- 1CAS Key Laboratory of Tropical Marine Bio-Resources and Ecology, Guangdong Provincial Key Laboratory of Applied Marine Biology, South China Sea Institute of Oceanology, Innovation Academy of South China Sea Ecology and Environmental Engineering, Chinese Academy of Sciences, Guangzhou, China
- 2Southern Marine Science and Engineering Guangdong Laboratory (Guangzhou), Guangzhou, China
- 3CAS-HKUST Sanya Joint Laboratory of Marine Science Research and Key Laboratory of Tropical Marine Biotechnology of Hainan Province, Tropical Marine Biological Research Station in Hainan, Chinese Academy of Sciences, Sanya, China
Global climate change has caused extensive loss of biodiversity and ecosystem function globally, particularly in coral reefs in shallow tropical waters. As the corallivorous snails represent considerable disturbances to coral reefs across Indo-Pacific region, it is becoming a threat to coral health and recovery in the Hainan Island, South China Sea, but current-knowledge gap remains on the spatial distribution, prey preferences, and the influence factors related. Thus, we investigated the abundance distribution of Drupella spp. in relation to coral-prey selectivity, availability, and nutritional value. The snail density was heterogeneously distributed in the study area with an average of 0.72 ± 0.32 ind m−2 (varying from 0.09 ± 0.03 to 1.78 ± 0.39 ind m−2), and the snail density was found generally higher in locations with higher coral cover, which resulted in much lower density where their preferred corals became scarce. Despite the snails showing a positive preference for Acroporidae corals, they displayed dietary plasticity by changing with prey abundance and availability. Further, we found the electivity index of the coral prey related to the substrate types, and the nutritional value of prey was an additional important determinant in the prey preference, suggesting that the specific reef habitat and environmental context may alter the prey preference of Drupella spp. Combined, these results provide preliminary evidence that Drupella spp. is among the common corallivories in the reef regions of Hainan Island, and we recommend that large-scale monitoring programs assess the spatial-temporal trends to better understand the dynamics of predation linked to both anthropogenic and natural impacts.
Introduction
Coral reefs are highly biodiverse but are among the most threatened habitats on Earth, facing unprecedented risk from global climate change, overfishing, and pollution (Hughes et al., 2018; Wolff et al., 2018; MacNeil et al., 2019). Due to these anthropogenic and natural disturbances, coral reefs are continuously deteriorating across the world (Gardner et al., 2003; Hoegh-Guldberg et al., 2007; Hughes et al., 2017). Although individual environmental threats (e.g., temperature, pH) to coral reefs have been well studied, the negative effect of corallivores could aggravate the reef degradation process (Rice et al., 2019). To mitigate losses in coral cover, it is essential to seek to identify the ecological processes and make well-informed conservation decisions that promote coral survivorship.
Feeding activities of corallivores have negative effects on coral growth and fitness, varying from minor to lethal damage and subsequently leading to fundamental changes in the reef state (Turner, 1994b; Rotjan and Lewis, 2008; Rice et al., 2019). For instance, an outbreak of crown-of-thorns starfish (Acanthaster spp., COTS) has caused high-coral mortality and reduced coral cover by up to 80% (Pratchett et al., 2017). High densities of other corallivores such as Coralliophila spp. and Drupella spp. have been reported to impact corals strongly in the Indo-Pacific, Western Indian Ocean, and Asia (Moyer et al., 1982; Boucher, 1986; Mcclanahan, 1994; Bruckner et al., 2017).
The genus Drupella is among the well-known corallivores occurring on coral reefs of the Indo-Pacific Ocean (Turner, 1994b; Claremont et al., 2011). Generally, these corallivorous gastropods are found in coral reef regions at a low density of 0–2 ind m−2 (Cumming, 1999; Cumming 2009a), while the outbreak of Drupella could reduce the live coral cover by more than 75% in the coral reef areas with a mean density of 14.73 ± 4.37 ind m−2 (Turner, 1994a; Turner 1994b). There were large aggregation or outbreak events of Drupella spp. have been observed in various regions, including Western Australia (Ayling and Ayling, 1987; Turner, 1994b), Great Barrier Reef (Cumming, 1999), Red Sea (Antonius and Riegl, 1998), Kenya (Mcclanahan, 1994), Thailand (Moerland et al., 2016; Scott et al., 2017), Maldives (Bruckner et al., 2017) and Hong Kong (Cumming and McCorry, 1998; Morton and Blackmore, 2009). Both anthropogenic (overfishing of natural predators, coral reef damage, and terrestrial run-off) and natural (changes in temperature and salinity) causes have been proposed to explain these events (Turner, 1994b; Turner, 1994a; Cumming, 2009b; Cumming, 2009a).
Previous studies have reported that these corallivorous snails display significant feeding selectivity and are affected by the relative abundance of each coral taxon (Morton and Blackmore, 2009). Many corallivores exhibit high dietary plasticity with respect to their coral prey, feeding on less preferred corals when their favored corals are not abundant. For instance, Drupella spp. had shifted their dietary preference for coral species of Acroporidae and Pocilloporidae to the less preferred fungiid corals after a major coral bleaching event in Thailand (Hoeksema et al., 2013; Moerland et al., 2016). Nevertheless, Morton et al. (2002) found that the snails of D. rugosa still preferred the low-abundance coral Acropora spp. in the reefs of Hong Kong despite being primarily dominated by massive corals. Hence, the coral assemblage composition might influence their diet varies as well. Additionally, the importance of the nutritional value of the prey species to prey preference has received attention in COTS (Pratchett et al., 2014; Keesing, 2021), while the data on the food selectivity of Drupella spp. is scarce.
In recent years, the aggregations of four species of Drupella spp., named D. cornus, D. rugose, D. fragum, and D. margariticola (Zhang and Zhang, 2007; Peng et al., 2014), have been constantly observed in the reef regions of Hainan Island. However, information regarding the snail aggregation and the coral status in these reef regions is still limited. Furthermore, the coral reefs in Hainan Island have suffered more from coastal development, pollution, overfishing, and destructive fishing practices than climate change (Hughes et al., 2013), which resulted in the live coral cover of the fringing reefs declining by more than 80% up to now. Nevertheless, both coral loss and recovery vary between each reef due to the different occurrence of disturbances (Lian et al., 2010; Zhang et al., 2016; Huang et al., 2020), and consequently, variation in both coral species abundance and cover may influence the ecology of the related organisms such as corallivores, in different ways.
The impact of corallivores in Hainan Province has recently attracted scientific attention, focusing on the outbreaks of A. planci (Li et al., 2019a; Li et al., 2019b). However, no information is available on the spatial distribution of Drupella spp., and the feeding ecology of these corallivorous snails is still poorly described in Hainan Island. The study aimed to investigate the distribution patterns and prey selection of Drupella spp. and the associated factors influencing its feeding preference in the southeastern Hainan Island. In particular, we tested the hypotheses that (1) the abundance distribution of Drupella spp. varies with coral abundance; and (2) reef substrate types and the nutritional value of coral prey are related to the prey selection of the corallivores.
Materials and methods
Study area
A field study was conducted from July 2019 to December 2020 along the coast of the southeastern Hainan Island, South China Sea. Our study area included five locations (Figure 1) with the intensive distribution of reef coral communities to enable convenient sampling and detection of Drupella spp. (Mollusca, Gastropoda, Muricidae).
Xiaozhou Island (XZD) and Xiaodonghai (XDH) are located at the east and west sides of the Luhuitou Peninsula of Sanya, respectively, and the marine environments are significantly affected severely by intensive human activities (e.g., coastal works, tourists activities, sewage discharges) (Titlyanov et al., 2019). Wuzhizhou Island (WZZ) is a small uninhabited island located about 2.7 km off the northern coast of Sanya. However, the marine environments are influenced by direct human activities (e.g., constructions, marine traffic, diving activities) (Huang et al., 2020). Fenjiezhou Island (FJZ) is a small island located at the junction of Lingshui County and Wanning City, and 2.2 km off the east coast of Hainan Island. Due to its exceptional coastal and diving tourism resources, the marine environment of this uninhabited island is affected by coastal construction, tourist activities, and disordered harvesting of marine resources, etc. (Bai et al., 2016). Dazhou Island (DZD) is another uninhabited small island located about 11 km away from the coast, and it has been the National Marine Ecological and Climatic Nature Reserve since September 1990. There are no constructive activity being conducting on it. Therefore, there are two types of sample sites among the five research stations: inshore sites (XZD and XDH) and offshore sites (WZZ, FJZ, and DZD).
