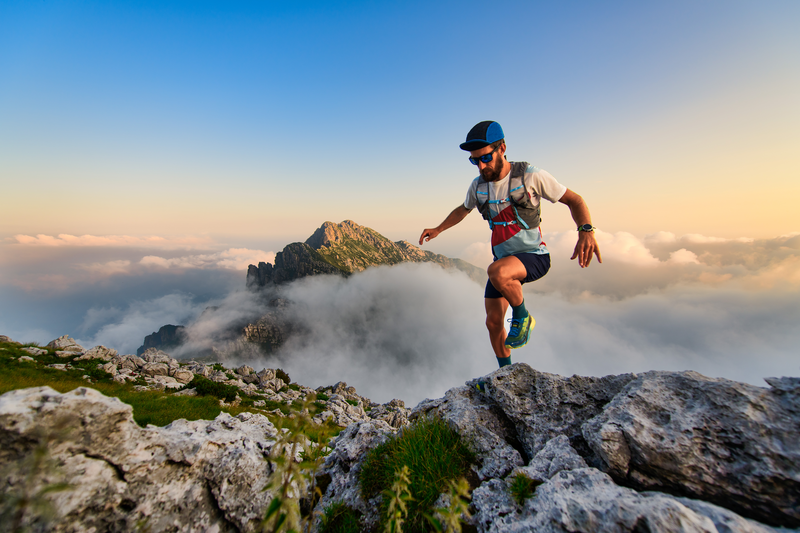
95% of researchers rate our articles as excellent or good
Learn more about the work of our research integrity team to safeguard the quality of each article we publish.
Find out more
TECHNOLOGY AND CODE article
Front. Mar. Sci. , 08 September 2022
Sec. Ocean Observation
Volume 9 - 2022 | https://doi.org/10.3389/fmars.2022.986752
This article is part of the Research Topic Ocean Observation based on Underwater Acoustic Technology View all 20 articles
Advancements in technologies have led to a rapid development of unmanned surface vehicles (USV) for marine ecosystem monitoring. The design, size, and scientific payload of the USVs differ as they are built for different purposes. Here, we present the design criteria and detailed technical solutions of a prototype USV which has been built to fulfill the following experimental and operational needs; the USV should be used for inshore and shallow water acoustic monitoring, offshore comparison of echo sounder recordings from the USV and research vessels, monitor natural fish schooling behavior and seabird-fish behavioral interactions. The prototype has been built over a period of 5 years with steadily quality improvements. As the hull is based on an expedition double kayak, the USV is named Kayak Drone, and we aimed at building the Kayak Drone using of-the-shelf hardware and existing open-source software. This allowed for the development of a modular and well-functioning USV at a relatively low cost. The Kayak Drone produces very little noise and in situ experiments show that the Kayak Drone can record echo sounder data of fish near the surface without disturbing their natural distribution and behavior. One in situ study shows that the Kayak Drone could navigate within a couple of meters from swimming puffin and other seabirds without triggering escape. These results demonstrate that the Kayak Drone can be utilized to produce unbiased survey estimates for fish distributed in shallow waters and near the surface, which is very important for many fish stock assessments and managements. Furthermore, it can also be used as a tool to observe the predation by seabirds on fish schools without interfering with their natural interspecific behavior, which traditionally has been very difficult. The use of the Kayak Drone is not restricted to these tasks, and we foresee that the Kayak Drone can be utilized in many different experiments where a silent platform is needed.
Worldwide, research vessel surveys have been crucial for monitoring marine ecosystems and for collection of information to assess the state of commercially important fish stocks (Gunderson, 1993). This traditional survey concept (Gunderson, 1993; Simmonds and MacLennan, 2008) has in recent years undergone a transition to also include additional platforms (Verfuss et al., 2019). Advancements in software, computers, sensors, and battery technologies have led to the development of a variety of unmanned surface vehicles (USV) for marine ecosystem monitoring. The USVs are partly replacing traditional tasks carried out by research vessels (De Robertis et al., 2019), but are more frequently used for other purposes (Shao et al., 2019; Chang et al., 2021) or as a supplement to traditional research vessels (Kum et al., 2020). With climate changes and other human induced stressors on the marine ecosystems, there is an increased need for more continuous and widespread monitoring, and it is highly likely that USVs will play a more important role in future data collection. The transition is ongoing, and several studies have tested USVs equipped with different sensors, and in a few cases the USVs are an integrated part of annual survey programs (De Robertis et al., 2019).
When USVs are used for abundance estimation and mapping of zooplankton and fish densities, they are usually equipped with scientific echo sounders. These echo sounders can be of the same type as installed on a research vessel, or of a more power efficient type (Benoit-Bird et al., 2018). Traditional use of echo sounders for fisheries research have been dependent on net-sampling for identification of the targets observed on the echograms. Such net-sampling requires relatively large, manned vessels, but more advanced multifrequency and broadband echo sounders together with deep learning acoustic classification may make trawling less important for identification of acoustic targets in the future (Johnsen et al., 2009; Brautaset et al., 2020; Handegard et al., 2021). Smaller unmanned vehicles can be used when regular biological sampling is of less importance or if samples can be obtained from fishing vessels or landing sites in the area. Nevertheless, the acoustic data format from the USVs is the same as recorded from a ship and makes comparison with data from research vessels feasible. This is important for the continuation of valuable survey time series that are repeated annually following standard protocols.
