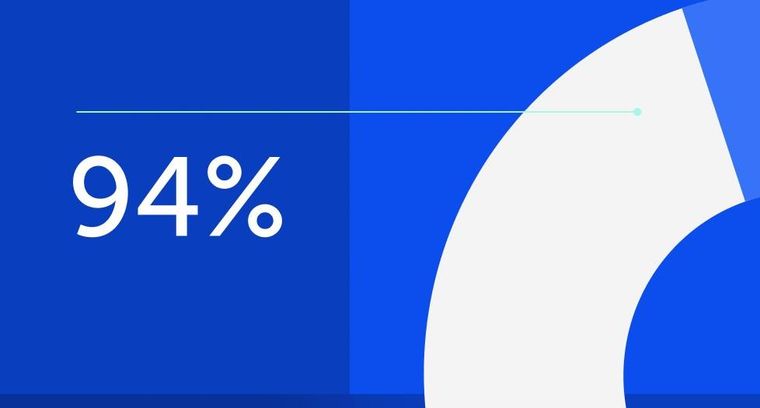
94% of researchers rate our articles as excellent or good
Learn more about the work of our research integrity team to safeguard the quality of each article we publish.
Find out more
REVIEW article
Front. Mar. Sci., 16 September 2022
Sec. Marine Conservation and Sustainability
Volume 9 - 2022 | https://doi.org/10.3389/fmars.2022.996263
The increases in chemical shipping volumes increase the risk of hazardous noxious substances (HNSs) spills at sea. As one of the most frequently transported HNSs, acrylonitrile (ACN) spill incidents occur from time to time. The spilled ACN will have a wide range of short-term and long-term impacts on the marine environment. To reasonably standardise and optimise the emergency response countermeasures for ACN spill and maintain marine ecological health, the marine ecological hazards and physico-chemical behaviours of ACN were summarised. Based on this, the emergency response countermeasures for ACN spill at sea were studied and summarised in five aspects: spill source control, rapid prediction, emergency monitoring, numerical simulation and impact assessment, and elimination of ACN. This review forms a set of systematic emergency response countermeasures for deployment in ACN maritime spill incidents.
With the rapid development of the chemical industry, global demand for various chemicals is increasing, and maritime transportation provides a low-cost solution for long-distance and large-scale chemical transportation (Cóccola and Mendez, 2013; Harold et al., 2014). The United Nations Conference on Trade and Development (UNCTAD) reported that the global maritime trade volume reached 11.08 billion tons, and there were more than 5000 types of chemical shipped (UNCTAD (United Nations Conference on Trade and Development), 2020). However, the increase of chemical shipping volume increases the risk of hazardous noxious substance (HNS) spills at sea (Cunha et al., 2015). International Maritime Organisation (IMO) defines HNS as “any substance other than oil that, if HNS is introduced into the marine environment, it is likely to create hazards to human health, to harm living resources and marine life, to damage amenities or to interfere with other legitimate uses of the sea” (IMO (International Maritime Organisation), 2000). Spilled HNS will have a wide range of short-term and long-term effects on the marine environment (Uherek et al., 2010; Neuparth et al., 2012). To control and eliminate spilled HNS at sea and reduce the severity of damage to the marine environment and ecology, targeted emergency response countermeasures must be taken (Kirby and Law, 2010). Since the end of last century, a series of HNS spill incidents [e.g. Aegean Sea 1992, Sea Empress 1996, Ievoli Sun 2000, Prestige, 2002, MSC Napoli, 2007, etc. (Kirby and Law, 2010; Neuparth et al., 2011; Neuparth et al., 2013; Häkkinen and Posti, 2014; Cunha et al., 2015)] have sparked more attention to improving the prevention and response capabilities of HNS spill accidents (Neuparth et al., 2011; Neuparth et al., 2013). Therefore, the world began to develop emergency response plan to deal with the threat of HNS spill. For example, IMO formulated the Protocol on Preparedness, Response and Co-operation to Pollution Incidents by Hazardous and Noxious Substances in 2000 (IMO/UNEP, 2000), and the Helsinki Commission (HELCOM) jointly established by European countries issued the HELCOM Response Manual in 2002 (HCBMEPC (Helsinki Commission Baltic Marine Environment Protection Commission), 2002). An emergency response is the key to prevention of further expansion of the impact of accidents and reduce losses accruing therefrom. However, HNS spill emergency responses cannot be the same since these chemicals have an assortment of possible behaviours and fates (ITOPF (The International Tanker Owners Pollution Federation Limited), 2014; Rocha et al., 2016).
Acrylonitrile (ACN, C3H3N), an HNS, can be used in the production of plastics and rubbers (Barza et al., 2018; Rezaie et al., 2020). In addition, ACN can also produce carbon fibres for aerospace and defence applications (Rezaie et al., 2020). In recent years, the production capacity of ACN has increased steadily, exceeding 5.0 million tons per annum (Guo et al., 2018). ACN is usually transported by sea, occasionally leading to its marine spillage. For example, the Alessandro Primo spilled 550 tons of ACN in the Adriatic Sea (Mamaca et al., 2009). ACN has been proved to be highly toxic, carcinogenic, mutagenic, immunotoxic, embryotoxic, and neurotoxic (Zheng et al., 2018; Kumar et al., 2020), and will cause great damage to the marine ecological environment once spilled at sea. In addition, ACN has complex environmental behavior in marine environment, that is, the chemical can be dissolved in sea water and evaporated into the air. The present work was designed to establish emergency response countermeasures for ACN spill incidents according to the marine ecological hazards and behaviour identification of ACN and provide scientific advice for a more effective response to ACN spills. Countermeasures include spill source control, rapid prediction, emergency monitoring, numerical simulation and impact assessment, and elimination.
