- 1College of Fisheries, Guangdong Ocean University, Zhanjiang, China
- 2Guangdong Provincial Key Laboratory of Pathogenic Biology and Epidemiology for Aquatic Economic Animals, Zhanjiang, China
- 3Guangdong Provincial Laboratory of Southern Marine Science and Engineering, Zhanjiang, China
The study investigates the outcomes of adding sodium humate and sodium humate with probiotics (Lysinibacillus, Bacillus subtilis) to culture water on the growth performance, enzyme activity, and microbial environment of shrimp. The sodium humate and probiotics concentrations were 3 mg/L and 105 CFU/mL in the culture water. Litopenaeus vannamei (0.31 ± 0.03 g) at a density of 300 shrimps/m3 were cultured in nine buckets for a 43-days culture experiment. The results showed that the survival rate (SR) of L. vannamei in sodium humate group and sodium humate with probiotics group were (88.44%) and (86.07%), with the sodium humate group outcome being significantly better than the control group. The shrimps’ final body weight and specific growth rate (SGR) in the two experimental groups were significantly higher than the control group. The feed conversion rate (FCR) was significantly lower than the control group. The ammonia nitrogen concentration in sodium humate with probiotics group was significantly lower than the control group on the 15th day. The activities of intestinal amylase, lipase, trypsin and hepatopancreas superoxide dismutase (SOD), phenol oxidase (PO), and catalase (CAT) in sodium humate group were significantly higher than the control group. Notably, SOD, PO, and lipase activities in sodium humate with probiotics group were significantly higher than the control group. Illumina MiSeq high-throughput sequencing showed that the Chao and Ace indices of the culture water microflora in experimental groups were higher than the control group, and the sodium humate group was significantly higher than the control group. At the phylum level, Proteobacteria, Bacteroidetes, and Actinobacteria were the dominant bacterial communities in the intestine and culture water of L. vannamei, Proteobacteria was the most abundant phyla. At the genus level, the relative abundance of Pseudohongiella of water microflora in the control group was significantly lower than the experimental groups. Ruggiella (15.22-19.56%) was the most abundant genera of intestinal microflora. These results infer that the addition of sodium humate enhances the growth performance, digestive enzymes, and some immune enzyme activities, improving the richness of the water microbial community of L. vannamei.
Introduction
Litopenaeus vannamei is one of the most economically important shrimps globally at present. The total culture production of L. vannamei in China was 1.86 × 106 t in 2020 (FBMA, 2020), the largest in the world. With the rapid development of shrimp aquaculture, the water environment is deteriorating daily, and diseases are frequent and spreading. Many antibiotics are used for disease prevention and control, causing problems such as pathogenic drug resistance and residual trace amounts of the drugs, seriously affecting the health of the shrimp industry and sustainable development (Flegel et al., 2008; Thitamadee et al., 2016). To solve the many contradictions in the shrimp aquaculture industry, such as breeding, food, and ecological safety, the search for green and environmentally friendly antibiotic alternatives and farming technologies has become urgent (Gatesoupe, 1999; Liu et al., 2014; Hai, 2015; Pinto et al., 2020).
Sodium humate is the sodium salt of humic acid, widely distributed in nature and extractable from lignite and peat (Motta and Santana, 2013). Sodium humate is a light powder or amorphous granule with bright black or brownish-black crystals and is easily soluble in water. It is an organic weak acid salt. Since sodium humate dissolves in water and turns black, sodium humate can be used for blanking the light in water to inhibit algae growth. Studies have shown that sodium humate solution can remove sulfur dioxide and nitrogen dioxide (Sun et al., 2010; Hu et al., 2010) and can also form hydrogels with starch or clay to adsorb Cu2+ or methyl blue dyes in aqueous solutions (Yi and Zhang, 2008; Zheng et al., 2010), indicating that sodium humate can chelate metal ions and adsorb pollutants, so it is widely used in biological agriculture and pollution control (Dou and Jiang, 2019). In addition, previous studies have shown that dietary supplementation with 0.6% humic acid for 60 days could improve fish meat quality and the immune parameters of rainbow trout (Yılmaz et al., 2018). Some studies reported that adding 0.28-0.37% sodium humate to the feed is beneficial to the growth and health of tilapia and can be used as a feed additive for tilapia (Deng et al., 2020). However, few research reports investigate sodium humate’s benefits in shrimp farming.
Probiotics are live microorganisms beneficial to health by implanting or colonizing the host’s microflora or the environment, thereby exerting beneficial effects on the host’s health (Hai, 2015; El-Saadony et al., 2021). Probiotics are expected to be an alternative to antibiotics because they do not leave residues in animals and do not confer resistance to pathogens (Reid and Friendship, 2002; Hai, 2015). Many studies have shown that probiotic supplementation can improve the survival rate and growth rate of aquatic animals and reduce the occurrence of diseases by improving the immune system of fish and shrimp (Amoah et al., 2019). In addition, probiotics can also improve the water quality of aquaculture (Zibiene and Zibas, 2019), increase the yield of aquatic animals (Jatobá et al., 2011; Sunitha and Padmavathi, 2013), regulate the intestinal micro-ecological balance of aquatic animals (Zhang et al., 2021), improve the activities of digestive enzymes (Zuo et al., 2019), improve the immunity of aquatic animals (Zokaeifar et al., 2012), and inhibit the growth and reproduction of pathogenic microorganisms (Reid and Burton, 2002). The Bacillus spp. has a better sporulation capacity among probiotics, allowing a higher survival rate and effectiveness (Abriouel et al., 2011). Some studies reported that fermented feed with 108 CFU/g Bacillus subtilis could improve growth, digestive enzyme activities, non-specific immune performance, and the immunity of L. vannamei (Lima et al., 2021). With the rapid development of next-generation sequencing technology, high-throughput sequencing based on 16S rRNA gene amplicons has been widely used in microbial research (Dougal et al., 2014; Niu et al., 2015). In this study, the probiotics (Lysinibacillus, Bacillus subtilis), which were isolated and identified from the shrimp culture pond, were used together with sodium humate to explore the effects on the growth, enzyme activity, and microbial environment of L. vannamei, to find a green and ecological way of L. vannamei farming.
Materials and methods
Experimental materials and feeding management
The experiment was carried out at the Donghai Island Marine Biological Research Base of Guangdong Ocean University over 43 days. The laboratory isolated and identified probiotics from Shrimp farm ponds, including Lysinibacillus and Bacillus subtilis which were identified by molecular analysis. The genomic DNA of strains was extracted by Bacterial Genomic DNA Extraction Kit (Tiangen Biochemical Technology (Beijing) Co., Ltd., China) and 16S rDNA was amplified by primers 27F (5’-AGAGTTTGA TCCTGGCTCAG-3’) and 1492R (5’-GGTTACCTTGTTACGACTT-3’). The reaction conditions of PCR: 94°C for 5 min followed by 35 cycles of 94°C for 30 s, 55°C for 30 s and 35 cycles of 72°C for 1 min, and 72°C for 5 min. The amplified products were sequenced (Bioengineering (Shanghai) Co., Ltd., China) and the 16S rDNA sequence was compared and identified in the GenBank database (http://blast.ncbi.nlm.nih.gov/Blast.cgi). The probiotics were ratified as colonies were identified on the bases of their morphological, and biochemical characteristics. The probiotics were activated by inoculation in a 2216E solid medium (Qingdao Haibo Biotechnology Co., Ltd., Qingdao, China) and incubated at a constant temperature of 28°C. Single colonies of activated probiotics were picked and incubated in 2216E liquid medium (Qingdao Haibo Biotechnology Co., Ltd., Qingdao, China) at 28°C with oscillation (150 rpm), then expanded with fresh 2216E liquid medium at 10% inoculum (100 mL) in a 1000-mL flat bottom flask. Overnight cultures of probiotic bacteria were collected by centrifugation (5000 rpm for 10 min at 4°C) and washed twice with phosphate-buffered saline (PBS) at a pH of 7.2. Finally, cell pellets were re-suspended, the optical density (OD) was measured at 600 nm (Gupta et al., 2014) using a spectrophotometer (Shanghai Jing Yi, China) and values were correlated with colony forming unit (CFU) counts using Total Plate Count method.
Sodium humate was commercial sodium humate produced in Wuhai City, Inner Mongolia, China. It was ground and dissolved in water with a 150-mesh sieve. The transparency of the 3 mg/L sodium humate solution was (68.3 ± 2.8) cm, and the total organic carbon (TOC) concentration was (2.34 ± 0.28) mg/L. L. vannamei was taken from the Donghai Island Marine Biological Research Base of Guangdong Ocean University. The body color of the shrimps was normal, healthy, and disease-free which was temporarily cultured in a pool (14.5 m3, 28 ± 1 °C, DO > 5 mg/L, salinity 32.5‰) for one week before the experiment, fed with artificial compound feed four times a day at 10% body weight, cleaned shrimp feces and bait regularly daily.
Active individuals with intact appendages and no damage were selected for the experiments. The shrimp’s body length was (3.30 ± 0.07) cm, and the body weight was (0.31 ± 0.03) g. The test seawater was extracted from the sea area and used after precipitation, sand filtration, and disinfection with chlorine-containing disinfectant, and the water body was continuously and evenly aerated. The experiment was undertaken in a blue plastic bucket (1.0 m deep) with a volume of 1 m3. The culture water body was 0.75 m3, the stocking density was 300 shrimps/m3, the water temperature was 28.1 ± 0.8 °C, and the salinity was 32-34‰. Each experimental bucket has three air stones (DO > 5 mg/L) and one feed observation desk, fed with artificial compound feed (Crude protein ≥ 43.0%, Crude fat ≥ 6.0%, Crude fiber ≤ 5.0%, Coarse Ash ≤ 16.0%, Crude fat ≥ 6.0%, water ≤ 12.0%, Total phosphorus ≥ 1.2% Lysine ≥ 2.2%, Guangdong Yuequn Marine Biological Research and Development Co., Ltd., China) four times a day. Feeding times are 07:00, 11:00, 15:00, and 19:00. The feeding amount was 5-10% of the body weight of the shrimps, which was adjusted according to the feeding and growth conditions of the shrimps, and there was only replenished water for evaporation once a week during the experiment.
