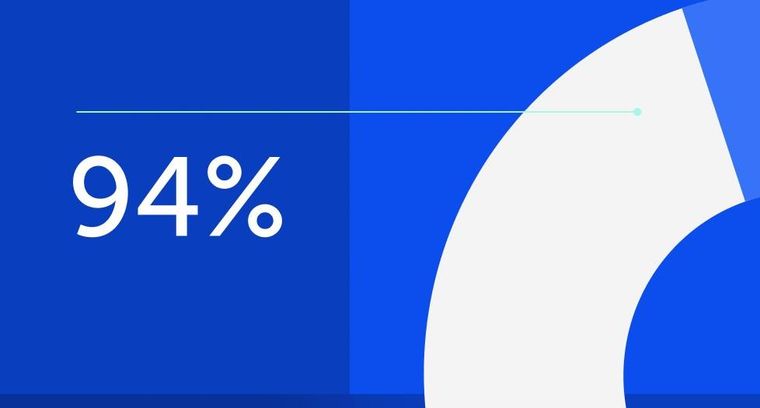
94% of researchers rate our articles as excellent or good
Learn more about the work of our research integrity team to safeguard the quality of each article we publish.
Find out more
ORIGINAL RESEARCH article
Front. Mar. Sci., 02 September 2022
Sec. Marine Evolutionary Biology, Biogeography and Species Diversity
Volume 9 - 2022 | https://doi.org/10.3389/fmars.2022.976255
The shallow-water hydrothermal vent (HV) system off Kueishan Island lies at the end of the Okinawa Trough to the northeast of Taiwan. Near its submarine vent openings, aperiodic vent discharges generate a dynamic acidic (pH 5.5-8.1) and sulfidic (9-3000 μM) ecosystem. The dominant metazoan in this unique environment is the brachyuran vent crab, Xenograpsus testudinatus, which has developed robust metabolic strategies and highly adaptive acid-base regulatory mechanisms to maintain its physiological homeostasis. X. testudinatus is considered a holobiont, but the symbiotic mechanisms underlying acid and sulfur tolerance in the host-microbe system remain largely unclear. In this study, we used LoopSeq long-read sequencing of the full-length 16S rRNA gene to identify the bacterial communities present in the gills and carapace surface of X. testudinatus. The alpha diversity analysis, Venn diagram, and principal coordinate analysis (PCoA) indicated that the gills and carapace surface exhibit different bacterial constituents. Further measurements of relative abundance, coupled with functional predictions and fluorescence in situ hybridization (FISH), revealed a predominance of Sulfurovum sp. NBC37-1, a key bacterium that can perform sulfur and hydrogen oxidation to support denitrification processes. Consequently, our findings suggest that the symbiotic bacteria may play a critical role in conferring the extraordinary acid and sulfur tolerances of X. testudinatus, allowing the crustacean holobiont to thrive in its ecological niche within one of the most extreme marine habitats on Earth.
Hydrothermal vent (HV) systems are widely recognized as being among the world’s most extreme marine environments. Many characteristics of these systems arise due to underwater volcanic activities, and the first HV system was discovered on the Galapagos rift in 1977 (Lonsdale, 1977; Glowka, 2003). The chemical-rich fluids gushing out of the vent opening create a highly acidic and megathermal environment (Elderfield and Schultz, 1996; Fisher et al., 2007), which is home to microbial populations that exhibit some of the highest diversity on the planet (Van Dover and Trask, 2000; Glowka, 2003; Van Dover, 2014). The diverse microorganisms in HVs build a biological bridge between the extreme environment and chemoautotrophic animals, serving as the primary biomass sources for food webs that support endemic crustacean, polychaete, echinoderm, coelenterate and mollusk species (Little and Vrijenhoek, 2003). Moreover, HV ecosystems are typically dominated by benthic invertebrate taxa that also host symbiotic and chemoautotrophic microorganisms (Dahms et al., 2018; Alfaro-Lucas et al., 2020). The chemoautotrophic microbes acquire electron donors (e.g., sulfide, sulfur, and hydrogen) in vent fluids, electron acceptors (e.g., nitrate and oxygen) in seawater, and inorganic carbon (e.g., CO2 or CH4) in vent fluids or seawater (Kuo et al., 2001; Takai et al., 2009).
HV fluids are typically enriched in inorganic carbon, ammonium, methane, sulfur, and hydrogen sulfide (H2S; Butterfield et al., 1990; Chen et al., 2005). HV dissolved gases or gas bubbles are enriched in carbon dioxide (CO2) and sulfur dioxide (SO2; Butterfield et al., 2011; Lin et al., 2019). The high sulfur concentrations in the HV fluids are significant in part because microorganisms that can perform sulfur/sulfide oxidation reactions may utilize the sulfur and sulfide as an energy source (Tunnicliffe, 1991; Tarasov et al., 2005; Akerman et al., 2013). Several prominent sulfur-oxidizing bacteria (SOB) have been identified in shallow-water HV areas, including Vulcano (Eolian Islands, Italy), Milos Island (Greece), Espalamaca (Faial, Azores), and El Hierro (Canary Islands, Spain; Gugliandolo and Maugeri, 1993; Giovannelli et al., 2013; Rajasabapathy et al., 2014; Gonzalez et al., 2020). In addition, chemoautotrophic bacteria have been isolated from crustaceans endemic to deep-sea HVs, such as the vent shrimp Rimicaris exoculate, yeti crab Kiwa hirsute and galatheid crab Shinkaia crosnieri (Goffredi et al., 2008; Zbinden et al., 2008; Tsuchida et al., 2011). These symbiotic bacteria play important roles in primary production and life-sustaining detoxification processes, especially within the gills of HV animals (Fialamedioni et al., 1986; Jouin and Gaill, 1990; Compere et al., 2002; Ponsard et al., 2013; Jan et al., 2014).
At the rifting end of the Okinawa Trough, the shallow-water HV system off Kueishan Island lies just northeast of Taiwan (Hu et al., 2012). This HV system consists of several smokers that discharge highly acidic (pH 1.75-4.60), sulfidic and hot fluids (65-116°C), resulting in relatively low pH (5.4-7.3) and dissolved oxygen (3.9-4.9 mg/L) values compared to the non-vent seawater (Jeng et al., 2004; Han et al., 2014; Chan et al., 2016). The shallow-water HV plumes cause several physicochemical parameters such as temperature, pH, and redox status to vary over an extreme range of values (Peng et al., 2011; Dahms et al., 2018; Pang et al., 2019; Misuraca et al., 2021). As such, vent-associated biological communities must exhibit adaptive behavioral, physiological, morphological and symbiotic features to sustain their lives (Adams et al., 2011; Hu et al., 2016; Allen et al., 2021). For example, previous reports have detailed unique biochemical mechanisms for sulfide detoxification (Wang et al., 2021), behavioral and molecular responses to high temperatures (Gurunathan et al., 2021), and the presence of specialized transepithelial ionocytes to cope with low-pH conditions (Hu et al., 2016). The shallow-water submarine vent field off the southeastern shore of Kueishan Island provides an accessible natural laboratory to study the effects of HV environments on local organisms (Han et al., 2014; Chan et al., 2016; Yang et al., 2016; Pang et al., 2019). Xenograpsus testudinatus, a brachyuran crab species, was originally reported to thrive in this extreme shallow-water HV system. It has also been found in shallow-water HV waters at Showa Iwo-jima in Kagoshima and Shikine-jima Island in the Izu archipelago of Japan (Jeng et al., 2004; Ng et al., 2014; Miyake et al., 2019; Yang et al., 2022). Since X. testudinatus is a vent-endemic species, it is expected to have strong ion and acid-base regulatory abilities that allow it to cope with the dynamic challenges presented by the environment. A previous study revealed the stimulation of mRNA expression, protein abundance and enzyme activity for basolateral Na+/K+-ATPase (NKA) and V-type H+-ATPase (VHA) in the gills of normal pH-acclimated X. testudinatus (pH 8.0) after exposure to acidified seawater (pH 6.5; Hu et al., 2016). The study showed that the subcellular localization of VHA was mainly within the cytosol and along with the apical membrane of the gill lamella epithelium, supporting the idea that VHA is involved in proton secretion as a mechanism of acid excretion (Hu et al., 2016). Additionally, similarly to vertebrate blood, crab hemolymph serves as a major transport medium for materials between cells and all the internal tissues in the body, such as hormones, waste materials, and nutrients, which have also been well documented (Allen et al., 2020; Allen et al., 2021). In this way, the physiochemical parameters in the hemolymph of crabs can be used to describe the physiological responses and adaptive characteristics.
In addition to adaptive mechanisms in the cells of the endemic macroorganisms, symbiotic microorganisms also support the survival of their hosts in extreme HV environments. The first step in understanding the interaction is to identify the microbial compositions in essential tissues, such as the gills and carapace, of the multicellular hosts. The two tissues directly contact the environment, and the carapace also serves as a shield for decapod crustaceans against the predators like fish (Farre et al., 2021). A white biofilm exists on the carapace surface of wild X. testudinatus (as we observed in Figure 1E), which may be a layer of the bacterial mat (Wang et al., 2014). Indeed, a previous study showed that the microbial community on the carapace of fiddler crabs (Uca panacea) was closely related to the community in the surrounding sediments (Cuellar-Gempeler and Leibold, 2019). Furthermore, the microbial epibiotic communities’ significance on the carapaces of deep-sea galatheid squat lobsters (Munidopsis alvisca) was interpreted as a mutualistic host-microbe relationship for sulfide detoxification (Leinberger et al., 2022). Up to now, only two studies have probed X. testudinatus tissues to examine the importance and potential contributions of symbiosis-associated bacteria (Ho et al., 2015; Yang et al., 2016); both studies showed diverse bacterial constitutions in different tissues of X. testudinatus. However, the two studies examined partial regions of the bacterial 16S rRNA gene, which usually identifies bacterial compositions only on the taxonomic levels above species or genus.
Figure 1 Landscape of the shallow-water hydrothermal vent system off Kueishan Island and representative images of hydrothermal vent crabs. Map shows Kueishan Island, which is located off the northeastern coast of Taiwan (A, upper left). Relative positions, latitude and longitude coordinates of the sample collection sites (vent and non-vent areas) (A). The photos show the southeastern tip of Kueishan Island, where white sulfur plumes are visible on the sea surface (B), which is emanated from the subsurface hydrothermal vent system (C). A large number of endemic crabs (Xenograpsus testudinatus) inhabit the vent area (D). A white biofilm can be observed on the carapace surface of the animals (E).
In the present study, the gills and carapace surface of HV crab X. testudinatus were examined in terms of the resident bacterial communities, in order to better understand how the microorganism mediates interactions between the HV environment and endemic animals. To gain in-depth knowledge about X. testudinatus-associated bacterial taxa, we used multiple approaches, including LoopSeq full-length 16S rRNA gene sequencing. Such full-length sequencing provides a higher data resolution. We also performed functional annotation of prokaryotic taxa (FAPROTAX) based on the taxonomic profiling results to predict the functions of major resident bacteria. Finally, we used fluorescence in situ hybridization (FISH) to profile the localizations of dominant gill-associated bacteria.
