- 1AZTI, Marine Research, Basque Research and Technology Alliance (BRTA), Pasaia, Spain
- 2Faculty of Marine Sciences, King Abdulaziz University, Jeddah, Saudi Arabia
- 3Joint Nature Conservation Committee (JNCC), Peterborough, United Kingdom
- 4Ifremer, ZI de la Pointe du Diable - CS 10070, Plouzané, France
- 5Department of Marine Ecological Environment, National Marine Data and Information Service, Tianjin, China
The EMOD-PACE project, funded by the European Commission, aimed to promote international ocean governance between EU and China. One of the objectives of EMOD-PACE is to compare European and Chinese modelling approaches for ecosystem vulnerability assessment. In particular, our objective was to test the applicability of the Chinese evaluation approach of resource-environment carrying capacity (MRECC) and spatial development suitability (abbreviated as “double evaluation”) to a European sea (the Bay of Biscay), in the context of marine spatial planning. The methodology involves three different steps: (i) an evaluation of areas of ecological importance, which includes species and habitats (i.e., biodiversity protection) and coastal characteristics; (ii) assessment of current marine development and utilization; and (iii) an ecological risk identification and the evaluation of the MRECC, by intersecting results from (i) and (ii). After collating information for 31 species of interest (fish, reptiles, mammals and birds), seven habitats (seagrass, seaweeds, saltmarshes, fish spawning areas, tidal flats, estuaries and unique habitats), marine protected areas and eight current human activities performed at sea (aquaculture, ports, ocean energy facilities, shipping, aggregate extraction and dredging, fisheries, military areas and tourism and recreation), they were aggregated and intersected (ecological data vs. human activities), and the ecological risk was determined. Since the total area covered by Marine Protected Areas and areas of high ecological importance is 135,372 km2, the available carrying capacity for development of marine activities within the Bay of Biscay is 229,266 km2. When we apply weighting to the calculation of the ecological importance and human activities, the high importance areas increase and the available carrying capacity decreases by 0.2%, to 228,637 km2. In this work we demonstrate that the Chinese double evaluation approach can be adapted and applied to a European sea, but to obtain more accurate results, and more extensive application to different areas are needed. Also, we have identified essential improvements, including better information for a number of species and habitats; more robust methods to identify biodiversity priorities; additional fish life-story traits; include future human activities; risks posed by multiple activities; and use appropriate weights through a stakeholder consultation.
Introduction
Historically, activities at sea have been diverse, including fishing, shipping, or leisure, among many others (Kleingärtner, 2018). Nowadays, both historical and new human activities at sea (the so-called ‘blue economy’; EUMOFA, 2020; UNESCO-IOC/European Commission, 2021; European Commission et al., 2022) are rapidly increasing, resulting in cumulative pressures on marine ecosystems (Borja et al., 2020). After different assessments (Halpern et al., 2015; Jouffray et al., 2020; United Nations, 2021a; United Nations, 2021b), those human pressures are impacting coastal ecosystems (e.g. seagrasses, mangroves and coral reefs), land- and sea-based activities are introducing pollutants to the sea (e.g. nutrients, metals, pesticides, pharmaceuticals, litter, microplastics or noise), overfishing has a pervasive effect on the trophic web, and on top of those, the gases released into the atmosphere are driving warming, acidification and sea-level rise, which are threatening biodiversity, ecosystem services delivery and even human health (Pörtner et al., 2021). Hence, although the ocean is the life-support system of our planet, it seems to be near its carrying capacity (United Nations, 2021b; United Nations, 2021a), which should be understood as the ability of the ocean to support a given amount of activity without damage for the environment.
To fight against these problems, different management responses have been implemented at global, supranational, or national scales. Hence, the United Nations Sustainable Development Goals (United Nations, 2016), try to find an equilibrium between growth and sustainability of human activities. In the case of marine systems, these can be achieved through marine spatial planning (MSP) of human activities, under an ecosystem-based management approach, in which the activities are undertaken in a sustainable way (Ehler and Douvere, 2009). In this context, many countries around the world have implemented MSP (Frazão Santos et al., 2019; Chalastani et al., 2021), based on specific legislation. For example, in the European Union (EU), the Maritime Spatial Planning Directive (MSPD; European Union, 2014) drives the future development of activities at sea, while at the same time good environmental status must be achieved in all regional seas, under the Marine Strategy Framework Directive (MSFD; European Commission, 2008). Other countries, such as China, have followed other spatial planning approaches, such as the Major Marine Functional Zoning Plan and Marine Functional Zoning (Tang et al., 2020), or the “multi-plan integration”, in which various types of spatial plans are integrated into one framework (Feng et al., 2016). The evaluation of resource-environment carrying capacity (MRECC) and spatial development suitability (abbreviated as “double evaluation”) is proposed along with these approaches, providing a scientific basis for spatial planning (Yue et al., 2020). By considering natural resources, environment and ecology, double evaluation provides an insight into ecological conservation and economic development trade-off (i.e., activities sustainability), as well as maximum carrying capacity estimation for each economic activity (Yue et al., 2020).
The relationships between economic growth and environmental status, as well as the link between economic activities and the carrying capacity and resilience of the environment, have been extensively discussed for decades (Arrow et al., 1995). The methodologies to determine the carrying capacity have been applied to several human activities at sea, including aquaculture (Duarte et al., 2003; Cai and Sun, 2007; Byron et al., 2011; Filgueira et al., 2015), beach uses (Epelde et al., 2021), tourism (Pearce and Kirk, 1986; Hughes and Furley, 1996; Han, 2018; Sha, 2020), harbors (Li et al., 2018a), land-uses (Li et al., 2018b), recreation (Shokri and Mohammadi, 2021), and multiple other coastal activities (Di et al., 2007; Fuju et al., 2011; Liu et al., 2020).
Historically, areas of cooperation between Europe and China have been diverse, ranging from science and technology, economy, and space occupancy, to environment and ecosystem health and protection. In recent years, the cooperation between Europe and China on ocean issues has increased considerably. Following high-level conferences and forums during the EU-China Blue Year 2017, the EU and China signed a Blue Partnership for the Ocean on 16th June 2018, which marked the beginning of a new phase of strategic EU-China ocean relations. The shared objective of this partnership is to ensure effective ocean governance for the conservation and sustainable use of the oceans and their resources. In this context, the EMOD-PACE project1 (“International Ocean Governance: Strengthening international ocean data through the EU’s ocean diplomacy with China”) was financed by the European Commission, with the aim of promoting international ocean governance between EU and China, and support the implementation of global commitments, by making marine data and data products more easily accessible and by providing better data and data products. One of the objectives of EMOD-PACE is to compare European and Chinese modelling approaches for ecosystem vulnerability assessment. Hence, applying the Chinese double evaluation approach to a European sea and looking for potential comparison with the MSP approach in Europe, is the main objective of this research.
Methodology
Case study: The Bay of Biscay
As case study, the Bay of Biscay was selected, due to the abundance of data available, as well as the availability of previous transboundary management information on the application of the MSFD (Cavallo et al., 2018) and the MSPD (Pinarbaşı et al., 2020). The Bay of Biscay is in the north-East Atlantic Ocean, between North-West France (Brittany) and North-West Spain (Galicia) (Figure 1). The boundaries of the Bay of Biscay are Cape Finisterre, at 43°N, in Galicia (NW Spain), and 48°N, in Brittany (NW France) (Lavín et al., 2006). In total, the Bay of Biscay occupies around 175,000 km2 (Borja et al., 2019a).
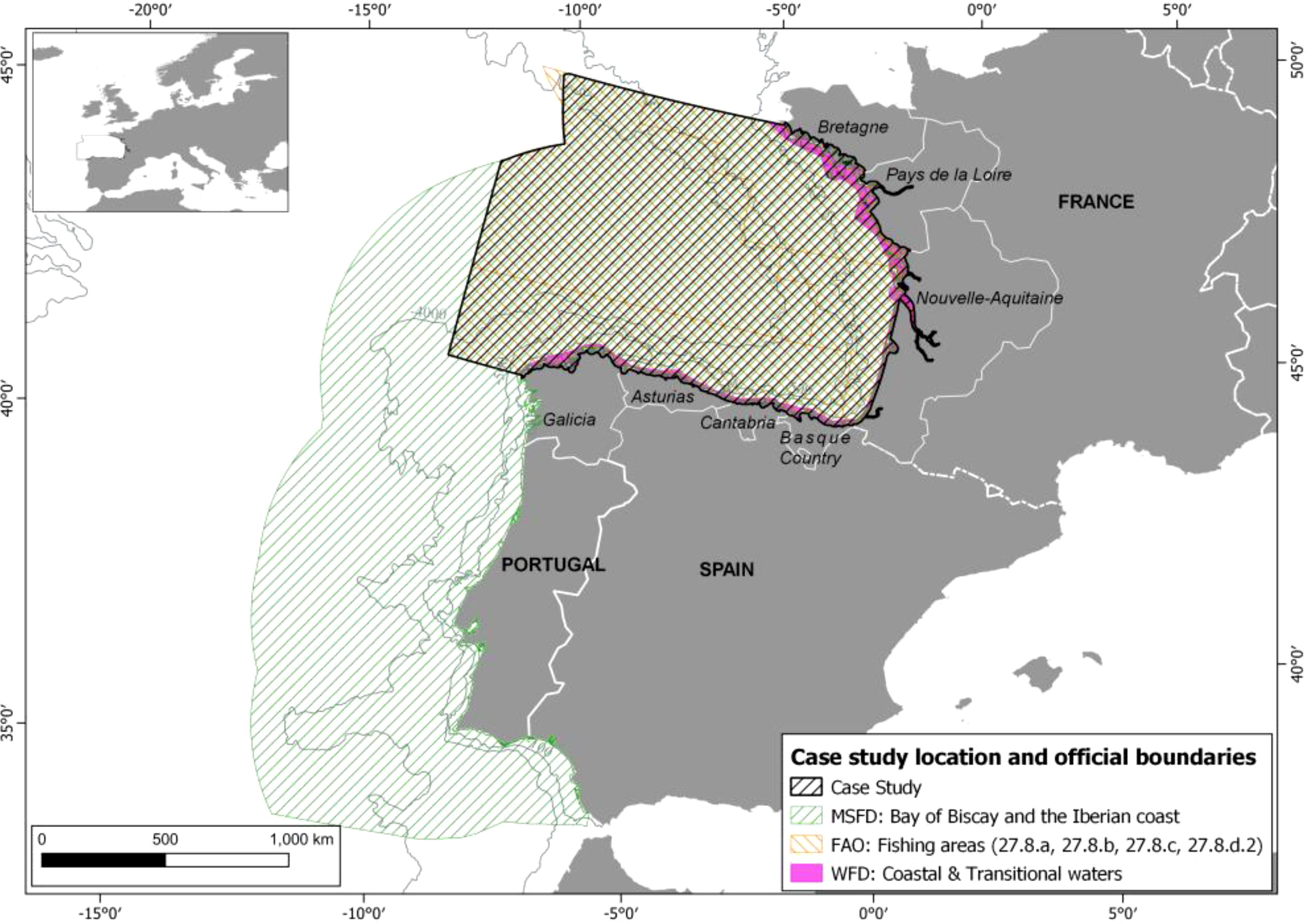
Figure 1 Location and geographic extension of the case study (Bay of Biscay), in the European context and other boundaries: Marine Strategy Framework Directive (MSFD), Food and Agriculture Organization (FAO) fishing areas, and Water Framework Directive (WFD).
The Bay of Biscay is a well-differentiated geomorphological unit prone to a long fetch and high oceanographic dynamics, in terms of winds, currents and waves (Borja et al., 2019a). The abyssal basin represents 50% of the total surface, with a mean depth of 4,800 m (Lavín et al., 2006). The continental shelf in the southern part of the bay is narrow (12-30 km), and it widens towards the French coast reaching a width of over 150 km in the northernmost part. The continental slope is very pronounced (slope of 10%–12%). This slope is cut by numerous canyons, which have generally narrow, steep-sided, linear, and sinuous channels, the most conspicuous being the Capbreton Canyon, where the 1,000 m isobath is found only 3 km from the coast (Lavín et al., 2006; Galparsoro et al., 2020).
To establish the geographical boundaries of the case study, different sources of information have been considered (Figure 1):
● Offshore boundaries in the south-west of the study area: the area corresponding to Food and Agriculture Organization (FAO) fishing areas 27.8.c and 27.8.d.22 and located inside the MSFD subregion “Bay of Biscay and Iberian Coast”3, coincident with the International Council for the Exploration of the Sea (ICES) ecoregion (“Bay of Biscay and the Iberian Coast”)4.
● Offshore boundaries in the north of the study area: FAO fishing areas 27.8.a and 27.8.d.2.
● Terrestrial boundaries in the south and east of the study area: MSFD subregion “Bay of Biscay and Iberian Coast” and coastal and transitional waters according to the Water Framework Directive (WFD) (European Commission, 2000)5. These bounding lines have been adjusted using both sources to achieve maximum coverage.
Additionally, a buffer was established along the coast to cover the marine-terrestrial interface, more precisely: five kilometers buffer around the coast and one kilometer buffer around the transitional water bodies (Figure 1).
In total, the Bay of Biscay study area covers 369,762 km2, much larger than the extent of the bay abovementioned, since it has been extended offshore, to accommodate FAO and ICES regions, and inshore, to include the transitional areas and the coastal buffer zone. Only 3% of the study area is terrestrial, and corresponds to the buffer area, while 97% is marine or coastal (including transitional waters).
To analyze the information in a standardized way, the study area polygon has been intersected with the European Environmental Agency (EEA) reference grid (1x1 km)6. All the cells that are, either totally or partially, inside the study area, were selected. It is important to note that an area in the north-west corner of the study area polygon was excluded from analysis as it is not covered by the EEA grid, because is out of the Exclusive Economic Zone (Supplementary Material -SM-, Figure SM1). A total of 364,638 cells have been selected: 349,703 are marine (including estuaries, lagoons, coastal and offshore waters), 6,865 terrestrial and the remaining 8,070 are partially terrestrial and marine.
Adapting the double evaluation methodology to Europe
Research has been carried out for many years related to carrying capacity in China and applied to several areas, such as in Liaoning Province (Di et al., 2007), Xiamen Bay (Liao et al., 2013), Dongtou Islands (Ma et al., 2017), the offshore Yangtse River area (Song and Du, 2019), or Fujian Province (Zhao et al., 2021), among others. Recently, in 2020, an official methodology of double evaluation was developed and was applied for MSP in coastal and marine areas. Technical text provided by the National Marine Data and Information Service (NMDIS), and translated from Chinese to English, is available as Supplementary Material (SM1). There, the MRECC refers to “the maximum and feasible volume of marine human activities which can be supported by marine resources and environment in a given sea area, which is associated with (based on) levels of development, economy and technology, production and lifestyle and goals for ecological protection.”
The terminology and the elements used in the Chinese version were adapted to the European context, in terms of the MSFD and the MSPD, resulting in the document included in Supplementary Material (SM2), which describes the approach that has been used in the application to the Bay of Biscay. The methodology involves three steps: (i) evaluation of areas of ecological importance; (ii) assessment of current marine development and utilization; and (iii) aggregation of the results (Figure 2), to identify the ecological risk and evaluate the MRECC, by intersecting results from (i) and (ii) as described below.
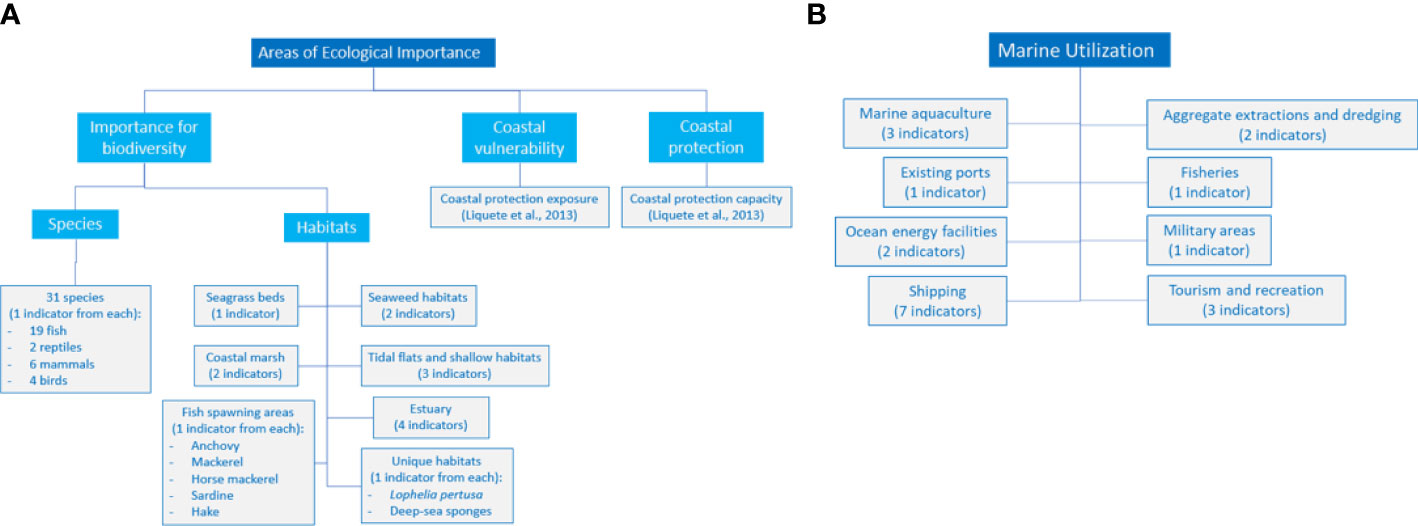
Figure 2 Aggregation scheme of the information layers to estimate (A) the areas of ecological importance and (B) the current marine development and utilization.