Experimental design
The study consisted of three groups with three replicates for each group. Group A was the control group that did not add any substances; group B was an experimental group which had 3 mg/L sodium humate in culture water; group C was another experimental group that had 3 mg/L sodium humate and 105 CFU/mL probiotics (Lysinibacillus, Bacillus subtilis) in culture water. Sodium humate was added once every ten days and probiotics were added once at the beginning of the experiment.
Measurement of growth performance
Thirty shrimps were randomly selected before the experiment, and the body length (using a vernier caliper) and mass (with an electronic balance after absorbing the water with filter paper) were measured. At the end of the breeding experiment, the number of each parallel shrimp was recorded, the body length and weight were measured, and the survival rate (SR), feed conversion rate (FCR), and specific growth rate (SGR) of the shrimp were calculated:
Water quality monitoring and measurement
The water indices were detected once every three days during the experiment, and the water passed through a 0.45μm glass fiber microfilter (Zinteng Laboratory Equipment Co., Tianjin, China). Water temperature and pH were detected using electric analyzers (SX-610, Shanghai Sanxin Instrument Factory), and the salinity was detected using an Optical salinometer. The concentration of ammonia nitrogen, nitrite nitrogen, and nitrate nitrogen was measured according to standard methods (Center, 2007).
Digestive enzymes and immune analysis
After the experiment, ten shrimps were randomly selected from each experimental bucket, and sterilized tweezers and scissors were used to dissect the shrimps. The obtained hepatopancreas and whole intestine were snap-frozen in liquid nitrogen and then transferred to -80°C for cryopreservation. The samples were cut, the weight was checked, and PBS was added (pH 7.2-7.4). Samples were rapidly frozen with liquid nitrogen and then maintained at 2-8°C after melting, with PBS added (pH 7.4). Samples from different organ were separately homogenized by hands, with centrifugation of 20-min at the speed of 3000 rpm to remove supernatant.
The activities of superoxide dismutase (SOD), phenol oxidase (PO), catalase (CAT), and lysozyme in Hepatopancreas (Aguirre-Guzman et al., 2009) were determined according to the kit instructions (Shanghai Enzyme Link Biotechnology Co., Ltd., China). The shrimp intestines were ground with buffer solution in the kit to prepare the sample, and the activities of amylase, lipase, and trypsin, in the intestinal tract were determined according to the kit’s requirements (Shanghai Enzyme Link Biotechnology Co., Ltd., China).
Microbial sample collection
Once the experiment had finished, samples of water microflora were collected. 500 mL of water was randomly taken from each experimental bucket, and a 0.45 μm filter membrane was used for suction filtration. It was quickly stored in a -80°C refrigerator to determine water microflora. The whole intestines of 30 shrimps were taken from each experimental bucket, combined into one sample, rinsed with sterile normal saline, put into a sterile antifreeze tube, placed quickly in liquid nitrogen, and stored in a -80°C refrigerator for the determination of intestinal microflora.
DNA extraction and miseq sequencing
The microbial community DNA of the intestine and culture water samples were extracted from 36 samples using the MagPure Stool DNA KF kit B(Magen, China) and PowerWater DNA Isolation Kit (MO BIO Laboratories, Carlsbad, California, USA) according to the manufacturer’s instructions. DNA was quantified with a Qubit Fluorometer using the Qubit dsDNA BR Assay kit (Invitrogen, USA). The quality was checked by running aliquots on 1% agarose gel.
To profile the diversity and structure of the microbial communities, the 16S rRNA V3-V4 region of the gene was amplified with degenerate PCR primers, 341F (5’-ACTCCTACGGGAGGCAGCAG-3’) and 806R (5’- GGACTACHVGGGTWTCTAAT-3’). The barcode is a sequence with eight-nucleotide unique to each sample. PCR enrichment was performed in a 50 μL reaction containing a 30 ng template, fusion PCR primer and PCR master mix. PCR cycling conditions were as follows: 94°C for 3 minutes, 30 cycles of 94°C for 30 seconds, 56°C for 45 seconds, 72°C for 45 seconds and finally, an extension for 10 minutes at 72°C. The PCR products were purified with AmpureXP beads and eluted in the Elution buffer. Libraries were qualified using the Agilent 2100 bioanalyzer (Agilent, USA). The validated libraries were used for sequencing on an Illumina MiSeq platform (BGI, Shenzhen, China), following the standard pipelines of Illumina and generating two 300 bp paired-end reads. The sequences obtained are available in the NCBI Sequence Read Archive (SRA) database (Accession Number: SRP379080).
Bioinformatic and statistical analysis
Raw reads were filtered to remove adaptors, low-quality and ambiguous bases, and then paired-end reads were added to tags by the Fast Length Adjustment of Short reads program FLASH, v1.2.11 (Magoc and Salzberg, 2011) to get the tags. The tags were clustered into OTUs with a cutoff value of 97% using UPARSE software v7.0.1090 (Edgar, 2013), and chimaera sequences were compared with the Gold database using UCHIME v4.2.40 (Edgar et al., 2011). The OTU representative sequences were taxonomically classified using Ribosomal Database Project (RDP) Classifier v.2.2 with a minimum confidence threshold of 0.6 and trained on the Greengenes database v201305 using QIIME v1.8.0 (Caporaso et al., 2010). The USEARCH global (Edgar, 2010) was used to compare all Tags back to OTU to get each sample’s OTU abundance statistics table.
The Venn plots in OTUs or taxa were plotted with R package “VennDiagram” version 3.1.1. Alpha diversity (Chao, Ace, Shannon and Simpson indices, Observed spices, Good’s coverage) was estimated at the OTU level using MOTHUR v1.31.2 (Schloss et al., 2009). Principal Coordinate Analysis (PCoA) and sample cluster and beta diversity at the OTU level were performed using QIIME v1.8.0 (Caporaso et al., 2010). Barplots and heatmaps of different classification levels were plotted using R package v3.4.1 and R package “gplots”. KEGG was predicted using the PICRUSt software (Wilkinson et al., 2018).
SPSS version 26 software (SPSS Inc., Chicago, IL, USA) was used to analyze the experimental data statistically, and one-way analysis of variance was used for the data. The results were expressed as mean ± standard deviation (SD). P< 0.05 was considered a significant difference. When the difference is significant, the Duncan test is used for multiple comparisons.
Results
Shrimp growth performance
The growth performance of L. vannamei in sodium humate (B) and sodium humate with Probiotics (C) groups is presented in Table 1. The survival rate of shrimps in groups B (88.44%) and C (86.07%) were higher than the control group (79.11%), and group B was significantly higher than the control group (P< 0.05). The final body weight (FBW) of shrimps in groups B and C were (2.12 ± 0.03) g and (1.90 ± 0.15) g, and the specific growth rate (SGR) was (4.35 ± 0.04%/d) and (4.09 ± 0.21%/d), significantly higher than the control group (P< 0.05). In addition, the feed conversion rates (FCR) of shrimps in groups B and C were 1.20 and 1.45, which was significantly lower than the control group (P< 0.05).

Table 1 Effects of sodium humate (B) and sodium humate with Probiotics (C) on growth performance of L.vannamei.
Water quality
During the experiment, the pH values were 7.4-8.1, with no significant differences between all groups (p > 0.05). The pH values showed a continuous downward trend in all groups (Figure 1A). The concentration of ammonia nitrogen was 0.01-3.03 mg/L (Figure 1B). The peak of ammonia nitrogen in sodium humate with probiotics group (C) reached 1.10 mg/L on day 12 but was delayed to day 15 in the sodium humate (B) and control groups (A) with 2.36 mg/L and 3.03 mg/L, respectively. The ammonia nitrogen in group C (0.73 mg/L) was significantly lower than the control group (3.03 mg/L) at 15 d (p< 0.05). The nitrite-nitrogen concentration was 0.01-18.61 mg/L (Figure 1C), with all groups trending with increasing and then decreasing levels. Nitrite nitrogen peaks were observed at 30 d, 33d, and 30d in control, groups B and C with 17.38, 18.61, and 17.27 mg/L, respectively. However, the nitrite-nitrogen in all groups was down to 0.05 mg/L at 42 d. The concentration of nitrate-nitrogen was 0.03-26.83 mg/L (Figure 1D), with no significant differences between all groups (p > 0.05). In the control group, groups B and C of nitrate-nitrogen reached peaks of 24.21, 24.64, and 25.15 mg/L at 42 d, respectively.