A long list of USVs for marine ecosystem monitoring have been developed in recent years and the range of purposes and designs are extensive (Othman, 2015). Some are designed for shallow waters such as the CatOne family (Romano and Duranti, 2012), whereas others are designed preliminary for ocean operations, e.g., Saildrone (De Robertis et al., 2019), Sailbouy (Ghani et al., 2014) and Kongsberg Sounder (Handegard et al., 2021b). The size ranges from the handheld USVs to the 22 m long Saildrone Surveyor USV (An et al., 2021). The prolusion may be sail, wave motion, underwater or air fan propeller, or a combination of these.
The scientific purposes affect the design of the USV, and our aim here is to present a new multipurpose prototype, the Kayak Drone USV, which was built to address a list of defined scientific challenges (Table 1). A detailed technical description of the Kayak Drone system is presented together with examples of research experiments carried out using the Kayak Drone. The paper is structured in scientific purposes, vehicle design, technical solutions, and examples of experimental field studies. We discuss advantages and limitation of the Kayak Drone and the future contribution of the Kayak Drone to marine research.
The design of the Kayak Drone was defined in accordance with a list of research questions and purposes as presented in Table 1. The addressed research challenges required a silent acoustic platform to observe undisturbed densities and natural behavior of fish, marine organisms, and seabirds, without affecting the targets of interest. The main payload is broadband scientific echo sounders, and the acoustic signal to noise ratio was required to be low to enable recording of high-quality data from small organisms in the deep.
The Kayak Drone is primarily made for small scale experimental studies and not as a platform for large scale extensive surveys. To fulfill the different experimental and operational needs, two operation modes for the USV were included; autonomously following waypoints defined in a chart, and real-time remote-control operation. The hull should be small enough to enable high maneuverability close to the shore and easy transport on a boat trailer to various experimental sites. It was a criterium that the Kayak Drone could be launched both from the shore and from a research vessel during moderate sea states.
To study potential behavioral effects of a research vessel on the marine organisms, the USV had to have a maximum speed similar to the standard survey speed of a research vessel (10 knots), thus enabling it to run in front or behind the research vessel during standard operation. For other experiments, a speed of about 5 knots is acceptable and we aimed at having enough battery capacity for an operation time of around 10 hours at a speed of 5 knots. It was furthermore important to make a flexible and adaptive system design that allowed for easy alteration of the payload and implementation of additional or alternative sensors and their associated software.
During the building of the Kayak Drone, it was important to utilize available open-source software and off-the-shelf products as far as possible to reduce the costs, development time, and to increase the technical reliability. Reaching scientific goals was the main aim (Table 1), rather than the development of complex and novel technology.
We purchased an existing and well-tested kayak hull after a review process using experts in kayaking. To satisfy the design criteria on speed, seaworthiness, and payload, we decided to modify a 6.7 m long, well-tested Triton expedition double kayak from Sea Kayaking UK as the basis for the USV (Figure 1). The total weight of the kayak, motor, batteries, and instrumentation is approximately 250 kg. The standard cockpits were converted to watertight chambers with lids. A well was made close to the stern where the propulsion motor is mounted, and a retractable keel was installed midships for acoustic transducers and to improve the sideway stability. The center board of the keel runs through a sheath in the hull and can be bolted in various draft positions (Figure 1). At the lower end of the keel there is a bulb, which houses the echo sounder transducers and lead weights (Figure 1). During survey operations, the adjustable keel is lowered to a bulb depth of 0.5 to 1.5 m below the sea surface. Air bubbles in the upper surface waters can attenuate or even block the acoustic signals, and lowering the transducers reduces this problem (Dalen and Løvik, 1981). During transport, the center board of the keel is fully retracted with only the bulb extending below the vehicle hull. A modified car trailer is used for transport, as a cradle onboard the research vessel and as a slipway during deployment from shore.
A Torqeedo Cruise 2.0 RS electric outboard motor (Figure 1) is used for propulsion, which provide a maximum speed of almost 9 knots (Table 2). This is in line with the defined speed criteria of the USV. The engine is installed with a large propeller (30 cm diameter) mounted directly on the motor shaft, giving a high energy efficiency. The large propeller and high torque provide a low RPM (revolutions per minute) and a low noise level. In combination with the slim shape of the kayak hull, which produce a very low hydrodynamic pressure field, the critical design criterion of a silent vehicle within the audible and sensory frequency range of the target organisms was achieved. One marinized Torqeedo LiIon battery (Power 26-104) is the power source for the engine. The USV is designed to house two such batteries, potentially doubling the current operation time of about 6 hours with an average speed of 5 knots (Table 2). In agreement with the Torqeedo company, we got access to the proprietary data protocols of the internal Torqeedo network, which allowed the USV software to alter the engine RPM and control the engine battery.
A Faulhaber brushless DC servo motor with position feedback (hall sensors) was marinized and attached to the propulsion engine to adjust rudder angle (Figure 1). Existing screw holes on the propulsion motor were used for attachment of the rudder motor and a cog. The corresponding cog on the rudder motor shaft transferred rudder positioning. Accurate control of rudder angle was achieved due to a Faulhaber 1:531 planetary gear attached to the servo motor. The rudder motor is powered by the Torqeedo battery. The charging time for fully discharged batteries is approximately 5 hours (Table 2).
