- 1Vantuna Research Group, Department of Biology, Occidental College, Los Angeles, CA, United States
- 2Department of Biological Sciences, California State Polytechnic University, Pomona, CA, United States
Life history parameters for fishes have generally been applied to species across their entire range, however, different ecological and environmental conditions and processes (e.g., sea surface temperature, habitat, primary productivity, fishing mortality, resource availability) influence life history patterns at smaller spatial scales. By focusing on a historically protected species, we determined how environmental and ecological factors shape patterns in growth and longevity, without the impact of fishing. The Garibaldi (Hypsypops rubicundus) is a territorial marine damselfish native to the shallow rocky reefs of southern California. Garibaldi were collected from five mainland locations and five Channel Islands throughout the Southern California Bight. Paired natural reef and artificial reef habitats (i.e., breakwaters and jetties) in each mainland location were sampled. Otolith-based ageing and biological data from these populations were used to assess how age and growth vary by location, reef type (natural/artificial), island or mainland, mean annual sea surface temperature, and/or sex. The annual formation of growth increments in otoliths was validated in-situ using tetracycline mark-recapture methods to confirm that increments are formed annually. Garibaldi grew significantly larger on artificial reefs than on natural reefs but tended to live longer on paired natural reefs. Regionally, growth and longevity followed mean annual sea surface temperature gradients, consistent with Bergmann’s rule. Garibaldi exhibited clear sexual size dimorphism; males grew larger, which is uncharacteristic for both damselfish and other marine fishes from the southern California. The Garibaldi collected for this study had a maximum age of 57 years, which makes this the longest lived damselfish species by two decades.
Introduction
Marine fish life history strategies (i.e., the allocation of limited resources to the competing demands of growth, reproduction, and survival) and demographic parameters can provide insight into population and community-level processes and ecological patterns. They are typically assumed to be consistent for a species across its entire range (King and McFarlane, 2003; Fonseca and Cabral, 2007). However, several recent studies concluded that different ecological and environmental conditions result in intraspecific spatial variation in fish life history patterns. This variation has been attributed to multiple factors including: differences in mean annual, inter-annual, or range in sea surface temperature (Trip et al., 2014; Trip et al., 2016; Tanner et al., 2019); habitat (DeMartini et al., 1989; DeMartini et al., 1994; Love et al., 2007; Granneman and Steele, 2014); primary productivity (Ruttenberg et al., 2005; Tanner et al., 2019); fishing mortality (Robertson et al., 2005; Hamilton et al., 2007; Hsieh et al., 2009; Hamilton et al., 2011; Taylor, 2013; Taylor et al., 2015); differing environmental conditions during larval/juvenile growth (Atkinson, 1994; Atkinson, 1996; Fonseca and Cabral, 2007; Williams et al., 2007); food resource availability (Hjelm et al., 2000); and density-dependent interactions (Figueira et al., 2007). Understanding the complexities of species-specific life histories for marine fishes is essential for informing ecosystem-based fisheries management (Jennings and Dulvy, 2008).
The Southern California Bight (SCB) spans 3.5° of latitude of the ocean from Point Conception, CA in the north to the United States-Mexico border in the south. The SCB encompasses 1,198 km of coastline that includes the mainland and an eight-island archipelago, known as the California Channel Islands (Pondella et al., 2015b). Roughly 26% of the nearshore (<30 m depth) habitat is composed of rocky reefs with the remainder dominated by soft substrata, exhibiting fragmented distributions of rocky reef habitat. The SCB has a unique surface current circulation pattern where the year-round, dominant California Current brings cool water to the region from the north. South of Point Conception, the flow of the California Current is disrupted by the Channel Islands and branches shoreward and poleward where it forms the Southern California Countercurrent, drawing warmer water from the south and creating a large eddy that circulates counterclockwise throughout the Bight (Hickey, 1992). This current pattern, when coupled with the complex bathymetry, results in strong abiotic gradients such as sea surface temperature (Figure 1) and diverse habitats that support various assemblages of fish fauna. This provides a prime opportunity to study how variability in environment and habitat type shape life history patterns (Engle, 1993; Allen and Pondella, 2006; Pondella et al., 2015a; Pondella et al., 2015b). Prior studies of spatial differences in growth patterns and demographic parameters for fishes in the SCB have concentrated on commercially and recreationally exploited species (Love et al., 1990; Love et al., 2007; Granneman, 2011; Hamilton et al., 2011; Granneman and Steele, 2014). Size-selective fishing mortality on larger, older members of a population can influence fish life history patterns including rapid growth to asymptotic size, early maturity, reduced reproductive output, skewed sex ratios, and age- and size-truncation of exploited populations, which can have evolutionary consequences (Robertson et al., 2005; Hamilton et al., 2007; Hsieh et al., 2009; Caselle et al., 2011; Hamilton et al., 2011; Taylor, 2013; Audzijonyte et al., 2016).
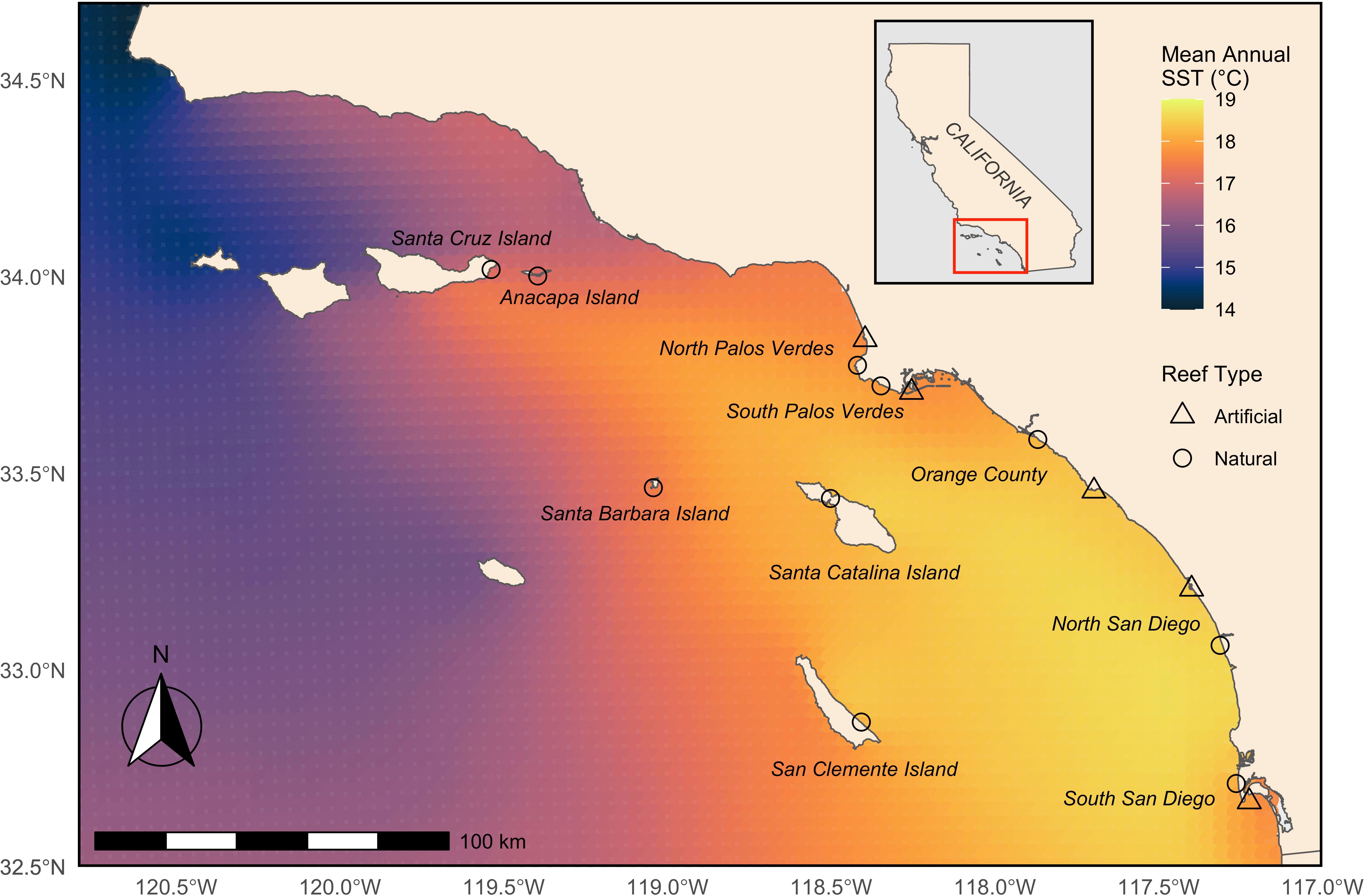
Figure 1 Map of sampled Garibaldi (Hypsypops rubicundus) populations (locations) in the Southern California Bight. Reef type is indicated by shape – triangles for artificial rocky reefs (i.e., breakwaters and jetties) and circles for natural rocky reefs. Mean annual sea surface temperature (SST;°C) values for January 2002-December 2018 are from merged 1 km resolution data.
In addition to natural rocky reefs, artificial or anthropogenic structures also provide habitat for reef-associated fishes in the SCB. Over the last 50 years, the population of southern California has nearly doubled and is one of the most densely populated areas in the United States, currently supporting roughly 24 million people. In addition, nearly 25% of the entire nation’s coastal population lives in the four counties bordering the shoreline of the SCB (Schiff et al., 2000; Wilson and Fischetti, 2010). The artificial structures in the SCB were primarily built as coastal infrastructure (e.g., breakwaters and jetties, oil and gas platforms, and outfall and discharge pipes) to support this urbanization. In addition, rapid population growth has put stress on marine resources through the discharge of pollutants, loss of habitat, and overfishing. In 1958, California Department of Fish and Game (currently California Department of Fish and Wildlife) began the Nearshore Sportfish Habitat Enhancement Program, which focused on restoring and enhancing fish habitat through the implementation of purpose-built artificial reefs in southern California (Lewis and McKee, 1989). The function of artificial structures, both infrastructure and purpose-built, as fish habitat is well-studied within the Bight (Ambrose and Swarbrick, 1989; DeMartini et al., 1989; DeMartini et al., 1994; Pondella and Stephens, 1994; Stephens et al., 1994; Pondella et al., 2002; Stephens and Pondella, 2002; Claisse et al., 2014; Granneman and Steele, 2015; Claisse et al., 2019; Love et al., 2019; Barilotti et al., 2020; Burns et al., 2020), however relatively few studies have compared the ecological performance of fish on artificial reefs to natural reefs (Love et al., 2007; Granneman and Steele, 2014).
