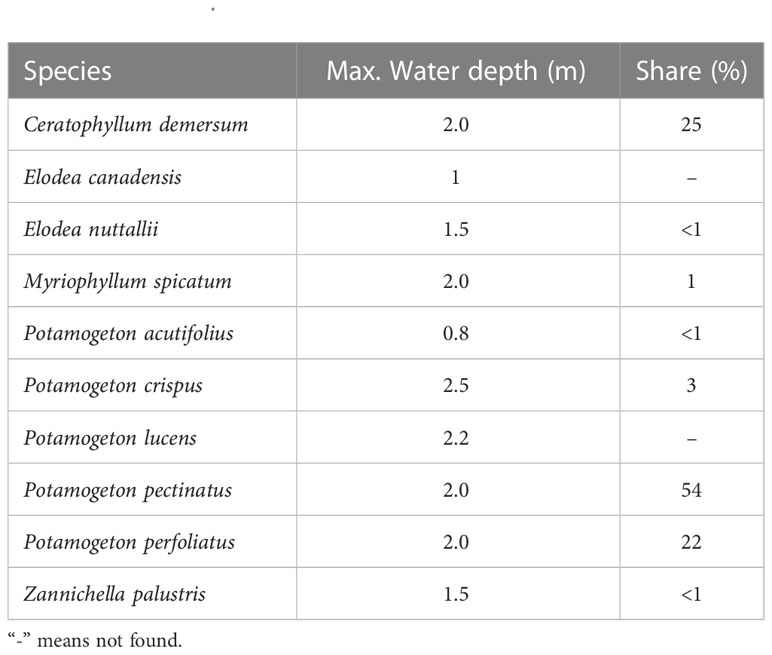
Table 1 Compilation of data on submerse macrophyte species and their maximum observed colonization depth in Kleines Haff based monitoring data and complementing literature (Selig et al., 2006; Gosselck and Schabelon, 2007; Porsche et al., 2008; Schadach, 2013). The shares are calculated based on the number of individuals (total=1920) found on all transects. Potamogeton pectinatus = Stuckenia pectinata.
A historical data compilation covering the last centuries (Schubert et al., 2007) documents the presence of seagrass (Zostera marina and Zostera noltei) in most of the south-western Baltic coastal waters. The data does not indicate the presence of seagrass in any part of the Oder Lagoon, because of its low salinity. Neubaur (1927) reports the dominance of charophytes in parts of the northern Wielki Zalew. Still in the 1960s, Garbacik-Wesolowska, 1969, 1973 in Wolnomiejski and Witek, 2013) mentions an area of 65 ha covered by charophytes in the Wielki Zalew and a 15.5% total macrophyte coverage of the Wielki Zalew. Until 2013, data does not prove the presence of charophytes in the Kleines Haff.
The most recent monitoring of 2015, 2018 and 2021, in the Kleines Haff reports 25 species for the Kleines Haff. The species spectrum includes the Charophytes Chara aspera and Chara baltica, which are found only sporadically, and the spermatophytes Ceratophyllum demersum, Elodea nuttallii, Myriophyllum spicatum, Phragmites australis, Potamogeton crispus, Potamogeton friesii, Potamogeton pectinatus, Potamogeton perfoliatus and, locally even, Zostera marina.
Based on the PHYtoBenthic Index used within the WFD assessment, the present state of macrophytes in the Oder Lagoon is classified as non-satisfactory. The PHYtoBenthic Index focusses on angiosperms and macrophytes (e.g. characean/charophytes), the ecological value of species, the percentage spatial coverage per water depth and the loss of colonization depth are criteria. Main reasons for the non-satisfactory state are a relatively poor species diversity and the lack of ecologically valuable species. The recent local observation of charophytes alone can hardly be interpreted as an improved ecological state of the lagoon. However, the data at least suggests a tendency towards an improvement.
Another important aspect that negatively affects the state assessment is the insufficient spatial coverage of macrophytes compared to the potential area at the present Secchi depth of 0.6 m (Figure 3). The reference value for the lower distribution limit of submerged macrophytes in the Kleines Haff is 3.0 m, according to the WFD assessment. The colonization depth for an excellent state is ≥2.7 m and for the good state between 2.4 m and 2.7 m, based on calculations by Domin et al. (2004). On average over the years 2015 and 2021 and over all transects, the present lower colonization depth is only 1.2 m and far below the threshold for a good status. Only very locally, on one transect at the northern coast near Gummlin, a colonization depth between 1.9 m and 2.2 m was recorded.
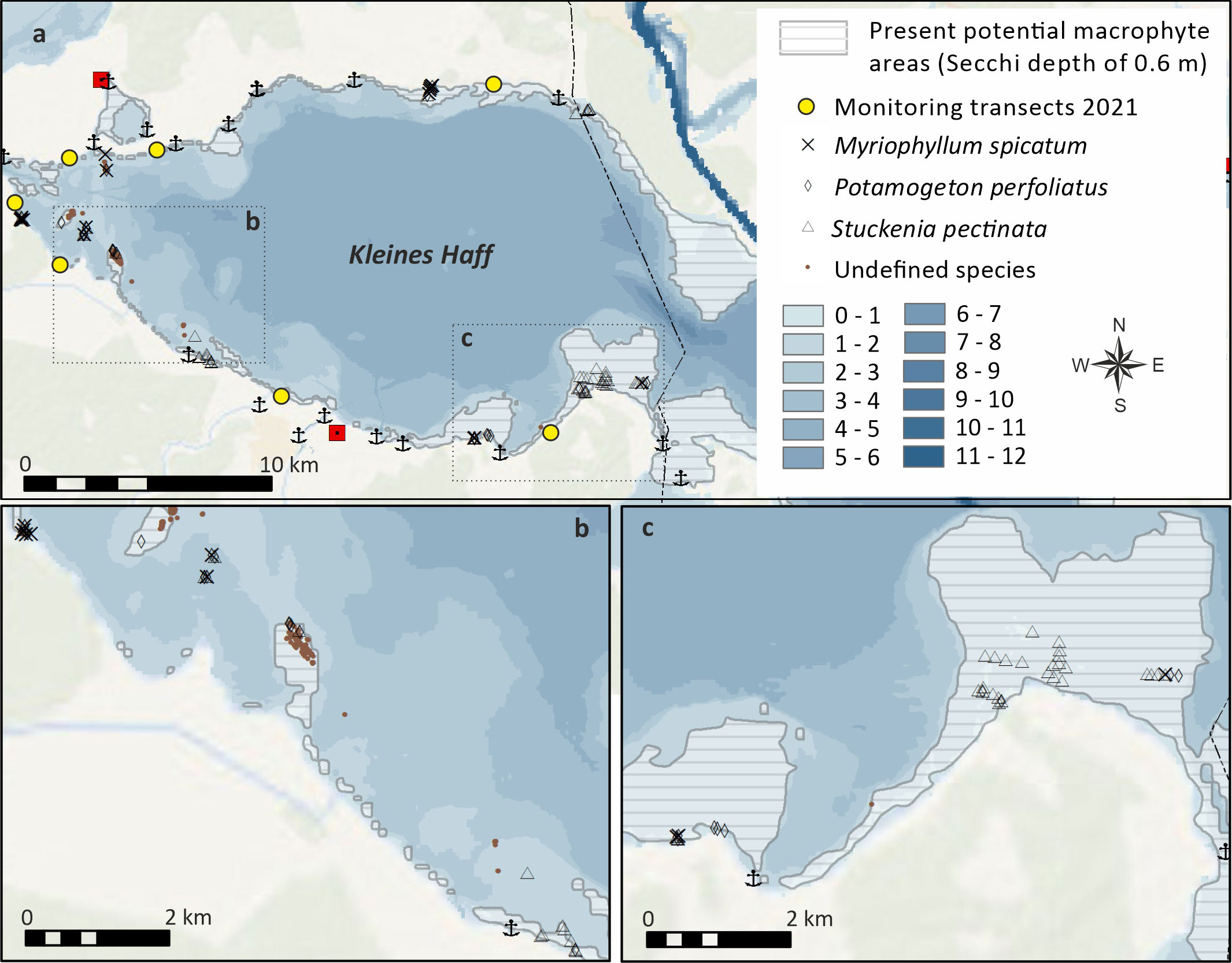
Figure 3 GIS-Maps showing coverage and species distribution today compared to the potential coverage area at the present Secchi depth of 0.6 m. (A) provides on overview and (B, C) show important areas enlarged.
Assuming that water depths down to 1.5 m potentially could be fully covered by macrophytes would result in an area of about 13% of the total areas of the Kleines Haff. Our survey data complemented with exiting WFD transect sampling data suggests a very patchy distribution and a real coverage close to 5% of the Kleines Haff surface area.
The loss of macrophytes in Baltic inner coastal waters is commonly regarded as indirect effect of eutrophication (Schiewer and Glocke, 1996). The annual riverine TN and TP loads to the lagoon increased between the 1880’s and the maximum in the 1980’s from about 14,000 t TN (1,000 t TP) to 115,000 t TN (10,500 t TP). In the early 1970’s, this increases intensified and caused strong eutrophication with increased phytoplankton concentrations, increased resuspendable organic material and subsequently declining light conditions. However, the limited macrophyte coverage 130 years ago suggests that macrophytes were either lost due to earlier eutrophication or as a result of long-lasting human impact.
3.3 Macrophyte coverage in a potential good water quality state
The question is how large would macrophytes covered areas be, compared to the situation today and in the past, assuming that good water transparency in the lagoon exists? The present water transparency threshold according to the WFD is a Secchi depth of 1.7 m in the Kleines Haff (Sagert et al., 2008). Based on model simulations, Friedland et al. (2019a) suggest 1.97 m for the Kleines Haff and 2.87 m Secchi depth for the Wielki Zalew. For a Secchi depth of 1.7 m, Middelboe and Markager (1997) provide a colonization depth for charophytes of 2.19 m and for angiosperms of 1.99 m for many Danish aquatic systems that are comparable to the Oder Lagoon. Comparing the thresholds for a good water transparency and the threshold for a good macrophyte colonization depth show an existing mismatch that requires a harmonization. It is likely that a good water transparency status of 1.7 m Secchi depth would not allow a colonization depth of above 2.4 m.
Reference state for the lagoon according to the WFD is a dominance of charophytes (Schubert et al., 2003; Selig et al., 2006). Therefore, charophytes and angiosperms represent the ecologically preferred target groups describing the good ecological status. As a consequence, we focus on the potential spatial coverage of these groups. The potential areas covered by angiosperms and charophytes are shown in Figure 4. At least 27% of the Kleines Haff areas would be covered by macrophytes in a good ecological status. Taking into account gradients between the two parts of the lagoons, with a higher transparency in the Wielki Zalew, this could result in a total macrophyte coverage of about 35%. This coverage is very close to our historic maximum coverage. Therefore, a Secchi depth of 1.7 m for the Kleines Haff represents a situation before the 1890’s and seems to be a too ambitious threshold for a good ecological status.
