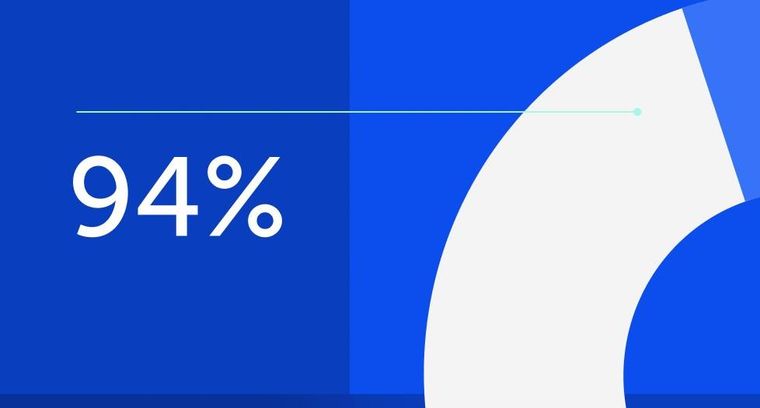
94% of researchers rate our articles as excellent or good
Learn more about the work of our research integrity team to safeguard the quality of each article we publish.
Find out more
ORIGINAL RESEARCH article
Front. Mar. Sci., 02 September 2022
Sec. Marine Biotechnology and Bioproducts
Volume 9 - 2022 | https://doi.org/10.3389/fmars.2022.981468
This article is part of the Research TopicBoosting the Potential of Algae for Biomass Production, Valorisation, and BioremediationView all 9 articles
Anthropogenic impacts on water resources, especially by nutrient discharge, is a worldwide problem in marine coastal areas. In this context, seaweed cultivation in aquaculture wastewater can be considered as an alternative for effluent mitigation, where the biomass becomes a source of valuable compounds. The current study examined the potential use of the seaweeds Ulva pseudorotundata and Ulva rigida to remove nutrients to treat effluents from the culture of Chelon labrosus. Two experiments were conducted under pilot-scale conditions to evaluate the nutrient uptake, photosynthetic activity, and biomass production of the seaweed species cultivated under 50 and 100% effluent concentrations. Photosynthetic parameters were determined by in vivo chlorophyll a fluorescence associated to photosystem II 3 times a day to estimate photosynthetic performance and seaweed physiology throughout the experiment: optimal quantum yield (Fv/Fm), in situ and ex situ electron transport rate (ETR), photosynthetic efficiency (αETR), saturation irradiance (Ek), and the maximum non-photochemical quenching (NPQmax). To evaluate seaweed metabolism and biomass compounds, elemental and biochemical composition were analyzed in the beginning and end of each experiment. Results regarding the nutrient source showed that both species removed more than 65% of ammonium after 3 hours of experimentation. At the end of the experiments, up to 94.8% of the initial ammonium was sequestered from the effluent. Additionally, after 5 days of cultivation under 50% fish effluent both Ulva species were able to remove more than 85% of the nitrate. Although a decrease in uptake efficiency was observed in cultures with 100% fish effluent, at the end of the experiment more than 440 µmol L-1 of nitrate was removed, considering all treatment conditions. The biomass values showed that growth rates of seaweed cultivated in 100% effluent were higher than those obtained in 50% effluent. Moreover, when cultivated in the 100% effluent concentration, a significant increment in protein content was detected in both Ulva species. Our results contribute to the understanding of biofiltration and photosynthetic performance of two different Ulva species in order to improve growth optimization, enhancement of biofiltration capacity and also to boost management practices of seaweed cultivation in aquaculture effluent treatment systems.
Eutrophication and the exploitation of natural resources resulting from anthropogenic activity have drastically modified marine coastal areas in the past few decades (Smith et al., 1999; Lotze et al., 2006). Furthermore, climate change is a threat to marine ecosystems, which is an emerging concern for coastal and ocean resilience. According to the United Nations Decade of Ocean Science for Sustainable Development (2021-2030) (IOC-UNESCO, 2020), political strategy and scientific knowledge will be required in the coming years in order to mitigate risk and develop sustainable practices. Hence, the search for innovative technologies that promote structural transformation and sustainable solutions is imperative, aiming to reduce the impact of economic activities on natural resources. The algal industry is an emerging sector that can be exploited in terms of blue bioeconomy, since it holds a very high potential from an economic, social and environmental perspective (Araújo et al., 2021). Algae production also plays an important role in ecosystem services and has the potential to mitigate climate change by reducing carbon emissions (Froehlich et al., 2019). The diversity of micro- and macroalgae (or seaweed) represents a source of a vast number of valuable compounds, which can be applied to biotechnological approaches in the cosmeceutical, pharmaceutical, agricultural and food industries (Buschmann et al., 2017; Jusadi et al., 2021; Vega et al., 2021)
According to the Food and Agriculture Organization of the United Nations (FAO, 2020), world algae aquaculture production has increased over the years, reaching 32.4 million tons of biomass in 2018, which is dominated by seaweeds. Much of the productivity is still limited to countries in Eastern and Southeastern Asia, however, as bioeconomic development grows worldwide, seaweed farming is gaining attention, especially in European countries. Many different technologies have been developed to produce seaweed, and their incorporation in fish, crustacean, and mollusc farms is growing as well. Integrating Multi-Trophic Aquaculture (IMTA) systems consists of the coupling of seaweed production with mariculture waste waters, which decreases the amount of inorganic nutrients in the discharge effluent. IMTA systems are considered an eco-friendly practice since they reduce the impact of the aquaculture industry and increase the overall profitability of fish production through the cultivation of algal biomass which can be applied in different economic sectors (Shpigel et al., 1993; Chopin et al., 2001; Neori et al., 2004). When practiced inland, IMTA systems require a deep understanding of broad concepts such as: systems design and scale, seaweed biomass quality and value, and how to improve the efficiency of algae species to absorb and remove nutrients from mariculture waste. Seaweed culture conditions (e.g. depth, light, stocking density, water turnover rates) and specific properties of the seaweed species (e.g. biology and physiology) are the main factors contributing to the nutrient reduction efficiency and uptake rate. Moreover, understanding 1) how seaweed biofiltering performance changes over diurnal and seasonal cycles, 2) which local seaweed strains/species can perform better in biofiltering effluents, and 3) what factors, other than light and nutrients, may narrow seaweed production, is also important for the successful implementation of integrated aquaculture systems (Troell et al., 2003). Thus, great efforts are made to understand algal physiology in order to improve growth optimization, upscaling of production volumes, enhancement of biofiltration capacity, and management practices of seaweed cultivation in aquaculture effluent treatment systems.
The green macroalgae genus Ulva is one of the most studied seaweeds for its use as a biofilter of dissolved nutrients from mariculture effluents. Ulva has a worldwide distribution, with 99 taxonomically recognized species (Guiry and Guiry, 2022), playing an important ecological role in marine nutrient cycling and provisioning a source of food and habitat for a diversity of species. Ulva spp. are frequently associated with the formation of massive algal aggregations denominated “green tides'', commonly in eutrophicated areas where elevated nutrient levels in seawater are observed (Ye et al., 2011; Liu et al., 2013; Smetacek and Zingone, 2013). Fast growth rates, phenotypic plasticity, the ability to rapidly remove nutrients, and the potential for various biomass applications make these robust seaweeds stand out for cultivation in onshore and offshore systems (Neori et al., 2003; Guttman et al., 2018; Steinhagen et al., 2022). Many studies demonstrate the efficiency of using Ulva spp. as a biofilter of fish effluents since it rapidly absorbs organic and inorganic nutrients, especially ammonium (Cohen and Neori, 1991; del Río et al., 1996; Ben-Ari et al., 2014; Nardelli et al., 2019). Under outdoor conditions, the efficiency of ammonia removal by Ulva in IMTA systems may reach more than 80%, which greatly improves the quality of mariculture effluents (Copertino et al., 2009; Al-Hafedh et al., 2014; Macchiavello and Bulboa, 2014). Moreover, dried Ulva spp. biomass can contain up to 40% protein (Shpigel et al., 2019; Shahar et al., 2020), and is a source of cell wall polysaccharides that are sought after for biotechnology applications (Kidgell et al., 2019). In this context, Ulva spp. can act as bioremediators by extracting waste nutrients from mariculture effluents and converting them into biomass that may be beneficial as a source of raw material. Nonetheless, nutrient uptake performance and growth rate can vary among species, depending on local environmental conditions (photoperiod and temperature) and the characteristics of the cropping system (nutrient loads, salinity, turbidity) which can affect the biofiltration performance and the cultivation biochemical composition.
Ulva species have also been used as a model organism in ecophysiology studies where relationships between photosynthesis and chlorophyll-a fluorescence have been explored (Osmond et al., 1993; Cruces et al., 2019). Photosynthesis assessments of Ulva spp. using Pulse Amplitude Modulation (PAM) of chlorophyll-a fluorescence has allowed inferences about the process of photoinhibition and the triggering of photoinhibitory damage and photoprotection mechanisms. Cruces et al. (2019) evaluated the photosynthetic performance of U. rigida using PAM and were able to quantify electron transport rates and aspects of photoinhibition in response to diurnal changes in solar radiation. Electron transport rates measured by PAM can also vary with nitrate concentration. Cabello-Pasini and Figueroa (2005) demonstrated that the maximum rate of electron transport (ETRmax) of U. rigida increased with the increase of nitrate in the medium. Figueroa et al. (2020) conducted experiments with U. rigida cultivated under predicted values of pCO2, nutrient enrichment and temperature that showed multiple aspects of photosynthetic parameters obtained by PAM measurements and provided information about the physiological impacts of future global changes.
