- 1Guangdong Provincial Key Laboratory of Fishery Ecology and Environment, Guangzhou, South China Sea Fisheries Research Institute, Chinese Academy of Fishery Sciences, Guangzhou, China
- 2Scientific Observation and Research Field Station of Pear River Estuary Ecosystem, Guangdong, Guangzou, China
The Jinwan Offshore Wind Farm project in the Pearl River Estuary (PRE) is a new stressor for the resident marine mammals there, especially for the Indo-Pacific finless porpoise. A broadband recording system was deployed in the Jinwan Offshore Wind Farm, before and during the construction period, in order to determine how the finless porpoise responded to pile driving activity. The results showed that the wind farm area was an important habitat for the finless porpoise during the monitoring period. The finless porpoise also showed avoidance behavior of pile driving activity. There was a significant negative correlation between porpoise detection and pile driving detection, and the time between porpoise’s acoustic detections increased during pile driving compared to periods without pile driving. Our results indicated that acoustic protection measures are strongly recommended in future offshore wind farm developments in order to protect finless porpoises.
1 Introduction
Anthropogenically accelerated climate change as a consequence of burning fossil fuels has led to many governments investing heavily in renewable energy sources (Gallagher, 2013; White et al., 2013; Dwyer and Teske, 2018; Johnsson et al., 2019; Sharif et al., 2019). This has been particularly noticeable in China, where the government has been rapidly constructing offshore wind farms with a potential total output of 600 GW (Yang et al., 2017). Installed wind energy capacity in China is said to be increasing at a rate of 9.56% through to 2025, including both on- and offshore wind farms (Yang et al., 2017). However, while the global benefits of offshore wind farms are not in question, the potential effects on marine mammals locally do need to be considered. This is because offshore wind farms are often constructed in shallow environments with high biodiversity and cover large areas. As such, there is often considerable physical overlap between offshore wind farms and core marine mammal habitats around China, particularly in the Pearl River Estuary (PRE)—leading to a range of potential impacts (Gill, 2005; OSPAR Commission, 2008; Bailey et al., 2014; Bergström et al., 2014). Public and stakeholder concerns about the potential impacts of the construction, operation and maintenance of offshore wind farms on marine mammals are therefore warranted.
Underwater noise from construction activities is well documented, and is a commonly listed contributor to habitat-use changes by marine mammals (Tyack, 2008; Richardson et al., 2013). Noise impacts relating to offshore wind farms are predominately associated with their installation, while increased vessel activity in the area, pile driving, dredging, blasting, and vibrations are the main sources of noise potentially impacting marine mammals (Madsen et al., 2006; Thomsen et al., 2006; Matuschek and Betke, 2009; Bailey et al., 2010; Thompson et al., 2010; Cheesman, 2016). Marine mammals are sensitive to underwater noise because they are heavily reliant on sound for communication, prey detection and capture, group cohesion, and navigation, and have evolved highly sensitive hearing to enable these diverse biological functions(Au et al., 2000; Au and Hastings, 2008). Therefore, underwater noise pollution can often affect the behavior, communications, acute physiology (such as hearing loss), and habitat-use of marine mammals(Weilgart, 2007a; Weilgart, 2007b).
The Indo-Pacific finless porpoise (Neophocaena phocaenoides) is a small and timid cetacean, occurring mainly in the shallow coastal waters (less than 50 m depth) of the Persian Gulf eastward to the Taiwan Strait and southward to Indonesia (Wang and Reeves, 2017). However, since it is difficult to survey this species in the wild by visual means, it has only been studied in a few areas (Wang and Reeves, 2017). The Pearl River Estuary (PRE) is an important habitat for the Indo-Pacific finless porpoise; however, nearly all of the surveys in the wild have been carried out in the waters around Hong Kong, which is located within the Pearl River Delta (Jefferson et al., 2002; Jefferson and Moore, 2020). The status of the finless porpoise population in the PRE is still controversial. Recently, a study of the abundance and population trends of this cetacean in the waters around Hong Kong suggested that there has been no significant change in population over the past 23 years (Jefferson and Moore, 2020), whereas an analysis of the population dynamics of the same species in the PRE region suggests that the population is fluctuating, which relates to the changing of fishery management (Lin et al., 2019). Offshore wind farm development in the PRE might therefore prove to be a new and serious challenge to finless porpoises in this area. Thus, it is critical to determine how the finless porpoise responds to underwater noise during wind farm construction.
Passive acoustic monitoring (PAM) of cetaceans is now a widely used and continuously evolving method due to its economic viability in providing detailed information on whale and dolphin activity (Zimmer, 2011; Sousa-Lima et al., 2013). The technology is also widely used in assessing the impacts of offshore wind farms on marine mammals (Brandt et al., 2009; Thompson et al., 2010; Scheidat et al., 2011; Brandt et al., 2018) and general habitat use by humpback dolphins within the PRE (Wang et al., 2015; Munger et al., 2016; Pine et al., 2016; Pine et al., 2017; Munger et al., 2018; Fang et al., 2020). Through the use of PAM, this study investigated the Indo-Pacific finless porpoise’s presence in response to pile driving activity during the construction of the Jinwan Offshore Wind Farm. The results of our study and the recommendations outlined below are also relevant to the future management of offshore wind farm developments elsewhere globally.
2 Methods
2.1 Study area and acoustic measuring device
The Jinwan Offshore Wind Farm is the second wind farm to be constructed in the PRE, located to the southeast of Zhuhai Jinwan Airport and northeast of Gaolan Island, a distance of 10.5 km to the nearest point of land (Figure 1A). The wind farm consists of 55 5.5 MW wind turbines arranged in five rows, with spacings of 1912 m between the rows and 512 m between the wind turbines. The wind farm covers an area of 44.5 km2, with the water depth ranging from 14 to 22 m. The foundations for the wind turbines were installed between August 2019 and May 2020. The turbine foundation piles are 7.5 m in diameter and 93.2 m in length. The pile driving for constructing the foundations was performed using an IHC S-3000 (IHC IQIP, USA) hydraulic hammer for steel piles.