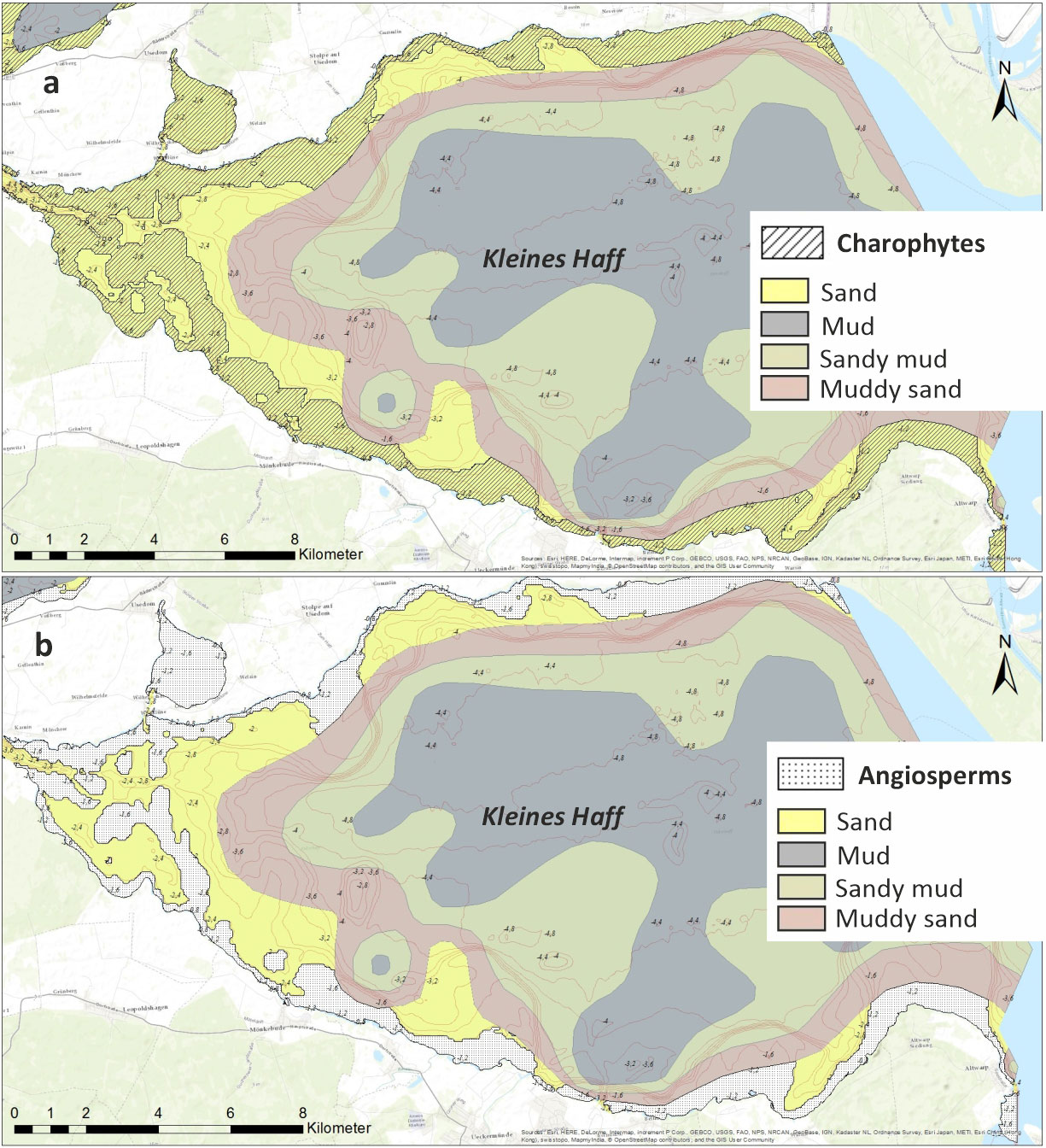
Figure 4 Potential spatial coverage of ecological target macrophytes in a potential good ecological status assuming a water transparency (Secchi depth) of 1.7 m according to Sagert et al. (2008): (A) charophytes and (B) angiosperms. Sediment distribution map after (Osadczuk and Wawrzyniak-Wydrowska, 1998).
The Secchi depths for a good ecological status suggested by Friedland et al. (2019a) is even much larger and would result a macrophyte coverage in above 50% of the lagoon. The same is true for the exiting target (> 2.4 m) describing a good ecological state according to the WFD. This is far beyond what we consider as maximum possible historic coverage of 36% and does not seem realistic, not even as reference state according to the WFD.
However, all these macrophyte coverage calculations are theoretical. It is well known that the distribution of macrophytes is not only controlled by light availability. The sediment plays an important role. Macrophytes usually prefer consolidated, stable sediments and are not able to settle on fine, muddy sediments. The sediment map (Figure 4) indicates that sandy sediments prevail near-shore and macrophyte growth in the lagoon is hardly restricted by unsuitable bottom conditions. Other important factors are exposition to wind, waves and currents (Scheffer, 1998; Yousef, 1999; Schneider, 2004). Since the lagoon is west-east oriented, it is exposed to the dominating westerly winds and frequent storms. Resulting waves, strong currents and high critical shear stress at the bottom seem to restrict the macrophyte distribution in reality, as well.
3.4 Effects of macrophytes on water quality
Guiding for this sub-chapter is one question: How relevant are macrophytes for the water quality in the lagoon? As mentioned before, the effects of macrophytes on aquatic ecosystems and especially water quality are well known and well documented (e.g. Scheffer, 1998; Horppila and Nurminen, 2003; Hilt et al., 2006; Blindow et al., 2014). Can macrophytes affect water quality in the entire lagoon, can changes in macrophyte coverage explain changes in water quality and have macrophytes to be taken into account when defining chemical water quality thresholds according to the WFD?
The model suggests that a macrophyte colonization depth of 2 m water depth would reduce the concentration of organic matter in the water column in a narrow near coast strip by more than 50% (Figure 5A). Especially sheltered shallow systems such as Lake Neuwarp and Lake Usedom are strongly affected. Macrophytes would affect even central parts of the lagoon by reducing organic matter concentration by 10%-20%. Changes in zooplankton grazing pressure (Figure 5B) are restricted to near shore areas and hardly affect central parts of the lagoon. Shading by macrophytes is limited to the coastal macrophyte covered areas (Figure 5C). The cumulative effect of the changes resulting from increased macrophyte coverage on the phytoplankton concentration in the lagoon, expressed in terms of chl.a, is shown in Figure 5D. Sheltered and semi-closed areas would face a chl.a reduction of about 10% and offshore areas of about 3%. Central parts of the lagoon are even less affected. This is especially true for the Kleines Haff. Altogether, macrophytes have effects on nearshore water quality, while open parts of the lagoon are not much affected. We cannot expect that changes in macrophyte coverage during the last 140 years affected water quality parameters in the central parts of the lagoon significantly. However, our model simulations take into account only major aspects.
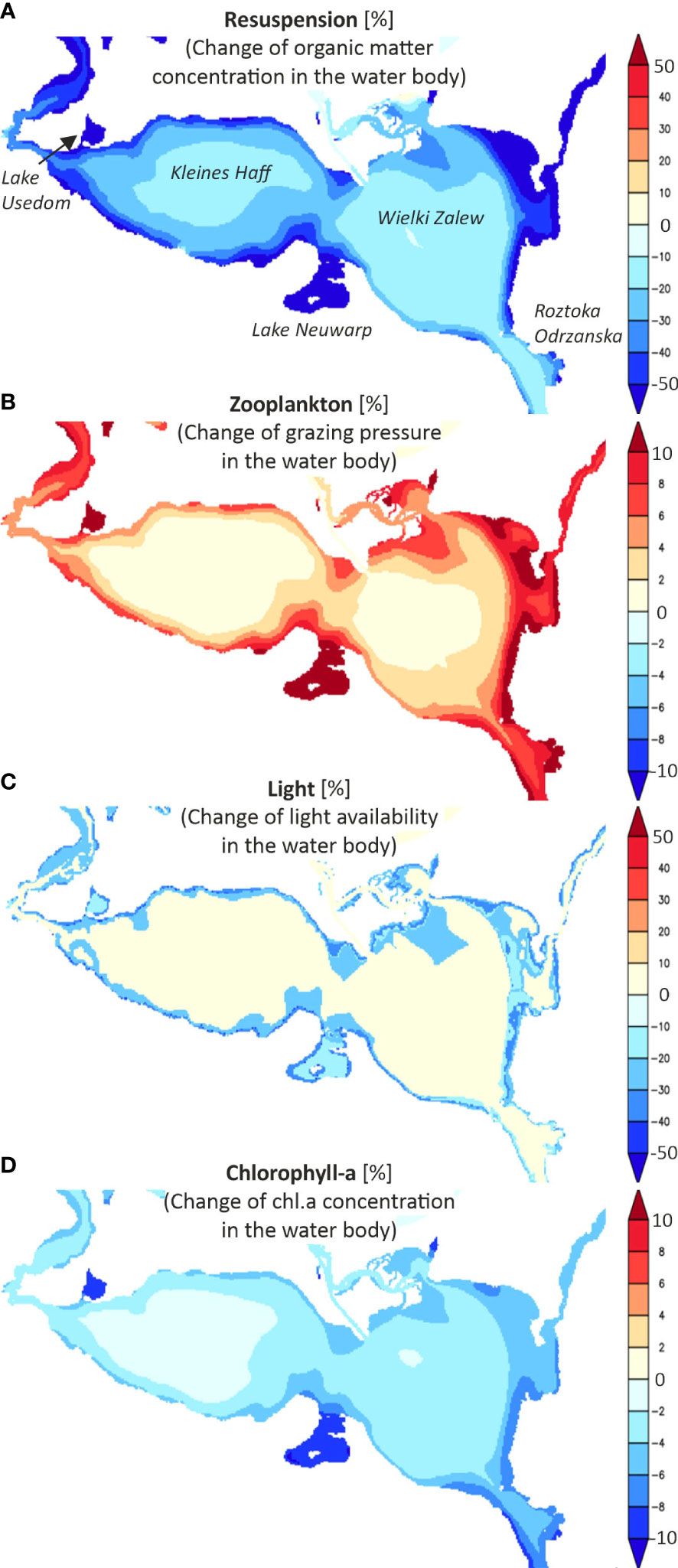
Figure 5 3D-Ecosystem model simulations of potential effects of macrophytes (colonization depth of 2 m) on ecologically relevant parameters in the lagoon: (A) resuspension, (B) zooplankton, (C) light availability at the bottom and (D) chlorophyll-concentrations in the surface layer. The simulations assume the present external nutrient loads.