Biological and environmental data collection
The shells of the snails are often encrusted with crustose coralline algae and other epifauna, which makes their in situ identification difficult. To avoid misidentification, the snail taxa were identified to the genus level and pooled as Drupella spp.
To examine the effects of variation in coral cover, abundance, and substrate pattern on the population structure, prey preferences, and spatial distribution of Drupella spp., we surveyed five locations along the southeast coast of Hainan Island. According to the accessibility of each site, three replicate belt transects of 20 m in length and 2.5 m on either side, lying parallel to the shoreline, were randomly selected at a depth of 1–3 m at each sample station. The survey depth was set in accordance with the previous studies, which reported that Drupella spp. is mainly distributed in shallower reef areas (Turner, 1994a; Cumming, 2009a; Scott et al., 2017; Hamman, 2018).
The benthic reef community composition was determined by the linear point intercept (LPI) method (Nadon and Stirling, 2006) on the same belt transects. Within each transect, types of substrate were identified as live coral, dead coral, rock, sand, macroalgae, and other sessile invertebrates. During the survey, special emphasis was given to the substrate directly under the Drupella individuals, while the corals were identified to species level to study the detailed prey preference and to broad categories for all other substrate types. Drupella spp. was considered relating to corals whether they were in an aggregation over the living tissue or a dead part of a living coral colony or near the feeding scar, because they should move from one coral colony to another to find food or shelter (Cumming, 1999; Morton et al., 2002).
Statistical analysis
Statistical comparisons of the spatial variation of the predator density and percentage of live coral cover between stations were analyzed using the permutational multivariate analysis of variance (PERMANOVA) with 999 permutations. Furthermore, the feeding preferences of Drupella spp. were investigated using the Ivlev (1961) electivity index (Ei). Ei was estimated using the formula:
For this equation, ri represents the proportion of prey in the diet and pi represents the proportion of prey in the environment. Values of Ei range between 1 (maximum preference) and −1 (maximum avoidance), whereby 0 indicates random feeding. We calculated ri as the frequency of corallivorous snails on a specific i species or genus of coral in relation to all the preyed colonies within each station.
The distribution and abundance of coral species, especially the species used as prey by Drupella spp., are likely to influence the distribution and abundance of the predator. Therefore, the similarity of coral species distribution for stations was analyzed using the ANOSIM test based on the Bray–Curtis similarity coefficient (Clarke and Gorley, 2015). Non-parametric multi-dimensional scaling (nMDS) ordinations were used to visualize the similarities and dissimilarities in coral community composition between stations, with a bubble plot showing the coral species preyed upon by Drupella spp. (Ei >0.5) in each sample station. We took the percentage of live coral cover as the relative abundance of corals at every station.
To explore the relationship between the electivity of coral prey and the benthic variables at each study station, we employed a nonparametric distance-based linear model (DISTLM) along with distance-based redundancy ordination analysis (dbRDA). The DISTLM models the relationship between these variables mentioned and the multivariate Ei of the coral dataset based on a multiple regression model as a way to determine the linear combination of variables that explains the greatest amount of variation in the elected coral community dataset, and it examines the amount of variance explained by each variable. Before analysis, benthic variable data were averaged, and a Draftsman plot was used to assess the collinearity between variables. Variables with a high degree of collinearity (Spearman’s correlation coefficient |r| >0.85) were removed from the DISTLM analyses. Additionally, DISTLM was initially used to analyze the marginal effects of each variable, followed by the application of the “best” model building process with a R2 selection to identify the best set of factors explaining the variation in the preferred coral community.
Additionally, principal component analysis (PCA) was used to investigate the features of the nutritional value of prey of the coral genus. The PCA multivariate analysis of the coral genera nutritional value of prey data, including lipid content (mg cm−2), protein content (mg cm−2), tissue biomass (ash-free dry weight, mg cm−2), Symbiodinium density (×106 cells cm−2), Chlorophyll-a content (Chl-a, μg cm−2), tissue thickness (mm), and polyp density (polyps cm−2). These data were obtained from the Coral Trait Database (Madin et al., 2016) and some published studies (Achituv et al., 1994; Loya et al., 2001; Anthony and Hoegh-Guldberg, 2003; Leuzinger et al., 2003; Edmunds et al., 2014; Qin et al., 2020; Keesing, 2021). The obtained species data were averaged as genus values, and the missing values were filled out using linear regression (Table S2).
All analyses were performed using PRIMER v7 software, including the PERMANOVA+ add-on package (Anderson et al., 2008; Clarke and Gorley, 2015).
Results
Abundance and spatial distribution
During the survey period, we counted individuals of Drupella spp. with an overall average density of 0.72 ± 0.32 ind m−2. Snail density was higher at the offshore sites (WZZ, FJZ, and DZD) compared to the inshore sites (XZD, XDH) (Figure 2A). The variation of snail densities among the stations was found to be statistically significant (p<0.05), with mean densities ranging from 0.09 ± 0.03 ind m−2 at station XDH, up to 1.78 ± 0.39 ind m−2 at station DZD. Similarly, the percent live hard coral cover varied significantly among the stations as well (p<0.05), with the mean value ranging from 0.83 ± 0.60% at station XDH, up to 37.52 ± 0.07% at station WZZ (Figure 2B). The mean number of individuals per aggregation was 4.56 ± 1.28 for all Drupella spp. individuals pooled together; ranging from a minimum of 1 to a maximum of 38 individuals found on a single coral species, Acropora hyacinthus (at DZD).
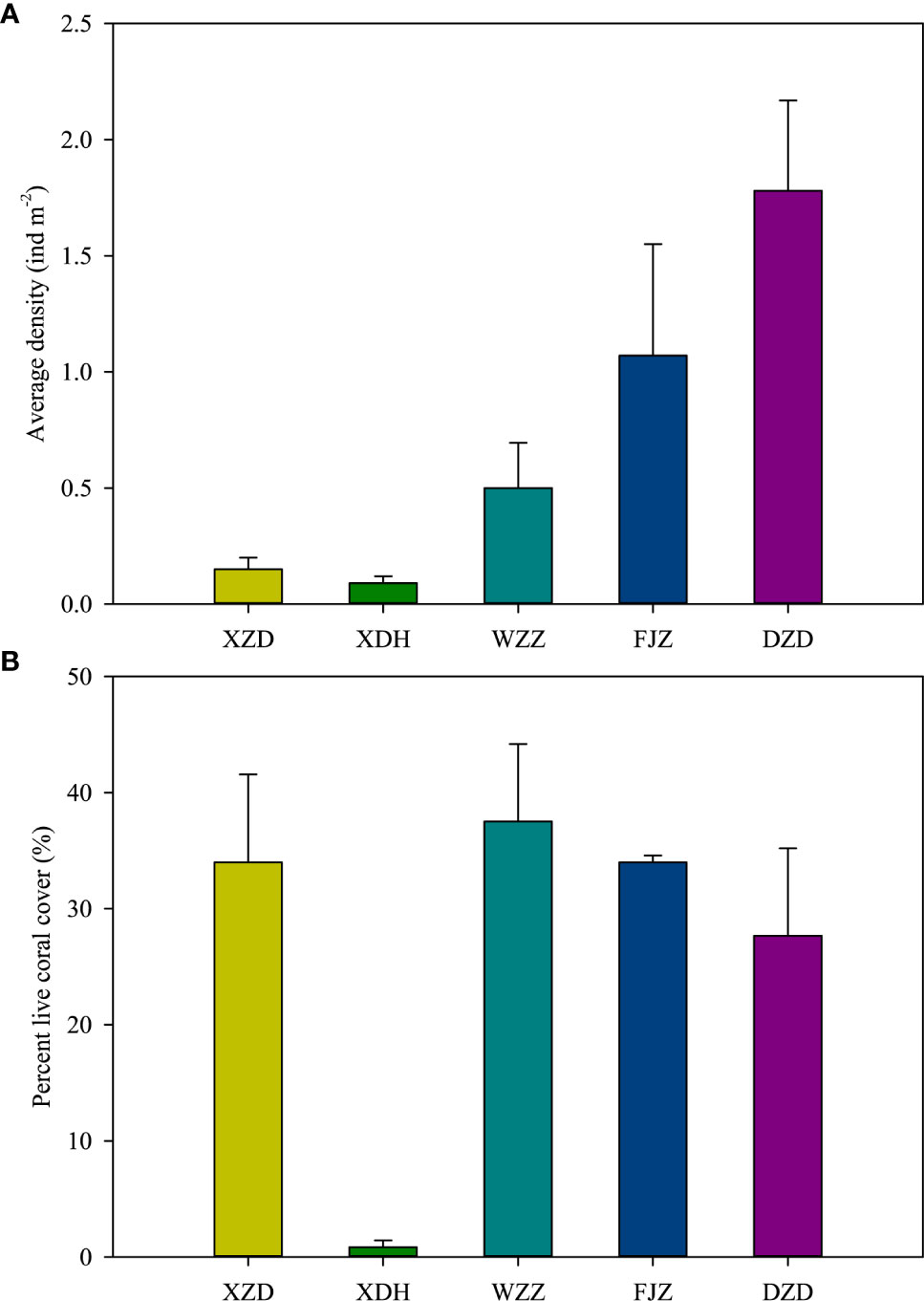
Figure 2 Spatial differences in (A) average snail density and (B) average percent live coral cover for each station. Bars indicate standard error.