Evaluation of areas of ecological importance
The first step of the double evaluation methodology requires the evaluation of the areas of ecological importance, which includes: (i) identifying areas of importance for biodiversity; (ii) assessing the coastal protection importance; and (iii) assessing the coastal vulnerability based on coastal erosion and sand loss.
Following their assessment, the three components (i.e., importance for biodiversity, coastal vulnerability, coastal protection) are integrated to identify the relevant ecological functional areas.
Importance for biodiversity
The method identifies the areas of importance for marine biodiversity at three different levels: species, habitats, and genes (Table 1).
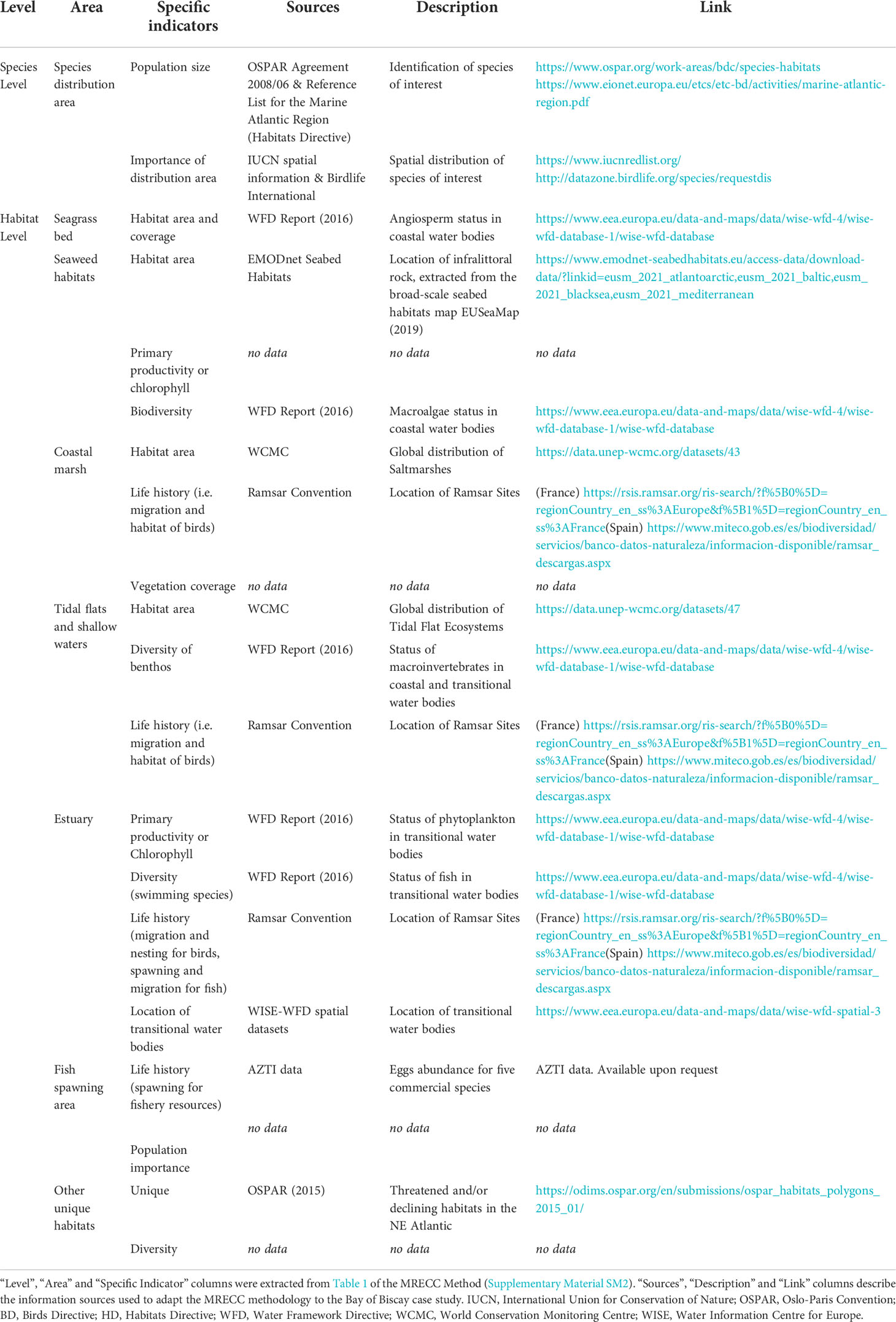
Table 1 Evaluation of areas of ecological importance at species and habitats levels, as described in the Marine Resource-Environment Carrying Capacity (MRECC) methodology.
At the species level, the approach requires the identification of the “species distribution areas” by means of two indicators: population size and importance of distribution area. The adaptation of the methodology to the European context and the data availability assessment were based on two sources of information: (i) a list of species of interest, and (ii) their spatial distribution within the Bay of Biscay.
To create the list of species of interest, the ‘OSPAR List of Threatened and/or Declining species’7 within OSPAR Region IV (OSPAR Agreement 2008-06)8, and the ‘Reference List for the Marine Atlantic Region’ of the Habitats Directive9 were used. Data on the spatial distribution of those species were obtained from the International Union for Conservation of Nature (IUCN) Red Lists10, and the bird species distribution data from Birdlife International11. Spatial data were obtained for 31 (19 fish, 2 reptiles, 6 mammals, 4 birds) out of the 33 species of interest (Table SM1).
The IUCN global species distribution maps are limited in their spatial resolution and a precautionary approach was necessary to classify grid cells into mid or high importance areas (Table SM2).
At the habitat level, from the eight habitat categories included in the method (i.e. seagrass bed, seaweed habitat, coastal marsh, tidal flats and shallow waters, estuary, island, fish spawning area, other unique habitats), a total of seven were included in the case study analysis. The “island” category was removed due to its low representativity. The MEP method requires that, independently of the number of specific indicators for each habitat category, all of them need to be aggregated into a single value in each cell, as explained below.
For seagrass beds, the method includes a single specific indicator, “Habitat area and coverage” (Table 1). Although a seagrass layer is available from the EMODnet Seabed Habitats data portal12, the information is not complete for the Bay of Biscay. Therefore, it was decided to use the angiosperm ecological status in coastal waters from the WFD 2016 Report13. The WFD classifies the status into five categories: high, good, moderate, poor, and bad. When the status cannot be assessed, the database contains information on unknown, unpopulated or no data. This information was transformed into three categories: high, when ecological status was high or good; mid, when angiosperm status was moderate; and low, when angiosperm status was any of the other categories, including those for which no data exist. This way, each cell in the case study grid was classified in one of those three categories for the seagrass beds habitat type (Table SM2).
The method includes three specific indicators for seaweed habitats: “habitat area”, “primary productivity or chlorophyll” and “biodiversity” (Table 1). For the first indicator, a macroalgal canopy extent data layer is available in EMODnet Seabed Habitats data portal14. However, as it is incomplete in the Bay of Biscay, the proxy “infralittoral rocks” was used (extracted from ‘EUSeaMap’ EMODnet broad-scale seabed habitat map 201915), since this habitat in the study area is mostly covered by macroalgae. The second indicator was discarded due to the lack of information. For the third indicator, the ecological status of macroalgae in coastal waters was used, as reported in the WFD Report 201616, using the same approach as for seagrasses. The two specific indicators for this habitat have been integrated into a single value per grid cell (Table SM2).
Coastal marshes assessment is based on three specific indicators (Table 1): “habitat area”, “life history” and “vegetation coverage”. Two sources of information have been used: (i) the global distribution of saltmarshes, from UNEP-WCMC17 Ocean Viewer portal; and (ii) the areas included as Ramsar sites (according to the Ramsar Convention or Convention on Wetlands)1819. The two specific indicators were aggregated into a single value per grid cell (Table SM2).
Tidal flats and shallow waters are analyzed according to three specific indicators: “habitat area”, “diversity of benthos” and “life history”. The first indicator has been adapted using the data layer “Global distribution of tidal flats”, from UNEP-WCMC20. For the second, the ecological status of macroinvertebrates reported under the WFD in 2016, both in coastal and transitional waters21. Finally, for “life history”, the designated Ramsar sites have been used16 (i.e. ‘Yes’ for cells with designated sites, and ‘No’ without sites). The three indicators were aggregated into a single value, as shown in Table SM2.
Estuarine habitats are characterized using three specific indicators: “primary productivity or chlorophyll”, “diversity of swimming species”, and “life history” (Table 1). The method has been adapted by aggregating four sources of information. First, for the location of these habitats, the “transitional water bodies”, as included in the WISE WFD reference spatial data sets22, have been used. As proxies of the specific indicators “primary productivity or chlorophyll” and “diversity of swimming species”, the ecological status of phytoplankton and fish in transitional waters, reported under the WFD, have been used, respectively23. Finally, for the “life history” indicator, the location of designated Ramsar sites has been used16. The aggregation of the information was done as described in Table SM2. Within the same grid cell, the ecological status of phytoplankton and fish could be different. In those cases, for aggregating the indicators, a precautionary approach was adopted, taking the highest of the two values as the value for the cell.
Information of five commercial fish species has been used to assess the fish spawning areas. More precisely, egg abundance data for anchovy (Engraulis encrasicolus), sardine (Sardina pilchardus), hake (Merluccius merluccius), Atlantic horse mackerel (Trachurus trachurus) and Atlantic mackerel (Scomber scombrus) were gathered. These species were selected because four of them are within the five most caught in the Bay of Biscay (>40% of the total landings in 2016 and 201724), and at the same time, they are within the 10 species of highest landing value (30% of the total in 2016 and 201725). The historical data of egg distribution and abundance (egg km-2), for the five species, was used to spatially delimit the spawning grounds. The data available per species are: (i) for anchovy, annual data from 1989 to 2020 (32 campaigns); (ii) for sardine, annual data from 1998 to 2020 (23 campaigns); (iii) for hake, data every three years, from 1995 to 2016 (8 campaigns); and (iv) for Atlantic horse mackerel and Atlantic mackerel, data every three years, from 1992 to 2019 (10 campaigns).
To transform the original point data into a layer covering the whole case study area, a geostatistical interpolation was performed in QGIS version 3.16.11-Hannover (QGIS.org, 2022) using the Ordinary Kriging technique. The data were split by species and year, obtaining between 8 and 32 raster layers per species. For each raster file, the pixel values were then aggregated to fit the case study grid using the zonal statistics tool in QGIS. Using these layers, the mean value of egg abundance per grid cell was estimated for each species. This value was then used to discretise data, following the work done previously to detect areas of high-density of marine organisms (Cañadas and Vázquez, 2014; García-Baron et al., 2019a): the mean abundance of eggs was ordered from highest to lowest values. The cells with the highest abundance values and comprising a cumulative abundance of 30%, were considered as “presence” (i.e., high) abundance cells, while the rest of the cells were considered as “absence” (i.e., low) importance cells. The threshold of 30% was chosen after exploring the options of 40%, 30%, 20% of cumulative abundance of eggs, since it includes most of the important areas for spawning of the five species, without being too large for a feasible protection and cost-effective monitoring.
For the last category of habitats, the Other unique habitats, the “OSPAR threatened and/or declining habitats in the northeast Atlantic” data layer has been used26. A total of three types of threatened and/or declining habitats can be found in the area: Deep-sea sponge aggregations, Lophelia pertusa reefs and Zostera spp. beds. To avoid double-counting with the information already collected for “seagrass habitats”, Zostera beds were removed from the database. Therefore, the other two habitats were included in “Other unique habitats”. The aggregation was done using a simple rule: if any of the two habitats was present in the cell, the cell was classified as high, and as low otherwise.
Finally, the areas of importance for biodiversity at genes level was not assessed due to the lack of accessible information for the case study, despite the recent advances in this topic in the area (Fraija-Fernández et al., 2020).
Importance of coastal protection function
The method assesses the importance of the coastal protection function by identifying biological and physical protection areas. Regarding the biological protection, the method considers areas with habitats such as coastal marshes or mangroves with indicators of habitat area, coverage and/or vegetation width. Regarding the physical protection importance (i.e. high, low), the method depends on whether the coast is rocky or sandy shore, and indicators of shoreline length, coast width and slope are adopted.
Instead of developing a new metric for the coastal protection function it was decided to use the Coastal Protection Capacity (CPcap) indicator, designed by Liquete et al. (2013). This indicator is described as “the natural potential that coastal ecosystems possess to protect the coast against inundation or erosion, based on geological and ecological characteristics”. The indicator, which requires input variables such as slope, geomorphology, submarine habitats and emerged habitats, has already been estimated for the whole European coast and the data are available on request. Based on the indicator description and the variables considered in its estimation, it was considered as a good proxy for the coastal protection function of the double evaluation method.
Liquete et al. (2013) calculated the CPcap indicator for the coastal zone (i.e., the area potentially affected by extreme hydrodynamic conditions), and delimited it by the 50 m depth isobath and the 50 m height contour line, with a minimum width of 1 nm offshore and 1 km inland from the coastline. Also, these authors established a maximum extent of 50 km landwards from the shoreline to avoid identifying far inland habitats as ‘coastal’. The CPcap values were normalized from 0 to 1 and presented as irregular polygons in the data layer. To include CPcap values to the case study grid and to the MRECC method, first, for each grid cell the CPcap was re-calculated as the mean of all the values contained within the cell. Second, considering the range and distribution of CPcap values, the percentiles 33rd and 67th (1/3 and 2/3) were used to discretize them in a three-category indicator: high, mid, and low importance.
Assessment of coastal vulnerability
In the method, the assessment of coastal vulnerability is done considering coastal erosion and sand loss, via parameters such as coastal sediment types, storm surge and erosion rate (Supplementary Material SM1).
For this assessment, it was decided to use the Coastal Protection Exposure (CPexp) indicator, designed by Liquete et al. (2013). This indicator is described as “The predicted need of CP based on the climatic and oceanographic conditions of each area”. The indicator integrates information on wave regime, tidal range, relative sea level and storm surge. As for CPexp has also been estimated for the whole European ‘coastal zone’ and the data are available on request. The adaptation of CPexp values to the case study grid and the method has been done as for CPcap.
Current marine development and utilization
Chinese methodology evaluates suitable areas for marine activities (e.g., port construction, aquaculture, oil and gas facilities), naming this as ‘Suitable Marine Development and Utilization’ (Supplementary Material SM1). However, due to the lack of information, it was decided to study the current utilization of the marine environment, selecting 8 out of the 11 activity themes included in the MSFD (European Commission, 2017a) i.e., physical restructuring (ports), production of energy, cultivation of living resources, extraction of non-living resources (aggregate extraction, dredging and disposal), extraction of living resources (fisheries), security-defense (military areas), tourism and leisure, and transport (shipping). We have included a ninth activity (Marine Protected Areas), which, although is a management activity, could be assimilable to ‘education and research’ under the European Commission (2017a) list, and can be related to the statutory European Union targets, under the Biodiversity Strategy 2030 (European Commission, 2020b). Hence, the main human activities within the Bay of Biscay, as defined by the MSFD, have been considered in the analysis.
Each of these was individually assessed. When an activity takes place in a cell, the area was considered as of high importance for the activity, irrespective of the intensity, except in the cases of fisheries, shipping and tourism and recreation. In these three cases the data available allow to classify the importance of the area according to the intensity of the activity (i.e., high, mid, low) (see Table SM4).
To delimit the Marine Protected Areas, three sources of information were used: OSPAR protected areas27, Natura 2000 areas28 and Nationally Designated areas29. The protected areas were transferred to the grid attending to possible overlaps. The cells where any of the protection figure abovementioned was present were classified as high importance areas, while the rest were classified as “not present”.
To characterize the marine aquaculture activity, the current location of marine aquaculture facilities for marine fish30, shellfish31 and algae32, from EMODnet Human Activities was used. The original data were point data, and those points were translated to the case study 1x1 km grid directly. The cells where any marine aquaculture activity was present were classified as high importance areas, while the rest were classified as “not present”.
Information on the location of main ports within the case study was obtained from the Word Port Index of the Maritime Safety Information web33. This webpage includes spatial location of the main ports of the world. The cells where a port was located were classified as high importance areas, while the rest were classified as “not present”.
Location of wind farms34 and ocean energy tests sites35 facilities were obtained from EMODnet Human Activities. The cells where any of these facilities was present were classified as high importance areas, while the rest were classified as “not present”.
