- 1Tjärnö Marine Laboratory, Department of Marine Sciences, University of Gothenburg, Strömstad, Sweden
- 2Swedish Food Agency, Uppsala, Sweden
- 3Division of Food and Nutrition Science, Department of Biology and Biological Engineering, Chalmers University of Technology, Gothenburg, Sweden
Ulva spp. (sea lettuce) has recently gained attention as a sustainable protein source due to its high productivity and many nutritional properties interesting for the food industry. In this study, we explored a possible industrial symbiosis between herring production processing industries and Ulva fenestrata cultivation. We show that U. fenestrata cultivated in herring production process waters had four to six times higher biomass yields (27.17 - 37.07 g fresh weight vs. 6.18 g fresh weight) and three times higher crude protein content (> 30% dry weight vs. 10% dry weight) compared to U. fenestrata cultivated in seawater. Along with the elevation of protein, the herring production process waters also significantly increased levels of all essential amino acids in the seaweed biomass. The content of some heavy metals (arsenic, mercury, lead, and cadmium) was well below the maximum allowed levels in foodstuff. Therefore, quantities of biomass around 100 g dry weight could be consumed daily following the US Environmental Protection Agency’s reference doses. Combined, the results show that cultivation of U. fenestrata in herring production process waters has great potential to produce sustainable proteins for the growing world population. At the same time, nutrients of currently discarded process waters are circulated back to the food chain.
Introduction
The demand for protein rich and nutritious food is steadily increasing, and it is evident that we need more sustainable food production systems to feed the growing human population (Aiking, 2014; Gephart et al., 2021). Seaweed aquaculture is considered one of the most environmentally friendly aquaculture practices, with a net uptake of both nitrogen and phosphorus (Buschmann et al., 2017; Gephart et al., 2021). The green seaweed Ulva spp. (sea lettuce) has received attention by the aquaculture sector due to its high productivity and high environmental tolerance (Bolton et al., 2016; Nardelli et al., 2019; Steinhagen et al., 2021). Ulva biomass has many nutritional properties interesting for food and feed applications (Bolton et al., 2016). Its protein content is often relatively high (10 – 20% of dry weight (dw)) (Fleurence, 2016; Toth et al., 2020; Steinhagen et al., 2021) compared to most vegetable staple foods, but still not as high as in, for example, soybean biomass (35 - 40% dw) (Grieshop and Fahey, 2001; Thakur and Hurburgh, 2007). Further, its proteins have a high proportion of essential amino acids (Machado et al., 2020). However, Ulva can increase growth and alter biochemical properties by responding to changing environmental conditions and higher nutrient availability (Bolton et al., 2016; Toth et al., 2020; Steinhagen et al., 2022). Recently, we found that Ulva fenestrata Postels & Ruprecht increases both its growth and crude protein content when cultivated in a wide variety of food production process waters (e.g. from shrimp, herring, and oat-milk production) (Stedt et al., 2022b), and there is a great potential to cultivate U. fenestrata with many different kinds of nutrient rich waters (Arumugam et al., 2018; Stedt et al., 2022a).
Water is used in almost every step of food processing, and for some seafood up to 50 m3 process water is used per tonne of final product (Forghani et al., 2020a; Forghani et al., 2020b). These process waters contain a wide range of nutrients and can be used for downstream recovery of macronutrients or as feed stock e.g. for microorganisms while they are still in a food grade state (Osman et al., 2015; Forghani et al., 2020a; Forghani et al., 2020b; Forghani et al., 2022). By integrating seaweed cultivation with industries that generate large quantities of nutrient rich process waters, some of the major challenges for land-based seaweed cultivations such as seawater intake and costly infrastructure, can be reduced. The possibility for an industrial symbiosis between food processing industries and seaweed cultivation is thus high.
Despite all the promising health benefits of eating seaweeds, it is important to determine possible toxic elements in the biomass to estimate potential health risks associated with its consumption (Almela et al., 2002; Mæhre et al., 2014; Neveux et al., 2016; Arumugam et al., 2018). To date, there are no general regulations from the European commission regarding maximum levels of heavy metals in seaweeds intended as food (European Commission, 2018). But as the interest for consuming seaweeds is growing outside of Asia, it is increasingly important to assess the seaweeds’ contribution to human exposure of heavy metals on the Western market. To establish the true potential of U. fenestrata as a food source, it is therefore important to quantify and evaluate heavy metals in the biomass originating from different cultivation settings, and to report realistic values of how much biomass that can safely be consumed each day.
Our goal in this study was twofold, firstly we wanted to assess if the increased growth and crude protein content of U. fenestrata cultivated in two herring production process waters (HPPWs) that we previously reported (Stedt et al., 2022b) were maintained when the cultivation setting was up-scaled. Secondly, we wanted to establish if U. fenestrata cultivated in these two HPPWs can become a nutritionally sound and safe protein source for the future. We answered these questions in two experiments. In the first experiment we investigated how growth, crude protein content, and content of some health concerning toxic elements (commonly grouped as “heavy metals”: arsenic, mercury, lead, and cadmium) in U. fenestrata were affected by cultivation for 28 days in the two different HPPWs. Based on the results from the first experiment, we ran a second experiment for 14 days where we cultivated U. fenestrata in the two HPPWs, this time monitoring growth, crude protein content and amino acid composition of U. fenestrata.
Materials and methods
Algal material and taxonomic identification
Algal material were collected from a long-term indoor tank culture at Tjärnö Marine Laboratory (TML, 58°52′33.7′′ N, 11°08′44.9′′ E), where gametophytes of U. fenestrata were cultivated in 90 L cultivation tanks, at 14°C, under 16:8 h (L:D) light cycle, at an irradiance of 100 μmol m-2 s-1 and in seawater supplemented by half strength Provasoli Enriched Seawater (PES) (Provasoli, 1968; Redmond et al., 2014). Molecular identification of the algal material used in the present study has been described by Toth et al. (2020) (GenBank accession numbers: MN240309, MN240310, MN240311).
Herring production process waters
The two herring production process waters (HPPWs) used in the experiments were collected in a food grade state in February 2021. The process waters included: (i) tub water (TUB) from in-house pre-processing storage of whole herring in 3% NaCl at a primary processor (Sweden Pelagic AB, Ellös, Sweden), and (ii) saturated salt brine (SAL) from maturation of herring fillets at a secondary processor (Klädesholmen Seafood AB, Rönnäng, Sweden). A detailed explanation of the HPPWs has been described by Stedt et al. (2022b). After collection, the waters were filtered to remove coarse particles (> 300 μm) and stored at - 60°C. The HPPWs were quantified for ammonium, nitrate and nitrite using commercial enzymatic kits following the method described in Stedt et al. (2022b), while the inorganic phosphorus/orthophosphate content was measured with a standard curve made with monopotassium phosphate as reported by Qvirist et al. (2015). The HPPWs were diluted with filtered (0.2 μm + UV-light treated) deep-sea (40 m) seawater to 25 μM NH4+ in both experiments (Table 1).

Table 1 Characterization of the seawater (SW) that was used as the control and to dilute the herring production process waters (HPPWs), as well as the undiluted HPPWs SAL and TUB (mean ± SEM, n = 3) collected in February 2021.
Experimental setup
The effect of HPPWs as cultivation media on growth and chemical composition of U. fenestrata was explored in two different land-based laboratory experiments. Both experiments used nine 14 L tanks filled to 10 L at 14°C, under 16:8 h (L:D) light cycle, and at an irradiance of 100 μmol m-2 s-1 (light source: INDY66 LED 60 W 4000 K 6000 lm). The experiments included the two HPPWs TUB and SAL diluted to 25 μM NH4+, as well as a seawater control (SW) set up in triplicates (Table 1). Both salinity (WTW MultiLine 3420, Xylem Analytics) and pH (Mettler Toledo FiveEasy bench meter) remained stable throughout the experiments in all treatments (34.0 ± 1.0 ppt and 7.9 ± 0.2 respectively, mean ± SD). Every second day the tanks were cleaned, and the respective waters were renewed to avoid nutrient depletion and microbial spoilage. The tanks were spatially interdispersed to avoid position biases.
