- 1Faculty of International Maritime Studies, Kasetsart University, Chonburi, Thailand
- 2National Hydraulic Research Institute of Malaysia, Ministry of Environment and Water, Seri Kembangan, Selangor, Malaysia
Sand bypassing is one of the promising solutions to rectify jetty-induced coastal erosion. Estimating alongshore sediment transport rate and understanding hydrodynamic conditions at a jetty are crucial for successful downdrift erosion management. This research investigated three major jetties in Thailand (Cha Am jetty, Krai jetty, and Na Saton jetty) that protrude across the surf zone and completely intercept alongshore sediment transport. Sub-aerial and inter-tidal field surveys by Real Time Kinematic (RTK) technique were undertaken in 2019 and 2020. The collected data was processed and overlaid to calculate the amount of sediment deposition at the updrift jetty. Numerical simulations using MIKE21 SW and MIKE21 HD were carried out in order to understand how waves and water currents interacted with the jetties. From the results, we found that the Cha Am jetty trapped approximately 38,187 cu.m/yr of the alongshore sediment. While the Krai jetty intercepted approximately 34,170 cu.m/yr of the alongshore drift, and approximately 65,951 cu.m/yr of longshore sediment transport was blocked by the Na Saton jetty. Such estimated amounts of deposited sediment are the quantities that should be bypassed at each jetty. Budgets and implementation plans for sand bypassing can be prepared. Decision makers can decide how to manage updrift deposition and downdrift erosion.
Introduction
Coastal zone is dynamic (Ariffin et al., 2018). Wind, waves, tides, and currents are major forcings that influence sediment transport (King et al., 2019; Fan et al., 2019). When waves break, they create longshore current within surf zone (United States Army Corps of Engineers, 1984; Lim et al., 2018). The effect of tidal currents is added to wave-generated currents, mobilizing sediment. Sediment transport, in turn, affects coastal geomorphology (Ariffin et al., 2019; Selamat et al., 2019; Saengsupavanich, 2020). Fan et al. (2019) found that swells induce intensified sediment resuspension and a flood-ebb symmetry of suspended sediment concentration. Net horizontal sediment fluxes are significantly increased in the presence of strong waves. Significant amounts of suspended sediments can be taken away from shoreline, implying the occurrence of coastal erosion. Sediment movement also varies seasonally, depending on incoming wave and water current characteristics, as well as monsoons (Amalan et al., 2018; Ratnayake et al., 2018; Ismail et al., 2020; Shetty and Jayappa, 2020; Zulfakar et al., 2021). Based on the theory of sediment budget (Kamphuis, 2010), coastal erosion can occur when the quantity of incoming sediment is less than the quantity of sediment leaving a certain coastal cell. Understanding and being able to estimate the net quantity of sediment transport is, therefore, one of the key requirements that lead to sustainable coastal management (Ariffin et al., 2020; Fortunato et al., 2021).
Many structures are constructed in coastal zones to protect the coast and to enhance the quality of living (Saengsupavanich, 2013; Oyegbile and Oyegbile, 2017; Saengsupavanich, 2017; Ariffin et al., 2020; Zulfakar et al., 2020). Jetties are one of the most encountered coastal structures. They help maintain safe navigation in and out of inlets. They also help fishermen, who are vulnerable to climate change (Muhammad et al., 2016), in terms of convenient catch transportations, while mitigating inland flooding. However, one of the critical environmental impacts of jetties is significant shoreline change. Ghashemizadeh and Tajziehchi (2013) and Rangel-Buitrago et al. (2015) showed that jetty construction influenced wave propagation, seabed evolution, and shoreline deposition as well as erosion. The sediment deposition associated with jetties is one of the key topics for sustainable coastal zone management. Previous studies have reported significant beach accretion on the updrift side in response to jetty constructions (Hapke et al., 2013; Garel et al., 2015; Wang et al., 2022). Salleh and Nadzir (2020) showed that flow velocity and current direction around a jetty significantly affected the deposition of coastal sediments. In the United States, Hein et al. (2019) presented that the northwards diffracted/refracted waves induced sediment accretion proximal to the south of the jetty and delivered the sediment across the jetty to an adjacent area. Silva et al. (2021) reported that the immediate updrift shoreline at the Tweed River, Australia, responded rapidly (from the first few months to two-three years) to the introduction of coastal management structures, while the extension of those impacts further updrift along the beach was gradual and took decades. In Europe, Žilinskas et al. (2020) showed that a sediment accretion occurred in a nearshore zone after completing a jetty. In Iran, Azarmsa et al. (2009) evidently showed the increased sedimentation, reduction in significant wave height, and flushing rate after a jetty construction at the Kiashahr lagoon. Anh et al. (2021) investigated the erosion-deposition process along a jetty in Vietnam and found that there was 56,442 m3 of deposited sediment during the northeast monsoon, which was ten times higher than the amount occurring in the southwest monsoon.
While a jetty creates an updrift coastline deposition, the downdrift shoreline is eroded (Bruun, 1995; Saengsupavanich, 2019; Ariffin et al., 2020). Installing more coastal protection structures, such as revetments or breakwaters, along the downdrift eroded shoreline cannot solve such a problem, rather the erosion will be migrated further downdrift (Nassar et al., 2018; Ariffin et al., 2020; Zulkafar et al., 2020). One of the possible solutions to downdrift erosion is sand-bypassing (Garel et al., 2014; Garel et al., 2015; Nassar et al., 2018), which involves moving the sand deposited at the updrift side to the downdrift zone. In Palm Beach County Florida, South Lake Worth Inlet (Boynton Beach Inlet) is a man-made inlet cut in 1927; a sand transfer plant was installed in 1937 to solve the problematic accretion and erosion (Zurmuhlen, 1957; Witmer et al., 2018). Boswood and Murray (2001) listed 53 different bypassing stations from around the world, including Oceanside Harbor, USA, with a pumping rate of 75,000–190,000 m3/year, and Channel Islands, USA, with an average pumping rate of 1,000,000 m3/year. Another example is the fixed system at the Tweed River Entrance in Australia where pumps located within and offshore of the entrance channel achieved a maximum daily rate of over 12,000 m3 (Dyson et al., 2002). The Indian River inlet, USA, constructed in 1940, experienced downdrift horizontal shoreline erosion between 10 and 60 m during the pre-bypassing period, but accreted 10–20 m during the bypassing period (Keshtpoor et al., 2013). The quantity of the sand being bypassed must be determined correctly, otherwise it will not solve the problem, and may create other issues. If the sediment is bypassed less than what it should be, downdrift erosion will still occur. On the other hand, if too much sediment is bypassed, the erosion will take place along the updrift shoreline instead. Overdredging may destabilize nearby coastal structures or induce slope instability and sliding because the counter-weight is taken away, as mentioned in many geotechnical engineering investigations (Murthy, 2003). Thus, accurately determining the quantity of sediment that needs to be bypassed is of utmost importance. Additionally, where to place the bypassed sediment on the downdrift shoreline must be clearly understood. For instance, when the bypassed sediment is blocked by any structure, it will be trapped, and less sediment will be supplied to another area (Ariffin et al., 2020).
Estimating deposited sediment at a jetty can be carried out by empirical calculation, numerical simulation, and field measurement. The empirical formula has been proposed by many researchers, such as Bayram et al. (2007) and Kamphuis (1991). Numerical simulation can be undertaken using many commercially available software packages such as MIKE21 or LITPACK (developed by Danish Hydraulics Institute) (Nassar et al., 2018; Rautenbach and Theron, 2018) or CMS (developed by US Army Corps of Engineers) (Wang and Beck, 2012). Although the implementations of both empirical equations and numerical simulations are useful, coastal engineers are well aware that there are many unknown parameters, as well as a lot of related calibration procedures, in such calculations that can lead to inaccurate results. On the other hand, the field measurements have been applied. Bergillos et al. (2017) applied topographic measurements over 36 days to compare them with numerical models predicting cross-shore distribution of alongshore sediment transport. Each topographic survey was performed under low tide conditions and the observations were referenced to the mean low water spring level. Masselink et al. (2016) collected subaerial beach morphological data by using RTK-GPS and total station to estimate the beach volume above the mean sea level along the Atlantic coast of Europe. Their surveys were then used to estimate storm impacts on beaches. Nevertheless, there is no previous publication about quantifying sediment deposition at jetties in Thailand. This study is the first one of its kind to estimate intertidal intercepted sediment quantity at jetties in Thailand. To determine net longshore sediment transport and to identify the volume of sediment that should be bypassed at certain jetties in Thailand, this study applies field surveys to determine the net alongshore sediment transport. Numerical simulations are carried out to help understand wave and water current around the jetties. This research will greatly help Thai coastal managers and the Thai government to solve jetty-induced downdrift erosion by appropriately estimating the required amount of sediment that should be bypassed.
Study locations and methodology
Study areas
This research focused on net alongshore sediment transport at three major jetties in Thailand, being Cha Am jetty, Krai jetty, and Na Saton jetty (Figure 1; Table 1). All of them extend across the surf zone, completely intercepting alongshore sediment transport. Each jetty has created severe downdrift coastal erosion. A responsible governmental department attempted to mitigate the downdrift erosion by constructing detached breakwaters at both the Krai jetty and the Na Saton jetty, while private property owners at the Cha Am jetty built a revetment along the downdrift shoreline. Such approaches did not solve the problems, but postponed erosion further downdrift. Historical coastline change analysis showed that each jetty simultaneously created updrift deposition and downdrift erosion. At the Cha Am jetty (Figures 2A, B), the accretion rate at the updrift jetty was greater than 4 m/yr, while the downdrift erosion occurring at the endpoint of the seawalls constructed by the private property owners was more severe than 1.5 m/yr. At the Krai jetty, the updrift jetty intercepted the alongshore sediment, inducing coastline deposition at a rate greater than 4 m/yr. Although the Marine Department attempted to solve the downdrift erosion by installing fourteen detached breakwaters, erosion still occurred further downdrift at a rate greater than 2 m/yr (Figures 2C, D). Similarly, the Na Saton jetty created updrift deposition with a rate of roughly 4.5 m/yr (Figures 2E, F). However, downdrift erosion was small because the Marine Department had constructed a series of detached breakwaters along the 7-km downdrift shoreline (Saengsupavanich, 2012).
