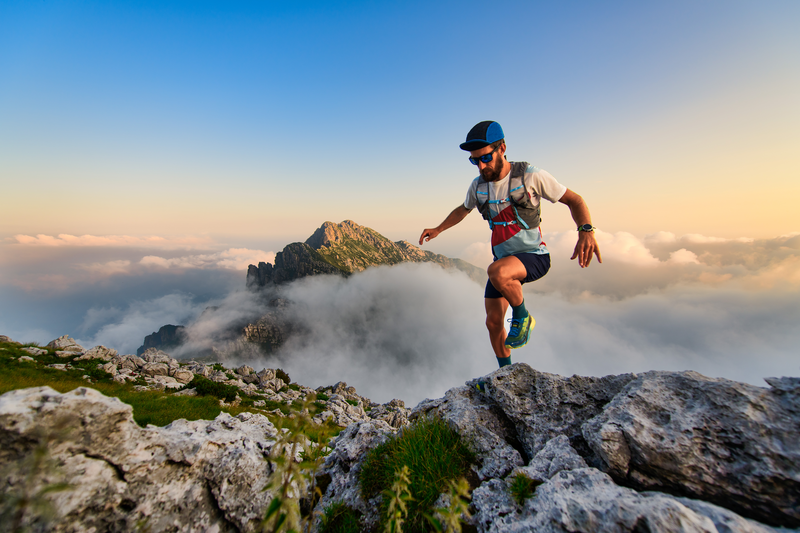
94% of researchers rate our articles as excellent or good
Learn more about the work of our research integrity team to safeguard the quality of each article we publish.
Find out more
ORIGINAL RESEARCH article
Front. Mar. Sci. , 30 August 2022
Sec. Marine Biogeochemistry
Volume 9 - 2022 | https://doi.org/10.3389/fmars.2022.973250
This article is part of the Research Topic Marine Microalgae and Biotoxins View all 5 articles
The dinoflagellate genus Prorocentrum includes several harmful toxigenic species, predominantly benthic ones. In the past, fast-acting toxicity in mice has been observed in extracts of the epibenthic species P. borbonicum, with the finding of two compounds termed as borbotoxins-A and -B. The presence of palytoxin-like compounds was also suggested from electrophysiological experiments. In the present study, a strain of P. borbonicum was isolated in the continental coast of Colombian Caribbean, in seagrass beds of Thalassia testudinum in Bonito Gordo, Tayrona National Natural Park (PNNT). The morphological and molecular characteristics were consistent with the original and former descriptions for this species. Typical haemolytic activity due to palytoxin was confirmed in P. borbonicum extracts in presence of ouabain, the toxin contents being estimated as 1.9 pg palytoxin equivalents cell-1. HPLC-HRMS analyses of these extracts unambiguously identified the presence of borbotoxins and 42-hidroxy-palytoxin (42-OH-PLTX). This is the first report of palytoxin-like compounds in another dinoflagellate genus than Ostreopsis and the first record of P. borbonicum in Colombia and the Caribbean region.
The genus Prorocentrum was first established by Ehrenberg (1834) with P. micans as the type species (Fukuyo, 1981). Prorocentroids are a group of dinoflagellates characterized by presenting laterally compressed cells, completely covered by two lateral thecal plates, with contours that can be rounded, oval, ovoid or pyriform, reviewed in detail by Hoppenrath et al. (2013). They do not have a cingulum or sulcus; with the insertion of its two flagella towards the apical area emerging through one of the two pores surrounded by a cluster composed of several fine platelets known as the periflagellar area, located in the right thecal plate. The vast majority are marine and, depending on the species, they can present planktonic and/or epibenthic habitat, living on inert substrates (dead coral, sediments, mollusk shells, sandy bottoms, submerged or floating detrital aggregates, among others) or living (macroalgae, marine grasses, mangrove roots and seagrasses, etc; Glibert et al., 2012). Currently 121 species have been reported, of which 79 have taxonomic acceptance according to the AlgaeBase (Guiry and Guiry, 2021). Approximately half of these display epibenthic habits.
In addition to their importance in the food web (Fukuyo, 1981), some species, generally epibenthic, are involved in harmful algal blooms, 13 of which are included in the IOC-UNESCO taxonomic reference list of harmful algae (Lundholm et al., 2021). These Prorocentrum species are known as producers of several biotoxins such as okadaic acid (OA) and its analogues, dinophysitoxins, borbotoxins, fast-acting toxins and haemolytic toxins (Ten-Hage et al., 2000; Heredia-Tapia et al., 2002; Pearce et al., 2005; Hoppenrath et al., 2013; Amar et al., 2018 and Nakajima et al., 1981; Yasumoto et al., 1987; Jackson et al., 1993; Hu et al., 1993; Ten-Hage et al., 2002). Some of them have been related with diarrhetic shellfish poisoning (DSP) intoxications in humans, like P. lima (Gayoso et al., 2002). However, until now it has not been clearly established a relationship between the presence of these organisms and DSP events (Levasseur et al., 2003; Foden et al., 2005).
In addition to the above mentioned biotoxins, a variety of secondary bioactive metabolites have been characterized in Prorocentrum spp., including belizeanolide, formosalides, hoffmaniolide, prorocentrolides and prorocentin (Kobayashi and Kubota, 2010). In the case of P. borbonicum, haemolytic and fast-acting toxins have been found, displaying symptoms of toxicity different to DSP and PSP (Ten-Hage et al., 2000; Ten-Hage et al., 2002). These authors suggested the presence of palytoxin-like compounds in the butanol-soluble fraction of P. borbonicum extracts based on results of electrophysiological experiments. However, only borbotoxin-A was isolated and purified, demonstrating that the main action of this compound is to block post-synaptic nicotinic ACh receptors (Ten-Hage et al., 2002).
In the present study, a strain of P. borbonicum from the continental coast of Colombian Caribbean was isolated and identified based on morphology and LSU rDNA phylogenetic analyses. Toxicity was examined using a haemolytic assay and P. borbonicum extracts were prepared for further HPLC-HRMS analyses. The obtained results provided the confirmation and first report of a palytoxin analogue (42-hidroxy-palytoxin (42-OH-PLTX) in another dinoflagellate genus than Ostreopsis. This finding adds a new species that should be considered as a potential source for these compounds in the marine food chain, associated with clupeotoxism. This study represents also the first report of P. borbonicum in Colombia.
The Prorocentrum strain INV MYZ0003 was obtained from leaves of Thalassia testudinum (Koenig, 1805) collected on March 2018 in a seagrass bed at Bonito Gordo (11°19´16,4 N; 74°07´36,5 W, 2 m depth), in Tayrona National Natural Park (PNNT), near the city of Santa Marta (Magdalena department, Colombian Caribbean; Figure 1). T. testudinum leaves were obtained by snorkeling at a depth of around 2 m, gently cut from their base to avoid detachment of epiphytes and carefully placed in hermetically sealed plastic bags (Ziploc®) with seawater and airtight closure.
Figure 1 Sampling localization at Concha bay (Bonito Gordo), in the Tayrona National Natural Park (TNNP), Colombia.
In the laboratory, bags were shaken to loosen the adhered organisms, after which most of the leaves were manually removed. The seawater containing the released organisms was passed through a 250 µm sieve to retain particle debris and remaining vegetal material. The obtained filtrate was used to search and isolate single dinoflagellate cells in aliquots on excavated slides under a Nikon inverted microscope, Eclipse TS100 (Nikon Corporation, Tokyo, Japan). Single cells isolation was carried out manually with capillary pipettes adapted to a silicone hose to pick up the organisms of interest. Once isolated, cells were transferred to another excavated slide with a drop of sterile filtered seawater. The procedure for transferring the cell into sterile seawater was repeated several times until no contaminants were observed.