In the first experiment, lasting 28 days, U. fenestrata (approximately 7 cm2) were cleaned and divided into the tanks at a density of 0.3 g L-1 fresh weight (fw). The total fw of the U. fenestrata in each tank was measured every seventh day, crude protein content was measured bi-weekly, while heavy metal content was measured at the end of the experiment.
Based on the significant differences in fw and crude protein content between the seawater control and the HPPWs recorded after 14 days in the first experiment, the second experiment lasted for only 14 days. The U. fenestrata were cleaned and cut into 30 cm2 discs and added to the tanks at a density of 0.3 g L-1 fw. The total fw and crude protein content of the U. fenestrata in each tank were measured on day 7 and 14. The amino acid composition of U. fenestrata was measured at the end of the experiment.
Growth
The biomass yield (fw) in each tank was determined in a standardized way by weighing the U. fenestrata on a lab-scale (Sartorius TE1502S, Göttingen, Germany), after removing the excess water with a salad spinner.
Crude protein content
Tissue samples from each tank were haphazardly collected, frozen, freeze-dried and homogenized to a fine powder. Total nitrogen content was analyzed using combustion GSL elemental analyzer coupled to an isotope-ratio mass spectrometer (EA-IRMS, 20 - 22, Sercon Ltd., Crewe UK). The nitrogen content was converted to crude protein content using a conversion factor of five (Angell et al., 2016).
Heavy metal content and exposure assessment
For the first experiment, random freeze-dried, homogenized tissue samples (0.5 g dw) from two tanks per HPPW treatment were analyzed for heavy metal content. Total arsenic (tAs), mercury (Hg), lead (Pb), and cadmium (Cd) were quantified at the Swedish Food Agency, Uppsala, Sweden, using inductively coupled plasma mass spectrometry (ICP-MS, Agilent 7700x) following the procedures of NMKL no. 186 and EN 15763:2009, which includes total microwave acid digestion of the samples using a mixture of concentrated nitric acid and hydrochloric acid (6 + 1 mL). The determination of inorganic arsenic (iAs) was performed at the Swedish Food Agency, Uppsala, Sweden, according to the European Standard EN 16802:2016. The method is based on extraction with dilute nitric acid and hydrogen peroxide in hot water bath, followed by analysis with strong anion exchange HPLC-ICP-MS (Agilent 1260 Infinity Quaternary LC and Agilent 7700x ICP-MS). The method performances are described in Kollander et al. (2019). The heavy metal contents were compared to the maximum allowed levels in foodstuff (MLs) set by the European Union’s Commission Regulation (EC) No. 1881/2006 (version 03/05/2022). Furthermore, a simple exposure assessment was performed to establish how much unprocessed dried seaweed biomass that can be consumed without exceeding the reference doses (RfD) set by US Environmental Protection Agency (US EPA):
Where RfDi is the reference dose for heavy metal i (μg kg bw-1 day-1) (US EPA, 2007), bw is reference body weight for adults (63.3 kg) (EFSA, 2017), and Ci is the content of heavy metal i in dry seaweed biomass (μg g dw-1). Because iAs is the most toxic species of arsenic, RfD values are only established for this form of As (US EPA, 2007). The US EPA RfD was chosen over the EU Tolerable daily intake (TDI) in order to simplify comparisons with other publications in the area.
Amino acid composition and human requirements
Total amino acids (TAA) of U. fenestrata in the second experiment were analyzed following the method in Trigo et al. (2021), with minor modifications. All measurements were made in triplicates. First, 100 mg of the homogenized seaweed tissue samples and 4 mL of 6 M HCl were mixed in a screw-cap glass tubes, after which the air in the tubes was replaced with nitrogen. The samples were hydrolyzed at 110°C for 24 h using a heat block, and the samples were then diluted with 0.2 M acetic acid and filtered (0.22 μm; Fisher Scientific). Two μL of each sample were run in a LC/MS (Agilent 1100 HPLC) with a Phenomenex column (C18 250 μm x 4.6 μm x 3 μm), coupled to an Agilent 6210 quadrupole in the SIM positive mode (Agilent Technologies). Separation at 0.7 mL min-1 for 40 min was performed using different ratios of mobile phase A (3% methanol, 0.2% formic acid and 0.01% acetic acid) and mobile phase B (50% methanol, 0.2% formic acid and 0.01% acetic acid). A mix of 17 amino acids (Thermo Scientific) was used for the calibration curve. Due to the use of acidic hydrolysis, (i) tryptophan and cysteine could not be recovered, and (ii) glutamine and asparagine were co-determined with glutamic and aspartic acid, respectively. MassHunter Quantitative Analysis software (version B.09.00, Agilent Technologies) was used to analyze the data.
The required consumption of dried seaweed biomass per day, in order to receive all the essential amino acids, was established with the following equation:
Where rEAAi is the essential amino acid requirement per day (mg kg bw-1 day-1) provided by World Health Organization (WHO/FAO/UNU, 2007), bw is the reference body weight for adults (63.3 kg) (EFSA, 2017), and EAAi is the amino acid content of amino acid i in dry seaweed biomass (mg g dw-1).
Statistical analysis
All statistical analyses were performed in RStudio (v. 1.2.5001). All data were visually checked for homogeneity and normality with diagnostic plots (density-, normality- and QQ-plots). Furthermore, normality was checked with Shapiro Wilks test for each combination of factor levels, and Levene’s test was used to test for homogeneity of variance at each level of time. Growth and crude protein content were analyzed using a two-way mixed model ANOVA with day as within (repeated measures) and treatment as between subject factors. Comparisons between treatment and day were analyzed post-hoc with pairwise paired t-test (Bonferroni corrected). Amino acids were analyzed with one-way ANOVA followed by Tukey’s post-hoc test for pairwise comparison.
Results
Growth
In the first experiment there was a statistically significant interaction between treatment and time in explaining growth (Table 2). After 28 days the biomass yield had increased 13 times in TUB (37.07 ± 2.05 g fw), nine times in SAL (27.17 ± 0.75 g fw), but only two times in SW (6.18 ± 0.21 g fw, mean ± SEM) (Figure 1A). This led to four to six times higher biomass yield in the HPPWs compared to in the seawater control. The effect of treatment was significant at day 14, 21, and 28 (p < 0.005), and the pairwise comparisons show that the mean fw was significantly higher in TUB and SAL compared to SW on day 14, 21 and 28 (p < 0.05). TUB had significantly higher fw compared to SAL on day 21 and 28 (p < 0.005).