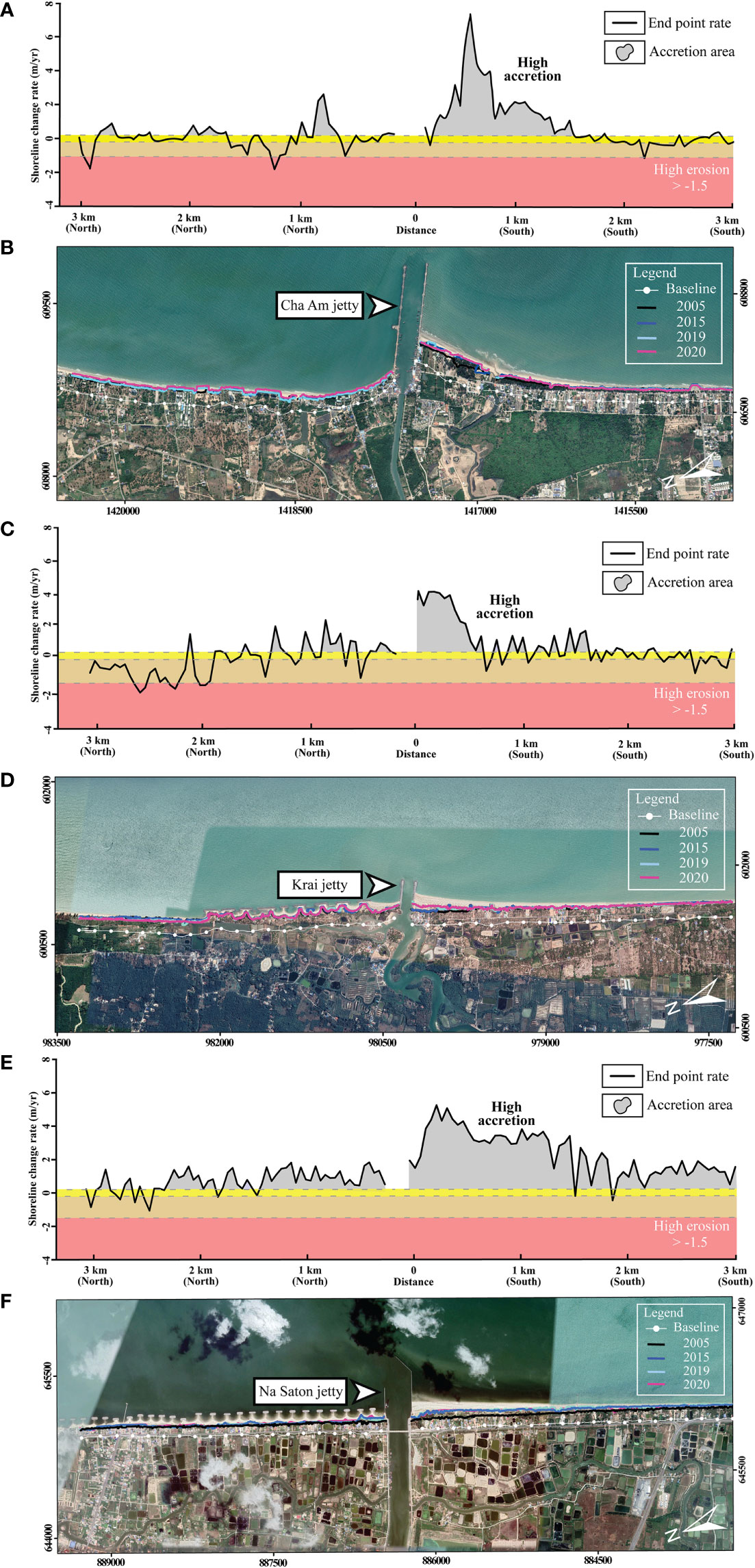
Figure 2 Updrift deposition and downdrift erosion at the jetties in this study. (A) Shoreline change rate at the Cha Am jetty, (B) historical coastline near the Cha Am jetty, (C) Shoreline change rate at the Krai jetty, (D) historical coastline near the Krai jetty, (E) Shoreline change rate at the Na Saton jetty, (F) historical coastline near the Na Saton jetty.
The jetties in this study are located along the east side of southern Thailand (Figure 1). They are exposed to seasonal storms during the northeast monsoon (November to February). Long-term tidal statistics near each jetty were collected from the Royal Thai Navy and the Marine Department (Table 2). To determine an appropriate time and scope for field surveys, information about wave climate at the jetties was indispensable. For each jetty, JONSWAP method (Kamphuis, 2010) was applied with data from the nearest wind station of the Thai Meteorological Department.
After calibrating the wave hindcasting calculation, the authors found that dominant waves at the Krai jetty were from the east. The annual calm period (having a significant wave height of less than 0.5 m or Beaufort scale level 3) was approximately 89% (Figure 3). Big waves with wave height greater than 4 m (Beaufort scale level 6) are from the east. When considering coastal alignment, it could be concluded that net alongshore sediment transport at this location was from the southeast to the northwest. Coastal deposition was expected to occur along the south side of the Krai jetty. Therefore, the field survey to determine the net alongshore sediment transport was carried out along the south side of the jetty. For the Na Saton jetty, the same approach was undertaken, because the annual wave characteristics, as well as the shoreline alignments, of the Krai jetty and the Na Saton jetty are very similar. At the Cha Am jetty, JONSWAP calculation showed that dominant waves with height greater than 2 m (Beaufort scale level 5) were from the south-southeast (SSE). The annual calm period (having a significant wave height of less than 0.5 m or Beaufort scale level 3) was approximately 91.4% (Figure 3). Therefore, sediment accretion was expected to occur along the south side of the Cha Am jetty, thus, the location of the field survey.
Survey methods
Time for the surveys was determined from the monsoon period (November to February). Direction of alongshore sediment transport depends on season. The basic theory of pre- and post-storm beach morphology suggests that the coast is likely to be eroded during storms when big waves are present (in this study, the stormy months are between November to February). Some sediment deposited at the updrift jetty will be carried offshore and will again return to the beach berm during the calm period (March to October). In the meantime, the calm waves that obliquely approach the shoreline will carry new sediment to deposit more at the updrift jetty. Thus, the suitable time for the survey along the south side of the jetties (Cha Am jetty, Krai jetty, and Na Saton jetty) was prior to the stormy season. The authors chose to conduct field surveys in August 2019, September 2019, August 2020, and September 2020.
The field investigations measured coastal elevation by Real Time Kinematic (RTK) technique. Benchmark descriptions can be found in Table 3. The coastal level along 2-km shoreline south from each jetty was surveyed, with each beach profile being spaced 25 m apart. In order to cover the sub-aerial and intertidal zones, the uppermost point of each beach profile was set at a beach dune foot, while the lowermost point was set at an elevation of -0.5 m from national mean sea level (MSL). The number of the RTK survey points at each jetty can be found in Table 4. Although net alongshore sediment transport should be measured from the beach dune down to the closure depth, in order to achieve the perfect result, no echo-sounding was undertaken because of self-supported financial limitations. This study focuses on the sub-aerial and intertidal sediment deposition at the updrift jetties, not in the surf zone. Such deposition would be a potential source of the sediment that might be bypassed. This survey scope, which measures only the sub-aerial and the intertidal beach profiles, has been applied by many researchers to quantify sediment volume (Masselink et al., 2016; Bergillos et al., 2017).
Analysis of field data
After acquiring relevant field data, the authors created a bathymetric map of each survey by AutoCAD 3D software package, using a bilinear interpolation technique. The ArcGIS software package was then applied afterwards to overlay the maps of each month at each jetty (August 2019 versus August 2020, and September 2019 versus September 2020). The results revealed how much of the sediment was deposited along the surveyed coastal strips. Volumetric change of the sediment was then calculated from multiplying the difference in vertical beach elevation with the horizontal area. Since finer sediment may bypass the jetty tip, leaving coarser sediment trapped at the updrift jetty, the amount of the deposited sediment at the updrift jetty should be the amount of the required sediment to be manually bypassed.
Numerical modelling
The two-dimensional MIKE 21 Spectral Wave (SW) and Flexible Mesh Hydrodynamic Module (FM-HD) model packages, developed by the Danish Hydraulic Institute, were implemented to study the 2-dimension significant wave field and free-surface flow respectively. A flexible mesh was used to simulate the hydrodynamic model, as it was suitable for irregular boundaries and to determine the wave and current parameters in coastal areas. The model domain, covering the whole southern South China Sea and the Gulf of Thailand, had two open boundaries; the north boundary started from east of Vietnam to west of the Philippines, while the south boundary started from south of Johor to west of Kalimantan. General Bathymetric Chart of the Oceans (GEBCO) and MIKE C-Map were utilized for better water depth coverage in offshore and coastal waters. The data was processed by a pre-processing tool called Mesh Generator in MIKE ZERO to prepare the bathymetry. The unstructured mesh had a medium resolution of 3 km in the southern South China Sea region and a fine resolution of 1 km in Thailand’s coastal waters, including the jetty locations.