Since water quality thresholds are determined based on data from central lagoon stations, an effect cannot be expected. The existing thresholds can be regarded as reliable. Another question is whether data from the central lagoon is really representative for the state of lagoon. The introduction of additional near shore stations would certainly provide a more complete picture of the state of the lagoon and is therefore recommendable.
Figure 6 summarizes the effects of macrophytes on chl.a concentrations integrated over the areas of the two parts of the lagoon. Reduced resuspension increases the light availability in the water body and favors phytoplankton while the other macrophyte effects, e.g. shading or increased zooplankton concentrations, hamper phytoplankton growth (Figures 6A, B). The combination of all effects result in a chl.a reduction of 5% in the Wielki Zalew and below 2% in the Kleines Haff. Assuming the much lower historic loads of 1880 in the model simulations result in a chl.a reduction of below 4% in the Wielki Zalew and below 1% in the Kleines Haff. The lower the loads, the lower are the effects of macrophytes on water quality. It becomes obvious, that the Wielki Zalew is and always was much more affected by macrophytes and changes in coverages than the Kleines Haff. Model results suggest that the effects of macrophytes on water quality in the entire Oder Lagoon is and always was very limited. For model based assessments within the WFD, such as the lagoon’s behavior on nutrient load increases and reductions, macrophytes can be neglected. The benefit of introducing state variables describing macrophytes in the model does not justify the effort and is not recommendable for the Oder Lagoon. Simplified approaches can be applied. In other smaller or shallower coastal waters this will certainly be different. A consequence is that the analysis of long-term changes and management perspectives for the lagoon can neglect macrophytes and focus on fundamental relationships between external loads and lagoon water quality. This is in agreement with Blindow and Meyer (2015) who mention a macrophyte containing volume of 15-20% as prerequisite for strong controlling effects in shallow lakes. Assuming the maximum colonization depth of 2.5 m in the Oder Lagoon, the macrophyte containing lagoon water volume would be close to 10% and assuming a colonization depth of 2 m the volume would be reduced to only 6-7%.
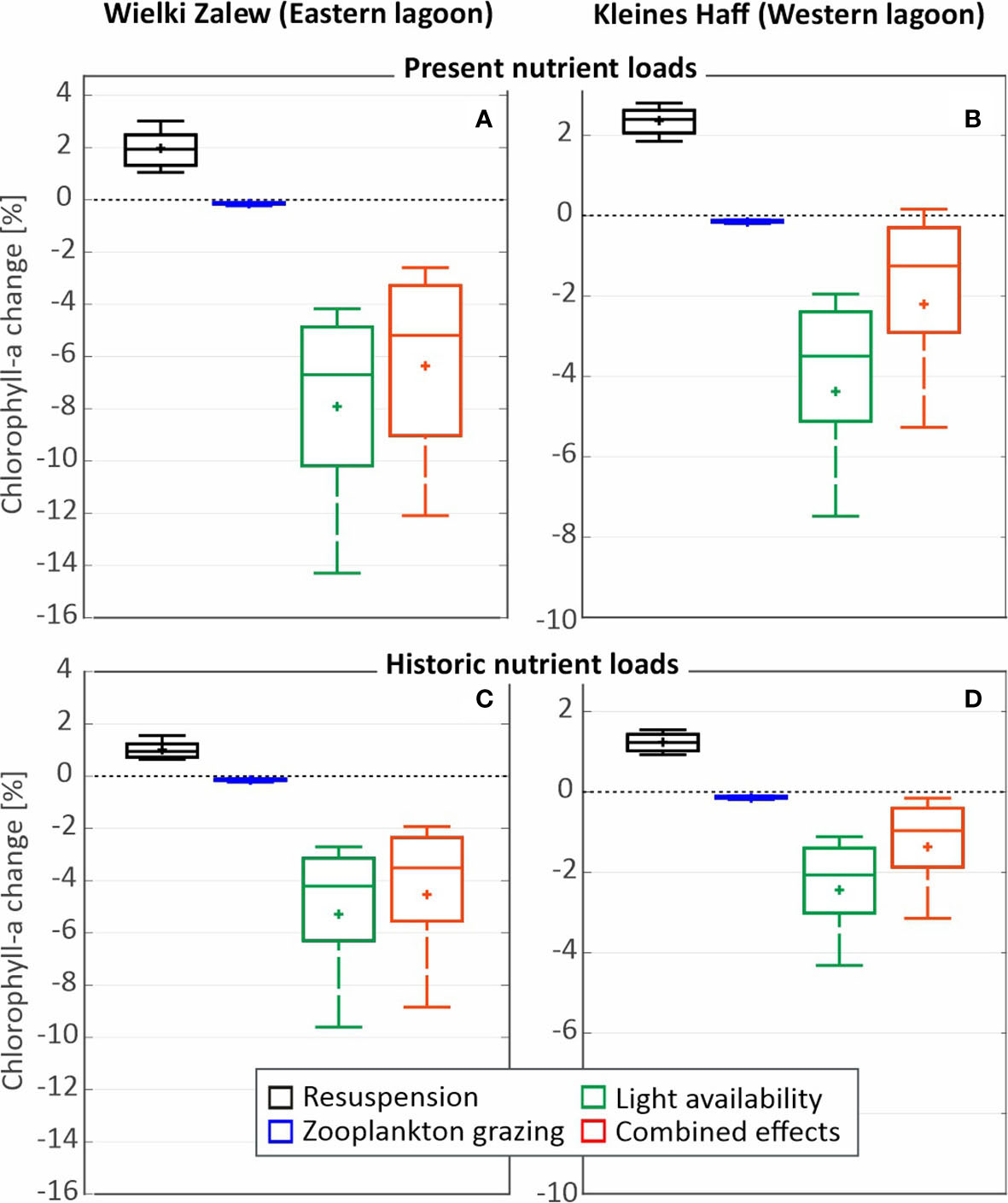
Figure 6 3D-Ecosystem model simulations of potential effects of increased macrophyte coverage (colonization depth of 2 m) and resulting reduced sediment resuspension, increased zooplankton grazing and reduced light availability at the bottom on chlorophyll a-concentrations in the two parts of the Oder Lagoon, the German Kleines Haff (A) and the Polish Wielki Zalew (B). (C, D) show similar results, but assume historical external nutrient loads around 1880.
3.5 Relationships between eutrophication controlling factors
Guiding question is whether eutrophication in the lagoon already took place centuries ago or if the lagoon is even a naturally eutrophied system. The latter would explain the relatively low coverage with macrophytes centuries ago. The old comprehensive OECD study of world-wide lakes by Vollenweider (1976) and later up-dates by Jones and Lee (1986) can give an insight into major relationships between nutrient loads and basic water quality parameters. Lee and Jones (1981) confirm the transferability of the relationships to estuaries and Reynolds (1992) introduce light as limiting factor. This allows answering the additional question, whether the lagoon can be regarded as a system with a behavior that is typical for lagoons and lakes.
Figure 7A shows that both parts of the lagoon have and had for the last 30 years a molar N/P close to 7/1 (expressed by weight). This indicates that P is not the limiting element for primary production in the lagoon, but that N may play an important role in controlling primary productivity. However, in comparison to the OECD lakes, the lagoon shows high concentrations for both nutrients in the water. The relationship between P and chl.a can be regarded as typical, as well (Figure 7B). Here too, the concentrations for both parameters are very high when compared to the OECD lakes. This is true for the situation today and 30 years ago. Water transparency in both parts of the lagoon is and was very low compared to the investigated OECD lakes. In the Wielki Zalew, the relationship between water transparency and average chl.a-concentrations is comparable to the lakes (Figure 7C). In contrast, the Kleines Haff shows a relatively low transparency at the given chl.a-concentration. Due to its shallowness, and longer retention time, sediment resuspension is more prominent in the Kleines Haff. The important role of sediment resuspension on water transparency is confirmed by frequent Secchi depths below 1 m even during winter seasons.
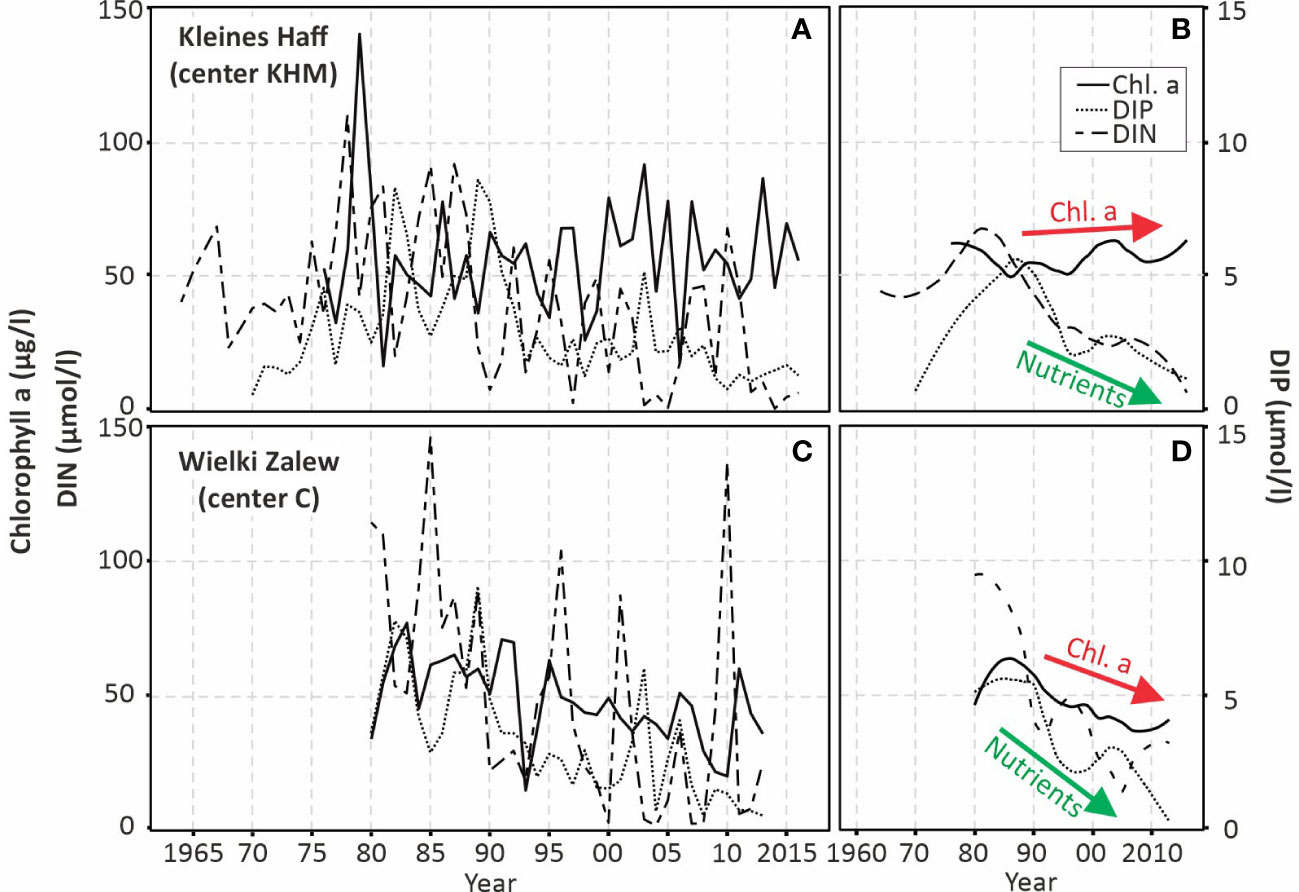
Figure 7 Chlorophyll a, dissolved inorganic nitrogen (DIN) and dissolved inorganic phosphorus (DIP) concentrations (averages April-August) in the two partsof the Oder Lagoon, the German Kleines Haff (A, B) and the Polish Wielki Zalew (C, D), during the last decades. (B, D) show smoothened trends.