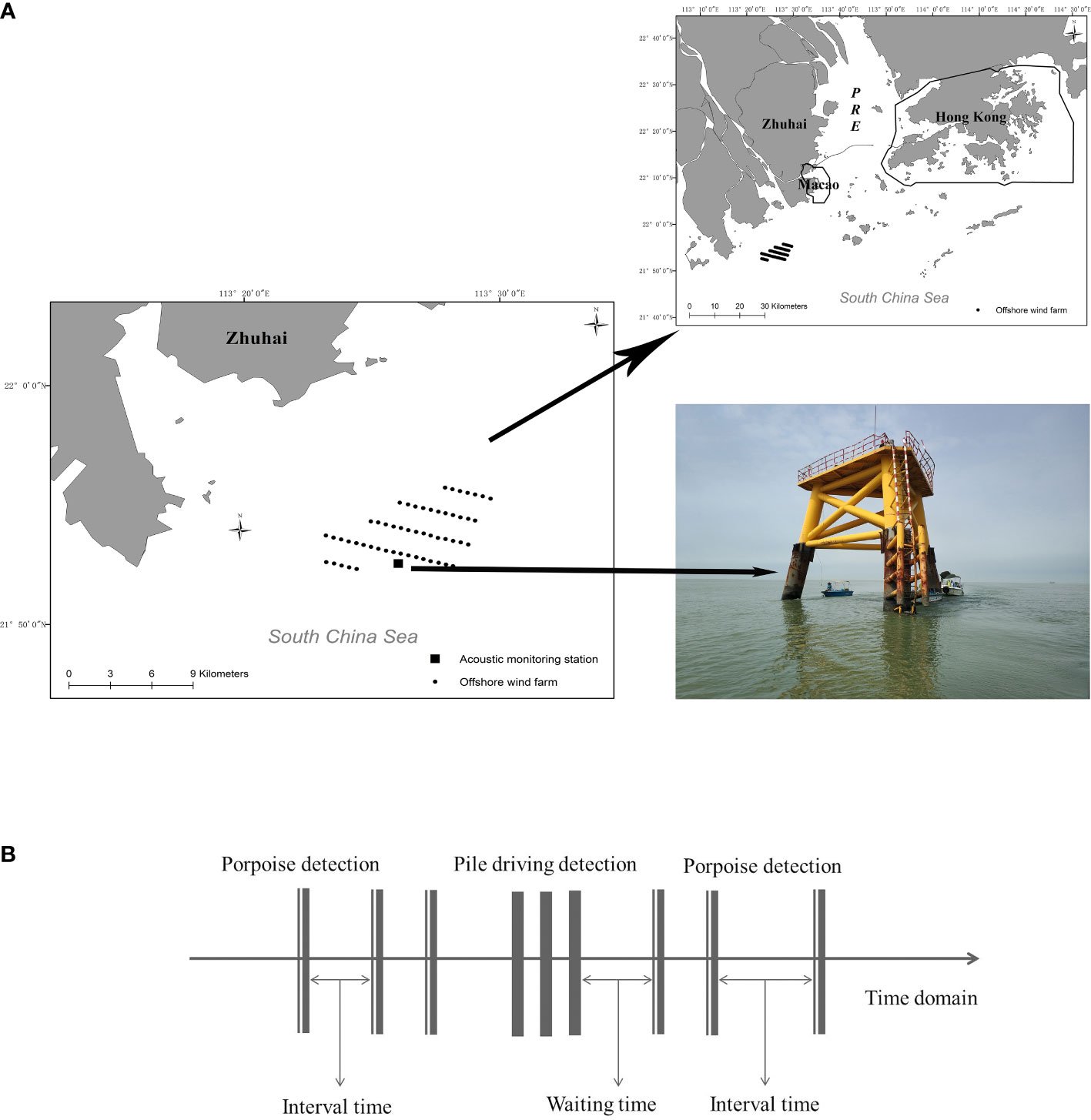
Figure 1 (A) Locations of the Jinwan Offshore Wind Farm turbine and the acoustic monitoring station; (B) Schematic of porpoise detection, pile driving detection, interval time of porpoise detection, and porpoise waiting time to pile driving.
To determine how the Indo-Pacific finless porpoise responded to the pile driving activity, a broadband acoustic recording system, Soundtrap 300 HF (Ocean Instruments Ltd, New Zealand), was used to record the sounds from the finless porpoises as well as the pile driving activity. The Soundtrap 300 HF is a compact autonomous unit with a frequency range between 20 Hz and 150 kHz. The duration of each deployment was dependent on the unit’s battery and memory, as well as the weather conditions prevailing at the time of measurement, but usually lasted between 40 and 60 days. The series number and sensitivities of soundtraps, gain setting and recording time were show in Table 1.
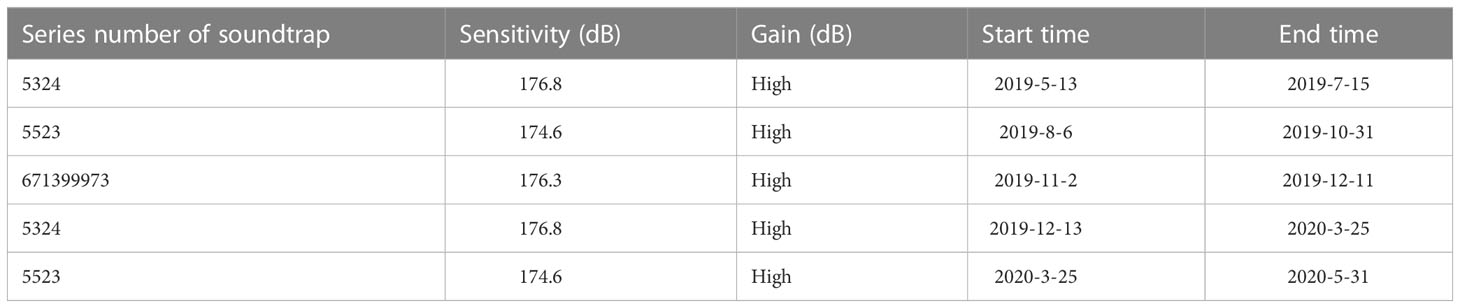
Table 1 The information of the recording instruments, including the series mumber of soundtrap, sensitivities, gain setting and recording time of each period.
During recording, the anemometer tower was used as the passive acoustic monitoring station (N21°52′33.17″, E 113°26′1.97″), where the distances were 780 m to the nearest and 6800 m to the furthest wind farm foundation (Figure 1A). The Soundtrap HF 300 was tied to the leg of the anemometer tower, 4 m below the water surface. The acoustic equipment was set to record for 5 min per hour, and the sampling rate was set at 288 kHz with high gain. The acoustic equipment was changed nearly two month once time to download data by diver.
2.2 Data analysis
All acoustic data were analyzed manually using Adobe Audition 3.0 (Adobe, Inc) digital audio workstation software. Finless porpoises emit narrow-band and high-frequency echolocation clicks, with a peak frequency higher than 120 kHz and barely have energy distributed less than 70 kHz. These special characteristics allow the clicks of finless porpoises to be easily distinguished in spectrograms from the background ocean noise (Li et al., 2005; Fang et al., 2015).
To better understand the effects of the offshore wind farm construction on the habitat used by the Indo-Pacific finless porpoise, several parameters were defined, including porpoise detection, pile driving activity detection, waiting time, and interval time. Porpoise detection was defined as the detection of echolocation clicks by finless porpoises in the acoustic file containing 5 min of recording time per hour, and pile driving detection was defined as the detection of pile driving sounds in the acoustic file of 5 min recording time. The detection rates in each month for finless porpoises and pile driving were calculated from the number of detections and the total number of recorded files (Fang et al., 2020). Waiting time was defined as the time interval between a pile driving detection and the first time of an acoustic occurrence from a finless porpoise, which is an important piece of data to show how long of the porpoise occurrence after the wind farm pile driving activity. The interval time was defined as the time between adjacent porpoise detections without the pile driving detection (Figure 1B).
