- 1Laboratório para a Inovação e Sustentabilidade dos Recursos Biológicos Marinhos e da Universidade de Aveiro (ECOMARE) & Centro de Estudos do Ambiente e do Mar (CESAM) Centre for Environmental and Marine Studies, Department of Biology, University of Aveiro, Campus Universitário de Santiago, Aveiro, Portugal
- 2Department of Fish Ecology and Evolution, Swiss Federal Institute of Aquatic Science and Technology (Eawag), Center for Ecology, Evolution, and Biogeochemistry, Kastanienbaum, Switzerland
- 3Mass Spectrometry Centre & Laboratório Associado para a Química Verde (LAQV-REQUIMTE), Department of Chemistry, University of Aveiro, Campus Universitário de Santiago, Aveiro, Portugal
- 4MARE - Marine and Environmental Science Centre, Escola Superior de Turismo e Tecnologia do Mar (ESTM), Polytechnic of Leiria, Peniche, Portugal
- 5Centre for Environmental and Marine Studies (CESAM), Department of Chemistry, University of Aveiro, Santiago University Campus, Aveiro, Portugal
Inter-individual variability plays a key role in species resilience. This, however, is difficult to assess in marine invertebrates with complex life cycles due to the inherent difficulty of sampling individuals in oceanic environments throughout their ontogeny. This study monitored the effect of contrasting oceanographic conditions, namely downwelling and upwelling, on the inter-variability of embryos and megalopae (the final larval stage) of the model brachyuran crab Carcinus maenas. We assessed i) the heterogeneity of energetic reserves, biomass, elemental composition, and isotopic niche in these early life stages, and ii) the effect of oceanographic conditions (up- and downwelling) on inter-individual variability. Indeed, organisms developing during downwelling consistently exhibited a higher heterogeneity than those developing during upwelling. While this finding suggests a higher resilience during downwelling, the overall condition of individuals was better during upwelling (i.e., higher C:N), irrespective of the developmental stage. Altogether, our data suggests that trophic history experienced under contrasting oceanographic conditions shape the plasticity of C. maenas populations and cascades over different life stages.
Introduction
A “deep trench of knowledge” separates developmental and ecological biologists focusing on marine crustaceans (Torres et al., 2019). The former mostly focus on specific processes that occur within a given developmental stage (e.g., neurogenesis) under optimal conditions. In contrast, ecological biologists usually attempt to link plastic responses (e.g., growth rates or trophic ecology consequences) to some key (yet specific) factors. Nevertheless, these two research lines share the need for information on real-world environmental contexts. Such information is key to address questions that link environmental conditions to inter-individual variability and its fitness consequences (Bosch et al., 2014; Gilbert, 2017; Sultan, 2017). In particular, inter-individual variability is typically associated with the levels of adaptability and resilience for a given species (Sunday et al., 2011; Foo et al., 2012; Schlegel et al., 2012; Forsman and Wennersten, 2016), which highlights the importance of understanding the key drivers of variability for both developmental and ecological disciplines.
Studying inter-individual variability in marine invertebrates with complex life cycles (i.e., those who experience metamorphosis from pelagic larvae to benthonic juveniles) is, however, beset by in situ logistic limitations to secure the monitoring of complete larval periods, and by the inherent difficulty of sampling in oceanic environments (Rey et al., 2019). As such, the extent of inter-individual variability in this type of organisms and the possible effects of varying environmental conditions on this trait have been largely overlooked (Calado and Leal, 2015). Indeed, marine invertebrates with complex life cycles often inhabit contrasting environments in their larval and adult forms, and shift dramatically from a pelagic to a benthic environment (e.g., Torres et al., 2021 and references therein). This change results in multiple potential drivers of inter-individual variability throughout their life cycle, thus highlighting the role of marine invertebrates with this type of development as model species to assess phenotypic variability throughout ontogeny (Duarte et al., 2014; Torres et al., 2019).
Aside from environmental drivers, inter-individual variability can also be affected by developmental processes. When considering embryogenesis, for example, it is important to know if the natural variability present in newly extruded embryos is propagated to later embryonic stages (Fernández et al., 2000; Leal et al., 2013) or if, on the other hand, initial embryonic inter-individual variability decreases over embryogenesis (Rosa et al., 2003; Rey et al., 2016a). Embryogenesis in marine invertebrates with complex life cycles, such as decapod crustaceans, is heavily dependent on lipidic reserves (Petersen and Anger, 1997 and references therein), which are known to vary throughout embryogenesis (Rey et al., 2015a) and with environmental conditions (e.g., Brillon et al., 2005) and/or with the nutritional status of females (e.g., Tuck et al., 1997; Racotta et al., 2003; Calado et al., 2010). A contrasting allocation of lipids from females to their embryos due to environmental or dietary drivers can determine the level of initial lipidic reserves available to fuel embryogenesis. Thence, the fitness of subsequent life stages can either be compromised or enhanced, and this effect likely repercusses on inter-individual variability throughout embryonic development (e.g., Rosa et al., 2007; Rey et al., 2016a). Ultimately, the performance and survival of decapod crustaceans with complex life cycles can be already compromised at hatching (e.g., Giménez, 2010; Rey et al., 2015b; Rey et al., 2016b) or even at oviposition if maternal effects are taken into consideration (see review by Calado and Leal, 2015). Indeed, early life stages can provide key information on how contrasting environmental conditions affect inter-individual variability (and performance) of these invertebrates with bi-phasic life cycles (Pechenik, 2006; Giménez, 2010; Rey et al., 2019; Rey et al., 2022).
The green crab Carcinus maenas develops through four planktonic zoeal stages, a single megalopae stage (semi-benthonic), and then undergoes metamorphosis to settle as a benthic juvenile that grows onto adulthood (Rice and Ingle, 1975). This species is a highly successful global invader (Carlton and Cohen, 2003; Young and Elliott, 2019), which makes it an optimal candidate to study the influence of environmental parameters (such as contrasting oceanographic conditions, namely downwelling and upwelling) as drivers of inter-individual variability. For instance, downwelling and upwelling repercuss on planktonic community composition and abundance (e.g., Ospina-Alvarez et al., 2010; Wilson et al., 2021). In turn, this affects C. maenas throughout its different life stages, as they ingest plankton during larval life (Pihl, 1985) and predate on important planktotrophs as adults (Palacios and Ferraro, 2003). Consequently, the variability experienced by C. maenas in such natural settings provides an excellent opportunity to investigate how these oceanographic events shape the inter-individual variability of this model species (Domingues et al., 2011).
The present study determined the effects of different developmental and environmental factors on inter-individual variability throughout the early life stages of the model species C. maenas. The following hypotheses were tested: i) the dominant oceanographic condition (up- or downwelling) is an important determinant of heterogeneity among embryos, and ii) variability at hatching (irrespective of the source) affects the degree of inter-individual variability of later developmental stages. The hypotheses were addressed by assessing the stable isotopic niche variability of both individual embryos and megalopae, and the elemental composition of the latter, sampled during contrasting oceanographic events (downwelling and upwelling). We also determined the biochemical profile (phospholipid, elemental composition and isotopic signature) of newly extruded and late-stage embryos to assess maternal effects and embryogenesis as potential sources of inter-individual variability. Altogether, the collected data provide an overview of different sources of inter-individual variability and their potential fitness consequences (e.g., Tang and Dam, 1999).
Materials and methods
Sampling procedure and location
Ovigerous C. maenas females and megalopae were collected at Ria de Aveiro, a shallow coastal lagoon on the north-western Portuguese coast (40°37’17’’ N, 8°44’56’’ W) influenced by downwelling and upwelling conditions (Fiúza et al., 1998). The ovigerous females were sampled in periods of downwelling (28th of March to 17th of April 2013) and upwelling (3rd and 4th of July 2013) events (see Rey et al., 2016b for a detailed analysis of the upwelling index during this period).
Ovigerous females were collected by trawling at low tide at ca. 2 m depth and pools of embryos were obtained by carefully removing them from their brooding chambers using forceps. Embryos were subsequently identified either as stage 1 (newly extruded embryos – characterised by uniform yolk and no embryonic structures visible, n = 10 females per oceanographic event) or stage 3 (embryos ready to hatch in less than 48 h – characterised by nearly no yolk and a fully developed embryo, n = 10 females per oceanographic event) according to Rosa et al. (2007). Accordingly, a total of 40 ovigerous females were sampled for this study, and individual carapace widths (CW, mm – measured between the first pair of lateral spines of the carapace) were recorded, ranging between 26.55 and 49.79 mm. These measurements were then converted to wet weight (WW, g) to minimize the effects of allometric growth following Equation 1 (Jungblut et al., 2017):
Immediately after collection, 30 embryos were randomly selected from the pool of embryos sampled from each female and measured using a calibrated micrometer eyepiece mounted on a stereomicroscope. Embryos were assumed to be approximately spheric (Rosa et al., 2007;Rey et al., 2016a). The remaining embryos pooled from each female were freeze-dried and stored at -32°C until biochemical analysis.
Megalopae were sampled by deploying two plankton nets with their entrance facing the inlet of the lagoon (constant depth of 0.1 m, as described by Queiroga et al. (2006)) for 24 h over several consecutive days within a period of downwelling (24th of March to 14th of April) and a period of upwelling (7th of May to 21st of May). The nets were allowed to passively collect plankton onto a collector cup, with specimens being gently transferred to a bucket with seawater and transported to the laboratory. Upon arrival, C. maenas megalopae were sorted live from the mixed plankton assemblage using a plastic pipette, rinsed with freshwater to remove salt, flash-frozen and freeze-dried. A total of 226 megalopae were collected during downwelling and 307 during upwelling conditions.
