- 1College of Ocean and Meteorology, Guangdong Ocean University, Zhanjiang, China
- 2Laboratory for Coastal Ocean Variation and Disaster Prediction, Guangdong Ocean University, Zhanjiang, China
- 3Guangdong AIB Polytechnic College, Guangzhou, China
- 4Key Laboratory of Climate, Resources and Environment in Continental Shelf Sea and Deep Sea of Department of Education of Guangdong Province, Guangdong Ocean University, Zhanjiang, China
Rapid urbanization and heavy industrialization generally result in serious aerosol pollution. Contrary to this conventional wisdom, Zhanjiang, one industrial city in the southernmost point of the Chinese mainland, is not accompanied by aerosol pollution and its air quality index always ranks high compared to other cities in China. To investigate this contradiction, 72-hour total suspended particles (TSPs) and water-soluble inorganic ions (WSIIs; including Mg2+, Ca2+, K+, Na+, NH4+, Cl-, NO3-, and SO42-) were collected in Zhanjiang, China, from November 2018 to November 2019. The relative humidity (RH) was higher than 80% throughout the whole year in Zhanjiang. However, the TSPs and WSIIs were not correlated with RH, indicating that RH can increase the particle size, but this had a minor impact on the dry deposition rate. The larger particles induced by RH were easily captured by wet precipitation, leading to a seasonal pattern with higher TSP and WSII mass concentrations during the dry and cool season and lower values during the hot and rainy season. This seasonal pattern and high aerosol acidity indicate that TSPs, WSIIs, and acidic gaseous precursors from the local sources were preferentially scavenged by the abundant rainfall and high precipitation frequency. Principal component analysis (PCA) results suggest that relatively clean marine emissions and secondary aerosols were the most important sources of TSPs and WSIIs. Our results indicate that the inconsistency between the heavy industrial activities and excellent air quality in Zhanjiang may be related to the high precipitation frequency (63%) and the marine dilution effect (27%).
Introduction
One consequence of the rapid urbanization and industrialization in developing countries is the increase in the total suspended particles (TSPs) in the atmosphere, i.e., air quality deterioration (Seinfeld, 2004; Huang et al., 2014). TSPs have long been regarded as a global air pollution concern due to their significant impacts on climate change, atmospheric chemistry, air quality, acidification, and human health (Fuzzi et al., 2015; Xiao et al., 2017). The TSPs (also called atmospheric aerosols) are primarily composed of various chemical species and water-soluble inorganic ions (WSIIs) (Ali-Mohamed, 1991). Therefore, observations of WSIIs may provide valuable information for controlling aerosol pollution.
Secondary inorganic aerosols, such as and particles, are important contributors to the WSIIs in the TSPs (Guo et al., 2014). The and particles in the atmosphere are mainly generated from oxidation of their representative gaseous precursors (SO2 and NOx) emitted from ambient fossil fuel combustion (Shon et al., 2012; Xu L. L. et al., 2017; Liu et al., 2019). These ions and gaseous precursors are largely linked to aerosol pollution and acidification (Cao et al., 2013; Weber et al., 2016; Liu et al., 2019; Tian et al., 2021). Previous studies have reported significant increases in the aerosol mass, which pose a potential threat to human health due to high fossil fuel combustion (Zhang et al., 2011). Additionally, Xiao and Liu (2004) found that air quality deterioration in Guiyang was caused by industrial activities, and some industries should be relocated to mitigate local haze pollution.
Zhanjiang city (20.00–21.58°N, 109.52–110.92°E), the site of our study, is located in Guangdong Province, southern China, and is a tropical coastal city close to the South China Sea (SCS,< 30 km). During the past decades, a variety of industries (especially heavy industries, i.e., petrochemical factory as well as iron and steel plant) have moved to and sprung up in Zhanjiang. Nowadays, Zhanjiang is a famous manufacturing and heavy industry base. According to the Guangdong Statistical Yearbook, the total output value of heavy industries above designated size in Zhanjiang accounts for ~50% of Zhanjiang’s gross regional domestic product. Additionally, the Basf Zhanjiang Integrated Base, the Lianjiang Nuclear Power Plant, and the Jingxin Zhanjiang Donghai Thermal Power Plant are under construction. Marine pollution caused by the industrialization and urbanization via terrestrial input in this region has been recently reported (Sun et al., 2018; Peng et al., 2020; Li J. C. et al., 2020). However, the influence of industrialization and urbanization on ambient air quality in Zhanjiang are not reported and still needs to be clarified, as TSPs and WSIIs in the atmosphere can be captured by rainfall and then enter into the marine systems. This may pose a potential risk to marine systems, for example, eutrophication (Cui et al., 2020; Wang J. J. et al., 2020).
Although the sources and impact factors of the WSIIs in the TSPs have been studied extensively in order to lessen aerosol pollution (Xiao and Liu, 2004; Kundu et al., 2010; Zhang et al., 2011; Xiao et al., 2017; Wang Q. Q. et al., 2020), limited research has been conducted on the impact of precipitation on WSIIs, which is considered to be an important process for removing TSPs and soluble gases in the ambient atmosphere (Hou et al., 2018). Thus, we hypothesize that the shorter aerosol lifetime and shortage of gaseous precursors in the atmosphere caused by precipitation may contribute to reducing aerosol pollution, especially in tropical regions with frequent precipitation and plentiful rainfall.
To investigate this contradiction and to verify this speculation, we monitored the TSPs in the atmosphere for one year and analyzed the WSIIs in the TSPs in the tropical city of Zhanjiang, China. The objectives of this study are as follows: (1) to determine the TSP and WSII concentrations and seasonal variations; (2) to verify the aerosol removal effect of precipitation; and (3) to use principal component analysis (PCA) to identify the possible sources of the WSIIs.
Methods and materials
Sampling site and sample collection
Zhanjiang in the southernmost point of the Chinese mainland (Figure 1) has a typical tropical monsoon climate, with a cool dry season from October to March (dry season) and a hot wet season from April to September (rainy season; Chen et al., 2019). The average annual temperature and rainfall in the city is 23°C and 1689 mm, respectively (Chen et al., 2019). The sampling campaign was conducted from 1 November 2018 to 1 November 2019 at 1.5 m above the roof of a building in Guangdong Ocean University in rural Zhanjiang City (21.15°N, 110.30°E). The TSPs in the atmosphere were collected on a 450°C pre-baked quartz fiber filter (Type MK360, 203 x 254 mm, Munktell Filter, Sweden) using a high-volume air sampler (1.37 ± 0.25 m3/min; KB-1000-180815, Xiamen, China), and the cumulative sampling time for each aerosol sample was about 72 hours. After sampling, the filter was stored at -20°C to prevent volatilization. A total of 116 aerosol samples were collected in this study.
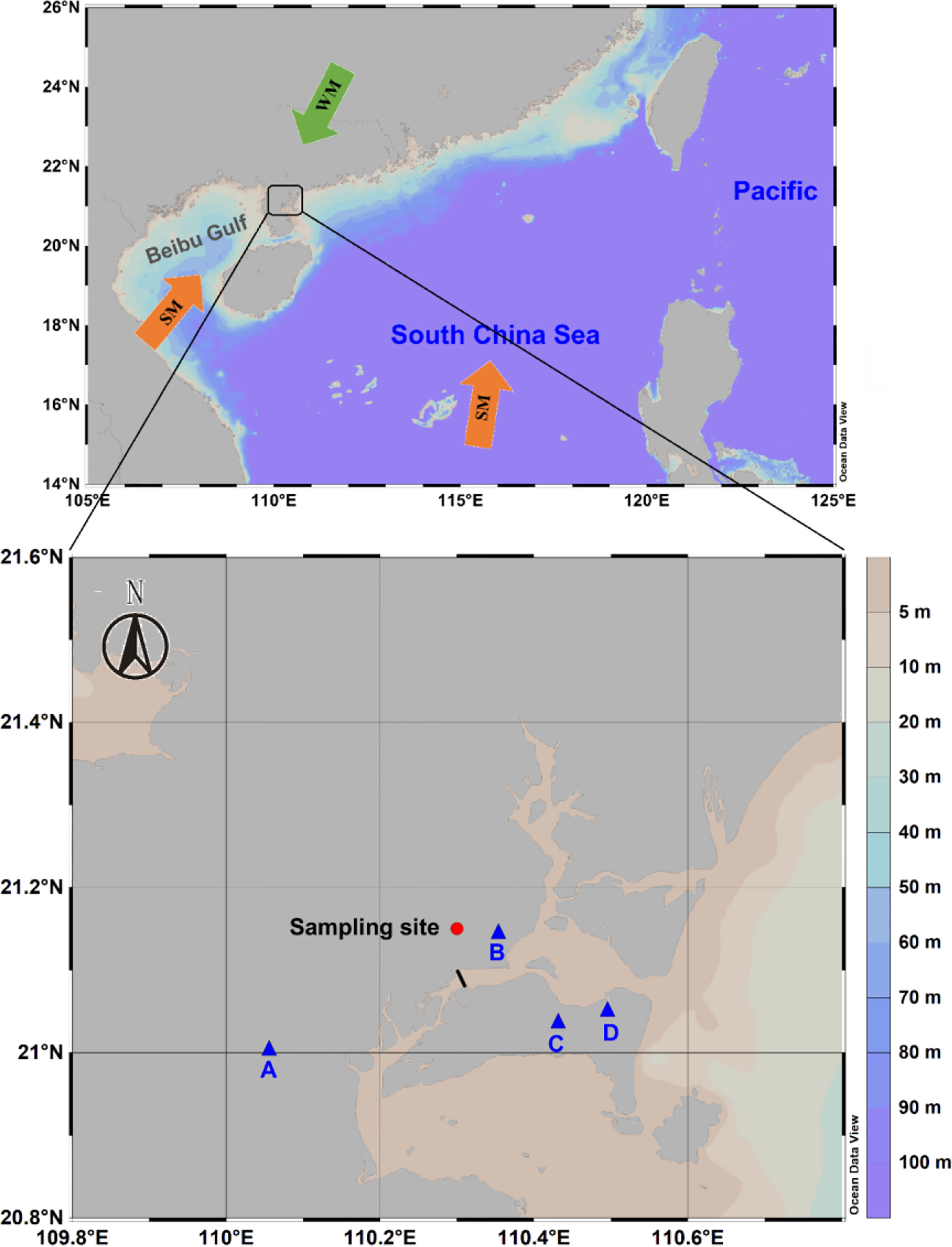
Figure 1 The sampling location of Zhanjiang (red dot). WM, winter monsoon; SM, summer monsoon. The blue triangles are the location of heavy industry. A, B, C, and D denote Chenming Paper Mill, Sinopec Zhanjiang Dongxing Petrochemcial Factory, Zhongke (Guangdong) Refinery & Petrochemical Factory, and Baosteel Zhanjiang Iron & Steel Plant, respectively.
pH measurements and analysis of the major WSIIs in the TSPs
The pH values of the samples were measured using a desktop pH meter (Orion STAR A211, Thermo Fisher Scientific, USA) immediately after obtaining the extracted aqueous solution. The remaining aqueous extracts were stored at -20°C until the water-soluble inorganic ions were analyzed.