In addition to environmental conditions, the impact of chemicals spilled at sea depends upon their toxicity, the sensitivity of the organisms to specific chemicals and the length of exposure time (ITOPF (The International Tanker Owners Pollution Federation Limited), 2014). Due to the large marine transport volume and high water-solubility of ACN, the ecological risks associated with marine accidental spillage of ACN are considerable (Neuparth et al., 2013). ACN has acute and chronic toxicity to different marine plants and animal taxa (Zheng et al., 2018), and has been classified as an U.S. Environmental Protection Agency (EPA) priority pollutant (Keith and Telliard, 1979). The Joint Group of Experts on the Scientific Aspects of Marine Environmental Protection (GESAMP) was established to provide scientific advice on the prevention, reduction and control of marine environmental degradation (GESAMP, 2014). GESAMP described the risks posed by the most frequently transported chemicals through the operational discharge of ships, accidental spilling or falling into the marine environment, and determined the hazard characteristics of eachchemical (GESAMP, 2016). According to GESAMP, the evaluation results for ACN are listed in Table 1. ACN has adverse effects in five aspects: bioaccumulation and biodegradation, aquatic toxicity, acute mammalian toxicity, irritation/corrosion/long-term health effects, and interference with other uses of sea. The reported toxicological end-points of ACN to marine organisms are compiled in Table 2. The toxicity of ACN to marine organisms is quite lacking. According to the GESAMP acute toxicity classification scheme for aquatic organisms, ACN showed low toxicity (10< LC50 or EC50 ≤ 100) or moderate toxicity (1< LC50 or EC50 ≤ 10) to marine organisms listed in Table 2, which is consistent with the assessment result in Table 1 (moderately acute aquatic toxicity). It has been reported that ACN can stimulate the antioxidant defense system, leading to oxidative damage, and significantly increase the levels of DNA damage in some aquatic organisms (Neuparth et al., 2013; Lin et al., 2018).
Table 2 Toxicological end-points for marine organisms exposed to acrylonitrile. (Supplement from Rocha et al., 2016).
In response to HNS spill incidents, the focus of emergency response is to identify all chemicals involved, pay attention to their mode of transportation (bulk, container, pallet cargo, drums, etc.) and the nature of spill or discharge (e.g. leaked chemicals, lost packaged dangerous goods) (ITOPF (The International Tanker Owners Pollution Federation Limited), 2014). For spilled HNSs in bulk, their environmental behaviour and marine ecological risk depend on external factors such as meteorological conditions and local topography, and internal factors such as the physico-chemical properties of HNSs (ITOPF (The International Tanker Owners Pollution Federation Limited), 2014; Rocha et al., 2016). It is essential to determine the physico-chemical properties and environmental behaviour of the HNS for the correct implementation of any emergency response. Due to the different properties of different HNSs, it may show a variety of behaviours such as dissolution, evaporation, floating, or sinking after spillage at sea (Neuparth et al., 2011). Standard European Behaviour Classification (SEBC) groups contained in the Bonn Agreement Counter Pollution Manual classifies HNSs according to their physical state and physical properties when spilled at sea (solubility, density, vapour pressure) (Bonn Agreement, 1994) and comprises 12 Groups (G/E (gas/evaporator), F (floater), D (dissolver), S (sinker), GD (gas and dissolver), ED (evaporator and dissolver), FE (floater and evaporator), FED (floater, evaporator and dissolver), FD (floater and dissolver), DE (dissolver and evaporator), SD (sinker and dissolver)) for bulk HNSs and three groups for package (PF (package floater), PI (package immerser), and PS (package sinker)) (Figure 1).
Figure 1 SEBC classification of HNS spills at sea. (adopted from the Bonn Agreement, 1994).
SEBC criteria are critical for emergency response professionals because they can be used to identify the major components of the ecosystem that will be affected in an emergency. Dissolvers and sinkers are listed as HNSs for priority disposal considering their highest ecological risk on the marine environment (Neuparth et al., 2011). Table 3 displays the solubility, density, vapour pressure and other physico-chemical characteristics of ACN, which determine their behaviour and persistence in the environment. According to the SEBC criteria, the physical behaviour of spilled ACN belongs to the DE Group, that is, the chemical can be dissolved in sea water and evaporated into the air (Figure 2). In addition, ACN has active chemical properties and readily reacts violently with reducing agents and releases toxic gas. ACN readily polymerises under hypoxia or exposure to visible light and can polymerise strongly under concentrated alkali conditions. When ACN steam is mixed with air, it readily forms explosive mixtures, which react strongly with oxidants, and will cause combustion and explosion in case of open fire and high heat. For ACN which belongs to the DE Group, the main emergency response countermeasures include spill source control, rapid prediction, emergency monitoring, numerical simulation, and elimination of ACN, note that emergency response countermeasures at sea and in the air should be carried out at the same time (Figure 2).
To avoid environmental damage caused by HNS spillage, it is necessary to identify the spill source in the shortest time and adopt different methods to quickly cut off the pollution source according to its release mode to reduce the amount of HNS entering the sea (Kirby et al., 2014). The location of ACN spill source can be divided into land tank area, dock port area, and shipping channel. Different spill source blocking measures should be taken in different areas. It should be noted that any action to block the source of the spillage should be conducted under the premise of ensuring personal safety (ITOPF (The International Tanker Owners Pollution Federation Limited), 2014).
The main causes of ACN spilled in land tank area are cracks in weld seams and corrosion cracking of the tank body. According to the different parts of spillage, the corresponding plugging method should be adopted. The plugging methods to cut off the continuous spill include adjusting the gap, mechanical plugging (plugging with the mechanical deformation of the sealing layer), air cushion plugging (using an air cushion or air bag fixed at the spill port to compress the spill port through the inflation pressure), sealant sealing (using the sealant to form a sealing layer at the spill port for plugging), magnetic pressure plugging (use the strong magnetic force of the magnet to press the gasket or sealant on the spill port of the equipment), etc. ACN may spill into harbour waters due to improper handling by dock and ship personnel during quayside loading and unloading operations. In case of a spill, the relevant valves shall be closed immediately, the connected equipment and pipelines shall be cut off, the operation shall be stopped, or the ACN spillage shall be prevented by changing the process flow. In circumstances where the spill cannot be stopped, the ACN should be pumped to another container. Various pump systems made of chemical-resistant materials can be used to deliver ACNs (e.g. centrifugal, submersible pumps). According to the statistics of international HNS marine spill cases in the past 50 years, human factors (ship collision, ship grounding/hitting a reef, fire/explosion, hull structure damage) are the main causes of channel ship spill accidents (Luo and Shin, 2019), and the consequence is that bulk carriers capsize or even sink, in which the chemicals carried are directly spilled at sea (Roberts et al., 2014). For such spillages, measures shall be taken to plug and repair the cracks according to the actual situation to stop further consequence. It is also possible to restrict the further outflow of ACN in sensitive areas and reduce other hazards by changing the location of the spill source (towing the vessel to a safe area).