Isotopic signatures and elemental composition
Currently, one of the best methods for the characterisation of a species’ trophic niche is the determination of its isotopic niche, as the two are tightly correlated (Jackson et al., 2011). The isotopic niche is typically inferred from a bi-plot that represents stable isotopic signatures (the variation in heavy to light ratios – 13C/12C and 15N/14N – i.e., δ13C and δ15N values) in a δ-space (Newsome et al., 2007). The stable isotopic signatures of C. maenas embryos and megalopae were thus determined. The information from embryos was used as a proxy to infer the isotopic niche of ovigerous females following previous studies (e.g., Kaufman et al., 2014; Dimitrijević et al., 2018).
Stable isotopic signatures were measured using a Thermo Fisher Delta V Advantage Isotope Ratio mass spectrometer coupled to a Thermo Flash 2000 Organic elemental analyser. The isotopic reference materials (acetanilide; Stable Isotope Research Facility, Indiana University, USA) were assessed at the beginning of each run and after every 10 samples. The average precision standards for δ13C and δ15N were 0.05 and 0.14‰, respectively. Carbon and nitrogen contents were also determined using this equipment. Before being processed (for isotopic and elemental analysis), a sub-sample of the pool of embryos sampled from each female (n = 40) were ground into a fine powder using a tissue lyser paired with tungsten beads (TissueLyser II, Qiagen, Hombrechtikon, Switzerland). Megalopas were individually placed and weighed in the tin cups before analysis in the mass spectrometer.
Decapod crustaceans possess a chitin-calcite exoskeleton and, therefore, their isotopic signature can be affected by the presence of inorganic C (Fantle et al., 1999; Kolasinski et al., 2008). We conducted a preliminary analysis to assess the effect of acidification (HCl) on megalopae δ13C and δ15N (Carabel et al., 2006). Although the δ15N remained unaltered (t = 1.42, df = 11.58. p = 0.2), δ13C changed, as expected, with acidification (t = 5.05, df = 11.53, p< 0.01). However, we decided not to acidify megalopa samples because i) the changes in δ13C with acidification were lower than the range of an average trophic level (ca. 0.39‰, whereas the average trophic level shift is 0.5 to 1‰ – see for instance Dimitrijević et al. (2018); ii) acidifying samples significantly (p< 0.05) affected the C and N content (C = 28.62% ± 6.62, N = 6.23% ± 0.91 in acidified samples versus C = 35.50% ± 0.49, N = 7.60% ± 0.16 in non-acidified samples) but not the C:N ratio (t = -1.72, df = 7.8113, p = 0.12); and iii) acidification decreased the dry weight of individual megalopae by ca. 18% (from 0.260 ± 0.039 to 0.213 ± 0.012) and, as a consequence, the accuracy of the δ13C and δ15N isotopic signatures were affected by the decrease in dry weight to values close to the detection limit of the mass spectrometer and elemental analyser, which in turn artificially increased the variability of results. Given that the main goal of this study was to assess sources of inter-individual variability, we have decided to mitigate any additional variability driven by technical artefacts, i.e., the detection limit of the equipment.
Phosphorus (P) content was only determined in embryos because megalopae could not be divided for different analyses. A sub-sample of ground embryonic tissue was diluted in potassium peroxydisulfate (10 g K2O8S2, 1.5 g NaOH, 1 L Milli-Q water) and autoclaved for 2 h at 121°C for P digestion prior to quantification. The concentration of P was determined colourimetrically using a continuous flow analyser (Skalar Analytical B.V., Breda, The Netherlands) following the ammonium molybdate method (Murphy and Riley, 1962; Parsons et al., 1984) and standard procedure ISO 13395:1996. Drift and baseline corrections were programmed every 18 samples.
Lipid analysis
Total lipids were extracted and quantified for the embryo samples, following the method by Bligh and Dyer (1959) as described by Rey et al. (2016a). For the quantification of total phospholipid (PL) content in the embryos, a phosphorus assay was conducted according to Bartlett and Lewis (1970). Briefly, we incubated 5% of the total lipid volume (previously dried under a nitrogen flow) with perchloric acid (70% V/V) for 1 h at 180°C. After this period, we added 3.3 mL of water, 0.5 mL of ammonium molybdate (2.5% w/V), and 0.5 mL of ascorbic acid (10% w/V) to each sample and incubated them for another 10 min at 100°C. Aside from the samples of C. maenas embryos, we also prepared standard solutions from 0.1 to 3.0 µg of phosphate (control samples) and applied the same protocol described above to them. The absorbance of both control and experimental samples was measured at room temperature (800 nm) in a microplate spectrophotometer (ultraviolet-visible).
Statistical analysis
Statistical analyses were conducted in R v 4.1.3 (R Development Core Team, 2021). The different physiological and biochemical parameters recorded for embryos and megalopae were compared between supply events (downwelling and upwelling) using Students’ t-test after checking for normality and homogeneity of variance. The isotopic profile (δ13C and δ15N) of embryos and megalopae were compared between supply events using a two-way MANOVA with embryo stage and supply events as categorical variables. The relationship between female biomass and embryo volume was tested with a general linear model, whereas the relationship between female biomass and embryo C:N content was tested with a logistic regression (higher power than transforming proportional data to use in linear models – Warton and Hui, 2011). A general linear model was used to assess how the relationship between % C and % N of megalopae interacted with supply event. Residuals for all models were tested for normality and heterogeneity of variance.
To infer the isotopic niche of females (using data from their embryos) and determine the isotopic niche of megalopae for each supply event, biplot ellipses were used to delineate the isotopic niche space (Jackson et al., 2011; Duarte et al., 2017). To test whether niche space differed between supply events, we used a Bayesian estimate of the standard ellipse and its area (‰2) using the package SIAR (Jackson et al., 2011).
Results
Embryos
Average embryonic volume and C:N content were similar across females, irrespective of their weight and sampling event (during downwelling or upwelling; Figure 1). The slope of the linear or logistic regressions was not significant (p > 0.05 in both cases). Irrespective of embryonic development stage and female biomass, embryonic volume did not differ significantly under downwelling or upwelling conditions (two-tailed Student’s t-test, stage 1: t(18) = -1.43; p = 0.171; stage 3: t(18) = -1.10; p = 0.302). Nevertheless, stage 3 eggs were significantly larger than stage 1 eggs (one-tailed Student’s t-test, t(38) = 21.63; p = 0.00).
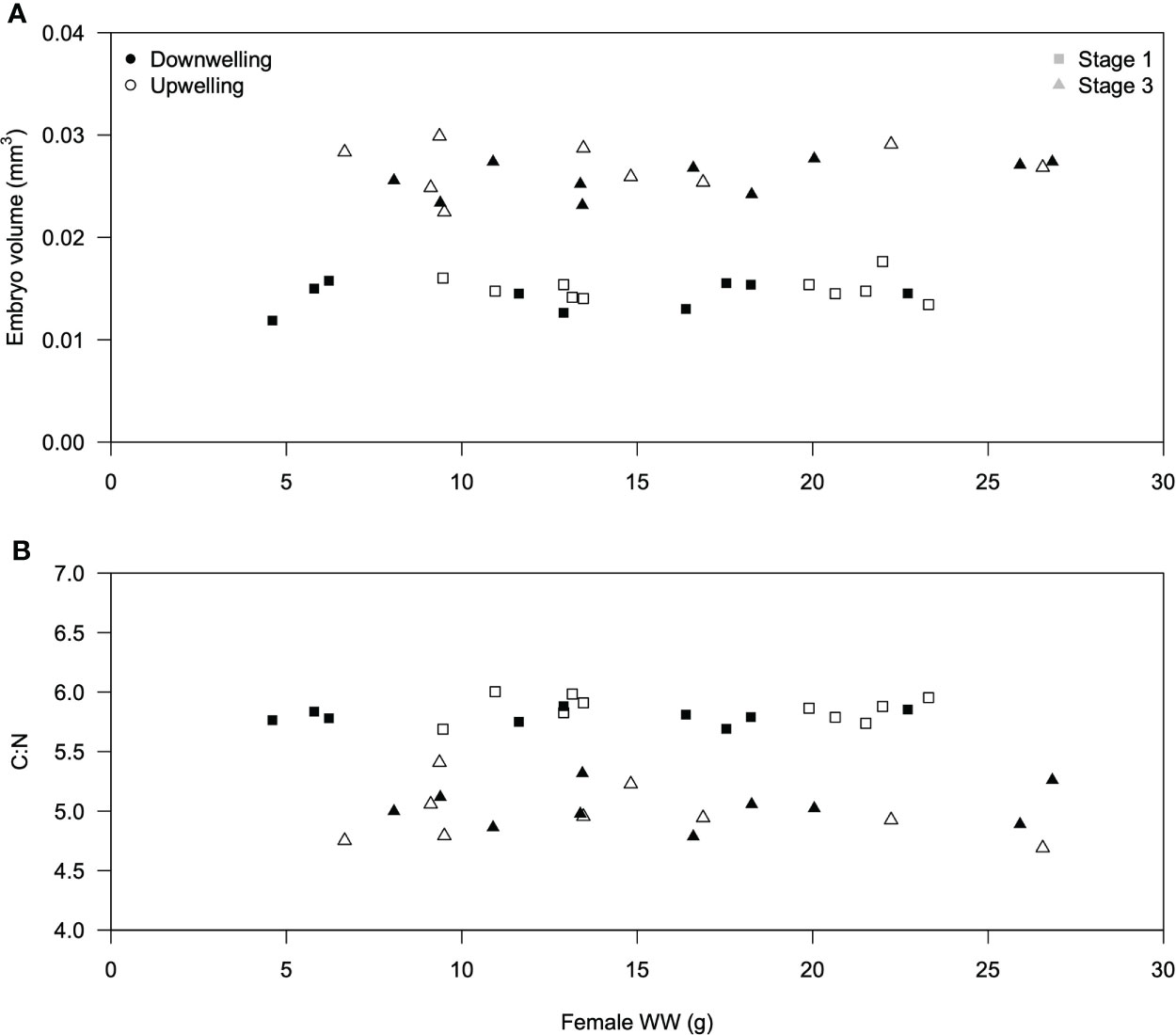
Figure 1 Relationship between female Carcinus maenas wet weight (g) and (A) average embryo volume (mm3, n = 40 females) or (B) average embryo C:N content (n = 40 females). Females collected during downwelling are depicted with white symbols, whereas upwelling ones are shown in black symbols. Squares and triangles are used to distinguish between stage 1 and stage 3 embryos, respectively.