The main scientific payload of the Kayak Drone is currently a SIMRAD EK80 broad band echo sounder (Demer et al., 2017) with a SIMRAD ES200-7C 200 kHz split-beam transducer mounted on the retractable keel (Figure 1). The full echo sounder system consists of the transducer, a SIMRAD Wide Band Transceiver (WBT) and the EK80 SIMRAD software installed on a ruggedized industrial Windows computer placed in the cockpit chamber in front of the keel. There is free space in the bulb of the keel for an additional transducer. A scientific echo sounder can measure small organisms at great distances despite the week return echoes by amplifying the incoming signal. This makes the echo sounder vulnerable to noise in the frequency range of operation, and electric noise from other equipment may be detected as input signals. High-frequency signal noise is a well-known problem which can corrupt the acoustic recordings of an echo sounder (Mitson and Knudsen, 2003). Therefore, the echo sounder, computer and other sensors are powered by their own set of lead gel-batteries, separate from the power source of the propulsion and rudder motors. To further reduce the risk of electric noise from the motors, communication between the computer and motors goes through galvanically shielded adapters. Furthermore, the echo sounder and transducer cabling is situated in a compartment away from other electronics. The chassis of the echo sounder is grounded to sea and the transducer cable is shielded.
A small vector compass containing two GPS receivers is mounted on a bracket above deck (Seapilot Vector Compact GPS Compass) (Figure 1). In addition to standard GPS navigation telegrams, the unit provides accurate heading information unaffected by magnetic fields, and information on roll, pitch and heave is also provided.
To increase navigation safety, a class B AIS transceiver is installed, which makes the Kayak Drone visible in other vessels’ charts and in online ship maps (e.g. Marine Traffic). In the Kayak Drone chart program (OpenCPN) ships with AIS is visualized.
The compartments of the USV have leakage sensors, and alarms are visible in the control application. Independent GoPro video cameras are normally mounted on deck and on the keel bulb during experiments.
The Kayak Drone can operate autonomously based on a preplanned survey route without real-time connection. However, during most experiments the distance between the USV and a mother-vessel is within visual distance. As the vehicle has no anti-collision system installed, the operation requires continuous access control of the USV in coastal and heavily trafficked waters. This is less important during operations in high seas. Furthermore, several of the studies require a real time observation of the echogram. A VNC connection (open-source program TightVNC version 4.8.8) gives remote access to the Kayak Drone computer via a radio link. Traditionally, when two communicating units are moving independently from each other, omnidirectional antennas are used. This setup has challenges such as short range and unstable communication. Initially, we had a system with an omnidirectional antenna on the USV, and a four-sector directional and switching antenna on the mother vessel. Due to frequent communication breakdowns and a too short range, the original wireless link system was replaced by Kongsberg Marine Broadband Radios (MBR) (Ludvigsen et al., 2016). MBR radios are automatically steerable, phased array radios, that directs the antenna beam towards the node it is communicating with, both when transmitting and receiving. The phase steering or beam forming is done electronically without any moving parts. This technology has several advantages compared to an omnidirectional solution. Due to the narrow lobe, the system is robust towards unwanted external interference and noise. The transmit and receive gains are both high, thus giving a very long range. The electronic phase-controlled communication allows for smooth communication between nodes. Several nodes can be appended to an MBR network, but in the Kayak Drone system, we currently have only two nodes, the USV itself, and the mother vessel. Depending on the required communication range, three sizes of radios with integrated antennas can be chosen. For the USV and the mother vessel, we have so far used the smallest (Figure 1) and the intermediate radios, respectibly. The radio onboard the drone has an option to directly connect a camera and include live video streaming onto the MBR network. On the mother vessel or from a land station, the virtual computer screen on board the Kayak Drone can be seen, and keyboard and mouse signals are transmitted using a VNC solution (TightVNC). This makes the Kayak Drone very flexible and new sensors and software may be installed in the Kayak Drone without altering the overall system. The maximum range of the radio link has not yet been tested. The USV was 1400 m away from the mother vessel during one of the experiments, and the connection was still flawless.
Two internal software programs on the USV computer work in a joint effort to operate the drone (Figures 2, 3). These are the open-source chart plotter navigation program, OpenCPN (https://opencpn.org), and the self-made EchoDrone program developed in Labview version 2013.
Figure 2 Overview of how the OpenCPN and EchoDrone programs share the task of controlling the Kayak Drone. The data flow between the two programs is shown as well as external sensors inputs and data outputs. Lat (latitude), Lon (longitude), HDG (heading), BNG (bearing), SOG (speed over ground), RNG (range), ETA (estimated time of arrival), XTE (cross track error), MBR (Maritime Broadband Radio), GPS (Global Positioning System) and AIS (Automatic Identification System), _USV (real time navigation data on the USV), _WP (navigation data on the active, next waypoint on the route), _Ext._Vessels (any vessel with AIS and withing range).
Figure 3 Illustration of the autonomous Kayak Drone navigation operation. A survey route with several legs (red arrows) are activated (similar to what is shown in the OpenCPN map). The Kayak Drone (red vessel) is sailing towards the next waypoint destination (WP_destination) marked as a red circle with yellow fill. Inactive waypoints are shown as blue diamonds. During operation, a set of calculations are continuously performed by OpenCPN and EchoDrone, and data are exchanged between the two programs. The calculation steps and resulting actions are described on the right side of the Figure. Note that the calculated WP_aim is used to compensate for drift due to wind and currents, which may result in a cross track error distance (XTE_Dist) to the left or right (XTE_LR) of the Active_leg. WP, way point; HDG, heading; XTE_Dist, cross track error (distance from active leg) and whether the USV is to the left or right side of the active leg (XTE_LR).