The porpoise clicks and pile driving were analyzed visually. The peak-to-peak sound pressure level (SPL) of pile driving detected by the acoustic measuring equipment was expressed as a peak-to-peak level given by Eq. (1):
Where SPL is the highest observed peak to peak sound pressure level from the acoustic recorder and P0 is the reference sound pressure, which is 1µPa. An example of pile driving activities with the waveform and spectrum of a single pulse was presented on Figure 2.
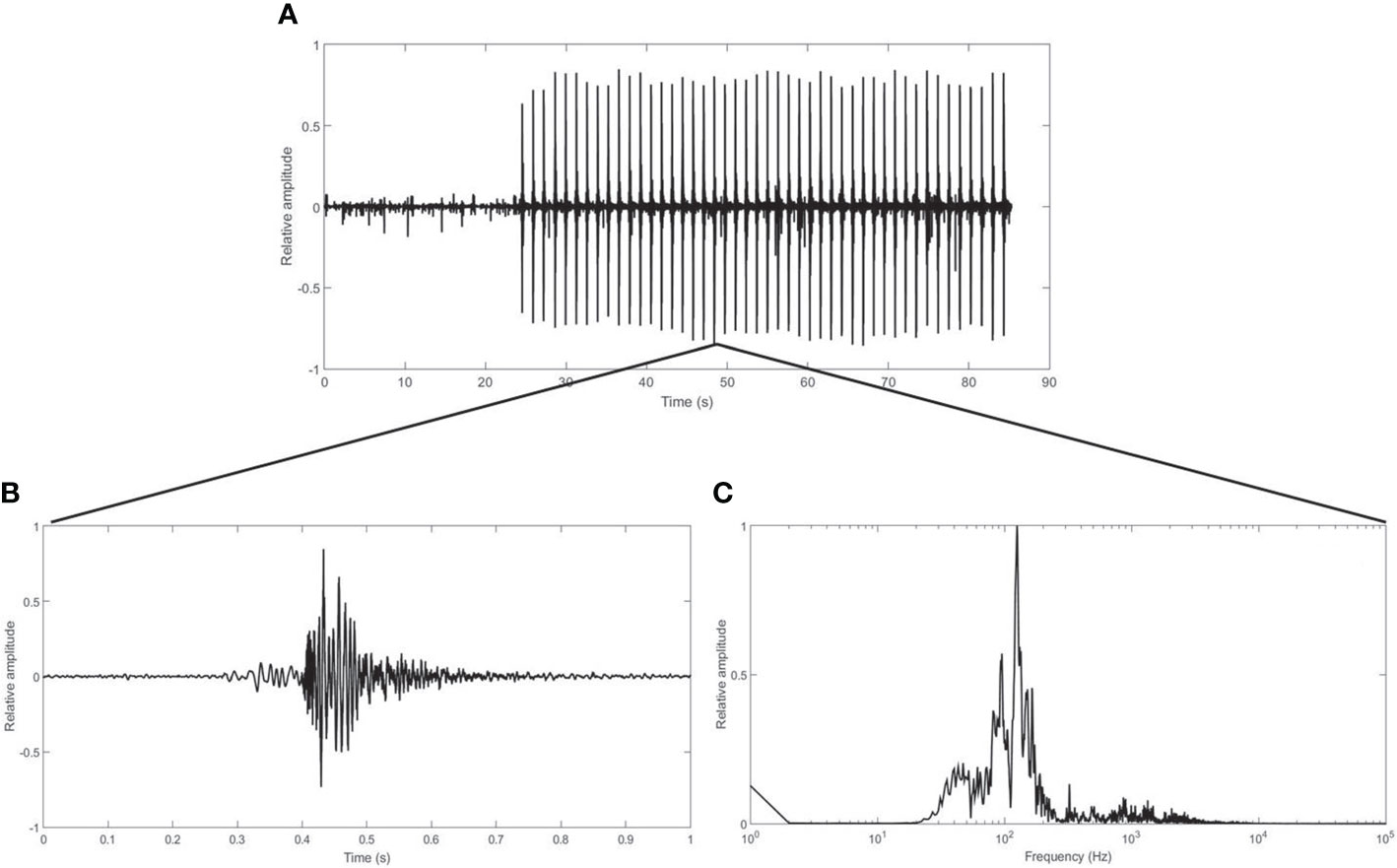
Figure 2 (A) Example of the high sound pressure level of pile driving activity; (B) waveform; (C) spectrum of a single pile driving pulse.
The data were analyzed by SPSS software (version 16.0; SPSS Inc., Chicago, Illinois). Significant difference between the waiting times and the interval times was tested using Mann-Whitney U-test with the given significant level P<0.01 and the relationship between the acoustic detection rates of finless porpoises and of pile driving in each month was described by Pearson correlation.
3 Results
From May 13, 2019 to May 31, 2020, a total of 13 months of continuous acoustic recording was conducted (Figure 3A) and a total of 8631 sound files were collected, including 1072 files of finless porpoise sounds and 92 files of pile driving activities.
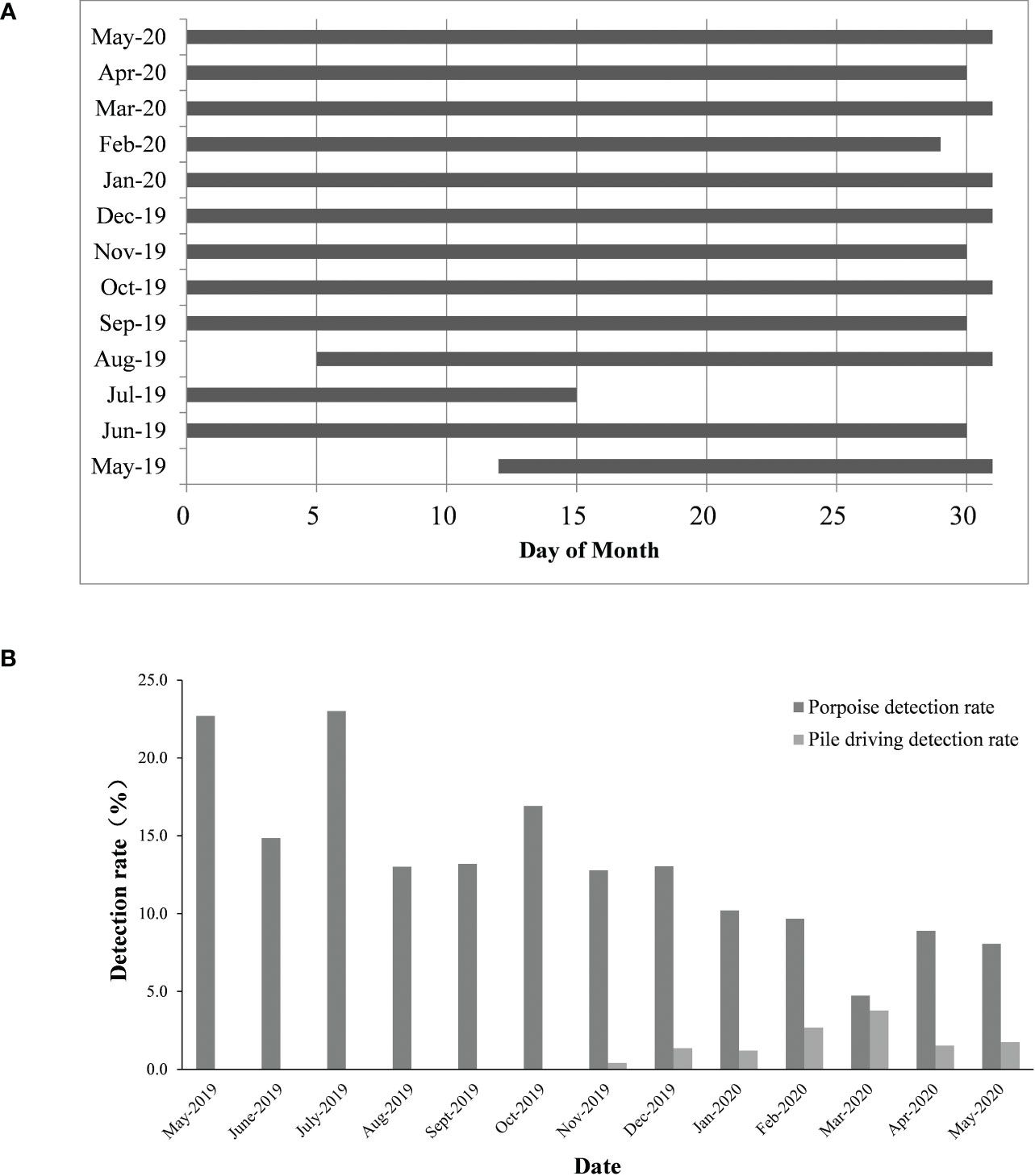
Figure 3 (A) The acoustic monitoring days of each month from May 2019 to May 2020; (B) histogram of detection rates of Indo-Pacific finless porpoises and pile driving activity in each month of monitoring.