Five cations (Mg2+, Ca2+, K+, Na+, and ) and three anions (Cl-, , and ) in the aqueous extracts of the aerosol samples were analyzed in this study. and were determined using standard colorimetric methods (Grasshoff et al., 1999), and their detection limits were< 0.1 mg/L. The other ions were analyzed using ion chromatography (Dionex Aquion, Thermo Fisher Scientific), and their uncertainties were< 5%.
Meteorological data
Daily meteorological parameters, i.e., air temperature (°C), wind speed (km/h), and relative humidity (RH, %), were obtained from the local weather station (< 15 km southwest of the sampling site) during the sampling period. The precipitation was documented after each rainfall event. Detailed information on the rainfall sampling protocols can be found in our previously published papers (Chen et al., 2019; Chen et al., 2020). The daily air pollutants, such as SO2, NO2, PM2.5, and PM10, presented during the sampling period in Zhanjiang were downloaded from https://www.zq12369.com/. A 72-hour average of the data described above (except for precipitation) was calculated and is presented in Figure 4.
Results
As shown in Figure 2, the mass concentration of the TSPs in the atmosphere ranged from 18.1 to 133.6 μg/m3, with an average and standard deviation of 56.5 ± 24.3 μg/m3. The average value of the TSPs falls within the recommended range of the international guidelines (150 to 230 μg/m3). There were significantly seasonal variations in the mass concentrations of the TSPs (Figure 2 and Table 1). The mass concentration of the TSPs in the dry season (with an average value of 68.5 ± 23.0 μg/m3; Table 1) were significantly higher than those in the rainy season (with an average value of 44.8 ± 19.2 μg/m3; Table 1; ANOVA, p< 0.01).
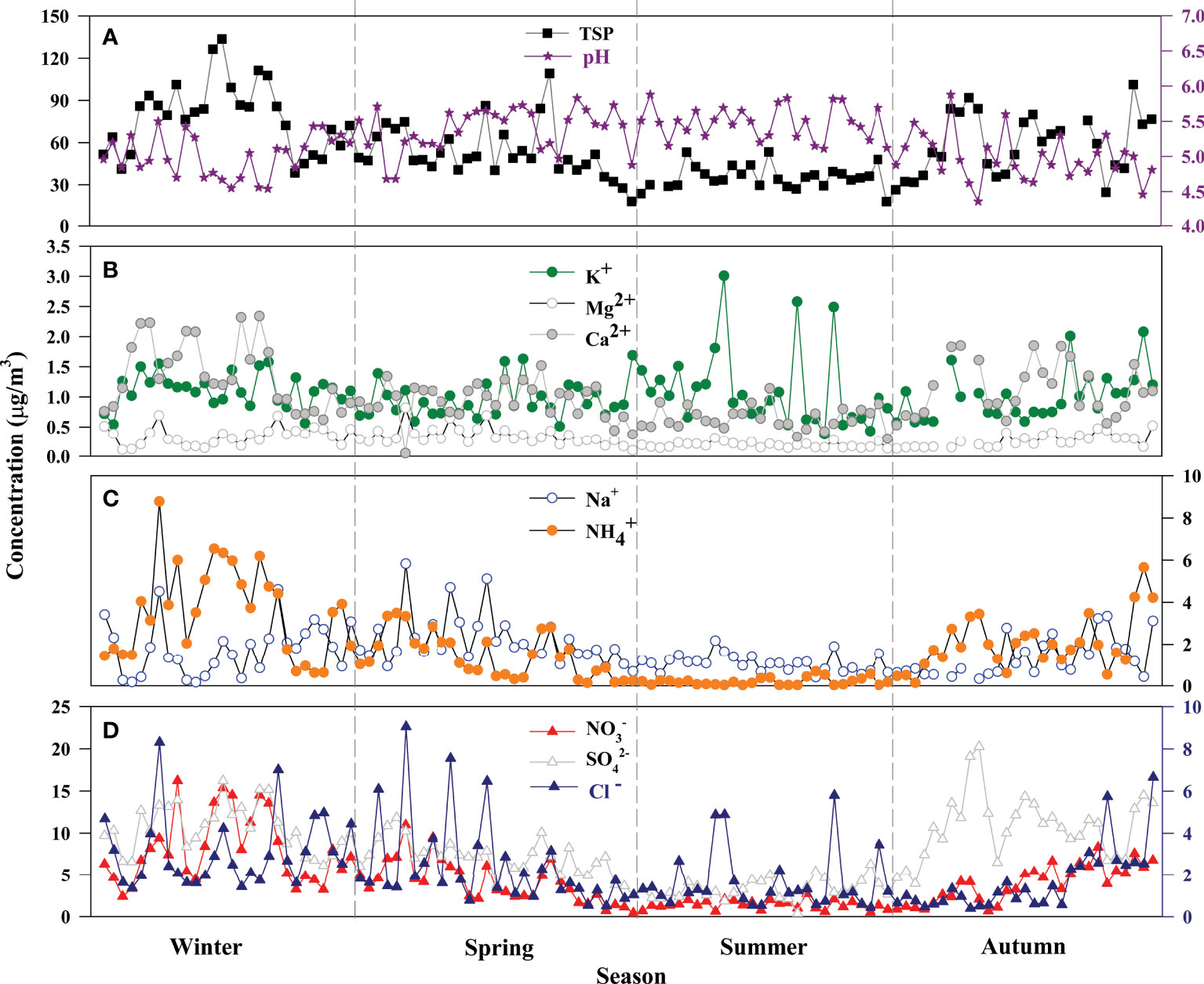
Figure 2 Seasonal variations in the pH, and mass concentrations of TSPs and WSIIs (i.e., K+, Mg2+, Ca2+, Na+, NH4+, NO3-, SO42- and Cl-) in the atmospheric aerosols in Zhanjiang.
The contents of the WSIIs in the TSPs increased in the order of . was the most abundant anion in the TSPs, followed by and Cl-, which accounted for 56.2%, 27.2%, and 16.6%, respectively, of the total anions. The predominant cations were Na+, , and K+, accounting for 28.8%, 26.8%, and 20.4%, respectively, of the total cations. Similar seasonal variation pattern could be observed in the WSIIs. Significantly higher Mg2+, Ca2+, , , and values occurred in the dry season, whereas lower values were observed in the rainy season (Figure 2D; Table 1; ANOVA, p< 0.01 for these ions). The mass concentrations of K+ were stable throughout the sampling campaign (Figure 2B and Table 1; ANOVA, p > 0.05).
Discussion
Influences of meteorological factors
The average mass concentration of the TSPs in the rainy season (with an average value of 44.8 ± 19.2 μg/m3) was 34.6% lower than that in the dry season (with an average of 44.8 ± 23.0 μg/m3) (ANOVA, p< 0.01). The backward air mass trajectories during the same sampling periods in our published study (Luo et al., 2022) showed that the air masses during the dry season could bring land-based aerosol to the Zhanjiang and the air parcels during the rainy season were mainly originated from the South China Sea. This suggested that the seasonal variation of TSPs concentrations in Zhanjiang were related to the air masses transition. However, it is hypothesized that this influence might be minor than local influences as discussed in the following sections. In addition, during the rainy season, the TSPs concentrations in the present study were lower than the value in the South China Sea (remote area, 60.4 ± 27.0 μg/m3; Xiao et al., 2017), Guangzhou (urban area, 62.1 μg/m3; Tan et al., 2009), and Guiyang (polluted area, 85.4 ± 29.1 μg/m3; Li Q. et al., 2020). During the dry season, the TSPs concentrations in the present study were significantly lower than the concentration observed in the South China Sea (remote area, 114.7 ± 82.1 μg/m3; Xiao et al., 2017), Shanghai (urban area, 94.64 μg/m3; Gao et al., 2011), Xi’an (urban area, 130 μg/m3; Gao et al., 2011), and Guiyang (polluted area, 140.2 ± 46.2 μg/m3; Li Q. et al., 2020), and were similar to Guangzhou (urban area, 66.7 μg/m3; Tan et al., 2009). These results implied that the seasonal variation of TSPs concentrations were greatly influenced by local conditions, for example, meteorological conditions (i.e., precipitation, temperature, wind speed, and RH) and local emissions (Gao et al., 2011; Meng et al., 2016; Xiao et al., 2017). Recent studies also found that local emission and meteorological factor have an important impact on air pollution (Wang et al., 2021; Zhou et al., 2022).
The meteorological conditions can affect chemical conversion and the deposition of TSPs (Meng et al., 2016) and thus affecting the mass fraction of WSIIs in TSPs. During the sampling periods, the mass fraction of WSIIs accounted for 35.7% of TSPs, displaying a higher fraction in the dry season (with an average value of 41.4%) and lower fraction in the rainy season (with an average value of 30.0%). This suggested that the seasonal variation of WSIIs was related to TSPs concentrations. The mass fraction of WSIIs in TSPs in the present study was higher than the value in the South China Sea (24.8%; Xiao et al., 2017), and were comparable to the value in urban Guangzhou (35%; Tao et al., 2017) and in suburban Zhuhai (34%; Tao et al., 2017). The significantly positive relationships between WSIIs and TSPs (p< 0.01 for , Ca2+, Cl-, , and ; p< 0.05 for Mg2+; p > 0.01 for K+ and Na+; Table 2) also supported the concept that WSIIs were important constituents of TSPs. Therefore, WSIIs might play a significant role in TSPs in the present study.