The very high nutrient concentrations, the tendency that N is the element with the shorter availability and the low water transparency indicates that light is the limiting factor for primary production in both parts of the lagoon, but that light limitation in the Kleines Haff is even stronger. The lagoon shows a situation beyond P-limitation as described by Reynolds (1992).
The OECD study of world-wide lakes by Vollenweider (1976) provides a relationship between external P-loads and the sensitiveness of a lake towards eutrophication. The shallower a system and the higher the water residence time, the higher the sensitiveness towards eutrophication and the lower the acceptable external P-load (Figure 7D). The P-loads to the entire Oder Lagoon and to each part of the lagoon is today and was 40 years ago above the acceptable loads for a non-eutrophied system. Compared to the Wielki Zalew, the higher water residence time and the slightly lower average depths makes the Kleines Haff more sensitive towards eutrophication. It is known that internal eutrophication, the release of P from the sediment during hypoxic conditions, in some years can play a role (Bangel et al., 2002), however a systematic study is still lacking.
The main source of external P is the Oder river, draining into the Wielki Zalew. As a consequence, the Kleines Haff receives significantly less external P compared to the Wielki Zalew. In both parts of the lagoon, the P-loads are far above the acceptable level and keep the system in a eutrophic state. Even if we assume that the maximum allowable P-input (MAI), required for a good ecological status of the Baltic Sea according to Helcom, would be reached in future this would not cause a change in the lagoon. The MAI is still far above the critical load and would keep all parts of the lagoon in a eutrophic state. The historic P-loads reflect the situation around the 1880’s, about 140 years ago (Gadegast et al., 2012; Hirt et al., 2014; Gadegast and Venohr, 2015). At that time, we can assume emissions into surface waters of the Oder catchment below 6 kg N/ha and around 0.1 kg P/ha. Very likely, the loads around the 1880’s were not significantly higher compared to earlier centuries. As a consequence, we can assume that the P-loads were above the critical level and kept the lagoon in a eutrophic state already for centuries. This allows to address the lagoon as a naturally eutrophied system, with limited submerse macrophyte coverage.
3.6 Long-term development of water quality
For the last decades, the question how water quality in the lagoon is and was affected by external nutrient load reductions, can be assessed in more detail. The external nutrient loads had a maximum in the 1980’s of 115,000 t TN (10,500 t TP) and strongly declined to 56,750 t TN (2,800 t TP) in 2010-2014 (Friedland et al., 2019a). Figure 8 compiles all existing data for N, P and chl.a from the central stations in Kleines Haff and Wielki Zalew. The dissolved inorganic N concentrations in the Wielki Zalew partly correspond to the Oder/Odra river nitrogen loads. For example, the flood year 2010 discharged about 90,000 t N to the lagoon and increased the DIN concentration to about 140 µmol/l. However, the strong variability between the years cannot be explained by external annual N loads (Figure 8C). The N concentrations in the Kleines Haff show a less strong inter-annual variability and do not follow the pattern in the Wielki Zalew (Figure 8A).
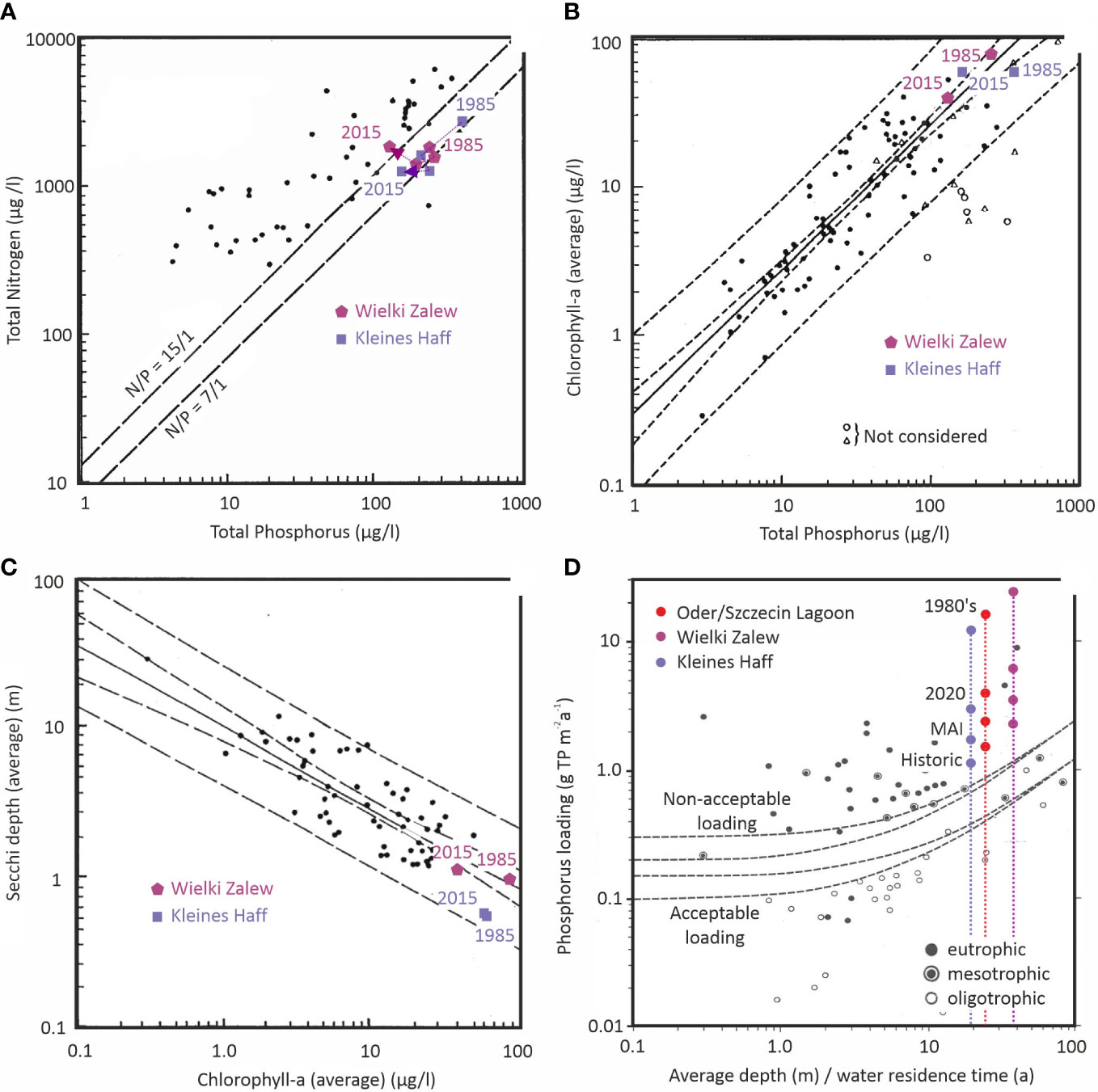
Figure 8 Functional relationships between nutrient loads and water quality parameters in Oder/Szczecin Lagoon and its two parts Wielki Zalew and Kleines Haff in the context of the OECD worldwide lake study (Vollenweider, 1976). (A) Total Nitrogen versus Total Phosphorus concentrations; (B) Chlorophyll-a versus Total Phosphorus concentrations; (C) Secchi depth versus Chlorophyll-a concentrations and (D) external P loading versus average depth divided by residence time.
The annual N and P concentrations in both parts of the lagoon do not show a systematic relationship to each other and the P concentrations in the lagoon are not clearly related to external P loads. Altogether the inter-annual variability of P-concentrations in Kleines Haff is stronger compared to Wielki Zalew. Schernewski et al. (2011) suggest that P-peaks in 1989 are resulting from temporal hypoxia and the release of iron-bound P from the sediments. This could be an explanation for the P-peak in the hot year 2003, as well. Bachor (2005) estimated an N content of 14,200 t and a P content of 2,400 t in the upper sediment layer (0-5 cm) for the Kleines Haff. In Wielki Zalew, P release from the sediment under temporary hypoxic conditions might explain the P peak in 2003, as well. This anoxic P-release is a process often observed in shallow aquatic systems (Boström and Pettersson, 1982; Jensen and Andersen, 1992). The Oder/Odra river influence, shipping induced turbulence and a higher water exchange with the Baltic Sea are reasonable explanations that internal eutrophication is less obvious in the Wielki Zalew data.
The chl.a-concentrations show a strong inter-annual variability in both parts of the lagoon. Especially in the Kleines Haff, the data suggests an opposite behavior of N and chl.a-concentrations and in the last decade, the N concentrations in both parts of the lagoon are in some years close to zero.
The aggregated annual data is not suitable to analyze processes in detail. For the Wielki Zalew, a higher temporal resolution of the data could possibly prove a relationship between especially external N loads and concentrations in the lagoon water. However, what we can conclude is that the Kleines Haff and the Wielki Zalew behave differently. While the first seems strongly influenced by internal lagoon processes, the latter is much stronger driven by external Oder/Odra river loads.
The smoothened data of the last 30-40 years for both parts of the lagoon indicate a strong decline of nutrient concentrations in the water that reflect the decline in external nutrient loads (Figures 8B, D). In Wielki Zalew, a slight decline of chl.a is visible during the last 30 years, while chl.a-concentrations in Kleines Haff remain stable.
Most important for macrophytes are changes in water transparency. From the 1990’s, summerly water transparency has slightly increased in the Kleines Haff from 0.5m to 0.6 m and in Wielki Zalew from 0.85 m to 1.1 m (Figure 9). However, the reliability of these trends is limited by the strong data variability and the non-homogeneous water transparency developments during other seasons. Reasons for different water transparencies between the two parts of the lagoon could be the Oder/Odra river water, which has a higher transparency, the lower water depth of the Kleines Haff, that favours sediment resuspension, and the availability of resuspendable organic material. The artificially deepened and regularly dredged shipping channel in the Wieki Zalew additionally serves as trap for organic matter (Minning, 2004) and in a longer perspective reduces the amount of available organic material. On the other hand, ship induced turbulence may even increase resuspension, at least locally.
