- 1Laboratory of Aquatic Nutrition and Feed, College of Fisheries, Guangdong Ocean University, Zhanjiang, China
- 2Integrity, Aquatic Animals Precision Nutrition and High Efficiency Feed Engineering Research Center of Guangdong Province, Zhanjiang, China
- 3Key Laboratory of Aquatic, Livestock and Poultry Feed Science and Technology in South China, Ministry of Agriculture, Zhanjiang, China
This study investigated the effect of lipid levels on growth, body composition, lipid metabolism enzymes activity and related genes expression in hybrid grouper (♀Epinephelus fuscointestinestatus × ♂E. lanceolatu). Three diets with different lipid levels of 6% (low-lipid feed, LF), 11% (medium-lipid feed, MF) and 16% (high-lipid feed, HF) were designed and each diet was fed to triplicate groups of 30 fish each (6.73 ± 0.00g) for eight weeks. The results revealed the following. 1) The maximum weight gain rate (WGR), specific growth rate (SGR), protein efficiency ratio (PER) and the lowest feed conversion ratio (FCR) values were found in the HF group. There was no significant difference in the survival rate (SR) among the groups (P > 0.05). 2) Condition factor (CF), hepatosomatic index (HSI) and visceralsomatic index (VSI) increased with increasing dietary lipid. 3) Crude lipid content in the whole-body and muscle increased significantly as the dietary lipid level increased (P < 0.05). 4) Principal component analysis of fatty acids showed that sum n-3 polyunsaturated fatty acids (∑n-3PUFA)/sum n-6 polyunsaturated fatty acids (∑n-6PUFA) was significantly higher in the HF group than in the other two groups (P < 0.05). 5) Biochemical serum indexes showed higher triglycerides (TG), total cholesterol (TC), alkaline phosphatase (AKP) and low-density lipoprotein-cholesterol (LDL-C) levels but lower high-density lipoprotein-cholesterol (HDL-C) level in the HF group. 6) Liver lipid metabolism enzymes activity and biochemical indicators showed that fish fed the MF diet had higher lipoprotein lipase (LPL), hepatic lipase (HL) and triglyceride lipase (ATGL) activity but lower fatty acid synthase (FAS) activity and very-low density lipoprotein-cholesterol (VLDL-C) level. 7) The relative expression levels of lipoprotein lipase (lpl), adipocyte-type fatty acid-binding protein (a-fabp), liver-type fatty acid-binding protein (l-fabp), heart-type fatty acid-binding protein (h-fabp) and hormone-sensitive lipase (hsl) genes in the fish liver were higher in the MF group with increasing dietary lipid (P < 0.05), while the fatty acid synthase (fas) gene was expressed at lower relative level (P < 0.05). 8) Histological oil-red sections of the liver showed that fish in the HF group had larger and more hepatic lipid droplets than those fed the other diets. It was concluded that fish fed the HF diet had better growth performance but this diet was not conducive to the progress of lipid metabolism, resulting in lipid accumulation and affecting the quality of the fish. This study provides useful information for the formulation of environmentally friendly and cost-effective diets for hybrid grouper.
Introduction
The grouper (Epinephelus spp.) is a valuable carnivorous fish of the lower and middle warm-water zones. It is one of the most economically important fish cultured in the southern coastal areas of China, especially in Guangdong, Fujian and Hainan provinces (Boonyaratpalin, 1997; Zhou et al., 2012). The hybrid grouper (♀Epinephelus fuscoguttatus × ♂Epinephelus lanceolatu) has the advantages of rapid growth, rich nutrition, delicate meat, high adaptability to the environment, strong disease resistance, high economic value and tolerance of a wide salinity range. It is suitable for pond, cage and factory farming. In recent years, large-scale farming has been carried out in Guangdong, Hainan and other places and the scale of farming and market demand have increased sharply (Liu et al., 2016; Fan et al., 2018; Zhao et al., 2019; Xu et al., 2021).
Fish meal, a high-quality protein source in feed, is in short supply due to limited fishery resources. Its price has increased continuously, making protein the most expensive feed ingredient. Therefore, reducing the content of fish meal in feed and improving the utilisation rate of feed protein can effectively save feed costs and decrease environmental pollution. The high digestibility of lipids can increase protein utilisation for tissue synthesis. Based on this principle that non-protein energy sources can decrease protein requirements, research is underway into an effective way to utilise lipids as energy to decrease feed protein requirements (Dias et al., 1998; Ai et al., 2004). Accordingly, the study of lipid-sparing protein has become a new research hotspot in aquaculture and the feed industry. Studies on hybrid grouper, Atlantic salmon (Salmo salar), cobia (Rachycentron canadum) and rainbow trout (Oncorhynchus mykiss) have shown that moderately increasing the lipid content in feed can save protein, improve feed utilisation and reduce nitrogenous waste emissions (Grisdale-Helland and Helland, 1997; Wang et al., 2005; Xie et al., 2021; Yight et al., 2002). The results of feeding hybrid grouper eight groups of diets containing 40%, 45%, 50% or 55% protein fraction and 7% or 14% lipid fraction (i.e. 40/7, 40/14, 45/7, 45/14, 50/7, 50/14, 55/7, 55/14), revealed a significant lipid-sparing protein effect, with the 50/14 group displaying the best utilisation of protein (Rahimnejad et al., 2015).
Feed lipid is important for the growth, development and reproduction of aquatic animals. Feed lipid not only serves as an important energy source to promote the growth and development of fish but also provides phospholipids, sterols and essential fatty acids for the body; facilitates the absorption, transfer and metabolism of lipid-soluble vitamins and other substances; and maintains the structure and physiological functions of biofilms (Lee et al., 2002; Leaver et al., 2008; Sun et al., 2013). Inadequate lipid content in feed can hinder the growth of farmed animals and reduce the quality of the product but excess lipid in feed has a detrimental effect on fish growth and development (Shiau and Lan, 1996; Perez‐Velazquez et al., 2017). Studies have shown that excessive lipid intake can lead to lipid deposition, metabolic disorders and immune reduction in farmed animals, which can lead to a fatty liver and affect the health status and growth rate (López et al., 2006; Han et al., 2014; Peng et al., 2017). Too much lipid in feed is also not conducive to feed storage (Song et al., 2010). For example, the liver lipid content of hybrid striped bass (♀Morone chrysops × ♂M. saxatilis) increased and growth declined when the dietary lipid content exceeded 20% (Gaylord and Gatlin Iii, 2000). The metabolism of lipid in the body requires a range of related enzymes. The expression of the lpl gene of red sea bream (Pagrus major) was affected by high lipid intake (Liang et al., 2002). Additionally, high-lipid supplementation of feed can reduce the stability of feed and increase its dissolution and loss, which can seriously affect the quality of aquafeeds, Too much lipid in feed can be easily oxidised, which is detrimental to feed storage (Tu et al., 2012). Therefore, an appropriate quantity of lipid in feed is essential to improve fish growth and optimise diet efficiency. For hybrid grouper fed with five groups of diets containing lipid fractions of 4.85%, 7.78%, 10.71%, 13.65% or 16.58%, the 13.65% group displayed a higher WGR, SGR and PER than the other four groups and the excretion rate of ammonia nitrogen was decreased (Xie et al., 2021).
Research on the nutrition of hybrid grouper is in its infancy. Some studies have shown that a diet with a crude protein content of 50%, a crude lipid content of 14% and an egg-to-energy ratio of 23.9 mg·kJ–1 can promote rapid growth. Thus, the purpose of this study was to investigate the effects of different dietary lipid levels on the growth, serum biochemical indexes, lipid metabolism enzymes and related genes of hybrid grouper.
Materials and methods
Based on the guidelines in the ‘Laboratory Animal Treatment and Usage Guide’, all animal researches were strictly conducted. Animal Ethics Committee of Guangdong Ocean University accepted the protocols (Zhanjiang, China).
Experimental diets
Three experimental diets with lipid contents of 6% (low-lipid, LF), 11% (medium-lipid, MF) and 16% (high-lipid, HF) were designed (Rahimnejad et al., 2015; Xie et al., 2021). All ingredients were crushed and passed through a 60-mesh sieve, then accurately weighed and thoroughly mixed using a progressive expansion method (Ayisi and Zhao, 2017), last mix well in the V-shaped stand mixer. The mixture was kneaded manually after the addition of fish oil and corn oil, then sieved and poured into the mixer. Added 30%-40% (mass fraction) distilled water and the ingredients were mixed evenly. The mixed ingredients were processed into 2.0 and 2.5 mm diameter pellets using a twin-screw extruder (F-26, South China University of Technology, Guangdong, China) and air-dried to a moisture content of approximately 10% at room temperature. The dried pellets were packed in bags, sealed and stored in a –20°C refrigerator for later use (Wei et al., 2021). The formulation and nutrient content are shown in Table 1.
Fish and feeding trial
Hybrid grouper (♀Epinephelus fuscointestinestatus × ♂E. lanceolatu) larvae were purchased from a grouper hatchery on Donghai Island (Zhanjiang, China). They were temporarily reared in an outdoor concrete pond (2.0 m × 4.0 m × 2.0 m) and fed a commercial diet for one week to adapt to the experimental conditions. The breeding experiments were conducted at the Breeding Base of Guangdong Ocean University (Zhanjiang, China). The fish were grouped after fasting for 24 h. According to the experimental design, 270 healthy fish of uniform size and an initial average weight of 6.73 ± 0.00 g were randomly divided into three groups of three replicates each. One 1000 L tank (0.8m water depth) was a replicate, with 30 fish in each tank. The breeding trial lasted for eight weeks. The grouper was fed twice a day (8:00 and 16:00) to obvious satiation and the feeding amount was adjusted according to the weather and food intake. During the breeding period, the aeration was uninterrupted with air stones, and about 500 L of water was exchanged every day to maintain the water quality. Each tank had a 20.0 cm (diameter) × 30.0 cm (length) polyvinyl chloride (PVC) pipe as a shelter for the fish. Throughout the experiment, the water temperature was 29–32°C, the salinity was 26–28, the dissolved oxygen was 5–6 mg·L–1 (Lu et al., 2022) and the nitrate was maintained at < 0.03 mg·L–1.
