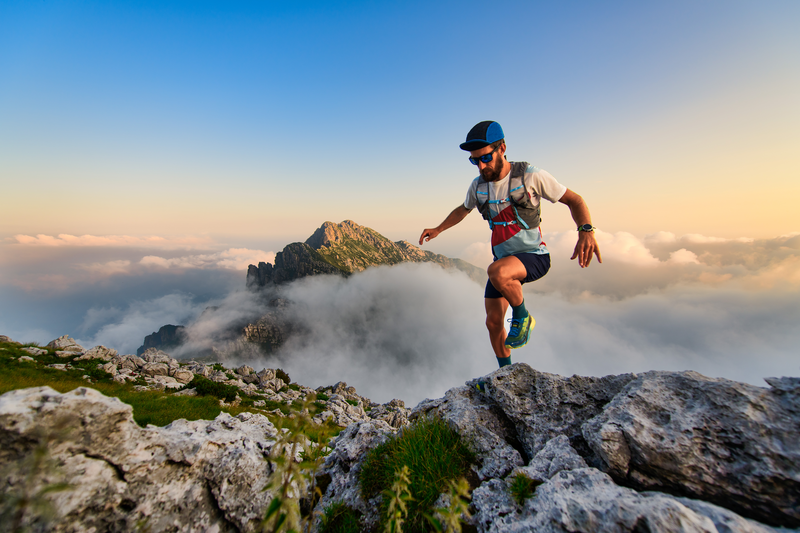
94% of researchers rate our articles as excellent or good
Learn more about the work of our research integrity team to safeguard the quality of each article we publish.
Find out more
ORIGINAL RESEARCH article
Front. Mar. Sci. , 26 July 2022
Sec. Marine Biotechnology and Bioproducts
Volume 9 - 2022 | https://doi.org/10.3389/fmars.2022.977234
This article is part of the Research Topic Comprehensive Utilization and Evaluation of Low-Value Marine Organisms and Seafood Processing Waste/By-products View all 5 articles
For efficiently utilizing the processing byproducts of miiuy croaker to prepare novel angiotensin I-converting enzyme (ACE) inhibitory (ACEi) peptides, in vitro gastrointestinal (GI) digestion method was screened and employed to prepare swim bladder hydrolysate with the highest ACEi activity. Subsequently, seven novel ACEi peptides were isolated from the hydrolysate and identified as DEGPE, EVGIQ, SHGEY, GPWGPA, GPFGTD, SPYGF, and VIGPF with molecular weights of 545.49, 544.58, 591.55, 583.63, 592.59, 569.60, and 531.63 Da, respectively. SHGEY and SPYGF exhibited remarkable ACEi activity with IC50 values of 0.86 ± 0.12 and 0.37 ± 0.06 mg/mL. Molecular docking experiment illustrated that the significant ACEi activity of SHGEY and SPYGF with the affinity of -8.7 and -9.7 kcal/mol mainly attributed to effectively combining with the ACEi active sites by hydrophobic interaction, electrostatic force and hydrogen bonding. Moreover, SHGEY and SPYGF could significantly up-regulate the nitric oxide (NO) production and decrease the endothelin-1 (ET-1) secretion in human umbilical vein endothelial cells (HUVECs), but also abolished the negative impacting of norepinephrine to the levels of NO and ET-1. Furthermore, SHGEY and SPYGF showed significant protection to HUVECs against H2O2 damage by increasing superoxide dismutase (SOD), glutathione peroxidase (GSH-Px) activity to lower the contents of reactive oxide species and malondialdehyde. Consequently, ACEi peptides derived from miiuy croaker swim bladders, especially SHGEY and SPYGF, are health-promoting ingredients for functional products as a supplementary treatment to hypertension and cardiovascular diseases.
Hypertension seriously affects the morbidity and mortality of cardiovascular and renal diseases (Kharazmi-Khorassani et al., 2019; Abedin et al., 2022). Because of the different lifestyles and genetic factors, the number of people with high blood pressure is now about 1.3×106, but this number is expected to rise to 1.56 ×106 by 2030 (Mabhida et al., 2021). The current and future situation will have a serious implication on human health and place a great strain on the finances of countries around the world (Abdelhedi and Nasri, 2019; Mabhida et al., 2021). The renin-angiotensin system bears crucial roles in maintaining blood pressure homeostasis and keeping the balance between fluid and salt (Zheng et al., 2022). Angiotensin (Ang) I-converting enzyme (ACE) is the key protease in participating the regulating of blood pressure through the renin-angiotensin system (Xu et al., 2021; Qiao et al., 2022), and its function is converting Ang I to Ang II for the inactivation of vasodilator bradykinin, which further induce the increase of blood pressure (BP). Then, inhibiting ACE activity is an ideal method in hypertension treatment (Fan et al., 2019; Chakraborty and Roy, 2022). Therefore, the chemosynthetic ACE inhibitors (captopril (Cap), lisinopril, enalapril, etc.) have been widely used in treating heart failure and hypertension in the clinical (Fan et al., 2019; Chakraborty and Roy, 2022), but the disturbing thing is those synthetic ACE inhibitors display some serious adverse symptoms, such as headache, impaired taste perception, dizziness, chronic dry cough, nausea, and hyperkalemia (Lee et al., 2010; Lee and Hur, 2017; Abedin et al., 2022). Therefore, researchers are focusing on searching safer ACE inhibitors from natural resources as the alternatives of synthetic ACE inhibitors to control hypertension (Abedin et al., 2022).
Presently, some natural ACE inhibitors have been isolated from diversified biological resources, and ACE inhibitory (ACEi) peptides drew great public attention due to their safe and effective therapeutic properties on hypertension (Pujiastuti et al., 2019; Abedin et al., 2022; Chakraborty and Roy, 2022). In the experiments on spontaneously hypertensive rats (SHRs), corn germ ACEi peptide with IC50 of 0.83 mg/mL could markedly lower the systolic BP, adjust the level of relaxing and contracting factors derived by endothelium in serum, and suppress the ACE activities in different organs (Guo et al., 2020). PPLLFAAL with IC50 of 28 μmmol/L could remarkably reduce the systolic BP and diastolic BP of SHRs after intravenous administration (Su et al., 2021). Similarly, LGF and GLFF from leaf hydrolysate of Moringa oleifera exhibited double inhibitory activity of ACE and renin and significantly reduced the systolic and diastolic BP of SHRs (Ma et al., 2021). VIPVPFF from yeast hydrolysate with IC50 of 10.27 μM could increase the nitric oxide (NO) levels, upregulate approximately 15-fold expression of GUCY1A1 gene, and activate several hypertension-related pathways in human umbilical vein endothelial cells (HUVECs) (Huang et al., 2021). Moreover, many ACEi peptides, such as LY, RALP, GHS (He et al., 2019a), EMFGTSSET (Pei et al., 2022), IWHHT (Gu et al., 2019), and VPP (Chakrabarti et al., 2017), show multi-activities, especially antioxidant and antiphlogistic activities. The multiple activities of those ACEi peptides should play synergistic roles with their ACEi activity in the control of hypertension (Majumder and Wu, 2015; Fan et al., 2019).
Globle fish production reached around 179 million tons and approximately 50% of these catches become byproducts during factory processing (Manikkam et al., 2016, Sila and Bougatef, 2016; Sheng et al., 2022). Those fish byproducts will lead to serious environmental problems if they can’t be handled properly (Zhang et al., 2019; Yang et al., 2019a). To make full use of these fish byproducts, many ACEi peptides were prepared from different processing byproducts, such as tuna black muscle and bone (Lee et al., 2010; Qiao et al., 2022), smooth-hound viscera (Abdelhedi et al., 2017; Abdelhedi et al., 2018), cuttlefish muscle (Balti et al., 2015), Nile tilapia skeleton (Borges-Contreras et al., 2019), rainbow trout viscera (Vásquez et al., 2022) [34], and Alaska pollack skins (Yang et al., 2021). Those sea-food derived ACEi peptides exhibit high potential application value in diet or clinical treatment on anti-hypertension (Abdelhedi and Nasri, 2019; Fan et al., 2019).
Miiuy croaker (Miichthys miiuy) is an important species of the Sciaenidae family, which is a popular marine economic fish (He et al., 2019b; Geng et al., 2021). In China, miiuy croaker is the most common materials used to produce dried croaker, but fish scales and viscus are discarded as byproducts in the process. Therefore, collagen and antioxidant peptides were prepared from those byproducts (Li et al., 2018; Zhao et al., 2018a; Zhao et al., 2018b). Moreover, FPYLRH from miiuy croaker showed notably cytoprotective effect against H2O2-injuried HUVECs by activating the intracellular antioxidant system to remove excess reactive oxygen species (ROS) (Cai et al., 2019). However, there was no literature on ACEi peptides produced using miiuy croaker swim bladders. Therefore, to make more efficient use of this resource, the objectives of this study were to (i) isolate ACEi peptides from swim bladder hydrolysate of miiuy croaker, (ii) identify the sequences and characterize the properties of isolated ACEi peptides, and (iii) evaluate the cytoprotective activity of the isolated ACEi peptides.