Researches with other seaweed species showed that PAM was a useful tool to evaluate photosynthetic responses of seaweed cultured under multiple factors such as irradiance (Figueroa et al., 2006; Mata et al., 2006; Kang et al., 2008), temperature (Figueroa et al., 2009), system aeration (Figueroa et al., 2006), and different nitrogen sources (Shahar et al., 2020). Understanding photosynthesis and the biofiltration process can help to improve aspects of IMTA cropping systems. In the present study, two seaweed species naturally occurring in the Mediterranean and with potential for use in biofiltration (U. pseudorotundata and U. rigida) were evaluated for their (1) ability to remove dissolved inorganic nutrients (NH4+, NO3- and PO43-), (2) photosynthetic performance, (3) biomass productivity, and (4) biomass elemental and biochemical composition. The experiments were carried out on a land-based pilot scale using fishpond effluents at two different concentrations. The main objective was to compare and parameterize the two species for use in biofiltration processes associated with IMTA systems.
The Ulva species used in this study were collected in two different places: in the bay of Cádiz (36°30'N, 6°10'W), where specimens were collected from salt marsh areas, and in the bay of Málaga (36°42’N, 4°19’W), where Ulva sp. specimens were collected fixed to rocky intertidal shores, both situated in Andalusia, Spain (Figure 1). The algae were transported to the Grice Hutchinson Research Center at Malaga University where the experiments were conducted. After collection, small pieces of algal material were separated for species-level identification using molecular methods. Clean and healthy fronds of Ulva were maintained in rectangular tanks (200 L; 1 m2 surface area and 50 cm depth) and exposed to full solar radiation in which air diffusers situated at the bottom of each tank kept the algae unattached and suspended. Prior to the experiments, seaweed biomass was grown in artificial seawater and supplemented twice a week with NH4Cl (300 µmol L-1), KNO3 (300 µmol L-1) and KH2PO4 (48 µmol L-1). The salinity and pH of the seawater were 35 and 8.0, respectively.
Algal samples were dried in silica-gel and submitted to DNA extraction, amplification, sequencing, and identification. The large subunit of the plastid-encoded Ribulose Bisphosphate Carboxylase-Oxygenase (RuBisCO) gene region (rbcL) was amplified in a polymerase chain reaction (PCR) using published primer pairs SHF1 (CCGTTTAACTTATTACACGCC) and SHR4 (TTACATCACCACCTTCAGATGC) (Heesch et al., 2009). The following were added to each sample: 0.25 µL of Taq DNA polymerase (5 unit µL-1) (Qiagen), 2.5 µL of CoralLoad PCR Buffer, 2.5 µL of dNTPS (2 mM), 5 µL of Q-solution, 3.5 µL MgCl (25 mM), 1 µL of each primer (10 µM), 2 µl of total DNA, and 7.25 µL of MiliQ water, leading to a final volume of 25 µL. PCR proceeded as follows: denaturation at 94 °C for 3 minutes, followed by 40 cycles of 94 °C for 1 minute and 30 seconds of denaturation, 37 °C for 2 minutes for annealing step , and then 72°C for 3 minutes for final extension. The strings were aligned and edited in Geneious 10.0.9. The species identification was carried out through BLASTn and compared in the GenBank database.
The evolutionary history was inferred by using the Maximum Likelihood method and Kimura 2-parameter model (Kimura, 1980). The bootstrap consensus tree inferred from 1000 replicates (Felsenstein, 1985) was taken to represent the evolutionary history of the taxa analyzed. Branches corresponding to partitions reproduced in less than 50% bootstrap replicates were collapsed. Initial tree(s) for the heuristic search were obtained by applying the Neighbor-Joining method to a matrix of pairwise distances estimated using the Maximum Composite Likelihood (MCL) approach. A discrete Gamma distribution was used to model evolutionary rate differences among sites (5 categories (+G, parameter = 0.1073)). The rate variation model allowed for some sites to be evolutionarily invariable ([+I], 45.04% sites). This analysis involved 19 nucleotide sequences. There were a total of 675 positions in the final dataset. Evolutionary analyses were conducted in MEGA11 (Tamura et al., 2021).
To assess the biofiltration capacity of the seaweed, two consecutive experiments were conducted using effluents from the cultivation of Chelon labrosus (Osteichthyes, Mugilidae). The fish effluent was obtained from a closed recirculating water system connected to a filter with chemoautotrophic nitrifying bacteria. Chemical composition of the effluents was characterized prior to experiments by ion chromatography (METROHM 863 Compact IC Autosampler). The first experiment was performed during 5 days using an effluent diluted to 50% with tap water. For the second one, we used pure effluent and it lasted 4 days. At the beginning of each experiment, U. pseudorotundata and U. rigida biomass was weighed at initial stocking densities of 6.0 g FW L–1 (n = 3). These seaweed stocking densities were established based on previous studies of U. lactuca cultivated in abalone culture effluent which demonstrated high uptake efficiency in removing NH4+, NO3-, and PO43- (Macchiavello and Bulboa, 2014). Both experiments started at 1:00 pm (local time) and the algae were kept without nutrients before the beginning of the experiments to provide starvation conditions. During experiments, pH, dissolved oxygen, and salinity were measured daily using portable devices (LAQUAact PH110, HANNA Instruments) (Table S1). Temperature and Photosynthetically Active Radiation (PAR, λ = 400-700 nm) were recorded every 15 minutes from HOBO data loggers (Zippo-HOBO U12, SQ-212 PAR) installed in one of the tanks. Integrated daily irradiance (kJ m-2) was calculated from PAR data to obtain total light energy received by cultures during the experiment.
To evaluate the nutrient removal performance of the algae, water samples were obtained from each tank three times a day for NO3- analysis (morning 8:00 - 9:00, noon 12:00 - 13:00 and evening 16:00 - 17:00); and once a day for NH4+ and PO43- analysis (evening 16:00 - 17:00), except the first day, when samples were taken at 13:00 and 16:00. Aliquots of 10 mL were filtered (GF/F Whatman) and stored at −20°C in polyethylene flasks for further analysis using a segmented flow analyzer (SFA; Seal Analytical autoanalyzer QuAAtro) according to the methods described by (Grasshoff et al., 1999).
Nutrient uptake efficiency (NUE) was calculated as:
where Ct represents the initial concentration of nutrients, Ct+1 represents the concentration of nutrients after t+1. The nutrient uptake rate (NUR), which represents the amount of nutrients removed per unit of time, per volume, per unit of seaweed dry weight, was determined from changes in nutrient concentrations according to the following equation:
where Ct represents the initial concentration of nutrients, Vt represents the initial volume of water (in L), Ct+1 represents the concentration of nutrients after t+1, Vt+1 represents the volume of water after t+1 (in L), B represents the dry biomass used (in grams), and Δt represents the time interval between t and t+1 (in hours).
Photosynthetic performance was assessed at the same time points as nitrate sampling described above by in vivo chlorophyll-a fluorescence of Photosystem II (PSII) measured in situ and ex situ. Measurements in situ were conducted using a Pocket-PAM (Gademann Instruments, Würzburg, Germany) on five algal samples from each tank to obtain the effective quantum yield of PSII (ΔF/Fm′) using the equation:
where Fm′ is the maximum fluorescence in light, induced by a saturating pulse, and Ft, the basal fluorescence emitted by an organism pre-acclimated to light conditions. The data of ΔF/Fm′ obtained was used to calculated the Electron Transport Rate (ETRsitu) associated with PSII according to Genty et al. (1989):
where EPAR is the PAR (μmol photons m-2 s-1) obtained by HOBO at the same time of the measurements, A was the absorptance of the algal thallus, and FII was a constant that represents the fraction of cellular chlorophyll a associated with the light harvesting complex of PSII being 0.5 the value for green algae (Figueroa et al., 2003). Absorptance of the algal thallus was calculated according to (Figueroa et al., 2009) as follows:
where Eo is the incident irradiance of a lamp determined by a sensor Li-189 (LI-COR Ltd, Nebraska, USAd) connected to a radiometer Li-250 (LI-COR Ltd, Nebraska, USA), and Et is the transmitted irradiance, measured by placing a small piece of the thallus above the PAR sensor.
Ex situ measurements consisted of rapid light-response curves (RLC), which were obtained using a Junior PAM (Walz GmbH, Effeltrich Germany) connected to a PC running WinControl software. Algal samples were transported under dark conditions from the outdoor tanks to the laboratory. After dark-adapted for at least 15 min, the maximum quantum yield of PSII Fv/Fm was obtained from the first saturating pulse and determined by the equation:
where F0 is the initial fluorescence of PSII (intrinsic fluorescence from the antenna of fully oxidized PSII); Fm is the maximum fluorescence of PSII after a saturating light pulse (0.4 s, approx. 9000 µmol photons m-2 s-1), and Fv is the variable fluorescence corresponding to the difference between Fm and F0. Afterwards, to estimate the electron transport rate (ETR), the same samples were exposed for 30s to twelve increasing irradiances (25, 45, 66, 90, 125, 190, 285, 420, 625, 845, 1150, and 1500 μmol photons m-2 . s-1) of actinic blue light, each one followed by a saturating blue light pulse. ETRs were calculated as previously described and plotted against the actinic light intensities generating light curves that were fitted according to the Eilers and Peeters (1988) model to obtain photosynthetic parameters (ETRmax,αETR, and Ek) in which ETRmax represents the maximum electron transport rates, αETR is the algal photosynthetic efficiency and Ek saturation irradiance for the photosynthetic electron transport. Non-photochemical quenching (NPQ), which corresponds to the total energy dissipation, was calculated after each saturating pulse during the RLCs, and NPQ versus irradiance relationships were determined. Curves were then fitted using Eilers and Peeters (1988) models, allowing the calculation of maximum NPQ values (NPQmax). All rapid light curves were fitted using the statistical environment R, version 4.2 (The R Development Core Team, 2022).