Sample collection
Samples were taken after 24 h of fasting at the end of the experiment (Liu et al., 2021). The total number of fish in each tank was counted and weighed. Three fish per tank were randomly selected from each tank and their length and weight were measured to calculate the condition factor. These fish were then stored at –20°C prior to body composition analysis. Another three fish per tank were selected and weighed and the liver and viscera were dissected and weighed to calculate the HSI and VSI. Then, the dorsal muscles were dissected, placed in 10 mL centrifuge tubes and stored at –20°C for subsequent fatty acid and nutrient content measurements. Additionally, four fish from each tank were randomly selected for blood collection using a 1 mL sterile syringe. The blood was placed in 1.5 mL centrifuge tubes and stored at 4°C for 12 h. The blood was later centrifuged and the serum was collected and stored at −20°C for enzyme activity analysis. The supernatant was collected and stored at –80°C for enzymes activity measurement. The liver and intestinal tissues of another three fish were dissected and placed in 2 mL cryopreservation tubes, frozen in liquid nitrogen and then stored at –80°C for enzyme activity measurement. The livers of an additional three fish per tank were dissected and placed in 2 mL enzyme-free tubes, frozen in liquid nitrogen and then stored at –80°C for relevant genes expression determination. Finally, the livers of three fish per tank were quickly removed and stored in 4% formaldehyde solution for the preparation of oil-red slices.
Methods of analysis
The experimental nutrient content of feed, whole body and muscle was determined by the AOAC method (AOAC, 1995). Moisture content was determined by the drying to constant weight method at 105°C. Crude protein content was measured using the Skalar Dumas TN/TC/IC/TOC automatic analyser. Crude lipid content was determined by the Soxhlet extraction method (the extraction solvent was petroleum ether). The crude ash content was determined using a muffle furnace at 550°C. The sections were observed by fluorescent inverted microscope (Nikon Eclipse Ti-E). The fatty acid content of the whole-body and muscle was determined by GC-MS (Agilent technologies 7890B-5977A, USA) according to the method of Qiu et al. (2017). The Total cholesterol (TC), triglycerides (TG), low-density lipoprotein-cholesterol (LDL-C), high-density lipoprotein-cholesterol (HDL-C), alkaline phosphatase (AKP) level of serum and lipoprotein lipase (LPL), hepatic lipase (HL), triglyceride lipase (ATGL), fatty acid synthase (FAS) activity and very low-density lipoprotein-cholesterol (VLDL-C) level of liver were analysed using ELISA kits (Shanghai Enzyme Linkage Biotechnology Co., Ltd., Shanghai, China).
RNA extraction and cDNA synthesis
Total RNA was extracted by adding 1 mL of TransZol UP (TransGen Biotech, Beijing, China) to each sample according to the manufacturer’s instructions. RNA quality and concentration were measured using a spectrophotometer Nanodrop 2000c (Thermo, USA) and RNA integrity was determined using 1% agarose gel. First-strand cDNA was synthesised using the PrimeScript™ RT kit with cDNA Eraser (Takara, Japan) according to the manufacturer’s instructions. The cDNA was stored at –80°C and used for real-time quantitative polymerase chain reaction (RT-qPCR).
Real-time quantitative polymerase chain reaction (RT-qPCR)
Real-time quantitative polymerase chain reaction (RT-qPCR) was performed on a 384-well plate in a reaction volume of 10 µL, containing 5 µL of 2X SYBR® Green Pro Taq HS Premix II (Accurate Biology, Hunan, China), 0.8µL of each primer, 1 µL of cDNA sample and 3.2 µL of Rnase Free dH2O. The PCR conditions were set using a thermal programmer: 95°C for 30 s, 40 cycles of 95°C for 5 s, then 60°C for 34s. Each experiment was conducted in triplicate. Primers for the reference gene (β-actin) and the target genes were designed with reference to published grouper sequences (Table 2). Threshold cycle (Ct) values were collected for each sample at the end of the treatment. The relative expression levels were calculated using the 2-ΔΔCt method (Livak and Schmittgen, 2001).
Calculation formula and statistical analysis
Growth performance and morphological indicators:
Weight gain rate (WGR, %) = 100 × (final weight - initial weight)/initial weight;
Specific growth rate (SGR, %/d) = 100 × [ln (final weight) - ln (initial weight)]/days of experiment;
Feed conversion ratio (FCR) = feed intake/weight gain;
Survival rate (SR, %) = 100 × (total number of fish at termination/total number of fish stocked);
Protein efficiency ratio (PER) = average weight gain/average protein intake;
Hepatosomatic index (HSI, %) = 100 × (liver weight/body weight);
Visceralsomatic index (VSI, %) = 100 × (viscera weight/body weight);
Condition factor (CF, g/cm3) = 100 × weight of fish/(length of fish)3.
All data were first tested for chi-square using SPSS version 21.0 (SPSS Inc., USA). Results were analysed using one-way ANOVA and Tukey test. P < 0.05 was considered a statistically significant difference. The results were expressed as mean ± standard error (SEM).
Results
Growth performance and morphological indexes
The results of growth performance are presented in Table 3. The maximum WGR, SGR and PER values were observed in the HF group and were significantly higher than those in the LF group (P < 0.05) but not significantly different from those in the MF group (P > 0.05). FCR was significantly higher in the LF group than in the MF and HF groups (P < 0.05), while PER was significantly lower in the LF group than in the other two groups (P < 0.05). There was no significant difference in SR among the groups (P > 0.05).
The morphological indexes are shown in Table 4. The highest values of CF and VSI were observed in the HF group and were significantly higher than those in the LF group (P < 0.05). There was no significant difference of VSI into the MF and HF groups (P > 0.05) and there was no significant difference in HSI among the treatments (P > 0.05).
Whole body and muscle composition
The results of whole-body composition are shown in Table 5. As the dietary lipid level increased, the moisture and crude protein content of the whole body significantly decreased (P < 0.05) and the lipid content significantly increased (P < 0.05). The whole-body crude ash content of the HF group was significantly lower than that of the LF group (P < 0.05) but was not significantly different from that of the MF group (P > 0.05).
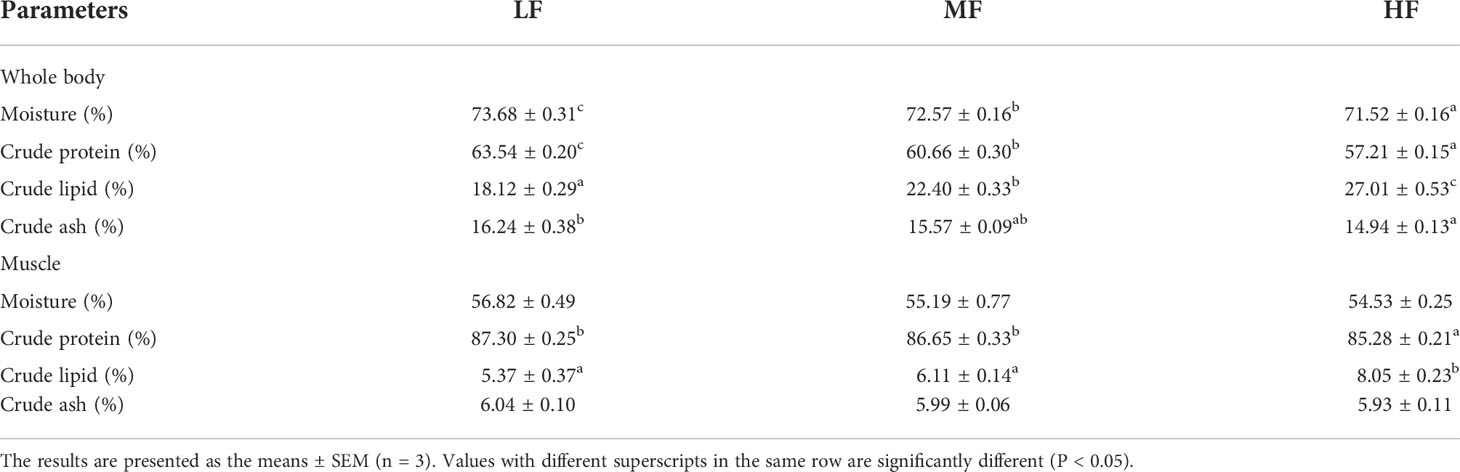
Table 5 Effect of dietary lipid levels on whole body and muscle composition of hybrid grouper (% dry matter).
There was no significant difference in the moisture and crude ash content in muscle among the groups (P > 0.05). The muscle crude lipid content was significantly higher in the HF group than in the other two groups (P < 0.05) and the trend in crude protein content in muscle as the dietary lipid content increased was opposite to that of crude lipid in muscle.
Fatty acid composition of whole body and muscle
The whole-body fatty acid composition is shown in Table 6. With the lipid level in the diet increased, the Total saturated fatty acids (∑SAFA) and total monounsaturated fatty acids(∑MUFA) content of the whole-body decreased gradually and was significantly higher in the LF group than in the other two groups (P < 0.05). The MF group had significantly higher whole-body sum n-3 polyunsaturated fatty acids (∑n-3PUFA) and sum n-3 highly unsaturated fatty acids (∑n-3HUFA) content than the other two groups (P < 0.05). The whole-body sum n-6 polyunsaturated fatty acids (∑n-6PUFA) content of the HF group was significantly higher than that of the other two groups(P < 0.05) and the n3/n6 ratio showed the opposite trend. There was no significant difference in the whole-body Eicosapentaenoic Acid (EPA)/Docosahexaenoic Acid (DHA) ratio among treatments (P > 0.05).