The two programs interact and solve various tasks such as navigation, autonomous operation, sensor data reading and storage, handling error messages and navigational warnings, and control of the propulsion and rudder. The EchoDrone reads data from the GPS vector compass (latitude, longitude, UTC time, true heading, tilt, roll and heave, speed over ground, coarse over ground) and AIS before transmitting the data onto the USV intranet. OpenCPN reads from this Ethernet and displays information in the chart (see Figures 2, 4), calculates range and bearing to the next waypoint, cross track error and estimated time of arrival. EchoDrone reads these calculated navigation data sent from OpenCPN for the active waypoint. Based on this information, the rudder motor is commanded to set the rudder to an appropriate angle for the Kayak Drone to steer towards the next waypoint (Figure 3). In default operation mode, the user sets up a route with waypoints in the OpenCPN chart via the remote radio link and VNC. Once the route and the first waypoint are activated, the USV can be set to automatically follow it. Once the active waypoint is reached, OpenCPN will automatically switch and activate the next waypoint on the route and send out new relevant navigation data. The USV will automatically stop when it reaches the last waypoint on the route. It is however possible to make a closed route that forms an eternal loop. The throttle and thereby the vessel speed is set in the EchoDrone front panel by the remote operator.
Figure 4 The Kayak Drone PC screen as seen in real time by the controller via the VNC radio link. To the left is the OpenCPN chart program. The route is indicated by consecutive red arrows. Next waypoint is indicated by a red circle with yellow fill. The drone is shown as a red ship and the actual sailed track of the drone is shown as a blue curved line. In the middle of the screen is the EchoDrone USV control software. To the right is the echo sounder software showing a school of sprat in shallow waters close to the current position near the shore. .
The integration of OpenCPN into the drone system plays a central role in the overall design. Taking advantage of all the functionality in OpenCPN and integrate its navigational outputs, has significantly reduced the development time for the USV and consequently also the costs. It is highly unlikely that the Kayak Drone could have been developed to the current advanced operational level without OpenCPN, as advanced charts operations and visualization are vital, especially in coastal areas and narrow fjords. All this to the credit of the many developers of this open-source application. OpenCPN chart program can read and display various sea chart formats. The chart forms the basis for all OpenCPN operations. The project has access to high accuracy s-57 format charts (Figure 4) from the Norwegian Mapping Authorities. These high-resolution maps are a prerequisite for Kayak Drone experiments in coastal areas and narrow fjord systems as described in the design criteria for the USV. The Kayak Drone and its track is shown in the OpenCPN map together with the route and waypoints (Figure 4). At any given time, the operator onboard the mother vessel may modify or make a new route.
In manual operation mode, the user can control the rudder angle through buttons in the EchoDrone front panel. The battery status is also read and displayed in the EchoDrone program. The EchoDrone program continuously write time tagged sensor data and operational parameters to a log file. The program can be set in replay mode and will read data from a previous and actual survey at sea. This option has been useful for system development in the lab, as well as debugging and improvement of the navigation algorithms in hindsight, in the office. The behavior of the EchoDrone program is governed by close to 100 parameters. These are stored in an ASCII parameter file and can be edited by the user. The parameter file is read by EchoDrone during initiation.
A remote Labview program, “Vessel”, may optionally be run on the mother vessel or at the command location on shore. In this program, a handshake option may be activated. Both the Vessel software operated by the controller and the EchoDrone software in the USV send and receive UDP ethernet telegrams via the MBR communication link at an interval of one second. If the remote communication link is lost, the USV will no longer receive handshake telegrams from the vessel, and propulsion is halted after a given time delay. The Vessel program can also automatically re-establish the VNC connection when the communication link is back and handshake telegram exchange resumes.
For specific experiments, the Vessel application can read GPS positions and transmit this as a moving target waypoint for the USV. This may typically be used to position the USV at a fixed distance and bearing relative to the mother ship to examine vessel avoidance reactions of fish to a large research vessel.
There are several challenges with traditional vessel based acoustic surveys relating to vessel avoidance, near sea-surface acoustic blind zone, cost of operation and full coverage in shallow water and near the coastline. The Kayak Drone has been used in several scientific studies relating to these challenges, and here we briefly present preliminary results as illustrated by three different experiments carried out with the Kayak Drone.
Sprat (Sprattus sprattus) is a small clupeid species with a wide distribution. It is an important key species in the ecosystems of the Norwegian fjords (Bakken, 1973). For almost five decades, changes in the biomass of sprat in these fjords have been monitored with acoustic trawl surveys carried out by research vessels. There has been a growing concern that a considerable and variable fraction of the sprat population have not been accessible for acoustic monitoring as the sprat may be distributed very close to the shore and the sea surface. To examine the full vertical and horizontal distribution of sprat in a fjord arm (Årdalsfjord) of Sognefjord, the Kayak Drone was used (Figure 5) in August 2020. The fjord arm was covered once at night and once during daytime, running zigzag transects (see survey report Johnsen et al., 2020). In each coverage, the Kayak Drone sailed in front of RV Kristine Bonnevie with a distance of at least 500 m. Echo sounder data were collected continuously from the two platforms and the data were analyzed using identical methods. The transducer depths were ~1.2 m and ~6 m, respectively, on the Kayak Drone and the RV Kristine Bonnevie.