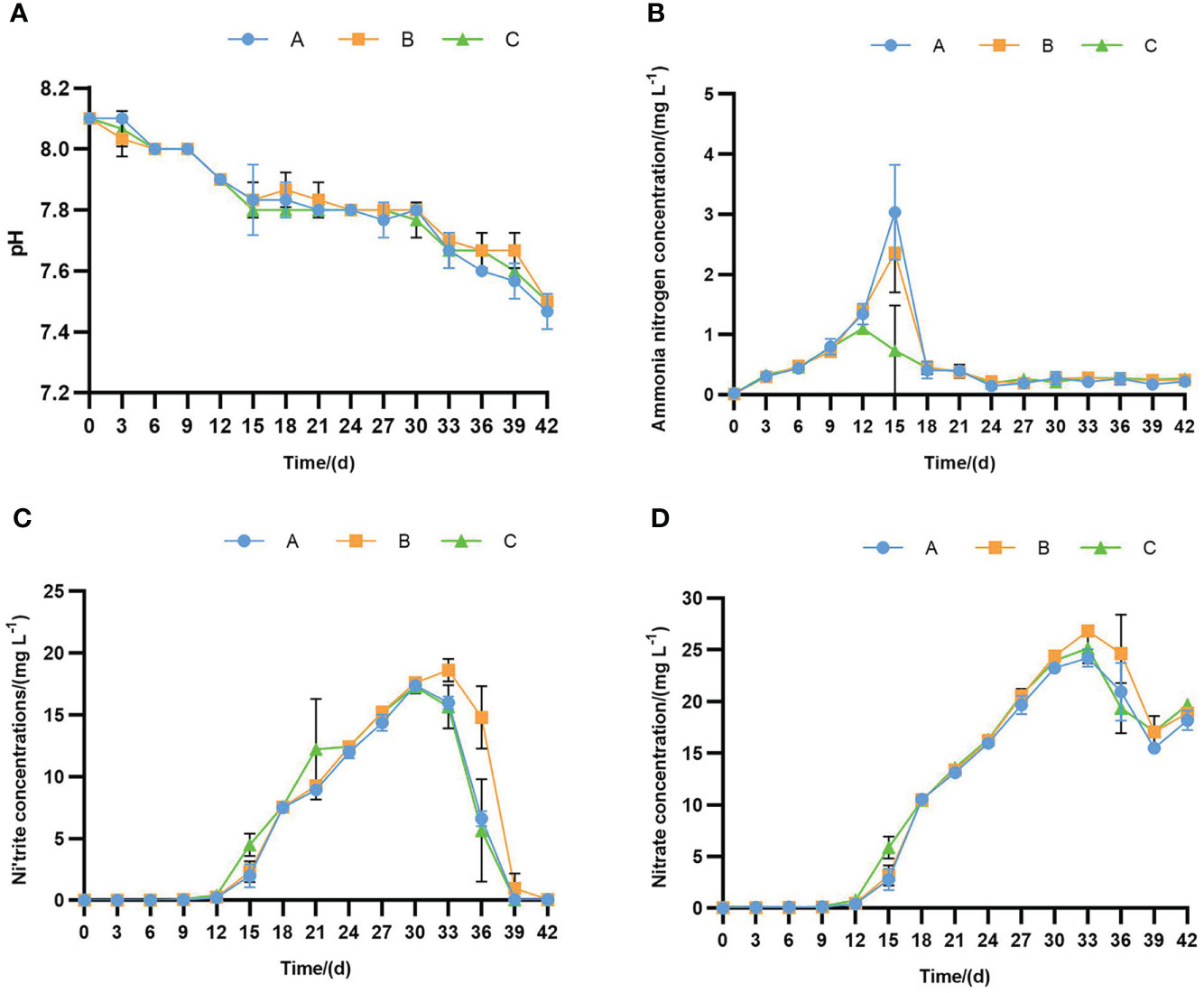
Figure 1 The pH values (A), ammonia nitrogen (B), nitrite (C) and nitrate (D) concentrations detected in the 43-days experiment culturing L. vannamei with sodium humate (B) and sodium humate with probiotics (C). Error bars indicate ± standard deviation (SD).
Digestive and immunological enzymes
At the end of the experiment, the activities of intestinal digestive enzymes (amylase, lipase, trypsin) and immune enzymes of superoxide dismutase (SOD), lysozyme, phenol oxidase (PO), and catalase (CAT) in the hepatopancreas of L. vannamei were analyzed in the sodium humate (B) and sodium humate with probiotics (C) groups (Figure 2). The activities of all enzymes in experimental groups increased to varying degrees when compared with the control group (A).
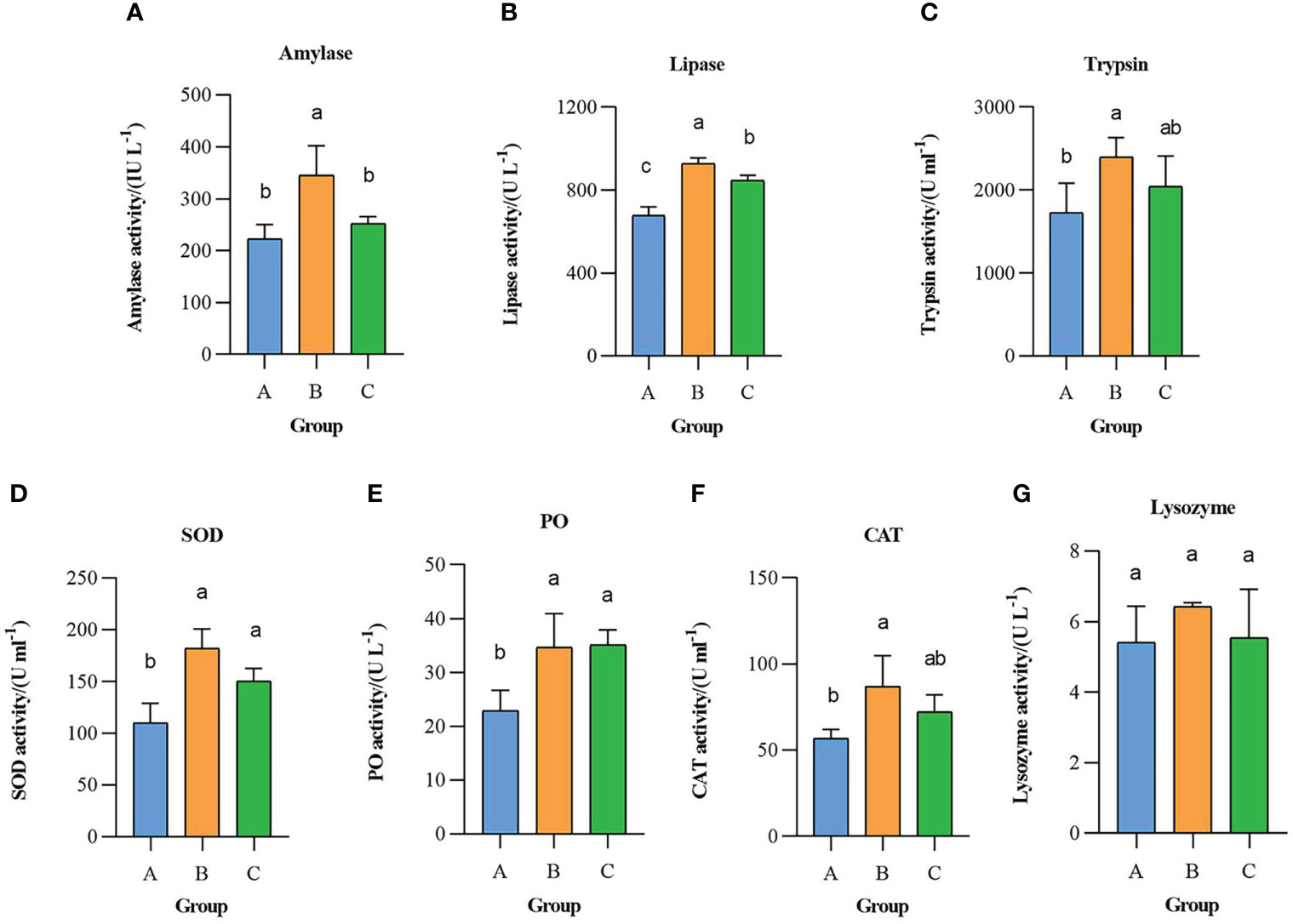
Figure 2 Effects of sodium humate (B) and sodium humate with Probiotics (C) on intestinal amylase (A), lipase (b), trypsin (C) and hepatopancreas SOD (D), PO (E), CAT (F) and lysozyme (G) of L. vannamei. Upright bars denote the mean ± SD (n = 6). Bars labelled with different letters denote significant differences (p < 0.05) between the experimental groups.
The amylase activity (Figure 2A) in group B was significantly higher than control and C groups (P< 0.05), but there was no significant difference between control and C groups (P > 0.05). The lipase activity (Figure 2B) in experimental groups was significantly higher than the control group (P< 0.05). The trypsin activity (Figure 2C) in group B was significantly higher than the control group (P< 0.05), while there was no significant difference between control and C groups (P > 0.05).
The SOD and PO activities (Figures 2D, E) in the experimental groups were significantly higher than the control group (P< 0.05), while there was no significant difference between groups B and C (P > 0.05). The CAT activity (Figure 2F) in group B was significantly higher than the control group (P< 0.05), while group C is not significantly different from the control group (P > 0.05). The highest lysozyme activity (Figure 2G) was detected in group B, with no significant difference between three groups (P > 0.05).
Analysis of culture water and intestinal microbiota
The raw HiSeq sequencing data yielded 862,629 and 824,553 high-quality sequences for the intestine and culture water samples of L. vannamei, respectively. The OTUs and Alpha diversity statistics in culture water and intestinal microbiota of L. vanamei were presented in Table 2. The average clean tags range from 44,674 to 48,616 per group, and the number of operational taxonomic units (OTUs) in the sodium humate group (BW) was significantly higher than the control group AW (p< 0.05). Moreover, the total number of OTUs was 1469. The three groups share 1037 OUTs in water microbiota, and the unique OTUs in the sodium humate with probiotics group (CW) were the highest (Figure 3A). For the intestinal microbiota of the shrimps, the total number of OTUs was 682, the three groups shared 440 OTUs, and the unique OTUs of each group were not different (Figure 3B).
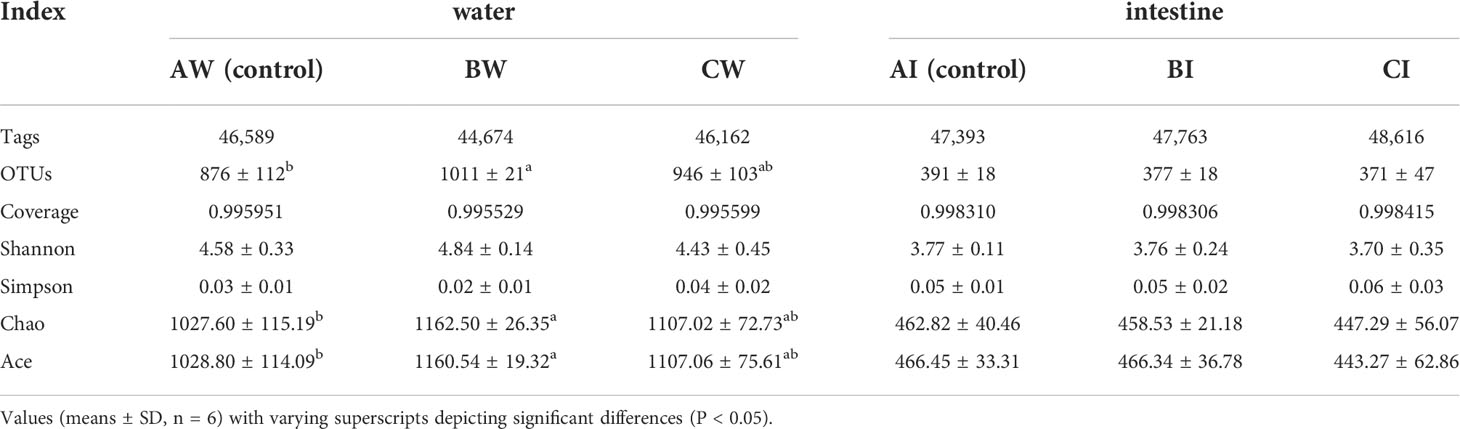
Table 2 OTU, diversity index and estimated OTU richness for the intestinal and water bacterial diversity analysis of L. vannamei with sodium humate (BI, BW) and sodium humate with probiotics (CI, CW).