The PL pool of embryos differed between the two oceanographic events (Table 1). Total PL of stage 1 and stage 3 embryos was significantly lower in females collected during downwelling, as compared to those sampled during the upwelling event (two-tailed Student’s t-test, p< 0.05 for all comparisons). In contrast, the elemental content of embryos (Table 1) was typically not statistically different (p > 0.05) between supply events, apart from N content in stage 1 embryos, which was slightly higher during downwelling (two-tailed Student’s t-test, t(18) = 2.18; p = 0.04).

Table 1 Phospholipid, elemental and isotopic content (mean ± SD) of embryos collected from wild Carcinus maenas females during downwelling (n = 10 females for stage 1 and 10 females for stage 3) and upwelling (n = 10 females for stage 1 and 10 females for stage 3).
The isotopic profile (δ13C and δ15N) of embryos was similar between developmental stages (MANOVA: F = 2.15, df = 2; p = 0.13) yet different between oceanographic event (MANOVA: F = 41.64, df = 2; p< 0.01; interaction F = 1.55, df = 2; p = 0.23). Therefore, we grouped females irrespective of the developmental stage of their embryos and determined their isotopic signature during both downwelling and upwelling (Figure 2). Ovigerous females showed a relatively similar δ13C signature between supply events, but a higher δ15N signature during downwelling than in the upwelling season (Table 1). In addition, the range of isotopic signatures varied between supply events, as highlighted by the standard Bayesian ellipse areas based on both δ13C and δ15N values (downwelling ellipse area = 14.14 ‰2, and upwelling ellipse area = 2.30 ‰2).
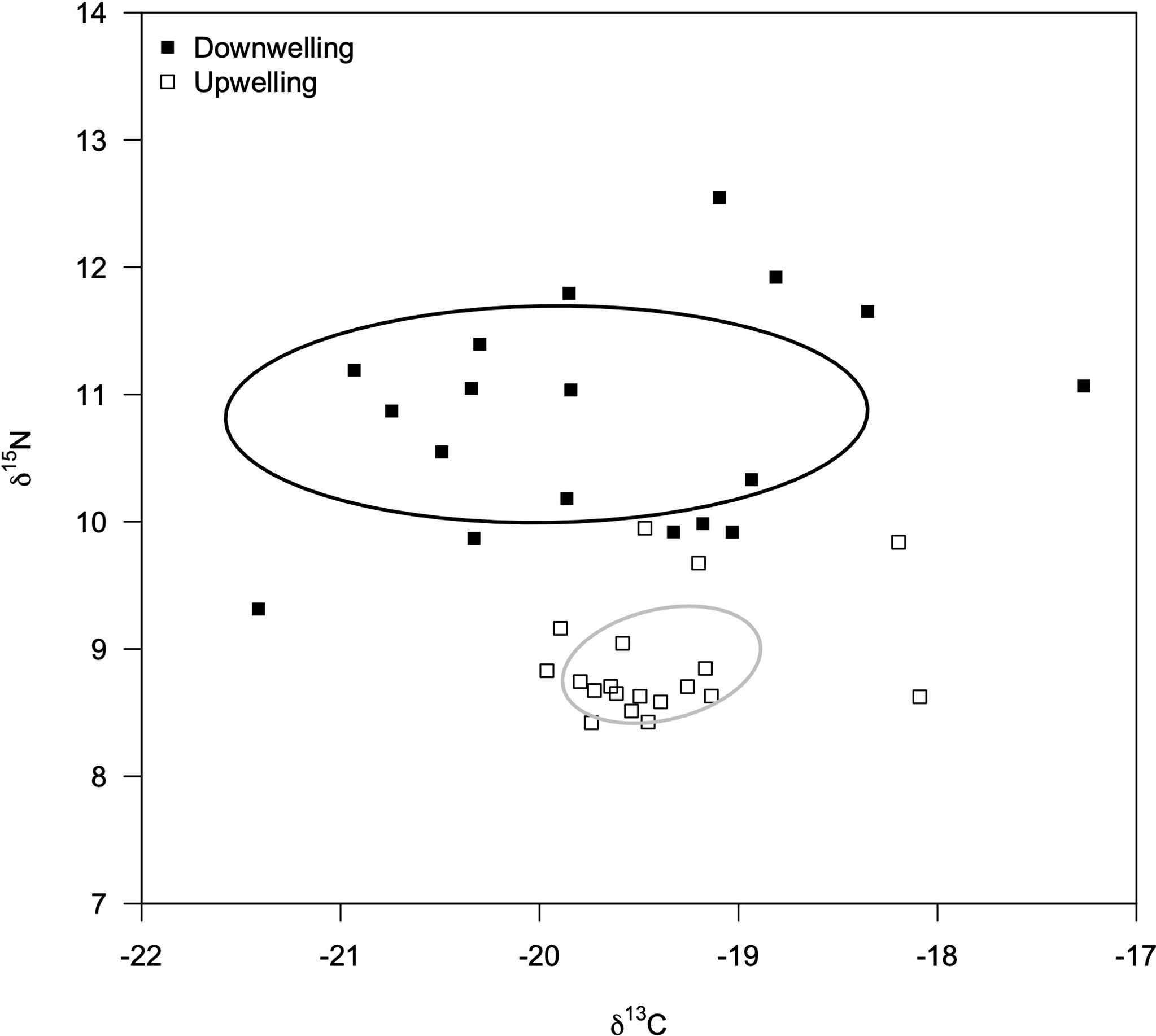
Figure 2 Isotopic niche area of Carcinus maenas females (δ13C and δ15N; ‰) inferred from embryonic tissues collected during downwelling (n = 20 females) and upwelling (n = 20 females) events. Stage 1 and stage 3 embryos were combined for each event given their due to similar isotopic profiles (MANOVA, p = 0.32) The standard Bayesian Ellipse areas are shown for each supply event.
Megalopae
The average ( ± SD) biomass of megalopae was significantly higher (t(531) = -20.78; p< 0.01) during upwelling (0.22 ± 0.04 mg DW) as compared to the downwelling event (0.15 ± 0.04 mg DW) (Figure 3). The two supply events surveyed also affected the C and N elemental content of megalopae (Figure 4; %C two-tailed Students’ t-test t(531) = -9.34; p< 0.01; %N two-tailed Students’ t-test t(531) = -5.32; p< 0.01), as well as their C:N ratio (C:N 5.28 ± 0.31 and 5.46 ± 0.25 during downwelling and upwelling, respectively; two-tailed Students’ t-test; p< 0.01). The results also demonstrate a significant interaction between the elemental profile of the megalopae and the oceanographic event (Table 2).
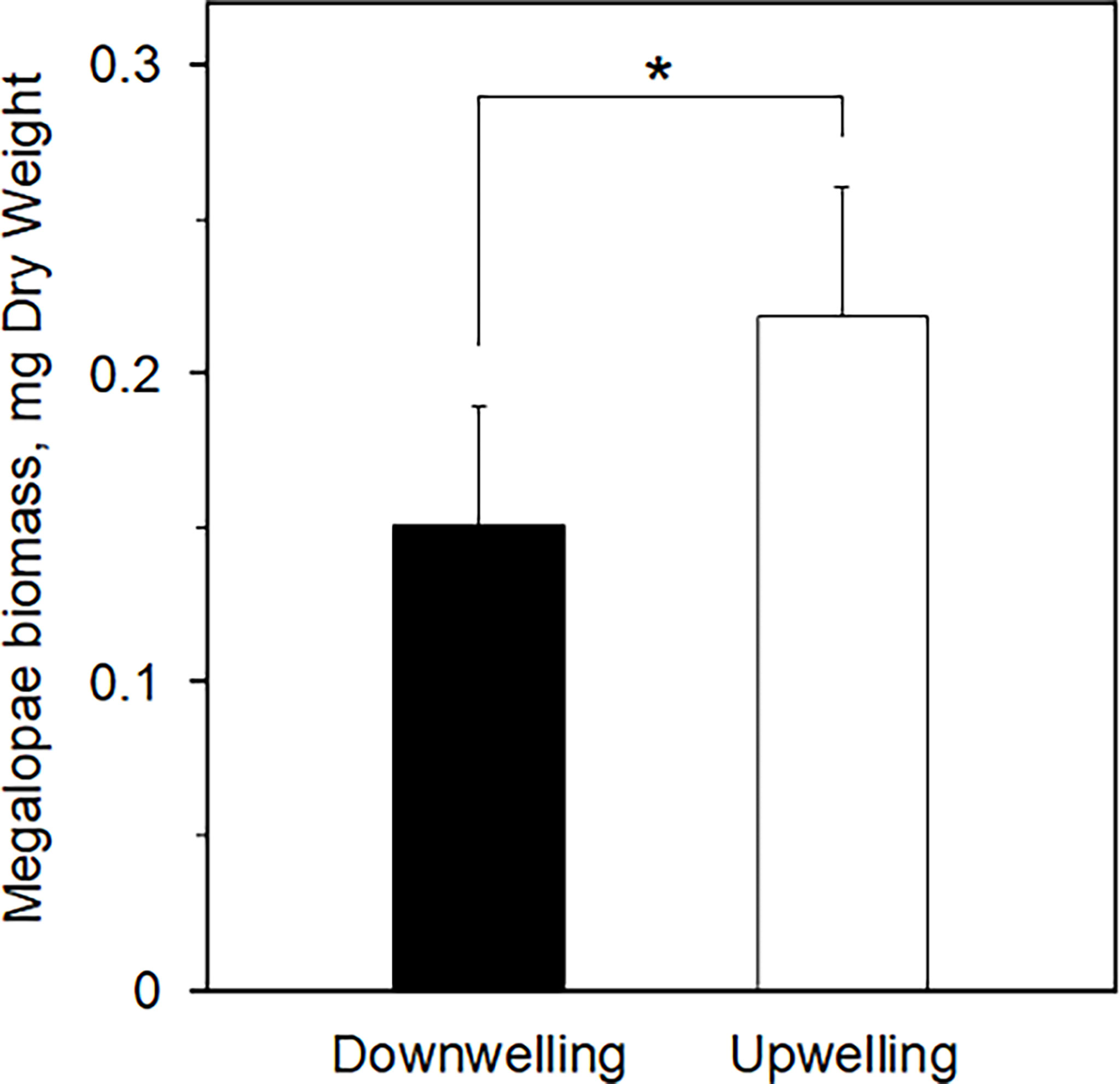
Figure 3 Biomass of Carcinus maenas megalopae (mg DW) collected during downwelling (n = 226) and upwelling (n = 307) conditions. The * denotes significant differences between supply events (one-tailed Students’ t-test, p = 0.00).