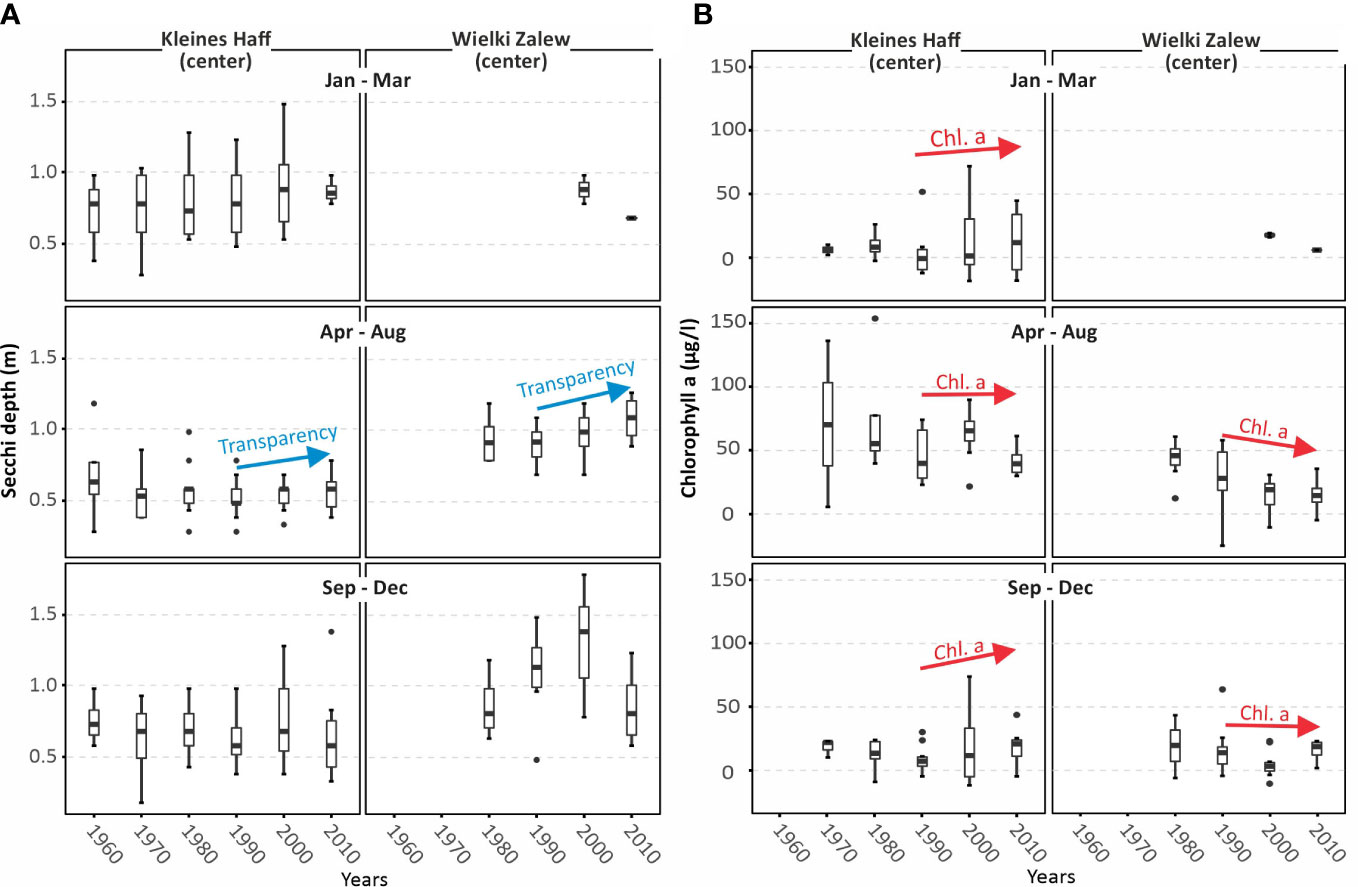
Figure 9 Seasonal data on water transparency (Secchi depth) (A) and chlorophyll a (B) in the two parts of the Oder Lagoon, the German Kleines Haff and the Polish Wielki Zalew, averaged over decades and covering the last 60 years.
In late winter and autumn the chl.a concentrations in the Kleines Haff seem to have increased during the last three decades. This could result from a climate warming. Higher temperatures in autumn and in winter, with less ice coverage, potentially enable a higher primary production during these seasons.
Obviously, the Kleines Haff is still light limited and changes in nutrient concentrations do not affect primary productions. Wielki Zalew shows a tendency to shift from a light limited towards a N controlled system. However, the chl.a-concentrations are still very high and one can hardly speak of a lasting N limitation. Shallow, turbid systems, such as the lagoon, enable a fast cycling of nutrients within days. Further, cyanobacteria are dominating in summer and have the potential to make atmospheric N accessible. A prove of N-fixation by cyanobacteria, that would indicate a real N shortage, is still lacking for the Szczecin Lagoon. This is very different in comparable lagoons, such as the Curonian Lagoon (Zilius et al., 2021).
4 Discussion
What are the ecological perspectives for the lagoon and to what extent can management measures improve its ecological state? Further, why is the macrophyte coverage today smaller than it potentially could be? Does this result from a hysteresis effect (Scheffer, 1998; Blindow and Meyer, 2015; Friedland et al., 2019a)?
The Szczecin Lagoon can be regarded as common with respect to the relationships between water quality parameters. The data does not show strong shifts in water quality during the intensified eutrophication period in the 20th century until the mid 1980’s. The data further indicates that the system was eutrophic already for centuries and can be regarded as a natural eutrophic system that was never dominated by macrophytes. The present external nutrient loads still keep the system in a eutrophic state.
The data of the last 30 years shows reduced external loads but, different to the expectations, only a limited or no reduction of chl.a-concentrations in the water. This indicates no or only a limited decline of phytoplankton biomass. Despite all efforts, the loads and subsequently the nutrient and chl.a-concentrations are still very high. Phytoplankton in the lagoon is controlled by light or partly by N. A light or N controlled system does not allow an eutrophication management via nutrient load reductions, because N shortages can be compensated by internal processes. The external P loads cannot be reduced to a level that the system becomes P-limited. The existing HELCOM maximum allowable inputs (MAI) for N and P are reasonable from a Baltic Sea protection perspective, but even in case they will be reached, they will leave the lagoon in a highly eutrophic state. The same is true if the nutrient concentrations in the Oder/Odra river would reach a level that reflect a good ecological status according to the WFD. The resulting P-loads with the river would still be too high for the lagoon. The new program of measures (IKSO, 2022b) for reducing nutrient loads in the river basin is, from a lagoon perspective, not sufficient for improving water quality in the lagoon significantly and hardly can be. However, the investigation of a large number of Dutch lakes (Portielje and van der Molen, 1999) shows that the relationship between external loads can vary in a wide range and depends on system specific properties and processes. In a large system with bays and differently exposed areas, like the Oder lagoon, local water quality improvements may be possible as a result of local changes in macrophyte coverage.
The lagoon serves as a sink for external nutrients and reduces the loads to the Baltic Sea. This function is usually neglected, for example, in calculation of the maximum allowable loads to the Baltic Sea. A consequence is that the Baltic Sea, in fact, receives less nutrients than assumed by HELCOM (2013). Wielgat and Witek (2004) calculate an annual nitrogen retention of about 20% of the N-loads and 17% of the P-loads in the lagoon. Burial is the only sink for P, while denitrification and burial each contribute about 10% to the N-retention. The important role of denitrification in the lagoon is confirmed by data, as well (Voss et al., 2010). All these processes are quantitatively relevant, have a seasonality and affect the lagoon’s sink function. In future, this should to be taken into account for calculating realistic, seasonal loads to the Baltic Sea.
For deriving water quality thresholds according to the WFD in Germany, values of the ‘reference’ state around 1880 were increased by 50%. The reference state was based on model simulations (Schernewski et al., 2015). Target is a good ecological status better than the threshold. Friedland et al. (2019a) follow the same approach to derive thresholds for both parts of the lagoon, namely 14.3 µg/l chl.a in the Kleines Haff and 17.3 µg/l chl.a in the Wielki Zalew. The present chl.a-concentrations in the Kleines Haff are about 4 times and in Wielki Zalew 2 times higher. It is questionable if the approach for deriving thresholds is suitable for a non-nutrient limited system, but more important is that these thresholds are not harmonized with approaches determining concentrations and loads in rivers. The nutrient concentration thresholds in the lagoon have to be related to the loads resulting from a good status in the river and would have to be 1.5-2 time higher compared to the suggested present values. It seems that in general, a new approach for deriving ecological targets and thresholds is required that better considers the relationships and dependencies between linked aquatic systems.
However, important for the Oder Lagoon is that by deriving thresholds based on the common technical implementation guidelines of the WFD, that are applied throughout Europe, the resulting chl.a-concentrations would describe a eutrophic situation. Usually, the good ecological status is derived from reference conditions that describe the system in a largely natural state. In Germany the reference period are the years around 1880 (Schernewski et al., 2015). In a naturally eutrophic system the ‘good ecological status’ according to WFD is a phytoplankton dominated, turbid, eutrophic status. Similar problems occur with respect to the water transparency thresholds. The thresholds are 1.7 m (Secchi depth, summer month) according to Sagert et al. (2008) and the modified values of 2.87 m (Wielki Zalew) and 1.97 m (Kleines Haff) by Friedland et al. (2019a). According to Middelboe and Markager (1997), a Secchi depth of 1.7 m would allow colonization depth of charophytes and angiosperms of above 2 m water depth and describe the situation observed by Brandt (1896) 130 years ago. A situation similar to what was observed 130 years ago is not a realistic target. The target values by Friedland et al. (2019a) and the present WFD macrophyte colonization depth thresholds for a good ecological status based on Domin et al. (2004) are even more ambitious. They reflect a situation that, very likely, never occurred in the lagoon, at least not in the Kleines Haff. In the Kleines Haff, a colonization depth of about 2 m would be a realistic target. It is obvious that present approaches for deriving WFD water quality threshold values in the lagoon show deficits. However, Chambers and Kalff (1985) show that the colonization depth of species very much depends on several other parameters. Therefore, reliable thresholds are not easy to obtain and a transfer of approaches from shallow coastal water system to another can be misleading. Further, the colonization depth alone seems an incomplete indicator for the ecological state because it does not provide information on the spatial coverage and stock densities of species. Further, the colonization is species dependent.
In German, in 2015, over 60% of all assessed coastal waters were considered to be in a poor or bad and none in a good or high status (BMUB/UBA, 2016). This results from an insufficient status of macroalgae, angiosperms as well as other aquatic flora. In comparison, less than 40% of all lakes were assessed as poor or bad and only less than 20% of the German transitional water (only occurring in the North Sea) were considered as poor (BMUB/UBA, 2016). This is a hint that natural eutrophic systems, like the Oder lagoon, which is defined as a coastal water, are no exception. It is further a hint that the definition of thresholds for a good ecological status, despite following the same approach, may require a reconsideration. This is at least true if the good ecological status is the politically defined target that needs to reached within the next years.