Miiuy croaker (M. miiuy) was provided by Zhejiang Hailisheng Group Co. Ltd. (China). HUVECs were purchased from Shanghai Cell Bank, Chinese Academy of Sciences (Shanghai, China). The assay kits of NO (Product no. A013-2-1), endothelin-1 (ET-1) (Product no. H093), superoxide dismutase (SOD) (Product no. A001-3-2), glutathione peroxidase (GSH-Px) (Product no. A005-1-2), and malondialdehyde (MDA) (Product no. A003-4-1) were purchased from Nanjing Jiancheng Bioengineering Institute Co., Ltd. (China). 2,4,6-Trinitrobenzene sulfonic acid (TNBS), Sephadex G-25 resin, Alcalase, 3-(4,5-dimethylthiazol-2yl)-2,5-diphenyltetrazolium bromide (MTT) (CAS no. 298-93-1), Neutrase, Cap, DMSO, acetonitrile (ACN), norepinephrine (NE), Glutathione (GSH), trypsin (enzyme activity ≥200000 U/g, CAS no. 9002-07-7), pepsin (enzyme activity ≥200000 U/g, CAS no. 9001-75-6), papain, N-[3-(2-Furyl)acryloyl]-Phe-Gly-Gly (FAPGG) (CAS no. 64967-39-1), Dulbecco’s Modified Eagle Medium (DMEM) (Product no. 11995), and ACE were purchased from Sigma-Aldrich (Shanghai) Trading Co., Ltd. (China). ACEi peptides of MMP3-MMP7 (purity > 95%) were synthesized in Shanghai Apeptide Co. Ltd. (China).
The degreasing process of miiuy croaker swim bladder was performed according to the described method (Chi et al., 2014; Zhao et al., 2018b). The swim bladders were homogenized and mixed with isopropanol at a material/ratio of 1:4 (w/v) for 6 h, and the solution was replaced each 2.0 h. Lastly, the solution was centrifuged at 6 000 g for 20 min and the resulted solid was air-dried at 35°C.
The hydrolytic process was performed on the previous methods (Chi et al., 2014). The dispersions of degreased swim bladders (1%, w/v) was treated by supersonic processing for 30 min and hydrolyzed respectively using four proteases under optimal conditions (Table 1).
The in vitro GI digestion method (pepsin-trypsin system) was carried out on the described method (Zhao et al., 2019a). The dispersions of degreased swim bladders (pH 1.5, 1%) were treated by supersonic processing for 30 min and firstly degraded by pepsin for 2 h, and the pH was adjusted to 7.0 and further degraded by trypsin for 2 h.
After digestion, the solutions were placed in 95°C for 15 min and centrifuged at 8 000 g for 20 min. The supernatant was lyophilized and stored in -20°C freezer. The protein hydrolysate prepared using in vitro GI digestion processing was referred to as MSCH.
MSCH (100.0 mg/mL) was fractionated by 1, 5 and 10 kDa ultrafiltration membrane and four fractions including MSCH-1 (< 1 kDa), MSCH-2 (1-5 kDa), MSCH-3 (5-10 kDa), and MSCH-4 (> 10 kDa) were prepared.
MSCH-1 solution (5 mL, 50.0 mg/mL) was fractionated with Sephadex G-25 column (3.6 × 150 cm) eluted with ultrapure water under the flow rate of 0.8 mL/min. Each eluate (2.4 mL) was collected by monitoring absorbance at 220 nm. Three subfractions (MSCH-1a, MSCH-1b, and MSCH-1c) were isolated from MSCH-I.
MSCH-1b solution (20 μL, 100.0 μg/mL) was finally separated by RP-HPLC on a Zorbax 300SB-C18 column (4.6 × 250 mm, 5 μm) with a linear gradient of ACN (5% ACN in 5 min; 5-25% ACN in 10 min; 25-50% ACN in 10 min; 50-100% ACN in 10 min; 100% ACN in 5 min) containing 0.06% trifluoroacetic acid at 1.5 mL/min flow rate. The eluate absorbance was monitored at 220 and 280 nm. Seven ACEi peptides (MMP1 to MMP7) were collected and lyophilized.
The sequences of MMP1 to MMP7 were analyzed using an Applied Biosystems 494 protein sequencer (Perkin Elmer, USA) (Zhao et al., 2018b). A quadrupole time-of-flight (Q-TOF) mass spectrometric device (Micromass, Waters, USA) in the combination of an ESI source were employed to determine the MWs of MMP1 to MMP7 (Chi et al., 2014).
The ACEi activity was determined according to the previous protocol by Zhao et al. (Zhao et al., 2019b). In brief, 50 μL FAPGG solution as substrate (1 mM) in HEPES-HCl buffer (0.5 mM, pH 8.3, containing 300 mM salt) were mixed with 40 μL sample (5, 10, 20, 40mg/mL) and 10 μL of ACE solution. The mixture was pre-incubated at 37°C for 5 min. Then, 50 μL of 1.0 mol/L FPAGG solution were added into the mixture to initiate the reaction and incubated at 37°C for 30 min. The control was prepared using 80 mM HEPES-HCl buffer containing 300 mM NaCl (pH 8.3) instead of the sample. The sample group and control group were run in the same manner. After that, measure the absorbance of the sample solution at 340 nm. All samples were measured as described above, respectively. The IC50 value was defined as the concentration of inhibitor required to inhibit 50% of the ACE activity. The ACEi activity was calculated by the following equation:
A0 and B0 represent the initial absorbance of the control group and the sample group; A30 and B30 represent the absorbance after 30 minutes for the control group and the sample group.
DH (%) was measure according to the previous method (Wang et al., 2013a). The hydrolysate (50 μL) was mixed with 0.5 mL of 0.2 M phosphate buffer, pH 8.2 and 0.5 mL of 0.05% TNBS reagent. TNBS was freshly prepared before use by diluting with deionized water. The mixture was incubated at 50°C for 1 h in a water bath. The reaction was stopped by adding 1 mL of 0.1 M HCl and incubating at room temperature for 30 min. The absorbance was monitored at 420 nm. L-Leucine was used as a standard. To determine the total amino acid content, hydrolysate was completely hydrolyzed with 6 M HCl with a sample to acid ratio of 1:100 at 120°C for 24 h. DH (%) was calculated using the following equation:
where At was the amount of a-amino acids released at time t, A0 was the amount of a-amino acids in the supernatant at 0 h, and Amax was the total amount of a-amino acids obtained after acid hydrolysis at 120°C for 24 h.
This assay of MMP3 and MMP6 was performed on the previous method (Zheng et al., 2022; Suo et al., 2022a) and commissioned to Shanghai NovoPro Biotechnology Co., Ltd (China). The crystal structures of human ACE-lisinopril complex (1O8A.pdb) and captopril were acquired from the RCSB PDB Protein Data Bank (PDB code: 1UZF). The interaction between ACE and MCO was analyzed to determine the position and size of the binding pocket using Chimera software. All non-standard residues in the 1UZF model were deleted, and AutodockTools were used to convert PDB files into PDBQT files (adding Gasteiger charge and setting key distortion). Peptide molecules were converted into SMILES format by PepSMI tool, and 3D models were drawn by Discovery Studio program and energy minimization was done using steepest descent and conjugate gradient techniques. Molecular docking and free energy calculation were carried out using flexible docking tool of Autodock Vina. Finally, the interaction between ACE and peptide molecules was analyzed by Chimera software. According to the binding-energy value and scores of MMP3 and MMP6, their best ranked docking poses in the active site of ACE were acquired.
The cytotoxic assay was carried out according to the previous method (Zhao et al., 2019b). HUVECs were cultured at a density of 1 × 104 cells/cm2 to confluence in DMEM at 37°C in a humidified 5% CO2 atmosphere. The cytotoxicity of MMP1-MMP7 on HUVECs was measured using MTT assay (Zhao et al., 2019b). In short, HUVECs in 96-well plates at a density of 1 × 104 cells/cm2 were separately treated with 20 μL samples at 50 and 200 μM, respectively, and cultured for 24 h. Then, 20 μL MTT solution (5 mg/mL) were put in and incubated for 4 h. In the end, DMSO was joined in each well plate and the absorbance (A) at 490 nm was measured. The cell viability was calculated: Cell viability (%) = (Asample/Acontrol) × 100.
HUVECs were cultured in 96 well-plates at a density of 1 × 104 cells/cm2 and treated with Cap (1 μM), NE (0.5 μM) or ACEi peptides (100 and 200 μM for MMP3 and MMP6) for 24 h, or incubated with both 200 μM ACEi peptide (MMP3 and MMP6) and NE (0.5 μM) for 24 h. The NO and ET-1 contents of HUVECs were determined after 24 h according to their assay kits as manufactures’ protocol (Zhao et al., 2019b; Suo et al., 2022b).
The cytoprotective assay was carried out on the described methods (Cai et al., 2019; Wang et al., 2021b). In short, HUVECs were cultured in a 96-well plate at a density of 1 × 104 cells/cm2 for 24 h. Soon afterwards, the supernatant was cleared away and 20 µL of peptide sample (MMP3 or MMP6) with the final concentrations of 100 and 200 μM were joined in the sample groups, respectively. ACEi peptides (MMP3 and MMP6) were removed after 8 h and H2O2 with the final concentration of 400 µM was added in and incubated for 24 h.
The ROS content was detected on the previous method and indicated as % of blank control (Cai et al., 2019); the levels of MDA, SOD, and GSH-Px were detected using their assay kits in accordance with the manufacturer’ protocols and expressed as U/mg prot (Cai et al., 2019).
All data are expressed as the mean ± standard deviation (SD) (n = 3) and analyzed by SPSS 19.0. Significant difference analysis was employed ANOVA test with Dunnett or Tukey Test (P < 0.05, P < 0.01, or P < 0.001).