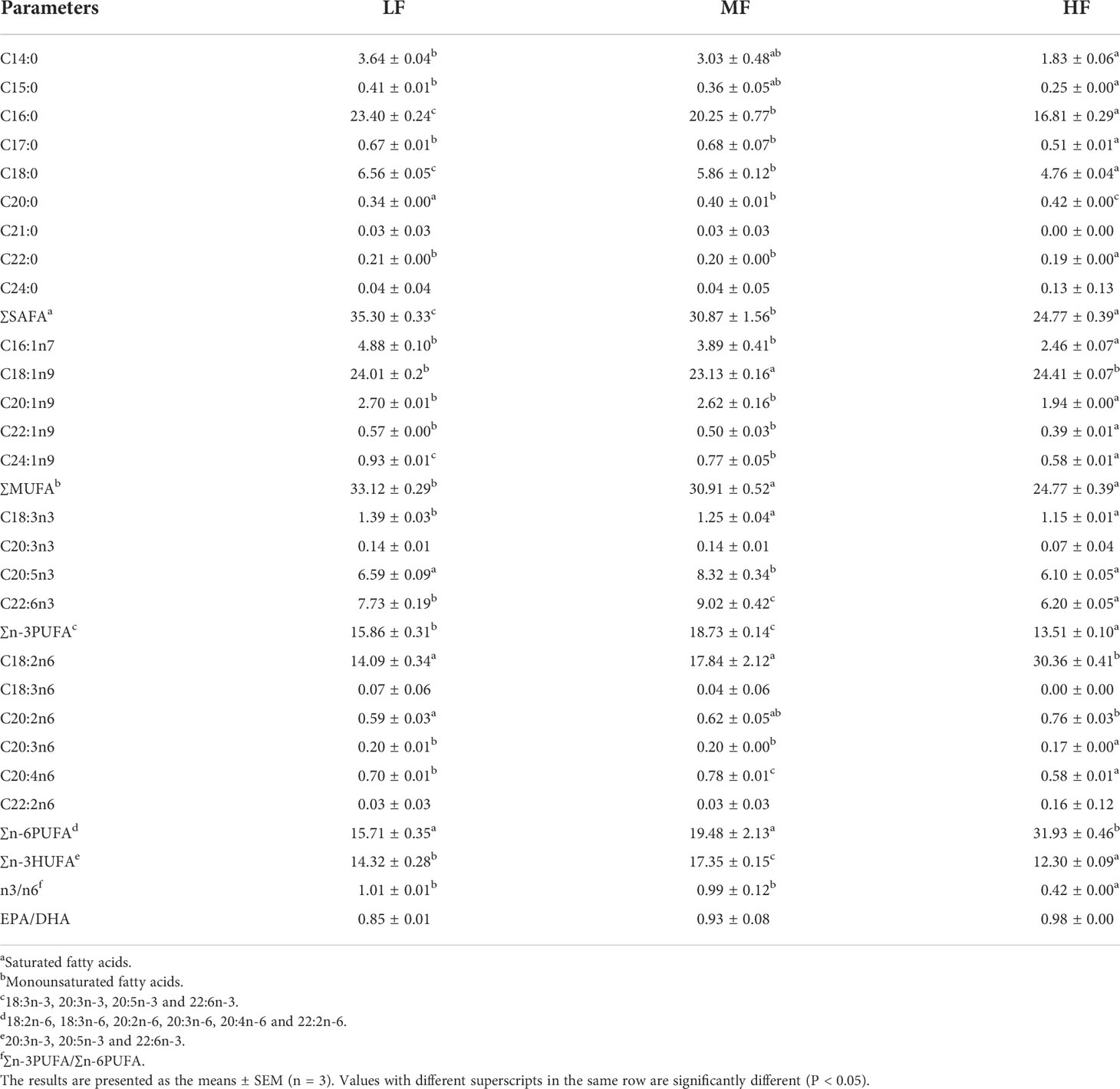
Table 6 Effect of dietary lipid levels on fatty acid composition of whole-body in hybrid grouper (% total fatty acids).
As shown in Table 7, the ∑SAFA and ∑MUFA content in muscle was significantly higher in the LF group than in the other two groups. The ∑n-3PUFA and ∑n-3HUFA in muscle increased and then decreased, with the highest value in the MF group, which was significantly higher than those in the other two groups (P < 0.05). As dietary lipid increased, ∑n-6PUFA and the EPA/DHA ratio of muscle significantly increased and the n3/n6 ratio significantly decreased (P < 0.05).
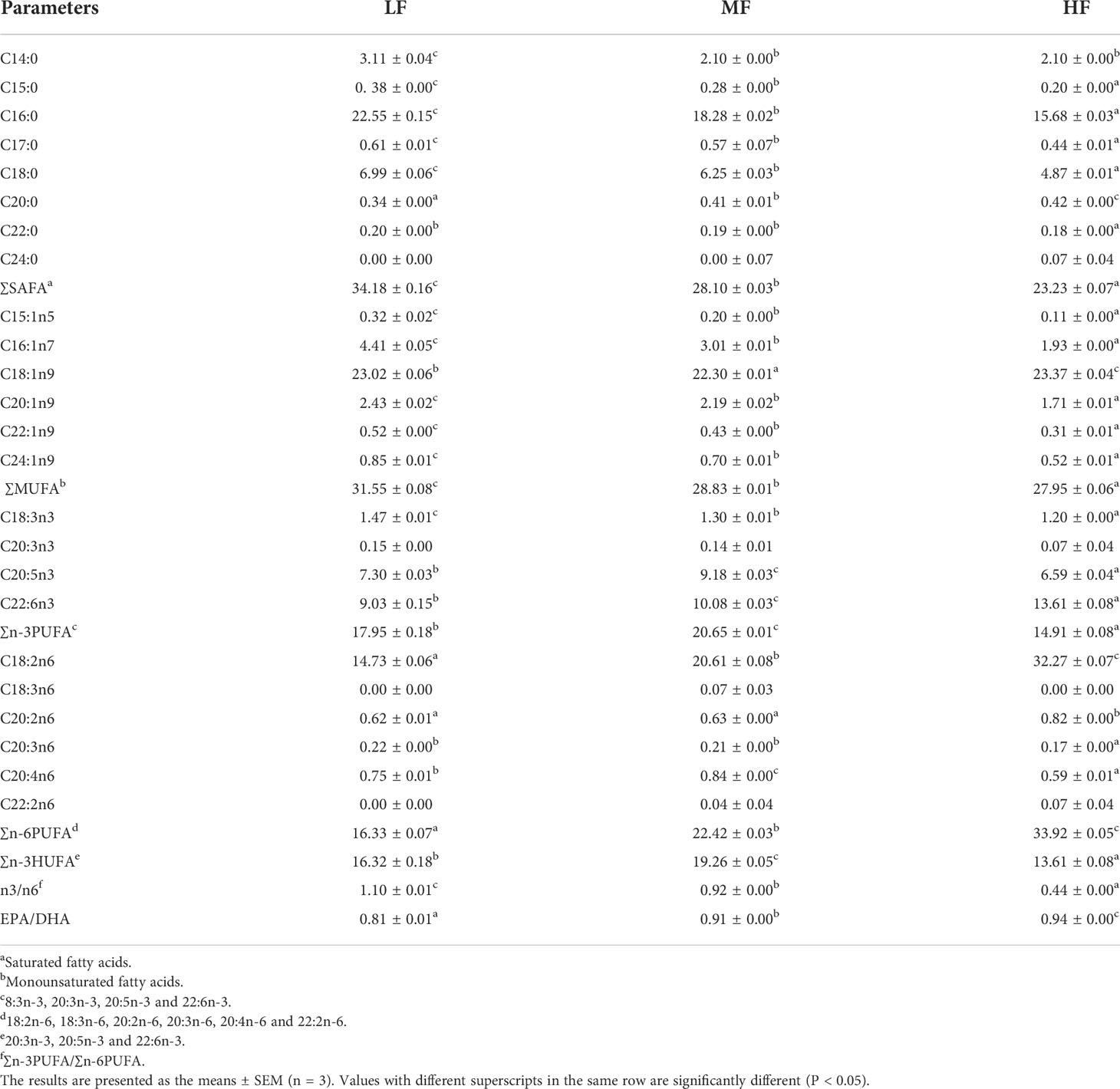
Table 7 Effect of dietary lipid levels on fatty acid composition of muscle in hybrid grouper (%total fatty acids).
Biochemical indices in serum
Serum biochemical indexes are shown in Table 8. The TG and TC content decreased and then increased. The lowest value was in the MF group, which was significantly lower than that in the HF group but not significantly different to that in the LF group (P < 0.05). The dietary lipid level did not affect HDL-C or LDL-C serum level (P > 0.05).
Lipid metabolism enzymes activity in liver
Liver lipid metabolism enzymes activity and biochemical indexes are shown in Table 9. LPL, HL and ATGL activity first increased and then decreased as the dietary lipid level increased. The greatest values were in the MF group, which were significantly higher than those in the HF group (P < 0.05) but not significantly different to those in the LF group (except for HL) (P > 0.05). FAS activity and VLDL-C level had the opposite trend to LPL, with minimum values obtained in the MF group; these were significantly lower than those in the HF group (P < 0.05) but were not significantly different from those in the LF group (P > 0.05).
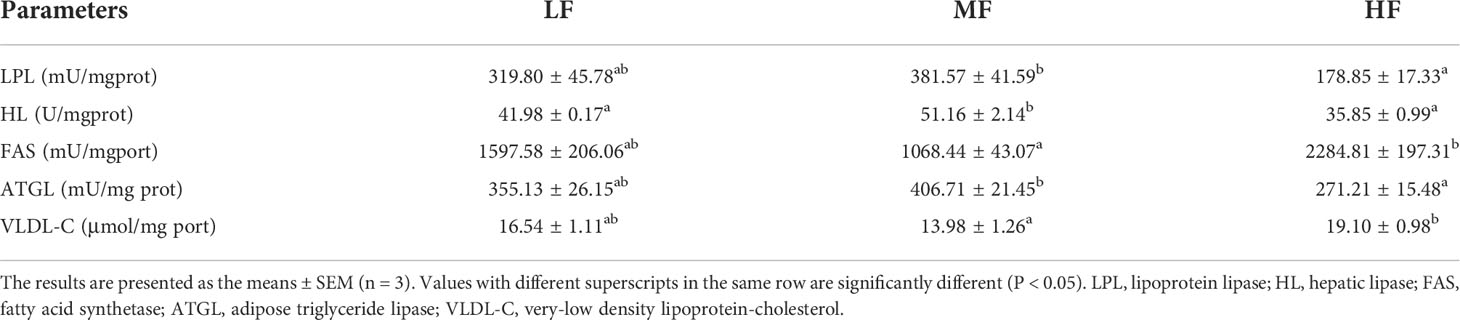
Table 9 Effect of dietary lipid levels on lipid metabolism enzymes activity and biochemical indexes in liver of hybrid grouper.
Liver oil-red slices
Figure 1 shows that, as dietary lipid increased, the hepatic lipid droplets in the liver oil-red slices gradually increased and enlarged. Serious lipid accumulation was found in the HF group.