There were 70 coral species in the total found among the five research stations (Table S1), of which 30 species belonging to 10 genera were preyed on by Drupella spp. (Tables 1, 2). The number of coral species ranged from four at XDH to 38 at WZZ, and the other benthic reef substrate variables showed significant differences among the research stations, except for sand, macroalgae, and the other sessile invertebrates (Table 3). The top three genera with the highest proportion at each station were Favites (30.39%), Acropora (25.00%), and Platygyra (15.20%) in XZD, Oulastrea (40.00%), Montipora (20.00%), and Favites (20.00%) in XDH, Porites (32.16%), Acropora (24.78%), and Montipora (13.19%) in WZZ, Galaxea (37.09%), Porites (24.48%), and Platygyra (7.09%) in FJZ, Acropora (22.29%), Favites (17.47%), and Acanthastrea (12.05%) in DZD, respectively. The coral community structure varied significantly among the research stations (ANOSIM, global R = 0.62, p = 0.001) (Figure 3).
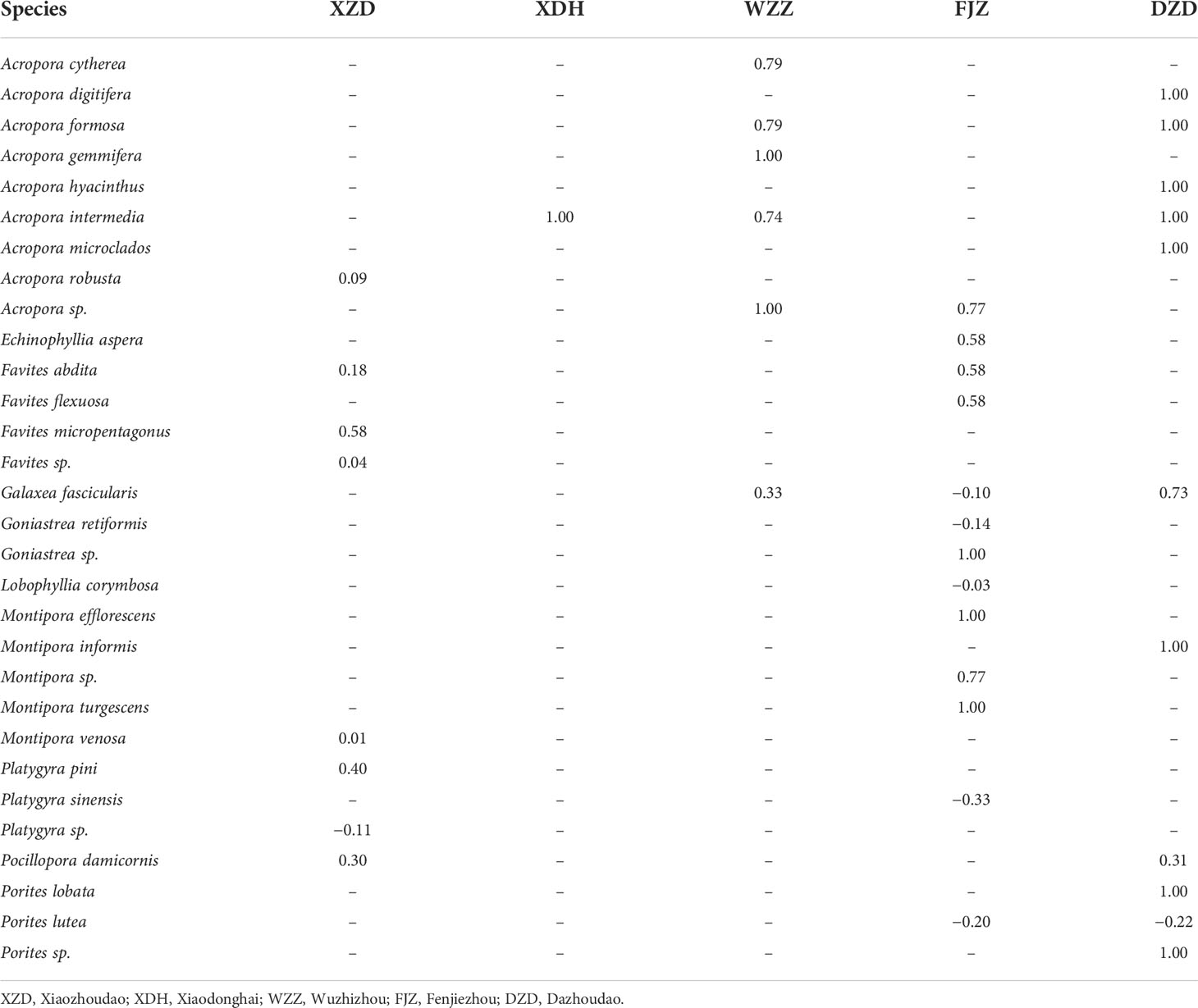
Table 1 Feeding preferences of Drupella spp. according to the Ivlev’s electivity index (Ei) considering the coral species preyed upon at each station.
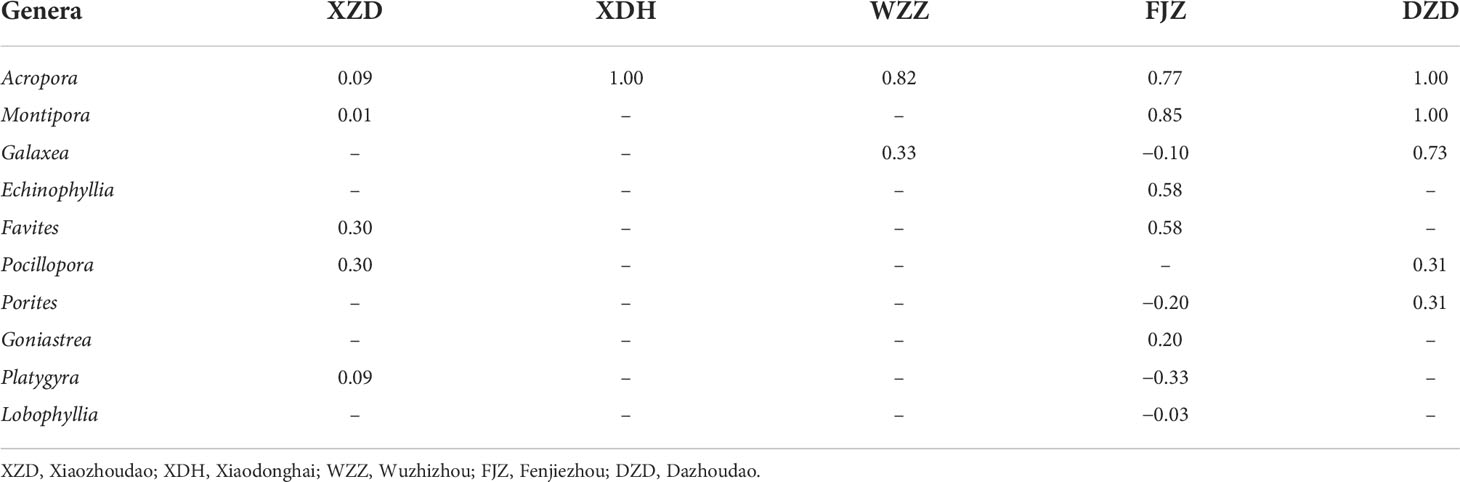
Table 2 Feeding preferences of Drupella spp. according to the Ivlev’s Electivity Index (Ei) considering the coral genera preyed upon at each station.