After an ACN spill, the risk of fire and explosion, as well as risks to adjacent areas must be assessed quickly. There are various computer models available that can elaborate a forecast of the spill’s future fate. In cases where the simulation model is not available, where it takes a long time to run, or where monitoring is not formally implemented, Hazardous & Noxious Substances Response Operation Guidelines prepared by United Nations Environment Programme (UNEP) and IMO provides quick and rough estimation methods to help emergency response personnel make rapid predictions of ACN migration and diffusion (IMO/UNEP, 2009).
After dissolving in seawater, the main diffusion direction of ACN is consistent with the direction of seawater flow, and it is distributed as a pyramid in three-dimensional space along the direction of the ocean current (Figure 3). Table 4 lists the corresponding relationship between the concentration of ACN with different spillages in water and its migration distance. When the ACN spill is between the quantities listed in Table 4, the interpolation method can be used. The concentration distribution of ACN in three-dimensional space can be quickly predicted combined with the distribution (orientation, distance) of coastal and ecologically sensitive areas near the spill point, as well as the flow direction and velocity of seawater, the time and concentration of chemical migration and diffusion to these areas can be estimated. Based on this, an early warning will be sent to the coast or ecologically sensitive areas that may be affected, so that evacuation, isolation, and other measures can be taken timeously.
Figure 3 Schematic representation of ACN migration in seawater. (adopted from IMO/UNEP, 2009).
Table 4 The concentrations of ACN with different spills in water and its migration distance. (adopted from IMO/UNEP, 2009).
ACN, which is volatilised and diffused in the air, poses a danger to people downwind. The distance of health risk and explosion risk caused by volatile gas cloud formed by ACN can be roughly calculated by use of Eq. (1). Based on the assessment, the risk area can be delineated and personnel evacuated to a position upwind of the leak.
Where: A represents the value (health risk or explosion risk) in Table 5 (m). Vp represents the vapor pressure of ACN (kPa) (11 kPa at 20 °C).
Table 5 Estimated extents of the health risk and explosion risk in the downwind direction for different quantities of gases spilled. (adopted from IMO/UNEP, 2009).
Once leaked into the marine environment, HNSs have a wide range of potential fate and environmental behaviour. Emergency monitoring shall run through the whole process of emergency response, to grasp the distribution and severity of pollution in the marine environment at different stages after the spill accident. There are essential differences between post-incident emergency monitoring and general environmental monitoring. Emergency monitoring is aimed at a specific purpose and requires the full preparation and participation of all departments and disciplines. Rapid deployment is crucial for post-spill emergency monitoring. The type, concentration, pollution range, rate of diffusion, and hazard posed by pollutants should be judged and measured in the shortest time, to provide a scientific basis for correct decision-making. In addition, specific factors such as the specific chemicals spilled and the receiving marine environment and ecological risks need to be considered when formulating the plan (Kirby and Law, 2010). The available information on HNS spills illustrates that many HNS accidents have incomplete records or no monitoring programs (Laffon et al., 2006).
At present, some developed countries have established monitoring organisations for HNS post-spill monitoring (Fuhrer et al., 2012; Neuparth et al., 2012; Radovic et al., 2012; Lee and Jung, 2013). The UK has launched an inter-governmental project called PREMIAM (Pollution Response in Emergencies: Marine Impact Assessment and Monitoring). The US National Incident Management System provides the Incident Command System (ICS) to organise operations of all levels of government during the incident response (Weidhaas et al., 2016). British scholars proposed a “monitoring and preparedness assessment” method. The overall monitoring and preparedness assessment score (MPAs) obtained can be used as a tool to assess the monitoring readiness and capacity under specific scenarios and point out areas for improvement (Kirby et al., 2014).
Emergency monitoring of ACN spilled at sea mainly includes physical parameter monitoring, chemical parameter monitoring, and ecological parameter monitoring. Sea conditions and weather conditions are the main constraints on the effectiveness of emergency response measures to ACN spilled at sea. Physical parameters such as ocean current (flow direction and velocity), ocean wave (wave height, period and direction), wind (sea surface wind direction and speed) and cloud (total cloud cover, low cloud cover) and precipitation affect the migration and attenuation of ACN in the ocean. Remote sensing monitoring, underwater robots and other advanced technologies have been used in HNS post-spill emergency monitoring (Roemmich et al., 2009; Grimaldi et al., 2011). The application of new technology will provide a strong boost to emergency monitoring capabilities. The monitoring data pertaining to physical parameters can also provide an immediate and accurate basis for the correction of numerical simulation results.
ACN is dissolved into seawater after spillage at sea. Therefore, a variety of chemical parameters of seawater around the spill point should be regularly monitored after the accident. The sensor-based detector can be used to measure inorganic parameters in the accident sea area, such as biological oxygen demand (BOD), turbidity, conductivity, pH value, ammonium ion, bromide ion, and chlorine ion concentrations. The concentration of ACN can be monitored by portable gas chromatography (GC), mass spectrometry connected with gas chromatography (portable GCMS), titration or ultraviolet (UV)/infrared (IR) spectroscopy (ITOPF (The International Tanker Owners Pollution Federation Limited), 2014). At the initial stage of emergency response, according to the rapid prediction results of ACN migration and diffusion in seawater, the monitoring stations are of dense-to-sparse arrangement from the spill source to downstream. To reflect the vertical distribution of ACN, each station shall be set with three points vertically: surface layer (0.5 m below the water surface), middle layer and bottom layer (within 0.5 m depth from the seabed). If the seawater depth in the area of the accident is greater than 10 m, the vertical sampling layer can be appropriately increased. If there are ecologically sensitive areas downstream of the spill point, monitoring stations must be set in these areas. Ideally, it should be monitored once every 6 h within 1-3 months after the accident. Thereafter, the monitoring frequency is gradually reduced until the measured value is close to the level before the incident. In the middle and late stages of the emergency response, the monitoring range can be adjusted appropriately according to the output from numerical simulations. ACN does not belong to the S (sinker) group, so it is not necessary to monitor marine sediments.