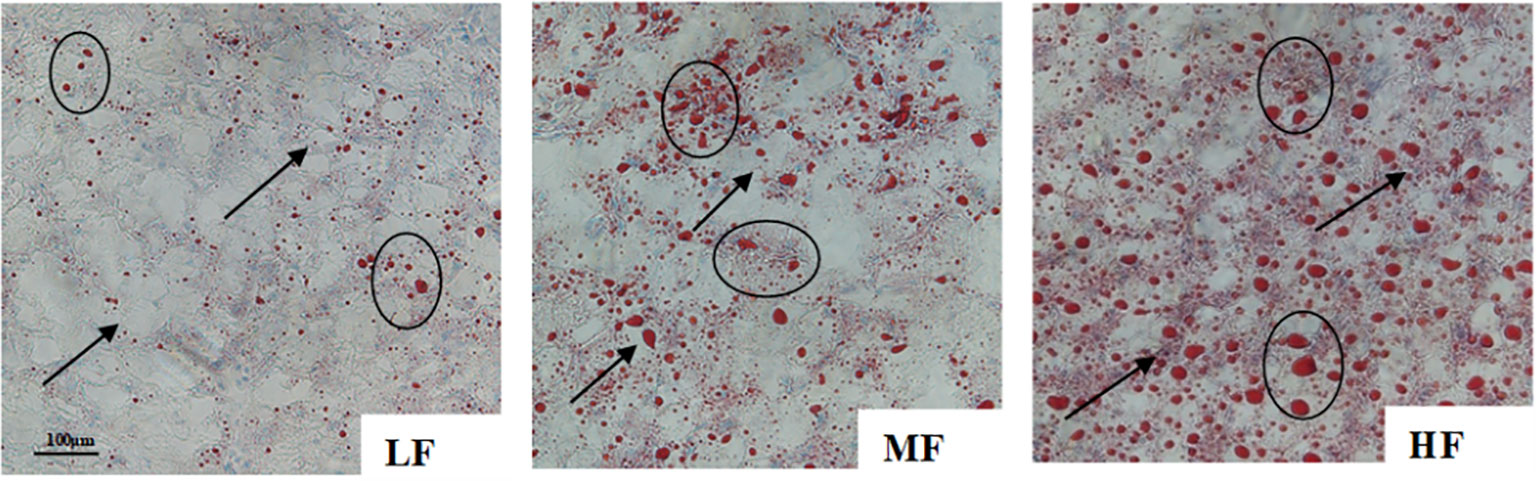
Figure 1 Effect of dietary lipid levels on hepatic histology of hybrid grouper (oil-red × 400; The lipid deposition status is shown by arrows and ovals).
Expression of genes related to hepatic lipid metabolism
As shown in Figure 2, lpl, a-fabp, l-fabp, h-fabp and hsl mRNA expression first increased and then decreased. The expression of lpl, a-fabp, l-fabp, h-fabp, and hsl mRNA was significantly higher in the MF group than in the other two groups (P < 0.05), while expression of these genes was significantly lower in the HF group than in the other two groups (P < 0.05). The expression of fas mRNA was significantly higher in the HF group than in the other two groups (P < 0.05) and its expression in the MF group was significantly lower than in the other two groupsm (P < 0.05).
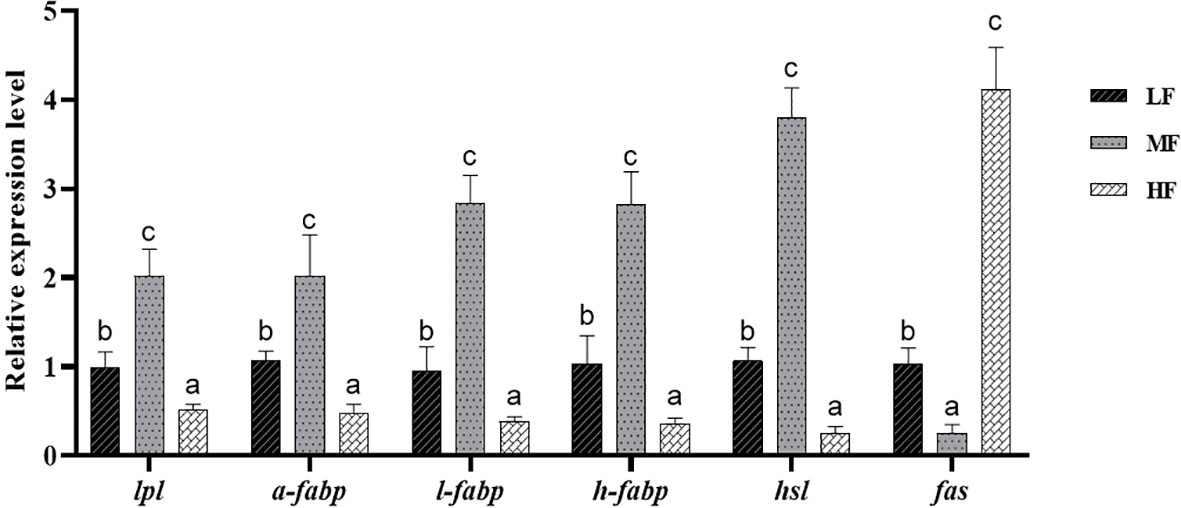
Figure 2 Effect of dietary lipid levels on relative expression of hepatic lipid metabolism-related genes of hybrid grouper. Values are means ± SEM (n = 3). Different letters assigned to the bars represent significant differences using Tukey’s test (P < 0.05). lpl, lipoprotein lipase; a-fabp, adipocyte-type fatty acid-binding protein; l-fabp, liver-type fatty acid-binding protein; h-fabp, heart-type fatty acid-binding protein; hsl, hormone-sensitive triglyceride lipase; fas, fatty acid synthetase.
Discussion
Lipid is an important source of energy, providing essential fatty acids and phospholipids to the body, and plays an important role in fish nutrition (Lu et al., 2013b). An appropriate amount of lipid is essential to maintain the vital functions of fish but levels that are too high or too low will have negative effects on fish (Hansen et al., 2008). The lipid requirements of fish vary not only by species but also depending on their feeding habits, living environment, growth stage, and the protein and carbohydrate content in their feed (Jiang et al., 2015). Carp (Cyprinus carpio) can have a feed lipid content of up to 18%, while some tilapia (Oreochromis mossambicus) can have a maximum feed lipid content of 10% (Guillaume et al., 2001). The results of this study showed that, after eight weeks of feeding, the HF group had significantly improved growth, which was consistent with the results of studies on orange-spotted grouper (Epinephelus bleekeri), Grass carp (Ctenopharyngodon idella) and Large yellow croaker (Larimichthy scrocea) (Jin et al., 2013; Yi et al., 2014; Li et al., 2016). The growth-promoting effects of high dietary lipids are usually associated with the protein-producing effects of dietary lipids (Boujard et al., 2004). Increasing the lipid level of the feed can conserve protein, thus allowing more energy to be used for fish growth. The FCR and PER values were significantly higher in the LF group than in the other two groups, which reflected good feed acceptability. The HF group had a lower SR, which may have been because high lipid levels are detrimental to the survival of grouper.
Three morphological indicators, CF, HSI and VSI are often used to evaluate the response of fish to different nutrient levels (Luo et al., 2011; Chang et al., 2018). This study showed that the HF group had the highest CF, HSI and VSI levels. This may have been due to the greater lipid deposition in the viscera and fish body for the HF group. Studies on fish such as Culter alburnus (Erythrocutler ilishaeformis), cobia and eye-plaque-like headfish (Sciaenops ocellatus) have shown similar results (Wang et al., 2005; Liu et al., 2008; Xu et al., 2020). In general, increasing dietary lipid levels will increase the lipid content of the liver. High dietary lipid levels promote an increase in the number of adipocytes in the liver (Umino et al., 1996), increasing the volume of hepatic adipocytes (Bellardi et al., 1995) and allowing the liver to accommodate more lipid (Nanton et al., 2001), ultimately leading to an increase in HSI. However, the HSI of fish such as Atlantic salmon, hybrid Clarias catfish (♀Clarias macrocephalus × ♂C.gariepinus) and largemouth bass (Micropterus salmoides) did not increase significantly with increasing levels of dietary lipid (Jantrarotai et al., 1994; Helland and Grisdale-Helland, 1998; Zhu et al., 2018). These fish can store large amounts of lipid in the abdominal cavity as cellulite, which reduces the amount of lipid stored in hepatocytes. This effect may also be due to the decreased feeding efficiency of fish as the level of dietary lipid increases but more in-depth studies are needed, including on liver lipid metabolism, to verify this.
In this study, the whole-body and muscle crude lipid content were significantly higher in the HF group than that in the other two groups. The HF group also showed the highest liver lipid accumulation in the oil-red sections. Increased dietary lipid levels were observed in Asian seabass (Lates calcarifer), freshwater black snapper (Hephaestus fuliginosus), Large yellow croaker, and hybrid snakehead (♀Channa argus × ♂Channa maculata) (Catacutan and Coloso, 1997; Song et al., 2010; Wang et al., 2015; Zhao et al., 2016), resulting in greater body lipid deposition. The study of Dongtingking crucian carp showed that the crude muscle lipid content increased as the dietary lipid level increased and was significantly higher in the 12% lipid group than in the 0 and 4% lipid groups (Lei et al., 2020). In Carassius auratus gibelio, the crude muscle lipid content increased within the dietary lipid range of 6.04–9.88% (Wang et al., 2010). A similar result was also found in cobia (Wang et al., 2005). However, in the present study, the whole-body and muscle crude protein content of the HF group was significantly lower than that in the other two groups, which may have been due to the increase in whole-body lipid content.
Fatty acids have attracted increasing research attention because of their important role in lipid digestion, absorption and synthesis (Monge‐Ortiz et al., 2018). Fatty acids can maintain the normal growth of fish, improve feed utilisation and improve the fish survival rate. When fatty acids are deficient, fish grow slowly, develop diseases and even die (Tocher, 2003; Torstensen et al., 2004). Differences in the fatty acids composition of fish can be attributed to differences in fish species and size, feed lipid types and levels, feeding time and environmental factors (Jin et al., 2013). Generally, the feed lipid level directly affects the digestion and absorption efficiency of different fatty acids by fish, the amount of fatty acid deposition in fish and the composition of fatty acids in fish (Qin et al., 2013). In the present study, there was a correlation between the feed lipid content and the concentration of specific fatty acids in the whole body and muscle. Additionally, the accumulation pattern of different fatty acids in fillets and muscle was different. The results showed that whole-body and muscle ∑n-6PUFA were highest in the HF group but there was an opposite outcome for ∑n-3PUFA, which caused a significant reduction in n3/n6 for the HF group. This may have been due to the increase in lipid levels in the diet changing the n3/n6 fatty acid ratio. An imbalance between n-3 and n-6 fatty acids can weaken the exchange capacity of lipids and accelerate the rate of lipid deposition in fish, resulting in higher lipid content in fish (Verreth et al., 1994). The HF group had the highest proportion of polyunsaturated fatty acids (PUFAs) in the total fatty acid content. This was because, when the level of feed lipid is higher than that required by fish, fish preferentially use SAFAs and MUFAs to meet their energy needs and store PUFAs (Torstensen et al., 2004). For example, the C22:1n-11 content in cod did not increase as the feed lipid content increased, whereas the content of PUFAs increased proportionally; this indicated that the cod preferentially used C22:1n-11 for energy and PUFAs were mainly used for growth and development (Santos et al., 1993). In fish such as white seabass (Atractoscion nobilis), it has been found that, the higher the level of dietary lipid, the higher the proportion of PUFAs of the total fatty acid content (López et al., 2009). Similar results have also been reported in studies of carp and Nile tilapia (Géret et al., 2002; Böhm et al., 2014). Thus, the feed lipid level not only regulates the composition pattern of saturated/monounsaturated/polyunsaturated fatty acids in fish tissues but also affects the n3/n6 ratio.