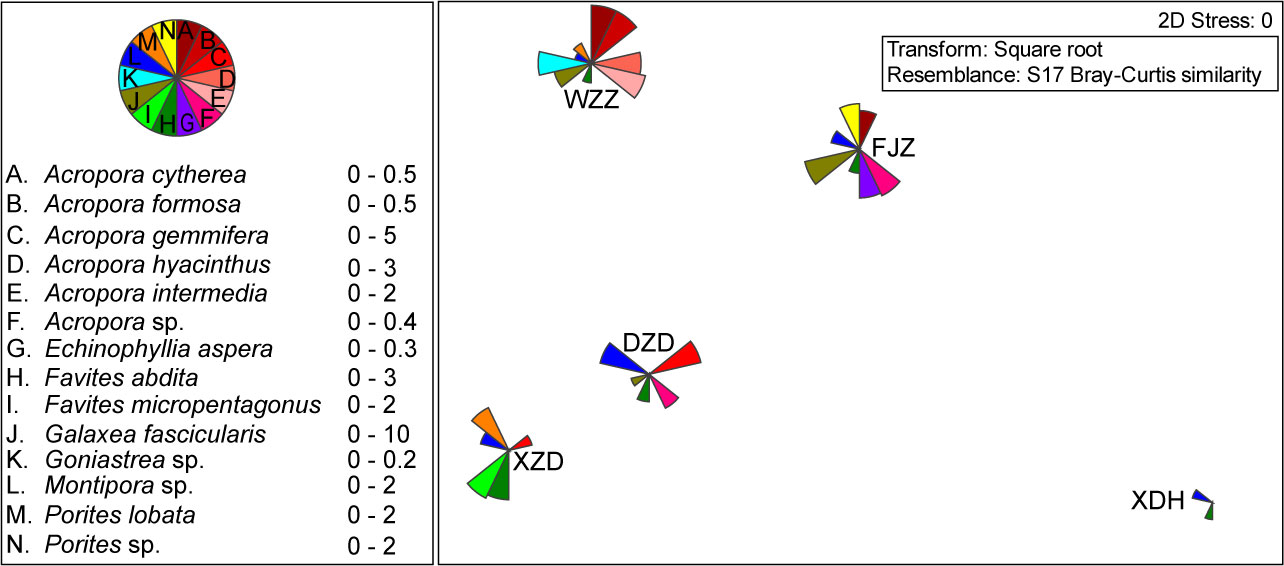
Figure 3 Non-parametric multi-dimensional scaling (nMDS) ordinations visualized the similarities and dissimilarities in coral community composition (living coral cover served as the abundance) between stations, with a bubble plot showing the live cover of the coral species preferred by Drupella spp. (Ei >0.5 at any station) in each sample station (XZD, Xiaozhoudao; XDH, Xiaodonghai; WZZ, Wuzhizhou; FJZ, Fenjiezhou; and DZD, Dazhoudao).
Prey selection and preferences
Prey preference varied at different stations according to the availability of coral prey. Coral species belonging to the family Acroporidae (Acropora and Montipora) were all preferred positively by Drupella spp. among the sample stations. Considering the preferred coral prey (Ei >0.5 at any station) of the snail, there were 20 coral species, and the abundance and distribution of these species were different in the sample stations (ANOSIM, global R = 0.52, p = 0.001), with species A. gemmifera, Montipora sp., and P. lobata being more abundant in the stations XZD and DZD, while the other eight species (A. cytherea, A. formosa, A. hyacinthus, A. intermedia, Acropora sp., G. fascicularis, Goniastrea sp., and Porites sp.) were more abundant in the stations WZD and FJZ (Figure 3, Table 1).
Species belonging to the genera Acropora, Echinophyllia, Favites, Montipora, and Pocillopora had positive values of the electivity index (Ei) in the five sample stations, while the other species had at least one negative value of the index in all stations (Table 1). At the genus level, all the genera of coral prey had positive values of Ei in the sample stations, except for the genera of Galaxea, Porites, Platygyra, and Lobophyllia, which were the avoided prey by Drupella spp. in the station FJZ (Table 2).
Relationship between feeding behavior and benthic variables
The benthic variables were different among the sample stations (SIMPEROF, global π = 0.59, p = 0.001). The Draftsman plots indicated that the number of coral species, other sessile invertebrate and macroalgae were all highly correlated with live coral cover (|r| >0.85, Table S3), and so these variables were excluded from the DISTLM analysis. Additionally, as the dead coral cover was highly correlated with rock (r = −0.95, Table S3), the rock was kept in the analysis based on the forward factor selection process.
The DISTLM analysis indicated that the three benthic variables, including live coral cover, rock, and sand, had a strong relationship with the electivity index of the coral prey at the research stations, although the marginal test showed no statistical significance (p >0.05). The dbRDA included vectors corresponding to these three variables on two axes that explained 91.6% of the fitted variation and 62.4% of the total variation (Figure 4). When considering the variables alone and ignoring all other variables, sand contributed to explaining the highest percentual variance, accounting for 21.03% of the variability in the coral prey communities, followed by rock, accounting for 13.51%, and live coral cover, accounting for 8.79%. The samples corresponding to the relatively high live coral cover stations were distributed along the dbRDA1 axis, while the samples of the different types of stations were dispersed along the dbRDA2 axis.
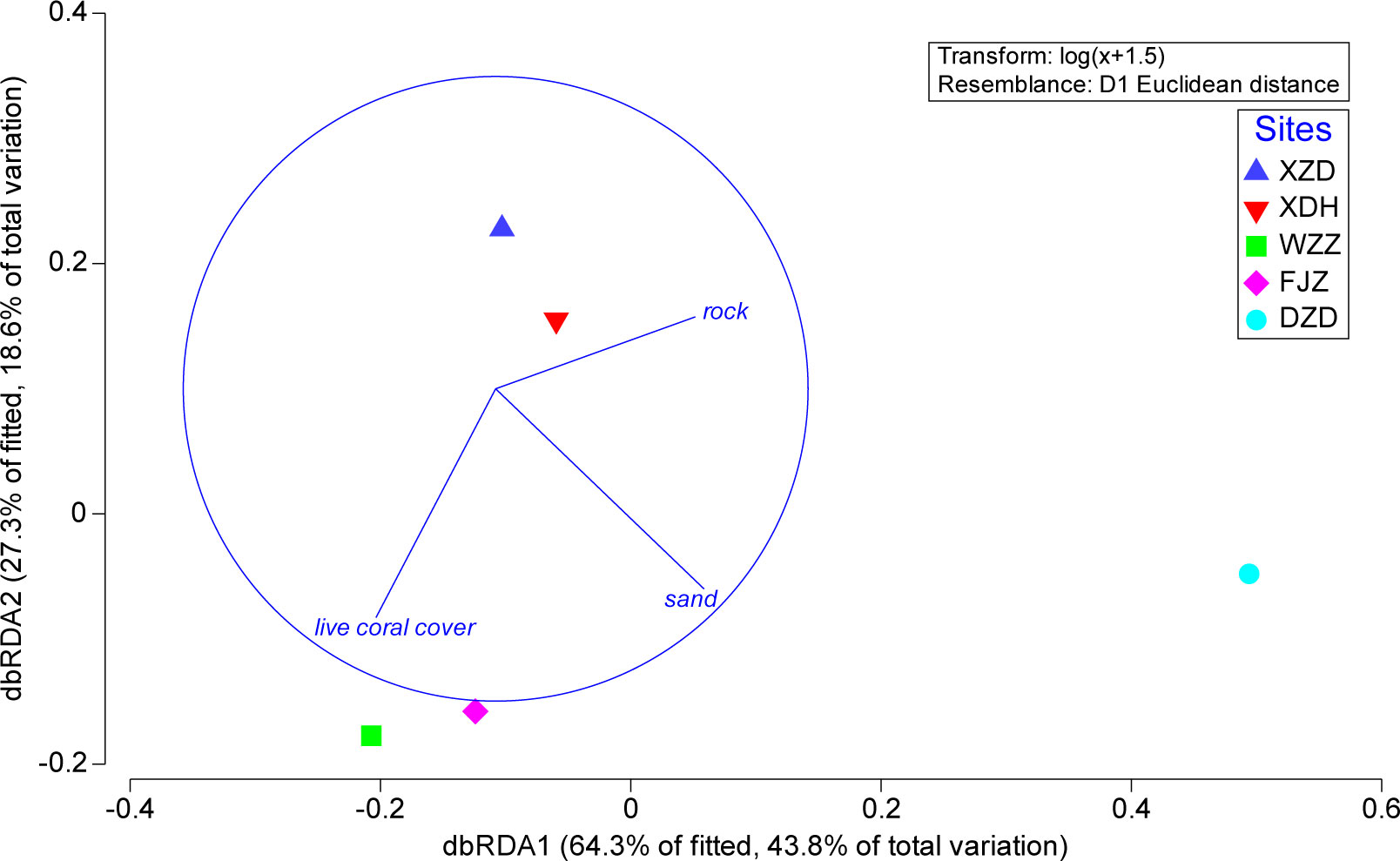
Figure 4 Distance-based redundancy analysis (dbRDA) of the Iviev’s electivity index (Ei) considering the coral prey for each station shows vector overlays of coral species preyed included in the best model by DISTLM analysis (XZD, Xiaozhoudao; XDH, Xiaodonghai; WZZ, Wuzhizhou; FJZ, Fenjiezhou; DZD, Dazhoudao).