The numerical simulations started from November 2018 to December 2019. They were spun up by the six-hourly wind and wave data retrieved from the Era-Interim European Centre for Medium-Range Weather Forecast (ECMWF), with a spatial resolution of 0.125° x 0.125°. Afterward, a model validation was performed by comparing the simulation outputs with field data measured by an Acoustic Doppler Current Profiler-Acoustic Wave and Currents (ADCP-AWAC) at the nearshore area in Terengganu, Malaysia (5°26’33.936” N, 103°9’37.548” E) for three months with every 10-min interval. The root-mean-square-error was found to be 0.17 for significant wave height and 0.13 for current speed, which were considered acceptable, referring to Ariffin et al. (2016) (0.28), Shariful et al. (2020) (0.18), and Zulfakar et al. (2021) (0.15).
Results
Intertidal intercepted sediment at Cha Am jetty
This research found that sediment accumulated at the updrift Cha Am jetty, as expected. Most deposition occurred on the beach berm. The increase of beach elevation was +0.80 to + 1.0 m in some areas, and the increase decreased further away from the jetty (Figure 4). On the other hand, the elevation in a further offshore area did not change much. Beach erosion was also found near a creek outlet, probably because of creek discharge. Multiplying the changes in the coastal elevation with the area and summing them up revealed a volumetric change of the intertidal intercepted sediment at the updrift jetty. It was found that the Cha Am jetty trapped approximately 38,187 cu.m/yr (Table 5).
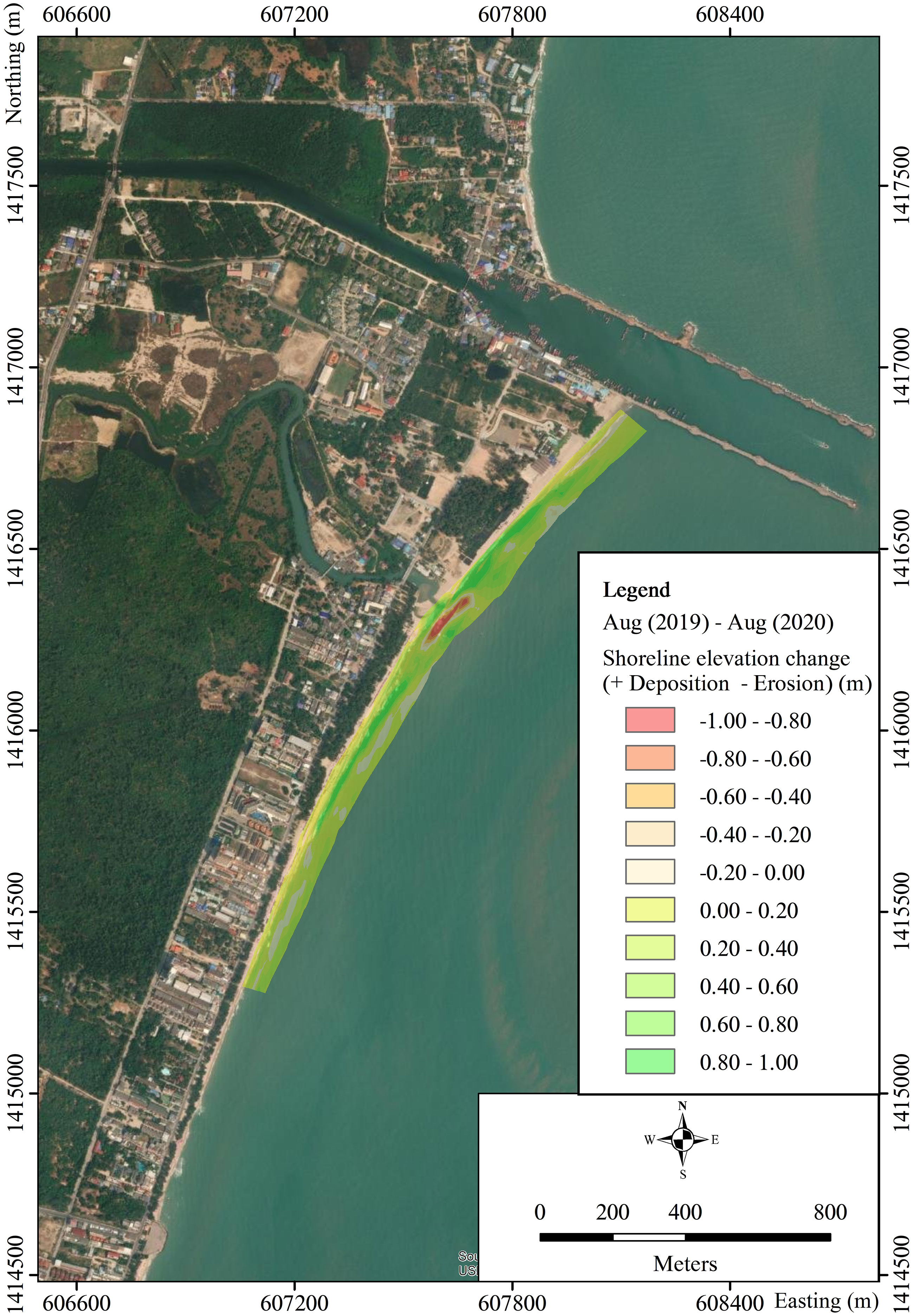
Figure 4 Differences in coastal elevation at the updrift Cha Am jetty (August 2019 versus August 2020).
The seasonal pattern of waves at the Cha Am jetty changes during the southwest (SW) and northeast (NE) monsoons (Figure 5). During the SW monsoon (May to October), predominant waves come from the southeast (SE) direction, transporting sediment northwards. They are not severe, having wave heights of less than 0.50 m (Figure 5A). However, the waves are stronger during February to April (the transitional period), also coming from the SE direction, carrying the sediment northwards. On the other hand, while the waves around the Cha Am jetty during the NE monsoon (November to January) are not aggressive, they come from the NE direction, moving the sediment southwards (Figure 5B). Since the Cha Am jetty is located near the bottom of the Gulf of Thailand, a NE fetch distance is not long enough to generate high waves. Therefore, at the Cha Am jetty, the waves during February to October move the sediment northwards, while the waves during November to January carry the sediment southwards. Moreover, alongshore water current is greatly affected by the Cha Am jetty (Figures 5C, D). The water current flows northwards during flood tides, and vice versa. Current speed is normally less than 0.3 m/s. The water current in the shallow zone near the jetty flows very slowly, while the current speed at the tips is much higher. The Cha Am jetty clearly changes the waves and the water currents, thus resulting in alongshore sediment transport.
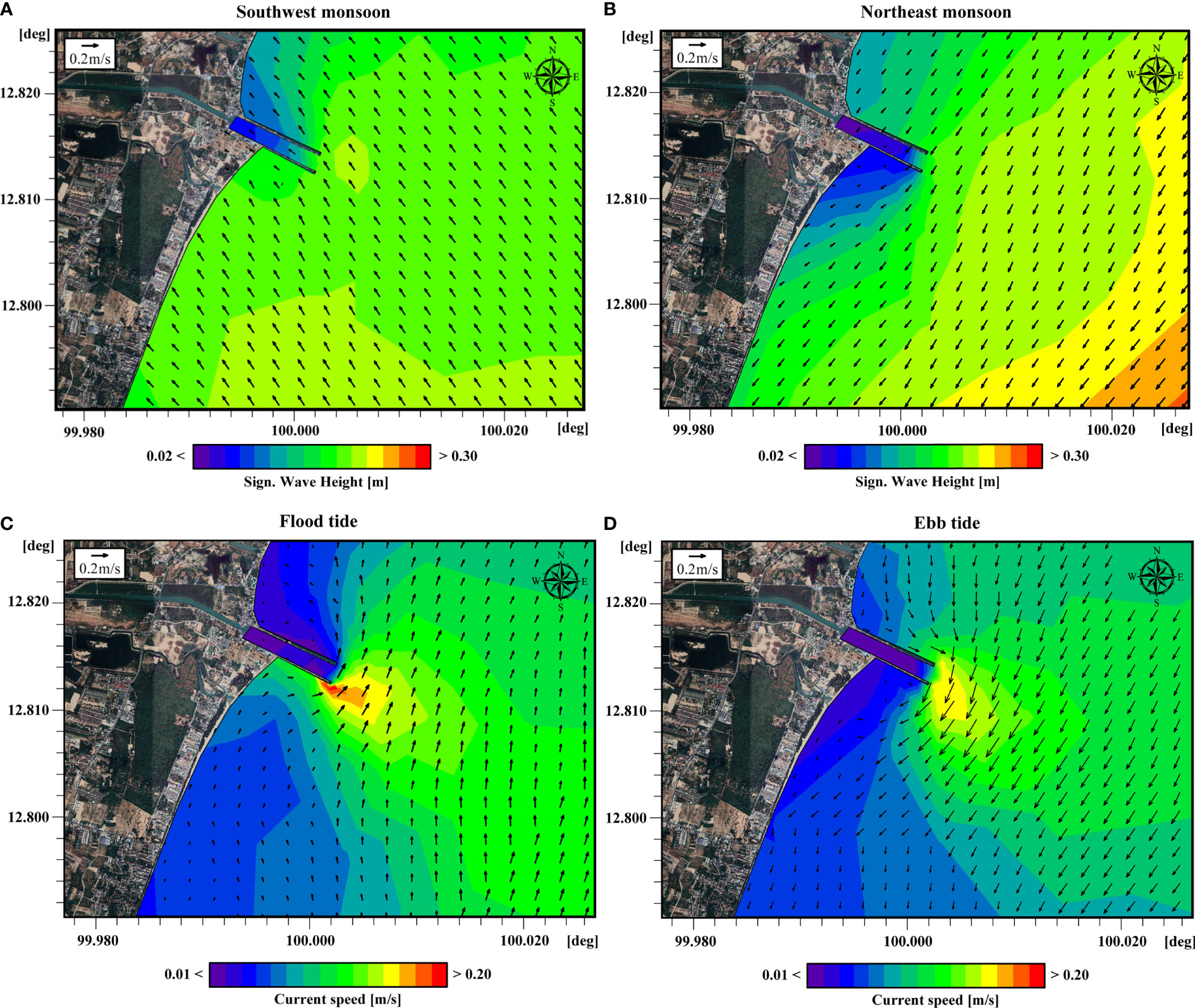
Figure 5 Results of MIKE 21 SW and HD at the Cha Am jetty (A) significant wave height and direction (arrows) during the southwest monsoon, (B) significant wave height and direction (arrows) during the northeast monsoon, (C) current speed and direction (arrows) during flood tide, (D) current speed and direction (arrows) during ebb tide.