In addition to affecting growth performance and fatty acid composition, feed lipid levels also affect the serum biochemical indicators in fish (Zhao et al., 2015; Xie et al., 2017). Fish blood is closely related to fish nutritional status, metabolism and disease. When fish are affected by external factors and undergo physiological or pathological changes, these are mainly reflected in blood indicators (Zhou et al., 2001). Therefore, blood lipid levels can reflect the level of lipid metabolism in fish. TG, an energy substance in fish, is involved in the release and storage of energy. Lipids in the form of TG are transported in the blood of fish, with the content of TG in serum being negatively correlated with the ability of the liver to decompose lipids (Hubacek and Bobkova, 2006). TC is used to assess the activity of endogenous lipid transport (Zhou et al., 2016). Thus, the levels of TG and TC in the blood are important physiological indicators reflecting the level of lipid metabolism in fish, with higher levels indicating greater lipid accumulation in the liver of fish (Kim et al., 2005; Tu et al., 2012). The HDL-C/LDL-C ratio can reflect the transport status of cholesterol in fish and act as an indicator of the degree of atherosclerosis (Yun et al., 2011). In the present study, the TG content of the HF group was significantly higher than that of the MF group and its TC content was significantly higher than that of the other two groups. The serum TG and TC levels of grass carp increased with increasing dietary lipid levels (Du et al., 2006), which was similar to the result of this study. Additionally, in the present study, the HF group had lower HDL-C level and higher LDL-C level compared to the other two groups. When the level of dietary lipid was too high, the level of serum HDL-C decreased; this is not conducive to metabolic energy production or the transport of TG and affects lipid metabolism in the liver. At the same time, the level of serum LDL-C increased; this causes TG to enter the bloodstream where excess TG accumulates on the vessel wall, increasing the risk of atherosclerosis (Fredenrich and Bayer, 2003).
The liver is the main metabolic centre of fish (Chi et al, 2017). It has the most active lipid metabolism and plays a central role in metabolic homeostasis, which is a complex process involving lipid uptake, transport and metabolism (Lu et al., 2013a). Dietary lipid levels not only affect the growth performance, fatty acid composition and serum biochemical parameters of grouper but also significantly influence the hepatic LPL, HL, ATGL, FAS enzymes activity and VLDL-C level. LPL and HL are two key enzymes involved in lipid catabolism, collectively referred to as total lipase (Chen et al., 2016). LPL can catalyse the hydrolysis of TG carried by VLDL-C and chylomicrons to glycerol and fatty acids for tissue storage and utilisation (Feoli-Fonseca et al., 1998; Huang et al., 2018). HL has a variety of lipase activity, acts as a ligand to facilitate the entry of LDL-C and chylomicrons into hepatocytes, and directly participates in the metabolism of TG and phospholipids in HDL-C. It is also one of the enzymes involved in the metabolism of endogenous TG in circulating blood and has a similar function to LPL (Cohen et al., 1999; Wong and Schotz, 2002). The activity of HL affects the content of HDL-C, producing small, dense LDL-C, which means that HL has a relieving effect on atherosclerosis (Schiekofer et al., 2017). ATGL is the rate-limiting enzyme for TC hydrolysis and it can also specifically hydrolyse the first ester bond of TG. ATGL deficiency leads to hepatic steatosis and the lipolytic process mediated by ATGL may be related to metabolic diseases such as fatty liver (Smirnova et al., 2006). Excessive deposition of VLDL in the liver affects the metabolic transport of lipids, leading to the disruption of lipid metabolism, which in turn leads to fatty liver disease (Young, 1990; Ma et al., 2015). FAS is a key enzyme involved in the ab initio synthesis of fatty acids in fish, with higher expression in liver and adipose tissue (Liu et al., 2010). The results of the present study showed that LPL and HL activity was lowest in the HF group and was significantly lower than in the MF group. This may have been because liver lipase activity gradually increased as the dietary lipid level increased, but excessive lipid caused liver damage and reduced lipase activity. ATGL activity was significantly lower in the HF group than in the MF group, which may have been because of the higher TC content in the HF group. The VLDL-C level in the HF group was significantly higher than that in the MF group, which may have affected lipid transport in the liver. The FAS content decreased in the MF group and increased in the HF group, which may have been because liver lipid synthase activity gradually decreased as the level of dietary lipid increased but excessive lipid caused liver damage and disrupted lipid synthesis.
The lipid metabolism process is a dynamic balance of lipid anabolism and catabolism (Zhu et al., 2012). The regulation of lipid synthesis and catabolism in animals is via the content and activity of key enzymes in lipid metabolism, with the expression levels of key enzyme genes determining the number and activity of enzyme proteins (Yue et al., 2012). LPL is related to lipid metabolism and obesity and can catalyse the breakdown of protein-related TG into glycerol and free fatty acids, thereby playing a key role in the metabolism of TG (Puigserver et al., 1999; Yue et al., 2012). It is generally believed that the measurement of HSL activity can reflect lipid breakdown, and hsl gene expression and HSL activity are affected by energy levels, lipid types and fasting (Sztalryd and Kraemer, 1994; Stich et al., 1997). FABPs are members of a multigene family of intracellular lipid-binding proteins (Huang et al., 2022). FABPS can increase the solubility of fatty acids and their transport from lipid membranes to oxidation sites and then to triglyceride or phospholipid sites, from where they enter the nucleus to exert regulatory effects (Di Pietro and Santomé, 2001). l-fabp mainly promotes the absorption, diffusion and metabolism of fatty acids (Her et al., 2003), while h-fabp mainly promotes the binding (Bransden et al., 2003) and transport of fatty acids and a-fabp mainly regulates the oxidative energy supply of fatty acids and the metabolism of phospholipids and triglycerides (Chmurzyńska, 2006). fas activity and gene expression level determine the rate of fatty acid synthesis. In the present study, the expression of lpl, hsl, a-fabp, l-fabp and h-fabp mRNA was significantly lower in the HF group than in the other two groups. This indicated that lipid transport and metabolism were most active in the MF group, while excessive dietary lipid levels inhibited the expression of lipolytic and lipid-binding enzymes, thus causing lipid accumulation. It has been shown that fas expression levels decrease as the level of ingested feed lipids increases (Leng et al., 2012; He et al., 2015). This is because exogenous lipid intake is sufficient at higher lipid levels in feed, thus reducing the synthesis of endogenous lipids. A dynamic balance between exogenous feed lipid absorption, utilisation and endogenous lipid synthesis is maintained (Rollin et al., 2003). In contrast, fas mRNA expression in the HF group was significantly higher than in the other two groups, showing the same trend as for enzyme activity. The reduction in fas expression in the MF group may have been to the inhibition of fatty acid synthesis and lipid accumulation, thus preventing cellular toxicity (Van Herpen and Schrauwen‐Hinderling, 2008). The increased expression of fas in the HF group may have been due to liver damage caused by excessive lipid, which led to the obstruction of endogenous lipid synthesis.
Conclusion
In summary, hybrid grouper fed a diet containing 16% lipid (HF) had better growth performance but this diet was not conducive to lipid metabolism in the serum and liver, resulting in the accumulation of lipid in the fish. Therefore, further research is needed to find feed additives that can alleviate the harm of high-lipid feed on the lipid metabolism of hybrid grouper.
Data availability statement
The original contributions presented in the study are included in the article/supplementary files. Further inquiries can be directed to the corresponding author.
Ethics statement
The studies involving human participants were reviewed and approved by Guangdong Ocean University. The patients/participants provided their written informed consent to participate in this study. The animal study was reviewed and approved by Guangdong Ocean University. Written informed consent was obtained from the individual(s) for the publication of any potentially identifiable images or data included in this article.
Author contributions
XS: Conduct breeding experiments, experimental analysis, data processing, and paper writing; XY, HL, SP, TL, and WH: Provide a lot of help in the process of breeding experiments and experimental analysis; XD, BT, SZ, and YY: Provided good breeding conditions, financial support and guidance for writing paper. All authors contributed to the article and approved the submitted version.
Funding
This study was supported financially by the Department of Education of Guangdong Province (2021ZDZX4005), General Program of Natural Science Foundation of Guangdong Province (2021A1515011165), the National Natural Science Foundation of China (NSFC31972808), Research and Demonstration of Precision Functional Compound Feed Technology of Major Cultured Fishes and Shrimps in South China (2021B0202050002), Science and Technology Bureau of Zhanjiang (2020A03010 & 2020A05003), and the China Agriculture Research System of MOF and MARA (CARS-47).
Conflict of interest
The authors declare that the research was conducted in the absence of any commercial or financial relationships that could be construed as a potential conflict of interest.
Publisher’s note
All claims expressed in this article are solely those of the authors and do not necessarily represent those of their affiliated organizations, or those of the publisher, the editors and the reviewers. Any product that may be evaluated in this article, or claim that may be made by its manufacturer, is not guaranteed or endorsed by the publisher.