The location of aggregate extraction areas and dredging activities were obtained from EMODnet Human Activities3637. The aggregate extraction database includes active and non-active extraction areas, in polygon format. Within the case study area, this activity is only allowed in France. The dredging activities database includes points where dredging operations have occurred, including dredged sediment disposal in coastal areas. For the two activities, the cells where the activity is present, were classified as high importance areas, while the rest were classified as “not present”.
To characterize the fishing activity, ‘fishing intensity’ extracted from EMODnet Human activities38 was used. More precisely, the average annual fishing effort (mW fishing hours) per gear type were used. In the Bay of Biscay, these values were estimated with 2018-2021 VMS (Vessel Monitoring System) and logbook data. It is important to note that only fishing vessels >15 m long are obliged to install a VMS. The original data was presented aggregated in a 0.05x0.05 degrees grid per gear type (beam trawls, bottom otter trawls, bottom seines, dredges, pelagic trawls and seines, and static gears). To adapt these data to the MRECC method, first, the summatory of all gear types was estimated per case study grid cell (1 x 1 km2). Next, the numeric values were transformed into categorical values using 70% and 90% quantiles: <0.7 as low’, 0.7-0.9 as mid, and >0.9 as high.
The location of military areas was obtained from EMODnet Human Activities39. Military areas are usually defined as exclusion areas for other human activities. Therefore, it was considered interesting to include them in the analysis. Unfortunately, within the case study area, only information for Spain was available. To codify the activity in categorical format, the cells where the activity was present were classified as high importance areas, while the rest were classified as “not present”.
Two indicators were used to characterize the tourism and recreation activities: (i) location of bathing waters40, as reported by EU Member States to the EEA (Reference year: 2019), and (ii) vessel density of different type of recreational vessels4142 and available from the EMODnet Human Activities portal. To translate bathing waters information to the case study, the cells that included bathing water were classified as high importance areas, while the rest were classified as “not present” importance areas. For vessel density, the subtypes ‘Sailing’ and ‘Pleasure Crafts’ were considered (monthly average measured in ‘hours per km2 per month’). Data for other type of recreational vessels was not available, as for such vessels holding an AIS (Automatic Identification System) is not mandatory. Although information is available for 2017-2020 years, it was decided to download 2019 data, as the outbreak of the COVID-19 pandemic in 2020 could have altered the activity (Pita et al., 2021; Ryan et al., 2021). The original information is in raster format and was transferred to the case study grid using the zonal statistics tool in QGIS. With this tool, the mean value for each vessel subtype and per grid cell was estimated. Then, the vessel density of the two vessel sub-types was summed. To adapt this numerical value to the requirements of the MRECC method (categorical value), the 70% and 90% quantiles were used: <0.7 as low, 0.7-0.9 as mid, and >0.9 as high.
Two sources of information were used to characterize the shipping activity: (i) vessel density of different types of vessels (monthly average measured in ‘hours per km2 per month’), and (ii) vessel route density of different types of vessels (unit: routes per km2). Both data were downloaded from the EMODnet Human Activities portal. For vessel density, information on cargo, tanker, passenger, and high-speed crafts was used, while for vessel route density, data on cargo, tanker and passenger vessels was used. The original information, available in raster format, was transformed using the methodology already explained for tourism and recreation.
Data aggregation
To estimate a single value per grid cell of ecological importance, the values of importance for biodiversity (species and habitats), coastal vulnerability and coastal protection function values have been considered (Figure 2A).
In each cell, the highest value reported for any of the components is considered as the overall value or the ecological importance value. Each component has also subcomponents with a different number of indicators, which are all integrated. By giving to all the elements the same weight (or importance) we followed a conservative approach, since having a single component classified as high is enough to classify the cell as high for its ecological importance.
To obtain a single value of current ‘human activity importance’ in each cell, all the activities except Marine Protected Areas were aggregated considering, as the overall value, the highest importance reported for any of the human activity indicators (Figure 2B). Marine Protected Areas were excluded from the analysis, since they are not activities causing pressures to the marine system, and only those resulting in harm to the ecosystem components have been considered in the aggregation.
The ‘Human activity importance’ and ‘areas of ecological importance’ data products were combined to estimate an indicator of the “Ecological Risk” in the cell. These ‘Risk’ values were estimated following the rules indicated in Table 2.

Table 2 Rules adopted to determine the ecological risks according to the ecological importance and human activities.
Considering that fishing could be one of the most extensive and pervasive human activity at sea, impacting different ecosystem components (Lewinson et al., 2014; Halpern et al., 2019; Pitcher et al., 2022), in addition to the above-mentioned overall ecological risk assessment the areas of ecological importance were separately intersected with the fishing activity. Two analyses were performed: first, in areas of high ecological importance, the intensity of fishing activity was estimated with the two indicators: fishing vessel density and fishing vessel route density. Secondly, the areas of high fishing activity intensity were filtered to check the values of ecological importance within the area.
The ecological importance was also separately intersected with the current protected areas, by analyzing the values of ecological importance within the Marine Protected Areas. This intersection can provide interesting information on the level of match between already set protected areas and the ecological importance of the areas. Finally, the original methodology was slightly adapted to calculate the MRECC within the study area, estimating it as the area in which neither high ecological importance nor Marine Protected Areas (i.e., areas not suitable for marine development and utilization) exist.
Results
Evaluation of areas of ecological importance
The species included in the lists of interest in the case study grid ranged between 1 and 27 spp. km-2 and its aggregated importance is presented in Figure SM2. In total, 20,115 km2 were classified as high importance (6% of the case study) and 104,502 km2 as mid importance (29%) due to their species-level relevance. There are three main high importance areas: two on the Spanish continental shelf and one on the French continental shelf. Other smaller areas of high importance are in estuarine (e.g. Gironde and Loire) and coastal zones. Mid importance areas are on the whole continental shelf of the Bay of Biscay, down to the slope, while most of the deep-sea areas have low importance (Figure SM2).
The maps of the individual assessments per habitat types are included in Figures SM3-SM9, and its aggregated importance for biodiversity in Figure SM10. The distribution is sparser than in the case of species, but again most of the high importance areas are located within the continental shelf and estuaries, with small areas in the deep-sea corresponding to unique habitats and/or fish spawning areas.
However, not all the high importance areas have the same number of habitats classified as high. From the potential seven habitats, up to five habitats of high importance overlap in the same cell. These 5-habitats cells are in the northern part of the Bay of Biscay, close to the coast (Figure SM11). In most cases, only one habitat of high importance occurs in a cell. Intermediate values (2-4 overlaps) are close to the coast or on the continental shelf slope. In the case of coastal areas, the overlaps are mainly due to the presence of seaweed and seagrass habitats in the north of the Bay of Biscay (Brittany), and fish spawning areas, whilst on the slope the overlaps come from fish spawning areas and unique habitats.
The area covered by each habitat category, classified by importance level, is summarized in Table SM3. Over the 364,638 km2 of the case study, the most extensive habitat of high importance is fish spawning areas, which covers 16% of the surface. The remainder habitats have much lower values, representing only between 0.2% (other habitats) and 4% (seagrass beds) of the total surface.
The highest coastal protection function areas correspond to a narrow strip along the whole northern coast of Spain and most part of the south of France, whilst the northern part of the Bay has mainly low or mid importance (Figure SM12). The coastal vulnerability is a near mirror image of the previous one, with low coastal vulnerability in northern Spain and south of France, and higher vulnerability in part of Galicia and the north of France (Figure SM13).
The assessment of areas of ecological importance in the Bay of Biscay, which is the result of aggregating areas of importance for biodiversity at species and habitat level, coastal protection and coastal vulnerability, is presented in Figure 3. The estuaries, coastal area and continental shelf concentrate most of the high importance areas, whilst mid importance areas are restricted to the continental shelf and part of the deep-sea and low importance areas are mainly offshore.
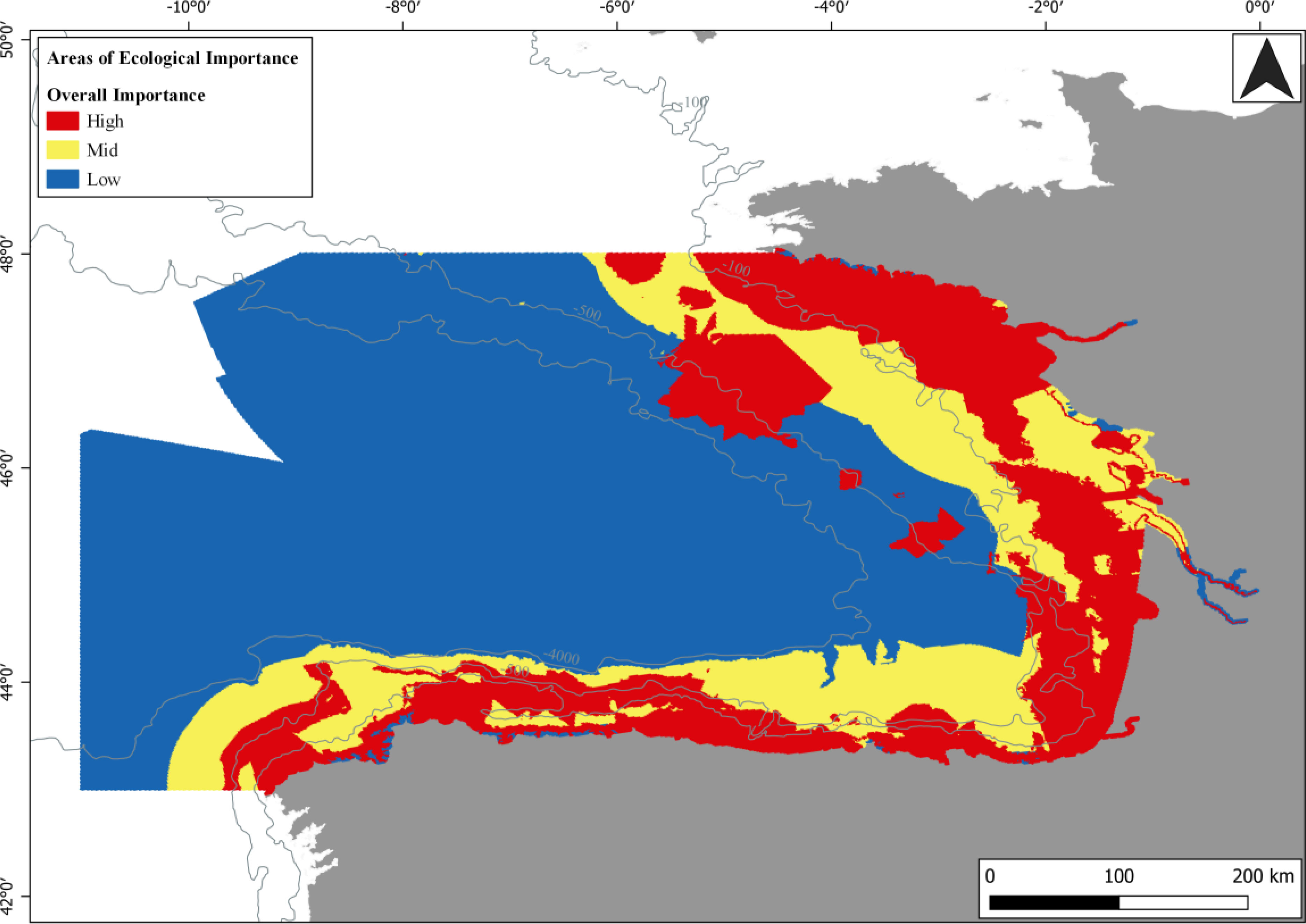
Figure 3 Areas of ecological importance in the Bay of Biscay, calculated by aggregating importance for marine biodiversity for species and habitats, coastal protection and coastal vulnerability.
A total of 81,759 km2 (22% of case study) were classified as areas of high ecological importance, 61,838 km2 as mid (17%) and 221,041 km2 as low (61%). It can be relevant to know, for the cells classified as high, for how many components the value is high per cell (Figure SM14). The case study had between zero and seven components ranked as high per km2, from the ten possible components (one for species, seven for habitats, one for coastal protection and one for coastal vulnerability).
Current marine development and utilization
After accounting for duplicates between Natura2000 sites (Special Protection Areas and Special Areas of Conservation, both under the Habitats Directive), OSPAR Marine Protected Areas and Nationally Designated Areas, a total area of 88,698 km2 (24%) was covered with one or various figures of protection and classified as of high importance (Figure SM15). Again, most of these areas are coastal, including estuaries, but also the slope of the continental shelf, with very few covering deep-sea areas.
In the Bay of Biscay, a total of 19 algae production facilities, 119 shellfish aquaculture and 8 finfish aquaculture facilities were found, most of them located in the French coast (Figure SM16).
Seventeen main commercial ports were identified within the case study, nine in France and eight in Spain (Figure SM17). Many other small ports (fishing ports, recreational ports) can be found in the case study but were not considered in this analysis.
Four wind farms (one in Spain and three in France) and four ocean energy test sites are located within the case study (Figure SM18). Currently, the three wind farms in France are planned but not operational, while the one in Spain is operational. These facilities occupied a total area of 283 km2 (0.1% of the case study area). Regarding the test sites, two are in Spain (one for waves and one for waves and wind) and two in France (one for waves and one for tidal energy). These facilities occupied a total area of 24 km2 in the case study grid.
A total of 31 aggregate extraction areas are located within the study area. All of them are in France, covering a total area of 1,283 km2 in the case study grid (Figure SM19). A total of 191 dredging points and sediment disposal areas are made available by EMODnet, 77 in France and 114 in Spain. Most of them are harbor maintenance and the associated sediment disposal areas at sea.
The fishing activity mostly occurs in the French continental shelf (see ‘High’ importance areas in Figure SM20). There is also a high and mid fishing activity parallel to the 500 m isobath, in the slope of the continental shelf. Some mid importance areas appear in the Spanish coast, between the 500 m and 4,000 m isobaths.
The information on military areas was only available for six areas in Spain (Figure SM21): four were associated with air force exercises and two with underwater exercise. Following checks for overlaps between areas, we calculated the total area covered by military use within the case study to be 23,890 km2 (6.6%).
For tourism and recreation, a total of 909 bathing waters are located within the case study (Figure SM22), 328 in Spain and 581 in France. Some of them occur in the same grid cell. Therefore, a total of 791 km2 contain bathing waters. For recreational vessel density, a total of 18,918 km2 were characterized as high importance areas, 37,835 km2 as mid importance areas and 132,423 km2 as low importance areas (Figure SM23). The remaining 175,462 km2 were considered equal to zero, in terms of recreational vessel occurrence.
For shipping activity, the two indicators show a similar pattern, with two high intensity areas in the coastal waters of Spain, and an area crossing the Bay of Biscay from the southwest boundary (i.e., Portugal) to the northern boundary (Figures SM24-25). The 9% of the case study was classified as of high importance for vessel density, and for the route density.
The aggregation of the ‘importance’ indicators of the eight activities (without including protected areas) shows that the Bay of Biscay is an area with a high concentration of human activities (Figure 4), with 26% of the area classified as high, 27% as mid and 45% as low importance area. In the remaining 3% of cells no human activity was detected.
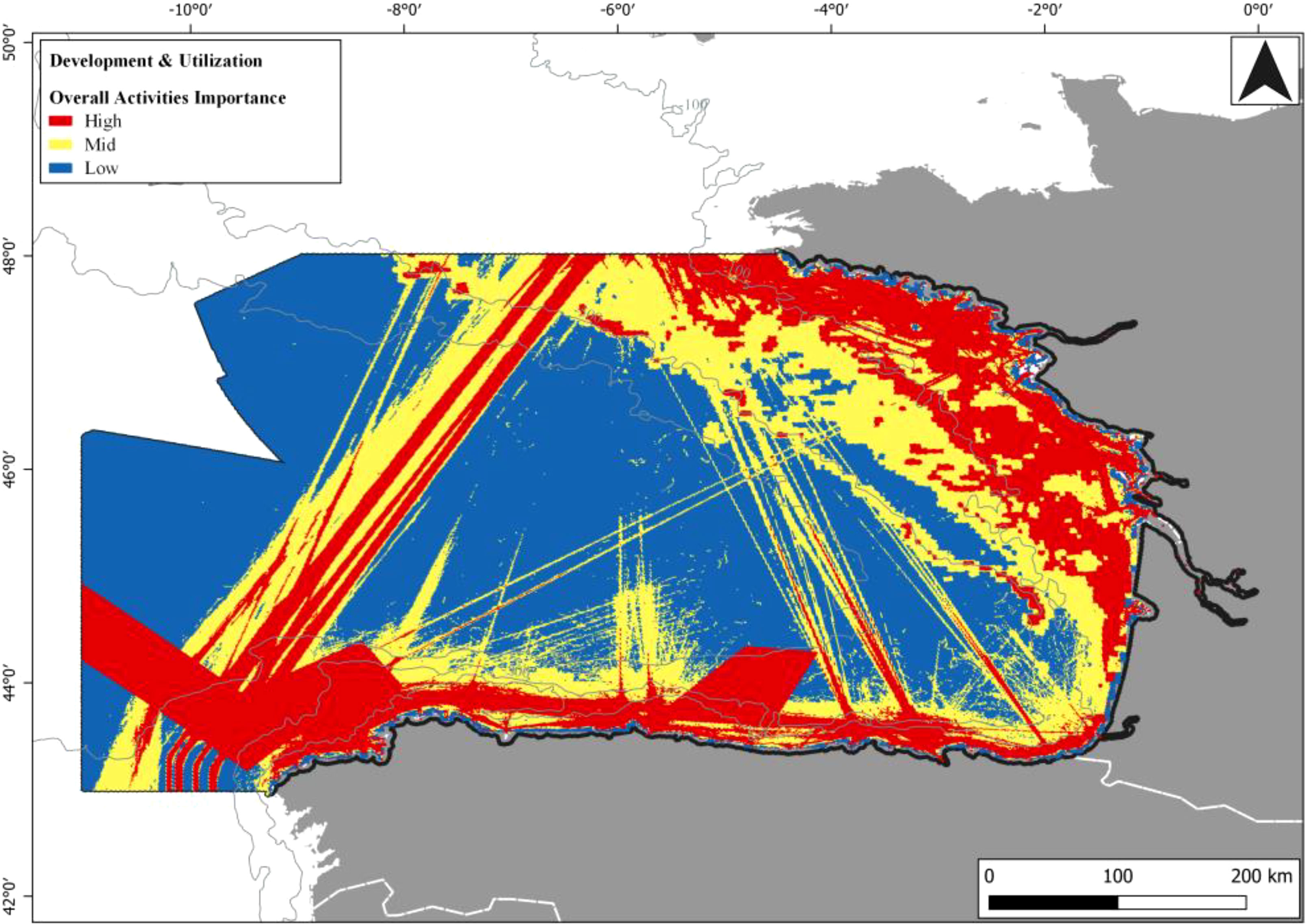
Figure 4 Aggregated assessment of the spatial distribution of important areas for human activities based on eight activities within the Bay of Biscay.