Growth rate, biomass productivity (also known as biomass yield), and biochemical composition were measured from the biomass obtained at the beginning and at the end of the experiments. Growth rates (GR) were calculated according to Lignell and Pedersén (1989):
where Wi = initial wet weight, Wt = wet weight after t days and t = days of cultivation. The productivity of the tanks was calculated according to (Mata et al., 2003):
where, Wt = wet weight after t days, Wi = initial wet weight, t = the number of days, (FW/DW) is the fresh weight/dry weight ratio, and A is the area covered by the tank in m2. To obtain the fresh weight/dry weight ratio, ten samples of ~3 g were dried to constant weight at 60˚C (48–72 h). Triplicate seaweed samples of each tank (~5 g FW) were taken at the beginning and end of the experiments, washed with MiliQ-water to remove salt and freeze-dried for 48h (Telstar Lyoquest-55) for tissue biochemical analysis. 2 mg of dried biomass were submitted for carbon, hydrogen, and nitrogen content analyses using a LECO CHNS-932 elemental analyzer (Michigan, USA). C, H and N values were expressed as percentage of dry weight and the C:N ratio was determined.
The daily N content of the produced biomass was also used as an estimate of the biofiltration capacity, being calculated by multiplying the biomass yield by the N content of the seaweeds (Mata et al., 2010). Total protein content was estimated according to Shuuluka et al. (2013) by multiplying the total N by a factor of 5.45. Chlorophyll a (Chl a), chlorophyll b (Chl b) and total carotenoids (Car) were extracted from samples of the Ulva spp. thalli (10-15 mg DW) by incubating them in 5-10 mL of acetone 90% + C4Mg4O12 for 12 h in the dark at 4°C and then centrifuging for 15 min at 4°C and 5000 rpm. Subsequently, the absorbances were measured photometrically at 480, 647, 664, and 750 nm (UV-2600 Shimadzu). The contents of Chl a, Chl b, and Car (mg g-1 DW) were calculated according to Ritchie (2006) and Parsons and Strickland (1963), respectively.
The averages of integrated daily irradiance and temperature were analyzed to compare experiment 1 and 2 by a test of independent samples (t-student) using the STATISTICA 12 software (StatSoft, Inc. 2011). We tested the effect of effluent (two levels), species identity (two levels), hour (five levels), day (five levels) and their interaction on nutrient concentrations (NO3-, NH4+ and PO43-) , algal biofiltration capacity (NUE and NUR), photosynthetic performance (Fv/Fm, ETRsitu, ETRmax), biomass growth (growth rate, productivity, N uptake) and composition (hydrogen, nitrogen, carbon, C:N ratio, protein, Chl a, Chl b, carotenoids) using analyses of variance (ANOVA) with permutation tests, as implemented in the ‘lmPerm’ package in R (Wheeler, 2016). We chose permutational methods since they are suitable when sample sizes are small and do not make assumptions about normal distribution. Permutation analysis randomizes the data set while retaining the data structure to generate all possible permutations of the values obtained in the experiment. Then, the p-value is obtained comparing each permuted data set to the raw data set to assess whether the treatment effects are the same or greater. When the null hypothesis was rejected after ANOVA, we followed with Tukey’s HSD post-hoc tests for multiple pairwise comparisons.
First, to test for changes in nutrient concentration during the experiment, days (between-group), hours (within-group), species identity (within-group) and their interaction were used as independent variables in a three-way ANOVA, where NO3-, NH4+ and PO43-, were used as response variables in each model. Second, to test for differences in the performance of nutrient removal between algal species during the experiment, days (between-group), species identity (within-group), and their interaction were used as predictors in a two-way ANOVA, whereas NUE and NUR were used as response variables in each model. Then, to test for differences in the photosynthetic performance between algal species during the experiment, days (between-group), hours (within-group), species identity (within-group) and their interaction were used as independent variables in a three-way ANOVA, where Fv/Fm, ETRsitu, ETRmax were used as response variables in each model. Finally, to test for differences in the biomass growth and composition between algal species and effluent concentration, effluent (between-group), species identity (within-group) and their interaction were used as independent variables in a two-way ANOVA, where growth rate, productivity, N uptake, hydrogen, nitrogen, carbon, C:N ratio, protein, Chl a, Chl b and carotenoids were used as response variables in each model.
Spearman’s correlation analyses were performed to measure the strength and direction of associations among chlorophyll-a fluorescence parameters (ETRsitu, Fv/Fm, ETRmax, αETR, Ek, and NPQmax), and algal nitrate biofiltration capacity (NUE and NUR) using the function ggpairs from the ‘GGally’ package (Schloerke et al., 2021). We used non-parametric statistics since no normal distribution was found (p-value > 0.05; Shapiro test).
Multiple linear models with permutation tests were performed using the ‘lmPerm’ package in R (Wheeler, 2016) to assess the relationship between nutrient uptake efficiency (NUE) and photosynthetic performance. The variables used as predictors in the models were Fv/Fm, αETR, Ek, ETRmax, NPQmax, and ETRsitu. Only significant (p-value < 0.05) predictors were retained in the model (αETR, NPQmax, and ETRsitu). Then, we used simple linear models to assess the relationship between each predictor and NUE. The adjusted coefficient of determination (adjusted-R2) was used to examine the fraction of the explained variance by the model. Statistical analyses described above were performed in the R statistical software version 4.2.0 (R Core Team 2022).
Ulva specimens collected from the Spanish Mediterranean coast were identified as Ulva pseudorotundata, from the bay of Cádiz, and Ulva rigida, from the bay of Málaga (Figure 2). Alignment of rbcL DNA sequences obtained from algal samples showed a monophyletic clade for each species in a phylogenetic tree with the sequences found in GenBank for U. rigida and U. pseudorotundata. Sequences obtained from U. pseudorotundata were grouped in the same clade as U. rotundata collected from Ireland (Loughnane et al., 2008; Wan et al., 2017) and, according to Guiry et al. (2014), could be considered as the same species since the specific epithet rotundata it's not usual anymore.
Figure 2 Ulva species identification utilized in this study. Molecular identification of algal material, considering a phylogenetic tree obtained by RbcL sequences (SHF1 and SHR4 markers). ⬛U. pseudorotundata; ⚫U. rigida.
The concentration of the main ions presented in Chelon labrosus effluents used as culture media for the algae are presented in Table 1. Clearly the dominant nitrogen form in the effluents was . Low values of were detected since the subsequent oxidation of this nutrient was carried out by chemoautotrophic nitrifying bacteria in the fishing system cultivation. The N:P molar ratios were approximately 12 for both effluents, which is about half the typical value for the main algal culture media used (e.g. F/2 and von Stosch; Andersen, 2005).
Table 1 Chemical composition of Chelons labrosus effluent used in experiment 1 (50% fish effluent) and experiment 2 (100% fish effluent) for U. pseudorotundata and U. rigida cultivation. Concentration (µmol L-1) values are mean ± SD, n = 2.
Temperature, PAR and daily integrated irradiance obtained from algal tanks during experiments 1 and 2 are presented in Figure 3. Temperature and PAR clearly presented daily patterns of low values in the mornings and evenings and higher values at noon. For experiment 1, daily mean values of irradiance varied from 289.88 to 748.29 μmol photons m−2 s−1 during the experimental period and temperature ranged from 8.0 to 28.0°C (Figure 3A). For experiment 2, the irradiance oscillated from 149.15 to 603.13 μmol photons m−2 s−1 and temperature from 12.0 to 35.0°C (Figure 3B). No significant differences were observed between the two experiments when comparing the average values of integrated daily irradiance (t-student, t(7) = 1.526, p = 0.17). The same was observed when comparing the averages of daily temperatures (t-student, t(7) = -0.745, p = 0.48).
Figure 3 Variation in temperature (°C, dotted line) and photosynthetically active radiation (PAR, µmol photons m-2 s-1, solid line) data during the experimental cultivation period of U. pseudorotundata and U. rigida in fish effluents. (A) daily temperature and daily solar irradiance for experiment 1 (50% fish effluent) and (B) daily temperature and daily solar irradiance for experiment 2 (100% fish effluent). PAR: λ = 400-700 nm. Gray areas correspond to dark periods. The integrated daily irradiance values (kJ m-2) are displayed at the top of the graphs.
Both Ulva species showed similar capacity for the removal of NO3-, when cultivated in C. labrosus fishpond effluents (Figures 4A–F) and significantly reduced concentrations of these nutrients over time in the two experimental conditions (Table S2). Considering , at the end of experiment 1 U. pseudorotundata reached a removal of 672.0 ± 86.2 µmol L-1 while U. rigida removed 700.6 ± 28.0 µmol L -1, but there was no statistical difference between the performances of the two species throughout the experiment (Figure 4A, ANOVA: F(1) = 6073.0, p = 0.134, Table S2). In experiment 2, cultivated with 100% effluent, U. pseudorotundata removed about 582.8 ± 41.8 µmol L-1 while U. rigida was responsible for the reduction of 441.3 ± 26.5 µmol L-1 from water, with no significant differences between the two species on the last day (Figure 4D, Tukey post hoc test, p = 1.0).