The Garibaldi (Hypsypops rubicundus) is a territorial marine damselfish endemic to the shallow reefs of southern California and Baja California, Mexico. Out of 385 species of damselfish (Family: Pomacentridae), Garibaldi is the largest, reaching up to 35 cm in length (Limbaugh, 1964; Clarke, 1970; Allen, 1991; Nelson et al., 2016). Their bright orange adult coloration, with spots of iridescent blue as juveniles, makes them the most conspicuous reef fish in southern California (Kritzler et al., 1950). Once an intense target of collection for the aquarium trade, as the State Marine Fish of California, this species has been fully protected in the United States since 1995. Although predation on young-of-the-year Garibaldi by Kelp Bass (Paralabrax clathratus) (Cook, 2011), adult Garibaldi have very few known natural predators (Kritzler et al., 1950; Clarke, 1970) and are currently unfished. Unlike other damselfish where males guard nest sites only during the mating season, both male and female adult Garibaldi typically defend year-round territories of approximately 3-10 m2, demonstrating high site fidelity (Limbaugh, 1964; Clarke, 1970; Foster, 1972; Sikkel, 1988; Sikkel, 1989; Alcalay and Sikkel, 1994). A territory includes a shelter hole, foraging area, and for adult males, a nest site comprised of perennial patches of red algae cultivated by the male. Females are allowed into the territory to deposit eggs into the nest during spawning season (April through September), the timing of which varies within and among populations (Clarke, 1970; Clarke, 1971; Sikkel, 1988). The eggs are guarded by the male until young hatch, and disperse into the plankton (Clarke, 1970). In early summer to early fall, juveniles settle on shallow rocky reefs on exposed or semi-protected coasts (Limbaugh, 1964; Clarke, 1970; Alcalay and Sikkel, 1994). Vagility is only through the larval stage making this taxon optimal for describing site-specific growth characteristics. Previous life history studies on Garibaldi are limited primarily to the San Diego, CA area and/or prior to 1995, when their protected status was implemented. Clarke (1970) reported that Garibaldi achieve adult coloration at around 5 years, sexual maturity at 6 years, and a maximum age of 17 years. However, Clarke’s study used scale-based ageing, which can drastically underestimate the ages of fish (Francis and Francis, 1992; Beamish and McFarlane, 1995; Campana, 2001), and was conducted prior to the implementation of protected status. During the period from when that study was conducted in 1965 to 2010, Garibaldi densities more than doubled at Clarke’s study sites (Cook, 2011), indicating that the status of Garibaldi populations have changed considerably over time. By focusing on a historically protected species with high site fidelity, like the Garibaldi, we can determine how habitat type, environmental, and ecological factors shape patterns in growth and longevity at distinct locations within the SCB.
For this study, otolith-based ageing was used to assess how life history patterns vary by reef type (natural/artificial), mean annual sea surface temperature, sex, island or mainland geographies, and/or location for Garibaldi populations throughout the SCB. The periodicity of increment formation in otoliths was assessed using in-situ tetracycline mark-recapture methods to confirm that increments form annually rather than conforming to some other periodicity (e.g., seasonality, lunar cycles) (Campana, 2001). Accounting for patterns in variation of fish life history observed in previous studies, the following predictions were made:
1. Garibaldi from artificial reef habitats will grow larger and faster than Garibaldi from natural reef habitats. Within the SCB, juvenile (young-of-the-year) Blue Rockfish (Sebastes mystinus) were found to have faster daily growth rates at oil platforms compared to nearby natural reefs (Love et al., 2007). Garibaldi were collected from breakwaters because they were consistent in habitat structure and presence in each mainland location. Breakwaters and jetties are built to protect coastal infrastructure and are made up of large (1-2 m) quarry rock boulders, providing a complex and ideal fish habitat with several open spaces to provide refuge and for Garibaldi to maintain territories (Stephens et al., 1994).
2. Garibaldi will be larger, slower-growing, and longer-lived at cooler locations, and smaller, faster-growing, and shorter-lived at warmer locations. Ectotherms tend to be larger and live longer in colder environments due to a trade-off between metabolism and longevity (Atkinson and Sibly, 1997; Mangel and Abrahams, 2001). In addition, fish populations in colder waters often have shorter reproductive seasons and therefore may allocate less of their annual energy budgets to reproduction, which results in larger and older fish in these populations (Ruttenberg et al., 2005).
3. Male Garibaldi will grow larger than female Garibaldi. Although previous Garibaldi life history studies found no apparent differences between growth rates for males and females, they did find that males lived longer and grew larger (Clarke, 1970). In addition, Sikkel (1988; 1989) determined that male size was positively correlated with female nest preference and posited that male size may be correlated with nest quality. Further, large males may be more successful in the defense of their nesting sites.
Methods
Study area
Garibaldi were collected from ten locations in the SCB (Figure 1). Locations were selected based on two criteria: they supported at minimum 100 Garibaldi per hectare (Pondella et al., 2015a); and were ecologically and environmentally distinct and geographically distant (Engle, 1993; Pondella et al., 2015b). Five locations were in the California Channel Islands of Santa Cruz Island, Anacapa Island, Santa Barbara Island, Santa Catalina Island, and San Clemente Island and the other five locations were on the mainland coast in Northern and Southern Palos Verdes, Orange County, and Northern and Southern San Diego. For locations on the mainland, paired natural rocky reefs and artificial or human-built rocky reefs (e.g., breakwaters and jetties at harbors nearby or adjacent to natural reef sites) were sampled. This investigated the influence of differences in habitat type under similar environmental conditions.
Fish collections
From 2013-2019, 1,111 Garibaldi were collected from the ten study locations (Figure 1) by scientific divers using pole spears. Divers attempted to collect a representative size sample of the fish present at each location. Upon collection, fish were transported to the laboratory where they were weighed to the nearest gram and measured head (HL), standard (SL), fork (FL), and total lengths (TL) to the nearest millimeter. The digestive tract and gonads were removed, weighed, and preserved for future studies. Sex was determined macroscopically, if possible. Macroscopic sex determination was difficult outside of the spawning season, especially in smaller male individuals, therefore sex was determined through histological preparations when necessary. Sagittal otoliths were extracted and stored dry. This study was reviewed and approved by the Institutional Care and Use Committees at both Cal Poly Pomona and Occidental College, and fish were collected under California Department of Fish and Wildlife Scientific Collecting Permits.
Otolith processing and age determination
Sagittal otoliths were used for ageing. Annual periodicity of sagittal otolith increment formation was validated using standard methods (Cappo et al., 2000) including in-situ tetracycline mark-recapture at Flat Rock in North Palos Verdes (Section 1 in Supplementary Material). Each whole sagittal otolith was weighed to the nearest 0.0001 g. One otolith from each fish was mounted on a glass slide using thermoplastic resin and sectioned using a 1200 grit diamond flat lap on a grinding wheel following Taylor and McIlwain (2010) to produce a thin transverse section. Next, otoliths were covered in resin and heated on a hotplate to 260 °C, to improve readability. This increased the contrast between alternating translucent and opaque annual zones producing results similar to the “break and burn method” (Forsberg, 2001). When the transverse sections were viewed under a stereomicroscope with reflected light, each displays a central, dense, opaque region (pre-settlement) and alternating translucent and opaque zones (annuli) (Figure S2 in Supplementary Material). Annuli were counted along a path from the center towards the tip of the inner face next to the sulcus, where annual growth increments are clearly defined (García-Mederos et al., 2016). Readings were done by a single reader (C. M. Williams) a minimum of two times. The final age (in years) of an individual was determined when two or more counts agree (Choat and Axe, 1996; Claisse et al., 2009). If at least two counts did not agree after three readings, the otolith was excluded from age-based analyses (1.1% of otoliths).
Age and growth analyses
All age and growth analyses were performed in R (R Core Development Team, 2021) and are reported in relation to fish total length (TL; mm). Growth patterns were evaluated by fitting the von Bertalanffy growth function (VBGF) using maximum likelihood using the ‘mle2’ function in the bbmle package (Bolker and R Core Development Team, 2020), which models the mean TL (Lt, in mm) at age (t, in years) following:
where L∞ is mean asymptotic length, k describes the rate at which Lt reaches L∞ (with units of yr–1), and t0 is the theoretical age at 0 mm in length. Since fish often demonstrate rapid initial growth, it is important to include small, young fish to obtain accurate estimations of mean size-at-age for early life stages when modeling growth (Kritzer et al., 2001; Berumen, 2005; Claisse, 2009). Therefore, for immature individuals where sex was unidentifiable via macroscopic identification and histological preparation, sex was assigned randomly following a 1:1 sex ratio. Additionally, because there were few individuals of age 0-3 within each distinct grouping, t0 was fixed to a value of -2.1 years which was determined by fitting the von Bertalanffy growth function to the global population.
The longevity of fishes is typically assessed using three methods: (1) the maximum age, or oldest individual in the population (Tmax) (Beverton and Holt, 1959), (2) as the mean age of the oldest 25% of fish collected (Tmax 25%) (Choat and Robertson, 2002), and (3) as the mean age of the oldest 10% of fish collected (Tmax 10%) (Trip et al., 2008). All three metrics were calculated for males and females for each location and reef type, and for the global population.
Environmental data
Measures of environmental variables were derived from remotely sensed data. Mean annual sea surface temperature (SST; °C) values were derived from merged MODIS 1 km resolution data from MODIS-Aqua and MODIS-Terra sensors composited over each month from January 2002- December 2018. This period was used to best represent the average conditions that fish experienced during their lifetime, with the data available from MODIS, which spans 2002-present. Surface chlorophyll-a (Chl-a; g C m-3) values for each location were derived using satellite data from MODIS-Aqua, MODIS-Terra, VIIRS-NPP, SeaWiFS, MERIS and OLCI sensors composited over each month during the same time period. Data were merged using simple pixel-wise averaging of valid pixel values to increase coverage and composited by the California Current Ecosystem Long-Term Ecological Research program based at Scripps Institution of Oceanography. Full-resolution 1 km data sets were downloaded in HDF5 format, clipped to the study area then converted to a data frame table format for plotting specific points using R (R Core Development Team, 2021). Values for mean annual SST and Chl-a were calculated by averaging the annual means. Because nearshore values for both Chl-a and SST often contain erroneous values due to some cells containing a portion of land, these data were then imported into ArcGIS, the cells that overlapped with land were deleted, and then the layers were converted to a 4 km raster using the Point to Raster tool (ESRI ArcMap 10.8.1 Spatial Analyst) and assigned a value using the average values of all the points within the cell. Cell values for SST and Chl-a for each study location were then extracted using the Extract Values to Points tool.
Age and growth model selection and confidence interval estimation
The level of evidence for effects of sex, reef type, island/mainland, and location on growth patterns was investigated using model selection. We used the corrected Akaike Information Criterion (AICc), which adds a correction for bias due to small sample size, effectively penalizing complex models with small data sets (Burnham and Anderson, 2002; Anderson, 2008). Akaike weights (wi) were calculated to assess the relative likelihood of each model in the set and were interpreted as the weight of evidence in favor of the model. Parameter estimates are reported for the highest ranked model, as indicated by wi, and 95% confidence intervals were produced using random bootstrap resampling with replacement (5,000 bootstraps) using the ‘bootstraps’ function in the rsample package (Silge et al., 2021) and mapped to the grouped data using the ‘map’ function in the purrr package (Henry and Wickham, 2020). Sampling with replacement assumes that the variation in the sampled group is representative of the population as a whole, and any additional fish collected would fall within the bounds of the sampled group (Kritzer et al., 2001). For models fit to data subsets with small sample sizes where bootstrap confidence intervals produced unrealistic values, the 95% confidence intervals from the likelihood profile are reported.
In total, 1,027 fish were used in the final data set for model selection. The sampled population from Anacapa Island was small (n = 55) so it was grouped with the sampled population from Santa Cruz Island (n = 101) for geographic analyses. Anacapa Island and Santa Cruz Island sites were close to each other and had similar environmental conditions and habitat types (Table 1 and Figure 1). Surface Chl-a was strongly correlated with the island/mainland categorization, likely due to localized upwelling events common at island sites (Kilpatrick et al., 2018) and terrestrial runoff at mainland sites (Schiff et al., 2000), so only island/mainland was used as a variable with the understanding that mainland sites often have significantly higher primary productivity than island sites.