The proteins of miiuy croaker swim bladder were separately hydrolyzed using four proteases and in vitro GI digestion method. Figure 1A manifested that the DH of hydrolysate produced using in vitro GI digestion method was 27.26 ± 1.38%, which was remarkedly higher than those of other hydrolysates produced using Alcalase, Neutrase, pepsin, and trypsin, respectively (P < 0.05). In addition, the ACEi activity of five hydrolysates showed a similar trend (Figure 1B). At 3.0 mg/mL, the hydrolysate produced using in vitro GI digestion method showed the highest ACEi activity (51.37± 2.06%), followed by the hydrolysates prepared using Alcalase, Neutrase, trypsin, and pepsin, respectively (P < 0.05).
Figure 1 Degree of hydrolysis (%) (A) and ACEi activity (%)(B) of swim bladder hydrolysates of miiuy croaker using four proteases and in vitro GI digestion method. All values are means ± SD (n = 3). a–d Same letters indicate no significant difference (p < 0.05).
Enzymatic hydrolysis method is a popular process to produce protein hydrolysates because of its multiple significant properties, such as easy to control, environmentally friendly, and no residual chemical reagents (Chi et al., 2015a; Chi et al., 2015b; Sila and Bougatef, 2016). The biological functions of protein hydrolysates are closely contacted with the chemical structures and composition of bio-peptides, which is dramatically affected by the specificity of protease (Wang et al., 2013b; Chi et al., 2015c). Therefore, single and multiplex enzyme hydrolysis are frequently applied to generate hydrolysates from diverse protein sources (Lan et al., 2019; Yang et al., 2019b). Then, in vitro GI digestion method is mostly employed as an inexpensive, effective, easy to operate and safe method to prepare the protein hydrolysates (Vieira et al., 2016; Zhao et al., 2019a). Therefore, the swim bladder hydrolysate produced by in vitro GI digestion method was named as MSCH and chosen for further purification.
MSCH was fractionated into MSCH-1, MSCH-2, MSCH-3, and MSCH-4 using ultrafiltration method. The ACEi activity of MSCH-I was 62.97 ± 2.39% at 3.0 mg/mL, and its inhibiting ability was prominently higher than those of MSCH (51.37± 2.06%), MSCH-2 (49.13 ± 2.35%), MSCH-3 (46.74 ± 1.82%), and MSCH-4 (21.96 ± 1.09%) (P < 0.05). Proteins and large MW peptides are difficult to approach and combine with the active site of ACE, leading to decreased inhibitory activity (Fan et al., 2019). Therefore, ultrafiltration process often serves to collect small MW bioactive peptides from protein hydrolysates (Abdelhedi et al., 2017). The current findings agreed with the reports that the lowest MW fractions of hydrolysates from Moringa oleifera leaf (Ma et al., 2021), tuna black muscle (Qiao et al., 2022) and frame (Lee et al., 2010), smooth-hound viscera (Abdelhedi and Nasri, 2019), Antarctic krill (Zhao et al., 2019b), Cyclina sinensis (Yu et al., 2018), and Takifugu flavidus skin (Su et al., 2021) presented the highest ACEi activities. Then, MSCH-I was selected for the next step isolation.
Using gel permeation chromatography of Sephadex G-25, MSCH-1 was divided into three peptide fractions (MSCH-1a, MSCH-1b, and MSCH-1c) and their ACEi rates were displayed at Figure 2. At 3.0 mg/mL, the ACEi rate of MSCH-1b was 76.25 ± 3.61%, which was markedly stronger than those of MSCH-1 (62.97 ± 2.39%), MSCH-1a (43.57 ± 1.59%), and MSCH-1c (35.24 ± 2.21%), respectively (Figure 2B) (P < 0.05). Gel filtration is a popular method to collect fractions with a particular MW dimension from a complex mixture and is generally applied in group isolation of protein hydrolysates from different sea food, such as by-catch shrimp waste (Joshi et al., 2020), miiuy croaker muscle (He et al., 2019b), skipjack tuna byproducts (Yang et al., 2019a; Wang et al., 2022a), red stingray (Dasyatis akajei) cartilages (Pan et al., 2019), rainbow trout viscera (Vásquez et al., 2022), Antarctic krill (Zhao et al., 2019b), and three-spot seahorse (Shi et al., 2020). In the experiment, MSCH-1b displayed the best ACEi activity, but it does not obtain the lowest MW. These finding suggested that some other influence factors besides MW, such as amino acid composition and linking sequences, also significantly affect the ACEi ability of peptides (Sila and Bougatef, 2016; Abdelhedi et al., 2017; Fan et al., 2019).
Figure 2 Chromatogram profiles of MSCH-1 isolated by Sephadex G-25 (A) and ACEi activity of prepared subfractions (MSCH-1a to MSCH-1c) from MSCH-I at 3.0 mg/mL (B). All values are means ± SD (n = 3). a-e Same letters indicated no significant difference (p < 0.05).
Finally, MSCH-1b was purified by RP-HPLC. According to the RP-HPLC profiles of MSCH-1b at 220 and 280 nm (Figure 3), seven ACEi peptides with retention time of 8.49 min (MMP1), 14.47 min (MMP2), 17.75 min (MMP3), 21.52 min (MMP4), 24.50 min (MMP5), 28.41 min (MMP6), and 33.25 min (MMP7) were concentrated on their HPLC peaks and freeze-dried (Table 2).
Table 2 Amino acid sequences, molecular weights (MWs), and ACEi activity (IC50 value) of seven isolated ACEi peptides (MMP1- MMP7) from swim bladder hydrolysate of miiuy croaker (MSCH).
By employing Protein/Peptide Sequencer, the sequences of seven ACEi peptides (MMP1- MMP7) were identified as Asp-Glu-Gly-Pro-Glu (DEGPE, MMP1), Glu-Val-Gly-Ile-Gln (EVGIQ, MMP2), Ser-His-Gly-Glu-Tyr (SHGEY, MMP3), Gly-Pro-Trp-Gly-Pro-Ala (GPWGPA, MMP4), Gly-Pro-Phe-Gly-Thr-Asp (GPFGTD, MMP5), Ser-Pro-Tyr-Gly-Phe (SPYGF, MMP6), and Val-Ile-Gly-Pro-Phe (VIGPF, MMP7), respectively, and their MWs were determined as 545.49, 544.58, 591.55, 583.63, 592.59, 569.60, and 531.63 Da, respectively (Figure 4), which agreed well with their theoretical MWs (Table 2).
Figure 4 Mass spectrogram of seven ACEi peptides (MMP1- MMP7) from protein hydrolysate of miiuy croaker swim bladders (MSCH). (A) MMP1; (B) MMP2; (C) MMP3; (D) MMP4; (E) MMP5; (F) MMP6; (G) MMP7.
Table 2 indicated that the IC50 values of MMP3 and MMP6 on ACE were 0.86 ± 0.12 and 0.37 ± 0.06 mg/mL, which were notably lower than those of other five ACEi peptides (P < 0.05). Additionally, the IC50 vales of MMP3 and MMP5 were lower than those of ACEi peptides from protein hydrolysates of Arthrospira platensis (PTGNPLSP: 1.54 mg/mL) (Wang et al., 2021a), Sepia officinalisn (VGLYP: 3.23 mg/mL) (Balti et al., 2015), Oncorhynchus keta (GLP: 2.91 mg/mL) (Lee et al., 2014), Salmo salar (YP: 1.54 mg/mL) (Neves et al., 2017), Katsuwonus pelamis muscle (IPK: 2.47 mg/mL; FEM: 2.18 mg/mL) (Qiao et al., 2022; Zheng et al., 2022), Okamejei kenojei (MVGSAPGVL 3.09 mg/ml) (Ngo et al., 2015), Ctenopharyngodon idella (VAP: 1.71 mg/ml) (Chen et al., 2012), and stone fish (EVLIQ: 1.44 mg/mL) (Auwal et al., 2019). The ACEi capabilities of MMP3 and MMP6 indicated that they might serve as active ingredients added in BP lowering products.
Molecular docking experiment of MMP3 and MMP6 was carried out to analyze their ACEi mechanisms (Figure 5). Figure 5A indicated that MMP3 (SHGEY) formed hydrogen bonds with Ala354, His353, Glu376, Ser284, His383, His387, and Tyr523 residues of ACE, therein, MMP3 (SHGEY) formed hydrogen bonds with S1 pocket (Ala354) and S2 pocket (His353). Additionally, MMP3 (SHGEY) interacted with Glu384, Glu411, Lys454, and Lys511 residues through hydrophobic effect, and contacted with Val380 and Val379 residues through electrostatic force. Figure 5B confirmed that MMP6 (SPYGF) formed hydrogen bonds with Tyr523(S1), His513(S2), Glu411, and Arg522 residues of ACE, therein, MMP6 (SPYGF) established hydrogen bonds with S1 (Tyr523) and S2 (His513) pockets. Furthermore, MMP6 (SPYGF) interacted with His387 residue through hydrophobic effect, and contacted with Trp357, Pro407, and His410 residues through electrostatic force. Molecular docking assay proved that MMP3 and MMP6 exhibited better ACEi activity attributing to the effectively interacting with the active sites of ACE by different forces, especially hydrophobic interaction, electrostatic force, and hydrogen bonding.
Figure 5 Molecular docking result of MMP3 (A) and MMP6 (B) with ACE (A1) 2-D details of ACE and MMP3 interaction. (A2) 3-D interaction details for MMP3; (B1) 2-D details of ACE and MMP6 interaction; (B2) 3-D interaction details for MMP6.
In addition, the affinity of MMP3 and MMP6 with ACE was -8.3 and -9.2 kcal/mol, which was close to those of YLLLK (-8.2 kcal/mol), GVQEGAGHYALL (-7.0 kcal/mol) (Zarei et al., 2019), YSK (-7.9 kcal/mol) (Wang et al., 2017), SP (-5.7 kcal/mol), VDRYF (-9.7 kcal/mol) (Zheng et al., 2022), PMHIR (-10.37 kcal/mol), and PQVSTPTL (-6.64 kcal/mol) (Li et al., 2022).