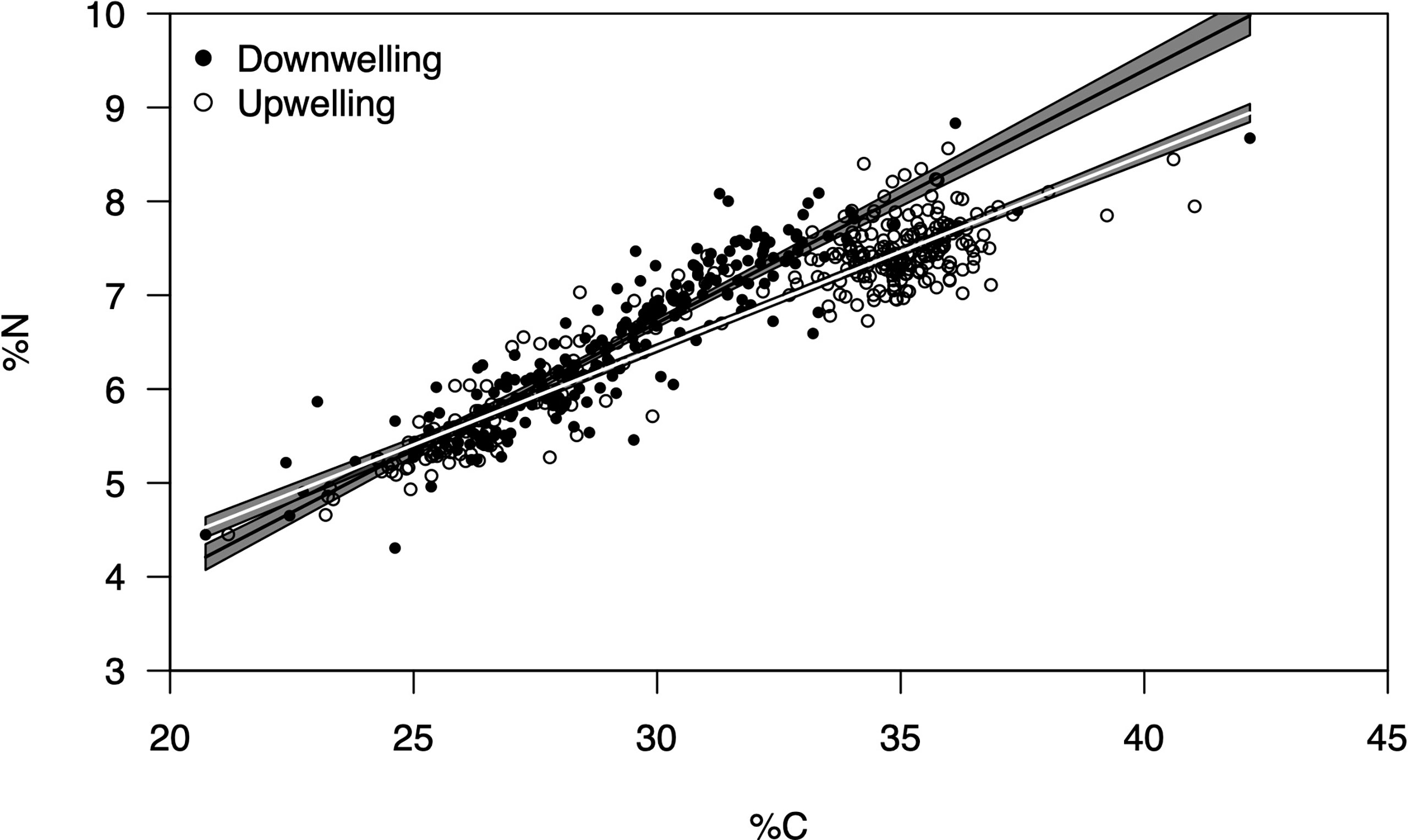
Figure 4 Relationship between % C and % N for Carcinus maenas megalopae collected during downwelling (n = 226) and upwelling (n = 307). The slopes of the predicted linear regressions (thick lines) represent the C:N ratio for the megalopae during the two contrasting supply events (black for downwelling, white for upwelling). The grey area depict the confidence intervals (2 times the St. Error). The C:N ratios were significantly different between supply events (two-tailed Students’ t-test, p < 0.05).
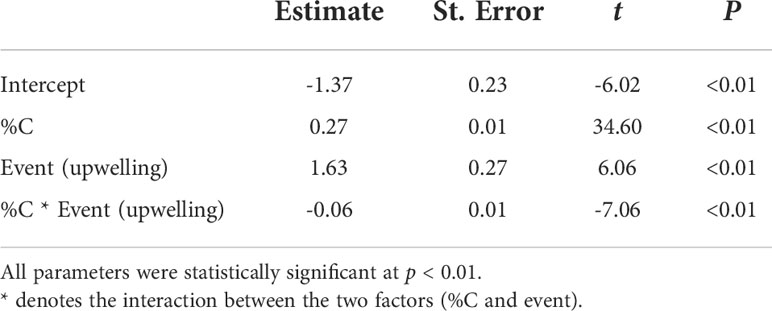
Table 2 Linear model testing the interaction between oceanographic events (downwelling and upwelling) and the elemental content of Carcinus maenas megalopae (%N as the response variable, %C and oceanographic event as explanatory variables).
Megalopae showed significantly different isotopic signatures (Figure 5) during downwelling and upwelling (MANOVA: F = 103.37, df = 2; p< 0.01). The δ13C ranged between -20.9 and -16.3‰ during downwelling and -19.9 and -17.0‰ during upwelling, being the average δ13C value lower during downwelling than upwelling (-19.27 ± 0.87‰ and -18.58 ± 0.49‰, respectively; p< 0.01). The opposite was observed for the δ15N signature, with an average of 7.91 ± 0.50‰ during downwelling and 7.66 ± 0.49‰ during upwelling (p< 0.01). Yet, the isotopic signature showed a similar range in both events (6.1 to 9.1‰ – downwelling; 6.1 to 9.2‰ – upwelling). In addition, the isotopic niche space occupied by megalopae during the downwelling event was notably larger than that during upwelling, as highlighted by the standard Bayesian ellipse areas based on both δ13C and δ15N values (downwelling = 1.36‰2, and upwelling = 0.71‰2).