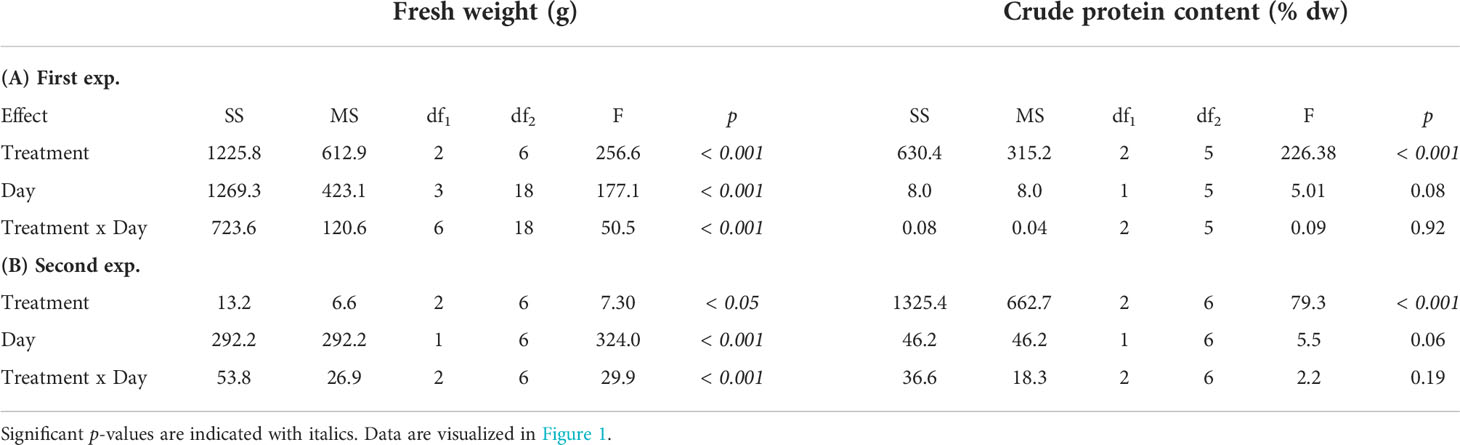
Table 2 Two-way mixed model ANOVA (Type III) of fresh weight (g) and crude protein content (% dw) in the (A) first, and (B) second experiment of Ulva fenestrata cultivated in the two different herring production process waters (SAL and TUB), as well as the seawater control (SW).
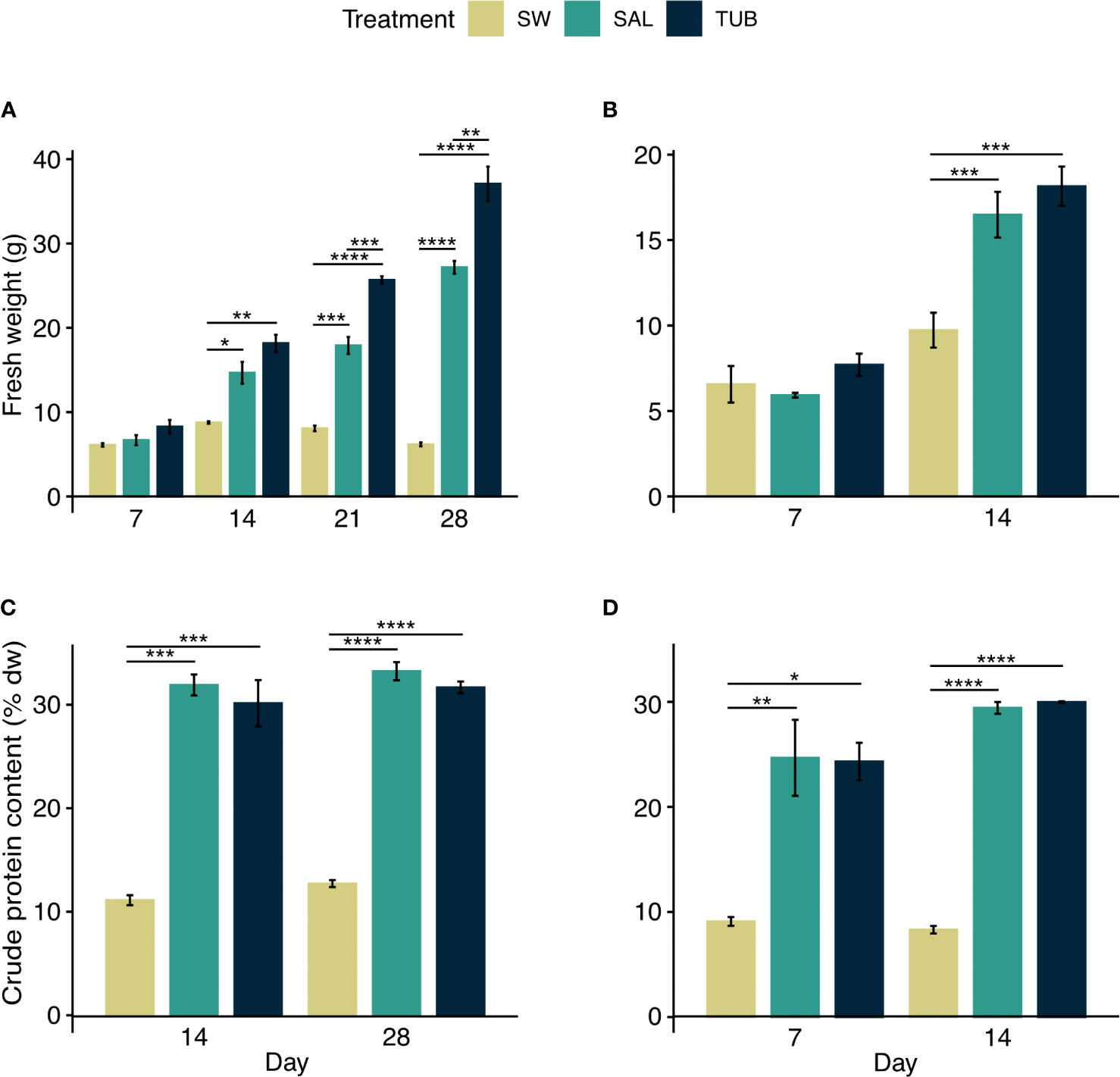
Figure 1 Fresh weight (g) of Ulva fenestrata cultivated in seawater control (SW) and the two herring production process waters salt brine (SAL) and tub water (TUB) during the (A) first experiment, and (B) second experiment, as well as their crude protein content (% dw) in the (C) first experiment, and (D) second experiment (mean ± SEM, n = 3). Significant differences from the pairwise paired t-test are denoted by asterisks.
In the second experiment, there was a statistically significant interaction between treatment group and time in explaining growth (Table 2). The biomass yield had increased six times in TUB (18.15 ± 1.15 g fw), five times in SAL (16.49 ± 1.33 g fw), and three times in SW (9.73 ± 1.02 g fw, mean ± SEM) after 14 days (Figure 1B), which led to double the biomass yield in the HPPWs compared to in the seawater control. The effect of treatment was significant after 14 days (p < 0.005), and the pairwise comparisons show that the mean fw was significantly higher in TUB and SAL compared to SW (p < 0.05).
Crude protein content
In the first experiment, seaweeds reached crude protein contents of 31.67 ± 0.57, 33.24 ± 0.88, and 12.72 ± 0.34% dw (mean ± SEM) in TUB, SAL, and SW, respectively (Figure 1C). There was no statistically significant interaction between treatment group and time explaining crude protein content (Table 2). The statistical analysis showed that treatment was the factor influencing the crude protein content, and that there was no statistically significant effect of days (Table 2). The crude protein content of the seaweed was nearly three times higher in both the HPPWs compared to in the seawater control after both 14 (p < 0.001) and 28 days (p < 0.001).
In the second experiment, seaweeds reached crude protein contents of 29.98 ± 0.08, 29.42 ± 0.56, and 8.31 ± 0.36% dw (mean ± SEM) in TUB, SAL, and SW, respectively (Figure 1D). There was no statistically significant interaction between treatment group and time explaining crude protein content (Table 2). The statistical analysis showed that treatment was the factor influencing the crude protein content, and that there was no statistically significant effect of days (Table 2). The crude protein content of the seaweed was almost three times higher in both the HPPWs compared to in the seawater control after seven days (p < 0.05), and four times higher after 14 days (p < 0.001).
Heavy metal content and exposure assessment
The heavy metal content of U. fenestrata cultivated in TUB and SAL from the first experiment were all below the EU maximum levels (MLs) in foodstuff. For TUB the Hg content of the biomass was below the method’s quantification limit (0.036 μg g dw-1) (Table 3). The tAs of U. fenestrata cultivated in TUB (106.95 ± 6.14 μg g dw-1) was higher than when cultivated in SAL (4.85 ± 0.10 μg g dw-1, mean ± SEM). However, the percentage of iAs was only 0.02% of tAs for biomass cultivated in TUB, and 0.32% in SAL. The level of iAs in both HPPWs was 0.02 μg g dw-1, which is well below the EU MLs for iAs in food (0.1 - 0.3 μg g-1, Table 3).