ACN can persist for two weeks in water with a half-life of 30-552 h (Rocha et al., 2016). The effect of ACN and its intermediate products on marine ecology cannot be ignored in case of a large-volume spill (De Smedt et al., 2014). Based on the physical behaviour of ACN, the ecological indicators of fish, shrimp, and intertidal benthos should be monitored in the ACN spill area. For a certain station, the effect of spilled ACN on marine ecology can be evaluated by comparing the species composition, catch (or density) and biodiversity of fish, shrimp, and intertidal benthos at different times after the accident. In addition, physiological and biochemical indicators indicating possible changes in marine organisms should be included in comprehensive monitoring of biological effects, which is an effective method with which to judge the health of species and aquatic systems (Connon et al., 2012; Vieira et al., 2017). The assessment of multiple biomarkers in the leaking sea area reflects the overall impact of the ACN on the marine ecosystem and provides important information for estimating the health status of species, ecosystem integrity, and site restoration/restoration (Rodrigues et al., 2014). Owing to the ease of use, inexpensive, and compact, microbial-derived biosensors have been successfully applied in environmental monitoring (Bilal and Iqbal, 2019).
It is important to monitor the concentration of ACN that is a volatile chemical in the air of the spill accident area. At present, there are various items of precision equipment available for air diffusion monitoring of HNSs, such as chemical detection paper (changes colour upon exposure to an HNS, according to the type of HNS), colorimetric tubes (160 substance-specific reagent tubes are available to identify different HNS), photo-lonisation detector, ion mobility spectroscopy, and infrared spectroscopy (ITOPF (The International Tanker Owners Pollution Federation Limited), 2014). Real time monitoring can be used to assess toxic risks and fire/explosion hazards, to help identify safe work areas or possible evacuation areas, and to determine the level of personal protective equipment for emergency response personnel. According to Occupational Safety and Health Administration (OSHA) Standards, the employer shall assure that no employee is exposed to an airborne concentration of ACN in excess of 2 ppm as an 8-hour time-weighted average (https://www.ecfr.gov). In addition, the monitoring results can test whether the risk range estimated based on the predictive model is appropriate. Key factors to be considered in selecting instruments and equipment include response time, portability, water resistance, etc. (ITOPF (The International Tanker Owners Pollution Federation Limited), 2014).
Although the ocean has considerable ability to assimilate pollutants and can show a high degree of elasticity through re-colonisation and recovery, the threat of HNS accidental spills on the ocean is significant and long-term, in terms of both catastrophic release and environmental damage and its unpredictability (Guterman, 2009). Therefore, effective post-spill simulation and impact assessment procedures need to be implemented (Kirby et al., 2014). Early maritime accident research methods are very limited, the major methods of which are case studies, descriptive reviews, and probability calculations (Talley et al., 2012; Luo and Shin, 2019). With the development of science and technology, numerical simulation estimation methods and other multidisciplinary methods have been used to more accurately predict the diffusion and impact of spilled HNS. (Goerlandt and Kujala, 2011; Faghih-Roohi et al., 2014). There are hundreds of highly sophisticated forecasting/modelling systems for prediction of the diffusion of HNSs. Modelling systems suitable for diffusion simulation and effect prediction of ACN include: ALOHA (https://www.epa.gov/cameo/aloha-software), CAFÉ (https://cafe.orr.noaa.gov/), CHEMMAP (French McCay et al., 2006), MOHID (Soares et al., 2020), MAMPEC (Kim et al., 2019), SMIS (Martin et al., 2004), PHAST (Wang et al., 2017), EPIcode (Thoman et al., 2006), etc. Due to the complexity of the actual situation and the limitations of the model algorithm and source code, different models have different application scenarios and advantages and disadvantages. For the purpose of exercise, ALOHA and CAFÉ models were used to predict the airborne diffusion and aquatic effect of ACN in maritime accident. The above two models are free, easy to operate and quick to respond.
ALOHA (Area Locations of Hazardous Atmospheres) is developed jointly by the National Oceanic and Atmospheric Administration (NOAA) and the EPA to model HNSs airborne diffusion for emergency responders (NOAA, 2014). It includes a database of nearly 1000 commonly used chemicals, and its key program features include chemical types, accident locations, weather conditions (temperature, wind speed and direction) and spill source conditions (storage materials, container shape and capacity, spill hole size, storage pressure), etc. Through the detailed information about the spill entered by the user, ALOHA evaluates the threat areas and levels of various types of risks, and displays them in a grid (NOAA, 2011). The classification of hazardous areas of chemical accidents (also known as the boundary control standard) is a measurement standard for the division of hazardous areas after such accidents. ALOHA has four built-in hazard levels to calculate the regional scope of various hazards: toxic level of concern, flammable level of concern, thermal radiation level of concern, and overpressure level of concern (NOAA, 2007; NAC/AEGL Meeting, 2019). ALOHA software divides the prediction results into red, orange, and yellow areas according to the hazard posed (the red area represents the area with the most serious hazard, and orange and yellow represent the area with reduced hazard in turn) (NOAA, 2011). ALOHA has become an important tool for HNS emergency rescue, planning and academic research, and is widely used in risk assessment and emergency decision-making (O’Mahony et al., 2008).
An ACN spill incident in Italy in 1991 was used to demonstrate the practical application of ALOHA. The assumptions about the details of spill are described as follows: The ACN is leaking out of a 500-gallon (2273 L), 4-foot-diameter (1219 mm), vertical tank through a 6-inch (152 mm) circular hole located 10 inches (254 mm) above the bottom of the tank. The prediction shows that most of the spilled ACN is dissolved in seawater. ALOHA estimates that the orange threat zone will extend 1308 yards (1196 m) downwind (Figures 4A, B). At concentrations above the Emergency Response Planning Guidelines (ERPG)-2 level (orange threat zones), people could experience serious health effects or find their ability to escape to be impaired (if they are exposed for about an hour). The red (ERPG-3 level) and yellow (ERPG-1 level) threat zones will extend 865 yards (791 m) and 1.5 miles (2.4 km), respectively. Unlike the toxic threat, if the spilled ACN is burning and forms a pool fire, the thermal radiation threat extends in all directions simultaneously. ALOHA estimates that the orange threat zone will extend 41 yards (38 m) in the downwind direction, and about 26 yards (24 m) in the upwind direction (Figures 4C, D). The red and yellow threat zones will extend 30 yards (27 m) and 61 yards (56 m) in the downwind direction, respectively.
Figure 4 Prediction results of ALOHA. Plots show the predicted averaged release rate (A) and the toxic threat zones (B) if the ACN is not burning and forms an evaporating puddle, and the predicted averaged burn rate (C) and thermal radiation threat zones (D) if the ACN is burning and forms a pool fire.