Molecular size greatly affects the affinity between peptide and ACE, which further remarkedly influences ACEi ability of antihypertensive peptides (Abdelhedi and Nasri, 2019; Fan et al., 2019). Crystallographic studies proved that potent ACEi peptides with 2 to 12 amino acid residues are easy to link to the acting site of ACE (Chen et al., 2012). Similarly, VPP and IPP could be easier access the ACE channel and effectively coordinate with Zn2+ with higher interaction scores than larger peptides (Abdelhedi et al., 2018). In addition, Su et al. proved that peptides with four to nine amino acid residues could passively pass through cell membranes to play their functions in cells (Su et al., 2021). In the experiment, MMP3 (SHGEY) and MMP6 (SPYGF) are pentapeptides, and the MWs increase their access to the active site of ACE, which was proved by their affinities with ACE (-8.7 and -9.7 kcal/mol for MMP3 and MMP6, respectively).
The amino acid composition and sequence could be more relevant with the ACEi activity of peptides than the MWs (Auwal et al., 2019). The C-terminal amino acids have been wildly discussed because of their crucial functions on the antihypertensive peptides (Fan et al., 2019). The aromatic (Tyr, Phe, and Trp) and branched-chain (Val, Leu and Ile) amino acids were in favor of combining with the C-terminal active site of ACE (Sun et al., 2017). Therefore, Tyr and Phe at the C-terminus of MMP3 and MMP6 are critical for their affinities with ACE. Ser was regarded as conducing to the high ACEi activity of EMFGTSSET because it could establish three hydrogen bonds with ACE residues Ala-356 and Tyr-523 (Pei et al., 2022). Also, molecular docking was found hydroxyl group of Ser residue played vital effects in the ACEi capability of SP because it could form hydrogen bond with the His383 residue of ACE (Zheng et al., 2022). Therefore, Ser residues of MMP3 and MMP6 could significantly strengthen their ACEi activity. Furthermore, ACEi peptides with reasonable proportion of hydrophilic and hydrophobic amino acids can helpfully bind with the active center of ACE to control its function (Yea et al., 2014; Auwal et al., 2019). In MMP3 and MMP6, MMP6 has a more reasonable ratio of hydrophobic and hydrophilic amino acids, and this should be the main reason that MMP6 showed more ACEi activity than MMP3.
The effects of MMP3 and MMP6 on the viability of HUVECs at 100-400 μM were shown in Figure 6A. At the designed concentration, the viability of MMP3 group was ranged from 98.67 ± 2.08% to 101.25 ± 0.98%, and the viability of MMP6 group was ranged from 98.81 ± 1.67% to 102.18 ± 1.52%. In normal tissues, the cell proliferation and death generally keep an appropriate balance, and the active substances with strong inhibiting ability on cell proliferation indicate their possible toxicity risk to the body, and are deemed to be inadequacy to developing healthy products with non-antitumor functions (Zhao et al., 2019b; Qiao et al., 2022). These data suggested that MMP3 and MMP6 didn’t show significant cell toxicity in HUVECs and should suite to developing anti-blood pressure health products.
Figure 6 The effects of MMP3 and MMP6 on the cell viability (A), NO production (B), and ET-1secretion (C) of HUVECs, respectively. Captopril (Cap) was designed as a positive control. All values are means ± SD (n= 3). ***P < 0.001 VS Control; ##P < 0.01 and ###P < 0.001 VS Cap; ▲▲▲P < 0.001 VS Norepinephrine (NE).
According to Figure 6B, the NO contents in MMP3 and MMP6 groups were significantly increased than control group (35.06±1.86 μmol/gprot) (P < 0.001), and the NO contents of MMP3 and MMP6 groups increased to 48.91 ± 2.46 and 51.38 ± 2.56 μmol/gprot at 200 μM. However, the NO contents in HUVECs treated with MMP3 and MMP6 were lower than that (60.82±2.67 μmol/gprot) of Cap treated group (P < 0.001). Moreover, NE could significantly decrease the content of NO (24.67±1.15 μmol/gprot) than control group (P < 0.001), but the NO content negative decreased by NE was separately compensated to 39.75 ± 1.83 and 42.26 ± 1.85 μmol/gprot in MMP3 and MMP6 group at 200 μM (P < 0.001).
Figure 6C indicated that the ET-1 secretion of HUVECs was negatively affected by MMP3 and MMP6, and the ET-1 levels of MMP3 and MMP6 groups reduced to 98.39 ± 3.65 and 95.38 ± 3.36 pg/mL at 200 μM. On the contrary, NE could significantly increase the ET-1 secretion (152.69 ± 5.38 pg/mL) than control group (125.39 ± 4.69 pg/mL) (P < 0.001), but the decrease of ET-1 secretion influenced by NE was partially restored by MMP3 and MMP6 treatment and decreased to 110.79 ± 4.28 and 105.72 ± 4.92 pg/mL at 200 μM (P < 0.001). The ET-1 secretion of HUVECs was significantly decreased to 93.54 ± 3.15 pg/mL by 0.5 μM Cap treatment than control group (125.39 ± 4.69 pg/mL) (P < 0.001).
Hypertension is a complicated chronic disease which leads to endothelial dysfunction by influencing the production of NO and ET-1 in endothelial cells, which further causes apoptosis and arterial vasoconstriction. Therefore, HUVECs are widely applied to analyze vascular endothelium characters and the key biological pathways on endothelium function and cardiovascular diseases. In pathologic situations, NO deficiency will give rise to the risk of cardiovascular, and improving the production of endothelial NO represents a good therapeutic approach for atherosclerosis (Abdelhedi and Nasri, 2019; Fan et al., 2019). Therefore, some ACEi peptides, such as KYIPIQ (Lin et al., 2020), IPIPATKT (Chen et al., 2021), WF (Zhao et al., 2019b), GRVSNCAA, TYLPVH (Zhang et al., 2021), IVTNWDDMEK, VGPAGPRG (Wang et al., 2022b), SP (Zheng et al., 2022), MKKS and LPRS (Qiao et al., 2022), play their hypotensive activity by enhancing the production of NO in HUVECs.
Literatures reported that increasing in Ang-II and ET-1 could cause endothelial abnormalities, which are closely correlated with hypertension and coronary heart disease (Jiang et al., 2021). Decreased production of ET-1 in the renal medulla can elevate systemic blood pressure (Kohan, 2008). ACEi oligopeptides of SP, YRK, MKKS, FQK, FAS, and LPRS from tuna muscle and Antarctic krill displayed same function of bringing down the ET-1 level (Zhao et al., 2019b; Qiao et alo, 2022). VIEPR and VVLYK from oil palm kernel expeller could dose-dependently inhibit the secretion of intracellular ET-1 in EA.hy926 cells (Zheng et al., 2017). GRVSNCAA and TYLPVH from Ruditapes philippinarum gave play to their function of lowering blood pressure through markedly lowering ET-1 generation (Zhang et al., 2021). Umami Peptide IPIPATKT from Sanhuang chicken hydrolysate could reduce the ET-1content in the insulin-resistant-HepG2 (IR-HepG2) and HUVEC models (Jiang et al., 2021). Moreover, IPIPATKT displayed hypotensive and decreased glucose level effects in SHRs and C57BL/6N mice. According to the finding, ACEi peptides of MMP3 and MMP6 prominently promote NO production while restrict ET-1 secretion in HUVECs. Moreover, MMP3 and MMP6 can reverse the negative effects of NE on NO and ET-1 production in HUVECs.
Figure 7A presented the cytoprotective effects of MMP3 and MMP6 on the H2O2-induced HUVECs at 100 and 200 μM. MMP3 and MMP6 revealed the dose-dependently protective effects on the H2O2-damaged HUVECs, and the cell viability of MMP3 and MMP6 groups at 200 μM were increased to 65.37 ± 2.49% and 70.03 ± 1.82%, respectively, which were significantly higher than that of model group (48.96 ± 3.46%) (P < 0.001). However, the cell viability of MMP3 and MMP6 groups was lower than that of the GSH group (78.63 ± 2.68%) (P < 0.001). Then, MMP3 and MMP6 could give a strong protection to H2O2-induced HUVECs by increasing their viability.
Figure 7 Effects of MMP3 and MMP6 on the cell viability (A), ROS (B), SOD (C), GSH-Px (D), and MDA (E) of H2O2-induced HUVECs. All values are means ± SD (n= 3). GSH was designed as a positive control. *** P < 0.001 < 0.01 VS control; ### P < 0.001 VS model; ▲▲▲ P < 0.001 VS GSH.
Figure 7B indicated that the ROS levels were markedly lowered after pretreating with MMP3 and MMP6 compared with model group (231.85 ± 5.62%) (P < 0.001). At 200 μM, the ROS levels of MMP3 and MMP6 groups were observably dropped to 158.92 ± 3.16% and 142.37 ± 4.36% of the control group, respectively. However, the ROS levels of MMP3 and MMP6 groups were higher than that of the GSH group (120.75 ± 3.98%) (P < 0.001). In addition, MMP6 showed the higher ability on scavenging ROS than MMP3 at the determined concentrations.