Histograms of the finless porpoise and pile driving detection rates in different months throughout the entire monitoring period are presented in Figure 3B. The results indicate the occurrence of finless porpoises in the monitoring area throughout the entire monitoring period. The highest detection rate of finless porpoises was 23% in July 2019, and the lowest rate was 4.7% in March 2020. Pile driving activity was first detected in November 2019 and continued until May 2020. The greatest number of occurrences was in March 2020, with a detection probability of 3.8%, while the lowest number of occurrences was in November 2019, with a detection probability of 0.4%.
A histogram of the SPL values of pile driving is presented in Figure 4. The SPL values ranged from 141.3 to 183.1 dB, nearly 41% of the SPL values were distributed between 170 and 180 dB, 17.85% were higher than 180 dB, and 28.21% were distributed between 160 and 170 dB.
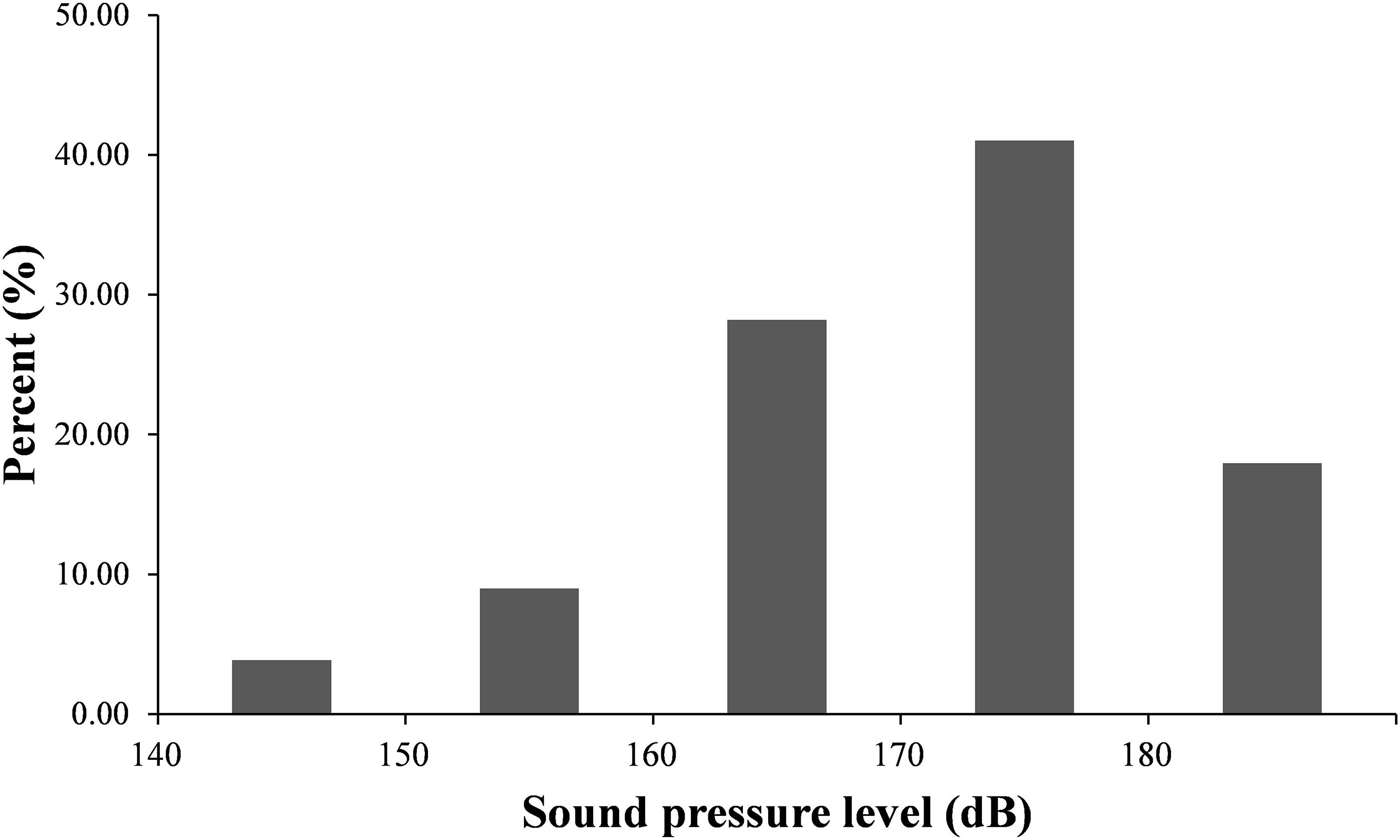
Figure 4 Distribution of sound pressure level (SPL) values of pile driving (peak-to-peak) detected by the passive acoustic measuring equipment.
A significant negative correlation between the detection rates of finless porpoises and pile driving activity was also observed (Pearson correlations, P = 0.025, R2 = 0.69, Figure 5). The interval time of porpoise occurrence without pile driving activity is presented in Figure 6A. Nearly 89.2% of the interval times of porpoise occurrence were less than 20 h, and 73.9% of interval times were less than 10 h. The waiting times of finless porpoises to pile driving events are also presented in Figure 6A. A total of 45.9% of waiting times were distributed between 0 and 10 h and 29.7% between 10 and 20 h. The median interval time of porpoise occurrence and the average waiting time are presented in Figure 6B, and these showed a significant difference (P< 0.01).