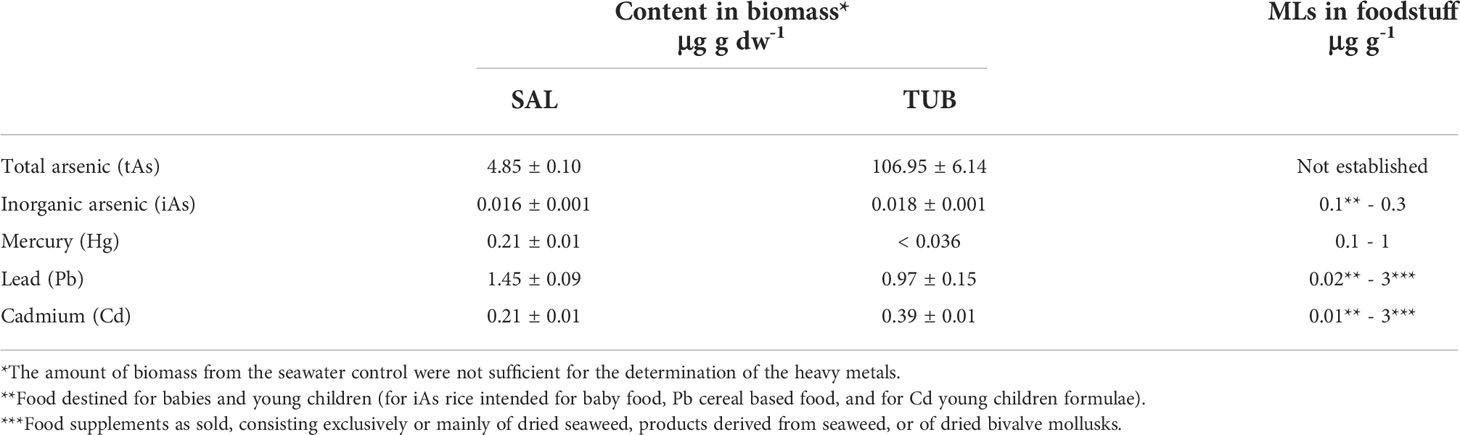
Table 3 Heavy metal content (μg g dw-1) in Ulva fenestrata cultivated in the two herring production process waters salt brine (SAL) and tub water (TUB) after 28 days (mean ± SEM, n = 2), as well as the maximum allowed levels in foodstuff (MLs) set by the European Union’s Commission Regulation (EC) No. 1881/2006 (version 03/05/2022).
The simple exposure assessment indicates that it is possible to eat approximately 90 g dw of U. fenestrata cultivated in SAL (limiting heavy metal Hg), and 160 g dw of U. fenestrata cultivated in TUB (limiting heavy metal Cd) daily before exceeding the RfDs of US EPA (Table 4).
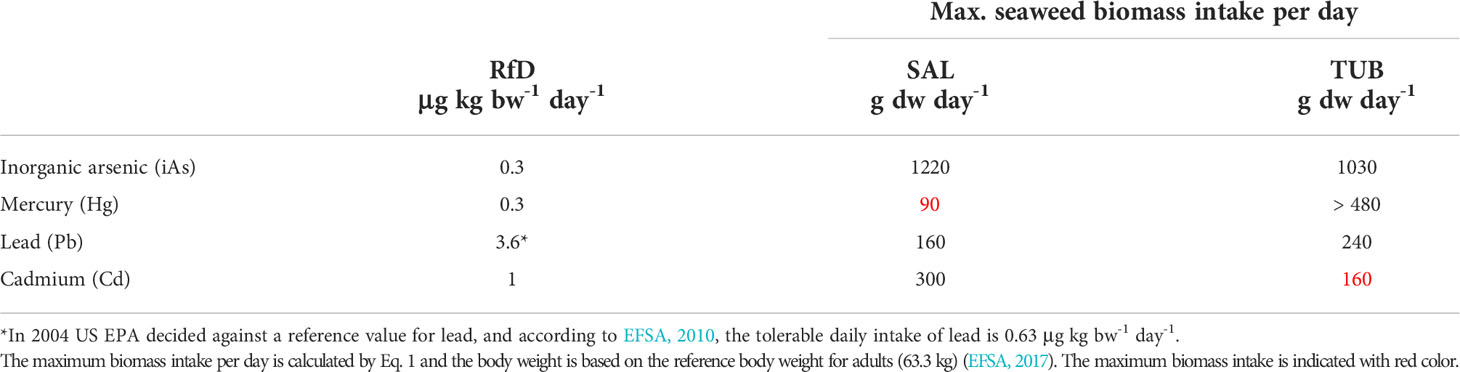
Table 4 Exposure assessment indicating how much dried biomass of Ulva fenestrata cultivated in the two herring production process waters salt brine (SAL) and tub water (TUB) that can be consumed before exceeding the reference dose (RfD) for each heavy metal, as indicated by the US Environmental Protection Agency (US EPA, 2007).
Amino acid composition and human requirements
All amino acids (AA) were present at significantly higher levels in U. fenestrata cultivated in the HPPWs compared to in the seawater control (Table 5), with no difference between TUB and SAL. Most AA were two to three times higher in seaweeds cultivated in HPPWs compared to in SW (p < 0.05), except for lysine and glutamic acid, which were four times higher (p < 0.05), and methionine and arginine which were six to eight times higher (p < 0.05). As a result of the high amount of some non-essential AA such as glutamic acid and arginine in biomass cultivated in the HPPWs, the relative TEAA was lower in the HPPWs compared to in the SW (p < 0.05).
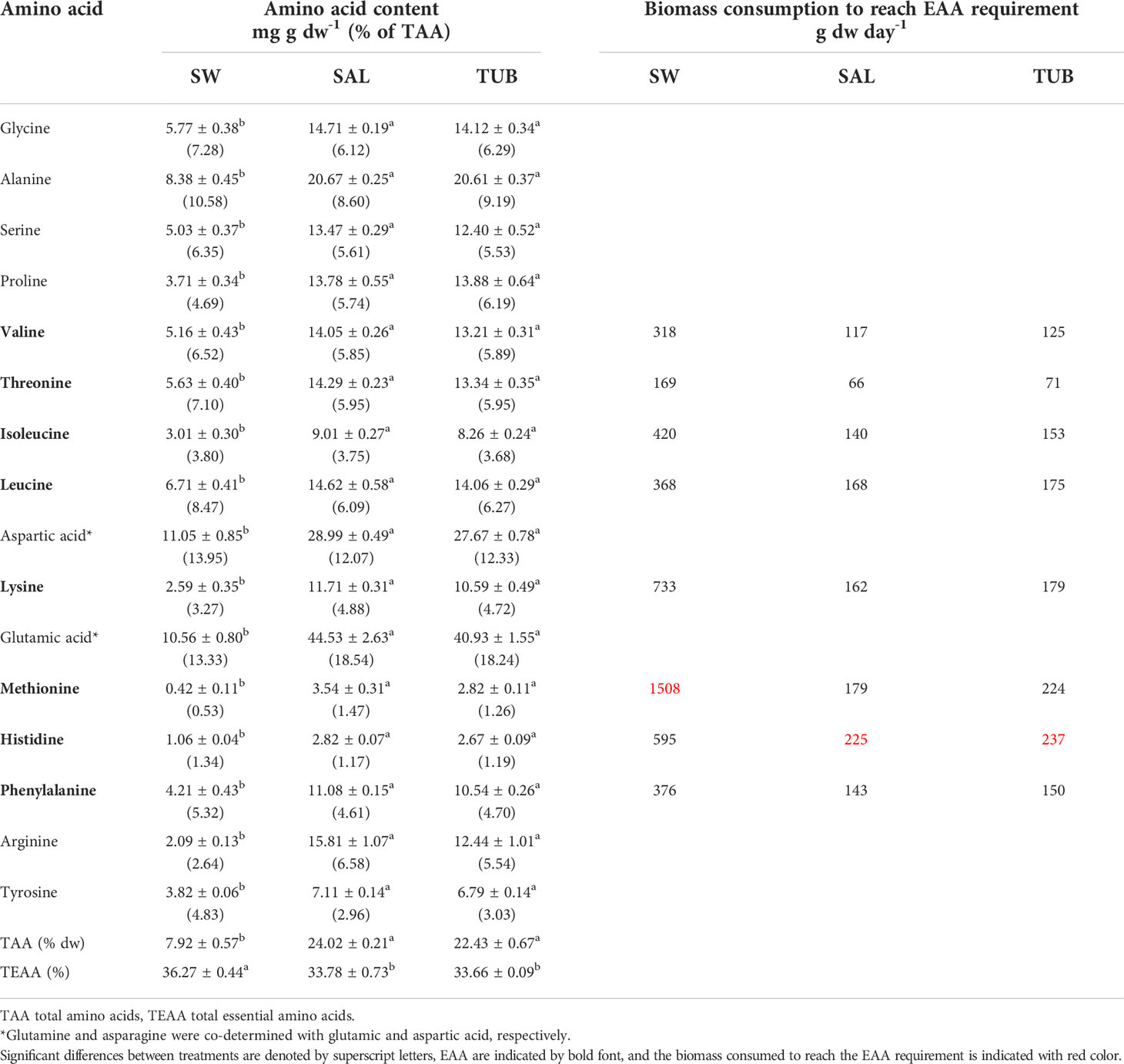
Table 5 Amino acid composition (mg g dw-1) of dried biomass of Ulva fenestrata cultivated in seawater control (SW) and the two herring production process waters salt brine (SAL) and tub water (TUB) (mean ± SEM, n = 3) after 14 days, as well as the biomass (g dw day-1) consumption needed to reach the recommended daily intake of essential amino acids (EAA) (Eq. 2, based on a body weight of 63.3 kg) as provided by the World Health Organization (WHO/FAO/UNU, 2007).