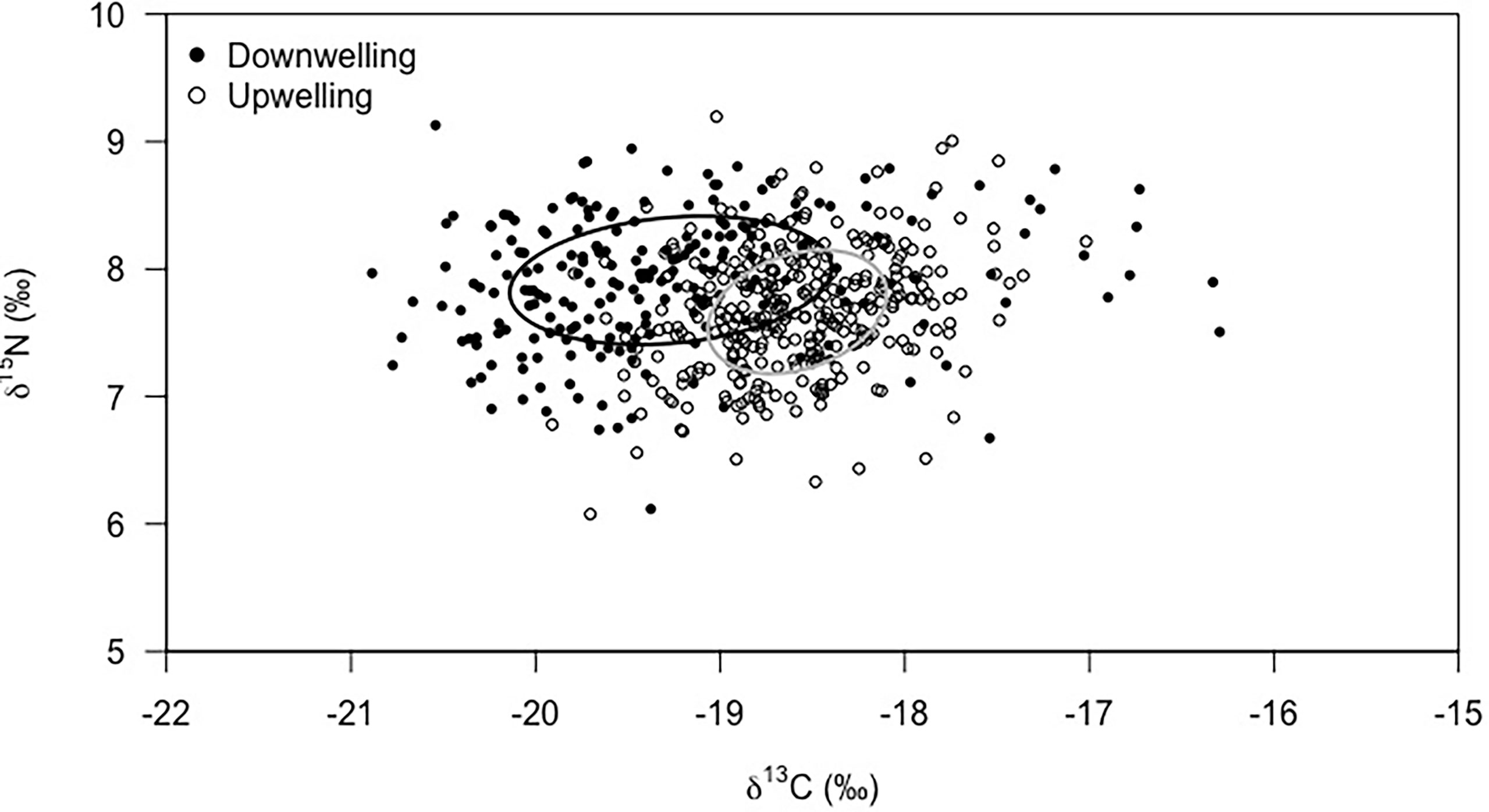
Figure 5 Isotopic niche area based on stable isotopes (δ13C and δ15N) of Carcinus maenas megalopae collected during downwelling (n = 226) and upwelling (n = 307) conditions. The standard Bayesian Ellipse areas were significantly different between supply events (MANOVA, p < 0.01).
Discussion
Two main questions addressed in this study using the brachyuran crab C. maenas as a model species were: i) whether downwelling and upwelling events differently affect inter-individual variability of organisms with complex life cycles, and ii) how different developmental stages can drive variability among individuals. To answer these questions we focused on the early life stages of this species, namely their embryos and megalopae, as their influence on early benthic life stages is already documented (e.g., Giménez, 2010; Rey et al., 2015b; Rey et al., 2016b) through carry-over effects (also known as legacy effects) with potential phenotypic and fitness consequences (e.g., Pechenik, 2006; Giménez, 2010; Rey et al., 2019). In summary, downwelling and upwelling events differently affected inter-individual variability, particularly through the trophic ecology of females and of megalopae during planktonic life.
One possible source of inter-individual variability in early life stages concerns maternal effects, which can be defined as “the effects of adult conditioning on offspring quality” as in Pechenik (2006) and references therein. Here, maternal effects were assessed through the consequences of female biomass on the volume and elemental composition of their embryos (Figure 1), despite acknowledging that these effects can also be accounted using other parameters (e.g., cortisol levels in McCormick, 1998). The reason why female size was considered as a plausible source of maternal effects concerns the fact that larger females can secure more and/or better food, which improves the allocation of reserves to their embryos (Calado and Leal, 2015) and ultimately allow larvae to hatch in better conditions than those from smaller females. Indeed, energetic reserves are significantly correlated with embryonic size in various decapod species (Rosa et al., 2007). We thus hypothesized that stage 1 embryos from larger C. maenas females could be fitter than those from smaller females, and consequently display a higher volume and/or C:N ratio. However, neither the volume of stage 1 embryos (Figure 1A) nor the C:N ratio (Figure 1B) were affected by the size of the ovigerous female (p > 0.05 on all cases), suggesting that female size does not significantly contribute as a driver of inter-individual variability. While this observation is in agreement with previous findings for Paralithodes camtschaticus, where female weight did not affect embryo size (Swiney et al., 2013), it contrasts with the findings for the European lobster Homarus gammarus (Moland et al., 2010).
A common feature of embryogenesis in crustaceans is the catabolism of lipidic reserves (Petersen and Anger, 1997; Rosa et al., 2003; Rosa et al., 2005), which is supported by the differences observed between the lipidic reserves of stage 1 and stage 3 embryos (Table 1). This consumption of the available lipid pool throughout embryo development could also explain the lower C and N contents, and C:N ratios observed in late-stage embryos, when compared with newly-extruded ones (e.g., Dawirs, 1981; Rosa et al., 2007). Nevertheless, it is worth mentioning that the C:N ratio remained unaltered within a given embryonic stage (p > 0.05 – Figure 1), despite these originating from females sampled at different oceanographic events, which is a clear sign of homeostasis in C. maenas’ embryos. Irrespective of developmental stage, different oceanographic events affected embryonic lipid content (PL content), with these being significantly lower during downwelling (p< 0.05 on all cases – Table 1). These differences in energetic reserves between supply events are natural (e.g., Brillon et al., 2005; Calado et al., 2010) and are expected to repercuss on the success of larval life, as well as post-metamorphosis (e.g., Giménez, 2010; Rey et al., 2015b; Rey et al., 2016b). Indeed, upwelling causes an ascent of nutrients from cooler and deeper waters to the surface, which reflects heavily on the food chain composition (e.g., Wilson et al., 2021) that becomes diatom-dominated (e.g., Fenchel, 1988; Sommer et al., 2002; Vidal et al., 2017). This shift in the dominant planktonic group alters the entire food chain, including the trophic ecology and nutritional status of C. maenas females that feed on planktivorous species (Pardal et al., 2006), as well as the extent of their energetic reserves (Tuck et al., 1997; Racotta et al., 2003).
The most prominent effect of different oceanographic events was recorded on the isotopic signature of embryos (Figure 2). As these tissues display fast turnover rates (e.g., Dimitrijević et al., 2018), their isotopic signature is strongly shaped by maternal diet prior oviposition. Thus, the isotopic signature of embryos can be used as a reliable proxy to estimate the isotopic niche of ovigerous females (e.g., Kaufman et al., 2014). A species’ isotopic niche is tightly correlated with its trophic niche, despite not being the same concept (Post, 2002; Jackson et al., 2011). The trophic niche of a predator is determined by its diet (Moore and Semmens, 2008; Parnell et al., 2010), whereas the isotopic niche is often beset by the consumer physiology (although in predictable amounts, thus the value of such comparison – e.g., (Post, 2002), Bearhop et al. (2002); Fox-Dobbs et al. (2007). In this regard, the δ13C values can be used to determine the variability of dietary carbon sources (e.g., Jackson et al., 2011; Yeakel et al., 2016) due to different incorporation rates across prey species (Layman et al., 2007). On the other hand, δ15N values are better suited to determine the trophic level of individuals (Layman et al., 2007; Jackson et al., 2011). Therefore, one can observe a direct effect of the supply event on the trophic ecology of ovigerous females: during downwelling females eat more diverse food (higher δ13C variability and range) and show a notably higher trophic level than females collected during upwelling conditions (higher δ15N value). The average δ15N signature during downwelling was ca. 1.97‰ higher than that during upwelling, which denotes an enrichment within the expected values of a one-level trophic variation; between 1.3 and 5.3‰, with an average of ca. 3.4‰ (Minagawa and Wada, 1984; Wada et al., 1987; Fry, 1988). In other words, C. maenas females predate on a shorter food chain during upwelling than they do during downwelling, with the diversity of prey items being lower. Adults C. maenas predate on important consumers of planktonic communities (e.g., Pihl, 1985; Palacios and Ferraro, 2003), whose abundance (e.g., Ospina-Alvarez et al., 2010) and composition (e.g., Wilson et al., 2021) is heavily influenced by oceanographic events and seasons (Pardal et al., 2006). Altogether, results suggest that downwelling increases the diversity of food sources being used and the length of the food chain, thus shaping the trophic ecology of adult C. maenas (Figure 2). These consequences of downwelling are in agreement with previous studies (e.g., Tarran et al., 1999). Indeed, the lack of a dominant planktonic group during downwelling events, such as diatoms that dominate the phytoplankton community during upwelling conditions (Fenchel, 1988; Sommer et al., 2002), might force a diversification of prey items (and increase competition for resources – e.g., Duarte et al., 2017); under this scenario the range of δ13C values increases for a given species (Jackson et al., 2011; Yeakel et al., 2016). Therefore, the change in the trophic ecology of C. maenas in response to contrasting oceanographic events can be deemed responsible for the changes in the lipidic reserves displayed by its embryos (Table 1). Additionally, results suggest that the higher inter-individual variability observed in the isotopic profile of ovigerous females during downwelling conditions is retained throughout embryogenesis.
Cascade-like effects, where early life histories determine the success or demise of later developmental stages, have been previously investigated in marine invertebrates, particularly in decapod crustaceans (e.g., Giménez, 2010; Rey et al., 2015b). Yet, few studies have addressed such impacts at the individual level due to the inherently complex process of sampling and analysing individual larvae (Rey et al., 2019). Here we assessed whether the inter-individual variability during embryonic development was associated with maternal effects, as well as contrasting oceanographic conditions. One other aspect was whether megalopae would still exhibit measurable inter-individual variability that could be traced back to the influence of distinct larval supply events. It is worth noticing that despite having data from distinct developmental stages in C. maenas, our study does not provide information on the effect of development per se as a possible source of inter-individual variability, as this would require following the same individuals of a given population across multiple developmental stages.