Intertidal intercepted sediment at Krai jetty
As anticipated, the updrift coastal strip at the Krai jetty experienced noticeable deposition. Most sediment accumulation took place within 1 km south from the jetty. Further down south, the coastal elevation did not exhibit much change (Figure 6). Multiplying the differences of the coastal elevation with the areas and adding them together showed a volumetric change of the intertidal deposited sediment at the updrift jetty. As a result, it was found that the Krai jetty intercepted approximately 34,170 cu.m/yr of the alongshore sediment (Table 5). Such an amount of the intertidal intercepted sediment was the quantity that should be bypassed to the downdrift shoreline.
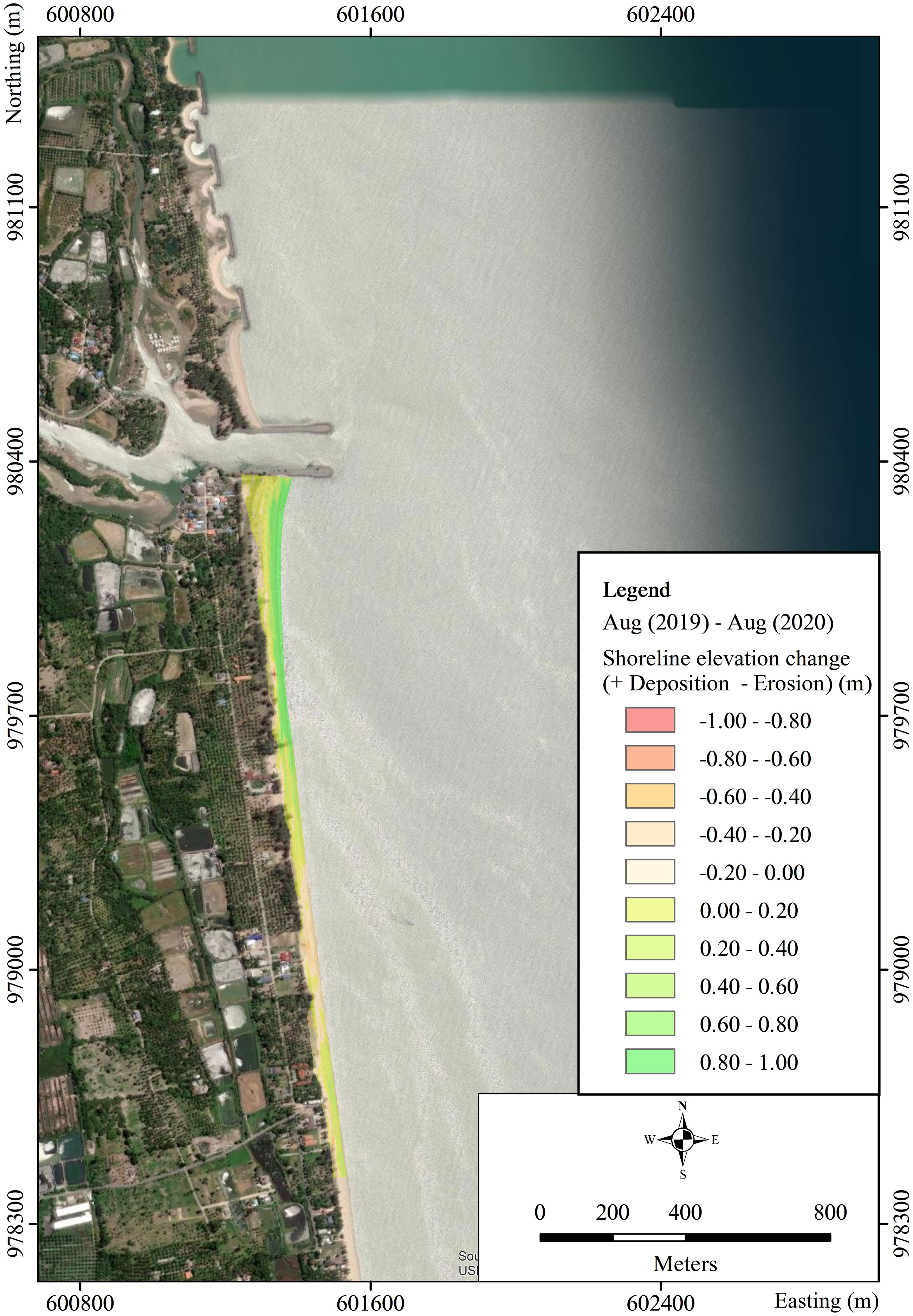
Figure 6 Differences in coastal elevation at the updrift Krai jetty (August 2019 versus August 2020).
Figure 7 reveals that the Krai jetty influences nearshore wave regime and water current pattern, resulting in the altered alongshore sediment transport. During the SW monsoon (May to October), waves at the Krai jetty are calm. They mainly come from the SE direction, moving sediment northwards (Figure 7A). The Krai jetty is also under the influence of the NE monsoon (November to January), when large waves approach the shoreline from the NE (Figure 7B), transporting the sediment southwards. During the transitional period (February to April), the waves come from the east, but with a smaller wave height. Therefore, at the Krai jetty, the waves during February to October transport the sediment northwards. On the other hand, the waves during November to January carry the sediment southwards. Moreover, water current around the Krai jetty is greatly deviated (Figures 7C, D). The water current flows northwards during flood tide, and vice versa. Current speed varies with a normal maximum value of less than 0.3 m/s. The water current flows very slowly around the downdrift detached breakwaters, while the offshore current speed is faster. The Krai jetty evidently has an impact on coastal hydrodynamics, resulting in altered alongshore sediment deposition.
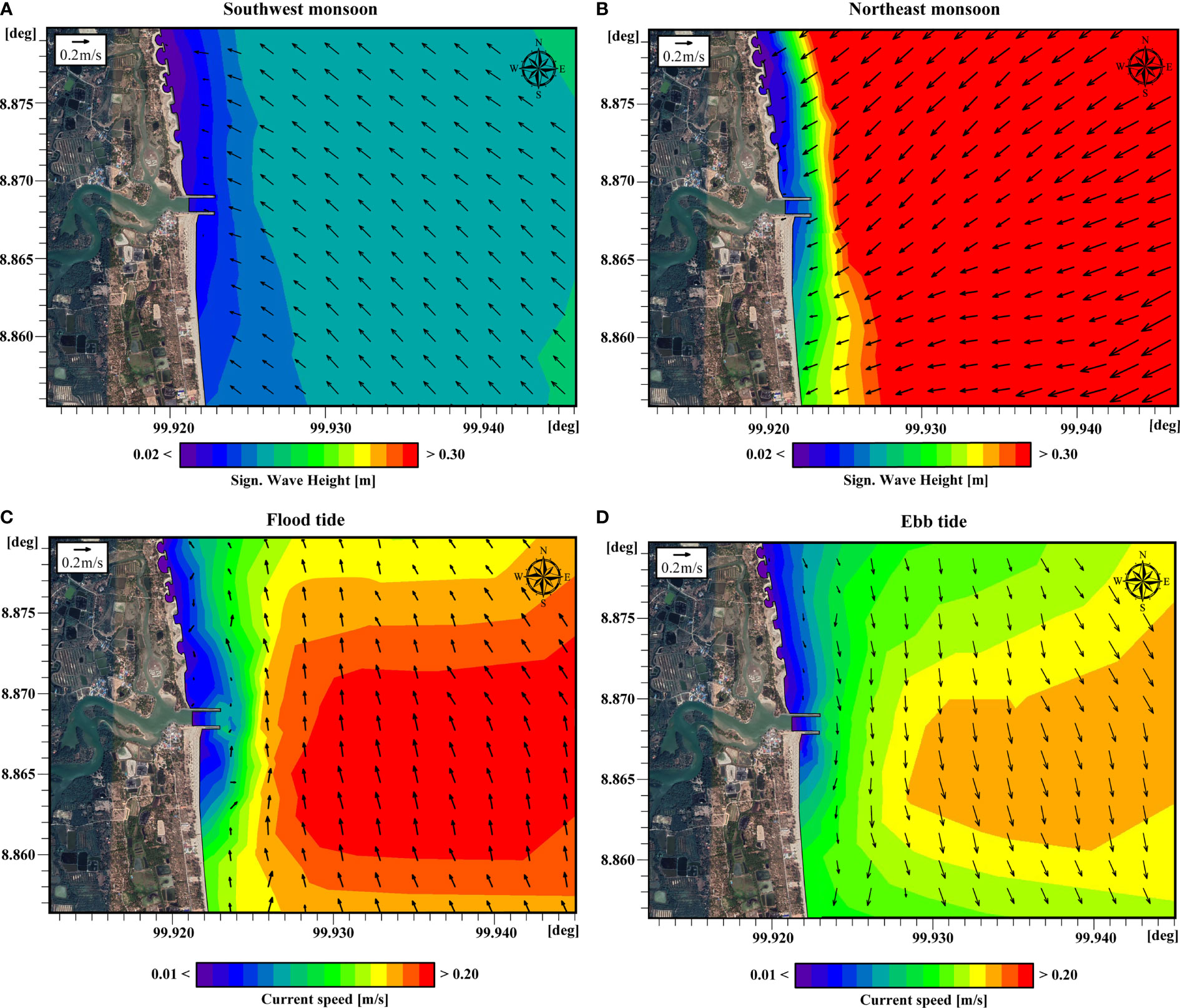
Figure 7 Results of MIKE 21 SW and HD at the Krai jetty (A) significant wave height and direction (arrows) during the southwest monsoon, (B) significant wave height and direction (arrows) during the northeast monsoon, (C) current speed and direction (arrows) during flood, (D) current speed and direction (arrows) during ebb tide.