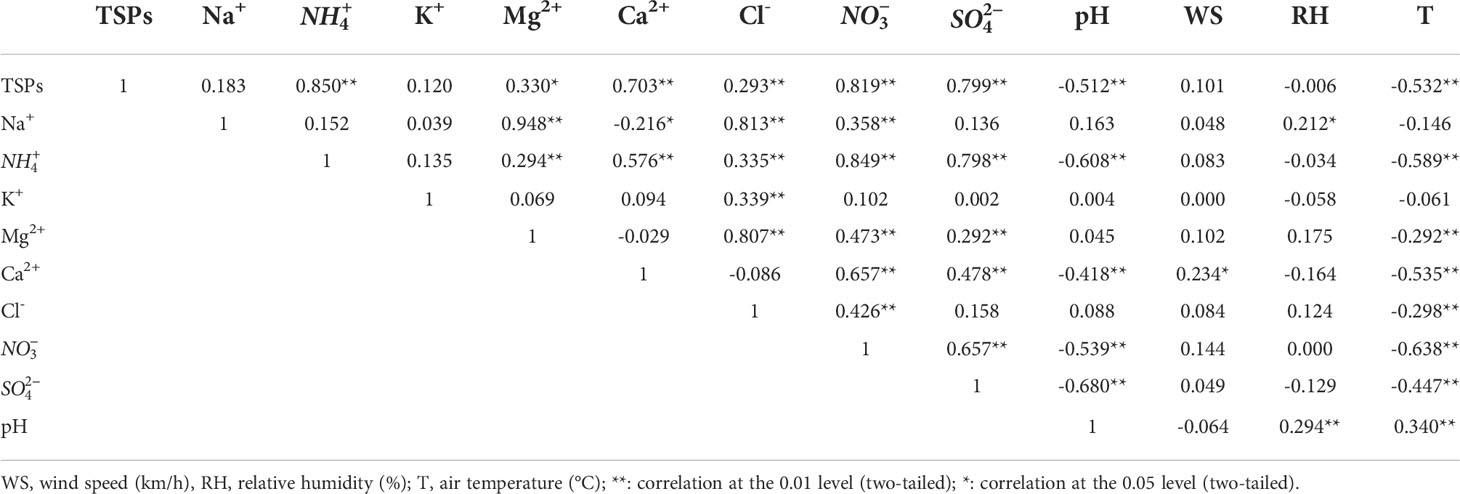
Table 2 Correlation coefficients between pH, the WSIIs in the atmospheric aerosols, and the meteorological parameters in Zhanjiang.
During the sampling periods, the WSIIs concentrations were also higher during the dry season and were lower during the rainy season, with an average reduction rate of 42.3% (Table 1). This result is consistent with the seasonal pattern in Guiyang, that is, decreases in the TSPs (decrease of 18%) and WSIIs concentrations during the rainy season (Xiao and Liu, 2004). However, in contrast to the findings for Guiyang, the percentages of , Cl-, , and in the total WSIIs were lower in the rainy season than those in the dry season, indicating that in the particles, these ions tend to be coarser, so they have a shorter residence time than the finer components. Thus, they were readily captured by precipitation and were preferentially scavenged below the clouds (Xiao and Liu, 2004). Notably, in the precipitation characteristics shown in Figure 3, the precipitation frequency (precipitation frequency was calculated from the fraction of rain events in each month during sampling periods; rainfall > 0.5 mm/day as the criterion; Hou et al., 2018; data sourced from https://en.tutiempo.net/world.html) and the amount of rainfall were significantly higher in the rainy season (especially in summer) and lower in the dry season, which further suggests a removal process driven by frequent and large amounts of precipitation (Hou et al., 2018).
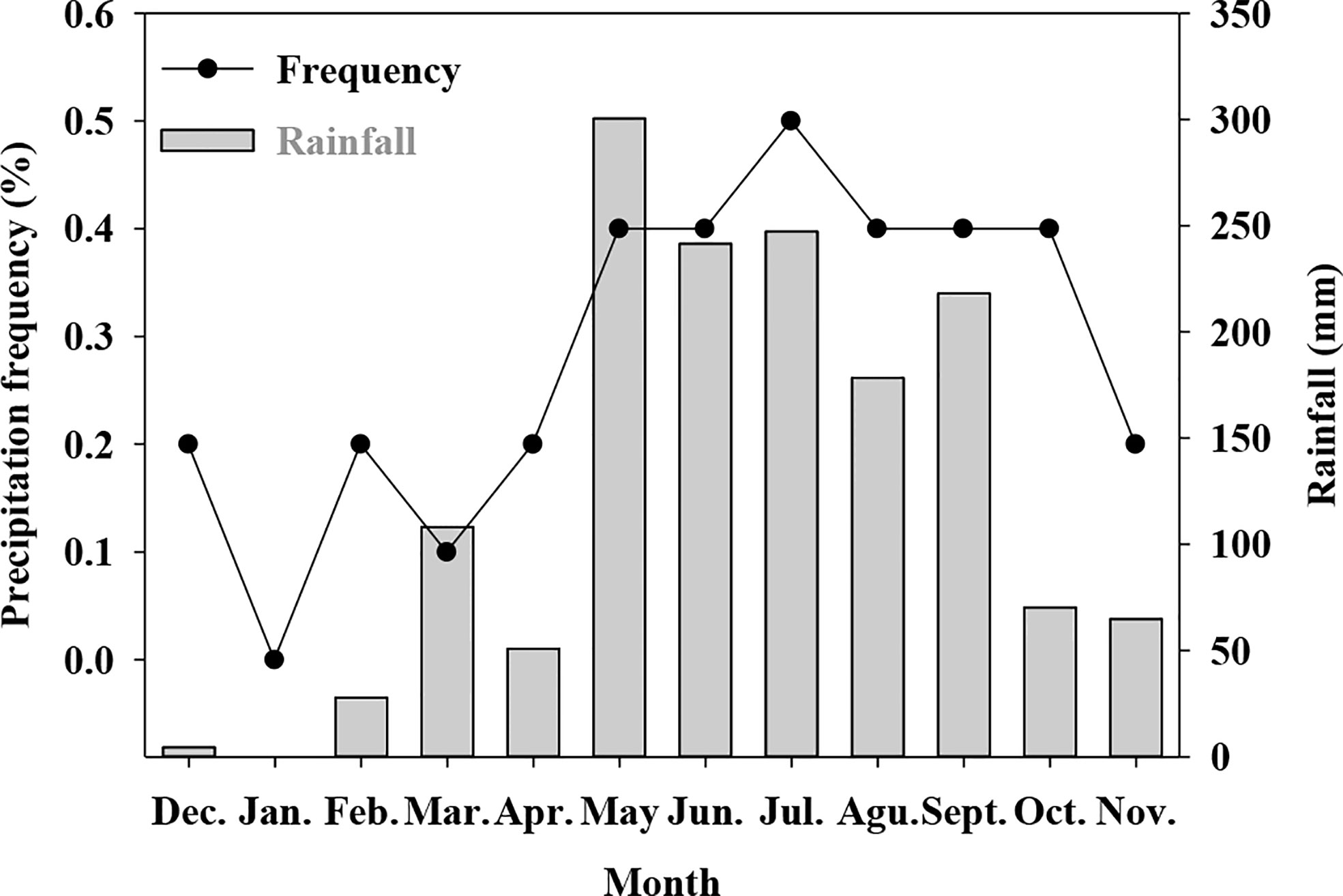
Figure 3 Monthly precipitation frequency and total rainfall amount in Zhanjiang. Precipitation frequency data was calculated from the fraction of rain events in each month during sampling periods (rainfall > 0.5 mm/day as the criterion; Hou et al., 2018; data sourced from https://en.tutiempo.net/world.html).
Temperature, wind speed, and RH also have impacts on the seasonal variations in the TSPs and WSIIs in atmospheric aerosols (Xiao and Liu, 2004; Xiao et al., 2017). In this study, the TSPs and WSIIs were strongly negatively correlated with the air temperature (p< 0.01 for TSPs, , Mg2+, Ca2+, Cl-, , and ; p > 0.05 for K+ and Na+; Table 2), suggesting that the hot and rainy season helps reduce the amount of these particles in the atmosphere, leading to a decrease in the TSPs and WSIIs concentrations (Figure 2 and Table 1). Only the mass concentration of Ca2+ in the TSPs was affected by wind speed (Table 2), and the resultant positive correlation (Table 2, p< 0.05) suggests that strong winds can bring dust containing Ca2+.
The high RH throughout the year (>80%) in Zhanjiang (Figure 4C) suggested that the particles in the atmospheric aerosols were wet and easily interacted with each other (Xiao and Liu, 2004), leading to an increase in particle size and the dry deposition rate (Tang et al., 2006). If the dry deposition rate increased during the sampling periods, a systematic decrease in the TSPs and WSIIs with increasing RH should be observed, and good correlations should be found among them. By contrast, the TSPs and WSIIs (with the exception of Na+) were not correlated with RH in Zhanjiang (Table 2), indicating that the increase in particle size caused by the RH had little impact on the dry deposition rate.