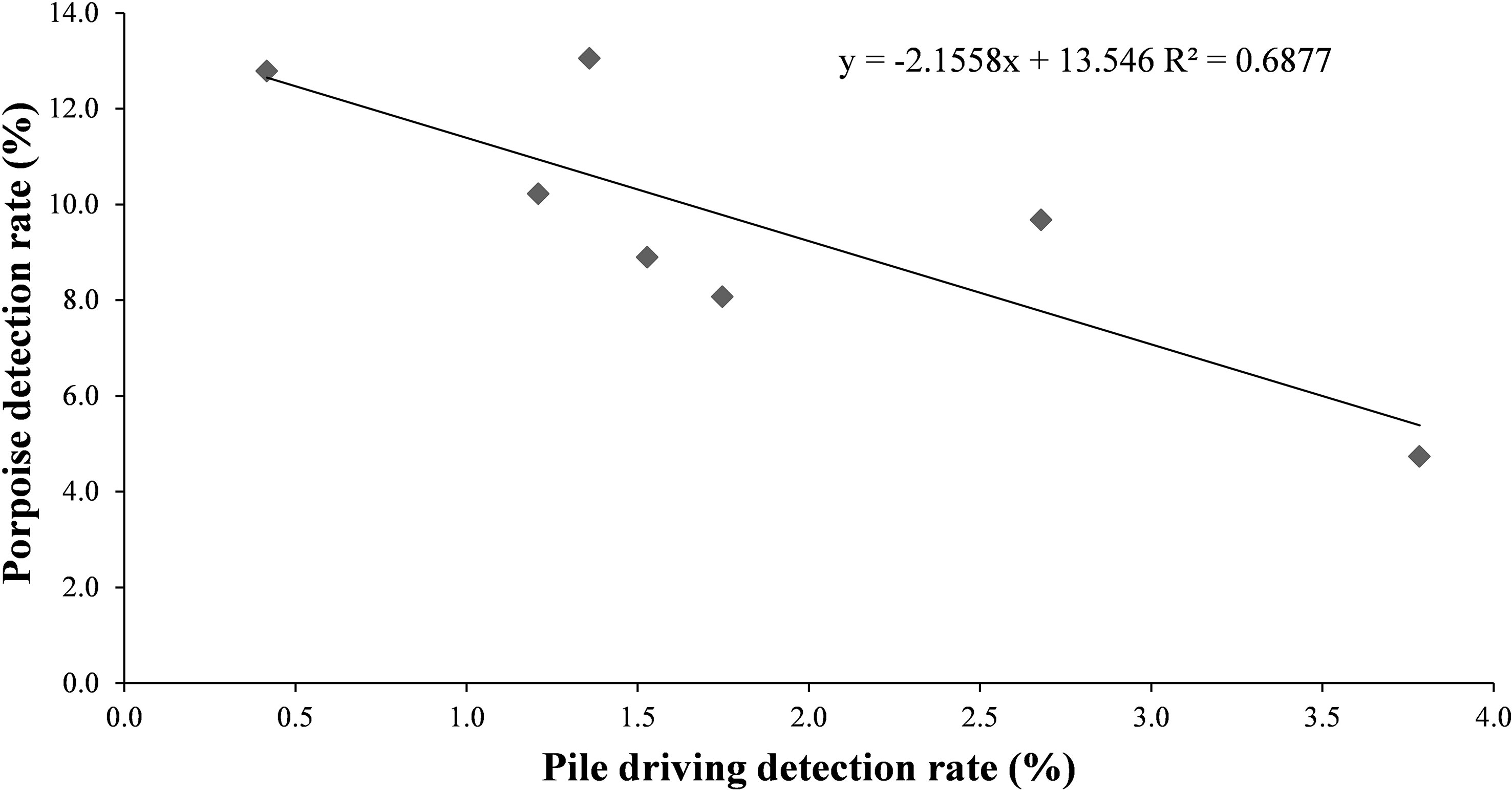
Figure 5 Relationship between the monthly detection rate of finless porpoises and pile driving activity (R2 = 0.69).
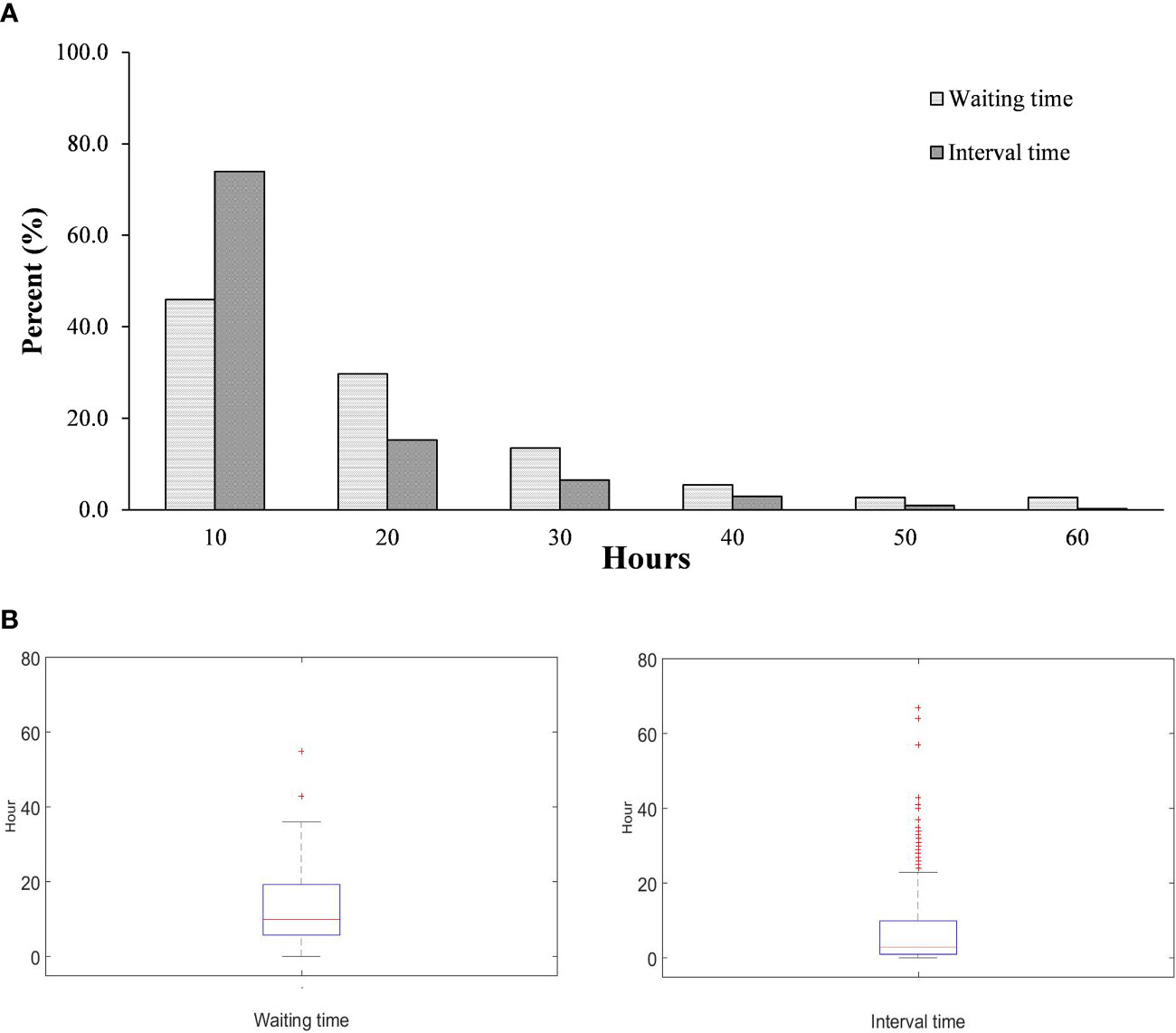
Figure 6 (A) Histograms of waiting time and interval time; (B) median waiting time and average interval time.