From August 2020 to April 2022, water samples were collected every 1 or 3 months (independent sample number = 10) by SCUBA divers in the vent area (24°50’02.6”N, 121°57’42.8”E; offshore the southeastern tip; 15-17 m depth) and non-vent area (24°50’29.9”N, 121°56’17.1”E; offshore the northwestern tip; 8-10 m depth) of Kueishan Island, Taiwan (Figure 1A). The data from each independently collected water sample was averaged from triplicate samples collected at each sampling time surrounding the hydrothermal vent crabs, but not directly above the vent opening. The crabs X. testudinatus were collected from the vent area (Figures 1B–E). Crabs were randomly selected for hemolymph collection (independent sample number = 9) as well from August 2020 to April 2022, and the hemolymph was carefully drawn from the coxa membrane of the 2nd walking legs (the 3rd pereopods) using a disposable 1 mL syringe with 24G X 1 Inch (0.55x25 mm) (Terumo Medical Corporation, Tokyo, Japan). The hemolymph was immediately used for pH measurements. To collect the bacterial community from X. testudinatus, the 5th gills (N = 29) were sampled, and the carapace surface was applied using cotton swabs (N = 19). The gill tissues and cotton swabs were separately placed into 1.5 mL tubes and stored in liquid nitrogen on the fisheries craft. Upon arriving at the laboratory, samples were frozen at -80°C until DNA extraction.
The water quality was assessed according to temperature, pH, dissolved oxygen (DO), alkalinity, sulfide content and bicarbonate () content. The pH, sulfide and content of crab hemolymph were compared with the corresponding measurements from water samples. The temperatures and pH values of the water samples were measured using a multi-parameter portable meter (Multiline® Multi 3620 IDS, WTW, Weilheim, Germany) equipped with an IDS pH electrode (SenTix® 940, WTW) on the fisheries craft. The crab hemolymph pH was determined using a portable pH meter (Profiline® pH 3310, WTW) equipped with a glass-body micro combination pH electrode (InLab® Micro, Mettler Toledo, Leicester, UK) immediately after hemolymph collection. The water alkalinity was spectrophotometrically assayed following a previously published method (Sarazin et al., 1999). Sulfide content was determined by a spectrophotometric method modified from (Cline, 1969), using a sulfide-sulfur specific mixed diamine reagent containing N, N-Dimethyl-p-phenylenediamine sulfate and ferric chloride in 50% hydrochloric acid. The dissolved inorganic carbon (DIC) content of water samples was analyzed in triplicate using a DIC analyzer (AS-C3, Apollo SciTech, Newark, DE, USA) with nitrogen as an inert gas, followed by quantification via a non-dispersive infra-red (NDIR) Li-7000 analyzer (LI-COR, Lincoln, NE, USA). The hemolymph DIC content was measured following a method described previously (Allen et al., 2021). The concentration of water samples was calculated according to DIC, pH, and temperature using a CO2SYS module undergone within Microsoft Excel (Pierrot et al., 2006). Dissociation constants were as provided and adjusted by two methodological studies (Mehrbach et al., 1973; Dickson and Millero, 1987). Alternatively, the hemolymph concentration was calculated based on DIC and pH using the Henderson–Hasselbalch equation, with dissociation constants and solubility coefficients as previously described (Truchot, 1976).
DNA from the crab gills was extracted using the Wizard Genomic DNA Purification Kit (Promega, Madison, WI, USA), and DNA from the carapace surface (cotton part) was extracted using the QIAamp DNA Microbiome Kit (Qiagen, Hilden, Germany). Both extractions were performed in accordance with the manufacturers’ instructions. The quantity and quality of extracted DNA were verified using a NanoDrop 2000™ spectrophotometer (Thermo Fisher Scientific, Wilmington, DE, USA). Afterward, the DNA samples were stored at -20°C before use.
LoopSeq™ 16S Long Read Kit (Loop Genomics, San Jose, CA, USA) was used to construct the bacterial full-length 16S rRNA gene libraries (V1-V9 region). The constructed DNA libraries were sequenced in a pair-end (2 × 150 bp) configuration on the NextSeq 550 platform (Illumina, San Diego, CA, USA) in accordance with the manufacturer’s instructions.
A Loop Genomics cloud platform (Microsoft Azure) was used to trim Illumina sequencing index and demultiplex the pair-end raw sequences for synthetic long-read reconstruction. The poor-quality reads and chimeric sequences were discarded. The remaining qualified sequences were uploaded to the CLC Genomic Workbench 20.0.1 (CLC Bio, QIAGEN, Aarhus, Denmark) for taxonomic profiling and abundance analysis based on a microbial genome database (2019-08-30, provided by CLC workbench). The total reads number of 16S rRNA gene sequences in each sequenced gill and carapace sample was shown in Table S1 (gill) and Table S2 (carapace). Taxonomic profiling was used to generate the abundance table based on the reads of the 16S rRNA sequence for each taxon. For each taxon, the number of reads in the sample was displayed along with the confidence score. The information was displayed using stacked bar charts. The Alpha diversity indices (Chao 1, Shannon and Simpson) were calculated to assess the heterogeneity of the bacterial community (species). Principal coordinate analysis (PCoA) was performed to determine the dissimilarity of bacterial compositions between the gill and carapace samples, based on the Bray-Curtis distance.
In order to predict the functions of bacteria collected from the gills and carapace surface of X. testudinatus, the taxonomic abundance table was used to retrieve functional annotations of identified prokaryotic taxa (FAPROTAX 1.2.5). FAPROTAX provides an informative database that can be used to sort prokaryotic taxa (e.g., classes, genera, or species) into presumptive functions based on studies of culture representatives (Louca et al., 2016). On the basis of the initial output, predicted functions were further classified into incorporative groups and represented as proportions of total predicted functions for each sample.
FISH was used to detect and locate the bacteria in the gills of X. testudinatus. The crab gills were fixed with 4% paraformaldehyde in phosphate-buffered saline (PBS) solution and temporarily stored in a box filled with ice on the fishing craft. Afterward, the fixed gills were embedded in paraffin wax in the laboratory, and the wax sections (8 μm) were mounted on the slide and stored at 4°C. To perform FISH, dewaxing was performed with Histo-Clear (National Diagnostics, Atlanta, GA), and Histo-Clear was removed using an ethanol series (100%, 75%, 50% and 25%). After rehydrating the section with 20 mM Tris-HCl, it was treated with proteinase K (5 μg/mL) and lysozyme (5 mg/mL) in Tris-HCl to permeabilize the bacterial walls. The gill sections were hybridized with probe Sulfurovum-target EPI653 (5’-ATCTTCCCCTCCCASACTCT-3’) (Watsuji et al., 2010), universal bacteria probe EUB338 (5’-GCTGCCTCCCGTAGGAGT-3’) (Amann et al., 1990), and a customized probe NBC371 (5’-CCAGTTAGCTTCCCCGCTT-3’) designed for Sulfurovum NBC37-1 and based on the 16S rRNA gene sequence retrieved from NCBI (AP009179). Fluorescence images were obtained with an Olympus FV3000 confocal laser scanning microscope (Olympus Corporation, Tokyo, Japan).
Statistical analyses of relative bacteria abundance (species) and predicted function proportions were performed with GraphPad Prism 9.1.2 (GraphPad Software, San Diego, CA, USA), using Welch’s t-test to compare the gill and carapace groups. Statistical analyses of alpha and beta diversity were performed with CLC Genomic Workbench 20.0.1 (CLC Bio, QIAGEN). The difference in alpha diversity indices (Chao 1, Shannon, and Simpson) between the gill and carapace groups was determined using the Kruskal-Wallis test. The Bray-Curtis-based permutational analysis of variance (PERMANOVA) was used to compare the difference in bacterial species compositions between gill and carapace groups, which was visualized by PCoA. The level of significance was set at p< 0.05.
Kueishan Island is located at the end of the Okinawa Trough and possesses a shallow-water HV system on its southeastern tip (vent area; Figures 1A, B). The water around the northwestern tip of Kueishan Island is a non-vent area that contains a coral reef ecosystem and exhibits relatively high biodiversity (Chan et al., 2016). According to our measurements, the environmental parameters of the vent area were more variable than those of the non-vent area (without vent openings). Parameters of water quality were measured in water samples collected from the vent and non-vent areas off Kueishan Island. The temperatures in both areas varied with the season. In the vent areas, water temperatures ranged from 18.6 to 28.3°C, while non-vent areas ranged from 20.2 to 27.9°C (Figure 2A). The dissolved oxygen (DO) ranged from 5.68 to 8.47 mg/L and from 7.16 to 8.68 mg/L in the vent and non-vent areas, respectively (Figure 2B). The alkalinity ranged from 1.56 to 2.43 mM and from 2.16 to 2.51 mM in the vent and non-vent areas, respectively (Figure 2C). Additionally, the pH, sulfide and contents of environmental water were compared to those of crab hemolymph. The pH ranged from 6.19 to 8.12 in the vent area, and from 7.87 to 8.28 in the non-vent area. However, the crabs inhabiting the vent area seemed to maintain their hemolymph pH in a narrow range of 7.49 to 7.78, in spite of environmental pH fluctuations (Figure 2D). The vent and non-ven sulfide contents ranged from 9 to 3480 μM and from 0 to 23 μM, respectively (Figure 2E). Thus, the vent area had a large variation and much elevated sulfide content. In the crab hemolymph, sulfide was detected at a range of 102 to 747 μM (Figure 2E). As shown in Figure 2F, the concentrations in the vent and non-vent areas respectively ranged from 0.76 to 2.03 mM and 1.90 to 2.21 mM. Notably, the hemolymph of crab exhibited a considerably large range of (9.30 to 16.40 mM). An extremely high concentration in the hemolymph is in line with the idea that may be held in reserve by the vent crabs for coping with pH drops in the vent area.
Figure 2 On-site measurement of physicochemical parameters from seawater and vent crab hemolymph. Temperature, dissolved oxygen (DO), and alkalinity of seawater samples collected from the vent and non-vent areas (A–C) were examined. The pH value, sulfide and - concentrations were estimated both in seawater and crab hemolymph samples (D–F). Parameters are presented as truncated violin plots with individual values also shown.
The bacterial compositions were profiled and represented as bar charts in Figure 3A and Table S1, S2. The analysis showed that the gills and carapace surface were both dominated by four classes, including Epsilonproteobacteria, Gammaproteobacteria, Flavobacteriia, and Alphaproteobacteria. Despite sharing overall similar bacterial compositions, there were clear differences in the relative abundances of classes between the gills and carapace. The five most abundant classes in the gills were Epsilonproteobacteria (73.8%), Gammaproteobacteria (9.9%), Flavobacteriia (9.3%), Alphaproteobacteria (3.0%), and Mollicutes (1.5%); the five most abundant classes on the carapace surface were Epsilonproteobacteria (42.2%), Alphaproteobacteria (16.9%), Gammaproteobacteria, Flavobacteriia (10.6%), and Saprospiria (4.7%; Figure S1A). At the genus level, the three most abundant genera in the gills were Sulfurovum (67.0%), Leucothrix (3.1%), and Leeuwenhoekiella (2.7%), while the five most abundant genera on the carapace surface were Sulfurovum (28.1%), Leucothrix (9.5%), Polaribacter (3.5%), Ruegeria (2.6%), and Arcobacter (2.5%; Figure S1B).
Figure 3 Relative abundance and alpha diversity of bacterial species. Box plots and whisker plots (A) illustrate the relative abundance of representative species within the five dominant classes of the gills and carapace surface. Asterisks indicate significant differences between gill and carapace groups (Welch’s t-test; *p< 0.05, ***p< 0.001, ****p< 0.0001; gill, N = 29; carapace, N = 19). The alpha diversity was analyzed at the species level (B) according to Chao 1, Shannon, and Simpson indices (Kruskal-Wallis test, p< 0.05).