The CAFÉ (Chemical Aquatic Fate and Effects) database aims to provide time-sensitive information to help emergency response personnel assess the environmental impact when a chemical or oil spill into water. CAFE is presented in two modules: The aquatic fate module, and the aquatic effect module. The aquatic fate module contains property data for more than 30,000 chemicals, which is helpful to understand and predict the chemical behaviour in the aquatic environment. The aquatic effect module more than 100,000 aquatic toxicity records for more than 3600 chemicals to help characterize the potential risk to the aquatic community through the Species Sensitivity Distribution curves (SSD). Toxicity data are summarised in the form of SSDs with associated first and fifth percentile hazard concentrations (HC1 and HC5) (Bejarano and Farr, 2013; Bejarano et al., 2016).
In the case of an ACN spill, CAFÉ predicted that ACN may slowly volatilise from water surfaces based on a Henry’s Law constant of 0.000138 atm m3/mole and is not expected to adsorb to suspended solids and sediment based upon a KOC of 8.511. The 24 h, 48 h, and 96 h-HC5 of ACN calculated by the aquatic effect module of CAFÉ are 12 mg/L, 6.7 mg/L, and 3.9 mg/L, respectively, and the 24 h, 48 h, and 96 h-HC1 are 6.7 mg/L, 3.6 mg/L, and 2 mg/L, respectively (Figure 5). Bejarano et al. (2016) use an incident in the Mississippi River in 2010 to demonstrate the application of CAFÉ in emergency response. They assumed that 625 barrels (c. 100 kL) of ACN had leaked into a river 600 m wide, 18 m deep, with a volume flow rate of 20,000 m3/s. Simulation results showed that the ACN concentration within 180 m of the source of the leak was 4520 mg/L in the first 10 min and rapidly decreased to less than 1 mg/L within 2 h. Comparisons of the ACN concentrations to 24h-HC5 suggested that most aquatic species may be potentially affected in the first 35 min after the accident occurred, with modelled environmental concentrations falling below the HC5 after 45 min.
In the actual emergency response, using various methods to accelerate the natural dissipation and dilution process may be the main way to deal with the spill of dissolved and volatile HNS chemicals such as ACN. ACN volatilised into the air or dissolved into seawater cannot be recovered. Generally, according to its transmission characteristics, spray dilution or strong dispersion can be used to reduce the concentration of toxic or combustible gases on site. Water-gun spray and other equipment can also dissolve ACN gas to a certain extent. At the same time, to reduce the rate of volatilisation of ACN, materials such as foam can be used to cover the spilled ACN and inhibit their volatilisation (Häkkinen and Posti, 2014). However, the above natural dilution method is inefficient, costly, and readily causes damage to the surrounding ecosystem. In view of the harm caused by spilled ACN to the environment, human activities, property losses and social stability, artificial enhanced degradation methods of ACN should be actively explored. For example, redox agents, ion exchangers, gels, and neutralisers were used to react with spilled ACN for oxidation-reduction or acid-base neutralisation (Shin et al., 2009; Guo et al., 2010; Popuri et al., 2011; Kumar et al., 2020). Compared with chemical methods, biological methods have the advantages of mild reaction conditions, low treatment cost, stable and continuous operation, and no secondary pollution. Some microorganisms can grow under natural marine conditions and use ACN as an organic carbon or nitrogen source to achieve long-term effective elimination of ACN (Sahu et al., 2019). The microbial metabolism of nitriles degradation mainly through nitrilase (EC 3.5.5.1) and nitrile hydratase (EC 4.2.1.84) (Dennett and Blamey, 2016; Prejano et al., 2017). Strains that have been found to synthesise the above two enzymes include Rhodococcus, Pseudomonas, Nocardia, Pseudonocardia, Alcaligenes, Corynebacterium, etc. (Mukram et al., 2015; Sahu et al., 2019). The degradation of cyanides by nitrilase and nitrile hydrolase has been determined (Cantarella et al., 2013). Under the premise of not affecting the original ecosystem, it is appropriate to consider introducing biological methods to purify ACN in an emergency response. However, it should be noted that the biodegradation of HNSs in seawater by biotechnology is mainly successful in the laboratory, and its application and its efficacy should be further assessed in the sea areas suffering such accidents.
ACN is transported at sea in large volumes; spills thereof cause strong ecological harm to the sea, and it has active physico-chemical properties. The spilled ACN can not only dissolve in seawater, but also volatilise into the air, resulting in the complex emergency response countermeasures for ACN spill incidents. Selection of the appropriate response countermeasures requires quick, simple, and comprehensive knowledge on the ecotoxicological and physico-chemical properties of the substance involved. Based on the full analysis of the marine ecological risks and physico-chemical behaviours of ACN, the emergency response countermeasures for ACN spill at sea were summarised and optimised. However, due to the different environmental conditions of the accident, the actual situation of chemical spill is very complex, so it is necessary to improve the emergency plan based on repeated drills for ACN spill accidents. For hundreds of pollutant diffusion models, the application conditions of various models are independent of each other. Developing global and high-performance diffusion simulation software is the focus of future research. After a spill incident, biological elimination of ACN at sea is a more efficient and environmentally friendly choice. At present, it has been found that a variety of bacteria containing nitrile hydratase and nitrile hydrolase can purify ACN, but whether these microorganisms are suitable for the accident scene has not been reported. The future research direction is to screen out microorganisms that can be used for efficient degradation of ACN in seawater through many studies, and to discuss its practical application effect.
XW drafted the manuscript. All authors contributed significantly to improve the manuscript by intensive discussions and substantial additions and revisions of text passages.
This work was financially supported by the National Natural Science Foundation of China (No. 42077335), and the National Marine Hazard Mitigation Service, Ministry of Natural Resources of the People’s Republic of China (No. 2016AA024).
The authors declare that the research was conducted in the absence of any commercial or financial relationships that could be construed as a potential conflict of interest.
All claims expressed in this article are solely those of the authors and do not necessarily represent those of their affiliated organizations, or those of the publisher, the editors and the reviewers. Any product that may be evaluated in this article, or claim that may be made by its manufacturer, is not guaranteed or endorsed by the publisher.
ANGroup (1997). Acrylonitrile: marina alga growth inhibition test (72 hr, EC50) (Tranent, Scotland: Inveresk Research). EU RAR.