Comparative analysis of food value of coral prey
PCA analyses revealed that the coral prey species fell into four significantly different groups (Figure 5). The first group comprised the genus Montipora, characterized by high Chl-a content and delivering high-polyp density, tissue biomass, and a low Symbiodinium density. The second group, comprising the genera Acropora, Pocillopora, and Porites, fell out together along axes of high Symbiodinium density, low Chl-a content, and delivering high-polyp density. The third group, comprising the genera Favites, Galaxea, and Platygyra, was correlated with high-tissue thickness, low-polyp density, and delivered high-lipid content and Symbiodinium density. The fourth group comprised the genera Goniastrea and Lobophyllia, characterized by high-lipid content, protein content, and tissue biomass, and delivered high-tissue thickness, high Chl-a content, and low-polyp density.
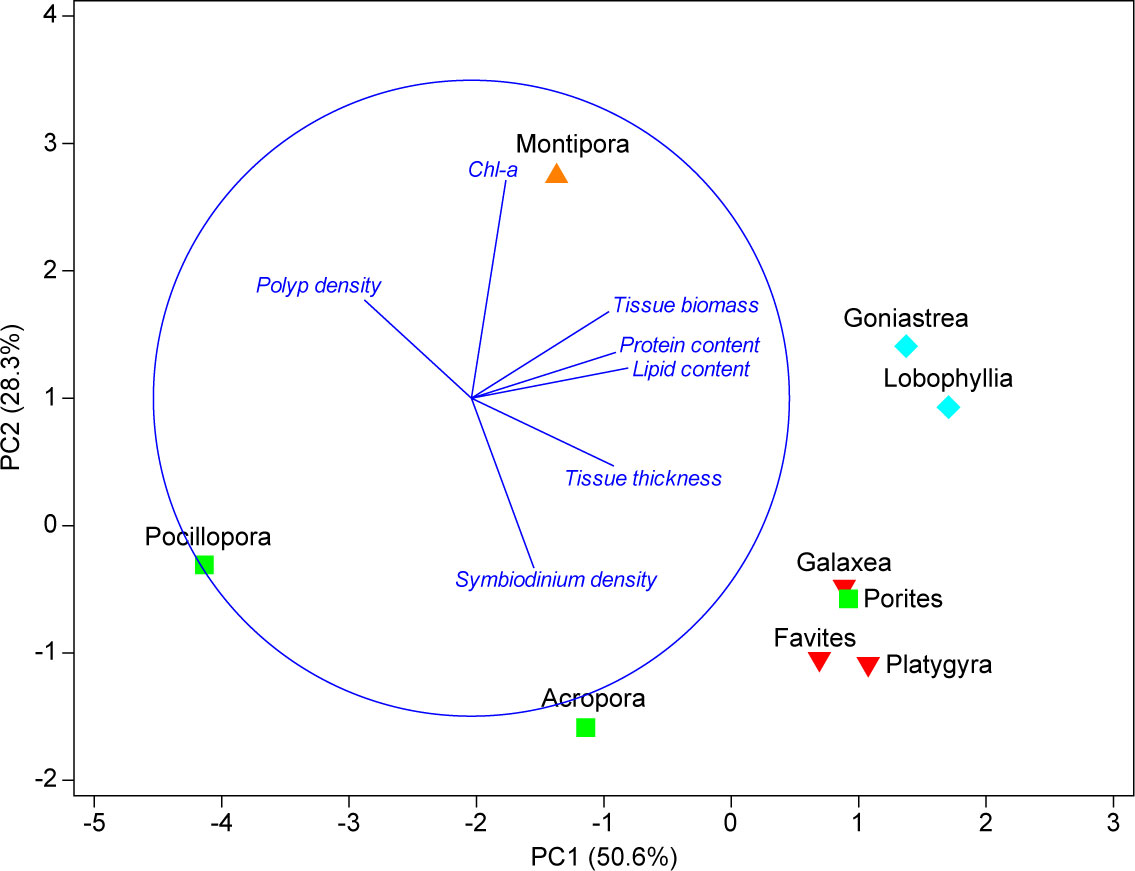
Figure 5 Principal components analysis (PCA) plot of multivariate analysis of coral genera nutritional value of prey data: lipid content, protein content, tissue biomass, Symbiodinium density, Chl-a content, tissue thickness, and polyp density. PCA analyses are based on the data obtained from the Coral Trait Database and published studies. The missing values were filled using a linear regression. The genus Echinophyllia was excluded in these analyses because the data were not available. The triangle, upside-down triangle, square, and diamond symbols indicate the four groups of significant different coral genera based on the SIMPEROF test.
Discussion
In recent years, the overabundance of corallivores has become an increasing threat to coral reef ecosystems across the Indo-Pacific Ocean. In the South China Sea, especially in the near-shore coral reef area, more and more aggregations of Drupella spp. have been observed, which would become a potential roadblock to the regional efforts to recover and restore the degraded coral communities in China. This study revealed the spatial differences in density and prey selection of the corallivorous snail Drupella spp. in the southeastern Hainan Island, South China Sea. Prey preferences of Drupella spp. vary in different stations according to the abundance and availability of coral prey. Corals of the family Acroporidae were preferred positively by Drupella spp. among all research stations, and their favored coral preys are of meaty, nutrition-packed, and vegetarian characteristics. Benthic variables, including live coral cover, rock, and sand, had a strong relationship with the coral prey communities among the research stations.
In this study, the overall mean density of Drupella spp. was lower than that in previous studies (Ayling and Ayling, 1987; Turner, 1994a; Cumming, 1999; Cumming, 2009b; Schoepf et al., 2010; Moerland et al., 2016), but higher than that in a recent study (Saponari et al., 2021). The corallivores did not outbreak because the mean density in each study site (0.09–1.78 ind m−2) never exceeded the outbreak threshold of >3 ind m−2 defined by (Cumming 2009a; Cumming, 2009b). However, the mean density exceeded the level of 0.62 ind m−2 suggested by Bessey et al. (2018) that the snails could outbreak on their preferred coral species if the density exceeded this level. In particular, we found there was a maximum of 38 individuals of a single coral species, Acropora hyacinthus at DZD. Thus, the high current density of the snails remained potentially destructive to the coral communities in the study regions.
The heterogeneity in the density of Drupella spp. recorded at different sites may have been responsible for the anthropogenic activities, such as over-fishing or boating traffic (Ayling and Ayling, 1987; Turner, 1994a), especially the coral collapse in XDH. Additionally, the snail density was found to be higher in the stations with relatively high coral cover than that in the stations with low coral cover across all sites surveyed, such as in FJZ and DZD, independently of the number of coral species. This finding was consistent with the theory of optimal foraging, which assumed that predators fed in such a way as to trade-off between the alternative available prey abundances (Cumming, 2009a; Keesing, 2021). However, we found that the coral cover was relatively high in XZD but with lower snail density. Hence, these between-site variations in the densities of Drupella were apparently not just dependent on the differences in the live coral cover at each station. Specifically, our statistical results indicated that the live coral cover only contributed to explaining the percentual variance of 8.79% for the electivity index of the coral prey, which highlights that the coral cover would not always be the main factor. Additionally, the different abundance of their preferred coral species (Acropora and Montipora) in each station may have been responsible (Morton and Blackmore, 2009). Although they are more mobile, the encounter probability with their preferred prey is probably an additional explanation. Thus, factors other than the live coral cover are probably important in controlling the density of Drupella in different reef regions.
For instance, the highest proportion of the coral taxa we found in each research station may be involved in the impact factors. For instance, the highest proportion of the genera Favites resulted in positive feeding in XZD, although they are the massive growth form of corals. Prior studies reported that the coral growth forms and intraspecific attraction probably played additional roles in the food selection of Drupella spp. (McClanahan, 1997; Schoepf et al., 2010). Corals with branching growth forms were always used as food and shelter (Ayling and Ayling, 1987; Hamman, 2018), especially for the juvenile snail Drupella spp. (McClanahan, 1997; Schoepf et al., 2010; Hamman, 2018).