4 Discussion
Our results for the detections of finless porpoises both before and during wind farm construction demonstrate for the first time that these waters are an important habitat for Indo-Pacific finless porpoises. However, there has been almost no consideration of the possible adverse effects of wind farm construction on the finless porpoise. Moreover, in recent years, offshore wind farm projects have been increasing rapidly in number in Pacific Ocean regions. Passive acoustic monitoring surveys, as a low-cost method, are strongly recommended for monitoring the activity of Indo-Pacific finless porpoises in the candidate areas for offshore wind farms, which has been found to be effective in numerous studies of the environmental impacts of wind farm construction on marine mammals (Brandt et al., 2009; Thompson et al., 2010; Brandt et al., 2011; Brandt et al., 2012; Graham et al., 2017; Graham et al., 2019).
Pile driving is one of the major activities taking place during wind farm construction, and the high noise levels created by it can result in a series of adverse impacts on marine organisms, especially marine mammals (Madsen et al., 2006). Over short distances, the loud noise from pile driving may cause a direct disturbance or a hearing injury, such as temporary damage to hearing thresholds (Finneran, 2015; Southall et al., 2019). The source level (SL) of pile driving activity was given by equation SL= SPL +TL=SPL+20log10(R)+ αR, TL is the transmission loss and R is the distance between pile driving site and acoustic equipment, α is the absorption coefficient of 0.0004dB (Bailey et al., 2010). In the present study, the highest peak-to-peak SPL received by the acoustic recorder was as much as 183.34 dB. Considering that the nearest turbine foundation was a distance of 780 m from the acoustic recorder, the actual noise level of the source pile driving activity was estimated to have been higher than 241.3 dB, which is much higher than the criterion 202 dB (unweight peak SPL) for the onset of permanent threshold shift (PTS) and 196 dB (unweight peak SPL) for the onset of temporary threshold shift (TTS) for very high-frequency cetaceans (Southall et al., 2019). The 241.3 dB pile driving sounds could induce the finless porpoise temporary and permanent hearing loss within range of 92.3 and 184.1 m. This means that there is quite a high risk of hearing damage to finless porpoises in close range to pile driving activities.
A significant negative correlation between the monthly detection rate of finless porpoises and pile driving activity was found, which suggests that finless porpoises reduce their use of their regular habitat in response to pile driving activity. In addition, the median waiting time 10 hour was significant longer than the median interval time 3 hour meant that harbor porpoises took more time to return to the wind farm habitat following completion of pile driving. This is probably because such a high-pressure level of pile driving noise can affect porpoises over a large spatial range. Pile driving can affect a quite large range of habitat for porpoises, which was observed in several studies. Brandt et al. (2011) found a clear negative effect on harbor porpoise acoustics at a distance of 17.8 km (Brandt et al., 2011). Dähne et al. (2013) showed a negative impact on harbor porpoise detection by pile driving at a distance of less than 10.8 km (Dähne et al., 2013). Even more, it has been reported as being more than 20 km by several studies (Tougaard et al., 2009; Thompson et al., 2010; Brandt et al., 2011). Our results show direct evidence for the porpoise avoidance of the pile driving activities and finless porpoise would reduce using this habitat in several months. It is unclear the effects of the porpoise away its habitat in several months, more work should be done during the construction, like monitoring the number of population and distribution of finless porpoise. In addition, continued concern for finless porpoises how to response and adapt the operation phase of off shore wind farm.
5 Conclusion and recommendations
Our results indicate that the waters surrounding the Jinwan Offshore Wind Farm are an important habitat for Indo-Pacific finless porpoises, which were found there both before and during the construction period. The frequency of finless porpoise detections was reduced after pile driving was detected, which correlated strongly with pile driving activity. Due to the noise level at the source of the pile driving being estimated to have been more than 240 dB, there was a high risk of temporary or even permanent hearing damage over short distances to finless porpoises according to the sound exposure criteria issued by the US Department of Commerce’s National Oceanic and Atmospheric Administration (NOAA). Offshore wind farms, as a new energy industry, are growing rapidly in China and Southeast Asia in general. However, little attention has been paid to their impact on marine mammals, especially the Indo-Pacific finless porpoise. The following recommendations are based on the lessons learned from this study, which are hoped will help to reduce the impact of further offshore wind farm developments on the finless porpoise in unsurveyed waters:
1. Passive acoustic monitoring was successfully used to show the porpoise acoustic presence in response to pile driving activity. We strongly recommend the use of passive and stationary acoustic monitoring methods for surveying candidate waters for offshore wind farms where there is limited funding support. Such data are urgently required for assessing the impact of offshore wind farms on finless porpoises in order to take appropriate action to ease the effects on the porpoise population in these areas. Furthermore, to better know how the finless porpoise response to the pile driving activity, multiple acoustic recorders should be deployed before and during the construction period in different distances to the pile driving sites.
2. Because of unknown the distance between the acoustic recorder and the pile driving sites, we can’t calculate the source level of pile driving. However, there is a need to monitor noise events and noise levels throughout the entire construction period, especially pile driving activities. The intensity of noise from pile driving is a key factor for evaluating the effects of offshore wind farm construction on marine mammals. Pile driving was always with multiple pulses. Analyzing the pile driving noise should also include the parameter of sound exposure level, which can provide the indication of pile driving energy with different number of pulses. Marine mammals show different acoustic activities to the pile driving noise in different distances, knowing the range of finless porpoise response to the pile driving noise can help the government making protect area for finless porpoies during the construction period.
3. High pile driving noise pressure level was observed in present study. The high intensity noise could damage the porpoise hearing system directly (Richardson et al., 2013; Southall et al., 2019). Noise mitigation measures should be developed during offshore wind farm construction. The principal source of noise coming from offshore wind farms during construction is foundation installation, including pile driving. Numerous measures are recommended for reducing noise and acoustic disturbance during construction, including bubble curtains, isolation casings, cofferdams, pingers, soft-start pile driving, and the use of low-noise pile driving equipment.