When activities were analyzed individually (Table SM4), shipping, fishing and tourism were the activities occupying the largest areas (95%, 66% and 52%, respectively, when adding high, mid and low activity). However, these activities were analyzed with dynamic indicators (e.g., vessel density and vessel route density), meaning that the occupation is not constant and has not the same intensity throughout the year in all the cells. Indeed, when those activities were analyzed considering only the cells classified as high and mid importance, the percentages were reduced to 34% for shipping, 20% for fishing and 16% for tourism.
The number of human activities per km2 ranged from 0 to 5 (Figure SM26). In most of the cells, the number of activities was 3 (34% of the case study) or 4 (37% of the case study). The two large areas in the Spanish coast with ~5 activities correspond with the two military areas.
Risk identification and evaluation of carrying capacity
When intersecting the human activities with the areas of ecological importance, the larger areas are found for protected areas (high importance for Marine Protected Areas and areas of high ecological importance), with 9.6% of the Bay of Biscay (Table 3). However, some activities harmful for the environment, such as fishing, with high or mid importance, are occurring in areas of high ecological importance (also 9.6%). When aggregating all the activities except Marine Protected Areas, 74,857 km2 (20.5%) include activities undertaken in areas of high ecological importance, and 60,594 (16.6%) in areas of mid ecological importance (Table 3).
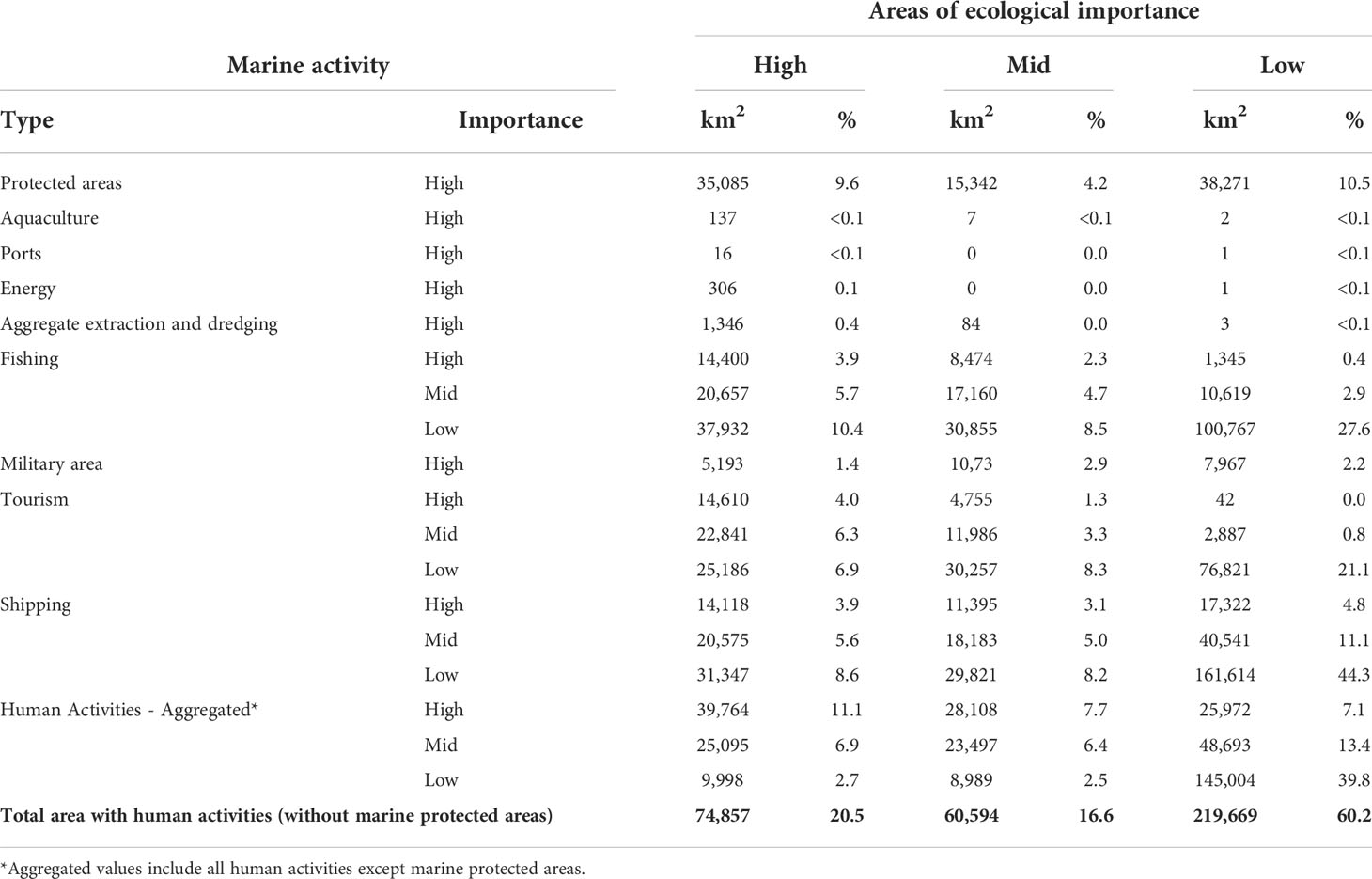
Table 3 Area (in km2 and percentage) used by each human activity within the Bay of Biscay according to the areas of ecological importance.
After intersecting human activities with the areas of ecological importance, it is possible to determine the risk caused by these eight marine activities on the areas of ecological importance. Most of the high and mid risk areas are located relatively close to the coast, on the continental shelf (Figure 5).
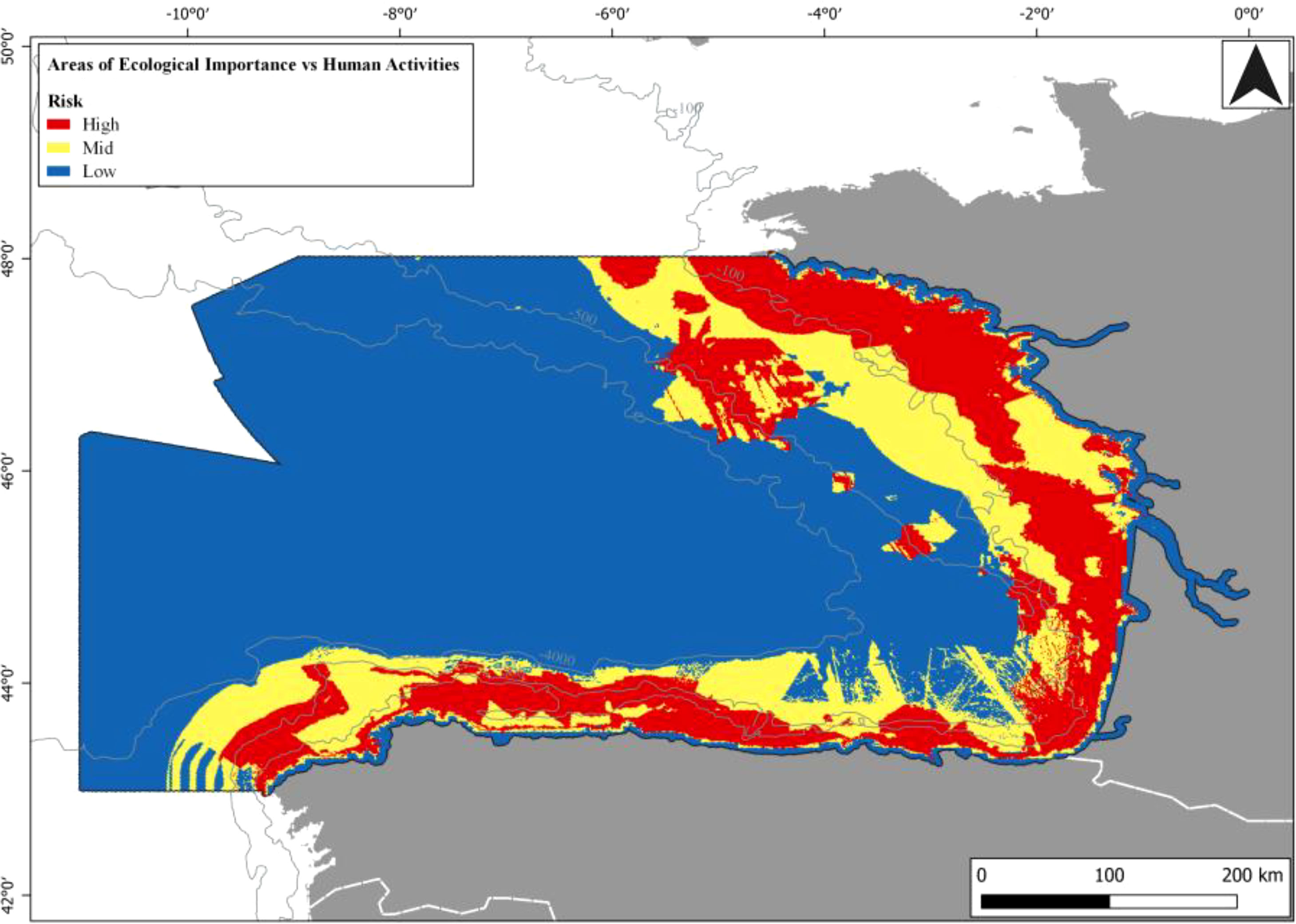
Figure 5 Ecological risk assessment based on the comparison of areas of ecological importance and human activities, within the Bay of Biscay.
Results show that 17.8% of the case study was at high risk and 17% at mid risk (Table SM5). A total of 238,176 km2 (65.3%) were classified as low risk areas, either because (i) the ecological importance was low (for any category of ‘Human Activity Importance’); (ii) the ecological importance was mid, and the importance of human activities was low; or (iii) no human activity was detected in the cell.
The number of human activities occurring within areas at high risk is represented in Figure SM27. From the 64,859 km2 classified as areas at high risk (17.8%), 98.4% have 2, 3 or 4 human activities (11,663, 45,321, and 6,807 km2, respectively) (Table SM6).
Considering that fishing is the most extensive human activity in the Bay of Biscay, a comparison with areas of ecological importance was undertaken. First, the level of fishing activity within the 81,759 km2 classified as of high ecological importance was analyzed (Figure 6), resulting in more than 66% classified as high or mid importance for fishing activity.
Secondly, the ecological importance values within high or mid importance areas for fishing activity were analyzed (Figure SM28). In this case, results suggest that from the 105,712 km2 important for fishing, most of them (52%) are classified as high according to ecological importance, while 32% and 17% are classified as areas of mid and low ecological importance, respectively.
On the other hand, Marine Protected Areas occupied a total of 88,698 km2. Of them, 40% were classified as high ecological importance, 17% as mid importance and 43% as low importance (Figure SM29). The highest extent of Marine Protected Areas classified as of low ecological importance are within two Natura2000 SPA in French waters, more precisely the SPA sites “Mers Celtiques- Talus du Golfe de Gascogne” (Natura2000 site code: FR5212016) and ‘Tête de Canyon du Cap Ferret’ (Natura2000 site code: FR7212019).
Finally, the MRECC was calculated as the area in which neither Marine Protected Areas nor areas of high ecological importance exist (both in red color in Figure 7). Since the total area covered by both is 135,372 km2, the available MRECC within the Bay of Biscay is 229,266 km2 (in blue color in Figure 7).
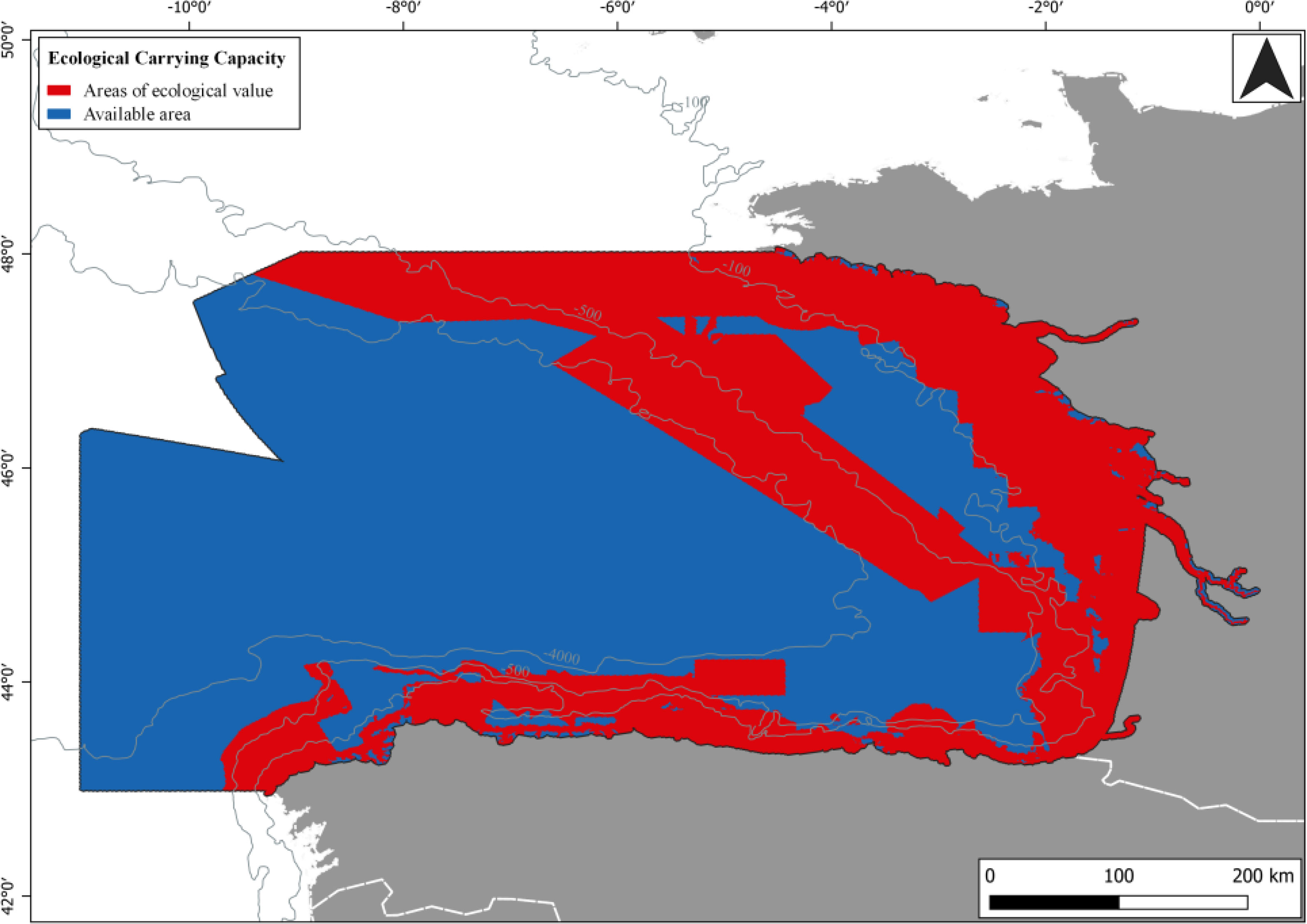
Figure 7 Marine Resource-Environment Carrying Capacity (in blue) within the Bay of Biscay. Areas where Marine Protected Areas are present and/or areas with high ecological importance are considered “areas of ecological value) (in red).