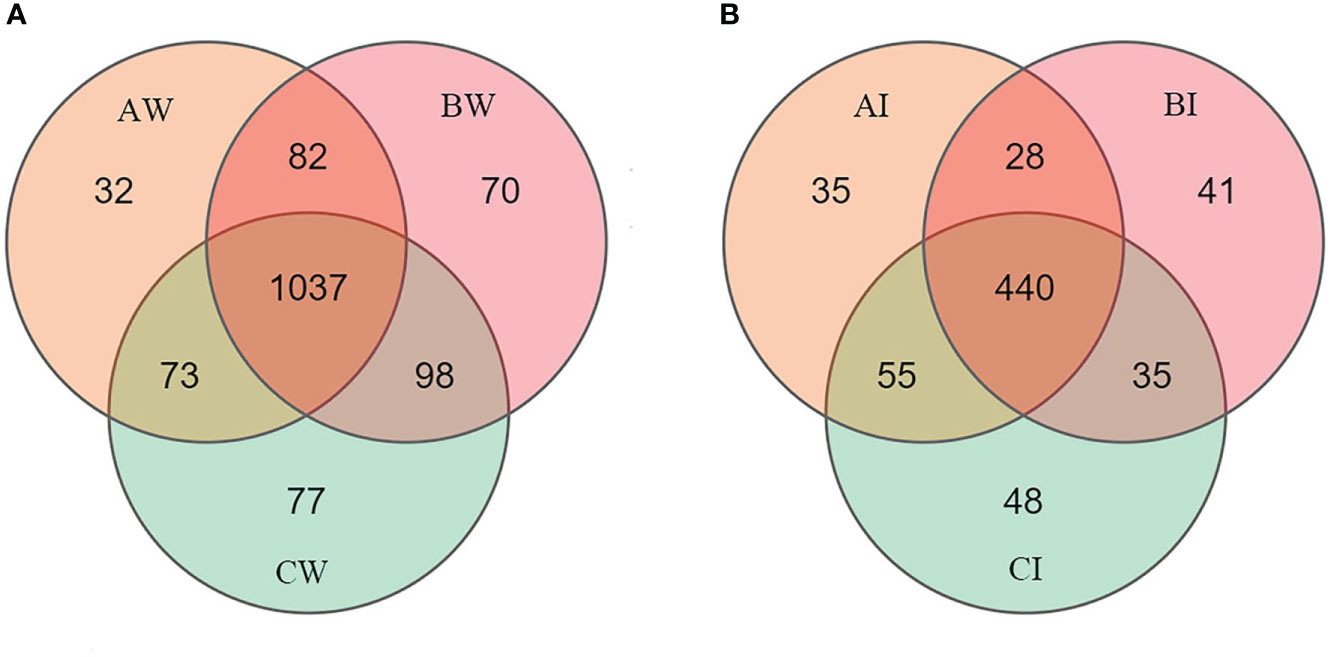
Figure 3 Venn diagram showing the unique and shared OTUs in culture water (A) and intestine (B) of L. vannamei with sodium humate (BW, BI) and sodium humate with probiotics (CW, CI). OTUs were defined at the 97% similarity level.
The coverage of each group ranged from 0.995529 to 0.998415, indicating the depth of sequencing had effectively covered the sample microflora. The Chao and Ace indices of the culture water microflora in BW and CW groups were higher than the control group, and group BW was significantly higher than the control group (p< 0.05). The Shannon and Simpson indices were not significantly different between all groups (p > 0.05). The Chao, Ace, Shannon, and Simpson indices of the intestinal microflora in groups BI and CI were not significantly different (p > 0.05) compared to the control group (Table 2).
There were 31 phyla in the water microflora of L. vannamei, with Proteobacteria, Bacteroidota, Actinobacteria, Chloroflexi, and Patescibacteria being the dominant phyla (relative abundance > 5% at least in one sample) (Figure 4A). Proteobacteria (42.77-52.31%) was the most abundant phyla, but there was no significant difference between the groups (p > 0.05). The relative abundance of Chloroflexi in sodium humate with probiotics group (CW) was significantly higher than the control group (P< 0.05). In contrast, the Patescibacteria and Bdellovibrionota in sodium humate with probiotics group (BW) were significantly higher than the control and CW groups (P< 0.05). The Verrucomicrobiota increased significantly (P< 0.05) in control group than other experimental groups (Figure 4C).
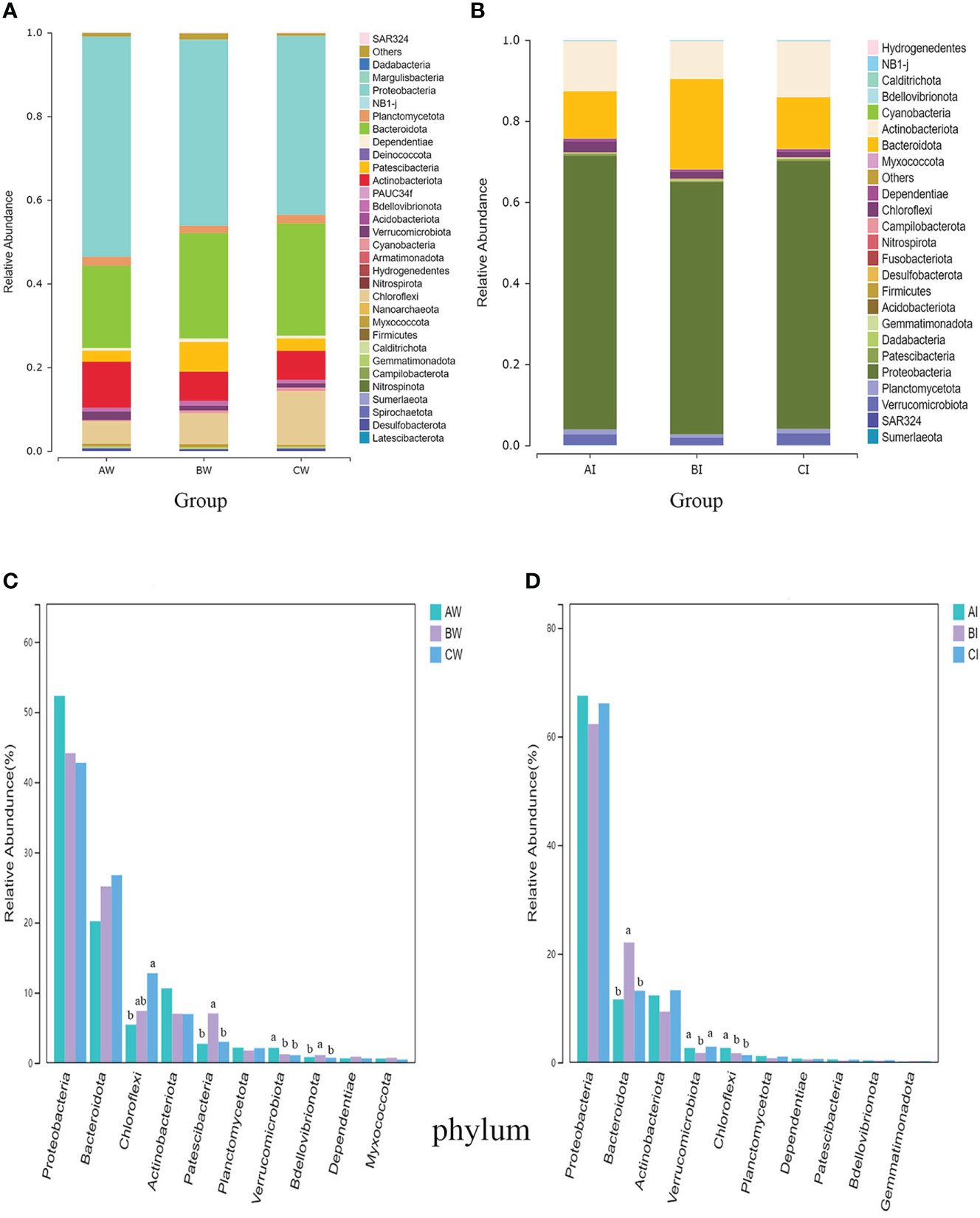
Figure 4 Effect of sodium humate (BW, BI) and sodium humate with probiotics (CW, CI) on bacterial community composition and the top 10 dominant bacteria at the phylum level in the culture water (A, C) and intestine (B, D) of L. vannamei. Bars labelled with different letters denote significant differences (p < 0.05) between the experimental groups.
There were 24 phyla in the intestinal microflora of L. vannamei, and Proteobacteria (62.30-67.51%) was the most abundant phylum (Figure 4B). The relative abundance of Bacteroidota in group BI was significantly higher than the control and CW groups (P< 0.05), but the Verrucomicrobiota in group BI was significantly lower than the control group (P< 0.05). Additionally, Chloroflexi increased significantly (P< 0.05) in the control group compared to other experimental groups (Figure 4D).
At the genus level, the top 5 genera were Marivita, Ruegeria, Halioglobus, Woeseia, and Pseudohongiella in the water microflora of L. vannamei (Figure 5A). The relative abundance of Marivita and Ruggiella in the control group was higher than experimental groups. However, Pseudohongiella in the control group was significantly lower than the experimental groups (P< 0.05). The relative abundance of Gracilibacteria increased significantly (P< 0.05) in group BW than the control group. Moreover, Polaribacter decreased significantly (P< 0.05) in experimental groups compared to the control group (Figure 5C).