Figure 4 Changes in concentrations of NO3-, NH4+, and PO43- (µmol L-1) during the experimental cultivation of U. pseudorotundata and U. rigida. (A–C) refer to experiment 1, conducted during 5 days with 50% fish effluent. (D–F) refer to experiment 2, conducted during 4 days with 100% fish effluent. Values are mean ± SD, n = 3.
removal was fast and efficient for the two species in both experiments. In experiment 1 both species removed more than 95% of in just 3 hours, reaching 78.7 ± 9.7 µmol L-1 for U. pseudorotundata and 69.0 ± 4.9 µmol L-1 for U. rigida (Figure 4B). A sharp decline was also observed in experiment 2 (Figure 4E), leading to a final removal of 225.1 ± 5.6 µmol L-1 for U. rigida and 236.0 ± 8.6 SD µmol L-1 for U. pseudorotundata. The removal of was less pronounced than the nitrogen sources, with both species being equally efficient at removing about 20 ± 3.3 µmol L-1 in both experiments (Figures 4C, F).
The two species evaluated showed similar patterns for both biofiltration parameters (NUR and NUE) in the two conditions tested (50% and 100% fish effluent) (Figures 5A–F). For nitrogenous nutrients, the uptake rates reached a maximum on the 1st day then decreased over time. In experiment 1, uptake rates for differed significantly (ANOVA: , p < 0.001; , p < 0.001, Table S3) between the two species (Figures 5A, B). On the 1st day, U. rigida exhibited the highest uptake rate (Tukey post hoc test, p < 0.001) reaching values of 105 ± 2.7 μmol g-1 DW h-1 (Figure 5A). However, the uptake rate was higher (Tukey post hoc test, p < 0.001) in U. pseudorotundata (31.9 ± 4.0 μmol g-1 DW h-1) than in U. rigida (19.7 ± 4.2 μmol g-1 DW h-1 ) when compared at the same moment (Figure 5B). After the initial drop, the uptake rates for showed similar patterns until the end of the experiment. In experiment 2, NUR values on the 1st day were different between the two species for both nitrogenous nutrients (Tukey post hoc test, p < 0.001), and U. pseudorotundata showed better performance, reaching 57.8 ± 4.5 μmol g-1 DW h-1 of and 81.8 ± 0.9 μmol g-1 DW h-1 of (Figures 5D, E).
Figure 5 NO3-, NH4+, and PO43- removal rate - NUR (bars) and uptake efficiency - NUE (symbols) by U. pseudorotundata and U. rigida in fish effluents. (A–C) refer to experiment 1, conducted during 5 days with 50% fish effluent. (D–F) refer to experiment 2, conducted during 4 days with 100% fish effluent. Values are mean ± SD, n = 3.
Maximum uptake efficiency for (NUE) was reached at the end of each experiment and values ranged between 85.1% and 91.3% in experiment 1 and from 29.9% to 36.9% in experiment 2, considering both species (Figures 5A, D). was biofiltered at a high percentage in all cultures just after 3 hours of seaweed treatment in both experiments. On the first day NUE reached at least 95% in experiment 1, while in experiment 2 the values ranged between 65.9-89.5%. At the end of both experiments, the two species removed up to 94.8% of (Figures 5B, D). Regarding , in experiment 1 both species showed equivalent removal rates (ANOVA: F(1) = 0.019, p = 0.155, Table S3), ranging from 2.4 to 0.2 μmol g-1 DW h-1 for U. pseudorotundata and 2.6 to 0.2 μmol g-1 DW h-1 for U. rigida (Figure 5C). However, in experiment 2 there was a significant difference associated with the interaction between species and time (ANOVA: F(3) = 0.139, p < 0.001, Table S3), with the NUR for being higher in U. pseudorotundata (0.42 ± 0.18 μmol g-1 DW h-1 ) than in U. rigida (0.34 ± 0.06 μmol g-1 DW h-1 (Figure 5F).
Photosynthetic parameters measured during the experimental period by PAM-fluorometers are presented in Figures 6A–F. In general, both Ulva species exhibited similar photosynthetic performance throughout the two experiments, except for electron transport rate measured directly under algal cultivation conditions (ETRsitu). Regarding the values of maximum quantum yield of PSII (Fv/Fm), although the ANOVA analysis showed a significant interaction between time and day (ANOVA: 50% = F(6) = 0.112, p = 0.014; 100% = F(4) = 0.101, p = 0.029, Table S4) such differences were not detected by the Tukey post hoc test (p > 0.05), which indicate that there was absence of photoinhibition even when irradiance was highest (Figures 6A, D). ETRsitu was significantly affected (ANOVA: F(6) = 1400.8, p = 0.008, Table S4) by the interaction of species, hour, and day just in experiment 1 (50% effluent) (Figures 6B, E). Maximum electron transport rate (ETRmax) was influenced by a significant interaction (ANOVA: F(4) = 1733.7, p < 0.001, Table S4) between hour and day only in experiment 2, and no significant difference has been detected in experiment 1 (Figures 6C, F).
Figure 6 Diurnal changes of photosynthetic parameters: maximum quantum yield (Fv/Fm), in situ electron transport rate (ETRsitu), and maximum electron transport rate (ETRmax) of U. pseudorotundata and U. rigida in two conditions: 50% fish effluent (5 days): (A–C); 100% fish effluent (4 days): (D–F). Values are mean ± SD, n = 3.
Spearman’s correlation analysis between the different fluorescence parameters and nitrate biofiltration measurements (NUR and NUE) obtained from U. pseudorotundata and U. rigida are shown in Table 2. The correlations obtained considering the data from experiments 1 and 2 showed that Fv/Fm, as an indicator of photoinhibition, was negatively correlated with ETRsitu and saturation irradiance (Ek), but positively correlated with photosynthetic capacity (αETR.). αETR was positively correlated with NUE, and NPQmax was positively correlated with ETRsitu..
Table 2 Spearman correlation values between nitrate biofiltration parameters and photosynthetic parameters obtained from U. pseudorotundata and U. rigida cultivation under two experimental conditions: (1) 50% fish effluent and (2) 100% fish effluent.
The linear regression model revealed that uptake efficiency (NUE) has a positive relationship with photosynthetic efficiency (αETR) in both Ulva species (Figure 7). In both experiments, the uptake efficiency significantly increased with increasing αETR (R2 = 69.77%, F-statistic = 97.14, p <0.001).
Figure 7 Linear regression model between NO3- uptake efficiency (NUE) and photosynthetic efficiency (αETR) for the species U. pseudorotundata and U. rigida grown in (A) 50% fish farm effluent and (B) 100% fish farm effluent. The solid line represents the fitted values of the overall model.
The growth rates of both species were higher when cultivated in 100% fish farm effluent (ANOVA: F(1) = 3.49, p = 0.015, Figure 8A; Table S5), with U. pseudorotundata showing higher values than U. rigida (Tukey post hoc test, p < 0.001). Productivity data were significantly influenced by effluent concentration (ANOVA: F(1) = 12.04, p = 0.009, Figure 8B and Table S5), and the highest values were also associated with U. pseudorotundata (Tukey post hoc test, p = 0.002). Total nitrogen assimilation per tank was higher in U. rigida than in U. pseudorotundata in the 50% effluent experiment (Tukey post hoc test, p = 0.005), but there was no difference between the two species in the 100% effluent experiment (Tukey post hoc test, p = 0.783, Figure 8C).
Figure 8 (A) Growth rate (% day-1), (B) productivity (g DW m-2 d-1), and (C) nitrogen uptake (g N m−2 day−1) of U. pseudorotundata and U. rigida grown in 50% and 100% fish farm effluents. Values are mean ± SD, n = 3. Different small letters represent differences related to the factor “species”. Different capital letters represent differences caused by the factor “effluent concentration”. Different italic letters represent the differences caused by the interaction between the two factors.
Biomass elemental and biochemical composition of the two Ulva species are presented in Table 3. The contents of carbon, nitrogen and proteins, as well as the C:N ratio, showed significant differences related to the interaction between the factors effluent concentration and time (ANOVA: C% = F(1) = 21.135; N% = F(1) = 1.131; Protein% = F(1) = 33.609; C:N = F(1) = 1.933, p – 0.05, Table S6) and an absence of significant differences between species. Both species showed a significant increase in nitrogen and protein content when cultivated in 100% effluent (Tukey post hoc test, p < 0.001) while a significant reduction was observed in the C:N ratio between the beginning and end of the experiment (Tukey post hoc test, p < 0.001). The hydrogen content were not affected by the effluent concentration (ANOVA, F(1) = 1.641, p = 0.615, Table S6), but differences were observed between the species (ANOVA, F(1) = 47.455, p = 0.004, Table S6), with higher values for U. rigida (Tukey post hoc test, p = 0.006). The chlorophyll-a content was influenced by the interaction of the factors effluent concentration, species and time (ANOVA, F(1) = 0.049, p = 0.018, Table S6) and the highest values were obtained at the end of experiment 1 for both species (Tukey post hoc test, U. pseudorotundata: p < 0.001; U. rigida: p < 0.001). Considering both Ulva species, there was an increment in Chl b when cultivated in 50% effluent (Tukey post hoc test, p < 0.001). For carotenoids, considering both Ulva species, the higher values were obtained when Ulva were cultivated in 50% fish effluent (Tukey post hoc test, p < 0.001).
Table 3 Elemental and biochemical composition of the dry biomass of U. pseudorotundata and U. rigida grown in different concentrations of fish pond effluent (experiment 1: 50% and experiment 2: 100%).