Discussion
Adapting the Chinese double evaluation approach to a European sea was challenging. Although environmental carrying capacity methods have been used in Europe for cities (Świąder et al., 2020), the circumstances are different for marine ecosystems. The terminology used in China sometimes does have not a similar meaning in Europe (e.g., ‘importance of life history’), in other cases the philosophy behind the method is different, even though the name might be the same (e.g., ‘areas of ecological importance’), or sometimes the habitats (e.g., mangroves) or threats (e.g., ‘marine disasters’) are inexistent or totally different in a European context. For these reasons this work required an adaptation of the methodology to European context, for which the MSFD (European Commission, 2008) and the MSPD (European Union, 2014), seem to be the closest references. This is because both directives aim at protecting the ocean, by making sustainable use of the marine resources (within the MSFD), and at the same time promoting the blue economy (within the MSPD) (Elliott et al., 2018; European Commission, 2020a; Stelzenmüller et al., 2020).
Despite the extensive related experience in applying the double evaluation in Chinese seas being of great support (e.g., Di et al., 2007; Fuju et al., 2011; Liao et al., 2013; Liu et al., 2020), we were able to adapt the approach only in part. The application of the double evaluation methodology to the Bay of Biscay has shown some limitations, but also interesting results, which are discussed below, considering the main components of the method.
Areas of ecological importance
The collation of information from different ecosystem components is essential for taking decisions on the future human activities that can be carried out at sea, as well as for their planning and management (Issaris et al., 2012). However, some methodological problems have been faced during the analysis process of the areas of ecological importance:
-Lack of data: for some topics, there was a total absence of data (e.g., aquatic genetic resources).
-Incompleteness of the available information: the method is based on a spatial analysis using a 1x1 km grid. Some datasets were found unfit for that purpose due to limitations with respect to spatial resolution or scale (e.g., grid size of several kilometers for some species with an ample distribution, such as mammals) or spatial coverage (e.g., lack of knowledge on the spatial distribution for some unique habitats, especially in deep-sea areas).
-In some cases, datasets provided qualitative information (e.g. presence/absence), making it difficult to evaluate the required information in a quantitative and accurate way.
-Specific indicators used in the analysis: despite the adaptation of the indicators used in Europe, in some cases it was difficult to translate them in the case study with the available information and, thus, expert judgment needed to be used (e.g., how to determine the importance of the distribution area of a species).
Sometimes, there were specific issues, associated to one of the methodological problems abovementioned. At the species level, the IUCN and Birdlife International spatial datasets were used to extract the spatial distribution of species of interest to determine the importance of distribution area for megafauna. However, the IUCN layers have low specificity, showing presence-absence of species within the Red List, with large distributions (Jefferson et al., 2021), which could result in a flawed approach, since taking a species richness approach to prioritization (ranking), does not take species representation into account. More accurate estimations for mammals and seabirds’ distribution exist (e.g., Waggitt et al., 2020). However, the number of species and the low correspondence with species of interest for conservation (e.g., after Oslo-Paris Convention, or Birds and Habitats Directives), make it difficult to use that dataset. Hence, it would be necessary to improve this part of the assessment in the future, when better information becomes available, also making the method more robust identifying biodiversity priorities by taking representation of all features into account (e.g. undertaking a systematic spatial prioritization using software like Marxan or Zonation), but studying the way in which these methods can match the original MRECC method.
In the case of habitats, the fish spawning areas were selected based on five of the most important commercial species in the Bay of Biscay, in terms of landings and economical value, representing more than 40% of total weight of landings and 30% of the total value of landings (STECF, 2019). Even if the approach implemented is robust enough, several areas for improvement have been identified. First only egg abundance data have been considered, but the inclusion of data on juvenile recruitment areas would be also of high interest (Irigoien et al., 2008). Second, the present approach considers the most captured species with the highest economic relevance, and this choice results in most species being pelagic. Future improvements would include other demersal and benthic species, helping also to cover the Descriptor 4 (food webs), under the MSFD. The inclusion of a higher number of species representative of different life-story traits, in the context of other human activities, would be of high relevance towards an ecosystem approach to fisheries (García et al., 2003).
In addition, not all habitats are represented in the analyses. Although we have included many, still some are largely absent (e.g., canyons, steep slopes, abyssal, etc.), which are likely to support species that are not represented elsewhere, and their needs should be also considered. However, although habitat maps of the Bay of Biscay exist (e.g., Galparsoro et al., 2014), the level of detail is insufficient to include the indicators needed to apply the method.
Data from Liquete et al. (2013) have been used for the assessment of coastal protection function and vulnerability. The objective of Liquete et al. (2013) was to estimate the coastal protection as an ecosystem service in Europe. They defined three indicators: ‘capacity’, ‘natural exposure’, and ‘human demand’ of coastal protection. The definition and approach followed to determine the coastal protection ‘capacity’ for coastal protection by Liquete et al. (2013) can be considered equivalent to the ‘coastal protection’ in the Chinese method. Regarding ‘coastal vulnerability’ as defined by the Chinese method, it could be considered like ‘natural exposure’ in Liquete et al. (2013); however, coastal vulnerability considers the erosion rate, and ‘natural exposure’ in Liquete et al. (2013) did not. Despite this difference, the natural exposure values as defined in Liquete et al. (2013) were used to estimate coastal vulnerability because the objective of both indicators is similar, and the information is accurate and useful in this context.
Finally, the aggregation method used to integrate the different indicators (“One high importance, all high importance”) is quite stringent, with no discrimination between areas of higher importance, due to the absence of good maps of abundance in the case of species or habitats status. To solve this issue, weighting factors can be applied to give more importance to certain indicators, as it is done when assessing the status in an integrative way (e.g. Levin et al., 2009; Langhans et al., 2014; Uusitalo et al., 2016), or to assign a higher biodiversity importance to areas where more than one component appears (e.g., a single cell where both a unique habitat such as deep-sea sponge aggregations and spawning ground of a commercial species can be found).
Despite the limitations of the methodology raised above, the aggregated assessment allowed to identify some of the most important ecological areas in the coastal zone, the continental shelf and the continental slope, in agreement with some of the assessments and recommendations undertaken in the Bay of Biscay (Lavín et al., 2006; García-Barón et al., 2020; Galparsoro and Borja, 2021). However, omitting from this study species not included in the OSPAR List of Threatened and/or Declining Species and Habitats, or listed in the Annex II of Habitats Directive, may have relevant implications. As an example, the fin whale (Balaenoptera physalus), is a non –listed species whose main critical area of distribution is in the deep waters of the south‐eastern part of the Bay of Biscay, and which could be protected by the implementation of a transboundary MPA (García-Barón et al., 2019a). In fact, this species can be considered of conservation interest as it is identified as indicator, within the functional group “marine mammals”, for achieving good environmental status under the MSFD, in the North-East Atlantic Ocean. On the other hand, it is difficult to properly assess the ecological importance of the extensive bathyal and abyssal area within the Bay of Biscay, because sufficient data are lacking and the available data are old (Laubier and Monnot, 1985; Elizalde et al., 1993; van Denderen et al., 2021; Watling and Lapointe, 2022).
Current marine development and utilization
In the analysis of activities at sea, we have included a total of 8 out of the 11 activity themes included in the MSFD (European Commission, 2017a), plus Marine Protected Areas. The methodological problems when collating information for human activities (or current marine development and utilization) were like those in the previous section (e.g., data availability, data completeness, layers creation, aggregation methodology, etc.). An example of issue with data completeness is the fact that some activities (e.g., aquaculture, ports, dredging sites, bathing waters) are represented by point data within the grid, when it is known that this does not represent the total extent of the activity, representing an underestimation of the total area covered by the activity and their footprints (Elliott et al., 2020; Solaun et al., 2021). Another example is, in the case of ports, that only main commercial ports have been included, when it is well-known the high number of small ports and marinas present within the Bay of Biscay.
Other problem refers to the activity itself and how to disentangle the activity from secondary data. For example, in the case of fisheries, the data used from EMODnet human activities come from the VMS (Marshall and Robert, 1998), in which disentangling the real fishing grounds from route data could be difficult, although its use has yielded good results in different studies (e.g., Campbell et al., 2014; Fernandes et al., 2019). Furthermore, not all the fishing activity can be characterized with VMS data, as not all the vessels are obliged to install them, e.g., small-scale fishing vessels fleet (<15 m long). This is an important gap due to the relevance of small-scale fishing vessels in the area (STECF, 2020), which can produce important pressures (Pascual et al., 2013). Similarly, for the characterization of tourism and recreation, vessel density has been used. This indicator is estimated using AIS data from sailing and pleasure crafts, but information is lacking for other recreational and sports vessels that are not obliged to install an AIS. Therefore, our method has underestimated the tourism and recreation activity.
As commented above, the designation of Marine Protected Areas, and associated management measures, are intended to reduce pressures produced by human activities; however, as this activity also requires space to be implemented, it has been included here as a type of marine space utilization but has been analyzed separately. Regarding Natura2000 areas, Special Protection Areas (under the Birds Directive) and Special Areas of Conservation (under the Habitats Directive) have been included, but no Sites of Community Importance were, since these are proposals but not already established protected areas (Pinarbaşı et al., 2020). Regarding the terminology, using the term ‘low importance’ for marine protected areas, could produce undesirable results in an MSP context, where decision-makers are looking to zone the marine space to build blue economies, since those areas could be considered as candidates for a reduction in its protection. Hence, this fact should be considered also in future improvements of the method.
This fact leads to another limitation when applying the double evaluation methodology: in China, this method includes suitable spatial development areas, and not only current ones (although some authors have used them, e.g., Ma et al., 2017). However, in the Bay of Biscay (both in France and Spain), the lack of information prevented the application of the original methodology and only current use was included. Also, this factor does not allow to make comparisons with areas in which the method has been applied in China. Hence, extraction activities (e.g. mining, oil/gas exploitation) and aquaculture, are very different in volume in China and the Bay of Biscay, and can be seen as a weakness when compared with the original Chinese model.
Despite these limitations, the aggregation of human activities shows a gradient of use, from intense use in coastal areas, to moderate use on the continental shelf and low level of use of the open sea (excepting the main shipping lanes and some areas used by fisheries). A similar gradient can be seen in the pressures produced by human activities, both at European level and in the Bay of Biscay, in a recent study by Korpinen et al. (2021). Marine Protected Areas occupy the largest area, which should serve to prevent the effects from pressures. The activities producing pressures and covering the largest areas are shipping, fishing, military and tourism, in that order, being the remaining anecdotic. These activities coincide with the most important pressures at sea, at European scale after climate change which are noise (coming from all the activities mentioned above), fish catches (from fishing) and introduction of alien species (from shipping, tourism, etc.) (Korpinen et al., 2021). In this way, clearer links between activities, pressures and their effects in the ecosystem components (and their specific targets), should be determined, for a more adequate evaluation of the carrying capacity.
Risk identification and evaluation of carrying capacity
In the MRECC, the risk assessment method has no mechanism for the risk posed to ecological features by the activities, i.e., the compatibility or lack thereof between activities and ecological features is not built into the risk assessment. Areas ranked as of ‘high’ ecological importance (because of high species richness, presence or high importance for certain habitats, high coastal protection or high coastal vulnerability) that overlap with areas of high human use (e.g. presence of coastal bathing, wind farms, or areas used highly for sailing) are then deemed ‘high risk’, with no mechanism by which those activities could or do impact the ecological features. Hence, to undertake this kind of risk assessment, there would need to be a clear, evidence-based rational for the possible impact an activity (or multiple activities) may have on an ecological feature, and this should be addressed in future improvements of the method.
Results from the combination of areas of ecological importance and human activities indicate that most of the importance come from presence of spawning areas for the most important commercial fish (Ibaibarriaga et al., 2007). However, the ecological importance of these areas tends to be seasonal, and not necessarily incompatible with all human activities, e.g., anchovy spawning takes place from March to August, peaking in May (Erauskin-Extramiana et al., 2019). Hence, an activity such as sediment disposal in September will not affect it, or other activities, such as shipping, could be hardly assessed in relation to anchovy spawning. Hence, determining the real ecological risk posed by human activities is problematic, and, for more accurate assessments, both the timing of the human activities throughout the year, and the potential overlap with areas of high ecological importance should be considered.
Also, although there are areas at high risk, with different number of human activities, it seems that, the higher the number of activities in a cell, the higher the risk, with a peak of five human activities. However, not all these activities produce the same type and intensity of pressure. Usually, methods for the calculation of the cumulative pressure effects integrate pressures without weighting the pressure effect (Ban et al., 2014; Korpinen et al., 2021). In other cases, weightings are applied to the sensitivity of the ecosystem components at risk (Halpern et al., 2015; Stelzenmüller et al., 2018; Galparsoro et al., 2021), but very few, if any, consider the additive, synergistic or antagonistic effects of multiple pressures in biodiversity importance risk (Piggott et al., 2015; Teichert et al., 2016), which are also not included in the double evaluation methodology. In this sense, the method in the Bay of Biscay could be adapted to provide a weighting factor for each human activity, based on its potential to impact the environment, as well as its temporal incidence (e.g., constant, punctual, concentrated in certain season, etc.).
Of the human activities studied, fishing could be considered one of the most extensive and pervasive human activities at sea, impacting different ecosystem components: overexploiting fish, damaging benthic communities by trawling, increasing mortality in mammals or seabirds due to bycatch, altering the food webs or introducing noise and litter into the system (Lewinson et al., 2014; Halpern et al., 2019; Pitcher et al., 2022). Significant overlaps occur between fishing and high ecological importance areas (until 52% of fishing important areas are placed in them). In some cases, it is because the fishing activity takes place in areas where fish concentrate for spawning [e.g., anchovy can only be fished during March-June, because the rest of the time is dispersed (Motos et al., 1996)]. However, in other cases, fishing grounds compete with migratory routes or feeding areas for mammals or seabirds, producing different threats to these faunistic groups in some periods of the year (Lewison et al., 2014; García-Barón et al., 2019b). The capacity of fishing activity to impact ecosystem component will differ depending on the fishing technique used, e.g., physical disturbance generated at benthic ecosystems is the main pressure produced by trawling vessels (Sciberras et al., 2016), while bycatch and lost gears are the main environmental problems generated by gillnet fleets (Shester and Micheli, 2011).
Hence, marine protection should be considered carefully when studying the human activities currently undertaken or likely to be developed. Currently, areas identified with this methodology as of high and moderate ecological importance represent 57% of the total surface protected within the Bay of Biscay. In turn, 43% of the protected areas are of low ecological importance and placed mostly on the continental shelf slope in French waters. However, this area is known to be important for some megafauna in some periods of the year, e.g., for several species of dolphins or fin and pilot whales (Laran et al., 2017; García-Barón et al., 2019a; Waggitt et al., 2020). This indicates that the methodology for delimiting areas of ecological importance probably is underestimating the importance of some areas, just because some species are not included in the ‘OSPAR List of Threatened and/or Declining species’ (within OSPAR Region IV) or in the ‘Reference List for the Marine Atlantic Region’ (Habitats Directive), or because there are not enough data. Perhaps, a regional approach to sustainable management of human activities in the Bay of Biscay should be established (van Denderen et al., 2021), like those approved by Member States within the MSFD (Cavallo et al., 2018).
The concept of carrying capacity can be diverse from different perspectives (e.g. pollutant management, ecosystem health, sustainable development), and can be categorized as environmental, ecological, resource-environmental, or social, among others (Arrow et al., 1995; Cai and Sun, 2007; Byron et al., 2011; Epelde et al., 2021). In China, much research has also been undertaken, such as Resource-Environment Carrying Capacity (Di et al., 2007; Liu et al., 2020; Zhao et al., 2021; Zhang and Niu, 2021), Environmental Carrying Capacity (Liao et al., 2013; Song and Du, 2019; Wang et al., 2021), or Ecological Carrying Capacity (Xie et al., 2011; Ma et al., 2017; Sun et al., 2022; Tang et al., 2022). Here, we used the methods described in the China official technical guidelines in the context of spatial planning, which define MRECC as the maximum volume of human activities considering resources, ecological and environmental elements. Yet, in the case of Bay of Biscay, due to the lack of some data, only the ecological part was carried out, and the suitability approach in terms of resources and environment was not taken. So, there exists some limitation about the carrying capacity, which only refers to potential space for all human activities with the constraints of ecological protection requirements. The result seems to be a broad and hard to subdivide into specific activities.
On the other hand, evaluating the available carrying capacity for development of marine activities, as the extent of the study area minus the areas highlighted as of ecological importance, could be problematic in the context of marine spatial planning. As such, the focus of MSP should be on sustainable development, which should take into account things like the intensity of activities and the compatibility of those activities with the biodiversity features in the area (e.g., areas outside of formal protection should still have some management measures to ensure sustainability). Hence, very intensive use outside Marine Protected Areas could have spill-over impacts inside Marine Protected Areas. Given the way the ecological priorities are identified, and how carrying capacity is measured, studies would need to be done to determine how multiple activities that are taking place in the area, can result in cumulative impacts compromising the carrying capacity.
