- 1National Marine Mammal Foundation, San Diego, CA, United States
- 2National Oceanic and Atmospheric Administration, National Marine Fisheries Service, Office of Protected Resources, Silver Spring, MD, United States
- 3Chicago Zoological Society, Brookfield Zoo, IL, United States
- 4ZooRadOne, IL, United States
- 5Zoological Pathology Program, University of Illinois College of Veterinary Medicine, IL, United States
- 6Bayside Hospital for Animals, Fort Walton Beach, FL, United States
- 7Chicago Zoological Society's Sarasota Dolphin Research Program, c/o Mote Marine Laboratory, Sarasota, FL, United States
The Deepwater Horizon (DWH) disaster resulted in large-scale contamination of bays, sounds, and estuaries in the northern Gulf of Mexico, home to multiple stocks of common bottlenose dolphins (Tursiops truncatus). Inhalation, aspiration, ingestion, and dermal absorption of oil and its toxic components were all considered possible routes of exposure for dolphins living within the oil spill footprint. To determine if dolphins were adversely impacted, catch-and-release health assessments were performed in heavily-oiled Barataria Bay (BB), Louisiana, and in Sarasota Bay (SB), Florida, a comparison site with no DWH oil contamination. Initial studies were conducted as part of a Natural Resource Damage Assessment (2011-2014) and follow-on studies were performed between 2016-2018 with support from the Gulf of Mexico Research Initiative. Ultrasound was used to evaluate the dolphins’ pulmonary health, including the presence/absence of pleural effusion, nodules, masses, consolidation, and alveolar interstitial syndrome (AIS). When present, AIS was further graded by severity (mild, moderate, or severe) and distribution. Based on the presence and severity of abnormalities, each dolphin was given an overall lung disease score (normal, mild, moderate, or severe). Normal to mild scores were considered within expected limits for a wild population, therefore the prevalence of normal-mild versus moderate-severe scores was compared between the oiled and unoiled sites. Separate analyses were conducted for dolphins alive in 2010 (and in BB, presumably exposed to DWH oil), and those born after 2010. For the dolphins alive in 2010, temporal trends were also examined using generalized additive models (GAMs). Results showed a strong difference (p=0.000357) in moderate to severe lung disease between the two sites for dolphins alive in 2010, but no significant difference (p=0.6745) between the sites for dolphins born after 2010. In BB dolphins, the prevalence of moderate to severe lung disease did not decrease in the years after the spill, and in fact, potentially worsened (p=0.0644 for trend over years), with the highest prevalence (0.61) being in 2018. Moderate to severe AIS remained a persistent finding in BB dolphins, and several animals had a pattern of AIS that was more severe ventrally than dorsally, with evidence of chronic, progressive disease states.
Introduction
In April 2010, the Deepwater Horizon (DWH) offshore drilling rig exploded and sank, resulting in large-scale release of oil that contaminated bays, sounds, and estuaries in the northern Gulf of Mexico (NGoM) (Michel et al., 2013). More than 3 million barrels of oil (~900 million pounds) were released before the well was sealed (U.S. v. BP et al., 2015), resulting in an oil slick that spanned ~43,000 square miles of water and oiled more than 1,000 miles of shoreline habitats (Michel et al., 2013; ERMA, 2015). Although clean-up efforts removed ~600 million pounds of oil-contaminated waste from Gulf waters and nearshore habitats of the NGoM (EPA, 2011), the oil spill caused substantial injury to marine life (Deepwater Horizon Natural Resource Damage Assessment (NRDA) Trustees, 2016).
As DWH oil spread throughout the NGoM, multiple cetacean species were observed in the oiled areas (Aichinger Dias et al., 2017). Response monitoring activities from April through September 2010 documented more than 1,100 individuals of 10 different cetacean species swimming through thick surface oil or surface oil sheen (Figure 1) (Aichinger Dias et al., 2017; Wilkin et al., 2017). Cetaceans living within the oil spill footprint were likely exposed through multiple routes, including inhalation of volatile organic compounds, direct aspiration of oil droplets, ingestion with or without subsequent aspiration, and dermal absorption of oil and its toxic components (Takeshita et al., 2017). Further, oral ingestion may have occurred through incidental ingestion of oil on the surface of the water and/or suspended in the water column, or through the ingestion of contaminated prey and/or sediments during foraging activities (Godard-Codding and Collier, 2018; Quigley et al., 2022).
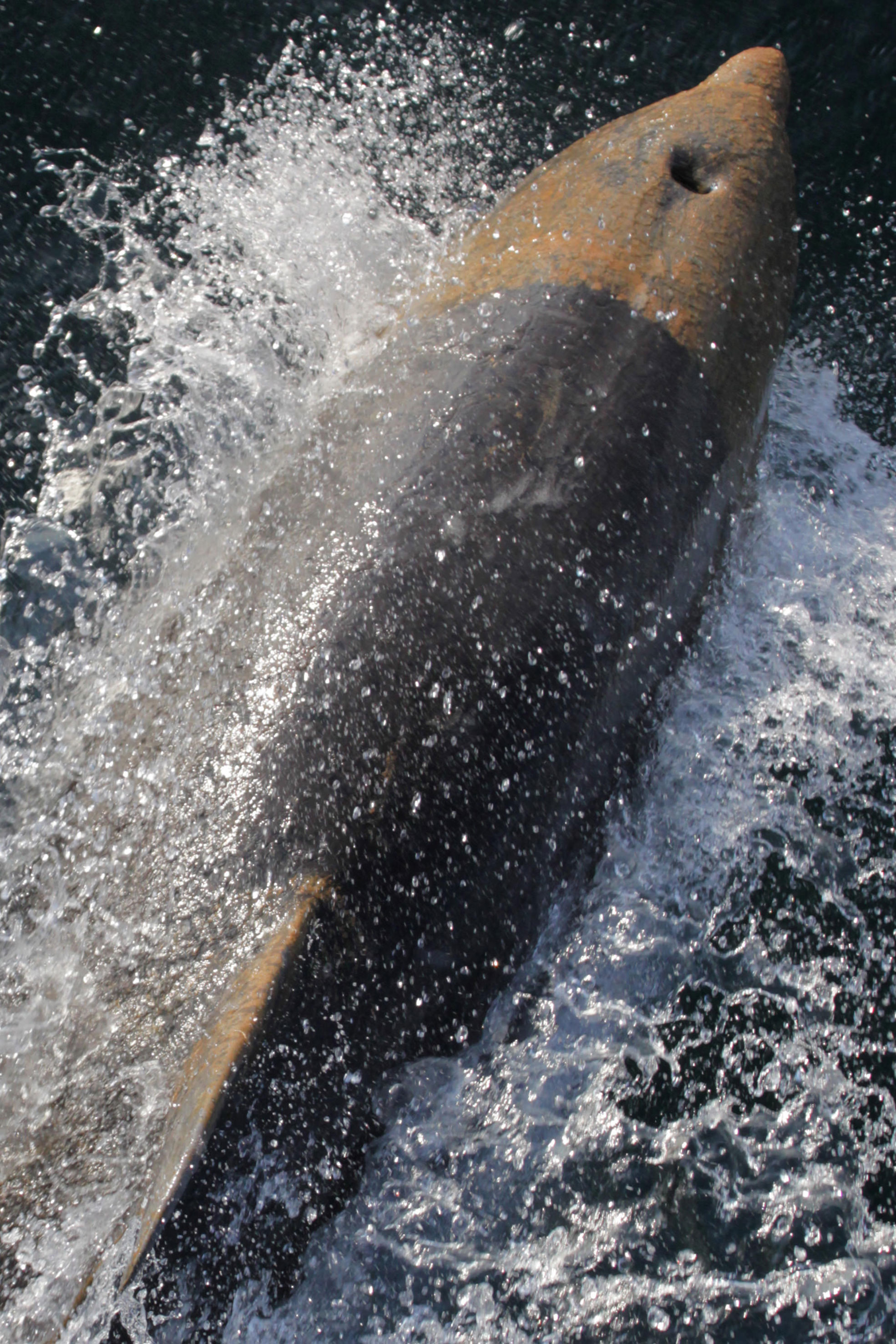
Figure 1 Bottlenose dolphin surfacing for a breath within the DWH oil spill footprint, with oil adhered to its head and dorsal fin (photo: NOAA, July 2010).
In the months that followed, one of the largest and longest-lasting marine mammal Unusual Mortality Events (UMEs) documented in the NGoM began and continued through 2014 (Litz et al., 2014). Marine mammal stranding network responders collected biological data and samples from carcasses, and after a comprehensive investigation into all potential causes of the mortality event, the most likely cause was determined to be the DWH oil spill (Venn-Watson et al., 2015a). Some of the most consistent necropsy findings within the oil spill footprint were bronchopneumonia and adrenal gland atrophy in non-perinate dolphins and an increased prevalence of fetal distress and in utero pneumonia in dead perinates (Venn-Watson et al., 2015b; Colegrove et al., 2016).
While the marine mammal UME investigation was underway, a Natural Resource Damage Assessment (NRDA) was being conducted to assess the potential impact of the DWH oil spill on the NGoM ecosystem, including marine mammals. Live bottlenose dolphins (Tursiops truncatus) living in heavily-oiled Barataria Bay (BB), Louisiana, and oil-impacted Mississippi Sound (MS), Mississippi/Alabama, received comprehensive health exams during catch-and-release field studies. Live dolphins were also examined in Sarasota Bay (SB), Florida, a comparison site with no DWH oil contamination. Dolphins in the heavily-oiled sites had multiple health issues, including moderate to severe lung disease, poor body condition, an impaired stress response, and hematological/serum chemistry indicators of inflammation, hypoglycemia, and abnormal iron levels (Schwacke et al., 2014). During 2011-2013, nearly half of the dolphins evaluated by experienced marine mammal veterinarians in oil-impacted habitats (BB and MS) were considered unhealthy, indicated by a guarded or worse prognosis, and 17% percent of examined dolphins received a poor or grave prognosis, meaning they were not expected to survive (Schwacke et al., 2014).