Within the five most abundant classes, a few representative species were evaluated in terms of relative abundance. The box and whisker plot shows the mean and variation of each bacterial species (Figure 3A). The relative abundances of Sulfurovum sp. NBC37-1, Leewuwenhoekiella sp. MAR_2009_32, and Ornithobacterium rhinotracheale were significantly higher in the gills (67.0%, 2.7% and 1.3%, respectively) than on the carapace surface (28.0%, 0.1% and 0.3%, respectively; Figures 3A, S2). In contrast, on the carapace, Nitratifactor salsuginis (2.9%), Arcobacter butzleri (2.1%), Leucothrix mucor (9.5%), Hydrogenvibrio sp. Milos-T1 (1.2%), Polaribacter sp. Hel1_33_49 (1.7%), Ruegeria sp. TM1040 (1.6%), and Pseudovibrio sp. FO-BEG1 (2.5%) were more abundant than in the gills (respectively 0.7%, 0.4%, 3.1%, 0.1%, 0.2%, 0.2% and 0.1%; Figure 3A; Figure S2).
Indices of alpha diversity, including Chao 1, Shannon, and Simpson, were obtained to characterize the richness and evenness of gill and carapace samples at the species level. The results showed that the carapace surface had more species (Chao 1) and higher diversity (Shannon and Simpson) compared to the gills (Figure 3B). Since the gills were profoundly dominated by Sulfurovum sp. NBC37-1 (67%), it is entirely reasonable that the bacterial diversity in the gills would be lower than that on the carapace surface. Additionally, according to the PCoA based on the Bray-Curtis distance, the bacterial species compositions of the gills and the carapace surface were significantly different (PERMANOVA, pseudo-F = 31.2942, p = 0.0001; Figure 4A). In the gills of X. testudinatus, a total of 80 genera and 91 species were identified (≥ 0.1% abundance). On the carapace surface, a total of 136 genera and 167 species were identified (≥ 0.1% abundance; Figures 4B, C). The Venn diagram analysis showed that the gills and carapace surface shared 58 genera and 64 species. There were 22 and 78 genera specific to the gills and carapace surface, respectively (Figure 4B). In the gills, the five most abundant specific species were Candidatus Hepatoplasma, Thiofilum, Lacinutrix, Chryseobacterium, and Thiolinea. On the carapace surface, they were Deinococcus, Cytophga, Truepera, Rhodovulum, and Rhodopirellula. In addition, 27 and 103 species were specific to the gills and carapace surface, respectively (Figure 4C). The five most abundant specific species in the gills were Candidatus Hepatoplasma crinochetorum, Thiofilum flexile, Lacinutrix sp. 5H-3-7-4, Thiolinea disciformis, and Methylomicrobium buryatense. On the carapace surface, Deinococcus peraridilitoris, Flavobacteria bacterium MS024-2A, Rhodovulum sulfidophilum, Deinococcus deserti, and Rubinisphaera brasiliensis were the five most abundant species (Figure 4C).
Figure 4 Principal coordinate analysis (PCoA) and Venn diagram of gill and carapace bacterial communities. PCoA was performed at the species level based on the Bray-Curtis distance, revealing the relative dissimilarity in bacterial community structures between the gill and carapace surface (A). The Venn diagram shows the distributions of bacterial genera (B) and species (C). Numbers of identified shared or specific groups are shown. For gills and carapace, the five most abundant specific genera and species are listed.
According to FAPROTAX analysis, several functional groups were predicted and identified based on the bacterial taxa profiles (Figure 5). Only two functional groups, methylotrophy (p = 0.3287) and hydrocarbon degradation (p = 0.3401), were not significantly different between the gill and the carapace samples. In the gills, there were higher proportions of bacteria involved in sulfur oxidation, hydrogen oxidation, and denitrification. On the other hand, the community on the carapace surface had higher functional proportions of sulfur reduction, nitrogen fixation, ammonification, chemoheterotrophy, phototrophy, cellulolysis, iron respiration, fumarate respiration, fermentation, ureolysis, and parasites, pathogens or symbionts. The different bacterial functions present in the gills and the surface of the carapace provide critical information for understanding how bacterial biochemical processes may be involved with the response of the vent crab to the HV environment.
Figure 5 Predicted function proportions based on the functional annotation of prokaryotic taxa (FAPROTAX). The proportions of predicted bacterial functions are shown in the barplot. Values are mean + SD. Asterisks indicate groups that are significantly higher in the carapace than the gill. The hash signs indicate groups that are significantly higher in the gill than in the carapace. (Welch’s t-test; **p< 0.01, ***p< 0.001, ****p< 0.0001, ##p< 0.01, ####p< 0.0001; gill, N = 29; carapace, N = 19).
Using FISH, it was possible to spatially identify Sulfurovum-related bacteria in the gills of X. testudinatus (Figures 6, S3). The signals from Sulfurovum-targeting probe EPI653 almost completely overlapped with those from universal bacteria probe EUB338, indicating that the signals were from bacteria and further supporting our finding that the most abundant genus in gill samples is Sulfurovum (Figure S3). Furthermore, the bacteria labeled with a customized probe designed for Sulfurovum sp. NBC37-1 were widely distributed in the afferent vessels of vent crab gills, and these signals also overlapped with EPI653 signals (Figure 6). Moreover, our microscopy analysis revealed that the Sulfurovum-related bacteria almost occupied the cells within the afferent vessel (Figures 6C–E), and fluorescence signals were also observed in the epithelial principal cells and pilaster cells on the lamella (Figures 6A, B, S3).
Figure 6 Spatial distribution of Sulfurovum sp. NBC37-1 in the gills of X. testudinatus. Fluorescence in situ hybridization (FISH) images show representative Cy5 signals (far-red, Sulfurovum sp. NBC37-1) and Alexa 488 signals (green, Sulfurovum-target probe EPI653) in the transverse sections of gill filaments. Scale bars = 20 μm (A, B) and 5 μm (C–E), respectively. L, lamella; AV, afferent vessel.
The fluids emitted by the shallow-water HVs off Kueishan Island contain high concentrations of sulfide (Fig. 2E), reduced gases and heavy metals (Peng et al., 2011). In addition to affecting the diversity, abundance, biology and ecology of phytoplankton and zooplankton (Tarasov et al., 2005; Mantha et al., 2013), the hydrothermal vent fluids are toxic to most organisms (Dahms et al., 2017; Lebrato et al., 2019). Thus, Kueishan Island HV provides unprecedented opportunities to study responses and adaptations at different levels of biotic integration (biosphere, ecosystem, community, population, cellular, omics-related) in a completely natural extreme environment (Dahms et al., 2018). In the present study, we continually monitored the water quality of the environment for over one year and found that the HV area located off the southeastern tip of the Kueishan Island is a highly dynamic acidic and sulfur-rich environment compared to the northwestern region (the non-vent area). Furthermore, the occurrence of photosynthesis in shallow-water HV systems may result in additional primary productivity, and higher species diversity than that found in deep-sea HV systems (Tarasov et al., 2005). Algae are one of the common species found in the Kueishan Island shallow-water HV system, which may also serve as an important energy source, followed by bacterial chemosynthesis (Chan et al., 2016; Wang et al., 2022). Additionally, 13 species of mollusks inhabit the vent area, the majority of which are grazers that feed on algae and biofilms (Chen et al., 2018a). While a number of studies have suggested that extreme and fluctuating high sulfide environments may limit the species richness of macrofauna in deep-sea environments (Goffredi and Barry, 2002; Sahling et al., 2002); this is not the case for shallow-water HV systems of Kueishan Island. In this regard, sulfur oxidation has been proposed as a major mechanism of sulfide detoxification in species either with or without bacterial symbionts (Zierenberg et al., 2000). Considering the extremely variable conditions, including the gradient of chemical and physical perturbations emanating from the vent to adjacent regions, the symbiosis of macro- and micro-organisms may provide an important platform for understanding the responsiveness of organisms to such gradients underlying interactions between the environment and endemic organisms (Chan et al., 2016).
We analyzed the bacterial compositions in the gills and carapace surface of shallow-water HV crab X. testudinatus. Notably, we identified Epsilonproteobacteria as the dominant class in both gills (73.8%) and carapace (42.2%). According to culture-based characterizations and genetic analyses, Epsilonproteobacteria are associated with the utilization of reduced sulfur, essentially as chemolithoautotrophs (Takai et al., 2003; Inagaki et al., 2004; Takai et al., 2005). Epsilonproteobacteria are also known to be a major class of symbiotic bacteria in other HV endemic creatures, such as yeti crabs (Kiwa hirsute), galatheid crabs (Shinkaia crosnieri) and vent gastropods (Cyathermia naticoide) (Goffredi et al., 2008; Tsuchida et al., 2011; Zbinden et al., 2015). In aquatic animals, the gills serve as an interface between the external environment and internal body fluid, playing primary roles in respiration, osmolyte transport, nitrogenous waste excretion and acid-base regulation (Henry and Wheatly, 1992; Evans et al., 2005). Despite this broad range of functions, few studies have investigated the physiological interactions between animal hosts and symbiotic bacteria in the gills. Sulfurovum, a genus of Epsilonproteobacteria, was especially well represented in the bacterial communities of the gills (67%) and the carapace surface (28%). Furthermore, Sulfurovum were abundantly labeled in the gills of X. testudinatus by FISH. As a SOB, Sulfurovum is likely to oxidize reduced sulfur (sulfide, S2-) into non-toxic forms (elemental sulfur S, sulfite , and sulfate ), mitigating the toxicity of hydrogen sulfide (H2S) in hydrothermal vent fluids (Nelson et al., 1995; Akerman et al., 2013).
We identified a few bacterial classes in X. testudinatus that are also present in the ambient HV environment. These HV-associated classes include Epsilonproteobacteria, Gammaproteobacteria and Alphaproteobacteria, which have been identified in the environmental waters or fluids of either deep-sea (Adams et al., 2011; Yamamoto and Takai, 2011) or shallow-water HV systems (Giovannelli et al., 2013; Yang et al., 2016). In the sulfur-rich environment, the most abundant class was Epsilonproteobacteria, a biologically and geologically significant bacterial class (Campbell et al., 2006). In line with these findings, Epsilonproteobacteria were also found both in the surrounding water and sediments of the shallow-water HV system located off the coast of Panarea Island in Italy (Maugeri et al., 2009). Phylogenic analysis also revealed a large Sulfurovum-related cluster in the shallow-water HV fields of Milos Island in Greece (Giovannelli et al., 2013). Similar results were reported for water and sediment samples collected from the Kueishan Island vent area, which were dominated by Sulfurovum (Wang et al., 2015; Yang et al., 2016; Wang et al., 2017). According to stable isotope analysis, the bacteria samples from the vent crab’s carapace and the surrounding water did not differ in 13C and 15N levels, which might be potentially cognate (Wang et al., 2022). Additionally, plenty of white-colored biofilms are growing on the biotic (crab carapace, gastropod shell, algae) and abiotic surfaces (sediment, rock, or artificial fragment) in the underwater environments of Kueishan Island, which are usually fed by HV metazoans, including X. testudinatus. Since the vent crab is a benthic crustacean, its bacterial community on the carapace surface could be decided by the environments, particularly sulfur-depositing sediments. The Kueishan Island HV system is a vent area exposed to the intermittent discharge of vent fluids. We found extremely high concentrations of sulfide in the vent area, with dynamically dropping DO concentrations and a great accumulation of sulfide in the vent crab’s hemolymph, which is also observed in marine soft sediments-inhabited priapulid worms (Halicryptus spinulosus) under anoxic conditions (Oeschger and Vetter, 1992). Thus, it is reasonable that a facultative symbiont of Epsilonproteobacteria-related chemoautotrophic SOB Sulfurovum and a sulfur-resistant species X. testudinatus would be found in such sulfidic extreme environment.