Discussion
Land-based cultivation of Ulva has several advantages over sea-based cultivations, such as full control of environmental cultivation conditions and easy accessibility and harvest of the biomass (Hafting et al., 2012). However, even if there are some commercial land-based cultivations (such as Algalplus, Monterey Bay Seaweed, Blue Evolution, and Nordic Seafarm), they are often costly to operate and maintain, which has limited the commercial interest of the systems (Titlyanov and Titlyanova, 2010; Hafting et al., 2012; Hafting et al., 2015). In this study, we show that one way to maximize the value of land-based produced seaweed biomass is to integrate U. fenestrata cultivation with high-nutrient food production process waters from the herring processing industry.
Proteins and amino acids
We show that U. fenestrata responds quickly to the addition of the process waters (HPPWs) by increasing its crude protein content and TAA significantly. After only seven days the U. fenestrata in HPPWs have reached crude protein contents of 24 - 25% dw compared to 9% dw in the seawater control. These values are already within the high range for U. fenestrata (Holdt and Kraan, 2011; Toth et al., 2020; Steinhagen et al., 2021), but after another seven days the crude protein content was over 30% dw. This crude protein content is comparable to soybean (35 - 40% dw) (Grieshop and Fahey, 2001; Thakur and Hurburgh, 2007), and pulses such as lentils, chickpeas and beans, in which crude protein makes up 17 - 30% dw (Boye et al., 2010). The rapid increase in crude protein content indicates that the biomass from these cultivation settings can become an alternative protein source for human consumption, comparable to high-protein terrestrial crops.
Most seaweed species contain all the essential amino acids (Fleurence, 2004), and the amino acid profile of the U. fenestrata cultivated in our experiment revealed that cultivation in HPPWs further increased the levels of all amino acids along with the buildup of proteins. The lower TAA content compared to the crude protein content can possibly be explained by the selected method for the amino acid analysis, in which the acidic hydrolysis prevents the recovery of tryptophan and cysteine, and the use of single hydrolysis time may destroy some amino acids (Angell et al., 2016). For U. fenestrata cultivated in the seawater control, the limiting amino acid was methionine, which is generally perceived as a limiting amino acid in seaweed proteins (Holdt and Kraan, 2011; Thiviya et al., 2022). However, when cultivating U. fenestrata in the HPPWs, methionine increased over six times and was no longer limiting. Lysine, another important EAA that is often limiting in proteins from seaweeds (Holdt and Kraan, 2011; Thiviya et al., 2022), follows the same pattern as methionine and was not limiting in U. fenestrata cultivated in the HPPWs. To receive all required EAA only from U. fenestrata, a person would need to eat around 230 g dw of the HPPW-treated seaweeds and 1510 g dw of the control seaweeds per day. These amount are very high compared to the average consumption of seaweed biomass in Japan (4.0 g dw day-1), China (5.2 g dw day-1), and South Korea (8.5 g dw day-1) (Chen et al., 2018). Nevertheless, when compared to the amount of soybean intake to reach the EAA recommendations (170 g dw, based on average soybean AA profile from Brazil, China, and the US) (Grieshop and Fahey, 2001), seaweeds should be considered an interesting future protein source. Furthermore, recent studies on Ulva show that its proteins can be concentrated by different extraction processes (Harrysson et al., 2019; Trigo et al., 2021; Juul et al., 2022), in a similar manner as is currently done with soy, which further enhances the prospects of U. fenestrata biomass as a protein source for human consumption.
Biomass yields
Land-based Ulva cultivations often use fertilizers to obtain high growth rates, as Ulva species are known to respond with increasing growth when cultivated in nutrient rich waters. Our results correspond well with previously reports on cultivation of Ulva spp. in nutrient rich side streams from the aquaculture sector (Ashkenazi et al., 2019; Nardelli et al., 2019). Extrapolating our results, using a dw:fw ratio of 20:80, indicates a production of 14 - 19 t dw ha-1 y-1 when cultivating U. fenestrata in the HPPWs. This is lower than estimates from fertilized tank cultivation of Ulva spp. (46 t dw ha-1 y-1) (Gadberry et al., 2018), but several folds higher than estimated average ocean-based Ulva spp. farming yields (4 t dw ha-1 y-1) (Savvashe et al., 2021; Nordic Seafarm, 2022) and soybean yields (< 3 t dw ha-1 y-1) (Ainsworth et al., 2012; FAO, 2021). It is important to note that this study did not focus on obtaining optimal biomass yields, and the start density used in this experiment (0.3 g L-1 fw) was very low compared to possible cultivation densities (> 12 g L-1 fw; Mata et al. (2010); Al-Hafedh et al. (2015); Gadberry et al. (2018), and personal experience). The biomass yields could therefore be optimized, further increasing the productivity of the cultivation system. However, the results need to be confirmed in an upscaled setting where a continuous supply of process water is ensured.
The decrease in fw between day 14 and 28 for U. fenestrata in the seawater control can be explained by a sporulation event that was observed during this experiment. When seaweeds sporulate, most of their energy is allocated from growing to releasing swarmers (in Ulva either zoids or gametes) (Han et al., 2003). Interestingly, we did not observe sporulation events in U. fenestrata cultivated in the HPPWs. It can only be speculated why the U. fenestrata did not sporulate in the HPPWs, as sporulation events are often triggered by changes in environmental conditions or nutrient availability (Balar and Mantri, 2019). Sporulation events can be detrimental to commercial Ulva cultivations, as large amounts of biomass are lost. Identifying potential sporulation inhibitors would further increase the value of the HPPWs as cultivation media.