The increased prevalence of dolphins with compromised health coincided with high mortality rates within the oil spill footprint. Follow-up studies of BB dolphins using mark-recapture survival models yielded estimated annual mortality rates of 13.2-19.6% in the years immediately following the spill (Lane et al., 2015; McDonald et al., 2017), which were much higher than mortality rates previously reported for bottlenose dolphins using similar techniques near Charleston, South Carolina (4.9%), and Sarasota, Florida (3.8%) (Wells and Scott, 1990; Speakman et al., 2010). Alternative hypotheses were considered, including exposure to harmful algal blooms (Deepwater Horizon Natural Resource Damage Assessment (NRDA) Trustees, 2016), persistent organic pollutants (Balmer et al., 2015), and infectious disease outbreaks (Venn-Watson et al., 2015a). These factors were ruled out as likely contributors to the increased mortality rates, and exposure to toxic oil components was determined to be the most likely cause of increased mortality within the DWH oil spill footprint (Deepwater Horizon Natural Resource Damage Assessment (NRDA) Trustees, 2016; Takeshita et al., 2017).
In the year following the DWH spill, dolphins examined within the oil spill footprint had an approximately 5-fold higher prevalence of moderate to severe lung disease compared to dolphins living outside the oiled region, based on pulmonary ultrasound examination (0.34 in BB versus 0.07 in SB, the comparison site) (Schwacke et al., 2014). During 2013 and 2014, the prevalence of moderate to severe lung disease among BB dolphins decreased slightly (0.23 and 0.25, respectively), but remained elevated relative to the prevalence at the SB non-oiled comparison site (Smith et al., 2017). Concurrent pathology investigations of dead dolphins recovered from the NGoM in the years following the spill documented similar pulmonary findings (Venn-Watson et al., 2015b). The prevalence of bacterial pneumonias, many severe, in carcasses recovered within the oil spill footprint was significantly higher than in comparison populations [0.22 (oiled) vs 0.02 (non-oiled)]. Pneumonias were caused by multiple bacterial pathogens, indicating that lung disease was not due to infection with a single, highly pathogenic bacterium, and may have been due to secondary infection of damaged lung tissue (Venn-Watson et al., 2015b).
The increased prevalence of lung disease in dolphins living within the DWH oil spill footprint was consistent with respiratory findings from humans exposed to oil and its toxic components (Zock et al., 2007; Jung et al., 2013; Alexander et al., 2018; Gam et al., 2018a; Gam et al., 2018b). However, relatively few studies have focused on the chronicity of respiratory disease post-exposure (Zock et al., 2012; Zock et al., 2014; Lawrence et al., 2020). Here we combined these previously reported data with additional data collected from 2016-2018 as part of a Gulf of Mexico Research Initiative study to examine temporal trends and assess if pulmonary disease persisted in wild dolphins as a chronic effect of the DWH oil spill.
Materials and methods
Dolphin catch-and-release health assessments
Dolphin health assessments were conducted in BB in 2016, 2017, and 2018, as well as SB (a non-oiled comparison site) in 2012 and 2015- 2018. For comparative analyses over time, we included previously reported pulmonary data from health assessments in BB (2011, 2013, 2014) and health assessments in SB (2011, 2013, 2014) (Schwacke et al., 2014; Smith et al., 2017). The SB 2012 health assessments were not previously included in the analysis because they were not part of the DWH NRDA. For the present study, we included the SB 2012 results to provide a more comprehensive analysis over time. Standardized, catch-and-release methodologies and diagnostic sampling techniques are described elsewhere and were similar during all study years and across locations (Wells et al., 2004; Schwacke et al., 2014). Briefly, dolphins were encircled with a seine net and supported by experienced handlers for examination. Small calves with a known or suspected age of less than two years were avoided. The amount of time each animal was restrained and the time spent out of the water were minimized (less than an hour), and veterinary staff continuously monitored animals throughout their health assessments.
Live dolphin health assessments in Barataria Bay were conducted under NMFS permit numbers 932-1905/MA-009526 and 18786 issued to the NMFS Marine Mammal Health and Stranding Response Program. All animal procedures were reviewed and approved by NMFS’s Institutional Animal Care and Use Committee. Sarasota Bay health assessments were performed under National Marine Fisheries Service Scientific Research Permit Nos. 15543 and 20455 approved annually by Mote Marine Laboratory’s Institutional Animal Care and Use Committee. All animal research was conducted in accordance with ARRIVE guidelines 2.0 (Percie du Sert et al., 2020).
Pulmonary ultrasound evaluations
To specifically evaluate pulmonary health, we utilized thoracic ultrasound techniques developed for U.S. Navy dolphins (Smith et al., 2012) and applied them to wild dolphin health exams. The dolphin body is well-suited for ultrasound, as their skin is smooth and hairless and doesn’t require any preparation. Portable ultrasound machines are powerful enough to penetrate dolphin blubber and rugged enough to be used in extreme field conditions (e.g. high air temperatures, saltwater environments, and unstable operating platforms). Therefore, we utilized portable Sonosite Edge® ultrasound machines (Sonosite, Bothell, Washington 98021, USA) and curvilinear 2-5MHz abdominal transducers to conduct pulmonary health evaluations.
Ultrasound exams were performed by experienced marine mammal sonographers (CRS, FMG, JMM) using the dorsal-ventral slide technique as previously described (Smith et al., 2012). Pulmonary abnormalities were detected following standardized methods (Smith et al., 2012; Schwacke et al., 2014) and divided into previously defined categories: (1) pleural effusion, or fluid surrounding the lungs; (2) superficial pulmonary nodules, or <2cm round/ovoid foci of non-aerated lung; (3) pulmonary masses, or ≥2cm well-defined areas of non-aerated lung; (4) alveolar-interstitial syndrome, or reduced air in the lung and replacement of air with cellular infiltrates or fluid, and (5) pulmonary consolidation, where fluid and/or cellular infiltrates completely occupy some portion of the lung. Alveolar-interstitial syndrome (AIS) was graded as mild, moderate, or severe as follows: mild – occasional clusters of ring-down artifacts; moderate – frequent clusters of ring-down artifacts, distributed throughout the dorsal, ventral, or entire lung field; severe – contiguous ring-down artifacts that created a ‘white-out’ effect and loss of reverberation artifact, detected in multiple areas. When present, distribution of AIS throughout the lung field was noted, such as a generalized distribution throughout the lung or localized to a specific area in the lung. Additionally, if AIS was more severe as the sonographer slid from dorsal lung to ventral lung, it was noted as ‘AIS worse D-V’. Ultrasound artifact identification was based on a large body of medical literature, as reviewed by Baad et al. (2017). Pulmonary nodules (<2cm) and masses (≥2cm) were measured and described.
Once examinations were complete, each lung (left and right) was given an overall score: normal (no evidence of disease), mild, moderate, or severe lung disease (Smith et al., 2017). Following the approach of Schwacke et al., (2014) and Smith et al. (2017), a binary variable was created to indicate lung scores that were normal-mild, which are within expected limits for a wild dolphin, versus moderate-severe disease.
Classification of age cohorts
In order to compare lung disease in BB dolphins presumably exposed to DWH oiling in 2010 versus those that were born after the spill, individual dolphins were classified as alive in 2010 (pre-2010 cohort), or born after 2010 (post-2010 cohort) following methods described by Schwacke et al. (2022). While age estimates from dental radiography and dentinal growth layer analysis were available for many of the sampled dolphins, there is uncertainty associated with these estimates (Herrman et al., 2020). Therefore, we chose to rely on observational life history evidence from photographic monitoring to obtain definitive classifications. Briefly, dorsal fin images of sampled dolphins were compared to photographic-identification (photo-ID) catalogs developed from long-term, small vessel studies in BB (2010-present) and SB (1970-present) (Wells and Scott, 1990; McDonald et al., 2017). Photo-ID surveys in SB were conducted regularly (monthly since 1992) and the vast majority of dolphins sampled in SB were known to the researchers prior to their health assessment. Those that had been observed in 2010 or earlier were classified as pre-2010; those observed as neonates in 2011 or later were classified as post-2010. Photo-ID surveys in BB were not initiated until 2010. For BB, a highly experienced photo-ID researcher (TS) searched the catalog for all sightings of the sampled dolphins prior to their health assessment. Dolphins that were sighted in 2010 were classified as pre-2010; dolphins sighted in 2011 or 2012 and deemed to be a non-calf (> 2 years) based on size at the time of the sighting were also classified as pre-2010. Dolphins observed as neonates in 2011, or in 2012 and later as a neonate or small calf were classified as post-2010. Cohort assignment could not be definitely determined for the remaining BB dolphins.
Statistical analysis
The prevalence of moderate-severe lung disease was compared between study sites separately for the pre-2010 and post-2010 cohorts. Right lungs were not routinely evaluated between 2011-2013 (due to health assessment time constraints), therefore only left lung scores were used for statistical analyses to facilitate comparison across years. For the pre-2010 cohort, we used a generalized additive model (GAM) with binomial response variable (normal or mild vs moderate or severe disease) as a function of study site (factor covariate BB or SB), year (continuous covariate), and sex (factor covariate male or female). A logit link function was used, and year was modeled using a thin plate regression spline with basis dimension k=3. We fit multiple models, including models with the main effects and an interaction of year and site, and then used Akaike Information Criteria (AIC) value to select the most parsimonious model.