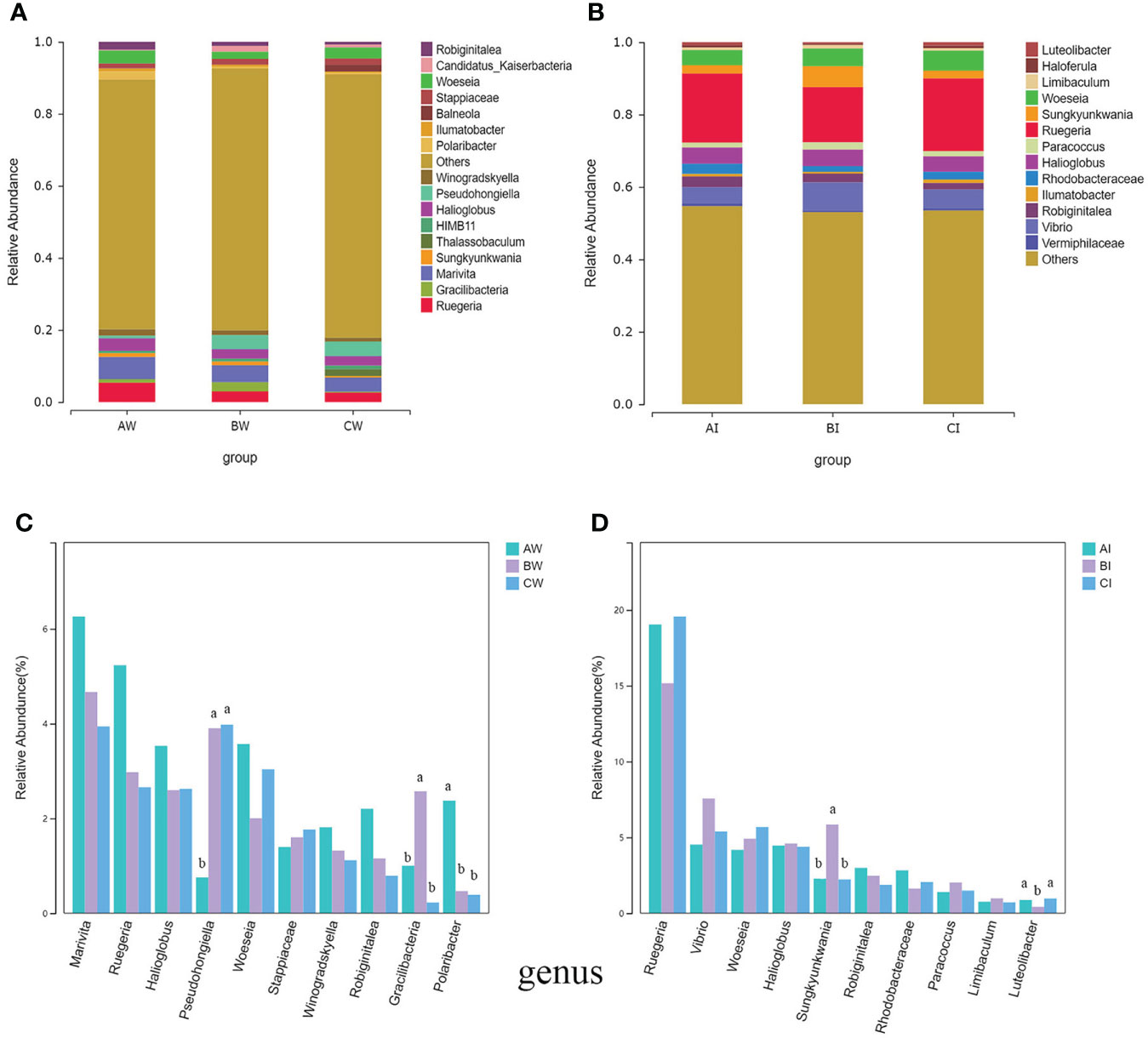
Figure 5 Effect of sodium humate (BW, BI) and sodium humate with probiotics (CW, CI) on bacterial community composition and the top 10 dominant bacteria at the genus level in the culture water (A, C) and intestine (B, D) of L. vannamei. Bars labelled with different letters denote significant differences (p < 0.05) between the experimental groups.
At the genus level, Ruegeria, Vibrio, Woeseia, Sungkyunkwania, and Halioglobus were the dominant genera (relative abundance > 5% at least in one sample) in the intestinal microflora of L. vannamei (Figure 5B). Ruggiella (15.22-19.56%) was the most abundant genus, but there was no significant difference between the groups (p > 0.05). The relative abundance of Sungkyunkwania in group BI was significantly higher than the control and CI groups (P< 0.05), but the abundance of Luteolibacter decreased significantly (P< 0.05) in group BI compared to control and CI groups (Figure 5D).
According to principal coordinates analysis (PCoA) based on unweighted-Unifrac, PCoA1 was 28.95%, and PCoA2 was 24.23% in water microflora. It could be seen that the water microflora of L. vannamei could be well clustered between samples of the same group and clearly distinguished between different groups. Group CW was further away from the control group on PCoA1, experimental group BW was further away from the control group on PCoA2, and experimental group BW was further away from group CW on PCoA2 (Figure 6A).
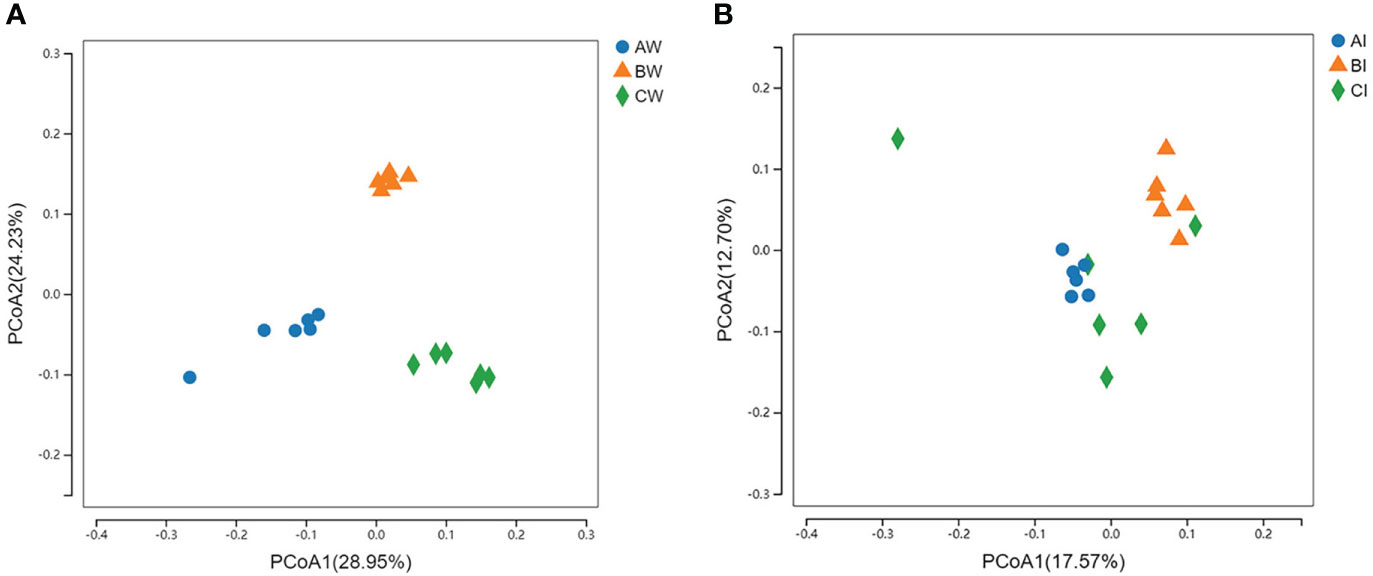
Figure 6 Effect of sodium humate (BW, BI) and sodium humate with probiotics (CW, CI) on principal co-ordinates analysis (PCoA) based on unweighted-Unifrac of the microbiota in the culture water (A) and intestine (B) of L. vannamei.
The PCoA of intestinal microflora in three groups, PCoA1 was 17.57%, and PCoA2 is 12.70%, indicated that the intestinal microflora of L. vannamei in control and group BI were clustered together, and the sample community of group CI was more dispersed. Notably, the group BI was farther away from the control group on PCoA1, and the group CI was closer to the control group (Figure 6B).
The conjectural functions of the microbiota in the culture water and intestine of L. vannamei were illustrated using PICRUSt. Compared to taxonomic profiles, the functional profiles of all groups were much more like each other (Figure 7). All groups were enriched with functions that were related to amino acid metabolism (relative abundance from 13.64% to 14.04%), carbohydrate metabolism (relative abundance from 13.03% to 13.37%), metabolism of cofactors and vitamins (relative abundance from 12.11% to 12.31%), metabolism of terpenoids and polyketides (8.52% to 9.40%), metabolism of other amino acids (8.17% to 8.47%), lipid metabolism (7.37% to 7.82%), xenobiotics biodegradation and metabolism (5.45% to 6.00%), and energy metabolism (5.29% to 5.41%). These KEGG function classification results indicated that the shrimp intestinal microbial taxa and cultured water microbial taxa maintained similar biological functions to those in culture water and intestine of L. vannamei.