Figure 7C and Figure 7D showed the activity of SOD and GSH-Px incubated with MMP3 and MMP6 at 100 and 200 μM was gradually increased. At the concentrations of 100 and 200 μM, SOD activity in MMP3 group was 143.59 ± 3.08 and 170.66 ± 5.32 U/mg prot, and the SOD activity in MMP6 groups was 165.38 ± 3.96 and 190.53 ± 4.67 U/mg prot. Moreover, the SOD activity in MMP3 and MMP6 groups was markedly higher than that of the model group (112.69 ± 3.57 U/mg prot) (P < 0.001). The changes of GSH-Px activity showed the same trend with the levels of SOD (Figure 7D). At the concentrations of 100 and 200 μM, the GSH-Px activity in MMP3 group was 46.58 ± 2.01 and 53.27 ± 2.85 U/mg prot, and the activity in MMP6 group was 50.39 ± 1.39 and 59.87 ± 1.88 U/mg prot. GSH-Px activity of peptide groups were observably higher than that of the model group (36.98 ± 1.23 U/mg prot) (P < 0.001).
Figure 7E revealed that the MDA levels were markedly lowered after pretreating with MMP3 and MMP6 compared with model group (33.19 ± 1.52 nmol/mg prot) (P < 0.001). At 200 μM, the MDA levels of MMP3 and MMP6 groups were dramatically decreased to 19.53 ± 0.76 and 18.75 ± 0.13 nmol/mg prot, respectively. MMP6 showed stronger ability on decreasing MDA content than MMP3, but it ability was still lower to that of GSH (16.85 ± 0.96 nmol/mg prot).
Oxidative stress is bound up with apoptosis, cell migration, hypertrophy, inflammation, angiogenesis, and endothelial dysfunction with reference to vascular remodeling of hypertension-related diseases (Sinha and Dabla, 2015). ROS is known as the mediums of angiotensin II-induced blood pressure, and inhibition of ROS content is contributed to normalizing endothelium function and decreased vascular inflammation and reaction, which further reduce blood pressure and prevent the hypertension development (Sinha and Dabla, 2015). For another, MDA is a key peroxidation product of the cell membrane lipid and serves as a well-known indicator for estimating the oxidative damage degree (Wang et al., 2021b). Presently, some food-derived peptides showed ACEi and antioxidant activities, such as. TPCPPQ, YSKA, and VLSTSFPPK from Pixian broad bean (Li et al., 2021), rice bran (Wang et al., 2017), and Kluyveromyces marxianus (Mirzaei et al., 2018), respectively. Moreover, some bioactive peptides showed significant protective function in Angiotensin II or H2O2-induced cell models. Zheng et al. found that ACEi peptides of VIEPR, LPILR, ADVFNPR and VVLYK from Oil palm kernel expeller could exert antihypertensive effect through scavenging excessive ROS and protect vascular endothelial cells from excessive ROS-induced damage (Zheng et al., 2017). Umami peptides of CC, CCNK, and HCHT played their cytoprotective effects by reducing the ROS content (Hao et al., 2020). ACEi peptides IVTNWDDMEK and VGPAGPRG could protect HUVECs against H2O2 damage by up-regulating the expression of nuclear factor erythroid 2-related factor 2 (Nrf2)/heme oxygenase-1 (HO-1) to decrease the production of ROS and MDA (Wang et al., 2022b). ACEi peptide EMFGTSSET (IC50 15.08 μM) from Isochrysis zhanjiangensis has excellent effect in regulating hypertension by reducing the contents of ROS, cytokines, and adhesion factors in Ang II-induced HUVECs (Pei et al., 2022). FNLRMQ from Takifugu bimaculatus skin could alleviated the viability and facilitated apoptosis of Ang-II-induced HUVECs by regulating phosphatidylinositol-3-kinase (PI3K)/protein kinase B (Akt)/endothelial NO synthase (eNOS) and Nrf2/HO-1 signaling pathways (Cai et al., 2021). FPYLRH, FWKVV, and FMPLH could promote the bioactivity of SOD/GSH-Px to bring down the oxidative damage of DNA and the production of ROS and MDA in H2O2-induced HUVECs (Wang et al., 2020). Moreover, the antioxidant mechanisms of bioactive peptides, such as FEIHCC (Chen et al., 2020), IVTNWDDMEK, VGPAGPRG (Wang et al., 2022b), KVLPVPEK (Tonolo et al., 2020), MHQPHQPLPPTVMF (Qin et al., 2021), APKGVQGPNG (Rahman et al., 2018), ICRD, LCGEC (Han et al., 2020), and VHVV (Tsai et al., 2020), indicated that they could regulate the Nrf2 pathway in cell model to induce the overexpression of antioxidases to decrease the damage of ROS. The current results suggested that the protective activities to H2O2-damaged HUVECs of MMP3 and MMP6 were similar to those previous reported peptides, and the acting mechanism should be connected with activating Nrf2 pathway to improve intracellular antioxidase bioactive.
In a conclusion, the swim bladder hydrolysate of miiuy croaker with high ACEi activity was produced using in vitro GI digestion processing, and seven novel ACEi peptides were isolated from the hydrolysate and identified as DEGPE, EVGIQ, SHGEY, GPWGPA, GPFGTD, SPYGF, and VIGPF, respectively. SHGEY and SPYGF displayed noticeable hypotensive activity through inhibiting ACE activity, increasing NO production and decreasing ET-1 secretion in HUVECs, and protecting HUVECs from H2O2-induced oxidative damage. Moreover, SHGEY and SPYGF exhibited significant ACEi activity attributing to their effective interaction with the active sites of ACE by hydrogen bonding, electrostatic force and hydrophobic interaction. Therefore, this study not only develops technical support for utilizing miiuy croaker swim bladders to produce novel ACEi peptides, but also contributes to dispose the environmental pollution problems of fish byproducts. More importantly, seven novel ACEi peptides, especially SHGEY and SPYGF, might be used as natural functional ingredients for developing noticeable hypotensive products. However, the antihypertensive activities and mechanisms of SHGEY and SPYGF in animal models should be performed in future experiments, which will provide better insight into their potential in the management of hypertension.
The original contributions presented in the study are included in the article/supplementary material. Further inquiries can be directed to the corresponding authors
W-YZ: investigation, methodology, data analysis, and validation. Y-MW: methodology, data analysis, and writing-original draft. S-KS and S-LZ: data curation, investigation, and methodology. BW: resources, funding acquisition, supervision, and writing-review and editing. All authors contributed to the article and approved the submitted version.
This work was funded by the National Natural Science Foundation of China (No. 82073764) and the Ten-Thousand Talents Plan of Zhejiang Province (No. 2019R52026).
The authors declare that the research was conducted in the absence of any commercial or financial relationships that could be construed as a potential conflict of interest.
All claims expressed in this article are solely those of the authors and do not necessarily represent those of their affiliated organizations, or those of the publisher, the editors and the reviewers. Any product that may be evaluated in this article, or claim that may be made by its manufacturer, is not guaranteed or endorsed by the publisher.
ACE, angiotensin I-converting enzyme; ACEi, angiotensin-I-converting enzyme inhibitory; GI, gastrointestinal; MMP1, Asp-Glu-Gly-Pro-Glu (DEGPE); MMP2, Glu-Val-Gly-Ile-Gln (EVGIQ); MMP3, Ser-His-Gly-Glu-Tyr (SHGEY); MMP4, Gly-Pro-Trp-Gly-Pro-Ala (GPWGPA); MMP5, Gly-Pro-Phe-Gly-Thr-Asp (GPFGTD); MMP6, Ser-Pro-Tyr-Gly-Phe (SPYGF); MMP7, Val-Ile-Gly-Pro-Phe (VIGPF); ET-1, endothelin-1; NO, nitric oxide; BP, blood pressure; Ang, angiotensin; Cap, captopril; SHRs, spontaneously hypertensive rats; HUVECs, human umbilical vein endothelial cells; SOD, superoxide dismutase; GSH-Px, glutathione peroxidase; ROS, reactive oxygen species; GSH, glutathione; TNBS, 2,4,6-Trinitrobenzene sulfonic acid; FAPGG, N-[3-(2-Furyl)acryloyl]-Phe-Gly-Gly; NE, norepinephrine; SD, standard deviation; GPC, gel permeation chromatography; MTT, 3-(4,5-Dimethylthiazol-2yl)-2,5-diphenyltetrazolium bromide; CAN, acetonitrile; MSCH, protein hydrolysate of miiuy croaker swim bladder prepared using in vitro GI digestion processing; RP-HPLC, reversed-phase high performance liquid chromatography; MW, molecular weight; Q-TOF, quadrupole time-of-flight; DH, degree of hydrolysis; HEPES, 4-(2-hydroxyethyl)-1-piperazineethanesulfonic acid; Nrf2, nuclear factor erythroid 2-related factor 2; HO-1, heme oxygenase-1; PI3K, phosphatidylinositol-3-kinase; Akt, protein kinase B; eNOS, endothelial NO synthase.