The isolated single cells were inoculated in a 96-well microplate containing K culture medium (Keller et al., 1987) diluted by half and without added silicates, prepared with seawater from the source area, sterilized and adjusted to salinity of 36. Cells were maintained at temperature of 25°C and a photoperiod of 12:12 h light/dark with a photon irradiance of about 90 µmol quanta m-2s-1 of PAR (Biospherical Instruments Inc. San Diego, CA, USA), using cool-white fluorescent lamps. In the wells where active growth was observed, cells were transferred and kept into new 7.4 mL glass vials and 20 mL flasks, under the same conditions.
Cells of the Prorocentrum strain INV MYZ0003 were observed under a Leica DMLA light microscope (Leica Microsystems GmbH, Wetzlar, Germany) equipped with epifluorescence with UV lamp and with an AxioCam HR3 photographic camera (Zeiss, Göttingen, Germany). The images in epifluorescence were obtained by applying a drop of calcofluor on the cells for staining their plates, before covering them with the coverslip (Fritz and Trimer, 1985), subsequently observing them under a UV light filter (Reguera et al., 2011). The autofluorescence of chloroplasts in living cells was registered using the same microscope and imaging equipment.
For SEM imaging, 2 mL of culture in exponential phase were treated with 4% Triton (20 min) and washed with distilled water through 10 µm membrane filters (TCTP, Isopore, Ireland) to remove external membranes. Then, cells were fixed with formaldehyde 4% for 24 hours at 5 °C, before being dehydrated by passing them (twice each step) through increasing ethanol concentrations: 30, 50, 75, 90, 95 and 100%. Then samples were dried using a critical point dryer equipment (Baltec CPD030). Afterwards, filters with specimens were mounted in a stub Finally, specimens were coated with gold using a Quorum Q150R ES sputter coater and observed with a FEI QUANTA 200-r with voltage acceleration between 20 and 25Kv. The working distance was between 8.8 and 9.6mm (Scanning Electron Microscopy Laboratory - Universidad Nacional de Colombia).
The terminology proposed by Hoppenrath et al. (2013) was followed to describe the cell orientation, as well as for the description, arrangement and enumeration of the periflagellar platelets. Therefore, we use the term anterior or “apical” for the site where the flagella emerge and “antapical” or posterior for the opposite end, with the right thecal plate being the most excavated anteriorly with respect to the left (both connected through a sagittal suture).
For DNA extraction, 3 to 5 cells of strain INV MYZ0003 were isolated by micropipetting, washed three times in drops of molecular grade distilled water and stored for 24 h at -40°C in a 200 μL tube. Then, samples were cold shocked with liquid nitrogen for 1 min and heated (94°C, 1 min) in an Eppendorf Mastercycler EP5345 (Eppendorf AG, New York, USA).
The D1-D3 regions of the LSU rRNA gene were amplified by PCR using the pair of primers D1R/LSUB (5´-ACCCGCTAATTTAAG-CATA-3´/5´-ACGAACGATTTGCACGTCAG-3’) (Scholin et al., 1994). Amplifications were performed in an Eppendorf Mastercycler EP5345, using Horse-Power™ Taq DNA Polymerase MasterMix 2x (Canvax, Spain) following manufacturer’s instructions.
PCR conditions for LSU rRNA gene amplifications were as follows: 5 minutes initial denaturing at 94°C, followed by 30 cycles of 35 s denaturing at 94°C, 35 s annealing at 51°C (LSU), and 1 min elongation at 72°C, with a final elongation step of 7 min at 72°C. The PCR products were purified with ExoSAP–IT Express (Applied Biosystems, Foster City, CA, USA). Sequencing reactions were performed using the Big Dye Terminator v.3.1 reaction cycle sequencing kit and migrated in a SeqStudio genetic analyzer (both at Applied Biosystems, Foster City, CA, USA) at the CACTI sequencing facilities (Universidade de Vigo).
LSU rRNA gene sequence of strain INV MYZ0003 (GenBank Acc. No. OM522663) was assembled in Bioedit (Hall, 1999), with a final length of 772 bp. An alignment including Prorocentrum spp. available from GenBank database was elaborated using MEGA 7 (Kumar et al., 2018), including 33 sequences from distinct species/phylotypes of Prorocentrum. Final LSU rRNA alignment included 757 positions. Sequences from Adenoides eludens (Herdman) Balech were used to root the LSU rRNA.
Phylogenetic model selection (Maximum Likelihood, ML) was performed using ModelTest tool in MEGA 7. A Kimura-2 parameter (K2+G, γ = 1.01) model was selected.
The phylogenetic relationships were also determined using Bayesian phylogenetic inference and in this case the substitution models were obtained by sampling across the entire GTR model space following the procedure described in Mr. Bayes v3.2 manuals. Bayesian trees were performed with Mr. Bayes v3.2 (Huelsenbeck and Ronquist, 2001) and the program parameters were statefreqpr = dirichlet (1,1,1,1), nst = mixed, rates = gamma. The phylogenetic analyses involved two parallel analyses, each with four chains. Starting trees for each chain were selected randomly using the default values for the Mr. Bayes program. The corresponding number of unique site patterns for LSU rRNA alignments were 276. The number of generations used in these analyses was 1,000,000. Posterior probabilities were calculated from every 100th tree sampled after log-likelihood stabilization (‘‘burn-in’’ phase). Maximum Likelihood phylogenetic analyses were conducted in MEGA 7. Bootstrap values were estimated from 1,000 replicates.
Overall topologies by ML and Bayesian inference (BI) method were very similar. The phylogenetic trees were represented using the ML method with bootstrap values and posterior probabilities from the BI. Uncorrected p-distances [proportion (p) of nucleotide sites at which two sequences are different (Transitions + Transversions), i.e. proportion of nucleotide sites that are different] were calculated using MEGA 7. Thus, no corrections for multiple substitutions at the same site, substitution rate biases (e.g., differences in the transitional and transversional rates), or differences in evolutionary rates among sites are considered (Nei and Kumar, 2000).
Prorocentrum borbonicum was grown in one 500 mL Erlenmeyer flask containing 218 mL of K culture medium (Keller et al., 1987) diluted by half and without added silicates under the same culture conditions previously detailed.
After the microalgal culture reached the end of the growth phase a Lugol-fixed aliquot was collected to determine cell density under the light microscope using a Sedgewick-Rafter chamber. P. borbonicum densities harvested for toxin analyses were 14880 cells mL-1. Cells were collected by filtering the whole culture through a GF/C glass fiber filter of 25 mm diameter (Whatman, International Ltd., Germany) and two consecutive extractions, each one with 1500 µL methanol:water (8:2), were performed. This methanol-water soluble extract was kept frozen (-20°C) until toxin analyses.
The haemolytic activity of the palytoxin (PLTX) standard solution obtained from Palythoa tuberculosa (Wako Chemical Industries, Ltd., Japan) and P. borbonicum extract was analyzed by delayed hemolysis of sheep erythrocytes using the PLTX antagonist ouabain following Riobó et al. (2008). Erythrocytes were separated from the plasma by centrifugation for 10 minutes at 400 x g at 10°C and washed twice with isotonic phosphate buffer (PBS) containing 0.1% bovine serum albumin (BSA), 1mM calcium chloride (CaCl2-2H2O) and 1mM boric acid (H3BO3). Then blood was diluted in PBS solution to a final concentration of 1.7 x 107 red blood cells mL-1.
One aliquot of the methanol-water soluble extract (500 µL) was dried and dissolved in 5mL of PBS solution (dilution 1:10). Assay was performed by using the following dilutions of P. borbonicum extract in the hemolysis buffer: 1:10, 1:102, 1:103 and 1:104.
Each assay was carried out in triplicate in a 96 microwell plate by mixing 150 µL of blood solution with 150 µL of diluted extract. Haemolysis controls for 0 and 100% lysis were prepared by mixing 150 µL of blood solution with 150 µL of PBS solution or distilled water respectively. Microplates were incubated for 22 h at 25°C and then the whole plate was centrifuged for 10 minutes at 400g at 10°C. From each well, 200 µL of the supernatant were transferred to a new plate and the absorbance at 405 nm was measured with a microplate reader (BioRad 550, USA). Measurements from three replicates were averaged.