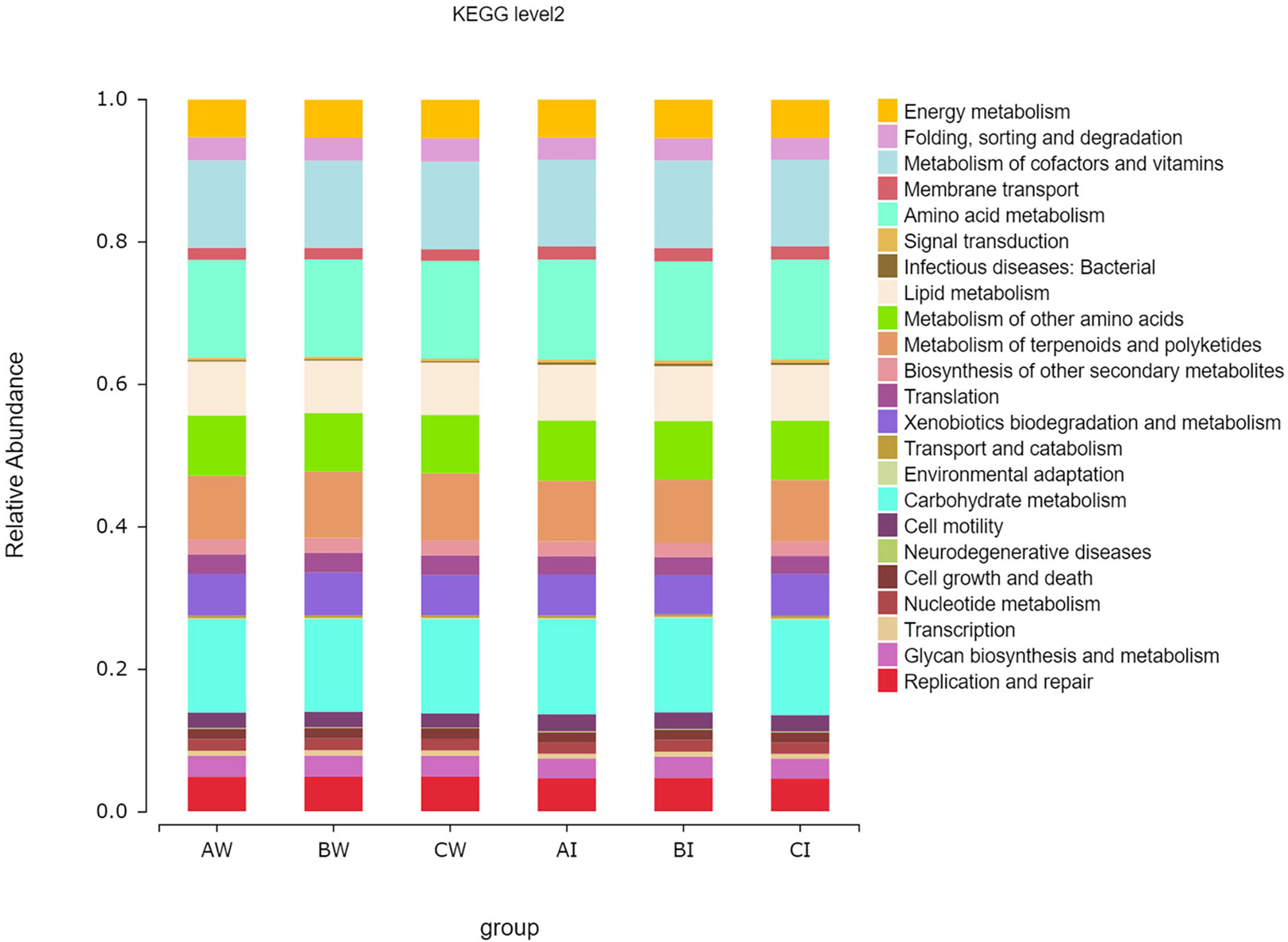
Figure 7 Effect of sodium humate (BW, BI) and sodium humate with probiotics (CW, CI) on KEGG function classification of microbiota in culture water and intestine in the three groups.
Discussion
Humic substances are organic compounds abundant in soils and sediments, divided into humic acids, fulvic acid, and humin according to pH-dependent aqueous solubility. (Alvarez-Puebla et al., 2007). Many studies on the biological effects of dietary humic acids have focused on livestock and poultry and indicate that dietary humic acids can promote animal growth and improve feed efficiency (Islam et al., 2005; Weber et al., 2014). Soluble humic acids are available in potassium humate and sodium humate. Animals prefer sodium humate because sodium is an essential inorganic electrolyte for animals. Deng et al. (2020) showed that the sodium humate content in the feed had a significant effect on tilapia’s growth performance and feed efficiency. However, few investigations have investigated the effects of dietary sodium humate on shrimps. In this study, the addition of sodium humate to culture water could promote growth, significantly increase the survival rate, and reduce the feed conversion rate of L. vannamei, which was similar to the above study results. The study outcome infers that sodium humate has a significant effect on the growth performance of L. vannamei.
Probiotics can maintain the micro-ecological balance of an organism by competing with harmful microorganisms for nutrients and space to survive and reproduce and by inhibiting the growth of harmful microorganisms (Balcázar et al., 2006; Akhter et al., 2015). In aquaculture, probiotics play an important role in maintaining water quality and improving productivity, nutrient cycling and disease control (Kuebutornye et al., 2019; El-Saadony et al., 2021). Many studies have shown that dietary supplementation with different probiotics improves the growth performance of L. vannamei and promotes the growth and health of the animals (Sadat Hoseini Madani et al., 2018; Zuo et al., 2019). In this study, adding sodium humate with probiotics to the culture water could promote growth, improve the survival rate of L. vannamei, and decrease the feed conversion rate. However, the results of probiotic addition were similar to sodium humate group on growth performance, it showed that probiotics had no function on shrimp which was inconsistent with the findings of other researchers (Linan-Vidriales et al., 2021). It may be related to the way of probiotic addition, which was only added once at the beginning of the experiment. Moreover, the ammonia nitrogen in group C was significantly lower than the control group at 15 d. The data validates that sodium humate with probiotics can reduce the accumulation of ammonia nitrogen to a certain extent in cultured water, thereby improving the growth and health of shrimp.
Digestion and nutrient absorption of food takes place in the intestine, which is closely related to the activity of intestinal digestive enzymes. The digestive capacity, growth, and development of aquatic animals are related to digestive enzyme activity, and nutrient absorption depends to a large extent on the level of enzyme activity (Assan et al., 2022). Shrimps, like other invertebrates, mainly rely on non-specific immunity to resist pathogenic bacteria (Li and Xiang, 2013; Holt et al., 2021). Shrimps’ immune system includes blood cells, several serum components, and multiple systems (coagulation protein cascade reaction, phenol oxidase progenitor system) (Song and Li, 2014). Hematocrit, lysozyme, and phenol oxidase are important indicators of shrimp immunity and play an important role in the defense response of shrimp (Rengpipat et al., 2000). Wang and Yin et al. reported that host-associated bacteria could potentially enhance enzyme production in the gut of juvenile spot shrimps (Wang et al., 2020). In the current study, both the intestinal digestive enzymes and some hepatopancreatic immune enzymes of the shrimps in the experimental groups increased to varying degrees compared to the control group, and all measured enzymes in sodium humate were significantly higher than the control group except for lysozyme, indicating that adding sodium humate to culture water improves the digestive and non-specific immune capacities of the L. vannamei. Thus, the growth performance and survival rate in sodium humate of the L. vannamei significantly increase, and the feed conversion rate reduces. However, only the SOD, PO, and Lipase activity in group C were significantly higher than the control group, and the amylase and lipase activities were significantly lower than the group B. It indicated that the addition of the probiotics was decreasing the positive effect of sodium humate addition in term of enzymatic activities. It may be directly related to the antibacterial properties of the enzymes with probiotics. The probiotics used in this study were composed of Lysinibacillus, and Bacillus subtilis strains, which produced a variety of metabolic products that could obstruct the growth of other microorganisms (Ahmad et al., 2014).
Intestinal microflora affects nutrient absorption, immune response, and intestinal mucosal morphology of the host is linked to the intestinal mucosa and constitutes a complex microbial system (Sha et al., 2016). The shrimp intestine hosts a structurally complex microflora that affects digestion efficiency and is relevant to the health and growth of the shrimp (Zheng et al., 2017). It has been shown that changes in environmental conditions can lead to an increase in the number of conditionally pathogenic bacteria, resulting in an imbalance in the intestinal microflora (Hou et al., 2017). Once the microflora has been disrupted, feeding beneficial microorganisms helps the host establish a normal microbiota (Adel et al., 2017). In this study, according to Alpha diversity statistics, sodium humate with probiotics had no significant effect on the diversity and abundance of intestinal microflora in L. vannamei, indicating a stable microflora in the L. vannamei intestine.
The bacterial diversity of culture water plays a vital role in the stability of the water environment. Bacteria in the culture environment play an important role in the nutrient cycling and mineralizing of organic compounds (Vaz-Moreira et al., 2011). Some researchers found that adding agricultural by-product sugarcane bagasse as a carbon source in biofloc systems with zero-water exchange increased the total number of heterotrophic bacteria, facilitated water recycling and improved the growth, and health of shrimp (Sharawy et al., 2022). In the current study, the Chao and Ace indices of water microflora in experimental groups are higher than the control group. The sodium humate group is significantly higher than the control group, suggesting that sodium humate as a carbon source increases the abundance of the water microbial community, with the enhanced bacterial respiration of bacteria leading to the pH reduction. The PCoA plot of the water microbial community has a clear shift with the addition of sodium humate and probiotics, suggesting that sodium humate and probiotics in the culture water have an obvious effect on the water microbial community of L. vannamei.
In this study, Proteobacteria, Bacteroidetes, and Actinobacteria are the dominant bacterial communities in the intestine and culture water of L. vannamei. Proteobacteria is the most abundant phylum, consistent with previous studies on shrimp ponds (Xiong et al., 2014; Mongkol et al., 2018). Several studies have shown that seawater microbiota is slightly more complex than the shrimp intestine (Sharawy et al., 2022). The microbial communities in water are more diverse than those in the shrimp intestine (Johnson et al., 2008). In this study, there are 31 main phyla within the water microflora of L. vannamei, and 24 main phyla in the shrimp intestine, indicating the microflora in culture water is more diverse than the intestinal microflora of L. vannamei. At the genus level, Ruegeria, Halioglobus, and Woeseia are the dominant genera in the intestine and culture water of L.vannamei, similar to the results of previous studies (Gao et al., 2022). Previous studies reported that Pseudohongiella spp. are representatives of the family Alcanivoraceae and can enhance abilities to transfer and metabolize organic and inorganic substances. In this study, the Pseudohongiella genus in culture water in the experimental groups is significantly higher than the control group, inferring that sodium humate and probiotics could indirectly improve the utilization of organic matter in culture water.