Abdelhedi O., Nasri M. (2019). Basic and recent advances in marine antihypertensive peptides: Production, structure-activity relationship and bioavailability. Trend. Food Sci. Tech. 88, 543–557. doi: 10.1016/j.tifs.2019.04.002
Abdelhedi O., Nasri R., Jridi M., Mora L., Oseguera-Toledo M. E., Aristoy M. C., et al. (2017). In silico analysis and antihypertensive effect of ACE-inhibitory peptides from smooth-hound viscera protein hydrolysate: Enzyme-peptide interaction study using molecular docking simulation. Process. Biochem. 58, 145–159. doi: 10.1016/j.procbio.2017.04.032
Abdelhedi O., Nasri R., Mora L., Jridi M., Toldra F., Nasri M. (2018). In silico analysis and molecular docking study of angiotensin I-converting enzyme inhibitory peptides from smooth-hound viscera protein hydrolysates fractionated by ultrafiltration. Food Chem. 239, 453–463. doi: 10.1016/j.foodchem.2017.06.112
Abedin M. M., Chourasia R., Phukon L. C., Singh S. P., Rai A. K. (2022). Characterization of ACE inhibitory and antioxidant peptides in yak and cow milk hard chhurpi cheese of the sikkim Himalayan region. Food Chem. X. 13, 100231. doi: 10.1016/j.fochx.2022.100231
Auwal S. M., Abidin N. Z., Zarei M., Tan C. P., Saari N. (2019). Identification, structure-activity relationship and in silico molecular docking analyses of five novel angiotensin I-converting enzyme (ACE)-inhibitory peptides from stone fish (Actinopyga lecanora) hydrolysates. PloS One 14, e0197644. doi: 10.1371/journal.pone.0197644
Balti R., Bougatef A., Sila A., Guillochon D., Dhulster P., Nedjar-Arroume N. (2015). Nine novel angiotensin I-converting enzyme (ACE) inhibitory peptides from cuttlefish (Sepia officinalis) muscle protein hydrolysates and antihypertensive effect of the potent active peptide in spontaneously hypertensive rats. Food Chem. 170, 519–525. doi: 10.1016/j.foodchem.2013.03.091
Borges-Contreras B., Martínez-Sánchez C. E., Herman-Lara E., Rodríguez-Miranda J., Hernández-Santos B., Juárez-Barrientos J. M., et al. (2019). Angiotensin-converting enzyme inhibition in vitro by protein hydrolysates and peptide fractions from mojarra of Nile tilapia (Oreochromis niloticus) skeleton. J. Med. Food. 22 (3), 286–293. doi: 10.1089/jmf.2018.0163
Cai S., Pan N., Xu M., Su Y., Qiao K., Chen B., et al. (2021). ACE inhibitory peptide from skin collagen hydrolysate of takifugu bimaculatus as potential for protecting HUVECs injury. Mar. Drugs 19 (12), 655. doi: 10.3390/md19120655
Cai S. Y., Wang Y. M., Zhao Y. Q., Chi C. F., Wang B. (2019). Cytoprotective effect of antioxidant pentapeptides from the protein hydrolysate of swim bladders of miiuy croaker (Miichthys miiuy) against H2O2-mediated human umbilical vein endothelial cell (HUVEC) injury. Int. J. Mol. Sci. 20 (21), 5425. doi: 10.3390/ijms20215425
Chakrabarti S., Liao W., Davidge S. T., Wu J. (2017). Milk-derived tripeptides IPP (Ile-Pro-Pro) and VPP (Val-Pro-Pro) differentially modulate angiotensin II effects on vascular smooth muscle cells. J. Funct. Foods. 30, 151–158. doi: 10.1016/j.jff.2016.12.022
Chakraborty R., Roy S. (2022). Angiotensin-converting enzyme inhibitors from plants: A review of their diversity, modes of action, prospects, and concerns in the management of diabetes-centric complications. J. Integr. Med. 19 (6), 478–492.
Chen M., Pan D., Zhou T., Gao X., Dang Y. (2021). Novel umami peptide IPIPATKT with dual dipeptidyl peptidase-IV and angiotensin I-converting enzyme inhibitory activities. J. Agric. Food Chem. 69 (19), 5463–5470. doi: 10.1021/acs.jafc.0c07138
Chen J., Tan L., Li C., Zhou C., Hong P., Sun S., et al. (2020). Mechanism analysis of a novel angiotensin-i-converting enzyme inhibitory peptide from isochrysis zhanjiangensis microalgae for suppressing vascular injury in human umbilical vein endothelial cells. J. Agric. Food Chem. 68 (15), 4411–4423. doi: 10.1021/acs.jafc.0c00925
Chen J., Wang Y., Zhong Q., Wu Y., Xia W. (2012). Purification and characterization of a novel angiotensin-I converting enzyme (ACE) inhibitory peptide derived from enzymatic hydrolysate of grass carp protein. Peptides 33, 52–58. doi: 10.1016/j.peptides.2011.11.006
Chi C. F., Hu F. Y., Wang B., Li Z. R., Luo H. Y. (2015c). Influence of amino acid compositions and peptide profiles on antioxidant capacities of two protein hydrolysates from skipjack tuna (Katsuwonus pelamis) dark muscle. Mar. Drugs 13 (5), 2580–2601. doi: 10.3390/md13052580
Chi C. F., Wang B., Hu F. Y., Wang Y. M., Zhang B., Deng S. G., et al. (2015a). Purification and identification of three novel antioxidant peptides from protein hydrolysate of bluefin leatherjacket (Navodon septentrionalis) skin. Food Res. Int. 73, 124–129. doi: 10.1016/j.foodres.2014.08.038
Chi C. F., Wang B., Wang Y. M., Deng S. G., Ma J. Y. (2014). Isolation and characterization of three antioxidant pentapeptides from protein hydrolysate of monkfish (Lophius litulon) muscle. Food Res. Int. 55 (1), 222–228. doi: 10.1016/j.foodres.2013.11.018
Chi C. F., Wang B., Wang Y. M., Zhang B., Deng S. G. (2015b). Isolation and characterization of three antioxidant peptides from protein hydrolysate of bluefin leatherjacket (Navodon septentrionalis) heads. J. Funct. Foods. 12, 1–10. doi: 10.1016/j.jff.2014.10.027
Fan H., Liao W., Wu J. (2019). Molecular interactions, bioavailability, and cellular mechanisms of angiotensin-converting enzyme inhibitory peptides. J. Food Biochem. 43 (1), e12572. doi: 10.1111/jfbc.12572
Geng S., Xu T., Sun Y. (2021). Genome-wide identification and analysis of chemokine receptor superfamily in miiuy croaker, miichthys miiuy. Fish. Shellfish. Immun. 118, 343–353. doi: 10.1016/j.fsi.2021.09.017
Gu Y., Liang Y., Bai J., Wu W., Lin Q., Wu J. (2019). Spent hen-derived ACE inhibitory peptide IWHHT shows antioxidative and anti-inflammatory activities in endothelial cells. J. Funct. Foods. 53, 85–92. doi: 10.1016/j.jff.2018.12.006
Guo Y., Wang K., Wu B., Wu P., Duan Y., Ma H. (2020). Production of ACE inhibitory peptides from corn germ meal by an enzymatic membrane reactor with a novel gradient diafiltration feeding working-mode and in vivo evaluation of antihypertensive effect. J. Funct. Foods. 64, 103584. doi: 10.1016/j.jff.2019.103584
Han J., Huang Z., Tang S., Lu C., Wan H., Zhou J., et al. (2020). The novel peptides ICRD and LCGEC screened from tuna roe show antioxidative activity via Keap1/Nrf2-ARE pathway regulation and gut microbiota modulation. Food Chem. 327, 127094. doi: 10.1016/j.foodchem.2020.127094
Hao L., Gao X., Zhou T., Caom J., Sun Y., Dang Y., et al. (2020). Angiotensin I-converting enzyme (ACE) inhibitory and antioxidant activity of umami peptides after in vitro gastrointestinal digestion. J. Agric. Food Chem. 68 (31), 8232–8241. doi: 10.1021/acs.jafc.0c02797
He Y., Pan X., Chi C. F., Sun K. L., Wang B. (2019b). Ten new pentapeptides from protein hydrolysate of miiuy croaker (Miichthys miiuy) muscle: Preparation, identification, and antioxidant activity evaluation. LWT 105, 1–8. doi: 10.1016/j.lwt.2019.01.054
He R., Wang Y., Yang Y., Wang Z., Ju X., Yuan J. (2019a). Rapeseed protein-derived ACE inhibitory peptides LY, RALP and GHS show antioxidant and anti-inflammatory effects on spontaneously hypertensive rats. J. Funct. Foods. 55, 211–219. doi: 10.1016/j.jff.2019.02.031
Huang Y., Jia F., Zhao J., Hou Y., Hu S. Q. (2021). Novel ACE inhibitory peptides derived from yeast hydrolysates: Screening, inhibition mechanisms and effects on HUVECs. J. Agric. Food Chem. 69 (8), 2412–2421. doi: 10.1021/acs.jafc.0c06053
Jiang S., Chen G., Yang Z., Wang D., Lu Y., Zhu L., et al. (2021). Testosterone attenuates hypoxia-induced hypertension by affecting NRF1-mediated transcriptional regulation of ET-1 and ACE. Hypertens. Res. 44 (11), 1395–1405. doi: 10.1038/s41440-021-00703-4
Joshi I., Janagaraj K., Noorani K. P. M., Nazeer R. A. (2020). Isolation and characterization of angiotensin I - converting enzyme (ACE-I) inhibition and antioxidant peptide from by-catch shrimp (Oratosquilla woodmasoni) waste. Biocatal. Agric. Biotechnol. 29, 101770. doi: 10.1016/j.bcab.2020.101770
Kharazmi-Khorassani J., Asoodeh A., Tanzadehpanah H. (2019). Antioxidant and angiotensin-converting enzyme (ACE) inhibitory activity of thymosin alpha-1 (Thα1) peptide. Bioorg. Chem. 87, 743–752. doi: 10.1016/j.bioorg.2019.04.003