4. Until now, most studies of the noise from offshore wind farms have focused on construction activity, while the effects of noise from the actual operation of offshore wind farms has been ignored. Since the operating times of offshore wind farms are much longer than their construction periods, the short-term and long-term noise impacts on marine mammals during operation period of offshore wind farms should remain a concern.
Data availability statement
The raw data supporting the conclusions of this article will be made available by the authors, without undue reservation.
Ethics statement
Ethical review and approval was not required for the animal study because There is no negative effect on marine mammals by a station passive acoustic monitoring.
Author contributions
LF conceived the study, carried out field work, analyzed data, and wrote the majority of the manuscript. ML,XW, YJC carried out filed work TC conceived the study, analyzed data and revised the manuscript All authors contributed to the article and approved the submitted version.
Funding
This research was supported by the grant of Central Public-interest Scientific Institution Basal Research Fund, South China Sea Fisheries Research Institute, CAFS (NO. 2019TS27, 2019ZD0202, 2021SD18), CAS Key Laboratory of Science and Technology on Operational Oceanography (No.OOST2021-06) and Special Foundation for National Science and Technology Basic Research Program of China (2019FY101905).
Acknowledgments
We grateful thank the captain Jianxiong Ruan and stuff of Haiyang diving company Changqing Luo.
Conflict of interest
The authors declare that the research was conducted in the absence of any commercial or financial relationships that could be construed as a potential conflict of interest.
Publisher’s note
All claims expressed in this article are solely those of the authors and do not necessarily represent those of their affiliated organizations, or those of the publisher, the editors and the reviewers. Any product that may be evaluated in this article, or claim that may be made by its manufacturer, is not guaranteed or endorsed by the publisher.
References
Bailey H., Brookes K. L., Thompson P. M. (2014). Assessing environmental impacts of offshore wind farms: lessons learned and recommendations for the future. Aquat. Biosyst. 10 (1), 8. doi: 10.1186/2046-9063-10-8
Bailey H., Senior B., Simmons D., Rusin J., Picken G., Thompson P. M. (2010). Assessing underwater noise levels during pile-driving at an offshore windfarm and its potential effects on marine mammals. Mar. pollut. Bull. 60 (6), 888–897. doi: 10.1016/j.marpolbul.2010.01.003
Bergström L., Kautsky L., Malm T., Rosenberg R., Wahlberg M., Capetillo N.Å., et al. (2014). Effects of offshore wind farms on marine wildlife–a generalized impact assessment. Environ. Res. Lett. 9 (3), 34012. doi: 10.1088/1748-9326/9/3/034012
Brandt M. J., Diederichs A., Betke K., Nehls G. (2011). Responses of harbour porpoises to pile driving at the horns rev II offshore wind farm in the Danish north Sea. Mar. Ecol. Prog. Ser. 421, 205–216. doi: 10.3354/meps08888
Brandt M. J., Diederichs A., Betke K., Nehls G. (2012). “Effects of offshore pile driving on harbor porpoises (Phocoena phocoena),” in The effects of noise on aquatic life (New York: Springer), 281–284.
Brandt M. J., Diederichs A., Nehls G. (2009). Harbour porpoise responses to pile driving at the Horns Rev II offshore wind farm in the Danish North Sea (Husum, Germany: BioConsult SH).
Brandt M. J., Dragon A. C., Diederichs A., Bellmann M. A., Wahl V., Piper W., et al. (2018). Disturbance of harbour porpoises during construction of the first seven offshore wind farms in Germany. Mar. Ecol. Prog. 596, 213–232. doi: 10.3354/meps12560
Cheesman S. (2016). Measurements of operational wind turbine noise in UK waters. Adv. Exp. Med. Biol. 875, 153–160. doi: 10.1007/978-1-4939-2981-8_18
Dähne M., Gilles A., Lucke K., Peschko V., Adler S., Krügel K., et al. (2013). Effects of pile-driving on harbour porpoises (Phocoena phocoena) at the first offshore wind farm in Germany. Environ. Res. Lett. 8 (2), 279–288.
Fang L., Lin W., Guo L., Cai H., Pine M. K., Wu Y. (2020). Monitoring indo-pacific humpback dolphin occurrences in a highly urbanized estuary for informing conservation and management. Aquat. Conservation: Mar. Freshw. Ecosyst. 31 (3), 685–696. doi: 10.1002/aqc.3475
Fang L., Wang D., Li Y., Cheng Z., Pine M. K., Wang K., et al. (2015). The source parameters of echolocation clicks from captive and free-ranging Yangtze finless porpoises (Neophocaena asiaeorientalis asiaeorientalis). PloS One 10 (6), e0129143. doi: 10.1371/journal.pone.0129143
Finneran J. J. (2015). Noise-induced hearing loss in marine mammals: a review of temporary threshold shift studies from 1996 to 2015. J. Acoustical Soc. America 138 (3), 1702–1726. doi: 10.1121/1.4927418
Gallagher K. S. (2013). Why & how governments support renewable energy. Daedalus 142 (1), 59–77. doi: 10.1162/DAED_a_00185
Gill A. B. (2005). Offshore renewable energy: ecological implications of generating electricity in the coastal zone. J. Appl. Ecol. 42 (4), 605–615. doi: 10.1111/j.1365-2664.2005.01060.x
Graham I. M., Merchant N. D., Farcas A., Barton T. R., Cheney B., Bono S., et al. (2019). Harbour porpoise responses to pile-driving diminish over time. R. Soc. Open Sci. 6 (6), 190335. doi: 10.1098/rsos.190335
Graham I. M., Pirotta E., Merchant N. D., Farcas A., Barton T. R., Cheney B., et al. (2017). Responses of bottlenose dolphins and harbor porpoises to impact and vibration piling noise during harbor construction. Ecosphere 8 (5), e01793. doi: 10.1002/ecs2.1793
Jefferson T. A., Hung S. K., Law L., Torey M., Tregenza N. (2002). Distribution and abundance of finless porpoises in Hong Kong and adjacent waters of China. Raffles Bull. Zool 50, 43–56.
Jefferson T. A., Moore J. E. (2020). Abundance and trends of indo-pacific finless porpoises (Neophocaena phocaenoides) in Hong Kong water 1996–2019. Front. Mar. Sci. 7. doi: 10.3389/fmars.2020.574381
Johnsson F., Kjärstad J., Rootzén J. (2019). The threat to climate change mitigation posed by the abundance of fossil fuels. Climate Policy 19 (2), 258–274. doi: 10.1080/14693062.2018.1483885
Li S., Wang K., Wang D., Akamatsu T. (2005). Echolocation signals of the free-ranging Yangtze finless porpoise (Neophocaena phocaenoides asiaeorientialis). J. Acoustical Soc. America 117 (5), 3288–3296. doi: 10.1121/1.1882945
Lin W., Karczmarski L., Li J., Chan S. C., Guo L., Wu Y. (2019). Differential population dynamics of a coastal porpoise correspond to the fishing effort in a large estuarine system. Aquat. Conservation: Mar. Freshw. Ecosyst. 29 (2), 223–234. doi: 10.1002/aqc.2998
Madsen P. T., Wahlberg M., Tougaard J., Lucke K., Tyack P. (2006). Wind turbine underwater noise and marine mammals: implications of current knowledge and data needs. Mar. Ecol. Prog. Ser. 309, 279–295. doi: 10.3354/meps309279
Matuschek R., Betke K. (2009). “Measurements of construction noise during pile driving of offshore research platforms and wind farms,” in Proc. NAG/DAGA int. conference on acoustics (Rotterdam, Netherlands) 262–265.