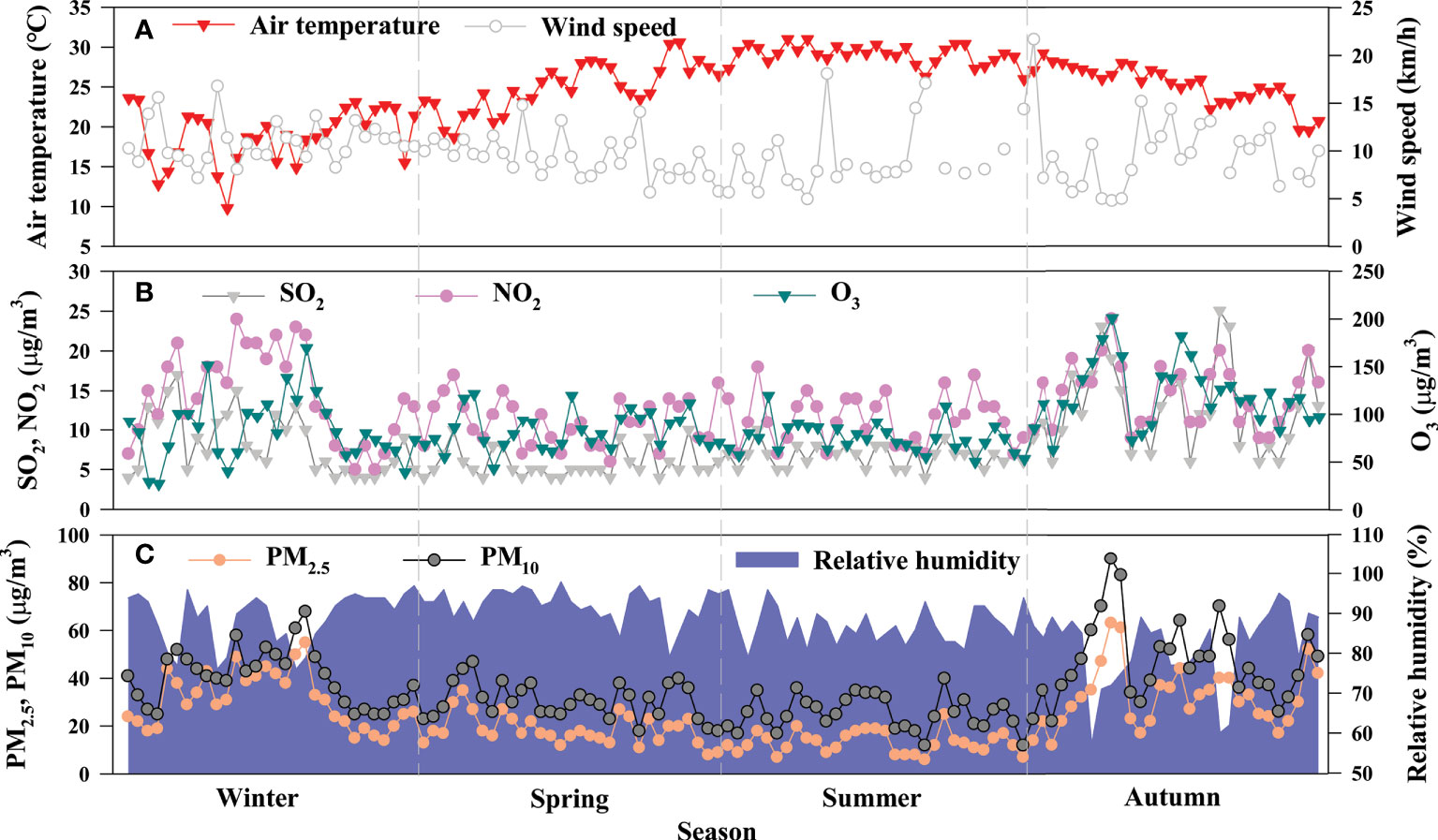
Figure 4 72-hour average values of air temperature, wind speed, SO2, NO2, O3, PM2.5, PM10, and relative humidity in Zhanjiang.
Indicators of aerosol acidity
In addition to the TSP and WSII concentrations, aerosol acidity is also a concerning environmental problem. Thus, the ion balance and neutralization factors were calculated to investigate the aerosol acidity (Xu J. S. et al., 2017; Agarwal et al., 2020). In order to evaluate the acid–base balance of the ions in the atmospheric aerosols, ion balance calculations, i.e., the ratio of the total anion equivalents to the total cations equivalents (ΣAE/ΣCE), have generally been conducted in previous studies (Zhang et al., 2011; Xu J. S. et al., 2017; Agarwal et al., 2020). The charge balance between the anions and cations was calculated as follows:
Here, , , [Cl-], [Na+], , [K+], [Mg2+], and [Ca2+] refer to the mass concentrations of the ions (μg/m3).
The plot of ΣAE vs ΣCE is presented in Figure 5A. The slope of the linear regression for all of the TSP samples (R2 = 0.87) was slightly higher than 1, indicating a cation deficient scenario, which may be the result of the omission of hydrogen ions from the calculations (Zhang et al., 2011). Furthermore, the average ratio of ΣAE/ΣCE in the four seasons followed the order autumn (1.34) > winter (1.12) > summer (1.03) > spring (1.02), which is in good agreement with the seasonal pattern of the pH values (Figure 2A). Therefore, these results demonstrate that the aerosols are more acidic in autumn and winter and are less acidic in spring and summer in Zhanjiang. This seasonal variation is consistent with the aerosol research conducted in Guangzhou (GZ) and Hong Kong (HK), where the samples were found to have lower pH values (pH = 4.33 and 5.21 in GZ and HK, respectively) in winter and higher pH values (pH = 5.21 and 5.72 in GZ and HK, respectively) in summer (Cao et al., 2013).
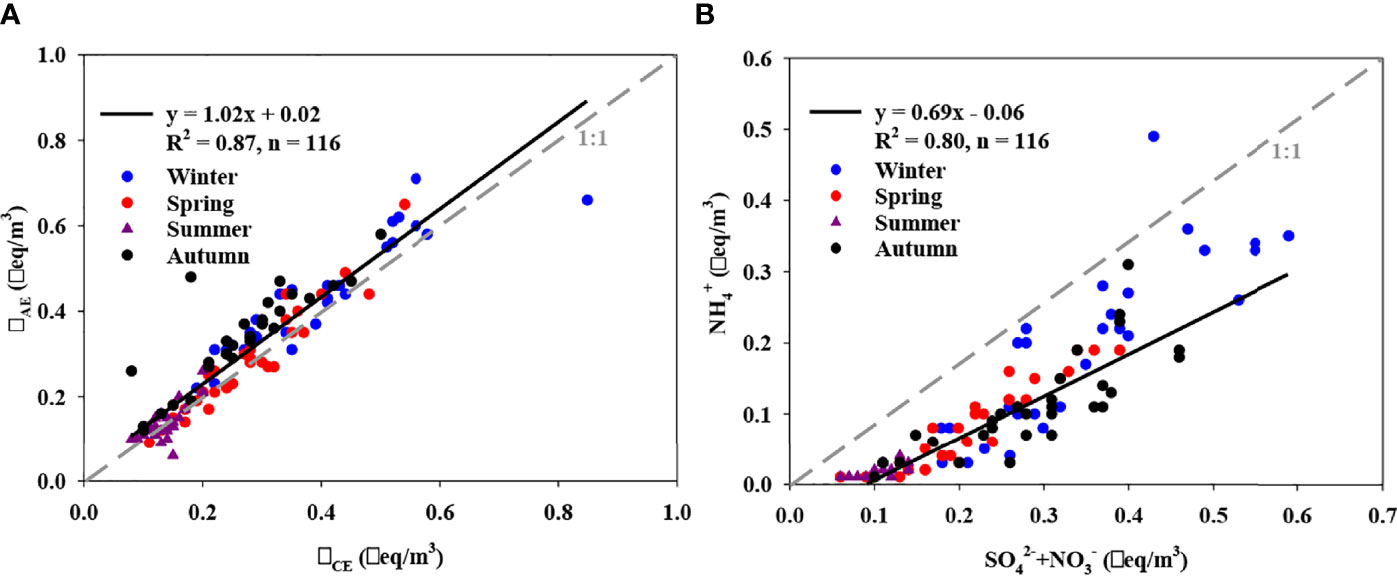
Figure 5 (A) Ion balance of the TSPs in Zhanjiang. (B) Relationship between the equivalent NH4+ concentration and the sum of the equivalent SO42- + NO3- concentrations in the TSPs in Zhanjiang.
The ratio of the equivalent concentration to the sum of the equivalent and concentrations (i.e., ) in the TSPs can be used to assess the neutralization reaction (secondary aerosol process) among , , and (Xu J. S. et al., 2017). In this study, the equivalent ratios of of the samples were less than 1:1 (Figure 5B), which indicates an deficiency in the ambient particles (Zhou et al., 2018). However, the measurements in this region are comparable to those on Jeju Island (average 1.7 ± 1.1 μg/m3; Kundu et al., 2010) and in Guiyang (average 3.81 ± 1.64 μg/m3; Xiao and Liu, 2004), are higher than those in Yurihonjo (rural site in Japan, average 0.38 ± 0.33 μg/m3; Kawashima and Kurahashi, 2011) and inadequate ambient (Jickells et al., 2003; Lin et al., 2016). This demonstrates that the in the atmosphere in Zhanjiang is adequate. One possible reason for this discrepancy is that the equivalent concentrations of the acidifying anions were considerably more abundant. That is, and were too abundant for the in the atmospheric aerosols to be neutralized, and thus the acid status of the atmospheric aerosols can be ascribed to an excess of acidifying anions (Cao et al., 2020). Thus, the higher acidity in autumn and winter could be related to the higher concentrations of and (Figure 2D).