The hemolytic effect of palytoxin compound from the microalgae extract was expressed as picograms of PLTX equivalents per cell (pg PLTX equiv cell-1) (Riobó et al., 2008).
HPLC-DAD analyses of methanolic-water soluble extracts of P. borbonicum and reference PLTX were performed by using an Alliance HPLC System consisting of a 2695 separations module and a Waters 996 photodiode array detector. Borbotoxins and PLTXs were determined according to Ten-Hage et al. (2002). Conditions for analyses were as follows: Toxins were separated on a reverse-phase Poroshell 120 EC-C18 column (100 x 2.1 mm, 2.7 μm particle size) maintained at 25 °C at a flow rate 0.2 mL min-1. The mobile phase consisted of (eluent A) (Milli-Q water acidified to pH 2.5 with trifluoroacetic acid) and (eluent B) acetonitrile. Gradient conditions were as follows: 20% B at 0 min, 60% B at 20 min, 100% B from 25 min to 28 min, 20% B from 32 min to 35 min (initial conditions). The column effluent was monitored by UV absorbance at 233nm (UV spectrum recorded between 200 and 325 nm). The injection volumes of P. borbonicum extract and PLTX standard (25 ng mL-1) were 100 μL and 40 μL respectively. For toxins identification a PLTX standard solution obtained from Palythoa tuberculosa (Wako Chemical Industries, Ltd., Japan) was used.
HPLC-HRMS analyses were performed by using a Thermo Scientific Dionex LC coupled to an Exactive mass spectrometer, equipped with an Orbitrap mass analyzer. Two different methods were applied in order to determine borbotoxins and palytoxins on the one hand and diarrheic shellfish toxins (DSTs) on the other hand. DSTs were determined according to the Standardized Operating Procedure (SOP) validated by the European Union Reference Laboratory for Lipophilic Marine Biotoxins (EURLMB, 2015). Borbotoxins and PLTXs were determined according to García-Altares et al. (2015).
Conditions for analyses of DSTs were as follows: analyses were performed in positive and negative modes (scan range: 100-1200 m/z). Toxins were separated on a Gemini NX C18 column (100 x 2 mm, 3µm particle size) maintained at 40 °C at a flow rate 0.4 mL min-1. The mobile phase consisted of (eluent A) 0.05% ammonia and (eluent B) 90% acetonitrile: 10% eluent A. Gradient conditions were as follows: 25%B from 0 to 1.5 min, 95%B from 7.5 to 9.5 min, 25%B from 12.5 to 16.5 min (initial conditions). For toxins identification a standard mix solution containing OA, DTX2, DTX1 and PTX2 (Cifga Laboratory, Spain) was used.
Conditions for analyses of borbotoxins and PLTXs: analyses were carried out in positive mode with and without all-ion fragmentation (AIF) (NCE 35 eV) (scan range for full MS and AIF mode: 200-3000 m/z). The column was identical to that used for HPLC-DAD analyses and eluted with the same flow rate but in this case was kept at 30°C. The mobile phases were Mili-Q water (eluent A) and 95% acetonitrile/water (eluent B), both containing 30 mM acetic acid. The following gradient elution was used: 28%B at 0 min, 50% B at 10 min, 100% B from 15 to 16 min, 28% B from 17 to 25 min (initial conditions). The volume of P. borbonicum extract and PLTX standard (25 ng mL-1) injected was 20µL. For toxins identification it was used the same PLTX standard as in the HPLC-DAD analyses.
Prorocentrum strain INV MYZ0003 exhibited symmetrical or slightly asymmetric broad oval to ovoid cells, with posterior area broadly rounded, in lateral view (Figures 2A−E). Length (L) varied between 19.0-27.4 μm (mean 23.5 ± 2.5 μm, n = 35), width (W) between 14.9-23.5 μm, (mean 20.2 ± 1.9 μm, n = 35) and L/W ratios between 0.94-1.32 (mean 1.17 ± 0.08, n = 35). Living cells containing a golden-brown chloroplast with a central pyrenoid with starch sheath (visible as ring) (Figure 2A). Both thecal plates are foveate (Figures 2D, E, 3A, B) with different sizes of scattered round pores usually inside the depressions (Figures 2D, E, 3A, B). The largest pores are less numerous and tend to be absent towards the center of the thecal plates (Figures 2B, D, E). Sometimes the pores appear to be arranged in a radial pattern. Depressions are round and more notorious at the edges and sometimes in a small central area of the cell (Figures 2D, E). Intercalary band is smooth (Figure 3B). Wide V-shaped periflagellar area (Figures 2A, E, 3A). Lacking structures such as ridges, protrusions, curved projections, wings or spines (Figure 3A). Collar on left plate was not visible. Thick flange surrounding the periflagellar area either was not observed (Figure 3A). Platelets surrounding flagellar and accessory pores may show platelet lists (Figures 2E, 3A). The accessory pore is smaller than flagellar pore (Figures 2E, 3A).
Figure 2 Prorocentrum borbonicum: light micrographs (A−C) and scanning electron micrographs (D, E). (A) Live cell, in right lateral view, p= pyrenoid, scale bar 10 μm. (B) Left thecal plate showing large thecal pores and (C) Chloroplasts. Scale bars 20 μm. (D) Cell in left lateral view and (E) Cell in right lateral view showing depressions, pores, and V-shaped periflagellar area. Scale bars 20 μm.
Figure 3 Prorocentrum borbonicum: scanning electron micrographs (A, B). (A) View of the periflagellar area and part of the right thecal plate, showing: Ap, small accessory pore; Fp, large flagellar pore; D, Depressions; Sp, small thecal pores; Lp, large thecal pore; Dp, depression with pore; and nine platelets of the periflagellar area (1, 2, 3, 4, 5, 6a, 6b, 7 and 8). (B) Theca in antapical view showing: S, suture; Ib, Intercalary band.
Following the nomenclature proposed by Hoppenrath et al. (2013), in this species nine platelets can be observed (1, 2, 3, 4, 5, 6a, 6b, 7, 8) (Figure 3A). Platelets 1 to 4 in contact to the border of the left thecal plate, while platelets 5, 6a, 6b and 1 in contact mainly with the right thecal plate (Figure 3A).
Platelet 1 triangular and excavated with one depression. This platelet connects with platelets 2, 6a, 7 and externally with the accessory pore (Figure 3A). Platelet 2 is small and square and has one depression. It is in contact with platelets 1, 3, 7 and 8, and forms the left side of the accessory pore (Figure 3A). Platelet 3 is large, rectangular and excavated in 1 depression. It is in contact with platelets 2, 4, 5 and 8 (Figure 3A). Platelet 4 is square and small, with one depression, it is in contact with platelets 3 and 5(Figure 3A). Platelet 5, characterized by being elongated and thin, with a wide curved arc shape, is in contact with platelets 3, 4, 6b forming altogether most of the right, left and ventral side of the flagellar pore; Platelet 5 generally presents an elongated depression that gives it a channel-like appearance (Figure 3A). Platelet 6 is characteristically divided two quadrangular platelets (6a and 6b), the 6a in contact with platelets 1, 6b, 8, 7 and with the right side of the accessory pore; the 6b in contact with the 6a, 5 and 8 platelets and with right side of the flagellar pore (Figure 3A). Platelet 7 adjacent to 1, is internal and rounds part of the accessory pore, it is also in contact with plate 2 (Figure 3A). Platelet 8 separates flagellar and accessory pores forming a bridge between platelet 6a and 6b in one side and platelets 2 and 3 on the other side (Figure 3A).
Partial LSU rRNA gene sequence obtained for P. borbonicum INV MYZ0003 was placed unambiguously in a molecular clade including other four P. borbonicum sequences with similar length retrieved from GenBank (Figure 4). Closest Prorocentrum species was P. sipadanense. In particular, the sequence from this study was identical to three strains originating from South China Sea. Other three sequences from P. borbonicum available in GenBank were excluded from the phylogeny given their shorter length (<600 bp). These belonged to South China Sea (MH381781, MH359126) and Florida (USA; KT898187; David, 2014) and were identical across their shared sequences to INV MYZ0003.