In our surveys, we identified the dominant bacteria species as Sulfurovum sp. NBC37-1 in both the gills and carapace samples. This strain was previously isolated from the Iheya North field and had 99.1% similarity (16S rRNA) with a strict SOB (Sulfurovum lithotrophicum 42BKT) that was also isolated from the Okinawa Trough (Nakagawa et al., 2005a; Nakagawa et al., 2007). The high functional proportions of sulfur oxidation in our study were mainly attributable to the predominance of Sulfurovum sp. NBC37-1. According to our FISH results, the presence of Sulfurovum sp. NBC37-1 within the afferent vessel, epithelial principal cells and pilaster cells in the gills suggests an endosymbiont relationship between the SOB and the vent crab. The widespread occurrence of the SOB in X. testudinatus gills is reminiscent of findings made in the gills of vesicomyid clams (Cruaud et al., 2019), mytilid mussels (Halary et al., 2008; Szafranski et al., 2015; Duperron et al., 2016), and bresiliid shrimps (Tokuda et al., 2008) from hydrothermal vent habitats. Since the gills serve as a boundary between an aquatic animal’s internal organs and the external environment, these tissues appear to be an enclave for SOBs to carry out sulfide detoxification and organic production for the hosts (Felbeck and Somero, 1982). Likewise, the high functional proportion of hydrogen (H2) oxidation in the gills was probably due to Sulfurovum sp. NBC37-1 with hydrogenase (Nakagawa et al., 2007). It was previously reported that H2 can also serve as an energy source in hydrothermal vent systems by powering chemosynthetic symbioses (Petersen et al., 2011). In the denitrification process, Sulfurovum may use nitrate () as an electron acceptor during sulfur oxidation. Then, Nitratifractor salsuginis can transfer electrons from H2 into to generate nitrogen (N2) (Inagaki et al., 2004; Nakagawa et al., 2005b; Vetriani et al., 2014). Intriguingly, the amino acid sequences of nitrate reductase (NapA) retrieved from a diffuse-flow HV area on the East Pacific Rise were related to Sulfurovum sp. NBC37-1 (44%), Arcobacter butzleri (14%) and N. salsuginis (7%), suggesting that these species may be involved in denitrification or ammonification (Smith et al., 2007; Vetriani et al., 2014). Notably, sulfur reduction and fumarate respiration were more active on the vent crab carapace surface, which had higher relative abundances of Wolinella succinogenes (another Epsilonproteobacterium). Previous studies demonstrated that W. succinogenes could oxidize H2S or bisulfide (HS-) coupled with fumarate respiration to form polysulfide, which is then reduced by polysulfide reductase in a periplasmic sulfur reduction process (Macy et al., 1986; Krafft et al., 1995; Hedderich et al., 1998; Kroger et al., 2002). Moreover, W. succinogenes contains a periplasmic NapA and a cytochrome c3 that are respectively involved in nitrate and nitrite reduction as an ammonification strategy, the functional proportion of which was higher on the carapace surface of the vent crab (Bokranz et al., 1983; Blackmore et al., 1986; Lovley et al., 1999; Simon, 2002; Simon et al., 2003; Strohm et al., 2007).
Among Gammaproteobacteria, Leucothrix mucor was the dominant species, as it had a higher relative abundance on the carapace surface than in the gills. Previous studies have shown that the presence of L. mucor on the surfaces of marine algae, fish eggs and aquatic invertebrates may occur as an infestation (Johnson et al., 1971; Payne et al., 2007). This filamentous heterotrophic bacterium is also known to be a sulfur oxidizer that utilizes reduced sulfur compounds as energy sources (Grabovich et al., 1999). The Gammaproteobacteria-related symbionts of vent shrimps (R. exoculate) were also dominated by L. mucor (Petersen et al., 2010). Another Gammaproteobacterium, Psychromonas ingrahamii was also more abundant on the vent crab carapace. Genomics analysis of P. ingrahamii revealed several reductases for nitrate, nitrite, sulfite, fumarate and fermentation-related enzymes, while culture studies further demonstrated that P. ingrahamii is a psychrophilic extremophile with the ability to perform nitrate reduction, fumarate utilization and fermentation (Auman et al., 2006; Riley et al., 2008; Markowitz et al., 2012). However, P. ingrahamii have mostly been isolated or identified from low-temperature marine environments, such as sea ice and polar sediments (Breezee et al., 2004; Auman et al., 2006; Canion et al., 2013). To the best of our knowledge, this is the first study to identify P. ingrahamii in a hydrothermal HV system. The Hydrogenvibrio sp. Milos-T1, which also had a higher abundance on the carapace, was previously isolated from a shallow-water HV system near the island of Milos in Greece and was closely related to Hydrogenovibrio marinus, an extremophilic hydrogen-oxidizing bacterium (Nishihara et al., 1997; Brinkhoff et al., 1999). In addition to hydrogen, H. marinus utilizes reduced sulfur compounds as electron donors, and it uses ammonium and urea as sole nitrogen sources, but not N2, nitrate or nitrite (Nishihara et al., 1991). The fact that ureolysis is performed much more actively on the carapace may be associated with a higher abundance of Hydrogenvibrio sp. Milos-T1.
Among Alphaproteobacteria, two species were identified. Pseudovibrio sp. FO-BEG1 and Ruegeria sp. TM1040, both of which were more abundant on the carapace. It was precisely the same that Pseudovibrio sp. FO-BEG1 was shown to be capable of sulfur oxidation, denitrification and fermentation as Pseudovibrio denitrificans, a representative species of the genus (Shieh et al., 2004; Romano, 2018). There is evidence that Psedovibrio-related bacteria may exhibit symbiotic relationships with several invertebrates, such as corals, sponges and tunicates (Fukunaga et al., 2006; Riesenfeld et al., 2008; Rypien et al., 2010; Santos et al., 2010; Penesyan et al., 2011). Nevertheless, this appears to be the first study to identify Psedovibrio in a crustacean. In the case of Ruegeria sp. TM1040 (formerly Silicibacter sp.), which is typically found in the phycosphere or on the surface of dinoflagellates, genomic analysis showed no relevant genes for sulfur oxidation, denitrification or ammonification, but dimethulsulfonioproponate (DMSP) demethylase was shown to metabolize DMSP which is produced by dinoflagellates and comprises a major source of organic sulfur in the ocean (Moran et al., 2007; Belas et al., 2009).
Flavobacteriia are known as polymer degraders and are found in a wide range of environments, including seawater and sediments (Alonso et al., 2007; Kappelmann et al., 2019). The relative abundances of Leeuwenhoekiella sp. MAR_2009_132 and Ornithobacterium rhinotracheale were both higher in the gills of X. testudinatus than on the carapace surface. Among these two constituents, Leeuwenhoekiella sp. MAR_2009_132 was previously isolated near the Island Sylt in the North Sea and was closely related to Leeuwenhoekiella aequorea (Hahnke and Harder, 2013; Chen et al., 2018b). Surprisingly, O. rhinotracheale is generally known as a species that causes respiratory disease in poultry (Barbosa et al., 2019). In our literature searches, we found no studies on this species related to marine organisms. Only two studies described the detection of O. rhinotracheale in seawater samples, but each provided limited information (Valenzuela-Gonzalez et al., 2016; Porchas-Cornejo et al., 2017). On the other hand, Polaribacter spp. and Tenacibaculum maritimum were more abundant on vent crab’s carapace. Polaribacter sp. Hel_33_49 and Hel_I_88 (also named Hel1_88) were first isolated from the North Sea during a spring bloom and were reported to feed on polysaccharides and proteins with carbohydrate-active enzymes (CAZymes) and peptidases (Zerillo et al., 2013; Xing et al., 2015). In the case of T. maritimum, a prominent pathogen without known host specificity, genomic analysis revealed similar metabolism preferences (saccharide and protein degradation) to those seen in other Flavobacteriia-related bacteria, such as Polaribacter spp. (Fukunaga et al., 2006; Perez-Pascual et al., 2017). The general consensus is that Flavobacteriia subsist on abundant organic matter in the epipelagic zone, which may explain why deep-sea HV systems usually show no or very low proportions of Flavobacteriia-related bacteria in contrast to the shallow-water HV system of Kueishan Island (Goffredi, 2010; Salazar et al., 2016; Cerqueira et al., 2017; Leinberger et al., 2022). Nevertheless, Flavobacteriia were not abundant in other shallow-water HV systems (Rajasabapathy et al., 2018). Since Kueishan Island lies to the southern rifting end of the Okinawa Trough (a back-arc depression of the Ryukyu arc-trench system), the active volcanic features cause unstable hydrothermal activities to occur in the geologically “young” vent system (Konstantinou et al., 2013; Deffontaines et al., 2022). The fluids spilling out of the vents around this young volcanic island aperiodically alter the surrounding water chemistry beneath the sea surface. In addition, frequent weather and current disturbances of typhoons, monsoons and the Kuroshio may cause strong turbulent mixing in Kueishan Island HV system (Liang et al., 2003; Lebrato et al., 2019). These climate features further contribute to the establishment of the marine ecosystem near Kueishan Island, off the northeastern coast of Taiwan. The combination of climate, status as a neritic zone (providing sufficient organic matters) and presence of an extreme HV system (emitting minerals, sulfur compounds, high temperature and low pH) make Kueishan Island a truly unique natural laboratory.
HV systems have taken a place in many critical biogeochemical processes that occur in the modern ocean (Tarasov et al., 2005) and were demonstrated to be an oasis of life in the deep sea, as the area is nourished by chemoautotrophic bacteria. Several known marine chemoautotrophic bacteria utilize sulfide and other reduced sulfur compounds for carbon fixation in food-limited and sulfide-rich deep-sea HV systems. Until recently, most studies on the sulfur detoxification mechanisms in marine species have been focused on those species inhabiting deep-sea HV systems of the mid-ocean ridge (Zierenberg et al., 2000; Zhang et al., 2017). Due to the limited accessibility and difficulty of keeping deep-sea organisms, such mechanisms on a holobiont cannot be practically studied in the deep-sea HV system. Thus, X. testudinatus crustacean holobiont residing in the shallow-water HV system at the south end of the Okinawa Trough, Kueishan Island serves as a unique experimental proxy.
HV areas are comprised of submarine fissures at the planet’s surface from which geothermally heated water is expelled. These areas are often characterized by variable and extreme physicochemical conditions, as well as the presence of microorganisms that can utilize and supply energy through sulfur-oxidizing processes. Due to the relatively low cost and ease of access to this shallow-water HV system, we are able to carry out a long-term monitoring with frequent observations rather than relying on snapshot estimates. Moreover, our current datasets reveal the bacterial community structure in the gills and the carapace surface of the vent crab X. testudinatus. These datasets are derived from the combination of field-based experiments and omic-based approaches, revealing the chemolithotrophic SOB Sulfurovum sp. NBC37-1 (an Epsilonproteobacterium) as the most dominant bacteria. Additionally, symbiotic processes involving hydrogen oxidation are also expected to occur in the wake of substrate respiration and reduction (e.g., fumarate and nitrogen oxides), thus supplying energy to metazoans in the sulfidic environments. This study provides profound insights into the mechanisms of bacteria-crustacean symbiosis from the vent ecosystem and demonstrates the significance of bacteria in marine holobionts with implications for the evolution of inter-kingdom symbiosis in Kueishan Island shallow-water HV system, one of the planet’s most extreme environments.