Heavy metals
Seaweeds may accumulate toxic elements, and there have been concerns about the risk of elevated levels of the carcinogenic inorganic form of arsenic (iAs) in seaweed biomass (Duinker et al., 2020; Blikra et al., 2021). In this study we show that iAs constitutes 0.02 μg g dw-1 of the U. fenestrata cultivated in HPPWs, which is below the EU maximum levels (MLs) in rice and rice products (0.1 - 0.3 μg g dw-1) (European Commission, 2006; European Commission, 2022). However, it is important to note that the MLs reflect the levels, size of consumption, and toxicity in foodstuff occurring at the market. New food items on the market and/or changed consumption patterns will eventually call for new risk assessments and new MLs (EFSA, 2005). The heavy metals limiting the seaweed biomass consumption were according to US EPA reference doses mercury in the biomass cultivated in SAL and cadmium when cultivated in TUB. To reach these reference doses, as much as 90 g dw of U. fenestrata biomass cultivated in SAL and 160 g dw cultivated in TUB can be consumed each day. It should be noted that the US EPA decided against a reference value for lead in 2004, and according to the European Food Safety Authority (EFSA) the tolerable daily intake is 0.63 μg kg bw-1 day-1 (EFSA, 2010). Using the EFSA reference value results in seaweed biomass consumption being limited by lead in both HPPW-treatments. Since both US EPA (2007) and EFSA (2010) stress the toxicity of lead, the intake should be kept as low as possible. When cultivating seaweeds in tanks on land it is possible to select for the best quality of water, and make sure there are no leakage of lead from pipes, while lead levels may also be reduced using ion exchange methods as a cleaning pre-step prior to using the process water for food production (EFSA, 2012; Lalmi et al., 2018; European Commission, 2022).
It should be noted that our estimations are based on the EFSA reference body weight for adults (63.3 kg), which is low compared to the average weight in America, Australia, and Europe (above 75 kg) (worlddata.info, 2022), and that all the heavy metals are bioavailable. When compared to the average seaweed biomass intake in Japan, China, or South Korea, and the level of heavy metals in U. fenestrata cultivated in HPPWs, the potential health risk for humans can be considered low. Furthermore, processing of the biomass, such as blanching, washing, and boiling, reduces the content of some heavy metals in seaweed biomass by up to 40 - 60% (Hanaoka et al., 2001; Stévant et al., 2018; Ownsworth et al., 2019; Blikra et al., 2021), and protein extraction processes may decrease the level of heavy metals which are not bound to proteins. Together, this further increases the possible amount of biomass that can safely be consumed.
Conclusion
In conclusion, our study shows that herring production process waters (HPPWs) can effectively be used as U. fenestrata cultivation media. In 7 - 28 days, we managed to produce U. fenestrata biomass with four to six times higher biomass yields (27.17 - 37.07 g fw vs. 6.18 g fw) and almost three times higher crude protein content (31.57 - 33.24% dw vs. 12.72% dw) when cultivated in HPPWs compared to in seawater. The increased protein content also translated to significantly higher EAA content with e.g. methionine content over six times higher in HPPWs compared to in seawater (2.82 - 3.54 mg g dw-1 vs. 0.42 mg g dw-1). Furthermore, the heavy metal content in the biomass was below the maximum allowed levels in foodstuff set by the commission of the European communities. Combined, our results show a great potential for the herring processing industry and the cultivators of U. fenestrata to jointly produce sustainable proteins for the future.
Data availability statement
The original contributions presented in the study are included in the article/supplementary material. Further inquiries can be directed to the corresponding author.
Author contributions
KS, conceptualization, formal analysis, investigation, methodology, project administration, supervision, validation, visualization, writing – original draft, and review and editing. OG, conceptualization, investigation, methodology, writing – review and editing. BK and IU, funding acquisition, resources, writing – review and editing. GT, supervision, validation, writing – review and editing. HP, funding acquisition, resources, supervision, validation, writing – review and editing. All authors contributed to the article and approved the submitted version.
Funding
This study was supported by the Swedish Research Council Formas (CirkAlg-project, Grant no. 2018-01839).
Acknowledgments
The authors are grateful to Dr. Sophie Steinhagen at the University of Gothenburg for sharing her Ulva expertise, and to João P. Trigo at the Chalmers University of Technology for helping out with the protocol for characterization of the process waters and the amino acid analysis. Some data from this study were collected and published online as a part of Oscar Gustavsson’s degree project for Master of Science at the department of Marine Sciences, University of Gothenburg, Sweden (Gustavsson, 2021).
Conflict of interest
The authors declare that the research was conducted in the absence of any commercial or financial relationships that could be construed as a potential conflict of interest.
Publisher’s note
All claims expressed in this article are solely those of the authors and do not necessarily represent those of their affiliated organizations, or those of the publisher, the editors and the reviewers. Any product that may be evaluated in this article, or claim that may be made by its manufacturer, is not guaranteed or endorsed by the publisher.
Abbreviations
HPPW(s), herring production process water(s); TUB, tub water from in-house pre-processing storage of whole herring at a primary processor; SAL, salt brine from maturation of herring fillets at a secondary processor; SW, seawater control; US EPA, United States Environmental Protection Agency; EFSA, European Food Safety Authority; RfD, reference doses for heavy metals set by US EPA; MLs, maximum allowed levels of heavy metals in foodstuff set by the European Union’s Commission Regulation (EC) No. 1881/2006 (version 03/05/2022).
References
Aiking H. (2014). Protein production: Planet, profit, plus people? Am. J. Clin. Nutr. 100 (suppl_1), 483S–489S. doi: 10.3945/ajcn.113.071209
Ainsworth E. A., Yendrek C. R., Skoneczka J. A., Long S. P. (2012). Accelerating yield potential in soybean: Potential targets for biotechnological improvement. Plant Cell Environ. 35 (1), 38–52. doi: 10.1111/j.1365-3040.2011.02378.x
Al-Hafedh Y. S., Alam A., Buschmann A. H. (2015). Bioremediation potential, growth and biomass yield of the green seaweed, Ulva lactuca in an integrated marine aquaculture system at the red Sea coast of Saudi Arabia at different stocking densities and effluent flow rates. Rev. Aquaculture 7 (3), 161–171. doi: 10.1111/raq.12060
Almela C., Algora S., Benito V., Clemente M. J., Devesa V., Suner M. A., et al. (2002). Heavy metal, total arsenic, and inorganic arsenic contents of algae food products. J. Agric. Food Chem. 50 (4), 918–923. doi: 10.1021/jf0110250
Angell A. R., Mata L., de Nys R., Paul N. A. (2016). The protein content of seaweeds: a universal nitrogen-to-protein conversion factor of five. J. Appl. Phycol. 28 (1), 511–524. doi: 10.1007/s10811-015-0650-1
Arumugam N., Chelliapan S., Kamyab H., Thirugnana S., Othman N., Nasri N. S. (2018). Treatment of wastewater using seaweed: A review. Int. J. Environ. Res. Public Health 15 (12), 2851. doi: 10.3390/ijerph15122851
Ashkenazi D. Y., Israel A., Abelson A. (2019). A novel two-stage seaweed integrated multi-trophic aquaculture. Rev. Aquaculture. 11 (1), 246–262. doi: 10.1111/raq.12238
Balar N. B., Mantri V. A. (2019). Insights into life cycle patterns, spore formation, induction of reproduction, biochemical and molecular aspects of sporulation in green algal genus Ulva: Implications for commercial cultivation. J. Appl. Phycol. 32, 473–484. doi: 10.1007/s10811-019-01959-7
Blikra M. J., Wang X., Philip J., Dagbjørn S. (2021). Saccharina latissima cultivated in northern Norway: Reduction of potentially toxic elements during processing in relation to cultivation depth. Foods 10 (6), 1–18. doi: 10.3390/foods10061290
Bolton J. J., Cyrus M. D., Brand M. J., Joubert M., Macey B. M. (2016). Why grow Ulva? Its potential role in the future of aquaculture. Perspect. Phycol. 3 (3), 113–120. doi: 10.1127/pip/2016/0058
Boye J., Zare F., Pletch A. (2010). Pulse proteins: Processing, characterization, functional properties and applications in food and feed. Food Res. Int. 43 (2), 414–431. doi: 10.1016/j.foodres.2009.09.003
Buschmann A. H., Camus C., Infante J., Neori A., Israel Á., Hernández-González M. C., et al. (2017). Seaweed production: Overview of the global state of exploitation, farming and emerging research activity. Eur. J. Phycol. 52 (4), 391–406. doi: 10.1080/09670262.2017.1365175
Chen Q., Pan X.-D., Huang B.-F., Han J.-L. (2018). Distribution of metals and metalloids in dried seaweeds and health risk to population in southeastern China. Sci. Rep. 8 (1), 1–7. doi: 10.1038/s41598-018-21732-z
Duinker A., Kleppe M., Fjære E., Biancarosa I., Heldal H. E., Dahl L., et al. (2020). Knowledge update on macroalgae food and safety: Based on data generated in the period 2014-2019 by the institute of marine research, Norway (Bergen, Norway:Havsforskningsinstitutet).