Cations (, nss-K+, nss-Mg2+, and nss-Ca2+) have individual neutralization capacities, and plays a major role in decreasing aerosol acidity (Xu J. S. et al., 2017; Agarwal et al., 2020). Based on the similar mass concentrations of the cations in this study (Figure 2A–C), we hypothesize that there were other major neutralization ions in addition to . Thus, the neutralization factors (NFs) were investigated to determine the dominant neutralization cations. The contributions of Na+ and Cl- to lessening the aerosol acidity are considered to be negligible since they are mainly sourced from sea salts (Safai et al., 2010; Satsangi et al., 2013). Although a good correlation was observed between Na+ and Cl- (p< 0.01; Table 2), the corresponding ratios of [Na+]/[Cl-] were found to be lower than that of seawater (1.1) (Xu et al., 2014), suggesting that coal combustion was also a source of Cl- in addition to sea salt (Xue et al., 2016; Jain et al., 2017; Xu J. S.et al., 2017). Therefore, the contribution of Cl- to the neutralization should be taken into account, and the non-sea salt Cl- ([nss-Cl-]) can be calculated by subtracting the sea salt Cl- ([ss-Cl-] = [Na+]/1.1). NFs in Zhanjiang were calculated using the following equations:
Here, , , [nss-Cl-], , [nss-K+], [nss-Mg2+], and [nss-Ca2+] refer to the mass concentration of the ions (μg/m3). For cases with [Na+]/[Cl-] ratios higher than 1.1, [Cl-] should be substituted for [nss-Cl-]. The non-sea salt ions were calculated as follows (Balasubramanian et al., 2003; Nair et al., 2005):
The NF values of the four cations (, nss-K+, nss-Mg2+, and nss-Ca2+) are listed in Table 3. As speculated, there were three major neutralization ions in Zhanjiang. Nss-K+ was the major neutralization ion in summer, which may be related to the fact that biomass burning is an important anthropogenic activity in rural sites (Xu J. S. et al., 2017; Agarwal et al., 2020). The NF values of were the highest in winter, spring, and autumn, suggesting its dominant impact in neutralizing aerosol acidity. This result is supported by a previous study (Xu J. S. et al., 2017). Nss-Ca2+ was the second largest contributor to the neutralization of aerosol acidity, possibly due to the enhanced soil dust brought by strong wind, as suggested by the significant positive correlation between Ca2+ and the wind speed (p< 0.01; Table 2). Nearby agricultural fields and unpaved roads (Xu J. S. et al., 2017; Agarwal et al., 2020) could be the origins of this soil dust. The NF values of nss-Mg2+ were the lowest during the sampling periods, indicating that it had a minor neutralization effect.

Table 3 The neutralization factors (NFs), i.e., NH4+, nss-K+, nss-Mg2+, and nss-Ca2+, in the TSPs in Zhanjiang.
The role of precipitation in chemical conversions
As was previously discussed, in this study, the aerosol acidity and concentration were correlated with excess and . Knowledge of the origin and processes of these ions could help lessen aerosol pollution. Aerosol acidity is greatly affected by the oxidation of nitric oxide (NOx) to and of sulfur dioxide (SO2) to in the atmosphere (Cao et al., 2020). The nitrogen oxidation ratio (NOR) and the sulfur oxidation ratio (SOR) J. S. were estimated using the following equations (Lin, 2002; Xu et al., 2017) in order to gain a better understanding of the conversions of and :
Here, , [SO2], , and [NO2] refer to the mass concentrations of the ions (μg/m3). The higher SOR and NOR values observed (threshold: 0.1) suggest the presence of more gaseous precursors that generate sulfate- and nitrate-containing secondary aerosols through oxidation (Colbeck and Harrison, 1984; Ohta and Okita, 1990; Kaneyasu et al., 1995).
The monthly and seasonal variations in air temperature, NOR, SOR, and the amount of rainfall are presented in Figure 6. As can be seen, the monthly average values of NOR and SOR were higher than 0.1, indicating a considerable conversion of gaseous precursors to and in the atmospheric aerosols during the sampling periods. The fact that NO2 and SO2 were relatively abundant in winter and autumn (Figure 4B) could contribute to the higher occurrences of and (Figure 2D), which were seasonally consistent with the lower pH values (Figure 2A). The decreased production of secondary aerosols in summer is indicated by the valleys in the SOR and NOR curves (Figure 6). This seasonal pattern is contrary to the results of previous studies (Zhang et al., 2011; Xu J. S. et al., 2020). The ambient oxidant (O3) for chemical conversion is relatively abundant (Figure 4B), so the different meteorological conditions may be the cause of the low conversion rates. The conversion of NO2 to and SO2 to is favored by warm and humid ambient conditions (Zhang et al., 2011; Xu J. S. et al., 2020). Since the city investigated in this study is warmer and moister than Xi’an (Zhang et al., 2011), the chemical conversion of NO2 and SO2 occurred year-round, and the calculated oxidation ratio was higher than that in the abovementioned area. Additionally, the amount of rainfall in Zhanjiang is about twice than that in the abovementioned area and is mainly concentrated in summer (Figure 4), which gives rise to a precipitation-driven removal process. Therefore, the coexistence of low gaseous precursors (Figure 4B), low precursor conversions (Figure 6), and frequent plentiful rainfall (Figure 3) in summer in Zhanjiang may be related to the removal effect caused by the precipitation. Gaseous precursors can be captured by precipitation below the clouds (Chen et al., 2020), and a lack of reactants may induce low oxidation ratios (Jiang, 2016).
It has been reported that gaseous precursors are predominantly emitted by fossil fuel combustion (> 70%; Lv, 2019; Liu et al., 2019), and high concentrations of NO2 and SO2 in winter are influenced by additional coal combustion for heating (Zhang et al., 2011). Thus, the reduction in NO2 and SO2 in summer seems to suggest a decrease in fossil fuel usage in addition to the removal effect caused by precipitation. However, we believe that the reduced combustion in summer is unlikely to be based on the ratio of to and power production.
The ratio of the atmospheric aerosols is recognized as an effective approach to assessing stationary and mobile sources of nitrogen and sulfur and has been widely used at various locations around the world (Arimoto et al., 1996; Tan et al., 2009; Safai et al., 2010; Begam et al., 2017). When this ratio is > 1, the emissions are considered to be produced by mobile sources; otherwise, they are emitted from stationary sources (Arimoto et al., 1996; Lv, 2019). As can be seen from Figure 7, the monthly average ratios during the sampling periods were<1 (from 0.05 to 2.57, averaged at of 0.55 ± 0.33), indicating that the predominance of stationary sources over mobile sources in Zhanjiang. Thus, the NO2 and SO2 in the atmosphere during the sampling periods were associated with local emissions.
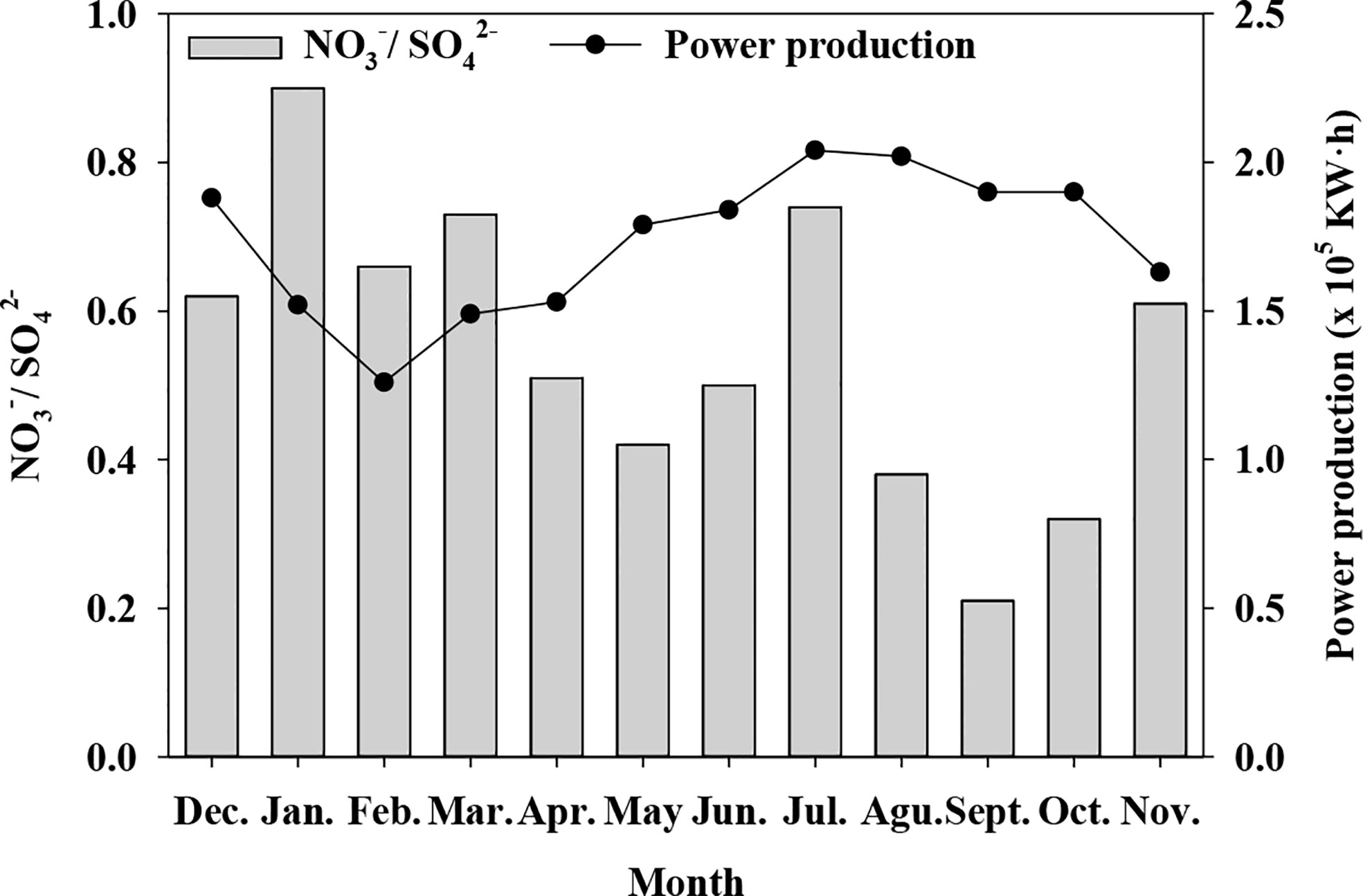
Figure 7 Monthly average values of the ratio (mass concentration ratio) and power production in Zhanjiang during the sampling periods (data from the Zhanjiang Bureau of Statistics, https://www.zhanjiang.gov.cn/tjj/).