Intertidal intercepted sediment at Na Saton jetty
The intertidal sediment accumulation rate at the Na Saton jetty was estimated by the same approach applied to the previous jetties. It was found that approximately 65,951 cu.m of longshore sediment transport was blocked by the Na Saton jetty annually (Table 5). The increase of beach elevation was +0.80 to + 1.0 m at some locations, and the accretion decreased further away from the jetty. The apparent deposition occurred within 1 km south from the updrift jetty (Figure 8). The alongshore sediment transport rate at the Na Saton jetty was larger than those of the other jetties in this study because the wave climate at the Na Saton jetty was more intense.
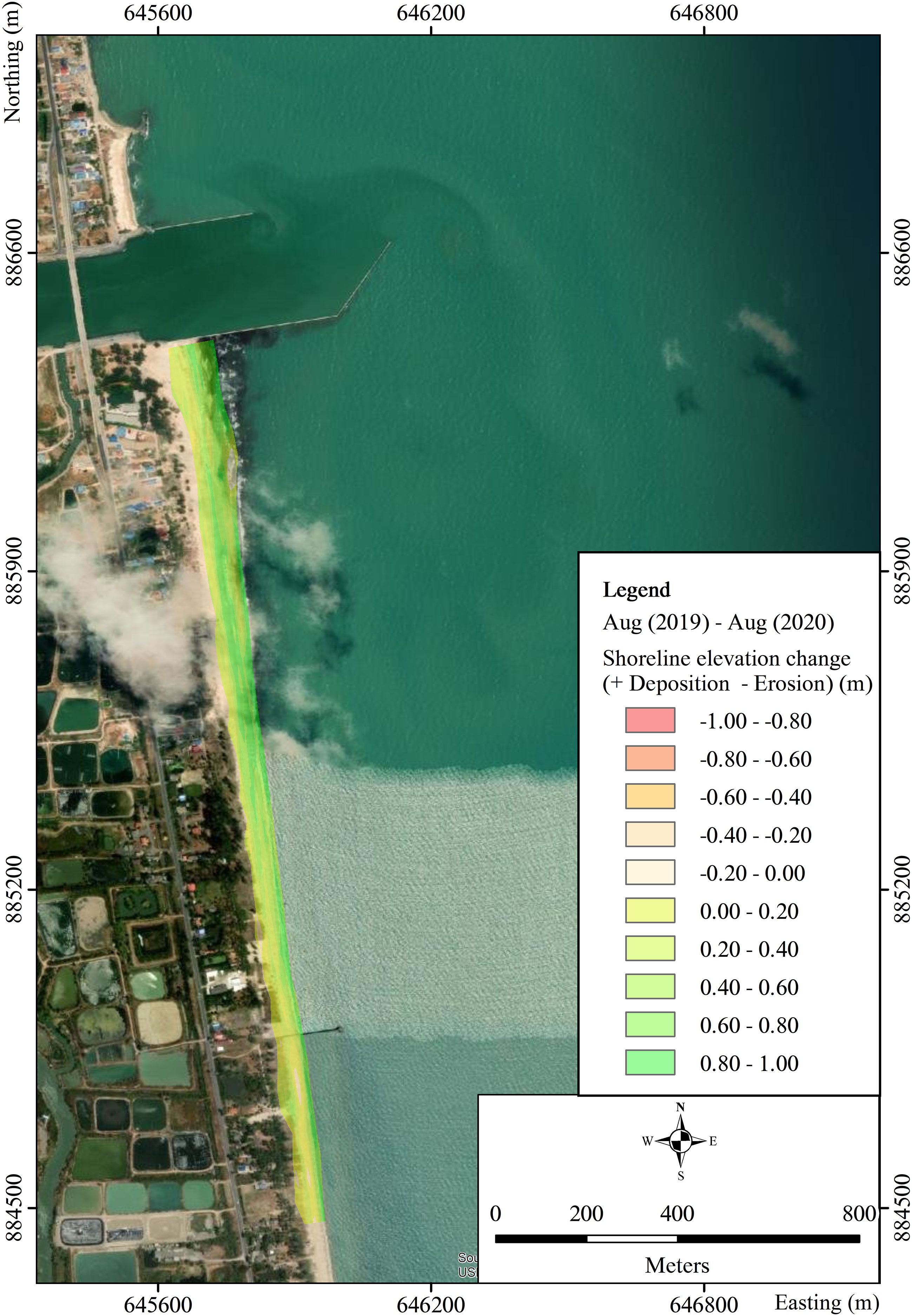
Figure 8 Differences in coastal elevation at the updrift Na Saton Jetty (August 2019 versus August 2020).
Wave and water current characteristics at the Na Saton jetty are somehow analogous to those of the Krai jetty because their coastline orientations are similar. During the SW monsoon (May to October), mild waves are predominantly from the SE direction, carrying the sediment northwards (Figure 9A). Big waves, happening during the NE monsoon (November to January), approaching the shoreline from the NE (Figure 9B), move the sediment southwards. During the transitional period (February to April), the waves come from the east, but with lesser magnitude. Therefore, at the Na Saton jetty, the waves during February to October bring the sediment northwards, while the waves during November to January carry the sediment southwards. Similar to other jetties in this study, the Na Saton jetty alters water current characteristics. The water current flows northwards during flood tide (Figure 9C), and vice versa (Figure 9D). The maximum current speed is less than 0.3 m/s. The nearshore water current direction is deviated by the jetty’s tip. The Na Saton jetty clearly changes coastal hydrodynamics, inducing a change in sediment transport.
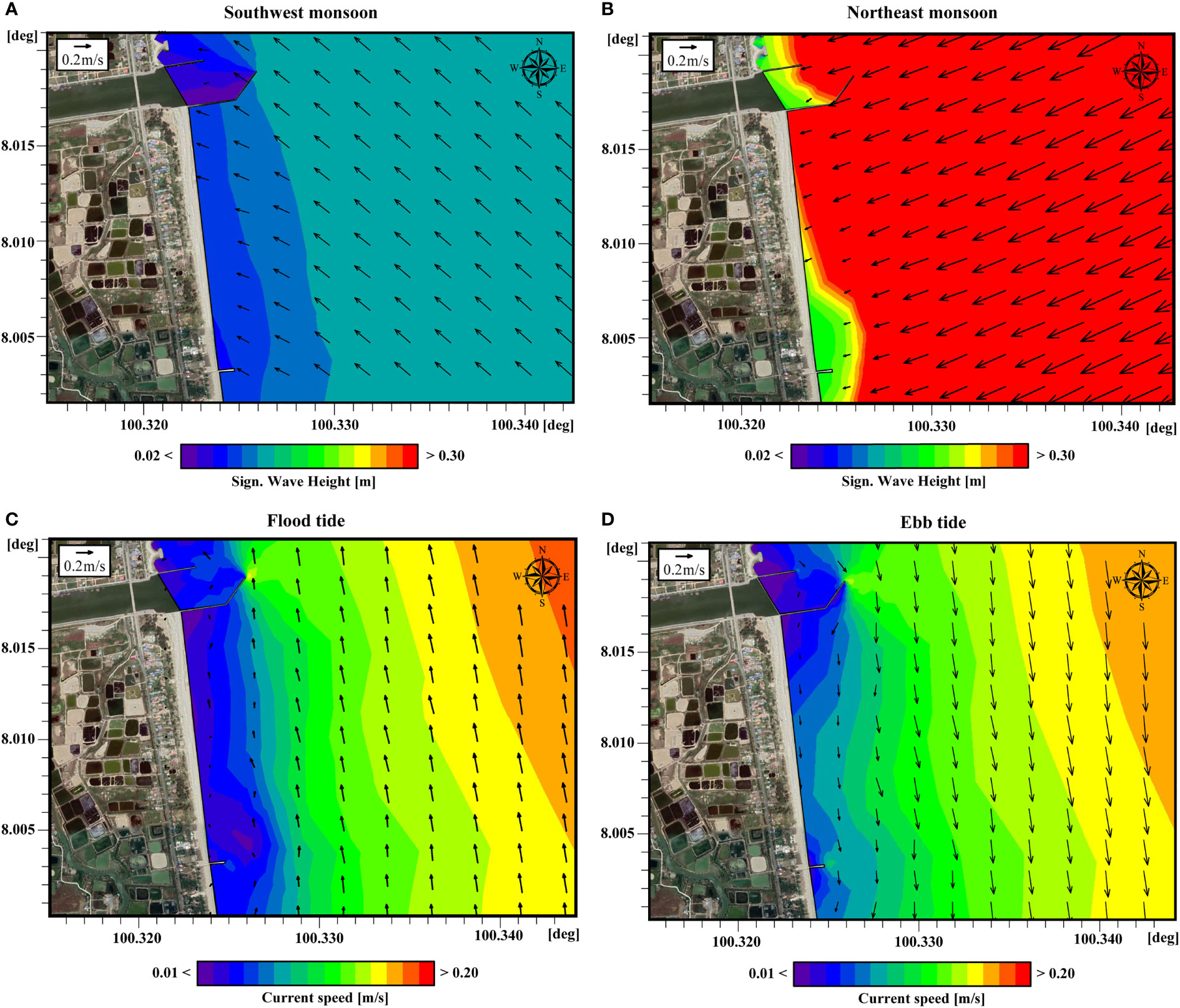
Figure 9 Results of MIKE 21 SW and HD at Na Saton jetty (A) significant wave height and direction (arrows) during the southwest monsoon, (B) significant wave height and direction (arrows) during the northeast monsoon, (C) current speed and direction (arrows) during flood tide, (D) current speed and direction (arrows) during ebb tide.