Figure 4 Bayesian-inferred phylogenetic tree showing relationships between Prorocentrum species. Sequence of Prorocentrum borbonicum, strain INV MYZ0003 is in bold. Node values indicate posterior probabilities and bootstrap values obtained by Maximum Likelihood method.
The methanol-water soluble extract of P. borbonicum showed typical haemolytic activity due to PLTX which was inhibited in presence of ouabain, a PLTX antagonist. Absorbance measurements of control haemolysis solutions: 0% (1 blood: 1 PBS solution) and 100% (1 blood: 1 distilled water) were used to calculate haemolysis percentages of standard and sample dilutions. Concentrations of twelve dilutions of PLTX standard ranging from 2.5 pg µL-1 to 0.025 pg µL-1 and their respective haemolysis percentages (from 88% to 5%) were used to plotting the calibration curve. Haemolytic activity percentages of dilutions 1:10 and 1:102 of the original sample extract were out of the linearity range of the calibration curve (96% and 93% respectively). Therefore, quantitation was achieved with dilutions 1:103 and 1:104 of the original extract, which percentages of haemolytic activity were 83% and 34% respectively. The calibration curve equation was obtained from the modified accumulative Weibull´s function in terms of Dose-Response model following Riobó et al. (2008). The toxin content in cells calculated with both dilutions was averaged. As result, the concentration of PLTX- like compounds in P. borbonicum strain INV MYZ0003 was estimated to be 1.9 pg PLTX equivalents cell-1.
As result of HPLC-HRMS analyses of P. borbonicum extract, lipophilic toxins (OA, DTX2, DTX1 and PTX2) were not detected while borbotoxin (A or B) and 42-hidroxy-palytoxin (42-OH-PLTX) were unambiguously identified.
Due to lack of borbotoxin calibration standard, the identification of this compound by HPLC-HRMS was based on the mass spectrum described for P. borbonicum (Reunion Island) by Ten-Hage (2002). One peak at 1.46 min was detected by monitoring pseudomolecular ions [M+H]+ at m/z 1038.6063 and the sodium adduct [M+H+Na]2+ at m/z 530.7981, both of them characteristic of borbotoxins A/B (Ten-Hage, 2002). Furthermore, the mass spectrum of this peak presented the sodium adduct [M+Na]+ at m/z 1060.5882, the potassium aduct [M+K]+ at m/z 1076.5838 and two other characteristic ions reported in borbotoxins at m/z 1054.6012 and at m/z 1070.5966 (Figure 5). The UV spectra of this compound was measured between 200 and 325 nm and showed two characteristic absorbance maxima at 234 nm and 283 nm. (Figure 6).
Figure 5 Methanolic-water soluble extract of P. borbonicum. LC-MS chromatogram monitored within the mass window m/z 1038-1039 Da (A) and mass spectrum for peak at 1.46 min (B).
Figure 6 Methanolic-water soluble extract of P. borbonicum. LC-DAD chromatogram monitored at 233 nm (left). Peaks at retention time 5.65 and 12.79 min correspond to borbotoxin A/B and 42OH-PLTX respectively. UV spectrum between 200 and 325 nm (right) at 5.65 min (at top) and 12.79 min (at bottom).
Taking into account that in reversed-phase HPLC the more polar solutes eluting first, this implies that borbotoxin compound (RT 1.46 min) is more polar than PLTX standard which was retained more time in column and eluted at 5.8 min. To analyze the presence of PLTX, extracted ion chromatograms were obtained from the HR full MS spectra for PLTX standard by selecting the most abundant ion peaks at m/z 1351.7469 [M+2H+Na]+2 and m/z 906.8226 [M+2H+Ca]+3 (corresponded to bi-charged and tri-charged ion clusters respectively) as well as the characteristic fragment ion at m/z 327.1927 (C6H27N2O5, Δ 3.825) attributed to the cleavage between carbons 8 and 9 (Figure 7).
Figure 7 Structure of PLTX molecule. The boxes show double bonds responsible of characteristic UV absorption profile. The circle shows the fragment ion at m/z 327.1927 attributed to the cleavage between carbons 8 and 9. The carbon 42 is also numbered. This carbon is bonded to one hydrogen atom or one hydroxyl group in PLTX and 42-OH-PLTX molecules respectively.
During the LC-HRMS analyses of P. borbonicum extract one major peak was detected at 5.72 min in the chromatogram monitored within the mass window m/z 326.5-327.5 Da. The mass spectrum of this peak showed abundant ion peaks at m/z 1359.7429 [M+2H+Na]+2 and m/z 912.1533 [M+2H+Ca]+3. This suggested that this compound contained 16 mass units more than PLTX (P. tuberculosa), just like 42-OH-PLTX (Ciminiello et al, 2009). The comparative analysis of the HRMS spectra of both, PLTX standard (1) and PLTX-like compound (2) showed evident structural similarities between the two compounds like the presence of the fragment ion at m/z 327.1927 and abundant peaks due to subsequent losses of water molecules from the precursor ions.
The HRMS spectra of (1) and (2) showed monocharged ions [M+2H]+ at m/z 2680.5044 (C129H225O54N3, RBD 19.0, 3.621 ppm) and m/z 2696.4996 (C129H225O55N3, RDB 19.0, 3.705 ppm) respectively. Both signals were very low to be used for assignment of molecular formulas. However, the elemental composition calculated by the Xcalibur data acquisition software agreed with the theoretical molecular formula of PLTX standard (P. tuberculosa) for (1) (C129H223O54N3) and also with subsequent evidences for assignment of molecular formula of compound 2 (C129H223O55N3) obtained from de comparative analysis of bicharged and tricharged ion clusters contained in the HRMS spectrum of (1) and (2). In particular, it was confirmed that bicharged ion clusters contained in HRMS spectrum of (2) were up-shifted by 8 mass units as compared to those of (1) and further confirmation was provided by exact masses of the most abundant tricharged ions. (Table 1).
Table 1 HRMS data of PLTX and PLTX-like compound 2 (42-OH-PLTX) obtained from full MS spectra acquired in the mass ranges m/z 2000-3000, m/z 1200-1400 and m/z 850-950.
The UV spectrum of PLTX showed two absorption peaks contributed by two chromophores at 233 and 264 nm, being the intensity at 233 nm double than 264 nm. This characteristic UV absorption profile was observed in 42-OH-PLTX (Figure 6).
Most of the morphological characters detailed in the present study for the INV MYZ0003 strain agree with the original description for P. borbonicum (Ten-Hage et al., 2000). Although, some differences were noted such as, e.g., the presence of an additional periflagellar platelet in the INV MYZ0003 strain compared to the eight reported in Ten-Hage et al. (2000), however, this characteristic fits the description of Hoppenrath et al. (2013) who consider that the number of platelets for this species is between eight or nine, as well as that of Zou et al. (2021) who count nine in a strain found in the South China Sea. The general notoriety of the depressions of the throughout thecal plates in the original description (Ten-Hage et al., 2000), which for INV MYZ0003 were more noticeable in those located towards the edges, and sometimes in small central areas of the thecal plates (Figures 2D, E), probably due to thin layers of mucilage and/or membranes that remained in our material. This also made difficult to clearly appreciate the thecal pores, because although various sizes could be distinguished (Figure 3A) it was not possible to appreciate the large pores located outside the depressions and pores of smaller sizes mentioned by Ten-Hage et al. (2000).
On the other hand, although the length and width sizes in some of the cells described in the present work exceeded the largest values reported in the original description, in general there was a good overlap between the size ranges of both strains (Table 2).
Table 2 Comparison of morphometric (min–max [mean ± SD]) and morphological features between the strain INV MYZ0003 and the original description for P. borbonicum (Ten-Hage et al., 2000).