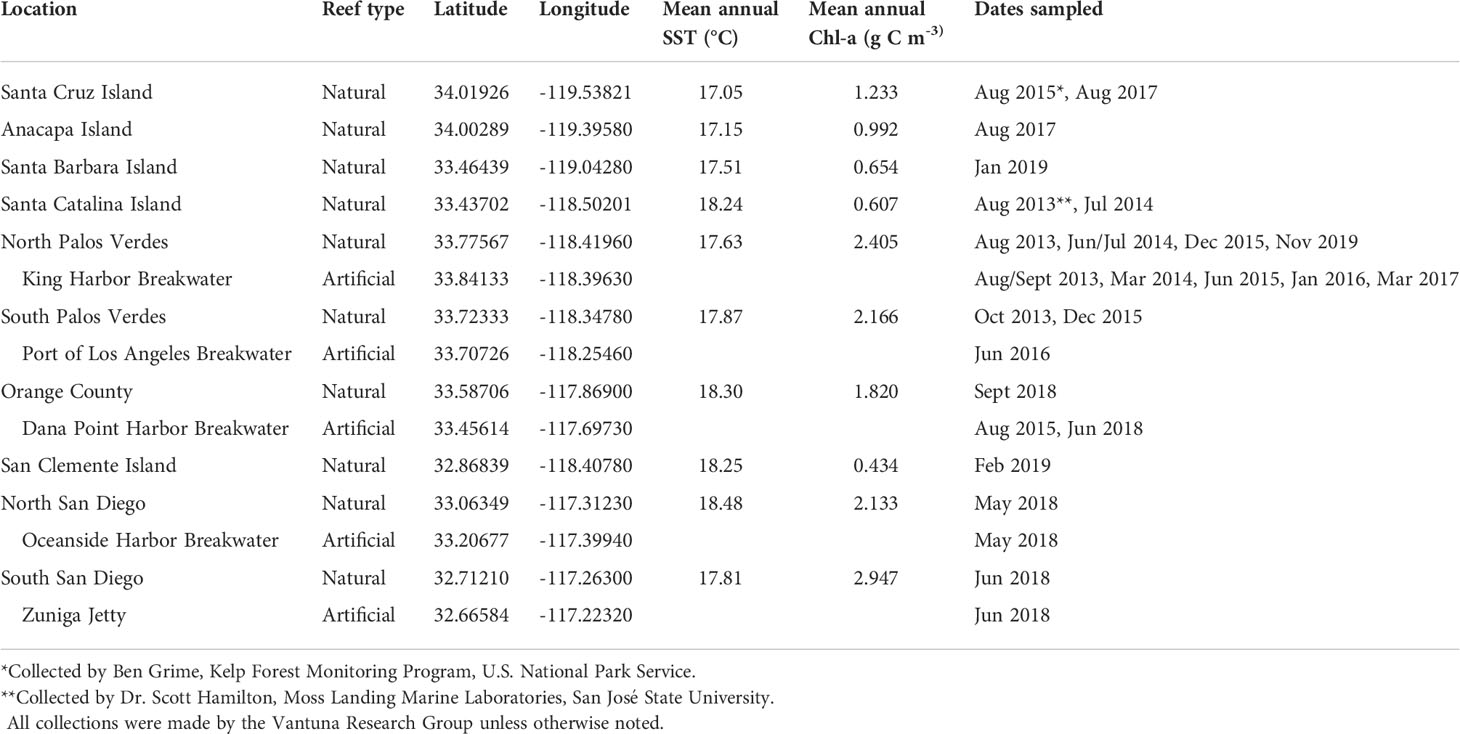
Table 1 Garibaldi (Hypsypops rubicundus) collection locations, environmental and geographic information including mean annual sea surface temperature (SST; °C) and mean annual Chlorophyll-a (Chl-a, g C m-3) from January 2002- December 2018.
Results
There is strong evidence that Garibaldi age and growth patterns vary by sex, reef type, mean annual sea surface temperature, and location within the SCB. The highest ranked model, where model parameters were fitted separately for each sex at each reef type within each location (Table 2; Figure S5 in Supplementary Material), was also the most complex model and received 100% of total Akaike weight (wi) (Table S4 in Supplementary Material).
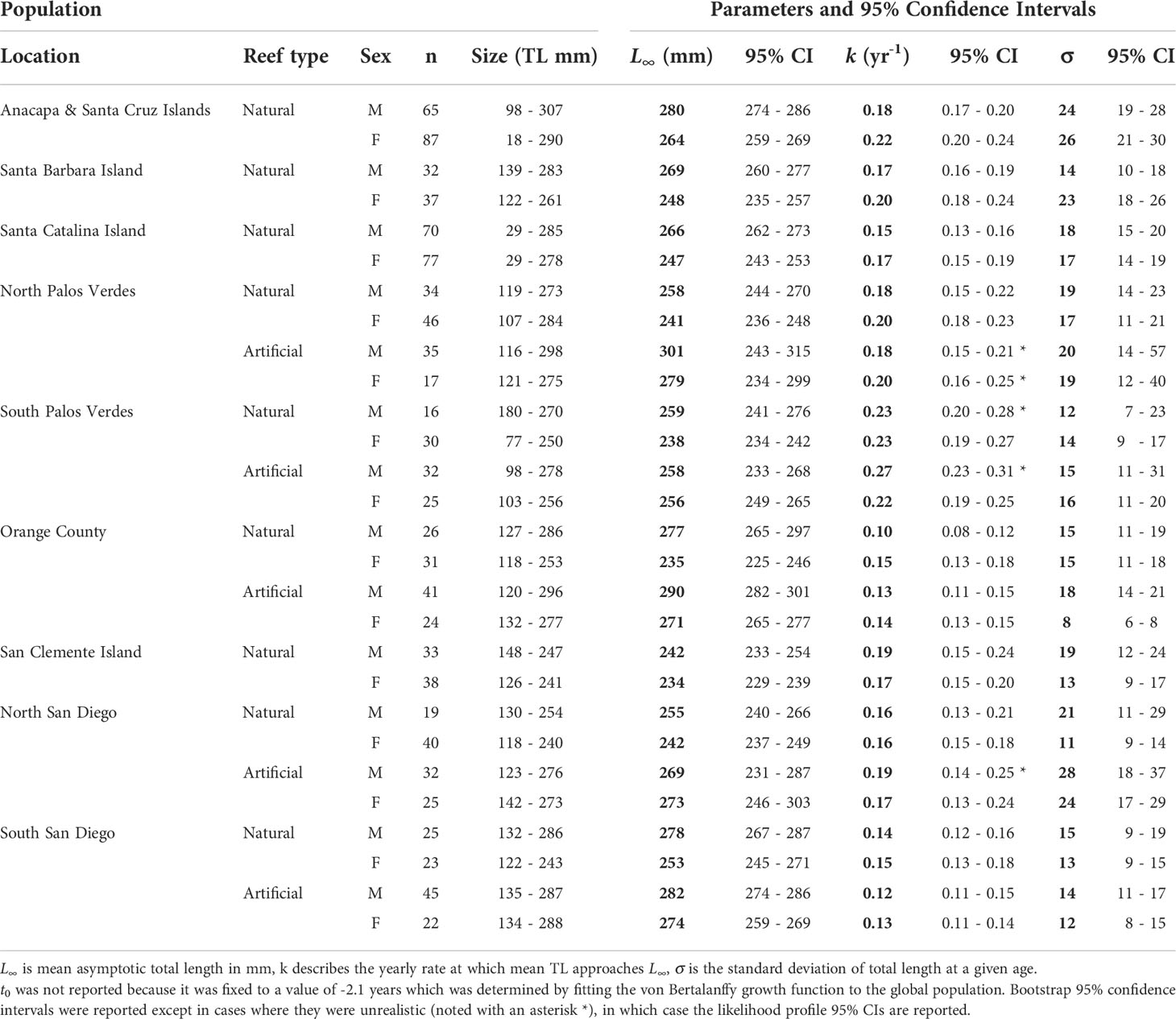
Table 2 Garibaldi (Hypsypops rubicundus) von Bertalanffy growth function parameters (bold) for the highest ranked (and most complex) model with bootstrapped 95% confidence intervals.
Garibaldi demonstrated clear sexual dimorphism in body length with males exhibiting greater mean asymptotic total length (L∞) than females at each location for each reef type, with only one exception, the artificial reef sampled in North San Diego, Oceanside Harbor Breakwater (Table 2; Figures 2A, S5 in Supplementary Material). In most instances, male-female pairwise L∞95% confidence intervals did not overlap (Table 2). When data from all reef types and locations were pooled, mean asymptotic length (L∞) was significantly higher for males (271 mm, 95% CI: 267 to 274 mm) than females (247 mm, 95% CI: 243 to 250 mm), with adult males being 24 mm larger than females on average (Table S5; Figure S8 in Supplementary Material). The greatest difference in L∞ between sexes was observed at the natural reef site in Orange County, where L∞ for males was 42 mm larger than for females. In contrast, the L∞ values between sexes were most similar at the artificial reef in South Palos Verdes, the Port of Los Angeles Breakwater, which differed by only 2 mm (Table 2).
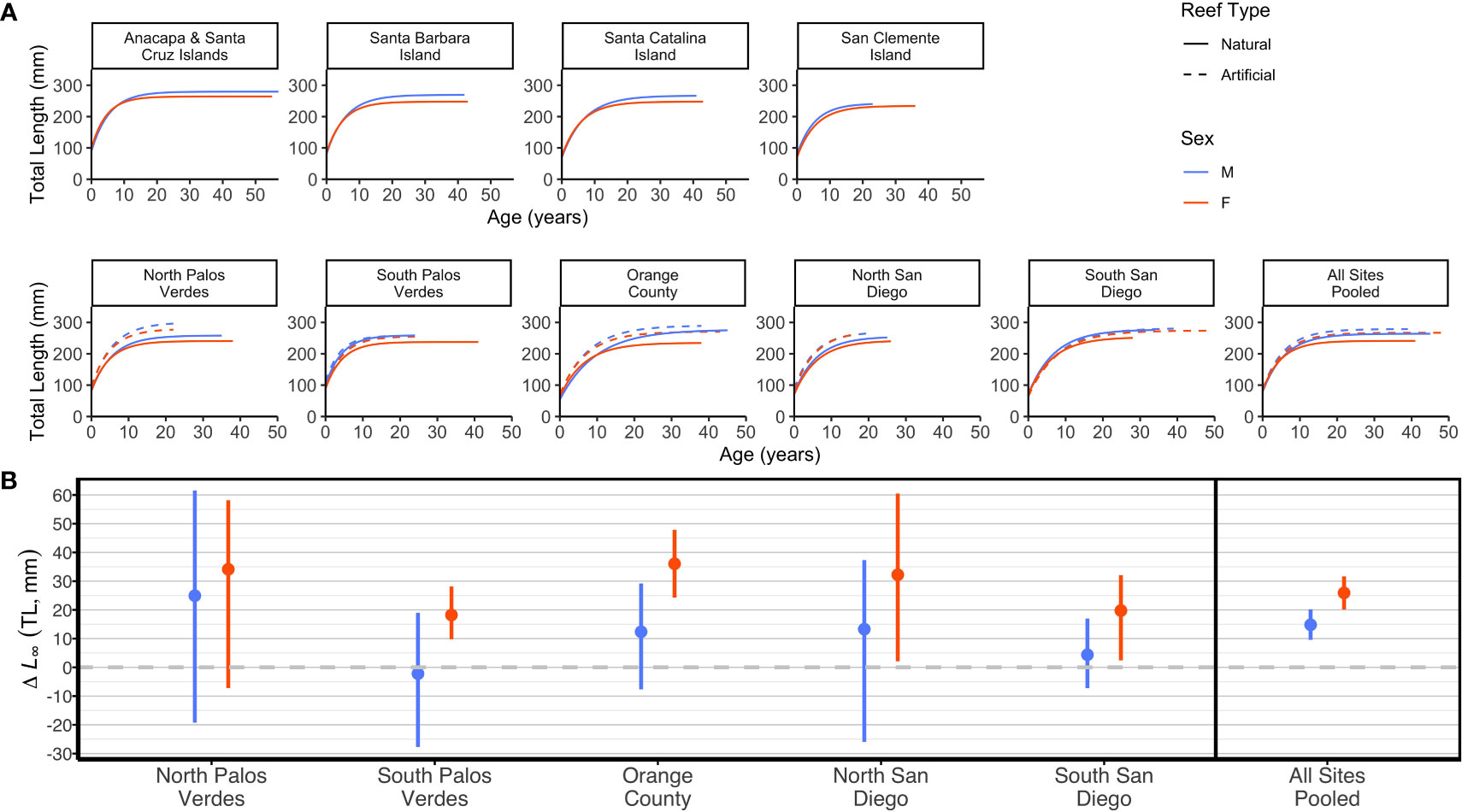
Figure 2 (A) Von Bertalanffy growth curves for Garibaldi (Hypsypops rubicundus) by sex (color) and reef type (dashed/solid line) for each location (Channel Islands on top and mainland locations below), and all mainland locations pooled fitted using maximum likelihood estimation. (B) Mean pairwise difference in L∞ values by sex (color) between artificial and natural rocky paired sites, and for all mainland sites pooled with bootstrapped 95% confidence intervals. The dashed line at Δ L∞ = 0 represents no difference between the paired artificial and natural reef sites, with a positive difference indicating adult Garibaldi were larger on the artificial reefs.