Due to the short time span since the spill and the fact that very young dolphins (< 2 years old) were avoided for sampling, the number of post-2010 was limited; therefore, we did not attempt a temporal analysis (i.e., we did not include year as a covariate). This left only factor covariates, meaning models for the post-2010 cohort were generalized linear models (GLMs) rather than GAMs.
To visualize the prevalence of various lung abnormalities that contributed to the lung disease scores, heatmaps were generated and stratified by study site and age cohort. Nodules, pleural effusion, consolidation, masses, and AIS worse D-V were treated as binary variables (absent=0; present=1), with prevalence calculated as the proportion of dolphins for which the abnormality was present. In order to visually capture the gradient of severity of AIS scores, dolphins with no or mild AIS were assigned a 0, dolphins with moderate AIS were assigned 0.5, and 1.0 for severe AIS. Note that this assignment infers that severe scores were twice as bad as moderate scores, which is not necessarily accurate; however, the numeric assignment was only used for visualization purposes, not for statistical inference.
To determine whether the prevalence of the AIS worse D-V pattern changed over time for pre-2010 BB dolphins, an additional GAM was conducted, again with year modeled using a thin plate regression spline with basis dimension 3. All analyses were conducted in R (R Core Team, 2021); we used the mgcv package for GAM analyses (Wood, 2017) and the glm function within the R stats package. The gam.check function from the mgcv package was used to confirm convergence and check basis dimension results to ensure that k was not too low.
Results
Between 2011 and 2018, we evaluated the pulmonary health of 171 BB dolphins: 132 were alive at the time of the spill (2010) and 18 were born post-spill. Age cohort classification could not be determined for 21 BB individuals. Over the same time period, we assessed the pulmonary health of 103 dolphins from the unoiled comparison site (SB): 85 were alive at the time of the spill (2010) and 18 were born post-spill.
Dolphins alive in 2010 during the DWH spill
In the first year post-spill (2011), the prevalence of moderate to severe lung disease was 0.34 in BB dolphins (Figure 2A). The lowest prevalence in BB was observed in 2013 (0.22), but prevalence had increased again by 2018 (0.61). In SB, no severe lung disease was observed and the prevalence of moderate lung disease was low (0.00-0.08) from 2011-2014, but increased between 2015-2018 (0.14-0.38) and returned to 0.08 in 2018. We note that there is significant uncertainty in the higher SB estimates (2015-2018) due to very low sample sizes (2015 N=7, 2016 N=5, 2017 N=8; Figure 2B).
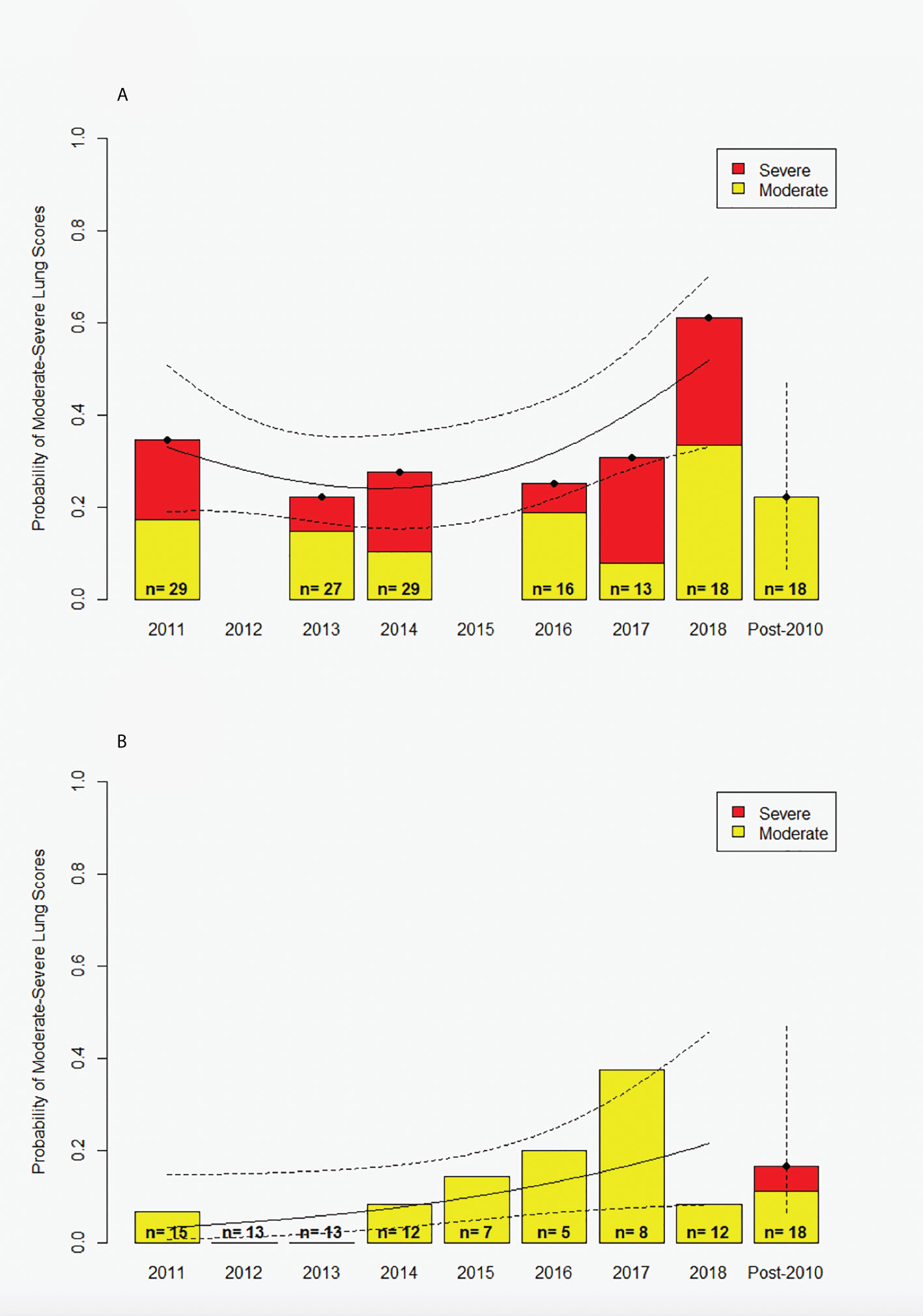
Figure 2 Probability of moderate and severe lung scores for (A) Barataria Bay and (B) Sarasota Bay. Probability for dolphins alive at the time of the spill is stratified by year (2011-2018); lung scores for dolphins born after 2010 were pooled within each site due to limited samples sizes (‘Post-2010’). Red and yellow portion of bars represent proportion of severe and moderate scores, respectively. Fitted curves (solid) and 95% CIs (dashed curves) are from the Generalized Additive Model (GAM) with separate smoothing splines for sampling year (Barataria Bay p=0.0644, Sarasota Bay p=0.0655). Vertical dashed lines for post-2010 cohorts represent 95% binomial confidence intervals.
Of the GAMs tested, the model with site and year covariates that had the lowest AIC value was selected for further inference (Table 1). Study site was strongly associated with the prevalence of moderate to severe lung disease (p=0.000357), with dolphins sampled in heavily-oiled BB having the higher prevalence. The GAM provided some evidence that prevalence of moderate to severe lung disease changed across years (p=0.0644 for BB, p=0.0655 for SB). However, in BB the prevalence did not consistently decrease over time after the spill (Figure 2A), and in fact the highest prevalence measured was in the final year of sampling.
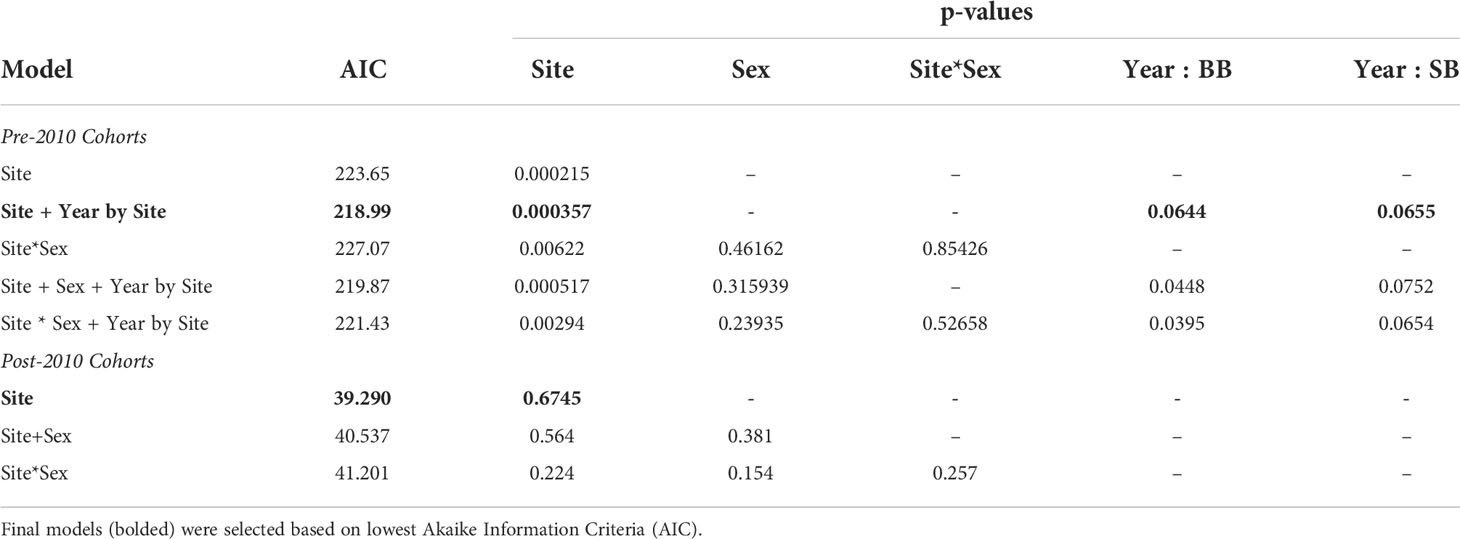
Table 1 Results from the generalized additive model analysis (pre-2010 cohorts) and generalized linear model analysis (post-2010 cohorts) of overall lung scores.