Besides, the application of double evaluation is a little different in China and Europe. In China, strict protection targets are set, and high ecological importance areas are applied in MSP as a basis to delimit Marine Ecological Red Line areas (excluding some of current or potential development areas through ecological impact assessment) (Lu et al., 2015). The double evaluation shares similarities with MSP (Ehler and Douvere, 2009) and its implementation in Europe (MSPD; European Union, 2014). MSPD required Member States to complete marine plans by 2021 within their administrative boundaries (see an initial analysis performed by Pinarbaşı et al. (2020) which compares background data and strategic objectives defined by France and Spain). Once national plans are approved, they could be compared with the results obtained from the implementation of the double evaluation approach. It is expected that some of the differences could be linked to availability to national authorities of detailed data, as well as to the public consultation process conducted during the implementation process and used to reach a consensus between interests of different sectors. Thus, the approved plans will serve to predict future scenarios of MRECC, especially considering activities that are more likely to increase in the region, such as renewable energies (Jay, 2010; Pinarbaşı et al., 2019; Galparsoro et al., 2021; Maldonado et al., 2022) or the designation of new Marine Protected Areas, or for potential future exploitations able to produce significant harm to the seabed, such as deep-sea mining (Levin et al., 2016; Tunnicliffe et al., 2020). The MRECC approach provides additional information towards the adoption of an ecosystem approach to MSP, especially used together with other tools, either used in the MSPD (UNESCO-IOC/European Commission, 2021) or in the MSFD (European Commission, 2017b), ensuring the sustainability of human activities at sea. In particular, the larger number of ecological features considered for the analysis (i.e., a detailed set of biodiversity-related data, not only related to habitats and species for conservation), information on land-sea interactions, and the transboundary region in which it has been implemented that goes beyond national jurisdiction.
Weighting the ecological elements and human activities
As discussed above, the methodology used here does not include the possibility of weighting either the elements for determining the ecological importance or the human activities, when calculating the risk and MRECC, representing a limitation of the method. Weighting factors, depending on the importance or sensitivity of certain indicators can be considered (e.g., Levin et al., 2009; Langhans et al., 2014; Uusitalo et al., 2016). Here, we have used the sensitivity scores of 30 marine habitats and species against 15 anthropogenic pressures in Europe’s seas, determined by Korpinen et al. (2021), to determine the differences in the method, when weighting or not. The scores range between 0 (not sensitive) and 5 (highly sensitive) for each single pressure. From the individual values provided by the authors mentioned above, we have calculated the mean values of the 15 pressures for: (i) species of interest (including fish, birds, reptiles and mammals), with a score of 2.498; (ii) seagrasses, with 2.873; (iii) seaweeds, with 2.8; (iv) coastal marshes, with 2.57; (v) tidal flats-shallow waters, with 2.807; (v) estuaries, with 2.553; (vi) fish spawning areas, with 3.273; and (vii) unique habitats, with 2.931.
These scores have been multiplied by the numeric importance of each component in each grid cell (see Figure SM2 and Table SM3), transforming the high importance to 3, moderate importance to 2, and low importance to 1. The numeric values obtained for each component have been summed up, obtaining a unique value that comprises information on species and habitats, i.e., the value of importance for biodiversity in each grid cell. The numeric value has been categorized using the percentiles 33rd and 67th (1/3 and 2/3) as high importance (>2/3), mid importance (>1/3 and ≤2/3) and low importance (≤1/3).
In each grid cell, the ecological importance value with weighting factors was calculated as the highest value reported for any of the three components: the new-weighted importance for biodiversity values, and the previous values for coastal protection and coastal vulnerability (Figure 8A).
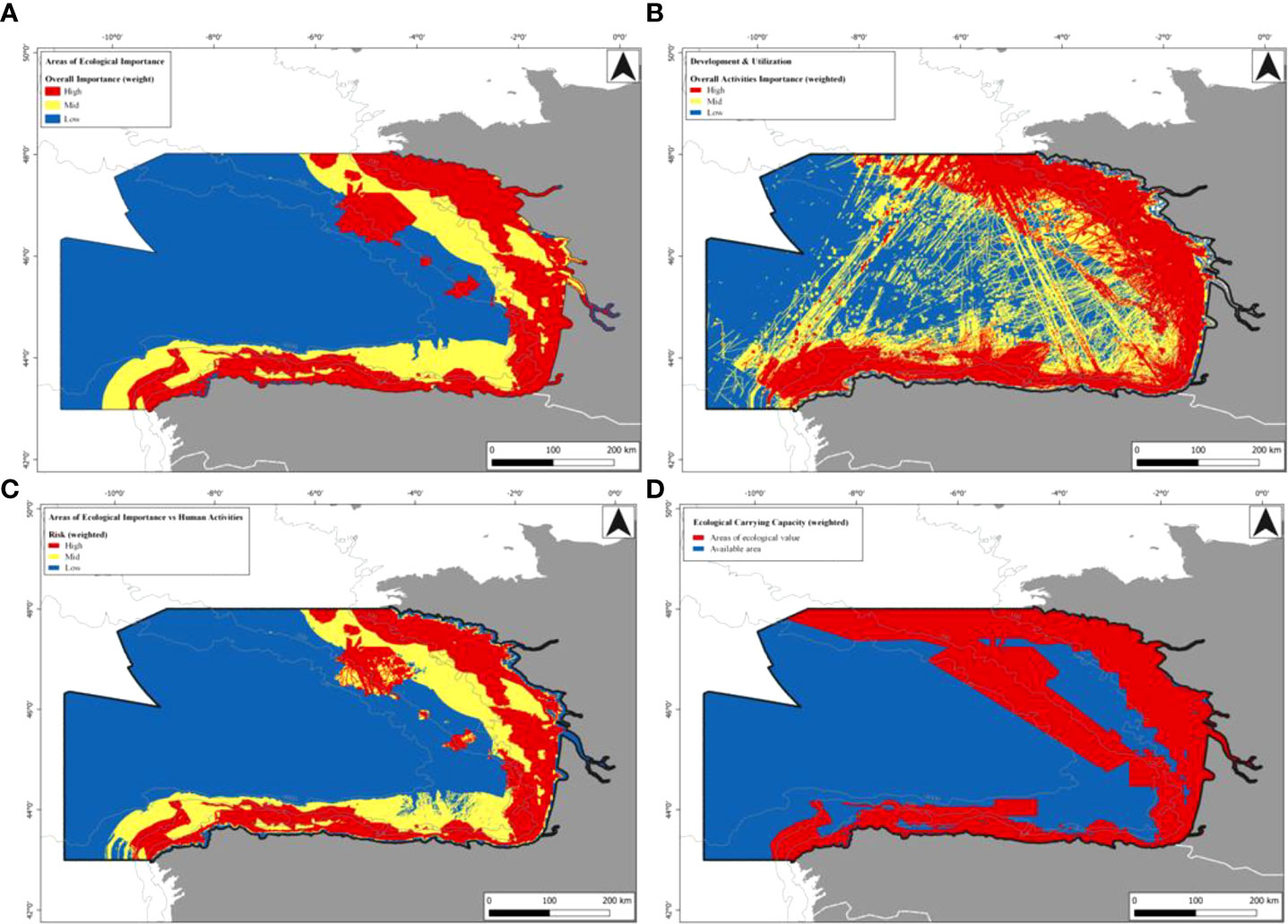
Figure 8 Results of the weighting approach: (A) Areas of Ecological Importance, (B) Aggregated assessment of the spatial distribution of important areas for human activities; (C) Ecological risk assessment; and (D) Marine Resource-Environment Carrying Capacity.
On the other hand, weights can be applied also to human activities, giving more weight to those able to increase the risk for the ecosystem components, due to pressures (Halpern et al., 2015; Stelzenmüller et al., 2018). In this case, ICES (2016) identified for the Bay of Biscay the pressures produced by the activities investigated in our study. We extracted the number of pressures by activity, as well as their intensity (value 1 for low intensity, 2 for mid and 3 for high) from the ICES report. Then, for each activity, we multiplied the number of pressures by its intensity, and we obtained the ‘pressure vs. intensity’ score: 6 for fishing, 5 for aquaculture, 4 for tourism, 3 for ports and shipping, and 2 for dredging, energy and military activities. Marine Protected Areas were not weighted, since we are looking here for risk coming from activities producing pressures.
To estimate the value of each weighted human activity per grid cell, the ‘pressure vs. intensity’ scores have been multiplied by the activity’s numeric importance (3 for high importance, 2 for mid importance and 1 for low importance), as obtained previously. A single value of weighted human activity importance was obtained by summing up weighted importance of each human activity. This numeric value has been categorized using the percentiles 33rd and 67th (1/3 and 2/3) as high importance (>2/3), mid importance (>1/3 and ≤2/3) and low importance (≤1/3) (Figure 8B).
The weighted values for ecological importance and human activities were combined to obtain risk values (Figure 8C), following the same methodology applied to not-weighted values. A total of 71,490 km2 resulted as areas at high risk, which implies an increase of 6,631 km2, compared to the original non-weighted risk values (Table 4).
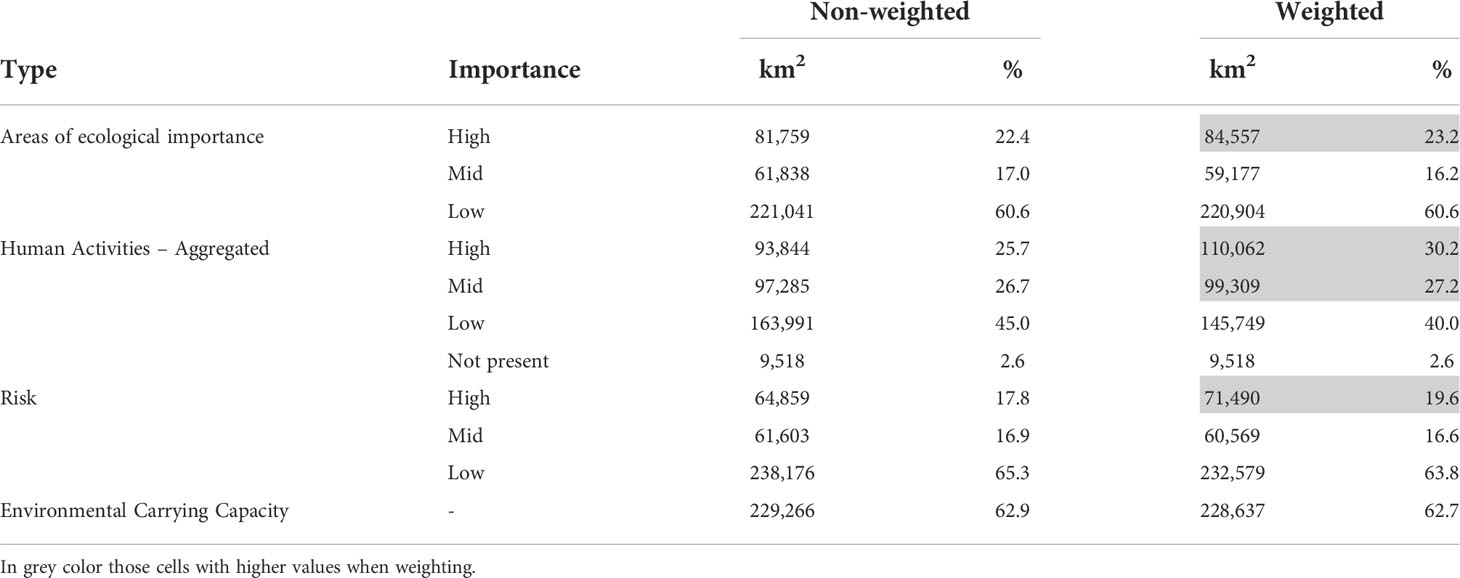
Table 4 Comparison of original non-weighted values vs. weighted values for areas of ecological importance, aggregated human activities (excluding marine protected areas), risk and environmental carrying capacity (in km2 and percentage of case study).
Applying weighted values to estimate ecological importance and human activities’ importance translates into changes for MRECC values (Figure 8D). Indeed, even if the weighting factors were not applied to the protected areas (i.e., Marine Protected Areas), the weighting approach increased the areas classified as high ecological importance, from 81,759 km2 to 84,557 km2, which means that the MRECC is slightly reduced from the 229,266 km2 (62.9% of case study) to 228,637 km2 (62.7%) (Table 4).
Hence, weighting ecological importance and human activities resulted in an increase of areas at high risk (19.6% of the Bay of Biscay) and a decrease in the MRECC. Although the changes are not very important, it seems that weighting could be more adequate to determine the risk and MRECC, since it represents a precautionary principle (O’Riordan and Jordan, 1995). In fact, this is in line with other methods to assess and manage the marine systems, in which weighting factors produce more accurate results when assessing the environmental status of marine ecosystems, and the results can change depending on the weightings used (Borja et al., 2019b). More appropriate weights (e.g. applying them differently on cells where there are multiple overlaps of data) could be chosen through a stakeholders’ consultation (Laurila-Pant et al., 2019), which in this initial application has not been taken into consideration.
Conclusions
The double evaluation methodology developed in China could not be applied directly to the European reality without some modifications. However, an adapted version of the approach was successfully applied to the Bay of Biscay. It was necessary to adapt the terminology used in the Chinese approach to better align with the current European policy needs (e.g., MSPD and MSFD), as well as to modify the list of human activities and ecosystem components (species, habitats, etc.) to be relevant to the European study area.
A number of limitations have been identified, including the lack of detailed data for certain ecosystem elements (e.g., distribution of some species and inclusion of more habitats of interest) and the availability of information regarding resource-environmental characteristics of certain human activities (e.g., aquaculture, port development, renewable energies, etc.). Thus, best available publicly accessible information was used, and human activities distribution was limited to current activities. The collation of all necessary information resulted to be the most time-consuming activity in the application of the methodology; whilst the subsequent integration and intersection of the information layers (human activities vs. areas of ecological importance) was straightforward. The future use of this methodology should include some essential improvements, for example: (i) improve the information for species distributions, probably using future data from MSFD or Regional Seas Conventions; (ii) more robust methods to identify biodiversity priorities (e.g., using Marxan); (iii) include spawning and nursery areas for fish, as well as more species representative of different life-story traits; (iv) habitats largely absent now (e.g., canyons, steep slopes, abyssal, etc.), which are likely to support species that are not represented elsewhere, should be included when information was available; (v) current and future activity plans should be considered for an accurate marine spatial planning, in the case of fishing including also vessels <15 m; (vi) the inclusion of the risks posed to ecological features by multiple activities, as well as clear evidence of links between multiple pressures and ecosystem components, will benefit and improve the application of this methodology; and (vii) the result of the approach strongly depends on the weightings applied to both sets of information, and it would be preferable to select appropriate weights through a stakeholder consultation, applying them differently on cells with multiple overlaps of data.
Applying this methodology to other areas in Europe, with different levels of data availability, could facilitate the intercomparison and assessment of the applicability of the approach. However, assumptions adopted for this approach are different to those made under the MSPD and thus, its application can be limited or will need further adaptations by Member States to accomplish with this Directive.
Data availability statement
Publicly available datasets were analyzed in this study. This data can be found here: all links are in Table 1.
Author contributions
AB developed the idea, WL and LY provided the double evaluation methodology, AB, SP, AU, and IG adapted the methodology to the European context. SP, AU, IG, AB, EM and MV collated the information. SP and AU made the calculations. WL and LY validated the results. AB wrote the first complete draft of the article and all authors contributed equally to the discussion-conclusions and in writing the final manuscript. All authors contributed to the article and approved the submitted version.
Funding
The project “International Ocean Governance: Strengthening international ocean data through the EU’s ocean diplomacy with China” is financed by the European Commission EuropeAid/139904/DH/SER/CN and this research is part of it. The content of this publication is the sole responsibility of the EMOD-PACE Consortium. The authors of this document have taken any available measure in order for its content to be accurate, consistent and lawful. The information and views set out in this research are those of the authors and do not necessarily reflect the official opinion of the European Commission. The European Commission does not guarantee the accuracy of the data included in this study. Neither the European Commission nor any person acting on the European Commission’s behalf may be held responsible for the use which may be made of the information.
Acknowledgments
Xiaoyu Fang and Jun She (EMOD-PACE project) translated the Chinese version into English. This is contribution number 1125 from AZTI’s Marine Research Division.
Conflict of interest
The authors declare that the research was conducted in the absence of any commercial or financial relationships that could be construed as a potential conflict of interest.
Publisher’s note
All claims expressed in this article are solely those of the authors and do not necessarily represent those of their affiliated organizations, or those of the publisher, the editors and the reviewers. Any product that may be evaluated in this article, or claim that may be made by its manufacturer, is not guaranteed or endorsed by the publisher.