China is a coal-fired country, and more than half of the coal is consumed by electric power plants, so power production can be considered to be an indicator of the local fossil fuel consumption. Power production continues at a high rate in summer; whereas it has a relatively low rate in winter and autumn (Figure 7), implying an adequate amount of gaseous precursors should be present in the hot and rainy season. However, the observations in summer showed low values (Figure 4B), which in turn supports the possibility of a precipitation-driven removal process.
Possible air quality improvement process and source identification in a heavy industry coastal city
Aerosol pollution is a significant global problem caused by rapid economic and industrial development. The inconsistency between heavy industrial activities and low aerosol concentrations during our sampling campaign in Zhanjiang may be related to a precipitation-driven removal effect (Hou et al., 2018) and the marine dilution effect. Aerosols from marine emissions are considered to be relatively clean (Jickells et al., 2003; Xiao et al., 2017; Zhou et al., 2019), which results in potential dilution and air quality improvement when mixed with polluted aerosols produced by terrestrial anthropogenic activities. Since the study area is bordered by the SCS, the marine effect should be taken into account in this region. Thus, the aerosols from non-sea salt contributions were evaluated, and the results are presented in Table 4. More than 50% of the Mg2+ in Zhanjiang was sourced from sea salt (Table 4), indicating that the Mg2+ is predominantly from marine emissions. The higher ratios (>90%) of nss-K+/K+, nss-Ca2+/Ca2+, and found during sampling campaign (Table 4) indicate minor contributions from marine emissions to these WSIIs in the aerosols.
In order to investigate the effects of precipitation on the TSPs, linear regression analysis (SPSS version 22.0, IBM Corp.) was conducted to quantitatively describe the impacts of precipitation on the TSPs. The frequency and amount of precipitation were selected as the precipitation characteristics, as was suggested by Hou et al. (2018). As can be seen from the data shown in Figure 8, the TSPs were negatively affected by precipitation, indicating that the atmospheric aerosol lifetime was sensitive to precipitation (Hou et al., 2018), and precipitation significantly contributed (> 50%) to the decrease in the TSPs pollution in Zhanjiang. The high coefficient of determination (R2 = 0.63) in Figure 8A further suggests that the precipitation frequency had a larger effect on the removal efficiency than the amount of precipitation. The model results of Hou et al. (2018) also highlight the importance of precipitation frequency in shortening aerosol lifetime.
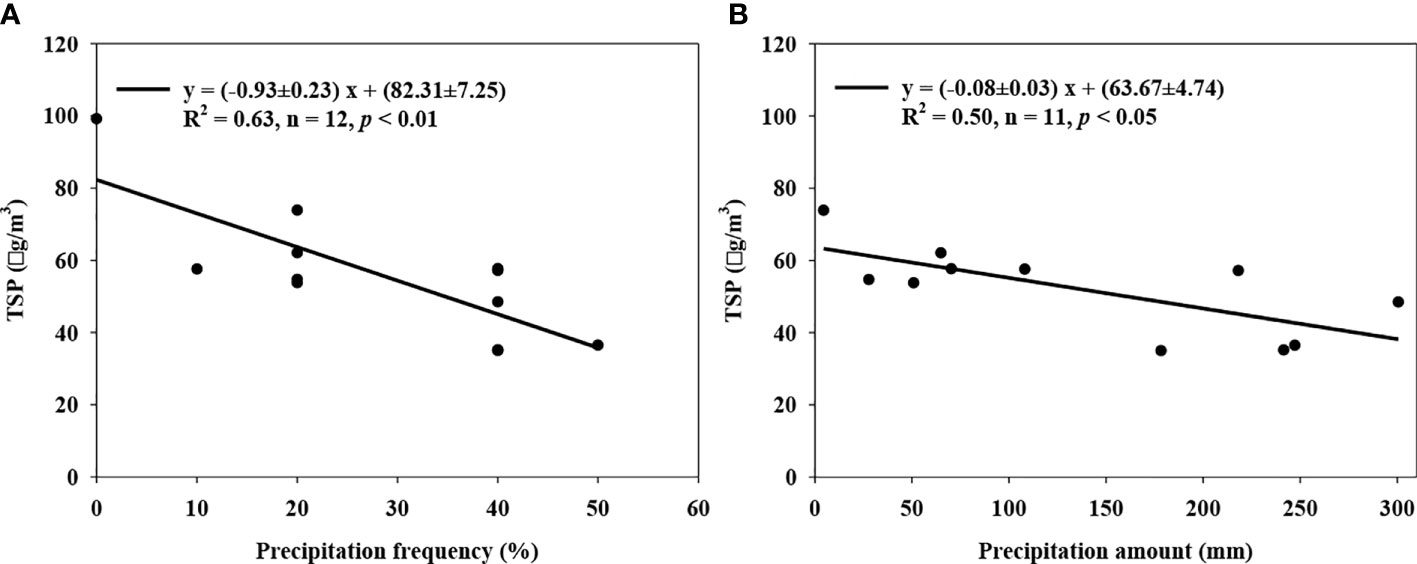
Figure 8 Linear regressions of (A) TSPs and precipitation frequency and (B) TSPs and amount of precipitation.
Principal component analysis (PCA, SPSS version 22.0, IBM Corp.) was conducted in this study and presented in Table 5. Principal Components Analysis (PCA) is an algorithm to transform the columns of a dataset into a new set of features called Principal Components (PC). According to the results of PCA, a large chunk of the information across the full dataset is effectively compressed in fewer feature columns (i.e., PC1, PC2, PC3, etc.), while still preserving as much information as possible. This enables dimensionality reduction and ability to visualize the separation of classes or clusters (https://builtin.com/data-science/step-step-explanation-principal-component-analysis). The extracted PC with eigenvalue > 1.0 is retained for interpretation and the loading above 0.5 of each component is determined to be the major contribution to each of the PC scores (Gao et al., 2011; Zhang et al., 2011; Agarwal et al., 2020; Liu et al., 2021a; Liu et al., 2021b). In these studies, the determined component is regarded as tracer of certain source (Gao et al., 2011; Zhang et al., 2011; Agarwal et al., 2020; Liu et al., 2021a; Liu et al., 2021b). Similarly, the determined component can be used for tracing sources in the present study.
As presented in Table 5, three factors were identified (eigenvalue > 1.0) as PCs and accounted for 90.0% of the total data variance. PC1 was strongly loaded with TSPs, , Mg2+, Ca2+, Cl-, , and . , , and were mainly from a secondary formation process (Agarwal et al., 2020). As was previously discussed, Cl- reflects the influence of coal combustion (Xue et al., 2016; Jain et al., 2017; Xu J.S. et al., 2017). Ca2+ and Mg2+ reflect the contributions of soil dust and are the principal crustal elements (Gao et al., 2011; Zhang et al., 2011; Meng et al., 2016; Saxena et al., 2017; Jaiprakash et al., 2017). Therefore, PC1 was identified as a mixture of secondary aerosols, coal combustion, and soil dust. PC2 explained 27.4% of the total variance and was observed to be heavily loaded for Na+, Mg2+, and Cl-, which were possibly influenced by sea salts. This is in agreement with the results for other coastal areas (Xu J. S. et al., 2017), that is, the aerosols in these areas exhibited oceanic characteristics. Thus, the presence of these ions indicates that PC2 was primarily sourced from marine emissions. PC3 was characterized by a high loading of K+ and accounted for 11.5% of the variance. Moreover, K+ was found to be uncorrelated with most of the WSIIs in Zhanjiang (Table 2), suggesting that the PC3 source is biomass burning.
We summarize the possible removal effect of TSPs in Zhanjiang in a conceptual diagram (Figure 9), showing frequent precipitation could remove a large amount of gas precursors and local secondary aerosols. The aerosols sourced from clean marine emissions therefore act as the supplement in the ambient, leading to the observance of relatively high contribution in the TSPs. This result may provide useful information for lessening aerosol pollution under the background of the pervasive anthropogenic aerosols pollution around the world, and the relocation site chosen for industrial activities.
As stated above, gas precursors and local secondary aerosols can be captured by frequent precipitation and abundant rainfall. These materials thus enter the marine environment in the coastal area as atmospheric deposition, which may aggravate nutrients loading and even result in eutrophication and hypoxia (Duce et al., 2008; Chen et al., 2017; Wu et al., 2018; Cui et al., 2020). The impacts of atmospheric deposition on marine environment require attention and need further exploration.
Conclusions
The goal of this study was to clarify the discrepancy between industrial activities and low aerosol concentrations in a tropical coastal city due to a precipitation-driven removal process and the marine dilution effect. In Zhanjiang, 72h TSPs collection and WSIIs analysis were conducted during a one-year sampling campaign. The results of this investigation show that the TSP mass concentrations and the total WSII concentrations in Zhanjiang were lower in the spring and summer and were higher in the winter and autumn. This seasonal pattern and high aerosol acidity (indicated by the ion balance, neutralization factors, and pH values) further suggest that the TSPs, WSIIs, and acidic gaseous precursors were removed by frequent precipitation and abundant rainfall. The PCA results revealed that secondary aerosols and sea salts were important sources of the TSPs and WSIIs. One of the more significant findings of this study is that a combination of high precipitation frequency and marine dilution can lessen aerosol pollution in a heavy industrial city.
Data availability statement
The original contributions presented in the study are included in the article/Supplementary Material. Further inquiries can be directed to the corresponding author.
Author contributions
XZ Conceptualization, Writing-Original draft, Writing-Review and Editing. Data Curation, Visualization, Software. ZL Methodology, Project administration, Writing-Review and Editing. CC Investigation, Methodology, Writing-Review and Editing. HL Investigation, Writing-Review and Editing. QZ Methodology, Project administration, Writing-Review and Editing. ZZ Methodology, Writing-Review and Editing. QL Writing-Review and Editing. All authors contributed to the article and approved the submitted version.
Funding
This work was supported by National Natural Science Foundation of China (92158201, U1901213); Innovation and Entrepreneurship Project of Shantou (2021112176541391); Special Projects in Key Fields of Colleges and Universities in Guangdong Province (2020ZDZX1099); Guangdong Provincial College Innovation Team Project (2019KCXTF021), First-class Discipline Plan of Guangdong Province (080503032101, 231420003).