Kohan D. E. (2008). Endothelin-1 and hypertension: from bench to bedside. Curr. Hypertens. Rep. 10 (1), 65–69. doi: 10.1007/s11906-008-0013-2
Lan C., Zhao Y. Q., Li X. R., Wang B. (2019). High Fischer ratio oligopeptides determination from antartic krill: Preparation, peptides profiles, and in vitro antioxidant activity. J. Food Biochem. 43 (5), e12827. doi: 10.1111/jfbc.12827
Lee S. Y., Hur S. J. (2017). Antihypertensive peptides from animal products, marine organisms, and plants. Food Chem. 228, 506–517. doi: 10.1016/j.foodchem.2017.02.039
Lee J. K., Jeon J. K., Byun H. G. (2014). Antihypertensive effect of novel angiotensin I converting enzyme inhibitory peptide from chum salmon (Oncorhynchus keta) skin in spontaneously hypertensive rats. J. Funct. Foods. 7, 381–389. doi: 10.1016/j.jff.2014.01.021
Lee S. H., Qian Z. J., Kim S. K. (2010). A novel angiotensin I converting enzyme inhibitory peptide from tuna frame protein hydrolysate and its antihypertensive effect in spontaneously hypertensive rats. Food Chem. 118, 96–102. doi: 10.1016/j.foodchem.2009.04.086
Li M., Fan W., Xu Y. (2021). Identification of angiotensin converting enzyme (ACE) inhibitory and antioxidant peptides derived from pixian broad bean paste. LWT 151, 112221. doi: 10.1016/j.lwt.2021.112221
Li X., Feng C., Hong H., Zhang Y., Luo Z., Wang Q., et al. (2022). Novel ACE inhibitory peptides derived from whey protein hydrolysates: Identification and molecular docking analysis. Food Biosci. 48, 101737. doi: 10.1016/j.fbio.2022.101737
Lin K., Ma Z., Ramachandran M., De Souza C., Han X., Zhang L. W. (2020). ACE inhibitory peptide KYIPIQ derived from yak milk casein induces nitric oxide production in HUVECs and diffuses via a transcellular mechanism in caco-2 monolayers. Process. Biochem. 99, 103–111. doi: 10.1016/j.procbio.2020.08.031
Li L. Y., Zhao Y. Q., He Y., Chi C. F., Wang B. (2018). Physicochemical and antioxidant properties of acid- and pepsin-soluble collagens from the scales of miiuy croaker (Miichthys miiuy). Mar. Drugs 16 (10), 394. doi: 10.3390/md16100394
Mabhida S. E., Mashatola L., Kaur M., Sharma J. R., Apalata T., Muhamed B., et al. (2021). Hypertension in African populations: Review and computational insights. Genes 12, 532. doi: 10.3390/genes12040532
Majumder K., Wu J. (2015). Molecular targets of antihypertensive peptides: Understanding the mechanisms of action based on the pathophysiology of hypertension. Int. J. Mol. Sci. 16, 256–283. doi: 10.3390/ijms16010256
Manikkam V., Vasiljevic T., Donkor O. N., Mathai M. L. (2016). A review of potential marine-derived hypotensive and anti-obesity peptides. Crit. Rev. Food Sci. Nutr. 56 (1), 92–112. doi: 10.1080/10408398.2012.753866
Ma K., Wang Y., Wang M., Wang Z., Wang X., Ju X., et al. (2021). Antihypertensive activity of the ACE-renin inhibitory peptide derived from moringa oleifera protein. Food Funct. 12 (19), 8994–9006. doi: 10.1039/D1FO01103K
Mirzaei M., Mirdamadi S., Ehsani M. R., Aminlari M. (2018). Production of antioxidant and ACE-inhibitory peptides from kluyveromyces marxianus protein hydrolysates: Purification and molecular docking. J. Food Drug Anal. 26 (2), 696–705. doi: 10.1016/j.jfda.2017.07.008
Neves A. C., Harnedy P. A., O'Keeffe M. B., FitzGerald R. J. (2017). Bioactive peptides from Atlantic salmon (Salmo salar) with angiotensin converting enzyme and dipeptidyl peptidase IV inhibitory, and antioxidant activities. Food Chem. 218, 396–405. doi: 10.1016/j.foodchem.2016.09.053
Ngo D. H., Kang K. H., Ryu B., Vo T. S., Jung W. K., Byun H. G., et al. (2015). Angiotensin-I converting enzyme inhibitory peptides from antihypertensive skate (Okamejei kenojei) skin gelatin hydrolysate in spontaneously hypertensive rats. Food Chem. 174, 37–43. doi: 10.1016/j.foodchem.2014.11.013
Pan X. Y., Wang Y. M., Li L., Chi C. F., Wang B. (2019). Four antioxidant peptides from protein hydrolysate of red stingray (Dasyatis akajei) cartilages: Isolation, identification, and in vitro activity evaluation. Mar. Drugs 17 (5), 263. doi: 10.3390/md17050263
Pei Y., Cai S., Ryu B., Zhou C., Hong P., Qian Z. (2022). An ACE inhibitory peptide from isochrysis zhanjiangensis exhibits antihypertensive effect via anti-inflammation and anti-apoptosis in HUVEC and hypertensive rats. J. Funct. Foods. 92, 105061. doi: 10.1016/j.jff.2022.105061
Pujiastuti D. Y., Ghoyatul Amin M. N., Alamsjah M. A., Hsu J. L. (2019). Marine organisms as potential sources of bioactive peptides that inhibit the activity of angiotensin I-converting enzyme: A review. Molecules 24 (14), 2541. doi: 10.3390/molecules24142541
Qiao Q. Q., Luo Q. B., Suo S. K., Zhao Y. Q., Chi C. F., Wang B. (2022). Preparation, characterization, and cytoprotective effects on HUVECs of fourteen novel angiotensin-i-converting enzyme inhibitory peptides from protein hydrolysate of tuna processing by-products. Front. Nutr. 9, 868681. doi: 10.3389/fnut.2022.868681
Qin D., Yang F., Hua Z., Liu J., Wu Q., Luo Y., et al. (2021). Peptide T8 isolated from yak milk residue ameliorates H2O2-induced oxidative stress through Nrf2 signaling pathway in HUVEC cells. Food Biosci. 44 (Part A), 101408. doi: 10.1016/j.fbio.2021.101408
Rahman M. S., Choi Y. H., Choi Y. S., Alam M. B., Lee S. H., Yoo J. C. (2018). A novel antioxidant peptide, purified from bacillus amyloliquefaciens, showed strong antioxidant potential via nrf-2 mediated heme oxygenase-1 expression. Food Chem. 239, 502–510. doi: 10.1016/j.foodchem.2017.06.106
Sheng Y., Qiu Y. T., Wang Y. M., Chi C. F., Wang B. (2022). Novel antioxidant collagen peptides of Siberian sturgeon (Acipenser baerii) cartilages: The preparation, characterization, and cytoprotection of H2O2-damaged human umbilical vein endothelial cells (HUVECs). Mar. Drugs 20 (5), 325. doi: 10.3390/md20050325
Shi J., Su R. Q., Zhang W. T., Chen J. (2020). Purification and the secondary structure of a novel angiotensin I-converting enzyme (ACE) inhibitory peptide from the alcalase hydrolysate of seahorse protein. J. Food Sci. Technol. 57 (11), 3927–3934. doi: 10.1007/s13197-020-04427-0
Sila A., Bougatef A. (2016). Antioxidant peptides from marine by-products: Isolation, identification and application in food systems. a review. J. Funct. Foods. 21, 10–26. doi: 10.1016/j.jff.2015.11.007
Sinha N., Dabla P. K. (2015). Oxidative stress and antioxidants in hypertension-a current review. Curr. Hypertens. Rev. 11 (2), 132–142. doi: 10.2174/1573402111666150529130922
Su Y., Chen S., Cai S., Liu S., Pan N., Su J., et al. (2021). A novel angiotensin-i-converting enzyme (ACE) inhibitory peptide from takifugu flavidus. Mar. Drugs 19 (12), 651. doi: 10.3390/md19120651
Sun L., Wu S., Zhou L., Wang F., Lan X., Sun J., et al. (2017). Separation and characterization of angiotensin I converting enzyme (ACE) inhibitory peptides from saurida elongata proteins hydrolysate by IMACNi2+. Mar. Drugs 15 (2), 29. doi: 10.3390/md20050325
Suo S. K., Zhao Y. Q., Wang Y. M., Pan X. Y., Chi C. F., Wang B. (2022a). Seventeen novel angiotensin converting enzyme (ACE) inhibitory peptides from protein hydrolysate of mytilus edulis: Isolation, identification, molecular docking study, and protective function on HUVECs. Food Funct. doi: 10.1039/D2FO00275B
Suo S. K., Zheng S. L., Chi C. F., Luo H. Y., Wang B. (2022b). Novel ACE inhibitory peptides from tuna byproducts-milts: Preparation, characterization, molecular docking study and antioxidant function on H2O2-damaged HUVECs. Front. Nutr. doi: 10.3389/fnut.2022.957778
Tonolo F., Folda A., Cesaro L., Scalcon V., Marin O., Ferro S., et al. (2020). Milk-derived bioactive peptides exhibit antioxidant activity through the Keap1-Nrf2 signaling pathway. J. Funct. Foods. 64, 103696. doi: 10.1016/j.jff.2019.103696
Tsai B. C., Kuo W. W., Day C. H., Hsieh D. J. Y., Kuo C. H., Daddam J., et al. (2020). The soybean bioactive peptide VHVV alleviates hypertension-induced renal damage in hypertensive rats via the SIRT1-PGC1α/Nrf2 pathway. J. Funct. Foods. 75, 104255. doi: 10.1016/j.jff.2020.104255
Vásquez P., Zapata J. E., Chamorro V. C., Fillería S. F. G., Tironi V. A. (2022). Antioxidant and angiotensin I-converting enzyme (ACE) inhibitory peptides of rainbow trout (Oncorhynchus mykiss) viscera hydrolysates subjected to simulated gastrointestinal digestion and intestinal absorption. LWT 154, 112834. doi: 10.1016/j.lwt.2021.112834