AIS was a substantial factor in the BB lung disease cases, and in some individual dolphins was more severe ventrally than dorsally (AIS worse D-V). This spatial distribution pattern of AIS in the lung was not seen in any of the SB dolphins sampled. The pattern was observed in BB dolphins alive at the time of the spill, and applying a GAM to examine the prevalence of the condition over time indicated an increase over the years (p=0.0002). The prevalence of this pattern was also highest in the final year of sampling (Figure 3). Other thoracic abnormalities included pleural effusion, pulmonary nodules, consolidation, and masses in BB (Figure 4A). Some of these abnormalities, including pulmonary nodules, were also seen in SB, but to a much lesser extent (Figure 4B).
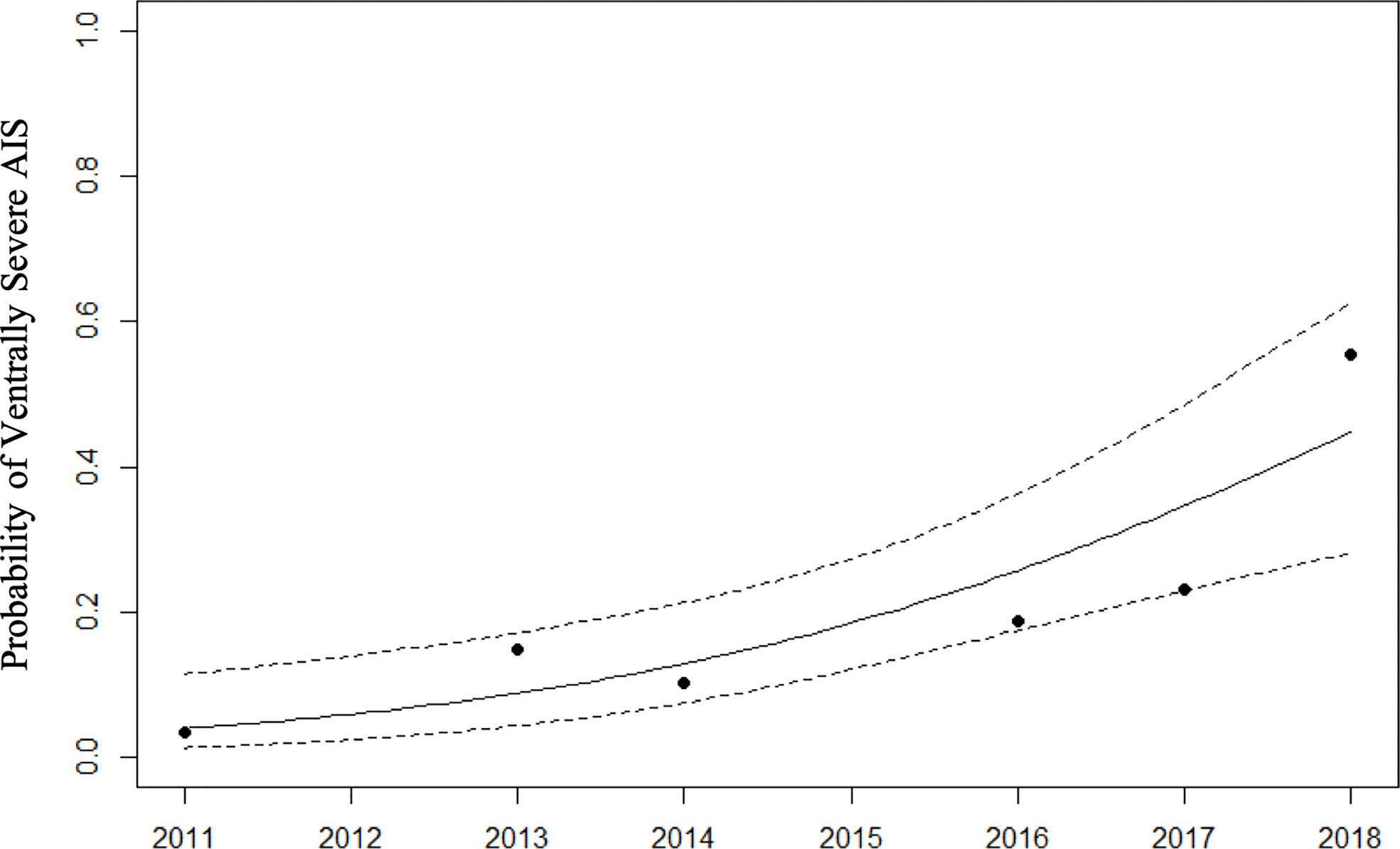
Figure 3 Probability of ventrally severe alveolar interstitial syndrome (AIS) in BB dolphins over years. Solid and dashed lines represent GAM model fit and 95% confidence intervals. p-value for smooth term < 0.001.
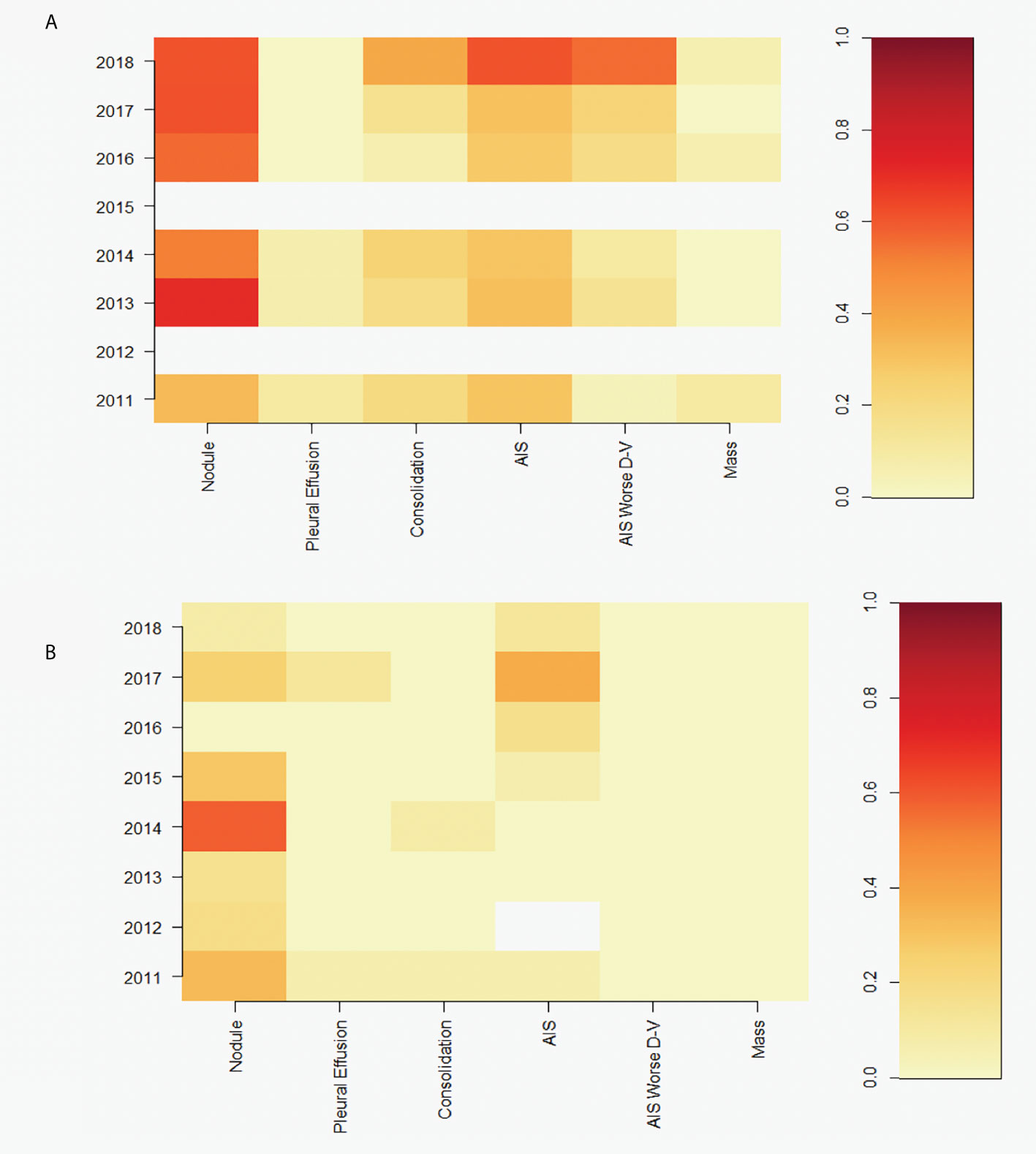
Figure 4 Heatmaps showing prevalence of pulmonary nodules (‘nodule’), pleural effusion, pulmonary consolidation (‘consolidation’), alveolar interstitial syndrome (‘AIS’), AIS with increased ventral severity (‘AIS Worse D-V’), and pulmonary masses (‘mass’) diagnosed between 2011-2018 in dolphins that were alive at the time of the DWH spill in (A) Barataria Bay (N=132 dolphins) and (B) Sarasota Bay comparison site (N=85 dolphins). White cells represent missing data. Colors represent prevalence of conditions, as represented in color key on the right.
Dolphins born after the DWH spill
For dolphins born after the spill, the GLM with only site as a factor had the lowest AIC (Table 1) and was selected for further inference. Prevalence of moderate or severe lung scores did not differ between BB and SB (p=0.6745), and was relatively low (Figure 2) for this younger cohort. Pulmonary nodules were the most commonly observed abnormality for both BB and SB cohorts (Figure 5).