The model, its validation and a critical discussion of the performance is provided by Neumann (2000); Schernewski et al. (2019) and Friedland et al. (2019a; 2019b). The biogeochemical model ERGOM does not explicitly represent macrophytes in form of state variables and wave induced resuspension is only considered in a simplified way. Therefore, the model simulations can hardly be regarded as reliable in itself, but the relative changes between the scenarios indicated by the model can provide some insights. Since monitoring takes place in central parts of the lagoon and data-based thresholds and assessments represent the conditions in these central parts, the existing model is generally suitable for simulating hydro-chemical and chlorophyll-a concentrations in past and future as well as quality thresholds. Further, in the Oder lagoon, the influence of macrophytes on chemical parameters and phytoplankton in the open lagoon seems limited. Against this background and for the purpose of overall lagoon investigations, it does not seem necessary to introduce macrophytes in the model in form of additional state variables. The present ERGOM model does not calculate water transparency in the desired quality, but requires lagoon specific adjustment factors to properly represent Secchi-depth. It seems that water transparency is only partly controlled by phytoplankton concentrations and water color. Short-term sediment resuspension seems to strongly affect water transparency in the lagoon and that these processes are still not represented in the model in a sufficient quality. Therefore, improvements are still required to increase the model’s practical relevance and applicability within the WFD. Further, a relationship between macrophyte colonization depth and water transparency as well as other controlling factors, such as sediment erosion, needs to be established. Detailed studies on the quantitative role of temporal internal P-release from the sediment require a higher spatial, especially horizontal, resolution of the hydrodynamic model.
However, if the lagoon cannot be transferred into a non-eutrophic system by external nutrient load reductions and has to be regarded as a naturally eutrophic system, what are the management options to improve its ecological state? A large variety of measures exists that can potentially be implemented in the lagoon to improve water quality and reduce eutrophication. For example, mechanical measures such as groin systems for reducing sediment resuspension, sediment dredging and dumping on land or sediment capping with clay to prohibit nutrient release from sediments (Oncken et al., 2022). The precipitation of P is a potential chemical measure. Biological measures are selective fisheries to favor piscivorous fish, macroalgae cultivation or the enlargement of mussel beds. The most promising measure, Dreissena mussel farming has been assessed in depth (Schernewski et al., 2012; Friedland et al., 2019b; Schernewski et al., 2019). Mussel farming can lead to local improvements, but none of these measures can realistically improve water quality in a lagoon of this size. Latest, this would be prohibited by legal and financial considerations. Commercial shipping in the lagoon is largely restricted to the dredged channel across the Wielki Zalew. With the recent channel deepening the intensity will very likely increase, but because of the large spatial distance to macrophyte areas, negative consequences seem unlikely. Increasing sport boat traffic and anchoring in shallow areas might become a problem in future.
The potential historic maximum macrophyte coverage is calculated to be 35% of the lagoons surface area. Presently, the patchy macrophyte coverage is only about 12% of the lagoon area. This suggests that the lagoon was never a macrophyte dominated, clear water system. However, it does not mean that macrophytes do not play an important role in the lagoon’s ecology. One aspect that has been neglected in our study, is the stepwise deepening of the shipping channel during the last century. In 1880 the first artificial channel had a depth of 5.7 m, was deepened to 5.7 m in 1939, to 10.5 m in 1984 and presently deepened to 12.5 m. This caused changes in flow cross-sections, a spatial re-allocation of the water discharge to the Baltic Sea between the three outlets and slightly changed transport pattern in the lagoon. The effects on the water residence time in the lagoon are negligible. However, the increasing cross-section favours short-termed intrusions of Baltic Sea water, with a salinity of about 6 psu, into the lagoon. Spatial data analysis covering the 1980’ and 1990’s show, that these intrusions into the lagoon are only local and temporary, close to the shipping channel (Bangel et al., 2004). They do not affect the salinity of the lagoon (presently 1-2 psu) significantly. However, in future ongoing sea-level rise may have consequences for the water exchange with the Baltic Sea. Further, it may increase shallow areas in size and increase the colonization area for macrophytes, especially for reed and bulrush. This may increase their relevance for the lagoon’s ecology.
Today the existing coverage with submerse macrophytes is below the potential coverage area considering the existing water transparency. It is known from many lakes that the artificial colonization with macrophytes can be successful and beneficial for the ecological state (Scheffer et al., 1992; James and Barko, 1994; Hilt et al., 2006; Hussner et al., 2010), since macrophytes are a quality element in itself in the WFD. Is it necessary to re-establish macrophytes stocks or to introduce species that are not present anymore? Recent studies by Nowak et al. (2018), Steinhardt and Selig (2008) and Blindow et al. (2016) document that germinable diaspores of many species are present in the sediments of all observed German Baltic coastal water. Nowak et al. (2018) conclude that diaspores have the potential to restore macrophyte communities. This can happen even decades after the stocks were lost. Recent field data of the Wielki Zalew already indicated Potamogeton perfoliatus and Myriophyllum spicatum at a depth of 2–2.2 m (Wozniczka, pers. com.) and the re-occurance of charophytes is reported by Brzeska et al. (2015). Charophytes were recently observed in the Kleines Haff, as well. The available data does not allow to speak of an improved ecological state, but indicates that macrophytes recover naturally as soon as the conditions are suitable. They do not require supporting artificial measures. Frequent sediment resuspension and storm induced sediment relocations should prevent a permanent burial of germinable diaspores and maintain a natural re-settlement potential. The investigation of organic sediment cores might provide new insights. The ongoing macrophyte monitoring within the WFD is providing more and more data. In future, a more detailed study on species level would make sense.
Friedland et al. (2019a) consider that hysteresis effects might hamper the re-settlement in the lagoon, but we do not see any indication for that. The lagoon seems to adapt continuously to changes, because it was never an oligotrophic, clear water system that performed a sudden regime shift into an eutrophic state. Therefore, the Oder Lagoon is very different to neighboring systems that are subject to much lower external loads (Blindow and Meyer, 2015). Further, Janssen et al. (2014) show that large aquatic systems generally behave differently, largely because of existing spatial heterogeneities.
Already in medieval times, the lagoon was known for its diverse and abundant fish fauna. Already in 1495, the intensive fisheries became regulated. Different types of fishing boats were used, for example the common up to 22 m long Zeesen boats. Already in the 16th century, ground touching fisheries with about 80 large Zeesen boats (Rudolph, 1969, Fircks, 2011) caused high pressure on fish stocks. Intensive fisheries already centuries ago indicates a productive, eutrophic system. It can be assumed, that around 1890 ground touching fisheries together with the common near shore gillnet and pot fisheries had caused large scale mechanical destructions on macrophyte stocks. Today, the mechanical destruction of underwater habitats by human activities, such as fisheries, dredging or sport boating, is ongoing, but more locally. A strict avoidance of mechanical disturbances down to a water depth of 2.5 would certainly be beneficial for a macrophyte re-colonization. The long lasting overexploitation of piscivorous fish might have amplified eutrophication, as suggested by Schindler (2006). Even today the fishing pressure on valuable and marketable piscivorous fish is high and several species are extinct from the lagoon. A systematic improvement of piscivorous fish stocks may be a supporting measure to reduce eutrophication in the lagoon.
An ongoing process that may increase water transparency and support the macrophyte recovery naturally is the recent invasion of the lagoon by Quagga mussels (Dreissena bugensis). First recorded in 2014 in the Wielki Zalew, it is still unclear if it replaces the existing Zebra mussel (Wawrzyniak-Wydrowska et al., 2018) and will affect water transparency in the lagoon significantly. In the Kleines Haff, the Quagga mussel already became the dominant mussel species. Potentially, the Quagga mussel is an efficient filter feeder with a high potential ability to clean water and it is larger and growths faster compared to the Zebra mussel (Baldwin et al., 2002; Rudstam and Gandino, 2020).
Altogether, we need to state that the possibilities to improve the water quality of the lagoon are very limited. However, improvements of the ecological state, especially with respect to macrophytes, are possible and should be implemented.
5 Conclusions
There is still a need for a harmonization of ecological targets between rivers, inner and outer coastal waters and the sea that fully recognizes the dependencies. Existing competing policies, in the Baltic the EU Water Framework Directive and the Baltic Sea Action Plan cause contradictions.
A harmonization of indicator thresholds describing the good ecological status indicator is needed, despite the existing EU Water Framework Directive. Indicator thresholds are derived based on different approaches that do not sufficiently reflect dependencies between parameters e.g. between water transparency and macrophyte colonization depth for good ecological state target species.
We need to accept that an eutrophic state can be the natural state of a system and that nutrient load reductions as common management approaches may remain insufficient. Consequence is that we need to reconsider ecological target states of ecosystems.
A further exploration of internal measures in coastal waters is needed, especially the cost-effectiveness and societal acceptance needs to be addressed.
Data availability statement
The raw data supporting the conclusions of this article will be made available by the authors, without undue reservation.
Author contributions
GS developed the article concept, took care of most data analyses and did most of the article writing. RF was responsible for the model simulations, visualizations and documentation. SP did the GIS and the literature analysis and contributed to the writing. MB and SD were involved in the field studies and commented the paper. MW contributed data, data interpretations and commented the paper. All authors contributed to the article and approved the submitted version.
Funding
The work was supported by project BONUS BALTCOAST (03F0717A) and partly German Environment Agency (UBA) project “The Baltic Sea Action Plan - Modelling of Water Quality Indicators” (3720252020). BONUS BALTCOAST project has received funding from BONUS (Art. 185), funded jointly by the EU and Baltic Sea national funding institutions. Supercomputing power was provided by HLRN (North-German Supercomputing Alliance).
Acknowledgments
We thank Dr. Miriam von Thenen for comments and critical remarks on the manuscript.
Conflict of interest
The authors declare that the research was conducted in the absence of any commercial or financial relationships that could be construed as a potential conflict of interest.
Publisher’s note
All claims expressed in this article are solely those of the authors and do not necessarily represent those of their affiliated organizations, or those of the publisher, the editors and the reviewers. Any product that may be evaluated in this article, or claim that may be made by its manufacturer, is not guaranteed or endorsed by the publisher.
References
Bachor A. (2005). Nährstoff- und schwermetallbilanzen der küstengewässer mecklenburg-vorpommerns unter besonderer berücksichtigung ihrer sedimente (Mecklenburg-Vorpommern: Schriftenreihe des Landesamtes für Umwelt, Naturschutz und Geologie).