References
Ai Q. H., Mai K. S., Li H. T., Zhang C. X., Zhang L., Duan Q. Y., et al. (2004). Effects of dietary protein to energy ratios on growth and body composition of juvenile Japanese seabass, Lateolabrax japonicus. Aquaculture 230 (1-4), 507–516. doi: 10.1016/j.aquaculture.2003.09.040
AOAC (1995). Official methods of analysis of AOAC. Trends Food Sci. Technol. 6 (11), 382–382. doi: 10.1016/0924-2244(95)90022-5
Ayisi C. L., Zhao J. L. (2017). Fatty acid composition, lipogenic enzyme activities and mRNA expression of genes involved in the lipid metabolism of Nile tilapia fed with palm oil. Turkish J. Aquat. Sci. 17, 405–415. doi: 10.4194/1303-2712-v17_2_20
Bellardi S., Bianchini M. L., Domenis L., Palmegiano G. B. (1995). Effect of feeding schedule and feeding rate on size and number of adipocytes in rainbow trout (Oncorhynchus mykiss). J. World Aquac. Soc. 26 (1), 80–83. doi: 10.1111/j.1749-7345.1995.tb00212.x
Böhm M., Schultz S., Koussoroplis A. M., Kainz M. J. (2014). Tissue-specific fatty acids response to different diets in common carp (Cyprinus carpio l.). PloS One 9 (4), e94759. doi: 10.1371/journal.pone.0094759
Boonyaratpalin M. (1997). Nutrient requirements of marine food fish cultured in southeast Asia. Aquaculture 151 (1-4), 283–313. doi: 10.1016/S0044-8486(96)01497-4
Boujard T., Gélineau A., Covès D., Corraze G., Dutto G., Gasset E., et al. (2004). Regulation of feed intake, growth, nutrient and energy utilisation in European sea bass (Dicentrarchus labrax) fed high lipid diets. Aquaculture 231 (1-4), 529–545. doi: 10.1016/j.aquaculture.2003.11.010
Bransden M. P., Carter C. G., Nichols P. D. (2003). Replacement of fish oil with sunflower oil in feeds for Atlantic salmon (Salmo salar): Effect on growth performance, tissue fatty acid composition and disease resistance. Comp. Biochem. Physiol. Part B: Biochem. Mol. Biol. 135 (4), 611–625. doi: 10.1016/S1096-4959(03)00143-X
Catacutan M. R., Coloso R. M. (1997). Growth of juvenile Asian seabass, lates calcarifer, fed varying carbohydrate and lipid levels. Aquaculture 149 (1-2), 137–144. doi: 10.1016/S0044-8486(96)01432-9
Chang J., Niu H. X., Jia Y. D., Li S. G., Xu G. F. (2018). Effects of dietary lipid levels on growth, feed utilization, digestive tract enzyme activity and lipid deposition of juvenile Manchurian trout, brachymystax lenok (Pallas). Aquac. Nutr. 24 (2), 694–701. doi: 10.1111/anu.12598
Chen Q., Liu H. Y., Tan B. P., Dong X. H., Chi S. Y., Yang Q. H., et al. (2016). Effects of dietary cholesterol on growth, blood biochemical parameters and lipid metabolism in juvenile cobia. J. Guangdong Ocean Univ. 36 (1), 35–43. doi: 10.3969/j.issn.1673-9159.2016.01.007
Chi S. Y., Zhong J. L., He Y. F., Li J. M., Luo Y. (2017). Amino acid transporter in Epinephelus coioides cloning and tissue expression of B0AT1 gene. J. Guangdong Ocean Univ. 37 (6), 1–6. doi: 10.3969/j.issn.1673-9159.2017.06.001
Chmurzyńska A. (2006). The multigene family of fatty acid-binding proteins (FABPs): Function, structure and polymorphism. J. Appl. Genet. 47 (1), 39–48. doi: 10.1007/BF03194597
Cohen J. C., Vega G. L., Grundy S. M. (1999). Hepatic lipase: new insights from genetic and metabolic studies. Curr. Opin. Lipidol. 10 (3), 259–267. doi: 10.1097/00041433-199906000-00008
Dias J., Alvarez M. J., Diez A., Arzel J., Corraze G., Bautista J. M., et al. (1998). Regulation of hepatic lipogenesis by dietary protein/energy in juvenile European seabass (Dicentrarchus labrax). Aquaculture 161 (1-4), 169–186. doi: 10.1016/S0044-8486(97)00268-8
Di Pietro S. M., Santomé J. A. (2001). Structural and biochemical characterization of the lungfish (Lepidosiren paradoxa) liver basic fatty acid binding protein. Arch. Biochem. Biophys. 388 (1), 81–90. doi: 10.1006/abbi.2001.2277
Du Z. Y., Clouet P., Zheng W. H., Degrace P., Tian L. X., Liu Y. J. (2006). Biochemical hepatic alterations and body lipid composition in the herbivorous grass carp (Ctenopharyngodon idella) fed high lipid diets. Br. J. Nutr. 95 (5), 905–915. doi: 10.1079/BJN20061733
Fan X. P., Qin X. M., Zhang C. H., Cheng J. P., Zhu Q. F. (2018). Analysis and evaluation of muscle nutritional components and volatile flavor components of hybrid grouper (♀Epinephelus fuscointestinestatus × ♂E. lanceolatu). J. Guangdong Ocean Univ. 38 (1), 39–46. doi: 10.3969/j.issn.1673-9159.2018.01.006
Feoli-Fonseca J. C., Lévy E., Godard M., Lambert M. (1998). Familial lipoprotein lipase deficiency in infancy: Clinical, biochemical, and molecular study. J. Pediatr. 133, 417–423. doi: 10.1016/S0022-3476(98)70280-X
Fredenrich A., Bayer P. (2003). Reverse cholesterol transport, high density lipoproteins and HDL cholesterol: Recent data. Diabetes Metab. 29 (3), 201–205. doi: 10.1016/S1262-3636(07)70029-0
Gaylord T. G., Gatlin Iii D. M. (2000). Dietary lipid level but not l-carnitine affects growth perfomance of hybrid striped bass (Morone chrysops♀× M. saxatilis♂). Aquaculture 190 (3-4), 237–246. doi: 10.1016/S0044-8486(00)00404-X
Géret F., Jouan A., Turpin V., Bebianno M. J., Cosson R. P. (2002). Influence of metal exposure on metallothionein synthesis and lipid peroxidation in two bivalve mollusks: the oyster (Crassostrea gigas) and the mussel (Mytilus edulis). Aquat. Living Resour. 15 (1), 61–66. doi: 10.1016/S0990-7440(01)01147-0
Grisdale-Helland B., Helland S. J. (1997). Replacement of protein by lipid and carbohydrate in diets for Atlantic salmon (Salmo salar) at the end of the freshwater stage. Aquaculture 152 (1-4), 167–180. doi: 10.1016/S0044-8486(97)00003-3
Guillaume J., Kaushik S., Bergot P., Metailler R. (2001). Nutrition and feeding of fish and crustaceans (London: Springer Science & Business Media, chichester), 297–307.
Han T., Li X. Y., Wang J. T., Hu S. X., Jiang Y. D., Zhong X. D. (2014). Effect of dietary lipid level on growth, feed utilization and body composition of juvenile giant croaker Nibea japonica. Aquaculture 434, 145–150. doi: 10.1016/j.aquaculture.2014.08.012
Hansen J.Ø., Berge G. M., Hillestad M., Krogdahl Å., Galloway T. F., Holm H., et al. (2008). Apparent digestion and apparent retention of lipid and fatty acids in Atlantic cod (Gadus morhua) fed increasing dietary lipid levels. Aquaculture 284 (1-4), 159–166. doi: 10.1016/j.aquaculture.2008.07.043
Helland S. J., Grisdale-Helland B. (1998). The influence of replacing fish meal in the diet with fish oil on growth, feed utilization and body composition of Atlantic salmon (Salmo salar) during the smoltification period. Aquaculture 162 (1-2), 1–10. doi: 10.1016/S0044-8486(98)00206-3
He A. Y., Ning L. J., Chen L. Q., Chen Y. L., Xing Q., Li J. M., et al. (2015). Systemic adaptation of lipid metabolism in response to low and high-lipid diet in Nile tilapia (Oreochromis niloticus). Physiol. Rep. 3 (8), e12485. doi: 10.14814/phy2.12485
Her G. M., Chiang C. C., Chen W. Y., Wu J. L. (2003). In vivo studies of liver-type fatty acid binding protein (L-FABP) gene expression in liver of transgenic zebrafish (Danio rerio). FEBS Lett. 538 (1-3), 125–133. doi: 10.1016/S0014-5793(03)00157-1
Huang W. B., Yan X. B., Liu H., Tan B. P., Suo X. X., Pan S. M., et al. (2022). Effects of vitamin e supplementation of a high-lipid diet on the growth and biochemical parameters of hybrid groupers (♀ Epinephelus fuscoguttatus × ♂ E. lanceolatus). Front. Mar. Sci. 9. doi: 10.3389/fmars.2022.924018
Huang H. L., Zhang Y., Cao M. Y., Xue L. Y., Shen W. L. (2018). Effects of fasting on the activities and mRNA expression levels oflipoprotein lipase (LPL), hormone-sensitive lipase (HSL) and fatty acid synthetase (FAS) in spotted seabass (Lateolabrax maculatus). Fish Physiol. Biochem. 44 (1), 387–400. doi: 10.1007/s10695-017-0442-4
Hubacek J. A., Bobkova D. (2006). Role of cholesterol 7α-hydroxylase (CYP7A1) in nutrigenetics and pharmacogenetics of cholesterol lowering. Mol. Diagn. Ther. 10 (2), 93–100. doi: 10.1007/BF03256448
Jantrarotai W., Sitasit P., Rajchapakdee S. (1994). The optimum carbohydrate to lipid ratio in hybrid clarias catfish (Clarias macrocephalus × C. gariepinus) diets containing raw broken rice. Aquaculture 127 (1), 61–68. doi: 10.1016/0044-8486(94)90192-9
Jiang Y. D., Wang J. T., Han T., Li X. Y., Hu S. X. (2015). Effect of dietary lipid level on growth performance, feed utilization and body composition by juvenile red spotted grouper (Epinephelus akaara). Aquac. Int. 23 (1), 99–110. doi: 10.1007/s10499-014-9801-7
Jin Y., Tian L. X., Zeng S. L., Xie S. W., Yang H. J., Liang G. Y., et al. (2013). Dietary lipid requirement on non-specific immune responses in juvenile grass carp (Ctenopharyngodon idella). Fish Shellfish Immunol. 34 (5), 1202–1208. doi: 10.1016/j.fsi.2013.01.008