Supplementary material
The Supplementary Material for this article can be found online at: https://www.frontiersin.org/articles/10.3389/fmars.2022.972448/full#supplementary-material
Footnotes
- ^ https://emodnet.ec.europa.eu/en/emod-pace
- ^ https://data.apps.fao.org/map/catalog/srv/eng/catalog.search#/metadata/ac02a460-da52-11dc-9d70-0017f293bd28
- ^ https://www.eea.europa.eu/data-and-maps/data/europe-seas-1
- ^ http://gis.ices.dk/geonetwork/srv/eng/catalog.search#/metadata/4745e824-a612-4a1f-bc56-b540772166eb
- ^ https://www.eea.europa.eu/data-and-maps/data/wise-wfd-spatial-3
- ^ https://www.eea.europa.eu/data-and-maps/data/eea-reference-grids-2
- ^ Only the species included in the OSPAR Agreement 2008/06 as under threat and/or declining in Region IV.
- ^ https://www.ospar.org/work-areas/bdc/species-habitats
- ^ Only the species included in the reference list as present in Spain and/or France: https://www.eionet.europa.eu/etcs/etc-bd/activities/marine-atlantic-region.pdf
- ^ https://www.iucnredlist.org/resources/spatial-data-download
- ^ http://datazone.birdlife.org/species/requestdis
- ^ https://www.emodnet-seabedhabitats.eu/access-data/download-data/
- ^ https://www.eea.europa.eu/data-and-maps/data/wise-wfd-4/wise-wfd-database-1/wise-wfd-database
- ^ https://www.emodnet-seabedhabitats.eu/access-data/download-data/?linkid=eov_macroalgae
- ^ https://www.emodnet-seabedhabitats.eu/access-data/download-data/?linkid=eusm_2019_atlantoarctic
- ^ https://www.eea.europa.eu/data-and-maps/data/wise-wfd-4/wise-wfd-database-1/wise-wfd-database
- ^ https://data.unep-wcmc.org/datasets/43
- ^ Spain:https://www.miteco.gob.es/es/biodiversidad/servicios/banco-datos-naturaleza/informacion-disponible/ramsar_descargas.aspx
- ^ France: https://rsis.ramsar.org/ris-search/?f%5B0%5D=regionCountry_en_ss%3AEurope&f%5B1%5D=regionCountry_en_ss%3AFrance
- ^ https://data.unep-wcmc.org/datasets/47
- ^ https://www.eea.europa.eu/data-and-maps/data/wise-wfd-4/wise-wfd-database-1/wise-wfd-database
- ^ https://www.eea.europa.eu/data-and-maps/data/wise-wfd-spatial-3
- ^ https://www.eea.europa.eu/data-and-maps/data/wise-wfd-4/wise-wfd-database-1/wise-wfd-database
- ^ Aggregated values were estimated with reported landings in 2016 and 2017 in the sub-regions 27.8.a, 27.8.b, 27.8.c and 27.8.d (STEFC Report 2019: https://stecf.jrc.ec.europa.eu/reports/economic/-/asset_publisher/d7Ie/document/id/2571760?inheritRedirect=false&redirect=https%3A%2F%2Fstecf.jrc.ec.europa.eu%2Freports%2Feconomic%3Fp_p_id%3D101_INSTANCE_d7Ie%26p_p_lifecycle%3D0%26p_p_state%3Dnormal%26p_).
- ^ Aggregated values were estimated with value of landings in 2016 and 2017 in the sub-regions 27.8.a, 27.8.b, 27.8.c and 27.8.d (STEFC Report 2019: https://stecf.jrc.ec.europa.eu/reports/economic/-/asset_publisher/d7Ie/document/id/2571760?inheritRedirect=false&redirect=https%3A%2F%2Fstecf.jrc.ec.europa.eu%2Freports%2Feconomic%3Fp_p_id%3D101_INSTANCE_d7Ie%26p_p_lifecycle%3D0%26p_p_state%3Dnormal%26p_).
- ^ https://odims.ospar.org/en/maps/map-threatened-or-declining-habitats/
- ^ https://carto.mpa.ospar.org/fr/1/ospar.map
- ^ https://www.eea.europa.eu/data-and-maps/data/natura-11
- ^ https://www.eea.europa.eu/data-and-maps/data/nationally-designated-areas-national-cdda-15
- ^ https://www.emodnet-humanactivities.eu/search-results.php?dataname=Finfish+Production
- ^ https://www.emodnet-humanactivities.eu/search-results.php?dataname=Shellfish+Production
- ^ https://www.emodnet-humanactivities.eu/search.php
- ^ https://msi.nga.mil/Publications/WPI
- ^ https://www.emodnet-humanactivities.eu/search-results.php?dataname=Wind+Farms+%28Polygons%29
- ^ https://www.emodnet-humanactivities.eu/search-results.php?dataname=Test+Sites
- ^ https://www.emodnet-humanactivities.eu/search-results.php?dataname=Aggregate+Extraction+Areas
- ^ https://www.emodnet-humanactivities.eu/search-results.php?dataname=Dredging
- ^ https://www.emodnet-humanactivities.eu/search-results.php?dataname=Fishing+Intensity
- ^ https://www.emodnet-humanactivities.eu/search- results.php?dataname=Military+Areas+%28Polygons%29
- ^ https://www.eea.europa.eu/data-and-maps/data/bathing-water-directive-status-of-bathing-water-12
- ^ Route density: https://www.emodnet-humanactivities.eu/search-results.php?dataname=Route+density+%28source%3A+EMSA%29
- ^ Vessel density https://www.emodnet-humanactivities.eu/search-results.php?dataname=Vessel+Density
References
Arrow K., Bolin B., Costanza R., Dasgupta P., Folke C., Holling C. S., et al. (1995). Economic growth, carrying capacity, and the environment. Science 268, 520–521. doi: 10.1126/science.268.5210.520
Ban S. S., Graham N. A. J., Connolly S. R. (2014). Evidence for multiple stressor interactions and effects on coral reefs. Global Change Biol. 20, 681–697. doi: 10.1111/gcb.12453
Borja A., Amouroux D., Anschutz P., Gómez-Gesteira M., Uyarra M. C., Valdés L. (2019a). “Chapter 5: The bay of Biscay,” in World seas: an environmental evaluation. volume I: Europe, the americas and West Africa, 2nd ed.Ed. Sheppard C. (Elsevier, London: Academic Press), 113–152.
Borja A., Andersen J. H., Arvanitidis C. D., Basset A., Buhl-Mortensen L., Carvalho S., et al. (2020). Past and future grand challenges in marine ecosystem ecology. Front. Mar. Sci. 7. doi: 10.3389/fmars.2020.00362
Borja A., Garmendia J. M., Menchaca I., Uriarte A., Sagarmínaga Y. (2019b). Yes, we can! Large-scale integrative assessment of European regional seas, using open access databases. Front. Mar. Sci. 6. doi: 10.3389/fmars.2019.00019
Byron C., Bengtson D., Costa-Pierce B., Calanni J. (2011). Integrating science into management: Ecological carrying capacity of bivalve shellfish aquaculture. Mar. Policy 35, 363–370. doi: 10.1016/j.marpol.2010.10.016
Cai H., Sun Y. (2007). Management of marine cage aquaculture: environmental carrying capacity method based on dry feed conversion rate. Environ. Sci. pollut. Res. 14, 463–469. doi: 10.1065/espr2007.05.423
Campbell M. S., Stehfest K. M., Votier S. C., Hall-Spencer J. M. (2014). Mapping fisheries for marine spatial planning: Gear-specific vessel monitoring system (VMS), marine conservation and offshore renewable energy. Mar. Policy 45, 293–300. doi: 10.1016/j.marpol.2013.09.015
Cañadas A., Vázquez J. A. (2014). Conserving cuvier’s beaked whales in the alboran Sea (SW mediterranean): Identification of high density areas to be avoided by intense man-made sound. Biol. Conserv. 178, 155–162. doi: 10.1016/j.biocon.2014.07.018
Cavallo M., Elliott M., Quintino V., Touza J. (2018). Can national management measures achieve good status across international boundaries? A Case study Bay Biscay Iberian Coast. sub-region. Ocean Coast. Manage. 160, 93–102. doi: 10.1016/j.ocecoaman.2018.04.005
Chalastani V. I., Tsoukala V. K., Coccossis H., Duarte C. M. (2021). A bibliometric assessment of progress in marine spatial planning. Mar. Policy 127, 104329. doi: 10.1016/j.marpol.2020.104329
Di Q., Han Z., Liu G., Chang H. (2007). Carrying capacity of marine region in liaoning province. Chin. Geogr. Sci. 17, 229–235. doi: 10.1007/s11769-007-0229-0
Duarte P., Meneses R., Hawkins A. J. S., Zhu M., Fang J., Grant J. (2003). Mathematical modelling to assess the carrying capacity for multi-species culture within coastal waters. Ecol. Model. 168, 109–143. doi: 10.1016/S0304-3800(03)00205-9
Ehler C., Douvere F. (2009). “A step-by-step approach toward ecosystem-based management. intergovernmental oceanographic commission and man and the biosphere programme,” in Marine spatial planning (Paris: UNESCO), 99.
Elizalde M., Sorbe J. C., Dauvin J. C. (1993). Las comunidades suprabentónicas batiales del golfo de vizcaya (margen sur del cañon de cap-ferret): composición faunística y estructura. Publ. Espec. Inst. Esp. Oceanogr. 11, 247–258.
Elliott M., Borja A., Cormier R. (2020). Activity-footprints, pressures-footprints and effects-footprints – walking the pathway to determining and managing human impacts in the sea. Mar. Pollut. Bull. 155, 111201. doi: 10.1016/j.marpolbul.2020.111201
Elliott M., Boyes S. J., Barnard S., Borja A. (2018). Using best expert judgement to harmonise marine environmental status assessment and maritime spatial planning. Mar. Pollut. Bull. 133, 367–377. doi: 10.1016/j.marpolbul.2018.05.029
Epelde I., Liria P., de Santiago I., Garnier R., Uriarte A., Picón A., et al. (2021). Beach carrying capacity management under covid-19 era on the Basque coast by means of automated coastal videometry. Ocean Coast. Manage. 208, 105588. doi: 10.1016/j.ocecoaman.2021.105588
Erauskin-Extramiana M., Alvarez P., Arrizabalaga H., Ibaibarriaga L., Uriarte A., Cotano U., et al. (2019). Historical trends and future distribution of anchovy spawning in the bay of Biscay. Deep Sea Res. Part II: Topical Stud. Oceanogr. 159, 169–182. doi: 10.1016/j.dsr2.2018.07.007
European Commission (2000). Directive 2000/60/EC of the European parliament and of the council of 23 October 2000 establishing a framework for community action in the field of water policy. Off. J. Eur. Union L327, 1–72.
European Commission (2008). Directive 2008/56/EC of the European parliament and of the council establishing a framework for community action in the field of marine environmental policy (Marine strategy framework directive). Off. J. Eur. Union L164, 19–40.
European Commission (2017a). Commission directive (EU) 2017/845 of 17 may 2017 amending directive 2008/56/EC of the European parliament and of the council as regards the indicative lists of elements to be taken into account for the preparation of marine strategies. Off. J. Eur. Communities L125, 27–33.
European Commission (2017b). Commission decision (EU) 2017/848 of 17 may 2017 laying down criteria and methodological standards on good environmental status of marine waters and specifications and standardised methods for monitoring and assessment, and repealing decision 2010/477/EU. Off. J. Eur. Communities L125, 43–74.
European Commission (2020a). Report from the commission to the European parliament and the council on the implementation of the marine strategy framework directive. Brussels 259, 307.
European Commission (2020b). Communication from the commission of the European parliament, the council, the European economic and social committee and the committee of the regions. EU Biodivers. Strat. 2030 Bringing Nat. back into our lives. Brussels 380, 27.
European Commission, Directorate General for Maritime Affairs and Fisheries, Addamo A., Calvo Santos A., Guillén J., et al. (2022). The EU blue economy report 2022. Publ. Office Eur. Union. 232. doi: 10.2771/793264
European Union (2014). Directive 2014/89/EU of the European parliament and of the council of 23 July 2014 establishing a framework for maritime spatial planning. Off. J. Eur. Union L257, 135–145.
Feng R., Chen X., Li P., Zhou L., Yu J. (2016). Development of china’s marine functional zoning: A preliminary analysis. Ocean Coast. Manage. 131, 39–44. doi: 10.1016/j.ocecoaman.2016.08.011
Fernandes J. A., Granado I., Murua H., Arrizabalaga H., Zarauz L., Mugerza E., et al. (2019). “Bay of Biscay VMS/logbook comparison (FAO subarea 27.8),” in Global atlas of AIS-based fishing activity - challenges and opportunities. Eds. Taconet M., Kroodsma D., Fernandes J. A. (Rome: FAO).
Filgueira R., Guyondet T., Bacher C., Comeau L. A. (2015). Informing marine spatial planning (MSP) with numerical modelling: A case-study on shellfish aquaculture in malpeque bay (Eastern Canada). Mar. pollut. Bull. 100, 200–216. doi: 10.1016/j.marpolbul.2015.08.048
Fraija-Fernández N., Bouquieaux M.-C., Rey A., Mendibil I., Cotano U., Irigoien X., et al. (2020). Marine water environmental DNA metabarcoding provides a comprehensive fish diversity assessment and reveals spatial patterns in a large oceanic area. Ecol. Evol. 10, 7560–7584. doi: 10.1002/ece3.6482
Frazão Santos C., Ehler C. N., Agardy T., Andrade F., Orbach M. K., Crowder L. B. (2019). “Chapter 30 - marine spatial planning,” in World seas: an environmental evaluation (Second edition). Ed. Sheppard C. (London: Academic Press), 571–592.
Fuju X. I. E., Mingxi Z., Hong Z. (2011). Research on ecological environmental carrying capacity in yellow river delta. Energy Proc. 5, 1784–1790. doi: 10.1016/j.egypro.2011.03.304
Galparsoro I., Borja Á. (2021). Defining cost-effective solutions in designing marine protected areas, using systematic conservation planning. Front. Mar. Sci. 8. doi: 10.3389/fmars.2021.683271
Galparsoro I., Borja A., Uyarra M. C. (2014). Mapping ecosystem services provided by benthic habitats in the European north Atlantic ocean. Front. Mar. Sci. 1. doi: 10.3389/fmars.2014.00023
Galparsoro I., Korta M., Subirana I., Borja Á., Menchaca I., Solaun O., et al. (2021). A new framework and tool for ecological risk assessment of wave energy converters projects. Renewable Sustain. Energy Rev. 151, 111539. doi: 10.1016/j.rser.2021.111539
Galparsoro I., Muxika I., Garmendia J. M., Rodríguez J. G. (2020). “Chapter 46 - continental shelf, canyons and pockmark fields in the southeastern bay of Biscay,” in Seafloor geomorphology as benthic habitat (Second edition). Eds. Harris P. T., Baker E. (Amsterdam: Elsevier), 769–781.
García-Barón I., Authier M., Caballero A., Vázquez J. A., Santos M. B., Murcia J. L., et al. (2019a). Modelling the spatial abundance of a migratory predator: A call for transboundary marine protected areas. Diversity Dis. 25, 346–360. doi: 10.1111/ddi.12877
García-Barón I., Santos M. B., Saavedra C., Astarloa A., Valeiras J., García Barcelona S., et al. (2020). Essential ocean variables and high value biodiversity areas: Targets for the conservation of marine megafauna. Ecol. Indic. 117, 106504. doi: 10.1016/j.ecolind.2020.106504
García-Barón I., Santos M. B., Uriarte A., Inchausti J. I., Escribano J. M., Albisu J., et al. (2019b). Which are the main threats affecting the marine megafauna in the bay of Biscay? Cont. Shelf Res. 186, 1–12. doi: 10.1016/j.csr.2019.07.009
García S. M., Zerbi A., Aliaume C., Do Chi T., Lasserre G. (2003). The ecosystem approach to fisheries. issues, terminology, principles, institutional foundations, implementation and outlook Vol. 71 (Rome: FAO)2003.
Halpern B. S., Frazier M., Afflerbach J., Lowndes J. S., Micheli F., O’Hara C., et al. (2019). Recent pace of change in human impact on the world’s ocean. Sci. Rep. 9, 11609. doi: 10.1038/s41598-019-47201-9
Halpern B. S., Frazier M., Potapenko J., Casey K. S., Koenig K., Longo C., et al. (2015). Spatial and temporal changes in cumulative human impacts on the world/’s ocean. Nat. Commun. 6. doi: 10.1038/ncomms8615
Han J. (2018). Carrying capacity of low carbon tourism environment in coastal areas from the perspective of ecological efficiency. J. Coast. Res. 83, 199–203. doi: 10.2112/SI83-031.1
Hughes G., Furley P. (1996). Threshold, carrying capacity and the sustainability of tourism: A case study of Belize. Caribb. Geogr. 7, 36–51.
Ibaibarriaga L., Irigoien X., Santos M., Motos L., Fives J. M., Franco C., et al. (2007). Egg and larval distributions of seven fish species in north-east Atlantic waters. Fish. Oceanogr. 16, 284–293. doi: 10.1111/j.1365-2419.2007.00430.x
ICES (2016). “ICES ecosystem overviews 7.1 bay of Biscay and the Iberian coast ecoregion – ecosystem overview,” in ICES advice, book(Copenhagen: ICES), vol. 7, 15.