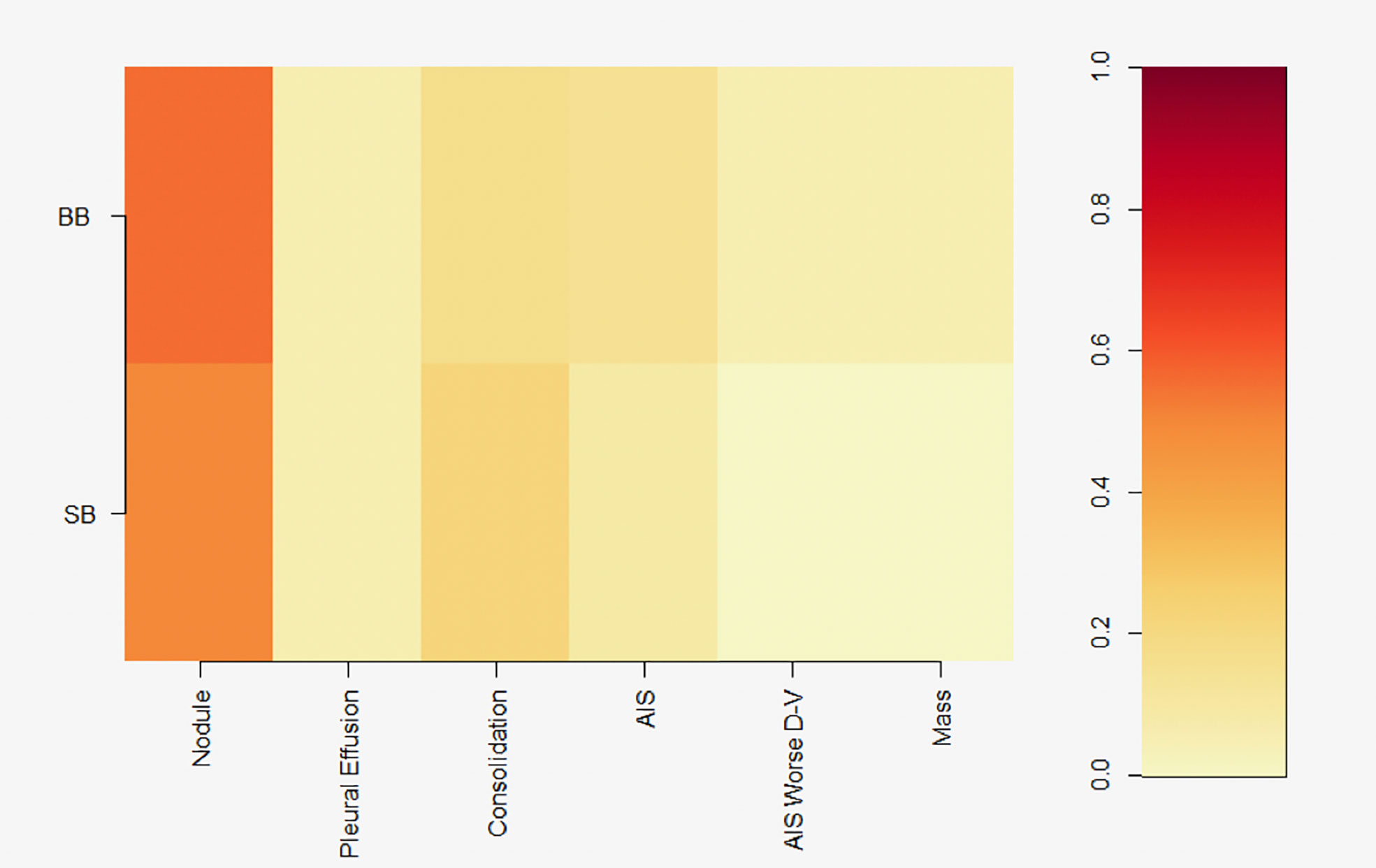
Figure 5 Heatmap showing prevalence of pulmonary nodules (‘nodule’), pleural effusion, pulmonary consolidation (‘consolidation’), alveolar interstitial syndrome (‘AIS’), AIS with increased ventral severity (‘AIS Worse D-V’), and pulmonary masses (‘mass’) diagnosed between 2011-2018 in dolphins born after the DWH spill. BB, Barataria Bay; SB, Sarasota Bay. Colors represent prevalence of conditions, as represented in color key on the right.
Discussion
In the aftermath of the DWH disaster and subsequent oiling of estuarine and coastal habitats, a high prevalence of moderate to severe lung disease was found in bottlenose dolphins living within Barataria Bay, one of the heaviest oiled estuaries. BB dolphins have high site fidelity, meaning that animals tend to live in the same region for multiple years and are unlikely to leave (Lane et al., 2015; Wells et al., 2017). Our results determined that dolphin pulmonary health has not improved for BB dolphins alive during the spill. The high prevalence of moderate to severe lung disease years following the spill suggests that the lung damage that occurred is chronic and likely progressive, which should be considered when investigating potential pathways and mechanisms of respiratory compromise. Other conditions that possibly contributed to the chronic lung disease included an increased susceptibility to lung infections due to oil-induced immune system aberrations (De Guise et al., 2021), cardiac damage with secondary pulmonary compromise (Linnehan et al., 2021), and/or age-related health changes.
In bottlenose dolphins and other cetaceans, the lungs serve a dual purpose of both respiration and buoyancy control (Ridgway et al., 1969). Investigations of dolphin pulmonary anatomy have shown that terminal airways are reinforced with cartilage, and myoelastic sphincters surround terminal bronchioles and alveolar entrances (Simpson & Gardner, 1972). The alveoli can completely collapse at depth, forcing air into the reinforced air spaces, presumably for prevention of decompression sickness (Ridgway et al., 1969). The presence of moderate to severe pulmonary disease would be expected to impair these physiologic mechanisms, negatively impact buoyancy, and increase energetic demands. This is supported by the authors’ (CRS, FMG, FIT, JMM, MI, RSW) personal observations of dolphins in human care diagnosed with moderate to severe pulmonary disease that have altered swim and dive patterns, likely due to decreased functional lung capacity and concomitant impaired buoyancy.
The physiological adaptions of dolphin lungs that support diving and swimming in marine ecosystems may make these species more susceptible than humans and other air-breathing animals to the negative impacts associated with inhaled or aspirated contaminants. Cetaceans breathe at the air-water interface, where high levels of volatile compounds and oil-containing aerosols and droplets were released from the DWH oil slick (de Gouw et al., 2011; Ryerson et al., 2012). Adult bottlenose dolphins take rapid breaths that begin with an explosive exhalation and are followed by deep inhalation of ~10 liters of surrounding air into their lungs, all in less than a second (Ridgway et al., 1969; Piscitelli et al., 2013). This air intake goes directly from the blowhole to the dolphin’s lungs, without protective cilia or nasal turbinates to filter the air. Dolphins often hold their breath for several minutes while swimming, foraging, and interacting with others, before returning to the surface of the water to take several breaths. During these respiratory cycles, dolphins exchange up to 90% of deep lung air (Ridgway et al., 1969), which could allow contaminants ample time and opportunity to impact the lung tissue.
A potential route of oil exposure in marine mammals is aspiration of liquid oil from the surface of the water and/or aerosolized oil droplets (Takeshita et al., 2017). During the DWH oil spill event, dolphins were seen surfacing in oil slicks and others were documented with crude oil on their heads (Figure 1) (Schwacke et al., 2014). In animals, aspiration of petroleum products (e.g. kerosene, crude oil) can cause direct injury to lung tissue or lead to aspiration pneumonia (Coppock et al., 2012; Mostrom, 2021). In dolphins, active infectious pneumonia of varying severity has been well documented (Smith et al., 2012). Less frequently, aspiration pneumonia has been diagnosed and managed (CRS, FMG, JMM unpublished). For animals in human care, clinical evidence typically includes elevated blood-based markers of systemic inflammation, confirmation of pneumonia on radiography and/or computed tomography, isolation of infectious agents from respiratory tract sampling, and a positive response to therapy. When treatment is effective, complete or near-complete resolution of the pulmonary abnormalities can occur. In wild dolphins with no access to medical treatment, aspiration pneumonia could be fatal, and if survived, could plausibly lead to chronic, pulmonary disease.
Moderate to severe AIS remains a persistent finding in BB dolphins (2011-2018). Possible causes include pneumonia, pulmonary edema, and pulmonary fibrosis (Smith et al., 2012). During the initial health assessments of BB dolphins (2011), infectious pneumonia was considered the most likely cause of AIS based on associated ultrasound findings (such as consolidation) and blood-based evidence of inflammation, anemia, and possible septicemia (Schwacke et al., 2014). In subsequent years (2013-2014), the animals’ overall health status stabilized somewhat, and chronic, pulmonary diseases were considered more likely than active, infectious pneumonias (Smith et al., 2017). From 2016-2018, the clinical picture slightly deteriorated, with increasing prevalence of severe lung disease. Several of the BB dolphins had a pattern of AIS that was more severe ventrally than dorsally (2016-2018).
This AIS pattern has been previously reported in three cetaceans with pulmonary edema; two geriatric bottlenose dolphins with congestive heart failure (Smith et al., 2012) and one mature vaquita porpoise (Phocoena sinus) that developed pulmonary edema secondary to capture myopathy (Rojas-Bracho et al., 2019). However, we considered pulmonary edema unlikely in most BB dolphin cases given the lack of additional clinical findings to support this diagnosis. Although a concurrent cardiac health study showed a higher prevalence of heart chamber abnormalities in BB dolphins than SB dolphins (e.g. thinner left ventricular walls, smaller left atria, tricuspid valve prolapse and thickening, aortic valve thickening) as diagnosed with echocardiography (Linnehan et al., 2021), the cardiac abnormalities in most animals were not considered significant enough to cause clinical manifestations. Additional data would be needed to determine if low-grade underlying cardiac abnormalities could lead to secondary pulmonary edema, specifically under brief capture conditions.