To develop an efficient integrated system of fish and marine algae biomass production, a deep understanding of the physiology of species used in the system is essential. Cultivation of Ulva spp. using fish farming effluents as a culture medium is a promising process, as these algae have a high capacity to remove nutrients. They act as a biofilter and generate biomass with several biomolecules of high economic value such as cell wall polysaccharides (ulvans) and pigments. The improvement of marine algae cultivation systems, as well as their use in bioremediation, implies the selection of native species that are adapted to local conditions, thus avoiding the introduction of exotic species. In the current study, we compared biofiltration capacity, photosynthetic performance, productivity and biomass characteristics of U. pseudorotundata and U. rigida cultivated in effluents of Chelon labrosus ponds. Comparisons were performed at two effluent concentrations under environmental conditions of light and temperature, simulating commercial production conditions. The results indicated that both Ulva species presented similar biofiltration performance to remove dissolved inorganic nutrients (NO3-, NH4+ and PO43-), from fish farm effluents, enabling them as good candidates for use in integrated aquaculture systems.
The investigation of the ability of different seaweed species to biofiltrate nutrients is fundamental for the use of these organisms as nutrient scrubbers of waste waters from mariculture activities. According to Gevaert et al. (2007), Ulva species can differ in their ability to uptake and assimilate nutrients due to their adaptation to stressful conditions, such as fluctuations of inorganic nitrogen concentrations. Based on this, we hypothesized that the species used in the present study could have different behaviors since they were collected from different environments. U. rigida was collected directly from intertidal rock pools and bare rocks, while U. pseudorotundata was obtained from salt marshes where they were floating suspended in the water column. Intertidal algae like U. rigida are exposed to a broad range of conditions such as variation in nutrients, oxygen and inorganic carbon concentrations, apart from changes in temperature, desiccation, and pH fluctuations. On the other hand, species like U. pseudorotundata, deal with constantly high temperatures, extreme salinities, and intense solar radiation. Despite these different stressful conditions, both species exhibited similar biofiltration performance, removing considerable amounts of nitrate (NO3-), ammonium , and phosphate from the experimental tanks. These similar responses suggest high phenotypic plasticity of both species, an important finding for use in bioremediation.
In our experiments both species showed higher uptake efficiency (NUE) for when compared to , quickly reducing concentrations at the beginning of the experiment. Our findings of this faster uptake efficiency is in agreement with previous studies on Ulva spp. (Shpigel et al., 2019; Shahar et al., 2020) and must be related to the mechanisms of uptake and assimilation of different nitrogen forms (Roleda and Hurd, 2019). Since less energy is required for assimilation and metabolization into amino acids, Ulva spp. tend to remove this nutrient more efficiently than . On the other hand metabolism is described as an energy-dependent mechanism that requires active transport to cross cell walls, followed by storage in intracellular pools such as nitrate and/or metabolization into ammonia through two-step sequential enzymes (nitrate and nitrite reductase) (Hurd et al., 2014). As observed by Shpigel et al. (2019), nitrate uptake by U. lactuca initiated only 24 h after total ammonia depletion, which was suggested by the authors as the time required for nitrate reductase synthesis. A study performed with U. lactuca (as U. fasciata) revealed nitrate reductase activity after 28 h of transfering the algae from a medium with total ammonia nitrogen as the only nitrogenous nutrient to enriched seawater (Shahar et al., 2020). Considering the high concentrations of and available in the effluents used, the remaining stock of measured at the end of the second experiment may be related to a saturation of nitrogen required by algae metabolism. Similar results were described by Fan et al. (2014), which observed that increasing levels, over a concentration of 503 µmol L-1, lead to a decay in uptake rate by U. prolifera.
concentrations in the fish farm effluents used in our study were enough to avoid phosphorus limitation for seaweed growth during experimental time. The lower values of removal, when compared to the removal of nitrogenous nutrients, are probably related to the differentiated assimilation rates of these nutrients (Redfield ratio), which is typical of photosynthetic organisms. If, on the one hand, this result suggests that fish farming effluents are not ideally balanced for algal growth, on the other hand, it indicates that this excess of P is not harmful to their growth, since the productivity values were similar in both effluent concentrations.
Unlike cultures developed in the laboratory, where algae are subjected to controlled and regular conditions of light and photoperiod, cultures performed under environmental conditions are subjected to considerable variations in the luminous environment. Under these conditions, photoinhibition and photochemical damage without proper recovery have been known to occur in the middle of the day, i.e. chronic photoinhibition, when increased irradiances reach the cultivation systems and the amount of absorbed light is higher than the photosystem’s capacity. In a practical way, photoinhibition has been detected by the determination of the maximum quantum yield of PSII (Fv/Fm) using PAM fluorometers (Hanelt and Nultsch, 1995; Figueroa et al., 2006; Cruces et al., 2019; Figueroa et al., 2020). In the present study, we did not detect significant differences in the values of Fv/Fm determined at different times of the day for the two algae, suggesting the absence of photoinhibition. These results differ from other studies carried out under similar conditions which observed a decrease in Fv/Fm at noon (Cruces et al., 2019; Figueroa et al., 2020; Masojídek et al., 2021), and can be explained by special adaptations of Ulva spp. to light fluctuations. Some of the adaptive mechanisms described for Ulva spp. are the synthesis of UV-screening and antioxidant compounds (e.g. phenolic compounds), dissipation of energy as heat (xanthophyll cycle), and enzyme-based antioxidant activity (e.g. superoxide dismutase and ascorbate peroxidase) (Bischof et al., 2002; Cruces et al., 2019). The regulation of internal physiological mechanisms of seaweeds to face photoinhibition and photodamage may be related to cultivation conditions, such as the supply of nutrients. Previous studies showed that photoinhibition caused by an increase in PAR was reduced under the increment of nutrient supply (Henley et al., 1991; Figueroa et al., 2006; Cabello-Pasini et al., 2011). Since our culture systems contained sufficient nutrients, we can expect that such mechanisms were active for both species.
Contrasting with results obtained in other studies (eg. Cruces et al., 2019) that showed that ETRmax fluctuations along the day had an inverse relationship with PAR irradiances, our study does not identify a decrease in ETRsitu and ETRmax during irradiance peaks, but an increasing trend confirming the absence of photoinhibition. Similar results were obtained by Figueroa et al. (2020), which described increased ETR values and decreased Fv/Fm at noon. Our findings of a negative correlation between Fv/Fm and ETRsitu obtained from both Ulva species corroborate the aforementioned inverse correlation. These results reflect that while photosynthetic activity (ETR) tends to increase, the maximum quantum yield (Fv/Fm) tends to decrease as solar irradiance rises as a result of the photoprotection mechanisms presented by algae. Also, the negative correlation we detected between Fv/Fm and Ek is consistent with results reported by Figueroa et al. (2020), being related to the diurnal variations in irradiance. Finally, the photochemical efficiency (αETR) extracted from rapid light curves and Fv/Fm, despite being obtained from different methods, showed a positive correlation, reinforcing the reliability of PAM fluorometer data.
Nitrogen metabolism requires energy supplied directly from photosynthetic electron transport since the assimilation of inorganic nitrogen into amino acids demands carbon skeletons (ketoacids), energy in the form of ATP and reductants (Huppe and Turpin, 1994). As described by Huppe and Turpin (1994), inorganic nitrogen metabolism of seaweeds, regardless of whether the original source is ammonium or nitrate, involves the incorporation of nitrogen into amino acids by the assimilation of ammonium through the glutamine synthetase (GS) and glutamine:2-oxoglutarate amino-transferase (GOGAT) pathway. For the maintenance of GOGAT activity, seaweed requires an adequate supply of carbon assimilated from photosynthetic activity. In this context, in the present study we present a mathematical relation between photosynthetic and biofiltration parameters. Spearman’s correlation showed a positive relationship between αETR and uptake efficiency (NUE) for the two algal species. In addition, a linear model was obtained and reveals a positive relationship (r2 = 69.77%) between αETR and NUE, which is largely in line with the evidence that nitrate removal requires a coordination of the metabolic interactions between photosynthesis, respiration, and N assimilation (Turpin et al., 1988). Moreover, assimilation demands larger quantities of carbon from the respiratory process and therefore we assume that the proposed model is valid only for nitrate as a source of nutrients, and does not include . The evidence for the integration of carbon and nitrogen assimilation has also been described in previous investigations with microalgae and seaweeds (Turpin, 1991; Huppe and Turpin, 1994; Figueroa et al., 2009). However, as far as we could verify, a mathematical model which correlated biofiltration and a photosynthetic parameter obtained with rapid measurements of in vivo chlorophyll a fluorescence has not yet been published.
Despite biomass productivity values were relatively low compared to other studies, U. pseudorotundata and U. rigida were able to grow in effluent concentrations tested in this study and, considering that the experiments were performed in a system exposed to natural environmental conditions, it confirms the tolerance of both species to non-controlled changes in temperature and light. The best results were achieved for the U. pseudorotundata cultivation with system productivity values reaching 8.0 g DW m-2 d-1. The feasibility of both Ulva species to keep growth even with temperature and light variations was in accordance with studies carried out with other Ulva species cultivated in mariculture effluent under open scale conditions. The maximum growth values obtained for U. pseudorotundata (4.8% ± 0.7) and U. rigida (2.4% ± 0.3) were lower than those previously obtained for U. lactuca (4-18% d-1, Al-Hafedh et al., 2014; Shpigel et al., 2019) and U. lactuca (as U. fasciata) (7.5-14.3% d-1, Shahar et al., 2020). This may be related to the difference in the system’s culture conditions such as nutrient source (Shahar et al., 2020), biomass stocking densities (Shpigel et al., 2019), and effluent flow rate (Al-Hafedh et al., 2014). Besides culture systems, environmental conditions directly influence biomass production. As verified by Friedlander et al. (1990), in land intensive seaweed cultivation not limited by nutrients, biomass productivity was influenced by changes in light and temperature as a result of season variability. In a study conducted in Portugal, Abreu et al. (2011) described the optimal relative growth rate and productivity for the red seaweed Gracilaria vermiculophylla during spring and summertime, with a decrease in growth rates and nutrient removal during autumn and winter months. In a 6-month study conducted with U. ohnoi, Mata et al. (2016) showed that biomass productivity had a temporal variation due to changes in temperature and light, and they also observed a positive relationship between light and biomass productivity. Those achievements indicate that a better understanding of cultivation conditions is essential to optimize system productivity.