Garibaldi grew significantly larger on artificial reefs than on paired natural reefs across the mainland locations, with females being 26 mm larger (95% CI: 20 to 32 mm) and males 15 mm larger (95% CI: 10 to 20 mm) on average, when data from all sites were pooled (pairwise differences in L∞; Figure 2B). For the smaller location-specific samples, there were significant pairwise differences (95% CIs did not include 0) for females at all but one location, (mean pairwise difference range: 18 to 36 mm), and males exhibited positive, albeit smaller and not significant pairwise differences at all but one mainland location (mean pairwise difference range: -2 to 24 mm). Female L∞values from all but one of the artificial reefs were also higher than any natural reef populations including fish from Anacapa and Santa Cruz Islands, which was the northernmost and coolest location sampled (Figure 3). Additionally, the L∞ of females from the South Palos Verdes artificial reef (i.e., the Los Angeles Harbor breakwater) was still higher than those from almost all natural reefs. In general, males exhibited a similar, but less distinct pattern, interrupted by males from the artificial reef in South Palos Verdes exhibiting relatively low L∞ and males from natural reef in Orange County and South San Diego exhibiting relatively high L∞ values (Figure 3).
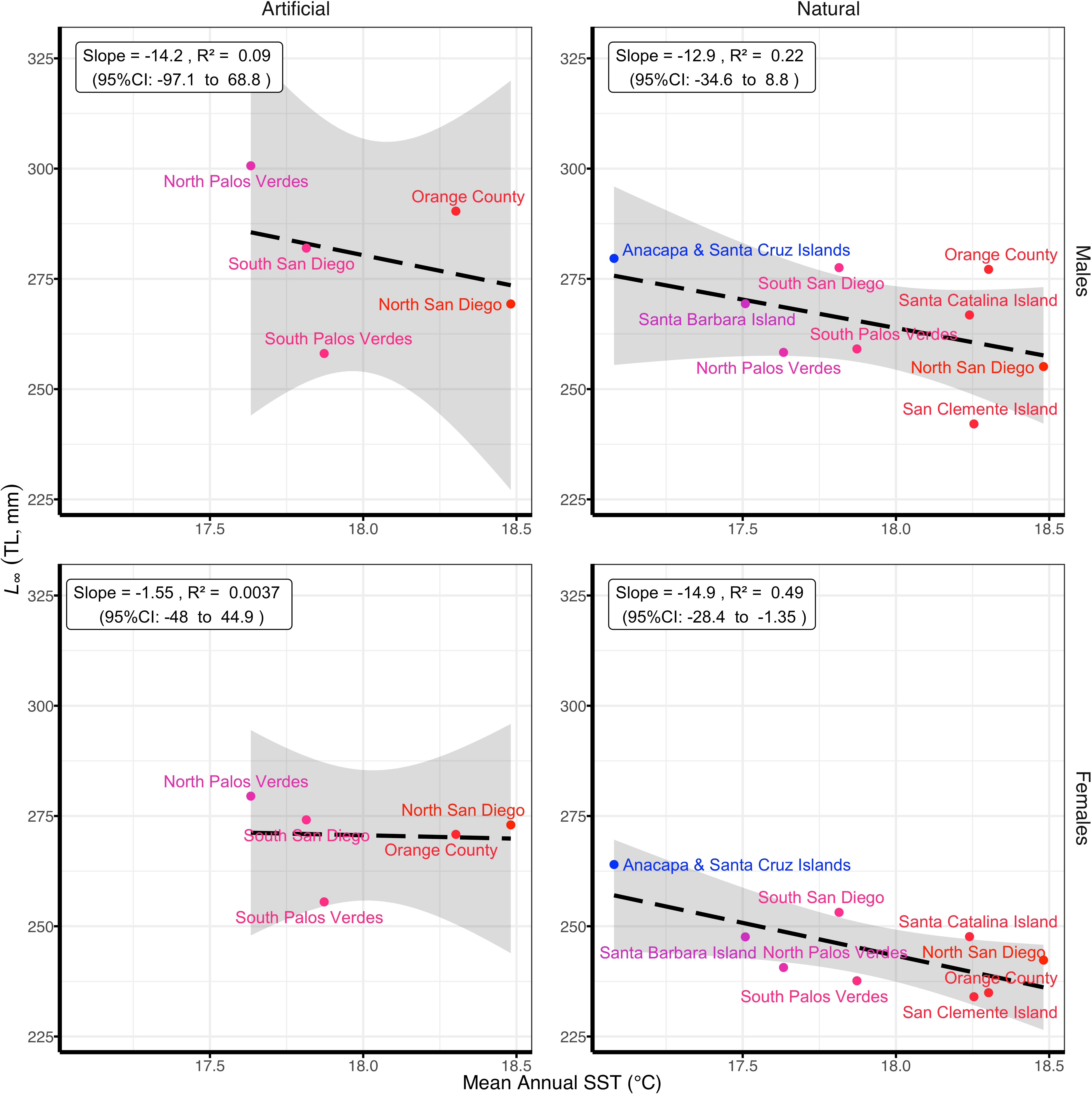
Figure 3 Relationship between mean asymptotic total length (L∞) of Garibaldi (Hypsypops rubicundus) from the highest ranked (and most complex) model (Table 2) and mean annual sea surface temperature (SST; °C) for January 2002-December 2018. Locations are colored from a scale of blue (cold) to red (warm).
The negative relationship between mean asymptotic total length (L∞) and mean annual SST was much stronger for natural reefs (male R2 = 0.22, slope = -12.9 mm/°C, 95% CI: -34.6 to 8.8 mm/°C ; female R2 = 0.49, slope = -14.8 mm/°C, 95% CI: -28.4 to -1.3 mm/°C) than at artificial reefs (male R2 = 0.09, slope = -14.2 mm/°C, 95% CI: -97.1 to 68.8 mm/°C; female R2 =< 0.01, slope = -1.55 mm/°C, 95% CI: -47.9 to 44.9 mm/°C) for both sexes (Figure 3). Among the Channel Islands, growth patterns followed both a latitudinal and SST gradient (Figure 3). Garibaldi from Anacapa and Santa Cruz Islands, the northernmost and coolest location, had the largest L∞ for both sexes at any island (male L∞ = 280 mm, female L∞ = 264 mm) whereas at the southernmost and warmest channel island, San Clemente Island, L∞ was the smallest at any island for both sexes (male L∞ = 242 mm, female L∞ = 234 mm). Mainland sites spanned a smaller range in both latitude and mean annual SST and the patterns were less distinct. The largest L∞ for Garibaldi from mainland natural reefs was in South San Diego (Point Loma) for both males and females (male L∞ = 278 mm, female L∞ = 253 mm). The main difference was for males from natural reefs in Orange County, which were similarly large (L∞ = 277 mm), while the females were the smallest (L∞ = 235 mm). Patterns in the growth coefficient (k) are generally more difficult to interpret than those for L∞ because they are dependent on L∞ in the von Bertalanffy growth model. However, when k and L∞ are plotted together, there was also a positive relationship with temperature for both males and females at natural reefs and a less distinct pattern for artificial reefs (Figure S7 in Supplementary Material).
Longevity (Tmax) exhibited a similar pattern with mean annual SST as observed for L∞ (Figure 4). Among natural reefs, maximum age (Tmax) was negatively correlated with mean annual SST (male R2 = 0.36 slope = -14.9 yrs/°C, 95% CI: -32.8 to 3.0 yrs/°C; female R2 = 0.46, slope = -12.9 yrs/°C, 95% CI: -25.3 to -0.4 yrs/°C). This supported the hypothesis that fish live longer in colder locations, while there was no relationship for fish from artificial reefs (male R2 =< 0.01, slope = 1.31 yrs/°C, 95% CI: -51.5 to 54.1 yrs/°C; female R2 = 0.01, slope = -4.5 yrs/°C, 95% CI: -76.8 to 67.8 yrs/°C) (Figure 4). The maximum ages of Garibaldi in this study were 57 years for males and 55 years for females (Figures 2, 4; Figures S5, S6, and Table S5 in Supplementary Material). Both fish were collected from Santa Cruz Island, the northernmost location with the coldest mean annual SST. Within locations, fish lived longer on natural reefs compared with their paired artificial reefs, except for the South San Diego population (Table S4 in Supplementary Material). There was no apparent difference in longevity between sexes (Figures S5, S6, and Table S6 in Supplementary Material), although the relationship between mean annual SST and maximum age was stronger for females than for males (Figure 4).