Discussion
Beach morphology and sediment volumetric change exhibit a seasonal variation (Yaacob et al., 2018). Alongshore sediment transport rate varies from location to location, depending on wave climate (wave height, wave period, and wave direction), sediment characteristics, and coastline alignment (Gunasinghe et al., 2021; Weerasingha and Ratnayake, 2022). Estimating the alongshore sediment transport quantity allows coastal managers to mitigate negative externalities generated by coastal structures that obstruct longshore sediment movement. Influences of wave propagation and water current pattern should be understood since they can create different shoreline adjustments between coastal structures (Adamo et al., 2014; Ratnayake et al., 2019).
Jetties are one of the most encountered coastal structures. They help maintain safe navigation in and out of inlets. However, one of the critical environmental impacts of jetties is significant shoreline change. An updrift coastline will be deposited, while the downdrift shoreline will be eroded. Numerical simulations at three jetties in this study indicated that the net sediment transport moves northwards while the longshore water current flows northwards and southwards along the shore, depending on flood or ebb tides. The beach sediment is transported and accumulated on the jetty’s updrift side as the jetty blocks the sediment movement. The jetty breaks the sediment budget equilibrium. Figure 10 illustrates how a jetty, waves, currents, and a beach interact. Swash and backwash promote sediment accretion at the updrift jetty. In this study, waves during February to October transport the sediment northwards (Figure 10A), while the waves during November to January carry the sediment southwards (Figure 10B). While wave direction changes seasonally, the water current direction changes daily. If the wave and the tidal current are in the same direction, a greater amount of the sediment will be transported. The same interaction was found by Ismail et al. (2020) and Shariful et al. (2020). Ariffin et al. (2020), who undertook a study in Malaysia, where the coastline is influenced by northeast monsoon like the jetties in this study, found that most of the deposition of sediment occurred near coastal protection structures.
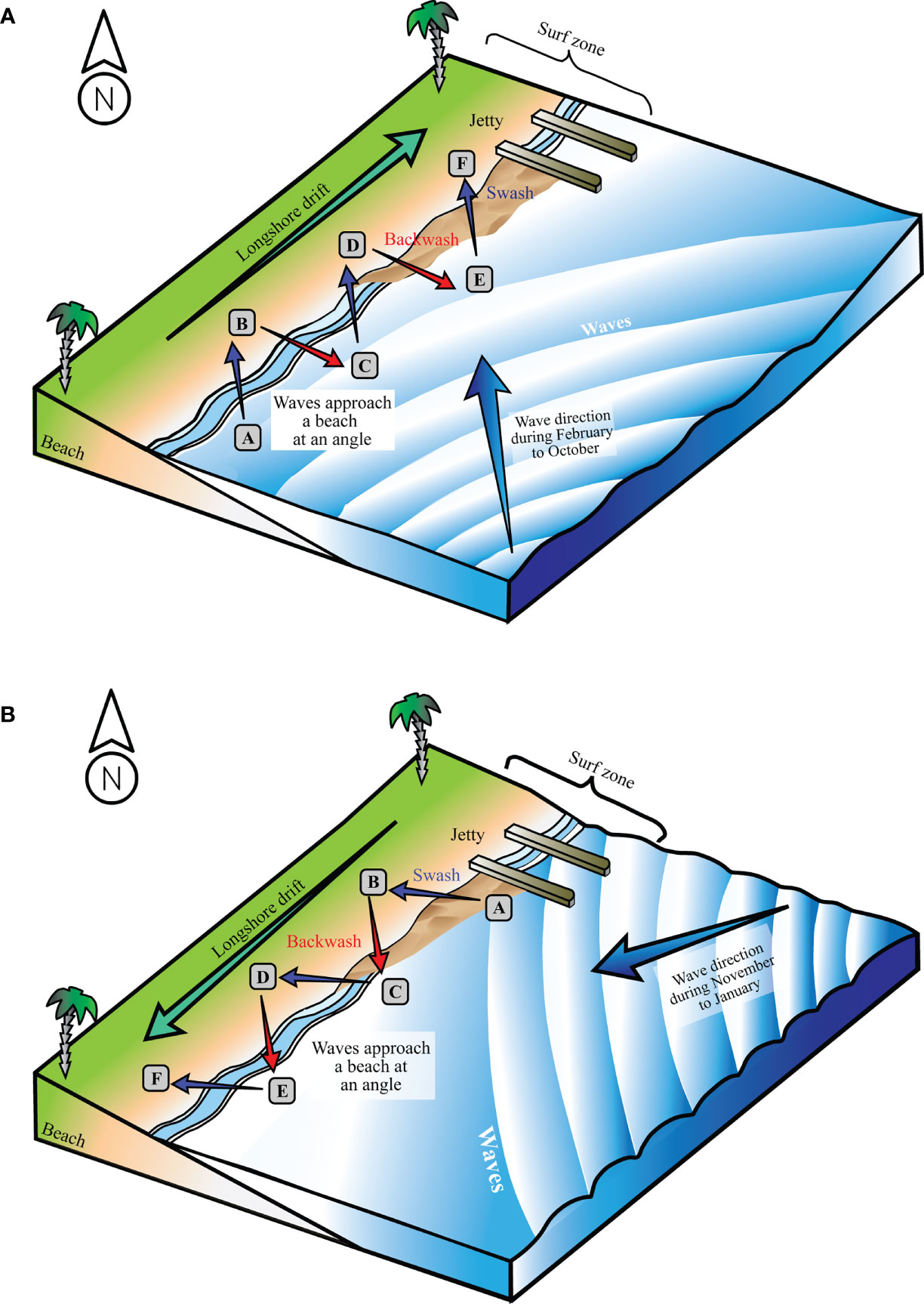
Figure 10 Interactions of a jetty, waves, currents, and beach morphology (A) during the southwest monsoon, waves transport sediment from A to F (northwards), creating accretion at the updrift jetty, (B) during the northeast monsoon, the waves carry the sediment away from the jetty from A to F (southwards).
Bypassing the sediment deposited at the updrift jetty can be one of the good solutions to solve downdrift coastal erosion (Garel et al., 2014, 2015; Franklin et al., 2021). Downdrift erosion can extend 8 to 10 times the length of the protrusion of a coastal structure (Kudale, 2010). However, how much of the sediment should be bypassed must be correctly estimated because it affects budget planning. This study is the first one in Thailand that estimates the sub-aerial and intertidal sediment deposition at jetties. Although there have been a few publications that have dealt with coastal erosion and protection in Thailand, none of them has elicited the amount of sediment that was intercepted by those coastal structures. This research found that the sub-aerial and intertidal sediment deposition at the Cha Am jetty was approximately 38,187 cu.m/yr, the rate at the Krai jetty was approximately 34,170 cu.m/yr, and 65,951 cu.m/yr at the Na Saton jetty. Nevertheless, the sediment transport rate estimated by this study might be less than the actual quantity, because the surveys were not expanded to cover the closure depth. The authors realized that there was a fraction of the alongshore sediment transport within the surf zone. Such sediment was carried by the longshore current and would accumulate at the updrift jetty. It would be washed ashore during calm periods (especially during the southwest monsoon) and deposited on the beach berm, as was concluded by a similar study in Sri Lanka (Ratnayake et al., 2018).
Bypassing sub-aerial and intertidal intercepted sediment can be a useful and an easy-to-implement approach to mitigate downdrift erosion, because it does not involve any advanced construction machinery. It can be seen from the literature that constructing coastal protection structures, such as revetments and offshore breakwaters, cannot solve the problem but rather move the erosion further downdrift (Kamphuis, 2010; Anfuso et al., 2012; Saengsupavanich, 2019). The construction costs of such coastal protection structures are not cheap. In Thailand, protecting a 1-km of shoreline with a revetment may cost approximately 2.2 million USD. Detached breakwaters may cost a little more, depending on their configurations such as gap width, crest height, and breakwater slope. The design lifetime of these coastal protection structures in Thailand is usually 50 years (Saengsupavanich, 2017). The overall construction cost will depend on how many kilometers of downdrift shoreline must be protected. Such approaches may induce more coastal erosion further downdrift, and more revetments/breakwaters will be kept going. On the other hand, bypassing sediment will not require any additional structural installation. In Thailand, the cost of sand excavation and dumping by an excavator is roughly 1 USD/cu.m, and 2 USD/cu.m by a dredger. The transportation cost varies, depending on the distance from the borrow to the dump sites. If the land-based sand bypassing (by excavators, trucks, and lorries) is implemented at the Na Saton jetty, it would cost at approximately 2 USD/cu.m or about 131,902 USD/yr, or a total of 6.6 million USD in 50 years. Decision makers can now weigh the benefits against the disadvantages before selecting which approach is more appropriate and sustainable.
Implementing sediment bypassing is not easy in reality. Many limitations may emerge (Beck & Wang, 2019; Saengsupavanich, 2020). Firstly, sediment bypassing is a continuous activity that needs continual budget allocation. Each year, Thailand must ration the national revenue, and there is no guarantee that sand bypassing will be given priority. Secondly, deposited sediment may be under the authority of different Subdistrict Administration Organizations (the smallest local government unit taking care of a group of villages) (SAOs). Moving the sediment from one SAO to another SAO can create social resistance, as has already happened in Thailand. The deposited beach at the updrift jetty is usually used as a recreational area. Bypassing the deposited sediment will inevitably destroy the wide beach, and deteriorate the updrift beach’s utilization.