Barza A., Mehri B., Pirouzfar V. (2018). Mathematical modeling of ethane cracking furnace of olefin plant with Coke formation approach. Int. J. Chem. React. Eng. 16 (9), 20170243. doi: 10.1515/ijcre-2017-0243
Bejarano A. C., Farr J. K. (2013). Development of short, acute exposure hazard estimates: a tool for assessing the effects of chemical spills in aquatic environments. Environ. Toxicol. Chem. 32 (8), 1918–1927. doi: 10.1002/etc.2255
Bejarano A. C., Farr J. K., Jenne P., Chu V., Hielscher A. (2016). The chemical aquatic fate and effects database (CAFE), a tool that supports assessments of chemical spills in aquatic environments. Environ. Toxicol. Chem. 35 (6), 1576–1586. doi: 10.1002/etc.3289
Bilal M., Iqbal H. M. N. (2019). Microbial-derived biosensors for monitoring environmental contaminants: Recent advances and future outlook. Process. Saf. Environ. 124, 8–17. doi: 10.1016/j.psep.2019.01.032
Bonn Agreement (1994). European Classification system, Bonn agreement: Counter-pollution manual (London: Bonn Agreement). (Chapter 25).
Cantarella L., Gallifuoco A., Spera A., Cantarella M. (2013). Nitrile, amide and temperature effects on amidase-kinetics during acrylonitrile bioconversion by nitrile-hydratase/amidase in situ cascade system. Bioresour. Technol. 142, 320–328. doi: 10.1016/j.biortech.2013.04.126
Cóccola M. E., Mendez C. A. (2013). Logistics management in maritime transportation systems. 11th. Int. Conf. Chem. Process. 32, 1291–1296. doi: 10.3303/CET1332216
Connon R. E., Geist J., Werner I. (2012). Effect-based tools for monitoring and predicting the ecotoxicological effects of chemicals in the aquatic environment. Sensors. (Basel). 12 (9), 12741–12771. doi: 10.3390/s120912741
Cunha I., Moreira S., Santos M. M. (2015). Review on hazardous and noxious substances (HNS) involved in marine spill incidents-an online database. J. Hazard. Mater. 285, 509–516. doi: 10.1016/j.jhazmat.2014.11.005
Dennett G. V., Blamey J. M. (2016). A new thermophilic nitrilase from an antartic hyperthermophilic microorganism. Front. Bioeng. Biotechnol. 4, 1–9. doi: 10.3389/fbioe.2016.00005
De Smedt T., De Cremer K., Vleminckx C., Fierens S., Mertens B., Van Overmeire I., et al. (2014). Acrylonitrile exposure in the general population following a major train accident in Belgium: a human biomonitoring study. Toxicol. Lett. 231 (3), 344–351. doi: 10.1016/j.toxlet.2014.09.009
EURAR (European Union Risk Assessment Report: Acrylonitrile) (2004). Institute for health and consumer protection Vol. 32 (European Chemicals Bureau, Varese, Italy).
Faghih-Roohi S., Xie M., Ng K. M. (2014). Accident risk assessment in marine transportation via Markov modelling and Markov chain Monte Carlo simulation. Ocean. Eng. 91, 363–370. doi: 10.1016/j.oceaneng.2014.09.029
French McCay D. P., Whittier N., Ward M., Santos C. (2006). Spill hazard evaluation for chemicals shipped in bulk using modeling. Environ. Modell. Software 21 (2), 156–169. doi: 10.1016/j.envsoft.2004.04.021
Fuhrer M., Peron O., Hofer T., Morrissette M., Le Floch S. (2012). Offshore experiments on styrene spillage in marine waters for risk assessment. Mar. pollut. Bull. 64 (7), 1367–1374. doi: 10.1016/j.marpolbul.2012.04.022
GESAMP (2014). Revised GESAMP hazard evaluation procedure for chemical substances carried by ships. 2nd Edition (London: International Maritime Organization).
Goerlandt F., Kujala P. (2011). Traffic simulation based ship collision probability modeling. Reliab. Eng. Syst. Safe. 96 (1), 91–107. doi: 10.1016/j.ress.2010.09.003
Grimaldi C. S. L., Casciello D., Coviello I., Lacava T., Pergola N., Tramutoli V. (2011). An improved RST approach for timely alert and near real time monitoring of oil spill disasters by using AVHRR data. Nat. Hazard. Earth Sys. 11 (5), 1281–1291. doi: 10.5194/nhess-11-1281-2011
Guo Y., Chang H., Wang Q., Shao C., Xu J. (2018). Hydrolytic denitrification and decynidation of acrylonitrile in wastewater with arthrobacter nitroguajacolicus ZJUTB06-99. AMB. Express. 8 (1), 191. doi: 10.1186/s13568-018-0719-8
Guo Y., Wang S., Xu D., Gong Y., Tang X., Zhang J. (2010). Hydrogen production by catalytic supercritical water gasification of nitriles. Int. J. Hydrog. Energy 35 (10), 4474–4483. doi: 10.1016/j.ijhydene.2010.02.108
Guterman L. (2009). Conservation biology. Exxon Valdez turns 20. Science 323 (5921), 1558–1559. doi: 10.1126/science.323.5921.1558
Häkkinen J. M., Posti A. I. (2014). Review of maritime accidents involving chemicals-special focus on the Baltic Sea. TransNav. Int. J. Mar. Navigation. Saf. Sea. Transport. 8 (2), 295–306. doi: 10.12716/1001.08.02.16
Harold P. D., de Souza A. S., Louchart P., Russell D., Brunt H. (2014). Development of a risk-based prioritisation methodology to inform public health emergency planning and preparedness in case of accidental spill at sea of hazardous and noxious substances (HNS). Environ. Int. 72, 157–163. doi: 10.1016/j.envint.2014.05.012
HCBMEPC (Helsinki Commission Baltic Marine Environment Protection Commission) (2002). HELCOM manual on co-operation in response to marine pollution within the framework of the convention on the protection of the marine environment of the Baltic sea area (Finland: Helsinki Convention).
IMO/UNEP (2000). Regional Information System, Part D, Operational Guides and Technical Manuals, Section 11, Practical Guide to Marine Chemical Spills (REMPEC, Malta).
IMO/UNEP (2009). Technical Report No.6. Hazardous & Noxious Substances (HNS) Response Operation Guidelines (Response Operation Guidelines, NOWPAP MERRAC, Japan).
IMO (International Maritime Organisation) (2000) Protocol on preparedness, response and Co-operation to pollution incidents by hazardous and noxious substances, (OPRC-HNS protocol). Available at: www.imo.org.