P. borbonicum stands out for being part of the small-sized epibenthic species of the genus Prorocentrum (L <28 µm) together with P. elegans Faust, P. formosum Faust, P. norrisianum, Faust & Morton and P. sipadanense Mohammad-Noor, Daugbjerg & Moestrup, differing P. borbonicum from the first three for presenting a foveate thecal surface (Ten-Hage et al., 2000) being smooth in P. elegans (Faust, 1993a), P. formosum (Faust, 1993b) and P. norrisianum (Faust, 1997).
Although P. sipadanense also presents a foveate thecal surface, it is easily differentiable from P. borbonicum by characteristics such as: asymmetrical oblique truncated apex in P. sipadanense (Saburova and Chomérat, 2016) being symmetrical truncate in P. borbonicum; thecal pores scarce and asymmetrically scattered in P. sipadanense (Saburova and Chomérat, 2016) noticeable and visible by LM, while for P. borbonicum are more or less arranged symmetrically and only the largest pores being visible to the LM (Figure 2B); the presence of conspicuous marginal pores in P. sipadanense irregularly distributed (Mohammad-Noor et al., 2007; Saburova and Chomérat, 2016) not present in P. borbonicum; and presence of prominent marginal crest in P. sipadanense (Saburova and Chomérat, 2016), absent in P. borbonicum.
P. borbonicum was at first time isolated and described in a fringing coral reef of St Leu from La Reunion Island (France, southwest Indian Ocean) by Ten-Hage et al. (2000). Since then, it has been also reported in some locations around the world such as South China Sea (Zou et al., 2021); Mediterranean Sea (Aligizaki et al., 2009) and Florida (David, 2014). Until 2019, P. borbonicum had not been reported for the coasts of Latin America (Durán-Riveroll et al., 2019) however, since that year it was reported on the beaches of the province of Santa Elena in Ecuador (Catuto, 2019) and then on the coast of Paraná in Brazil (Junqueira de Azevedo, 2020). According to the results of this work, this would be the first report of the presence of this species in the Colombian Caribbean and for the Caribbean area.
It is noteworthy, the few places where this organism has been reported compared to the reports of other organisms of the same genus, perhaps because it is not usually found in high densities and/or because of its small size that would make it go unnoticed (Saburova and Chomérat, 2016).
During 2018, in the PNNT, small Prorocentrum species that highly resembles in appearance and size of epiphytic P. borbonicum were found on leaves of T. testudinum, a seagrass with a wide and permanent distribution in the area, and also in fiberglass mesh collectors placed for 24 hours in these meadows in the months of March (major dry season), June (minor rainy season) and October (major rainy season). During this last month the highest abundances were found, both in mesh and in T. testudinum; temperature values between 26,5 and 31.6° C and salinity between 32.6 and 36.8 were recorded.
Although T. testudinum like other phanerogams can serve as a substrate for a large number of organisms (González and Díaz, 2008), among them P. borbonicum, it is not ruled out that this species can be found in other types of substrates, if it is considered that the PNNT presents a high diversity, with 218 Rhodophyta, 52 Phaeophyceae and 83 Chlorophyta recorded species (Díaz-Pulido and Díaz-Ruíz, 2003). Aligizaki et al. (2009) also found P. borbonicum on sediment in Greek coastal 398 waters.
The TNNP is a hotspot of coral reef biodiversity, with some patches of phanerogams and mangroves also present, located between the city of Santa Marta (>455,000 inhabitants) and several smaller river mouths (Bayraktarov et al., 2014). The general geomorphological characteristics are influenced by the northwestern foothills of the Sierra Nevada de Santa Marta which enter towards the sea. These configure an indented coastline in which rocky headlands and inlets alternate with beaches, between which Bahía Concha (Bonito Gordo) is located. The region experiences a strong seasonal variation in physical parameters (temperature, salinity, wind, and water currents) due to alternating dry seasons with coastal upwelling and rainy seasons. For example, temperature (decreasing in average from 28 to 21°C) (Salzwedel and Müller, 1983; Bayraktarov et al., 2014), salinity (increasing from 33 to 38) (Salzwedel and Müller, 1983; Bayraktarov et al., 2014) and higher wind in dry seasons (Salzwedel and Müller, 1983).
In cultures, P. borbonicum cells generally exhibited sedentary behavior, primarily located at the bottom of the containers, with some cells actively swimming in the water column. There were strong attachments towards the containers usually forming aggregations which increased as the cultures became old.
Phylogenetically, P. borbonicum represents a well-defined clade by means of LSU rDNA sequences, given the large genetic distances regarding closest related species (P. panamense, P. sipadanense and P. playfairi). Therefore, the use of additional molecular markers were not necessary to depict its position. This is not the case always for other Prorocentrum spp. which given their genetic similarity need at least additional molecular markers (ITS rDNA, ITS2 secondary structure) to outline their differences between close ribotypes (e.g. Nascimento et al., 2017).
Morphologically, P. borbonicum shares with P. playfairi and P. panamense the presence of a foveate-reticulated surface of its thecal plates, differing mainly from these in the sizes that are much larger in the latter (length> 42.5 and width> 30). Other differences were the absence of pyrenoid in P. playfairi (Croome and Tyler, 1987); the asymmetric (heart-shaped) in the thecal plates of P. panamense cells (Grzebyk et al., 1998). On the other hand, P. playfairi has as a particular ecophysiological characteristic that of inhabiting fresh waters (Croome and Tyler, 1987).
Carrying out bioassays in mice with crude extracts of P. borbonicum, Ten-Hage et al. (2000); Ten-Hage et al. (2002) found lethality, previously presenting symptoms associated with neurotoxicity such as prostration, progressive limp paralysis (from hind- to fore-limbs), dyspnea, and terminal convulsions, without detecting diarrheal or paralytic toxins using HPLC analyze. These symptoms could be probably due to any PLTX analog since they are characteristic in mice after intraperitoneal injection with samples containing PLTX like compounds (Riobó et al., 2011); Similarly, P. borbonicum isolated from Greek coastal waters was also lethal to the brine shrimp Artemia without detecting the presence of okadaic acid (Aligizaki et al., 2009). According to Ten-Hage et al. (2002), electrophysiological experiments suggested the presence of palitoxy-like compounds; it was also suggested that P. borbonicum possessed polar fast acting toxins. The same authors, after HPLC-MS and MS/MS analyses revealed the presence of two new isomeric toxins named as borbotoxin-A and borbotoxin-B.
The mechanism of action for borbotoxin-A was found to be the blocking of the post-synapse of the nicotinic-type acetylcholine receptors. These receptors are involved in several central functions, including: voluntary control of movement, memory and attention, sleep and alertness, pain and anxiety (Le Novère et al., 2002). In this study, presence of borbotoxin was confirmed, but the most relevant result was the identification and quantitation of the PLTX analogue 42-hidroxy-palytoxin (42-OH-PLTX) responsible for haemolytic activity in P. borbonicum INV MYZ003.
Palytoxin (PLTX) and its analog 42-OH-PLTX are among the most potent non-protein marine biotoxins in existence (Ciminiello et al., 2009). These compounds were first isolated in 1971 and 2009, respectively, from zoanthid of the genus Palythoa in Hawaii (Moore and Scheuer, 1971; Ciminiello et al., 2009), with 42-OH-PLTX being the main palytoxin analog present in Palythoa toxica samples and a main component of the toxic extract from the P. tuberculosa sample, with biological effects similar to that of PLTX itself (Ciminiello et al., 2009). Kerbrat et al. (2011) also report the presence of these toxins in the cyanobacterium Trichodesmium erythraeum in New Caledonia (Oceania). In bioassays by intraperitoneal injection with extracts of this cyanobacterium in mice Kerbrat et al. (2011) observed symptoms such as reduced activity and responsiveness, frequent convulsive spasms, respiratory distress, and partial paralysis, which quickly progressed to total paralysis, which could lead to their death. On the other hand, Mori et al. (2016), discovered a compound with identical activity to PLTX in Chondria armata, when studying the vermifuge and insecticide properties in this red alga.