EFSA (2005). Opinion of the scientific committee on a request from EFSA related to a harmonised approach for risk assessment of substances which are both genotoxic and carcinogenic. EFSA J. 282, 1–31. doi: 10.2903/j.efsa.2005.282
EFSA (2010). Panel on contaminants in the food chain (CONTAM); scientific opinion on lead in food. EFSA J. 8 (4), 1570(151). doi: 10.2903/j.efsa.2010.1570
EFSA (2012). Lead dietary exposure in the European population. EFSA J. 10 (7), 2831. doi: 10.2903/j.efsa.2012.2831
EFSA (2017). Dietary reference values for nutrients summary report. EFSA Supporting Publications 14 (12), e15121E. doi: 10.2903/sp.efsa.2017.e15121
European Commission (2006). Commission regulation (EC) no 1881/2006 of 19 December 2006 setting maximum levels for certain contaminants in foodstuffs. Off. J. Eur. Union Strasbourg, France. L364/5, 5–24. Available at: http://data.europa.eu/eli/reg/2006/1881/oj
European Commission (2018). Commision recommendation (EU) 2018/464 of 19 march 2018 on the monitoring of metals and iodine in seaweed, halophytes and products based on seaweed. Off. J. Eur. Union. L78/16, 16–18. Available at: http://data.europa.eu/eli/reco/2018/464/oj
European Commission (2022). “European Committee for food contract materials and articles (partial agreement) (CD-P_MCA),” in Technical guide on metals and alloys used in food contact materials and articles, 2nd edition (Strasbourg, France: Draft for public consultation of stakeholders).
FAO (2021) FAOSTAT statistical database. Available at: https://www.fao.org/faostat/en/#data (Accessed 5 July 2022).
Fleurence J. (2004). “Seaweed proteins ”, in Proteins in food processing, vol. 197-211 . Ed. Yada R. Y. (Cambridge, UK:Food Science, Technology and Nutrition: Woodhead Publishing Limited).
Fleurence J. (2016). “Chapter 5 - seaweeds as food,” in Seaweed in health and disease prevention. Eds. Fleurence J., Levine I. (San Diego: Academic Press), 149–167.
Forghani B., Bordes R., Strom A., Undeland I. (2020a). Recovery of a protein-rich biomass from shrimp (Pandalus borealis) boiling water: A colloidal study. Food Chem. 302, 125299. doi: 10.1016/j.foodchem.2019.125299
Forghani B., Mayers J. J., Albers E., Undeland I. (2022). Cultivation of microalgae - Chlorella sorokiniana and Auxenochlorella protothecoides - in shrimp boiling water residues. Algal Res. 65, 102753. doi: 10.1016/j.algal.2022.102753
Forghani B., Sørensen A. D. M., Johannesson J., Svendsen T. C., Undeland I. (2020b). Flocculation and flotation to recover protein-enriched biomasses from shrimp boiling and peeling process waters: A case study. ACS Sustain. Chem. Eng. 8 (26), 9660–9668. doi: 10.1021/acssuschemeng.0c01154
Gadberry B. A., Colt J., Maynard D., Boratyn D. C., Webb K., Johnson R. B., et al. (2018). Intensive land-based production of red and green macroalgae for human consumption in the pacific Northwest: An evaluation of seasonal growth, yield, nutritional composition, and contaminant levels. Algae 33 (1), 109–125. doi: 10.4490/algae.2018.33.2.21
Gephart J. A., Henriksson P. J. G., Parker R. W. R., Shepon A., Gorospe K. D., Bergman K., et al. (2021). Environmental performance of blue foods. Nature 597 (7876), 360–365. doi: 10.1038/s41586-021-03889-2
Grieshop C. M., Fahey G. C. (2001). Comparison of quality characteristics of soybeans from Brazil, China, and the united states. J. Agric. Food Chem. 49 (5), 2669–2673. doi: 10.1021/jf0014009
Gustavsson O. (2021). Cultivation of ulva fenestrata in food production process water (Gothenburg, Sweden:University of Gothenburg). Master of Science (MSc).
Hafting J. T., Craigie J. S., Stengel D. B., Loureiro R. R., Buschmann A. H., Yarish C., et al. (2015). Prospects and challenges for industrial production of seaweed bioactives. J. phycology 51 (5), 821–837. doi: 10.1111/jpy.12326
Hafting J. T., Critchley A. T., Cornish M. L., Hubley S. A., Archibald A. F. (2012). On-land cultivation of functional seaweed products for human usage. J. Appl. Phycol. 24 (3), 385–392. doi: 10.1007/s10811-011-9720-1
Hanaoka K.i., Yosida K., Tamano M., Kuroiwa T., Kaise T., Maeda S. (2001). Arsenic in the prepared edible brown alga hijiki, Hizikia fusiforme. Appl. organometallic Chem. 15 (6), 561–565. doi: 10.1002/aoc.195
Han T. J., Han Y. S., Kain J. M., Hader D. P. (2003). Thallus differentiation of photosynthesis, growth, reproduction, and UV-b sensitivity in the green alga Ulva pertusa (Chlorophyceae). J. Phycol. 39 (4), 712–721. doi: 10.1046/j.1529-8817.2003.02155.x
Harrysson H., Konasani V. R., Toth G. B., Pavia H., Albers E., Undeland I. (2019). Strategies for improving the protein yield in pH-shift processing of Ulva lactuca Linnaeus: Effects of ulvan lyases, pH-exposure time, and temperature. ACS Sustain. Chem. Eng. 7 (15), 12688–12691. doi: 10.1021/acssuschemeng.9b02781
Holdt S. L., Kraan S. (2011). Bioactive compounds in seaweed: Functional food applications and legislation. J. Appl. Phycol. 23 (3), 543–597. doi: 10.1007/s10811-010-9632-5
Juul L., Steinhagen S., Bruhn A., Jensen S. K., Undeland I., Dalsgaard T. K. (2022). Combining pressing and alkaline extraction to increase protein yield from Ulva fenestrata biomass. Food Bioproducts Processing 134, 80–85. doi: 10.1016/j.fbp.2022.05.006
Kollander B., Sand S., Almerud P., Ankarberg E. H., Concha G., Barregård L., et al. (2019). Inorganic arsenic in food products on the Swedish market and a risk-based intake assessment. Sci. Total Environ. 672, 525–535. doi: 10.1016/j.scitotenv.2019.03.289
Lalmi A., Bouhidel K.-E., Sahraoui B., Anfif C. (2018). Removal of lead from polluted waters using ion exchange resin with Ca(NO3)2 for elution. Hydrometallurgy 178, 287–293. doi: 10.1016/j.hydromet.2018.05.009
Machado M., Machado S., Pimentel F. B., Freitas V., Alves R. C., Oliveira M. (2020). Amino acid profile and protein quality assessment of macroalgae produced in an integrated multi-trophic aquaculture system. Foods 9 (10), 1382. doi: 10.3390/foods9101382
Mæhre H. K., Malde M. K., Eilertsen K. E., Elvevoll E. O. (2014). Characterization of protein, lipid and mineral contents in common Norwegian seaweeds and evaluation of their potential as food and feed. J. Sci. Food Agric. 94 (15), 3281–3290. doi: 10.1002/jsfa.6681
Mata L., Schuenhoff A., Santos R. (2010). A direct comparison of the performance of the seaweed biofilters, Asparagopsis armata and Ulva rigida. J. Appl. Phycol. 22 (5), 639–644. doi: 10.1007/s10811-010-9504-z
Nardelli A. E., Chiozzini V. G., Braga E. S., Chow F. (2019). Integrated multi-trophic farming system between the green seaweed Ulva lactuca, mussel, and fish: A production and bioremediation solution. J. Appl. Phycol. 31 (2), 847–856. doi: 10.1007/s10811-018-1581-4
Neveux N., Magnusson M., Mata L., Whelan A., de Nys R., Paul N. A. (2016). The treatment of municipal wastewater by the macroalga Oedogonium sp and its potential for the production of biocrude. Algal Research-Biomass Biofuels Bioproducts 13, 284–292. doi: 10.1016/j.algal.2015.12.010
Nordic Seafarm (2022) Ulva farm, an EU-backed project dedicated to scaling up the green seaweed sea lettuce (Ulva). Available at: https://www.nordicseafarm.com/ulva-farm (Accessed 15 August 2022).