Baldwin B. S., Mayer M. S., Dayton J., Pau N., Mendilla J., Sullivan M., et al. (2002). Comparative growth and feeding in zebra and quagga mussels (Dreissena polymorpha and dreissena bugensis): implications for north American lakes. Can. J. Fisheries Aquat. Sci. 59 (4), 680–694. doi: 10.1139/f02-043
Balls H., Moss B., Irvine K. (1989). The loss of submerged plants with eutrophication i. experimental design, water chemistry, aquatic plant and phytoplankton biomass in experiments carried out in ponds in the Norfolk broadland. Freshw. Biol. 22 (1), 71–87. doi: 10.1111/j.1365-2427.1989.tb01085.x
Bangel H., Schernewski G., Bachor A., Landsberg-Uczciwek M. (2004). Spatial pattern and long-term development of water quality in the Oder estuary. Mar. Sci. Rep. 57, 17–65.
Bangel H., Schernewski G., Wielgat M. (2002). Spatial, seasonal and long-term changes of phosphorus concentrations in the oder estuary. Deutsche Gesellschaft für Limnologie (DGL) - Tagungsbericht 2001 (Kiel) Tutzing 2002, 466–470.
Barko J. W., James W. F. (1998). Effects of submerged aquatic macrophytes on nutrient dynamics, sedimentation, and resuspension (New York: Springer).doi: 10.1007/978-1-4612-0695-8_10.pdf
BLANO (2014) Harmonisierte hintergrund- und orientierungswerte für nährstoffe und chlorophyll-a in den deutschen küstengewässern der ostsee sowie zielfrachten und zielkonzentrationen für die einträge über die gewässer. Available at: https://www.meeresschutz.info/sonstige-berichte.html.
Blindow I., Dahlke S., Dewart A., Flügge S., Hendreschke M., Kerkow A., et al. (2016). Long-term and interannual changes of submerged macrophytes and their associated diaspore reservoir in a shallow southern Baltic Sea bay: influence of eutrophication and climate. Hydrobiologia 778, 121–136. doi: 10.1007/s10750-016-2655-4
Blindow I., Hargeby A., Hilt S. (2014). Facilitation of clear-water conditions in shallow lakes by macrophytes: differences between charophyte and angiosperm dominance. Hydrobiologia 737, 99–110. doi: 10.1007/s10750-013-1687-2
Blindow I., Meyer J. (2015). Submerse makrophyten während eutrophierung und re-mesotrophierung – ein vergleich von inneren und äußeren boddengewässern. Rostocker Meeresbiologische Beiträge 25, 105–118.
BMUB/UBA (2016). Water framework directive – the status of German waters 2015 (Bonn, Dessau: Umweltbundesamt). Available at: https://www.gewaesser-bewertung.de/files/wrrl_englische_version_dez_2016.pdf.
Boström B., Pettersson K. (1982). Different patterns of phosphorus release from lake sediments in laboratory experiments. Hydrobiologia 91, 415–429. doi: 10.1007/PL00020032
Brandt K. (1896). Wissenschaftliche meeresuntersuchungen Vol. 2 (Kiel und Leipzig: Kommission zur wissenschaftlichen Untersuchung der Deutschen Meere in Kiel und der Biologischen Anstalt auf Helgoland).
Bruggeman J., Bolding K. (2014). A general framework for aquatic biogeochemical models. Environ. Model. Software 61, 249–265. doi: 10.1016/j.envsoft.2014.04.002
Brzeska P., Wozniczka A., Pelechaty M., Blindow I. (2015). New records of chara connivens p. salzmann ex a. Braun 1835-an extremely rare and protected species in polish brackish waters. Acta Soc Bot. Poloniae 84, 143–146. doi: 10.5586/asbp.2015.010
Burchard H., Bolding K. (2002). GETM–a general estuarine transport model. scientific documentation. technical report EUR 20253 EN (Brussels: European Commission).
Carpenter S. R., Lodge D. M. (1985). Effects of submersed macrophytes on ecosystem processes. Aquat. Bot. 26, 341–370. doi: 10.1016/0304-3770(86)90031-8
Chambers P. A., Kalff J. (1985). Depth distribution and biomass of submersed aquatic macrophyte communities in relation to secchi depth. Can. J. Fisheries Aquat. Sci. 42 (4), 701–709. doi: 10.1139/f85-090
Domin A., Schubert H., Krause H. C., Schiewer U. (2004). Modelling of pristine depth limits for macrophyte growth in the southern Baltic Sea. Hydrobiologia 514, 29–39. doi: 10.1023/B:hydr.0000018204.47081.ae
Dumke A. (2001). Substratkartierungen im kleinen stettiner haff. Jahresheft Des. Vereins Fisch und Umwelt Mecklenburg-Vorpommern e.V. 2001, 61–70.
Fircks Jv (2011). “Der zeesenkahn vom stettiner haff: bericht über die rekonstruktion,” in Deutsches schiffahrtsarchiv, vol. 34, 87–104. Available at: https://nbn-resolving.org/urn:nbn:de:0168-ssoar-65982-0.
Friedland R., Buer A. L., Dahlke S., Schernewski G. (2019b). Spatial effects of different zebra mussel farming strategies in an eutrophic Baltic lagoon. Front. Environ. Sci. 6. doi: 10.3389/fenvs.2018.00158
Friedland R., Neumann T., Schernewski G. (2012). Climate change and the Baltic Sea action plan: model simulations on the future of the western Baltic Sea. J. Mar. Syst. 105, 175–186. doi: 10.1016/j.jmarsys.2012.08.002
Friedland R., Schernewski G., Gräwe U., Greipsland I., Palazzo D., Pastuszak M. (2019a). Managing eutrophication in the szczecin (Oder) lagoon-development, present state and future perspectives. Front. Mar. Sci. 5. doi: 10.3389/fmars.2018.00521
Gadegast M., Hirt U., Opitz D., Venohr M. (2012). Modelling changes in nitrogen emissions into the oder river system 1875–1944. Regional Environ. Change 12, 571–580. doi: 10.1007/s10113-011-0270-5
Gadegast M., Venohr M. (2015). “Modellierung historischer nährstoffeinträge und -frachten zur ableitung von nährstoffreferenz- und orientierungswerten für mitteleuropäische flussgebiete,” in Bericht erstellt im auftrag des NLWKN (Berlin: Leibniz-Institut für Gewässerökologie und Binnenfischerei), 39. Available at: http://www.nlwkn.niedersachsen.de/download/98787.
Garcia N. V., Wasilewicz M., Tronscoso R. A., Zalewski T., Soszka H., Kolada A., et al. (2012). Weryfikacja warto’sci granicznych dla oceny stanu ekologicznego rzek i jezior w zakresie elementόw fizykochemicznych z uwzglednieniem warunków charakterystycznych dla poszczególnych typów wód. ADASA, IO´S-PIB, 180.
Gosselck F., Schabelon H. (2007). Aktueller zustand und historische entwicklung des makrozoobenthos und des makrophytobenthos des oderästuars- ein Überblick. IKZM-Oder Berichte 36, 33.
Gräwe U., Holtermann P., Klingbeil K., Burchard H. (2015). Advantages of vertically adaptive coordinates in numerical models of stratified shelf seas. Ocean Modell 92, 56–68. doi: 10.1016/j.ocemod.2015.05.008
HELCOM (2013) Summary report on the development of revised maximum allowable inputs (MAI) and updated country allocated reduction targets (CART) of the Baltic Sea action plan. Available at: http://www.helcom.fi/.
Hilt S., Gross E. M., Hupfer M., Morscheid H., Mählmann J., Melzer A., et al. (2006). Restoration of submerged vegetation in shallow eutrophic lakes – a guideline and state of the art in Germany. Limnologica 36 (3), 155–171. doi: 10.1016/j.limno.2006.06.001
Hirt U., Mahnkopf J., Gadegast M., Czudowski L., Mischke U., Heidecke C., et al. (2014). Reference conditions for rivers of the German Baltic Sea catchment: reconstructing nutrient regimes using the model MONERIS. Reg. Environ. Change 14, 1123–1138. doi: 10.1007/s10113-013-0559-7
Holtz L. (1892). Die characeen neuvorpommerns und der insel rügen und der insel usedom. Mitt. Nat. Ver. Neuvorpommern und Rügen 23, 99–156.
Horppila J., Nurminen L. (2003). Effects of submerged macrophytes on sediment resuspension and internal phosphorus loading in lake hiidenvesi (southern Finland). Water Res. 37 (18), 4468–4474. doi: 10.1016/S0043-1354(03)00405-6
Hussner A., Hoelken H. P., Jahns P. (2010). Low light acclimated submerged freshwater plants show a pronounced sensitivity to increasing irradiances. Aquat. Bot. 93 1, 17–24. doi: 10.1016/j.aquabot.2010.02.003
IKSO (2022a). Zweite aktualisierung des bewirtschaftungsplans für die internationale flussgebietseinheit (Internationale Kommission zum Schutz der Oder gegen Verunreinigung Oder). Available at: http://www.mkoo.pl/download.php?fid=6996&lang=DE.
IKSO (2022b). Strategie zur nährstoffreduzierung in den gewässern der internationalen flussgebietseinheit oder (Internationale Kommission zum Schutz der Oder gegen Verunreinigung). Available at: http://www.mkoo.pl/show.php?fid=7068&lang=DE.
James W. F., Barko J. W. (1994). Macrophyte influences on sediment resuspension and export in a shallow impoundment. Lake Reserv. Manag. 10 (2), 95–102. doi: 10.1080/07438149409354180
Janssen A. B., Teurlinkx S., An S., Janse J. H., Paerl H. W., Mooij W. M. (2014). Alternative stable states in large shallow lakes? J. Great Lakes Res. 40 (4), 813–826. doi: 10.1016/j.jglr.2014.09.019
Jensen H. S., Andersen F. O. (1992). Importance of temperature, nitrate, and ph for phosphate release from aerobic sediments of four shallow, eutrophic lakes. Limnol. Oceanogr. 37, 577–589. doi: 10.4319/lo.1992.37.3.0577
Jeppesen E., Jensen J. P., Sondergaard M., Lauritzen T. (1999). Trophic dynamics in turbid and clearwater lakes with special emphasis on the role of zooplankton for water clarity. Hydrobiologia 408/409, 217–231. doi: 10.1007/978-94-017-2986-4_24
Jones R. A., Lee G. F. (1986). Eutrophication modeling for water quality management: an update of the vollenweider-OECD model. World Health Organization's Water Qual. Bull. 11, 67–174.
Lee G. F., Jones R. A. (1981). “Application of the OECD eutrophication modeling approach to estuaries,” in Estuaries and nutrients (Clifton, NJ: Humana Press), 549–568.
LUNG (2016) Monitoringprogramm zur Überwachung der oberflächengewässer und des grundwassers in mecklenburg-vorpommern im zeitraum 2016-2021. Available at: http://www.lung.mv-regierung.de/.