Vieira E. F., das Neves J., Vitorino R., Dias da Silva D., Carmo H., Ferreira I. M. (2016). Impact of in vitro gastrointestinal digestion and transepithelial transport on antioxidant and ACE-inhibitory activities of brewer's spent yeast autolysate. J. Agric. Food Chem. 64 (39), 7335–7341. doi: 10.1021/acs.jafc.6b02719
Wang X., Chen H., Fu X., Li S., Wei J. (2017). A novel antioxidant and ACE inhibitory peptide from rice bran protein: Biochemical characterization and molecular docking study. LWT 75, 93–99. doi: 10.1016/j.lwt.2016.08.047
Wang B., Li L., Chi C. F., Ma J. H., Luo H. Y., Xu Y. F. (2013a). Purification and characterisation of a novel antioxidant peptide derived from blue mussel (Mytilus edulis) protein hydrolysate. Food Chem. 138 (2-3), 1713–9171. doi: 10.1016/j.foodchem.2012.12.002
Wang K., Luo Q., Hong H., Liu H., Luo Y. (2021a). Novel antioxidant and ACE inhibitory peptide identified from arthrospira platensis protein and stability against thermal/pH treatments and simulated gastrointestinal digestion. Food Res. Int. 139, 109908. doi: 10.1016/j.foodres.2020.109908
Wang Y. M., Pan X., He Y., Chi C. F., Wang B. (2020). Hypolipidemic activities of two pentapeptides (VIAPW and IRWWW) from miiuy croaker (Miichthys miiuy) muscle on lipid accumulation in HepG2 cells through regulation of AMPK pathway. Appl. Sci. 10 (3), 817. doi: 10.3390/app10030817
Wang C. X., Song C. C., Liu X. T., Qiao B. W., Song S., Fu Y. H. (2022b). ACE inhibitory activities of two peptides derived from volutharpa ampullacea perryi hydrolysate and their protective effects on H2O2 induced HUVECs injury. Food Res. Int. 157, 111402. doi: 10.1016/j.foodres.2022.111402
Wang B., Wang Y. M., Chi C. F., Luo H. Y., Deng S. G., Ma J. Y. (2013b). Isolation and characterization of collagen and antioxidant collagen peptides from scales of croceine croaker (Pseudosciaena crocea). Mar. Drugs 11, 4641–4661. doi: 10.3390/md11114641
Wang J., Wang Y. M., Li L. Y., Chi C. F., Wang B. (2022a). Twelve antioxidant peptides from protein hydrolysate of skipjack tuna (Katsuwonus pelamis) roe prepared by flavourzyme: Purification, sequence identification, and activity evaluation. Front. Nutr. 8, 813780. doi: 10.3389/fnut.2021.813780
Wang Y. Z., Wang Y. M., Pan X., Chi C. F., Wang B. (2021b). Antioxidant mechanisms of the oligopeptides (FWKVV and FMPLH) from muscle hydrolysate of miiuy croaker against oxidative damage of HUVECs. Oxid. Med. Cell. Longev. 2021, 9987844. doi: 10.1155/2021/9987844
Xu Z., Wu C., Sun-Waterhouse D., Zhao T., Waterhouse G. I. N., Zhao M., et al. (2021). Identification of post-digestion angiotensin-I converting enzyme (ACE) inhibitory peptides from soybean protein isolate: Their production conditions and in silico molecular docking with ACE. Food Chem. 345, 128855. doi: 10.1016/j.foodchem.2020.128855
Yang G., Qin S., Li W. (2021). Purification and characterization of a novel angiotensin I-converting enzyme-inhibitory peptide derived from Alaska pollack skins. J. Food Sci. 86 (6), 2457–2467. doi: 10.1111/1750-3841.15754
Yang X. R., Zhang L., Zhao Y. Q., Chi C. F., Wang B. (2019b). Purification and characterization of antioxidant peptides derived from protein hydrolysate of the marine bivalve mollusk tergillarca granosa. Mar. Drugs 17 (5), 251. doi: 10.3390/md17050251
Yang X. R., Zhao Y. Q., Qiu Y. T., Chi C. F., Wang B. (2019a). Preparation and characterization of gelatin and antioxidant peptides from gelatin hydrolysate of skipjack tuna (Katsuwonus pelamis) bone stimulated by in vitro gastrointestinal digestion. Mar. Drugs 17 (2), 78. doi: 10.3390/md17020078
Yea C. S., Ebrahimpour A., Hamid A. A., Bakar J., Muhammad K., Saari N. (2014). Winged bean [Psophorcarpus tetragonolobus (L.) DC] seeds as an underutilised plant source of bifunctional proteolysate and biopeptides. Food Funct. 5 (5), 1007–1016. doi: 10.1030/c3fo60667h
Yu F., Zhang Z., Luo L., Zhu J., Huang F., Yang Z., et al. (2018). Identification and molecular docking study of a novel angiotensin-I converting enzyme inhibitory peptide derived from enzymatic hydrolysates of cyclina sinensis. Mar. Drugs 16, 411. doi: 10.3390/md16110411
Zarei M., Abidin N. B. Z., Auwal S. M., Chay S. Y., Haiyee Z. A., Sikin A. M., et al. (2019). Angiotensin converting enzyme (ACE)-peptide interactions: Inhibition kinetics, in silico molecular docking and stability study of three novel peptides generated from palm kernel cake proteins. Biomolecules 9 (10), 569. doi: 10.3390/biom9100569
Zhang Y., Pan D., Yang Z., Gao X., Dang Y. (2021). Angiotensin I-converting enzyme (ACE) inhibitory and dipeptidyl peptidase-4 (DPP-IV) inhibitory activity of umami peptides from ruditapes philippinarum. LWT 144, 111265. doi: 10.1016/j.ltw.2021.111265
Zhang L., Zhao G. X., Zhao Y. Q., Qiu Y. T., Chi C. F., Wang B. (2019). Identification and active evaluation of antioxidant peptides from protein hydrolysates of skipjack tuna (Katsuwonus pelamis) head. Antioxid. (Basel). 8 (8), 318. doi: 10.3390/antiox8080318
Zhao W. H., Chi C. F., Zhao Y. Q., Wang B. (2018a). Preparation, physicochemical and antioxidant properties of acid- and pepsin-soluble collagens from the swim bladders of miiuy croaker (Miichthys miiuy). Mar. Drugs 16 (5), 161. doi: 10.3390/md16050161
Zhao W. H., Luo Q. B., Pan X., Chi C. F., Sun K. L., Wang B. (2018b). Preparation, identification, and activity evaluation of ten antioxidant peptides from protein hydrolysate of swim bladders of miiuy croaker (Miichthys miiuy). J. Funct. Foods. 47, 503–511. doi: 10.1016/j.jff.2018.06.014
Zhao G. X., Yang X. R., Wang Y. M., Zhao Y. Q., Chi C. F., Wang B. (2019a). Antioxidant peptides from the protein hydrolysate of Spanish mackerel (Scomberomorous niphonius) muscle by in vitro gastrointestinal digestion and their in vitro activities. Mar. Drugs 17 (9), 531. doi: 10.3390/md17090531
Zhao Y. Q., Zhang L., Tao J., Chi C. F., Wang B. (2019b). Eight antihypertensive peptides from the protein hydrolysate of Antarctic krill (Euphausia superba): Isolation, identification, and activity evaluation on human umbilical vein endothelial cells (HUVECs). Food Res. Int. 121, 197–204. doi: 10.1016/j.foodres.2019.03.035
Zheng Y., Li Y., Zhang Y., Ruan X., Zhang R. (2017). Purification, characterization, synthesis, in vitro ACE inhibition and in vivo antihypertensive activity of bioactive peptides derived from oil palm kernel glutelin-2 hydrolysates. J. Funct. Foods. 28, 48–58. doi: 10.1016/j.jff.2016.11.021
Keywords: miiuy croaker (Miichthys miiuy), swim bladder, angiotensin I converting enzyme, peptide, molecular docking experiment, cytoprotection
Citation: Zhu W-Y, Wang Y-M, Suo S-K, Zheng S-L and Wang B (2022) Isolation, identification, molecular docking analysis, and cytoprotection of seven novel angiotensin I-converting enzyme inhibitory peptides from miiuy croaker byproducts-swim bladders. Front. Mar. Sci. 9:977234. doi: 10.3389/fmars.2022.977234
Received: 24 June 2022; Accepted: 07 July 2022;
Published: 26 July 2022.
Edited by:
Haibin Tong, Wenzhou University, ChinaReviewed by:
Zhongshan Zhang, Huzhou University, ChinaCopyright © 2022 Zhu, Wang, Suo, Zheng and Wang. This is an open-access article distributed under the terms of the Creative Commons Attribution License (CC BY). The use, distribution or reproduction in other forums is permitted, provided the original author(s) and the copyright owner(s) are credited and that the original publication in this journal is cited, in accordance with accepted academic practice. No use, distribution or reproduction is permitted which does not comply with these terms.
*Correspondence: Bin Wang, d2FuZ2JpbjQxNTlAaG90bWFpbC5jb20=; Wang-Yu Zhu, emh1d2FuZ3l1MjRAc2luYS5jbg==
†These authors have contributed equally to this work
Disclaimer: All claims expressed in this article are solely those of the authors and do not necessarily represent those of their affiliated organizations, or those of the publisher, the editors and the reviewers. Any product that may be evaluated in this article or claim that may be made by its manufacturer is not guaranteed or endorsed by the publisher.
Research integrity at Frontiers
Learn more about the work of our research integrity team to safeguard the quality of each article we publish.