ITOPF (The International Tanker Owners Pollution Federation Limited) (2014). Technical information papers 17: response to marine chemical incidents (United Kingdom: London EC1Y 1HQ). Available at: http://www.itopf.com/knowledge-resources/documents-guides/document/tip-17-response-to-marine-chemical-incidents/.
Keith L. H., Telliard W. A. (1979). Priority pollutants. i. a perspective view. Environ. Sci. Technol. 13, 416–423. doi: 10.1021/es60152a601
Kim Y. R., Lee M., Jung J. Y., Kim T. W., Kim D. (2019). Initial environmental risk assessment of hazardous and noxious substances (HNS) spill accidents to mitigate its damages. Mar. Pollut. Bull. 139, 205–213. doi: 10.1016/j.marpolbul.2018.12.044
Kirby M. F., Gioia R., Law R. J. (2014). The principles of effective post-spill environmental monitoring in marine environments and their application to preparedness assessment. Mar. Pollut. Bull. 82 (1-2), 11–18. doi: 10.1016/j.marpolbul.2014.01.038
Kirby M. F., Law R. J. (2010). Accidental spills at sea–risk, impact, mitigation and the need for co-ordinated post-incident monitoring. Mar. Pollut. Bull. 60 (6), 797–803. doi: 10.1016/j.marpolbul.2010.03.015
Kumar A., Prasad B., Garg K. K. (2020). Efficiently degradation of acrylonitrile from aqueous solution by La0.5Ce0.5MO3 (M = Fe, Cu and Co) perovskite-like catalyst: Optimization and reaction pathways. J. Water Process. Eng. 36, 101314. doi: 10.1016/j.jwpe.2020.101314
Laffon B., Rabade T., Pasaro E., Mendez J. (2006). Monitoring of the impact of prestige oil spill on mytilus galloprovincialis from Galician coast. Environ. Int. 32 (3), 342–348. doi: 10.1016/j.envint.2005.07.002
Lee M., Jung J. Y. (2013). Risk assessment and national measure plan for oil and HNS spill accidents near Korea. Mar. Pollut. Bull. 73 (1), 339–344. doi: 10.1016/j.marpolbul.2013.05.021
Lin P., Miao J., Pan L., Zheng L., Wang X., Lin Y., et al. (2018). Acute and chronic toxicity effects of acrylonitrile to the juvenile marine flounder paralichthys olivaceus. Environ. Sci. Pollut. Res. Int. 25 (35), 35301–35311. doi: 10.1007/s11356-018-3430-z
Luo M., Shin S. H. (2019). Half-century research developments in maritime accidents: Future directions. Accid. Anal. Prev. 123, 448–460. doi: 10.1016/j.aap.2016.04.010
Mamaca E., Girin M., Floch S., Zir R. (2009). Review of chemical spills at sea and lessons learnt. A Technical Appendix to the INTERSPILL 2009 Conference White Paper “Are HNS spills more dangerous than oil Spills?”. Available at: www.itopf.com
Martin P. H., LeBoeuf E. J., Daniel E. B., Dobbins J. P., Abkowitz M. D. (2004). Development of a GIS-based spill management information system. J. Hazard. Mater. 112 (3), 239–252. doi: 10.1016/j.jhazmat.2004.05.014
Mukram I., Nayak A. S., Kirankumar B., Monisha T. R., Reddy P. V., Karegoudar T. B. (2015). Isolation and identification of a nitrile hydrolyzing bacterium and simultaneous utilization of aromatic and aliphatic nitriles. Int. Biodeterior. Biodegrad. 100, 165–171. doi: 10.1016/j.ibiod.2015.03.002
NAC/AEGL Meeting (2019) Access acute exposure guideline levels (AEGLs) values [EB/OL]. Available at: https://www.epa.gov/aegl/access-acuteexposure-guideline-levels-aegls-values#chemicals.
Neuparth T., Capela R., Rey-Salgueiro L., Moreira S. M., Santos M. M., Reis-Henriques M. A. (2013). Simulation of a hazardous and noxious substances (HNS) spill in the marine environment: lethal and sublethal effects of acrylonitrile to the European seabass. Chemosphere 93 (6), 978–985. doi: 10.1016/j.chemosphere.2013.05.064
Neuparth T., Moreira S., Santos M. M., Reis-Henriques M. A. (2011). Hazardous and noxious substances (HNS) in the marine environment: prioritizing HNS that pose major risk in a European context. Mar. Pollut. Bull. 62 (1), 21–28. doi: 10.1016/j.marpolbul.2010.09.016
Neuparth T., Moreira S. M., Santos M. M., Reis-Henriques M. A. (2012). Review of oil and HNS accidental spills in Europe: identifying major environmental monitoring gaps and drawing priorities. Mar. Pollut. Bull. 64 (6), 1085–1095. doi: 10.1016/j.marpolbul.2012.03.016
NOAA (2007) Acute exposure guideline levels (AEGLs) (AEGLS on 191 chemicals on 12th February 2007). Available at: http://www.epa.gov/oppt/aegl/pubs/chemlist.htm.
NOAA (2011) ALOHA manual [M/OL]. Available at: http://www.epa.gov/oem/content/cameo/aloha.html.
NOAA (2014). ALOHA Arenl Locations of Hazardous Atmosperes user's Manual [EB/OL]. Available at: https://www.epa.gov/cameo/aloha-software.