Osman A., Gringer N., Svendsen T., Yuan L. F., Hosseini S. V., Baron C. P., et al. (2015). Quantification of biomolecules in herring (Clupea harengus) industry processing waters and their recovery using electroflocculation and ultrafiltration. Food Bioproducts Process. 96, 198–210. doi: 10.1016/j.fbp.2015.08.002
Ownsworth E., Selby D., Ottley C. J., Unsworth E., Raab A., Feldmann J., et al. (2019). Tracing the natural and anthropogenic influence on the trace elemental chemistry of estuarine macroalgae and the implications for human consumption. Sci. Total Environ. 685, 259–272. doi: 10.1016/j.scitotenv.2019.05.263
Provasoli L. (1968). “Media and prospects for the cultivation of marine algae,” in Cultures and collections of algae. proceedings of US-Japan conference, hakone, September 1966 (Tokyo, Japan:Japan Society of Plant Physiology).
Qvirist L., Carlsson N.-G., Andlid T. (2015). Assessing phytase activity–methods, definitions and pitfalls. J. Biol. Methods 2 (1), e16. doi: 10.14440/jbm.2015.58
Redmond S., Lindsay G., Yarish C., Kim J., Neefus C. (2014). New England seaweed culture handbook (Groton, US:University of Connecticut).
Savvashe P., Mhatre-Naik A., Pillai G., Palkar J., Sathe M., Pandit R., et al. (2021). High yield cultivation of marine macroalga ulva lactuca in a multi-tubular airlift photobioreactor: A scalable model for quality feedstock. J. Cleaner Production 329, 129746. doi: 10.1016/j.jclepro.2021.129746
Stedt K., Pavia H., Toth G. B. (2022a). Cultivation in wastewater increases growth and nitrogen content of seaweeds: A meta-analysis. Algal Res. 61, 102573. doi: 10.1016/j.algal.2021.102573
Stedt K., Trigo J. P., Steinhagen S., Nylund G. M., Forghani B., Pavia H., et al. (2022b). Cultivation of seaweeds in food production process waters: Evaluation of growth and crude protein content. Algal Res. 63, 102647. doi: 10.1016/j.algal.2022.102647
Steinhagen S., Enge S., Cervin G., Larsson K., Edlund U., Schmidt A. E. M., et al. (2022). Harvest time can affect the optimal yield and quality of sea lettuce (Ulva fenestrata) in a sustainable sea-based cultivation. Front. Mar. Sci. 9. doi: 10.3389/fmars.2022.816890
Steinhagen S., Enge S., Larsson K., Olsson J., Nylund G. M., Albers E., et al. (2021). Sustainable large-scale aquaculture of the northern hemisphere sea lettuce, Ulva fenestrata, in an off-shore seafarm. J. Mar. Sci. Eng. 9 (6), 615. doi: 10.3390/jmse9060615
Stévant P., Marfaing H., Duinker A., Fleurence J., Rustad T., Sandbakken I., et al. (2018). Biomass soaking treatments to reduce potentially undesirable compounds in the edible seaweeds sugar kelp (Saccharina latissima) and winged kelp (Alaria esculenta) and health risk estimation for human consumption. J. Appl. Phycol. 30 (3), 2047–2060. doi: 10.1007/s10811-017-1343-8
Thakur M., Hurburgh C. R. (2007). Quality of US soybean meal compared to the quality of soybean meal from other origins. J. Am. Oil Chemists’ Soc. 84 (9), 835–843. doi: 10.1007/s11746-007-1107-8
Thiviya P., Gamage A., Gama-Arachchige N. S., Merah O., Madhujith T. (2022). Seaweeds as a source of functional proteins. Phycology 2 (2), 216–243. doi: 10.3390/phycology2020012
Titlyanov E. A., Titlyanova T. V. (2010). Seaweed cultivation: Methods and problems. Russian J. Mar. Biol. 36 (4), 227–242. doi: 10.1134/S1063074010040012
Toth G. B., Harrysson H., Wahlstrom N., Olsson J., Oerbekke A., Steinhagen S., et al. (2020). Effects of irradiance, temperature, nutrients, and pCO(2) on the growth and biochemical composition of cultivated Ulva fenestrata. J. Appl. Phycol. 32, 3243–3254. doi: 10.1007/s10811-020-02155-8
Trigo J. P., Engström N., Steinhagen S., Juul L., Harrysson H., Toth G. B., et al. (2021). In vitro digestibility and caco-2 cell bioavailability of sea lettuce (Ulva fenestrata) proteins extracted using pH-shift processing. Food Chem. 356, 129683. doi: 10.1016/j.foodchem.2021.129683
US EPA (2007). Concepts, methods, and data sources for cumulative health risk assessment of multiple chemicals, exposures and effects: A resource document (Washingtong, DC: US Environmental Protection Agency).
WHO/FAO/UNU (2007). Protein and amino acid requirements in human nutrition”, in: WHO technical report series 935. Rep. Joint WHO/FAO/UNU Expert Consultation 935, 1–265. Available at: https://apps.who.int/iris/handle/10665/43411
worlddata.info (2022) Average sizes of men and women. Available at: https://www.worlddata.info/average-bodyheight.php (Accessed 5 July 2022).
Keywords: arsenic, wastewater, proteins, aquaculture, seaweed, macroalgae, process water, food production
Citation: Stedt K, Gustavsson O, Kollander B, Undeland I, Toth GB and Pavia H (2022) Cultivation of Ulva fenestrata using herring production process waters increases biomass yield and protein content. Front. Mar. Sci. 9:988523. doi: 10.3389/fmars.2022.988523
Received: 07 July 2022; Accepted: 23 August 2022;
Published: 13 September 2022.
Edited by:
Morten Omholt Alver, Norwegian University of Science and Technology, NorwayReviewed by:
Leonel Pereira, University of Coimbra, PortugalAlejandro H. Buschmann, University of Los Lagos, Chile
Copyright © 2022 Stedt, Gustavsson, Kollander, Undeland, Toth and Pavia. This is an open-access article distributed under the terms of the Creative Commons Attribution License (CC BY). The use, distribution or reproduction in other forums is permitted, provided the original author(s) and the copyright owner(s) are credited and that the original publication in this journal is cited, in accordance with accepted academic practice. No use, distribution or reproduction is permitted which does not comply with these terms.
*Correspondence: Kristoffer Stedt, kristoffer.stedt@gu.se