Among the most representative cases due to their negative impacts at the socio-economic, ecological and human health levels, the production of PLTX and several of its analogs by different species of dinoflagellates of the genus Ostreopsis stands out, such as: ostreocins in Ostreopsis siamensis (Usami et al., 1995; Terajima et al., 2019); mascarenotoxins in Ostreopsis mascarenensis (Lenoir et al., 2004) and ovatoxins in Ostreopsis ovata (Ciminiello et al., 2008; Garcia-Altares et al., 2015). In accordance with the above, this study reports for the first time the presence of a palytoxin analogue (42-OH-PLTX), in a dinoflagellate of a genus other than Ostreopsis, such as Prorocentrum, represented by P. borbonicum, confirming the suspicions of Ten-Hage et al. (2002) on the presence of palytoxin analogs in this organism.
Although palytoxin and several of its congeners have also been detected in various marine organisms such as fish (Fukui et al., 1987), molluscs, crabs, starfish, corals, sponges, polychaetes, gorgonians (Aligizaki et al., 2011; Biré et al., 2013), its presence is generally attributed to its transit and bioaccumulation through the trophic network, also associated by its close coexistence observed with zoanthids that produce these toxins, without clearly establishing its original producer.
The presence of 42-OH-PLTX and borbotoxins in P. borbonicum implies it as another of the producers and potential dispersers of these biotoxins, not only in Colombia where it is reported for the first time, but possibly in other areas of the world where it has been found. The presence of P. borbonicum in the Caribbean should be considered a potential problem for human health and well-being, even more so when the economy of the Caribbean region is based on tourism, an industry that has registered income of 25 billion US dollars per year, which represents 20% of the Gross Domestic Product (Burke and Maidens, 2005; Kingsbury, 2005; Pantojas, 2006). The finding of this article should serve to strengthen the Benthic Harmful Algae Blooms (BHABs) monitoring and design and implement an early warning system.
The datasets presented in this study can be found in online repositories. The names of the repository/repositories and accession number(s) can be found in the article/Supplementary Material.
EA-S led the investigation with the isolation, culture and morphological analysis of P. borbonicum, including writing of the manuscript. PR undertook toxin analyses and wrote the corresponding section. FR performed the phylogenetic analysis and writing of the manuscript. JM-P participated in the sampling design of the doctoral thesis of the first author (EA-S). JF-A takes part in the cultivation and processing of the organisms for SEM, as well as analysis, writing and final revision of the article. All authors provided input into the drafting and review of the manuscript prior to submission.
This work was funded by the following entities: the Ministry of Science, Technology and Innovation of Colombia - MINCIENCIAS (COLCIENCIAS), the Marine and Coastal Research Institute “Jose Benito Vives de Andreis” (INVEMAR, Contribution number: 1340) and Environmental and Sustainable Development Ministry (MINAMBIENTE) through BPIN project, the International Atomic Energy Agency (IAEA) through the Research Contract RC#18827 (“Bentox Project”) and the Technical Cooperation projects RLA/7/014 and RLA/7/020, the Spanish national project CCVIEO (CNIEO-CSIC), with additional funds of the Axencia Galega de Innovación (agreement GAIN-IEO), la Universidad Nacional de Colombia-UNAL Sede Caribe (within the Project “Influencia de recursos y reguladores en la abundancia de dinoflagelados bentónicos del Caribe”, Hermes Code: 40410). EA-S was supported by a COLCIENCIAS grant (call No. 727–2015).
We would like to acknowledge VGOHAB staff (CNIEO-CSIC, Vigo), involved in culturing facilities, namely Patricia Loures, Pilar Rial, Isabel Ramilo and Nuria Lluch. We are also indebted to Carlos Carballeira and Jose Luis Garrido from IIM-CSIC for HPLC-DAD analyses. Finally, the authors also appreciate the technical assistance by CACTI (University of Vigo) for sequencing (Sebastián Comesaña and Verónica Outeiriño).
The authors declare that the research was conducted in the absence of any commercial or financial relationships that could be construed as a potential conflict of interest.
All claims expressed in this article are solely those of the authors and do not necessarily represent those of their affiliated organizations, or those of the publisher, the editors and the reviewers. Any product that may be evaluated in this article, or claim that may be made by its manufacturer, is not guaranteed or endorsed by the publisher.
The Supplementary Material for this article can be found online at: https://www.frontiersin.org/articles/10.3389/fmars.2022.973250/full#supplementary-material
Aligizaki K., Katikou P., Milandri A. (2011). Occurrence of palytoxin-group toxins in seafood and future strategies to complement the present state of the art. Toxicon 57, 390–399. doi: 10.1016/j.toxicon.2010.11.014
Aligizaki K., Nikolaidis G., Katikou P., Baxevanis A. D., Abatzopoulos T. J. (2009). Potentially toxic epiphytic Prorocentrum (Dinophyceae) species in Greek coastal waters. Harmful. Algae. 8, 299–311. doi: 10.1016/j.hal.2008.07.002
Amar M., Aráoz R., Iorga B. I., Yasumoto T., Servent D., Molgó J. (2018). Prorocentrolide-a from cultured Prorocentrum lima dinoflagellates collected in Japan blocks sub-types of nicotinic acetylcholine receptors. Toxins 10, 97. doi: 10.3390/toxins10030097
Bayraktarov E., Pizarro V., Wild C. (2014). Spatial and temporal variability of water quality in the coral reefs of tayrona national natural park, Colombian Caribbean. Environ. Monit. Assess. 186, 3641–3659. doi: 10.1007/s10661-014-3647-3
Biré R., Trotereau S., Lemée R., Delpont C., Chabot B., Aumond Y., et al. (2013). Occurrence of palytoxins in marine organisms from different trophic levels of the French Mediterranean coast harvested in 2009. Harmful. Algae. 28, 10–22. doi: 10.1016/j.hal.2013.04.007
Catuto R. E. (2019). “Composición y abundancia de dinoflagelados epibentónicos asociados a las macroalgas en dos playas de la provincia de Santa Elena,” in Trabajo de pregrado de tesis (Santa Elena, Ecuador: Universidad Estatal Provincia de Santa Helena).
Ciminiello P., Dell’Aversano C., Dello Iacovo E., Fattorusso E., Forino M., Grauso L., et al. (2009). Stereostructure and biological activity of 42-hydroxy palytoxin: a new palytoxin analog from Hawaiian palythoa spp. Chem. Res. Toxicol. 22, 1851–1859. doi: 10.1021/tx900259v
Ciminiello P., Dell’Aversano C., Dello Iacovo E., Forino M., Tartaglione L., Pelin M., et al. (2014). Stereoisomers of 42-hydroxy palytoxin from Hawaiian Palythoa toxica and p. tuberculosa: Stereostructure elucidation, detection, and biological activities. J. Nat. Prod. 77, 2, 351–357. doi: 10.1021/np4009514
Ciminiello P., Dell’Aversano C., Fattorusso E., Forino M., Tartaglione L., Grillo C., et al. (2008). LC-MS/MS and HRMS/MS data demonstrated the presence of putative palytoxin and the novel ovatoxin-a in plankton collected during the ligurian 2006 toxic outbreak. J. Am. Soc Mass. Spectrom. 19, 111–120. doi: 10.1021/ac060250j
Croome R. L., Tyler P. A. (1987). Prorocentrum playfairi and Prorocentrum foveolata, two new dinoflagellates from Australian freshwaters. Br. Phycological. J. 22 (1), 67–75. doi: 10.1080/00071618700650091
David H. (2014). Characterization of potentially toxic dinoflagellates of the genera coolia, ostreopsis and prorocentrum from the Atlantic Iberian peninsula. [dissertation] (País Vasco, Spain: Universidad del País Vasco).