The datasets in this study are deposited in the NCBI Sequence Read Archive (SRA). The 16S rRNA metagenome sequences from each tissue sample can be found according to accession number PRJNA858606 (https://www.ncbi.nlm.nih.gov/bioproject/PRJNA858606).
LC, M-CW, S-HY and Y-CT conceived and designed the study, and carried out the analysis. LC and M-CW performed animals collection and biochemical experiments. K-YT, LC and M-CW carried out the functional annotation analysis. LC performed the FISH image acquisition. LC, M-CW, C-LW, H-TL, S-HY and Y-CT wrote the first draft of the paper, and all co-authors commented on and approved the manuscript. All authors contributed to the article and approved the submitted version.
This study was financially supported by the grants from the National Science and Technology Council, Taiwan, Republic of China (MOST 111-2621-M-019-003).
The authors would like to thank Captain Lan for the sample collection on the fisheries craft, and Cheuk-King Johny Leung for the bacteria sampling of the vent crabs. The authors also express appreciation to the Medical Microbiota Center of the First Core Laboratory, National Taiwan University College of Medicine, for technical support with synthetic long-read 16S rRNA gene sequencing.
The authors declare that the research was conducted in the absence of any commercial or financial relationships that could be construed as a potential conflict of interest.
The reviewer BC declared a shared affiliation with the authors LC and M-CW to the handling editor at the time of review
All claims expressed in this article are solely those of the authors and do not necessarily represent those of their affiliated organizations, or those of the publisher, the editors and the reviewers. Any product that may be evaluated in this article, or claim that may be made by its manufacturer, is not guaranteed or endorsed by the publisher.
The Supplementary Material for this article can be found online at: https://www.frontiersin.org/articles/10.3389/fmars.2022.976255/full#supplementary-material
Adams D. K., McGillicuddy D. J. Jr., Zamudio L., Thurnherr A. M., Liang X., Rouxel O., et al. (2011). Surface-generated mesoscale eddies transport deep-sea products from hydrothermal vents. Science 332 (6029), 580–583. doi: 10.1126/science.1201066
Akerman N. H., Butterfield D. A., Huber J. A. (2013). Phylogenetic diversity and functional gene patterns of sulfur-oxidizing subseafloor Epsilonproteobacteria in diffuse hydrothermal vent fluids. Front. Microbiol. 4. doi: 10.3389/fmicb.2013.00185
Alfaro-Lucas J. M., Pradillon F., Zeppilli D., Michel L., Martinez-Arbizu P., Tanaka H., et al. (2020). High environmental stress and productivity increase functional diversity along a deep-sea hydrothermal vent gradient. Ecology 101 (11), e03144. doi: 10.1002/ecy.3144
Allen G. J. P., Kuan P. L., Tseng Y. C., Hwang P. P., Quijada-Rodriguez A. R., Weihrauch D. (2020). Specialized adaptations allow vent-endemic crabs (Xenograpsus testudinatus) to thrive under extreme environmental hypercapnia. Sci. Rep. 10 (1), 1–13. doi: 10.1038/s41598-020-68656-1
Allen G. J. P., Wang M. C., Tseng Y. C., Weihrauch D. (2021). Effects of emersion on acid–base regulation, osmoregulation, and nitrogen physiology in the semi-terrestrial mangrove crab, Helice formosensis. J. Comp. Physiol. B 191 (3), 455–468. doi: 10.1007/s00360-021-01354-0
Alonso C., Warnecke F., Amann R., Pernthaler J. (2007). High local and global diversity of flavobacteria in marine plankton. Environ. Microbiol. 9 (5), 1253–1266. doi: 10.1111/j.1462-2920.2007.01244.x
Amann R. I., Binder B. J., Olson R. J., Chisholm S. W., Devereux R., Stahl D. A. (1990). Combination of 16S rRNA-targeted oligonucleotide probes with flow cytometry for analyzing mixed microbial populations. Appl. Environ. Microbiol. 56 (6), 1919–1925. doi: 10.1128/aem.56.6.1919-1925.1990
Auman A. J., Breezee J. L., Gosink J. J., Kampfer P., Staley J. T. (2006). Psychromonas ingrahamii sp. nov., a novel gas vacuolate, psychrophilic bacterium isolated from Arctic polar sea ice. Int. J. Syst. Evol. Microbiol. 56 (5), 1001–1007. doi: 10.1099/ijs.0.64068-0
Barbosa E. V., Cardoso C. V., Silva R. D. C. F., Cerqueira A. D. M. F., Liberal M. H. T., Castro H. C. (2019). Ornithobacterium rhinotracheale: An update review about an emerging poultry pathogen. Vet. Sci. 7 (1), 3. doi: 10.3390/vetsci7010003
Belas R., Horikawa E., Aizawa S., Suvanasuthi R. (2009). Genetic determinants of silicibacter sp. TM1040 motility. J. Bacteriol. 191 (14), 4502–4512. doi: 10.1128/JB.00429-09
Blackmore R., Roberton A. M., Brittain T. (1986). The purification and some equilibrium properties of the nitrite reductase of the bacterium Wolinella succinogenes. Biochem. J. 233 (2), 547–552. doi: 10.1042/bj2330547
Bokranz M., Katz J., Schröder I., Roberton A. M., Kröger A. (1983). Energy metabolism and biosynthesis of Vibrio succinogenes growing with nitrate or nitrite as terminal electron acceptor. Arch. Microbiol. 135 (1), 36–41. doi: 10.1007/BF00419479
Breezee J., Cady N., Staley J. T. (2004). Subfreezing growth of the sea ice bacterium ‘‘Psychromonas ingrahamii”. Microb. Ecol. 47 (3), 300–304. doi: 10.1007/s00248-003-1040-9
Brinkhoff T., Sievert S. M., Kuever J., Muyzer G. (1999). Distribution and diversity of sulfur-oxidizing thiomicrospira spp. at a shallow-water hydrothermal vent in the Aegean Sea (Milos, Greece). Appl. Environ. Microbiol. 65 (9), 3843–3849. doi: 10.1128/aem.65.9.3843-3849.1999
Butterfield D. A., Massoth G. J., Mcduff R. E., Lupton J. E., Lilley M. D. (1990). Geochemistry of hydrothermal fluids from axial seamount hydrothermal emissions study vent field, Juan de fuca ridge: Subseafloor boiling and subsequent fluid-rock interaction. J. Geophys. Res. Solid Earth 95 (B8), 12895–12921. doi: 10.1029/JB095iB08p12895
Butterfield D. A., Nakamura K., Takano B., Lilley M. D., Lupton J. E., Resing J. A., et al. (2011). High SO2 flux, sulfur accumulation, and gas fractionation at an erupting submarine volcano. Geology 39 (9), 803–806. doi: 10.1130/G31901.1
Campbell B. J., Engel A. S., Porter M. L., Takai K. (2006). The versatile epsilon-proteobacteria: Key players in sulphidic habitats. Nat. Rev. Microbiol. 4 (6), 458–468. doi: 10.1038/nrmicro1414
Canion A., Prakash O., Green S. J., Jahnke L., Kuypers M. M., Kostka J. E. (2013). Isolation and physiological characterization of psychrophilic denitrifying bacteria from permanently cold Arctic fjord sediments (Svalbard, Norway). Environ. Microbiol. 15 (5), 1606–1618. doi: 10.1111/1462-2920.12110
Cerqueira T., Pinho D., Froufe H., Santos R. S., Bettencourt R., Egas C. (2017). Sediment microbial diversity of three deep-sea hydrothermal vents southwest of the Azores. Microb. Ecol. 74 (2), 332–349. doi: 10.1007/s00248-017-0943-9
Chan B. K. K., Wang T.-W., Chen P.-C., Lin C.-W., Chan T.-Y., Tsang L.-M. (2016). Community structure of macrobiota and environmental parameters in shallow water hydrothermal vents off kueishan island, Taiwan. PloS One 11 (2), e0148675. doi: 10.1371/journal.pone.0148675
Chen C., Chan T. Y., Chan B. K. K. (2018a). Molluscan diversity in shallow water hydrothermal vents off kueishan island, Taiwan. Mar. Biodivers. 48 (1), 709–714. doi: 10.1007/s12526-017-0804-2
Chen J., Robb C. S., Unfried F., Kappelmann L., Markert S., Song T., et al. (2018b). Alpha- and beta-mannan utilization by marine Bacteroidetes. Environ. Microbiol. 20 (11), 4127–4140. doi: 10.1111/1462-2920.14414
Chen C. T. A., Zeng Z. G., Kuo F.-W., Yang T. Y. F., Wang B.-J., Tu Y.-Y. (2005). Tide-influenced acidic hydrothermal system offshore NE Taiwan. Chem. Geol. 224 (1-3), 69–81. doi: 10.1016/j.chemgeo.2005.07.022
Cline J. D. (1969). Spectrophotometric determination of hydrogen sulfide in natural waters. Limnol. Oceanogr. 14 (3), 454–458. doi: 10.4319/lo.1969.14.3.0454
Compere P., Martinez A. S., Charmantier-Daures M., Toullec J. Y., Goffinet G., Gaill F. (2002). Does sulphide detoxication occur in the gills of the hydrothermal vent shrimp, Rimicaris exoculata? C. R. Biol. 325 (5), 591–596. doi: 10.1016/S1631-0691(02)01466-X
Cruaud P., Decker C., Olu K., Arnaud-Haond S., Papot C., Le Baut J., et al. (2019). Ecophysiological differences between vesicomyid species and metabolic capabilities of their symbionts influence distribution patterns of the deep-sea clams. Mar. Ecol. 40 (3), e12541. doi: 10.1111/maec.12541
Cuellar-Gempeler C., Leibold M. A. (2019). Key colonist pools and habitat filters mediate the composition of fiddler crab-associated bacterial communities. Ecology 100 (4), e02628. doi: 10.1002/ecy.2628
Dahms H.-U., Schizas N. V., James R. A., Wang L., Hwang J.-S. (2018). Marine hydrothermal vents as templates for global change scenarios. Hydrobiologia 818 (1), 1–10. doi: 10.1007/s10750-018-3598-8