O’Mahony M. T., Doolan D., O’Sullivan A., Hession M. (2008). Emergency planning and the control of major accident hazards (COMAH/Seveso II) directive: an approach to determine the public safety zone for toxic cloud releases. J. Hazard. Mater. 154 (1-3), 355–365. doi: 10.1016/j.jhazmat.2007.10.065
Popuri S. R., Chang C. Y., Xu J. (2011). A study on different addition approach of fenton’s reagent for DCOD removal from ABS wastewater. Desalination 277 (1-3), 141–146. doi: 10.1016/j.desal.2011.04.017
Prejano M., Marino T., Rizzuto C., Madrid Madrid J. C., Russo N., Toscano M. (2017). Reaction mechanism of low-spin Iron(III)- and Cobalt(III)-containing nitrile hydratases: A quantum mechanics investigation. Inorg. Chem. 56 (21), 13390–13400. doi: 10.1021/acs.inorgchem.7b02121
Radovic J. R., Rial D., Lyons B. P., Harman C., Vinas L., Beiras R., et al. (2012). Post-incident monitoring to evaluate environmental damage from shipping incidents: chemical and biological assessments. J. Environ. Manage. 109, 136–153. doi: 10.1016/j.jenvman.2012.04.042
Rezaie F., Pirouzfar V., Alihosseini A. (2020). Technical and economic analysis of acrylonitrile production from polypropylene. Thermal. Sci. Eng. Prog. 16, 100463. doi: 10.1016/j.tsep.2019.100463
Roberts S. E., Nielsen D., Kotlowski A., Jaremin B. (2014). Fatal accidents and injuries among merchant seafarers worldwide. Occup. Med. (Lond). 64 (4), 259–266. doi: 10.1093/occmed/kqu017
Rocha A. C., Reis-Henriques M. A., Galhano V., Ferreira M., Guimaraes L. (2016). Toxicity of seven priority hazardous and noxious substances (HNSs) to marine organisms: Current status, knowledge gaps and recommendations for future research. Sci. Total. Environ. 542 (Pt A), 728–749. doi: 10.1016/j.scitotenv.2015.10.049
Rodrigues A. P., Oliva-Teles T., Mesquita S. R., Delerue-Matos C., Guimaraes L. (2014). Integrated biomarker responses of an estuarine invertebrate to high abiotic stress and decreased metal contamination. Mar. Enviro. Res. 101, 101–114. doi: 10.1016/j.marenvres.2014.10.001
Roemmich D., Johnson G., Riser S., Davis R., Gilson J., Owens W. B., et al. (2009). The argo program: Observing the global oceans with profiling floats. Oceanography 22 (2), 34–43. doi: 10.5670/oceanog.2009.36
Sahu R., Meghavarnam A. K., Janakiraman S. (2019). A simple, efficient and rapid screening technique for differentiating nitrile hydratase and nitrilase producing bacteria. Biotechnol. Rep. (Amst). 24, e00396. doi: 10.1016/j.btre.2019.e00396
Shin Y. H., Shin N. C., Veriansyah B., Kim J., Lee Y. W. (2009). Supercritical water oxidation of wastewater from acrylonitrile manufacturing plant. J. Hazard. Mater. 163 (2-3), 1142–1147. doi: 10.1016/j.jhazmat.2008.07.069
Soares J., Fernandes R., Brito D., Oliveira H., Neuparth T., Martins I., et al. (2020). Environmental risk assessment of accidental marine spills: A new approach combining an online dynamic hazardous and noxious substances database with numerical dispersion, risk and population modelling. Sci. Total. Environ. 715, 136801. doi: 10.1016/j.scitotenv.2020.136801
Talley W. K., Yip T. L., Jin D. (2012). Determinants of vessel-accident bunker spills. Transport. Res. D-Tr. E. 17 (8), 605–609. doi: 10.1016/j.trd.2012.07.005
Thoman D. C., O’Kula K. R., Laul J. C., Davis M. W., Knecht K. D. (2006). Comparison of ALOHA and EPIcode for safety analysis applications. J. Chem. Health Saf. 13 (6), 20–33. doi: 10.1016/j.jchas.2006.02.003
Uherek E., Halenka T., Borken-Kleefeld J., Balkanski Y., Berntsen T., Borrego C., et al. (2010). Transport impacts on atmosphere and climate: Land transport. Atmospheric. Environ. 44 (37), 4772–4816. doi: 10.1016/j.atmosenv.2010.01.002
UNCTAD (United Nations Conference on Trade and Development) (2020) Review of maritime transport 2020. Available at: https://unctad.org/webflyer/review-maritime-transport-2020.
Vieira C. E. D., Costa P. G., Cabrera L. C., Primel E. G., Fillmann G., Bianchini A., et al. (2017). A comparative approach using biomarkers in feral and caged Neotropical fish: Implications for biomonitoring freshwater ecosystems in agricultural areas. Sci. Total. Environ. 586, 598–609. doi: 10.1016/j.scitotenv.2017.02.026
Wang K., Liu Z., Qian X., Huang P. (2017). Long-term consequence and vulnerability assessment of thermal radiation hazard from LNG explosive fireball in open space based on full-scale experiment and PHAST. J. Loss. Prevent. Proc. 46, 13–22. doi: 10.1016/j.jlp.2017.01.001
Weidhaas J. L., Dietrich A. M., DeYonker N. J., Ryan Dupont R., Foreman W. T., Gallagher D., et al. (2016). Enabling science support for better decision-making when responding to chemical spills. J. Environ. Qual. 45 (5), 1490–1500. doi: 10.2134/jeq2016.03.0090
Zhang T., Jin H., Zhu H. (1996). Quality criteria of acrylonitrile for the protection of aquatic life in China. Chemosphere 32 (10), 2083–2093. doi: 10.1016/0045-6535(96)00110-5
Zheng L., Pan L., Lin P., Miao J., Wang X., Lin Y., et al. (2017). Evaluating the toxic effects of three priority hazardous and noxious substances (HNS) to rotifer brachionus plicatilis. Environ. Sci. Pollut. Res. Int. 24 (35), 27277–27287. doi: 10.1007/s11356-017-0298-2
Keywords: acrylonitrile, spill, marine ecological risk, physico-chemical behavior, emergency response
Citation: Wang X and Meng F (2022) Emergency responses to acrylonitrile maritime spills from the perspective of marine ecological protection. Front. Mar. Sci. 9:996263. doi: 10.3389/fmars.2022.996263
Received: 17 July 2022; Accepted: 29 August 2022;
Published: 16 September 2022.
Edited by:
Xiutang Yuan, Yantai Institute of Coastal Zone Research, Chinese Academy of Sciences (CAS), ChinaCopyright © 2022 Wang and Meng. This is an open-access article distributed under the terms of the Creative Commons Attribution License (CC BY). The use, distribution or reproduction in other forums is permitted, provided the original author(s) and the copyright owner(s) are credited and that the original publication in this journal is cited, in accordance with accepted academic practice. No use, distribution or reproduction is permitted which does not comply with these terms.
*Correspondence: Fanping Meng, bWVuZ2ZhbnBpbmdAb3VjLmVkdS5jbg==
Disclaimer: All claims expressed in this article are solely those of the authors and do not necessarily represent those of their affiliated organizations, or those of the publisher, the editors and the reviewers. Any product that may be evaluated in this article or claim that may be made by its manufacturer is not guaranteed or endorsed by the publisher.
Research integrity at Frontiers
Learn more about the work of our research integrity team to safeguard the quality of each article we publish.