Díaz-Pulido G., Díaz-Ruíz M. (2003). Diversity of benthic marine algae of the Colombian Atlantic. Biota Colombiana. 4(2), 203–246. doi: 10.21068/bc.v4i2.134
Durán-Riveroll L. M., Cenbella A. D., Okololodkov Y. B. (2019). A review on the biodiversity and biogeography of toxigenic benthic marine dinoflagellates of the coasts of Latin America. Front. Mar. Sci. 6. doi: 10.3389/fmars.2019.00148
EURLMB (2015) EU-Harmonised standard operating procedure for determination of lipophilic marine biotoxins in molluscs by LC–MS/MS. EU reference laboratory for monitoring of marine biotoxins. Available at: https://www.aesan.gob.es/en/CRLMB/web/public_documents/seccion/crlmb_standard_operating_procedures.htm (Accessed May 10, 2022).
Faust M. A. (1993a). Prorocentrum belizeaum, Prorocentrum elegans, and Prorocentrum caribbaeum, three new benthic species (Dinophyceae) from a mangrove island, twin cays, Belize. J. Phycol. 29, 100–107. doi: 10.2216/i0031-8884-32-6-410.1
Faust M. A. (1993b). Three new benthic species of Prorocentrum (Dinophyceae) from twin cays, Belize: P. maculosum sp. nov., P. foraminosum sp. nov. and P. formosum sp. nov. Phycologia 32 (6), 410–418. doi: 10.2216/i0031-8884-32-6-410.1
Faust M. A. (1997). Three new benthic species of Prorocentrum (Dinophyceae) from Belize: P. norrisianum, sp. nov., P. tropicalis sp. nov., and P. reticulatum sp. nov. J. Phycol. 33, 851–858. doi: 10.1111/j.0022-3646.1997.00851.x
Foden J., Purdie D. A., Morris S., Nascimento S. M. (2005). Epiphytic abundance and toxicity of Prorocentrum lima populations in the fleet lagoon, UK. Harmful. Algae. 4, 1063–1074. doi: 10.1016/j.hal.2005.03.004
Fritz L., Trimer R. E. (1985). A rapid and simple technique utilizing calcofluor white MR2 for visualization of dinoflagellates technology. J. Phycol. 21, 664–664. doi: 10.1111/j.0022-3646.1985.00662.x
Fukui M., Murata M., Inoue A., Gawel M., Yasumoto T. (1987). Occurrence of palytoxin in the trigger fish Melichtys vidua. Toxicon 25, 1121–1124. doi: 10.1016/0041-0101(87)90269-8
Fukuyo Y. (1981). Taxonomical study on benthic dinoflagellates collected in corals reefs. Bull. Jap. Soc Sci. Fish. 47, 967–978. doi: 10.2331/suisan.47.967
García-Altares M., Tartaglione L., Dell’Aversano C., Carnicer O., de la Iglesia P., Forino M., et al. (2015). The novel ovatoxin-g and isobaric palytoxin (so far referred to as putative palytoxin) from Ostreopsis cf. ovata (NW Mediterranean sea): structural insights by LC-high resolution MS(n.). Anal. Bioanal. Chem. 407, 1191–1204. doi: 10.1007/s00216-014-8338-y
Gayoso A. M., Dover S., Morton S. L., Busman M., Moeller P., Fulco V. K., et al. (2002). Diarrhetic shellfish poisoning associated with Prorocentrum lima (Dinophyceae) in Patagonian gulfs (Argentina). J. Shellfish. Res. 21, 461–436. doi: 10.2983/035.034.0339
Glibert P. M., Burkholder J. M., Kana T. M. (2012). Recent insights about relationships between nutrient availability, forms, and stoichiometry, and the distribution, ecophysiology, and food web effects of pelagic and benthic Prorocentrum species. Harmful. Algae. 14, 231–259. doi: 10.1016/j.hal.2011.10.023
González L., Díaz J. (2008). Distribución de dinoflagelados epifitos potencialmente tóxicos asociados a praderas de Thalassia testudinum en la isla la tortuga, la bahía de mochima y el golfo de cariaco, Venezuela. Boletín del Instituto. Oceanográfico de Venezuela. 47, 47–58. Corpus ID: 128771941
Grzebyk D., Sako Y., Berland B. (1998). Phylogenetic analysis of nine species of Prorocentrum (Dinophyceae) inferred from 18S ribosomal DNA sequences, morphological comparisons, and description of Prorocentrum panamensis sp. nov. J. Phycology. 34(6), 1055–1068. doi: 10.1046/j.1529-8817.1998.341055.x.
Guiry M. D., Guiry G. M. (2021) AlgaeBase. world-wide electronic publication, national university of Ireland, Galway. Available at: http://www.algaebase.org (Accessed May 10, 2022).
Hall T. (1999). BioEdit: a user-friendly biological sequence alignment editor and analysis program for windows 95/98/NT. Nucleic Acids 41, 95–98. doi: 10.14601/Phytopathol_Mediterr-14998u1.29
Heredia-Tapia A., Arredondo-Vega B. O., Núñez-Vázques E. J., Yasumoto T., Yasuda M., Ochoa J. L. (2002). Isolation of Prorocentrum lima (Syn Exuviaella lima) and diarrhetic shellfish poisoning (DPS) risk assessment in the gulf of California, México. Toxicon 40, 1121–1127. doi: 10.1016/s0041-0101(02)00111-3
Hoppenrath M., Chomérat N., Horiguchi T., Schweikert M., Nagahama Y., Shauna S. (2013). Taxonomy and phylogeny of the benthic Prorocentrum species (Dinophyceae)-a proposal and review. Harmful. Algae. 27, 1–28. doi: 10.1016/j.hal.2013.03.006
Hu T., Defreitas A. S., Doyle J., Jackson D., Marr J., Nixon E., et al. (1993). “New DSP toxin derivatives isolated from toxic mussels and the dinoflagellates, prorocentrum lima and prorocentrum concavum,” in Toxic phytoplankton blooms in the sea. Eds. Smayda T. J., Shimizu Y. (Amsterdam: Elsevier Scientific Publishing), 507–512.
Huelsenbeck J. P., Ronquist F. (2001). MRBayes: Bayesian inference of phylogenetic trees. Bioinformatics 17, 754–755. doi: 10.1093/bioinformatics/17.8.754
Jackson A. E., Marr J. C., McLachlan J. L. (1993). “The production of diarrhetic shellfish toxin by an isolate of prorocentrum lima from Nova Scotia, canadá,” in Toxic phytoplankton blooms in the sea. Eds. Smayda T. J., Shimizu Y. (Amsterdam: Elsevier Scientific Publishing), 513–518.
Junqueira de Azevedo C. E. (2020). “Dinoflagelados bénticos em áreas de costões rochosos e recifes distribucão, toxinas e impactos potenciais,” in Tesis de doctorao (Estado de Paraná, Brasil: Universidade Federal do Paraná).
Keller M. D., Sevin R. C., Claus W., Guillard R. L. L. (1987). Media for the culture of oceanic ultraphytoplankton. J. Phycol. 23, 633–638. doi: 10.1111/j.1529-8817.1987.tb04217.x
Kerbrat A. S., Amzil Z., Pawlowiez R., Golubic S., Sibat M., Darius H. T., et al. (2011). First evidence of palytoxin and 42-hydroxy-palytoxin in the marine cyanobacterium Trichodesmium. Mar. Drugs. 9, 543–560. doi: 10.3390/md9040543
Kingsbury P. (2005). Jamaican Tourism and the politics of enjoyment. Geoforum 36, 113e132. doi: 10.1016/j.geoforum.2004.03.012.