Kim K. N., Joo E. S., Kim K. I., Kim S. K., Yang H. P., Jeon Y. J. (2005). Effect of chitosan oligosaccharides on cholesterol level and antioxidant enzyme activities in hypercholesterolemic rat. J. Korean Soc. Food Sci. Nutr. 34 (1), 36–41. doi: 10.3746/jkfn.2005.34.1.036
Leaver M. J., Bautista J. M., Björnsson B. T., Jönsson E., Krey G., Tocher D. R., et al. (2008). Towards fish lipid nutrigenomics: current state and prospects for fin-fish aquaculture. Rev. Fish. Sci. 16 (1), 73–94. doi: 10.1080/10641260802325278
Lee S. M., Jeon I. G., Lee J. Y. (2002). Effects of digestible protein and lipid levels in practical diets on growth, protein utilization and body composition of juvenile rockfish (Sebastes schlegeli). Aquaculture 211 (1-4), 227–239. doi: 10.1016/S0044-8486(01)00880-8
Lei Y. J., Yan J., Tang Z. T., Zou C. X., Yang P. H., Zhang Y. S., et al. (2020). Effects of dietary lipid levels on growth performance and meat quality of juvenile Dongtingking crucian carp. Chin. J. Anim. Nutr. 32 (12), 5839–5849. doi: 10.3969/j.issn.1006-267x.2020.12.037
Leng X. J., Wu X. F., Tian J., Li X. Q., Guan L., Weng D. C. (2012). Molecular cloning of fatty acid synth ase from grass carp (Ctenopharyngodon idella) and the regulation of its expression by dietary lipid level. Aquac. Nutr. 18 (5), 551–558. doi: 10.1111/j.1365-2095.2011.00917.x
Liang X. F., Oku H., Ogata H. Y. (2002). The effects of feeding condition and dietary lipidlevel on lipoprotein lipase gene expression in liver and visceral adipose tissue of red sea bream Pagrus major. Comp. Biochem. Physiol. Part A: Mol. Integr. Physiol. 131 (2), 335–342. doi: 10.1016/S1095-6433(01)00481-0
Li S. L., Mai K. S., Xu W., Yuan Y. H., Zhang Y. J., Zhou H. H., et al. (2016). Effects of dietary lipid level on growth, fatty acid composition, digestive enzymes and expression of some lipid metabolism related genes of orange-spotted grouper larvae (Epinephelus coioides). Aquac. Res. 47 (8), 2481–2495. doi: 10.1111/are.12697
Liu H., Li L., Stephen A., Ze T., Wei F., Tan B., et al. (2021). Effects of dietary yeast culture supplementation on growth, intestinal morphology, immunity, and disease resistance in Epinephelus fuscoguttatus ♀ × Epinephelus lanceolatu ♂. J. Guangdong Ocean Univ. 41, 1–11. doi: 10.3969/j.issn.1673-9159.2021.03.001
Liu B., Tang Y. K., Yu J. H., Xie J., Ge X. P. (2008). Effects of dietary lipid on growth, glucokinase and glucose-6-phosphatase activity and gene expression of culter alburnus. J. Fish. Sci. China 15 (6), 1024–1033. doi: 10.3969/j.issn.1005-8737-(2008)06-1024-10
Liu Y., Tian W. X., Ma X. F., Ding W. J. (2010). Evaluation of inhibition of fatty acid synthase by ursolic acid: Positive cooperation mechanism. Biochem. Biophys. Res. Commun. 392 (3), 386–390. doi: 10.1016/j.bbrc.2010.01.031
Liu Y., Wang J. Y., Li B. S., Qiao H. J., Liu X. D., Hao T. T., et al. (2016). Effects of cobalt methionine on growth, mineral element deposition and liver enzyme activity in juveniles of hybrid grouper (Epinephelus fuscointestinestatus♀ × E. lanceolatu ♂). J. Fish. Sci. China 23 (3), 574–583. doi: 10.3724/SP.J.1118.2016.15351
Livak K. J., Schmittgen T. D. (2001). Analysis of relative gene expression data using real-time quantitative PCR and the 2–ΔΔCT method. Methods 25 (4), 402–408. doi: 10.1006/meth.2001.12ja62
López L. M., Durazo E., Viana M. T., Drawbridge M., Bureau D. P. (2009). Effect of dietary lipid levels on performance, body composition and fatty acid profile of juvenile white seabass (Atractoscion nobilis). Aquaculture 289 (1-2), 101–105 doi: 10.1016/j.aquaculture.2009.01.003
López L. M., Torres A. L., Durazo E., Drawbridge M., Bureau D. P. (2006). Effects of lipid on growth and feed utilization of white seabass (Atractoscion nobilis) fingerlings. Aquaculture 253 (1-4), 557–563. doi: 10.1016/j.aquaculture.2005.08.007
Lu Z., He H., Huang X., Huang W. (2022). Effects of hypoxic stress on antioxidant and energy metabolism of hybrid grouper (♀ Epinephelus fuscoguttatus × ♂ Epinephelus lanceolatus). J. Guangdong Ocean Univ. 42, 13–19. doi: 10.3969/j.issn.1673-9159.2022.01.003
Luo Z., Tan X. Y., Liu X. J., Wen H. (2011). Effect of dietary betaine levels on growth performance and hepatic intermediary metabolism of GIFT strain of Nile tilapia Oreochromis niloticus reared in freshwater. Aquac. Nutr. 17 (4), 361–367. doi: 10.1111/j.1365-2095.2010.00805.x
Lu K. L., Xu W. N., Li J. Y., Li X. F., Huang G. Q., Liu W. B. (2013a). Alterations of liver histology and blood biochemistry in blunt snout bream (Megalobrama amblycephala) fed high lipid diets. Fish. Sci. 79 (4), 661–671. doi: 10.1007/s12562-013-0635-4
Lu K. L., Xu W. N., Li X. F., Liu W. B., Wang L. N., Zhang C. N. (2013b). Hepatic triacylglycerol secretion, lipid transport and tissue lipid uptake in blunt snout bream (Megalobrama amblycephala) fed high lipid diet. Aquaculture 408, 160–168. doi: 10.1016/j.aquaculture.2013.06.003
Ma F., Li X. Q., Li B. A. (2015). Effects of extruded and pelleted diets with differing protein levels on growth and nutrient retention of tilapia, (Oreochromis niloticus♀ × O. aureus♂). Aquac. Int. 23 (6), 1341–1356. doi: 10.1007/s10499-015-9888-5
Monge‐Ortiz R., Tomás-Vidal A., Rodriguez-Barreto D., Martínez-Lllorens S., Pérez J. A., Jover-Cerdá M., et al. (2018). Replacement of fish oil with vegetable oil blends in feeds for greater amberjack (Seriola dumerili) juveniles: Effect on growth performance, feed efficiency, tissue fatty acid composition and flesh nutritional value. Aquac. Nutr. 24 (1), 605–615. doi: 10.1111/anu.12595
Nanton D. A., Lall S. P., McNiven M. A. (2001). Effects of dietary lipid level on liver and muscle lipid deposition in juvenile haddock (Melanogrammus aeglefinus). Aquac. Res. 32 (1), 225–234. doi: 10.1046/j.1355-557x.2001.00019.x
Peng S. M., Zhang C. J., Gao Q. X., Shi Z. H., Chen C., Wang J. G. (2017). Growth performance and metabolic response of juvenile grouper (Epinephelus moara) fed low dietary protein and high lipid levels. J. Appl. Ichthyol. 33 (4), 790–796. doi: 10.1111/jai.13361
Perez‐Velazquez M., Minjarez-Osorio C., González-Félix M. L. (2017). Effect of dietary lipid level on growth performance, feed utilization and body composition of totoaba (Totoaba macdonaldi). Aquac. Res. 48 (5), 2607–2617. doi: 10.1111/are.13002
Puigserver P., Adelmant G., Wu Z., Fan M., Xu J., O'Malley B., et al. (1999). Activation of PPARγ coactivator-1 through transcription factor docking. Science 286 (5443), 1368–1371. doi: 10.1126/science.286.5443.1368
Qin C. J., Chen L. Q., Li E. C., Xie P. Y., Yue X. J., Wang Y. M. (2013). Effect of feed lipid level on growth and lipid metabolism of fish. Fish. Sci. 32 (8), 485–491. doi: 10.16378/j.cnki.1003-1111.2013.08.011
Qiu H., Jin M., Li Y., Lu Y., Hou Y. M., Zhou Q. C. (2017). Dietary lipid sources influence fatty acid composition in tissue of Large yellow croaker (Larmichthys crocea) by regulating triacylglycerol synthesis and catabolism at the transcriptional level. PloS One 12 (1), e0169985. doi: 10.1371/journal.pone.0169985
Rahimnejad S., Bang I. C., Park J. Y., Sade A., Choi J., Lee S. M. (2015). Effects of dietary protein and lipid levels on growth performance, feed utilization and body composition of juvenile hybrid grouper, (Epinephelus fuscointestinestatus♀×E.lanceolatus♂). Aquaculture 446, 308–313. doi: 10.1016/j.aquaculture.2015.05.019
Rollin X., Médale F., Gutieres S., Blanc D., Kaushik S. J. (2003). Short- and long-term nutritional modulation of acetyl-CoA carboxylase activity in selected tissues of rainbow trout (Oncorhynchus mykiss). Br. J. Nutr. 89 (6), 803–810. doi: 10.1079/BJN2003844
Santos J. D., Burkow I. C., Jobling M. (1993). Patterns of growth and lipid deposition in cod (Gadus morhua) fed natural prey and fish-based feeds. Aquaculture 110 (2), 173–189. doi: 10.1016/0044-8486(93)90271-Y
Schiekofer S., Kleber M. E., Maerz W., Rasche F. M., Schneider J. G. (2017). The proline 7 substitution in the preproneuropeptide y is associated with higher hepatic lipase activity In vivo. Int. J. Endocrinol. 2017, 1–7. doi: 10.1155/2017/2869090
Shiau S. Y., Lan C. W. (1996). Optimum dietary protein level and protein to energy ratio for growth of grouper (Epinephelus malabaricus). Aquaculture 145 (1-4), 259–266. doi: 10.1016/S0044-8486(96)01324-5
Smirnova E., Goldberg E. B., Makarova K. S., Lin L., Brown W. J., Jackson C. L. (2006). ATGL has a key role in lipid droplet/adiposome degradation in mammalian cells. EMBO Rep. 7 (1), 106–113. doi: 10.1038/sj.embor.7400559
Song L. P., Han B., Mao S. Q., Wang A. Y., Hu B. (2010). Effects of lipid levels on the growth and intestinal lipase activity of Hephaestus fuliginosus. J. Guangdong Ocean Univ. 30 (1), 13–17. doi: 10.3969/j.issn.1673-9159(2010)01-0013-05