Chronic, progressive pulmonary fibrosis was also considered as a potential explanation for the AIS seen in BB dolphins. Pulmonary fibrosis occurs as a consequence of significant lung injury and is associated with scarring that can progress over time and eventually lead to death. There are numerous types and causes of pulmonary fibrosis, including etiologies related to environmental exposure to chemicals and pathogens (Huang and Tang, 2021; Vlahovich and Sood, 2021; Moss et al., 2022). Although pulmonary fibrosis hasn’t been definitely diagnosed with ultrasound in dolphins, ultrasound findings have been described in human patients. These include pleural thickening, pleural irregularity, and an increase in B-line (or ring-down) artifacts that can coalesce with severity to create a ‘white out’ appearance (Manolescu et al., 2018). Although pleural thickness measurements have not been standardized in dolphins, we subjectively noted thickened and irregular pleura in the majority of BB dolphins, more severe ventrally than dorsally, and dolphins with severe AIS had a characteristic ‘white-out’ appearance to the lung. Therefore, it is reasonable that at least some of the BB dolphins examined had pulmonary fibrosis. Even so, there is still no clear explanation for why the fibrosis would worsen ventrally, unless it reflected a gravity-dependent inhalation pattern or previous aspiration injury within the lungs that progressed over time.
Pulmonary angiomatosis has been observed in stranded Gulf of Mexico dolphins and can cause interstitial and pleural thickening, often increasing with age (Venn-Watson et al., 2015b). While the potential contribution of underlying pulmonary angiomatosis to AIS needs to be further examined, there are no current data to support that angiomatosis worsens ventrally in the lung, or would be more prevalent in BB versus SB dolphins. A critical need exists to collect and study the carcasses of BB dolphins. Histopathologic analysis of their lung tissue could help determine if chronic progressive pulmonary fibrosis, or another pulmonary interstitial disease, has emerged as a health issue in BB dolphins as a consequence of the DWH oil spill.
Pulmonary damage and subsequent respiratory compromise are not unusual findings several years after exposure to an oil spill. Respiratory symptoms following inhalation exposure from various spills were reported in humans, including the DWH disaster (Alexander et al., 2018; Rusiecki et al., 2018). Experimental studies involving fish and mice exposed to DWH oil and/or associated chemicals reported respiratory injury (Brown-Peterson et al., 2015; Jaligama et al., 2015; Pan et al., 2018). Potential mechanisms of primary injury have been compared across taxa, including oxidative damage, cellular damage, and cellular necrosis (Takeshita et al., 2021). Secondary pathways have also been investigated, including immunotoxicity, cardiotoxicity, and chronic stress.
Based on the comprehensive examination of all available data collected to date, chronic pulmonary disease was likely a significant factor in the overall poor health of dolphins living within the DWH oil spill footprint. There were additional consequences to dolphins that sustained this injury. To help define clinical significance to individual animals, oxygenation and blood gas analyses were conducted (2016 and 2017), which identified evidence of compensatory acid-base disturbances in dolphins with lung disease (Sharp et al., 2017). Poor pulmonary health and acid-base imbalances could contribute to the sustained high rates of dolphin reproductive failure in BB dolphins (Lane et al., 2015; Kellar et al., 2016; Smith et al., 2020), as maternal illness and related adverse health outcomes could put pregnancies at risk and impact a female dolphin’s ability to adequately care for her calf. Additionally, overall health scores in BB dolphins showed that dolphin population health has not improved over time (2011-2018) and in some cases has worsened (Schwacke et al., 2022).
A slight increase in prevalence of moderate lung disease was seen in pre-spill SB dolphins (comparison site) sampled during 2016 and 2017. Sample size for this cohort was very limited (5 in 2016; 8 in 2017), so caution is warranted when interpreting these findings. Furthermore, there were no documented cases of pulmonary masses, pulmonary consolidation, or ventrally severe AIS in SB during 2017 or 2018. Additionally, no cases of severe pulmonary disease were diagnosed in SB during the entire post-DWH disaster study period, compared to a prevalence of 0.23 in 2017 and 0.22 in 2018 in oiled BB.
Dolphins born after the DWH oil spill did not have an elevated prevalence of pulmonary disease. In fact, the prevalence of moderate to severe lung disease in BB dolphins born post-spill was similar to SB dolphins not impacted by the DWH oil spill, which is an encouraging finding for this generation of BB dolphins. Although these animals were relatively young at the time of exam (<10 years old), our previous study showed that age was not a significant factor in the prevalence of moderate to severe lung disease in BB dolphins (Smith et al., 2017). Additional monitoring studies will be needed to determine if the prevalence of lung disease in dolphins born post-spill remains similar to SB over time.
This study showed strong evidence of chronic and potentially progressive respiratory injury in bottlenose dolphins living within the DWH oil spill footprint. Other cetacean species living farther off-shore were also exposed, but their health was difficult to evaluate and few data exist to determine the potential adverse health impacts. However, the nearshore bottlenose dolphin data suggests that any cetacean with a similar exposure to DWH oil or its byproducts could sustain a similar injury. Long-term monitoring of dolphin populations living within the oil spill footprint is critical to fully understand the potential for and timeline of individual and population recovery from the impacts of a such a large-scale oil spill event, and to extrapolate impacts on other cetacean populations in the NGoM and elsewhere. As long-lived, air-breathing mammals that live, feed, and breed along the coastline, dolphins can be considered a sentinel species and provide valuable insight into the chronic effects of oil and associated contaminants on animal, human, and ecosystem health.
Data availability statement
Data are publicly available through the Gulf of Mexico Research Initiative Information & Data Cooperative (GRIIDC) at https://data.gulfresearchinitiative.org (doi: 10.7266/N7H41PTV, 10.7266/n7-76aj-rp39, 10.7266/n7-sv57-1h12).
Ethics statement
The animal study was reviewed and approved by National Marine Fisheries Service’s Institutional Animal Care and Use Committee and Mote Marine Laboratory’s Institutional Animal Care and Use Committee.
Author contributions
CS: conceptualization, methodology, formal analysis, investigation, writing – original draft, visualization, supervision, project administration, funding acquisition. TR: conceptualization, methodology, writing – review and editing, supervision, resources, project administration, funding acquisition. FG: investigation, formal analysis, writing – review and editing, supervision, project administration, funding acquisition. MI: investigation, formal analysis, writing – review and editing, funding acquisition. KC: investigation, formal analysis, writing – review and editing. RT: formal analysis, data curation, validation, writing – review and editing. FT: investigation, writing – review and editing. EZ: investigation, writing – review and editing. JM: data curation, synthesis, and analysis, writing – review and editing. VC: investigation, writing – review and editing. JM: investigation, writing – review and editing. WM: investigation, data synthesis, writing – review and editing. TS: investigation, writing – review and editing. AB: data synthesis, writing – review and editing. RW: investigation, writing – review and editing, resources, funding acquisition. LS: conceptualization, methodology, statistical analysis, validation, writing – review and editing, visualization, project administration, funding acquisition. All authors contributed to the article and approved the submitted version.
Acknowledgments
This research was made possible in part by a grant from The Gulf of Mexico Research Initiative (Award SA16-17), and as part of the Deepwater Horizon Natural Resource Damage Assessment. We appreciate the efforts of numerous organizations and their researchers, veterinarians, technicians, students, and volunteers who provided support for the health assessment projects, which include our home institutions as well as the Audubon Nature Institute, Dolphin Quest, International Fund for Animal Welfare, Louisiana Department of Wildlife and Fisheries, National Centers for Coastal Ocean Science, National Institute of Standards and Technology, and SeaWorld and Busch Gardens. We especially thank Brian Balmer, Brenda Bauer, Brian Quigley, Ross Martinson, and Larry Fulford for their valuable contributions to this research. We thank Jason Allen, Aaron Barleycorn, Sunnie Brenneman, Kerry Coughlin, James Daugomah, Sylvain De Guise, Deborah Fauquier, Gavin Goya, Larry Hansen, Craig Harms, Jean Herrman, Trip Kolkmeyer, Stephen Manley, Amanda Moors, Lydia Staggs, Jay Sweeney, Christina Toms, Blaine West, and Rob Yordi. This is the National Marine Mammal Foundation’s contribution #336 to peer-reviewed scientific literature. Data are publicly available through the Gulf of Mexico Research Initiative Information & Data Cooperative (GRIIDC) at https://data.gulfresearchinitiative.org (doi:10.7266/N7H41PTV, 10.7266/n7-76aj-rp39, 10.7266/n7-sv57-1h12).
Conflict of interest
The authors declare that the research was conducted in the absence of any commercial or financial relationships that could be construed as a potential conflict of interest.
Publisher’s note
All claims expressed in this article are solely those of the authors and do not necessarily represent those of their affiliated organizations, or those of the publisher, the editors and the reviewers. Any product that may be evaluated in this article, or claim that may be made by its manufacturer, is not guaranteed or endorsed by the publisher.