The larger megalopae of C. maenas observed during upwelling (Figure 3) suggest that the higher lipidic reserves observed in upwelling embryos (Table 1) likely benefited the organisms in subsequent developmental stage (also confirmed by the higher C:N ratio recorded during upwelling – Figure 4). Yet, it could have been beneficial to account for other biochemical parameters (other than lipidic reserves) that also affect a larvae’s development into megalopae, such as the protein or carbohydrate content, for example (McAlister and Moran, 2012). In any case, our observations are in accordance with Rey et al. (2017), who suggested that upwelling produced higher quality lipids in C. maenas embryos and improved larval quality and chances to successfully metamorphose. Furthermore, our results match the model predictions of Rey et al. (2016b), where being a zoea during upwelling conditions promoted the growth of juvenile crabs. The larger an organism is, the faster it undergoes metamorphosis (Giménez, 2010; Calado and Leal, 2015), which can be useful both from a prey’s but also from a predator’s point of view. From the prey’s perspective, metamorphosis can make an organism large enough to attain a so called “safe size”, hence escape predation (Werner, 1991; Abrams et al., 1996) or, in the specific case of C. maenas, most likely cannibalism (Moksnes, 2004; Almeida et al., 2011). As a predator, metamorphosis in C. maenas involves the formation of claws and improves the overall strength of the organism, which grants it a competitive advantage over its competitors for food (Duarte et al., 2014). As such, the biomass data record (larger megalopae during upwelling conditions) suggest that individuals are better suited to deal with various biotic constraints during upwelling than during downwelling, as confirmed by the C:N ratio (Figure 4) and the significant interaction of supply event (Table 2). The reason behind having higher biomass and C:N ratios during upwelling may be explained by the dietary shifts that occur in the plankton, a direct consequence of shifting oceanographic conditions (see discussion below). Indeed, the fact that megalopae feed on plankton paired with the observations of healthy megalopae during downwelling, imply that embryonic reserves cannot completely explain the success or demise of later life stages. In other words, a lipid-poor embryo does not necessarily imply failure as megalopae since success also depends on the conditions and resources (such as food) encountered as a megalopae, thus compensating the lack of initial energetic reserves (see Swearer et al., 2019 and references therein). It is also important to highlight that the higher and less variable C:N ratio (in megalopae) during upwelling suggests no absolute homeostasis and implies that these individuals are in better conditions when upwelling is the dominant oceanographic condition. Our results thus agree with previous observations that organisms deal with a changing environment by adjusting homeostatic mechanisms over time i.e., exhibit rheostasis (e.g., Villar-Argaiz et al., 2002; Leal et al., 2017).
Another important aspect to highlight is the lower inter-individual variability of megalopae isotopic signature during upwelling than downwelling. As previously discussed, the available range of preys greatly affects the trophic ecology of C. maenas. The trophic level of the megalopae (Figure 5) suggests that, during upwelling, the organisms are a part of a shorter and more efficient food chain (e.g., Fenchel, 1988; Sommer et al., 2002). As observed by Duarte et al. (2017) for a different decapod species, a habitat with a surplus of food (equivalent to upwelling conditions in our study) enables feeding on preferred food items, which narrows down the isotopic niche of a species. In the opposite scenario (downwelling), megalopae cannot be selective and must feed on whatever preys are available. This generalist feeding behaviour often implies a higher competition for resources, with intra/inter-specific competition being known to affect the magnitude of inter-individual variability within a population (Araújo et al., 2011). Altogether, this information supports our observation of a wider (i.e., more variable) isotopic niche in megalopae developing during downwelling events when contrasted to conspecifics developing during upwelling (Figure 5).
Our combined observations in C. maenas adults, newly extruded and late-stage embryos, and megalopae suggest that contrasting oceanographic conditions of downwelling and upwelling greatly affect inter-individual variability. However, it must be highlighted that the conclusions attained at adult level (i.e., with ovigerous females) should be taken with caution, as sampling 20 females per oceanographic event may underestimate diversity at population level. Overall, variability was higher during downwelling irrespective of the life stage, which can indicate that organisms can be more adaptable and resilient during these oceanographic events (Sunday et al., 2011; Foo et al., 2012; Schlegel et al., 2012). However, upwelling resulted in higher lipidic reserves and C:N ratios in embryos. Altogether, it appears that hatching and growing during upwelling conditions results not only in larger individuals but also in better fitness conditions and, ultimately, more resilient populations. Nevertheless, during downwelling the higher inter-individual variability recorded highlights the remarkable ability of C. maenas to cope and thrive in highly variable environments, likely being key for this species success as a global invader.
Data availability statement
Data is available at Zenodo and can be cited using this reference: Leal, Miguel Costa, Rey, Felisa, Ferreira, Guilherme Duarte, Domingues, M. Rosário, & Calado, Ricardo. (2022). Inter-individual variability of early life stages of a model marine invertebrate with a bi-phasic life cycle is shaped by contrasting oceanographic conditions [Data set]. https://doi.org/10.3389/fmars.2022.984569.
Author contributions
ML, FR, MD, and RC conceptualized the experiments. RC, MD and ML acquired funding and provided materials and facilities. FR collected the samples and conducted the laboratory experiments. ML performed the laboratory analysis. ML and GF analysed the data and prepared the figures. GF and ML prepared the original draft manuscript. All authors commented and contributed to the final version of the manuscript thus justifying all authorships.
Funding
This work was supported by national funds, through Fundação para a Ciência e Tecnologia (FCT), in the scope of the Individual Call to Scientific Employment Stimulus 2017 with a Junior Researcher contract [CEECIND/00580/2017 to FR] and an Assistant Researcher contract [CEECIND/01618/2020 to ML]. We also acknowledge financial support to CESAM by FCT/MCTES (UIDP/50017/2020+UIDB/50017/2020+ LA/P/0094/2020), through national funds, and to national funding through FCT, within the research project, NO RESET PTDC/BIA-BIC/116871/2010. ML was also supported by EAWAG funding. The authors also acknowledge the contribution of Serge Robert and Inês Carvalho during the laboratory analysis.
Acknowledgments
The authors acknowledge the contribution of Serge Robert and Inês Carvalho during the laboratory analysis.
Conflict of interest
The authors declare that the research was conducted in the absence of any commercial or financial relationships that could be construed as a potential conflict of interest.
Publisher’s note
All claims expressed in this article are solely those of the authors and do not necessarily represent those of their affiliated organizations, or those of the publisher, the editors and the reviewers. Any product that may be evaluated in this article, or claim that may be made by its manufacturer, is not guaranteed or endorsed by the publisher.
References
Abrams P. A., Leimar O., Nylin S., Wiklund C. (1996). The effect of flexible growth rates on optimal sizes and development times in a seasonal environment. Proc. Am. Soc Zool. 147, 381–395. doi: 10.1086/285857
Almeida M. J., González-Gordillo J. I., Flores A., Queiroga H. (2011). Cannibalism, post-settlement growth rate and size refuge in a recruitment-limited population of the shore crab Carcinus maenas. J. Exp. Mar. Biol. Ecol. 410, 72–79. doi: 10.1016/j.jembe.2011.10.011
Araújo M. S., Bolnick D. I., Layman C. A. (2011). The ecological causes of individual specialisation. Ecol. Lett. 14, 948–958. doi: 10.1111/j.1461-0248.2011.01662.x
Bartlett E. M., Lewis D. H. (1970). Spectrophotometric determination of phosphate esters in the presence and absence of orthophosphate. Anal. Biochem. 36, 159–167. doi: 10.1016/0003-2697(70)90343-X
Bearhop S., Waldron S., Votier S. C., Furness R. W. (2002). Factors that influence assimilation rates and fractionation of nitrogen and carbon stable isotopes in avian blood and feathers. Physiol. Biochem. Zool. 75, 451–458. doi: 10.1086/342800
Bligh E. G., Dyer W. J. (1959). A rapid method of total lipid extraction and purification. Can. J. Biochem. Physiol. 37, 911–917. doi: 10.1139/y59-099
Bosch T. C. G., Adamska M., Augustin R., Domazet-Loso T., Foret S., Fraune S., et al. (2014). How do environmental factors influence life cycles and development? An experimental framework for early-diverging metazoans. BioEssays 36, 1185–1194. doi: 10.1002/bies.201400065
Brillon S., Lambert Y., Dodson J. (2005). Egg survival, embryonic development, and larval characteristics of northern shrimp (Pandalus borealis) females subject to different temperature and feeding conditions. Mar. Biol. 147, 895–911. doi: 10.1007/s00227-005-1633-6
Calado R., Leal M. C. (2015). Trophic ecology of benthic marine invertebrates with bi-phasic life cycles: What are we still missing? Adv. Mar. Biol. 71, 1–70. doi: 10.1016/bs.amb.2015.07.001