Conflict of interest
The authors declare that the research was conducted in the absence of any commercial or financial relationships that could be construed as a potential conflict of interest.
Publisher’s note
All claims expressed in this article are solely those of the authors and do not necessarily represent those of their affiliated organizations, or those of the publisher, the editors and the reviewers. Any product that may be evaluated in this article, or claim that may be made by its manufacturer, is not guaranteed or endorsed by the publisher.
References
Agarwal A., Satsangi A., Lakhani A., Kumari K. M. (2020). Seasonal and spatial variability of secondary inorganic aerosols in PM2.5 at Agra: Source apportionment through receptor models. Chemosphere 242, 125132. doi: 10.1016/j.chemosphere.2019.125132
Ali-Mohamed A. Y. (1991). Estimation of inorganic particulate matter in the atmosphere of isa town, Bahrain, by dry deposition. Atmos. Environ. Part B. 25, 397–405. doi: 10.1016/0957-1272(91)90011-3
Arimoto R., Duce R. A., Savoie D. L., Prospero J. M., Talbot R., Cullen J. D., et al. (1996). Relationships among aerosol constituents from Asia and the north pacific during PEM-West a. J. Geophys. Res. Atmos. 101, 2011–2023. doi: 10.1029/95JD01071
Balasubramanian R., Qian W. B., Decesari S., Facchini M. C., Fuzzi S. (2003). Comprehensive characterization of PM2.5 aerosols in Singapore. J. Geophys. Res. Atmos. 108 (D16), 4523. doi: 10.1029/2002JD002517
Begam G. R., Vachaspati C. V., Ahammed Y. N., Kumar K. R., Reddy R. R., Sharma S. K., et al. (2017). Seasonal characteristics of water-soluble inorganic ions and carbonaceous aerosols in total suspended particulate matter at a rural semi-arid site, kadapa (India). Environ. Sci. pollut. Res. 24, 1719–1734. doi: 10.1007/s11356-016-7917-1
Cao J. J., Tie X. X., Dabberdt W. F., Jie T., Zhao Z. Z., An Z. S., et al. (2013). On the potential high acid deposition in northeastern China. J. Geophys. Res. Atmos. 118, 4834–4846. doi: 10.1002/jgrd.50381
Cao Y. S., Zhang Z. Y., Xiao H. W., Xie Y. J., Liang Y., Xiao H. Y. (2020). How aerosol pH responds to nitrate to sulfate ratio of fine-mode particulate. Environ. Sci. pollut. Res. 27, 35031–35039. doi: 10.1007/s11356-020-09810-0
Chen F. J., Chen C. Q., Zhou F. X., Lao Q. B., Zhu Q. M., Zhang S. W. (2017). Nutrients in atmospheric wet deposition in the zhanjiang bay. China Environ. Sci. 37 (6), 2055–2063.
Chen F. J., Lao Q. B., Jia G. D., Chen C. Q., Zhu Q. M., Zhou X. (2019). Seasonal variations of nitrate dual isotopes in wet deposition in a tropical city in China. Atmos. Environ. 196, 1–9. doi: 10.1016/j.atmosenv.2018.09.061
Chen F. J., Lao Q. B., Li Z. Y., Bian P. W., Zhu Q. M., Chen C. Q., et al. (2020). Monthly variations of the nitrogen isotope of ammonium in wet deposition in a tropical city of south China. Aerosol. Air. Qual. Res. 20, 1062–1069. doi: 10.4209/aaqr.2019.06.0303
Colbeck I., Harrison R. M. (1984). Ozone–secondary aerosol–visibility relationships in north-West England. Sci. Total. Environ. 34, 87–100. doi: 10.1016/0048-9697(84)90043-3
Cui X., Huang C. Z., Wu J. P., Liu X. H., Hong Y. G. (2020). Temporal and spatial variations of net anthropogenic nitrogen inputs (NANI) in the pearl river basin of China from 1986 to 2015. PloS One 15 (2), e0228683. doi: 10.1371/journal.pone.0228683
Duce R. A., LaRoche J., Altieri K., Arrigo K. R., Baker A. R., Capone D. G., et al. (2008). Impacts of atmospheric anthropogenic nitrogen on the open ocean. Science 320 (16), 893–897. doi: 10.1126/science.1150369
Fuzzi S., Baltensperger U., Carslaw K., Decesari S., Facchini M. C., Fowler D., et al. (2015). Particulate matter, air quality and climate: Lessons learned and future needs. Atmos. Chem. Phys. 14 (15), 8217–8299. doi: 10.5194/acp-15-8217-2015
Gao X. M., Yang L. X., Cheng S. H., Gao R., Zhou Y., Xue L. K., et al. (2011). Semi-continuous measurement of water-soluble ions in PM2.5 in jinan, China: Temporal variations and source apportionments. Atmos. Environ. 45, 6048–6056. doi: 10.1016/j.atmosenv.2011.07.041
Grasshoff K., Kremling K., Ehrhardt M. (1999). Methods of seawater analysis, 3rd edn. Wiley. New York. pp, 159–228. doi: 10.1002/9783527613984
Guo S., Hu M., Zamora M. L., Peng J. F., Shang D. J., Zheng J., et al. (2014). Elucidating severe urban haze formation in China. PNAS 111, 17373–17378. doi: 10.1073/pnas.1419604111
Hou P., Wu S. L., McCarty J. L., Gao Y. (2018). Sensitivity of atmospheric aerosol scavenging to precipitation intensity and frequency in the context of global climate change. Atmos. Chem. Phys. 18, 8173–8182. doi: 10.5194/acp-18-8173-2018
Huang R. J., Zhang Y. L., Bozzetti C., Ho K. F., Cao J. J., Han Y. M., et al. (2014). High secondary aerosol contribution to particulate pollution during haze events in China. Nature 514, 218–222. doi: 10.1038/nature13774
Jain S., Sharma S. K., Choudhary N., Masiwal R., Saxena M., Sharma A., et al. (2017). Chemical characteristics and source apportionment of PM2.5 using PCA/APCS, UNMIX, and PMF at an urban site of Delhi, India. Environ. Sci. pollut. Res. 24, 14637–14656. doi: 10.1007/s11356-017-8925-5
Jaiprakash, Singhai A., Habib G., Raman R. S., Gupta T. (2017). Chemical characterization of PM1.0 aerosol in Delhi and source apportionment using positive matrix factorization. Environ. Sci. pollut. Res. 24, 445–462. doi: 10.1007/s11356-016-7708-8
Jiang B. (2016). Analysis of fine particle's chemical characteristics and secondary pollution formation at heshan atmospheric supersite. dissertation (Guangzhou: Jinan University).
Jickells T. D., Kelly S. D., Baker A. R., Biswas K., Dennis P. F., Spokes L. J., et al. (2003). Isotopic evidence for a marine ammonia source. Geophys. Res. Lett. 30(7), 1374. doi: 10.1029/2002GL016728
Kaneyasu N., Ohta S., Murao N. (1995). Seasonal variation in the chemical composition of atmospheric aerosols and gaseous species in Sapporo, Japan. Atmos. Environ. 29, 1559–1568. doi: 10.1016/1352-2310(94)00356-P
Kawashima H., Kurahashi T. (2011). Inorganic ion and nitrogen isotopic compositions of atmospheric aerosols at yurihonjo, Japan: Implications for nitrogen sources. Atmos. Environ. 45, 6309–6316. doi: 10.1016/j.atmosenv.2011.08.057
Kundu S., Kawamura K., Lee M. (2010). Seasonal variation of the concentrations of nitrogenous species and their nitrogen isotopic ratios in aerosols at gosan, jeju island: Implications for atmospheric processing and source changes of aerosols. J. Geophys. Res. Atmos. 115, D20305. doi: 10.1029/2009JD013323
Li J. C., Cao R. X., Lao Q. B., Chen F. J., Chen C. Q., Zhou X., et al. (2020). Assessing seasonal nitrate contamination by nitrate dual isotopes in a monsoon-controlled bay with intensive human activities in south China. Int. J. Environ. Res. Public Health 17, 1921. doi: 10.3390/ijerph17061921
Li Q., Li X. D., Yang Z., Cui G., Ding S. (2020). Diurnal and seasonal variations in water-soluble inorganic ions and nitrate dual isotopes of PM2.5: Implications for source apportionment and formation processes of urban aerosol nitrate. Atmos. Res. 248 (15), 105197. doi: 10.1016/j.atmosres.2020.105197
Lin J. J. (2002). Characterization of water-soluble ion species in urban ambient particles. Environ. Int. 28, 55–61. doi: 10.1016/S0160-4120(02)00004-1
Lin C. T., Jickells T. D., Baker A. R., Marca A., Johnson M. T. (2016). Aerosol isotopic ammonium signatures over the remote Atlantic ocean. Atmos. Environ. 133, 165–169. doi: 10.1016/j.atmosenv.2016.03.020
Liu M. Y., Chen M., Duan M., Lin Y., Liu X., Liang J., et al. (2021b). Particulate export of PAHs firstly traced by 210Po/210Pb disequilibrium: Implication on the “Shelf sink effect” in the Arctic ocean. J. Geophys. Res.: Oceans. 126, e2021JC017384. doi: 10.1029/2021JC017384
Liu M. X., Huang X., Song Y., Tang J., Cao J. J., Zhang X. Y., et al. (2019). Ammonia emission control in China would mitigate haze pollution and nitrogen deposition, but worsen acid rain. PNAS 116, 7760–7765. doi: 10.1073/pnas.1814880116
Liu M. Y., Zheng H. W., Wang W. M., Ke H. W., Huang P., Liu S. G., et al. (2021a). Enhanced sinks of polycyclic aromatic hydrocarbons due to kuroshio intrusion: Implications on biogeochemical processes in the ocean-dominated marginal seas. Environ. Sci. Technol. 55 (10), 6838–6847. doi: 10.1021/acs.est.1c01009
Luo H. J., Lao Q. B., Chen F. J., Chen C. Q., Zhou X., Jin G. Z., et al. (2022). Nitrate sources and formation in aerosol and precipitation in a tropical city in south China: Insight from nitrate dual isotopes. Atmos. Environ. 278, 119087. doi: 10.1016/j.atmosenv.2022.119087
Lv Z. (2019). Chemical characteristics and source apportionment of water-soluble ions in PM2.5 in shijiazhuang. dissertation (Nanchang: East China University of Technology).