References
Aichinger Dias L., Litz J., Garrison L., Martinez A., Barry K., Speakman T. (2017). Exposure of cetaceans to petroleum products following the deepwater horizon oil spill in the gulf of Mexico. Endang. Species Res. 33, 119–125. doi: 10.1016/j.envres.2017.11.044
Alexander M., Engel L. S., Olaiya N., Wang L., Barrett J., Weems L., et al. (2018). The deepwater horizon oil spill coast guard cohort study: A cross-sectional study of acute respiratory health symptoms. Environ. Res. 162, 196–202. doi: 10.1016/j.envres.2017.11.044
Baad M., Feng Lu Z., Reiser I., Paushter D. (2017). Clinical significance of US artifacts. RadioGraphics 37, 1408–1423. doi: 10.1148/rg.2017160175
Balmer B. C., Ylitalo G. M., McGeorge L. E., Baugh K. L., Boyd D., Mullin K. D., et al. (2015). Persistent organic pollutants (POPs) in blubber of common bottlenose dolphins (Tursiops truncatus) along the northern gulf of Mexico coast, USA. Sci. Total Environ. 527, 306–312. doi: 10.1016/j.scitotenv.2015.05.016
Brown-Peterson N. J., Krasnec M., Takeshita R., Ryan C. N., Griffitt K. J., Lay C., et al. (2015). A multiple endpoint analysis of the effects of chronic exposure to sediment contaminated with deepwater horizon oil on juvenile southern flounder and their associated microbiomes. Aquat. Tox. 165, 197–209. doi: 10.1016/j.aquatox.2015.06.001
Colegrove K. M., Venn-Watson S., Litz J., Kinsel M. J., Terio K. A., Fougeres E., et al. (2016). Fetal distress and in utero pneumonia in perinatal dolphins during the northern gulf of Mexico unusual mortality event. Dis. Aquat. Org. 119, 1–16. doi: 10.3354/dao02969
Coppock R. W., Christian R. G., Gupta R. C. (2012). “Petroleum,” in Veterinary toxicology basic and clinical principals (New York: Elsevier), 745–778.
Deepwater Horizon Natural Resource Damage Assessment (NRDA) Trustees (2016) Deepwater horizon oil spill: Final programmatic damage assessment and restoration plan and final programmatic environmental impact statement. Available at: http://www.gulfspillrestoration.noaa.gov/restoration-planning/gulf-plan.
de Gouw J. A., Middlebrook A. M., Warneke C., Ahmadov R., Atlas E. L., Bahreini R., et al. (2011). Organic aerosol formation downwind from the deepwater horizon oil spill. Science 331, 1295–1299. doi: 10.1126/science.1200320
De Guise S., Levin M., Jasperse L., Herrman J., Wells R. S., Rowles T., et al. (2021). Long-term immunological alterations in bottlenose dolphins a decade after the deepwater horizon oil spill in the northern gulf of Mexico: Potential for multigenerational effects. Environ. Toxicol. Chem. 40 (5), 1308–1321. doi: 10.1002/etc.49
EPA (2011). EPA Should clarify and strengthen its waste management oversight role with respect to oil spills of national significance. office of the inspector general report no. 11-P-0706 (Washington, DC: U.S: . Environmental Protection Agency). Available at: https://www.epa.gov/sites/default/files/2015-10/documents/20110926-11-p-0706.pdf.
ERMA (2015). Environmental response management application (Washington, DC: National Oceanic and Atmospheric Administration).
Gam K. B., Engel L. S., Kwok R. K., Curry M. D., Stewart P. A., Stenzel P. R., et al. (2018b). Association between deepwater horizon oil spill response and cleanup work experiences and lung function. Environ. Int. 121 (Pt 1), 695–702. doi: 10.1016/j.envint.2018.09.058
Gam K. B., Kwok R. K., Engel L. S., Curry M. D., Stewart P. A., Stenzel M. R., et al. (2018a). Lung function in oil spill response workers 1-3 years after the deepwater horizon disaster. Epidemiology 29 (3), 315–322. doi: 10.1097/EDE.0000000000000808
Godard-Codding C. A., Collier T. K. (2018). The effects of oil exposure on cetaceans. In Marine Mammal Ecotoxicology: Impacts of Multiple Stressors on Population Health (Elsevier Inc.) 75–93. doi: 10.1016/B978-0-12-812144-3.00003-6
Herrman J. M., Morey J. S., Takeshita R., De Guise S., Wells R. S., McFee W., et al. (2020). Age determination of common bottlenose dolphins (Tursiops truncatus) using dental radiography pulp:tooth area ratio measurements. PLoS one 15(11): e0242273. doi: 10.1371/journal.pone.0242273
Huang W. J., Tang X. X. (2021). Virus infection induced pulmonary fibrosis. J. Transl. Med. 19, 496. doi: 10.1186/s12967-021-03159-9
Jaligama S., Chen Z., Saravia J., Yadav N., Lomnicki S. M., Dugas T. R., et al. (2015). Exposure to deepwater horizon crude oil burnoff particulate matter induces pulmonary inflammation and alters adaptive immune response. Environ. Sci. Technol. 49 (14), 8769–8776. doi: 10.1021/acs.est.5b01439
Jung S. C., Kim K. M., Lee K. S., Roh S., Jeong W. C., Kwak S. J., et al. (2013). Respiratory effects of the hebei spirit oil spill on children in taean, Korea. Allergy Asthma Immunol. Res. 5 (6), 365–370. doi: 10.4168/aair.2013.5.6.365
Kellar N. M., Lane S. M., Smith C. R., Speakman T. R., Balmer B. C., Trego M. L., et al. (2016). Low reproductive success rates of common bottlenose dolphins (Tursiops truncatus) in the northern gulf of Mexico following the deepwater horizon disaster, (2010–2015). Endang. Species Res. 33, 143–158. doi: 10.3354/ESR00775
Lane S. M., Smith C. R., Mitchell J., Balmer B. C., Barry K. P., McDonald T., et al. (2015). Reproductive outcome and survival of common bottlenose dolphins sampled in barataria bay, Louisiana, USA, following the deepwater horizon oil spill. Proc. Biol. Sci. 282, 20151944. doi: 10.1098/rspb.2015.1944
Lawrence K. G., Keil A. P., Garantziotis S., Umbach D. M., Stewart P. A., Stenzel M. R., et al. (2020). Lung function in oil spill responders 4-6 years after the deepwater horizon disaster. J. Toxicol. Environ. Health A. 83 (6), 233–248. doi: 10.1080/15287394.2020.1745111
Linnehan B. K., Gomez F. M., Huston S. M., Hsu A., Takeshita R., Colegrove K. M., et al. (2021). Cardiac assessments of bottlenose dolphins (Tursiops truncatus) in the northern gulf of Mexico following exposure to deepwater horizon oil. PloS One 16 (12), e0261112. doi: 10.1371/journal.pone.0261112
Litz J., Baran M. A., Bowen-Stevens S. R., Carmichael R. H., Colegrove K. M., Garrison L. P., et al. (2014). Review of historical unusual mortality events (UMEs) in the gulf of mexico, (1990–2009): Providing context for the multi-year northern gulf of Mexico cetacean UME declared in 2010. Dis. Aquat. Org. 112, 161–175. doi: 10.3354/dao02807
Manolescu D., Davidescu L., Traila D., Oancea C., Tudorache V. (2018). The reliability of lung ultrasound in assessment of idiopathic pulmonary fibrosis. Clin. Interventions Aging 13, 437–449. doi: 10.2147/CIA.S156615
McDonald T. L., Hornsby F., Speakman T., Zolman E., Mullin K. D., Sinclair C., et al. (2017). Survival, density, and abundance of common bottlenose dolphins in barataria bay following the deepwater horizon oil spill. Endang. Species Res. 33, 193–209. doi: 10.3354/ESR00806
Michel J., Owens E. H., Zengel S., Graham A., Nixon Z., Allard T., et al. (2013). Extent and degree of shoreline oiling: Deepwater horizon oil spill, gulf of Mexico, USA. PloS One 8 (6), e65087. doi: 10.1371/journal.pone.0065087
Moss B. J., Stefan W. R., Rosas I. O. (2022). Pathogenic mechanisms underlying idiopathic pulmonary fibrosis. Annu. Rev. Pathol. 17, 515–546. doi: 10.1146/annurev-pathol-042320-030240
Mostrom M. S. (2021). “Petroleum product poisoning in animals,” in Merck Veterinary manual (Rahway, NJ: Merck & Co., Inc). Available at: https://www.merckvetmanual.com/toxicology/petroleum-product-poisoning/petroleum-product-poisoning-in-animals.
Pan Y. K., Khursigara A. J., Johansen J. L., Esbaugh A. J. (2018). The effects of oil induced respiratory impairment on two indices of hypoxia tolerance in Atlantic croaker (Micropogonias undulatus). Chemosphere 200, 143–150. doi: 10.1016/j.chemosphere.2018.02.028
Percie du Sert N., Hurst V., Ahluwalia A., Alam S., Avey M. T., Baker M., et al. (2020). The ARRIVE guidelines 2.0: Updated guidelines for reporting animal research. PloS Biol. 18 (7), e3000410. doi: 10.1371/journal.pbio.3000410
Piscitelli M. A., Raverty S. A., Lillie M. A., Shadwick R. E. (2013). A review of cetacean lung morphology and mechanics. J. Morphol. 274 (12), 1425–1440. doi: 10.1002/jmor.20192
Quigley B. M., Speakman T. R., Balmer B. C., Gorgone A. M., Rowles T. K., Sinclair C., et al. (2022). Observations of a benthic foraging behavior used by common bottlenose dolphins (Tursiops truncatus) in barataria basin, Louisiana, USA. Aquat. Mammals 48 (2), 159–166. doi: 10.1578/AM.48.2.2022.159
R Core Team (2021). The r project for statistical computing (Vienna, Austria: R Foundation for Statistical Computing). Available at: http://www.R-project.org/.