Seaweed biomass is a source of bioactive compounds like pigments (chlorophyll and carotenoids), proteins, phenolic compounds, sulfated polysaccharides, which are known for their beneficial properties for human health (Fleurence et al., 1999; Farvin and Jacobsen, 2013; Schneider et al., 2020; Kalasariya et al., 2021). Our results showed that U. rigida and U. pseudorotundata present similar protein content, ranging from 17.1 to 24.4% DW. These values are similar to those found in studies with U. lactuca (e.g. 10-25%, Shuuluka et al., 2013), U. lacinulata (as U. armoricana) (18%-24%, Fleurence et al., 1999), and U. fenestrata (20.79%, Steinhagen et al., 2022). In general, the protein content of Ulva species could vary between 10 and 47% DW (Dominguez and Loret, 2019). Higher values (up to 40%) were described for U. lactuca (as U. fasciata) (30-38%, Shahar et al., 2020) and U. lactuca (24.9-41.1%, Shpigel et al., 2019) grown in nutrient-enriched seawater. In the present study, after cultivated in pure fish effluent, an increment in protein content of 5.1% was detected in both Ulva species. Since concentrations were higher in pure effluent and complete removal of this nutrient was observed, it is plausible to assume that the higher uptake of N may be associated with a higher assimilation of N for amino acids and proteins. This result is in agreement with those reported by Shahar et al. (2020), which observed an increase in protein content in Ulva that was cultivated using only total ammonia nitrogen (TAN) rather than as a sole nitrogen source.
Chlorophylls and carotenoids are pigments of interest to the cosmetic and pharmaceutical areas mainly due to their antioxidant and anticancer capacity (Boominathan and Mahesh, 2015; Pérez-gálvez et al., 2020; Kalasariya et al., 2021). When cultivated under conditions tested in our experiment, both Ulva species showed similar amounts of chlorophyll a, but not chlorophyll b and carotenoids. Moreover, although a variation in pigment composition was detected over the experimental time, it was less pronounced than that reported by Steinhagen et al. (2022), who observed a sharp decay in chlorophyll a and b and carotenoid content from U. lactuca (as U. fenestrata) biomass grown in a sea based cultivation, under non-controlled conditions. So, the biomass of Ulva species used in this study remained with available pigments to be profitable in the above mentioned application, in a complementary alternative for biomass utilization.
Our study advanced toward biofiltration capacity, photosynthetic performance, productivity and biomass characteristics of U. pseudorotundata and U. rigida cultivated in effluents of Chelon labrosus ponds under natural environmental conditions of light and temperature. In conclusion, our findings show that U. pseudorotundata and U. rigida possess similar capabilities for removing inorganic nutrients from fish effluents. Both seaweed species, naturally found on the Spanish Mediterranean coast, presented equivalent photosynthetic performance and biomass composition. A linear regression model between uptake efficiency and photosynthetic efficiency (αETR) is presented. In addition, we highlighted the application of U. pseudorotundata and U. rigida as robust species for nutrient scrubbing of mariculture effluents, and their potential biomass composition as a sustainable source of raw material for the development of industrial products.
The data presented in the study are deposited in the GenBank - National Center for Biotechnology Information (NCBI) repository. The accession numbers of data are: OP020918.1 and OP020919.1
TM, participated in the experimental design, experimental execution, water chemical analysis, statistical analysis, data interpretation, and original draft. VR-C and JV, participated in the experimental design and execution. BM and PC-V, participated in the experimental execution. LP-S, participated in the execution of statistical analysis and data interpretation. WO, provided the species phylogenetic analysis. AA, dedicated to water chemical analysis. JB-B, participated in the experimental design and data interpretation. LR and FF, reviewed, edited the manuscript text, supervised and provided funding acquisition. All authors contributed to the article and approved the submitted version.
Financial resources and scholarships were provided by: Andalusia Government (Spain) in the frame of the Project FACCO - UMA18-FEDER JA-162, Santa Catarina State Foundation for Research and Innovation Support (FAPESC): Research Grant: n° 12/2020 – 2021TR000671; Scholarship: n° 03/2017, Brazil), and Coordination for the Improvement of Higher Education Personnel (CAPES/PRINT process n°. 88887.470102/2019-00, Brazil). We also thanks the Conselho Nacional de Desenvolvimento Científico e Tecnológico (CNPq) for financial support through research grants (n°305367/2019-6). P.C-V, thanks to Asociación Iberoamericana de Postgrado (AUIP) and Malaga University for PhD Scholarship.
We sincerely thank the Federal University of Santa Catarina (UFSC) and University of Malaga (UMA), especially the faculty members and technical staff of the Laboratory of Phycology (LAFIC - UFSC), Laboratory of Fotobiología y Biotecnología de Organismos acuáticos (FYBOA - UMA), research center Grice Hutchinson (UMA), and Servicios Centrales de Apoyo a la Investigación (SCAI - UMA) for providing space and resources for this work. We also thank Marta G. Sanchez, Cristina V. G. Fernández, Fabian Lopez, David Paniagua, Roberto Abdala, Nathalie K. Peinado, and Ellie Bergstrom for their support and assistance in carrying out experiments, data collection and manuscript revision. This study is part of the Doctoral thesis of the first author to the UFSC Graduate Program in Biotechnology and Bioscience, Santa Catarina, Brazil.
The authors declare that the research was conducted in the absence of any commercial or financial relationships that could be construed as a potential conflict of interest.
All claims expressed in this article are solely those of the authors and do not necessarily represent those of their affiliated organizations, or those of the publisher, the editors and the reviewers. Any product that may be evaluated in this article, or claim that may be made by its manufacturer, is not guaranteed or endorsed by the publisher.
The Supplementary Material for this article can be found online at: https://www.frontiersin.org/articles/10.3389/fmars.2022.981468/full#supplementary-material
Abreu M. H., Pereira R., Yarish C., Buschmann A. H., Sousa-Pinto I. (2011). IMTA with gracilaria vermiculophylla: Productivity and nutrient removal performance of the seaweed in a land-based pilot scale system. Aquaculture 312, 77–87. doi: 10.1016/j.aquaculture.2010.12.036
Al-Hafedh Y. S., Alam A., Buschmann A. H. (2014). Bioremediation potential, growth and biomass yield of the green seaweed, ulva lactuca in an integrated marine aquaculture system at the red Sea coast of Saudi Arabia at different stocking densities and effluent flow rates. Rev. Aquac. 7, 1–11. doi: 10.1111/raq.12060
Andersen R. A. (2005). Algal culturing techniques (1st ed.). Burlington, San Diego, London: Elsevier Academic Press.