Conclusion
Under the experimental conditions of this present study. The addition of sodium humate to culture water enhanced not only the growth performance and activities of intestinal digestive enzymes and some hepatopancreas immunoenzymes but also the richness of the water microflora of L. vannamei. However, the positive effect of growth performance and enzymatic activities in the addition of sodium humate with probiotics (Lysinibacillus, Bacillus subtilis) was relatively lower than the addition of sodium humate, indicating that the addition of the probiotics was actually decreasing the positive effect of sodium humate addition, but the addition of the probiotics could effectively reduce the accumulation of ammonia nitrogen to a certain extent in the water. In a word, sodium humate can be used as a beneficial water additive for L. vannamei farming, and further research is needed on the use of sodium humate together with probiotics, especially the frequency and concentration of probiotic additions.
Data availability statement
The datasets presented in this study can be found in online repositories. The names of the repository/repositories and accession number(s) can be found below: NCBI Sequence Read Archive (SRA) database (Accession Number: SRP379080).
Ethics statement
Ethical review and approval was not required for the animal study because This experiment is a study of the shrimp.
Author contributions
WL and CS contributed to conception and design of the study. ML organized the database. ZL performed the statistical analysis. WL wrote the first draft of the manuscript. YX, JZ, YW, and DH wrote sections of the manuscript. All authors contributed to manuscript revision, read, and approved the submitted version.
Funding
This research was funded by the key research and development projects in Guangdong Province (Grant No.2020B0202010009), the project of the innovation team for the innovation and utilization of Economic Animal Germplasm in the South China Sea (Grant No.2021KCXTD026) and the Fangchenggang Science and Technology Plan Project (Grant No. AD19008017).
Acknowledgement
We are appreciative of all the support offered for this work.
Conflict of interest
The authors declare that the research was conducted in the absence of any commercial or financial relationships that could be construed as a potential conflict of interest.
Publisher’s note
All claims expressed in this article are solely those of the authors and do not necessarily represent those of their affiliated organizations, or those of the publisher, the editors and the reviewers. Any product that may be evaluated in this article, or claim that may be made by its manufacturer, is not guaranteed or endorsed by the publisher.
References
Abriouel H., Franz C. M., Omar N. B., Gálvez A. (2011). Diversity and applications of Bacillus bacteriocins. FEMS Microbiol. Rev. 35, 201–232. doi: 10.1111/j.1574-6976.2010.00244.x
Adel M., Yeganeh S., Dawood M. A. O., Safari R., Radhakrishnan S. (2017). Effects of pediococcus pentosaceus supplementation on growth performance, intestinal microflora and disease resistance of white shrimp, Litopenaeus vannamei. Aquac. Nutr. 23, 1401–1409. doi: 10.1111/anu.12515
Aguirre-Guzman G., Sanchez-Martinez J. G., Campa-Cordova A. I., Luna-Gonzalez A., Ascencio F. (2009). Penaeid shrimp immune system. Thai J. Vet. Med. 39, 205–215.
Ahmad V., Iqbal A., Haseeb M., Khan M. S. (2014). Antimicrobial potential of bacteriocin producing Lysinibacillus jx416856 against foodborne bacterial and fungal pathogens, isolated from fruits and vegetable waste. Anaerobe 27, 87–95. doi: 10.1016/j.anaerobe.2014.04.001
Akhter N., Wu B., Memon A. M., Mohsin M. (2015). Probiotics and prebiotics associated with aquaculture: a review. Fish shellfish Immunol. 45, 733–741. doi: 10.1016/j.fsi.2015.05.038
Alvarez-Puebla R. A., dos Santos D. S. Jr., Aroca R. F. (2007). SERS detection of environmental pollutants in humic acid–gold nanoparticle composite materials. Analyst 132, 1210–1214. doi: 10.1039/b711361g
Amoah K., Huang Q.-C., Tan B.-P., Zhang S., Chi S.-Y., Yang Q.-H., et al. (2019). Dietary supplementation of probiotic Bacillus coagulans ATCC 7050, improves the growth performance, intestinal morphology, microflora, immune response, and disease confrontation of pacific white shrimp, Litopenaeus vannamei. Fish shellfish Immunol. 87, 796–808. doi: 10.1016/j.fsi.2019.02.029
Assan D., Kuebutornye F. K. A., Hlordzi V., Chen H., Mraz J., Mustapha U. F., et al. (2022). Effects of probiotics on digestive enzymes of fish (finfish and shellfish); status and prospects: a mini review. Comp. Biochem. Physiol. Part B: Biochem. Mol. Biol. 257, 110653. doi: 10.1016/j.cbpb.2021.110653
Balcázar J. L., De Blas I., Ruiz-Zarzuela I., Cunningham D., Vendrell D., Múzquiz J. L. (2006). The role of probiotics in aquaculture. Vet. Microbiol. 114, 173–186. doi: 10.1016/j.vetmic.2006.01.009
Caporaso J. G., Kuczynski J., Stombaugh J., Bittinger K., Bushman F. D., Costello E. K., et al. (2010). QIIME allows analysis of high-throughput community sequencing data. Nat. Methods 7, 335–336. doi: 10.1038/nmeth.f.303
Center N. M. E. M. (2007). The specification for marine monitoring. Part 4:Seawater analysis. general administration of quality supervision, inspection and quarantine of the people's republic of china; standardization administration of China standard number GB 17378.4-2007.
Deng J., Lin B., Zhang X., Guo L., Chen L., Li G., et al. (2020). Effects of dietary sodium humate on growth, antioxidant capacity, non-specific immune response, and resistance to Aeromonas hydrophila in genetic improvement of farmed tilapia (GIFT, Oreochromis niloticus). Aquaculture 520, 734788. doi: 10.1016/j.aquaculture.2019.734788
Dougal K., de la Fuente G., Harris P. A., Girdwood S. E., Pinloche E., Geor R. J., et al. (2014). Characterisation of the faecal bacterial community in adult and elderly horses fed a high fibre, high oil or high starch diet using 454 pyrosequencing. PloS One 9, e87424. doi: 10.1371/journal.pone.0087424
Dou G., Jiang Z. (2019). Preparation of sodium humate-modified biochar absorbents for water treatment. ACS omega 4, 16536–16542. doi: 10.1021/acsomega.9b02227
Edgar R. C. (2010). Search and clustering orders of magnitude faster than BLAST. Bioinformatics 26, 2460–2461. doi: 10.1093/bioinformatics/btq461
Edgar R. C. (2013). UPARSE: highly accurate OTU sequences from microbial amplicon reads. Nat. Methods 10, 996–998. doi: 10.1038/nmeth.2604
Edgar R. C., Haas B. J., Clemente J. C., Quince C., Knight R. (2011). UCHIME improves sensitivity and speed of chimera detection. Bioinformatics 27, 2194–2200. doi: 10.1093/bioinformatics/btr381
El-Saadony M. T., Alagawany M., Patra A. K., Kar I., Tiwari R., Dawood M. A., et al. (2021). The functionality of probiotics in aquaculture: an overview. Fish Shellfish Immunol. 117, 36–52. doi: 10.1016/j.fsi.2021.07.007
Flegel T. W., Lightner D. V., Lo C. F., Owens L. (2008). Shrimp disease control: past, present and future. Dis. Asian Aquac. VI. Fish Health Section Asian Fish. Society Manila Philippines 505, 355–378.
Gao F., Zhang Y., Wu P., Chen M., He L., Xu Q., et al. (2022). Bacterial community composition in gut content and ambient sediment of two tropical wild sea cucumbers (Holothuria atra and H. leucospilota). J. Oceanol. Limnol. 40, 360–372. doi: 10.1007/s00343-021-1001-5
Gatesoupe F. J. (1999). The use of probiotics in aquaculture. Aquaculture 180, 147–165. doi: 10.1016/S0044-8486(99)00187-8
Gupta A., Gupta P., Dhawan A. (2014). Dietary supplementation of probiotics affects growth, immune response and disease resistance of Cyprinus carpio fry. Fish Shellfish Immunol. 41, 113–119. doi: 10.1016/j.fsi.2014.08.023
Hai N. (2015). The use of probiotics in aquaculture. J. Appl. Microbiol. 119, 917–935. doi: 10.1111/jam.12886
Holt C. C., Bass D., Stentiford G. D., van der Giezen M. (2021). Understanding the role of the shrimp gut microbiome in health and disease. J. invertebr. Pathol. 186, 107387. doi: 10.1016/j.jip.2020.107387
Hou D., Huang Z., Zeng S., Liu J., Wei D., Deng X., et al. (2017). Environmental factors shape water microbial community structure and function in shrimp cultural enclosure ecosystems. Front. Microbiol. 82359. doi: 10.3389/fmicb.2017.02359
Hu G., Sun Z., Gao H. (2010). Novel process of simultaneous removal of SO2 and NO2 by sodium humate solution. Environ. Sci. Technol. 44, 6712–6717. doi: 10.1021/es101892r
Islam K. M. S., Schuhmacher A., Gropp J. (2005). Humic acid substances in animal agriculture. Pakistan J. Nutr. 4, 126–134. doi: 10.3923/pjn.2005.126.134
Jatobá A., Vieira F., d. N., Buglione-Neto C. C., Mourino J. L. P., Silva B. C., et al. (2011). Diet supplemented with probiotic for Nile tilapia in polyculture system with marine shrimp. Fish Physiol. Biochem. 37, 725–732. doi: 10.1007/s10695-011-9472-5
Johnson C. N., Barnes S., Ogle J., Grimes D. J., Chang Y. J., Peacock A. D., et al. (2008). Microbial community analysis of water, foregut, and hindgut during growth of pacific white shrimp, Litopenaeus vannamei, in closed-system aquaculture. J. World Aquac. Soc. 39, 251–258. doi: 10.1111/j.1749-7345.2008.00155.x