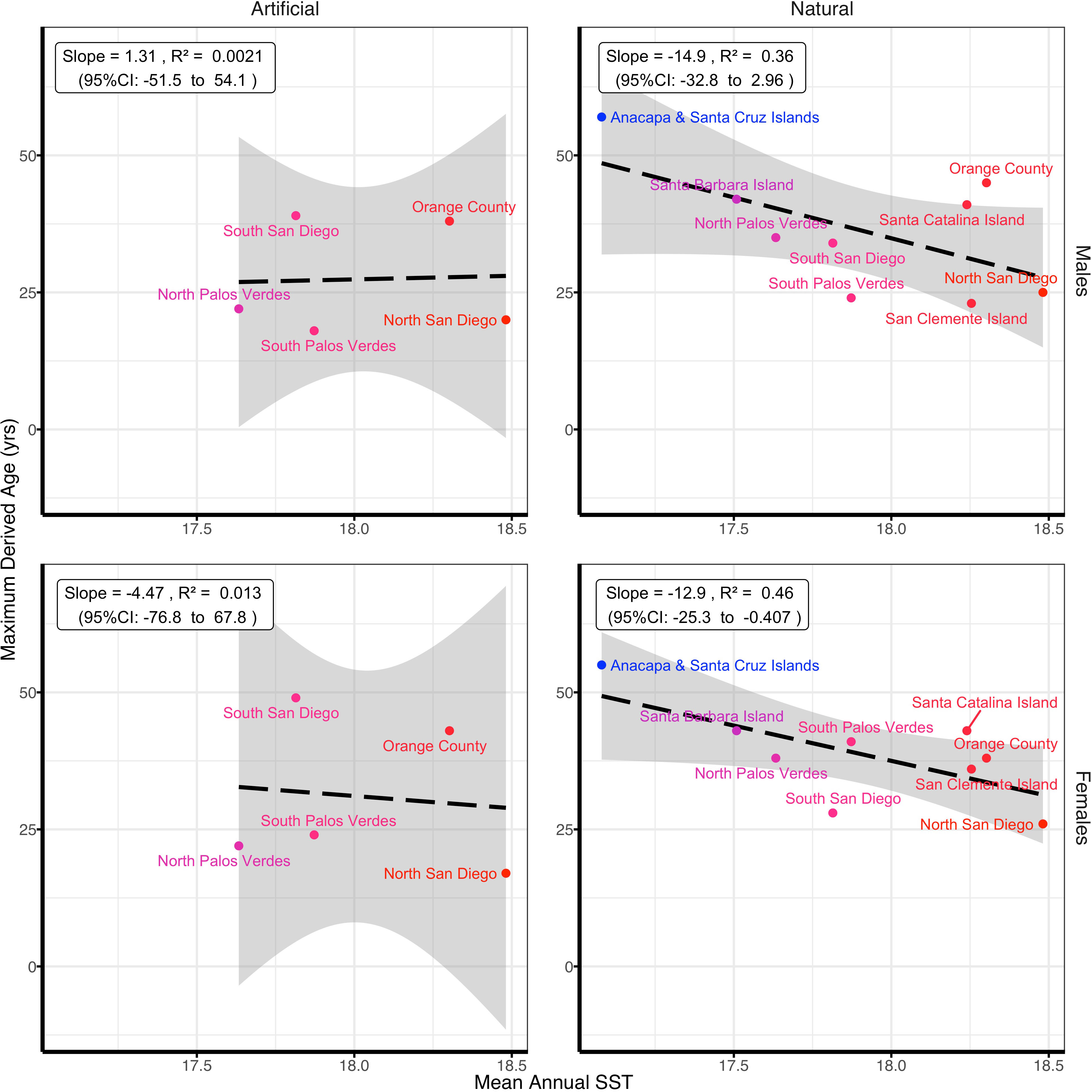
Figure 4 Relationship between maximum derived age (life span) of Garibaldi (Hypsypops rubicundus) and mean annual sea surface temperature (SST; °C) for January 2002-December 2018. Locations are colored from a scale of blue (cold) to red (warm).
Discussion
Spatial and habitat variability in the life history of Garibaldi is apparent at the relatively small spatial scale of this study with growth patterns differing by reef type, location, and sex. Garibaldi exhibited clear sexual size dimorphism; males grew larger than females in all populations. Garibaldi grew significantly larger on artificial quarry rock reefs (i.e., breakwaters and jetties) compared to natural rocky reefs, but tended to live longer on natural reefs. Regionally, growth and longevity patterns followed gradients in mean annual sea surface temperature, consistent with the hypothesis that Garibaldi are larger and live longer in cooler locations than in warmer locations. Sex-specific average adult asymptotic size (L∞) was greater for most artificial reef sites than the coldest natural reef sites, indicating, at least across the temperature range sampled, that habitat can have a greater effect than environmental conditions on this life history parameter, particularly for females. The Garibaldi collected for this study had a maximum age of 57 years, which makes them the longest lived of any species of damselfish by two decades (Tzioumis and Kingsford, 1999). Annual otolith increment formation was validated using the in-situ tetracycline mark-recapture method which is an ideal technique for age validation for species with high site fidelity (Campana, 2001). This technique is uncommon for fish life history studies conducted in temperate marine systems.
Sexual dimorphism in size was observed within all populations of Garibaldi and agreed with Clarke’s (1970) observation that males achieve larger body size than females. This appears to be an unusual trait for damselfish species (Dulčić and Kraljević, 1995; Kim et al., 2016) and nearshore dioicous reef-associated fish in southern California (Love and Westphal, 1981; Love et al., 1987; MacNair et al., 2001; Laidig et al., 2003; Pondella et al., 2008; Grebel and Cailliet, 2010; Williams et al., 2012; Miller et al., 2014), where adult females are typically larger. Only one other damselfish species, the Canary Damsel (Similiparma lurida) from the northeastern Atlantic (García-Mederos et al., 2016), shows the same pattern of males growing larger than females. Other than average body size, there are no visually discernable phenotypic sexually dimorphic traits for Garibaldi, as researchers were unable to distinguish between sexes both underwater and in the laboratory. In addition, length-weight relationships were very similar between sexes (Figure S4 in Supplementary Material), which demonstrates that males simply grow larger than females, but there are no apparent body shape or weight differences when at similar lengths. Garibaldi exhibit polygyny, where multiple females sequentially lay eggs in one male’s nest site. Females visit multiple nest sites before selecting one for spawning, and tend to prefer nests that have a high percentage of dense turf algae and contain eggs in the early phase of development (i.e., another female had deposited eggs there less than 5 days prior) (Sikkel, 1988; Sikkel, 1989; Sikkel, 1994; Sikkel, 1995). Sikkel, 1988; 1989 also found that male size was correlated with female nest preference, and posited that male size is correlated with nest quality, although the relationship between the two variables was not strong. Further, large males may be more successful in the defense of their nesting sites (Sikkel, 1995), suggesting that females are choosing mates based on size.
For mainland locations, Garibaldi grew larger on average (L∞) at artificial reefs than at paired natural reefs. The breakwater and jetty artificial reefs sampled in this study were primarily built to protect coastal infrastructure and were made up of large (1-2 m) quarry rock boulders, providing a complex and ideal habitat with vertical rock surfaces and several open spaces for Garibaldi to maintain territories (Stephens et al., 1994; Cook, 2011). Natural rocky reefs within the SCB, although much larger in area, characteristically have lower vertical relief and less habitat complexity than breakwaters (Pondella et al., 2015b). For rocky reefs within the SCB, vertical relief and variation in vertical relief is correlated with increased fish density, biomass, and production (Ambrose and Swarbrick, 1989; Pondella et al., 2002; Pondella et al., 2018; Pondella et al., 2019) indicating that more complex reefs are more productive than homogenous habitats. Garibaldi from artificial reefs grew significantly larger, on average, than fish on natural reefs (sexes pooled, artificial L∞ = 276, 95% CI: 271 to 281 mm; natural L∞ = 252; 95% CI: 249 to 255 mm) (Table S7 and Figure S10 in Supplementary Material). However, the largest Garibaldi were from natural reefs at Anacapa & Santa Cruz Islands (290 mm TL for females, 307 mm TL for males). This may be due to not sampling artificial habitats in coolest locations, where fish tend to be larger overall, and sampling effort being greater overall for natural reefs (n = 732) than for artificial reefs (n = 298).
Garibaldi from South Palos Verdes and South San Diego did not exhibit as strong of a difference in growth patterns between natural and artificial habitats as the other mainland locations. Both the natural and artificial reefs at South Palos Verdes are degraded habitats, which may have affected growth. The natural rocky reef (Bunker Point) is characterized by low structural relief and is impacted by sedimentation from ongoing landslides from 1956 to present, resulting in reef burial, scour, and chronic turbidity (Pondella et al., 2018). Scour and sedimentation affect benthic sessile invertebrate communities (e.g., sponges, anemones), the primary component in the diet of Garibaldi, interfering with filter and suspension feeding, or by denuding or burying the reef and eliminating sessile invertebrates entirely in severe instances (Figurski et al., 2016). In addition, the reduction in light intensity due to persistent turbidity limits benthic algal recruitment and growth (Airoldi and Virgilio, 1998; Gibson and Atkinson, 2003), which is necessary for successful nest-building (Sikkel, 1995). The South Palos Verdes artificial reef (Port of Los Angeles breakwater) borders the busiest harbor in the United States. In South San Diego, both natural (Point Loma) and artificial (Zuniga Jetty) reefs are adjacent to San Diego Bay, a highly urbanized estuary and home to the Port of San Diego. Point Loma is an expansive shelf of natural reef characterized by low relief bedrock with minimal slope and lacking boulders that would provide hiding spaces and good habitat for Garibaldi. Unlike the other breakwaters sampled, Zuniga Jetty is often fully submerged and Garibaldi typically recruit to rocky reefs depths of less than 5m (Clarke, 1970). The distance from the shallow subtidal to the reef where fish were collected at Point Loma, compounded with the relatively high density of adult Garibaldi (Cook, 2011) and skewed sex ratio (2/3 of fish were male) at Zuniga Jetty could explain why natural and artificial reefs in South San Diego are performing similarly in relation to Garibaldi growth patterns.
Growth and longevity patterns followed gradients in mean annual sea surface temperature with fish getting larger and living longer in cooler environments than in warmer environments, particularly for females from natural reefs. This is a common intraspecific pattern in damselfishes and marine fishes in general and has been observed on spatial scales larger and smaller than the present study (Meekan et al., 2001; Choat and Robertson, 2002; Choat et al., 2003; Ruttenberg et al., 2005; Hamilton et al., 2011; Trip et al., 2014; Taylor et al., 2019). Within the SCB, this pattern of fish growing larger at cooler locations has also been observed in the California Sheephead (Bodianus pulcher), a fished protogynous hermaphroditic wrasse (Hamilton et al., 2011) and in recreationally and commercially targeted conspecific rockfish species (Scorpaenidae: Sebastes) (Love et al., 1990). Further, California Halibut (Paralichthys californicus) grew larger in the cooler waters of central California than in southern California (MacNair et al., 2001). The pattern in the present study was not as strong as in other studies, likely due to the mean annual SST values throughout the SCB varying by only ~ 1.5°C. Further, sample sizes were relatively small for a given location, especially when grouping populations by sex, which can increase variability in estimates of L∞ and Tmax (Kritzer et al., 2001). The relationship between age and growth and SST was not present for Garibaldi from artificial reefs, but these reefs spanned a lower temperature range than natural reefs. Further, annual and seasonal temperature patterns in the SCB are dynamic (Dong et al., 2009; Kim and Cornuelle, 2015) and long-term annual SST means over the relatively small spatial scale may not be indicative of the environmental conditions that influence Garibaldi life history at local scales. Finally, fish were collected opportunistically over a six-year period, with some locations only being visited once and others being visited multiple times. Differences in sample dates across some sites could mean that the younger fish in a sample may have experienced different environmental conditions during their early faster growth years prior to when they were collected, possibly confounding temporal and spatial effects on growth. However, this was unlikely to affect the major results of our study. Most natural-artificial reef pairs were collected in the same or similar sets of years. Further, we focused our comparisons mostly on estimates of L∞, and for the long-lived Garibaldi, this parameter is going to primarily be determined by the 10- to 40-year-old fish in our sample which would not be impacted by the relatively small differences in collection dates.