Conclusion
Estimating sub-aerial and intertidal sediment deposition is necessary to mitigate downdrift coastal erosion caused by jetties. Along the southern coast of Thailand, there are a few jetties that have created severe downdrift erosion, particularly the Cha Am jetty, the Krai jetty, and the Na Saton jetty. At every jetty, understanding the governing hydrodynamic interaction and accurately determining the quantities of sediment that should be bypassed influence a successful solution of the downdrift erosion. The magnitude of the sub-aerial and intertidal sediment that should be bypassed, estimated by this study, was in the range of 34,170 to 65,951 cu.m/yr. Budget planning and implementation preparations can be set up. Decision makers can choose between 1) a one-off solution, such as constructing revetments/offshore breakwaters to solve erosion occurring adjacent to downdrift jetties, which may induce more erosion further downdrift, or 2) bypassing the sediment once in a few years without having to install any coastal protection structures. Each option has different shortcomings that need to be addressed before downdrift coastal protection can be achieved.
Data availability statement
The raw data supporting the conclusions of this article will be made available by the corresponding author, without undue reservation, to any qualified researcher upon request.
Author contributions
CS conceived and planned the research, including the main ideas and theoretical background, led the survey teams, analyzed the field data, undertook numerical simulations, and wrote the manuscript. LY prepared the figures and helped conduct the numerical modeling. LL commented on the design of the research. SS-N-A assisted in the field survey and took part in analyzing the field data. All authors provided critical feedback and helped shape the research, analysis, and manuscript.
Funding
This research did not receive any specific grant from funding agencies in the public, commercial, or not-for-profit sectors. CS provided full financial support of field surveys, and partly paid the article processing fee. Kasetsart University greatly supported the article processing fee. SS-N-A partly supported the article processing fee.
Acknowledgments
The authors would like to express their heartfelt appreciation to the anonymous reviewers for their meticulous work and thoughtful suggestions, which have significantly improved this paper.
Conflict of interest
The authors declare that the research was conducted in the absence of any commercial or financial relationships that could be construed as a potential conflict of interest.
Publisher’s note
All claims expressed in this article are solely those of the authors and do not necessarily represent those of their affiliated organizations, or those of the publisher, the editors and the reviewers. Any product that may be evaluated in this article, or claim that may be made by its manufacturer, is not guaranteed or endorsed by the publisher.
References
Adamo F., De Capua C., Filianoti P., Lanzolla A. M. L., Morello R. (2014). A coastal erosion model to predict shoreline changes. Measurement 47 (1), 734–740. doi: 10.1016/j.measurement.2013.09.048
Amalan K., Ratnayake A. S., Ratnayake N. P., Weththasinghe S. M., Dushyantha N., Lakmali N., et al (2018). Influence of nearshore sediment dynamics on the distribution of heavy mineral placer deposits in Sri Lanka. Environ. Earth Sci. 77, 737. doi: 10.1007/s12665-018-7914-4
Anfuso G., Martínez-del-Pozo J. Á., Rangel-Buitrago N. (2012). “Bad practice in erosion management: The southern Sicily case study,” in Pitfalls of shoreline stabilization. Eds. Cooper J. A. G., Pilkey O. (Netherland: Springer), 215–233. doi: 10.1007/978-94-007-4123-2
Anh V. T., Trung P. B., Nguyen K. A., Liou Y. A., Phan M. T. (2021). Human impacts on estuarine erosion-deposition in southern central Vietnam: Observation and hydrodynamic simulation. Sustainability 13 (15), 8303. doi: 10.3390/su13158303
Ariffin E. H., Mathew M. J., Yaacob R., Akhir M. F., Shaari H., Zulfakar M. S. Z., et al (2018). Beach morphodynamic classification in different monsoon seasons at terengganu beaches, Malaysia. J. Sustain. Sci. Manage. 13 (5), 65–74.
Ariffin E. H., Sedrati M., Akhir M. F., Yaacob R., Husain M. L. (2016). Open sandy beach morphology and morphodynamic as response to seasonal monsoon in Kuala terengganu, Malaysia. J. Coast. Res. 75 (10075), 1032–1036. doi: 10.2112/SI75-207.1
Ariffin E. H., Sedrati M., Daud N. R., Mathew M. J., Akhir M. F., Awang N. A., et al (2019). “Shoreline evolution under the influence of oceanographic and monsoon dynamics: The case of terengganu, Malaysia,” in Coastal zone management. Eds. Ramkumar M., James R. A., Menier D., Kumaraswamy (Netherland: Elsevier), 113–130. doi: 10.1016/B978-0-12-814350-6.00005-7
Ariffin E. H., Zulfakar M. S. Z., Redzuan N. S., Mathew M. J., Akhir M. F., Baharim N. B., et al (2020). Evaluating the effects of beach nourishment on littoral morphodynamics at Kuala nerus, terengganu (Malaysia). J. Sustain. Sci. Manage. 15 (5), 29–42. doi: 10.46754/jssm.2020.07.005
Azarmsa S. A., Esmaeili M., Khaniki A. K. (2009). Impacts of jetty construction on the wave heights off the kiashahr lagoon. Aquat. Ecosyst. Health Manage. 12 (4), 358–363. doi: 10.1080/14634980903354726
Bayram A., Larson M., Hanson H. (2007). A new formula for the total longshore sediment transport rate. Coast. Eng. 54 (9), 700–710. doi: 10.1016/j.coastaleng.2007.04.001
Beck T. M., Wang P. (2019). Morphodynamics of barrier-inlet systems in the context of regional sediment management, with case studies from west-central Florida, USA. Ocean Coast. Manage. 177, 31–51. doi: 10.1016/j.ocecoaman.2019.04.022
Bergillos R. J., Masselink M., Ortega-Sanchez M. (2017). Coupling cross-shore and longshore sediment transport to model storm response along a mixed sand-gravel coast under varying wave directions. Coast. Eng. 129, 93–104. doi: 10.1016/j.coastaleng.2017.09.009
Boswood P. K., Murray R. J. (2001) World-wide sand bypassing systems: Data report (Queensland, Australia). Available at: https://repository.tudelft.nl/islandora/object/uuid%3Af05038b9-3e0d-4d95-be99-f2266600e1fe (Accessed 19 October 2021).
Dyson A., Lawson S., Victory S., Boswood P., Mahon B., Trucchi L., et al (2002). “Tweed River entrance sand bypassing project post commissioning coastal behaviour,” in Paper presented at proceedings of the 28th international conference on coastal engineering. American society of civil engineers (Cardiff, Wales, UK: World Scientific).
Fan R., Wei H., Zhao L., Zhao W., Jiang C., Nie H. (2019). Identify the impacts of waves and tides to coastal suspended sediment concentration based on high-frequency acoustic observations. Mar. Geol. 408, 154–164. doi: 10.1016/j.margeo.2018.12.005
Fortunato A. B., Freire P., Mengual B., Bertin X., Pinto C., Martins K., et al (2021). Sediment dynamics and morphological evolution in the tagus estuary inlet. Mar. Geol. 440, 106590. doi: 10.1016/j.margeo.2021.106590
Franklin G. L., Medellín G., Appendini C. M., Gómez J. A., Torres-Freyermuth A., González J. L., et al (2021). Impact of port development on the northern Yucatan peninsula coastline. Reg. Stud. Mar. Sci. 45, 101835. doi: 10.1016/j.rsma.2021.101835
Garel E., Sousa C., Ferreira O. (2015). Sand bypass and updrift beach evolution after jetty construction at an ebb-tidal delta. Estuar. Coast. Shelf Sci. 167, 4–13. doi: 10.1016/j.ecss.2015.05.044
Garel E., Sousa C., Ferreira O., Morales J. A. (2014). Decadal morphological response of an ebb-tidal delta and downdrift beach to artificial breaching and inlet stabilisation. Geomorphology 216, 13–25. doi: 10.1016/j.geomorph.2014.03.031
Ghashemizadeh N., Tajziehchi M. (2013). Impact of long jetty on shoreline evaluation (Case study: Eastern coast of Bandar abbas). J. Basic Appl. Sci. Res. 3 (3), 1256–1266.
Gunasinghe G. P., Ruhunage L., Ratnayake N. P., Ratnayake A. S., Samaradivakara G. V. I., Jayaratne R. (2021). Influence of manmade effects on geomorphology, bathymetry and coastal dynamics in a monsoon-affected river outlet in southwest coast of Sri Lanka. Environ. Earth Sci. 80, 238. doi: 10.1007/s12665-021-09555-0
Hapke C. J., Kratzmann M. G., Himmelstoss E. A. (2013). Geomorphic and human influence on large-scale coastal change. Geomorphology 199, 160–170. doi: 10.1016/j.geomorph.2012.11.02
Hein C. J., Fallon A. R., Rosen P., Hoagland P., Georgiou I. Y., FitzGerald D. M., et al (2019). Shoreline dynamics along a developed river mouth barrier island: Multi-decadal cycles of erosion and event-driven mitigation. Front. Earth Sci. 7. doi: 10.3389/feart.2019.00103
Ismail N. I., Ariffin E. H., Yaacob R., Lokman M. H., Baharim N. B. (2020). The impact of seasonal monsoons on the morphology of beaches protected by barrier islands in setiu, terengganu, Malaysia. J. Sustain. Sci. Manage. 15 (4), 1–10. doi: 10.46754/jssm.2020.06.012
Kamphuis J. W. (1991). Alongshore sediment transport rate. J. Waterw. Port Coast. Ocean Eng. 117 (6), 624–640. doi: 10.1061/(ASCE)0733-950X(1991)117:6(624
Kamphuis J. W. (2010). Introduction to coastal engineering and management (Singapore: World Scientific), 472 p.