Figure 5 The Kayak Drone in operation during the sprat acoustic survey in Årdalsfjorden, Norway, 2020.
The mean acoustic density measured from the Kayak Drone was more than three times higher the density measured with the vessel, and the Kayak Drone measured approximately the same density of sprat in both coverages. RV Kristine Bonnevie measured about 40% higher mean density during daytime than at nighttime. As expected, the vertical density distribution of sprat was considerably closer to the sea surface at night where about 65% of the density was found shallower than 8 m (Figure 6), which is approximately the upper depth from which the echo sounder on the large vessel can detect fish. During the day coverage, 30% of the fish density was found above 8 meters (Figure 6). In addition, during night many schools of sprat were found closer to the shore than the large vessel can navigate safely (Johnsen et al., 2020). Clearly, the Kayak Drone data shows that a high and variable fraction of the sprat density is not available during standard acoustic surveys using large research vessel. The large difference in diel vertical distributions and day-night density measurements made by the research vessel show that the standard survey estimates are biased as the fjord surveys are carried out day and night.
Figure 6 The echo density of sprat by depth, measured from the Kayak Drone and the research vessel Kristine Bonnevie (Årdalsfjorden, Norway, August 2020).
It is well known that many fish species are very sensitive to approaching research vessels, and therefore may lead to biased survey estimates (De Robertis and Handegard, 2013). The vessel avoidance of the Norwegian spring spawning herring (Clupea harengus), which is one of the largest fish stocks in the North Atlantic (Devold, 1963), is well documented (Vabø et al., 2002; Ona et al., 2007). Despite large scientific effort to overcome the source of error caused by the blind zone and vessel avoidance, neither advance modelling of fish behavior (Vabø and Nøttestad, 1997) nor the development of 3D sonars for near surface monitoring (Holmin et al., 2016) have succeeded. In November 2020, we investigated the avoidance of the herring during their overwintering in fjords in northern Norway. The test site is very good as the herring is responsive to noise in this period due to large predation by whales (Vogel et al., 2021). The behavior is predictable and makes the experimental setup relatively simple as the herring emerges close to the surface at night, in a large dense layer (Ona et al., 2007).
In our experiment, we anchored an upward facing echo sounder below the herring layer and continuously monitored the behavior and backscattering strength of the herring layer at night without disturbing their natural behavior. We identified the exact position the upward facing echo sounder and repeatedly sailed over this position with the Kayak Drone, and later with a large research vessel G.O. Sars (Gross Registered Tonnage (GRT) 4067 tons, Length Overall (LOA) 77,5m). The upper part of the herring layer was about 20 m below the sea surface (Figure 7). The herring did not show any avoidance reaction when the Kayak Drone passed over the upward facing echo sounder (Figure 7). In contrast, the herring showed a strong diving response when the research vessel passed (Figure 7). The latter is in accordance with results previously presented by Ona et al. (2007). Clearly, surveys carried out by the Kayak Drone will lead to more accurate survey estimates as strong vessel avoidance of fish affects the survey estimate accuracy. The horizontal escapement reduces the number of individuals observed in the acoustic beam and the diving fish has a tilt angle that results in a reduced acoustic backscatter compared to fish with natural behavior (Vabø et al, 2002).
Figure 7 Echograms from an upward looking echo sounder mooring when the Kayak Drone (A) and a research vessel (B) pass over the mooring. A dense layer of herring can be observed from 20 to 40 meters below the surface. Below the herring layer, dispersed individual fish can be seen. Air bubbles from the propeller of the vessel (B) can be seen down to approx. 10 meters depth, while no bubbles are produced by the Kayak Drone (A). The time of passing the mooring is shown as vertical black lines.
The noise produced by the USV, and the research vessel (with diesel electric propulsion) was measured in situ following ANSI S12.64-2009 (Grade C) (ANSI/ASA, 2009). A single hydrophone (Ocean Instruments Soundtrap HF) was deployed at 36.4 m depth on a bottom anchored mooring, which corresponds to a 20-degree angle from the hydrophone to the point of closest approach of the vessels (100 m from the surface above the hydrophone (see Figure 2 in ANSI S12.64-2009). Both the USV and the research vessel performed multiple measurement runs (port and starboard). Using the received sound pressure levels between 50 to 10 000 Hz, and assuming geometric sound propagation only, the average difference in source levels was estimated, with the USV source level being significantly lower than the source level of the research vessel (~ -37 dB re 1 μPa at 1m).
It is difficult to observe the predation by seabirds on of fish schools without interfering with their natural interspecific behavior (Safina and Burger, 1988; Hentati-Sundberg et al., 2018), and there is a need to develop methods to study such fine-scale predator–prey patterns (Hentati-Sundberg et al., 2018). As presented above, several experiments shows that the Kayak Drone can observe fish near the sea surface using echo sounders without affecting their natural behavior. To test the avoidance reaction of swimming seabirds such as puffin (Fratercula arctica), common guillemot (Uria aalge) and razorbills (Alca torda) to the Kayak Drone, an experiment was carried out near the bird cliffs at the island of Runde, Norway, May 2018.