Our study showed there were significant differences in the coral community composition among the research stations. The different Drupella densities in each site were probably correlated to the distribution pattern of the coral assemblage (Schoepf et al., 2010). Moreover, variation among coral assemblages has also been linked to the snail relative density because the abundance and distribution of their preferred coral prey were different in each sample station. Specifically, the low abundance of their preferred prey (Acropora and Montipora) at XZD was probably responsible for the low density of Drupella. Previous studies revealed that a decrease in the abundance of preferred coral species might result in a higher density of snails in the reefs (Schoepf et al., 2010). Nonetheless, Acropora abundance does not always explain the distribution pattern of Drupella (Turner, 1994b), for the snails could aggregate on the remaining corals if the coral population decreased (Hoeksema et al., 2013; Saponari et al., 2021).
Results from our analysis of spatially explicit data of 30 prey species demonstrated that the corallivorous snail displayed dietary plasticity, consistent with the previous findings that they were able to switch to their less preferred corals in the areas where their preferred prey became scarce (Turner, 1994b; Shafir et al., 2008; Schoepf et al., 2010; Hoeksema et al., 2013; Tsang and Ang, 2015; Saponari et al., 2021). In this study, we found coral species of the family Acroporidae were preyed on positively where the abundance of them was relatively high, such as at WZZ, FJZ, and DZD. However, the snails were confined to their less favorite prey. For instance, they had to prey on Favites spp. and Platygyra spp. when the coral species of Acroporidae were less available at XZD. Similarly, previous observations revealed that Drupella were restricted to preying on massive or encrusting corals where branching corals were not available (Mcclanahan, 1994; Cumming and McCorry, 1998; Morton et al., 2002; Hoeksema et al., 2013; Moerland et al., 2016). Moreover, as found in our study, the snails were found expanding their prey range from branching corals (Acropora and Pocillopora) to a few massive corals (Porites, Favites, Galaxea, etc.) in the Maldives (Bruckner et al., 2017; Saponari et al., 2021) according to the availability of their preferred prey.
Interestingly, previous studies found that some coral species that were not preferentially preyed upon by Drupella, such as corals of the genus Porites (Morton et al., 2002; Hamman, 2018), while they had a positive tendency to be preyed on in the station DZD in this investigation. These results showed that the complex change of coral community composition in this study area influenced the predation preference of the snail Drupella. Additionally, during the survey period, we also found the snails preferred aggregating near the freshly damaged tissues of the corals, which supports evidence from previous observations (Morton et al., 2002; Bright et al., 2015; Bruckner et al., 2017; Hamman, 2018). Kita et al. (2005) reported that the chemicals in the mucus released by damaged coral tissue showed potent feeding-attractant activity toward D. cornus.
Besides the influence factors to the prey preferences of the snail Drupella spp. proposed by previous studies above, other researches indicated that coral growth forms, such as branching growth form, could strongly influence their feeding preferences (Turner, 1994a; Cumming and McCorry, 1998; Cumming, 1999; Schoepf et al., 2010; Saponari et al., 2021). In this research, we found that benthic variables, including live coral cover, rock, and sand, had a strong relationship with the electivity index of the coral prey at the research stations. This indicated that the substrata condition played an important role in determining the prey preference of Drupella spp. This finding is consistent with the reports that the snails showed different reef habitat preferences (Turner, 1994a; Cumming, 1999), such as a high cover of rubble and dead coral (Moerland et al., 2016). However, the correlation was not statistically significant in the present study. This was presumably due to the insufficient sample collection and/or some remaining unclear drivers, although various aspects had been discussed in previous studies, such as coral communities (Morton and Blackmore, 2009; Schoepf et al., 2010; Saponari et al., 2021); depth (Schoepf et al., 2010; Saponari et al., 2021); reef status (Mcclanahan, 1994; Morton et al., 2002). Furthermore, prey selection by Drupella spp. could not only be considered in the multivariable analysis because coral species tested were insufficient. Additionally, environmental stressors, including elevated seawater temperature, reduced salinity, mechanical damage, and coral diseases, were also correlated with the feeding preference of Drupella (Morton et al., 2002; Al-Horani et al., 2011; Nicolet et al., 2013; Tsang and Ang, 2019).
The comparative analysis suggested that the preferred coral genera were composed of the theoretically favorable traits of high nutritional value, such as high protein content, lipid content, tissue biomass, and thickness. (Keesing, 2021; Keesing, 1990) reported that the nutritional value of coral prey was an important determinant in the evolution of the prey preferences of crown-of-thorns starfish. Our results here demonstrate that the favored coral preys of Drupella spp. were among those with high nutritional value, e.g., high protein content, lipid content, tissue biomass, and thickness. In particular, their preferred corals (Acropora and Montipora) contained high Chl-a content, Symbiodinium density, polyp density, and intermediate tissue nutritional value. These indicated that, to a certain extent, the corallivorous snails were smart and their preferred coral prey were full of nutritional value with the features of being meaty, energy-enriching, and vegetarian. However, the location of energy reserves in the coral tissue varied from species to species. For instance, the lipid was typically deposited in the basal half to one-third of the polyp column in hard corals (Stimson, 1987). This would explain why the corals with massive growth form, such as Porites spp., had resulted in a random prey choice, because the lipid reserved in polyps penetrating further down into the skeleton (Keesing, 2021). This suggests that linking feeding preference to food nutritional value for Drupella spp. feeding on numerous coral species in such a complex environment as coral reef requires more than an assessment of the absolute nutritional content of food.
Conclusions
Overall, our surveys revealed that the abundance of corallivorous snails, Drupella spp., varied spatially on the reefs throughout southeastern Hainan Island, South China Sea, while the snail density was found generally related to coral cover, independent of the number of coral species. However, the high coral cover was not always responsible for the high density of the snails. Drupella spp. showed a positive preference for Acroporidae corals, and random choice resulted for Porites, Galaxea, Platygyra, and Lobophyllia corals, when their favored taxa were abundant in situ. The results of our study suggest that the prey preference of Drupella spp. exhibits a dietary plasticity according to the abundance and availability of their favored coral prey, as well as the encounter probability between them. Furthermore, benthic types, including live coral cover, rock, and sand, had a strong relationship with the electivity index of the coral prey, reflecting the prey preference of Drupella spp. related to the substrata condition. The nutritional value of coral preys was an additional important determinant in their prey preference, while the location of the nutrient deposits in the corals and the complex environmental context should not be ignored.
As the corals in the shallow water suffering increasing influences, further works should focus on larger geographical areas, and expend to the deeper areas of the coral reefs to make a better understanding of the spatial distribution of Drupella spp. In addition, the prey preference and the microhabitat use in each life stage should be well studied. With the future research on how to increase the local predators of the corallivorous snails, there will be more benefit to reducing the cost and need for human interventions.
Data availability statement
The original contributions presented in the study are included in the article/Supplementary Material. Further inquiries can be directed to the corresponding authors. Data pertaining to this study are available at South China Sea Ocean Data Center, National Earth System Science Data Center, National Science & Technology Infrastructure of China(http://data.scsio.ac.cn/metaData-detail/1534808395246669824).
Author contributions
XL: Conceptualization, methodology, investigation, data acquisition and curation, formal analysis, and writing-original draft. CL: Investigation, data acquisition and curation, writing-review and editing. YZ: Analytic tools, investigation, data acquisition, and curation. XY: Investigation, data acquisition, and curation. JY: Investigation, data acquisition, and curation. YL: Investigation, data acquisition, and curation. GZ: Methodology, funding, supervision, writing–review and editing. HH: Funding, supervision, writing–review and editing. All authors contributed to the article and approved the submitted version.
Acknowledgments
This research was funded by the National Natural Science Foundation of China (41876192, 41976120), the Guangdong Basic and Applied Basic Research Foundation (2019A1515011532 and 2019A1515111045), the Key Research and Development Project of Hainan Province, China (ZDYF2020200), the Science and Technology Planning Project of Guangdong Province, China (2020B1212060058), the Innovation Academy of South China Sea Ecology and Environmental Engineering, Chinese Academy of Sciences (ISEE2021ZD03), and the Key Special Project for Introduced Talent Teams of Southern Marine Science and Engineering Guangdong Laboratory (Guangzhou) (GML2019ZD0404). The authors thank the staff of Tropical Marine Biological Research Station in Hainan for their logistical and administrative support. We appreciate the constructive comments from the reviewers and the editor who have greatly improved the initial manuscript. We also thank the data archive support from the National Earth System Data Center, National Science & Technology Infrastructure of China (http://www.geodata.cn).