Dahms H.-U., Tseng L.-C., Hwang J.-S. (2017). Are vent crab behavioral preferences adaptations for habitat choice? PloS One 12 (9), e0182649. doi: 10.1371/journal.pone.0182649
Deffontaines B., Chang K. J., Huang P. C., Hsu H. H., Hsu S. K., Liu C. S., et al. (2022). Neotectonics of the volcanic kuei-shan Tao island, and geodynamic implications (NE Taiwan-SW Okinawa trough). Tectonophysics 832, 229362. doi: 10.1016/j.tecto.2022.229362
Dickson A. G., Millero F. J. (1987). A comparison of the equilibrium constants for the dissociation of carbonic acid in seawater media. Deep-Sea Res. I: Oceanogr. Res. Pap. 34 (10), 1733–1743. doi: 10.1016/0198-0149(87)90021-5
Duperron S., Quiles A., Szafranski K. M., Leger N., Shillito B. (2016). Estimating symbiont abundances and gill surface areas in specimens of the hydrothermal vent mussel Bathymodiolus puteoserpentis maintained in pressure vessels. Front. Mar. Sci. 3. doi: 10.3389/fmars.2016.00016
Elderfield H., Schultz A. (1996). Mid-ocean ridge hydrothermal fluxes and the chemical composition of the ocean. Annu. Rev. Earth Planet. Sci. 24, 191–224. doi: 10.1146/annurev.earth.24.1.191
Evans D. H., Piermarini P. M., Choe K. P. (2005). The multifunctional fish gill: dominant site of gas exchange, osmoregulation, acid-base regulation, and excretion of nitrogenous waste. Physiol. Rev. 85 (1), 97–177. doi: 10.1152/physrev.00050.2003
Farre M., Lombarte A., Tuset V. M., Abello P. (2021). Shape matters: Relevance of carapace for brachyuran crab invaders. Biol. Invasions 23 (2), 461–475. doi: 10.1007/s10530-020-02378-3
Felbeck H., Somero G. N. (1982). Primary production in deep-sea hydrothermal vent organisms: Roles of sulfide-oxidizing bacteria. Trends Biochem. Sci. 7 (6), 201–204. doi: 10.1016/0968-0004(82)90088-3
Fialamedioni A., Metivier C., Herry A., Lepennec M. (1986). Ultrastructure of the gill of the hydrothermal-vent mytilid bathymodiolus sp. Mar. Biol. 92 (1), 65–72. doi: 10.1007/Bf00392747
Fisher C. R., Takai K., Le Bris N. (2007). Hydrothermal vent ecosystems. Oceanography 20 (1), 14–23. doi: 10.5670/oceanog.2007.75
Fukunaga Y., Kurahashi M., Tanaka K., Yanagi K., Yokota A., Harayama S. (2006). Pseudovibrio ascidiaceicola sp. nov., isolated from ascidians (sea squirts). Int. J. Syst. Evol. Microbiol. 56 (2), 923–923. doi: 10.1099/00207713-56-4-923
Giovannelli D., d'Errico G., Manini E., Yakimov M., Vetriani C. (2013). Diversity and phylogenetic analyses of bacteria from a shallow-water hydrothermal vent in milos island (Greece). Front. Microbiol. 4. doi: 10.3389/fmicb.2013.00184
Glowka L. (2003). Putting marine scientific research on a sustainable footing at hydrothermal vents. Mar. Policy 27 (4), 303–312. doi: 10.1016/S0308-597x(03)00042-3
Goffredi S. K. (2010). Indigenous ectosymbiotic bacteria associated with diverse hydrothermal vent invertebrates. Environ. Microbiol. Rep. 2 (4), 479–488. doi: 10.1111/j.1758-2229.2010.00136.x
Goffredi S. K., Barry J. P. (2002). Species-specific variation in sulfide physiology between closely related vesicomyid clams. Mar. Ecol. Prog. Ser. 225, 227–238. doi: 10.3354/meps225227
Goffredi S. K., Jones W. J., Erhlich H., Springer A., Vrijenhoek R. C. (2008). Epibiotic bacteria associated with the recently discovered yeti crab, Kiwa hirsuta. Environ. Microbiol. 10 (10), 2623–2634. doi: 10.1111/j.1462-2920.2008.01684.x
Gonzalez F. J., Rincon-Tomas B., Somoza L., Santofimia E., Medialdea T., Madureira P., et al. (2020). Low-temperature, shallow-water hydrothermal vent mineralization following the recent submarine eruption of tagoro volcano (El hierro, canary islands). Mar. Geol. 430, 106333. doi: 10.1016/j.margeo.2020.106333
Grabovich M. Y., Muntyan M. S., Lebedeva V. Y., Ustiyan V. S., Dubinina G. A. (1999). Lithoheterotrophic growth and electron transfer chain components of the filamentous gliding bacterium leucothrix mucor DSM 2157 during oxidation of sulfur compounds. FEMS Microbiol. Lett. 178 (1), 155–161. doi: 10.1111/j.1574-6968.1999.tb13772.x
Gugliandolo C., Maugeri T. L. (1993). Chemolithotrophic, sulfur-oxidizing bacteria from a marine, shallow hydrothermal vent of vulcano (Italy). Geomicrobiol. J. 11 (2), 109–120. doi: 10.1080/01490459309377939
Gurunathan R., Huang B., Ponnusamy V. K., Hwang J.-S., Dahms H.-U. (2021). Novel recombinant keratin degrading subtilisin like serine alkaline protease from Bacillus cereus isolated from marine hydrothermal vent crabs. Sci. Rep. 11 (1), 1–14. doi: 10.1038/s41598-021-90375-4
Hahnke R. L., Harder J. (2013). Phylogenetic diversity of Flavobacteria isolated from the north Sea on solid media. Syst. Appl. Microbiol. 36 (7), 497–504. doi: 10.1016/j.syapm.2013.06.006
Halary S., Riou V., Gaill F., Boudier T., Duperron S. (2008). 3D FISH for the quantification of methane- and sulphur-oxidizing endosymbionts in bacteriocytes of the hydrothermal vent mussel Bathymodiolus azoricus. ISME J. 2 (3), 284–292. doi: 10.1038/ismej.2008.3
Han C. H., Ye Y., Pan Y. W., Qin H. W., Wu G. H., Chen C. T. A. (2014). Spatial distribution pattern of seafloor hydrothermal vents to the southeastern kueishan Tao offshore Taiwan island. Acta Oceanol. Sin. 33 (4), 37–44. doi: 10.1007/s13131-014-0405-x
Hedderich R., Klimmek O., Kröger A., Dirmeier R., Keller M., Stetter K. O. (1998). Anaerobic respiration with elemental sulfur and with disulfides. FEMS Microbiol. Rev. 22 (5), 353–381. doi: 10.1111/j.1574-6976.1998.tb00376.x
Henry R. P., Wheatly M. G. (1992). Interaction of respiration, ion regulation, and acid-base balance in the everyday life of aquatic crustaceans. Am. Zoologist 32 (3), 407–416. doi: 10.1093/icb/32.3.407
Ho T. W., Hwang J. S., Cheung M. K., Kwan H. S., Wong C. K. (2015). Dietary analysis on the shallow-water hydrothermal vent crab Xenograpsus testudinatus using illumina sequencing. Mar. Biol. 162 (9), 1787–1798. doi: 10.1007/s00227-015-2711-z
Hu M. Y., Guh Y.-J., Shao Y.-T., Kuan P.-L., Chen G.-L., Lee J.-R., et al. (2016). Strong ion regulatory abilities enable the crab Xenograpsus testudinatus to inhabit highly acidified marine vent systems. Front. Physiol. 7. doi: 10.3389/fphys.2016.00014
Hu M. Y. A., Hagen W., Jeng M. S., Saborowski R. (2012). Metabolic energy demand and food utilization of the hydrothermal vent crab Xenograpsus testudinatus (Crustacea: Brachyura). Aquat. Biol. 15 (1), 11–25. doi: 10.3354/ab00396
Inagaki F., Takai K., Nealson K. H., Horikoshi K. (2004). Sulfurovum lithotrophicum gen. nov., sp nov., a novel sulfur-oxidizing chemolithoautotroph within the epsilon-proteobacteria isolated from Okinawa trough hydrothermal sediments. Int. J. Syst. Evol. Microbiol. 54 (5), 1477–1482. doi: 10.1099/ijs.0.03042-0
Jan C., Petersen J. M., Werner J., Teeling H., Huang S. X., Glockner F. O., et al. (2014). The gill chamber epibiosis of deep-sea shrimp Rimicaris exoculata: An in-depth metagenomic investigation and discovery of zetaproteobacteria. Environ. Microbiol. 16 (9), 2723–2738. doi: 10.1111/1462-2920.12406
Jeng M. S., Ng N. K., Ng P. K. L. (2004). Feeding behaviour: Hydrothermal vent crabs feast on sea 'snow'. Nature 432 (7020), 969. doi: 10.1038/432969a
Johnson P. W., Sieburth J. M., Sastry A., Arnold C. R., Doty M. S. (1971). Leucothrix mucor infestation of benthic crustacea, fish eggs, and tropical algae. Limnol. Oceanogr. 16 (6), 962–969. doi: 10.4319/lo.1971.16.6.0962
Jouin C., Gaill F. (1990). Gills of hydrothermal vent annelids: Structure, ultrastructure and functional implications in two alvinellid species. Prog. Oceanogr. 24 (1-4), 59–69. doi: 10.1016/0079-6611(90)90019-X
Kappelmann L., Kruger K., Hehemann J. H., Harder J., Markert S., Unfried F., et al. (2019). Polysaccharide utilization loci of north Sea Flavobacteriia as basis for using SusC/D-protein expression for predicting major phytoplankton glycans. ISME J. 13 (1), 76–91. doi: 10.1038/s41396-018-0242-6
Konstantinou K. I., Pan C. Y., Lin C. H. (2013). Microearthquake activity around kueishantao island, offshore northeastern Taiwan: Insights into the volcano-tectonic interactions at the tip of the southern Okinawa trough. Tectonophysics 593, 20–32. doi: 10.1016/j.tecto.2013.02.019
Krafft T., Gross R., Kröger A. (1995). The function of Wolinella succinogenes psr genes in electron transport with polysulphide as the terminal electron acceptor. Eur. J. Biochem. 230 (2), 601–606. doi: 10.1111/j.1432-1033.1995.0601h.x
Kroger A., Biel S., Simon J., Gross R., Unden G., Lancaster C. R. (2002). Fumarate respiration of Wolinella succinogenes: Enzymology, energetics and coupling mechanism. Biochim. Biophys. Acta 1553 (1-2), 23–38. doi: 10.1016/s0005-2728(01)00234-1
Kuo J., Shibuno T., Kanamoto Z., Noro T. (2001). Halophila ovalis (R. br.) hook. f. from a submarine hot spring in southern Japan. Aquat. Bot. 70 (4), 329–335. doi: 10.1016/S0304-3770(01)00162-0