Garibaldi have a much longer lifespan than has previously been reported. The next longest lifespan for a Pomacentrid is for the White-Ear Scalyfin (Parma microlepis) endemic to Eastern Australia at 37 years maximum age (Tzioumis and Kingsford, 1999), and most damselfishes considered to be “long-lived” live to be only 10-20 years old (Fowler, 1990; Fowler and Doherty, 1992; Kohda, 1996; Meekan et al., 2001; Schwamborn and Ferreira, 2002; Ruttenberg et al., 2005). Maximum age for both sexes was negatively correlated with mean annual SST for natural reefs, supporting the hypothesis that fish live longer in colder locations. There was also no apparent difference in longevity between sexes although the relationship between mean annual SST and maximum age was stronger for females than for males. The oldest 55-year-old female and 57-year- old male were from the highest latitude and the coolest Santa Cruz Island location. It is important to note that mean annual SST and latitude do not follow the same pattern within the SCB due to the complex current system. The furthest south location, South San Diego had the second oldest female fish (49 years) and is cooler than locations that are further north due to the Southern California Countercurrent (Table 1; Figure 1). There were consistently multiple fish around 40 years old collected from many sites (Table S4, Figure S6 in Supplementary Material). The long lifespan of this species and relatively small sample sizes for each site and sex, make it less likely to collect very old fish and thus limits the ability to make precise longevity comparisons across sites.
This study has demonstrated that habitat and environmental conditions can have a substantial effect on localized age and growth patterns in the absence of fishing effects. Our results contribute to the growing body of evidence that age and growth patterns for marine fish vary spatially and among habitats, even at small geographical scales. In addition, this study provided location-, habitat- and sex-specific parameters enabling researchers to effectively apply appropriate life history traits for specific localities to estimate secondary production (e.g., Claisse et al., 2014, Granneman and Steele, 2014) and as a basis to further investigate differences in habitat quality. The effects of habitat and temperature on both age and growth patterns were stronger for female Garibaldi than for males. Behavioral factors such as sexual selection and competition amongst males for apparently preferable nesting sites (Sikkel, 1988; Sikkel, 1989; Sikkel, 1995) may play a larger role in shaping the localized life history patterns for male Garibaldi than for females. Additional variability in male growth rates and longevity could result from variability in energy expenditures for territoriality, courting behaviors, nest defense, and/or differences in habitat quality at the scale of individual male territories. That adult female Garibaldi grow significantly larger on artificial reefs is a potential indicator of habitat quality and may have regional population-level implications. Estimates of total reproductive-energy output (i.e., combining fecundity, egg size, egg energy content) per spawn scaled hyperallometrically with body size for Garibaldi and other demersal spawning damselfish species, and therefore larger mothers contribute disproportionately to population replenishment (Barneche et al., 2018). The amount of human-built structures in the marine environment is expected to increase in the coming decades with the continued expansion of marine infrastructure associated with activities such as port construction, marine aquaculture, sea level rise adaptation, and marine renewable energy development (Tickell et al., 2019; Bugnot et al., 2020), and with the growing interest to design artificial reefs for reef habitat restoration and mitigation (e.g., Pondella et al., 2006; Reed et al., 2006; Pondella et al., 2018; Komyakova et al., 2019) and/or for fisheries enhancement (e.g., Polovina and Sakai, 1989; Santos et al., 2011; Roa-Ureta et al., 2019). Fish life history studies that compare ecological performance across habitat types, such as this one, are essential to inform the design and assessment of these future reefing projects.
Data availability statement
The raw data supporting the conclusions of this article will be made available by the authors, without undue reservation.
Ethics statement
The animal study was reviewed and approved by Institutional Care and Use Committees at both Cal Poly Pomona and Occidental College.
Author contributions
CW envisioned the project, managed and contributed to field work and dissections, performed computational analyses, and wrote the manuscript. JE contributed to field work and dissections, prepared histological preparations of gonads and performed microscopic determination of sex. DP contributed to field work and writing. JC envisioned the project, contributed to field work, and oversaw writing and data analysis. All authors contributed to the article and approved the submitted version.
Funding
Funding for this project was provided by the Vantuna Research Group at Occidental College, the Claisse Lab at Cal Poly Pomona, the Southern California Academy of Sciences, the American Society of Ichthyologists and Herpetologists, the Department of Biological Sciences at Cal Poly Pomona, the Western Society of Naturalists, and CSU Council on Ocean Affairs, Science & Technology (COAST) and the University of Southern California Sea Grant Program through a Sea Grant Traineeship to C. M. Williams.
Acknowledgments
This project, particularly the field component, was an immense undertaking and would not have been completed without the help of several people. Thanks to those who participated in field collections, dissections, and gonad histological preparations, particularly J. Williams, M. Robart, M. Hansler, B. Power, B. Grime, A. Pyles, J. Eagleton, Z. Scholz, S. Schwab, A. Obaza, C. Pollock, D. Erana, K. Lau, E. Jaco, S. Soule, A. Sugimoto, A. Roeper, C. Pollock, G. Coogan, H. Hoefs, M. Winston, N. Myers, R. Stokes, S. Moffly, S. Akiyama, S. Ashey, S. D’Orazio, S. Franck, E. Liang, G. Yang, J. Sturges, and B. Calderon. Several Garibaldi were collected from Catalina Island by Dr. S. Hamilton at Moss Landing Marine Laboratories. J. Davis, C. Dobbins, K. Walker at California Department of Fish and Wildlife provided ship time to collect Garibaldi at Santa Cruz and Anacapa Islands. We thank the anonymous reviewers for their careful reading, insightful comments, and suggestions that improved the quality of this manuscript. The content of this manuscript was previously published as a master’s thesis (Williams, 2021) and benefitted greatly from the guidance of committee members A. Zellmer, A. Bonisoli-Alquati, and Á. Valdés.
Conflict of interest
The authors declare that the research was conducted in the absence of any commercial or financial relationships that could be construed as a potential conflict of interest.
Publisher's note
All claims expressed in this article are solely those of the authors and do not necessarily represent those of their affiliated organizations, or those of the publisher, the editors and the reviewers. Any product that may be evaluated in this article, or claim that may be made by its manufacturer, is not guaranteed or endorsed by the publisher.
Supplementary material
The Supplementary Material for this article can be found online at: https://www.frontiersin.org/articles/10.3389/fmars.2022.988158/full#supplementary-material
References
Airoldi L., Virgilio M. (1998). Responses of turf-forming algae to spatial variations in the deposition of sediments. Mar. Ecol. Prog. Ser. 165, 271–282. doi: 10.3354/meps165271
Alcalay O. M., Sikkel P. C. (1994). Diel periodicity of hatching of demersal eggs in the temperate damselfish, Hypsypops rubicundus. Bull. Mar. Sci. 54 (2), 565–569.
Allen L. G., Pondella II D. J. (2006). “Ecological Classification,” in The ecology of marine fishes: California and adjacent waters. Eds. Allen L. G., Pondella D. J. II, Horn M. (Los Angeles, CA: University of California Press), 81–113.
Ambrose R. F., Swarbrick S. L. (1989). Comparison of fish assemblages on artificial and natural reefs off the coast of southern California. Bull. Mar. Sci. 44, 718–733.
Anderson D. R. (2008). Model based inferences in the life sciences: A primer on evidence (New York, New York, USA: Springer Science + Business Media, LLC).
Atkinson D. (1994). Temperature and organism size - a biological law for ectotherms? Adv. Ecol. Res. 25, 1–58. doi: 10.1016/0306-4565(94)00028-H
Atkinson D. (1996). “Ectotherm life-history responses to developmental temperature,” in Animals and temperature: Phenotypic and evolutionary adaptation. Eds. Johnston I. A., Bennett A. F. (New York: Cambridge University Press), 183–204.
Atkinson D., Sibly R. M. (1997). Why are organisms usually bigger in colder environments? making sense of a life history puzzle. Trends Ecol. Evol. 12 (6), 235–239. doi: 10.1016/S0169-5347(97)01058-6
Audzijonyte A., Fulton E., Haddon M., Helidoniotis F., Hobday A. J., Kuparinen A., et al. (2016). Trends and management implications of human-influenced life-history changes in marine ectotherms. Fish Fish. 7, 1005–1028. doi: 10.1111/faf.12156
Barilotti A. A., White C. F., Lowe C. G. (2020). Are fishes attracted to piers? Movements and association of marine fishes to a public fishing pier within a commercial harbor. Bulletin South. Cal. Acad. Sci. 119 (1), 18–34. doi: 10.3160/0038-3872-119.1.18
Barneche D. R., Robertson D. R., White C. R., Marshall D. J. (2018). Fish reproductive-energy output increases disproportionately with body size. Science 360 (6389), 642–645. doi: 10.1126/science.aao6868
Beamish R. J., McFarlane G. A. (1995). “A discussion of the importance of aging errors, and an application to walleye pollock: the world ‘s largest fishery,” in Recent developments in fish otolith research. Eds. Secor D. H., Dean J. M., Campana S. E. (Columbia: University of South Carolina Press).
Berumen M. L. (2005). The importance of juveniles in modelling growth: butterflyfish at lizard island. Environ. Biol. Fish. 72 (4), 409–413. doi: 10.1007/s10641-004-2595-0
Beverton R. J. H., Holt S. J. (1959). “A review of the lifespans and mortality rates of fish in nature, and their relation to growth and other physiological characteristics,” in Ciba foundation symposium - the Lifespan of animals, colloquia on aging. (Boston, MA: Little, Brown), 142–180.
Bolker B., R Core Development Team (2020). Bbmle: Tools for general maximum likelihood estimation. R package version 1.0.23.1.
Bugnot A. B., Mayer-Pinto M., Airoldi L., Heery E. C., Johnston E. L., Critchley L. P., et al. (2020) Current and projected global extent of marine built structures. Nat. Sustain. doi: 10.1038/s41893-020-00595-1
Burnham K. P., Anderson D. R. (2002). Model selection and multimodel inference, a practical information-theoretic approach (New York, New York, USA: Springer Science + Business Media, LLC).
Burns E. S., Clevenstine A. J., Logan R. K., Lowe C. G. (2020). Evidence of artificial habitat use by a recovering marine predator in southern California. J. Fish Biol. 97 (6), 1857–1860. doi: 10.1111/jfb.14539
Campana S. E. (2001). Accuracy, precision and quality control in age determination, including a review of the use and abuse of age validation methods. J. Fish Biol. 59, 197–242. doi: 10.1111/j.1095-8649.2001.tb00127.x
Cappo M., Eden P., Newman S. J., Robertson S. (2000). A new approach to validation of periodicity and timing of opaque zone formation in the otoliths of eleven species of Lutjanus from the central great barrier reef. Fish. Bull. 98, 474–488.
Caselle J. E., Hamilton S. L., Schroeder D. M., Love M. S., Standish J. D., Rosales-Casian J. A., et al. (2011). Geographic variation in density, demography, and life history traits of a harvested, sex-changing, temperate reef fish. Can. J. Fish. Aquat. Sci. 68 (2), 288–303. doi: 10.1139/f10-140
Choat J. H., Axe L. M. (1996). Growth and longevity in fishes of the family scaridae. Mar. Ecol. Prog. Ser. 145, 33–41. doi: 10.3354/meps145033
Choat J. H., Robertson D. R. (2002). “Age-based studies,” in Coral reef fishes: dynamics and diversity in a complex ecosystem (San Diego, California, USA: Academic Press), 57–80.