Conflict of interest
The authors declare that the research was conducted in the absence of any commercial or financial relationships that could be construed as a potential conflict of interest.
Publisher’s note
All claims expressed in this article are solely those of the authors and do not necessarily represent those of their affiliated organizations, or those of the publisher, the editors and the reviewers. Any product that may be evaluated in this article, or claim that may be made by its manufacturer, is not guaranteed or endorsed by the publisher.
Supplementary material
The Supplementary Material for this article can be found online at: https://www.frontiersin.org/articles/10.3389/fmars.2022.990113/full#supplementary-material
References
Achituv Y., Ben-Zion M., Mizrahi L. (1994). Carbohydrate, lipid, and protein composition of zooxanthellae and animal fractions of the coral pocillopora damicornis exposed to ammonium enrichment. Pacific Sci. 48 (3), 224–233.
Al-Horani F. A., Hamdi M., Al-Rousan S. A. (2011). Prey selection and feeding rates of drupella cornus (Gastropoda: Muricidae) on corals from the Jordanian coast of the gulf of aqaba, red Sea. Jordan J. Biol. Sci. 4 (4), 191–198.
Anderson M. J., Gorley R. N., Clarke K. R. (2008). PERMANOVA+ for PRIMER: Guide to software and statistical methods (Plymouth, UK: PRIMER-E Ltd).
Anthony K. R. N., Hoegh-Guldberg O. (2003). Variation in coral photosynthesis, respiration and growth characteristics in contrasting light microhabitats: an analogue to plants in forest gaps and understoreys? Funct. Ecol. 17, 246–259. doi: 10.1046/j.1365-2435.2003.00731.x
Antonius A., Riegl B. (1998). Coral diseases and drupella cornus invasion in the red Sea. Coral Reefs 17 (1), 48–48. doi: 10.1007/s003380050093
Ayling T., Ayling A. L. (1987). Ningaloo marine park: preliminary fish density assessment and habitat survey: with information on coral damage due to drupella cornus grazing (Western Australia: Department of Conservation and Land Management).
Bai L., Zhao Z., Liu Q., Cui J. (2016). Investigation on shellfish species living in the intertidal zone to subtidal zone of fenjiezhou island of hainan. Agric. Sci. Technol. 17 (5), 1204–1210. doi: 10.16175/j.cnki.1009-4229.2016.05.037
Bessey C., Babcock R. C., Thomson D. P., Haywood M. D. E. (2018). Outbreak densities of the coral predator drupella in relation to in situ acropora growth rates on ningaloo reef, Western Australia. Coral Reefs 37 (4), 985–993. doi: 10.1007/s00338-018-01748-7
Boucher L. M. (1986). Coral predation by muricid gastropods of the genus drupella at enewetak, Marshall islands. Bull. Mar. Sci. 38 (1), 9–11.
Bright A. J., Cameron C. M., Miller M. W. (2015). Enhanced susceptibility to predation in corals of compromised condition. PeerJ 3, e1239. doi: 10.7717/peerj.1239
Bruckner A. W., Coward G., Bimson K., Rattanawongwan T. (2017). Predation by feeding aggregations of drupella spp. inhibits the recovery of reefs damaged by a mass bleaching event. Coral Reefs 36 (4), 1181–1187. doi: 10.1007/s00338-017-1609-2
Claremont M., Reid D. G., Williams S. T. (2011). Evolution of corallivory in the gastropod genus drupella. Coral Reefs 30 (4), 977–990. doi: 10.1007/s00338-011-0788-5
Cumming R. L. (1999). Predation on reef-building corals: multiscale variation in the density of three corallivorous gastropods, drupella spp. Coral Reefs 18 (2), 147–157. doi: 10.1007/s003380050170
Cumming R. L. (2009a). Case study: impact of drupella spp. on reef-building corals of the great barrier reef (Townsville, QLD, Australia: Great Barrier Reef Marine Park Authority).
Cumming R. L. (2009b). Population outbreaks and large aggregations of drupella on the great barrier reef (Townsville, QLD, AustraliaGreat Barrier Reef Marine Park Authority).
Cumming R. L., McCorry D. (1998). Corallivorous gastropods in Hong Kong. Coral Reefs 17 (2), 178–178. doi: 10.1007/s003380050112
Edmunds P. J., Burgess S. C., Putnam H. M., Baskett M. L., Bramanti L., Fabina N. S., et al. (2014). Evaluating the causal basis of ecological success within the scleractinia: an integral projection model approach. Mar. Biol. 161 (12), 2719–2734. doi: 10.1007/s00227-014-2547-y
Gardner T. A., Cote I. M., Gill J. A., Grant A., Watkinson A. R. (2003). Long-term region-wide declines in Caribbean corals. Science 301 (5635), 958–960. doi: 10.1126/science.1086050
Hamman E. A. (2018). Aggregation patterns of two corallivorous snails and consequences for coral dynamics. Coral Reefs 37 (3), 851–860. doi: 10.1007/s00338-018-1712-z
Hoegh-Guldberg O., Mumby P. J., Hooten A. J., Steneck R. S., Greenfield P., Gomez E., et al. (2007). Coral reefs under rapid climate change and ocean acidification. Science 318 (5857), 1737–1742. doi: 10.1126/science.1152509
Hoeksema B. W., Scott C., True J. D. (2013). Dietary shift in corallivorous drupella snails following a major bleaching event at koh Tao, gulf of Thailand. Coral Reefs 32 (2), 423–428. doi: 10.1007/s00338-012-1005-x
Huang J. Z., Wang F. X., Zhao H. W., Xu H. L., Liu S., Xu Q., et al. (2020). Reef benthic composition and coral communities at the wuzhizhou island in the south China Sea: The impacts of anthropogenic disturbance. Estuarine Coast. Shelf. Sci. 243, 106863. doi: 10.1016/j.ecss.2020.106863
Hughes T. P., Barnes M. L., Bellwood D. R., Cinner J. E., Cumming G. S., Jackson J. B. C., et al. (2017). Coral reefs in the anthropocene. Nature 546 (7656), 82–90. doi: 10.1038/nature22901
Hughes T. P., Huang H., Young M. A. L. (2013). The wicked problem of china's disappearing coral reefs. Conserv. Biol. 27 (2), 261–269. doi: 10.1111/j.1523-1739.2012.01957.x
Hughes T. P., Kerry J. T., Baird A. H., Connolly S. R., Dietzel A., Eakin C. M., et al. (2018). Global warming transforms coral reef assemblages. Nature 556 (7702), 492–496. doi: 10.1038/s41586-018-0041-2
Keesing J. K. (1990). Feeding biology of the crown-of-thorns starfish, acanthaster planci (Linnaeus) doctor PhD, James cook university.(Townsville: James Cook University of North Queensland).
Keesing J. K. (2021). Optimal foraging theory explains feeding preferences in the Western pacific crown-of-thorns sea star acanthaster sp. Biol. Bull. 241 (3), 303–329. doi: 10.1086/718141
Kita M., Kitamura M., Koyama T., Teruya T., Matsumoto H., Nakano Y., et al. (2005). Feeding attractants for the muricid gastropod drupella cornus, a coral predator. Tetrahedron Lett. 46 (49), 8583–8585. doi: 10.1016/j.tetlet.2005.09.182
Leuzinger S., Anthony K. R., Willis B. L. (2003). Reproductive energy investment in corals: scaling with module size. Oecologia 136 (4), 524–531. doi: 10.1007/s00442-003-1305-5
Lian J. S., Huang H., Huang L. M., Wang D. R. (2010). Coral reef and its biodiversity of sanya (Beijing: Marine Press).
Li Y. C., Liang J. L., Wu Z. J., Chen S. Q. (2019a). Outbreak and prevention of acanthaster planci (in Chinese). Ocean Dev. Manag. 8, 9–12.