Irigoien X., Cotano U., Boyra A., Santos M., Alvarez P., Otheguy P., et al. (2008). From egg to juvenile in the bay of Biscay: spatial patterns of anchovy (Engraulis encrasicolus) recruitment in a non-upwelling region. Fish. Oceanogr. 17, 446–462. doi: 10.1111/j.1365-2419.2008.00492.x
Issaris Y., Katsanevakis S., Pantazi M., Vassilopoulou V., Panayotidis P., Kavadas S., et al. (2012). Ecological mapping and data quality assessment for the needs of ecosystem-based marine spatial management: case study Greek Ionian Sea and the adjacent gulfs. Mediterr. Mar. Sci. 13, 297–311. doi: 10.12681/mms.312
Jay S. (2010). Strategic environmental assessment for energy production. Energy Policy 38, 3489–3497. doi: 10.1016/j.enpol.2010.02.022
Jefferson T., Costello M. J., Zhao Q., Lundquist C. J. (2021). Conserving threatened marine species and biodiversity requires 40% ocean protection. Biol. Conserv. 264, 109368. doi: 10.1016/j.biocon.2021.109368
Jouffray J.-B., Blasiak R., Norström A. V., Österblom H., Nyström M. (2020). The blue acceleration: The trajectory of human expansion into the ocean. One Earth 2, 43–54. doi: 10.1016/j.oneear.2019.12.016
Kleingärtner S. (2018). “A short history of the use of seas and oceans,” in Handbook on marine environment protection: Science, impacts and sustainable management. Eds. Salomon M., Markus T. (Cham: Springer International Publishing), 519–531.
Korpinen S., Laamanen L., Bergström L., Nurmi M., Andersen J. H., Haapaniemi J., et al. (2021). Combined effects of human pressures on europe’s marine ecosystems. Ambio 50, 1325–1336. doi: 10.1007/s13280-020-01482-x
Langhans S. D., Reichert P., Schuwirth N. (2014). The method matters: A guide for indicator aggregation in ecological assessments. Ecol. Indic. 45, 494–507. doi: 10.1016/j.ecolind.2014.05.014
Laran S., Authier M., Blanck A., Doremus G., Falchetto H., Monestiez P., et al. (2017). Seasonal distribution and abundance of cetaceans within French waters- part II: The bay of Biscay and the English channel. Deep Sea Res. Part II: Topical Stud. Oceanogr. 141, 31–40. doi: 10.1016/j.dsr2.2016.12.012
Laurila-Pant M., Mäntyniemi S., Venesjärvi R., Lehikoinen A. (2019). Incorporating stakeholders’ values into environmental decision support: A Bayesian belief network approach. Sci. Total Environ. 697, 134026. doi: 10.1016/j.scitotenv.2019.134026
Lavín A., Valdés L., Sánchez F., Abaunza P., Forest A., Bouucher J., et al. (2006). “Chapter 24. the bay of Biscay: The encountering of the ocean and the shelf,” in The Sea, volume 14. Eds. Robinson A. R., Brink K. H. (Harvard: Harvard University Press), 933–1001.
Levin P. S., Fogarty M. J., Murawski S. A., Fluharty D. (2009). Integrated ecosystem assessments: Developing the scientific basis for ecosystem-based management of the ocean. PloS Biol. 7, e1000014. doi: 10.1371/journal.pbio.1000014
Levin L. A., Mengerink K., Gjerde K. M., Rowden A. A., Van Dover C. L., Clark M. R., et al. (2016). Defining “serious harm” to the marine environment in the context of deep-seabed mining. Mar. Policy 74, 245–259. doi: 10.1016/j.marpol.2016.09.032
Lewison R., Crowder L. B., Wallace B. P., Moore J. E., Cox T., Zydelis R., et al. (2014). Global patterns of marine mammal, seabird, and sea turtle bycatch reveal taxa-specific and cumulative megafauna hotspots. Proc. Natl. Acad. Sci. 111, 5271–5276. doi: 10.1073/pnas.1318960111
Liao E., Jiang Y., Yan X.-H., Chen Z., Wang J., Zhang L. (2013). Allocation of marine environmental carrying capacity in the xiamen bay. Mar. Pollut. Bull. 75, 21–27. doi: 10.1016/j.marpolbul.2013.08.023
Li J., Jiang B., Lin N. (2018a). A study on the influence mechanism of port environmental carrying capacity. Asian J. Shipping Log. 34, 191–197. doi: 10.1016/j.ajsl.2018.09.002
Liquete C., Zulian G., Delgado I., Stips A., Maes J. (2013). Assessment of coastal protection as an ecosystem service in Europe. Ecol. Indic. 30, 205–217. doi: 10.1016/j.ecolind.2013.02.013
Liu R., Pu L., Zhu M., Huang S., Jiang Y. (2020). Coastal resource-environmental carrying capacity assessment: A comprehensive and trade-off analysis of the case study in jiangsu coastal zone, eastern China. Ocean Coast. Manage. 186, 105092. doi: 10.1016/j.ocecoaman.2020.105092
Li M., Yin P., Duan X., Liu J., Qiu J., Phung V. P. (2018b). Land-use changes and human driving in and near the Yangtze river delta from 1995–2015. J. Ocean Univ. China 17, 1361–1368. doi: 10.1007/s11802-018-3735-z
Lu W.-H., Liu J., Xiang X.-Q., Song W.-L., Mcllgorm A. (2015). A comparison of marine spatial planning approaches in China: marine functional zoning and the marine ecological red line. Mar. Policy 62, 94–101. doi: 10.1016/j.marpol.2015.09.004
Maldonado A. D., Galparsoro I., Mandiola G., de Santiago I., Garnier R., Pouso S., et al. (2022). A Bayesian network model to identify suitable areas for offshore wave energy farms, in the framework of ecosystem approach to marine spatial planning. Sci. Total Environ. 156037, 838. doi: 10.1016/j.scitotenv.2022.156037
Marshall P., Robert T. (1998). FAO technical guidelines for responsible fisheries, no.1 Suppl.1 (Rome: Food and Agriculture Organization of the United Nations).
Ma P., Ye G., Peng X., Liu J., Qi J., Jia S. (2017). Development of an index system for evaluation of ecological carrying capacity of marine ecosystems. Ocean Coast. Manage. 144, 23–30. doi: 10.1016/j.ocecoaman.2017.04.012
Motos L., Uriarte A., Valencia V. (1996). The spawning environment of the bay of Biscay anchovy (Engraulis encrasicolus l.). Sci. Marina 60, 117–140.
O’Riordan T., Jordan A. (1995). The precautionary principle in the contemporary environmental policy. Environ. Values 4, 191–212. doi: 10.3197/096327195776679475
Pascual M., Borja A., Galparsoro I., Ruiz J., Mugerza E., Quincoces I., et al. (2013). Total fishing pressure produced by artisanal fisheries, from a marine spatial planning perspective: A case study from the Basque country (Bay of Biscay). Fish. Res. 147, 240–252. doi: 10.1016/j.fishres.2013.06.010
Piggott J. J., Townsend C. R., Matthaei C. D. (2015). Reconceptualizing synergism and antagonism among multiple stressors. Ecol. Evol. 5, 1538–1547. doi: 10.1002/ece3.1465
Pita P., Ainsworth G. B., Alba B., Anderson A. B., Antelo M., Alós J., et al. (2021). First assessment of the impacts of the COVID-19 pandemic on global marine recreational fisheries. Front. Mar. Sci. 8. doi: 10.3389/fmars.2021.735741
Pitcher C. R., Hiddink J. G., Jennings S., Collie J., Parma A. M., Amoroso R., et al. (2022). Trawl impacts on the relative status of biotic communities of seabed sedimentary habitats in 24 regions worldwide. Proc. Natl. Acad. Sci. 119, e2109449119. doi: 10.1073/pnas.2109449119
Pınarbaşı K., Galparsoro I., Alloncle N., Quemmerais F., Borja Á. (2020). Key issues for a transboundary and ecosystem-based maritime spatial planning in the bay of Biscay. Mar. Policy 120, 104131. doi: 10.1016/j.marpol.2020.104131
Pınarbaşı K., Galparsoro I., Depellegrin D., Bald J., Perez-Moran G., Borja A. (2019). A modelling approach for offshore wind farm feasibility with respect to ecosystem-based marine spatial planning. Sci. Total Environ. 667, 306–317. doi: 10.1016/j.scitotenv.2019.02.268
Pörtner H. O., Scholes R. J., Agard J., Archer E., Arneth A., Bai X., et al. (2021). Scientific outcome of the IPBES-IPCC co-sponsored workshop on biodiversity and climate change (Report). Bonn, Germany: IPBES secretariat. 256. doi: 10.5281/zenodo.4659158
QGIS.org. (2022) QGIS geographic information system. Available at: http://www.qgis.org.
Ryan K. L., Desfosses C. J., Denham A. M., Taylor S. M., Jackson G. (2021). Initial insights on the impact of COVID-19 on boat-based recreational fishing in Western Australia. Mar. Policy 132, 104646–104646. doi: 10.1016/j.marpol.2021.104646
Sciberras M., Parker R., Powell C., Robertson C., Kröger S., Bolam S., et al. (2016). Impacts of bottom fishing on the sediment infaunal community and biogeochemistry of cohesive and non-cohesive sediments. Limnol. Oceanogr. 61, 2076–2089. doi: 10.1002/lno.10354
Sha S. (2020). The early warning model of tourism environmental carrying capacity measurement in coast and island regions. J. Coast. Res. 103, 1042–1046. doi: 10.2112/SI103-217.1
Shester G. G., Micheli F. (2011). Conservation challenges for small-scale fisheries: Bycatch and habitat impacts of traps and gillnets. Biol. Conserv. 144, 1673–1681. doi: 10.1016/j.biocon.2011.02.023
Shokri M. R., Mohammadi M. (2021). Effects of recreational SCUBA diving on coral reefs with an emphasis on tourism suitability index and carrying capacity of reefs in kish island, the northern Persian gulf. Regional Stud. Mar. Sci. 45, 101813. doi: 10.1016/j.rsma.2021.101813
Solaun O., Sagarminaga Y., Menchaca I., Galparsoro I. (2021). Spatial overlap between human activities and seabed habitats in European seas: Insights into EMODnet’s data for management purposes. Mar. Policy 130, 104551. doi: 10.1016/j.marpol.2021.104551
Song S., Du Y. (2019). The environmental carrying capacity of marine resources in the offshore areas of the Yangtze river economic belt in China. J. Coast. Res. 98, 14–17. doi: 10.2112/SI98-004.1
STECF. (2019). The 2019 annual economic report on the EU fishing fleet (STECF 19-06) Eds. Carvalho N., Keatinge M., Guillen Garcia J. (Luxembourg; Publications Office of the European Union).
STECF. (2020). The 2020 annual economic report on the EU fishing fleet (STECF 20-06) (Luxembourg: Publications Office of the European Union).
Stelzenmüller V., Coll M., Cormier R., Mazaris A. D., Pascual M., Loiseau C., et al. (2020). Operationalizing risk-based cumulative effect assessments in the marine environment. Sci. Total Environ. 724, 138118. doi: 10.1016/j.scitotenv.2020.138118
Stelzenmüller V., Coll M., Mazaris A. D., Giakoumi S., Katsanevakis S., Portman M. E., et al. (2018). A risk-based approach to cumulative effect assessments for marine management. Sci. Total Environ. 612, 1132–1140. doi: 10.1016/j.scitotenv.2017.08.289
Sun J., Miao J., Mu H., Xu J., Zhai N. (2022). Sustainable development in marine economy: Assessing carrying capacity of Shandong province in China. Ocean Coast. Manage. 216, 105981. doi: 10.1016/j.ocecoaman.2021.105981
Świąder M., Lin D., Szewrański S., Kazak J. K., Iha K., van Hoof J., et al. (2020). The application of ecological footprint and biocapacity for environmental carrying capacity assessment: A new approach for European cities. Environ. Sci. Policy 105, 56–74. doi: 10.1016/j.envsci.2019.12.010
Tang Y., Wang M., Liu Q., Hu Z., Zhang J., Shi T., et al. (2022). Ecological carrying capacity and sustainability assessment for coastal zones: A novel framework based on spatial scene and three-dimensional ecological footprint model. Ecol. Model. 466, 109881. doi: 10.1016/j.ecolmodel.2022.109881
Tang H., Yue Q., Wang C., Zhou L., Yu J., Wu W., et al. (2020). Relationships between two kinds of MSP in China. Mar. Econ. Manage. 3, 1–11. doi: 10.1108/MAEM-12-2019-0013
Teichert N., Borja A., Chust G., Uriarte A., Lepage M. (2016). “Restoring fish ecological quality in estuaries: Implication of interactive and cumulative effects among anthropogenic stressors,” in Science of the total environment. 542, 383–393.
Tunnicliffe V., Metaxas A., Le J., Ramirez-Llodra E., Levin L. A. (2020). Strategic environmental goals and objectives: Setting the basis for environmental regulation of deep seabed mining. Mar. Policy 114, 103347. doi: 10.1016/j.marpol.2018.11.010
UNESCO-IOC/European Commission (2021). MSPglobal international guide on Marine/Maritime spatial planning (Paris: UNESCO (IOC Manuals and Guides no 89).
United Nations (2016). Report of the inter-agency and expert group on sustainable development goal indicators. (E/CN.3/2016/2/Rev.1). new York. United Nations Econ. Soc. Council, 49.
United Nations (2021a). The second world ocean assessment. volume I (New York: United Nations publication), 570.
United Nations (2021b). The second world ocean assessment. volume II (New York: United Nations publication), 520.
Uusitalo L., Blanchet H., Andersen J., Beauchard O., Berg T., Bianchelli S., et al. (2016). Indicator-based assessment of marine biological diversity – lessons from 10 case studies across the European seas. Front. Mar. Sci. 3. doi: 10.3389/fmars.2016.00159
van Denderen P. D., Holah H., Robson L. M., Hiddink J. G., Menot L., Pedreschi D., et al. (2021). A policy-based framework for the determination of management options to protect vulnerable marine ecosystems under the EU deep-sea access regulations. ICES J. Mar. Sci. 79, 34–49. doi: 10.1093/icesjms/fsab237
Waggitt J. J., Evans P. G. H., Andrade J., Banks A. N., Boisseau O., Bolton M., et al. (2020). Distribution maps of cetacean and seabird populations in the north-East Atlantic. J. Appl. Ecol. 57, 253–269. doi: 10.1111/1365-2664.13525
Wang X., Liu L., Zhang S. (2021). Integrated model framework for the evaluation and prediction of the water environmental carrying capacity in the guangdong-Hong Kong-Macao greater bay area. Ecol. Indic. 130, 108083. doi: 10.1016/j.ecolind.2021.108083
Watling L., Lapointe A. (2022). Global biogeography of the lower bathyal (700–3000 m) as determined from the distributions of cnidarian anthozoans. Deep Sea Res. Part I: Oceanogr. Res. Pap. 181, 103703. doi: 10.1016/j.dsr.2022.103703
Xie F., Zheng M., Zhang H. (2011). Research on ecological environmental carrying capacity in yellow river delta. Energy Proc. 5, 1784–1790. doi: 10.1016/j.egypro.2011.03.304
Yue W. Z., Wu T., Wang T. Y., Xia H. X. (2020). “Double evaluations” for territorial spatial planning: Challenges and responses. J. Natural Resour. 35 (10), 2299–2310. doi: 10.31497/zrzyxb.20201001
Zhang T., Niu X. (2021). Analysis on the utilization and carrying capacity of coastal tidal flat in bays around the bohai Sea. Ocean Coast. Manage. 203, 105449. doi: 10.1016/j.ocecoaman.2020.105449
Keywords: carrying capacity, vulnerability, human activities, marine protection, marine spatial planning, cumulative effects
Citation: Borja A, Pouso S, Galparsoro I, Manca E, Vasquez M, Lu W, Yang L and Uriarte A (2022) Applying the China’s marine resource-environment carrying capacity and spatial development suitability approach to the Bay of Biscay (North-East Atlantic). Front. Mar. Sci. 9:972448. doi: 10.3389/fmars.2022.972448
Received: 18 June 2022; Accepted: 26 August 2022;
Published: 20 September 2022.
Edited by:
Nadia Papadopoulou, Hellenic Centre for Marine Research (HCMR), GreeceReviewed by:
Andrea Barbanti, Department of Earth System Sciences and Technologies for the Environment (CNR), ItalyAndrew Conway, Marine Institute, Ireland
Copyright © 2022 Borja, Pouso, Galparsoro, Manca, Vasquez, Lu, Yang and Uriarte. This is an open-access article distributed under the terms of the Creative Commons Attribution License (CC BY). The use, distribution or reproduction in other forums is permitted, provided the original author(s) and the copyright owner(s) are credited and that the original publication in this journal is cited, in accordance with accepted academic practice. No use, distribution or reproduction is permitted which does not comply with these terms.
*Correspondence: Angel Borja, aborja@azti.es