Kobayashi J., Kubota T. (2010). “Bioactive metabolites from marine dinoflagellates,” in Comprehensive natural products II. Eds. H. -W. (Elsevier, Oxford: Mander, Liu and L.) 263–325. doi: 10.1016/B978-008045382-8.00040-X
Kumar S., Stecher G., Li M., Knyaz C., Tamura K. (2018). MEGA X: Molecular evolutionary genetics analysis across computing platforms. Mol. Biol. Evol. 35, 1547–1549. doi: 10.1093/molbev/msy096
Lenoir S., Ten-Hage L., Turquet J., Quod J. P., Bernard C., Hennion M. C. (2004). First evidence of palytoxin analogues from an Ostreopsis mascarenensis (Dinophyceae) benthic bloom in southwestern Indian ocean. J. Phycol. 40, 1042–1051. doi: 10.1111/j.1529-8817.2004.04016.x
Le Novère N. P., Corringer J., Changeux J. P. (2002). The diversity of subunit composition in nAChRs: evolutionary origins, physiologic and pharmacologic consequences. J. Neurobiol. 53, 447–456. doi: 10.1002/neu.10153
Levasseur M., Couture J. Y., Weise A. M., Michaud S., Elbrächter M., Sauvé G., et al. (2003). Pelagic and epiphytic summer distributions of Prorocentrum lima and P. mexicanum at two mussel farms in the gulf of st. Lawrence, Canada. Aquat. Microb. Ecol. 30, 283–293. doi: 10.3354/ame030283
Lundholm N., Churro C., Fraga S., Hoppenrath M., Iwataki M., Larsen J., et al. (2021) Prorocentrales, in IOC-UNESCO taxonomic reference list of harmful micro algae. Available at: http://www.marinespecies.org/hab (Accessed May 10, 2022).
Mohammad-Noor N., Daugbjerg N., Moestrup Ø., Anton A. (2007). Marine epibenthic dinoflagellates from Malaysia - a study of live cultures and preserved samples based on light and scanning electron microscopy. Nord. J. Bot. 24, 629–690. doi: 10.1111/j.1756-1051.2004.tb01938.x
Moore R. E., Scheuer P. J. (1971). Palytoxin: A new marine toxin from a coelenterate. Sci. (Washington. D.C.) 172(3982), 495–498. doi: 10.1126/science.172.3982.495.
Mori S., Sugahara K., Maedab M., Nomotoa K., Iwashitaa T., Yamagakia T. (2016). Insecticidal activity guided isolation of palytoxin from a red alga, Chondria armata. Tetrahedron Letters. 5732, 3612–3617. doi: 10.1016/j.tetlet.2016.06.108
Nakajima I., Oshima Y., Yasumoto T. (1981). Toxicity of benthic dinoflagellates in Okinawa. Bull. Japanese. Soc. Sci. Fisheries. 47 (8), 1029–1033. doi: 10.2331/suisan.47.1029.
Nascimento S. M., Mendes M. C. Q., Menezes M., Rodríguez F., Alves-de-Souza C., Branco S., et al. (2017). Morphology and phylogeny of Prorocentrum caipirignum sp. nov. (Dinophyceae), a new tropical toxic benthic dinoflagellate. Harmful Algae. 70, 73–89. doi: 10.1016/j.hal.2017.11.001
Pantojas G. E. (2006). De la plantación al resort: El caribe en la era de la globalización. Rev. Cienc. Soc 15, 82–99. Corpus ID: 149238380
Pearce I., Handlinger H. J., Hallegraeff G. H. (2005). Histopathology in pacific oyster (Crassostrea gigas) spat caused by the dinoflagellate Prorocentrum rhathymum. Harmful. Algae. 4, 61–74. doi: 10.1016/j.hal.2003.11.002
Reguera B., Alonso R., Moreira A., Méndez S. (2011). “Guía para el diseño y puesta en marcha de un plan de seguimiento de microalgas productoras de toxinas,” in IOC manual and guides 59, vol. pp. (París y Viena: IOC UNESCO and IAEA), 1–46.
Riobó P., Franco J. M. (2011). Palytoxins: Biological and chemical determination. Toxicon 57, 368–375. doi: 10.1016/j.toxicon.2010.09.012
Riobó P., Paz B., Franco J. M., Vázquez J., Murado M. A. (2008). Proposal for a simple and sensitive haemolytic assay for palytoxin. toxicological dynamics, kinetics, ouabain inhibition and thermal stability. Harmful Algae. 7, 415–429. doi: 10.1016/j.hal.2007.09.001.
Saburova M., Chomérat N. (2016). An emended description and phylogeny of the little-known Prorocentrum sipadanense mohammad-noor, daugbjerg & moestrup (Prorocentrales, dinophyceae) from the Indian ocean, Oman. Eur. J. Phycol. 51, 270–281. doi: 10.1080/09670262.2015.1134815
Salzwedel H., Müller K. (1983). A summary of meteorological and hydrological data from the bay of Santa Marta, Colombian Caribbean. Bull. Mar. Coast. Res. 13, 67–83. doi: 10.25268/bimc.invemar.1983.13.0.483
Scholin C. A., Herzog M., Sogin M., Anderson D. M. (1994). Identification of group and strain specific genetic markers for globally distributed Alexandrium (Dinophyceae). II. sequence analysis of a fragment of the LSU rRNA gene. J. Phycology. 30, 999–1011. doi: 10.1111/j.0022-3646.1994.00999.x
Ten-Hage L., Robillot C., Turquet J., Le Gall F., Le Caer J. P., Bultel V., et al. (2002). Effects of toxic extracts and purified borbotoxins from Prorocentrum borbonicum (Dinophyceae) on vertebrate neuromuscular junctions. Toxicon 40, 137–148. doi: 10.1016/S0041-0101(01)00200-8
Ten-Hage L., Turquet J., Quod J.-P., Piuseux-Dao S., Couté A. (2000). Prorocentrum borbonicum sp. nov. (Dinophyceae), a new toxic benthic dinoflagellate from southwestern Indian ocean. Phycología. 394, 296–301. doi: 10.2216/i0031-8884-39-4-296.1
Terajima T., Uchida H., Abe N., Yasumoto T. (2019). Structure elucidation of ostreocin-a and ostreocin-E1, novel palytoxin analogs produced by the dinoflagellate Ostreopsis siamensis, using LC/Q-TOF MS. Bioscience. Biotechnology. Biochem. 83:3, 381–390. doi: 10.1080/09168451.2018.1550356
Usami M., Satake M., Ishida S., Inoue A., Kan Y., Yasumoto T. (1995). Palytoxin analogs from the dinoflagellate Ostreopsis siamensis. J. Am. Chem. Soc 117, 5389–5390. doi: 10.1021/ja00124a034
Yasumoto T., Seino N., Murakami Y., Murata M. (1987). Toxins produced by benthic dinoflagellates. Biol. Bull. 172, 128–131. doi: 10.2307/1541612
Keywords: Prorocentrum, palytoxin, Tayrona National Natural Park, Colombian Caribbean, HPLC-HRMS
Citation: Arteaga-Sogamoso E, Riobó P, Rodríguez F, Mancera-Pineda JE and Franco-Angulo J (2022) First record of the dinoflagellate Prorocentrum borbonicum in the continental coast of Colombian Caribbean: A new 42 hydroxi-palytoxin producer. Front. Mar. Sci. 9:973250. doi: 10.3389/fmars.2022.973250
Received: 19 June 2022; Accepted: 27 July 2022;
Published: 30 August 2022.
Edited by:
Marius Nils Müller, Federal University of Pernambuco, BrazilReviewed by:
Haifeng Gu, State Oceanic Administration, ChinaCopyright © 2022 Arteaga-Sogamoso, Riobó, Rodríguez, Mancera-Pineda and Franco-Angulo. This is an open-access article distributed under the terms of the Creative Commons Attribution License (CC BY). The use, distribution or reproduction in other forums is permitted, provided the original author(s) and the copyright owner(s) are credited and that the original publication in this journal is cited, in accordance with accepted academic practice. No use, distribution or reproduction is permitted which does not comply with these terms.
*Correspondence: Edgar Arteaga-Sogamoso, ZWFydGVhZ2FzQHVuYWwuZWR1LmNv
Disclaimer: All claims expressed in this article are solely those of the authors and do not necessarily represent those of their affiliated organizations, or those of the publisher, the editors and the reviewers. Any product that may be evaluated in this article or claim that may be made by its manufacturer is not guaranteed or endorsed by the publisher.
Research integrity at Frontiers
Learn more about the work of our research integrity team to safeguard the quality of each article we publish.