Stich V., Harant I., Glisezinski I. D., Crampes F., Berlan M., Kunesova M., et al. (1997). Adipose tissue lipolysis and hormone-sensitive lipase expression during very-low-calorie diet in obese female identical twins. J. Clin. Endocrinol. Metab. 82 (3), 739–744. doi: 10.1210/jcem.82.3.3793
Sun Y., Jiang Z. Q., Li Y. Q., Mao M. G., Wu H., Gong X. (2013). Effects of dietary lipid levels on the activity of antioxidant enzymes and tissue structure in the liver of juvenile Takifugu rubripes. J. Guangdong Ocean Univ. 33 (3), 27–32. doi: 10.3969/j.issn.1673-9159(2013)03-0027-06
Sztalryd C., Kraemer F. B. (1994). Regulation of hormone-sensitive lipase during fasting. Am. J. Physiol. Endocrinol. Metab. E179. 266 (2), 179–185. doi: 10.1152/ajpendo.1994.266.2
Tocher D. R. (2003). Metabolism and functions of lipids and fatty acids in teleost fish. Rev. Fish. Sci. 11 (2), 107–184. doi: 10.1080/713610925
Torstensen B. E., Frøyland L., Lie Ø. (2004). Replacing dietary fish oil with increasing levels of rapeseed oil and olive oil–effects on Atlantic salmon (Salmo salar l.) tissue and lipoprotein lipid composition and lipogenic enzyme activities. Aquac. Nutr. 10 (3), 175–192. doi: 10.1111/j.1365-2095.2004.00289.x
Tu G. X., Chen G., Zhou H., Wang S., Ji D. L., Zhang J. D., et al. (2012). Effects of 3 protein sources in place of fishmeal on blood parameters of juvenile Epinephelus fuscoguttatus. J. Guangdong Ocean Univ. 32 (4), 12–19. doi: 10.3969/j.issn.1673-91599(2012)04-0012-08
Umino T., Nakagawa H., Arai K. (1996). Development of adipose tissue in the juvenile red sea bream. Fish. Sci. 62 (4), 520–523. doi: 10.2331/fishsci.62.520
Van Herpen N. A., Schrauwen‐Hinderling V. B. (2008). Lipid accumulation in non-adipose tissue and lipotoxicity. Physiol. Behav. 94 (2), 231–241. doi: 10.1016/j.physbeh.2007.11.049
Verreth J., Coppoolse J., Segner H. (1994). The effect of low HUFA- and high HUFA-enriched artemia, fed at different feeding levels, on growth, survival, tissue fatty acids and liver histology of clarias gariepinus larvae. Aquaculture 126 (1-2), 137–150. doi: 10.1016/0044-8486(94)90255-0
Wang X. X., Li Y. J., Hou C. L., Gao Y., Wang Y. Z. (2015). Physiological and molecular changes in large yellow croaker (Pseudosciaena crocea) with high-lipid diet-induced fatty liver disease. Aquac. Res. 46 (2), 272–282. doi: 10.1111/are.12176
Wang J. T., Liu Y. J., Tian L. X., Mai K. S., Du Z. Y., Wang Y., et al. (2005). Effect of dietary lipid level on growth performance, lipid deposition, hepatic lipogenesis in juvenile cobia (Rachycentron canadum). Aquaculture 249 (1-4), 439–447. doi: 10.1016/j.aquaculture.2005.04.038
Wang A. M., Lyu F., Yang W. P., Yu Y. B., Han G. M., Wang C. W., et al. (2010). Effects of dietary fat levels on growth performance, body fat deposition, muscle composition and digestive enzyme activity in the Carassius auratus gibelio. Chin. J. Anim. Nutr. 22 (3), 625–633. doi: 10.369/j.issn.1006-267x.2010.03.016
Wei H., Li R., Yang Q., Tan B., Ray A., Dong X., et al. (2021). Effects of zn on growth performance, immune enzyme activities, resistance to disease and intestinal flora for juvenile pearl gentian grouper (Epinephelus lanceloatu ♂ × Epinephelus fuscoguttatus ♀) under low fishmeal diet. Aquac. Rep. 21, 100880. doi: 10.1016/j.aqrep.2021.100880
Wong H., Schotz M. C. (2002). The lipase gene family. jour. Lipid Res. 43 (7), 993–999. doi: 10.1194/jlr.R200007-JLR200
Xie R. T., Amenyogbe E., Chen G., Huang J. S. (2021). Effects of feed fat level on growth performance, body composition and serum biochemical indices of hybrid grouper (Epinephelus fuscoguttatus× Epinephelus polyphekadion). Aquaculture 530, 735813. doi: 10.1016/j.aquaculture.2020.735813
Xie D. Z., Yang L. P., Yu R., Chen F., Lu R., Qin C. B., et al. (2017). Effects of dietary carbohydrate and lipid levels on growth and hepatic lipid deposition of juvenile tilapia (Oreochromis niloticus). Aquaculture 479, 696–703. doi: 10.1016/j.aquaculture.2017.07.013
Xu S. L., Huang H., Gao P., Cheng R. M., Zeng D. D., Cheng Y. S. (2021). Residue elimination rule of quinaldine in hybrid grouper (♀Epinephelus fuscointestinestatus × ♂E. lanceolatu). J. Guangdong Ocean Univ. 41 (6), 18–24. doi: 10.3969/j.issn.1673-9159.2021.06.003
Xu L., Wen H. B., Sun G. X., Ma X. Y., Jin W., Hua D., et al. (2020). Effects of fish oil addition on the growth, fat metabolism and blood physiology of eye-plaque-like headfish. J. Dalian Ocean Univ. 35 (1), 39–46. doi: 10.16535/j.cnki.dlhyxb.2019-045
Yi X. W., Zhang F., Xu W., Li J., Zhang W. B., Mai K. S. (2014). Effects of dietary lipid content on growth, body composition and pigmentation of large yellow croaker (Larimichthys croceus). Aquaculture 434, 355–361. doi: 10.1016/j.aquaculture.2014.08.035
Yigit M., Yardim Ö, Koshio S. (2002). The protein sparing effects of high lipid levels in diets for rainbow trout (Oncorhynchus mykiss) with specil reference to reduction of total nitrogen excretion[J]. The Israeli Journal of Aquaculture–Bamidgeh 54(2):79–88.
Young S. G. (1990). Recent progress in understanding apolipoprotein b. Circulation 82 (5), 1574–1594. doi: 10.1161/01.CIR.82.5.1574
Yue Y., Liu G. H., Zheng A. J., Zhang H., Wang X. F., Li T. T., et al. (2012). Modulating the expression of genes regulating key enzymes for lipid metabolism in growing animals. Chin. J. Anim. Nutr. 24, 232–238. doi: 10.3969/j.issn.1006-267x.2012.02.007
Yun B., Mai K. S., Zhang W. B., Xu W. (2011). Effects of dietary cholesterol on growth performance, feed intake and cholesterol metabolism in juvenile turbot (Scophthalmus maximus) fed high plant protein diets. Aquaculture 319 (1-2), 105–110. doi: 10.1016/j.aquaculture.2011.06.028
Zhao P. F., Li F. J., Chen X. R., Chen Y. J., Lin S. M., Zhang L., et al. (2016). Dietary lipid concentrations influence growth, liver oxidative stress, and serum metabolites of juvenile hybrid snakehead (Channa argus♀×Channa maculata♂). Aquac. Int. 24 (5), 1353–1364. doi: 10.1007/s10499-016-9993-0
Zhao J., Wen X. B., Li S. K., Zhu D. S., Li Y. Y. (2015). Effects of dietary lipid levels on growth, feed utilization, body composition and antioxidants of juvenile mud crab (Scylla paramamosain). Aquaculture 435, 200–206. doi: 10.1016/j.aquaculture.2014.09.018
Zhao J. K., Zhang J. D., Chen G., Tang B. G., Wang Z. L., Huang J. S. (2019). Operational effect of hybrid grouper (Epinephelus fuscointestinestatus ♀ × E. lanceolatu ♂) cultured in circulating water system. J. Guangdong Ocean Univ. 39 (2), 118–125. doi: 10.3969/j.issn.1673-9159.2019.02.015
Zhou H., Chen G., Ji D. L., Wang S., Zhang J. D. (2012). Effects of 3 kinds of protein substitutes for fishmeal on growth, body composition and energy budget of Epinephelus fuscoguttatus. J. Guangdong Ocean Univ. 32 (6), 10–16. doi: 10.3969/j.issn.1673-9159(2012)06-0010-07
Zhou Y., Guo W. C., Yang Z. G., Zhang K. (2001). Advances in fish hematological index research. J. Shanghai Ocean Univ. 10 (2), 163–165.doi: 10.3969/j.issn.1004-7271(2001)02-0163-03
Zhou P., Wang M., Xie F., Deng D. F., Zhou Q. (2016). Effects of dietary carbohydrate to lipid ratios on growth performance, digestive enzyme and hepatic carbohydrate metabolic enzyme activities of large yellow croaker (Larmichthys crocea). Aquaculture 452, 45–51. doi: 10.1016/j.aquaculture.2015.10.010
Zhu T. T., Jin M., Sun P., Li C. C., Ma H. N., Luo J. X., et al. (2018). Effects of dietary lipid levels on body parameters, tissue fatty acid composition, serum biochemical parameters and liver antioxidant properties in largemouth bass. Chin. J. Anim. Nutr. 30 (1), 126–137. doi: 10.3969/j.issn.1006-267x.2018.01.017
Keywords: dietary lipid levels, ♀Epinephelus fuscointestinestatus × ♂ E. lanceolatu, growth performance, lipid metabolism, hepatic histology
Citation: Suo X, Yan X, Tan B, Pan S, Li T, Liu H, Huang W, Zhang S, Yang Y and Dong X (2022) Lipid metabolism disorders of hybrid grouper (♀Epinephelus fuscointestinestatus × ♂E. lanceolatu) induced by high-lipid diet. Front. Mar. Sci. 9:990193. doi: 10.3389/fmars.2022.990193
Received: 09 July 2022; Accepted: 16 September 2022;
Published: 05 October 2022.
Edited by:
Youji Wang, Shanghai Ocean University, ChinaReviewed by:
Ting Chen, South China Sea Institute of Oceanology, (CAS), ChinaYong Tian, Zhejiang Academy of Agricultural Sciences, China
Copyright © 2022 Suo, Yan, Tan, Pan, Li, Liu, Huang, Zhang, Yang and Dong. This is an open-access article distributed under the terms of the Creative Commons Attribution License (CC BY). The use, distribution or reproduction in other forums is permitted, provided the original author(s) and the copyright owner(s) are credited and that the original publication in this journal is cited, in accordance with accepted academic practice. No use, distribution or reproduction is permitted which does not comply with these terms.
*Correspondence: Xiaohui Dong, dongxiaohui2003@163.com