Araújo R., Vázquez Calderón F., Sánchez López J., Azevedo I. C., Bruhn A., Fluch S., et al. (2021). Current status of the algae production industry in Europe: An emerging sector of the blue bioeconomy. Front. Mar. Sci. 7. doi: 10.3389/fmars.2020.626389
Ben-Ari T., Neori A., Ben-Ezra D., Shauli L., Odintsov V., Shpigel M. (2014). Management of ulva lactuca as a biofilter of mariculture effluents in IMTA system. Aquaculture 434, 493–498. doi: 10.1016/j.aquaculture.2014.08.034
Bischof K., Kräbs G., Wiencke C., Hanelt D. (2002). Solar ultraviolet radiation affects the activity of ribulose-1,5-bisphosphate carboxylase-oxygenase and the composition of photosynthetic and xanthophyll cycle pigments in the intertidal green alga ulva lactuca l. Planta 215, 502–509. doi: 10.1007/s00425-002-0774-9
Boominathan M., Mahesh A. (2015). ““Seaweed carotenoids for cancer therapeutics,”,” in Handbook of anticancer drugs from marine origin, ed. Kim S.-K. (Springer International Publishing Switzerland), 185–203. doi: 10.1007/978-3-319-07145-9
Buschmann A. H., Camus C., Infante J., Neori A., Israel Á., Hernández-González M. C., et al. (2017). Seaweed production: Overview of the global state of exploitation, farming and emerging research activity. Eur. J. Phycol. 52, 391–406. doi: 10.1080/09670262.2017.1365175
Cabello-Pasini A., Figueroa F. L. (2005). Effect of nitrate concentration on the relationship between photosynthetic oxygen evolution and electron transport rate in ulva rigida (Chlorophyta). J. Phycol. 41, 1169–1177. doi: 10.1111/j.1529-8817.2005.00144.x
Cabello-Pasini A., Macías-Carranza V., Abdala R., Korbee N., Figueroa F. L. (2011). Effect of nitrate concentration and UVR on photosynthesis, respiration, nitrate reductase activity, and phenolic compounds in ulva rigida (Chlorophyta). J. Appl. Phycol. 23, 363–369. doi: 10.1007/s10811-010-9548-0
Chopin T., Buschmann A. H., Halling C., Troell M., Kautsky N., Neori A., et al. (2001). Integrating seaweeds into marine aquaculture systems: a key toward sustainability. J. Phycol. 37, 975–986. doi: 10.1046/j.1529-8817.2001.01137.x
Cohen I., Neori A. (1991). Ulva lactuca biofilters for marine fishpond effluents. i. ammonia uptake kinetics and nitrogen content. Bot. Mar. 34:475–482. doi: 10.1515/botm.1991.34.6.475
Copertino M. D. S., Tormena T., Seeliger U. (2009). Biofiltering efficiency, uptake and assimilation rates of ulva clathrata (Roth) j. agardh (Clorophyceae) cultivated in shrimp aquaculture waste water. J. Appl. Phycol. 21, 31–45. doi: 10.1007/s10811-008-9357-x
Cruces E., Rautenberger R., Cubillos V. M., Ramírez-Kushel E., Rojas-Lillo Y., Lara C., et al. (2019). Interaction of photoprotective and acclimation mechanisms in ulva rigida (Chlorophyta) in response to diurnal changes in solar radiation in southern Chile. J. Phycol. 55, 1011–1027. doi: 10.1111/jpy.12894
del Río M., Ramazanov Z., García-Reina G. (1996). Ulva rigida (Ulvales, chlorophyta) tank culture as biofilters for dissolved inorganic nitrogen from fishpond effluents. Hydrobiologia. 66, 61–66. doi: 10.1007/978-94-009-1659-3_7
Dominguez H., Loret E. P. (2019). Ulva lactuca, a source of troubles and potential riches. Mar. Drugs 17(6):357. doi: 10.3390/md17060357
Eilers P. H. C., Peeters J. C. H. (1988). A model for the relationship between light intensity and the rate of photosynthesis in phytoplankton. Ecol. Modell. 42, 199–215. doi: 10.1016/0304-3800(88)90057-9
Fan X., Xu D., Wang Y., Zhang X., Cao S., Mou S., et al. (2014). The effect of nutrient concentrations, nutrient ratios and temperature on photosynthesis and nutrient uptake by ulva prolifera: Implications for the explosion in green tides Journal of Applied Phycology 26, 537–544. doi: 10.1007/s10811-013-0054-z
FAO (2020). The state of world fisheries and aquaculture 2020. sustainability in action. doi: 10.4060/ca9229en
Farvin K. H. S., Jacobsen C. (2013). Phenolic compounds and antioxidant activities of selected species of seaweeds from Danish coast. Food Chem. 138, 1670–1681. doi: 10.1016/j.foodchem.2012.10.078
Felsenstein J. (1985). Confidence limits on phylogenies: An approach using the bootstrap. Evol. (N. Y). 39, 783. doi: 10.2307/2408678
Figueroa F. L., Bonomi-Barufi J., Celis-Plá P. S. M., Nitschke U., Arenas F., Connan S., et al. (2020). Short-term effects of increased CO2, nitrate and temperature on photosynthetic activity in ulva rigida (Chlorophyta) estimated by different pulse amplitude modulated fluorometers and oxygen evolution. J. Exp. Bot. 72, 491–509. doi: 10.1093/jxb/eraa473
Figueroa F., Conde-Alvarez R., Gomez I. (2003). Relations between electrion transport rates determined by pulse amplitude modulated chlorophyll fluorescence and oxygen evolution in macroalgae under different light conditions. Photosynth. Res. 75, 259–275. doi: 10.1023/A:1023936313544
Figueroa F. L., Israel A., Neori A., Martínez B., Ang P. O., Inken S., et al. (2009). Effects of nutrient supply on photosynthesis and pigmentation in ulva lactuca ( chlorophyta ): responses to short-term stress Aquatic Biology 7, 173–183. doi: 10.3354/ab00187
Figueroa F. L., Santos R., Conde-Álvarez R., Mata L., Gómez Pinchetti J. L., Matos J., et al. (2006). The use of chlorophyll fluorescence for monitoring photosynthetic condition of two tank-cultivated red macroalgae using fishpond effluents. Bot. Mar. 49, 275–282. doi: 10.1515/BOT.2006.035
Fleurence J., Chenard E., Luçon M. (1999). Determination of the nutritional value of proteins obtained from ulva armoricana. J. Appl. Phycol. 11, 231–239. doi: 10.1023/A:1008067308100
Friedlander M., Galai N., Farbstein H. (1990). A model of seaweed growth in an outdoor culture in Israel. Hydrobiologia, 204(1):367–373. doi: 10.1007/BF00040258
Froehlich H. E., Afflerbach J. C., Frazier M., Halpern B. S. (2019). Blue growth potential to mitigate climate change through seaweed offsetting. Curr. Biol. 29, 3087–3093.e3. doi: 10.1016/j.cub.2019.07.041
Genty B., Briantais J.-M., Baker N. R. (1989). Electron transport and quenching of chlorophyll fluorescence. BBA - Gen. Subj. 990, 87–92. doi: 10.1016/S0304-4165(89)80016-9
Gevaert F., Barr N. G., Rees T. A. V. (2007). Diurnal cycle and kinetics of ammonium assimilation in the green alga ulva pertusa. Mar. Biol. 151, 1517–1524. doi: 10.1007/s00227-006-0588-6
Grasshoff K., Kremling K., Ehrhardt M. (1999). Methods of seawater analysis. 3nd ed (Weinheim: WILEY-VCH).
Guiry M. D., Guiry G. M., et al. (2011). AlgaeBase. World-wide electronic publication. National University of Ireland, Galway. Available online at: https://www.algaebase.org/search/genus/detail/?genus_id=33; [Accessed August 18, 2022]
Guttman L., Boxman S. E., Barkan R., Neori A., Shpigel M. (2018). Combinations of ulva and periphyton as bio fi lters for both ammonia and nitrate in mariculture fi shpond e ffl uents. Algal research 34, 235–243. doi: 10.1016/j.algal.2018.08.002
Hanelt D., Nultsch W. (1995). Field studies of photoinhibition show non-correlationsbetween oxygen and fluorescence measurements in the Arctic red alga palmaria palmata. J. Plant Physiol. 145, 31–38. doi: 10.1016/S0176-1617(11)81842-0
Heesch S., Broom J. E. S., Neill K. F., Farr T. J., Dalen J. L., Nelson W. A. (2009). Ulva, umbraulva and gemina: Genetic survey of new Zealand taxa reveals diversity and introduced species. Eur. J. Phycol. 44, 143–154. doi: 10.1080/09670260802422477
Henley W. J., Levavasseur G., Franklin L. A., Osmond C. B., Ramus J. (1991). Photoacclimation and photoinhibition in ulva rotundata as influenced by nitrogen availability. Planta 184, 235–243. doi: 10.1007/BF01102423
Huppe H. C., Turpin D. H. (1994). Integration of carbon and nitrogen metabolism in plant and algal cells. Annu. Rev. Plant Biol. 45, 577–607. doi: 10.1146/annurev.pp.45.060194.003045
Hurd C. L., Harrison P. J., Bischof K., Lobban C. S. (2014). Seaweed ecology and physiology. (2nd ed.). Cambridge: Cambridge University Press. doi: 10.1017/CBO9781139192637
IOC-UNESCO (2020). “United Nations Decade of Ocean Science for Sustainable Development 2021-2030 Implementation Plan version 2.0.Available online at: https://www.oceandecade.org/resource/108/Version-20-of-the-Ocean-Decade-Implementation-Plan [accessed August 18, 2022].
Jusadi D., Ekasari J., Suprayudi M. A., Setiawati M., Fauzi I. A. (2021). Potential of underutilized marine organisms for aquaculture feeds. Front. Mar. Sci. 7. doi: 10.3389/fmars.2020.609471
Kalasariya H. S., Yadav V. K., Yadav K. K., Tirth V., Algahtani A., Islam S., et al. (2021). Seaweed-based molecules and their potential biological activities: An eco-sustainable cosmetics. Molecules 26, 1–22. doi: 10.3390/molecules26175313
Kang Y. H., Shin J. A., Kim M. S., Chung I. K. (2008). A preliminary study of the bioremediation potential of codium fragile applied to seaweed integrated multi-trophic aquaculture (IMTA) during the summer. J. Appl. Phycol. 20, 183–190. doi: 10.1007/s10811-007-9204-5
Kidgell J. T., Magnusson M., de Nys R., Glasson C. R. K. (2019). Ulvan: A systematic review of extraction, composition and function. Algal. Res. 39, 101422. doi: 10.1016/j.algal.2019.101422
Kimura M. (1980). A simple method for estimating evolutionary rates of base substitutions through comparative studies of nucleotide sequences. J. Mol. Evol. 16, 111–120. doi: 10.1007/BF01731581
Lignell A., Pedersén M. (1989). Agar composition as a function of morphology and growth rate. studies on some morphological strains of graciliaria secundata and graciliaria verrucosa (Rhodophyta). Bot. Mar. 32, 219–227. doi: 10.1515/botm.1989.32.3.219
Liu D., Keesing J. K., He P., Wang Z., Shi Y., Wang Y. (2013). The world’s largest macroalgal bloom in the yellow Sea, China: Formation and implications. Estuar. Coast. Shelf. Sci. 129, 2–10. doi: 10.1016/j.ecss.2013.05.021
Lotze H. K., Lenihan H. S., Bourque B. J., Bradbury R. H., Cooke R. G., Kay M. C., et al. (2006). Depletion, degradation, and recovery potential of estuaries and coastal seas. Science 312, 1806–1809. doi: 10.1126/science.1128035
Loughnane C. J., McIvor L. M., Rindi F., Stengel D. B., Guiry M. D. (2008). Morphology, rbcL phylogeny and distribution of distromatic ulva (Ulvophyceae, chlorophyta) in Ireland and southern Britain. Phycologia 47, 416–429. doi: 10.2216/PH07-61.1
Macchiavello J., Bulboa C. (2014). Nutrient uptake efficiency of gracilaria chilensis and ulva lactuca in an IMTA system with the red abalone haliotis rufescens. Lat. Am. J. Aquat. Res. 42, 523–533. doi: 10.3856/vol42-issue3-fulltext-12
Masojídek J., Ranglová K., Rearte T. A., Celis Plá P. S. M., Torzillo G., Benavides A. M. S., et al. (2021). Changes in photosynthesis, growth and biomass composition in outdoor chlorella g120 culture during the metabolic shift from heterotrophic to phototrophic cultivation regime. Algal. Res. 56:102303. doi: 10.1016/j.algal.2021.102303
Mata L., Magnusson M., Paul N. A., De Nys R. (2016). The intensive land-based production of the green seaweeds derbesia tenuissima and ulva ohnoi : biomass and bioproducts. Journal of Applied Phycology 1, 365–375. doi: 10.1007/s10811-015-0561-1
Mata L., Santos R., Chapman A. R. O., Anderson R. J., Vreeland V. J., Davison I. R. (2003). “Cultivation of ulva rotundata (Ulvales, chlorophyta) in raceways using semi-intensive fishpond effluents: Yield and biofiltration,” in Proc. 17th int. seaweed symp. 2001 (Cape Town, South Africa: Oxford University Press), 237–242. Available at: http://www.cabdirect.org/abstracts/20033183342.html;jsessionid=913580A26234A2B1E97F538D9D5BD6AE.