Choat J. H., Robertson D. R., Ackerman J. L., Posada J. M. (2003). An age-based demographic analysis of the Caribbean stoplight parrotfish Sparisoma viride. Mar. Ecol. Prog. Ser. 246, 265–277. doi: 10.3354/meps246265
Claisse J. T. (2009). The life history and movements of yellow tang, Zebrasoma flavescens, with implications for fisheries management using protected areas (Manoa: University of Hawaii).
Claisse J. T., Kienzle M., Bushnell M. E., Shafer D. J., Parrish J. D. (2009). Habitat- and sex-specific life history patterns of yellow tang Zebrasoma flavescens in Hawaii, USA. Mar. Ecol. Prog. Ser. 389, 245–255. doi: 10.3354/meps08114
Claisse J. T., Love M. S., Meyer-Gutbrod E. L., Williams C. M., Pondella D. J. II, Daniel J. (2019). Fishes with high reproductive output potential on California offshore oil and gas platforms. Bull. Mar. Sci. 95 (4), 515–534. doi: 10.5343/bms.2019.0016
Claisse J. T., Pondella D. J. II, Love M., Zahn L. A., Williams C. M., Williams J. P., et al. (2014). Oil platforms off California are among the most productive marine fish habitats globally. Proc. Natl. Acad. Sci. USA 111 (43), 15462–15467. doi: 10.1073/pnas.1411477111
Clarke T. A. (1970). Territorial behavior and population dynamics of a pomacentrid fish, the Garibaldi Hypsypops rubicunda. Ecol. Soc. America 40 (2), 189–212. doi: 10.2307/1942295
Clarke T. A. (1971). Territory boundaries, courtship, and social behavior in the garibaldi, Hypsypops rubicunda (Pomacentridae). Copeia 1971, 295–299. doi: 10.2307/1442830
Cook G. S. (2011). Rocky reef fish connectivity: Patterns, processes, and population dynamics (San Diego: University of California).
DeMartini E. E., Barnett A. M., Johnson T. D., Ambrose R. F. (1994). Growth and production estimates for biomass-dominant fishes on a southern California artificial reef. Bull. Mar. Sci. 55, 484–500.
DeMartini E. E., Roberts D. A., Anderson T. W. (1989). Contrasting patterns of fish density and abundance at an artificial rock reef and a cobble-bottom kelp forest. Bull. Mar. Sci. 44 (2), 881–892.
Dong C., Idica E. Y., McWilliams J. C. (2009). Circulation and multiple-scale variability in the Southern California Bight. Prog. Oceanogr. 82 (3), 168–190. doi: 10.1016/j.pocean.2009.07.005
Dulčić J., Kraljević M. (1995). Age, growth and mortality of damselfish (Chromis chromis l.) in the eastern middle Adriatic. Fish. Res. 22 (3-4), 255–264. doi: 10.1016/0165-7836(94)00318-Q
Engle J. (1993). “Distribution patterns of rocky subtidal fishes around the California islands,” in Third California islands symposium: recent advances in research on the California islands (Santa Barbara, California, USA: Santa Barbara Museum of Natural History), 475–484.
Figueira W. F., Lyman S. J., Crowder L. B., Rilov G. (2007). Small-scale demographic variability of the biocolor damselfish, Stegastes partitus, in the Florida keys USA. Environ. Biol. Fish. 81 (3), 297–311. doi: 10.1007/s10641-007-9201-1
Figurski J. D., Freiwald J., Lonhart S. I., Storlazzi C. D. (2016). Seasonal sediment dynamics shape temperate bedrock reef communities. Mar. Ecol. Prog. Ser. 552, 19–29. doi: 10.3354/meps11763
Fonseca V., Cabral H. (2007). Are fish early growth and condition patterns related to life-history strategies? Rev. Fish Biol. Fish. 17 (4), 545–564. doi: 10.1007/s11160-007-9054-x
Forsberg J. E. (2001). “Aging manual for pacific halibut: procedures and methods used at the International pacific halibut commission“, in International Pacific Halibut Commission Technical Report 46. (Seattle, WA).
Foster M. S. (1972). “The algal turf community in the nest of the ocean goldfish, Hypsypops rubicunda,” in Proceedings of the 7th international seaweed symposium. Ed. Nisizawa K. (Japan: University of Tokyo Press), 55–60.
Fowler A. J. (1990). Validation of annual growth increments in the otoliths of a small, tropical coral reef fish. Mar. Ecol. Prog. Ser. Oldendorf 64 (1), 25–38. doi: 10.3354/meps064025
Fowler A. J., Doherty P. J. (1992). Validation of annual growth increments in the otoliths of two species of damselfish from the southern great barrier reef. Mar. Freshw. Res. 43 (5), 1057–1068. doi: 10.1071/MF9921057
Francis M. P., Francis R. I. C. C. (1992). Growth rate estimtes for new Zealand rig (Mustelus lenticulatus). Aust. J. Freshw. Res. 43, 1157–1176. doi: 10.1071/MF9921157
García-Mederos A. M., Tuya F., Tuset V. M. (2016). Life-history strategies of a conspicuous reef fish, the canary damsel Similiparma lurida (Pomacentridae) in the northeastern Atlantic. Sci. Mar. 8 (1), 57–68. doi: 10.3989/scimar.04343.28A
Gibson R. N., Atkinson R. J. A. (2003). The effects of sedimentation on rocky coast assemblages. Oceanogr. Mar. Biol.: an Annu. Rev. 41, 161–236. doi: 10.1201/9780203180570-23
Granneman J. E. (2011). A comparison of fish production on artifical and natural reefs in southern California (Northridge: California State University).
Granneman J. E., Steele M. A. (2014). Fish growth, reproduction, and tissue production on artificial reefs relative to natural reefs. ICES J. Mar. Sci. 71 (9), 2494–2504. doi: 10.1093/icesjms/fsu082
Granneman J. E., Steele M. A. (2015). Effects of reef attributes on fish assemblage similarity between artificial and natural reefs. ICES J. Mar. Sci. 72 (8), 2385–2397. doi: 10.1093/icesjms/fsv094
Grebel J. M., Cailliet G. M. (2010). Age, growth, and maturity of cabezon (Scorpaenichthys marmoratus) in California. Cal. Fish Game 96 (1), 36–52.
Hamilton S. L., Caselle J. E., Standish J. D., Schroeder D. M., Love M. S., Rosales-Casian J. A., et al. (2007). Size-selective harvesting alters life histories of a temperate sex-changing fish. Ecol. Appl. 17 (8), 2268–2280. doi: 10.1890/06-1930.1
Hamilton S. L., Wilson J. R., Ben-Horin T., Caselle J. E. (2011). Utilizing spatial demographic and life history variation to optimize sustainable yield of a temperate sex-changing fish. PloS One 6 (9), e24580. doi: 10.1371/journal.pone.0024580
Hickey B. M. (1992). Circulation over the Santa Monica-San Pedro basin and shelf. Prog. Oceanogr. 30 (1-4), 37–115. doi: 10.1016/0079-6611(92)90009-O
Hjelm J., Persson L., Christensen B. (2000). Growth, morphological variation and ontogenetic niche shifts in perch (Perca fluviatilis) in relation to resource availability. Oecologia 122(2):190–199. doi: 10.1007/PL00008846
Hsieh C.-h., Yamauchi A., Nakazawa T., Wang W.-F. (2009). Fishing effects on age and spatial structures undermine population stability of fishes. Aquat. Sci. 72(2):165–178. doi: 10.1007/s00027-009-0122-2
Jennings S., Dulvy N. K. (2008). Beverton and Holt’s insights into life history theory: Influence, application and future use. Adv. Fish. Sci. 50, 434–450. doi: 10.1002/9781444302653.ch18
Kilpatrick T., Xie S. P., Miller A. J., Schneider N. (2018). Satellite observations of enhanced chlorophyll variability in the Southern California Bight. J. Geophys. Res.: Ocean. 123 (10), 7550–7563. doi: 10.1029/2018JC014248
Kim S. Y., Cornuelle B. D. (2015). Coastal ocean climatology of temperature and salinity off the Southern California Bight: Seasonal variability, climate index correlation, and linear trend. Prog. Oceanogr. 138, 136–157. doi: 10.1016/j.pocean.2015.08.001
Kim H., Na J., Oh C. W. (2016). Age and growth of damselfish Chromis notata (Temminck & schlegel 1843), Jeju Island, Korea. J. Appl. Ichthyol. 32 (6), 1179–1185. doi: 10.1111/jai.13137
King J., McFarlane G. (2003). Marine fish life history strategies: applications to fishery management. Fish. Manage. Ecol. 10 (4), 249–264. doi: 10.1046/j.1365-2400.2003.00359.x
Kohda M. (1996). A damselfish living for more than 15 years: a longevity record for small reef fishes. Ichthyol. Res. 43 (4), 459–462. doi: 10.1007/BF02347643
Komyakova V., Chamberlain D., Jones G. P., Swearer S. E. (2019). Assessing the performance of artificial reefs as substitute habitat for temperate reef fishes: Implications for reef design and placement. Sci. Total Environ. 668, 139–152. doi: 10.1016/j.scitotenv.2019.02.357
Kritzer J. P., Davies C. R., Mapstone B. D. (2001). Characterizing fish populations: effects of sample size and population structure on the precision of demographic parameter estimates. Can. J. Fish. Aquat. Sci. 58 (8), 1557–1568. doi: 10.1139/cjfas-58-8-1557
Kritzler H., Fox D. L., Hubbs C. L., Crane S. C. (1950). Carotenoid pigmentation of the pomacentrid fish Hypsypops rubicunda. Copeia 1950 (2), 125–138. doi: 10.2307/1438955
Laidig T. E., Pearson D. E., Sinclair L. L. (2003). Age and growth of blue rockfish (Sebastes mystinus) from central and Northern California. Fish. Bull. 101 (4), 800–808.
Lewis R. D., McKee K. K. (1989). A guide to the artificial reefs of southern California. Dep. Fish Game Sacramento Cal. 73, 1–67.
Limbaugh C. (1964). Notes on the life history of two Californian pomacentrid: Garibaldis, Hypsypops rubicunda (Girard), and blacksmiths, Chromis punctipinnis (Cooper). Pac. Sci. 18, 41–50.
Love M. S., Axell B., Morris P., Collins R., Brooks A. (1987). Life history and fishery of the California scorpionfish, Scorpaena guttata, within the Southern California Bight. Fish. Bull. 85 (1), 99–116.
Love M. S., Brothers E. B., Schroeder D., Lenarz W. H. (2007). Ecological performance of young-of-the-year blue rockfish (Sebastes mystinus) associated with oil platforms and natural reefs in California as measured by daily growth rates. Bull. Mar. Sci. 80 (1), 147–157.
Love M. S., Claisse J. T., Roeper A. (2019). An analysis of the fish assemblages around 23 oil and gas platforms off California with comparisons with natural habitats. Bull. Mar. Sci. 95 (4), 477–514. doi: 10.5343/bms.2018.0061
Love M. S., Morris P., McCrae M., Collins R. (1990). Life history aspects of 19 rockfish species (Scorpaenidae: Sebastes) from the Southern California Bight. NOAA Tech. Rep. NMFS 827, 1–38.
Love M. S., Westphal W. V. (1981). Growth, reproduction, and food habits of olive rockfish, Sebastes serranoides, off central California. Fish. Bull. 79, 533–543.
MacNair L. S., Domeier M. L., Chun C. S. (2001). Age, growth, and mortality of California halibut, Paralichthys californicus, along southern and central California. Fish. Bull. 99 (4), 588–601.