Meng C. C., Wang L. T., Zhang F. F., Wei Z., Ma S. M., Ma X., et al. (2016). Characteristics of concentrations and water-soluble inorganic ions in PM2.5 in handan city, hebei province, China. Atmos. Res. 171, 133–146. doi: 10.1016/j.atmosres.2015.12.013
Nair P. R., Parameswaran K., Abraham A., Jacob S. (2005). Wind-dependence of sea-salt and non-sea-salt aerosols over the oceanic environment. J. Atmos. Sol. Terr. Phys. 67, 884–898. doi: 10.1016/j.jastp.2005.02.008
Ohta S., Okita T. (1990). A chemical characterization of atmospheric aerosol in Sapporo. Atmos. Environ. Part A. 24, 815–822. doi: 10.1016/0960-1686(90)90282-R
Peng S. Y., Kong D. M., Li L. T., Zou C. L., Chen F. J., Li M. J., et al. (2020). Distribution and sources of DDT and its metabolites in porewater and sediment from a typical tropical bay in the south China Sea. Environ. pollut. 267, 115492. doi: 10.1016/j.envpol.2020.115492
Safai P. D., Budhavant K. B., Rao P. S. P., Ali K., Sinha A. (2010). Source characterization for aerosol constituents and changing roles of calcium and ammonium aerosols in the neutralization of aerosol acidity at a semi-urban site in SW India. Atmos. Res. 98, 78–88. doi: 10.1016/j.atmosres.2010.05.011
Satsangi A., Pachauri T., Singla V., Lakhani A., Kumari K. M. (2013). Water soluble ionic species in atmospheric aerosols: Concentrations and sources at Agra in the indo-gangetic plain (IGP). Aerosol. Air. Qual. Res. 13, 1877–1889. doi: 10.4209/aaqr.2012.08.0227
Saxena M., Sharma A., Sen A., Saxena P., Saraswati, Mandal T. K., et al. (2017). Water soluble inorganic species of PM10 and PM2.5 at an urban site of Delhi, India: Seasonal variability and sources. Atmos. Res. 184, 112–125. doi: 10.1016/j.atmosres.2016.10.005
Seinfeld J. H. (2004). Air pollution: A half century of progress. Energy Environ. 50, 12. doi: 10.1002/aic.10102
Shon Z. H., Kim K. H., Song S. K., Jung K., Kim N. J., Lee J. B. (2012). Relationship between water-soluble ions in PM2.5 and their precursor gases in Seoul megacity. Atmos. Environ. 59, 540–550. doi: 10.1016/j.atmosenv.2012.04.033
Sun R. X., Sun Y., Li Q. X., Zheng X. B., Luo X. J., Mai B. X. (2018). Polycyclic aromatic hydrocarbons in sediments and marine organisms: Implications of anthropogenic effects on the coastal environment. Sci. Total. Environ., 640–641: 264-272. doi: 10.1016/j.scitotenv.2018.05.320
Tan J. H., Duan J. C., Chen D. H., Wang X. H., Guo S. J., Bi X. H., et al. (2009). Chemical characteristics of haze during summer and winter in guangzhou. Atmos. Res. 94, 238–245. doi: 10.1016/j.atmosres.2009.05.016
Tang X., Zhang Y., Shao M. (2006). Atmospheric environmental chemistry. 2nd edition (Beijing: Higher Education press).
Tao J., Zhang L. M., Cao J. J., Zhong L. J., Chen D. S., Yang Y. H., et al. (2017). Source apportionment of PM2.5 at urban and suburban areas of the pearl river delta region, south China -with emphasis on ship emissions. Sci. Total. Environ. 574, 1559–1570. doi: 10.1016/j.scitotenv.2016.08.175
Tian J., Guan H., Zhou Y. H., Zheng N. J., Xiao H. W., Zhao J. J., et al. (2021). Isotopic source analysis of nitrogen-containing aerosol: A study of PM2.5 in guiyang (SW, China). Sci. Total. Environ. 760, 143935. doi: 10.1016/j.scitotenv.2020.143935
Wang J. J., Beusen A. H. W., Liu X. C., Dingenen R. V., Dentener F., Yao Q. Z., et al. (2020). Spatially explicit inventory of sources of nitrogen inputs to the yellow Sea, East China Sea, and south China Sea for the period 1970–2010. Earth's. Future 8, e2020EF001516. doi: 10.1029/2020EF001516
Wang Q. Q., Li J., Yang J. X., Chen Y., Li Y. Y., Li S. Y., et al. (2020). Seasonal characterization of aerosol composition and sources in a polluted city in central China. Chemosphere 258, 127310. doi: 10.1016/j.chemosphere.2020.127310
Wang G., Zhu Z. Y., Zhao N., Wei P., Li G. H., Zhang H. Y. (2021). Variations in characteristics and transport pathways of PM2.5 during heavy pollution episodes in 2013–2019 in jinan, a central city in the north China plain. Environ. Pollut. 284, 117450. doi: 10.1016/j.envpol.2021.117450
Weber R. J., Guo H. Y., Russell A. G., Nenes A. (2016). High aerosol acidity despite declining atmospheric sulfate concentrations over the past 15 years. Nat. Geosci. 9, 282–285. doi: 10.1038/ngeo2665
Wu Y. C., Zhang J. P., Liu S. L., Jiang Z. J., Huang X. P. (2018). Aerosol concentrations and atmospheric dry deposition fluxes of nutrients over daya bay, south China Sea. Mar. Pollut. Bull. 128, 106–114. doi: 10.1016/j.marpolbul.2018.01.019
Xiao H. Y., Liu C. Q. (2004). Chemical characteristics of water-soluble components in TSP over guiyang, SW China 2003. Atmos. Environ. 38, 6297–6306. doi: 10.1016/j.atmosenv.2004.08.033
Xiao H. W., Xiao H. Y., Luo L., Shen C. Y., Long A. M., Chen L., et al. (2017). Atmospheric aerosol compositions over the south China Sea: temporal variability and source apportionment. Atmos. Chem. Phys. 17, 3199–3214. doi: 10.5194/acp-17-3199-2017
Xu L. L., Duan F. K., He K. B., Ma Y. L., Zhu L. D., Zheng Y. X., et al. (2017). Characteristics of the secondary water-soluble ions in a typical autumn haze in Beijing. Environ. Pollut. 227, 296–305. doi: 10.1016/j.envpol.2017.04.076
Xue Y. F., Zhou Z., Nie T., Wang K., Nie L., Pan T., et al. (2016). Trends of multiple air pollutants emissions from residential coal combustion in Beijing and its implication on improving air quality for control measures. Atmos. Environ. 142, 303–312. doi: 10.1016/j.atmosenv.2016.08.004
Xu J. Z., Wang Z. B., Yu G. M., Qin X., Ren J. W., Qin D. H. (2014). Characteristics of water soluble ionic species in fine particles from a high altitude site on the northern boundary of Tibetan plateau: Mixture of mineral dust and anthropogenic aerosol. Atmos. Environ. 143, 43–56. doi: 10.1016/j.atmosres.2014.01.018
Xu J. S., Xu M. X., Snape C., He J., Behera S. N., Xu H. H., et al. (2017). Temporal and spatial variation in major ion chemistry and source identification of secondary inorganic aerosols in northern zhejiang province, China. Chemosphere 179, 316–330. doi: 10.1016/j.chemosphere.2017.03.119
Zhang T., Cao J. J., Tie X. X., Shen Z. X., Liu S. X., Ding H., et al. (2011). Water-soluble ions in atmospheric aerosols measured in Xi'an, China: Seasonal variations and sources. Atmos. Res. 102, 110–119. doi: 10.1016/j.atmosres.2011.06.014
Zhou X., Luo H. J., Chen F. J., Chen C. Q., Li Z. Y., Zhu Q. M., et al. (2022). Regime shift in aerosol ammonium between the rainy and dry season: Perspective from stable isotopes in bulk deposition. Atmos. Pollut. Res. 13, 101462. doi: 10.1016/j.apr.2022.101462
Zhou X. D., Xu Z. F., Liu W. J., Wu Y., Zhao T., Jiang H., et al. (2019). Chemical composition of precipitation in shenzhen, a coastal mega-city in south China: Influence of urbanization and anthropogenic activities on acidity and ionic composition. Sci. Total. Environ. 662, 218–226. doi: 10.1016/j.scitotenv.2019.01.096
Keywords: aerosol, air quality improvement, water-soluble inorganic ions, precipitation, sources, Zhanjiang City
Citation: Zhou X, Li Z, Chen C, Luo H, Zhu Q, Zeng Z and Lao Q (2022) The removal of atmospheric aerosols in a heavy industrial coastal city in China with frequent rainfall. Front. Mar. Sci. 9:977120. doi: 10.3389/fmars.2022.977120
Received: 24 June 2022; Accepted: 04 August 2022;
Published: 23 August 2022.
Edited by:
Meilin Wu, South China Sea Institute of Oceanology, Chinese Academy of Sciences, ChinaReviewed by:
Guo Wei, East China University of Technology, ChinaPeixue Song, Zaozhuang University, China
Zuobing Liang, Sun Yat-sen University, China
Copyright © 2022 Zhou, Li, Chen, Luo, Zhu, Zeng and Lao. This is an open-access article distributed under the terms of the Creative Commons Attribution License (CC BY). The use, distribution or reproduction in other forums is permitted, provided the original author(s) and the copyright owner(s) are credited and that the original publication in this journal is cited, in accordance with accepted academic practice. No use, distribution or reproduction is permitted which does not comply with these terms.
*Correspondence: Qingmei Zhu, zhuqingmei1987@163.com