Madsen J. D., Chambers P. A., James W. F., Koch E. W., Westlake D. F. (2001). The interaction between water movement, sediment dynamics and submersed macrophytes. Hydrobiologia 444 (1-3), 71–84. doi: 10.1023/A:1017520800568
Middelboe A. L., Markager S. (1997). Depth limits and minimum light requirements of freshwater macrophytes. Freshw. Biol. 37, 553–568. doi: 10.1046/j.1365-2427.1997.00183.x
Minning M. (2004). Der Einfluss des schifffahrtskanals auf den stoffhaushalt des oderhaffs. Mar. Sci. Rep. 57, 179–222.
Neubaur R. (1927). Beiträge zur kenntnis der molluskenfauna des stettiner haffs und der swinemünder bucht. Zeitschr. f. Fischerei 25, 245–261.
Neuhaus E. (1933). “Untersuchung über die allgemeinen hydrographischen und biologischen verhältnisse zeitschrift für fischerei und deren hilfswissenschaften,” in Studien über das stettiner haff und seine nebengewässer, 427–489.
Neumann T. (2000). Towards a 3D-ecosystemmodel of the Baltic Sea. J. Mar. Syst. 25, 405–419. doi: 10.1016/S0924-7963(00)00030-0
Neumann T., Fennel W., Kremp C. (2002). Experimental simulations with an ecosystem model of the Baltic Sea: a nutrient load reduction experiment. Global Biogeochem. Cycles 16, 3. doi: 10.1029/2001GB001450
Nickel J., Wilken H., Meyer T. (2019) Handlungsanweisung zum bewertungsverfahren PHYBIBCO, version 2. Available at: https://mhb.meeresschutz.info/files/meeresschutz/Dokumente/makrophyten/Handlungsanweisung_PHYBIBCO_Mai2019_deu.pdf.
Nowak P., Steinhardt T., Ammon U. V., Rohde H., Schoor H., Holzhausen A., et al. (2018). Diaspore bank analysis of Baltic coastal waters. Botany Lett. 165 (1), 159–173. doi: 10.1080/23818107.2017.1400464
Oncken N. S., Lange T., Kristensen E., Quintana C. O., Steinfurth R. C., Flindt M. R. (2022). Sand-capping – a large-scale approach to restore organic-enriched estuarine sediments. Mar. Envir Res. 173, 105534. doi: 10.1016/j.marenvres.2021.105534
Osadczuk A., Wawrzyniak-Wydrowska B. (1998). “Sediments in the szczecin lagoon: selected elements and macrozoobenthos,” in Greifswalder bodden und oderästuar - austauschprozesse (GOAP). Ed. Lampe R. (Greifswald: Universität Greifswald).
Porsche C., Schubert H., Selig U. (2008). Rezente verbreitung submerser makrophyten in den inneren küstengewässern der deutschen ostseeküste. Rostocker Meeresbiologische Beiträge 20, 109–122.
Portielje R., van der Molen D. T. (1999). Relationships between eutrophication variables: from nutrient loading to transparency. Hydrobiologia 408-409, 375–387. doi: 10.1023/A:1017090931476
Radtke H., Neumann T., Voss M., Fennel W. (2012). Modeling pathways of riverine nitrogen and phosphorus in the Baltic Sea. J. Geophysical Research Oceans 117 (C9), 2156–2202. doi: 10.1029/2012JC008119
Radziejewska T., Schernewski G. (2008). “The szczecin (Oder-) lagoon,” in Ecology of Baltic coastal waters. Ed. Schiewer U. (Berlin: Springer), 115–129.
Reynolds C. S. (1992). Eutrophication and the management of planktonic algae: what Vollenweider couldn't tell us. Freshwater Biol. Assoc. 3, 4–29.
Rudstam L. G., Gandino C. J. (2020). Zebra or quagga mussel dominance depends on trade-offs between growth and defense–field support from Onondaga lake, NY. PloS One 15 (6), e0235387. doi: 10.1371/journal.pone.0235387
Sagert S., Selig U., Schubert H. (2008). Phytoplankton indicators for ecological classification of coastal waters along the German Baltic coast. Rost. Meeresbiolog. Beitr. 20, 45–69.
Schadach M. (2013). “Muschelfarmen im stettiner haff,” in Eignungsgebiete vor dem hintergrund eines integrierten regionalmanagements (Rostock: Universität Rostock).
Scheffer M., Carpenter S., Foley J. A., Folke C., Walker B. (2001). Catastrophic shifts in ecosystems. Nature 413, 591–596. doi: 10.1038/35098000
Scheffer M., Redelijkheid M. R. D., Noppert F. (1992). Distribution and dynamics of submerged vegetation in a chain of shallow eutrophic lakes. Aquat. Bot. 42 3, 199–216. doi: 10.1016/0304-3770(92)90022-B
Schernewski G., Friedland R., Buer A. L., Dahlke S., Drews B., Höft S., et al. (2019). Ecological-social-economic assessment of zebra-mussel cultivation scenarios for the oder (Szczecin) lagoon. J. Coast. Conserv. 23, 913–929. doi: 10.1007/s11852-018-0649-2
Schernewski G., Friedland R., Carstens M., Hirt U., Leujak W., Nausch G., et al. (2015). Implementation of European marine policy: new water quality targets for German Baltic waters. Mar. Policy 51, 305–321. doi: 10.1016/j.marpol.2014.09.002
Schernewski G., Neumann T., Behrendt H. (2011). “Sources, dynamics and management of phosphorus in a southern Baltic estuary,” in The Baltic Sea basin as a natural laboratory. Eds. Harff J., Björck S., Hoth P. (Springer), 373–388.
Schernewski G., Stybel N., Neumann T. (2012). Managing eutrophication: cost-effectiveness of zebra mussel farming in the oder (Szczecin) lagoon. Ecol. Soc. 17 (2), 4. doi: 10.5751/ES-04644-170204
Schiewer U., Glocke K. (1996). “Ökologie der bodden und förden,” in Meereskunde der ostsee. 2. auflage. Ed. Rheinheimer G. (Verlag, Berlin, Heidelberg, New York: Springer).
Schindler D. W. (2006). Recent advances in the understanding and management of eutrophication. Limnol. Oceanogr. 51 (1,2), 356–363. doi: 10.4319/lo.2006.51.1_part_2.0356
Schneider S. (2004). “Indikatoreigenschaften und Ökologie aquatischer makrophyten in stehenden und fließenden gewässern. habilitationsschrift,” in Wissenschaftszentrum weihenstephan (München: Technische Universität München).
Schriever P., Bogestrand J., Jeppesen E., Søndergaard M. (1995). Impact of submerged macrophytes on fish-zooplanl phytoplankton interactions: large-scale enclosure experiments in a shallow eutrophic lake. Freshw. Biol. 33, 255–270. doi: 10.1111/j.1365-2427.1995.tb01166.x
Schubert H., Blümel C., Eggert A., Rieling T., Schubert M., Selig U. (2003). Entwicklung von leitbildorientierten bewertungsgrundlagen für innere küstengewässer der deutschen ostseeküste nach der EU-WRRL: endbericht zum forschungsvorhaben 0330014 des BMBF (Rostock: Universität Rostock).
Schubert H., Schubert M., Krause J. C. (2007). Reconstruction of XIXth century submerged vegetation of coastal lagoons of the German Baltic Sea. Sea Environ. 1 (14), 16–27.
Selig U., Sagert S., Reincke T. (2006). Erarbeitung eines ökologischen gesamtansatzes für die bewertung der küstengewässer an der deutschen ostseeküste entsprechend der vorgabender EU-WRRL (Rostock: Zwischenbericht zum BMBF).
Steinhardt T., Selig U. (2008). Diasporen von characeen und angiospermen in sedimenten von küstengewässern der südlichen ostseeküste. Rostocker Meeresbiologische Beiträge 20, 175–190.
Van den Berg M., Scheffer M., van Nes E., Coops H. (1999). Dynamics and stability of chara sp. and potamogeton pectinatus in a shallow lake changing in eutrophication level. Hydrobiologia 408, 335–342. doi: 10.1007/978-94-017-2986-4_37.pdf
Van Donk E., van de Bund W. J. (2002). Impact of submerged macrophytes including charophytes on phyto- and zooplankton communities: allelopathy versus other mechanisms. Ecol. Charophytes 72 (3–4), 261–274. doi: 10.1016/S0304-3770(01)00205-4
Vollenweider R. A. (1976). Advances in defining critical loading levels for phosphorus in lake eutrophication. Memorie dell' Istituto Italiano di Idrobiologia 33, 53–83.
Voss M., Deutsch B., Liskow I., Pastuszak M., Schulte I., Sitek S. (2010). Nitrogen retention in the szczecin lagoon, Baltic Sea. Isotopes Environ. Health Stud. 46 (3), 355–369. doi: 10.1080/10256016.2010.503895
Wawrzyniak-Wydrowska B., Skrzypacz A., Wozniczka A., Radziejewska T. (2018). Has dreissena polymorpha, an older immigrant in a coastal Baltic lagoon, responded to appearance of a new non-indigenous dreissenid? PeerJ. doi: 10.7287/peerj.preprints.26797v1
Wielgat M., Witek Z. (2004). A dynamic box model of the Szczecin Lagoon nutrient cycling and its first application to the calculation of the nutrient budget. Mar. Sci. Rep. 57, 99–119.
Wolnomiejski N., Witek Z. (2013). “The szczecin lagoon ecosystem,” in The biotic community of the great lagoon and its food web model versita (London, England: Versita), 294. Available at: www.degruyter.com/view/product/209571.
Yousef M. A. M. (1999). “Ökophysiologie von makrophyten und epiphyten in flachwasserökosystem-en,” in Dissertation (Rostock: Universität Rostock).
Keywords: angiosperms, charophytes, eutrophication, good ecological status, water transparency, chlorophyll, Szczecin Lagoon, target
Citation: Schernewski G, Friedland R, Paysen S, Bucas M, Dahlke S and von Weber M (2023) Macrophytes and water quality in a large Baltic lagoon: relevance, development and restoration perspectives. Front. Mar. Sci. 10:1049181. doi: 10.3389/fmars.2023.1049181
Received: 20 September 2022; Accepted: 14 April 2023;
Published: 29 May 2023.
Edited by:
Ronald Osinga, Wageningen University and Research, NetherlandsReviewed by:
Georgios Sylaios, Democritus University of Thrace, GreeceEmma Cebrian, Centre d’Estudis Avançats de Blanes, Spain
Copyright © 2023 Schernewski, Friedland, Paysen, Bucas, Dahlke and von Weber. This is an open-access article distributed under the terms of the Creative Commons Attribution License (CC BY). The use, distribution or reproduction in other forums is permitted, provided the original author(s) and the copyright owner(s) are credited and that the original publication in this journal is cited, in accordance with accepted academic practice. No use, distribution or reproduction is permitted which does not comply with these terms.
*Correspondence: Gerald Schernewski, Z2VyYWxkLnNjaGVybmV3c2tpQGlvLXdhcm5lbXVlbmRlLmRl