Ridgway S. H., Scronce B. L., Kanwisher J. (1969). Respiration and deep diving in the bottlenose porpoise. Science 166 (3913), 1651–1654. doi: 10.1126/science.166.3913.1651
Rojas-Bracho L., Gulland F. M. D., Smith C. R., Taylor B., Wells R. S., Thomas P. O., et al. (2019). A field effort to capture critically endangered vaquitas Phocoena sinus for protection from entanglement in illegal gillnets. Endang. Species Res. 38, 11–27. doi: 10.3354/esr00931
Rusiecki J., Alexander M., Schwartz E. G., Wang L., Weems L., Barrett J., et al. (2018). The deepwater horizon oil spill coast guard study. Occup. Environ. Med. 75 (3), 165–175. doi: 10.1136/oemed-2017-104343
Ryerson T. B., Camilli R., Kessler J. D., Kujawinski E. B., Reddy C. M., Valentine D. L., et al. (2012). Chemical data quantify deepwater horizon hydrocarbon flow rate and environmental distribution. Proc. Natl. Acad. Sci. U.S.A. 109 (50), 20246–20253. doi: 10.1073/pnas.1110564109
Schwacke L. H., Marques T. A., Thomas L., Booth C. G., Balmer B. C., Barratclough A., et al. (2022). Modeling population effects of the deepwater horizon oil spill on a long-lived species. Conserv. Biol. 17, 36(4), e13878. doi: 10.1111/cobi.13878
Schwacke L. H., Smith C. R., Gomez F. M., Rowles T. K., Wells R. S., Zolman E. S., et al. (2020). “Health trends for bottlenose dolphins in the 8 years following the deepwater horizon oil spill: Evidence for lack of resilience,” in Proceedings of the gulf of Mexico oil spill & ecosystem science conference(Tampa, FL), 3–6.
Schwacke L. H., Smith C. R., Townsend F. I., Wells R. S., Hart L. B., Balmer B. C., et al. (2014). Health of common bottlenose dolphins (Tursiops truncatus) in barataria bay, Louisiana, following the deepwater horizon oil spill. Environ. Sci. Technol. 48 (1), 93–103. doi: 10.1021/es403610f
Sharp S. M., Baird M., Cendejas V., Gomez F. G., Meegan J. M., Townsend F. I., et al. (2017). “A pilot study to evaluate the utility of blood gas analysis and capnography in determining maternal oxygenation status in bottlenose dolphins (Tursiops truncatus) following the deepwater horizon oil spill,” in Proceedings of the international association of aquatic animal medicine annual meeting, may 20-24, 2017(Cancun, Mexico).
Simpson J. G., Gardner M. B. (1972). “Comparative microscopic anatomy of selected marine mammals,” in Mammals of the Sea: Biology and medicine. Ed. Ridgway S. H. (Springfield, IL: Charles C. Thomas Publishers), 298–418.
Smith C. R., Gomez F. G., Colegrove K. M., Rowles T. K., Zolman E. S., Speakman T. R., et al. (2020). “Sustained maternal illness and low reproductive success rate in barataria bay bottlenose dolphins following the deepwater horizon oil spill,” in Proceedings of the gulf of Mexico oil spill & ecosystem science conference, Feb 3-6, 2017(Tampa, FL).
Smith C. R., Rowles T. K., Hart L. B., Townsend F. I., Wells R. S., Zolman E. S., et al. (2017). Slow recovery of barataria bay dolphin health following the deepwater horizon oil spill, (2013-2014), with evidence of persistent lung disease and impaired stress response. Endang. Species Res. 33, 127–142. doi: 10.3354/ESR00778
Smith C. R., Solano M., Lutmerding B. A., Johnson S. P., Meegan J. M., Le-Bert C. R., et al. (2012). Pulmonary ultrasound findings in a bottlenose dolphin Tursiops truncatus population. Dis. Aquat. Org. 101, 243–255. doi: 10.3354/dao02537
Speakman T. R., Lane S. M., Schwacke L. H., Fair P. A., Zolman E. S. (2010). Mark-recapture estimates of seasonal abundance and survivorship for bottlenose dolphins (Tursiops truncatus) near Charleston, south Carolina. J. Cetacean Res. Manage. 11 (2), 153–162.
Takeshita R., Bursian S. J., Colegrove K. M., Collier T. K., Deak K., Dean K. M., et al. (2021). A review of the toxicology of oil in vertebrates: What we have learned following the Deepwater horizon oil spill. J. Toxicol. Environ. Health Part B Crit. Rev. 24 (8), 355–394. doi: 10.1080/10937404.2021.1975182
Takeshita R., Sullivan L., Smith C., Collier T., Hall A., Brosnan T., et al. (2017). The deepwater horizon oil spill marine mammal injury assessment. Endang. Species Res. 33, 95–106. doi: 10.3354/esr00808
U.S. v. BP, et al. (2015). “Findings of fact and conclusions of law: Phase two trial,” in Oil spill by the oil rig deepwater horizon in the gulf of Mexico, on April 20, 2010, no. MDL 2179, 2015 WL 225421. LA. E.D. jan. 15, 2015. doc. 14021. U.S. district court for the Eastern district of Louisiana. Available at: http://www.laed.uscourts.gov/sites/default/files/OilSpill/Orders/1152015FindingsPhaseTwo.pdf.
Venn-Watson S., Colegrove K. M., Litz J., Kinsel M., Terio K., Saliki J., et al. (2015b). Adrenal gland and lung lesions in gulf of Mexico common bottlenose dolphins (Tursiops truncatus) found dead following the deepwater horizon oil spill. PloS One 10 (5), e0126538. doi: 10.1371/journal.pone.0126538
Venn-Watson S., Garrison L., Litz J., Fougères E. M., Mase B., Rappucci G., et al. (2015a). Demographic clusters identified within the northern gulf of Mexico common bottlenose dolphin (Tursiops truncatus) unusual mortality event: January 2010-June 2013. PloS One 10 (2), e0117248. doi: 10.1371/journal.pone.0117248
Vlahovich K. P., Sood A. (2021). A 2019 update on occupational lung diseases: A narrative review. Pulmonary Ther. 7 (1), 75–87. doi: 10.1007/s41030-020-00143-4
Wells R. S., Rhinehart H. L., Hansen L. J., Sweeney J. C., Townsend F. I., Stone R., et al. (2004). Bottlenose dolphins as marine ecosystem sentinels: Developing a health monitoring system. EcoHealth 1, 246–254. doi: 10.1007/s10393-004-0094-6
Wells R. S., Schwacke L. H., Rowles T. K., Balmer B. C., Zolman E., Speakman T., et al. (2017). Ranging patterns of common bottlenose dolphins Tursiops truncatus in Barataria bay, Louisiana, following the deepwater horizon oil spill. Endang. Species Res. 33, 159–180. doi: 10.3354/esr00732
Wells R. S., Scott M. D. (1990). Estimating bottlenose dolphin population parameters from individual identification and capture-release techniques. Rep. Int. Whaling Commission. Special Issue 12, 407–415.
Wilkin S. M., Rowles T. K., Stratton E., Adimey N., Field C. L., Wissmann S., et al. (2017). Marine mammal response operations during the MC-252/Deepwater horizon oil spill. Endang. Species Res. 33, 107–118. doi: 10.3354/esr00811
Wood S. N. (2017). Generalized additive models: An introduction with r. 2nd edition (New York: Chapman and Hall/CRC).
Zock J. P., Rodríguez-Trigo G., Pozo-Rodríguez F., Barberà J. A., Bouso L., Torralba Y., et al. (2007). Prolonged respiratory symptoms in clean-up workers of the prestige oil spill. Am. J. Respir. Crit. Care Med. 176 (6), 610–616. doi: 10.1164/rccm.200701-016OC
Zock J. P., Rodríguez-Trigo G., Pozo-Rodríguez E., Espinosa A., Pozo-Rodríguez F., Gómez F., et al. (2012). Persistent respiratory symptoms in clean-up workers 5 years after the prestige oil spill. Occup. Environ. Med. 69 (7), 508–513. doi: 10.1136/oemed-2011-100614
Zock J. P., Rodríguez-Trigo G., Pozo-Rodríguez E., Souto-Alonso A., Espinosa A., Pozo-Rodríguez F., et al. (2014). Evaluation of the persistence of functional and biological respiratory health effects in clean-up workers 6 years after the prestige oil spill. Environ. Int. 62, 72–77. doi: 10.1016/j.envint.2013.09.020
Keywords: Deepwater Horizon, oil spill, dolphin, pulmonary, health
Citation: Smith CR, Rowles TK, Gomez FM, Ivančić M, Colegrove KM, Takeshita R, Townsend FI, Zolman ES, Morey JS, Cendejas V, Meegan JM, Musser W, Speakman TR, Barratclough A, Wells RS and Schwacke LH (2022) Poor pulmonary health in Barataria Bay dolphins in the eight years following the Deepwater Horizon oil spill. Front. Mar. Sci. 9:975006. doi: 10.3389/fmars.2022.975006
Received: 21 June 2022; Accepted: 18 August 2022;
Published: 08 September 2022.
Edited by:
Samantha Shields, St. Matthew’s University, Cayman IslandsReviewed by:
Vanessa Labrada Martagón, Autonomous University of San Luis Potosí, MexicoMaurizio Migliaccio, Parthenope University of Naples, Italy
Meaghan McCormack, SUNY College of Environmental Science and Forestry, United States
Copyright © 2022 Smith, Rowles, Gomez, Ivančić, Colegrove, Takeshita, Townsend, Zolman, Morey, Cendejas, Meegan, Musser, Speakman, Barratclough, Wells and Schwacke. This is an open-access article distributed under the terms of the Creative Commons Attribution License (CC BY). The use, distribution or reproduction in other forums is permitted, provided the original author(s) and the copyright owner(s) are credited and that the original publication in this journal is cited, in accordance with accepted academic practice. No use, distribution or reproduction is permitted which does not comply with these terms.
*Correspondence: Cynthia R. Smith, Y3ludGhpYS5zbWl0aEBubW1mLm9yZw==