Lebrato M., Wang Y. V., Tseng L. C., Achterberg E. P., Chen X. G., Molinero J. C., et al. (2019). Earthquake and typhoon trigger unprecedented transient shifts in shallow hydrothermal vents biogeochemistry. Sci. Rep. 9 (1), 1–14. doi: 10.1038/s41598-019-53314-y
Leinberger J., Milke F., Christodoulou M., Poehlein A., Caraveo-Patino J., Teske A., et al. (2022). Microbial epibiotic community of the deep-sea galatheid squat lobster Munidopsis alvisca. Sci. Rep. 12 (1), 2675. doi: 10.1038/s41598-022-06666-x
Liang W. D., Tang T. Y., Yang Y. J., Ko M. T., Chuang W. S. (2003). Upper-ocean currents around Taiwan. Deep-Sea Res. Part II Top. Stud. Oceanogr. 50 (6-7), 1085–1105. doi: 10.1016/S0967-0645(03)00011-0
Lin Y. S., Lui H. K., Lee J., Chen C. T. A., Burr G. S., Chou W. C., et al. (2019). Fates of vent CO2 and its impact on carbonate chemistry in the shallow-water hydrothermal field offshore kueishantao islet, NE Taiwan. Mar. Chem. 210, 1–12. doi: 10.1016/j.marchem.2019.02.002
Little C. T., Vrijenhoek R. C. (2003). Are hydrothermal vent animals living fossils? Trends Ecol. Evol. 18 (11), 582–588. doi: 10.1016/j.tree.2003.08.009
Lonsdale P. (1977). Structural geomorphology of a fast-spreading rise crest: the East pacific rise near 3°25'S. Mar. Geophys. Res. 3 (3), 251–293. doi: 10.1007/BF00285656
Louca S., Parfrey L. W., Doebeli M. (2016). Decoupling function and taxonomy in the global ocean microbiome. Science 353 (6305), 1272–1277. doi: 10.1126/science.aaf4507
Lovley D. R., Fraga J. L., Coates J. D., Blunt-Harris E. L. (1999). Humics as an electron donor for anaerobic respiration. Environ. Microbiol. 1 (1), 89–98. doi: 10.1046/j.1462-2920.1999.00009.x
Macy J. M., Schröder I., Thauer R. K., Kröger A. (1986). Growth the Wolinella succinogenes on H2S plus fumarate and on formate plus sulfur as energy sources. Arch. Microbiol. 144 (2), 147–150. doi: 10.1007/BF00414725
Mantha G., Awasthi A. K., Al-Aidaroos A. M., Hwang J.-S. (2013). Diversity and abnormalities of cyclopoid copepods around hydrothermal vent fluids, kueishantao island, north-eastern Taiwan. J. Nat. Hist. 47 (5-12), 685–697. doi: 10.1080/00222933.2012.747638
Markowitz V. M., Chen I. M., Palaniappan K., Chu K., Szeto E., Grechkin Y., et al. (2012). IMG: The integrated microbial genomes database and comparative analysis system. Nucleic Acids Res. 40 (D1), D115–D122. doi: 10.1093/nar/gkr1044
Maugeri T. L., Lentini V., Gugliandolo C., Italiano F., Cousin S., Stackebrandt E. (2009). Bacterial and archaeal populations at two shallow hydrothermal vents off panarea island (Eolian islands, Italy). Extremophiles 13 (1), 199–212. doi: 10.1007/s00792-008-0210-6
Mehrbach C., Culberson C. H., Hawley J. E., Pytkowicx R. M. (1973). Measurement of the apparent dissociation constants of carbonic acid in seawater at atmospheric pressure. Limnol. Oceanogr. 18 (6), 897–907. doi: 10.4319/lo.1973.18.6.0897
Misuraca L., Demé B., Oger P., Peters J. (2021). Alkanes increase the stability of early life membrane models under extreme pressure and temperature conditions. Commun. Chem. 4 (1), 1–8. doi: 10.1038/s42004-021-00467-5
Miyake H., Oda A., Wada S., Kodaka T., Kurosawa S. (2019). First record of a shallow hydrothermal vent crab, Xenograpsus testudinatus, from shikine-jima island in the izu archipelago. Biogeography 21), 31–36. doi: 10.11358/biogeo.21.31
Moran M. A., Belas R., Schell M. A., Gonzalez J. M., Sun F., Sun S., et al. (2007). Ecological genomics of marine Roseobacters. Appl. Environ. Microbiol. 73 (14), 4559–4569. doi: 10.1128/AEM.02580-06
Nakagawa S., Takai K., Inagaki F., Hirayama H., Nunoura T., Horikoshi K., et al. (2005a). Distribution, phylogenetic diversity and physiological characteristics of epsilon-proteobacteria in a deep-sea hydrothermal field. Environ. Microbiol. 7 (10), 1619–1632. doi: 10.1111/j.1462-2920.2005.00856.x
Nakagawa S., Takai K., Inagaki F., Horikoshi K., Sako Y. (2005b). Nitratiruptor tergarcus gen. nov., sp. nov. and Nitratifractor salsuginis gen. nov., sp. nov., nitrate-reducing chemolithoautotrophs of the epsilon-proteobacteria isolated from a deep-sea hydrothermal system in the mid-Okinawa trough. Int. J. Syst. Evol. Microbiol. 55 (2), 925–933. doi: 10.1099/ijs.0.63480-0
Nakagawa S., Takaki Y., Shimamura S., Reysenbach A. L., Takai K., Horikoshi K. (2007). Deep-sea vent epsilon-proteobacterial genomes provide insights into emergence of pathogens. Proc. Natl. Acad. Sci. U.S.A. 104 (29), 12146–12150. doi: 10.1073/pnas.0700687104
Nelson D. C., Hagen K. D., Edwards D. B. (1995). The gill symbiont of the hydrothermal vent mussel Bathymodiolus thermophilus is a psychrophilic, chemoautotrophic, sulfur bacterium. Mar. Biol. 121 (3), 487–495. doi: 10.1007/BF00349457
Ng N. K., Suzuki H., Shih H. T., Dewa S., Ng P. K. L. (2014). The hydrothermal crab, Xenograpsus testudinatus ng, Huang & ho 2000 (Crustacea: Decapoda: Brachyura: Grapsidae) in southern Japan. Proc. Biol. Soc Wash. 127 (2), 391–399. doi: 10.2988/0006-324x-127.2.391
Nishihara H., Igarashi Y., Kodama T. (1991). Hydrogenovibrio marinus gen. nov., sp. nov., a marine obligately chemolithoautotrophic hydrogen-oxidizing bacterium. Int. J. Syst. Evol. Microbiol. 41 (1), 130–133. doi: 10.1099/00207713-41-1-130
Nishihara H., Miyashita Y., Aoyama K., Kodama T., Igarashi Y., Takamura Y. (1997). Characterization of an extremely thermophilic and oxygen-stable membrane-bound hydrogenase from a marine hydrogen-oxidizing bacterium Hydrogenovibrio marinus. Biochem. Biophys. Res. Commun. 232 (3), 766–770. doi: 10.1006/bbrc.1997.6369
Oeschger R., Vetter R. D. (1992). Sulfide detoxification and tolerance in Halicryptus spinulosus (Priapulida): A multiple strategy. Mar. Ecol. Prog. Ser. 86, 167-179.
Pang K.-L., Guo S.-Y., Chen I.-A., Burgaud G., Luo Z.-H., Dahms H. U., et al. (2019). Insights into fungal diversity of a shallow-water hydrothermal vent field at kueishan island, Taiwan by culture-based and metabarcoding analyses. PloS One 14 (12), e0226616. doi: 10.1371/journal.pone.0226616
Payne M. S., Hall M. R., Sly L., Bourne D. G. (2007). Microbial diversity within early-stage cultured Panulirus ornatus phyllosomas. Appl. Environ. Microbiol. 73 (6), 1940–1951. doi: 10.1128/AEM.02520-06
Penesyan A., Tebben J., Lee M., Thomas T., Kjelleberg S., Harder T., et al. (2011). Identification of the antibacterial compound produced by the marine epiphytic bacterium pseudovibrio sp. D323 and related sponge-associated bacteria. Mar. Drugs 9 (8), 1391–1402. doi: 10.3390/md9081391
Peng S.-H., Hung J.-J., Hwang J.-S. (2011). Bioaccumulation of trace metals in the submarine hydrothermal vent crab Xenograpsus testudinatus off kueishan island, Taiwan. Mar. pollut. Bull. 63 (5-12), 396–401. doi: 10.1016/j.marpolbul.2011.05.013
Perez-Pascual D., Lunazzi A., Magdelenat G., Rouy Z., Roulet A., Lopez-Roques C., et al. (2017). The complete genome sequence of the fish pathogen Tenacibaculum maritimum provides insights into virulence mechanisms. Front. Microbiol. 8. doi: 10.3389/fmicb.2017.01542
Petersen J. M., Ramette A., Lott C., Cambon-Bonavita M. A., Zbinden M., Dubilier N. (2010). Dual symbiosis of the vent shrimp Rimicaris exoculata with filamentous gamma-and Epsilonproteobacteria at four mid Atlantic ridge hydrothermal vent fields. Environ. Microbiol. 12 (8), 2204–2218. doi: 10.1111/j.1462-2920.2009.02129.x
Petersen J. M., Zielinski F. U., Pape T., Seifert R., Moraru C., Amann R., et al. (2011). Hydrogen is an energy source for hydrothermal vent symbioses. Nature 476 (7359), 176–180. doi: 10.1038/nature10325
Pierrot D., Lewis E., Wallace D. W. R. (2006). “). MS excel program developed for CO2 system calculations. ORNL/CDIAC-105a,” in Carbon dioxide information analysis center, oak ridge national laboratory (Oak Ridge, Tennessee: US Department of Energy).
Ponsard J., Cambon-Bonavita M. A., Zbinden M., Lepoint G., Joassin A., Corbari L., et al. (2013). Inorganic carbon fixation by chemosynthetic ectosymbionts and nutritional transfers to the hydrothermal vent host-shrimp Rimicaris exoculata. ISME J. 7 (1), 96–109. doi: 10.1038/ismej.2012.87
Porchas-Cornejo M. A., Martinez-Porchas M., Vargas-Albores F., Gollas-Galvan T., Martinez-Cordova L. R., Vazquez-Euan R., et al. (2017). High-resolution detection of bacterial profile of ocean water, before and after being used by shrimp farms. Aquac. Int. 25 (5), 1833–1843. doi: 10.1007/s10499-017-0160-z
Rajasabapathy R., Mohandass C., Bettencourt R., Colaco A., Goulart J., Meena R. M. (2018). Bacterial diversity at a shallow-water hydrothermal vent (Espalamaca) in Azores island. Curr. Sci. 115 (11), 2110–2121. doi: 10.18520/cs/v115/i11/2110-2121
Rajasabapathy R., Mohandass C., Colaco A., Dastager S. G., Santos R. S., Meena R. M. (2014). Culturable bacterial phylogeny from a shallow water hydrothermal vent of espalamaca (Faial, Azores) reveals a variety of novel taxa. Curr. Sci. 106 (1), 58–69.