A Gopro camera mounted on the antenna mast was used to observe the swimming seabirds, and the echo sounder was set to record fish aggregations and diving birds. By following the instructions of the local ornithologists, the Kayak Drone was steered slowly (~2 knots) into the aggregations of seabirds. No reaction was observed from the birds until the USV was about 2 meters away (Figure 8). Then the seabirds started swimming fast away from the approaching Kayak Drone. Observing diving seabirds targeting fish was also an objective in this experiment, but little fish and no diving birds were acoustically observed. Furthermore, the video recordings did not show any seabird with fish in their beaks. According to the experts, the non-feeding behavior was not very surprising as e.g. puffins typically aggregate near the cliffs in the afternoon before they return to their burrows at sunset. Nevertheless, the results are promising as the Kayak Drone seems to be applicable when observing seabird-fish interaction without affecting their natural behavior.
The Kayak Drone project started with some scientific questions that could not easily be answered using traditional vessels or measurement platforms. To address these scientific objectives, at set of requirements and design criteria was listed (Table 1) and the Kayak Drone was built accordingly. The Kayak Drone is a very silent, fast-moving platform that supports high quality data collection from scientific broadband echo sounders. It can operate with high position accuracy in narrow fjords, lakes, shallow coastal waters and in open sea during favorable sea states. To do so, electric propulsion and battery power is required. Long-time survey operations covering large areas is therefore not within the scope. That USV niche is better covered by sail drones or petroleum powered USVs. The results from several experiments, shows that the Kayak Drone has answered the defined scientific needs. It is now in regular use and operates without any major technical problems.
The modular concept with regards to hardware and software design turned out to be important as is it difficult to foresee all future needs and challenges during the planning phase of such a project. Since the first sea trial in February 2018, the performance of the Kayak Drone has been considerably improved as a result of lessons learnt during sea operations. We gained practical experience with the USV during field experiments, leading to improvements in the control software, introduction of high accuracy maps and new compartment lids that does not leak. These upgrades have, together with a number of minor technical improvements, advanced the system to its current well-functioning level. One of the most significant upgrades was the installation of the MBR radio link which substantiality improved the stability of the remote communication. Thereafter, the USV has performed as originally intended, and without any significant challenges during surveys.
The Kayak Drone was not design as a prototype for future mass production, however, there is some lessons learnt that may be useful for others. The inclusion of the open-source program OpenCPN as a key component in the system, is probably the most consequential decision that we made. We regard this as a great success, and it has allowed safe USV operations in narrow fjords by using high resolution sea charts. The project would not have had the resources to develop a self-made, high quality chart system as an integrated part of the USV control program.
Despite the successful use of the Kayak Drone, it still has weaknesses and a potential for improvement. Upgrading and improvement of the current USV could include a more hydrodynamic keel/bulb to reduce drag, increase speed and make the USV more energy efficient. Some air bubbles also seem to originate from a sub-optimal keel design. These bubbles interfere negatively on the acoustic recordings. The GoPro cameras could be replaced by integrated MBR cameras to allow real time video streaming. The weight of the USV could be reduced and operation time increased if the batteries were shifted to more modern types. There is also space for more batteries than currently installed. For the analysis of the acoustic recordings, an additional echo sounder operating at a different frequency would enhance the scientific outcome. When exposed to large ocean waves, the roll becomes evident on the echograms as changes in density of school registrations. This could be mitigated by mechanical transducer gimbles, thus improving the quality of the acoustic recordings. It has been a challenge that the fiber glass hull is vulnerable to damage during retrieval in open sea and large waves. Cracks in the hull sometimes occur with consequential insignificant leakage. On the other hand, it is easy to repair, and the leakage alarms give a good real time overview of the situation. To mitigate this problem, a new hull might be built in a more rugged material than fiber glass.
The field experiments with the Kayak Drone have clearly shown that some types of marine science surveys cannot optimally be conducted by large research vessels. Their size may prevent adequate sampling of the target population as the acoustic sensor is mounted too deep, or because the vessel cannot navigate into the shallow habitats. In regions of the world with gradually sloping bottom from deep to shallow waters, this may negatively influence the research vessel’s coverage of some target species and their distribution. For abundance estimation surveys it is a prerequisite that the survey brackets the target population (Gunderson, 1993), this is not always possible with large traditional research vessels. The field results have been very promising and support the assumption that USV technologies will become important new tools that will advance marine research in the future. The silent acoustic Kayak Drone has produced novel results within some fields of marine research. From the Kayak Drone experiments, new knowledge has been derived that possibly will lead to alteration of the current survey design for the Norwegian sprat monitoring.
Sometimes new tools provide unexpected observations. In the seabird-fish interaction experiment, several bird species showed almost no avoidance from the Kayak Drone, however, we observed that the northern gannets (Morus bassanus) circled the sky above the USV. It seems like the Kayak Drone acted as an aggregating device for the gannets. In another experiment on the sandeel grounds in the North Sea we carried an experiment in 2021 with the objective of measuring sandeel near the sea surface. From the GoPro video recordings on the keel, we observed a minke whale (Balaenoptera acutorostrata) that approached below the Kayak Drone three times over a period of 15 minutes. This may have been a random event, but such observations are nevertheless worth some reflection. A silent measuring platform may attract some species rather than scaring them away. In either case, a silent platform may be well suited to observe natural behavior of some species, but not necessarily others.