Mata L., Schuenhoff A., Santos R. (2010). A direct comparison of the performance of the seaweed biofilters, asparagopsis armata and ulva rigida. J. Appl. Phycol. 22, 639–644. doi: 10.1007/s10811-010-9504-z
Mata L., Silva J., Schuenhoff A., Santos R. (2006). The effects of light and temperature on the photosynthesis of the asparagopsis armata tetrasporophyte (Falkenbergia rufolanosa), cultivated in tanks. Aquaculture 252, 12–19. doi: 10.1016/j.aquaculture.2005.11.045
Nardelli A. E., Chiozzini V. G., Braga E. S., Chow F. (2019). Integrated multi-trophic farming system between the green seaweed ulva lactuca, mussel, and fish: a production and bioremediation solution. J. Appl. Phycol. 31, 847–856. doi: 10.1007/s10811-018-1581-4
Neori A., Chopin T., Troell M., Buschmann A. H., Kraemer G. P., Halling C., et al. (2004). Integrated aquaculture : Rationale, evolution and state of the art emphasizing seaweed biofiltration in modern mariculture. Aquaculture 231, 361–391. doi: 10.1016/j.aquaculture.2003.11.015
Neori A., Msuya F. E., Shauli L., Schuenhoff A., Kopel F. (2003). A novel three-stage seaweed (ulva lactuca) biofilter design for integrated mariculture a novel three-stage seaweed (ulva lactuca) biofilter design for integrated mariculture. J. Appl. Phycol. 15, 543–553. doi: 10.1023/B
Osmond C. B., Ramus J., Levavasseur G., Franklin L. A., Henley W. J. (1993). Fluorescence quenching during photosynthesis and photoinhibition of ulva rotundata blid. Planta 190, 97–106. doi: 10.1007/BF00195680
Parsons T. R., Strickland J. D. H. (1963). Marine-plant pigments, with revised equations far ascertaining chlorophylls and carotenoids. J. Mar. Res. 21, 155–163.
Pérez-gálvez A., Viera I., Roca M. (2020). Carotenoids and chlorophylls as antioxidants. Antioxidants 9, 1–39. doi: 10.3390/antiox9060505
Ritchie R. J. (2006). Consistent sets of spectrophotometric chlorophyll equations for acetone, methanol and ethanol solvents. Photosynth. Res. 89, 27–41. doi: 10.1007/s11120-006-9065-9
Roleda M. Y., Hurd C. L. (2019). Seaweed nutrient physiology: application of concepts to aquaculture and bioremediation. Phycologia 58, 552–562. doi: 10.1080/00318884.2019.1622920
Schneider G., Figueroa F. L., Vega J., Chaves P., Álvarez-Gómez F., Korbee N., et al. (2020). Photoprotection properties of marine photosynthetic organisms grown in high ultraviolet exposure areas: Cosmeceutical applications. Algal. Res. 49, 101956. doi: 10.1016/j.algal.2020.101956
Schloerke B., Cook D, Larmarange J, Briatte F, Marbach M, Thoen E, Elberg A, Crowley J, et al. (2021). GGally: Extension to ‘ggplot2’. R package version 2.1.2. https://CRAN.R-project.org/package=GGally
Shahar B., Shpigel M., Barkan R., Masasa M., Neori A., Chernov H., et al. (2020). Changes in metabolism, growth and nutrient uptake of ulva fasciata (Chlorophyta) in response to nitrogen source. Algal. Res. 46, 101781. doi: 10.1016/j.algal.2019.101781
Shpigel M., Guttman L., Ben-Ezra D., Yu J., Chen S. (2019). Is ulva sp. able to be an efficient biofilter for mariculture effluents? J. Appl. Phycol. 31, 2449–2459. doi: 10.1007/s10811-019-1748-7
Shpigel M., Neori A., Popper D. M., Gordin H. (1993). A proposed model for “environmentally clean” land-based culture of fish, bivalves and seaweeds. Aquaculture 117, 115–128. doi: 10.1016/0044-8486(93)90128-L
Shuuluka D., Bolton J. J., Anderson R. J. (2013). Protein content , amino acid composition and nitrogen-to-protein conversion factors of ulva rigida and ulva capensis from natural populations and ulva lactuca from an aquaculture system , in south Africa. Journal of Applied Phycology 25, 677–685. doi: 10.1007/s10811-012-9902-5
Smetacek V., Zingone A. (2013). Green and golden seaweed tides on the rise. Nature 504, 84–88. doi: 10.1038/nature12860
Smith V. H., Tilman G. D., Nekola J. C. (1999). Eutrophication : Impacts of excess nutrient inputs on freshwater , marine , and terrestrial ecosystems. Environ. pollut. 100, 179–196. doi: 10.1016/S0269-7491(99)00091-3
Steinhagen S., Enge S., Cervin G., Larsson K., Edlund U., Schmidt A. E. M., et al. (2022). Harvest time can affect the optimal yield and quality of Sea lettuce (Ulva fenestrata) in a sustainable Sea-based cultivation. Front. Mar. Sci. 9. doi: 10.3389/fmars.2022.816890
Tamura K., Stecher G., Kumar S. (2021). MEGA11: Molecular evolutionary genetics analysis version 11. Mol. Biol. Evol. 38, 3022–3027. doi: 10.1093/molbev/msab120
Troell M., Halling C., Neori A., Chopin T., Buschmann A. H., Kautsky N., et al. (2003). Integrated mariculture: Asking the right questions. Aquaculture, 226(1):69–90. doi: 10.1016/S0044-8486(03)00469-1
Turpin D. H. (1991). Effects of inorganic n availability on algal photosynthesis and carbon metabolism. J. Phycol. 27, 14–20. doi: 10.1111/j.0022-3646.1991.00014.x
Turpin D. H., Elrifi I. R., Birch D. G., Weger H. G., Holmes J. J. (1988). Interactions between photosynthesis, respiration, and nitrogen assimilation in microalgae. Can. J. Bot. 66, 2083–2097. doi: 10.1139/b88-286
Vega J., Schneider G., Moreira B. R., Herrera C., Bonomi-Barufi J., Figueroa F. L. (2021). Mycosporine-like amino acids from red macroalgae: Uv-photoprotectors with potential cosmeceutical applications. Appl. Sci. 11(11):5112. doi: 10.3390/app11115112
Wan A. H. L., Wilkes R. J., Heesch S., Bermejo R., Johnson M. P., Morrison L. (2017). Assessment and characterisation of ireland’s green tides (Ulva species). PloS One 12(1):e0169049. doi: 10.1371/journal.pone.0169049
Keywords: Aquaculture, biofiltration, in vivo chlorophyll-a fluorescence, nitrogen, photosynthesis, Ulva pseudorotundata, Ulva rigida
Citation: Massocato TF, Robles-Carnero V, Moreira BR, Castro-Varela P, Pinheiro-Silva L, Oliveira WdS, Vega J, Avilés A, Bonomi-Barufi J, Rörig LR and Figueroa FL (2022) Growth, biofiltration and photosynthetic performance of Ulva spp. cultivated in fishpond effluents: An outdoor study. Front. Mar. Sci. 9:981468. doi: 10.3389/fmars.2022.981468
Received: 29 June 2022; Accepted: 27 July 2022;
Published: 02 September 2022.
Edited by:
Elena Ficara, Politecnico di Milano, ItalyReviewed by:
Leonel Pereira, University of Coimbra, PortugalCopyright © 2022 Massocato, Robles-Carnero, Moreira, Castro-Varela, Pinheiro-Silva, Oliveira, Vega, Avilés, Bonomi-Barufi, Rörig and Figueroa. This is an open-access article distributed under the terms of the Creative Commons Attribution License (CC BY). The use, distribution or reproduction in other forums is permitted, provided the original author(s) and the copyright owner(s) are credited and that the original publication in this journal is cited, in accordance with accepted academic practice. No use, distribution or reproduction is permitted which does not comply with these terms.
*Correspondence: Thaís Fávero Massocato, dGhhaXNtYXNzb2NhdG9AZ21haWwuY29t
†These authors have contributed equally to this work and share last authorship
Disclaimer: All claims expressed in this article are solely those of the authors and do not necessarily represent those of their affiliated organizations, or those of the publisher, the editors and the reviewers. Any product that may be evaluated in this article or claim that may be made by its manufacturer is not guaranteed or endorsed by the publisher.
Research integrity at Frontiers
Learn more about the work of our research integrity team to safeguard the quality of each article we publish.