Riesenfeld C. S., Murray A. E., Baker B. J. (2008). Characterization of the microbial community and polyketide biosynthetic potential in the palmerolide-producing tunicate Synoicum adareanum. J. Nat. Prod. 71 (11), 1812–1818. doi: 10.1021/np800287n
Riley M., Staley J. T., Danchin A., Wang T. Z., Brettin T. S., Hauser L. J., et al. (2008). Genomics of an extreme psychrophile, Psychromonas ingrahamii. BMC Genom. 9 (1), 1–19. doi: 10.1186/1471-2164-9-210
Romano S. (2018). Ecology and biotechnological potential of bacteria belonging to the genus Pseudovibrio. Appl. Environ. Microbiol. 84 (8), e02516–e02517. doi: 10.1128/AEM.02516-17
Rypien K. L., Ward J. R., Azam F. (2010). Antagonistic interactions among coral-associated bacteria. Environ. Microbiol. 12 (1), 28–39. doi: 10.1111/j.1462-2920.2009.02027.x
Sahling H., Rickert D., Lee R. W., Linke P., Suess E. (2002). Macrofaunal community structure and sulfide flux at gas hydrate deposits from the cascadia convergent margin, NE pacific. Mar. Ecol. Prog. Ser. 231, 121–138. doi: 10.3354/meps231121
Salazar G., Cornejo-Castillo F. M., Benitez-Barrios V., Fraile-Nuez E., Alvarez-Salgado X. A., Duarte C. M., et al. (2016). Global diversity and biogeography of deep-sea pelagic prokaryotes. ISME J. 10 (3), 596–608. doi: 10.1038/ismej.2015.137
Santos O. C., Pontes P. V., Santos J. F., Muricy G., Giambiagi-deMarval M., Laport M. S. (2010). Isolation, characterization and phylogeny of sponge-associated bacteria with antimicrobial activities from Brazil. Res. Microbiol. 161 (7), 604–612. doi: 10.1016/j.resmic.2010.05.013
Sarazin G., Michard G., Prevot F. (1999). A rapid and accurate spectroscopic method for alkalinity measurements in sea water samples. Water Res. 33 (1), 290–294. doi: 10.1016/S0043-1354(98)00168-7
Shieh W. Y., Lin Y. T., Jean W. D. (2004). Pseudovibrio denitrificans gen. nov., sp. nov., a marine, facultatively anaerobic, fermentative bacterium capable of denitrification. Int. J. Syst. Evol. Microbiol. 54 (6), 2307–2312. doi: 10.1099/ijs.0.63107-0
Simon J. (2002). Enzymology and bioenergetics of respiratory nitrite ammonification. FEMS Microbiol. Rev. 26 (3), 285–309. doi: 10.1111/j.1574-6976.2002.tb00616.x
Simon J., Sänger M., Schuster S. C., Gross R. (2003). Electron transport to periplasmic nitrate reductase (NapA) of Wolinella succinogenes is independent of a NapC protein. Mol. Microbiol. 49 (1), 69–79. doi: 10.1046/j.1365-2958.2003.03544.x
Smith C. J., Nedwell D. B., Dong L. F., Osborn A. M. (2007). Diversity and abundance of nitrate reductase genes (narG and napA), nitrite reductase genes (nirS and nrfA), and their transcripts in estuarine sediments. Appl. Environ. Microbiol. 73 (11), 3612–3622. doi: 10.1128/AEM.02894-06
Strohm T. O., Griffin B., Zumft W. G., Schink B. (2007). Growth yields in bacterial denitrification and nitrate ammonification. Appl. Environ. Microbiol. 73 (5), 1420–1424. doi: 10.1128/AEM.02508-06
Szafranski K. M., Piquet B., Shillito B., Lallier F. H., Duperron S. (2015). Relative abundances of methane- and sulfur-oxidizing symbionts in gills of the deep-sea hydrothermal vent mussel Bathymodiolus azoricus under pressure. Deep-Sea Res. I: Oceanogr. Res. Pap. 101, 7–13. doi: 10.1016/j.dsr.2015.03.003
Takai K., Campbell B. J., Cary S. C., Suzuki M., Oida H., Nunoura T., et al. (2005). Enzymatic and genetic characterization of carbon and energy metabolisms by deep-sea hydrothermal chemolithoautotrophic isolates of Epsilonproteobacteria. Appl. Environ. Microbiol. 71 (11), 7310–7320. doi: 10.1128/AEM.71.11.7310-7320.2005
Takai K., Inagaki F., Nakagawa S., Hirayama H., Nunoura T., Sako Y., et al. (2003). Isolation and phylogenetic diversity of members of previously uncultivated epsilon-proteobacteria in deep-sea hydrothermal fields. FEMS Microbiol. Lett. 218 (1), 167–174. doi: 10.1016/S0378-1097(02)01147-3
Takai K., Miyazaki M., Hirayama H., Nakagawa S., Querellou J., Godfroy A. (2009). Isolation and physiological characterization of two novel, piezophilic, thermophilic chemolithoautotrophs from a deep-sea hydrothermal vent chimney. Environ. Microbiol. 11 (8), 1983–1997. doi: 10.1111/j.1462-2920.2009.01921.x
Tarasov V. G., Gebruk A. V., Mironov A. N., Moskalev L. I. (2005). Deep-sea and shallow-water hydrothermal vent communities: Two different phenomena? Chem. Geol. 224 (1-3), 5–39. doi: 10.1016/j.chemgeo.2005.07.021
Tokuda G., Yamada A., Nakano K., Arita N. O., Yamasaki H. (2008). Colonization of sulfurovum sp. on the gill surfaces of Alvinocaris longirostris, a deep-sea hydrothermal vent shrimp. Mar. Ecol. 29 (1), 106–114. doi: 10.1111/j.1439-0485.2007.00211.x
Truchot J. P. (1976). Carbon dioxide combining properties of the blood of the shore crab Carcinus maenas (L): Carbon dioxide solubility coefficient and carbonic acid dissociation constants. J. Exp. Biol. 64 (1), 45–57. doi: 10.1242/jeb.64.1.45
Tsuchida S., Suzuki Y., Fujiwara Y., Kawato M., Uematsu K., Yamanaka T., et al. (2011). Epibiotic association between filamentous bacteria and the vent-associated galatheid crab, Shinkaia crosnieri (Decapoda: Anomura). J. Mar. Biolog. Assoc. U.K. 91 (1), 23–32. doi: 10.1017/S0025315410001827
Tunnicliffe V. (1991). The biology of hydrothermal vents: ecology and evolution. Oceanogr. Mar. Biol. 29, 319–407.
Valenzuela-Gonzalez F., Martinez-Porchas M., Villalpando-Canchola E., Vargas-Albores F. (2016). Studying long 16S rDNA sequences with ultrafast-metagenomic sequence classification using exact alignments (Kraken). J. Microbiol. Methods 122, 38–42. doi: 10.1016/j.mimet.2016.01.011
Van Dover C. L. (2014). Impacts of anthropogenic disturbances at deep-sea hydrothermal vent ecosystems: A review. Mar. Environ. Res. 102, 59–72. doi: 10.1016/j.marenvres.2014.03.008
Van Dover C. L., Trask J. L. (2000). Diversity at deep-sea hydrothermal vent and intertidal mussel beds. Mar. Ecol. Prog. Ser. 195, 169–178. doi: 10.3354/meps195169
Vetriani C., Voordeckers J. W., Crespo-Medina M., O'Brien C. E., Giovannelli D., Lutz R. A. (2014). Deep-sea hydrothermal vent Epsilonproteobacteria encode a conserved and widespread nitrate reduction pathway (Nap). ISME J. 8 (7), 1510–1521. doi: 10.1038/ismej.2013.246
Wang T. W., Chan T. Y., Chan B. K. K. (2014). Trophic relationships of hydrothermal vent and non-vent communities in the upper sublittoral and upper bathyal zones off kueishan island, Taiwan: A combined morphological, gut content analysis and stable isotope approach. Mar. Biol. 161 (11), 2447–2463. doi: 10.1007/s00227-014-2479-6
Wang L., Cheung M. K., Kwan H. S., Hwang J. S., Wong C. K. (2015). Microbial diversity in shallow-water hydrothermal sediments of Kueishan Island, Taiwan as revealed by pyrosequencing. J. Basic Microbiol. 55 (11), 1308–1318. doi: 10.1002/jobm.201400811
Wang L., Cheung M. K., Liu R., Wong C. K., Kwan H. S., Hwang J. S. (2017). Diversity of total bacterial communities and chemoautotrophic populations in sulfur-rich sediments of shallow-water hydrothermal vents off Kueishan Island, Taiwan. Microb. Ecol. 73 (3), 571–582. doi: 10.1007/s00248-016-0898-2
Wang S., Jiang L., Hu Q., Liu X., Yang S., Shao Z. (2021). Elemental sulfur reduction by a deep-sea hydrothermal vent campylobacterium sulfurimonas sp. NW10. Environ. Microbiol. 23 (2), 965–979. doi: 10.1111/1462-2920.15247
Wang T. W., Lau D. C., Chan T. Y., Chan B. K. K. (2022). Autochthony and isotopic niches of benthic fauna at shallow-water hydrothermal vents. Sci. Rep. 12 (1), 1–14. doi: 10.1038/s41598-022-09839-w
Watsuji T., Nakagawa S., Tsuchida S., Toki T., Hirota A., Tsunogai U., et al. (2010). Diversity and function of epibiotic microbial communities on the galatheid crab, Shinkaia crosnieri. Microbes Environ. 25 (4), 288–294. doi: 10.1264/jsme2.ME10135
Xing P., Hahnke R. L., Unfried F., Markert S., Huang S., Barbeyron T., et al. (2015). Niches of two polysaccharide-degrading Polaribacter isolates from the north Sea during a spring diatom bloom. ISME J. 9 (6), 1410–1422. doi: 10.1038/ismej.2014.225
Yamamoto M., Takai K. (2011). Sulfur metabolisms in epsilon- and gamma-proteobacteria in deep-sea hydrothermal fields. Front. Microbiol. 2. doi: 10.3389/fmicb.2011.00192
Yang S. H., Chiang P. W., Hsu T. C., Kao S. J., Tang S. L. (2016). Bacterial community associated with organs of shallow hydrothermal vent crab Xenograpsus testudinatus near Kuishan Island, Taiwan. PloS One 11 (3), e0150597. doi: 10.1371/journal.pone.0150597
Yang C. H., Wang T. W., Ng P. K. L., Chan T. Y., Lin Y. Y., Chan B. K. K. (2022). Population genetic differentiation on the hydrothermal vent crabs Xenograpsus testudinatus along depth and geographical gradients in the Western pacific. Diversity 14 (3), 162. doi: 10.3390/d14030162
Zbinden M., Marqué L., Gaudron S. M., Ravaux J., Léger N., Duperron S. (2015). Epsilonproteobacteria as gill epibionts of the hydrothermal vent gastropod Cyathermia naticoides (North East-pacific rise). Mar. Biol. 162 (2), 435–448. doi: 10.1007/s00227-014-2591-7
Zbinden M., Shillito B., Le Bris N., de Montlaur C. D., Roussel E., Guyot F., et al. (2008). New insigths on the metabolic diversity among the epibiotic microbial communitiy of the hydrothermal shrimp Rimicaris exoculata. J. Exp. Mar. Biol. Ecol. 359 (2), 131–140. doi: 10.1016/j.jembe.2008.03.009
Zerillo M. M., Adhikari B. N., Hamilton J. P., Buell C. R., Levesque C. A., Tisserat N. (2013). Carbohydrate-active enzymes in Pythium and their role in plant cell wall and storage polysaccharide degradation. PloS One 8 (9), e72572. doi: 10.1371/journal.pone.0072572
Zhang J., Sun Q. L., Luan Z. D., Lian C., Sun L. (2017). Comparative transcriptome analysis of rimicaris sp. reveals novel molecular features associated with survival in deep-sea hydrothermal vent. Sci. Rep. 7 (1), 1–16. doi: 10.1038/s41598-017-02073-9
Keywords: shallow-water hydrothermal vent, brachyuran crab, Xenograpsus testudinatus, sulfide-rich fluids, crustacean holobiont, detoxification, Sulfurovum, Kueishan Island
Citation: Chiu L, Wang M-C, Tseng K-Y, Wei C-L, Lin H-T, Yang S-H and Tseng Y-C (2022) Shallow-water hydrothermal vent system as an extreme proxy for discovery of microbiome significance in a crustacean holobiont. Front. Mar. Sci. 9:976255. doi: 10.3389/fmars.2022.976255
Received: 24 June 2022; Accepted: 11 August 2022;
Published: 02 September 2022.
Edited by:
Jin Sun, Ocean University of China, ChinaReviewed by:
Yan Sun, Institute of Oceanology, Chinese Academy of Sciences (CAS), ChinaCopyright © 2022 Chiu, Wang, Tseng, Wei, Lin, Yang and Tseng. This is an open-access article distributed under the terms of the Creative Commons Attribution License (CC BY). The use, distribution or reproduction in other forums is permitted, provided the original author(s) and the copyright owner(s) are credited and that the original publication in this journal is cited, in accordance with accepted academic practice. No use, distribution or reproduction is permitted which does not comply with these terms.
*Correspondence: Shan-Hua Yang, c2hhbmh1YUBudHUuZWR1LnR3; Yung-Che Tseng, eWN0c2VuZ0BnYXRlLnNpbmljYS5lZHUudHc=
†These authors have contributed equally to this work
Disclaimer: All claims expressed in this article are solely those of the authors and do not necessarily represent those of their affiliated organizations, or those of the publisher, the editors and the reviewers. Any product that may be evaluated in this article or claim that may be made by its manufacturer is not guaranteed or endorsed by the publisher.
Research integrity at Frontiers
Learn more about the work of our research integrity team to safeguard the quality of each article we publish.