The raw data supporting the conclusions of this article will be made available by the authors upon request, without undue reservation.
EJ has been the project leader and responsible for defining the scientific requirements that the USV needed to meet the research goals. In addition, he is responsible for all the experimental survey designs. AT has been responsible for the technical design and construction of the USV. EJ and AT have authored the manuscript in a joint effort. All authors contributed to the article and approved the submitted version.
Norwegian Research Council, project number 243941/E40. Center for Research-based Innovation in Marine Acoustic Abundance Estimation and Backscatter Classification (CRIMAC; number 309512). Nordic Council research project, Hydroacoustic, project number 169 (2017). Internal research projects at Institute of Marine Research, Norway.
The authors want to thank Torqeedo GmbH, Germany for giving us access to the internal data protocols for their propulsion system. Nigel Dennis at Sea Kayaking UK and Ronny Riise at God Tur, Norway made the necessary rebuilding of the standard Triton kayak hull. Harald Fitje at IMR Norway made the housing and adaptation of the rudder motor. Kongsberg Seatex and Kongsberg Simrad have given us invaluable assistance throughout. Furthermore, Runde Environmental Centre and Cecilie Kvamme, IMR have invited us to participate in their field activities, and Geir Pedersen provided the noise measurement results from the herring experiments.
The authors declare that the research was conducted in the absence of any commercial or financial relationships that could be construed as a potential conflict of interest.
All claims expressed in this article are solely those of the authors and do not necessarily represent those of their affiliated organizations, or those of the publisher, the editors and the reviewers. Any product that may be evaluated in this article, or claim that may be made by its manufacturer, is not guaranteed or endorsed by the publisher.
ANSI/ASA (2009). “ANSI S12.64-2009,” in Quantities and procedures for description and measurement of underwater sound from ships–part 1: General requirements” (New York: American National Standards Institute/Acoustical Society of America).
An Y., Yu J., Zhang J. (2021). Autonomous sailboat design: A review from the performance perspective. Ocean Engineering. 238, 109753. doi: 10.1016/j.oceaneng.2021.109753
Bakken E. (1973). Sprat in Norwegian waters, a short review of biology, fishery and current research. Coun. Meet. Int. Coun. Explor Sea, 1–13.
Benoit-Bird K. J., Patrick Welch T., Waluk C. M., Barth J. A., Wangen I., McGill P., et al. (2018). Equipping an underwater glider with a new echosounder to explore ocean ecosystems. Limnol. Oceanog.: Methods 16 (11), 734–749. doi: 10.1002/lom3.10278
Brautaset O., Waldeland A. U., Johnsen E., Malde K., Eikvil L., Salberg A. B., et al. (2020). Acoustic classification in multifrequency echosounder data using deep convolutional neural networks. ICES J. Mar. Sci. 77 (4), 1391–1400. doi: 10.1093/icesjms/fsz235
Chang H. C., Hsu Y. L., Hung S. S., Ou G. R., Wu J. R., Hsu C. (2021). Autonomous water quality monitoring and water surface cleaning for unmanned surface vehicle. Sensors 21 (4), 1102. doi: 10.3390/s21041102
Dalen J., Løvik A. (1981). The influence of wind-induced bubbles on echo integration surveys. J. Acoustical Soc. America 69 (6), 1653–1659. doi: 10.1121/1.385943
Demer D. A., Andersen L. N., Bassett C., Berger L., Chu D., Condiotty J., et al. (2017). evaluation of a wideband echosounder for fisheries and marine ecosystem science. In: ICES cooperative research report. Available at: https://archimer.ifremer.fr/doc/00585/69730/67619.pdf (Accessed May 23, 2022).
De Robertis A., Handegard N. O. (2013). Fish avoidance of research vessels and the efficacy of noise-reduced vessels: a review. ICES J. Mar. Sci. 70 (1), 34–45. doi: 10.1093/icesjms/fss155
De Robertis A., Lawrence-Slavas N., Jenkins R., Wangen I., Mordy C. W., Meinig C., et al. (2019). Long-term measurements of fish backscatter from saildrone unmanned surface vehicles and comparison with observations from a noise-reduced research vessel. ICES J. Mar. Sci. 76 (7), 2459–2470. doi: 10.1093/icesjms/fsz124
Devold F. (1963). The life history of the atlanto-scandian herring. Rapp Proc. Verb Cons Int. Explor. Mer 154, 98–108.
Ghani M. H., Hole L. R., Fer I., Kourafalou V. H., Wienders N., Kang H., et al. (2014). The SailBuoy remotely-controlled unmanned vessel: Measurements of near surface temperature, salinity and oxygen concentration in the northern gulf of mexico. Methods Oceanography 10, 104–121. doi: 10.1016/j.mio.2014.08.001
Handegard N. O., Algrøy T., Eikvil L., Hammersland H., Tenningen M., Ona E. (2021b)Smart fisheries in Norway: Partnership between science, technology, and the fishing sector (Accessed May 23, 2022).