Kuebutornye F. K., Abarike E. D., Lu Y. (2019). A review on the application of Bacillus as probiotics in aquaculture. Fish shellfish Immunol. 87, 820–828. doi: 10.1016/j.fsi.2019.02.010
Lima P. C. M., Silva A. E. M., Silva D. A., Silva S. M. B. C., Brito L. O., Gálvez A. O. (2021). Effect of stocking density of crassostrea sp. in a multitrophic biofloc system with Litopenaeus vannamei in nursery. Aquaculture 530, 735913. doi: 10.1016/j.aquaculture.2020.735913
Linan-Vidriales M. A., Pena-Rodriguez A., Tovar-Ramirez D., Elizondo-Gonzalez R., Barajas-Sandoval D. R., Ponce-Gracia E. I., et al. (2021). Effect of rice bran fermented with Bacillus and Lysinibacillus species on dynamic microbial activity of pacific white shrimp (Penaeus vannamei). Aquaculture 531, 8. doi: 10.1016/j.aquaculture.2020.735958
Liu L., Hu Z., Dai X., Avnimelech Y. (2014). Effects of addition of maize starch on the yield, water quality and formation of bioflocs in an integrated shrimp culture system. Aquaculture 418, 79–86. doi: 10.1016/j.aquaculture.2013.10.005
Li F., Xiang J. (2013). Signaling pathways regulating innate immune responses in shrimp. Fish shellfish Immunol. 34, 973–980. doi: 10.1016/j.fsi.2012.08.023
Magoc T., Salzberg S. L. (2011). FLASH: fast length adjustment of short reads to improve genome assemblies. Bioinformatics 27, 2957–2963. doi: 10.1093/bioinformatics/btr507
Mongkol P., Bunphimpapha P., Rungrassamee W., Arayamethakorn S., Klinbunga S., Menasveta P., et al. (2018). Bacterial community composition and distribution in different segments of the gastrointestinal tract of wild-caught adult Penaeus monodon. Aquac. Res. 49, 378–392. doi: 10.1111/are.13468
Motta F. L., Santana M. (2013). Production of humic acids from oil palm empty fruit bunch by submerged fermentation with trichoderma viride: Cellulosic substrates and nitrogen sources. Biotechnol. Prog. 29, 631–637. doi: 10.1002/btpr.1715
Niu Q., Li P., Hao S., Zhang Y., Kim S. W., Li H., et al. (2015). Dynamic distribution of the gut microbiota and the relationship with apparent crude fiber digestibility and growth stages in pigs. Sci. Rep. 5, 1–7. doi: 10.1038/srep09938
Pinto P. H. O., Rocha J. L., do Vale Figueiredo J. P., Carneiro R. F. S., Damian C., de Oliveira L., et al. (2020). Culture of marine shrimp (Litopenaeus vannamei) in biofloc technology system using artificially salinized freshwater: Zootechnical performance, economics and nutritional quality. Aquaculture 520, 734960. doi: 10.1016/j.aquaculture.2020.734960
Reid G., Burton J. (2002). Use of Lactobacillus to prevent infection by pathogenic bacteria. Microbes infect. 4, 319–324. doi: 10.1016/S1286-4579(02)01544-7
Reid G., Friendship R. (2002). Alternatives to antibiotic use: probiotics for the gut. Anim. Biotechnol. 13, 97–112. doi: 10.1081/ABIO-120005773
Rengpipat S., Rukpratanporn S., Piyatiratitivorakul S., Menasaveta P. (2000). Immunity enhancement in black tiger shrimp (Penaeus monodon) by a probiont bacterium (Bacillus S11). Aquaculture 191, 271–288. doi: 10.1016/S0044-8486(00)00440-3
Sadat Hoseini Madani N., Adorian T. J., Ghafari Farsani H., Hoseinifar S. H. (2018). The effects of dietary probiotic bacilli (Bacillus subtilis and Bacillus licheniformis) on growth performance, feed efficiency, body composition and immune parameters of whiteleg shrimp (Litopenaeus vannamei) postlarvae. Aquac. Res. 49, 1926–1933. doi: 10.1111/are.13648
Schloss P. D., Westcott S. L., Ryabin T., Hall J. R., Hartmann M., Hollister E. B., et al. (2009). Introducing mothur: open-source, platform-independent, community-supported software for describing and comparing microbial communities. Appl. Environ. Microbiol. 75, 7537–7541. doi: 10.1128/AEM.01541-09
Sha Y., Liu M., Wang B., Jiang K., Qi C., Wang L. (2016). Bacterial population in intestines of Litopenaeus vannamei fed different probiotics or probiotic supernatant. J. Microbiol. Biotechnol. 26, 1736–1745. doi: 10.4014/jmb.1603.03078
Sharawy Z. Z., Abbas E. M., Abdelkhalek N. K., Ashry O. A., Abd El-Fattah L. S., El-Sawy M. A., et al. (2022). Effect of organic carbon source and stocking densities on growth indices, water microflora, and immune-related genes expression of Litopenaeus vannamei larvae in intensive culture. Aquaculture 546, 737397. doi: 10.1016/j.aquaculture.2021.737397
Song Y.-L., Li C.-Y. (2014). Shrimp immune system-special focus on penaeidin. J. Mar. Sci. Technol. 22, 1–8. doi: 10.6119/JMST-013-0813-1
Sunitha K., Padmavathi P. (2013). Influence of probiotics on water quality and fish yield in fish ponds. Int. J. Pure Appl. Sci. Technol. 19, 48–60.
Sun Z., Zhao Y., Gao H., Hu G. (2010). Removal of SO2 from flue gas by sodium humate solution. Energy fuels 24, 1013–1019. doi: 10.1021/ef901052r
Thitamadee S., Prachumwat A., Srisala J., Jaroenlak P., Salachan P. V., Sritunyalucksana K., et al. (2016). Review of current disease threats for cultivated penaeid shrimp in Asia. Aquaculture 452, 69–87. doi: 10.1016/j.aquaculture.2015.10.028
Vaz-Moreira I., Egas C., Nunes O. C., Manaia C. M. (2011). Culture-dependent and culture-independent diversity surveys target different bacteria: a case study in a freshwater sample. Antonie Van Leeuwenhoek 100, 245–257. doi: 10.1007/s10482-011-9583-0
Wang Y., Al Farraj D. A., Vijayaraghavan P., Hatamleh A. A., Biji G. D., Rady A. M. (2020). Host associated mixed probiotic bacteria induced digestive enzymes in the gut of tiger shrimp Penaeus monodon. Saudi J. Biol. Sci. 27, 2479–2484. doi: 10.1016/j.sjbs.2020.07.010
Weber T., Van Sambeek D., Gabler N., Kerr B., Moreland S., Johal S., et al. (2014). Effects of dietary humic and butyric acid on growth performance and response to lipopolysaccharide in young pigs. J. Anim. Sci. 92, 4172–4179. doi: 10.2527/jas.2013-7402
Wilkinson T. J., Huws S. A., Edwards J. E., Kingston-Smith A. H., Siu-Ting K., Hughes M., et al. (2018). CowPI: a rumen microbiome focussed version of the PICRUSt functional inference software. Front. Microbiol. 91095. doi: 10.3389/fmicb.2018.01095
Xiong J., Zhu J., Wang K., Wang X., Ye X., Liu L., et al. (2014). The temporal scaling of bacterioplankton composition: high turnover and predictability during shrimp cultivation. Microbial. Ecol. 67, 256–264. doi: 10.1007/s00248-013-0336-7
Yi J.-Z., Zhang L.-M. (2008). Removal of methylene blue dye from aqueous solution by adsorption onto sodium humate/polyacrylamide/clay hybrid hydrogels. Bioresource technology 99, 2182–2186. doi: 10.1016/j.biortech.2007.05.028
Yılmaz S., Ergun S., Çelik E.Ş., Yigit M. (2018). Effects of dietary humic acid on growth performance, haemato-immunological and physiological responses and resistance of rainbow trout, Oncorhynchus mykiss to Yersinia ruckeri. Aquac. Res. 49, 3338–3349. doi: 10.1111/are.13798
Zhang M., Pan L., Fan D., He J., Su C., Gao S., et al. (2021). Study of fermented feed by mixed strains and their effects on the survival, growth, digestive enzyme activity and intestinal flora of Penaeus vannamei. Aquaculture 530, 735703. doi: 10.1016/j.aquaculture.2020.735703
Zheng Y., Hua S., Wang A. (2010). Adsorption behavior of Cu2+ from aqueous solutions onto starch-g-poly (acrylic acid)/sodium humate hydrogels. Desalination 263, 170–175. doi: 10.1016/j.desal.2010.06.054
Zheng Y., Yu M., Liu J., Qiao Y., Wang L., Li Z., et al. (2017). Bacterial community associated with healthy and diseased pacific white shrimp (Litopenaeus vannamei) larvae and rearing water across different growth stages. Front. Microbiol. 8. doi: 10.3389/fmicb.2017.01362
Zibiene G., Zibas A. (2019). Impact of commercial probiotics on growth parameters of European catfish (Silurus glanis) and water quality in recirculating aquaculture systems. Aquac. Int. 27, 1751–1766. doi: 10.1007/s10499-019-00428-9
Zokaeifar H., Balcazar J. L., Saad C. R., Kamarudin M. S., Sijam K., Arshad A., et al. (2012). Effects of Bacillus subtilis on the growth performance, digestive enzymes, immune gene expression and disease resistance of white shrimp, Litopenaeus vannamei. Fish Shellfish Immunol. 33, 683–689. doi: 10.1016/j.fsi.2012.05.027
Keywords: sodium humate, probiotics, Litopenaeus vannamei, enzyme activity, microflora
Citation: Liao WQ, Lin ZX, Liao MZ, Xue Y, Zhou JN, Wang Y, Hou DQ and Sun CB (2022) Effects of sodium humate and probiotics on growth performance enzyme activity and microbial environment of Litopenaeus vannamei in high-density zero‐water exchange systems. Front. Mar. Sci. 9:989325. doi: 10.3389/fmars.2022.989325
Received: 08 July 2022; Accepted: 05 September 2022;
Published: 20 September 2022.
Edited by:
Johan Verreth, Wageningen University and Research, NetherlandsReviewed by:
Yafei Duan, South China Sea Fisheries Research Institute, ChinaMagdalena Lenny Situmorang, Bandung Institute of Technology, Indonesia
Copyright © 2022 Liao, Lin, Liao, Xue, Zhou, Wang, Hou and Sun. This is an open-access article distributed under the terms of the Creative Commons Attribution License (CC BY). The use, distribution or reproduction in other forums is permitted, provided the original author(s) and the copyright owner(s) are credited and that the original publication in this journal is cited, in accordance with accepted academic practice. No use, distribution or reproduction is permitted which does not comply with these terms.
*Correspondence: Chengbo Sun, suncb@gdou.edu.cn