Calado R., Pimentel T., Cleary D. F. R., Dionísio G., Nunes C., Da Silva T. L., et al. (2010). Providing a common diet to different marine decapods does not standardize the fatty acid profiles of their larvae: A warning sign for experimentation using invertebrate larvae produced in captivity. Mar. Biol. 157, 2427–2434. doi: 10.1007/s00227-010-1507-4
Carabel S., Godínez-Domínguez E., Verísimo P., Fernández L., Freire J. (2006). An assessment of sample processing methods for stable isotope analyses of marine food webs. J. Exp. Mar. Biol. Ecol. 336, 254–261. doi: 10.1016/j.jembe.2006.06.001
Carlton J. T., Cohen A. N. (2003). Episodic global dispersal in shallow water marine organisms: The case history of the European shore crabs Carcinus maenas and C. aestuarii. J. Biogeogr. 30, 1809–1820. doi: 10.1111/j.1365-2699.2003.00962.x
Dawirs R. R. (1981). Elemental composition (C, n, h) and energy in the development of Pagurus bernhardus (Decapoda: Pagurida) megalopa. Mar. Biol. 64, 117–123. doi: 10.1007/BF00397100
Dimitrijević D., Paiva V. H., Ramos J. A., Seco J., Ceia F. R., Chipev N., et al. (2018). Isotopic niches of sympatric gentoo and chinstrap penguins: Evidence of competition for Antarctic krill? Polar Biol. 41, 1655–1669. doi: 10.1007/s00300-018-2306-5
Domingues C. P., Almeida M. J., Dubert J., Nolasco R., Cordeiro N., Waap S., et al. (2011). Supply of crab larvae to an estuary in the eastern Atlantic upwelling system exhibits predictable and haphazard variation at different temporal scales. Mar. Ecol. Prog. Ser. 425, 113–124. doi: 10.3354/meps08992
Duarte R. C., Flores A., Vinagre C., Leal M. C. (2017). Habitat-dependent niche partitioning between colour morphs of the algal-dwelling shrimp Hippolyte obliquimanus. Mar. Biol. 164, 1–12. doi: 10.1007/s00227-017-3247-1
Duarte R. C., Ré A., Flores A., Queiroga H. (2014). Conspecific cues affect stage-specific molting frequency, survival, and claw morphology of early juvenile stages of the shore crab Carcinus maenas. Hydrobiologia 724, 55–66. doi: 10.1007/s10750-013-1712-5
Fantle M. S., Dittel A. I., Schwalm S. M., Epifanio C. E., Fogel M. L. (1999). A food web analysis of the juvenile blue crab, Callinectes sapidus, using stable isotopes in whole animals and individual amino acids. Oecologia 120, 416–426. doi: 10.1007/s004420050874
Fenchel T. (1988). Marine plankton food chains. Annu. Rev. Ecol. Syst. 19, 19–38. doi: 10.1146/annurev.es.19.110188.000315
Fernández M., Bock C., Pörtner H. O. (2000). The cost of being a caring mother: the ignored factor in the reproduction of marine invertebrates. Ecol. Lett. 3, 487–494. doi: 10.1046/j.1461-0248.2000.00172.x
Fiúza A. F. G., Hamann M., Ambar I., Del RıíO G. D. A., González N., Cabanas J. M. (1998). Water masses and their circulation off western Iberia during may 1993. Deep Sea Res. Part I 45, 1127–1160. doi: 10.1016/S0967-0637(98)00008-9
Foo S. A., Dworjanyn S. A., Poore A. G. B., Byrne M. (2012). Adaptive capacity of the habitat modifying sea urchin Centrostephanus rodgersii to ocean warming and ocean acidification: Performance of early embryos. PLoS One 7, e42497. doi: 10.1371/journal.pone.0042497
Forsman A., Wennersten L. (2016). Inter-individual variation promotes ecological success of populations and species: Evidence from experimental and comparative studies. Ecography 39, 630–648. doi: 10.1111/ecog.01357
Fox-Dobbs K., Bump J., Peterson R., Fox D., Koch P. (2007). Carnivore-specific stable isotope variables and variation in the foraging ecology of modern and ancient wolf populations: Case studies from isle royale, Minnesota, and la brea. Can. J. Zool. 85, 458–471. doi: 10.1139/Z07-018
Fry B. (1988). Food web structure on georges bank from stable c, n, and s isotopic compositions. Limnol. Oceanogr. 33, 1182–1190. doi: 10.4319/lo.1988.33.5.1182
Gilbert S. F. (2017) “Ecological developmental biology” in eLSvol. 1-8 Ed. L J. W. & Sons.(Chichester: Wiley)
Giménez L. (2010). Relationships between habitat conditions, larval traits, and juvenile performance in a marine invertebrate. Ecology 91, 1401–1413. doi: 10.1890/09-1028.1
Jackson A. L., Inger R., Parnell A. C., Bearhop S. (2011). Comparing isotopic niche widths among and within communities: SIBER – stable isotope Bayesian ellipses in r. J. Anim. Ecol. 80, 595–602. doi: 10.1111/j.1365-2656.2011.01806.x
Jungblut S., Beermann J., Boos K., Saborowski R., Hagen W. (2017). Population development of the invasive crab Hemigrapsus sanguineus (De haa) and its potential native competitor Carcinus maenas (Linnaeu) at helgoland (North Sea) between 2009 and 2014. Aquat. Invasions 12:85–96. doi: 10.3391/ai.2017.12.1.09
Kaufman T. J., Pajuelo M., Bjorndal K. A., Bolten A. B., Pfaller J. B., Williams K. L., et al. (2014). Mother-egg stable isotope conversions and effects of lipid extraction and ethanol preservation on loggerhead eggs. Conserv. Physiol. 2, cou049–cou049. doi: 10.1093/conphys/cou049
Kolasinski J., Rogers K., Frouin P. (2008). Effects of acidification on carbon and nitrogen stable isotopes of benthic macrofauna from a tropical coral reef. Rapid Commun. Mass Spectrom. 22, 2955–2960. doi: 10.1002/rcm.3694
Layman C. A., Arrington D. A., Montaña C. G., Post D. M. (2007). Can stable isotope ratios provide for community-wide measures of trophic structure? Ecology 88, 42–48. doi: 10.1890/0012-9658(2007)88[42:CSIRPF]2.0.CO;2
Leal M. C., Pochelon P. N., Da Silva T. L., Reis A., Rosa R., Calado R. (2013). Variable within-brood maternal provisioning in newly extruded embryos of Homarus gammarus. Mar. Biol. 160, 763–772. doi: 10.1007/s00227-012-2131-2
Leal M. C., Seehausen O., Matthews B. (2017). The ecology and evolution of stoichiometric phenotypes. Trends Ecol. Evol. 32, 108–117. doi: 10.1016/j.tree.2016.11.006
McAlister J. S., Moran A. L. (2012). Relationships among egg size, composition, and energy: A comparative study of geminate sea urchins. PLoS One 7, e41599. doi: 10.1371/journal.pone.0041599
McCormick M. I. (1998). Behaviorally induced maternal stress in a fish influences progeny quality by a hormonal mechanism. Ecology 79, 1873–1883. doi: 10.1890/0012-9658(1998)079[1873:BIMSIA]2.0.CO;2
Minagawa M., Wada E. (1984). Stepwise enrichment of 15N along food chains: further evidence and the relation between δ15N and animal age. Geochim. Cosmochim. Acta 48, 1135–1140. doi: 10.1016/0016-7037(84)90204-7
Moksnes P.-O. (2004). Self-regulating mechanisms in cannibalistic populations of juvenile shore crabs Carcinus maenas. Ecology 85, 1343–1354. doi: 10.1890/02-0750
Moland E., Olsen E. M., Stenseth N. C. (2010). Maternal influences on offspring size variation and viability in wild European lobster homarus gammarus. Mar. Ecol. Prog. Ser. 400, 165–173. doi: 10.3354/meps08397
Moore J. W., Semmens B. X. (2008). Incorporating uncertainty and prior information into stable isotope mixing models. Ecol. Lett. 11, 470–480. doi: 10.1111/j.1461-0248.2008.01163.x
Murphy J., Riley J. P. (1962). A modified single solution method for the determination of phosphate in natural waters. Anal. Chim. Acta 27, 31–36. doi: 10.1016/S0003-2670(00)88444-5
Newsome S. D., Martinez Del Rio C., Bearhop S., Phillips D. L. (2007). A niche for isotopic ecology. Front. Ecol. Environ. 5, 429–436. doi: 10.1890/1540-9295(2007)5[429:ANFIE]2.0.CO;2
Ospina-Alvarez N., Prego R., Álvarez I., Decastro M., Álvarez-Ossorio M. T., Pazos Y., et al. (2010). Oceanographical patterns during a summer upwelling–downwelling event in the northern Galician rias: Comparison with the whole ria system (NW of Iberian peninsula). Cont. Shelf Res. 30, 1362–1372. doi: 10.1016/j.csr.2010.04.018
Palacios K. C., Ferraro S. P. (2003). Green crab (Carcinus maenas Linnaeus) consumption rates on and prey preferences among four bivalve prey species. J. Shellfish Res. 22, 865–872.
Pardal M. A., Baeta A., Marques J. C., Cabral H. N. (2006). Feeding ecology of the green crab, Carcinus maenas (L., 1758) in a temperate estuary, Portugal. Crustaceana 79 (10), 1181–1193. doi: 10.1163/156854006778859506
Parnell A. C., Inger R., Bearhop S., Jackson A. L. (2010). Source partitioning using stable isotopes: Coping with too much variation. PLoS One 5, e9672. doi: 10.1371/journal.pone.0009672
Parsons T. R., Maita Y., Lalli C. M. (1984). A manual of chemical and biological methods for seawater analysis (Oxford, England: Pergamon Press Ltd).
Pechenik J. A. (2006). Larval experience and latent effects - metamorphosis is not a new beginning. Integr. Comp. Biol. 46, 323–333. doi: 10.1093/icb/icj028
Petersen S., Anger K. (1997). Chemical and physiological changes during the embryonic development of the spider crab, Hyas araneus L.(Decapoda: Majidae). Comp. Biochem. Physiol. 117B, 299–306. doi: 10.1016/S0305-0491(97)00091-6
Pihl L. (1985). Food selection and consumption of mobile epibenthic fauna in shallow marine areas. Mar. Ecol. Prog. Ser. 22, 169–179. doi: 10.3354/meps022169
Post D. M. (2002). Using stable isotopes to estimate trophic position: models, methods, and assumptions. Ecology 83, 703–718. doi: 10.1890/0012-9658(2002)083[0703:USITET]2.0.CO;2
Queiroga H., Almeida M. J., Alpuim T., Flores A., Francisco S., Gonzàlez-Gordillo I., et al. (2006). Tide and wind control of megalopal supply to estuarine crab populations on the Portuguese west coast. Mar. Ecol. Prog. Ser. 307, 21–36. doi: 10.3354/meps307021
Racotta I. S., Palacios E., Ibarra A. M. (2003). Shrimp larval quality in relation to broodstock condition. Aquaculture 227, 107–130. doi: 10.1016/S0044-8486(03)00498-8
R Development Core Team (2021). R: A language and environment for statistical computing (Vienna, Austria: R Foundation for Statistical Computing). Available at: http://www.R-project.org.