Handegard N. O., Tonje F. N., Johnsen E., Pedersen G., Tenningen M., Korneliussen R., et al. (2021). Fisheries acoustics in Norway–wide band data, autonomous platforms and deep learning. J. Acoustical Soc. America 150 (4), A254–A254. doi: 10.1121/10.0008196
Hentati-Sundberg J., Evan T., Osterblom H., Hjelm J., Larson N., Bakken V., et al. (2018). “Fish and seabird spatial distribution and abundance at the largest seabird colony in the Baltic sea”. Mar. Ornithol. 46, 61–68.
Holmin A. J., Korneliussen R. J., Tjøstheim D. (2016). Estimation and simulation of multi-beam sonar noise. J. Acoustical Soc. America 139 (2), 851–862. doi: 10.1121/1.4941913
Johnsen E., Kvamme C., Totland A. (2020). Measuring distribution and density of sprat in Årdalsfjorden with a kayak drone. Rapport fra Havforskningen 2020-28, 1893–4536.
Johnsen E., Pedersen R., Ona E. (2009). Size-dependent frequency response of sandeel schools. ICES J. Mar. Sci. 66 (6), 1100–1105. doi: 10.1093/icesjms/fsp091
Kum B. C., Shin D. H., Jang S., Lee S. Y., Lee J. H., Moh T., et al. (2020). Application of unmanned surface vehicles in coastal environments: Bathymetric survey using a multibeam echosounder. J. Coast. Res. 95 (SI), 1152–1156. doi: 10.2112/SI95-223.1
Ludvigsen M., Albrektsen S. M., Cisek K., Johansen T. A., Norgren P., Skjetne R., et al. (2016). Network of heterogeneous autonomous vehicles for marine research and management. OCEANS 2016 MTS/IEEE Monterey, 1–7. doi: 10.1109/OCEANS.2016.7761494
Mitson R. B., Knudsen H. P. (2003)Causes and effects of underwater noise on fish abundance estimation. Available at: https://www.alr-journal.org/articles/alr/pdf/2003/03/alr3043.pdf (Accessed May 23, 2022).
Ona E., Godø O. R., Handegard N. O., Hjellvik V., Patel R., Pedersen G. (2007). Silent research vessels are not quiet. J. Acoustical Soc. America 121 (4), EL145–EL150. doi: 10.1121/1.2710741
Othman E. H. (2015)A review on current design of unmanned surface vehicles (usvs) (Accessed May 23, 2022).
Romano A., Duranti P. (2012)Autonomous unmanned surface vessels for hydrographic measurement and environmental monitoring. In: FIG working week 2012 (Rome Italy). Available at: https://www.fig.net/resources/proceedings/fig_proceedings/fig2012/papers/ts04d/TS04D_romano_duranti_6118.pdf (Accessed May 23, 2022).
Safina C., Burger J. (1988). Use of sonar and a small boat for studying foraging ecology of seabirds. Colonial Waterbirds 11 (2), 234–244. doi: 10.2307/1521005
Shao G., Ma Y., Malekian R., Yan X., Li Z. (2019). A novel cooperative platform design for coupled USV–UAV systems. IEEE Trans. Ind. Inf. 15 (9), 4913–4922. doi: 10.1109/TII.2019.2912024
Simmonds J., MacLennan D. N. (2008). Fisheries acoustics: Theory and practice. John Wiley & Sons, 1–472.
Vabø R., Nøttestad L. (1997). An individual based model of fish school reactions: Predicting antipredator behaviour as observed in nature. Fisheries oceanog. 6 (3), 155–171. doi: 10.1046/j.1365-2419.1997.00037.x
Vabø R., Olsen K., Huse I. (2002). The effect of vessel avoidance of wintering Norwegian spring spawning herring. Fisheries Res. 58 (1), 59–77. doi: 10.1016/S0165-7836(01)00360-5
Verfuss U. K., Aniceto A. S., Harris D. V., Gillespie D., Fielding S., Jiménez G., et al. (2019). A review of unmanned vehicles for the detection and monitoring of marine fauna. Mar. Pollut. Bull. 140, 17–29. doi: 10.1016/j.marpolbul.2019.01.009
Keywords: echo sounder, fish behavior, seabird - fish interactions, silent and nonintrusive vehicle, unmanned surface vehicle (USV), vessel avoidance, vertical fish distribution
Citation: Totland A and Johnsen E (2022) Kayak Drone – a silent acoustic unmanned surface vehicle for marine research. Front. Mar. Sci. 9:986752. doi: 10.3389/fmars.2022.986752
Received: 05 July 2022; Accepted: 18 August 2022;
Published: 08 September 2022.
Edited by:
Haixin Sun, Xiamen University, ChinaReviewed by:
Adwitiya Sinha, Jaypee Institute of Information Technology, IndiaCopyright © 2022 Totland and Johnsen. This is an open-access article distributed under the terms of the Creative Commons Attribution License (CC BY). The use, distribution or reproduction in other forums is permitted, provided the original author(s) and the copyright owner(s) are credited and that the original publication in this journal is cited, in accordance with accepted academic practice. No use, distribution or reproduction is permitted which does not comply with these terms.
*Correspondence: Atle Totland, YXRsZS50b3RsYW5kQGhpLm5v
Disclaimer: All claims expressed in this article are solely those of the authors and do not necessarily represent those of their affiliated organizations, or those of the publisher, the editors and the reviewers. Any product that may be evaluated in this article or claim that may be made by its manufacturer is not guaranteed or endorsed by the publisher.
Research integrity at Frontiers
Learn more about the work of our research integrity team to safeguard the quality of each article we publish.