Keshtpoor M., Puleo J. A., Gebert J., Plant N. G. (2013). Beach response to a fixed sand bypassing system. Coast. Eng. 73, 28–42. doi: 10.1016/j.coastaleng.2012.09.006
King E. V., Conley D. C., Masselink G., Leonardi N., McCarroll R. J., Scott T. (2019). The impact of waves and tides on residual sand transport on a sediment-poor, energetic, and macrotidal continental shelf. J. Geophys. Res.: Oceans 124 (7), 4974–5002. doi: 10.1029/2018JC014861
Kudale M. D. (2010). Impact of port development on the coastline and the need for protection. Indian J. Geo-Mar. Sci. 39 (4), 597–604.
Lim H. S., Kim M., Do J., Kim S., Lee H. J., Kim D. H. (2018). Variability of wave-induced current analyzed with intensive field measurements in the coastal waters of anmok. J. Coast. Res. 85, 686–690. doi: 10.2112/SI85-138.1
Masselink G., Castelle B., Scott T., Dodet G., Suanez S., Jackson D., et al (2016). Extreme wave activity during 2013/2014 winter and morphological impacts along the Atlantic coast of Europe. Geophys. Res. Lett. 43, 2135–2143. doi: 10.1002/2015GL067492
Muhammad M., Idris K., Ariffin E. H., Shaffril H. A. M., Samah B. A., Suandi T. (2016). The impact of climate change on small-scale fishermen in Malaysia. Soc. Sci. (Pakistan) 11 (13), 3352–3356. doi: 10.3923/sscience.2016.3352.3356
Murthy V. N. S. (2003). Geotechnical engineering: Principles and practices of soil mechanics and foundation engineering (New York: Marcel Dekker), 1029 p.
Nassar K., Mahmod W. E., Masria A., Fath H., Nadaoka K. (2018). Numerical simulation of shoreline responses in the vicinity of the western artificial inlet of the bardawil lagoon, Sinai peninsula, Egypt. Appl. Ocean Res. 74, 87–101. doi: 10.1016/j.apor.2018.02.015
Oyegbile B. O., Oyegbile B. A. (2017). Applications of geosynthetic membranes in soil stabilization and coastal defence structures. Int. J. Sustain. Built Environ. 6, 636–662. doi: 10.1016/j.ijsbe.2017.04.001
Rangel-Buitrago N. G., Anfuso G., Williams A. T. (2015). Coastal erosion along the Caribbean coast of Colombia: Magnitudes, causes and management. Ocean Coast. Manage. 114, 129–144. doi: 10.1016/j.ocecoaman.2015.06.024
Ratnayake N. P., Ratnayake A. S., Azoor R. M., Weththasinghe S. M., Seneviratne I. D. J., Senarathne N., et al (2019). Erosion processes driven by monsoon events after a beach nourishment and breakwater construction at uswetakeiyawa beach, Sri Lanka. SN Appl. Sci. 1, 52. doi: 10.1007/s42452-018-0050-7
Ratnayake N. P., Ratnayake A. S., Keegle P. V., Mallawa Arachchi M. A. K. M., Premasiri H. M. R. (2018). An analysis of beach profile changes subsequent to the Colombo harbor expansion project, Sri Lanka. Environ. Earth Sci. 77, 24. doi: 10.1007/s12665-018-7234-8
Rautenbach C., Theron A. K. (2018). Study of the Durban bight shoreline evolution under schematised climate change and sand−bypassing scenarios. J. South Afr. Inst. Civ. Eng. 60 (4), 2–15. doi: 10.17159/2309-8775/2018/v60n4a1
Saengsupavanich C. (2012). Unwelcome environmental impact assessment for coastal protection along a 7-km shoreline in southern Thailand. Ocean Coast. Manage. 61, 20–29. doi: 10.1016/j.ocecoaman.2012.02.008
Saengsupavanich C. (2013). Detached breakwaters: Communities' preferences for sustainable coastal protection. J. Environ. Manage. 115, 106–113. doi: 10.1016/j.jenvman.2012.11.029
Saengsupavanich C. (2017). Coastal revetment design process in Thailand. WIT Trans. Built Environ. 170, 33–44. doi: 10.1016/j.jenvman.2012.11.029
Saengsupavanich C. (2019). Willingness to restore jetty-created erosion at a famous tourism beach. Ocean Coast. Manage. 178, 104817. doi: 10.1016/j.ocecoaman.2019.104817
Saengsupavanich C. (2020). Deconstructing a jetty to rectify the downdrift erosion. J. Sustain. Sci. Manage. 15 (2), 79–88.
Salleh A. M., Nadzir N. (2020). Study of sediment deposition at tanjung laboh due to jetty structural. IOP Conference Series: Earth and Environmental Science. 498, 1, 012016. doi: 10.1088/1755-1315/498/1/012016. IOP Publishing.
Selamat N. S., Maulud K. N., Mohd F. A., Rahman A. A. A., Zainal M. K., Wahid M. A. A., et al (2019). Multi method analysis for identifying the shoreline erosion during northeast monsoon season. J. Sustain. Sci. Manage. 14 (3), 43–54.
Shariful F., Sedrati M., Ariffin E. H., Shubri S. M., Akhir M. F. (2020). Impact of 2019 tropical storm (Pabuk) on beach morphology, terengganu coast (Malaysia). J. Coast. Res. 95 (Special Issue), 346–350. doi: 10.2112/SI95-067.1
Shetty A., Jayappa K. S. (2020). Seasonal variation in longshore sediment transport rate and its impact on sediment budget along the wave-dominated karnataka coast, India. J. Earth Syst. Sci. 129, 234. doi: 10.1007/s12040-020-01504-y
Silva A. P., Vieira da Silva G., Strauss D., Murray T., Tomlinson R. (2021). Updrift morphological impacts of a coastal protection strategy. how far and for how long? Mar. Geol. 441, 106625. doi: 10.1016/j.margeo.2021.106625
United States Army Corps of Engineers (1984). Shore protection manual (Washington: Coastal Engineering Research Center), 652 p.
Wang P., Beck T. M. (2012). Morphodynamics of an anthropogenically altered dual-inlet system: John's pass and blind pass, west-central Florida, USA. Mar. Geol. 291–294, 162–175. doi: 10.1016/j.margeo.2011.06.001
Wang Y. H., Wang Y. H., Deng A. J., Feng H. C., Wang D. W., Guo C. S. (2022). Emerging downdrift erosion by twin long-range jetties on an open mesotidal muddy coast, China. J. Mar. Sci. Eng. 10 (5), 570. doi: 10.3390/jmse10050570
Weerasingha W. A. D. B., Ratnayake A. S. (2022). Coastal landform changes on the east coast of Sri Lanka using remote sensing and geographic information system (GIS) techniques. Remote Sens. Appl.: Soc. Environ. 26, 100763. doi: 10.1016/j.rsase.2022.100763
Witmer A. D., Ammons A. W., Bell A. C., Rowe J. B. (2018). Anthropogenic transport of macrofauna through a sand transfer plant. Ocean Coast. Manage. 155, 1–7. doi: 10.1016/j.ocecoaman.2018.01.026
Yaacob R., Shaari H., Sapon N., Ahmad M. F., Arifin E. H., Zakariya R., et al (2018). Annual changes of beach profile and nearshore sediment distribution off dungun-Kemaman,Terengganu, Malaysia. J. Teknol. 80 (5), 57–66.
Žilinskas G., Janušaitė R., Jarmalavičius D., Pupienis D (2020). The impact of Klaipėda Port entrance channel dredging on the dynamics of coastal zone, Lithuania. Oceanologia. 62 (4), 489–500. doi: 10.1016/j.oceano.2020.08.002
Zulfakar M. S. Z., Akhir M. F., Ariffin E. H., Awang N. A., Yaakob M. A. M., Muslim A. M. (2020). The effect of coastal protections on the shoreline evolution at Kuala nerus, terengganu (Malaysia). J. Sustain. Sci. Manage. 15 (3), 71–85.
Zulfakar M. S. Z., Ariffin E. H., Akhir M. F., Awang N. A. (2021). Variation of seasonal monsoon wave-driven circulation pattern in the lee of groyne in Kuala nerus beach. IOP Conf. Ser.: Earth Environ. Sci. 698 (1), 012042. doi: 10.1088/1755-1315/698/1/012042
Keywords: coastal erosion, beach survey, sediment deposition, beach management, coastal engineering
Citation: Saengsupavanich C, Yun LS, Lee LH and Sanitwong-Na-Ayutthaya S (2022) Intertidal intercepted sediment at jetties along the Gulf of Thailand. Front. Mar. Sci. 9:970592. doi: 10.3389/fmars.2022.970592
Received: 16 June 2022; Accepted: 11 July 2022;
Published: 02 August 2022.
Edited by:
Effi Helmy Ariffin, University of Malaysia Terengganu, MalaysiaReviewed by:
Amila Ratnayake, Uva Wellassa University, Sri LankaUmakanta Pradhan, National Centre for Coastal Research, India
Mohamad Hidayat Jamal, Universiti Teknologi Malaysia, Malaysia
Copyright © 2022 Saengsupavanich, Yun, Lee and Sanitwong-Na-Ayutthaya. This is an open-access article distributed under the terms of the Creative Commons Attribution License (CC BY). The use, distribution or reproduction in other forums is permitted, provided the original author(s) and the copyright owner(s) are credited and that the original publication in this journal is cited, in accordance with accepted academic practice. No use, distribution or reproduction is permitted which does not comply with these terms.
*Correspondence: Cherdvong Saengsupavanich, Y2hlcmR2b25nLnNhZW5nc3VwYXZhbmljaEBob3RtYWlsLmNvbQ==; Y2hlcmR2b25nLnNhQGt1LnRo