Li Y. C., Wu Z. J., Liang J. L., Chen S. Q., Zhao J. M. (2019b). Analysis on the outbreak period and cause of acanthaster planci in xisha islands in recent 15 years (in Chinese). Chin. Sci. Bull. 64, 3478–3484. doi: 10.1360/TB-2019-0152
Loya Y., Sakai K., Yamazato K., Nakano Y., Sambali H., van Woesik R. (2001). Coral bleaching: the winners and the losers. Ecol. Lett. 4 (2), 122–131. doi: 10.1046/j.1461-0248.2001.00203.x
MacNeil M. A., Mellin C., Matthews S., Wolff N. H., McClanahan T. R., Devlin M., et al. (2019). Water quality mediates resilience on the great barrier reef. Nat. Ecol. Evol. 3 (4), 620–627. doi: 10.1038/s41559-019-0832-3
Madin J. S., Anderson K. D., Andreasen M. H., Bridge T. C., Cairns S. D., Connolly S. R., et al. (2016). The coral trait database, a curated database of trait information for coral species from the global oceans. Sci. Data 3, 160017. doi: 10.1038/sdata.2016.17
Mcclanahan T. R. (1994). Coral-eating snail drupella cornus population increases in Kenyan coral reef lagoons. Mar. Ecol. Prog. Ser. 115 (1-2), 131–137. doi: 10.3354/meps115131
McClanahan T. R. (1997). Dynamics of drupella cornus populations on Kenyan coral reefs. Proc. 8th Coral Reef. Sym. 1, 633–638.
Moerland M. S., Scott C. M., Hoeksema B. W. (2016). Prey selection of corallivorous muricids at koh Tao (Gulf of Thailand) four years after a major coral bleaching event. Contrib. Zool. 85 (3), 291–309. doi: 10.1163/18759866-08503003
Morton B., Blackmore G. (2009). Seasonal variations in the density of and corallivory by drupella rugosa and cronia margariticola (Caenogastropoda: Muricidae) from the coastal waters of Hong Kong: ‘plagues’ or a’ggregations’? J. Mar. Biol. Assoc. U. K. 89 (1), 147–159. doi: 10.1017/s002531540800218x
Morton B., Blackmore G., Kwok C. T. (2002). Corallivory and prey choice by drupella rugosa (Gastropoda : Muricidae) in Hong Kong. J. Molluscan Stud. 68, 217–223. doi: 10.1093/mollus/68.3.217
Moyer J. T., Emerson W. K., Ross M. (1982). Massive destruction of scleractinian corals by the muricid gastropod, drupella in Japan and the Philippines. Nautilus 96 (2), 69–82.
Nadon M. O., Stirling G. (2006). Field and simulation analyses of visual methods for sampling coral cover. Coral Reefs 25 (2), 177–185. doi: 10.1007/s00338-005-0074-5
Nicolet K. J., Hoogenboom M. O., Gardiner N. M., Pratchett M. S., Willis B. L. (2013). The corallivorous invertebrate drupella aids in transmission of brown band disease on the great barrier reef. Coral Reefs 32 (2), 585–595. doi: 10.1007/s00338-013-1010-8
Peng F. M., Wu Z. H., Shen Y. C., Li Z. M., Liu L. (2014). Systematic observation on morphological structure of drupella margariticola (Broderip 1833). J. Guangdong Ocean Univ. 34 (6), 12–17.
Pratchett M. S., Caballes C. F., Rivera-Posada J. A., Sweatman H. P. A. (2014). Limits to understanding and managing outbreaks of crown-of-thorns starfish (Acanthaster spp.). Oceanography Mar. Biol.: Annu. Rev. 52, 133–199. doi: 10.1201/b17143
Pratchett M., Caballes C., Wilmes J., Matthews S., Mellin C., Sweatman H., et al. (2017). Thirty years of research on crown-of-thorns starfish, (1986–2016): scientific advances and emerging opportunities. Diversity 9 (4), 1–49. doi: 10.3390/d9040041
Qin Z., Yu K., Liang Y., Chen B., Huang X. (2020). Latitudinal variation in reef coral tissue thickness in the south China Sea: Potential linkage with coral tolerance to environmental stress. Sci. Total Environ. 711, 134610. doi: 10.1016/j.scitotenv.2019.134610
Rice M. M., Ezzat L., Burkepile D. E. (2019). Corallivory in the anthropocene: interactive effects of anthropogenic stressors and corallivory on coral reefs. Front. Mar. Sci. 5. doi: 10.3389/fmars.2018.00525
Rotjan R. D., Lewis S. M. (2008). Impact of coral predators on tropical reefs. Mar. Ecol. Prog. Ser. 367, 73–91. doi: 10.3354/meps07531
Saponari L., Dehnert I., Galli P., Montano S. (2021). Assessing population collapse of drupella spp. (Mollusca: Gastropoda) 2 years after a coral bleaching event in the republic of Maldives. Hydrobiologia 848 (11), 2653–2666. doi: 10.1007/s10750-021-04546-5
Schoepf V., Herler J., Zuschin M. (2010). Microhabitat use and prey selection of the coral-feeding snail drupella cornus in the northern red Sea. Hydrobiologia 641 (1), 45–57. doi: 10.1007/s10750-009-0053-x
Scott C. M., Mehrotra R., Hein M. Y., Moerland M. S., Hoeksema B. W. (2017). Population dynamics of corallivores (Drupella and acanthaster) on coral reefs of koh Tao, a diving destination in the gulf of Thailand. Raffles Bull. Zool. 65, 68–79.
Shafir S., Gur O., Rinkevich B. (2008). A drupella cornus outbreak in the northern gulf of eilat and changes in coral prey. Coral Reefs 27 (2), 379–379. doi: 10.1007/s00338-008-0353-z
Stimson J. S. (1987). Location, quantity and rate of change in quantity of lipids in tissue of Hawaiian hermatypic corals. Bull. Mar. Sci. 41 (3), 889–904.
Titlyanov E. A., Titlyanova T. V., Scriptsova A. V., Ren Y. X., Li X. B., Huang H. (2019). Interannual and seasonal changes in the benthic algae flora of coral reef in xiaodong hai (Hainan island, China). J. Mar. Sci. Eng. 7 (8), 243. doi: 10.3390/jmse7080243
Tsang R. H. L., Ang P. (2015). Cold temperature stress and predation effects on corals: their possible roles in structuring a nonreefal coral community. Coral Reefs 34 (1), 97–108. doi: 10.1007/s00338-014-1210-x
Tsang R. H. L., Ang P. Jr. (2019). Resistance to temperature stress and drupella corallivory may promote the dominance of platygyra acuta in the marginal coral communities in Hong Kong. Mar. Environ. Res. 144, 20–27. doi: 10.1016/j.marenvres.2018.11.009
Turner S. J. (1994a). The biology and population outbreaks of the corallivorous gastropod drupella on indo-pacific reefs. Oceanography Mar. Biol.: Annu. Rev. 32, 461–530.
Turner S. J. (1994b). Spatial variability in the abundance of the corallivorous gastropod drupella cornus. Coral Reefs 13 (1), 41–48. doi: 10.1007/Bf00426434
Wolff N. H., Mumby P. J., Devlin M., Anthony K. R. N. (2018). Vulnerability of the great barrier reef to climate change and local pressures. Global Change Biol. 24 (5), 1978–1991. doi: 10.1111/gcb.14043
Zhang Y. Y., Huang H., Huang J. Y., You F., Lian J. S., Yang J. H., et al. (2016). The effects of four transplantation methods on five coral species at the sanya bay. Acta Oceanol. Sin. 35 (10), 88–95. doi: 10.1007/s13131-016-0916-8
Keywords: Corallivory, coral, prey selection, spatial variation, Drupella
Citation: Lei X, Liu C, Zhang Y, Yu X, Yang J, Luo Y, Zhou G and Huang H (2022) Spatial variability in the abundance and prey selection of the corallivorous snail Drupella spp. in the southeastern Hainan Island, China. Front. Mar. Sci. 9:990113. doi: 10.3389/fmars.2022.990113
Received: 09 July 2022; Accepted: 03 August 2022;
Published: 24 August 2022.
Edited by:
Dilip Kumar Jha, National Institute of Ocean Technology, IndiaReviewed by:
Xinqing Zheng, State Oceanic Administration, ChinaWentao Niu, Ministry of Natural Resources, China
Jiayuan Liang, Guangxi University, China
Copyright © 2022 Lei, Liu, Zhang, Yu, Yang, Luo, Zhou and Huang. This is an open-access article distributed under the terms of the Creative Commons Attribution License (CC BY). The use, distribution or reproduction in other forums is permitted, provided the original author(s) and the copyright owner(s) are credited and that the original publication in this journal is cited, in accordance with accepted academic practice. No use, distribution or reproduction is permitted which does not comply with these terms.
*Correspondence: Guowei Zhou, zhougw@scsio.ac.cn; Hui Huang, huanghui@scsio.ac.cn