Munger L., Lammers M. O., Cifuentes M., Würsig B., Jefferson T. A., Hung S. K. (2016). Indo-pacific humpback dolphin occurrence north of lantau island, Hong Kong, based on year-round passive acoustic monitoring. J. Acoustical Soc. America 140 (4), 2754–2765. doi: 10.1121/1.4963874
Munger L., Piwetz S., Lammers M. O., Wursig B. (2018). Passive acoustic monitoring and concurrent theodolite observations of indo-pacific humpback dolphins (Sousa chinensis) in Hong Kong: a case study. Aquat. Mammals 44 (6), 729–735. doi: 10.1578/am.44.6.2018.729
OSPAR Commission (2008). Assessment of the environmental impact of offshore windfarms. OSPAR commission biodiversity series.
Pine M. K., Wang K., Wang D. (2016). “Monitoring rising ambient sound levels from vessels and impacts on indo-pacific humpback dolphin (Sousa chinensis) occurrences,” in Proceedings of meetings on acoustics 4ENAL: acoustical society of America (Dublin, Ireland), vol. 27. 070003.
Pine M. K., Wang K., Wang D. (2017). Fine-scale habitat use in indo-pacific humpback dolphins, Sousa chinensis, may be more influenced by fish rather than vessels in the pearl river estuary, China, Mar. Mammal Sci. 33 (1), 291–312. doi: 10.1111/mms.12366
Richardson W. J., Greene C. R. Jr., Malme C. I., Thomson D. H. (2013). Marine mammals and noise (San Diego, CA: Academic Press).
Scheidat M., Tougaard J., Brasseur S., Carstensen J., Petel T. V. P., Teilmann J., et al. (2011). Harbour porpoises (Phocoena phocoena) and wind farms: a case study in the Dutch north Sea. Environ. Res. Lett. 6 (2), 025102. doi: 10.1088/1748-9326/6/2/025102
Sharif A., Raza S. A., Ozturk I., Afshan S. (2019). The dynamic relationship of renewable and nonrenewable energy consumption with carbon emission: a global study with the application of heterogeneous panel estimations. Renewable Energy 133, 685–691. doi: 10.1016/j.renene.2018.10.052
Sousa-Lima R. S., Norris T. F., Oswald J. N., Fernandes D. P. (2013). A review and inventory of fixed autonomous recorders for passive acoustic monitoring of marine mammals. Aquat. Mammals 39 (1), 23–53. doi: 10.1578/AM.39.1.2013.23
Southall B. L., Finneran J. J., Reichmuth C., Nachtigall P. E., Ketten D. R., Bowles A. E., et al. (2019). Marine mammal noise exposure criteria: updated scientific recommendations for residual hearing effects. Aquat. Mammals 45 (2), 125–232. doi: 10.1578/AM.45.2.2019.125
Thompson P. M., Lusseau D., Barton T., Simmons D., Rusin J., Bailey H. (2010). Assessing the responses of coastal cetaceans to the construction of offshore wind turbines. Mar. Pollut. Bull. 60 (8), 1200–1208. doi: 10.1016/j.marpolbul.2010.03.030
Thomsen F., Lüdemann K., Kafemann R., Piper W. (2006). “Effects of offshore wind farm noise on marine mammals and fish,” in Biola, Hamburg, Germany on behalf of COWRIE Ltd (Biola, Hamburg, Germany on behalf of COWRIE Ltd), 62.
Tougaard J., Carstensen J., Teilmann J., Skov H., Rasmussen P. (2009). Pile driving zone of responsiveness extends beyond 20 km for harbor porpoises (Phocoena phocoena (L.)). J. Acoustical Soc. America 126 (1), 11–14. doi: 10.1121/1.3132523
Tyack P. L. (2008). Implications for marine mammals of Large-scale changes in the marine acoustic environment. J. Mammal 89 (3), 549–558. doi: 10.1644/07-MAMM-S-307R.1
Wang Z.-T., Nachtigall P. E., Akamatsu T., Wang K.-X., Wu Y.-P., Liu J.-C., et al. (2015). Passive acoustic monitoring the diel, lunar, seasonal and tidal patterns in the biosonar activity of the indo-pacific humpback dolphins (Sousa chinensis) in the pearl river estuary, China. PloS One 10 (11), e0141807. doi: 10.1371/journal.pone.0141807
Wang J., Reeves R. (2017). “Neophocaena phocaenoides,” in IUCN red list of threatened species 2017-2013.
Weilgart L. S. (2007a). A brief review of known effects of noise on marine mammals. Int. J. Comp. Psychol. 20 (2), 159–168. doi: 10.46867/IJCP.2007.20.02.09
Weilgart L. S. (2007b). The impacts of anthropogenic ocean noise on cetaceans and implications for management. Can. J. Zool 85 (11), 1091–1116. doi: 10.1139/Z07-101
White W., Lunnan A., Nybakk E., Kulisic B. (2013). The role of governments in renewable energy: the importance of policy consistency. Biomass Bioenergy 57, 97–105. doi: 10.1016/j.biombioe.2012.12.035
Yang J., Liu Q., Li X., Cui X. (2017). Overview of wind power in China: status and future. Sustainability 9 (8), 1454. doi: 10.3390/su9081454
Keywords: off shore wind farm, pile driving noise, finless porpoise, passive acoustic monitoring, habitat
Citation: Fang L, Li M, Wang X, Chen Y and Chen T (2023) Indo-Pacific finless porpoises presence in response to pile driving on the Jinwan Offshore Wind Farm, China. Front. Mar. Sci. 10:1005374. doi: 10.3389/fmars.2023.1005374
Received: 28 July 2022; Accepted: 05 June 2023;
Published: 19 June 2023.
Edited by:
Lijun Dong, Chinese Academy of Sciences (CAS), ChinaReviewed by:
Chongwei Peng, Beibu Gulf University, ChinaZhongchang Song, Xiamen University, China
Ghulam Nabi, Hebei Normal University, China
Copyright © 2023 Fang, Li, Wang, Chen and Chen. This is an open-access article distributed under the terms of the Creative Commons Attribution License (CC BY). The use, distribution or reproduction in other forums is permitted, provided the original author(s) and the copyright owner(s) are credited and that the original publication in this journal is cited, in accordance with accepted academic practice. No use, distribution or reproduction is permitted which does not comply with these terms.
*Correspondence: Tao Chen, chent@scsfri.ac.cn