Rey F., Alves E., Melo T., Domingues P., Queiroga H., Rosa R., et al. (2015a). Unravelling polar lipids dynamics during embryonic development of two sympatric brachyuran crabs (Carcinus maenasand necora puber) using lipidomics. Sci. Rep. 5, 1–13. doi: 10.1038/srep14549
Rey F., Domingues M. R. M., Domingues P., Rosa R., Orgaz M. D. M., Queiroga H., et al. (2017). Effect of maternal size, reproductive season and interannual variability in offspring provisioning of Carcinus maenas in a coastal lagoon. Estuaries Coasts 40, 1732–1743. doi: 10.1007/s12237-017-0235-0
Rey F., Greenacre M., Neto G. M. S., Bueno-Pardo J., Domingues M. R., Calado R. (2022). Fatty acid ratio analysis identifies changes in competent meroplanktonic larvae sampled over different supply events. Mar. Environ. Res. 173, 105517. doi: 10.1016/j.marenvres.2021.105517
Rey F., Moreira A. S. P., Ricardo F., Coimbra M. A., Domingues M. R. M., Domingues P., et al. (2016a). Fatty acids of densely packed embryos of Carcinus maenas reveal homogeneous maternal provisioning and no within-brood variation at hatching. Biol. Bull. 230, 120–129. doi: 10.1086/BBLv230n2p120
Rey F., Neto G. M. S., Brandão C., Ramos D., Silva B., Rosa R., et al. (2016b). Contrasting oceanographic conditions during larval development influence the benthic performance of a marine invertebrate with a bi-phasic life cycle. Mar. Ecol. Prog. Ser. 546, 135–146. doi: 10.3354/meps11629
Rey F., Neto G. M. S., Bueno-Pardo J., Bispo R., Calado R. (2019). Supply and larval traits at metamorphosis of a coastal marine invertebrate with a bi-phasic life cycle under contrasting oceanographic conditions. Progr. Oceanogr. 178, 102201. doi: 10.1016/j.pocean.2019.102201
Rey F., Neto G. M. S., Rosa R., Queiroga H., Calado R. (2015b). Laboratory trials reveal that exposure to extreme raining events prior to metamorphosis affect the post-settlement performance of an estuarine crab. Estuar. Coast. Shelf Sci. 154, 179–183. doi: 10.1016/j.ecss.2015.01.005
Rice A. L., Ingle R. W. (1975). The larval development of Carcinus maenas (L.) and C. mediterraneus czerniavsky (Crustacea, brachyura, portunidae) reared in the laboratory. Bull. Br. Mus. Nat. Hist. 28, 103–119. doi: 10.5962/p.314136
Rosa R., Calado R., Andrade A., Narciso L., Nunes M. (2005). Changes in amino acids and lipids during embryogenesis of European lobster, Homarus gammarus (Crustacea: Decapoda). Comp. Biochem. Physiol. Part B: Biochem. Mol. Biol. 140, 241–249. doi: 10.1016/j.cbpc.2004.10.009
Rosa R., Calado R., Narciso L., Nunes M. L. (2007). Embryogenesis of decapod crustaceans with different life history traits, feeding ecologies and habitats: a fatty acid approach. Mar. Biol. 151, 935–947. doi: 10.1007/s00227-006-0535-6
Rosa R., Morais S., Calado R., Narciso L., Nunes M. L. (2003). Biochemical changes during the embryonic development of Norway lobster, Nephrops norvegicus. Aquaculture 221, 507–522. doi: 10.1016/S0044-8486(03)00117-0
Schlegel P., Havenhand J. N., Gillings M. R., Williamson J. E. (2012). Individual variability in reproductive success determines winners and losers under ocean acidification: A case study with sea urchins. PLoS One 7, e53118. doi: 10.1371/journal.pone.0053118
Sommer U., Stibor H., Katechakis A., Sommer F., Hansen T. (2002). Pelagic food web configurations at different levels of nutrient richness and their implications for the ratio fish production: Primary production. Hydrobiologia 484, 11–20. doi: 10.1023/A:1021340601986
Sultan S. E. (2017). “Eco-Evo-Devo,” in Evolutionary developmental biology: A reference guide. Eds. Nuno de la Rosa L., Müller G. (Cham: Springer).
Sunday J. M., Crim R. N., Harley C. D. G., Hart M. W. (2011). Quantifying rates of evolutionary adaptation in response to ocean acidification. PLoS One 6, e22881. doi: 10.1371/journal.pone.0022881
Swearer S. E., Treml E. A., Shima J. S. (2019). A review of biophysical models of marine larval dispersal. Oceanogr. Mar. Biol.: Annu. Rev. 57, 325–356. doi: 10.1201/9780429026379-7
Swiney K. M., Eckert G. L., Kruse G. H. (2013). Does maternal size affect red king crab, Paralithodes camtschaticus, embryo and larval quality? J. Crustacean Biol. 33, 470–480. doi: 10.1163/1937240X-00002162
Tang K. W., Dam H. G. (1999). Limitation of zooplankton production: beyond stoichiometry. Oikos 84, 537–542. doi: 10.2307/3546434
Tarran G. A., Burkill P. H., Edwards E. S., Woodward E. M. S. (1999). Phytoplankton community structure in the Arabian Sea during and after the SW monsoo. Deep Sea Res. Part II 46, 655–676. doi: 10.1016/S0967-0645(98)00122-2
Torres G., Melzer R. R., Spitzner F., Šargač Z., Harzsch S., Gimenez L. (2021). Methods to study organogenesis in decapod crustacean larvae. i. larval rearing, preparation, and fixation. Helgol. Mar. Res. 75, 3. doi: 10.1186/s10152-021-00548-x
Torres G., Spitzner F., Harzsch S., Giménez L. (2019). “Ecological developmental biology and global ocean change: brachyuran crustacean larvae as models,” in Perspectives on evolutionary and developmental biology. Ed. Fusco. G. (Padova, Italy: Padova University Press) 283–306.
Tuck I. D., Taylor A. C., Atkinson R. J. A., Gramitto M. E., Smith C. (1997). Biochemical composition of nephrops norvegicus: Changes associated with ovary maturation. Mar. Biol. 129, 505–511. doi: 10.1007/s002270050191
Vidal T., Calado A. J., Moita M. T., Cunha M. R. (2017). Phytoplankton dynamics in relation to seasonal variability and upwelling and relaxation patterns at the mouth of ria de aveiro (West Iberian margin) over a four-year period. PLoS One 12, e0177237. doi: 10.1371/journal.pone.0177237
Villar-Argaiz M., Medina-Sánchez J. M., Carrillo P. (2002). Linking life history strategies and ontogeny in crustacean zooplankton: Implications for homeostasis. Ecology 83, 1899–1914. doi: 10.1890/0012-9658(2002)083[1899:LLHSAO]2.0.CO;2
Wada E., Terazaki M., Kabaya Y., Nemoto T. (1987). 15N and 13C abundances in the antartic ocean with emphasis on the biogeochemical structure of the food web. Deep-Sea Res. Part A 34, 829–841. doi: 10.1016/0198-0149(87)90039-2
Warton D. I., Hui F. K. C. (2011). The arcsine is asinine: The analysis of proportions in ecology. Ecology 92, 3–10. doi: 10.1890/10-0340.1
Werner E. E. (1991). Nonlethal effects of a predator on competitive interactions between two anuran larvae. Ecology 72, 1709–1720. doi: 10.2307/1940970
Wilson J. R., Wilkerson F. P., Blaser S. B., Nielsen K. J. (2021). Phytoplankton community structure in a seasonal low-inflow estuary adjacent to coastal upwelling (Drakes estero, CA, USA). Estuaries Coasts 44, 769–787. doi: 10.1007/s12237-020-00792-3
Yeakel J. D., Bhat U., Elliott Smith E. A., Newsome S. D. (2016). Exploring the isotopic niche: Isotopic variance, physiological incorporation, and the temporal dynamics of foraging. Front. Ecol. Evol. 4. doi: 10.3389/fevo.2016.00001
Keywords: downwelling, upwelling, carcinus maenas, inter-individual variability, maternal effects, isotopic niche, developmental stages
Citation: Leal MC, Rey F, Ferreira GD, Domingues MR and Calado R (2022) Inter-individual variability of early life stages of a model marine invertebrate with a bi-phasic life cycle is shaped by contrasting oceanographic conditions. Front. Mar. Sci. 9:984569. doi: 10.3389/fmars.2022.984569
Received: 02 July 2022; Accepted: 22 August 2022;
Published: 12 September 2022.
Edited by:
Francesca Porri, South African Institute for Aquatic Biodiversity, South AfricaReviewed by:
Nancy O’Connor, University of Massachusetts Dartmouth, United StatesSimone Baldanzi, Universidad de Valparaiso, Chile
Copyright © 2022 Leal, Rey, Ferreira, Domingues and Calado. This is an open-access article distributed under the terms of the Creative Commons Attribution License (CC BY). The use, distribution or reproduction in other forums is permitted, provided the original author(s) and the copyright owner(s) are credited and that the original publication in this journal is cited, in accordance with accepted academic practice. No use, distribution or reproduction is permitted which does not comply with these terms.
*Correspondence: Miguel Costa Leal, miguelcleal@gmail.com; Ricardo Calado, rjcalado@ua.pt