Mangel M., Abrahams M. V. (2001). Age and longevity in fish, with consideration of the ferox trout. Exp. Gerontol. 36 (4-6), 765–790. doi: 10.1016/S0531-5565(00)00240-0
Meekan M., Ackerman J., Wellington G. (2001). Demography and age structures of coral reef damselfishes in the tropical eastern pacific ocean. Mar. Ecol. Prog. Ser. 212, 223–232. doi: 10.3354/meps212223
Miller E. F., Pondella D. J., Goldberg S. (2014). Life history and historic trends in salema (Haemulon californiensis) in Southern California. Cal. Coop. Ocean. Fish. Invest. Prog. Rep. 55, 144–157.
Nelson J. S., Grande T. C., Wilson M. V. H. (2016). Fishes of the world. 5th edition (Hoboken, New Jersey: John Wiley & Sons, Inc).
Polovina J. J., Sakai I. (1989). Impacts of artificial reefs on fishery production in Shimamaki, Japan. Bull. Mar. Sci. 44 (2), 997–1003.
Pondella D. J. II, Allen L. G., Craig M. T., Gintert B. (2006). Evaluation of eelgrass mitigation and fishery enhancement structures in San Diego Bay, California. Bull. Mar. Sci. 78 (1), 115–131.
Pondella D. J. II, Caselle J. E., Claisse J. T., Williams J. P., Davis K., Williams C. M., et al. (2015a). “Baseline characterization of the shallow rocky reef and kelp forest ecosystems of the south coast study region,” in South coast baseline program final report: Kelp and shallow rock ecosystems. SCMPA-27.
Pondella D. J. II, Froeschke J. T., Wetmore L. S., Miller E., Valle C. F., Medeiros L. (2008). Demographic parameters of yellowfin croaker, Umbrina roncador (Perciformes: Sciaenidae), from the Southern California Bight. Pac. Sci. 62 (4), 555–568. doi: 10.2984/1534-6188(2008)62[555:DPOYCU]2.0.CO;2
Pondella D. J. II, Piacenza S. E., Claisse J. T., Williams C. M., Williams J. P., Zellmer A. J., et al. (2019). Assessing drivers of rocky reef fish biomass density from the Southern California Bight. Mar. Ecol. Prog. Ser. 628, 125–140. doi: 10.3354/meps13103
Pondella D. J. II, Stephens J. S. (1994). Factors affecting the abundance of juvenile fish species on a temperate artificial reef. Bull. Mar. Sci. 55 (2-3), 1216–1223.
Pondella D. J. II, Stephens J. S., Craig M. T. (2002). Fish production of a temperate artificial reef based on the density of embiotocids (Teleostei: Perciformes). ICES J. Mar. Sci. 59, S88–S93. doi: 10.1006/jmsc.2002.1219
Pondella D. J. II, Williams J. P., Claisse J. T., Schaffner R., Ritter K., Schiff K. (2015b). The physical characteristics of nearshore rocky reefs in the Southern California Bight. Bull. South. Cal. Acad. Sci. 114 (3), 105–122. doi: 10.3160/soca-114-03-105-122.1
Pondella D. J. II, Williams J. P., Williams C. M., Claisse J.T., Witting D (2018). “Restoring a nearshore rocky reef ecosystem in the challenge of an urban setting,”Bortone S Marine Artificial Reef Research and Development: Integrating Fisheries Management Objectives Location: Bethesda, MD:American Fisheries Society Symposium. 86, 165–186
R Core Development Team (2021). “R: A language and environment for statistical computing,” in R foundation for statistical computing (Vienna, Austria:R Core Development Team 2021). Available at: http://www.R-project.org.
Reed D. C., Schroeter S. C., Huang D., Anderson T. W., Ambrose R. F. (2006). Quantitative assessment of different artificial reef designs in mitigating losses to kelp forest fishes. Bull. Mar. Sci. 78 (1), 133–150.
Roa-Ureta R. H., Santos M. N., Leitão F. (2019). Modelling long-term fisheries data to resolve the attraction versus production dilemma of artificial reefs. Ecol. Model. 407, 1–12. doi: 10.1016/j.ecolmodel.2019.108727
Robertson D. R., Choat J. H., Posada J. M., Pitt J., Ackerman J. L. (2005). Ocean surgeonfish Acanthurus bahianus. II. Fishing effects on longevity, size and abundance? Mar. Ecol. Prog. Ser. 295, 245–256. doi: 10.3354/meps295245
Ruttenberg B. I., Haupt A. J., Chiriboga A. I., Warner R. R. (2005). Patterns, causes and consequences of regional variation in the ecology and life history of a reef fish. Oecologia 145 (3), 394–403. doi: 10.1007/s00442-005-0150-0
Santos M. N., Monteiro C. C., Leitão F. (2011). The role of artificial reefs in the sustainability of artisanal fisheries. Artificial reefs in fisheries management (New York:Taylor and Francis Group), 221–237 edited by. Bortone SA, Brandini FP, Fabi G, Otake S
Schiff K. C., Allen M. J., Zeng E. Y., Bay S. M. (2000). Southern California. Mar. Pollut. Bull. 41 (1-6), 76–93. doi: 10.1016/S0025-326X(00)00103-X
Schwamborn S. H., Ferreira B. P. (2002). Age structure and growth of the dusky damselfish, Stegastes fuscus, from tamandare reefs, Pernambuco, Brazil. Environ. Biol. Fish. 63 (1), 79–88. doi: 10.1023/A:1013851532298
Sikkel P. C. (1988). Factors influencing spawning site choice by female garibaldi, Hypsypops rubicundus (Pisces: Pomacentridae). Copeia 1988, 710–718. doi: 10.2307/1445392
Sikkel P. C. (1989). Egg presence and developmental stage influence spawning-site choice by female garibaldi. Anim. Behav. 38 (3), 447–456. doi: 10.1016/S0003-3472(89)80038-7
Sikkel P. C. (1994). Why female garibaldi prefer males with young eggs: A test of the parental investment hypothesis. Ethol. Ecol. Evol. 6 (2), 191–211. doi: 10.1080/08927014.1994.9522994
Sikkel P. C. (1995). Effects of nest quality on male courtship and female spawning-site choice in an algal-nesting damselfish. Bull. Mar. Sci. 57 (3), 682–689.
Silge J., Chow F., Kuhn M., Wickham H. (2021). in Rsample: General resampling infrastructure. R package version 0.0.9.
Stephens J. S., Morris P. A., Pondella D. J., Koonce T. A., Jordan G. A. (1994). Overview of the dynamics of an urban artificial reef fish assemblage at King Harbor, California, USA 1974-1991: A recruitment driven system. Bull. Mar. Sci. 55 (2-3), 1224–1239.
Stephens J. S., Pondella D. J. II (2002). Larval productivity of a mature artificial reef: the ichthyoplankton of King Harbor, California 1974-1997. ICES J. Mar. Sci. 59, S51–S58. doi: 10.1006/jmsc.2002.1189
Tanner S. E., Vieira A. R., Vasconcelos R. P., Dores S., Azevedo M., Cabral H. N., et al. (2019). Regional climate, primary productivity and fish biomass drive growth variation and population resilience in a small pelagic fish. Ecol. Indic. 103, 530–541. doi: 10.1016/j.ecolind.2019.04.056
Taylor B. M. (2013). Drivers of protogynous sex change differ across spatial scales. Proc. R. Soc. B: Biol. Sci. 281 (1775), 20132423. doi: 10.1098/rspb.2013.2423
Taylor B. M., Choat J. H., DeMartini E. E., Hoey A. S., Marshell A., Priest M. A., et al. (2019). Demographic plasticity facilitates ecological and economic resilience in a commercially important reef fish. J. Anim. Ecol. 88 (12), 1888–1900. doi: 10.1111/1365-2656.13095
Taylor B. M., Lindfield S. J., Choat J. H. (2015). Hierarchical and scale-dependent effects of fishing pressure and environment on the structure and size distribution of parrotfish communities. Ecography 38 (5), 520–530. doi: 10.1111/ecog.01093
Taylor B. M., McIlwain J. L. (2010). Beyond abundance and biomass: effects of marine protected areas on the demography of a highly exploited reef fish. Mar. Ecol. Prog. Ser. 411, 243–258. doi: 10.3354/meps08672
Tickell S., Sáenz-Arroyo A., Milner-Gulland E. J. (2019). Sunken worlds: The past and future of human-made reefs in marine conservation. BioScience 69 (9), 725–735. doi: 10.1093/biosci/biz079
Trip E. D. L., Choat J. H., Wilson D. T., Robertson D. R. (2008). Inter-oceanic analysis of demographic variation in a widely distributed indo-pacific coral reef fish. Mar. Ecol. Prog. Ser. 373, 97–109. doi: 10.3354/meps07755
Trip E. D. L., Clements K. D., Raubenheimer D., Choat J. H. (2014). Temperature-related variation in growth rate, size, maturation and life span in a marine herbivorous fish over a latitudinal gradient. J. Anim. Ecol. 83 (4), 866–875. doi: 10.1111/1365-2656.12183
Trip E. D. L., Clements K. D., Raubenheimer D., Choat J. H. (2016). Temperate marine herbivorous fishes will likely do worse, not better, as waters warm up. Mar. Biol. 163, 24. doi: 10.1007/s00227-015-2790-x
Tzioumis V., Kingsford M. J. (1999). Reproductive biology and growth of the temperate damselfish Parma microlepis. Copeia 1999, 348–361. doi: 10.2307/1447480
Williams C. M. (2021). Spatial and habitat variation in life history of the Garibaldi, Hypsypops rubicundus, including validation of annual growth increments (Pomona: California State Polytechnic University).
Williams J. P., Allen L. G., Steele M. A., Pondella D. J. (2007). El Niño periods increase growth of juvenile white seabass (Atractoscion nobilis) in the Southern California Bight. Mar. Biol. 152 (1), 193–200. doi: 10.1007/s00227-007-0673-5
Williams J. P., Claisse J. T., Pondella D. J., Medeiros L., Valle C. F., Shane M. A. (2012). Patterns of life history and habitat use of an important recreational fishery species, spotfin croaker, and their potential fishery implications. Mar. Coast. Fish. 4 (1), 71–84. doi: 10.1080/19425120.2012.661392
Keywords: artificial reefs, southern California, fish life history, growth patterns, spatial variation, Garibaldi, Hypsypops rubicundus
Citation: Williams CM, Eagleton JL, Pondella DJ II and Claisse JT (2022) Habitat type and environmental conditions influence the age and growth of a temperate marine damselfish. Front. Mar. Sci. 9:988158. doi: 10.3389/fmars.2022.988158
Received: 07 July 2022; Accepted: 09 September 2022;
Published: 06 October 2022.
Edited by:
Bernardo Antonio Perez Da Gama, Fluminense Federal University, BrazilReviewed by:
Anthony Fowler, South Australian Research and Development Institute, AustraliaSahar Fahmy Mehanna, National Institute of Oceanography and Fisheries (NIOF), Egypt
Copyright © 2022 Williams, Eagleton, Pondella and Claisse. This is an open-access article distributed under the terms of the Creative Commons Attribution License (CC BY). The use, distribution or reproduction in other forums is permitted, provided the original author(s) and the copyright owner(s) are credited and that the original publication in this journal is cited, in accordance with accepted academic practice. No use, distribution or reproduction is permitted which does not comply with these terms.
*Correspondence: Chelsea M. Williams, Y211bm96QG94eS5lZHU=